- 1Geological Survey of Spain (IGME, CSIC), Madrid, Spain
- 2Portuguese Institute for Sea and Atmosphere IPM, Lisbon, Portugal
- 3Spanish Institute of Oceanography (IEO, CSIC), Málaga, Spain
The aim of this work is to make a synthesis at regional scale focused on the geophysical characterization of submarine faults around the Iberian margin to identify active structures and analyze their development in the framework of the present plate organization. Most of these submarine faults show seabed morphological expressions mapped with high-resolution swath bathymetry data, high-resolution parametric sub-bottom profiles and multichannel seismic profiles. Present active tectonics, deformation, seismicity, and tsunami-affected coastal areas is mainly focused on south Iberia at the Eurasian and Nubia plate boundary. Submarine active faults in these areas are represented by long strike-slip fault systems and arcuate fold-thrust systems. Their development takes place in response to present NW-SE convergence between the Eurasian and Nubia plates. We propose a strain partitioning model of the plate boundary into simple and pure shear zones to explain the distribution and mechanisms of active submarine faults along the Gulf of Cádiz, Gibraltar Arc and Alborán Sea in response to the present-day shear stress orientation. Nevertheless, deformation is also focused in the NW Iberian margin. Thus, along the Galician and Portuguese margin, several submarine faults mapped as thrust fault systems with high-seismic activity along the Iberian ocean-continent transition reflect the re-activation of former structures. We suggest that submarine active faults in the NW and W Iberia are also the response to the eastwards transfer of short-offset transform faults of the Mid Atlantic Ridge into the oceanic Iberian along a weakness as the former plate boundary between the oceanic Iberia and Eurasia domains. The distribution and activity of submarine faults mapped in this work from geophysical and bathymetric data are in good agreement with geodetic data and focal mechanisms.
Introduction
The Iberian Peninsula is bounded by a Cenozoic convergent margin between the Eurasia and former Iberian plates along their northern edge (North and Northwest Iberian Margin), and by a complex transform boundary (Gulf of Cádiz and Alborán Sea) to the south, between the Eurasia and Nubia plates (Figure 1). The changes in stress direction from N-S during the early Cenozoic to NW-SE since late Neogene have triggered the development of new tectonic structures together with the reactivation of older structures around the plate boundaries of the Iberian Peninsula. A representative case is the accretionary wedge of the Gulf of Cadiz which appears affected by a later complex system of long submarine strike-slip faults (Medialdea et al., 2004; Rosas et al., 2009; Zitellini et al., 2009).
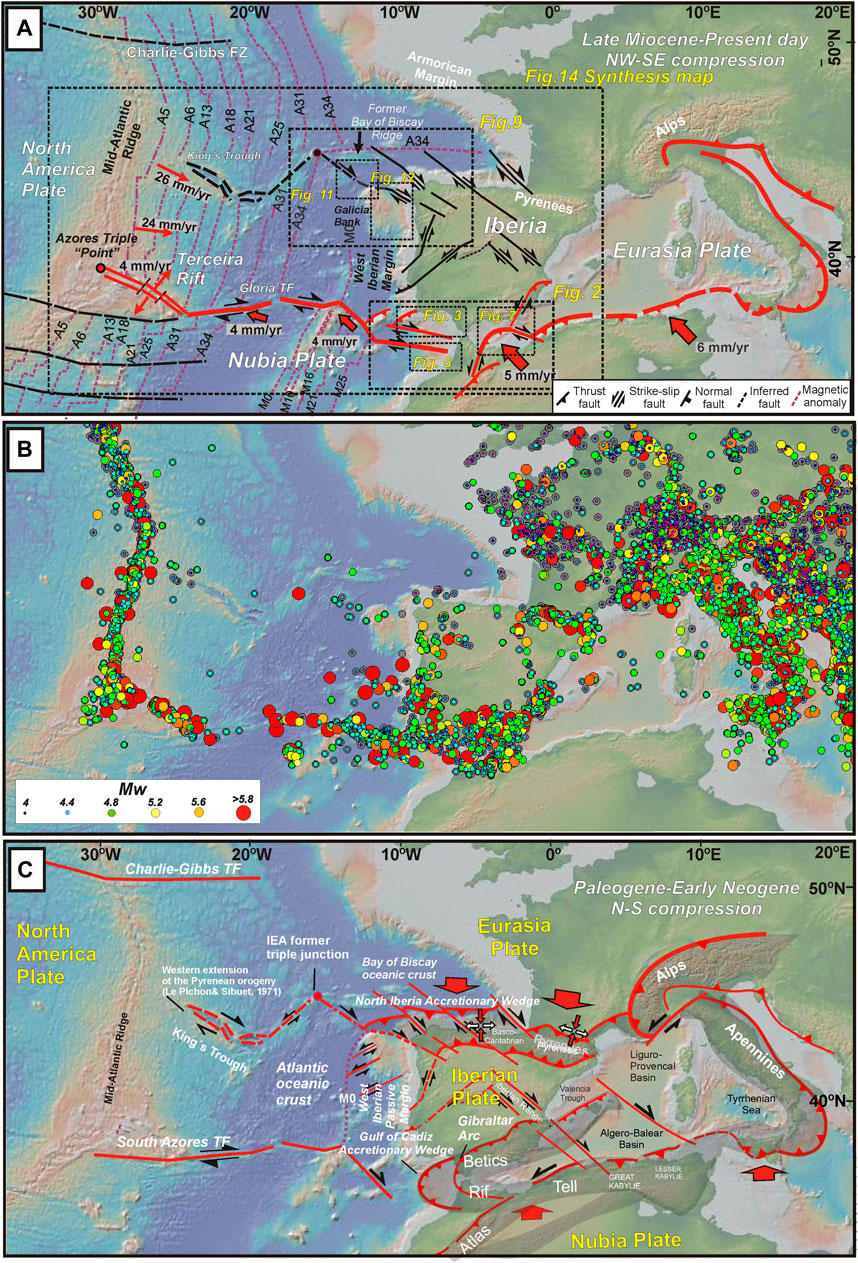
FIGURE 1. (A) Summary of tectonic structures affecting the Iberian Peninsula. Offshore data modified from Maldonado et al. (1999), Somoza et al. (2019). Onshore data comes from Vegas et al. (2008). Location of regional figures is also located. FZ: Fracture Zone. GoC: Gulf of Cádiz; GA: Gibraltar Arc; Alb: Alborán Sea. (B) Distribution of earthquakes of magnitude Mw > 4 around the Iberia Peninsula. Source: Seismic Hazard Harmonization in Europe (SHARE), Giardini et al. (2013). (C) Alpine tectonic structures in Iberian in response to the convergence between Iberia, Africa, and Eurasia. Modified from Somoza et al. (2019); Terrinha et al., (2019), and Terrinha et al. (2020). Background bathymetry from http://www.geomapapp.org (Ryan et al., 2009).
Several works have aimed to determine the source of the famous Mw 8.5–8.7, 1755 Lisbon tsunami earthquake event in the Gulf of Cádiz, Southwest Iberian Margin (e.g, Terrinha et al., 2003; Zitellini et al., 2009). The source of the aforementioned tsunami in the cities of Cádiz and Lisbon was initially attributed to the NE-SW Marques of Pombal Fault (e.g., Zitellini et al., 1999; Gràcia et al., 2003b) (Figure 1). However, scaling the source characteristics of the February 12th, 2007, Mw 6.0 Horseshoe earthquake, it was suggested another fault with a length of 230–315 km as potential source (Stich et al., 2006). This led to relate the potential source of the 1755-Lisbon tsunami to large-scale WNW-ESE dextral strike-slip faults affecting the sedimentary cover over the continental and the oceanic basements. These structures were identified on multichannel seismic profiles by Medialdea et al. (2009b) and seabed mapping as SWIM (South West Iberian Margin) lineaments on the compilation of multibeam bathymetry made by Zitellini et al. (2009) in the Gulf of Cádiz.
Otherwise, the North and Northwest Iberian Margin have been affected by subduction of the oceanic lithosphere of the Bay of Biscay as a consequence of the early Cenozoic collision between the Eurasian and Iberian plates (e.g., Le Pichon et al., 1971; Malod et al., 1993). The oblique convergence between the Eurasian and Iberian plates since the late Cretaceous caused the formation of the Alps-Pyrenees intracontinental collisional orogen to the east (e.g., Srivastava et al., 1990; Sibuet et al., 2004), that progressed westwards to a continent-ocean collision with subduction of the Bay of Biscay oceanic lithosphere beneath the North Iberian Margin (Sibuet and Collette, 1991). Afterwards the stress field transmitted to the Iberian Peninsula changed from N-S to NW-SE from late Miocene to present-day (e.g., Andeweg et al., 1999). In addition, the West and Northwest Iberia margins are also affected by the propagation of stress generated by the spreading of the Mid-Atlantic Ridge (MAR) and the Bay of Biscay Ridge during the Cenozoic (Figure 1). At present, the spreading of the Mid-Atlantic Ridge north of the Azores Triple Junction has been estimated to be at rated of 24 and 26 mm/yr (Argus et al., 1989; Miranda et al., 2014) (Figure 1).
The aim of this work is to make a synthesis at regional scale focused on the geophysical characterization of submarine faults around the Iberian margins to identify offshore active structures, with seismogenic and tsunamigenic potential. In this work faults are considered to be active if they show, at least, one of these characteristics: 1) seafloor expression on high-resolution bathymetry; 2) Deformation and/or displacement of the sea floor and the most recent sediments on ultra-high resolution seismic sections; 3) prominent fault scarps uncovered by recent sediments; 4) location of swarms of earthquakes near the submarine fault trace.
Following these criteria, we review and map the main active submarine faults around the Iberian Margins, which are mainly concentrated along the southern Eurasia-African plate boundary (Figure 1B), along with re-activated tectonic structures related to the former Cenozoic subduction in the northern Iberian Margin. Finally, based on our new synthesis map, we present a model of distribution of the present active submarine faults linked to seismicity around Iberia and analysed the available geodetic data. We propose that this distribution is the result of the remnant NW-SE convergence between the Eurasian and Nubia plates, but also by the propagation of stress from the ocean spreading of MAR to the west Iberian margin.
Geological Setting
The South Iberian Margin: Oblique Convergence Between the Eurasia and African Plates
The Gulf of Cádiz region, located to the west of the Gibraltar Arc, offshore SW Iberia and NW Morocco, has been increasingly recognized as a critical site for tectonics related to the Africa (Nubia)-Iberia plate boundary (e.g., Sartori et al., 1994; Maldonado et al., 1999, Gutscher et al., 2002, 2009; Medialdea et al., 2004; Terrinha et al., 2003; Rosas et al., 2009; Zitellini et al., 2009; Martínez-Loriente et al., 2014; Ramos et al., 2017a, b, c, 2020). This plate boundary extends along the Gloria fault zone to the Azores Triple Junction to the west (Miranda et al., 2014) (Figure 1A). In the Gulf of Cádiz the average direction of the Maximum Horizontal Compressive Stress (Shmax) deduced from earthquake focal mechanisms is N45W (Ribeiro et al., 1996; Stich et al., 2006; Pedrera et al., 2011). Present-day rate of approximately 4–5 mm yr−1 of oblique convergence between Africa and Iberia has been reported at this plate boundary (e.g., Nocquet and Calais, 2004; Stich et al., 2006) (Figure 1A).
The bathymetric map shows a huge lobe occupying almost the entire Gulf of Cádiz, which is attributed to an accretionary wedge emplaced in the late Miocene times associated with an east-dipping subduction zone close to the Gibraltar Arc (Maldonado et al., 1999; Gutscher et al., 2002). The accretionary wedge is represented in seismic profiles by the Allochthonous Unit of the Gulf of Cádiz (AUGC), characterized by a chaotic seismic signature and covered by late Miocene to Quaternary sediments (Maldonado et al., 1999; Medialdea et al., 2004). The Late Miocene to present-day NW–SE convergent movement of Africa with respect to Iberia generates deformation in the Gulf of Cádiz through strain partitioning accommodated by strike-slip faults and shear zones (Terrinha et al., 2009) and thrust reactivation along the Southwest Iberian Margin, offshore Algarve Basin (south Portugal and southwest Spain; Ramos et al., 2017a). Moreover, this thrust system presents an associate set of oblique ramps with NW-SE orientation, the location of which is inherited from the Mesozoic extensional transfer zones of the passive margin (Ramos et al., 2020). Numerous authors have also documented the domain of the Algarve Basin and the Gulf of Cádiz as tectonically active (Gràcia et al., 2003a; Gràcia et al., 2003b; Duarte et al., 2009; Duarte et al., 2010; Terrinha et al., 2009; Zitellini et al., 2009; Martínez-Loriente et al., 2013; Martínez-Loriente et al., 2014). These major compressional structures are interpreted as south-verging basement-involving blind thrusts, being responsible for the southeastward stair-stepped geometry of the SW Iberian margin observable in the basement, the base of the Miocene unconformity and the present-day bathymetry, as the case of the uplift and tilting of the Guadalquivir Bank. Their orientation is perpendicular to the orientation of the present-day convergence between Africa and Eurasia (e.g., Olaiz et al., 2009).
A distributed deformation in the Alborán Sea region, to the East of the Gibraltar Arc, has been classically defined as diffuse in relation to the seismicity pattern (Vegas, 1991; Jímenez-Munt et al., 2001; Buforn et al., 2015; Grevemeyer et al., 2015; Palano et al., 2015), however recent geophysical and geological studies points to concentration of crustal deformation along several striking NE-SW and NW-SE fault zones in this region (Negredo et al., 2002; Serpelloni et al., 2007; Neres et al., 2016). The current plate boundary between Nubia (West Africa) and Eurasia is related to the evolution of the Betic-Rif orogenic system which was generated by the westward drift of the Alborán Crustal Domain in relation to the westward retreat of a subduction slab (Lonergan and White, 1997; Jolivet and Faccenna, 2000; Pedrera et al., 2011; Molina-Aguilera et al., 2019) (Figure 1C). This orogen is characterized by an arcuate front (Gibraltar Arc) and the development of the Alborán Basin in the backarc region between two main cordilleras, respectively in the south of Iberia (Betic Ranges) and northern Africa (Rif Ranges). During the late Tortonian, the regional change of convergence from N-S to NW-SE between the main plates caused a general inversion of the region. As a consequence an indenter deformation has been developed in the area, the Alborán Ridge constituted by an African crustal domain (Gómez de la Peña et al., 2018) works as an indenter towards the northern margin of the Alborán Basin (Estrada et al., 2018a) producing a conjugated system of left lateral (NE-SW to NNE-SSW) and right-lateral strike-slip faults (Figure. 2). The left-lateral strike-slip family correspond to the Trans Alborán Shear Zone currently represented by the Al Idrissi fault zone (Galindo-Zaldívar et al., 2018; Gràcia et al., 2019; Vázquez et al., 2021a). This fault zone connects southwards with the active onshore faults of the Al Hoceima region and the southwestern Rif deformation (Chalouan et al., 2014; d’Acremont et al., 2014; Galindo-Zaldívar et al., 2015; Lafosse et al., 2018), and northwards with the active faults of the Adra region in southern Iberia (Gràcia et al., 2012; Galindo-Zaldívar et al., 2013) and the Eastern Betic Shear Zone by means of La Serrata-Carboneras Fault (Gràcia et al., 2006; Borque et al., 2019). The right-lateral strike-slip family corresponds to the Yusuf Fault (Mauffret et al., 2007; Martínez García et al., 2017), connected to the east with the Algerian compressive region, which accomodates the current deformation between Nubia and Eurasia (Vázquez et al., 2021b).
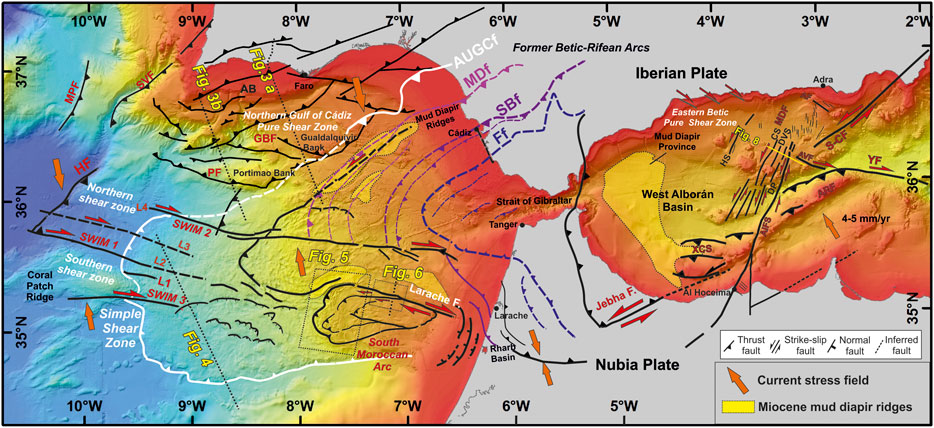
FIGURE 2. Synthetic map of active submarine faults in the Gulf of Cadiz and the Alborán Sea along the Iberia-Africa plate boundary and location of figures. The plate boundary is partitioned into the main stress areas: (i) the SW Iberian Margin simple shear zone; (ii) the Gulf of Cadiz pure shear zone; (iii) the South Moroccan compressional arc; and (iv) the Eastern Betic pure shear zone. Abbreviations of the submarine active faults are listed in Table 1. CS: Calahonda Sound Fault; DP:Djibouti Passage Fault; DVS: Djibouti Ville Fault: HS: Herradura Sound Fault; XCS: Xauen Compressive System; AB: Algarve Basin; Units of the Betic-Rifian arc (modified from Medialdea et al., 2009a) are also shown: Ff: Flysch Units front; SBf: Subbetic front; MDf: Mud diapiric front, AUGCf: Boundary of the Allochthonous Unit of the Gulf of Cádiz; IB:Ibn-Batouta Bank LHB: La Herradura Bank Bathymetry from SWIM compilation (Zitellini et al., 2009) and EMODnet project (http://www.emodnet.eu). Present-day stress fields from Pedrera et al. (2011).
The North and Northwest Iberian Margin: A Cenozoic Convergent Plate Boundary Between Iberia and Eurasia
The North Iberian Margin was deformed by subduction of the oceanic lithosphere of the Bay of Biscay due to the early Cenozoic convergence between the Eurasian and Iberian plates (e.g., Le Pichon et al., 1971; Malod et al., 1993). The oblique convergence between the Eurasian and Iberian plates progressed to a continent-ocean collision with subduction of the Bay of Biscay oceanic lithosphere beneath the North Iberian margin, thus provoking uplift and deformation of the Cantabrian range (Figure 1C; e.g., Le Pichon and Sibuet, 1971; Boillot et al., 1979; Pulgar et al., 1996; Cadenas et al., 2018). The North Iberian margin was modeled with a south or southeast dipping oceanic crust beneath the outer part of the margin (Boillot et al., 1979), thereby forming an accretionary prism (Figure 1C). The estimated amount of underthrusting of the southern Bay of Biscay varies from a maximum of 120 km to a minimum of 40 km (e.g., Pulgar et al., 1996; Gallastegui and Pulgar, 2002). Since Oligocene times, the compressional deformation was transferred to the south through the Iberian plate, resulting in the development of several mountain ranges. Convergence between Eurasia (Iberia being incorporated) and African plates through the Alborán microplate transmitted to the Iberian Peninsula changed from N-S to NW-SE from late Miocene to present-day (e.g., Dewey et al., 1989; Andeweg et al., 1999), defining the current location of the Eurasian-African plate boundary in south Iberia (Figure 1A).
General Methodology and Data
This study is mainly based on a large array of data including multichannel seismic (MCS), ultra high-resolution parasound sub-bottom profilers (SBP) and high-resolution multibeam data (MBES) acquired during the MOUNDFORCE-2007 cruise aboard the RV L´Atalante (Somoza, 2007), MVSEIS-2008 cruise aboard the RV Hespérides (Somoza and UTM-CSIC, 2018), and SUBVENT-2 cruise aboard the RV Sarmiento de Gamboa (Somoza et al., 2019) in the Gulf of Cádiz and West Moroccan margin and BREOGHAM-2005, aboard RV Hespérides in the NW Iberian Margin (Somoza et al., 2005). A complete list of data used in this work with detail information on the cruises, methods and configurations is provided in the Supplementary Table S1.
The following MBES have been used to make 3d bathymetric images from the Galicia margin and the Gulf of Cadiz: Simrad EM-12S, 13 kHz, Konsberg EM-120 (12 kHz), KONSBERG EM-12 dual (12 kHz), KONSBERG EM-120 (13 kHz), and Atlas DS 1x1 14–16 kHz (12 kHz).
In the N and NW Iberian Margin (Galicia and Cantabrian regions), we use a MBES dataset acquired for the Spanish Exclusive Economic Zone (EEZ) and Extended Continental Shelf (ECS) mapping programs of the Galicia region at 150 m resolution (Somoza et al., 2005; Somoza et al., 2019). In the Gulf of Cádiz, we use the SWIM bathymetric compilation as multibeam background data at an average resolution of 250 m (Zitellini et al., 2009). For background bathymetry in other areas, the EMODnet project data (http://www.emodnet.eu/bathymetry) and Marine Geoscience Data Systems (MGDS), Global Multi-Resolution Topography data (GMRT, Ryan et al., 2009) were used. Multi-resolution DTMs was used to generate regional sun-shaded image renders, perspective views and to extract margin-wide bathymetric profiles using Fledermaus™ software in order to interpret the submarine landscapes. It was also used to generate derivative products such as slope angle maps by means of ArcGIS™.
Two main types of parasound sub-bottom profilers (SBP) has been used to acquire ultra-high resolution profiles: TOPAS (Topographic Parametric Echosounder) and CHEOPS. System details are summarized in Supplementary Table S1.
Multichannel seismic profiles were acquired during two cruises. The BREOGHAM-2005 survey in the Galicia Margin and Celtic Sea used a seismic source of six BOLT™ guns (1500 LL model) and two SLEEVE™ guns with a total volume of 22.85 L and 50 m shooting interval. The acquisition consisted of an analogical TELEDYNE streamer composed of 24 sections with a total length of 2,400 m. The MOUNDFORCE-2007 in the Gulf of Cádiz and western Moroccan Margin used as a source an array of 14–16 guns G.I. GUN and BOLT with a total volume of 56.1 L and 50–75 m shooting interval. The acquisition was performed with a SERCEL streamer composed of 360 channels with a length of active sections of 4,500 m, and a total length of 5.000 m. Kingdom Suite software has been used to perform seismic images both of the SBP and MCS profiles. For the SW Iberian margin, we took into account 2D and 3D multichannel reflection seismic data (i.e., Ramos et al., 2017a). The seismic interpretation was calibrated with 72 wells in the area, both offshore and onshore.
Results
Mapping Quaternary Active Submarine Faults Along the Africa-Eurasian Plate Boundary
A new map with a synthesis of the submarine faults between the Gulf of Cádiz and Alborán Sea has been made in this work (Figure 2). This map has been constructed on the basis of high-resolution multibeam bathymetry of the Gulf of Cádiz and Alborán Sea, combined with multichannel and high-resolution seismic data (Medialdea et al., 2009b; Zitellini et al., 2009). This map allows linking the main faults in the Gulf of Cádiz and in the Alborán Sea along the Africa-Eurasian plate boundary (Figure 2).
Quaternary Active Submarine Faults in the Gulf of Cádiz
The Case of the SW Iberian Margin: Active Inversion of a Passive Margin
The thrust system that affects the morphology of the SW Iberian margin locally controls the present-day bathymetry and consequently the pathway of the Mediterranean Outflow Water as it flows along the Gulf of Cádiz middle continental slope from the Strait of Gibraltar (Figure 3). The Mediterranean Outflow Water has determined the development of a complex contourite depositional system during Pliocene-Quaternary times (Hernández-Molina et al., 2003). Moreover, the main clusters of seismic events in the northern Gulf of Cadiz (Ribeiro et al., 1996; Pedrera et al., 2011) lay parallel and aligned to the thrust faults interpreted by Ramos et al. (2017a) (Figures 3C,D). The focal mechanisms measured in the margin are coherent with N-S to NW-SE directed shortening (e.g., Palano et al., 2013). Thrust fault solutions are compatible with the presence of the E-W o WSW-ENE trending basement-involved thrust faults affecting the Algarve Basin, while the location of the strike-slip fault solutions is consistent with the N-S to NW-SE trending lateral ramps and tear faults of the thrust system. The main seismic cluster sits on the most representative bathymetric highs (Guadalquivir and Portimão banks), which correspond to inverted Cenozoic structures associated to the activity of the most southern thrust (Figure 3). These highs related to neotectonic activity can control in turn the activity of the bottom currents and genesis of contourite features. South of the Algarve Basin however, the generalized absence of seismic activity could indicate that the deformation is shallow (Sallarès et al., 2011) and related to the gravitational effects of shale and evaporite mobility (Medialdea et al., 2004).
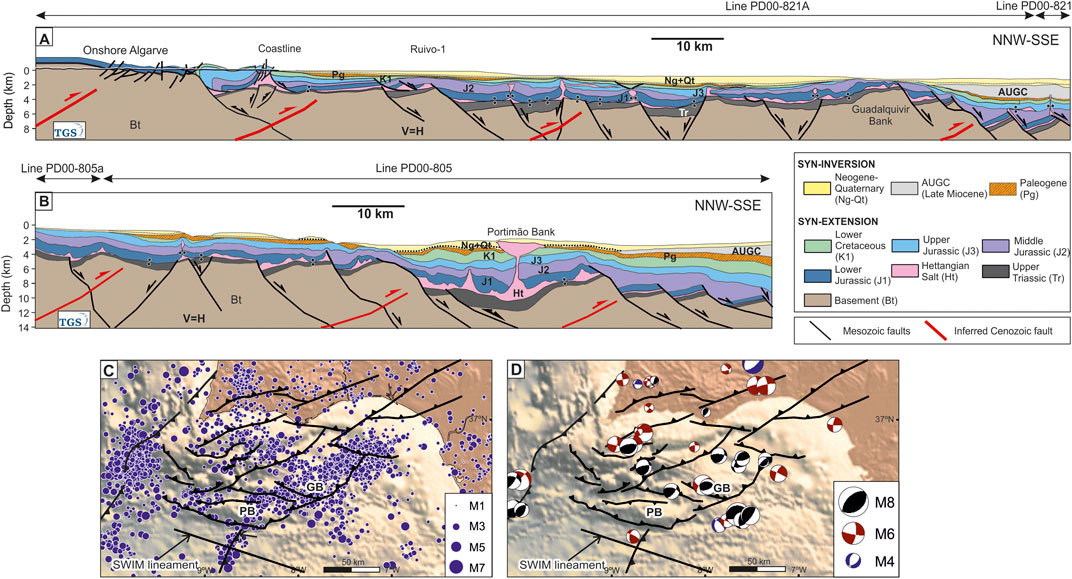
FIGURE 3. Cross-sections through the Algarve Basin showing the major ontractional structures responsible for the inversion of the SW Iberian margin. (A) Interpreted seismic section through the central Algarve Basin, including interpretation of the onshore basin. (B) Interpreted seismic section through the western Algarve Basin. Pairs of black dots represent salt welds. See Figure 2 for location. Modified after Ramos et al. (2017a). See this reference for further discussion on the seismic interpretation and supplementary data for seismic sections. (C) Location of earthquake epicentres in the SW Iberian margin in relation with the thrust fault system described in the text in more detail. (D) Location of focal mechanisms compiled from several earthquakes: red for strike-slip faulting, black for thrust faulting, and blue for normal faulting in relation with the thrust faults of SW Iberia. Modified after Palano et al. (2013) and Ramos et al. (2017a). GB: Guadalquivir Bank; PB: Portimão Bank.
On seismic profiles, the AUGC lies folded directly on the southern flank of the Guadalquivir (Figure 3) and Portimão banks, demonstrating that these uplifted structures acted as physiographical barriers to the progression of the AUGC to the NW, and therefore, attesting a more complex inversion history of the margin. Although these structures are active nowadays, at least four main stages of shortening were documented by interpreting the seismic data present in the Gulf of Cadiz: late Cretaceous to early Paleogene, late Paleogene to early Miocene, middle to late Miocene, and late Pliocene to present day (Ramos et al., 2017a).
The opposing dip between the Mesozoic extensional faults and the south-verging thrust system (Figure 3) is interpreted by the reactivation of low-angle thrusts and cleavage within the Paleozoic basement during the Cenozoic inversion, in contrast with the south-dipping extensional faults accommodating extension towards the south. The main inversion structure (the southern thrust) coincides in orientation and location with the necking domain of the margin (Figure 3). This suggests that the thrust fault would have taken advantage of the north-tilted continental crust and Moho during the Mesozoic extensional necking phase (Ramos et al., 2017b).
Simple Shear Zone: Deep-Rooted Right-Lateral Strike-Slip Faults
Along the Gulf of Cádiz, a major system of linear and sub-parallel strike-slip faults has been reported based on the SWIM compilation of multibeam bathymetry (Zitellini et al., 2009). This system has a clear reflection on the seafloor morphology. The angular relationship between en-echelon fold axes affecting the surface sediments and the SWIM faults indicates a dextral strike-slip movement (Rosas et al., 2009). Four SWIM faults were described (Zitellini et al., 2009): The SWIM-1 and SWIM-3 bounds the Coral Patch Ridge (CPR), whereas the SWIM-2 is located further north (Figure 2). In contrast, Rosas et al. (2009) show a system of major bathymetric lineaments termed as L1 to L4 from south to north. L1 and L2 coincides with two of the faults already mapped by Medialdea et al. (2004), Medialdea et al. (2009a) in the Gulf of Cádiz. SWIM-1 and SWIM-2 from Zitellini et al. (2009) coincides with L2 and L4 lineaments from Rosas et al. (2009), but L1 and L3 are intercalated between SWIM faults (Figure 2).
Interpretation of the seismic profile MOUNDFORCE-06 shows that SWIM-1 (L2), L1 and SWIM-3 lineaments linked in depth and belong to the same flower-like structure (Figure 4). Mud volcanoes as Porto and Soloviev (Pinheiro et al., 2003; Medialdea et al., 2009b) appear to be closely related to the SWIM-1 and SWIM-3 strike-slip faults respectively (Figure 2). This points to active fluid expulsion along the transpressive faults. This structure corresponds to a mega-shear zone rooted into the basement and affecting both the autochthonous Mesozoic oceanic sequence (Upper Jurassic-Lower Aptian) and the AUGC. The mega-shear zone is up to 33 km in width reaching a depth up to 10 s two-way travel time (TWT) into the basement and extends to the African margin where it links with the South Moroccan Arc (Figure 2).
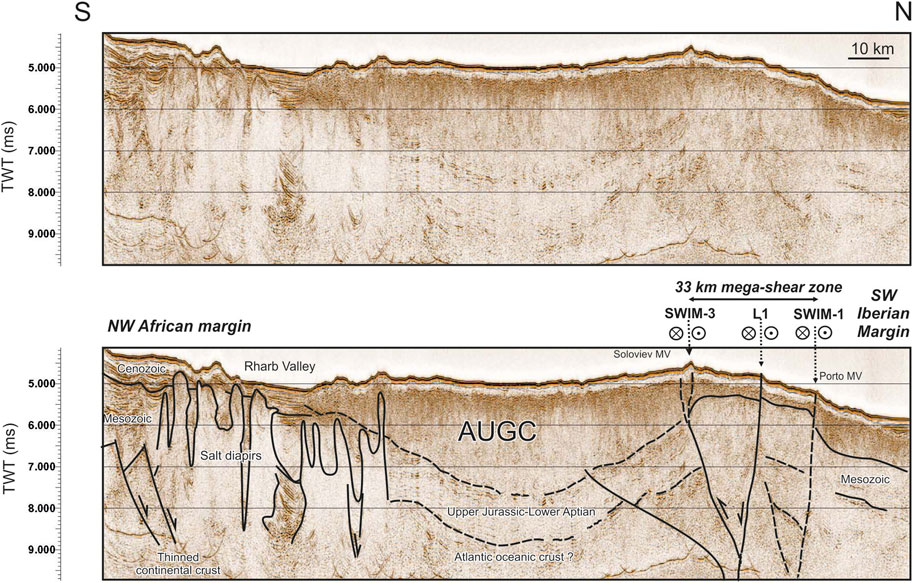
FIGURE 4. Multichannel seismic profiles MOUNDFORCE-06 showing the SWIM lineaments that belong to a flower structure rooted in the basement between the African and Iberian Margins. The flower structure comprises the SWIM-1, SWIM-3, and L1-faults large-scale right-lateral strike slip faults. The relationship between Faults and mud volcanoes as Soloviev and Porto MVs are clearly observed in the seismic profile The accretionary wedge is represented in the seismic profile by the Allochthonous Unit of the Gulf of Cádiz (AUGC). See Figure 2 for location of seismic profile.
The Fold-Thrust System of the South Moroccan Arc and Larache strike-Slip Fault: A Recent Major Seafloor Deformation
Based on a first interpretation of the SWIM compilation of swath bathymetry, a prominent arc was identified in the Atlantic Moroccan margin (Zitellini et al., 2009). This arc had been previously observed on side scan sonar data (Ivanov et al., 2000).
Here we present a 3d model of the South Moroccan Arc (SMA) combining high-resolution swath bathymetry data and seismic reflection profiles from several cruises as MOUNDFORCE-2007, MVSEIS-2008 and SUBVENT-2014 (Somoza, 2007; Somoza and UTM-CSIC, 2018; Somoza et al., 2019) (Figure 5A). The northern boundary of the South Moroccan Arc corresponds to a major ESE-striking fault termed as the Larache fault (Figures 2, 5). This major fault splits to the west into an arcuate deformation front. The Larache fault is composed by several segments showing local pull-apart basins. This major ESE strike-slip fault was considered as the prolongation of the SWIM lineaments (Zitellini et al., 2009). However, our bathymetric model shows that the easternmost SWIM lineaments are not aligned with the Larache fault and the South Moroccan Arc is superimposed over the eastern prolongation of the SWIM faults (Figure 2).
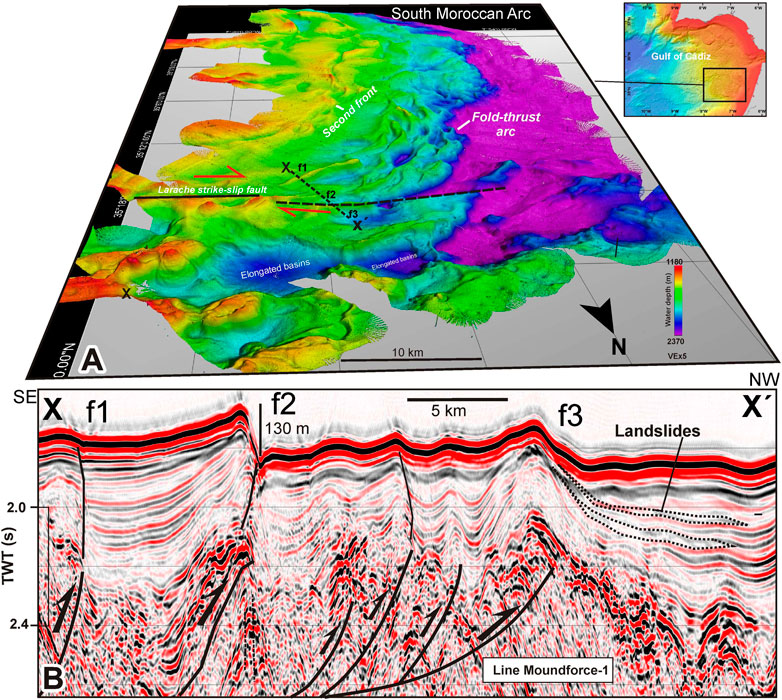
FIGURE 5. (A) 50 × 60 km 3 days image of the multibeam bathymetry of the South Moroccan Arc -SMA [data from Somoza (2007), Somoza et al.(2008)]. f1 to f3 are splays of the Larache right-lateral strike-slip fault. (B) Multichannel seismic profile MOUNDFORCE-01 crossing the SMA. The Larache strike-slip fault is split to the west into several fold-thrusts systems (f1 to f3) which deform the seabed in the South Moroccan Arc (Inset location in Figure 2).
Multichannel seismic profiles crossing the deformation front of the South Moroccan Arc show that it is composed of a series of fold-thrust systems linked to the main strike-slip faults (f1 to f3 system in Figure 5B). The thrusts are rooted on the AUGC unit and deform the uppermost sedimentary units including the seafloor, forming prominent diapiric ridges (Figure 2). A strong discontinuity is observed within the fold-thrust system as the beginning of the deformation (Figure 5B).
The generation of the SMA deformation front is associated with the right-lateral movement of the southern side of the Larache strike-slip fault (Figure 6). The seabed expression of this major fault is a narrow valley, 2.2 km width and 75 m depth, that splits into two elongated ridges, 45 m in height (Figure 6A). The elongated ridges are separated by a 2.6 km width depression. Pull-part mini basins are also associated with the right-lateral movements of this fault (Figure 6A).
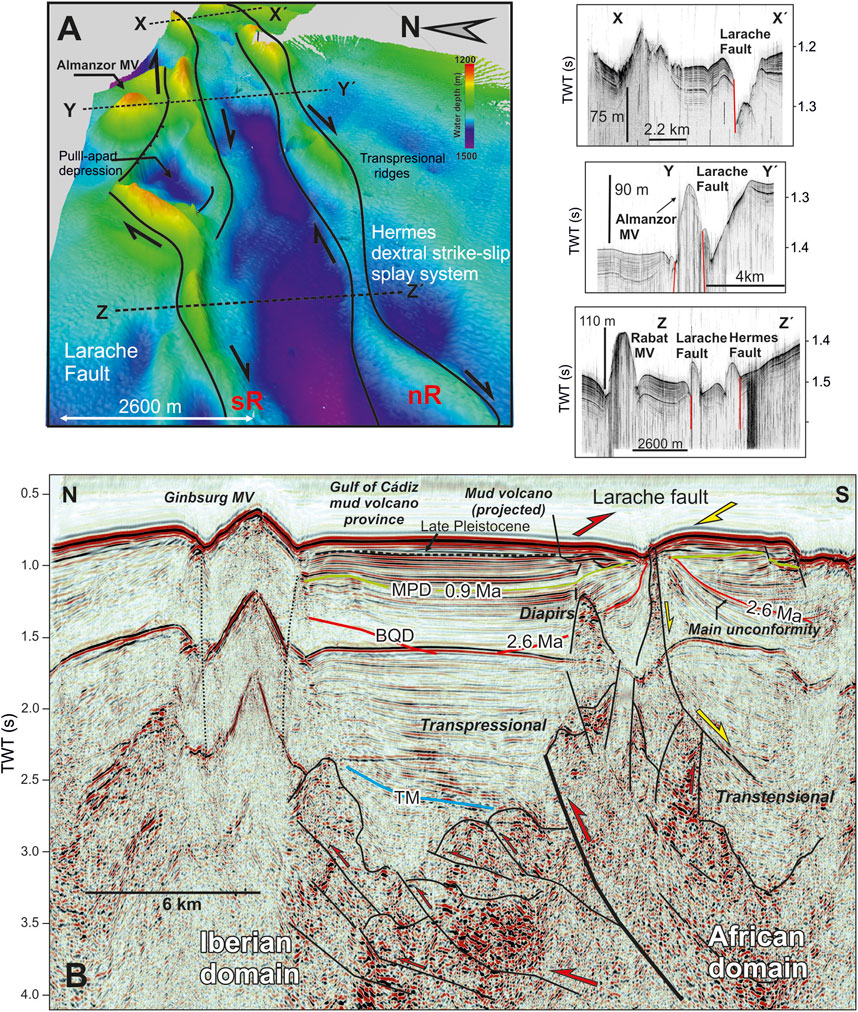
FIGURE 6. (A) Upper left: 3 days image of the swath bathymetry showing seafloor expression of the ESE strike-slip Larache fault: nR and sR = north and south ridges; Upper right: Ultra high-resolution profiles across the fault. See location in Figure 2. (B) Multichannel seismic section (Moundforce 02) showing the subseafloor expression of the Larache fault composed by a flower-like structure dividing two main domains: Iberian and Africa. Unconformities are taken from Toyos et al. (2016).
The subsurface expression of the Larache strike-slip fault, as seen in multichannel seismic profiles (Figure 6B), shows a complex flower-type structure composed of a northern transpressional edge and an eastern transtensional zone. The lower part of this flower-type structure, down to 2 s TWT, is transformed into major thrust system that affect the AUGC units forming subsidiary thrust systems towards the north (Figure 6B). These thrust systems affect the southernmost mud volcanoes of the Moroccan mud volcano province as the Ginsburg, Rabat and Almanzor mud volcanoes (Figure 6B) (Ivanov et al., 2000; Medialdea et al., 2009a; León et al., 2012).
The age of initiation of the Larache strike-slip fault activity can be inferred from the definition of the main unconformities associated with its movement (Figure 6B). A main unconformity marking a pronounced basin at the southern edge of the Larache fault allows to determine the beginning of the fault activity. We correlate this discontinuity with the base of Quaternary Discontinuity (BQD, ∼2.6 My) reported by Toyos et al. (2016) and associated with the development of the Ginsburg MV. An overlying major unconformity is interpreted as the Mid-Pleistocene Discontinuity (MPD, ∼0.9 My). Finally, a third unconformity can be dated as Late Pleistocene. On ultra high-resolution sub-bottom profiles, these seabed ridges related to the Larache fault deform the sea-floor sediments indicating recent activity, at least, from Late Pleistocene times.
Based on this correlation, we estimate the onset of the Larache strike-slip fault activity at the beginning of the Quaternary (∼2.6 My), even though, main activity has taken place during Mid and Late Pleistocene times. This major fault triggered the generation of the South Moroccan Arc structure overlapping the former AUGC unit, estimated to be emplaced in the Gulf of Cadiz during the Late Tortonian (Maldonado et al., 1999).This strike-slip fault and the associated thrust-fold system of the South Moroccan Arc show a total rupture length of 200 km, 80 km of the Larache strike-slip fault plus 120 km of the thrust-folds system, and therefore reach the required scale to be a potential source candidate for large earthquakes (Stich, 2007). Moreover, vertical displacements of the seabed up to 75 m have been observed along the Larache fault and up to 100 m high in the fold-thrust system (Figures 5, 6). Therefore we propose that these related vertical components are potential sources candidates for large tsunamis in the Gulf of Cádiz.
Quaternary Active Submarine Faults in the Alborán Sea
The Alborán Sea region has been highly deformed during the Pliocene and Quaternary as a consequence of the indentation of the Alborán Ridge block to the north and the generation of two main families of strike-slip faults in this process, left-lateral transcurrent NNE-SSW to NE-SW faults and right-lateral transcurrent WNW-ESE to NW-SE faults, together with the uplifting of several ENE-WSW compressive structures mainly focused in the Alborán Ridge (Figure 2). In addition, minor N-S to NNW-SSE normal faults have also played an important role in the northern margin. This general fault system explains the main crustal seismic activity in the region (Stich et al., 2006; Grevemeyer et al., 2015; Buforn et al., 2017; Peláez et al., 2018; Stich et al., 2020) and its development strongly affects the seafloor morphology (Estrada et al., 1997; Gràcia et al., 2006; Martínez García et al., 2013, 2017; Estrada et al., 2018a; Galindo-Zaldívar et al., 2018; Perea et al., 2018; Soumaya et al., 2018; Gràcia et al., 2019; d’Acremont et al., 2020; Gómez de la Peña et al., 2020; Lafosse et al., 2020; Vázquez et al., 2021b), where several penetrative morphotectonic features such as linear scarps, ridges, elongated pressure push-up swells, and longitudinal or rhomb-shaped depressions show the contemporary variety of Quaternary tectonics (Ballesteros et al., 2008; Vázquez et al., 2008b; Gràcia et al., 2012; d’Acremont et al., 2014; Giaconia et al., 2015; Gómez de la Peña et al., 2016; Vázquez et al., 2016; Lafosse et al., 2018). The seismicity event distribution appoints that the NNE-SSW to NE-SW left lateral strike-slip fault system assumes most of the regional deformation (Figure 2). Secondarily, earthquakes epicenters are focused in the WNW-ESE to NW-SE right lateral strike-slip conjugate fault system and in the ENE-WSW compressive structures.
Left-Lateral Strike-Slip Fault Systems
These fault systems are concentrated in the central sector of the Alborán Sea basin, where at least five NNE-SSW and one NE-SW trending fault zones were identified from seafloor morphotectonic deformation (Figure 7).
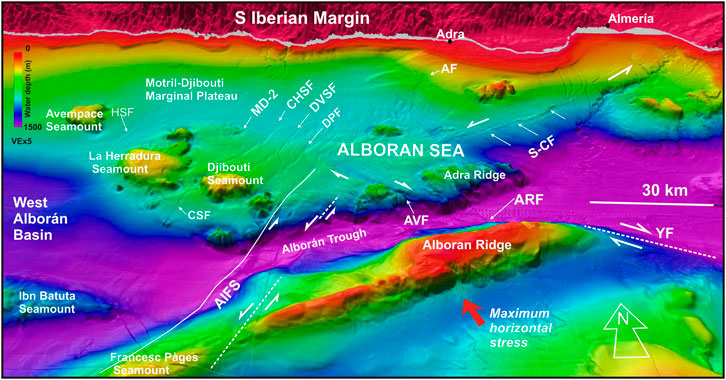
FIGURE 7. Three days multibeam bathymetry of the Alborán Sea showing the main active submarine faults. See location in Figure 1A. AF: Adra Fault; AIFS: Al Idrissi Fault System; ABR: Alboran Ridge Fault; AVF: Averroes Fault; CSF: Calahonda Sound Fault; DVSF: Djibouti Seamount Sound Fault; DPF: Djibouti Passage Fault; HSF: Herradura Sound Fault, MD-2 secondary fault zones, S-CF: Serrata-Carboneras Fault, YF: Yussuf Fault. Nomenclature from Vázquez et al. (2018).
They comprise the Al Idrissi (AIFS), the Motril-Djibouti Marginal Plateau (MDF) and La Serrata-Carboneras (S-CF) fault zones (Figures 7, 8 and Table 1) (Vázquez et al., 2018). The lengths of the fault zones vary between 40 (La Herradura Sound Fault Zone) and 140 km (La Serrata-Carboneras Fault Zone), although the later extends approximately 50 km onland and 90 km on the continental margin, with the Al Idrissi fault being the longest on the continental margin (125 km). The fault zones varies between 0.7 and 4 km in wide and are characterized by intense internal brittle deformation. Locally they have transtensive segments characterized by longitudinal grabens, rhomboidal depressions and transtensional relays (in the cases of The Herradura Sound, Calahonda Sound, Djibouti Ville Seamount, and Djibouti Passage fault zones), specially to the north of the La Herradura and Djibouti Ville seamounts, or with transpressive segments characterized by pressure ridges as in the cases of La Serrata-Carboneras and Al Idrissi faults (Figure 8). These last two structures are the most significant. The onland extension of La Serrata-Carboneras Fault Zone has been described as part of the Eastern Betic Shear Zone (Figure 7) and affects geological units during the last 133 ka (Silva et al., 1993; Bell et al., 1997; Moreno et al., 2015; Masana et al., 2018 Even though there are no large instrumental seismic events concentrations along this fault, several authors suggest that some historical events may be related to this fault (Keller et al., 1995; Gràcia et al., 2006). Finally, the Al Idrissi fault zone connects the Al Hoceima and Adra seismic areas, which are respectively located on the southern and northern margins of the basin and clearly displaces the Alborán Ridge (Galindo-Zaldívar et al., 2018; Gràcia et al., 2019; d’Acremont et al., 2020). This fault is divided at least into three segments: 1) the northeastern one is located on the Motril-Djibouti Marginal Plateau and has a transtensive character (Vázquez et al., 2016, 2018; Gràcia et al., 2019), 2) the central segment extends from the Alborán Trough to the Alborán Ridge towards the SSW; it corresponds to the western boundary of the Alborán Ridge Indenter (Estrada et al., 2018b) and has a transpressive configuration (Martínez-García et al., 2013) constituted by elongated pressure ridges and restraining bends with a set of successive high-angle reverse faults (Galindo-Zaldívar et al., 2018; Gràcia et al., 2019; d’Acremont et al., 2020); 3) the southwestern segment extends from the Alborán Ridge to the Al-Hoceima Bay towards the SSW and has an extensional horsetail splay (d’Acremont et al., 2014). In this SSW area a fault zone of similar characteristics has been defined at crustal levels extended both onshore and offshore regions, which could explain the three main earthquake series between 1994 and 2016 (Galindo-Zaldívar et al., 2018; Gràcia et al., 2019). This fault system has been explained by these authors as the growth of a continental strike-slip fault from the African margin to the north and include the eastern set of faults previously described in the of Motril-Djibouti Marginal Plateau.
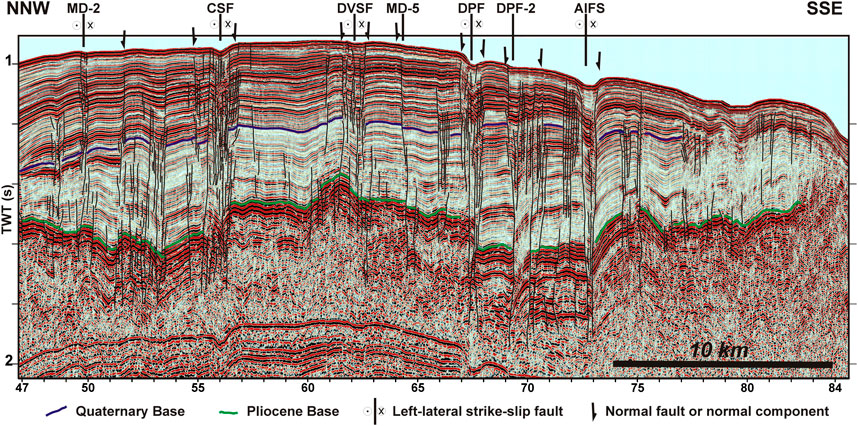
FIGURE 8. High-resolution multichannel seismic profiles crossing the set of NNW-SSE left-lateral strike-slip faults that composes the Motril-Djibouti Marginal Plateau (MDF): MD-2 and MD-5 secondary fault zones, CSF: Calahonda Sound Fault, DVSF: Djibouti Ville Seamount Fault; DPF and DPF-2: Djibouti Passage Fault and AIFS: Al Idrissi Fault System. See location in Figures 2, 7.
Right-Lateral Strike-Slip to Normal Faults System
The faults of this system are concentrated in the northeastern continental margin of the basin and in the eastern part of the Alborán Sea Basin, where they show NW-SE to WNW-ESE trends and constitute the outstanding Yusuf Fault Zone, that corresponds to the eastern boundary of the Alborán Ridge Indenter (Moreno et al., 2016; Estrada et al., 2018b; Perea et al., 2018).
The Yusuf Fault Zone (YF) is a right-lateral strike-slip fault with a transtensional component (Figure 7). It is 175 km long and 15 km wide and is divided into two main segments separated by a relay zone (Mauffret et al., 1992; Mauffret et al., 2007; Gràcia et al., 2014; Gómez de la Peña et al., 2016). The fault zone is characterized by the development of several strike-slip faults with a general transtensive geometry. Its morphology is characterized by a rectilinear escarpment with a relief ranging from 800 to 2000 m in the western part and an elongated ridge in the eastern one, with the development of a pull-apart basin (20 km in length and 10 km in width). The fault trace in its northern segment bends to the west at the connection with the northern Alborán Ridge Fault (ARF, Figure 7), where it shows a reverse component (Martínez-García et al., 2010). It can also continue to the northwest with the NW-SE Averroes system (Perea et al., 2018).
At least five WNW-ESE fault zones have been identified in the eastern part of the Motril-Djibouti Marginal Plateau (MDF) (Figure 8).The most penetrative is the Averroes Fault Zone (AVF), but at least other four fault zones have been identified to the northeast, sub-parallel to the Averroes Fault Zone and called NAF1 to NAF4 by Perea et al. (2018). These faults separate elongated ridges that have been interpreted as anticlines between faults (Moreno et al., 2016). The Averroes Fault Zone is constituted by at least two high-angle faults of 46 km in length and 2 km in maximum width, made up of at least two segments. The southeastern one displaces the seafloor across the Adra Ridge and the Alborán Channel and ends in the Alborán Ridge, generating a longitudinal escarpment and an elongated ridge (Figure 7). Meanwhile the northwestern segment corresponds to a narrow trough formed by a half-graben structure around 15 km long, with a vertical offset of up to 470 m with a downthrown block to the NE (Figure 7) (Estrada et al., 2018b; Perea et al., 2018) The other four remaining fault zones have similar characteristics to the Averroes Fault Zone: high angle fault surface, affect the Motril-Djibouti Marginal Plateau, the Adra Ridge and the Alborán Channel and generate elongated depressions in the seafloor related to negative flower structure geometries and rectilinear scarps, allowing to define a right-lateral to normal movement. Some of them have several branches and their length approximately ranges between 17 and 36 km (Perea et al., 2018).
In addition, another fault zone of this system has been located in the upper continental slope in front of the Adra region that has been called as the Adra Fault (Gràcia et al., 2012), interpreted as a right lateral strike-slip fault (Figure 7). This fault zone is constituted at least by three different faults, with lengths ranging between 10 and 16 km. It is characterized for producing minor rectilinear changes in the slope gradient and small scarps caused by the normal component of these faults (Vázquez et al., 2014).
Compressive ENE-WSW Structures
These structures include antiformal folding related to the main elongated ENE-WSW ridges and banks, as well as thrust faults. Two main structures are defined: the Northern Alborán Ridge Fault and the Xauen Compressive System (Figure 2).
The Alborán Ridge fault (ARF) has an ENE-WSW direction and is constituted by at least two or three thrusts. It is located north of the ridge and corresponds to the front of the Alborán Ridge Indenter (Estrada et al., 2018a) bounded by the Yusuf faults to the east and the Al Idrissi fault (AIFS) to the west, with an approximate length of 75 km and a width of the fault zone around 6 km, presenting an arcuate geometry on the surface (Figure 7). This structure and its southwest extension are considered as a 165 km long left-lateral strike-slip fault zone, with a compressive component that has been active since late Miocene times (Bourgois et al., 1992; Woodside and Maldonado, 1992; Watts et al., 1993; Comas et al., 1999). However, it had an important uplift phase as a tectonic relief, through folding and reverse faults in the Pliocene-Quaternary (Martínez-García et al., 2013, 2017; Vázquez et al., 2015; Estrada et al., 2018a).
The Xauen Compressive System generates the current relief of the banks located to the west of the Alborán Ridge (Francesc Pages and Xauen banks), that are left-laterally displaced by the Al Idrissi fault with respect to the eastern Alborán Ridge (Figure 7). This system has almost 60 km in length and 30 km in width and is constituted by at least three north-verging thrust faults gently arched and a fourth thrust with southern vergence (Bourgois et al., 1992; d’Acremont et al., 2020). The geometry of the Xauen Bank corresponds to a pop-up structure. Two main thrusts bound this positive relief and reach the seafloor; the northern thrust of this system corresponds to a blind thrust that constitutes the deformation front (d’Acremont et al., 2020).
In addition, three gently ridges with a N50-60 trend and around 20 km long affect the continental margin seafloor in front of the Adra coast. The most prominent feature has two high-angle reverse faults at the top affecting the Upper Pleistocene-Holocene units (Vázquez et al., 2014, 2016). These ridges are interpreted as anticline folds associated with blind thrusts verging to the NW, affecting the Quaternary units and bulging the seafloor (Comas et al., 1992; Vázquez et al., 2008b).
N-S to NNW-SSE Normal Faults
Several normal faults trending N-S to NNW-SSE affect the seafloor in the Alborán Sea region generating sets of rectilinear scarps and longitudinal depressions. They have been described in both northern and southern margins. In the southern margin, they are focused in the southern end of the Al Idrissi fault zone into the Al Hoceima Bay where a homogeneous set of N155 oriented normal faults and close to 10 km in length has been described by Lafosse et al. (2018). Normal faults generate small rectilinear scarps on the seafloor (Figure 7). In the northern margin, faults of this system are better represented along the Motril-Djibouti Marginal Plateau (MDF) (Figure 8), although they are more concentrated in the northern end of the Al Idrissi fault zone, where they are located in a corridor of 5 km in width. Normal faults have trends from N165 to N15 and generate rectilinear scarps and elongated tectonic depressions ranging between 2 and 7 km in length, that affect the seafloor (Vázquez et al., 2014; Vázquez et al., 2016). These faults have been explained as the surficial expression of the NNE propagation of Al Idrissi fault strike-slip system (Vázquez et al., 2014; Gràcia et al., 2019).
Quaternary Reactivation of Submarine Faults Along the Northwest Iberian Margin
In the Galicia region (Figure 9) Three main zones with active submarine faults related to seismicity have been identified in the NW Iberian margin (Figure 10): 1) The arcuate Finisterre thrust fault (FSF) to the NW of the Galicia Bank; 2) The Burato Shear Zone, located between the eastern Galicia Bank and the NNW-SSE Castelao Fault (CSF); 3) The NW-SE Ortegal Fault, split onshore into the Meirama and As Pontes strike-slip faults (e.g., Andeweg, 2002).
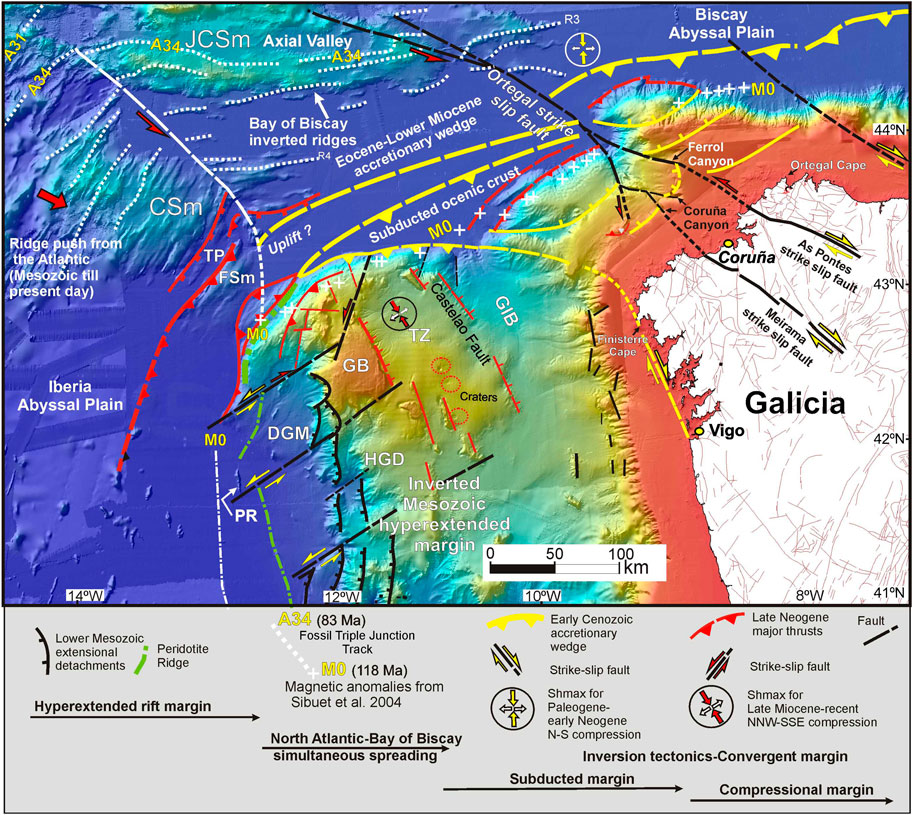
FIGURE 9. Structure map of the Galicia margin showing the main Alpine-Pyrenes structures along North Iberia subduction margin (yellow lines) as the buried front of the accretionary wedge segmented by NW-SE large right-lateral strike-slip faults. CSm: Coruña Seamount; HGD, Half-Graben Domain; DGM: Deep Galicia Margin; FSm: Finisterre Seamount; GB:Galicia Bank; TZ, Transition Zone; GIB: Galicia Interior Basin; JSm: Jean Charcot Seamount; PR: Peridotite Ridge; TP:Theta Passage; MO: Magnetic anomaly MO.
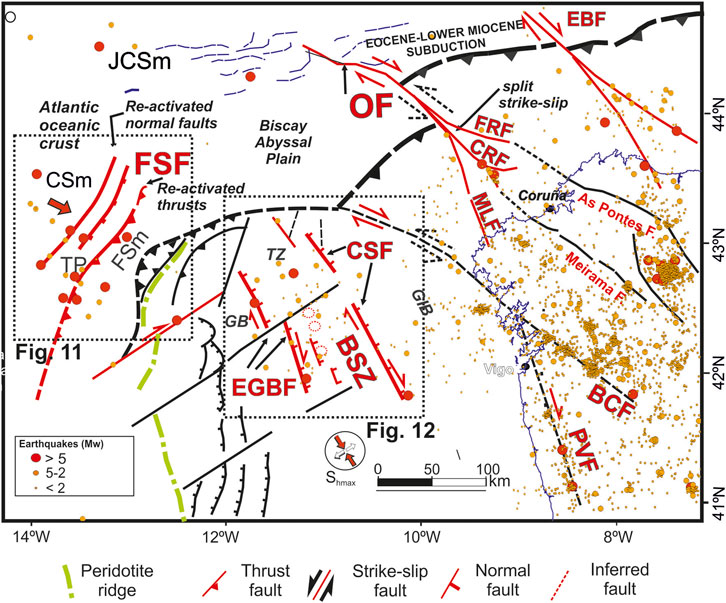
FIGURE 10. Main active submarine faults (red lines) and distribution of earthquakes in the Northwestern Iberia Margin. These active submarine faults are related to re-activation of former structures in response to present-day NW-SE convergence. (A) Reactivation of the Finisterre thrust fault (FSF); (B) The Burato Shear Zone (BSZ), an area of tension gashes and craters bounded by NNW-SSE normal to strike-slip faults (CSF: Castelao Fault); and (C) NW-SE strike-slip faults: Ortegal Fault (OF) split into three strike-slip faults in the margin (MLF: Malpica Fault, CRF: Coruña Fault and FRF: Ferrol Fault) and two main faults onshore (As Pontes and Meirama faults); and Estaca de Bares Fault (EBF). Onshore faults: Padrón-Vigo Fault (PVF) and Becerreá Fault (BCF) a highly seismic zone. Earthquakes location taken from the online database of the Instituto Geográfico Nacional (IGN). Faults are also listed in Table 1. Other labels same than Figure 9.
The Finisterre Thrust System: Reactivation of the Former Cenozoic Subduction Zone
A cluster of earthquakes Mw > 5 is associated with the Finisterre thrust system (FSF, Figure 10). This system consists of an array of thrust faults that deform the recent sedimentary sequence at the northern area of the Finisterre Seamount (Figure 11). The Theta Passage is a deep passage that separates the Iberian continental margin from the Atlantic oceanic ridges of the Coruña Seamount (Figure 11A). (e.g., Vázquez et al., 2009a; Somoza et al., 2019). The Finisterre Seamount fault system (FSF) is interpreted as landward-dipping thrusts which presently deforms the seafloor forming a serie of ridges (Figure 11B). This zone has been considered as a zone of obduction of the serpentinized mantle and Atlantic oceanic crust during the Alpine-Pyrenean compression due to the outcropping lherzholites that were collected at the foot of the NW slope of the Galicia Bank (Boillot et al., 1979; Malod et al., 1993). The Finisterre external deformation front has a length of 75 km, at the seabed, extending from the SW to NE (Figure 11).
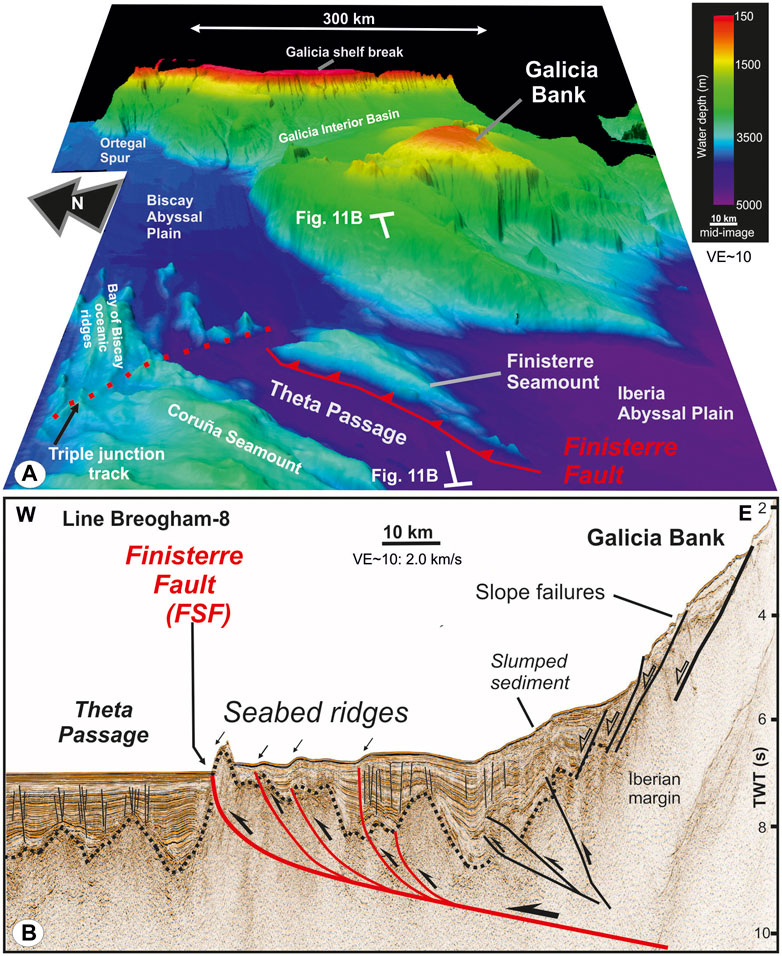
FIGURE 11. (A) Multibeam bathymetry of the NW Iberian Margin showing the location of the Finisterre Thrust Fault interpreted as the former boundary between the North Atlantic plate and Iberia. (B) Multichannel seismic line BREOGHAM-08 showing the Finisterre thrust system deforming the uppermost seafloor. See location in Figure 1.
The Burato Shear Zone and the Castelao Fault System: Seabed Tension Gashes and Deep Craters
The second zone with high seismic activity is the so-called Transitional Zone (Murillas et al., 1990) located between the Galicia Bank and the Galicia Interior Basin (Vázquez et al, 2009c). This area extends over 75 km and is bounded by two main NW-SE trending faults from the Galicia Interior Basin (Castelao Fault, CSF) and the Galicia Bank (Eastern Galicia Bank Fault, EGBF) (Figure 12). This area is characterized by an elongated WNW-ESE dome-like morphology at water depths from 1,500 to 2,500 m with gentle slopes of 1.2° (Figures 12, 13). The seafloor floor exhibits an array of en-echelon NW-SE to N-S trending ridges with lengths up to 10 km and circular depressions (Figure 12). The largest depression, named as the “Burato Hole” (Vázquez et al., 2009c), is a 3 km in diameter crater-like depression of 300 m deep with average flank slopes of 12° (Figure 13).
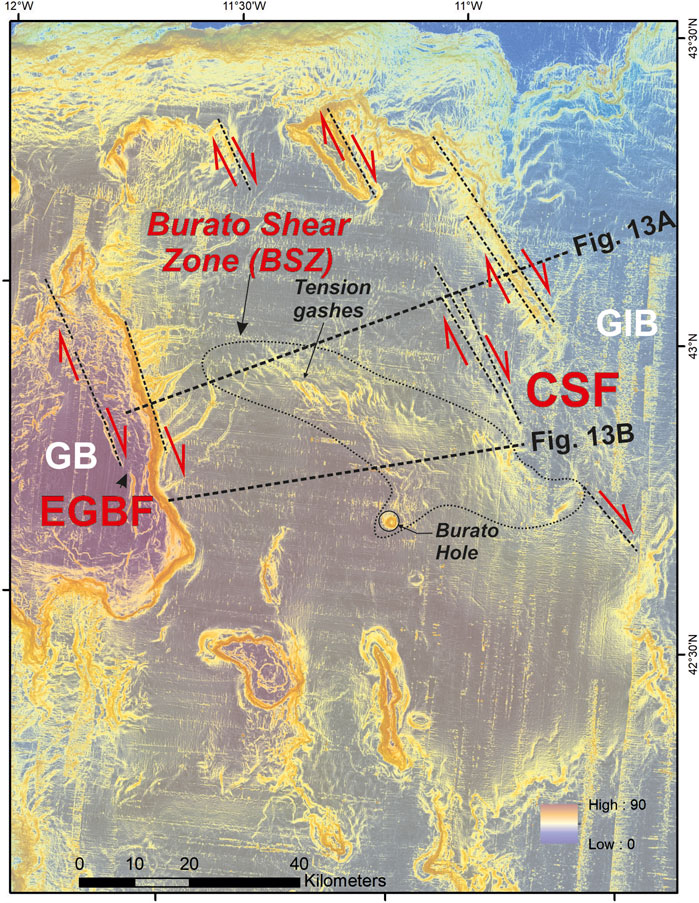
FIGURE 12. Slope gradient map of the high-resolution bathymetry of the Burato Shear Zone (BSZ) between the Galicia Bank (GB) and the Galicia Interior Basin (GIB). Seismic profiles shown in Figure 13 are also displayed. This shear zone is located between two main systems of NNW-SSE fault systems acting as right-lateral strike-slip system, i.e., the Castelao fault (CSF) and the East Galicia Bank fault (EGBF). Other labels same than in Figure 9. See also Figure 10 for location of the Burato Shear Zone.
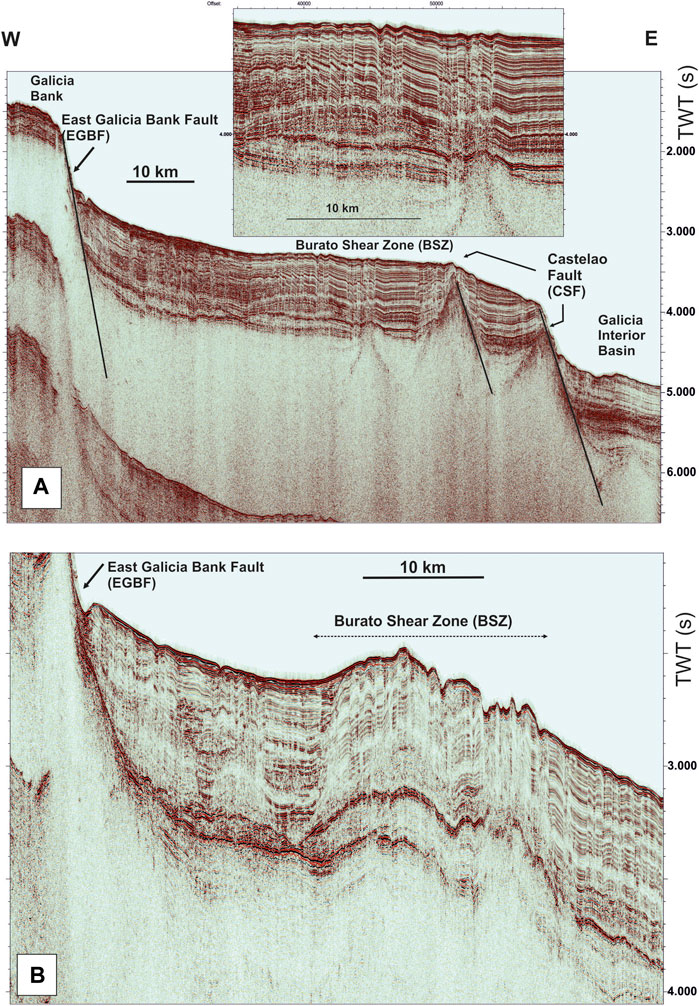
FIGURE 13. High-resolution multichannel seismic profiles crossing the Burato Shear Zone (BSZ) bounded by the Castelao Fault (CSF in Figure 12). The Burato Shear Zone shows a prominent elongated WNW-ESE trending dome bounded by a dense network of sub-vertical faults. Location of profiles is shown in Figure 12.
The Burato Shear Zone shows a prominent elongated NW-SE trending ridge bounded by a dense network of tensional gashes (Figure 12). High-resolution multichannel seismic profiles show that the Burato shear zone is characterized by a dense array of sub-vertical faults identified as tension gashes on the multibeam bathymetry (Figure 13).
Inherited Right-Lateral Strike-Slip Faults Split Into the Iberian Margin and Forming Deep-Incised Submarine Canyons
The third structure associated with relatively moderate activity of earthquakes MW > 5 is the NW-SE Ortegal right lateral strike-slip fault (OF in Figure 10). This type of NW-SE structure was formed, at least, during the Cenozoic Alpine-Pyrenean orogeny, dissecting the accretionary wedge of the North Iberian Margin.
The Ortegal Fault (OF) is the westernmost of a system of large Cenozoic strike-slip faults as Estaca de Bares (EBF) or Ventaniella (VF) (Figure 10), developed from the Northwest Iberian margin to the Pyrenees (Figure 1). These faults were formed, at least, during the Pyrenean phase 9 in response to Paleogene-early Neogene N-S and NE-SW compression between Eurasia and Iberia (Figure 1C) (e.g., Andeweg, 2002). The Ortegal Fault offsets the Mesozoic oceanic ridges of the Jean Charcot Seamounts and the buried front of the accretionary wedge formed along the N Iberia margin (Figure 9). The high-resolution bathymetry shows that this fault is split into several branches when enter into the continental margin, controlling the development of deep submarine canyons named as Ferrol and La Coruña canyons (Figure 9). We have termed, each of these branches affecting the continental margin from south to north, as the Malpica (MLF), La Coruña (CRF) and Ferrol (FRF) faults (Figure 10). The activity of these faults is revealed by the occurrence of earthquakes Mw > 5 on the upper margin, especially along the central branch (CRF) (Figure 10). The onshore prolongation of these strike-slip faults (Figure 10) is constituted by the As Pontes and Meirama strike-slip faults and the Padrón-Vigo Fault (PVF) (de Vicente, 2009), that form the main deep-intracontinental Tertiary basins in the Galicia region (e.g., Andeweg et al., 1999). The Becerreá Fault (BCF in Figure 10) holds a remarkable concentration of earthquakes within the Galicia Region (e.g., López-Fernández et al., 2012) This high-seismic onshore lineament might be linked to the offshore front of the Galicia Bank and would represent the crustal boundary between the former convergent N Iberian Margin and the hyperextended-rifting W Iberian Margin (Somoza et al., 2019) (Figure 10).
Discussion and Conclusion
Synthesis Map of Active Submarine Faults Around Iberia
A synthesis map of the main active submarine faults around the Iberian Peninsula has been carried out. In this synthesis map (Figure 14), we only include those active tectonics structures that show the following characteristics: 1) The submarine fault/fold shows seafloor morphological expression on high-resolution MBES images with resolution < 250 m; 2) The structure shows deformation and/or displacement of the seabed affecting the most recent sediments, at least, since the late Quaternary unconformity identified on ultra-high SBP sections with vertical resolution <1 m; 3) The seafloor expressions of fault/fold surface has to be linked to deep-seated structures on MCS profiles, affecting at least Cenozoic units; 4) The fault/fold structures or surface shear zones have to be linked to swarms of Mw < 5 earthquakes or single Mw > 5 earthquakes hypocenters.
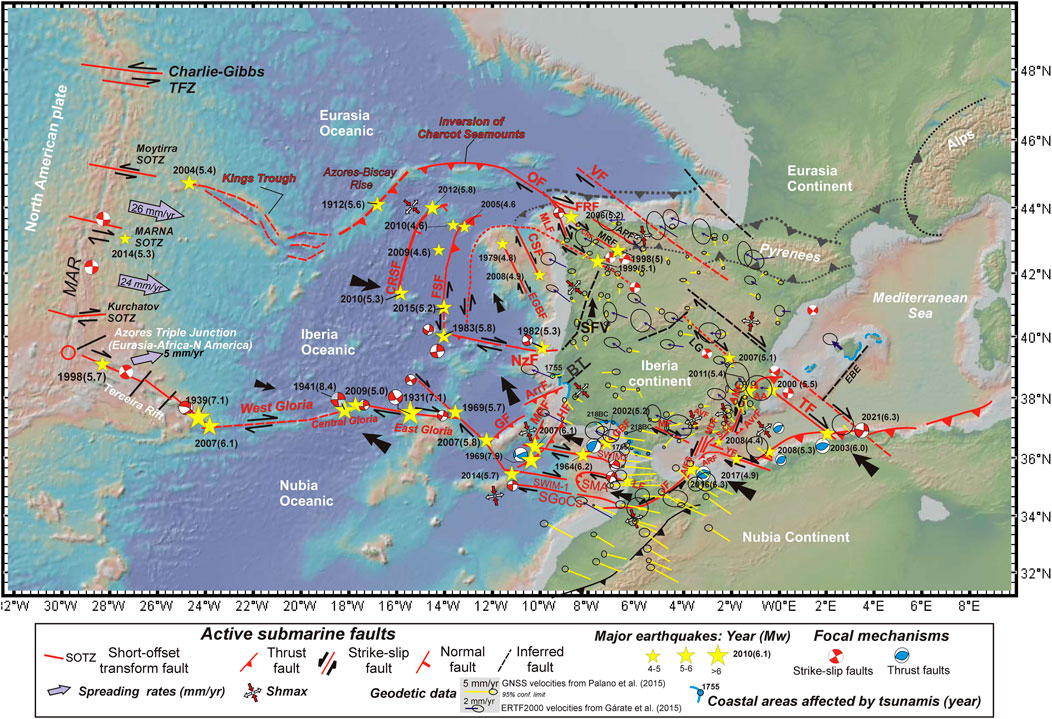
FIGURE 14. Synthesis map of the main active submarine faults around Iberia with location of the main Mw > 5 earthquakes (yellow stars). Active submarine faults by regions; (A) Gulf of Cádiz. ArrF: Arrabida Fault; GBF: Gualdalquivir Bank Fault; GF: Gorringe Fault; HF: Horseshoe Fault; LF: Larache Fault; MPF: Marques de Pombal Fault; SGoCs: South Gulf of Cadiz Shear Zone; SMA: South Moroccan Arc. (B) Alborán Sea. AIFS: Al Idrisi Fault System; ARF: Alborán Ridge Fault; AVF: Averroes Fault; JF: El Jebha Fault; MF: Maro-Nerja Fault; MDF: Motril-Djibouti Fault System; S-CF: La Serrata Carboneras Fault; YF: Yusuf Fault. (C) SE and E Iberia. AA: Aguilas Arc; AMF: Alhama de Murcia Fault; AvrF: Abubacer volcanic ridge Fault; TF: Torrevieja Fault; EME: Emile Baudot Escarpment. (D) N and NW Iberia Margin. CRF: Coruña Fault; CSF: Castelao Fault; CRSF: Coruña Seamount Fault; EGBF: East Galicia Bank Fault; FRF: Ferrol Fault; FSF: Finisterre Seamount Fault; MLF: Malpica Fault; OF: Ortegal Fault; VF: Ventaniella Fault. (E) Iberia intraplate active fault lineations (from Vegas et al., 2008). BT = Bajo Tagus; SFV = Vilarica Fault System; LG: Guadiana Lineaments. (F) Onshore faults. APF: As Pontes Fault; BF: Becerreá Fault; MRF: Meirama. Geodetic GPS velocities and 95 per cent confidence ellipses data from Gárate et al. (2015) and Palano et al. (2015). Shmax directions from Andeweg et al. (1999), Fernández-Ibáñez et al. (2007) and Pedrera et al. (2011). Earthquake distribution taken from the Instituto Geográfico Nacional (www.ign.es) and SHARE database (Giardini et al., 2013). Tsunamis from Lario et al. (2011). Background bathymetry from Ryan et al. (2009). Abbreviations are also listed in the Supplementary Table S2.
Shear Zones, Submarine Faults and Potential Tsunamigenic Sources Along the Plate Boundary Between Nubia and Eurasia
Along the southern boundary, the oblique convergence between Africa and Iberia is not a well-defined plate boundary but constituted by, at least, five main shear zones (Vegas et al., 2008). Thus, in the Gulf of Cadiz, we define three main shear zones (Figure 2): 1) A pure-shear zone along the northern margins; 2) a simple shear zone along the central Gulf of Cadiz; and 3) a convergence zone along the SMA South Moroccan Arc (Figure 14).
The pure shear zone along the southern Iberian margin in the northern Gulf of Cadiz is characterized by SW-NE thrusts as the Gorringe (GF), Marques de Pombal (MPF), Horseshoe (HF), Portimao (PF), and Guadalquivir (GF) faults (e.g., Terrinha et al., 2003; Medialdea et al., 2004; Martínez-Loriente et al., 2018) (Figures 2, 12).
The simple shear zone along the central Gulf of Cadiz is formed by 200–315 km length, 33 km width, ENE-WSW shear zones composed of arrays of large right lateral strike-slip faults, which are linked at 10 s TWT depth assumed as the oceanic crust. Two major simple shear structures are identified (Figure 2): 1) the southern shear zone composed by a set of seafloor trace faults like the L-3, SWIM-1 (L-2), L-1 and SWIM-3 right-lateral faults.(e.g., Medialdea, 2007; Rosas et al., 2009; Zitellini et al., 2009; Bartolome et al., 2012) and the northern shear zone composed mainly by the SWIM-2 (L-4) fault (e.g. Medialdea et al., 2004; Terrinha et al., 2009; Zitellini et al., 2009). Best documented offshore historical large earthquake events (Imax > VIII) around Iberia Peninsula have occurred in 1,531, 1,356, 881, 241, and 245 BC along these shear structures in the Gulf of Cadiz (Udias, 2015).
The South Moroccan margin in the south of the Gulf of Cadiz is composed of a fold-thrust system migrating westwards and bounded to the north by the Larache right lateral strike-slip fault and to the south by the offshore prolongation of the El Jebha left lateral strike-slip fault (Figure 2). Therefore, we suggest that this fault might have acted as a link between the active submarine structures of the Gulf of Cádiz and Alborán Sea. A slight change in the orientation of the Shmax to ENE-WSW identified onshore Morocco from focal mechanisms (Pedrera et al., 2011) could explain the present inactivity of the El Jebha Fault as a left lateral strike-slip fault onshore (Figure 2).
In the Alborán Sea, the convergence between Africa and Iberia is assumed by an oblique pure shear regime forming a conjugated system (e.g., Vegas and Vázquez, 2000; Vegas et al., 2008). In the Iberian margin, the eastern Betic pure shear zone is formed by the NE-SW La Serrata-Carboneras left lateral strike-slip fault (S-CF in Figure 7) (e.g., Rutter et al., 2012; Gràcia et al., 2014), the conjugated NW-SE Maro-Nerja right lateral strike-slip fault (MF in Figure 14) (Fernández-Ibáñez et al., 2007) and the Motril-Djibouti shear zone (MDF in Figure 14) (Vázquez et al., 2018), composed by an array of near N-S dextral strike-slip faults with tensional components due to the N-S direction (Vázquez et al., 2008c).
Towards the north, the active submarine La Serrata-Carboneras fault continues onshore into the Eastern Betics through the NNE-SSW Palomares and Alhama de Murcia left lateral strike-slip faults (AMF), forming the Aguilas Arc in the eastern Betics (Figure 14) (e.g., Silva et al., 1993; Somoza, 1993).
In the African margin, the conjugated system of this pure shear system, which accommodate most of convergence, is formed by the NNE-SSW left lateral strike-slip Al Idrisi Fault System (AIFS in Figure 14) (Gràcia et al., 2019; d’Acremont et al., 2020) and the WNW-ESE Yusuf right lateral strike-slip fault (YF in Figure 14). These two conjugated faults are linked through the Alborán ridge (ARF in Figure 14), bounded by a thrust fault as response to a near NNW-SS convergence in the region (Vázquez et al., 2021a; Vázquez et al., 2021b).
Re-Activation of the Inherited Faults Along the Northwest and Southwest Iberian Margin
Besides the high record of earthquakes and tsunamis along the southern boundary of Iberia, a remarkable concentration of earthquakes can be observed, onshore and offshore, along the NW margin of Iberia. In this region, the present-day stress field caused by the convergence between Africa and Iberia is modified by the opening of the Mid-Atlantic Ridge with near E-W spreading rates up to 26 mm/yr at 43° N decreasing southwards to 24 mm/yr at 42°N (Figure 14). Short-offset transforms of the Mid-Atlantic Ridge as the Kurchatov, MARNA and Moytirra are almost 30° oblique to the direction of plate motion showing secondary strike-slip faults with N°120 trend (Figure 14) (Somoza et al., 2019). For the oceanic Iberia, especially for the northern part, the incorporation of these forces leads to a dramatic change in the stress field orientation and magnitude (Andeweg et al., 1999). Therefore, the local stress data derived from focal mechanism solutions, active faults and bore-hole breakout analysis (e.g., Stich et al., 2006; Pedrera et al., 2011; Custódio et al., 2016) of the Iberian Peninsula show a certain anticlockwise deviation of the Shmax direction from the south to north Iberia margins (Figure 14). This would explain the inversion and re-activation as thrust faults of northern oceanic seamounts as the North Jean-Charcot Seamounts (Medialdea et al., 2009b), the Coruña Seamount Fault (CRSF) and the Finisterre Seamount (FSF), which show occurrence of earthquakes reaching magnitudes between 5.6 and 5.8 (Figure 14). The northernmost system, the North Charcot structure, connects with the Ortegal strike-slip fault that splits as it enters into the continental margin forming deep incised submarine canyons and continues onshore with major active faults during the Tertiary (Meirama and As Pontes faults) (e.g, Andeweg, 2002). Both later faults are aligned with the main intraplate lineaments as the Iberic Range (LG in Figure 14). At the same time, the Cenozoic deformational structures of the Galicia Bank region (Vázquez et al., 2008a) seem to be re-activated by two faults as the East Galicia Bank Fault (EGBF) and Castelao Fault (CSF), which form a vast shear zone expressed on the seafloor as craters and tension gashes.
This northern domain is bounded by the Nazaré Fault (NzF) which runs near E-W from the Estremadura Spur to the Tore Seamount. This fault shows earthquakes with Mw between 5.3 and 5.8 and strike-slip fault solutions. This fault-plane mechanism could be explained by the relative movement propagated westwards from the Mid-Atlantic Ridge segment north of Kurchatov short-offset transform fault (Figure 14). Southwards of this boundary along the SW Iberian margin, the northwards propagation of the deformation takes place between major thrusts as the Arrabida Fault (ArrF), Gorringe (GB), Marques de Pombal (MPF) and Horseshoe Faults (HS), and along the westward prolongation of the southern Gulf of Cádiz shear zone, linked to the Gloria Fault (Figure 14).
Submarine Faults and Geodetic Constraints
The dense spatial coverage of geodetic velocities in the Iberian Peninsula and North Africa, comprising over 380 stations (Gárate et al., 2015; Palano et al., 2015), helps to understand the dynamic of the offshore submarine faults (Figure 14). Geodetic data reveals that significant deformation occurs prevailing along the southern margins of the Iberian Peninsula, from the Alborán Sea to the Gulf of Cádiz, including the Gibraltar Arc, and to a lesser extent along its W and NW margins, while on the inner parts of the Peninsula, the crustal deformation occurs locally at rates <15 nano strain/year (Palano et al., 2014). Stations located in central and northern Portugal move northwards with rates of ∼1 mm/yr (Palano et al., 2015). Along the NW margin, geodetic data evidences an E-W oriented contraction up to 55 nanostrain/year. In contrast, along the Gibraltar Arc, ∼2–5 mm/year WSW motion can be detected (Palano et al., 2014). The Al-Hoceima region (south Alboran domain, Figure 2) shows an E-W-oriented elongation up to 90 nanostrain/year, and the Alboran domain is characterized by elongation strain-rate axes WSW-ENE oriented coupled with shortening strain-rate axes of ∼25–40 nanostrain/year. Finally, along the SE Iberian margin a prevailing NNW-SSE-oriented contraction up to 30 nanostrain/year can be recognized (Palano et al., 2014). Thus, the main deformation around Iberia takes place along the southern margin (Alboran Sea-Eastern Betics), SW (Gulf of Cádiz-S Portuguese margin) and NW Iberian margins (N Portuguese and Galicia margins).
In the Alborán Sea, the NW motion up to 5 mm/yr of the stations located in the eastern Rif clearly depicts a contraction of the Alborán Sea and explain the dynamics of the Alborán indentation system and westwards escape tectonics (Chalouan et al., 2014) (Figure 14). Therefore, the Al Idrissi left lateral strike-slip fault system in the south propagates to the north into the system of the Motril-Djibouti Marginal Plateau and the La Serrata-Carboneras Fault in the north. The conjugated right-lateral system is composed of the Yusuf Fault in the south and the Averroes Fault and related system to the north (Figure 7). In SE Iberia (Eastern Betics e.g., Almería–Murcia-Alicante region), geodetic values show a deformation pattern that strongly differs from that observed for surrounding areas (Palano et al., 2015). Thus, geodetic velocities clearly show a NW-to- NE fan-shaped pattern with rates ranging from ∼3 mm/yr near the coastline to ∼0.8 mm/yr inland (Figure 14). This pattern could explain the dynamics of the Palomares and Alhama de Murcia faults as left-lateral strike-slip faults, and specially the clockwise rotation from NE to NW of the Aguilas Arc, probably forced by the confluence with NW-SE faults as the Torrevieja Fault or the Ibiza-Alicante right-lateral strike-slip faults, that continues into the South Balearic Basin and the Algerian margin.
The westward motion up to 5 mm/yr of the stations located on the central sector of the Gibraltar Arc and eastern Gulf of Cádiz could explain the activity of the South Moroccan Arc (SMA), interpreted as the most prominent thrust structure with seafloor expression (Figure 5). Otherwise, the left lateral focal mechanism of the southernmost shear structure (SGoCs) in the Gulf of Cádiz is explained by the NW motion with rates of ∼1.1 mm/yr. In contrast, stations located in the northern Gulf of Cádiz and the south Portuguese margin show NW motions with rates of ∼3 mm/yr, suggesting the re-activation of thrust faults along the SW Iberian continental margins such as the Guadalquivir Bank (GBF), Portimao (PF), Horseshoe (HF), Marques de Pombal (MPF), or Gorringe (GF) faults.
Northwards along the Atlantic margin, stations located in central and northern Portugal and Galicia region indicate northward motion with rates of ∼1 mm/yr (Figure 14). This could explain the right-lateral mechanism of the Nazaré Fault and the strike-slip mechanism of the Eastern Galicia Bank (EGBF) and Castelao (CSF) faults in the Galicia Bank region. In the NW Iberian margin, this motion could also explain the inversion of former oceanic crust seamounts as the Jean Charcot, Coruña (CRSF) and Finisterre (FSF) seamounts that could also be affected by the propagation of tectonic stress from the Mid-Atlantic Ridge.
Geodynamic Model: Oceanic Vs. Continental Iberia
Based on geodetic data, Palano et al. (2015), proposed a present-day large-scale clockwise rotation of Iberia acting as a microplate with a southern boundary at the Nubia-Eurasia convergent boundary without describing its north and northwest boundaries. According to geophysical data, the main weakness zones along the N and NW Iberia are the former boundaries between 1) the Eurasia and Iberia oceanic domains, and between 2) the Eurasia oceanic and Iberian continent domains (Figure 14). Based on our synthesis of submarine faults, we propose that the weakness zone between the Iberian and Eurasia oceanic domains is constituted by the westward prolongation of the Moytirra short offset-transform fault (SOTZ) into the Kings Trough, Azores Biscay Rise and Jean Charcot Seamounts (Figure 14). This boundary shows earthquakes higher than Mw five and links with the NW-SE Ortegal strike-slip faults. These NW-SE faults continue onshore and split into two main high seismicity zones in Galicia with earthquakes higher than Mw five and strike-slip mechanisms (Figure 14). Otherwise, based on offshore submarine faults, we suggest that the clockwise rotation of Iberia proposed by Palano et al. (2015) would only affect the Iberian Continental domain, e.g., the SW and NW margins. Westwards, deformation affecting the Iberian oceanic domain is mainly dominated by stress derived from the spreading of the Mid-Atlantic Ridge in the segment between the Azores Triple Junction and the Moytirra SOTZ with a main direction of propagation trending N-120° (Somoza et al., 2021). Furthermore, we suggest that a major left-lateral shear-zone results as consequence of the N motion of the Iberian continental domain and SE motion of the Iberia oceanic domain along the west Iberian continent-oceanic transition. This zone is characterized by the occurrence of serpentinized mantle which probably buffers the magnitude of earthquakes along this area.
Concluding Remarks
As the main conclusion of the synthesis map presented in this work, we propose that the present active submarine faults and their associated seismicity around the Iberia margins (Figure 14) can be explained by the present-day, roughly NNW-SSE compressional stress field related to the convergence between Eurasia and Africa plates. The distribution and activity of submarine faults mapped in this work from geophysical and bathymetric data are in good agreement with geodetic and seismological observations. Major deformation is located in the south Iberia margin along the Nubia-Eurasia plate boundary according to submarine fault distribution, earthquake distribution pattern and geodetic data. Nevertheless, deformation is also focused in the NW Iberian margin. We suggest that deformation in this area is derived from the westward motion of the Iberian oceanic domain due to differential spreading rates of the MAR and the clockwise rotation of Iberian continental domain with respect to stable Eurasia proposed by Palano et al. (2015). This interaction takes place over a crustal weakness zone that corresponds to the transition between the oceanic and the continental Iberian crusts.
Data Availability Statement
The datasets presented in this study can be found in online repositories. The names of the repository/repositories and accession number(s) can be found in the article/Supplementary Material.
Author Contributions
LS: Data acquisition, writing, and overall organizations; TM: Seismic Interpretation and Writing (Gulf of Cádiz and Galicia regions); PT: Geological interpretation SW Margin; AR: Seismic interpretation and writing. JV: Interpretation and writing Alborán Sea.
Funding
This work is funded by the Spanish Minister for Science and Innovation, projects EXPLOSEA (grant CTM201675947-R) and FAUCES (CTM 2015-65461-C2-2-R). This study is a contribution to the EMODNET-Geology project (EASME/EMFF/2018/1.3.1.8-Lot 1/SI2.811048), the European project H2020 GeoERA-MINDeSEA (Grant Agreement No. 731166, project GeoE.171.001), the IEO project RIGEL and the AGORA PAIDI project.
Conflict of Interest
The authors declare that the research was conducted in the absence of any commercial or financial relationships that could be construed as a potential conflict of interest.
The handling editor declared a past co-authorship with one of the author PT.
Acknowledgments
This study also benefits from the Atlantic Seabed Mapping International Working Group (ASMIWG) as part of the Atlantic Ocean Research Alliance Coordination and Support Action (AORA-CSA). Thanks to the two reviewers, Satish Chandra Singh and Jacques Deverchere, for their useful comments and suggestions. Special thanks to the guest editor, Hector Perea, for his valuable helpful and suggestions.
Supplementary Material
The Supplementary Material for this article can be found online at: https://www.frontiersin.org/articles/10.3389/feart.2021.653639/full#supplementary-material
References
Andeweg, B. (2002). Cenozoic Tectonic Evolution of the Iberian Peninsula: Causes and Effects of Changing Stress fields. PhD Thesis, Amsterdam: Vrije Universiteit, 178.
Andeweg, B., De Vicente, G., Cloetingh, S., Giner, J., and Muñoz Martin, A. (1999). Local Stress fields and Intraplate Deformation of Iberia: Variations in Spatial and Temporal Interplay of Regional Stress Sources. Tectonophysics 305, 153–164. doi:10.1016/s0040-1951(99)00004-9
Argus, D. F., Gordon, R. G., DeMets, C., and Stein, S. (1989). Closure of the Africa-Eurasia-North America Plate Motion Circuit and Tectonics of the Gloria Fault. J. Geophys. Res. 94 (B), 5585–5560. doi:10.1029/jb094ib05p05585
Ballesteros, M., Rivera, J., Muñoz, A., Muñoz-Martín, A., Acosta, J., Carbó, A., et al. (2008). Alboran Basin, Southern Spain-Part II: Neogene Tectonic Implications for the Orogenic Float Model. Mar. Pet. Geology. 25, 75–101. doi:10.1016/j.marpetgeo.2007.05.004
Bartolome, R., Gràcia, E., Stich, D., Martínez-Loriente, S., Klaeschen, D., de Lis Mancilla, F., et al. (2012). Evidence for Active Strike-Slip Faulting along the Eurasia-Africa Convergence Zone: Implications for Seismic hazard in the Southwest Iberian Margin. Geology 40 (6), 495–498. doi:10.1130/g33107.1
Bell, J. W., Amelung, F., and King, G. C. P. (1997). Preliminary Late Quaternary Slip History of the Carboneras Fault, southeastern Spain. J. Geodynamics 24, 51–66. doi:10.1016/s0264-3707(96)00029-4
Boillot, G., Dupeuble, P. A., and Malod, J. (1979). Subduction and Tectonics on the continental Margin off Northern Spain. Mar. Geology. 32, 53–70. doi:10.1016/0025-3227(79)90146-4
Borque, M. J., Sánchez‐Alzola, A., Martin‐Rojas, I., Alfaro, P., Molina, S., Rosa‐Cintas, S., et al. (2019). How Much Nubia‐Eurasia Convergence Is Accommodated by the NE End of the Eastern Betic Shear Zone (SE Spain)? Constraints from GPS Velocities. Tectonics 38, 1824–1839. doi:10.1029/2018TC004970
Bourgois, J., Mauffret, A., Ammar, A., and Demnati, A. (1992). Multichannel Seismic Data Imaging of Inversion Tectonics of the Alboran Ridge (Western Mediterranean Sea). Geo-Marine Lett. 12, 117–122. doi:10.1007/bf02084921
Buforn, E., Pro, C., Sanz de Galdeano, C., Cantavella,., J. V., Cesca, S., Caldeira, B., et al. (2017). The 2016 South Alboran Earthquake (Mw= 6.4): A Reactivation of the Ibero-Maghrebian Region?. Tectonophysics 712-713, 704–715. doi:10.1016/j.tecto.2017.06.033
Buforn, E., Udías, A., and Pro, C. (2015). Large Earthquakes at the Ibero-Maghrebian Region: Basis for an EEWS. Pure Appl. Geophys. 172 (9), 2387–2396. doi:10.1007/s00024-014-0954-0
Cadenas, P., Fernández-Viejo, G., Pulgar, J. A., Tugend, J., Manatschal, G., and Minshull, T. A. (2018). Constraints Imposed by Rift Inheritance on the Compressional Reactivation of a Hyperextended Margin: Mapping Rift Domains in the North Iberian Margin and in the Cantabrian Mountains. Tectonics 37 (3), 758–785. doi:10.1002/2016tc004454
Chalouan, A., Gil, A. J., Galindo-Zaldívar, J., Ahmamou, M. F., Ruano, P., De Lacy, M. C., et al. (2014). Active Faulting in the Frontal Rif Cordillera (Fes Region, Morocco): Constraints from GPS Data. J. Geodynamics 77, 110–122. doi:10.1016/j.jog.2014.01.002
Comas, M. C., García-Dueñas, V., and Jurado, M. J. (1992). Neogene Tectonic Evolution of the Alboran Sea from MCS Data. Geo-mar. Lett. 12 (2-3), 157–164. doi:10.1007/bf02084927
Comas, M. C., Platt, J. P., Soto, J. I., and Watts, A. B. (1999). The Origin and Tectonic History of the Alboran Basin: Insights from Leg 161 Results. in Proceedings of the Ocean Drilling Program Scientific Results. Editors M. C. Zahn, and A. ComasKlaus (College Station, TX: Ocean Drilling Program), 161, 555–580.
Custódio, S., Lima, V., Vales, D., Cesca, S., and Carrilho, F. (2016). Imaging Active Faulting in a Region of Distributed Deformation from the Joint Clustering of Focal Mechanisms and Hypocentres: Application to the Azores-Western Mediterranean Region. Tectonophysics 676, 70–89. doi:10.1016/j.tecto.2016.03.013
d'Acremont, E., Gutscher, M.-A., Rabaute, A., Mercier de Lépinay, B., Lafosse, M., Poort, J., et al. (2014). High-resolution Imagery of Active Faulting Offshore Al Hoceima, Northern Morocco. Tectonophysics 632, 160–166. doi:10.1016/j.tecto.2014.06.008
d'Acremont, E., Lafosse, M., Rabaute, A., Teurquety, G., Do Couto, D., Ercilla, G., et al. (2020). Polyphase Tectonic Evolution of Fore-Arc basin Related to STEP Fault as Revealed by Seismic Reflection Data from the Alboran Sea (W.Mediterranean). Tectonics 39, e2019TC005885. doi:10.1029/2019tc005885
de Vicente, G. (2009). Partición de la deformación Cenozoica intraplaca en el Sistema Central. Geogaceta 46, 23–26. Available at: https://sge.usal.es/archivos/geogacetas/geo46/art06.pdf
Dewey, J. F., Helman, M. L., Knott, S. D., Turco, E., and Hutton, D. H. W. (1989). “Kinematics of the Western Mediterranean,” in Alpine Tectonics. Editors M P. Coward, D. Dietrich, and R G. Park (London: Geol. Soc. London Spec. Publ.), 45, 265–283. doi:10.1144/gsl.sp.1989.045.01.15
Duarte, J. C., Terrinha, P., Rosas, F. M., Valadares, V., Pinheiro, L. M., Matias, L., et al. (2010). Crescent-shaped Morphotectonic Features in the Gulf of Cadiz (Offshore SW Iberia). Mar. Geology. 271, 236–249. doi:10.1016/j.margeo.2010.02.017
Duarte, J. C., Valadares, V., Terrinha, P., Rosas, F., and ZitelliniGràcia, N. E. (2009). Anatomy and Tectonic Significance of WNW-ESE and NE-SW Lineaments at a Transpressive Plate Boundary (Nubia-Iberia). Trab. Geol. 29 (29), 237–241. Available at: https://oceanrep.geomar.de/27147/
Estrada, F., Ercilla, G., and Alonso, B. (1997). Pliocene-Quaternary Tectonic-Sedimentary Evolution of the NE Alboran Sea (SW Mediterranean Sea). Tectonophysics 282, 423–442. doi:10.1016/s0040-1951(97)00227-8
Estrada, F., Galindo-Zaldívar, J., González-Vida, J. M., Vázquez, J. T., Ercilla, G., d’Acremont, E., et al. (2018b). “Indentación Tectónica en la Zona Central del Mar de Alborán”Tercera Reunión Ibérica sobre Fallas Activas y Paleosismología, Alicante,” in Avances en el estudio de Fallas Activas, Terremotos y Peligrosidad Sísmica de Iberia. Eds. C. Canora, F. Martín, E. Masana, R. Pérez, and M. Ortuño Spain: University of Alicante, 121–124.
Estrada, F., Galindo-Zaldívar, J., Vázquez, J. T., Ercilla, G., D'Acremont, E., Alonso, B., et al. (2018a). Tectonic Indentation in the central Alboran Sea (Westernmost Mediterranean). Terra Nova 30 (1), 24–33. doi:10.1111/ter.12304
Fernández-Ibáñez, F., Soto, J. I., Zoback, M. D., and Morales, J. (2007). Present-day Stress Field in the Gibraltar Arc (Western Mediterranean). J.Geophys. Res. 112, B08404 (88), 1–25. doi:10.1029/2006JB004683
Galindo-Zaldívar, J., Azzouz, O., Chalouan, A., Pedrera, A., Ruano, P., Ruiz-Constán, A., et al. (2015). Extensional Tectonics, Graben Development and Fault Terminations in the Eastern Rif (Bokoya-Ras Afraou Area). Tectonophysics 663, 140–149. doi:10.1016/j.tecto.2015.08.029
Galindo-Zaldívar, J., Borque, M. J., Pedrera, A., Marín-Lechado, C., Gil, A. J., and López-Garrido, A. C. (2013). Deformation Behaviour of the Low-Rate Active Balanegra Fault Zone from High-Precision Levelling (Betic Cordillera, SE Spain). J. Geodynamics 71, 43–51. doi:10.1016/j.jog.2013.07.003
Galindo-Zaldívar, J., Ercilla, G., Estrada, F., Catalán, M., d'Acremont, E., Azzouz, O., et al. (2018). Imaging the Growth of Recent Faults: The Case of 2016-2017 Seismic Sequence Sea Bottom Deformation in the Alboran Sea (Western Mediterranean). Tectonics 37, 2513–2530. doi:10.1029/2017TC004941
Gallastegui, J., Pulgar, J. A., and Gallart, J. (2002). Initiation of an Active Margin at the North Iberian Continent-Ocean Transition. Tectonics 21 (4), 15. doi:10.1029/2001tc901046
Gárate, J., Martin-Davila, J., Khazaradze, G., Echeverria, A., Asensio, E., Gil, A. J., et al. (2015). Topo-Iberia Project: CGPS Crustal Velocity Field in the Iberian Peninsula and Morocco. GPS Solut 19, 287–295. doi:10.1007/s10291-014-0387-3
Giaconia, F., Booth-Rea, G., Ranero, C. R., Gràcia, E., Bartolome, R., Calahorrano, A., et al. (2015). Compressional Tectonic Inversion of the Algero-Balearic basin: Latemost Miocene to Present Oblique Convergence at the Palomares Margin (Western Mediterranean). Tectonics 34 (7), 1516–1543. doi:10.1002/2015TC003861
Giardini, D., Woessner, J., Danciu, L., Crowley, H., Cotton, F., Grónthal, G., et al. (2013). Seismic Hazard Harmonization in Europe (SHARE): Zurich, Switzerland: Swiss Seism, Serv ETH Zurich. Online Data Resource. doi:10.12686/SED-00000001-SHARE
Gómez de la Peña, L., Gràcia, E., Muñoz, A., Acosta, J., Gómez-Ballesteros, M., R. Ranero, C., et al. (2016). Geomorphology and Neogene Tectonic Evolution of the Palomares continental Margin (Western Mediterranean). Tectonophysics 689, 25–39. doi:10.1016/j.tecto.2016.03.009
Gómez de la Peña, L., Grevemeyer, I., Kopp, H., Díaz, J., Gallart, J., Booth-Rea, G., et al. (2020). The Lithospheric Structure of the Gibraltar Arc System from Wide-Angle Seismic Data. J. Geophys. Res. 125, e2020JB019854. doi:10.1029/2020JB019854
Gómez de la Peña, L., Ranero, C. R., and Gràcia, E. (2018). The Crustal Domains of the Alboran Basin (Western Mediterranean). Tectonics 37 (10), 3352–3377. doi:10.1029/2017tc004946
Gràcia, E., Bartolome, R., Lo Iacono, C., Moreno, X., Stich, D., Martínez-Diaz, J. J., et al. (2012). Acoustic and Seismic Imaging of the Adra Fault (NE Alboran Sea): in Search of the Source of the 1910 Adra Earthquake. Nat. Hazards Earth Syst. Sci. 12 (11), 3255–3267. doi:10.5194/nhess-12-3255-2012
Gràcia, E., Bartolome, R., Perea, H., Moreno, X., Gómez de la Peña, L., Ranero, C. R., et al. (2014). ““Seismic hazard of Active Faults in the Alboran Sea Inferred from Submarine Paleosismology”,” in Una aproximación multidisciplinar al estudio de las fallas activas, los terremotos y el riesgo sísmicoSegunda reunión ibérica sobre fallas activas y paleosismología. Editors J. A. Álvarez-Gomez, and F. Martín González. (Lorca: Murcia, España), 101–104.
Gràcia, E., Dañobeitia, J., Vergés, J., Bartolomé, R., and Córdoba, D. (2003b). Crustal Architecture and Tectonic Evolution of the Gulf of Cadiz (SW Iberian Margin) at the Convergence of the Eurasian and African Plates. Tectonics 22 (4), a–n. doi:10.1029/2001TC901045
Gràcia, E., Dañobeitia, J., Vergés, J., and Team, P. (2003a). Mapping Active Faults Offshore Portugal (36°N-38°N): Implications for Seismic hazard Assessment along the Southwest Iberian Margin. Geol 31, 83–86. doi:10.1130/0091-7613(2003)031<0083:MAFOPN>2.0.CO;2
Gràcia, E., Grevemeyer, I., Bartolomé, R., Perea, H., Martínez-Loriente, S., Gómez de la Peña, L., et al. (2019). Earthquake Crisis Unveils the Growth of an Incipient continental Fault System. Nat. Commun. 10, 3482. doi:10.1038/s41467-019-11064-5
Gràcia, E., Pallàs, R., Soto, J. I., Comas, M., Moreno, X., Masana, E., et al. (2006). Active Faulting Offshore SE Spain (Alboran Sea): Implications for Earthquake hazard Assessment in the Southern Iberian Margin. Earth Planet. Sci. Lett. 241 (3-4), 734–749. doi:10.1016/j.epsl.2005.11.009
Grevemeyer, I., Gràcia, E., Villaseñor, A., Leuchters, W., and Watts, A. B. (2015). Seismicity and Active Tectonics in the Alboran Sea, Western Mediterranean: Constraints from an Offshore-Onshore Seismological Network and Swath Bathymetry Data. J. Geophys. Res. 121, 767–787. doi:10.1002/2015JB012352
Gutscher, M.-A., Dominguez, S., Westbrook, G. K., Gente, P., Babonneau, N., Mulder, T., et al. (2009). Tectonic Shortening and Gravitational Spreading in the Gulf of Cadiz Accretionary Wedge: Observations from Multi-Beam Bathymetry and Seismic Profiling. Mar. Pet. Geology. 26 (5), 647–659. doi:10.1016/j.marpetgeo.2007.11.008
Gutscher, M.-A., Malod, J., Rehault, J.-P., Contrucci, I., Klingelhoefer, F., Mendes-Victor, L., et al. (2002). Evidence for Active Subduction beneath Gibraltar. Geol 30 (12), 1071–1074. doi:10.1130/0091-7613(2002)030<1071:efasbg>2.0.co;2
Hernández-Molina, J., Llave, E., Somoza, L., Fernández-Puga, M. C., Maestro, A., León, R., et al. (2003). Looking for Clues to Paleoceanographic Imprints: A Diagnosis of the Gulf of Cadiz Contourite Depositional Systems. Geol 31, 19–22. doi:10.1130/0091-7613(2003)031<0019:lfctpi>2.0.co;2
Ivanov, M. K., Kenyon, N., Nielsen, T., Wheeler, A., Monteiro, J., Gardner, J., et al. (2000). Scientific Party, TTR-9 Cruise Goals and Principal Results of the TTR-9 Cruise. Ioc/ UNESCO Work Rep. 168, 3–4 .
Jiménez-Munt, I., Fernàndez, M., Torné, M., and Bird, P. (2001). The Transition from Linear to Diffuse Plate Boundary in the Azores-Gibraltar Region: Results from a Thin-Sheet Model. Earth Planet. Sci. Lett. 192, 175–189. doi:10.1016/s0012-821x(01)00442-3
Jolivet, L., and Faccenna, C. (2000). Mediterranean Extension and the Africa–Eurasia Collision. Tectonics 1265 (19), 1095–1106. doi:10.1029/2000tc900018
Lafosse, M., d'Acremont, E., Rabaute, A., Estrada, F., Jollivet-Castelot, M., Vázquez, J. T., et al. (2020). Plio-Quaternary Tectonic Evolution of the Southern Margin of the Alboran Basin (Western Mediterranean). Solid Earth 11, 714–765. doi:10.5194/se-11-741-2020
Lafosse, M., Gorini, C., Le Roy, P., Alonso, B., d’Acremont, E., Ercilla, G., et al. (2018). Late Pleistocene-Holocene History of a Tectonically Active Segment of the continental Margin (Nekor basin, Western Mediterranean, Morocco). Mar. Pet. Geology. 97, 370–389. doi:10.1016/j.marpetgeo.2018.07.022
Lario, J., Zazo, C., Goy, J. L., Silva, P. G., Bardají, T., Cabero, A., et al. (2011). Holocene Palaeotsunami Catalogue of SW Iberia. Quat. Int. 242 (1), 196–200. doi:10.1016/j.quaint.2011.01.036
Le Pichon, X., Bonnin, J., Francheteau, J., and Sibuet, J. C. (1971). “Une hypothèse d’évolution tectonique du golfe de Gascogne,” in Editor Debyseret al. Histoire structurale du Golfe de Gascogne, (Technip, Eds, Paris).
Le Pichon, X., and Sibuet, J. C. (1971). “Western Extension of Boundary Between European and Iberian Plates During the Pyrenean Orogen. Earth Planetary Sci. Letter., 12(1), 83–88.
León, R., Somoza, L., Medialdea, T., Vázquez, J. T., González, F. J., López-González, N., et al. (2012). New Discoveries of Mud Volcanoes on the Moroccan Atlantic continental Margin (Gulf of Cádiz): Morpho-Structural Characterization. Geo-mar Lett. 32, 473–488. doi:10.1007/s00367-012-0275-1
Lonergan, L., and White, N. (1997). Origin of the Betic-Rif Mountain belt. Tectonics 16, 504–522. doi:10.1029/96tc03937
López-Fernández, C., Pulgar, J. A., Díaz, J., Gallart, J., González-Cortina, J. M., and Ruiz, M. (2012). Seismotectonic Characterization of the Becerreá Area (NW Spain). Geologica Acta 10 (1), 1–11. doi:10.1344/105.000001750
Maldonado, A., Somoza, L., and Pallarés, L. (1999). The Betic Orogen and the Iberian-African Boundary in the Gulf of Cadiz: Geological Evolution (central North Atlantic). Mar. Geology. 155, 9–43. doi:10.1016/s0025-3227(98)00139-x
Malod, J. A., Murillas, J., Kornprobst, J., and Boillot, G. (1993). Oceanic Lithosphere at the Edge of a Cenozoic Active continental Margin (Northwestern Slope of Galicia Bank, Spain). Tectonophysics 221, 195–206. doi:10.1016/0040-1951(93)90332-e
Martínez‐Loriente, S., Gràcia, E., Bartolome, R., Sallarès, V., Connors, C., Perea, H., et al. (2013). Active Deformation in Old Oceanic Lithosphere and Significance for Earthquake hazard: Seismic Imaging of the Coral Patch Ridge Area and Neighboring Abyssal plains (SW Iberian Margin). Geochem. Geophys. Geosyst. 14, 2206–2231. doi:10.1002/ggge.20173
Martínez-García, P., Comas, M., Lonergan, L., and Watts, A. B. (2017). From Extension to Shortening: Tectonic Inversion Distributed in Time and Space in the Alboran Sea, Western Mediterranean. Tectonics 36 (12), 2777–2805. doi:10.1002/2017tc004489
Martínez-García, P., Comas, M., Soto, J. I., Lonergan, L., and Watts, A. B. (2013). Strike-slip Tectonics and basin Inversion in the Western Mediterranean: the Post-Messinian Evolution of the Alboran Sea. Basin Res. 25 (4), 361–387. doi:10.1111/bre.12005
Martínez-García, P., Soto, J. I., and Comas, M. (2010). Recent Structures in the Alboran Ridge and Yusuf Fault Zones Based on Swath Bathymetry and Sub-bottom Profiling: Evidence of Active Tectonics. Geo-mar. Lett. 31, 19–36. doi:10.1007/s00367-010-0212-0
Martínez-Loriente, S., Gràcia, E., Bartolome, R., Perea, H., Klaeschen, D., Dañobeitia, J. J., et al. (2018). Morphostructure, Tectono-Sedimentary Evolution and Seismic Potential of the Horseshoe Fault, SW Iberian Margin. Basin Res. 30, 382–400. doi:10.1111/bre.12225
Martínez-Loriente, S., Sallarès, V., Gràcia, E., Bartolome, R., Dañobeitia, J. J., and Zitellini, N. (2014). Seismic and Gravity Constraints on the Nature of the Basement in the Africa-Eurasia Plate Boundary: New Insights for the Geodynamic Evolution of the SW Iberian Margin. J. Geophys. Res. Solid Earth 119 (1), 127–149. doi:10.1002/2013jb010476
Masana, E., Moreno, X., Gràcia, E., Pallás, R., Ortuño, M., López, R., et al. (2018). First Evidence of Paleoearthquakes along the Carboneras Fault Zone (SE Iberian Peninsula): Los Trances Site. Geol. Acta 16 (4), 461–476. doi:10.1344/GeologicaActa2018.16.4.8
Mauffret, A., Ammar, A., Gorini, C., and Jabour, N. (2007). The Alboran Sea (Western Mediterranean) Revisited with a View from the Moroccan Margin. Terra Nova 19, 195–203. doi:10.1111/j.1365-3121.2007.00734.x
Mauffret, A., Maldonado, A., and Campillo, A. C. (1992). Tectonic Framework of the Eastern Alboran and Western Algerian Basins, Western Mediterranean. Geo-Marine Lett. 12, 104–110. doi:10.1007/bf02084919
Medialdea, T. (2007). Estructura y evolución tectónica del Golfo de Cádiz. Pub. Instituto Geológico y Minero de España. Madrid: Serie Tesis Doctorales n° 8.
Medialdea, T., Somoza, L., Bohoyo, F., Vázquez, J. T., Vegas, R., Patriat, M., et al. (2009b). Cenozoic Compression in the Union Basin and Armorican Seamount (Biscay Abyssal Plain). 6th Symposium on the Iberian Atlantic Margin MIA09. Spain: Oviedo, 77–80.
Medialdea, T., Somoza, L., Pinheiro, L. M., Fernández-PugaVázquez, M. C. J. T., Vázquez, J. T., León, R., et al. (2009a). Tectonics and Mud Volcano Development in the Gulf of Cádiz. Mar. Geology. 261, 48–63. doi:10.1016/j.margeo.2008.10.007
Medialdea, T., Vegas, R., Somoza, L., Vázquez, J. T., Maldonado, A., Dı́az-del-Rı́o, V., et al. (2004). Structure and Evolution of the "Olistostrome" Complex of the Gibraltar Arc in the Gulf of Cádiz (Eastern Central Atlantic): Evidence from Two Long Seismic Cross-Sections. Mar. Geology. 209, 173–198. doi:10.1016/j.margeo.2004.05.029
Miranda, J. M., Luis, J. F., Lourenço, N., and Goslin, J. (2014). Distributed Deformation Close to the Azores Triple “Point”. Mar. Geology. 355, 27–35. doi:10.1016/j.margeo.2014.05.006
Molina-Aguilera, A., Mancilla, F. L., Morales, J., Stich, D., Yuan, X., and Heit, B. (2019). Connection between the Jurassic Oceanic Lithosphere of the Gulf of Cádiz and the Alboran Slab Imaged by Sp Receiver Functions. Geology, 47, 227–230. doi:10.1130/G45654.1
Moreno, X., Gràcia, E., Bartolomé, R., Martínez-Loriente, S., Perea, H., de la Peña, L. G., et al. (2016). Seismostratigraphy and Tectonic Architecture of the Carboneras Fault Offshore Based on Multiscale Seismic Imaging: Implications for the Neogene Evolution of the NE Alboran Sea. Tectonophysics 689, 115–132. doi:10.1016/j.tecto.2016.02.018
Moreno, X., Masana, E., Pallàs, R., Gràcia, E., Rodés, Á., and Bordonau, J. (2015). Quaternary Tectonic Activity of the Carboneras Fault in the La Serrata Range (SE Iberia): Geomorphological and Chronological Constraints. Tectonophysics 663, 78–94. doi:10.1016/j.tecto.2015.08.016
Murillas, J., Mougenot, D., Boulot, G., Comas, M. C., Banda, E., and Mauffret, A. (1990). Structure and Evolution of the Galicia Interior Basin (Atlantic Western Iberian continental Margin). Tectonophysics 184, 297–319. doi:10.1016/0040-1951(90)90445-e
Negredo, A. M., Bird, P., Sanz de Galdeano, C., and Buforn, E. (2002). Neotectonic Modeling of the Ibero-Maghrebian Region. J. Geophys. Res. 107 (B11), 2292. doi:10.1029/2001JB000743
Neres, M., Carafa, M. M. C., Fernandes, R. M. S., Matias, L., Duarte, J. C., Barba, S., et al. (2016). Lithospheric Deformation in the Africa-Iberia Plate Boundary: Improved Neotectonic Modeling Testing a Basal-Driven Alboran Plate. J. Geophys. Res. Solid Earth 121, 6566–6596. doi:10.1002/2016JB013012
Nocquet, J.-M., and Calais, E. (2004). Geodetic Measurements of Crustal Deformation in the Western Mediterranean and Europe. Pure Appl. Geophys. 161, 661–681. doi:10.1007/978-3-0348-7899-9_11
Olaiz, A. J., Muñoz-Martín, A., De Vicente, G., Vegas, R., and Cloetingh, S. (2009). European Continuous Active Tectonic Strain-Stress Map. Tectonophysics 474, 33–40. doi:10.1016/j.tecto.2008.06.023
Palano, M., González, P. J., and Fernández, J. (2014). “An Update GPS Velocity and Strain Rate Fields for the Iberian Region,” in Mathematics of Planet Earth. Editor E. Pardo-Igúzquiza (369, Lecture Notes in Earth System Sciences) Berlin, Heidelberg: Springer-Verlag, 369–372. doi:10.1007/978-3-642-32408-6_82
Palano, M., González, P. J., and Fernández, J. (2013). Strain and Stress fields along the Gibraltar Orogenic Arc: Constraints on Active Geodynamics. Gondwana Res. 23 (3), 1071–1088. doi:10.1016/j.gr.2012.05.021
Palano, M., González, P. J., and Fernández, J. (2015). The Diffuse Plate Boundary of Nubia and Iberia in the Western Mediterranean: Crustal Deformation Evidence for Viscous Coupling and Fragmented Lithosphere. Earth Planet. Sci. Lett. 430, 439–447. doi:10.1016/j.epsl.2015.08.040
Pedrera, A., Ruiz-Constán, A., Galindo-Zaldívar, J., Chalouan, A., Sanz de Galdeano, C. S., et al. (2011). Is There an Active Subduction beneath the Gibraltar Orogenic Arc? Constraints from Pliocene to Present-Day Stress Field. J. Geodynamics 52 (2), 83–96. doi:10.1016/j.jog.2010.12.003
Peláez, J. A., Henares, J., Hamdache, M., and Sanz de Galdeano, C. (2018). “A Seismogenic Zone Model for Seismic Hazard Studies in Northwestern Africa,” in Moment Tensor Solutions. A Useful Tool for Seismotectonics. Editor S. d’Amico (Springer Natural Hazards, Springer, Cham), 643–680. doi:10.1007/978-3-319-77359-9_29
Perea, H., Gràcia, E., Martínez-Loriente, S., Bartolome, R., de la Peña, L. G., de Mol, B., et al. (2018). Kinematic Analysis of Secondary Faults within a Distributed Shear-Zone Reveals Fault Linkage and Increased Seismic hazard. Mar. Geology. 399, 23–33. doi:10.1016/j.margeo.2018.02.002
Pinheiro, L. M., Ivanov, M. K., Sautkin, A., Akhmanov, G., Magalhães, V. H., Volkonskaya, A., et al. (2003). Mud Volcanism in the Gulf of Cadiz: Results from the TTR-10 Cruise. Mar. Geology. 195, 131–151. doi:10.1016/S0025-3227(02)00685-0
Pulgar, J. A., Gallart, J., Fernández-Viejo, G., Pérez-Estaún, A., and Álvarez-Marrón, J. (1996). ESCIN GroupSeismic Image of the Cantabrian Mountains in the Western Extension of the Pyrenees from Integrated ESCIN Reflection and Refraction Data. Tectonophysics 264, 1–19. doi:10.1016/s0040-1951(96)00114-x
Ramos, A., Fernández, O., Muñoz, J. A., and Terrinha, P. (2017c). Impact of basin Structure and Evaporite Distribution on Salt Tectonics in the Algarve Basin, Southwest Iberian Margin. Mar. Pet. Geology. 88, 961–984. doi:10.1016/j.marpetgeo.2017.09.028
Ramos, A., Fernández, O., Terrinha, P., Muñoz, J. A., and Arnaiz, Á. (2020). Paleogeographic Evolution of a Segmented Oblique Passive Margin: the Case of the SW Iberian Margin. Int. J. Earth Sci. (Geol Rundsch) 109, 1871–1895. doi:10.1007/s00531-020-01878-w
Ramos, A., Fernández, O., Terrinha, P., and Muñoz, J. A. (2017a). Neogene to Recent Contraction and basin Inversion along the Nubia-Iberia Boundary in SW Iberia. Tectonics 36, 257–286. doi:10.1002/2016TC004262
Ramos, A., Fernández, O., Torné, M., Sánchez de la Muela, A., Muñoz, J. A., Terrinha, P., et al. (2017b). Crustal Structure of the SW Iberian Passive Margin: the Westernmost Remnant of the Ligurian Tethys?. Tectonophysics 705, 42–62. doi:10.1016/j.tecto.2017.03.012
Ribeiro, A., Cabral, J., Baptista, R., and Matias, L. (1996). Stress Pattern in Portugal mainland and the Adjacent Atlantic Region, West Iberia. Tectonics 15 (3), 641–659. doi:10.1029/95tc03683
Rosas, F. M., Duarte, J. C., Terrinha, P., Valadares, V., and Matias, L. (2009). Morphotectonic Characterization of Major Bathymetric Lineaments in Gulf of Cadiz (Africa–Iberia Plate Boundary): Insights from Analogue Modelling Experiments. Mar. Geol. 261 (1-4), 33–47. doi:10.1016/j.margeo.2008.08.002
Rutter, E. H., Faulkner, D. R., and Burgess, R. (2012). Structure and Geological History of the Carboneras Fault Zone, SE Spain: Part of a Stretching Transform Fault System. J. Struct. Geology. 45, 68–86. doi:10.1016/j.jsg.2012.08.009
Ryan, W. B. F., Carbotte, S. M., Coplan, J. O., O'Hara, S., Melkonian, A., Arko, R., et al. (2009). Global Multi-Resolution Topography Synthesis. Geochem. Geophys. Geosyst. 10, a–n. doi:10.1029/2008GC002332
Sallarès, V., Gailler, A., Gutscher, M.-A., Graindorge, D., Bartolomé, R., Gràcia, E., et al. (2011). Seismic Evidence for the Presence of Jurassic Oceanic Crust in the central Gulf of Cadiz (SW Iberian Margin). Earth Planet. Sci. Lett. 311 (1–2), 112–123. doi:10.1016/j.epsl.2011.09.003
Sartori, R., Torelli, L., Zitellini, N., Peis, D., and Lodolo, E. (1994). Eastern Segment of the Azores-Gibraltar Line (central-eastern Atlantic) : An Oceanic Plate Boundary with Diffuse Compressional Deformation. Geol 22 (6), 555–558. doi:10.1130/0091-7613(1994)022<0555:esotag>2.3.co;2
Serpelloni, E., Vannucci, G., Pondrelli, S., Argnani, A., Casula, G., Anzidei, M., et al. (2007). Kinematics of the Western Africa-Eurasia Plate Boundary from Focal Mechanisms and GPS Data. Geophys. J. Int. 169, 1180–1200. doi:10.1111/j.1365-246X.2007.03367.x
Sibuet, J.-C., and Collette, B. J. (1991). Triple Junctions of Bay of Biscay and North Atlantic: New Constraints on the Kinematic Evolution. Geol 19 (5), 522–525. doi:10.1130/0091-7613(1991)019<0522:tjobob>2.3.co;2
Sibuet, J.-C., Srivastava, S. P., and Spakman, W. (2004). Pyrenean Orogeny and Plate Kinematics. J. Geophys. Res. 109. doi:10.1029/2003JB002514
Silva, P. G., Goy, J. L., Somoza, L., Zazo, C., and Bardají, T. (1993). Landscape Response to Strike-Slip Faulting Linked to Collisional Settings: Quaternary Tectonics and basin Formation in the Eastern Betics, southeastern Spain. Tectonophysics 224 (4), 289–303. doi:10.1016/0040-1951(93)90034-H
Somoza, L. (1993). Study of the Quaternary coast between Cabo de Palos and Guardamar (Murcia-Alicante) regarding the variations of sea level in relation to the geodynamic context. Spec. Issue. Boletin Instituto Oceanográfico 12, 4.
Somoza, L., Ayala, C., Bárcenas, P., Berndt, J., Bohoyo, F., Fernández, F., et al. (2005). Informe Científico Campaña BREOGHAM. Hespérides 2005, 319. doi:10.20351/29HE20050906
Somoza, L., and UTM-CSIC, . (2018). MVSEIS Cruise, RV Hespérides. [Data set]. UTM-CSIC. doi:10.20351/29HE20080516
Somoza, L., Medialdea, T., González, F. J., León, R., Palomino, D., Rengel, J., et al. (2019). Morphostructure of the Galicia continental Margin and Adjacent Deep Ocean Floor: From Hyperextended Rifted to Convergent Margin Styles. Mar. Geology. 407, 299–315. doi:10.1016/j.margeo.2018.11.011
Somoza, L., Medialdea, T., González, F. J., Machancoses, S., Candón, J. A., Cid, C., et al. (2021). High-Resolution Multibeam Bathymetry of the Northern Mid-Atlantic Ridge at 45‐46°N: the Moytirra Hydrothermal Field. J. Maps 17 (22), 184–196.
Soumaya, A., Ben Ayed, N., Rajabi, M., Meghraoui, M., Delvaux, D., Kadri, A., et al. (2018). Active Faulting Geometry and Stress Pattern Near Complex Strike‐Slip Systems along the Maghreb Region: Constraints on Active Convergence in the Western Mediterranean. Tectonics 37, 3148–3173. doi:10.1029/2018TC004983
Srivastava, S. P., Roest, W. R., Kovacs, L. C., Oakey, G., Lévesque, S., Verhoef, J., et al. (1990). Motion of Iberia since the Late Jurassic: Results from Detailed Aeromagnetic Measurements in the Newfoundland Basin. Tectonophysics 184, 229–260. doi:10.1016/0040-1951(90)90442-b
Stich, D., Mancilla, F. d. L., Pondrelli, S., and Morales, J. (2007). Source Analysis of the February 12th 2007, Mw6.0 Horseshoe Earthquake: Implications for the 1755 Lisbon Earthquake. Geophys. Res. Lett. 34, 12. doi:10.1029/2007GL030012
Stich, D., Martín, R., Morales, J., López-Comino, J. Á., and Mancilla, F. d. L. (2020). Slip Partitioning in the 2016 Alboran Sea Earthquake Sequence (Western Mediterranean). Front. Earth Sci. 8, 587356. doi:10.3389/feart.2020.587356
Stich, D., Serpelloni, E., de Lis Mancilla, F., and Morales, J. (2006). Kinematics of the Iberia-Maghreb Plate Contact from Seismic Moment Tensors and GPS Observations. Tectonophysics 426, 295–317. doi:10.1016/j.tecto.2006.08.004
Terrinha, P., Matias, L., Vicente, J., Duarte, J., Luís, J., Pinheiro, L., et al. (2009). Morphotectonics and Strain Partitioning at the Iberia-Africa Plate Boundary from Multibeam and Seismic Reflection Data. Mar. Geology. 267, 156–174. doi:10.1016/j.margeo.2009.09.012
Terrinha, P., Medialdea, T., Batista, L., Somoza, L., Magalhães, V., González, F. J., et al. (2020). “Integrated Thematic Geological Mapping of the Atlantic Margin of Iberia,” in From Continental Shelf to Slope: Mapping the Oceanic Realm. Editors K. Asch, H. Kitazato, and H. Vallius (London: Geological Society, Special Publications), 505. doi:10.1144/SP505-2019-90
Terrinha, P., Pinheiro, L. M., Henriet, J.-P., Matias, L., Ivanov, M. K., Monteiro, J. H., et al. (2003). Tsunamigenic-seismogenic Structures, Neotectonics, Sedimentary Processes and Slope Instability on the Southwest Portuguese Margin. Mar. Geology. 195, 55–73. doi:10.1016/s0025-3227(02)00682-5
Terrinha, P., Ramos, A., Neres, M., Valadares, V., Duarte, J., Martínez-Loriente, S., et al. (2019). The Alpine Orogeny in the West and Southwest Iberia Margins. (Cham, Switzerland: Springer), 487–505. doi:10.1007/978-3-030-11295-0_11
Toyos, M. H., Medialdea, T., León, R., Somoza, L., González, F. J., and Meléndez, N. (2016). Evidence of Episodic Long-Lived Eruptions in the Yuma, Ginsburg, Jesús Baraza and Tasyo Mud Volcanoes, Gulf of Cádiz. Geo-mar. Lett. 36 (3), 197–214. doi:10.1007/s00367-016-0440-z
Udías, A. (2015). Historical Earthquakes (Before 1755) of the Iberian Peninsula in Early Catalogs. Seismological Res. Lett. 86 (3), 999–1005. doi:10.1785/0220140200
Vázquez, J. T., Somoza, L., Medialdea, T., Ercilla, G., Bohoyo, F., León, R., Estrada, F., and Iglesias, J. (2009a). “The Finisterre Seamount: A Submarine Elevation of Compressive Origin in the North-Western Corner of the Galicia Bank Continental Margin”, in: Editors J. S. Troncoso, I. Alejo, and J. López. II International Symposium in Marine Sciences (ISMSO9), 27-30 April 2009, Vigo, Spain: Uninersity of Vigo, Abstracts Volume, 259.
Vázquez, J. T., Alonso, B., Fernández-Puga, M. C., Gómez-Ballesteros, M., Iglesias, J., Palomino, D., et al. (2015). Seamounts along the Iberian continental Margins: A Key Morphological Feature. Bol. Geol. Min 126 (2-3), 483–514. Available at: https://www.igme.es/boletin/2015/126_2y3/13-Articulo%209.pdf
Vázquez, J. T., Ercilla, G., Alonso, B., Pelaez, J. P., Palomino, D., León, R., et al. (2021a). ““Triggering Processes of Tsunamis in the Alboran Sea and Gulf of Cádiz: A General Review”, in Historical Earthquakes and Tsunamis in the Iberian Peninsula - an Interdiciplinary Dialogue. Editors M. Álvarez Martí-Aguilar, and F. Machuca Prieto (Springer Earth System Sciences).
Vázquez, J. T., Ercilla, G., Catalán, M., Do Couto, D., Estrada, F., Galindo-Zaldívar, J., et al. (2021b). “A Geological History for the Alboran Sea Region”, in Alboran Sea and its Marine Resources. Editors J. C. Baéz, J. A. Camiñas, J. T. Vázquez, and M. Malouli (Switzerland, AG: Springer Nature).
Vázquez, J. T., Ercilla, G., Medialdea, T., Somoza, L., Bohoyo, F., Casas, D., et al. (2009b). “El colapso BURATO ERGAP: Un rasgo morfo-tectónico de primera magnitud en el Banco de Galicia. in eds. G. Flor Rodríguez, J. Gallastegui, G. Flor Blanco, and J. Martín Llaneza, 6th Symposium on the Iberian Atlantic Margin MIA09, 1-5 December 2009, (Spain: Oviedo), 205–208.
Vázquez, J. T., Ercilla, G., Medialdea, T., Somoza, L., Bohoyo, F., Estrada, F., et al. (2009c). “Deformaciones neotectónicas en la Zona Transicional de la región del Banco de Galicia.” in eds. G. Flor Rodríguez, J. Gallastegui, G. Flor Blanco, and J. Martín Llaneza, 6th Symposium on the Iberian Atlantic Margin MIA09. (Spain: Oviedo), 45–48.
Vázquez, J. T., Estrada, F., Ercilla, G., d’Acremont, E., Fernández-Salas, L. M., Galindo-Zaldívar, J., et al. (2018). “Geomorfología y deformaciones cuaternarias en zonas de falla de la plataforma marginal de Motril-Djibouti,” in Avances en el estudio de Fallas Activas Terremotos y Peligrosidad Sísmica de Iberia. Editors C. Canora, F. Martín, E. Masana, R. Pérez, and M. Ortuño (Alicante (España): Tercera reunión ibérica sobre fallas activas y paleosismología), 137–140.
Vázquez, J. T., Estrada, F., Vegas, R., Ercilla, G., Alonso, B., Fernández-Salas, L. M., et al. (2016). “Geomorfología submarina en relación con la actividad tectónica Cuaternaria en la Cuenca del Mar de Alborán”,” in Comprendiendo el relieve: del pasado al futuro, Editors J. J Durán, M. Montes, A. Robador, and A. Salazar (Madrid, Spain: Pub IGME Geología y Geofísica) 5, 587–595.
Vázquez, J. T., Estrada, F., Vegas, R., Ercilla, G., d’Acremont, E., Fernández-Salas, L. M., et al. (2014). ““Quaternary Tectonics Influence on the Adra continental Slope Morphology (Northern Alboran Sea)”,” in Una aproximación multidisciplinar al estudio de las fallas activas, los terremotos y el riesgo sísmico. Editors J A. Álvarez-Gómez, and F. Martín-González (Murcia, España: Segunda reunión ibérica sobre fallas activas y paleosismología, Lorca), 89–92.
Vázquez, J. T., Medialdea, T., Ercilla, G., Somoza, L., Estrada, F., Fernández Puga, M. C., et al. (2008a). Cenozoic Deformational Structures on the Galicia Bank Region (NW Iberian continental Margin). Mar. Geology. 249, 128–149. doi:10.1016/j.margeo.2007.09.014
Vázquez, J. T., Medialdea, T., Somoza, L., Vegas, R., and Fernández-Puga, M. C. (2008b). Revisión de las estructuras neotectónicas en la región del Golfo de Cadiz. Margen continental y Llanuras abisales adyacentes. Geotemas 10, 592–594. Available at: https://sociedadgeologica.org/publicaciones/geotemas/geo-temas-10
Vázquez, J. T., Vegas, R., and Medialdea, T. (2008c). Recent Deformational Structures in the continental Margin of the Alborán Sea (Málaga-Adra Sector). Geo-Temas 10, 596–598. Available at: https://sociedadgeologica.org/publicaciones/geotemas/geo-temas-10
Vegas, R. (1991). “Present-dat Geodynamics of the Ibero-Maghrebian Region,” in Seismicity, Seismotectonics and Seismic Risk of the Ibero-Maghrebian Región, eds.: J. Mezcua, and A. Udías (Monografias, Instituto Geogrófico Nacional, Madrid, Spain), 193–203.
Vegas, R., Medialdea, T., and Vázquez, J. T. (2008). On the Nature of the Present-Day Plate Boundary between the Iberian Peninsula and Northern Africa. Geo-Temas 10, 1535–1538. Available at: https://sociedadgeologica.org/publicaciones/geotemas/geo-temas-10
Watts, A. B., Piatt, J. P., and Buhl, P. (1993). Tectonic Evolution of the Alboran Sea basin. Basin Res. 5, 153–177. doi:10.1111/j.1365-2117.1993.tb00063.x
Woodside, J. M., and Maldonado, A. (1992). Styles of Compressional Neotectonics in the Eastern Alboran Sea. Geo-Marine Lett. 12, 111–116. doi:10.1007/bf02084920
Zitellini, N., Chierici, F., Sartori, R., and Torelli, L. (1999). The Tectonic Source of the 1755 Lisbon Earthquake and Tsunami. Ann. Geofis 42 (1), 49–55. doi:10.4401/ag-3699
Keywords: submarine faults, tsunami and earthquake assessment, geodynamic activity, Iberian, plate tectonics, marine geophysical data, Atlantic-Mediterranean margins
Citation: Somoza L, Medialdea T, Terrinha P, Ramos A and Vázquez J-T (2021) Submarine Active Faults and Morpho-Tectonics Around the Iberian Margins: Seismic and Tsunamis Hazards. Front. Earth Sci. 9:653639. doi: 10.3389/feart.2021.653639
Received: 14 January 2021; Accepted: 15 June 2021;
Published: 30 June 2021.
Edited by:
Hector Perea, Complutense University of Madrid, SpainReviewed by:
Satish Chandra Singh, UMR7154 Institut de Physique du Globe de Paris (IPGP), FranceJacques Deverchere, Université de Bretagne Occidentale, France
Copyright © 2021 Somoza, Medialdea, Terrinha, Ramos and Vázquez. This is an open-access article distributed under the terms of the Creative Commons Attribution License (CC BY). The use, distribution or reproduction in other forums is permitted, provided the original author(s) and the copyright owner(s) are credited and that the original publication in this journal is cited, in accordance with accepted academic practice. No use, distribution or reproduction is permitted which does not comply with these terms.
*Correspondence: Luis Somoza, bC5zb21vemFAaWdtZS5lcw==