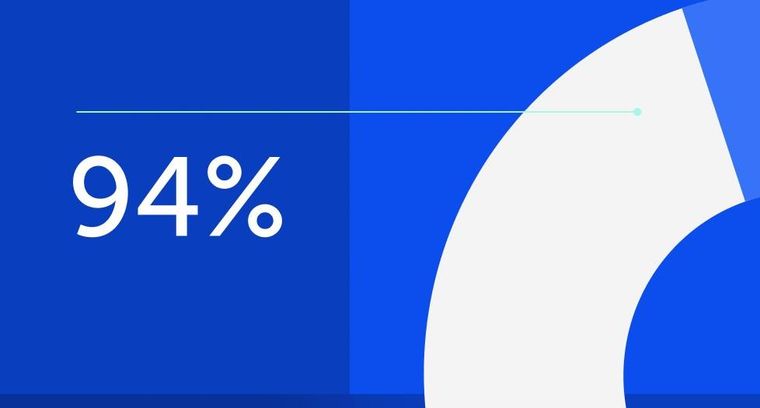
94% of researchers rate our articles as excellent or good
Learn more about the work of our research integrity team to safeguard the quality of each article we publish.
Find out more
ORIGINAL RESEARCH article
Front. Earth Sci., 04 June 2021
Sec. Quaternary Science, Geomorphology and Paleoenvironment
Volume 9 - 2021 | https://doi.org/10.3389/feart.2021.662172
This article is part of the Research TopicHuman-Environmental Interactions in Prehistoric PeriodsView all 16 articles
Bronze Age Shang China is characterized by its large-scale production system and distinctive ritual world. Both are vividly materialized by a large number of bronze ritual vessels with added lead. Whilst a remarkable amount of research effort has been channeled into the trace elemental and lead isotopic analysis of these ritual vessels, and successfully revealed some important fingerprints such as highly radiogenic lead (HRL), there is as yet no consensus on the metal source(s) which supplied the entire bronze production during the Shang period. In addition to the traditional method to look for matching and mismatching between ores and objects, we propose that environmental archaeological studies can provide crucial clues to address some long-standing questions in archaeometallurgy. In the first part of the paper, we attempt to illustrate the potential and complexity of combining these two subjects together. The second part of the paper offers a case study by reviewing the debate on Yunnan as the source of HRL. Synthesis of various lines of evidence published by most recent studies on environmental archaeology, archaeometallurgy, field reports and radiocarbon dating suggests that this hypothesis appears much less likely than previously suspected.
Archaeometallurgy and environmental archaeology have become increasingly important in the study Bronze Age China in recent years (Xu et al., 2011; Zhang et al., 2017; Qiu et al., 2019; Storozum et al., 2020). In fact, these two subjects share a variety of research interests and objectives (Nriagu, 1972; Abbott and Wolfe, 2003; Thevenon et al., 2011; Iles, 2016; McConnell et al., 2018). Archaeometallurgy is primarily focused on all the archaeological records related to metallurgy (Rehren and Pernicka, 2008; Roberts and Thornton, 2014). Metallurgical production is a highly complex process, encompassing mining, ore crushing and selection, smelting, mould-making, casting and recycling. Each of these processes can certainly leave some markers which are detectable by environmental research. However, in the current literature we find very little attempt to correlate these two branches of archaeology in Chinese prehistoric studies. In this paper, we argue that there is great potential for archaeometallurgists and environmental scientists to work together and resolve many critical questions in both disciplines. To exemplify this, we choose to revisit the long-standing issue of highly radiogenic lead (HRL) in Chinese Bronze Age archaeology by integrating the most recent work on archaeometallurgy, environmental science and archaeological excavations. Synthesis of these various lines of information implies increasingly less possibility for Yunnan to be the source of HRL used in Bronze Age Shang China (ca. 1500–1045 BC).
Two features of metallurgical production in Bronze Age China make it particularly relevant for environmental research. The first one is the large scale of production (Ledderose, 2000; Pollard et al., 2017a; Rawson, 2017a). Since the early Shang period, especially from the upper Erligang phase (ca. 1500–1450 BC), one starts to see strong evidence of mass production of bronzes in the Central Plains (Chen, 2016; Rawson, 2017b; Xu, 2020). Although the major part of Shang Zhengzhou is still underneath the present-day Zhengzhou city, the three bronze hoards demonstrate the remarkable skills of bronze casting and the high volume of raw metal accessible to the early Shang power (Henan Institute of Archaeology, 2001). More crucially, they have also provided us with the first robust evidence for bronze ritual vessels being used in sets, which was central to ritual practice throughout the Chinese Bronze Age, and is well-documented in later Eastern Zhou period (ca. 770–256 BC) (Bagley, 1999; Rawson, 1999; Zhang, 2014). This becomes even more prominent in the Anyang period (ca. 1300–1045 BC) where thousands of elite tombs were discovered containing bronze objects. In the tomb of Fu Hao, the only intact royal tomb so far excavated at Anyang, archaeologists recovered 1.6 tonnes of bronze objects (The Institute of Archaeology, 1980; Bagley, 1999). Another good example of the large-scale of bronze production at Anyang is the famous Houmuwu Ding, containing over 830 kg of metal. Both Fu Hao’s metal assemblage and the Houmuwu Ding mean that craftspeople had to process probably tens or even hundreds of tons of ores and slags, depending on the type and quality of the ores. It is important to note that although Anyang was probably the largest bronze consumer during the late Shang dynasty, it is nevertheless located in the Central Plains with the least abundant nearby metal resources (Liu and Chen, 2009; Li, 2014; Liu, 2016). The majority of metal resources in China are distributed in the middle and lower Yangtze river valley, Yunnan, the Hexi Corridor and Northeast China. Therefore, apart from the workshop practice, more labor was certainly necessary for mining, smelting and transporting metals from other places to the Central Plains. Moreover, metal production in the entire Chinese Bronze Age was based on ceramic piece-mould casting. Preparing the ceramic molds obviously required more fuel (i.e., charcoal), leading to the expectation that intensive markers would be left in various environmental records (e.g., deforestation, pollution or other anthropogenic proxies, see below).
The second feature of metal production for Bronze Age China is the addition of lead (Pollard et al., 2017a; Pollard et al., 2017b). Although metallurgy was introduced into the Central Plains from the steppe (Roberts et al., 2009; Linduff and Mei, 2014), with specific timing and route(s) still to be ascertained, the prevailing alloying recipes on the steppe are tin bronze, arsenical copper and arsenical bronze (Chernykh, 1992; Mei, 2009; Hsu, 2016). In contrast, it was only in the Central Plains that lead became a major alloying element. It is so far still impossible to illustrate when exactly lead started to be deliberately added to copper. Some evidence derives from the range of lead percentages in the objects. Since Erlitou Phase IV, one can see an increasing number of copper-alloyed objects containing lead over 5% (Jin, 2008; IA; CASS, 2014). This is undoubtedly beyond the general level of impurity in coper ores and can therefore be considered as a deliberate addition. Further evidence comes from Anyang. In the top-elite tomb M1046 (dated to Anyang Phase IV), the nine ritual vessels show a remarkably high percentage of lead, but with very narrow variation (between 30 and 35% Pb), suggesting a well-controlled alloying practice for lead (Zhao et al., 2008; Liu et al., 2020b).
Both large-scale production and the widespread use of lead could exert profound impacts on the environment. However, there is considerable complexity involved in integrating archaeometallurgy with environmental records (Figure 1). The most characteristic environmental marker left by metallurgical production is probably the increased levels of metallic elements, such as copper, tin, lead, arsenic, nickel, zinc in the environment. Each step of metallurgical production can significantly increase the concentrations of metallic elements in the atmosphere or biosphere. Therefore, the rise and fall of metallurgy in one region is often used as a reason to explain metal variations in the environmental records, such as ice cores, lake sediments and excavation profiles (Abbott and Wolfe, 2003; Zhang et al., 2017; McConnell et al., 2018). This is particularly true if regional metallurgical production can be linked to state expansion or warfare, when more metal objects (i.e., weapons) became widely needed. The Greenland ice core shows various rises and falls in the level of lead since ca. 1000 BC, which were ultimately sourced to the lead pollution in Europe by the atmospheric circulation modeling. Further archaeological and historical evidence back up such linkages. Based on a refined high-resolution chronology (ca. 1–10 years), McConnell et al. (2018) were able to correlate the changing levels of lead in the ice core to a variety of human factors that affected the production of silver in Europe, such as the Phoenician expansion, new silver mines being opened, along with Roman expansion and plagues that destroyed local mining industries. Silver is generally extracted from argentiferous lead ores, so the increased production of silver gives rise to increased levels of lead in the atmosphere. Similar examples can be also found in the silver extraction in Potosi, South America from the 16th century CE. The patterns of metallic elements (Pb, Sb, Ag, Bi and Sn) reveal critical information on not only the scale of local silver production (approximated by the lead spikes in the lake sediments), but also the technological changes brought about by local people or early Spanish arrival. The rapid disappearance of spikes for Ag, Bi and Sn were caused by the improved capability of local smelters to control these volatile elements. However, the new Spanish smelting technology used stone furnaces to overheat the ores, which not only failed to extract their targeted silver, but also triggered higher metallic pollution in atmosphere, which was also registered in the lake sediment. It was only in 1572 CE when mercury amalgamation was introduced into Bolivia from Mexico which allowed people to extract low-grade ores more efficiently and reduce these atmospheric fluxes (Abbott and Wolfe, 2003).
The ultimate reason for the complexity of combining archaeometallurgy and environmental data is that, apart from metallurgy, a range of different anthropogenic activities and natural factors can also result in the rise of metallic concentrations in sediments. One vivid example comes from four Neanderthal cave sites in the Iberian Peninsula. Scholars have discovered a substantial elevation in the concentrations of copper, zinc, nickel and lead in the profile of the cave sites, which were clearly unrelated to metallurgy. Copper, zinc and nickel are crucial micronutrients for plant growth, and therefore the resultant wood ash after combustion may well concentrate these metallic elements in cave deposits. For some samples, guano (bird or bat droppings) is also an important source for zinc and copper. Lead is slightly different in this case, as it is more likely to be derived from galena (or lead ornaments) leaching down from upper layers (Monge et al., 2015). The scope of catchment is another key issue in interpreting metal pollution and other environmental markers. For some environmental records such as the Greenland ice cores, the lead pollution was mainly transported from continental Europe by air movement (macro-scale) (McConnell et al., 2018). In other cases, local sources and activities can be more significant, particularly for lakes which were linked to local rivers associated with metallurgical activities (mining or smelting). Other issues are related to chronological resolution. Whilst it is sometimes possible to use isotopic analysis to source metal pollution, in most cases scholars have to rely on chronology (e.g., synchronicity) to 1) establish correlation between metallurgy and environmental changes and 2) deduce underlying causality. The exact sequence of events is therefore of uttermost importance but due to the lack of good-quality materials for dating, the chronological resolution for environmental or archaeological records could be more than one hundred years for some key periods, making it less useful to interpret potential cause and effect between different events in Bronze Age.
In Chinese archaeology, highly radiogenic lead, or HRL, refers to a specific type of lead characterized by higher values of the lead isotopic signature (e.g., 206Pb/204Pb > 19, 207Pb/204Pb > 15.75 and 208Pb/204Pb > 39.0) (Jin, 2008; Liu et al., 2015; Jin et al., 2017). These higher isotopic ratios are ultimately derived from excessive amounts of uranium and/or thorium in the geological environment (Russell and Farquhar, 1960; Pollard et al., 2017c). It was first discovered in the bronzes at Anyang, the last capital of the Shang dynasty (ca. 1300 BC–1050 BC), by Prof Jin Zhengyao and his Chinese and Japanese colleagues (Jin, 1987). This soon rose to world-wide academic attention because HRL has to be formed through a very unique geological history, in which uranium and thorium have to be mobilized together (Prof D. Killick pers. comm). This is easier for uranium which can be dissolved in water and incorporated with other minerals. But thorium is insoluble and thus requires a different geological mechanism to be emplaced in the deposits (Chen et al., 2020; Liu et al., 2021). Several surveys of the geological literature show that the number of metallic deposits which can yield HRL is far less than those giving common lead (Jin et al., 2017; Hsu and Sabatini, 2019; Hsu et al., 2021).
Decades of effort in tracking HRL have produced a comprehensive illustration of the spatial-temporal pattern of HRL during the Shang period (ca. 1500–1050 BC). The current consensus is that HRL firstly came in to use around the Erligang Lower Phase (Jin, 2008), though one bronze fragment at Erlitou is also highly radiogenic (Jin et al., 2001; Liu et al., 2021b). It soon came to dominate the supply of metal during the Erligang period. Not only at Erligang, now known as Zhengzhou, one of the capitals for the early Shang dynasty, but also at Panlongcheng along the Yangtze River, at Hanzhong along the Han River and at Mogou in the east Hexi Corridor exist a considerable number of objects been discovered with HRL. It became even more widely distributed during the Anyang period, covering northeast China, the Central Plains, middle and lower Yangtze River Valley, Hanzhong Basin and Sichuan Basin. However, the use of HRL suddenly ceased after the Shang dynasty. To be more specific, at Anyang, it once dominated over 75% of metal supply in Phase II and 50% in Phase III, but virtually disappeared in Phase IV. So far, the latest site which was heavily dependent on radiogenic lead is Jinsha in the Sichuan basin, dated to the early Western Zhou dynasty (for more details see Jin et al., 2017 and Liu et al., 2021).
The provenance of HRL has been a matter of intense debate since Jin and colleagues discovered this signal in Shang bronzes in the 1980s. It is of critical importance for reconstructing the flow of metal during the Shang period and the organization of mass bronze production that underpinned the ritual world across the Yellow and Yangtze River. Based on a comparison of lead isotopic ratios between the Shang bronzes and geological data (ores such as galena), Jin proposed that Northeast Yunnan was where the “best match can be found between the Shang bronzes and local ores”, although he admitted himself that they are not completely the same (NE Yunnan is much less radiogenic than the Shang bronzes, see Jin, 2008: 21). This stimulated an extensive debate between archaeologists and geochemists. No other archaeological evidence has been found to support such long-distance communication between Yunnan and the Central Plains in the Bronze Age. Moreover, the earliest metallurgical evidence at Yunnan can only be traced to ca. 1000 BC (see below for updated chronology). The archaeological sites discovered after this pioneering isotopic work at Anyang, such as Sanxingdui, Jinsha, Xin’gan and Hanzhong, all subsequently yielded a significant proportion of bronzes with HRL. The finding of HRL at Sanxingdui and Jinshan in the Sichuan Basin, which is obviously closer to Yunnan than the other sites, seem to support the argument of a Yunnan provenance for this metal. Jin further proposed two routes via which metal could be moved from Yunnan to the Central Plains (Figure 2; Jin, 2008: 59–60, 147,285–289). The southern route was more favored as it takes advantage of the river system and also explains the wide distribution of HRL along the middle and lower range of the Yangtze River.
FIGURE 2. Key archaeological sites mentioned in the text and the proposed northern and southern routes of highly radiogenic lead (HRL) from Yunnan to the Central Plains.
A Yunnan provenance has, however, been challenged by new data from both geologists and archaeologists. More and more lead isotopic data has been published for lead ores in China, showing that a variety of regions can produce HRL, including not only Yunnan, but also the Zhongtiao Mountains (Tong, 2012; Qin et al., 2020), the Qinling Mountains (Chen et al., 2019; Zhangsun et al., 2021), metallic deposits along the Yangtze River (Peng et al., 1999), Northeast China (Wang et al., 2020), the Hexi Corridor (Chen et al., 2020; Liu et al., 2021b: Hexi type HRL) and Xinjiang (Liu et al., 2020a). Recently, even South Africa has been listed as one potential source of HRL for Chinese bronzes (Sun et al., 2016; Liu et al., 2018b; Liu et al., 2018c; Sun et al., 2018). Liu et al. (2018a) reviewed lead isotopic data for a wider range of lead-bearing materials (e.g., copper-based objects, glaze, glass, and pigments) and pointed out that HRL sources of lead were explored across Chinese antiquity. It is highly unlikely that one specific source of HRL could have been repetitively exploited over such a long period. However, what remains uncertain is how many sources were used within the chronological range of the Shang dynasty. Sources such as Zhongtiao, the middle and lower Yangtze and Qinling are favored by archaeologists as they already showed clear evidence for a mining industry during the Shang period. Data accumulated in the last ten years from environmental archaeology, field archaeology and archaeometallurgy allows us to revisit this debate from a fresh perspective and perhaps allows us to exclude some possible sources previously discussed.
Much research effort has been devoted to reconstructing the human-environment relationship in prehistoric Yunnan. The analytical data from the cores taken from the lakes of Erhai in Northwest Yunnan, Dian and Xingyun in Central Yunnan, are particularly interesting as their environmental data include the key metal concentrations (e.g., copper, lead, silver and zinc, Figure 3) (Dearing et al., 2008; Hillman et al., 2014; Hillman et al., 2015; Hillman et al., 2019). Dearing et al. (2008) discovered that in the core of Erhai the copper concentration started to rise around 1400 BC, and linked this to the metallurgical production around the Erhai Lake. The earliest evidence for metallurgical production in Yunnan comes from Haimenkou and Yinsuodao, which fall in the catchment area of Erhai Lake (see Earliest Archaeological Evidence at Yunnan Metallurgy). However, lead in the core remained at a rather low level until AD 400. Its rapid increase in the later period was probably due to local silver production, in which cupellation was employed to extract silver from lead ores, and lead was the major by-product released into the atmosphere. Hillman et al. (2015) present similar results for Erhai, showing a clear rise in copper concentration, from 26.8 ± 1 μg/g (2500–2000 BC) to 51.1 μg/g (ca. 1500 BC) but very low levels of lead. They also confirm a synchronous rise of lead and silver starting at around AD 200, which is earlier than the period proposed by Dearing et al. (2008). The Xingyun lake is located in central Yunnan and thus represents a different area of catchment. Although the chronology of the core data only extends to 1BC/AD, it still presents some useful data to infer the earlier metallurgy. The lead concentration remains at a very low level (ca. 10 μg/g) until the rise of the Nanzhao Kingdom (AD 738–AD 902), which was probably also due to an increasing scale of silver smelting. The low level of lead pollution in the Xingyun lake in the earlier stage might suggest the absence or very limited scale of metallurgical activities around the eastern part of Yunnan. This assumption is further confirmed by the most recent data from the Dian lake, which is around 60–80 km to the northwest of the Xingyu lake. It also shows a consistently low and stable level of lead pollution (ca. 10 μg/g) between 2000–1 BC/AD (Hillman et al., 2019).
FIGURE 3. Synthesis of metal pollution data in ancient Yunnan (modified from Hillman et al. (2019); Hillman et al. (2015); Hillman et al. (2014); Dearing et al. (2008)).
In addition to metal pollution, the other major environmental impact which could be caused by large-scale prehistoric metallurgy is deforestation, since both smelting, casting and mold-making (clay), sometimes also mining (use of fire to crack the rocks), required large amounts of charcoal as fuel. This is nevertheless not completely inevitable, depending on the scale of wood exploitation, and on the forest management practices such as coppicing. Charcoal cannot be made from large trunks, so charcoal production is usually associated with coppicing to manage the trees to produce small branches. Figure 4 summarizes the variation of pollen data from four lake cores. There appears no evident decrease in the tree pollen data around 2000–500 BC, suggesting the absence of large-scale deforestation in Yunnan. This implies that the possibility of discovering very large-scale metallurgical production in Yunnan dating to this period is very low. The data from the Erhai lake however shows a visible decrease in tree pollen data after 500 BC as well as an increase in poaceae pollen, suggesting stronger human activities probably due to expansion of settlement and agriculture. Whilst textual records in the Eastern Zhou and later periods do testify to metallurgical activities in Yunnan, it is also important to bear in mind that settlement and agricultural production could also result in a similar pattern in the pollen record.
FIGURE 4. Summary of pollen data for ancient Yunnan (Green lines: tree pollen percentage; Blue lines: poaceae pollen percentage; data source: Shen et al. (2006); Chen et al. (2014); Xiao et al. (2014); Wu et al. (2015); Xiao et al. (2015)).
The date of the earliest metallurgical production in Yunnan has been debated for decades. Although Jianchuan Haimenkou is widely regarded as the site with the earliest metallurgical evidence, the lack of systematic excavation and high-quality radiocarbon dates has prevented scholars from engaging in further discussion. Two small-scale excavations were carried out at Haimenkou in the years 1957 and 1978, respectively, but only two radiocarbon dates were published. Moreover, a huge chronological discrepancy of 500 years is indicated by these two dates, leading to deep confusion among archaeologists as to which period the archaeological records should be assigned (Min, 2013; Li and Min, 2016).
Local archaeologists decided to perform a much larger and more systematic excavation from 2008. A series of well-defined stratigraphies and high-quality radiocarbon dates are now published, allowing a much more detailed investigation into the development of metallurgy at Haimenkou. The local metal objects are markedly different from those bronze ritual vessels in the Central Plains or the standing figures and human heads at Sanxingdui. At Haimenkou, the objects are all in small sizes, including a bell, knives, chisels, awls, arrows and bracelets. A piece of stone mold has also been discovered, indicating a different metallurgical tradition to that elsewhere in China. The new excavation identified ten stratigraphic layers in total, and the evidence of metallurgy (one bell, one copper tube, one needle, one chisel, one copper block, one awl and one knife) starts in layer VI. Surprisingly, two iron objects were also recovered from the same layer (Min, 2009).
Defining the chronology of the layer VI is however not an easy task. Li and Min (2016) argue that the metal objects in layer VI were most likely dated after the Western Zhou, with the Spring and Autumn Period (ca. 770–475 BC) as the terminus post quem and the early Warring States (ca. 475–221 BC) as the terminus ante quem. Their argument is primarily based on the iron pieces (one small iron circle and one small iron bracelet), the “alloying technology” of the copper objects and one specific radiocarbon date. Metallographic analysis shows that the iron objects were smelted iron rather than meteoritic, and a similar cast iron technology was encountered at the Guo state cemetery at Sanmenxia, which can be safely dated to the early Western Zhou at the earliest (ca.11th–10th century BC). The copper block contains 5.3% antimony, which is rare compared to the prevailing tin-bronze (Cu-Sn) or leaded bronze (Cu-Sn-Pb) elsewhere in China. They argue that such “alloying technology” is more likely to be dated to later periods such as the Warring States, with no further evidence being provided. Moreover, one millet grain discovered in layer VI is radiocarbon dated to the early Warring States (730 BC–410 BC, 68.2% probability; 760 BC–400 BC, 95.4% probability).
The occurrence of Cu-Sb should not be considered on its own as evidence for dating. As Li and Min (2016) pointed out, smelting of some natural mixed copper ores such as tetrahedrite Cu12Sb4S13 could also result in such an unusual chemical composition. The word “alloying technology” is sometimes used too casually in archaeometallurgical literature, which is ultimately rooted in the modern industrial metallurgy in which many archaeometallurgists were trained. It is a common assumption in the Chinese literature that any element beyond 2% in the metal can be considered as an intentional addition to copper (thus an alloying element), added in order to modify the physical properties of the finished objects. This is potentially meaningful if one is dealing with a large number of analyses, but should by no means be treated as a golden rule when applied to a single object. A similar case can be found in the early Shang metal assemblage at Hanzhong, where a variety of rare chemical combinations (Cu-As, Cu-Sb, Cu-As-Ni) can be found (Chen et al., 2009; Mei et al., 2009; Chen et al., 2016). This is almost certainly to do with local types of ores rather than artificial alloying. A more detailed discussion of alloying technology/practice can be found in Pollard et al., 2018, Pollard et al., 2019 (chapter 3 and 2019).
The radiocarbon date from the millet seems to be the most compelling evidence. However, three other radiocarbon dates from the same layer suggest a rather earlier chronology of around 1300–1100 BC. Figure 5 shows a new Bayesian model for the Haimenkou chronology, which integrates not only the radiocarbon dates but also the stratigraphy. Given the fact that two samples are charcoal from unknown species (therefore of unknown ring position relative to the outer ring), we chose to apply Charcoal Plus Outlier Model to these samples, in order to minimize the potential effect of inbuilt-age (Dee and Bronk Ramsey, 2014). For the other samples, we have applied a General_Outlier model to reveal possible outliers due to laboratory analysis or erroneous stratigraphy (Bronk Ramsey, 2009). It is rather obvious that the posterior range of the date from the layer VI millet (BA081097) is far later than the other three dates and is completely inconsistent with the stratigraphic sequence (Figure 5B). Bayesian analysis also confirms that the probability for this sample to be an outlier is extremely high (ca. 88%). As all the samples followed the same analytical protocol, it is highly unlikely that this offset is due to laboratory analysis. We think it is most likely to be an intrusion from upper layers, therefore giving a much later date. In this light, it is also necessary to reconsider whether or not the two iron pieces were also intrusions.
FIGURE 5. Remodeling of the radiocarbon dates from Haimenkou ((A). the modeled start and end date for each stratigraphic layer at Haimenkou; (B). the four radiocarbon dates assigned to the Layer VI; (C). some metal objects recovered at Haimenkou; data source: Li and Min (2016)).
After careful investigation into the complexity associated with chronology, it is reasonable to argue that so far the earliest metallurgical production at Haimenkou can be dated to around 1200 BC. This is still approximately three hundred years later than the first use of HRL in the Central Plains. The most recent excavation shows a scale of metallurgical activity at Haimenkou that is far from being equivalent to any major metal producers or consumers along the Yangtze or the Yellow River. All the recovered metal objects are small in size, designed for daily use or personal ornaments. No evidence of large metal production/consumption has been found. This raises the question that, if Yunnan was the producer of HRL which supported the metal production at Zhengzhou, Anyang, Sanxingdui, Hanzhong, Panlongcheng, Xin’gan and many others, why the contemporary metal production within Yunnan was still so primitive.
The alloying pattern can also throw some extra light on to this issue. Figure 6 compares the distribution of lead and tin of the objects from Haimenkou (Cui and Wu, 2008; Li and Min, 2016), Zhengzhou and Anyang. Approximately 90% of Haimenkou objects (dated to all periods from ca. 1200 BC to 200 BC) contain lead at no more than 3%. The majority of objects are tin-bronzes. However, almost all the objects at Zhengzhou and Anyang are leaded bronze, except for those of Anyang Phase II, which is well-known for high-tin bronze (Zhao, 2004). There has been a long-standing question concerning the radiogenic lead, namely, whether the highly radiogenic signal derives from the lead or the copper. Some objects without added lead, for instance, less than 1–2%, do have HRL, so they may come from a copper source containing HRL lead, the majority have added lead which must be HRL (Jin, 2008; Jin et al., 2017; Liu et al., 2020c). If HRL did indeed originate from Yunnan, it is then hard to comprehend why lead was not used to alloy local objects.
Whilst a large number of publications have focused on comparative studies of lead isotopic data among various metal assemblages, there is still very little attention paid to the bronzes from Southeast Asia, which are arguably more relevant to interpret the Yunnan bronzes (Higham et al., 2011; Higham et al., 2020; Yao et al., 2020; Higham and Cawte, 2021). Yunnan and Southeast Asia bear a range of resemblances in terms of material culture. Both regions lie in the crunch area of Himalaya, which is very active in geological history, leading to many folds therefore rich metal deposits such as copper, tin and lead (Zaw et al., 2014; Cheng et al., 2016). Thanks to the decades of research made by Pryce and his colleagues (Pryce et al., 2011; Pryce et al., 2014; Pryce et al., 2018), 466 sets of lead isotopic ratios have been published for the metal assemblages excavated in Southeast Asia, ranging from 1000 BC to 700 AD. Figure 7 summarizes almost all the lead isotopic data for bronzes from Bronze Age Southeast Asia and China, with Yunnan being highlighted. It is important to observe that Yunnan bronzes and those from Southeast Asia are directly comparable in terms of both typology and lead isotopic ratios, reflecting mutual exchange of metal and similar geological background. Nonetheless, neither of them can be matched to HRL. Intriguingly, one copper adze from the site of Wangjiadun in Yunnan (around 1200–1050 BC) shows a typical Shang HRL (Cui and Wu, 2008). Without further information, it is difficult to tell whether this was made from recycling of objects in other regions.
FIGURE 7. Comparison of lead isotopic data from copper-based artifacts from Yunnan and SE Asia (data from https://flame.arch.ox.ac.uk/, see also Perucchetti et al. (2021)).
The discovery of HRL is one of the most crucial keys to reconstructing the supply network and management system of the metal resources during the Shang period. However, its unresolved issue of provenance makes the whole discussion like a floating chronology, which still lacks an absolute chronological point to be anchored to the real calendar years. In addition to the commonly used archaeometallurgical approaches, evidence produced by environmental archaeology can be equally thought-provoking. Metallurgical production should reshape as well as adapt to the local environment. Both environmental archaeology and archaeometallurgical research in China have made marked progress in their own field in the last two decades or so. Each discipline has produced crucial and stimulating results of interest to the other. It becomes increasingly meaningful and practical for scholars to carry out more cross-disciplinary cooperation to resolve many shared issues that combine environmental studies and archaeometallurgy. Reviewing the recent published environmental records, together with new excavation results, radiocarbon chronology and alloying practice, reveals no further evidence to support Yunnan as the source of HRL. Rather, environmental archaeology suggests no large-scale metallurgical activities at Yunnan until the historical period. Cooperation between environmental archaeology and archaeometallurgy in China is still surrounded by various issues. It is imperative to establish systematic theoretical framework in order to trigger more dynamic dialogue between the two subjects and more environmental records should be collected and analyzed for more specific questions in archaeometallurgy.
The original contributions presented in the study are included in the article/Supplementary Material, further inquiries can be directed to the corresponding author.
RL design the research and wrote the whole paper; AP edited the manuscript; FL produced Figure 4; LH produced Figures 2, 3; All the authors contributed to the discussion.
This work is funded by European Research Council Horizon 2020 advanced project FLAME (Flow of Ancient Metal Across Eurasia, 670010).
The authors declare that the research was conducted in the absence of any commercial or financial relationships that could be construed as a potential conflict of interest.
We are grateful for the comments from the two reviewers and the platform provided by the Northwest University 111 Project of Ministry of Education of China (D18004) for us to perform this research.
Abbott, M. B., and Wolfe, P. A. (2003). Intensive Pre-incan Metallurgy Recorded by Lake Sediments from the Bolivian Andes. Science 301 (5641), 1893–1895. doi:10.1126/science.1087806
Bagley, R. (1999). “Shang Archaeology,” in The Cambridge History of Ancient China: From the Origins of Civilization to 221 B.C. Editors E. L. Shaughnessy, and M. Loewe (Cambridge: Cambridge University Press), 124–231. doi:10.1017/chol9780521470308.005
Bronk Ramsey, C. (2009). Dealing with Outliers and Offsets in Radiocarbon Dating. Radiocarbon 51(3) 1023–1045. doi:10.1017/S0033822200034093
Chen, F., Chen, X., Chen, J., Zhou, A., Wu, D., Tang, L., et al. (2014). Holocene Vegetation History, Precipitation Changes and Indian Summer Monsoon Evolution Documented from Sediments of Xingyun Lake, South-West China. J. Quat. Sci. 29 (7), 661–674. doi:10.1002/jqs.2735
Chen, G., Cui, Y., Liu, R., Wang, H., Yang, Y., Pollard, A. M., et al. (2020). Lead Isotopic Analyses of Copper Ores in the Early Bronze Age central Hexi Corridor, north‐west China. Archaeometry 62 (5), 952–964. doi:10.1111/arcm.12566
Chen, G. (2016). Rediscussion on the Metallurgycial Remians at Erlitou. Zhongyuan wenwu 3, 35–44. (in Chinese).
Chen, K., Mei, J., Rehren, T., Liu, S., Yang, W., Martinón-Torres, M., et al. (2019). Hanzhong Bronzes and Highly Radiogenic lead in Shang Period China. J. Archaeological Sci. 101, 131–139. doi:10.1016/j.jas.2018.11.010
Chen, K., Mei, J., Rehren, T., and Zhao, C. (2016). Indigenous Production and Interregional Exchange: Late Second-Millennium BC Bronzes from the Hanzhong basin, China. Antiquity 90 (351), 665–678. doi:10.15184/aqy.2016.94
Chen, K., Rehren, T., Mei, J., and Zhao, C. (2009). Special Alloys from Remote Frontiers of the Shang Kingdom: Scientific Study of the Hanzhong Bronzes from Southwest Shaanxi, China. J. Archaeological Sci. 36(10), 2108–2118. doi:10.1016/j.jas.2009.04.016
Cheng, Y., Mao, J., and Liu, P. (2016). Geodynamic Setting of Late Cretaceous Sn-W Mineralization in southeastern Yunnan and Northeastern Vietnam. Solid Earth Sci. 1, 79–88. doi:10.1016/j.sesci.2016.12.001
Chernykh, E. N. (1992). Ancient Metallurgy in the USSR: The Early Metal Age. Cambridge: Cambridge University Press.
Cui, J., and Wu, X. (2008). Lead Isotopic Archaeology – Case Studies of Bronzes at Yunnan in China and Veitanam. Beijing: Wenwu press (in Chinese).
Dearing, J. A., Jones, R. T., Shen, J., Yang, X., Boyle, J. F., Foster, G. C., et al. (2008). Using Multiple Archives to Understand Past and Present Climate-Human-Environment Interactions: the lake Erhai Catchment, Yunnan Province, China. J. Paleolimnol 40, 3–31. doi:10.1007/s10933-007-9182-2
Dee, M. W., and Ramsey, C. B. (2014). High-precision Bayesian Modeling of Samples Susceptible to Inbuilt Age. Radiocarbon 56 (1), 83–94. doi:10.2458/56.16685
Higham, C. F. W., and Cawte, H. (2021). Bronze Metallurgy in Southeast Asia with Particular Reference to Northeast Thailand. J. World Prehist 34, 1–46. doi:10.1007/s10963-020-09151-3
Higham, C., Higham, T., Ciarla, R., Douka, K., Kijngam, A., and Rispoli, F. (2011). The Origins of the Bronze Age of Southeast Asia. J. World Prehist 24, 227–274. doi:10.1007/s10963-011-9054-6
Higham, T. F. G., Weiss, A. D., Higham, C. F. W., Ramsey, C. B., D'Alpoim Guedes, J., Hanson, S., et al. (2020). A Prehistoric Copper-Production centre in central Thailand: Its Dating and Wider Implications. Antiquity 94 (376), 948–965. doi:10.15184/aqy.2020.120
Hillman, A. L., Abbott, M. B., Yu, J., Bain, D. J., and Chiou-Peng, T. (2015). Environmental Legacy of Copper Metallurgy and Mongol Silver Smelting Recorded in Yunnan Lake Sediments. Environ. Sci. Technol. 49, 3349–3357. doi:10.1021/es504934r
Hillman, A. L., Yao, A., Abbott, M. B., and Bain, D. J. (2019). Two Millennia of Anthropogenic Landscape Modification and Nutrient Loading at Dian Lake, Yunnan Province, China. The Holocene 29 (3), 505–517. doi:10.1177/0959683618816504
Hillman, A. L., Yu, J., Abbott, M. B., Cooke, C. A., Bain, D. J., and Steinman, B. A. (2014). Rapid Environmental Change during Dynastic Transitions in Yunnan Province, China. Quat. Sci. Rev. 98, 24–32. doi:10.1016/j.quascirev.2014.05.019
Hsu, Y.-K., O'Sullivan, R., and Li, H. (2021). Sources of Western Zhou lead: a New Understanding of Chinese Bronze Age Supply Networks. Archaeological Anthropological Sci. 13 (2), 1–15. doi:10.1007/s12520-021-01279-3
Hsu, Y.-K., and Sabatini, B. J. (2019). A Geochemical Characterization of lead Ores in China: An Isotope Database for Provenancing Archaeological Materials. PLOS ONE 14 (4), e0215973. doi:10.1371/journal.pone.0215973
Hsu, Y.-k. (2016). The Dynamic Flow of Copper and Copper Alloys across the Prehistoric Eurasian Steppe from 2000 to 300 BCE. PhD, University of Oxford.
Iles, L. (2016). The Role of Metallurgy in Transforming Global Forests. J. Archaeol Method Theor. 23, 1219–1241. doi:10.1007/s10816-015-9266-7
Jin, Z. (1987). “The Central Plain during the Late Shang Period,” in Sources of Metals for the Bronze Production in Lead Isotope Archaeology in China (China): Press of Univeristy of Science and Technology), 292–302.Hefei
Jin, Z. (2008). Lead Isotopic Archaeology in China. Hefei: Press of University of Science and Technology (in Chinese).
Jin, Z., Liu, R., Rawson, J., and Pollard, A. M. (2017). Revisiting lead Isotope Data in Shang and Western Zhou Bronzes. Antiquity 91 (360), 1574–1587. doi:10.15184/aqy.2017.149
Jin, Z., Zheng, G., Hirao, Y., and Hayakawa, Y. (2001). “Lead Isotopic Ratios of Early Chinee Bronzes,” in Circulation of Metal in Ancient East Asia. Editors Y. Hirao, J. Enomoto, and H. Takahama (Tokyo, Japan: Tsuruyamado), 295–304. (in Chinese).
Ledderose, L. (2000). Ten Thousand Things: Module and Mass Production in Chinese Art. Princeton: Princeton University Press.
Li, S., Joseph, E., and Min, Q. (2016). Remote Sensing of Ground-Level PM2.5 Combining AOD and Backscattering Profile. Remote Sensing Environ. 183 (3), 120–128. doi:10.1016/j.rse.2016.05.025 (in Chinese).
Li, Y. (2014). Preliminary Exploration of the Broad Organization of Metallurgy in the Central Plains and Northern China. Zhongguo Wenwubao Wenwu Kaogu Zhoukan 5, 1–4. (in Chinese Newspaper).
Linduff, K. M., and Mei, J. (2014). “Metallurgy in Ancient Eastern Asia: Retrospect and Prospects,” in Archaeometallurgy in Global Perspective: Methods and Syntheses. Editors B. W. Roberts, and C. P. Thornton (New York: Springer), 785–803. doi:10.1007/978-1-4614-9017-3_27
Liu, C., Liu, R., Zhou, P., Lu, C., Yang, Z., Pollard, A. M., et al. (2020a). Metallurgy at the Crossroads: New Analyses of Copper‐based Objects at Tianshanbeilu, Eastern Xinjiang, China. Acta Geologica Sinica ‐ English Edition 94 (3), 594–602. doi:10.1111/1755-6724.14531
Liu, L., and Chen, X. (2009). State Formation in Early China. London: Duckworth. doi:10.1093/oxfordhb/9780199271016.013.0020
Liu, R., Bray, P., Pollard, A. M., and Hommel, P. (2015). Chemical Analysis of Ancient Chinese Copper-Based Objects: Past, Present and Future. Archaeological Res. Asia 3, 1–8. doi:10.1016/j.ara.2015.04.002
Liu, R. (2016). Capturing Changes: Applying the Oxford System to Further Understand the Movement of Metal in Shang China. PhD, University of Oxford.
Liu, R., Hsu, Y.-K., Pollard, A. M., and Chen, G. (2021). A New Perspective towards the Debate on Highly Radiogenic lead in Chinese Archaeometallurgy. Archaeol Anthropol. Sci. 13 (2), 1–7. doi:10.1007/s12520-021-01280-w
Liu, R., Pollard, A. M., Cao, Q., Liu, C., Sainsbury, V., Howarth, P., et al. (2020b). Social Hierarchy and the Choice of Metal Recycling at Anyang, the Last Capital of Bronze Age Shang China. Scientnfic Rep. 10, 18794. doi:10.1038/s41598-020-75920-x
Liu, R., Pollard, A. M., Liu, C., and Rawson, J. (2020c). Every Cloud Has a Silver Lining: Using Silver Concentration to Identify the Number of Sources of Lead Used in Shang Dynasty Bronzes. Acta Geologica Sinica ‐ English Edition 94 (3), 585–593. doi:10.1111/1755-6724.14530
Liu, R., Rawson, J., and Pollard, A. M. (2018a). Beyond Ritual Bronzes: Identifying Multiple Sources of Highly Radiogenic lead across Chinese History. Sci. Rep. 8, 11770. doi:10.1038/s41598-018-30275-2
Liu, S., Chen, K. L., Rehren, T., Mei, J. J., Chen, J. L., Liu, Y., et al. (2018c). Did China Import Metals from Africa in the Bronze Age? Archaeometry 60 (1), 105–117. doi:10.1111/arcm.12352
Liu, S. R., Chen, K. L., Rehren, T., Mei, J. J., Chen, J. L., Liu, Y., et al. (2018b). Lead isotope and metal source of Shang bronzes: a response to Sun et al .'s comments. Archaeometry 60 (5), 1040–1044. doi:10.1111/arcm.12411
McConnell, J. R., Wilson, A. I., Stohl, A., Arienzo, M. M., Chellman, N. J., Eckhardt, S., et al. (2018). Lead Pollution Recorded in Greenland Ice Indicates European Emissions Tracked Plagues, Wars, and imperial Expansion during Antiquity. Proc. Natl. Acad. Sci. USA 115 (22), 5726–5731. doi:10.1073/pnas.1721818115
Mei, J., Chen, K., and Cao, W. (2009). Scientific Examination of Shang-Dynasty Bronzes from Hanzhong, Shaanxi Province, China. J. Archaeological Sci. 36(9), 1881–1891. doi:10.1016/j.jas.2009.04.017
Mei, J. (2009). “Early Metallurgy and Socio-Cultural Complexity: Archaeological Discoveries in Northwest China,” in Social Complexity in Prehistoric Eurasia: Monuments, Metals, and Mobility. Editors B. K. Hanks, and K. M. Linduff (Cambridge: Cambridge University Press), 2108–2118.
Min, R. (2013). Synthesis of Archaeological Excavations at Haimenkou Jianchuan. Xuyuan (15), 6–9. (in Chinese).
Min, R. (2009). The Third Excavation at Haimenkou, Jianchuan, Yunnan. Kaogu (8), 3–22. (in Chinese).
Monge, G., Jimenez-Espejo, F. J., García-Alix, A., Martínez-Ruiz, F., Mattielli, N., Finlayson, C., et al. (2015). Earliest Evidence of Pollution by Heavy Metals in Archaeological Sites. Scientific Rep. 5, 14252. doi:10.1038/srep14252
Nriagu, J. O. (1972). A Hisotry of Global Metal Pollution. Science 272, 223. doi:10.2475/ajs.272.5.476
Peng, Z., Liu, Y., Liu, S., and Hua, J. (1999). Preliminary of the Provenance of Bronzes and Raw Copper and lead Materials in Southern China. Ziran kexueshi yanjiu 18 (3), 241–249. (in Chinese).
Perucchetti, L., Bray, P., Felicetti, A., Sainsbury, V., Howarth, P., Saunders, M. K., et al. (2021). FLAME‐D Database: An Integrated System for the Study of Archaeometallurgy. Archaeometry 63 (3), 651–667. doi:10.1111/arcm.12616
Pollard, A. M., Bray, P., Hommel, P., Hsu, Y.-K., Liu, R., and Rawson, J. (2017a). Bronze Age Metal Circulation in China. Antiquity 91 (357), 674–687. doi:10.15184/aqy.2017.45
Pollard, A. M., Bray, P., Hommel, P., Hsu, Y.-K., Liu, R., and Rawson, J. (2017b). Further Understanding of Chinese Bronzes by the Oxford System. Kaogu (1), 95–106.
Pollard, A. M., Bray, P., Hommel, P., Liu, R., Pouncett, J., Saunders, M., et al. (2018). Beyond Provenance : New Approaches to Interpreting the Chemistry of Archaeological Copper Alloys. Belgium, Leuven: Leuven University Press. doi:10.2307/j.ctv7xbs5r
Pollard, A. M., Heron, C., and Armitage, R. A. (2017c). Archaeological Chemistry. London, United Kingdom: Royal Society of Chemistry: Royal Society of Chemistry.
Pollard, A. M., Liu, R., Rawson, J., and Tang, X. (2019). From Alloy Composition to Alloying Practice: Chinese Bronzes. Archaeometry 61 (1), 70–82. doi:10.1111/arcm.12415
Pryce, T. O., Baron, S., Bellina, B. H. M., Bellwood, P. S., Chang, N., Chattopadhyay, P., et al. (2014). More Questions Than Answers: the Southeast Asian Lead Isotope Project 2009-2012. J. archaeological Sci. 42, 273–294. doi:10.1016/j.jas.2013.08.024
Pryce, T. O., Htwe, K. M. M., Georgakopoulou, M., Martin, T., Vega, E., Rehren, T., et al. (2018). Metallurgical Traditions and Metal Exchange Networks in Late Prehistoric central Myanmar, C. 1000 BC to C. AD 500. Archaeol Anthropol. Sci. 10 (5), 1087–1109. doi:10.1007/s12520-016-0436-7
Pryce, T. O., Pollard, M., Martinón-torres, M., Pigott, V. C., and Pernicka, E. (2011). Southeast Asia's First Isotopically Defined Prehistoric Copper Production System: when Did Extractive Metallurgy Begin in the Khao Wong Prachan Valley of Central Thailand? Archaeometry 53 (1), 146–163. doi:10.1111/j.1475-4754.2010.00527.x
Qin, Y., Wei, G., and HUang, Q. (2020). The Provenance of Yin-Shang Bronzes with Highly Radiogenic lead Isotopes. Archaeological Anthropological Sci. 12, 1–10.
Qiu, L., Zhuang, X., HUang, X., Liu, P., and Zhao, X. (2019). Study on Heavy Metals' Influence on Buried Cultural Relics in Soil of an Archaeological SiteEnviron. Tech. 42. Environmental Technology Online available (12), 1955–1966. doi:10.1080/09593330.2019.1684569
Rawson, J. (2017a). China and the Steppe: Reception and Resistance. Antiquity 91 (356), 375–388. doi:10.15184/aqy.2016.276
Rawson, J. (2017b). Shimao and Erlitou: New Perspectives on the Origins of the Bronze Industry in Central China. Antiquity Project Gallery 91. doi:10.15184/aqy.2016.234
Rawson, J. (1999). “Western Zhou Archaeology,” in Western Zhou Archaeology " in the Cambridge History of Ancient China : From the Origins of Civilization to 221 B.C. Editors E. L. Shaughnessy, and M. Loewe (Cambridge: Cambridge University Press), 352–449. doi:10.1017/chol9780521470308.008
Rehren, T., and Pernicka, E. (2008). Coins, Artefacts and Isotopes-Archaeometallurgy and Archaeometry. Archaeometry 50 (2), 232–248. doi:10.1111/j.1475-4754.2008.00389.x
Roberts, B. W., and Thornton, C. P. (2014). Archaeometallurgy in Global Perspective: Methods and Syntheses. Springer Science & Business Media.
Roberts, B. W., Thornton, C. P., and Pigott, V. C. (2009). Development of Metallurgy in Eurasia. Antiquity 83 (322), 1012–1022. doi:10.1017/s0003598x00099312
Russell, R. D., and Farquhar, R. M. (1960). Lead Isotopes in Geology. New York: Interscience Publishers.
Shen, J., Jones, R. T., Yang, X., Dearing, J. A., and Wang, S. (2006). The Holocene Vegetation History of Lake Erhai, Yunnan Province Southwestern China: the Role of Climate and Human Forcings. The Holocene 16 (2), 265–276. doi:10.1191/0959683606hl923rp
Storozum, M. J., Qin, Z., Wang, Y., and Liu, H. (2020). Buried Soils as Archives of Paleo-Pollution in the North China Plain. Anthropocene 31, 1–16. doi:10.1016/j.ancene.2020.100251
Sun, W.-d., Zhang, L.-p., Guo, J., Li, C.-y., Jiang, Y.-h., Zartman, R. E., et al. (2016). Origin of the Mysterious Yin-Shang Bronzes in China Indicated by lead Isotopes. Sci. Rep. 6, 23304. doi:10.1038/srep23304
Sun, W.-D., Zhang, L.-P., Guo, J., Li, C.-Y., Jiang, Y.-H., and Zhang, Z.-F. (2018). Comment on 'Did China Import Metals from Africa in the Bronze Age?' Archaeometry 60 (5), 1036–1039. doi:10.1111/arcm.12412
The Institute of Archaeology, C. (1980). Yinxu Fuhao Tomb. Beijing: Cultural Relics Publishing House (in Chinese).
Thevenon, F., Guedron, S., Chiaradia, M., Loizeau, J.-L., and Pote, J. (2011). (Pre-) Historic Changes in Natural and Anthropogenic Heavy Metals Deposition Inferred from Two Contrasting Swiss Alpine Lakes. Quat. Sci. Rev. 30 (1-2), 224–233. doi:10.1016/j.quascirev.2010.10.013
Tong, W. (2012). “Yuanqu Shang Site and the Copper Resources in the Zhongtiao Mountains,” in Kaoguxue Yanjiu (jiu). Editor B.U. School of Archaeology and Museology (Beijing: Science Press), 346–361. ((in Chinese).
Wang, Y., Mei, J., Li, Y., Chen, K., Cao, J. e., and Dang, Y. (2020). Research on the Ore Sources of Some Bronze Objects from the Lower Xiajiadian Culture Sites. Sci. Conservation Archaeology 3, 1–7. (in Chinese).
Wu, D., Zhou, A., Liu, J., Chen, X., Wei, H., Sun, H., et al. (2015). Changing Intensity of Human Activity over the Last 2,000 Years Recorded by the Magnetic Characteristics of Sediments from Xingyun Lake, Yunnan, China. J. Paleolimnol 53 (1), 47–60. doi:10.1007/s10933-014-9806-2
Xiao, X., Haberle, S. G., Shen, J., Yang, X., Han, Y., Zhang, E., et al. (2014). Latest Pleistocene and Holocene Vegetation and Climate History Inferred from an alpine Lacustrine Record, Northwestern Yunnan Province, Southwestern China. Quat. Sci. Rev. 86, 35–48. doi:10.1016/j.quascirev.2013.12.023
Xiao, X., Shen, J. I., Haberle, S. G., Han, Y., Xue, B., Zhang, E., et al. (2015). Vegetation, Fire, and Climate History during the Last 18 500 Cal a BP in South-Western Yunnan Province, China. J. Quat. Sci. 30 (8), 859–869. doi:10.1002/jqs.2824
Xu, M. X., H, W. S., Zhou, S. L., Liao, F. Q., Ma, C. M., and Zhu, C. (2011). Hyperspectral Reflectance Models for Retrieving Heavy Metal Content: Application in the Archaeological Soil. J. Infrared Millimeter Waves 30 (2), 109–114. (in Chinese with English abstract).
Yao, A., Darre, V., Jiang, Z., Lam, W., and Wei, Y. (2020). Bridging the Time gap in the Bronze Age of Southeast Asia and Southwest China. Archaeological Res. Asia 22, 1–19. doi:10.1016/j.ara.2020.100189
Zaw, K., Meffre, S., Lai, C. K., Burrett, C., Santosh, M., Graham, I., et al. (2014). Tectonics and Metallogeny of mainland Southeast Asia—A Review and Contribution. Gondwana Res. 26 (1), 5–30.
Zhang, C. (2014). “Erligang a Perepctive from Panlongcheng,” in Art and Archaeology of the Erligang Civilization. Editors K. Steinke, and D. C. Y. Ching (Princeton: Princeton University Press), 51–63.
Zhang, S., Yang, Y., Storozum, M. J., Li, H., Cui, Y., and Dong, G. (2017). Copper Smelting and Sediment Pollution in Bronze Age China: a Case Study in the Hexi Corridor, Northwest China. Catena 156, 92–101. doi:10.1016/j.catena.2017.04.001
Zhangsun, Y. Z., Jin, Z. Y., Feng, F. Z., Tian, J. H., Wang, H. G., Zhao, C. C., et al. (2021). Revisiting the lead Isotopic Compositions of the Shang Bronzes at Hanzhong and the New Hypothesis of Qinling as the Source of Highly Radiogenic lead. Archaeometry 63 (1), 122–141. doi:10.1111/arcm.12630
Zhao, C. (2004). Chemical Analysis of Bronzes at Anyang. Kaoguxue jikan (15), 243–268. (in Chinese).
Keywords: environmental archaeology, archaeometallurgy, Yunnan, highly radiogenic lead, metal pollution
Citation: Liu R, Pollard AM, Lv F, Huan L, Zhang S and Ma M (2021) Two Sides of the Same Coin: A Combination of Archaeometallurgy and Environmental Archaeology to Re-Examine the Hypothesis of Yunnan as the Source of Highly Radiogenic Lead in Early Dynastic China. Front. Earth Sci. 9:662172. doi: 10.3389/feart.2021.662172
Received: 31 January 2021; Accepted: 11 May 2021;
Published: 04 June 2021.
Edited by:
Jade D'Alpoim Guedes, University of California, San Diego, United StatesReviewed by:
Nadia Solovieva, University College London, United KingdomCopyright © 2021 Liu, Pollard, Lv, Huan, Zhang and Ma. This is an open-access article distributed under the terms of the Creative Commons Attribution License (CC BY). The use, distribution or reproduction in other forums is permitted, provided the original author(s) and the copyright owner(s) are credited and that the original publication in this journal is cited, in accordance with accepted academic practice. No use, distribution or reproduction is permitted which does not comply with these terms.
*Correspondence: Ruiliang Liu, cnVpbGlhbmcubGl1QGFyY2gub3guYWMudWs=
Disclaimer: All claims expressed in this article are solely those of the authors and do not necessarily represent those of their affiliated organizations, or those of the publisher, the editors and the reviewers. Any product that may be evaluated in this article or claim that may be made by its manufacturer is not guaranteed or endorsed by the publisher.
Research integrity at Frontiers
Learn more about the work of our research integrity team to safeguard the quality of each article we publish.