- 1National and Local Joint Engineering Research Center of Shale Gas Exploration and Development, Chongqing Institute of Geology and Mineral Resources, Chongqing, China
- 2Key Laboratory of Shale Gas Exploration, Ministry of Natural Resources, Chongqing Institute of Geology and Mineral Resources, Chongqing, China
- 3Chongqing Key Laboratory of Complex Oil and Gas Exploration and Development, Chongqing, China
- 4Chongqing Institute of Geological Survey, Chongqing, China
- 5Chongqing Shale Gas Exploration and Development Company Limited, Chongqing, China
- 6China Huaneng Group Co., Ltd., Chongqing, China
Compared with the overpressure conditions of shale reservoirs in the Jiaoshiba area, exploring the controlling factors of changes in shale reservoir physical properties under normal-pressure accumulation is of great significance to shale gas exploration. To achieve this, X-ray powder diffraction, nitrogen adsorption, and scanning electron microscopy were carried out to determine mineral content, permeability, porosity, and pore structure for well core and outcrop samples of the Wufeng–Longmaxi Formation in southeastern Chongqing, China. Field observations and drilling cores revealed abundant structural fractures in southeastern Chongqing, offering potential main storage space for shale gas. Findings also determined that high-quality source rocks are located in the lower part of the Wufeng–Longmaxi Formation, which features low porosity and low permeability, yet existing structural fractures could potentially improve permeability to a certain extent. The main factors controlling the shale reservoir with the above characteristics include the depositional environment, mineral components, total organic carbon content, and tectonic movement. The deep-water anoxic depositional environment was conducive to the complete preservation of organic matter and sedimentation of the biogenic siliceous minerals, which had a strong effect on improving the porosity of organic matter and brittleness of the rocks. The high content of quartz was found to improve rock compression resistance and brittleness, and increasing clay proportion was found to enhance the interlayer pores of clay minerals. Total organic carbon content, specific surface area, pore volume, and fracture development were all found to have direct contributions to shale gas entrapment. Overall, the most important factor resulting in normal-pressure accumulation in southeastern Chongqing was the intense tectonic movements since the Late Jurassic period which destroyed the original overpressure accumulation conditions.
Introduction
As an unconventional gas, shale gas is of great significance to the global energy supply. China’s shale gas exploration blocks are concentrated in the Upper Yangtze Fuling, Weiyuan, Pengshui, and Changning areas. The main strata for exploration and development are located in the Upper Ordovician Wufeng Formation (O3w)–Lower Silurian Longmaxi Formation (S1l), which are rich in organic black shale and have been commercialized (Wang et al., 2013; Guo and Zhang, 2014; He Z. et al., 2017). Among them, the Fuling gas field has reserves amounting to 7,685.16 × 108 m3, making it the second-largest shale gas field in the world after one field located in North America (Jiang et al., 2016; Dai et al., 2017). Therefore, the black shale of the Wufeng–Longmaxi Formation in the Upper Yangtze District has good prospects for exploration and development. Previous research on this area’s black shale has mainly been concentrated on the Sichuan Basin. The Changning–Weiyuan block is mainly composed of organic-rich black shales enriched in graptolites, brachiopods, and other fossils, along with good organic matter types, relatively high thermal maturity, moderate content of brittle mineral components in shale matrix, and the presence of diverse types of pores and fractures, all of which make the Longmaxi Formation shale favorable for shale gas accumulation (Pu et al., 2010; Pan et al., 2016). Most research into black shale has focused on the Jiaoshiba shale gas reservoir in Fuling, southeastern Sichuan, including studies on the division of graptolite belts, sedimentary responses, shale distribution laws, accumulation models, and structural influences (Su et al., 2007; Mu et al., 2011; Guo, 2014; Guo et al., 2014a; Chen et al., 2015; Wang, 2015; Nie et al., 2016; Jin et al., 2018; Zhou et al., 2018; Gao et al., 2019; Gou and Xu, 2019; Liang et al., 2018; Lv et al., 2019, 2020; Tang et al., 2019; Wang Q. et al., 2019; Wang R. Y. et al., 2019; Wang et al., 2020). Overall conclusions agree that Jiaoshiba experiences overpressure, with a pressure coefficient of more than 1.74 and a burial depth of >6,200 m. The reservoir space mainly consists of pores, and the thermal evolution of organic matter hydrocarbon-generation pressurization is the main mechanism of overpressure formation (Gao et al., 2019; Wang Q. et al., 2019). Gao et al. (2019) divided the evolution of the Jiaoshiba overpressure conditions into four stages: (i) the rapid pressurization stage caused by the generation of thermal catalytic dry gas, (ii) the rapid pressure release stage, (iii) the overpressure preservation stage, and (iv) the slow-release pressure stage. Regarding this last stage, researchers have suggested that pressure has been released since the initial overpressure was first formed. Guo and Zhang (2014) proposed that the shale gas in the Longmaxi Formation of Jiaoshiba has undergone three stages of accumulation: (i) the early-stage formation of a small amount of carbohydrates, (ii) deep burial and massive carbohydrate formation, and (iii) uplift, denudation, and fracture development, all of which correspond well with Gao et al.’s (2019) perspective. In contrast, although southeastern Chongqing is located relatively close to Jiaoshiba, the Wufeng–Longmaxi Formation shale is situated within a different atmospheric environment with a pressure coefficient between 0.8 and 1.2 (He X. et al., 2017). Yuan et al. (2020) proposed that the Pengshui–Longmaxi Formation shale gas reservoir also experienced overpressure during its geological formation period, yet during uplift, the overpressure caused ruptures and fractures, resulting in the loss of shale gas and release of overpressure, ultimately transitioning the pressure environment to atmospheric. This is because the tectonic deformation experienced in the periphery of the Sichuan Basin was earlier and more intense than that in the interior of the basin, which resulted in the formation of distinctly contrasting accumulation environments between Jiaoshiba and Pengshui (Fan et al., 2019). Therefore, owing to a lack of relevant research, the characteristics, vertical evolution laws, and main controlling factors of normal-pressure Wufeng–Longmaxi Formation shale reservoirs in southeastern Chongqing need to be determined. This study therefore uses petrographic observations, low-temperature nitrogen adsorption, and scanning electron microscopy to better evaluate shale gas reservoir characteristics and guide the division of shale gas-favorable blocks in southeastern Chongqing.
Geological Setting
Parts of southeastern Chongqing are adjacent to the southeastern margin of the Sichuan Basin, western Hunan, and northern Guizhou (Figure 1A). This area has undergone the superimposition and transformation of multiple tectonic movements (Guangxi, Indo-China, Yanshan, and Himalayan), forming the upper Yangtze intra-platform depression structural unit, with folds and faults trending north–northeast (Xie et al., 2013). According to the changes in structural styles, the northwestern part of the area is classified as a trough-fold belt, the middle part as a transitional fold belt, and the southeastern part as a barrier-fold belt (Figure 1B). The partition and trough structure are successively controlled by the Yanshan and Himalayan movements (Wang et al., 2017). The outcropping strata in the area are dominated by Paleozoic-age rocks, lacking the Carboniferous, Cretaceous, and Tertiary eras. The basement of the strata is the Precambrian Banxi Group, and the total thickness of deposits of Sinian to Jurassic-age is nearly 10,000 m (Xu et al., 2015). This area developed two main source rocks: the Lower Cambrian Niutitang Formation (ϵ1n) and Upper Ordovician Wufeng Formation–Lower Silurian Longmaxi Formation (O3w–S1l) (i.e., the Wufeng–Longmaxi Formation or separate formations thereof) (Wang et al., 2017). The sedimentation of the Niutitang shale most likely occurred in a weak hydrodynamic, deep water, oxygen-deficient reducing environment, sourced from continental margins (Liu et al., 2016a). The Wufeng and Longmaxi formations are the main horizons for marine shale gas exploration and development in the Sichuan–Chongqing area. The two sets of shale have relatively similar characteristics, such as “high organic carbon content, high thermal evolution, and deep burial,” and are generally incorporated into an overall stratum for research (Liang et al., 2018). The original thickness of the Wufeng–Longmaxi Formation varies between 300 and 4,500 m. Owing to the influence of structural uplift and denudation, the current residual thickness is 20–120 m (70 m on average), which is thinner in the southeast and thicker in the northwest (Tan et al., 2015; Wang et al., 2017; Figure 1B). He X. et al. (2017) proposed that the Wufeng–Longmaxi Formation in the Pengshui area of southeastern Chongqing is under normal pressure, with a pressure coefficient between 0.8 and 1.2 which is far below that of the Jiaoshiba area. During the Late Ordovician–Early Silurian, the Sichuan Basin and its periphery were affected by the Guangxi Movement in which an intracontinental depression occurred under a background of compression, forming a large-scale deep-water shelf environment. The long-term closed and stable anaerobic environment was conducive to the preservation of organic matter, which provided superior conditions for high shale gas production (Mu et al., 2011; He et al., 2016; Fan et al., 2019). The argillaceous limestone and calcareous mudstone of the Guanyinqiao section are locally deposited, conforming to the overlying Longmaxi Formation and underlying Wufeng Formation (Liang et al., 2018; Zhao et al., 2020).
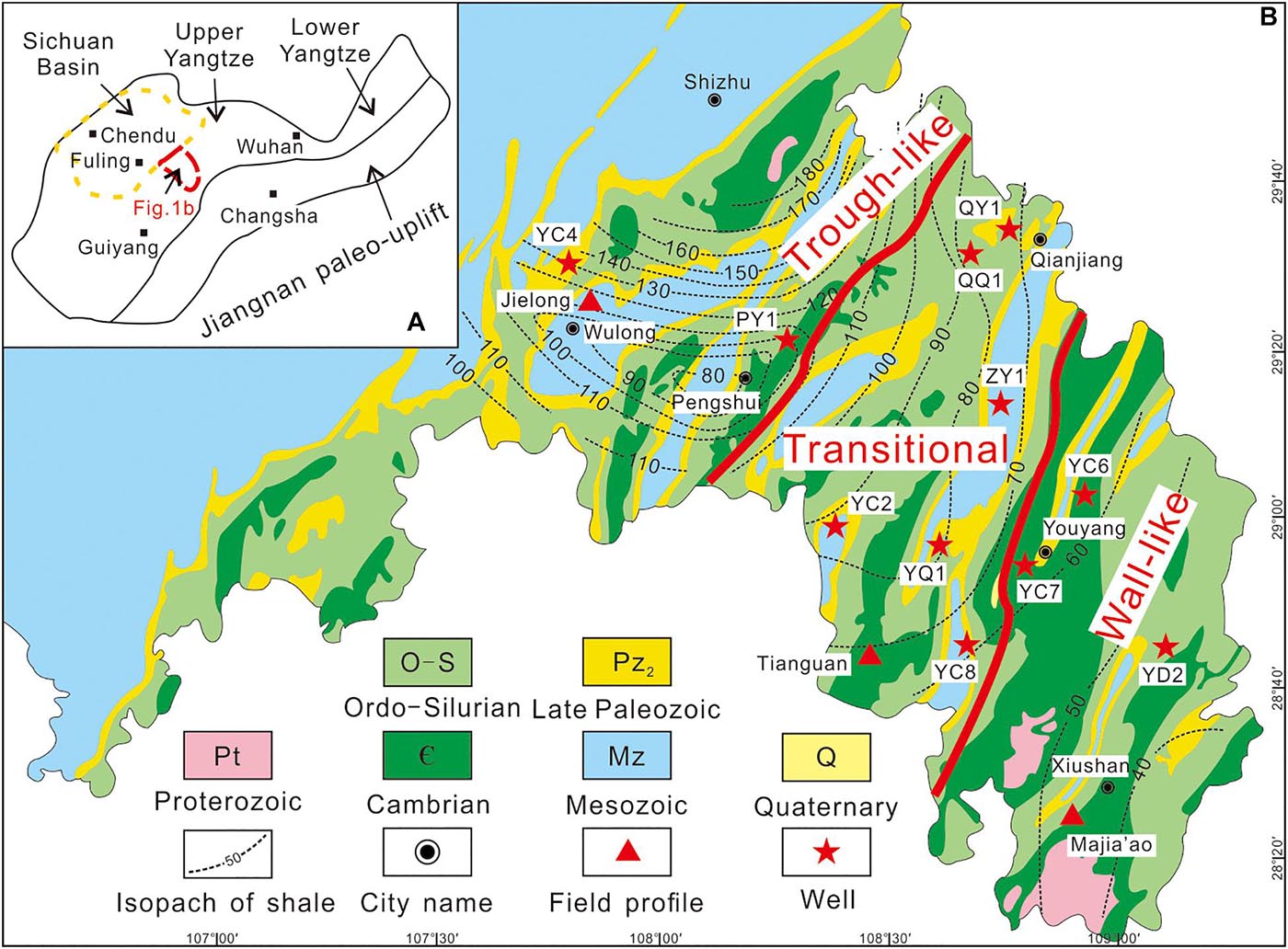
Figure 1. (A) Map of the study area; (B) geological map of southeastern Chongqing and sampling points (refer to Wu et al., 2011; Tan et al., 2015; Luo et al., 2016).
Samples and Methods
Samples of the Wufeng–Longmaxi Formation were collected from nine wells: Yushen 4, Yushen 6, Yushen 7, Yushen 8, Zhuoye 1, Qianqian 1, Youqian 1, Youshen 2, and Youdi 2 (Figure 1B).
TOC Content Analysis
Two grams of a powder sample was placed into a centrifuge tube, and 10% dilute hydrochloric acid was added. The sample was then dissolved by shaking and allowed to stand for 24 h. Then, the supernatant was poured out, and deionized water was added. This step was repeated several times until the supernatant was neutral, and then the sample was dried. Finally, a Leco CS-230 carbon and sulfur analyzer was used to determine the total organic carbon (TOC) content.
Mineral Composition Analysis
Quantitative analysis of the mineral composition was carried out using a Rigaku D/MAX RAPID II diffractometer for X-ray powder diffraction, and the experimental conditions used a Mo target. The rotation angle was 2.5–60°, the tube pressure was 40 kV, the tube flow was 100 mA, the incident spot diameter was 0.1 mm, and the sample tube diameter was 0.5 mm; no background sample tube was utilized.
Scanning Electron Microscopy
Scanning electron microscopy analysis was performed using a Quanta 200F (Field Electron and Ion Company, FEI; Oregon, United States). Secondary electron imaging was performed in high-vacuum mode at 3.0 nm and 30 kV, and at 8 nm and 3 kV; in high-vacuum deceleration mode at 7 nm and 3 kV; in low-vacuum mode at 3.0 nm and 30 kV, and at 10 nm and 3 kV; and in ambient-vacuum mode at 3.0 nm and 30 kV. The backscattered electron (BSE) imaging passes were conducted at 4.0 nm and 30 kV, the sample chamber pressure extended up to 2600 Pa, the acceleration voltage was between 200 V and 30 kV, and the moving range of the sample stage was continuously adjusted (Quanta 200F X = Y = 100 mm).
Porosity and Permeability Analysis
The analysis of porosity and permeability was performed with a CMS-300 (Temco, United States). Before the experiment, the core samples were dried at 104.4°C for 2 days, and the permeability was measured at a confining pressure of 1000 to 6000 psia. The instrument uses a pressure attenuation method to measure the permeability and porosity according to Boyle’s law, with a permeability range of 0.00005–15 mD and porosity range of 0.01–40%.
Pore Structure Analysis
Pore structure analysis was performed on the Autosorb-1 specific surface area and pore size measuring instrument (Quantachrome Company, United States). The instrument utilizes the isothermal physical adsorption static volume method, with a minimum resolvable relative pressure of 2.6 × 10–7, specific surface area of ≥0.0005 m2/g, test pore size range of 0.35–500 nm, and test pore volume of <0.0001 cm3/g. The test requires degassing, which was performed at a temperature of 97°C for 5 h. The Brunauer–Emmett–Telle (BET) model (Brunauer et al., 1938) was used to calculate the specific surface area of shale and determine the pore size distribution characteristics, including pore volume calculation of mesopores and some macropores (Barratt et al., 1951).
Results
Material Composition
Petrology
The Wulong–Jielong section in the northwest of the area reveals the complete profile of the Wufeng Formation–Guanyinqiao Section–Longmaxi Formation (Figure 2A). The lithology of the Wufeng Formation is mainly black siliceous shale, carbonaceous shale, and silty shale with a deposition thickness of 10.4 m (Figure 2B). The lithology of the Guanyinqiao section (in the upper part of the Wufeng Formation) is mainly marl and lime shale, and the thickness is very thin at only 31 cm (Figure 2B). The lower Longmaxi Formation comprises black shale with extremely thick deposits, and the silty content increases upward.
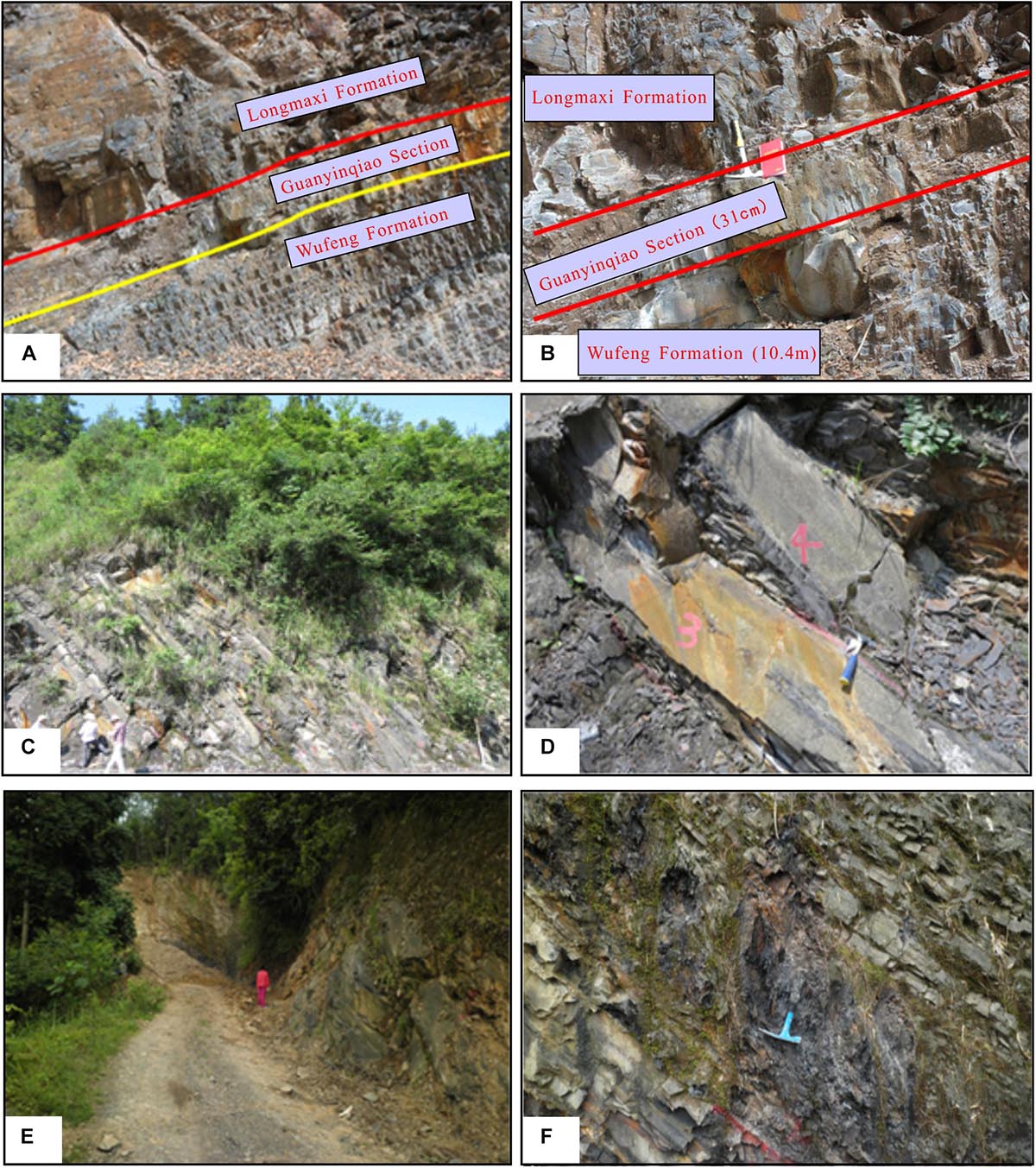
Figure 2. Field sedimentary characteristics of the Wufeng–Longmaxi Formation shale. (A) shale at the Wulong–Jielong Section; (B) interface of Wufeng–Guanyinqiao Section–Longmaxi Formation at the Wulong–Jielong Section; (C) shale of the Youyang–Tianguan Section; (D) black carbonaceous mud shale at the bottom of the formation at the Tianguan Section in Youyang; (E) shale at the Majiaao Section in Xiushan; and (F) black carbon at the bottom of the formation at the Majiaao Section in Xiushan mudstone.
The Youyang–Tianguan section (Figure 2C) in the central part of the study area outcrops black carbonaceous shale and carbonaceous siliceous shale at the bottom of the Wufeng–Longmaxi Formation (Figure 2D), where many graptolite fossils can be seen and with horizontal bedding of 22.39 m thickness. The upper lithology is black and gray-black carbon-bearing silt shale and silt stone, and the overall silt content increases upward, with a thickness of 36.79 m.
At the Xiushan–Majiaao section in the southeastern part of the study area (Figures 1B, 2E), the organic-rich mud shale at the bottom of the Wufeng–Longmaxi Formation is thinner, having a thickness of 10.2 m (Figure 2F). The lithology is mainly black and gray-black carbonaceous shale and carbonaceous siliceous shale with a large number of graptolite fossils and pyrite and reduced carbon content after weathering. The lithology in the central part is mainly gray-black and black argillaceous siltstone with occasional gray-white ultra-thin layered porphyrite and a thickness of 6.53 m. In the upper part, the argillaceous content is increased, and the lithology is mainly gray-black and gray silty mudstone. The local carbon content is high, and a large number of graptolite fossils can be seen with a thickness of 28.19 m.
Mineralogy
The Wufeng–Longmaxi Formation shale in the area is mainly composed of brittle minerals and clay minerals and a small amount of pyrite (Table 1). The content of the brittle minerals is between 39.5 and 90.7% (65.5% on average), which mainly consists of quartz (22–79%, average 41.1%), followed by feldspar (1.7–28.7%, average 14.9%), and a small amount of carbonate minerals (0–27.9%, average 9.3%). The content of the clay minerals is 7.1–58.2% with an average of 30.3%, which is mainly illite (7–68%, 29.9% on average) and illite/smectite minerals (8–85%, 54.1% on average) (Figure 3D). A small amount of chlorite (1–42%, average 11.5%) and a chlorite/smectite layer (0–17%, average 3.7%) are present, and the illite/smectite layer mainly reflects the properties of illite. The pyrite content ranges from 0 to 14.6%, with an average of 3%. Vertically, the content of the brittle minerals such as quartz feldspar in the lower shale section is highest, generally reaching more than 60% and gradually decreasing upward; the content of the clay minerals gradually increases upward (Figures 3A,B). Horizontally, the quartz content gradually decreases from the barrier-fold belt to trough-fold belt. The content of feldspar and carbonate minerals gradually increases, and the clay mineral content gradually decreases (Figures 3C,D).
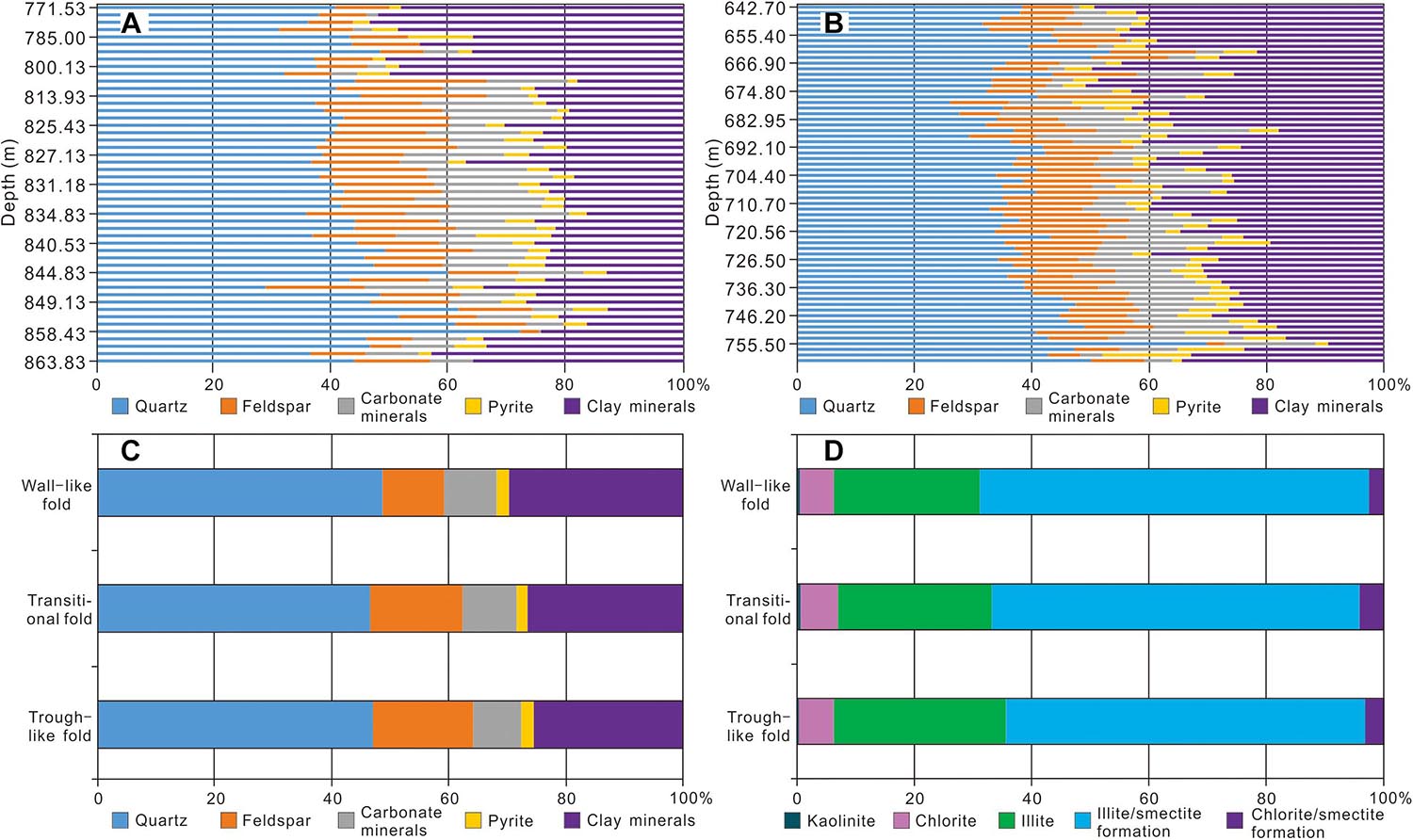
Figure 3. Variation trend of mineral composition in the Wufeng–Longmaxi Formation shale: (A) Vertical distribution map of the shale mineral composition in the Yucan 7 well; (B) vertical distribution map of the shale mineral composition in the Yucan 4 well; (C) shale mineral composition distribution map according to structural style; and (D) shale clay mineral composition distribution map according to structural style.
Organic Matter Characteristics
The overall TOC content of Wufeng–Longmaxi Formation shale in the research area has a large variation range of 0.29–8.49%. In terms of total TOC content of the samples, samples with TOC greater than 1% account for 50.92%, and those greater than 2% account for 24.51%. According to the vertical lithology changes, the Wufeng–Longmaxi Formation shale is divided into three sections in the northwestern part of the area, characterized by high TOC (first section; 2.0–8.49%, average 3.43%); low TOC (second section; 0.63–1.97%, average 1.37%); and moderate TOC (third section; 1.28–2.64%, average 2.07%). However, the lithological changes of the Wufeng–Longmaxi Formation in the research area are not obvious, nor is the vertical change in TOC. These phenomena may be related to the distribution of sedimentary facies.
Based on the classification criterion of kerogen type index from Shen et al. (2013), the Wufeng–Longmaxi Formation kerogen type in the area is mainly saprolite I (Table 2). The organic-rich shale has a relatively high degree of evolution with a maturity of 1.6–3.38% (average 2.56%). The overall Ro did not change much (1.8–2.8%). The Youyang East–Xiushan area has the characteristics of thin deposits, low maturity, and Ro values of less than 2.0%. The Wulong–Pengshui area has the characteristics of thick deposits, high maturity, and Ro values of greater than 2.5%.
Reservoir Characteristics
Type of Storage Space
According to the observational statistics of scanning electron microscopy and core samples, the Wufeng–Longmaxi Formation mud shale developed organic matter pores, clay mineral interlayer pores, intragranular and intergranular pores, and structural fractures. Of these, the structural fractures are the main storage space for shale gas, followed by organic pores, which have the lowest proportion of intragranular pores. There appears to be no change in the type of storage space from the southeast to the northwest of the study area.
(1) Organic pores
The shale in the formation considered herein has well-developed organic pores, which are circular or elliptical in shape and distributed in a honeycomb shape; the pore diameters are concentrated at 30–100 nm (Figures 4A,B). The facies ratios of the organic matter in the shale show that the separate Wufeng and Longmaxi formations account for 19.67% and 9.84% respectively, and that of the Wufeng–Longmaxi Formation shale accounts for 39.9% of the total pores.
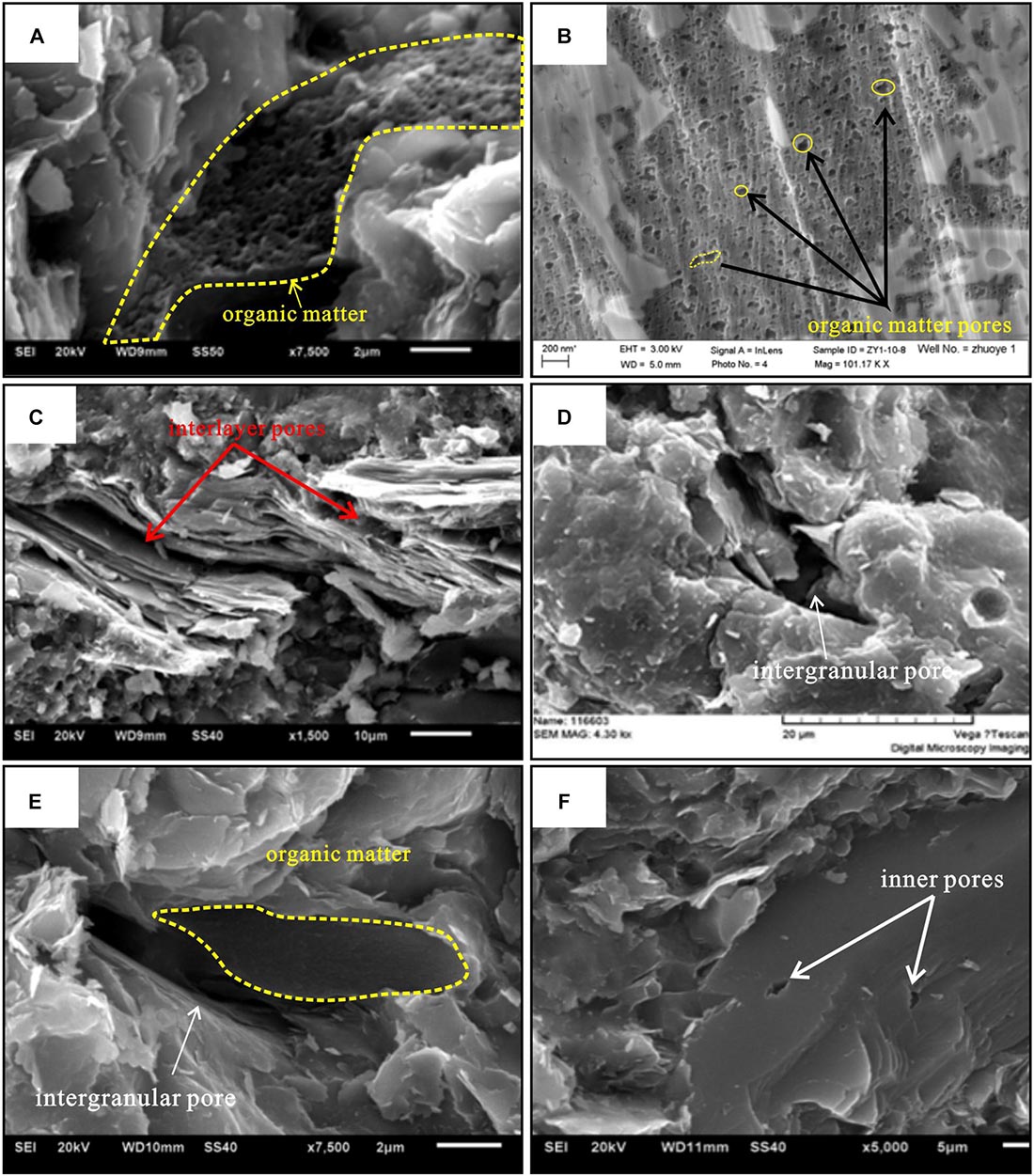
Figure 4. Types and characteristics of pore spaces of the Longmaxi Formation shale in the study area (scanning electron microscopy). (A) Nano-scale organic pores, Yucan 4 well; (B) nano-scale organic pores, Zhuoye 1 well; (C) interlayer pores of clay minerals, Youdi 2 well; (D) intergranular pores, Qianqian 1 well; (E) micron-scale organic matter and mineral intergranular pores, Yucan 6 well; (F) nano-scale feldspar grain inner pores, Yucan 6 well.
(2) Clay mineral interlayer pores
The shale structure of the Longmaxi Formation is relatively dense with fewer intergranular and intragranular pores of inorganic minerals, but large-scale interlayer pores of clay minerals are observed, distributed among clay minerals such as the filamentous and curly, flaky illite (Figure 4C). The width of these interlayer pores varies from tens of nanometers to several micrometers with good connectivity and the pores are linear and parallel to each other, accounting for 28% of the total pores.
(3) Intergranular pores
The intergranular pores of the Wufeng–Longmaxi Formation shale mainly include mineral pores and intermediate pores of organic matter and minerals, accounting for 20.2% of the total pores. The larger the mineral particles, the larger the inter-particle pores, and the pore diameters range from nanometers to 5 μm (Figure 4D). Organic matter and mineral intermediate pores only account for a small part of the shale pores (Figure 4E), but such pores open up organic matter pores and mineral pore channels, enabling natural gas to migrate to mineral pores. The Wufeng Formation shale structure is relatively fragmented, and a large number of intergranular pores are developed between quartz grains and between quartz and clay minerals, which are usually distributed linearly along the grain edges.
(4) Intragranular pores
The edges of the dissolved pores are irregular harbors or zigzags (Figure 4F), and the pore walls are curved and developed in groups. The pores are nanometer-sized, accounting for 11.9% of the total pores.
(5) Structural fractures
The Wufeng–Longmaxi Formation shale has structural fractures that formed in the Yanshanian and Himalayan movement periods (Figures 5A,B); these fractures are the main storage space for shale gas. The width of typical open fractures is large, while the extension is short, and the fracture surface is non-straight. In contrast, shear fractures have a relatively straight fracture surface and high density. The same group of fractures has better equidistance, and the different groups of fractures cut through one another. The surface of the slippage fracture is non-smooth, yet the scratches, steps, and mirror features are visible (Figures 5C,D).
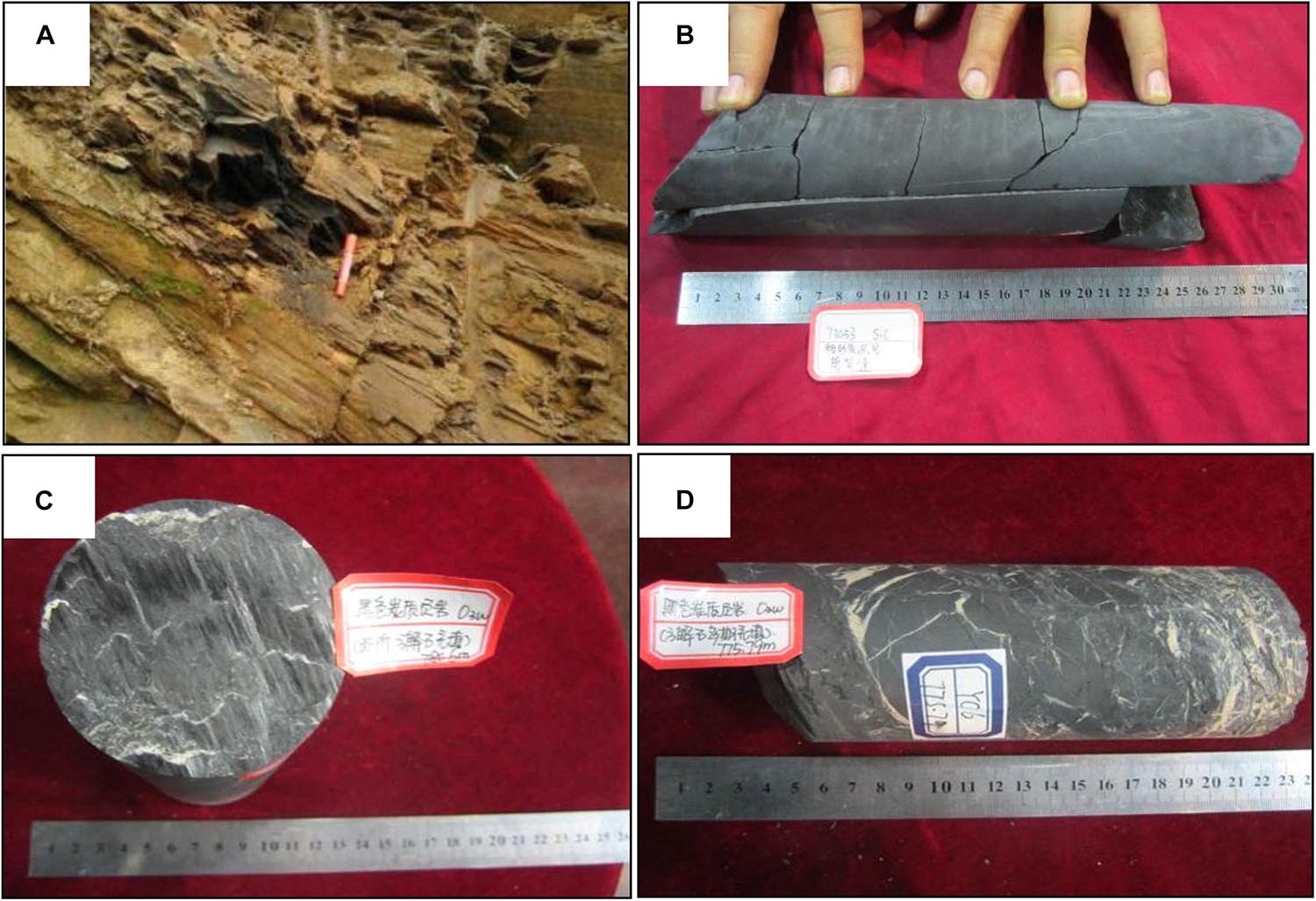
Figure 5. Distribution characteristics of fractures in field section and drilling cores. (A) Wufeng–Longmaxi Formation structural fractures in the Majiaao section of Xiushan; (B) multi-stage structural fractures in the lower part of the Wufeng–Longmaxi Formation, Yucan 4 well; (C,D) attachment and detachment zones in the lower part of the Wufeng–Longmaxi Formation scratches, Yucan 6 well.
The fracture length is dominated by short fractures (<10 cm), which represent close to 90% of the total length (Figure 6A). The longest fracture was observed to be up to 88 cm. The fractures with openings ≤0.2 mm and 0.5–1 mm represent 40% of the total, while fractures with openings of 0.2–0.5 mm are not developed (Figure 6B). Low-angle oblique fractures and high-angle cutting joints are roughly the same, accounting for 34.95 and 33.49% of the total, respectively. The frequencies of occurrence of the horizontal and vertical slits were also very close at 15.73 and 15.83%, respectively (Figure 6C).
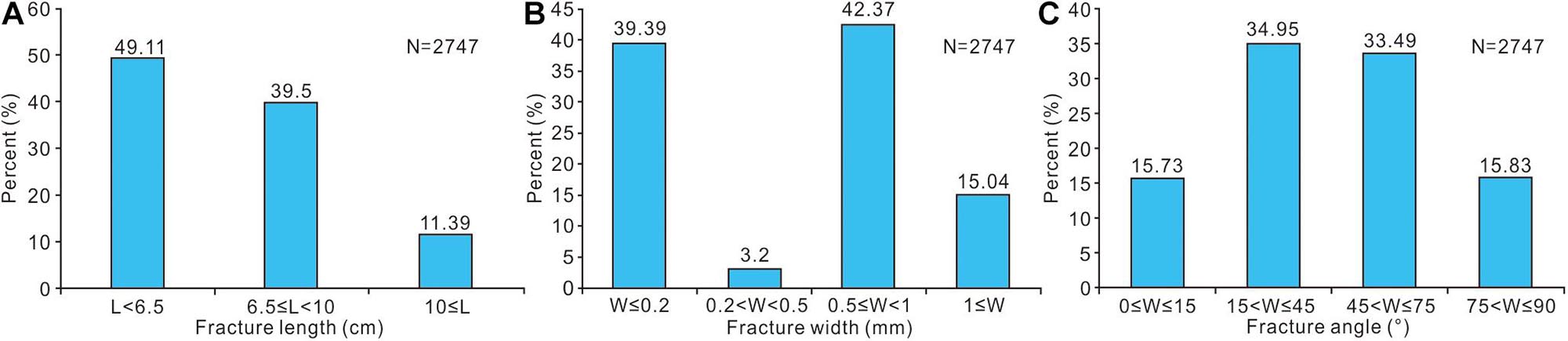
Figure 6. (A) Percent of fracture length with the different scale from the Wufeng-Longmaxi Formation, southeastern Chongqing. (B) Percent of different fracture width in the Wufeng-Longmaxi Formation, southeastern Chongqing. (C) Percent of different Fracture angle in the Wufeng-Longmaxi Formation, southeastern Chongqing. N denotes the number of fractures.
The Wufeng–Longmaxi Formation has a relatively high degree of fracture filling. More than half of the fractures are completely filled, and unfilled fractures account for 40.81% of the total number of fractures measured; semi-filled fractures are basically not developed and are characterized by a large amount of pyrite and calcite filled together. These types of fractures are widely distributed in the stratum, with stable extension and spreading.
Porosity and Permeability Characteristics
The porosity and permeability of the Wufeng–Longmaxi shale in the study area were lower than those in the Jiaoshiba area (Figure 7A), indicating a low-porosity and low-permeability reservoir. The study area porosity ranges from 0.05% to 5.61% (average 1.25%), while the porosity of 81.1% of the region ranges from 0 to 2%. Likewise, the study area permeability is between 5 × 10–7 and 16.26 × 10–3 μm2, while the permeability of 72.1% of the region is 1 × 10–4 to 1 × 10–5 μm2. Therefore, the correlation between the porosity and permeability of the Wufeng–Longmaxi Formation in the study area is not obvious; however, the southeastern Yucan 6 well, the central Qianqian 1 well, and the northwestern Yucan 4 well all show the characteristics of low porosity and high permeability (Figure 7A). Further, Liu et al. (2017a) previously revealed the vertical heterogeneity of the physical properties of the Longmaxi shale reservoir resulting from diagenesis based on the comparison of the single well. According to TOC content, the shale in the study area is divided into organic-rich shale (TOC > 2%), organic shale (1% < TOC < 2%), and organic-poor shale (TOC < 1%). From the cross-plot of porosity and permeability of different lithofacies (Figure 7B), the permeability of all three lithofacies is the same, in that the porosity of organic-rich shale is the largest, followed by organic shale, and that of organic-poor shale is the smallest. This shows that the higher TOC content of shale, the more developed the organic porosity and the higher the porosity.
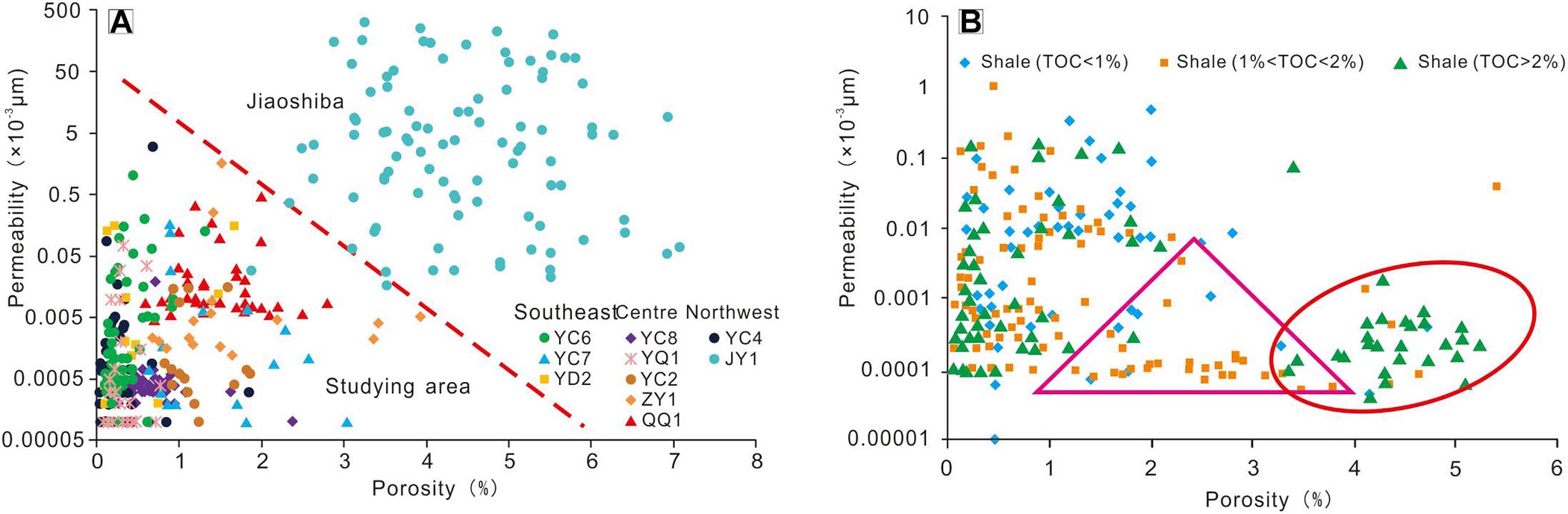
Figure 7. (A) Pore-permeability intersection diagram of shale from the Wufeng-Longmaxi Formation in southeastern Chongqing, compared with those in Jiaoshiba area (data from JY 1 well were obtained from Guo et al., 2014b). (B) Pore-permeability intersection diagram of shale with different percent of TOC content from the Wufeng-Longmaxi Formation in southeastern Chongqing. YC6 denotes the serial number of well drilling.
Pore Structure
According to the nitrogen gas adsorption/desorption curves (Figure 8), the internal pore structure of the particles has the characteristics of slit-like pores with parallel walls and contains other pores with multiple morphologies. The pores are in an open state and have mainly cylindrical holes with open ends and parallel plate-shaped holes with four sides open. The average pore size of specific surface area (as determined using the method of Barrett, Joyner, and Halenda) (hereafter referred to as BJH) ranges from 2.97 to 8.21 nm (average 5.28 nm). The average diameter of the pores in the Yucan 6 well is the largest (6.58 nm); the average pore diameter in the Zhuoye 1 well is the smallest (3.65 nm) (Figure 9). The average pore size of the lower part of the Wufeng-longmaxi Formation was smaller than that of the upper part (Figure 9). Pore properties can affect the specific surface area of shale and the relative abundance of micropores. The results of nitrogen adsorption experiments show that the specific surface area (i.e., BET) of the Wufeng–Longmaxi Formation shale is large, ranging from 2.1 to 35.1 m2/g (average 9.2 m2/g). Horizontally, the BET-specific surface area of the Zhuoye 1 well is the largest, with a distribution range of 12.1–35.1 m2/g (20.2 m2/g on average). The Yucan 6 well BET-specific surface area is the smallest, ranging from 2.8–7.7 m2/g (5.2 m2/g on average) (Figure 9). Vertically, the BET-specific surface area of the lower Wufeng-longmaxi Formation is relatively large, and the micropores are more developed but gradually decrease upward (Figure 9). The Wufeng–Longmaxi Formation shale BJH has a relatively large pore volume, ranging from 0.0012–0.026 ml/g (0.0064 ml/g on average). The BJH pore volume of the Zhuoye 1 well is largest, distributed between 0.0122–0.026 ml/g (average 0.018 ml/g). The BJH pore volumes of the Yushen 7 and Yushen 8 wells are similar in size, with an average of ∼0.0045 ml/g. The BJH pore volume of the Youdi 2 well is the smallest at 0.0012–0.0048 ml/g, with an average of 0.0026 ml/g (Figure 9). Among the different types of pores, the mesopore volume (2–50 nm) accounts for 90.8–97.2% of the total pore volume (average 94.9%). Micropores smaller than 2 nm account for 2.8–9.3% (average 5.1%), with almost no large holes (>50 nm) present.
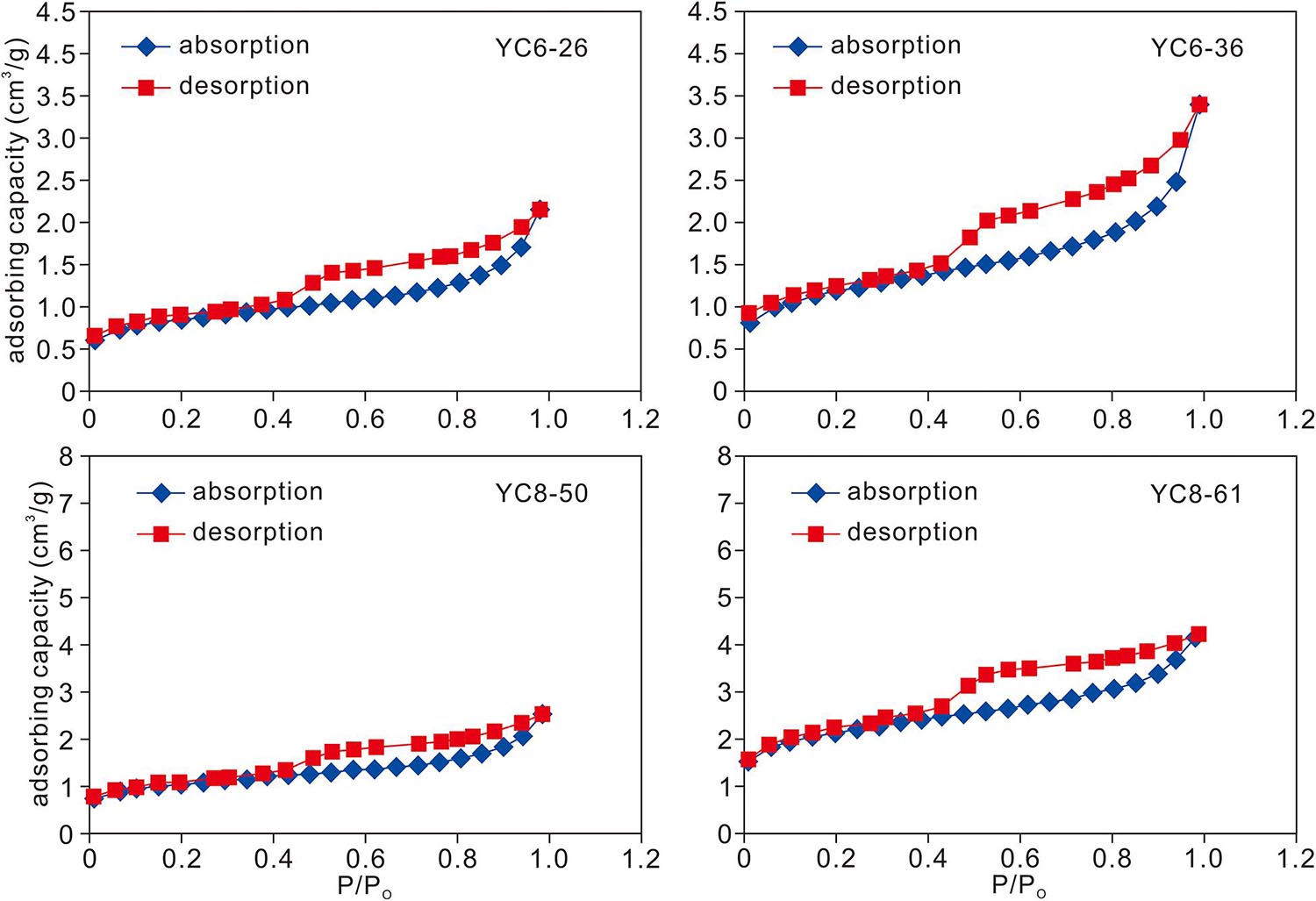
Figure 8. Nitrogen gas adsorption/desorption curves of representative samples from YC 6 well and YC 8 well in the Wufeng–Longmaxi Formation.
Discussion
Mud shale gas reservoirs are self-generating, self-storing, and self-covering. The physical characteristics of the reservoir determine whether it can become an industrial gas flow; however, the physical properties of shale reservoirs are constrained by multiple factors, and identifying the formation mechanisms of reservoir physical characteristics can provide guidance for the determination and categorization of shale gas-favorable areas. The Wufeng–Longmaxi Formation shale reservoir in southeastern Chongqing is of low porosity and low permeability. Structural fractures are the main storage space for shale gas, followed by organic pores. The main factors controlling its physical properties include the sedimentary environment, mineral composition, organic carbon content, and tectonic movement. The difference in the depositional environment determines the changes in the mineral composition and TOC content of the Wufeng–Longmaxi Formation in the southeast and northwest areas of southeastern Chongqing, which controls the anti-compaction ability, degree of fracture development, and organic matter pore ratio of the shale reservoir, as well as the tectonic movement. The depositional environment is a power source that affects the physical properties of shale reservoirs.
Depositional Environment
The Late Cambrian Niutitang Formation was deposited in a neritic-to-slope environment with a weak hydrodynamic and oxygen-deficient reducing environment (Liu et al., 2016a). In the Late Ordovician–Early Silurian period, a famous intracontinental orogeny occurred in the South China Plate (Shu, 2012). During this, the continuous compression of the Yangtze and Cathaysia landmass formed the Middle–Upper Yangtze Craton into a rugged and uneven sedimentary terrain, and a deformed depression area developed in eastern Chongqing and northern Guizhou (Mou et al., 2014). Previous research has been conducted thoroughly on the depositional environment of the Middle–Upper Yangtze Region in the Late Ordovician–Early Silurian period. Mou et al. (2014) proposed that the topographic depression area was a deep-water basin facies in the late Kaidi–Henantian period, which developed black carbonaceous shale and radiolarian siliceous shale. Wang G. et al. (2019) suggested that the late Hernnant period corresponds to the global ice age, which caused an eventual regression of global sea levels, allowing limestone and argillaceous siltstone to be deposited in the Guanyinqiao section. During the Early Silurian period, the climate warmed and the ice caps melted, and this rapid event transgression deposited the graptolite-rich carbonaceous shale at the bottom of the Longmaxi Formation. After that, the area was affected by structural uplift, and the sea level gradually declined. Fan et al. (2019) indicated that northeastern Sichuan entered a rapid transgression stage during the Late Ordovician–Early Silurian period, forming a large-scale and deep-water shelf sedimentary environment. Lower microorganisms began to proliferate in large numbers, and lower planktonic microalgae settled out in the deep-water environment after death, which transformed the deepened and blocked deep-water shelf environment into a reducing environment. This was conducive to the burial and preservation of microbial remains and allowed for organic matter-rich shale layers in this area. Earlier research on the sequence stratigraphy in the Wufeng–Longmaxi Formation in southeast Chongqing showed that the deep-water shelf facies occurred in the Wufeng period. Since the Longmaxi period, the sea level gradually receded to the northwest, and the depositional environment evolved into deep-water shelf, shallow-water shelf, and shallow sandy shelf facies (Liang et al., 2018). In addition, previous researchers have also used geochemical methods to define and further verify the depositional environment. Zhang et al. (2013) used nutrient elements and redox indicators to demonstrate that the bottom of the Longmaxi Formation was deposited in a reducing environment, and the middle–upper spherical chemical indicators were mainly in the oxygen-poor stage, indicating the transition from a reducing to an oxidizing environment. This effectively confirmed that the southeastern Sichuan to northern Guizhou region during the early Longmaxi period mainly experienced deep cementitious shelf deposition and that the middle–late period was a shallow-water shelf depositional environment. Therefore, the high-quality source rocks of the Longmaxi Formation mainly developed at its bottom. Moreover, Fan et al. (2019) have suggested that the Longmaxi Formation in the Dingshan area was deposited in a weakly oxidizing–strongly reducing environment, mainly in sub–high energy and low-energy sedimentary water bodies. Zhou et al. (2011) studied the Mo isotope of the Longmaxi Formation and concluded that the Longmaxi Formation black shale was deposited in an anaerobic-poor environment. Therefore, southeastern Chongqing was in a deep-water shelf and shallow-water shelf environment during the Late Ordovician Wufeng period–Early Silurian–Longmaxi period (Figure 9). In addition, the Wufeng–Longmaxi Formation mud shale in the study area has sedimentary characteristics of thickening from the southeast to northwest and increasing sand content from the bottom to top, which may be related to changes in the depositional environment. A set of organic-rich black siliceous shale and carbonaceous shale was deposited at the bottom of the Wufeng and Longmaxi formations in the area, which corresponds well to the deep-water shelf environment; however, the increase in upward sand content and the decrease in organic matter content may be effects of the collision orogeny between the Yangtze and Cathaysia plates, resulting in large-scale northwestward regression (Figure 10A). The Youyang–Xiushan area in the southeast of the study area is characterized by thin sediment thickness, low TOC (average 1.69%), and average siliceous mineral content of 35.8%, suggesting that the region may belong to a shallow-water shelf environment (Figure 10B). The Beixi Shizhu–Pengshui-Qianjiang area has the characteristics of thick deposit thickness and high TOC, which suggests that it may belong to a deep-water shelf environment (Figure 10B) with an average TOC of 3.6% and an average composition of 49% siliceous minerals. Tang et al. (2019) proposed that the Wufeng–Longmaxi Formation deposition center in southeastern Chongqing has high porosity, high TOC, and high quartz content. Based on this, the deposition center is located in the northwest of the study area, and the provenance area may be the Xuefeng Mountain uplift area in the southeast (Figure 10B). Mud shale with high TOC and high brittle mineral content not only has significantly greater adsorption capacity for methane, but it also helps to improve the physical properties of mud reservoirs.
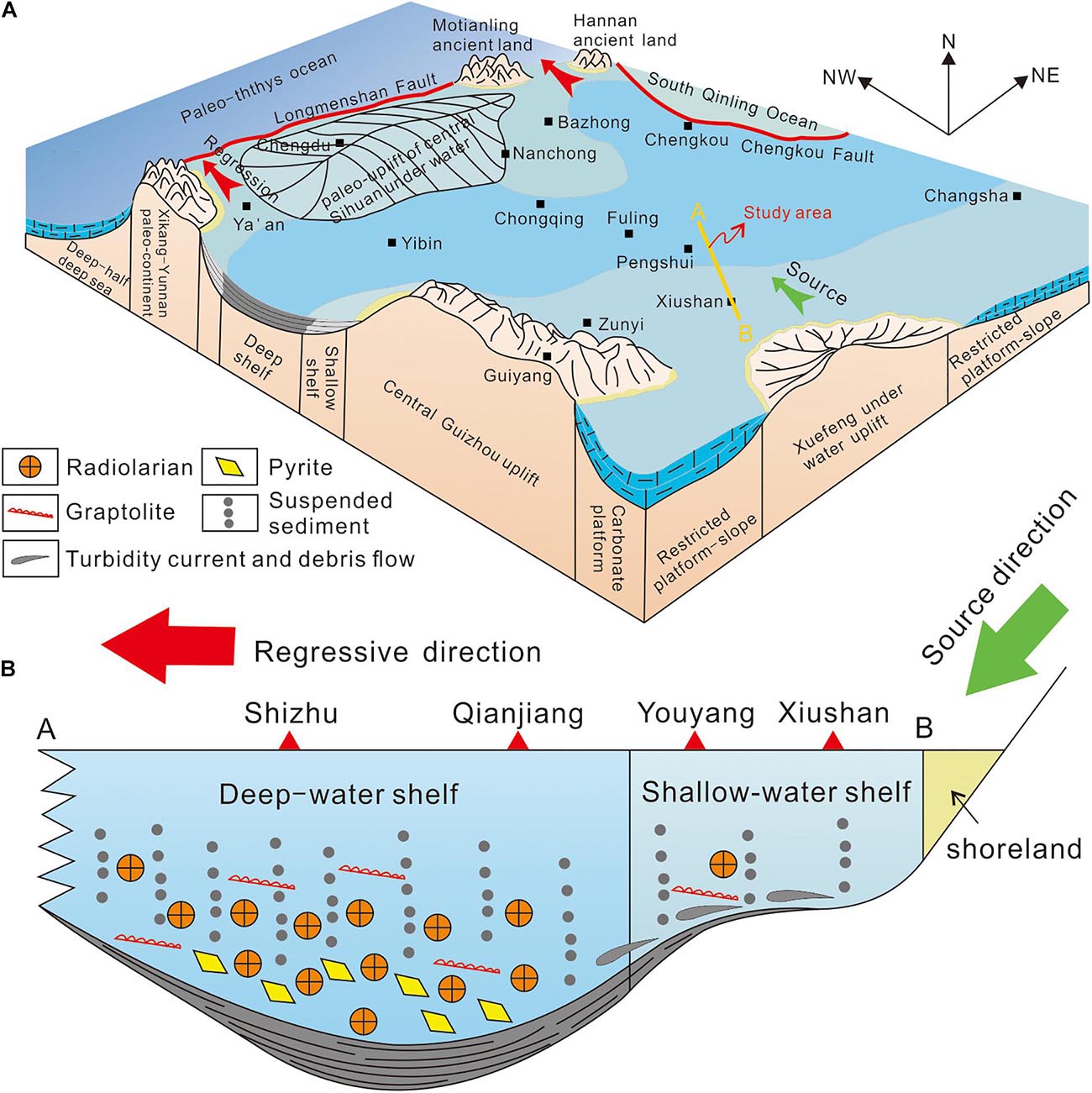
Figure 10. (A) Late Ordovician–Early Silurian sedimentary environment model map of the Sichuan Basin and its periphery (modified from Fan et al., 2019); (B) sedimentary environment distribution model map of the Wufeng–Longmaxi Formation in southeastern Chongqing (modified from Liang et al., 2018).
Mineral Composition
The Wufeng–Longmaxi Formation organic-rich shale contains two main mineral groups: brittle minerals and clay minerals, consistent with the viewpoint of Liu et al. (2016b). The brittle minerals are mainly quartz, and the clay minerals are mainly illite and illite/smectite (Figure 3). The deep-water anoxic depositional environment is conducive to the complete preservation of microorganisms and plankton. The Wufeng–Longmaxi Formation deposited in the deep-water shelf environment has higher TOC and quartz content (Tang et al., 2019), indicating that SiO2 mainly comes from the slow sedimentation of seawater rather than terrigenous debris, which is consistent with the view of biogenic siliceous minerals (Guo et al., 2020).
The high content of brittle minerals not only improves the anti-compaction ability of pores but also allows for easier shale fracturing, producing a large number of fractures and microfractures, which is conducive to the preservation of shale gas (oil). According to the statistics of quartz content and fractures in the Longmaxi Formation of the Yucan 8 well from 789 to 860 m (Figure 11), there is a clear exponential relationship between the quartz content and fracture line density. The higher the quartz content, the greater the fracture line density. Combined with the characteristics of high permeability but low porosity in the study area (Figure 7), this indicates that incompletely filled natural fractures in the shale layer likely improve the permeability of the shale reservoir. Vertically, the bottom of the Wufeng–Longmaxi Formation has a higher content of brittle minerals (Figures 3A,B), indicating that the bottom is more prone to fractures and has better permeability. Horizontally, the northwest has the highest content of brittle minerals, and the southeast has the lowest content (Figure 3C), indicating that the northwestern area has more developed fractures and better permeability.
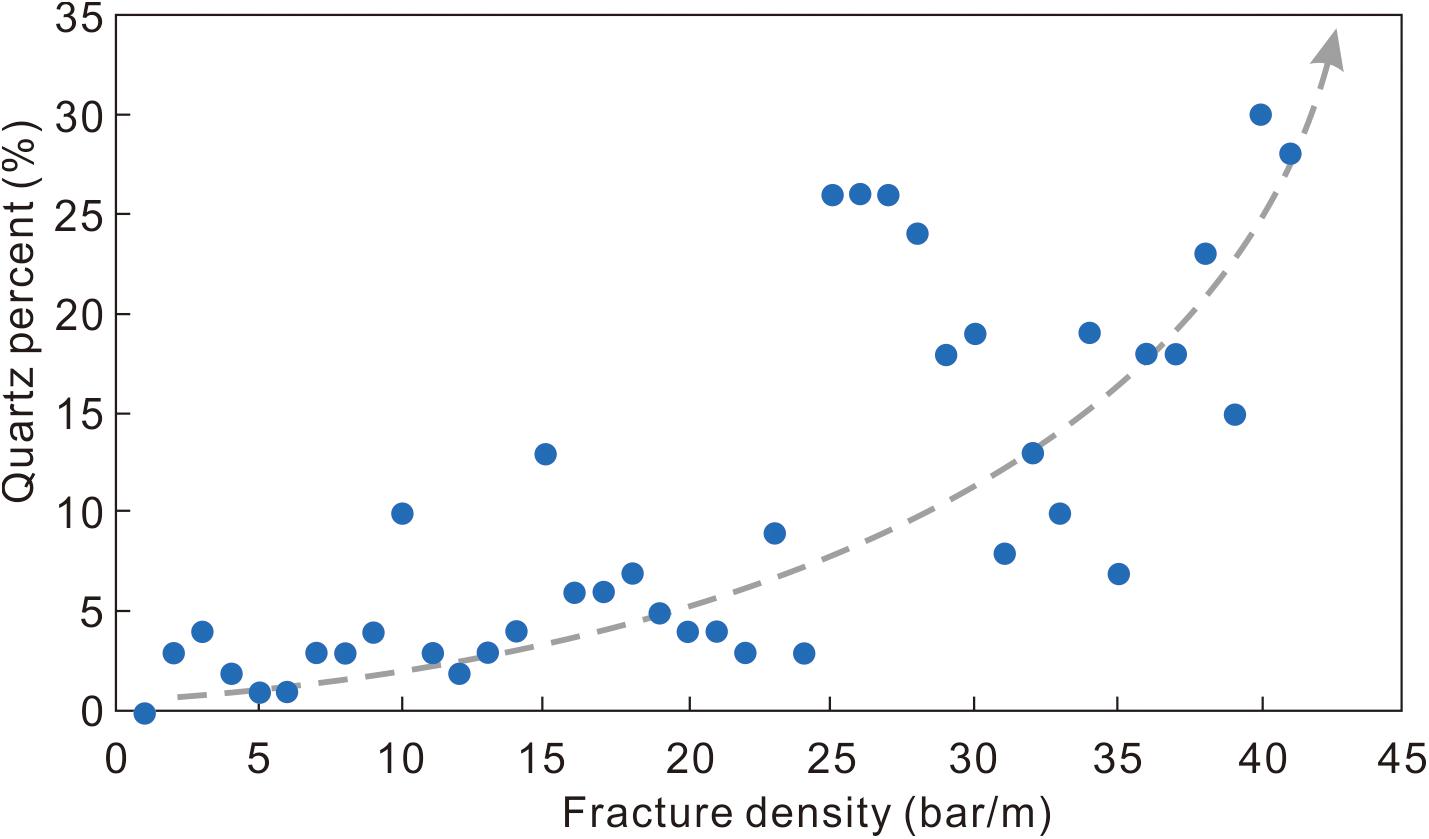
Figure 11. Relationship between fracture development and quartz content in the Longmaxi Formation shale at the Yushen 8 well.
Ross and Bustin (2008) suggested that clay minerals in shale gas reservoirs have higher micropore volumes and larger specific surface areas. The analysis of clay minerals, pore volume, and specific surface area in the Longmaxi Formation shale of the Yushen 6 and Yushen 8 wells in southeastern Chongqing in this study shows a definite positive correlation between these characteristics, indicating that the pore-specific surface area and pore volume of organic-rich mud shale vary with the increase in clay mineral content (Figures 12A,B). However, the crystal layer and pore structure of different clay minerals are distinct, and the pore-specific surface area is also very different (Ji et al., 2013). The clay minerals contained in the organic-rich shale of the Longmaxi Formation in southeastern Chongqing are mainly illite and an illite/smectite mixed layer (Figure 3D). There is a good exponential relationship between the illite/smectite mixed-layer mineral content and the pore volume and specific surface area of the Yushen 6 and Yushen 8 wells (Figures 12C,D), indicating that the organic-rich shale pore volume and pore-specific surface area increase as the mineral content of the illite/smectitemixed layer increases. Therefore, the mineral content of the illite/smectite mixed layer is one of the main factors controlling the microscopic pore volume and pore-specific surface area of the organic-rich shale of the Longmaxi Formation.
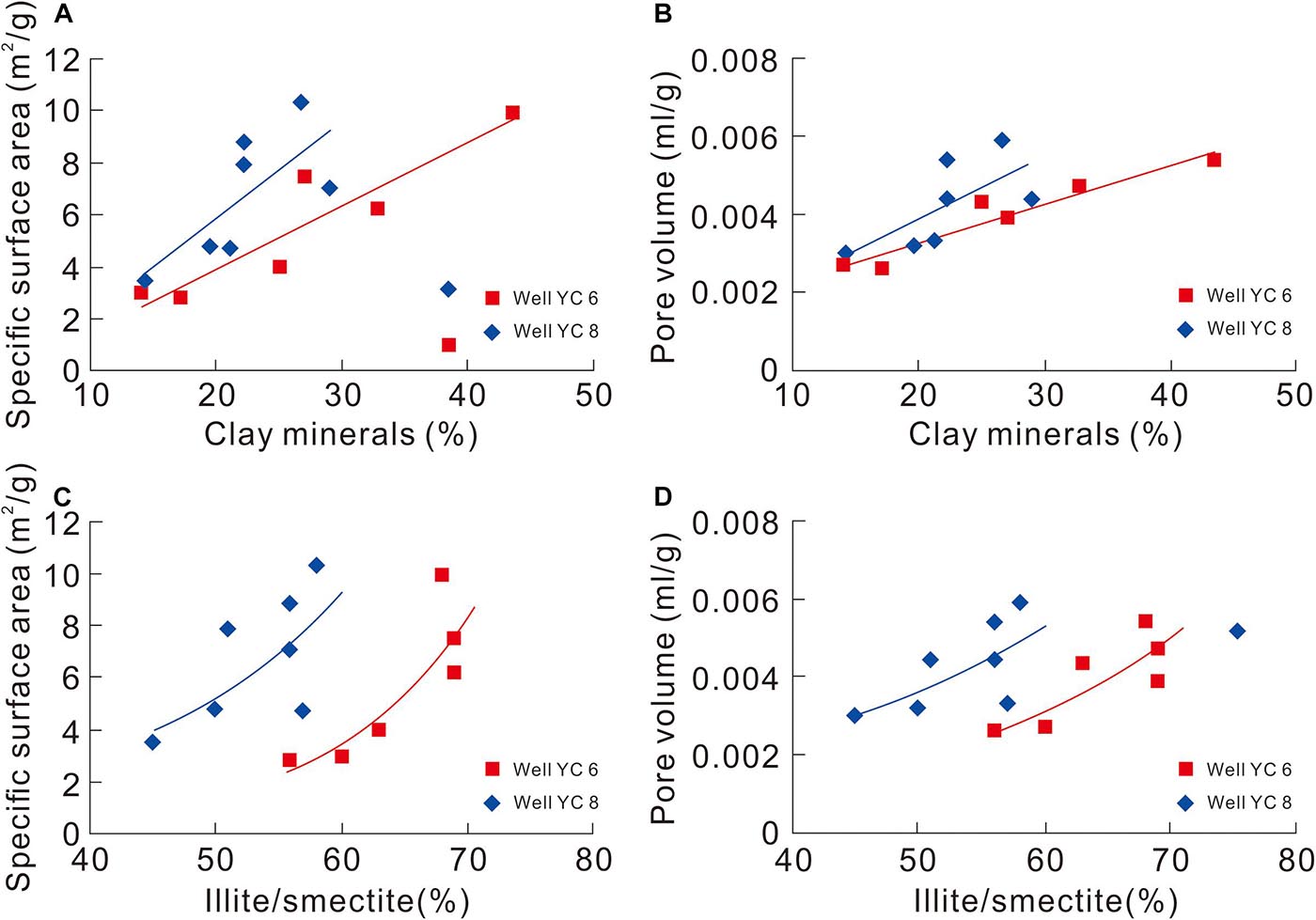
Figure 12. (A,B) Correlation diagram of the clay mineral content, specific surface area, and pore volume of the Longmaxi Formation shale in southeastern Chongqing; (C,D) correlation diagram of the mineral content, specific surface area, and pore volume of the shale illite/smectite layer of the same formation.
TOC Content
The TOC content of shale is an important parameter for measuring the hydrocarbon-generation potential of source rocks, and also an important controlling factor for the development of organic matter pores. A large number of nano-scale pores develop in organic matter, providing the specific surface area and pore volume (Bustin et al., 2008). From this study, according to the correlation analysis between the TOC and the specific surface area and pore volume of the Longmaxi Formation in southeastern Chongqing (Figures 13A,B), TOC has a significant positive correlation with both characteristics, indicating that as the TOC value increases, the pore volume and pore-specific surface area of organic-rich shale also increase accordingly. Generally, organic matter pores have a complicated internal structure with rough pore surfaces and diverse internal morphologies. This special structure can increase the specific surface area and pore volume, thereby improving the adsorption and storage capacity. The lower part of the Wufeng–Longmaxi Formation in the study area has a high TOC content which gradually decreases upward. In addition, the TOC content in the northwest is high (>2.4%), and that in the southeast is low. The corresponding specific surface area and pore volume also have the same characteristics (Figure 8). Therefore, TOC is one of the important factors controlling the micropores of organic-rich shale in the Longmaxi Formation, which plays an important role in shale gas enrichment.
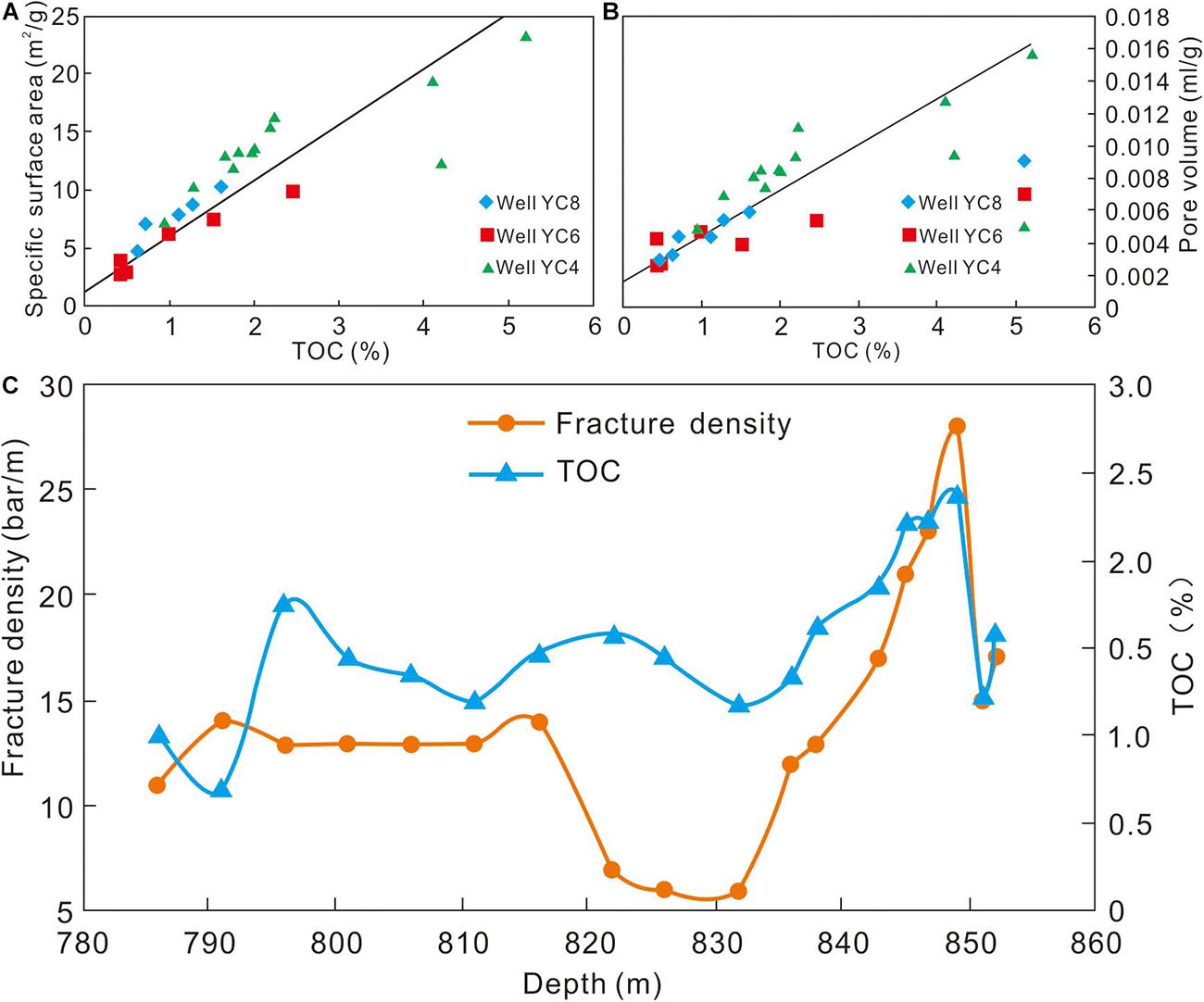
Figure 13. (A,B) Correlation between the TOC and the specific surface area and pore volume of the Longmaxi shale in southeastern Chongqing; (C) correlation between the vertical fracture development and TOC of the Longmaxi shale at the Yushen 7 well.
In addition, TOC can also control the development of shale fractures to a certain extent. This is mainly due to the fact that the deep-water reduced depositional environment could completely preserve siliceous organisms (such as radiolarians) and biological skeleton remains, which increases the siliceous content of shale reservoirs. Shales with high organic matter and quartz content are more brittle, making it easy to form natural fractures and induce fractures under tectonic stress, which is conducive to the desorption of shale gas as well as free gas accumulation and seepage. According to the correlation statistics between the fracture line density and TOC in the Yucan 7 well (Figure 13C), the TOC is lower than 1.6% in the 786–836 m well section, the fracture line density and TOC are not high, and there is no obvious change trend. In the 838–849 m well section, the organic matter content is between 1.6 and 2.5%, and the fracture line density increases from 13 to 28 lines/m, indicating that, when the TOC is greater than 1.6%, the fracture density is largely affected by the TOC changes. Regarding the regional tectonics, Tang et al. (2019) proposed that the natural fractures in the upper Yangtze area mainly developed in the Qiyaoshan fault zone in the northwest and the Xuefengshan fault zone in the southeast. The TOC content was the highest (>2.4) in the northwest of the study area and was low in the southeast, which has a good corresponding relationship with the fracture development area. Therefore, the TOC can affect the physical properties of shale reservoirs by controlling the degree of fracture development.
Tectonic Movement
Shale gas belongs to the “self-generation and self-storage” accumulation model. Based on the characteristics of rock compactness of the study area, as well as the low porosity, degree of development, and distribution characteristics of fractures, these factors can have important geological significance for the accumulation and preservation of shale gas. However, tectonic movement has a larger significance on the reformation of the reservoir, in dual effect. On one hand, uplifting and squeezing are beneficial to the formation of fractures, increasing the storage space, connecting isolated pores, and constituting the main channel for shale gas desorption and flow. On the other hand, tectonic movement can also destroy the reservoir structure, transforming structural fractures into channels for shale gas to escape, causing the shale reservoirs to be invalidated. Therefore, determining whether the tectonic movement has been constructive or destructive to the Wufeng–Longmaxi Formation high-quality shale reservoir in southeastern Chongqing is a particularly important question.
Southeastern Chongqing has undergone multiple periods of tectonic movement after the deposition during the Early Silurian, presenting an NNE-trending tectonic pattern and developing a large number of fault zones. Combined with the characteristics of rich brittle minerals in the Wufeng–Longmaxi Formation, a large number of structural fractures have easily formed. The Wufeng–Longmaxi shales in the southeastern Chongqing show high porosity and permeability resulting from the developed pores and fracture systems (Liu et al., 2017b). Wu et al. (2011) predicted the fracture distribution in the black shale of the Longmaxi Formation in southeastern Chongqing and divided the fracture-favorable areas into three types. The first type of fracture-favorable area is mostly located in the large-scale fault development area and the axis of the anticline; the second type is located in the vicinity of some secondary faults and the axis of the syncline; and the third type is mostly located on the two wings of anticlines and synclines as well as on some small synclines and anticlines with weak structural deformations. Tang et al. (2019) indicated that a large number of natural fractures developed around the Qiyaoshan and Xuefengshan fault zones, which agrees with the findings of Wu et al. (2011).
The well-known large-scale Fuling shale gas field is the result of overpressure accumulation. Overpressure is a direct sign of a well-preserved shale gas reservoir, and the thermal evolution of organic matter and the pressurization of hydrocarbon generation are the main reasons for the abnormally high pressure in this area (Gao et al., 2019; Wang Q. et al., 2019). However, the Wufeng–Longmaxi Formation in the Pengshui area of southeastern Chongqing is under normal pressure, with a pressure coefficient between 0.8 and 1.2 (He X. et al., 2017). Nie et al. (2019) suggested that atmospheric shale gas reservoirs can be divided into four main types: (i) high-quality shale missing/thinning, (ii) early escape, (iii) fault failure, and (iv) residual syncline types, and their distribution and enrichment are mainly controlled by tectonic processes. Li et al. (2016) proposed that early and late tectonic uplift, the superimposition of multi-stage stress fields, and the development of high-angle fractures are the controlling factors that affect the maintenance of shale gas overpressure in the later stages. Yuan et al. (2020) then studied the atmospheric formation mechanism of the Longmaxi Formation in Pengshui and revealed that the Longmaxi Formation shale gas reservoir experienced overpressure during its geological development period. During the uplifting process, an overpressure rupture occurred, resulting in fractures that led to the loss of shale gas and the release of overpressure, transitioning the area from overpressure to normal pressure. It is therefore unclear why the Fuling and Pengshui areas are located so geographically close together with starkly opposing accumulation environments. One explanation may be related to the fact that since the Late Jurassic period, the South China Plate has been affected by the subduction of the Pacific slab, causing an intracontinental uplift movement from the southeast to northwest (Shu, 2012). The southeastern area of Chongqing is located in the periphery of the Sichuan Basin; therefore, it was uplifted and suffered strong denudation and deformation at ∼125 Ma. That tectonic movement was relatively long-lived, leading to the destruction of the preservation conditions of the Wufeng–Longmaxi Formation shale reservoir (Fan et al., 2019). However, the Fuling Jiaoshiba is located in the Sichuan Basin, and the structural uplift occurred at ∼80 Ma, with short duration, weak deformation, and thin denudation thickness (Fan et al., 2019). Therefore, it is likely that earlier structural uplift and longer-term and more intense denudation and deformation are the main factors that caused the two completely opposite accumulation environments. The structural fractures that have often developed in southeastern Chongqing are generally destructive to shale reservoirs.
Nevertheless, some structural fractures in southeastern Chongqing still exhibit constructive performance. As mentioned above, the Wufeng–Longmaxi Formation in southeastern Chongqing is divided into three sections, of which the optimal section for comprehensive evaluation of shale gas enrichment conditions is the first section: the black organic shale section at the bottom of the formation. An obvious detachment zone can be seen in the first section of the Wufeng–Longmaxi Formation in the Yucan 4 and Yucan 6 wells, and obvious scratches, steps, or smooth mirror surface characteristics can be seen in the core observations with well-developed fractures (Figures 5B–D). In addition, the on-site analytical gas content of the Wufeng–Longmaxi Formation in the Yucan 4 well is 0.96–2.7 m3/t, with an average of 1.62 m3/t, indicating that fractures still have a beneficial effect on the gas-bearing properties of shale and confirming the constructive effect of fracture development on shale gas enrichment.
Conclusion
The Wufeng–Longmaxi Formation mud shales in the northwestern part of the study area have a higher content of brittle minerals and organic carbon than those in the southeastern part in the southeastern Chongqing. The types of storage spaces include organic pores, clay mineral interlayer pores, intergranular pores, intragranular pores, and structural fractures. The high-quality shale reservoir is located in the lower part of the Wufeng–Longmaxi Formation, and features low porosity and low permeability. Tectonic fractures are the main shale gas storage spaces, and effectively improve the permeability of the reservoir to a certain extent.
The depositional environment, mineral component, TOC content, and tectonic movement are the main factors that cause the obvious differences from shale gas productions in the Jiaoshiba area. The deep-water anoxic depositional environment is conducive to the complete preservation of organic matter and has an important effect on improving the pores of organic matter and the brittleness of rocks. A high content of quartz was found to improve the rock compression resistance, brittleness, and a high proportion of clay minerals was found to enhance the interlayer pores of clay minerals. Specific surface area, pore volume, and degree of fracture development all have a positive correlation with the TOC content. As the most important factor controlling the shale reservoir environment of normal-pressure accumulation, tectonic movement destroyed the original overpressure accumulation that would have been similar to that of the modern Jiaoshiba area, yet also improved the permeability of the reservoir. Overall, tectonic movement was ultimately destructive to the reservoir.
Data Availability Statement
The original contributions presented in the study are included in the article/supplementary material, further inquiries can be directed to the corresponding author/s.
Author Contributions
JinW completed the writing and editing of the manuscript. XT and JiaW focused on the scientific issues involved in the manuscript. HZ, YZ, and DG were responsible for the physical property analysis of shale reservoir involved in the manuscript. XW, ZL, CZ, and GY have carried out the data analysis of shale mineral composition and sedimentary environment. All authors contributed to the article and approved the submitted version.
Funding
We are grateful to all teachers at the Chongqing University of Science and Technology for the analysis and guidance of experimental samples. This work was financially supported by the National Science Foundation of China (Grant Numbers 42072140 and 41502150), Natural Science Foundation Project of CQ CSTC (Grant Numbers cstc2018jcyjAX0523 and cstc2020jcyj), the Performance Incentive and Guidance Project for Scientific Research Institutions in Chongqing (Grant Number cstc2020jxjlX0002), the Special Project of performance incentive and guidance for scientific research institutions in Chongqing (Grant Number cstc2019jxjl90001), and the Pre-project of Chongqing Shale Gas Exploration and Development Company Limited (Grant Number CYS-FW-2020-0047).
Conflict of Interest
ZL was employed by the Chongqing Shale Gas Exploration and Development Company Limited and GY was employed by the China Huaneng Group Co., Ltd.
The remaining authors declare that the research was conducted in the absence of any commercial or financial relationships that could be construed as a potential conflict of interest.
Acknowledgments
We thank our scientific research team for their help and guidance in the field investigation.
References
Barratt, E., Joyner, L., and Halenda, P. (1951). The determination of pore volume and area distributions in porous substances. I. Computations from nitrogen isotherms. J. Manag. Eng. 24, 373–380. doi: 10.1021/ja01145a126
Brunauer, S., Emmet, P., and Teller, E. (1938). Adsorption of gases in multimolecular layers. J. Am. Chem. Soc. 60, 309–319. doi: 10.1021/ja01269a023
Bustin, R., Bustin, A., Cui, X., Ross, D., and Pathi, V. (2008). Impact of shale properties on pore structure and storage characteristics. Paper Presented at the Society of Petroleum Engineers shale Gas Production Conference in Fort Worth, Texas, SPE Paper 119892, Fort Worth, TX.
Chen, X., Fan, J., Zhang, Y., Wang, D., Chen, Q., Wang, W., et al. (2015). Subdivision and delineation of the Wufeng and Lungmachi black shales in the subsurface areas of the Yangtze platform. J. Stratigr. 39, 351–358.
Dai, J., Ni, Y., Gong, D., Feng, Z., and Han, W. (2017). Geochemical characteristics of gases from the largest tight sand gas field (Sulige) and shale gas field (Fuling) in China. Mar. Petrol. Geol. 79, 426–438. doi: 10.1016/j.marpetgeo.2016.10.021
Fan, C., Zhong, C., Zhang, Y., Qin, Q., and He, S. (2019). Geological factors controlling the accumulation and high yield of marine–facies shale gas: case study of the wufeng– longmaxi formation in the dingshan area of southeast sichuan china. Acta Geol. Sin. (Eng. Ed.) 3, 536–560. doi: 10.1111/1755-6724.13857
Gao, J., Zhang, J., He, S., Zhao, J., He, Z., Wo, J., et al. (2019). Overpressure generation and evolution in Lower Paleozoic gas shales of the Jiaoshiba region China: implications for shale gas accumulation. Mar. Petrol. Geol. 102, 844–859. doi: 10.1016/j.marpetgeo.2019.01.032
Gou, Q., and Xu, S. (2019). Quantitative evaluation of free gas and adsorbed gas content of Wufeng-Longmaxi shales in the Jiaoshiba area, Sichuan Basin, China. Adv. Geo Ener. Res. 3, 258–267. doi: 10.26804/ager.2019.03.04
Guo, T., and Zhang, H. (2014). Formation and enrichment mode of jiaoshiba shale gas field Sichuan Basin. Petrol. Expl. Dev. 41, 28–36. (in Chinese).
Guo, X. (2014). Rules of two-factor enrichment for marine shale gas in southern china—understanding from the longmaxi formation shale gas in sichuan basin and its surrounding area. Acta Geol. Sin. 88, 1209–1218. (in Chinese).
Guo, X., Hu, D., Li, Y., Liu, R., and Wang, Q. (2014a). Geological features and reservoiring mode of shale gas reservoirs in longmaxi formation of the jiaoshiba area. Acta Geol. Sin. (Eng. Ed.) 88, 1811–1821. doi: 10.1111/1755-6724.12347
Guo, X., Hu, D., Wen, Z., and Liu, R. (2014b). Major factors controlling the accumulation and high productivity in marine shale gas in the Lower Paleozoic of Sichuan Basin and its periphery: a case study of the Wufeng-Longmaxi Formation of Jiaoshiba area. Geol. China 41, 893–901. (in Chinese).
Guo, X., Li, Y., Tenger, B., Wang, Q., Yuan, T., Shen, B., et al. (2020). Hydrocarbon generation and storage mechanisms of deep–water shelf shales of Ordovician Wufeng Formation–Silurian Longmaxi Formation in Sichuan Basin China. Petrol. Explor. Dev. 47, 1–9. (in Chinese).
He, X., Gao, Y., Tang, X., Zhang, P., and He, G. (2017). Analysis of major factors controlling the accumulation in normal pressure shale gas in the southeast of Chongqing. Nat. Gas Geosci. 28, 654–664. (in Chinese).
He, Z., Hu, Z., Nie, H., Li, S., and Xu, J. (2017). Characterization of shale gas enrichment in the Wufeng Formation–Longmaxi Formation in the Sichuan Basin of China and evaluation of its geological construction–transformation evolution sequence. J. Nat. Gas Geosci. 28, 724–733. (in Chinese).
He, Z., Nie, H., and Zhang, Y. (2016). The main factors of shale gas enrichment of Ordovician Wufeng Formation–Silurian Longmaxi Formation in the Sichuan Basin and its adjacent area. Earth Sci. Front. 23, 8–17. (in Chinese).
Ji, L., Qiu, J., and Xia, Y. (2013). Micro-pore characteristics and methane adsorption properties of common clay minerals by electron microscope scanning. Acta Petrol. Sin. 2, 249–256.
Jiang, S., Peng, Y., Gao, B., Zhang, J., Cai, D., Xue, G., et al. (2016). Geology and shale gas resource potentials in the Sichuan Basin China. Ener. Explor. Expl. 34, 689–710. doi: 10.1177/0144598716657442
Jin, Z., Nie, H., Liu, Q., Zhao, J., and Jiang, T. (2018). Source and seal coupling mechanism for shale gas enrichment in upper Ordovician Wufeng Formation – Lower Silurian Longmaxi Formation in Sichuan Basin and its periphery. Mar. Petrol. Geol. 97, 78–93. doi: 10.1016/j.marpetgeo.2018.06.009
Li, S., Yuan, Y., Sun, W., Sun, D., and Jin, Z. (2016). The formation and destroyment mechanism of shale gas overpressure and its main controlling factors in Silurian of Sichuan Basin. Nat. Gas Geosci. 27, 924–931. (in Chinese).
Liang, M., Tan, X., Chen, X., Wang Ran, T., Wang, P., Tan, D., et al. (2018). Sequence stratigraphy of Wufeng–Longmaxi Formation in the southeastern Chongqing area and its geological significance. Coal Geol. Explor. 46, 40–51. (in Chinese).
Liu, J., Yao, Y., Elsworth, D., Liu, D., Cai, Y., and Dong, L. (2017a). Vertical heterogeneity of the shale reservoir in the lower Silurian Longmaxi Formation: Analogy between the southeastern and northeastern Sichuan Basin, SW China. Minerals 7:151. doi: 10.3390/min7080151
Liu, J., Yao, Y., Elsworth, D., Pan, Z., Sun, X., and Ao, W. (2016a). Sedimentary characteristics of the Lower Cambrian Niutitang shale in the southeast margin of Sichuan Basin, China. J. Nat. Gas Sci. Eng. 36, 1140–1150. doi: 10.1016/j.jngse.2016.03.085
Liu, J., Yao, Y., Liu, D., Pan, Z., and Cai, Y. (2017b). Comparison of three key marine shale reservoirs in the southeastern margin of the Sichuan basin, SW China. Minerals 7:179. doi: 10.3390/min7100179
Liu, J., Yao, Y., Zhu, Z., Cheng, L., and Wang, G. (2016b). Experimental investigation of reservoir characteristics of the upper Ordovician Wufeng Formation shale in middle–upper Yangtze region, China. Ener. Explor. Expl. 34, 527–542. doi: 10.1177/0144598716650553
Luo, Q., Zhong, N., Dai, N., and Zhang, W. (2016). Graptolite-derived organic matter in the Wufeng–Longmaxi Formations (Upper Ordovician–Lower Silurian) of southeastern Chongqing China: implications for gas shale evaluation. Int. J. Coal Geol. 153, 87–98. doi: 10.1016/j.coal.2015.11.014
Lv, D. W., Li, Z. X., Wang, D. D., Li, Y., Liu, H. Y., Liu, Y., et al. (2019). Sedimentary model of coal and shale in the paleogene lijiaya formation of the huangxian basin: insight from petrological and geochemical characteristics of coal and shale. Ener. Fuels 33, 10442–10456. doi: 10.1021/acs.energyfuels.9b01299
Lv, D. W., Song, Y., Shi, L. Q., Wang, Z. L., Cong, P. Z., and Loon, A. J. V. (2020). The complex transgression and regression history of the northern margin of the Palaeogene Tarim Sea (NW China), and implications for potential hydrocarbon occurrences. Mar. Petrol. Geol. 112:104041. doi: 10.1016/j.marpetgeo.2019.104041
Mou, C., Ge, X., Xu, X., Zhou, K., Liang, W., and Wang, X. (2014). Lithofacies palaeogeography of the late ordovician and its petroleum geological significance in middle-upper yangtze region. J. Palaeogeogr. 16, 427–440. (in Chinese).
Mu, C., Zhou, K., and Liang, W. (2011). Early paleozoic sedimentary environment of hydrocarbon source rocks in the middle–upper yangtze region and petroleum and gas exploration. Acta Geol. Sin. 85, 526–532. (in Chinese).
Nie, H., Jin, Z., Bian, R., and Du, W. (2016). The “source-cap hydrocarbon-controlling” enrichment of shale gas in Upper Ordovician Wufeng Formation-Lower Silurian Longmaxi Formation of Sichuan Basin and its periphery. Acta Petrol. Sin. 37, 557–571. (in Chinese).
Nie, H., Wang, H., He, Z., Wang, R., Zhang, P., and Peng, Y. (2019). Formation mechanism distribution and exploration prospect of normal pressure shale gas reservoir: a case study of Wufeng-Longmaxi Formation in Sichuan Basin and its periphery. Acta Petrol. Sin. 40, 131–143. (in Chinese).
Pan, R., Gong, Q., Yan, J., and Jin, J. (2016). Elements and gas enrichment laws of sweet spots in shale gas reservoir: a case study of the Longmaxi Fm in Changning Block, Sichuan Basin. Nat. Gas Industr. B 3, 195–201. doi: 10.1016/j.ngib.2016.05.003
Pu, B., Jiang, Y., Wang, Y., Bao, S., and Liu, X. (2010). Reservoirforming conditions and favorable exploration zones of shale gas in lower Silurian Longmaxi formation of Sichuan Basin. Acta Petrol. Sin. 31, 225–230. (in Chinese).
Ross, D., and Bustin, R. (2008). Characterizing the shale gas resource potential of Devonian–Mississippian strata in the Western Canada sedimentary basin: application of an integrated formation evaluation. AAPG Bull. 92, 87–125. doi: 10.1306/09040707048
Shen, J., Yang, J., Zhou, F., Zhang, J., Yang, G., Qiu, Y., et al. (2013). Study of kerogen type index through the calculation of organic elements. J. North. Petrol. Univ. 37, 24–31.
Shu, L. (2012). Liangshu. an analysis of principal features of tectonic evolution in South China Block. Geol. Bull. China 31, 1035–1053. (in Chinese).
Su, W., Li, Z., Ettensohn, F., Johson, M., Huff, W., Wang, W., et al. (2007). Tectonic and eustatic control on the distribution of black–shale source beds in the Wufeng and Longmaxi formations (Ordovician–Silurian), South China. Front. Earth Sci. China 1, 470–481. doi: 10.1007/s11707-007-0058-6
Tan, L., Xu, Y., Li, D., Cheng, L., and Zeng, C. (2015). Geological condition of shale gas accumulation and favorable area prediction for the wufeng-longmaxi formations in southeastern chongqing. Acta Geol. Sin. 89, 1308–1317. (in Chinese).
Tang, X., Jiang, S., Jiang, Z., Li, Z., He, Z., and Zhu, D. (2019). Heterogeneity of paleozoic wufeng–longmaxi formation shale and its effects on the shale gas accumulation in the Upper Yangtze Region China. Fuel 239, 387–402. doi: 10.1016/j.fuel.2018.11.022
Wang, G., Zhu, T., Wang, H., Wu, J., Du, W., Feng, D., et al. (2019). Integrated Sequence stratigraphic division and vertical distribution characteristics of marine shale: a case study of the wufeng formation–longmaxi formation in southeastern sichuan basin. Acta Sedimentol. Sin. 37, 330–344. (in Chinese).
Wang, H., Liu, Y., Dong, D., Zhao, Q., and Du, D. (2013). Scientific issues on effective development of marine shale gas in southern China. Petrol. Explor. Dev. 40, 574–579. (in Chinese).
Wang, Q., Wei, X., Wei, F., Yan, J., and Wan, L. (2019). Overpressure in shale gas reservoirs of Wufeng–Longmaxi formations Fuling area southeastern Sichuan Basin. Petrol. Geol. Exp. 41, 333–340. (in Chinese).
Wang, R. Y., Hu, Z. Q., Long, S. X., Zhao, J. H., Dong, L., Du, W., et al. (2019). Differential characteristics of the Upper Ordovician-Lower Silurian Wufeng-Longmaxi shale reservoir and its implications for exploration and development of shale gas in/around the Sichuan Basin. Acta Geol. Sin. (Eng. Ed.) 93, 520–535. doi: 10.1111/1755-6724.13875
Wang, R. Y., Nie, H. K., Hu, Z. Q., Liu, G. X., Xi, B. B., and Liu, W. X. (2020). Controlling effect of pressure evolution on shale reservoirs: a case study of the Wufeng-Longmaxi Formation in the Sichuan Basin. Nat. Gas Indust. 40, 1–11. (in Chinese).
Wang, S., Jiao, W., Fang, G., Wang, F., Zhang, Z., Wang, Q., et al. (2017). Geochemical features and genesis of shale gas of Wufeng–Longmaxi formation in southeastern chongqing. Mar. Origin Petrol. Geol. 22, 77–84. (in Chinese).
Wang, Z. (2015). Breakthrough of Fuling shale gas exploration and development and its inspiration. Oil Gas Geol. 36, 1–6. (in Chinese).
Wu, L., Ding, W., Zhang, J., Li, Y., Zhao, S., and Hu, L. (2011). Fracture prediction of organic–enriched shale reservoir in lower silurian longmaxi formation of southeastern chongqing area. J. Oil Gas Technol. 33, 43–46. (in Chinese).
Xie, C., Zhang, J., Li, Y., and Wang, X. (2013). Characteristics and gas content of the lower cambrian dark shale in well yuke 1 southeast chongqing. Oil Gas Geol. 34, 11–15. (in Chinese).
Xu, E., Li, Z., and Yang, Z. (2015). Thermal and hydrocarbon generation history of Wufeng and longmaxi shales in pengshui area eastern sichuan basin: a well PY1 case study. Etrol. Geol. Exp. 37, 494–499. (in Chinese).
Yuan, Y., Fang, Z., He, X., Li, S., Peng, Y., and Long, S. (2020). Normal pressure formation mechanism of longmaxi shale gas in Pengshui and its adjacent areas. Reserv. Eval. Dev. 10, 9–16. (in Chinese).
Zhang, C., Jiang, Z., Guo, Y., and Zhang, W. (2013). Geochemical Characteristics and paleoenvironment reconstruction of the longmaxi formation in southeast sichuan and northern guizhou. Geol. Sci. Technol. Inform. 32, 124–130. (in Chinese).
Zhao, D., Guo, Y., Wang, G., Bai, W., Zeng, C., Jiao, W., et al. (2020). Sedimentary characteristics of shales graptololite and Its implications for high–quality shale gas reservoirs in the Wufeng-Longmaxi formation shales in southeast Chongqing. J. Henan Polyt. Univ. (Nat. Sci.) 39, 26–36. (in Chinese).
Zhou, L., Su, J., Huang, J., Yan, J., Xie, X., Gao, S., et al. (2011). A new paleoenvironmental index for anoxic events—Mo isotopes in black shales from Upper Yangtze marine sediments. Sci. China Earth Sci. 41, 309–317. (in Chinese).
Keywords: shale reservoir, physical characteristics, the Wufeng formation, the Longmaxi formation, southeastern Chongqing
Citation: Wang J, Tan X, Wang J, Zhang H, Zhang Y, Guo D, Wang X, Lei Z, Zeng C and Yao G (2021) Characteristics and Genetic Mechanisms of Normal-Pressure Fractured Shale Reservoirs: A Case Study From the Wufeng–Longmaxi Formation in Southeastern Chongqing, China. Front. Earth Sci. 9:661706. doi: 10.3389/feart.2021.661706
Received: 31 January 2021; Accepted: 26 March 2021;
Published: 05 May 2021.
Edited by:
Dawei Lv, Shandong University of Science and Technology, ChinaReviewed by:
Yanbin Yao, China University of Geosciences, ChinaRuyue Wang, SINOPEC Exploration & Production Research Institute, China
Copyright © 2021 Wang, Tan, Wang, Zhang, Zhang, Guo, Wang, Lei, Zeng and Yao. This is an open-access article distributed under the terms of the Creative Commons Attribution License (CC BY). The use, distribution or reproduction in other forums is permitted, provided the original author(s) and the copyright owner(s) are credited and that the original publication in this journal is cited, in accordance with accepted academic practice. No use, distribution or reproduction is permitted which does not comply with these terms.
*Correspondence: Xianfeng Tan, eGlhbmZlbmd0YW44Mjk5QDE2My5jb20=; Jia Wang, d2FuZ2ppYUBjcXVzdC5lZHUuY24uY29t