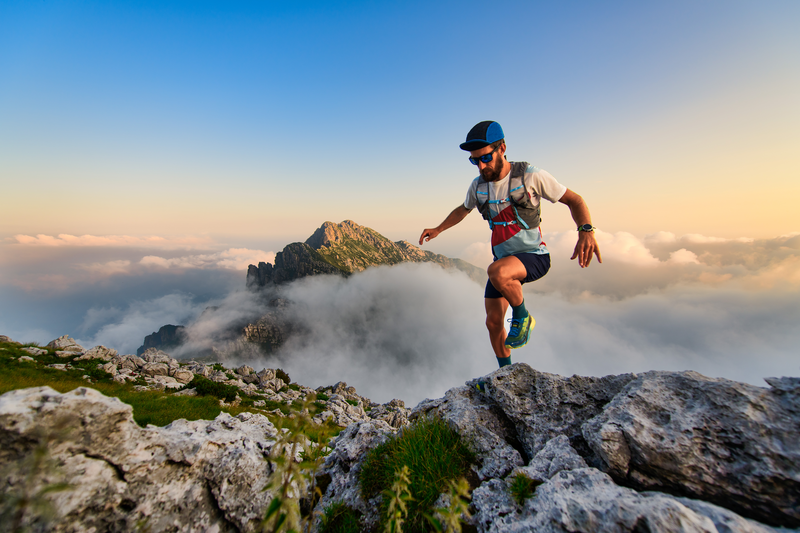
94% of researchers rate our articles as excellent or good
Learn more about the work of our research integrity team to safeguard the quality of each article we publish.
Find out more
ORIGINAL RESEARCH article
Front. Earth Sci. , 28 May 2021
Sec. Quaternary Science, Geomorphology and Paleoenvironment
Volume 9 - 2021 | https://doi.org/10.3389/feart.2021.640172
This article is part of the Research Topic Late Quaternary Hydrological, Paleoenvironmental and Geomorphological Processes in the Tibetan Plateau and Its Adjacent Areas View all 21 articles
There are more than 1,000 lakes within the Tibetan Plateau (TP), all of which are sensitive to changes in regional climate and local hydrology. Lacustrine sediments within these lakes preserve a good record of these changes. However, determining their precise ages is difficult due to the complex nature of lake reservoir effects (LRE), which limit our understanding of paleoenvironmental changes. Focusing on an exposed 600 cm thick lacustrine sediment profile located in western Zhari Namco, we used a combination of both radiocarbon and optically stimulated luminescence (OSL) dating methods in order to evaluate the carbon reservoirs of bulk organic matter (BOM) and aquatic plant remnants (APR), and to explore the age differences between 14C and OSL and their respective reliability. We demonstrated that (i) OSL ages were changed in stratigraphic order, and the OSL age just below the beach gravel layer was consistent with previously reported paleoshoreline ages; (ii) 14C ages were divergent between BOM and grass leaves; (iii) 14C ages of BOM were older than 14C ages of APR; and (iv) all 14C ages were older than OSL ages. This could be attributed to changing LRE in the past, causing the 14C ages to appear unstable during the deposition period. Although the 14C ages of terrestrial plant remnants (TPR) were not affected by LRE, an analyzed twig nonetheless returned a 14C age older than its respective layer’s OSL age, suggesting it may have been preserved on land prior to transportation into the lake. Our study suggests that OSL ages are more reliable than 14C ages with respect to Zhari Namco lacustrine sediments. We recommend caution when interpreting paleoenvironmental changes based on lacustrine sediment 14C ages alone.
The Tibetan Plateau (TP) is the largest and highest plateau on Earth, often regarded as the “Third Pole” due to both its high elevations and expansive c. 2.5 million km2 coverage (Yao et al., 2012; Liu et al., 2019). Of the more than 1,400 lakes (larger than 1 km2) distributed across the TP, c. 60% are within the endorheic TP (Zhang et al., 2020), and their lacustrine sediments preserve a wealth of information that helps researchers track past environmental and hydrological changes within the region (Hou et al., 2010; Long et al., 2014; Liu et al., 2018; Chongyi et al., 2018).
Radiocarbon (14C) dating is the most commonly used method for dating lacustrine sediments within the TP (Shen et al., 2005; Hou et al., 2010; Chen H. et al., 2019; Wang H. et al., 2019), with most age frameworks for borehole lacustrine sediments constructed using 14C ages (Hou et al., 2012). Terrestrial plant remnants (TPR), aquatic plant remnants (APR), and bulk organic matter (BOM) are typical materials for 14C dating of lacustrine sediments within the TP (Zhang et al., 2007). Although 14C dating is a mature dating technology with high precision, the harsh environmental conditions in the region limit overall biomass. This makes it difficult to find TPR in lacustrine sediments (Mischke et al., 2013). However, 14C ages taken from the BOM and APR of lacustrine sediments 14C ages are often influenced by the disequilibrium with atmospheric radiocarbon, thus causing the corresponding BOM and APR 14C ages to appear older than the 14C ages of contemporaneous TPR. This phenomenon is called the lake reservoir effect (LRE), which is commonly encountered during radiocarbon dating of lacustrine sediments within the TP. The accurate estimation of LRE is a crucial prerequisite for establishing reliable 14C age-depth relationships (Stuiver and Braziunas, 1993; Ascough et al., 2011). Hou et al. (2012) and Mischke et al. (2013) summarized several approaches for evaluating LRE within the TP. These methods include: 14C-based estimation of reservoir ages (RAs) using modern lacustrine sediments (Mischke et al., 2010), linear extrapolation of the 14C age-depth model to the sediment-water interface depth (Fontes et al., 1996; Shen et al., 2005), geochemical models for 14C reservoir correction (Wang et al., 2007; Yu et al., 2007), stratigraphic alignment (Liu et al., 2008), independent age determinations such as 210Pb and 137Cs dating (Zhu et al., 2008), optically stimulated luminescence (OSL) dating (Long et al., 2014; Wang Y. X. et al., 2019), U-series dating (Zhu et al., 2004), magnetic susceptibility comparison (Li et al., 2021), and varve counting (Zhou et al., 2011). In the above methods, OSL is unaffected by variations in LRE and lake hydrochemistry, and it is convenient for extracting dating materials (e.g., quartz and feldspar grains). Because of these advantages, OSL dating is frequently used for Quaternary sediments dating.
The OSL age of sediment directly reflects its time of burial, i.e., when it was last exposed to sunlight (Aitken, 1998). Recent studies have successfully applied the OSL dating method to TP loess (Liu et al., 2012, 2017; Wang et al., 2018; Kang et al., 2020; Li et al., 2020), dunes (Chen T. Y. et al., 2019), sand wedges (Liu et al., 2010; Liu and Lai, 2013), river sediments (An et al., 2018) and lacustrine sediments (Liu et al., 2011, 2015) in order to reveal their respective accumulation histories. Moreover, many recent studies have used a combination of 14C and OSL dating to discuss the reliability of ages from lacustrine sediment cores. Zhang et al. (2007) used OSL and 14C dating in organic-rich lacustrine sediments cored from Lake Gucheng (Jiangsu, China), and found the 14C ages of BOM were c. 2,000 years (a) older than the OSL ages. Shen et al. (2008) applied OSL dating using fine silt quartz, together with 14C dating of terrestrial plant macrofossils, which should not have been affected by the LRE, to establish a chronology for a lacustrine sediment core from Crummock Water (in the northwestern part of the English Lake District). Long et al. (2011) used OSL and 14C dating of lacustrine sediments from Qingtu Lake (northern China); the apparent agreement between OSL and 14C dating suggested that the RAs of 14C samples in Qingtu Lake were much lower than in other lakes of northern China (e.g., Bangong Lake: 6,670 a, Fontes et al., 1996, Qinghai Lake: 1,039 a, Shen et al., 2005, Ahung Lake: 600–700 a, Morrill et al., 2006, Bositeng Lake: 1,140 a, Chen et al., 2006). However, only few studies of lacustrine sediments from the TP have used multi-dating approaches to establish age-depth models (Hu et al., 2017). Hu et al. (2017) used a combination of 210Pb, 14C, and OSL dating in Linggo Co (central TP) and found that the 210Pb and OSL ages were roughly concordant, and all 14C ages were much older than the 210Pb and OSL ages at the same depths.
Therefore, a comparison between OSL and 14C ages may help assess the reliability of the established chronology. In this study, 14C ages sampled from different materials were compared with independently obtained OSL ages from Zhari Namco with the aim of determining their respective accuracy and reliability and to gain a better understanding of the intrinsic variability of the LRE.
Zhari Namco (30°44′–31°05′N, 85°20′–85°54′E) is a brackish lake located in the Cuoqin Basin, situated in the southern TP and possessing a cold and arid climate (Figure 1). The lake is formed by an east-west extending structural fault and is irregular in shape with narrow northern and southern banks, whereas its eastern and western banks are relatively wide. According to GPS measurements in the field, Zhari Namco sits at an elevation of 4,613 m above sea level (a.s.l.). With an overall length of 54.3 km from east to west, a length of 26.2 km from north to south, a present surface area of 996.9 km2, and a catchment area of c. 15,433.2 km2, it is the third-largest lake in Tibet after Siling Co and Namco. It is supplied mainly by precipitation and meltwater from snow and ice, with the Cuoqin Zangbo River—which drains into the lake from the south—representing the major water input. This river originates at the Gangdisê Mountains (c. 6,000 m a.s.l.) and has a total length of 253 km and a catchment area of about c. 9,930 km2 (Wang and Dou, 1998).
Figure 1. (a) Location of Zhari Namco Lake within the Tibetan Plateau. (b) The position of section CQ (white circle) investigated in this study.
An exposed section, named CQ (31°0′56.086″N, 85°8′51.238″E, elevation 4,664 m a.s.l.), was discovered in Cuoqin County, which is located on the west side of the lake. Section CQ (Figure 2) is composed of subsection CQ1 (Figure 2A) and CQ2 (Figure 2B). CQ1 is located at the top and is 400 cm thick. CQ2 is 200 cm thick and connects with the bottom of CQ1. The surface section of CQ is comprised mainly of modern soil and some gravels (0–5 cm). Moving up the section, the sediments change from fine to coarse, with the color undergoing an attendant change from dark to light. CQ can roughly be divided into five stratigraphic units: In the bottommost part (600–230 cm depth), the sediments are unstratified silty clay and fine sands with dark black coloration and exuding a strong smell of organic decay, indicating continuous sedimentation within a deep and stable lake environment (Figure 2a). In the second part (230–150 cm depth), the sediments change from blackish to grayish medium-fine sands (Figure 2b). The third part (150–130 cm depth) is comprised of a layer of well-sorted, yellowish to grayish, medium-fine sands, indicating they were deposited at or near the lake shoreline (Figure 2c). The sediments of the fourth part (130–70 cm depth) consist of coarse sands and pebbles, which are round or dish-shaped with a long axis of 0.5 cm to 3 cm, indicative of a high-energy shoreline deposit (Figure 2d). Within the uppermost part (70–0 cm depth) the sediment is characterized by slope wash and colluvial deposits composed of soils and angular rock pieces (Figure 2C).
Figure 2. Profile of section CQ. (A) section CQ1, (B) section CQ2, and (C) overall profile of section CQ. (a–d) are details of section CQ.
Samples for OSL dating were collected from both CQ subsections. The vertical sections were first cleaned and polished to ensure access to freshly exposed sediments. Stainless steel tubes (6 cm in diameter and 30 cm in length) were then inserted into the profile to obtain the samples. Once extracted from the profile, both the ends of the tube were immediately sealed by cotton and wrapped with dark-colored tape before being sealed in a black plastic bag in order to avoid light exposure and water loss. A total of five OSL samples were collected from section CQ (Figure 2). Additionally, radiocarbon dating samples were collected from the middle-lower parts of the profile. They included eight aquatic plant leaves along with a terrestrial plant twig (Figure 3), as well as three BOM samples.
Figure 3. Photos of radiocarbon dating samples. (a–d) Are aquatic plant remnants, (e) is terrestrial plant remnants.
We selected quartz as the dating material due to the ease with which it can be bleached compared to feldspar. All OSL sample preparation and luminescence measurements were conducted under subdued red light in the Luminescence Dating Laboratory at the Qinghai Institute of Salt Lakes, Chinese Academy of Sciences. Then the outer 3 cm of sediment at the end of each steel tube were scraped away and reserved for measurements of water content and environmental dose rate. The unexposed middle section of the tube was used for equivalent dose (De) determination. The five raw samples were first treated with 10% HCl and 30% H2O2 to remove carbonates and organic materials, followed by wet sieving to obtain three fractions: grains smaller than 38 μm, middle-grained quartz (MG, 38–63 μm), and coarse-grained quartz (CG, 90–125 μm). All grains smaller than 38 μm were treated in glass cylinders using Stokes’ law in order to obtain fine-grained quartz (FG, 4–11 μm). The MG and FG were treated with silica saturated fluorosilicic acid (H2SiF6) for about 2 weeks, whereas the CG was etched with 40% HF for 60 min. All three fractions were then treated with 10% HCl to remove any fluorides, followed by the use of a magnet to remove any magnetic minerals. The purity of the isolated quartz was tested via infrared (IR) stimulation, with samples that showed obvious infrared stimulated luminescence (IRSL) signals retreated with H2SiF6 or HF again until no obvious IRSL was observed in any sample. We also ensured that all feldspar was removed in order to protect against age underestimation (Duller, 2003; Roberts, 2007; Lai and Brückner, 2008). Finally, we chose sufficient fractions by the content of different grain sizes for De measurements (Table 1).
The quartz was mounted as a mono-layer on the central part (0.65 cm diameter) of a stainless-steel disc (0.97 cm diameter) using silicone oil. The OSL signal was measured using an automated Risø TL/OSL-DA-20 reader. Laboratory irradiation was carried out using 90Sr/90Y sources mounted within the reader, with a dose rate of 0.086 Gy/s. The OSL signal was obtained after passage through a U-340 filter, while the IRSL signal was detected using a photomultiplier tube with the IRSL passing through BG-39 and coring-759 filters.
The environmental dose rate was calculated by measuring the radioactive element concentrations of surrounding sediments with a small contribution from cosmic rays. For all samples, U and Th concentrations and K contents were determined using inductively coupled plasma mass spectrometry (ICP-MS) analysis at the Institute of Earth Environment, Chinese Academy of Sciences in Xian. The cosmic ray dose was estimated for each sample as a function of depth, elevation, and geomagnetic latitude (Prescott and Hutton, 1994). The water content was calculated from sample weights measured before and after drying in an oven. The measured water contents of three deep lake samples CQ1-91, CQ2-26, and CQ2-60 were 43.8, 48.1, and 61.8%, respectively, and for the two shoreline samples CQ1-A and CQ1-B, the measured water contents were 20.5 and 19.7%, respectively. Due to water content variability during the burial period and based on the measured water content, approximations of measured water content were used to calculate OSL ages; an error of 5% of the measured water content was applied given the uncertainty of water changes in the lake sediments after burial (Table 1). The α-value for fine-grained quartz and middle-grained quartz was taken as 0.035 ± 0.003 (Lai et al., 2008). Radionuclide concentrations, water content, and dose rates are summarized in Table 1. Lastly, we converted the elemental concentrations into annual dose rates according to Aitken (1998).
The equivalent dose for each sample was measured using the single aliquot regenerative dose (SAR) protocol (Murray and Wintle, 2000). For each sample, at least 65 aliquots were measured by SAR. The OSL signal was calculated using the integral of the initial 0.64 s of the OSL signals minus the last 8 s. The characteristics of the quartz luminescence growth and decay curves for sample CQ1-A and CQ2–60 are shown in Supplementary Figure 1. It is important to investigate the influence of preheating on the charge transfer from light-insensitive traps to light-sensitive ones, a process called thermal transfer (Aitken, 1998). To select the appropriate preheat conditions for De determination using the SAR protocol, a preheat temperature plateau test was conducted for samples CQ1-A and CQ1-B. Preheat temperatures from 140 to 240°C at 20°C intervals for 10 s were tested and the cut-heat was 140°C for 10 s, using a heating rate of 5°C/s. As a plateau was observed from 160 to 200°C, we therefore selected a preheat temperature of 200°C for routine De determination (Supplementary Figure 2). The suitability of the SAR procedure for De determination was further checked with a “dose recovery test” (Murray and Wintle, 2003). The dose recovery test was performed on sample CQ1-A. Six natural aliquots were stimulated for c. 56 h under direct sunlight. The aliquots were then given a laboratory dose of 9.2 Gy, close to the natural De (9.19 Gy). The measured De was 9.4 ± 1.8 Gy. Thus, the ratio of the measured to the given De was c. 1.02, suggesting that the SAR conditions were appropriate for De determination.
For OSL age calculation, because the sediments in nearby lakes within the TP did not experience long-distance transport or multiple sedimentation-transportation cycles, samples drawn from these lakes may be partially bleached, and the stimulated signal intensities will be low when compared with sediments from other regions, such as the Chinese Loess Plateau (Lee et al., 2009). To reduce this impact, CQ1–90 removed one test aliquot with abnormal data, CQ2–60 and CQ2–26 removed two test aliquots with abnormal data. Because each sample had at least 65 aliquots, the influence of removed data on the results could be ignored. The abnormal data did not occur in CQ1-A and CQ1-B. Supplementary Table 1 shows the raw data for Des. Moreover, Arnold et al. (2007) proposed the single-aliquot age selection model to determine whether the 3-parameter minimum-age model (MAM-3) or the central-age model (CAM) was applied to calculate the Des. We used the R-language-based luminescence data analysis package “numOSL” to select the suitable age model (Peng et al., 2013), with all the samples’ Des ultimately determined using MAM-3 (Table 1).
Radiocarbon dating of 12 samples took place at Beta Analytic Inc. (Miami, FL, United States). Eight samples were APR, three samples were BOM, and the final sample was a terrestrial plant twig. Three samples (BOM) were simply treated with acid washes to remove any carbonates, whereas the nine plant samples were pre-treated with acid/alkali/acid to remove carbonates and mobile humic acids. They were then subjected to accelerator mass spectrometry (AMS) to measure their 14C ratios. The calibration of 14C dates was performed using the “CALIB 8.1.0” program (Stuiver et al., 2021) and the IntCal20 calibration curve (Reimer et al., 2020). The 13C/12C ratios (δ13C, ‰) of all dating materials were measured using an isotope ratio mass spectrometer (IRMS). All 14C ages from section CQ are summarized in Table 2. Radiocarbon ages and OSL ages are shown together against the depth scale in Figure 4.
Figure 4. Stratigraphy of the lacustrine sediment sequences and the ages of section CQ discussed in the text. The three shaded areas are the age-depth models of lacustrine sediment. The yellow shaded area is the OSL age-depth model, and the green and gray shaded areas are the age-depths of aquatic plant remnants and bulk organic matter, respectively. Three dotted lines are the median ages. Because there was only one 14C age of TPR, we did not use it to build the age-depth model.
Our results showed that the ages for all five OSL samples increased with respect to their depth within the section, indicating that these lacustrine sediments were deposited between 5 and 2 ka BP (Table 2). The uppermost sample CQ1-A, just below the paleo-shoreline deposits, returned an OSL age of 2.1 ± 0.2 ka BP. Accordingly, the oldest OSL age was 5.1 ± 0.4 ka BP, which was from the sample collected from the bottom of section CQ (Figure 2 and Table 2). The ages of the three mid-depth samples collected from 220, 375, and 445 cm were 2.0 ± 0.2 ka BP, 3.1 ± 0.3 ka BP, and 4.2 ± 0.5 ka BP, respectively (Table 2). We suggest that the lake level was higher than section CQ (4,664 m) during the interval from 5.1 to 3.1 ka BP on account of the lacustrine nature of the sediments, and because the paleo-shoreline deposits within the upper part of the section were deposited at c. 2 ka BP—the lake has further regressed since then. Our speculation is consistent with previous research indicating that the lake level of Zhari Namco declined by 42.5 m between 3.9 and 2.0 ka BP (from 4,680.6 to 4,637.9 m) (Chen et al., 2013).
During the deposition process, the OSL signal bleaching degree is the key factor affecting the accuracy of De determination in lacustrine sediments. In different minerals, quartz is more easily bleached than feldspar (Long et al., 2010). According to the OSL dating results from other lacustrine sediments within the TP (Long et al., 2012), the OSL signal of lacustrine sediments was well bleached during the deposition. In our study, the OSL signal quickly decreased to background levels within the first 2 s (Supplementary Figure 1), indicating that it was dominated by the fast component (Singarayer and Bailey, 2003). It also suggested that the quartz in our samples was relatively bright, which should have bleached easily when the grains were exposed to sunlight. Even so, our samples showed broad De distributions that may have been heterogeneously bleached before deposition (Supplementary Figure 3). To mitigate the influence of partial bleaching on age estimation, we used MAM-3 to calculate Des. Moreover, in De determination using the SAR protocol, inaccuracies may have been caused by improper heating conditions. However, dose recovery tests and preheat temperature plateau tests showed that Des was measured correctly for all samples from the CQ section using the SAR protocol (Aitken, 1998; Murray and Wintle, 2003). Therefore, we concluded that the OSL dating correctly measured and calculated De in this study.
The dose rate is determined by the content of the radionuclides in the sediments. It is usually assumed the dose rate of a sample is constant during the burial period in OSL dating (Zhang et al., 2007). Cupper (2006) measured sediment radioactivity from three salt lakes within southeastern Australia, finding it was in secular equilibrium from one of the salt lakes. Sediment radioactivity was in disequilibrium in the other two salt lakes, but this had a minimal effect on the dose rate. As shown in Table 1, the radionuclides contents of our samples were roughly constant, similar to other lacustrine sediments within the TP (Long et al., 2014; Hu et al., 2017). This suggests that radionuclides disequilibrium was likely to be absent in our samples (Long et al., 2015).
Water content is also the main factor affecting dose rate except for radionuclides. The water molecules in the sediments will absorb some radiation energy from the environment, reducing the absorption of the radiation energy by the sediments themselves (Zhang et al., 2007). In the field, we found that sediments in the profile were well preserved in a permanently frozen condition (the elevation of the site was c. 4,664 m), the water content was stable throughout their burial stage, and no evidence of a disturbance was observed from the structural features of the profile. Therefore, the measured water content at present could represent the real water content of sediments from section CQ during the burial period.
The twig, with a depth of 258 cm (CQ1–61), had a 14C age of 4,189–4,417 Cal a BP. The aquatic plant leaf, although coming from a similar depth of 267 cm (CQ1–64), returned a 14C age of 5,315–5,466 Cal a BP. Moreover, at depths of 339, 400, and 525 cm, the 14C ages of these aquatic plant leaf samples were 5,051–5,309, 5,046–5,301, and 5,766–5,994 Cal a BP, respectively. However, at a similar depth, the 14C ages of BOM were 7,011–7,253, 7,431–7,569, and 7,177–7,421 Cal a BP. The rest of aquatic plant leaves were sampled at depths of 178, 184, 375, and 475 cm, with reported 14C ages of 4,849–5,027, 5,052–5,313, 5,324–5,575, and 5,475-5,588 Cal a BP, respectively.
In general, the 14C ages of TPR are not affected by LRE, and their ages are often considered to be consistent with those of the sedimentary horizons in which they are found (Hou et al., 2012). However, the 14C age of the terrestrial twig (CQ1–61, 4,372 a) was roughly 2.3 ka older than the OSL sample (CQ1-B, 2.0 ± 0.2 ka BP) taken at a similar stratigraphic depth in section CQ. It is worth noting, however, that this discrepancy could possibly be explained due to a delay between the death of the twig and its ultimate transportation to, and burial within, the lacustrine sediments of the lake (Chen et al., 2017).
As shown in Table 2 and Figure 4, the 14C ages of APR were older than the OSL ages at similar depths, an inconsistency that was possibly due to the influence of LRE. This line of reasoning is supported, firstly, by the massive marine carbonate rocks which are widely distributed throughout the Coqen Basin (Zhong et al., 2010). These rocks have been eroded by precipitation runoff recharge and glacial meltwater from the catchment area, which enters the lake as dissolved inorganic carbon (DIC). In addition to DIC, dissolved organic carbon (DOC) and particulate organic carbon (POC) enters the lake by various means (Chen H. et al., 2019; Meng et al., 2020), with some underground brine water also infiltrating the lake via underlying faults (Fontes et al., 1996). Secondly, Zhari Namco is centrally located within the TP, which is one of the coldest and most arid regions. Extensive evaporation has resulted in a high concentration of carbon ions in the water, with the lake struggling to maintain equilibrium with atmospheric carbon (Zhou et al., 2020). Aquatic plants are therefore absorbing greater quantities of old or dead carbon (Olsson, 2009; Meng et al., 2020), possibly leading to inflated age estimates.
The 14C ages for BOM were consistently older than that of OSL and 14C ages for APR of similar depth (Figure 4). However, determining the precise LRE to explain this discrepancy was complicated by the complex nature of BOM. Firstly, in addition to the carbon disequilibrium between water and atmosphere, as well as the abundance of old and dead carbon from the catchment area, microalgae and bacteria which lived in Zhari Namco represent yet another source of LRE affecting BOM (Meyers and Ishiwatari, 1993; Li et al., 2015; Chen H. et al., 2019). Secondly, bulk masses of terrestrial organic matter and external POC can be directly deposited without dissolution in lacustrine sediments, which can also produce inflated 14C age estimates for BOM (Meng et al., 2020). Moreover, HCL washing, which is typically used to remove carbonate during the preparation of BOM samples, sometimes has the unintended corollary of mixing carbonate remnants into the organic material, which can also make the age of the sample appear older than it actually is (Harkness, 1975; Gilet-Blein et al., 1980). However, because all 14C samples were pretreated and dated in Beta’s laboratory, we did not know the exact details for this performance, hence this factor could not easily be estimated in this study. In conclusion, the 14C ages of BOM from Zhari Namco were also clearly subject to a host of LRE, and caution should be taken before drawing inferences from 14C ages based on this material.
Although the error margins for radiocarbon dating are lower compared with luminescence dating, and TPR are generally exempt from the influence of LRE (Hou et al., 2012), TPR are nonetheless rare in the lacustrine sediments of the TP due to low vegetation cover. Additionally, as terrestrial plants typically grow on the land surrounding the lake, they are not necessarily deposited directly into the lake upon death. This means that the timing of both their death and their retention on land remains uncertain (Chen et al., 2017), further illustrating the caution required when using the 14C ages of TPR. Most 14C ages from loess sediments (Wang et al., 2014; Song et al., 2015; Song et al., 2017; Cheng et al., 2019) have underestimated ages. In contrast, due to the effects of LRE, the 14C ages of APR and BOM are overestimated in Zhari Namco. This phenomenon has also been observed in QingHai Lake (Meng et al., 2020), Tangra Yumco (Long et al., 2014), and Linggo Co (Hu et al., 2017), all of which contain BOM with 14C ages older than that of stratigraphically associated aquatic plant material, and all 14C ages of APR and BOM appearing older than its actual age.
OSL dating is not affected by the LRE of lacustrine sediments. The equivalent dose and dose rate used in the calculation of OSL age in this study were reliable. The OSL ages were in stratigraphic sequence order, with no age reversals, and we used MAM-3 to mitigate the influence of partial bleaching on age estimation and to make sure that any overestimation of the equivalent dose had less impact on OSL ages (Peng et al., 2013; Shi et al., 2017). An age-depth model for the CQ section’s lacustrine sediments was established using the Bacon age-depth modeling package in “R” software (Blaauw and Christen, 2011) for five OSL ages, eight 14C ages of APR, and three 14C ages of BOM. As shown in Figure 4, age-depth model for OSL calculations found that for the 14C ages of APR and 14C ages of BOM, the average sedimentation rates of section CQ section c. 1.06 mm⋅a–1, c. 5.03 mm⋅a–1, c. 19.8 mm⋅a–1. The average sedimentation rate was c. 1.03 mm⋅a–1 within Linggo Co (Hu et al., 2017), which was also similar to the average sedimentation rate found by using OSL age from section CQ. Due to this convergence in multiple lines of evidence, we are confident of the general reliability of OSL ages in section CQ.
The chronology framework for a series of paleo-shorelines and section CQ in Zhari Namco showed that lake level dropped by 128 m since c. 8.2 ka BP (Chen et al., 2013) (Figure 5). Notably, there was a “dog-leg” change in Figure 5. Based on the OSL ages and the sedimentary facies of section CQ, the sediments from the bottom experienced continuous sedimentation within a deep and stable lake environment. As depth decreased, sediments changed to a high-energy shoreline deposition (Figure 4). This meant that the lake level of Zhari Namco dropped rapidly between c. 5.1 ka BP and c. 2.0 ka BP, which was also consistent with a similarly rapid decline in the levels of Seling Co Lake and Zabuye Lake—reported as occurring during the late Holocene (Shi et al., 2017; Jonell et al., 2020). Therefore, we suggested that the rapidly dropping lake level caused the “dog-leg” change. Another possible cause might be that section CQ was located at the mountainous area far from the lakefront area, with undulating landforms and significant elevation change.
Figure 5. OSL ages plotted against elevation for the 10 paleo-shorelines sediments of Zhari Namco. Blue circles are the ages of each shoreline from Chen et al. (2013). Yellow circles are the OSL ages of the CQ section.
The major reason behind the dropping lake level may have been the weakening Indian summer monsoon, which caused the climate in Zhari Namco to change from relatively warm-humid to cold-arid during this period (Chen et al., 2013). Because of this, the recharge of the catchment area into the lake also underwent a gradual decrease during this period. Moreover, it was clear that the RAs were not constant, neither in APR nor in BOM (Figure 6). This was probably related to the drop in lake level. From stratigraphy and age fluctuation, during c. 5.1 ka BP to c. 2 ka BP, Zhari Namco experienced a drop in lake level. With this decrease, the RAs of APR increased from c. 0.1 ka to c. 4.1 ka (6–0.7 m core depth), and the RAs of BOM increased from c. 1.7 ka to c. 6.5 ka (6–0.7 m core depth). We suggest that the major contributor to LRE variation in Zhari Namco is salinity. Salinity, in addition to being associated with climate-driven fluctuations in lake capacity and level (Fontes et al., 1996; Wu et al., 2007), has been tightly influenced by changes in carbon ion concentration (Xu et al., 2006; Lei et al., 2010; Li and Liu, 2012, 2014; Li et al., 2015; Batanero et al., 2017; Zhou et al., 2020), causing RAs to increase or decrease in different periods. During warm-humid periods and the attendant increase in lake capacity and water level, lake desalination would occur, which, accompanied by a decrease in carbon ion concentration (Yu et al., 2016), caused a concomitant decrease in RAs. However, the opposite situation occurred during cold-arid periods, which caused RAs to increase. Similar results were found in Qinghai Lake: Chongyi et al. (2018) suggested that the highest RAs may have occurred when the lake had its highest salinity and lowest water levels. In addition, the RAs of BOM were greater than APR, possibly due to the increased complexity of the LRE sources acting on it (Gilet-Blein et al., 1980). Either way, because of the noted influence of LRE, the 14C age estimates of APR and BOM cannot be considered to be wholly accurate.
Figure 6. The two shaded areas are the RAs-depth models. The green shaded area is the RAs-depth model for aquatic plant remnants, the gray shaded area is the RAs-depth of bulk organic matter. The two dotted lines represent the RAs median values.
In this study, radiocarbon and OSL dating were used to date lacustrine sediments from Zhari Namco Lake in the southern Tibetan Plateau. Through the comparison and analysis of 14C and OSL ages, we found that the OSL dating can produce reliable ages and has good potential for lacustrine sediments dating. By contrast, even though 14C dating is a mature dating method with high accuracy for dating lacustrine sediments, 14C ages of both aquatic plants remnants and BOM are negatively impacted by LRE, which can lead to apparent 14C ages being older than their actual ages. During warm-humid periods/cold-arid periods, RAs decrease/increase in response to higher/lower water levels, indicating that fluctuating that RA values are clearly associated with variations in the overarching hydrological regime. Due to the multitude of factors affecting LRE, it can be difficult to obtain reliable ages from APR and BOM, especially as the timing of both the death and ultimate deposition of terrestrial plants is often impossible to establish. In summary, OSL ages were more reliable than 14C ages in this study. Accordingly, we suggest that radiocarbon dating alone may be inappropriate for dating lacustrine sediments and that OSL dating could be applied when evaluating the reliability of 14C ages. Only by exploring areas of agreement and disagreement between multiple dating methods can we work toward building an accurate chronological framework for lacustrine sediments within the TP.
The original contributions presented in the study are included in the article/Supplementary Material, further inquiries can be directed to the corresponding author/s.
LC designed the research. LC, YW, XZ, TC, DG, and FA performed the research. LC and YW analyzed the data and wrote the manuscript. All authors contributed to the article and approved the submitted version.
This work was funded by the Second Tibetan Plateau Scientific Expedition and Research Program (2019QZKK0202) and the National Natural Science Foundation of China (41671006) to X.-J. Liu, the Science and Technology foundation Platform Project of Qinghai Province (2018-ZJ-T10), and the Science and Technology program of Qinghai Province (2018-ZJ-723).
The authors declare that the research was conducted in the absence of any commercial or financial relationships that could be construed as a potential conflict of interest.
We thank two reviewers for their valuable suggestions and comments on the manuscript. Furthermore, we thank X.-J. Liu who firstly designed and guided the performance of this study; Aijun Sun and Jun Zhou for their help in the field; and Guoqiang Li, Jun Peng, and He Yang for help with analysis of the data.
The Supplementary Material for this article can be found online at: https://www.frontiersin.org/articles/10.3389/feart.2021.640172/full#supplementary-material
Supplementary Figure 1 | Characteristics of quartz luminescence growth curves and decay curves of samples CQ1-A and CQ2–60.
Supplementary Figure 2 | Preheat plateau test results for 90–125 μm quartz in samples CQ1-A and CQ1-B.
Supplementary Figure 3 | Radial plots showing distributions of equivalent dose (De) and uncertainties of the OSL samples.
Supplementary Table 1 | The raw data for equivalent dose (De) determination; the red numbers indicate abnormal data, which were removed before further analysis.
An, F. Y., Liu, X. J., Zhang, Q. X., Wang, Y. X., Chen, T. Y., Yu, L. P., et al. (2018). Drainage geomorphic evolution in response to paleoclimatic changes since 12.8 ka in the eastern Kunlun Mountains, NE Qinghai-Tibetan Plateau. Geomorphology 319, 117–132. doi: 10.1016/j.geomorph.2018.07.016
Arnold, L. J., Bailey, R. M., and Tucker, G. E. (2007). Statistical treatment of fluvial dose distributions from southern Colorado arroyo deposits. Quat. Geochronol. 2, 162–167. doi: 10.1016/j.quageo.2006.05.003
Ascough, P., Cook, G. T., Kinch, H., Dunbar, E., Church, M. J., Einarsson, A., et al. (2011). An Icelandic fresh-water radiocarbon reservoir effect: implications for lacustrine 14C chronologies. Holocene 21, 1073–1080. doi: 10.1177/0959683611400466
Batanero, G. L., León-Palmero, E., Li, L. L., Green, A. G., Rendón-Martos, M., Suttle, C. A., et al. (2017). Flamingos and drought as drivers of nutrients and microbial dynamics in a saline lake. Sci. Rep. 7:12173. doi: 10.1038/s41598-017-12462-9
Blaauw, M., and Christen, J. (2011). Flexible paleoclimate age-depth models using an autoregressive gamma process. Bayesian Anal. 6, 457–474. doi: 10.1214/ba/1339616472
Chen, F. H., Huang, X. Z., Zhang, J. W., Holmes, J. A., and Chen, J. H. (2006). Humid Little Ice age in arid central Asia documented by Bosten Lake, Xinjiang, China. Sci. China Ser. D Earth Sci. 49, 1280–1290.
Chen, H., Zhu, L. P., Ju, J. T., Wang, J. B., and Ma, Q. F. (2019). Temporal variability of 14C reservoir effects and sedimentological chronology analysis in lake sediments from Chibuzhang Co, North Tibet (China). Quat. Geochronol. 52, 88–102. doi: 10.1016/j.quageo.2019.02.009
Chen, T. Y., Lai, Z. P., Liu, S. W., Wang, Y. X., Wang, Z. T., Miao, X. D., et al. (2019). Luminescence chronology and palaeoenvironmental significance of limnic relics from the Badain Jaran Desert, northern China. J. Asian Earth Sci. 177, 240–249. doi: 10.1016/j.jseaes.2019.03.024
Chen, T. Y., Liu, S. W., Lai, Z. P., Wang, Z. T., Han, F. Q., and Wang, Y. X. (2017). A preliminary study of AMS 14C dating of young lacustrine sediments in the Badain Jaran Desert. J. Salt Lake Res. 25, 60–66.
Chen, Y. W., Zhong, Y. Q., Li, B., Li, S. H., and Aitchison, J. C. (2013). Shrinking lakes in Tibet linked to the weakening Asian monsoon in the past 8.2ka. Quat. Res. 80, 189–198. doi: 10.1016/j.yqres.2013.06.008
Cheng, P., Burr, G., Zhou, W. J., Chen, N., Hou, Y. Y., Du, H., et al. (2019). The deficiency of organic matter 14C dating in Chinese Loess-paleosol sample. Quat. Geochronol. 56:101051. doi: 10.1016/j.quageo.2019.101051
Chongyi, E., Sun, Y. J., Liu, X. J., Hou, G. L., Lv, S. C., Yuan, J., et al. (2018). A comparative study of radiocarbon dating on terrestrial organisms and fish from Qinghai Lake in the northeastern Tibetan Plateau, China. Holocene 28, 1712–1719. doi: 10.1177/0959683618788671
Cupper, M. L. (2006). Luminescence and radiocarbon chronologies of playa sedimentation in the Murray Basin, Southeastern Australia. Quat. Sci. Rev. 25, 2594–2607. doi: 10.1016/j.quascirev.2005.09.011
Duller, G. A. T. (2003). Distinguishing quartz and feldspar in single grain luminescence measurements. Radiat. Meas. 37, 161–165. doi: 10.1016/S1350-4487(02)00170-1
Fontes, J. C., Gasse, F., and Gibert, E. (1996). Holocene environmental changes in Lake Bangong basin (Western Tibet). Part 1: chronology and stable isotopes of carbonates of a Holocene lacustrine core. Palaeogeogr. Palaeoclimatol. Palaeoecol. 120, 25–47. doi: 10.1016/0031-0182(95)00032-1
Gilet-Blein, N., Marien, G., and Evin, J. (1980). Unreliability of 14C dates from organicmatter of soils. Radiocarbon 22, 919–929. doi: 10.1017/S0033822200010328
Harkness, D. (1975). “The role of the archaeologist in C-14 measurement,” in Radiocarbon: Calibration and Prehistory, ed. T. Watkins (Edinburgh: Edinburgh University Press), 128–135.
Hou, J. Z., D’Andrea, W. J., and Liu, Z. H. (2012). The influence of 14C reservoir age on interpretation of paleolimnological records from the Tibetan Plateau. Quat. Sci. Rev. 48, 67–79. doi: 10.1016/j.quascirev.2012.06.008
Hou, J. Z., Huang, Y. S., Brodsky, C., Alexandre, M. R., Mcnichol, A. P., King, J. W., et al. (2010). Radiocarbon dating of individual lignin phenols: a new approach for establishing chronology of late quaternary lake sediments. Anal. Chem. 82, 7119–7126. doi: 10.1021/ac100494m
Hu, G., Yi, C. L., Zhang, J. F., Cao, G. R., Pan, B. L., Liu, J. H., et al. (2017). Chronology of a lacustrine core from Lake Linggo Co using a combination of OSL, 14C and 210Pb dating: implications for the dating of lacustrine sediments from the Tibetan Plateau. Boreas 47, 656–670. doi: 10.1111/bor.12291
Jonell, T. N., Aitchison, J. C., Li, G. Q., Shulmeister, J., Zhou, R. J., and Zhang, H. X. (2020). Revisiting growth and decline of late Quaternary mega-lakes across the south-central Tibetan Plateau. Quat. Sci. Rev. 248:106475. doi: 10.1016/j.quascirev.2020.106475
Kang, S. G., Wang, X. L., Roberts, M. H., Duller, G. A. T., Song, Y. G., Liu, W. G., et al. (2020). Increasing effective moisture during the Holocene in the semiarid regions of the Yili Basin, Central Asia: evidence from loess sections. Quat. Sci. Res. 246:106553. doi: 10.1016/j.quascirev.2020.106553
Lai, Z. P., and Brückner, H. (2008). Effects of feldspar contamination on equivalent dose and the shape of growth curve for OSL of silt-sized quartz extracted from Chinese loess. Geochronometria 30, 49–53. doi: 10.2478/v10003-008-0010-0
Lai, Z. P., Zöllerr, L., Fuchs, M., and Brückner, H. (2008). Alpha efficiency determination for OSL of quartz extracted from Chinese loess. Radiat. Meas. 43, 767–770. doi: 10.1016/j.radmeas.2008.01.022
Lee, J., Li, S. H., and Aitchison, J. C. (2009). OSL dating of paleoshorelines at Lagkor Tso, western Tibet. Quat. Geochronol. 4, 335–343. doi: 10.1016/j.quageo.2009.02.003
Lei, Y. B., Zhang, H. C., Li, S. J., Yang, L. Q., Yao, S. C., Li, C. H., et al. (2010). Variation of 13C value in authigenic carbonates from Zigetang Co, Tibetan Plateau since 1950 AD. J. Lake Sci. 1, 143–150. doi: 10.18307/2010.0120
Li, C. G., Zheng, Y., Wang, M. D., Sun, Z., and Jin, C. S. (2021). Refined dating using palaeomagnetic secular variations on a lake sediment core from Guozha Co, northwestern Tibetan Plateau. Quat. Geochronol. 62:101146. doi: 10.1016/j.quageo.2020.101146
Li, G. Q., Zhang, H. X., Liu, X. J., Yang, H., Wang, X. Y., Zhang, X. J., et al. (2020). Paleoclimatic changes and modulation of East Asian summer monsoon by high-latitude forcing over the last 130,000 years as revealed by independently dated loess-paleosol sequences on the NE Tibetan Plateau. Quat. Sci. Rev. 237:106283. doi: 10.1016/j.quascirev.2020.106283
Li, X. Z., and Liu, W. G. (2012). The stable oxygen isotopic composition of ostracoda Limnocythere inopinata Bird and its possible response to water salinity in Lake Qinghai. J. Lake Sci. 24, 623–628. doi: 10.18307/2012.0417
Li, X. Z., and Liu, W. G. (2014). Water salinity and productivity recorded by ostracod assemblages and their carbon isotopes since the early Holocene at Lake Qinghai on the northeastern Qinghai-Tibet Plateau, China. Palaeogeogr. Palaeoclimatol. Palaeoecol. 407, 25–33. doi: 10.1016/j.palaeo.2014.04.017
Li, X. Z., Liu, W. G., Zhou, X., Xu, L. M., and Cheng, P. (2015). A 700-year macrophyte productivity record inferred from isotopes of macrophyte remains and bulk carbonates in Lake Koucha, northeast Qinghai–Tibetan Plateau. Quat. Int. 430, 32–40. doi: 10.1016/j.quaint.2015.11.053
Liu, W. M., Hu, K. H., Carling, P. A., Lai, Z. P., Cheng, T., and Xu, Y. L. (2018). The establishment and influence of Baimakou paleo-dam in an upstream reach of the Yangtze River, southeastern margin of the Tibetan Plateau. Geomorphology 321, 167–173. doi: 10.1016/j.geomorph.2018.08.028
Liu, X. J., and Lai, Z. P. (2013). Optical dating of sand wedges and ice-wedge casts from Qinghai Lake area on the northeastern Qinghai-Tibetan Plateau and its palaeoenvironmental implications. Boreas 42, 333–341. doi: 10.1111/j.1502-3885.2012.00288.x
Liu, X. J., Lai, Z. P., Fan, Q. S., Long, H., and Sun, Y. J. (2010). Timing for high lake levels of Qinghai Lake in the Qinghai-Tibetan Plateau since the Last Interglaciation based on quartz OSL dating. Quat. Geochronol. 5, 218–222. doi: 10.1016/j.quageo.2009.03.010
Liu, X. J., Lai, Z. P., Madsen, D., Yu, L. P., Liu, K., and Zhang, J. R. (2011). Lake level variations of Qinghai Lake in northeastern Qinghai-Tibetan Plateau since 3.7 ka based on OSL dating. Quat. Int. 236, 57–64. doi: 10.1016/j.quaint.2010.08.009
Liu, X. J., Lai, Z. P., Madsen, D., and Zeng, F. M. (2015). Last Deglacial and Holocene lake level variations of Lake Qinghai, northeastern Qinghai-Tibetan Plateau. J. Quat. Sci. 30, 245–257. doi: 10.1002/jqs.2777
Liu, X. J., Lai, Z. P., Yu, L. P., Sun, Y. J., and Madsen, D. B. (2012). Luminescence chronology of aeolian deposits from the Qinghai Lake area in the Northeastern Qinghai-Tibetan Plateau and its palaeoenvironmental implications. Quat. Geochronol. 10, 37–43. doi: 10.1016/j.quageo.2012.01.016
Liu, X. J., Xiao, G. Q., Chongyi, E., Li, X. Z., Lai, Z. P., Yu, L. P., et al. (2017). Accumulation and erosion of aeolian sediments in the northeastern Qinghai-Tibetan Plateau and implications for provenance to the Chinese Loess Plateau. J. Asian Earth Sci. 135, 166–174. doi: 10.1016/j.jseaes.2016.12.034
Liu, X. J., Zhang, X. J., Lin, Y. L., Jin, L. Y., and Chen, F. H. (2019). Strengthened Indian summer monsoon brought more rainfall to the western Tibetan Plateau during the early Holocene. Sci. Bulletin. 64, 1482–1485. doi: 10.1016/j.scib.2019.07.022
Liu, X. Q., Dong, H. L., Rech, J. A., Matsumoto, R., Yang, B., and Wang, Y. B. (2008). Evolution of Chaka Salt Lake in NW China in response to climatic change during the Latest Pleistocene–Holocene. Quat. Sci. Rev. 27, 867–879. doi: 10.1016/j.quascirev.2007.12.006
Long, H., Haberzettl, T., Tsukamoto, S., Shen, J., Kasper, T., Daut, G., et al. (2014). Luminescence dating of lacustrine sediments from Tangra Yumco (southern Tibetan Plateau) using post-IR IRSL signals from polymineral grains. Boreas 44, 139–152. doi: 10.1111/bor.12096
Long, H., Lai, Z. P., Frenzel, P., Fuchs, M., and Haberzettl, T.(2012). Holocene moist period recorded by the chronostratigraphy of a lake sedimentary sequence from Lake Tangra Yumco on the south Tibetan Plateau. Quat. Geochronol. 10, 136–142. doi: 10.1016/j.quageo.2011.11.005
Long, H., Lai, Z. P., Wang, N. A., and Li, Y. (2010). Holocene climate variations from Zhuyeze terminal lake records in East Asian monsoon margin in arid northern China. Quat. Res. 74, 46–56. doi: 10.1016/j.yqres.2010.03.009
Long, H., Lai, Z. P., Wang, N. A., and Zhang, J. R. (2011). A combined luminescence and radiocarbon dating study of Holocene lacustrine sediments from arid northern China. Quat. Geochronol. 6, 1–9. doi: 10.1016/j.quageo.2010.06.001
Long, H., Shen, J., Wang, Y., Gao, L., and Frechen, M. (2015). High-resolution OSL dating of a late Quaternary sequence from Lake Xingkai (NE Asia): chronological challenge of the “MIS 3a Mega-palaeolake”hypothesis in China. Earth Planet. Sci. Lett. 428, 281–292. doi: 10.1016/j.epsl.2015.07.003
Meng, B. W., Zhou, A. F., Zhang, Y. C., Song, M., Liu, W. G., Xie, Z. Q., et al. (2020). Downcore variations of carbon reservoir ages linked to lake level changes in northwest China. Quat. Geochronol. 60, 101–105. doi: 10.1016/j.quageo.2020.101105
Meyers, P. A., and Ishiwatari, R. (1993). Lacustrine organic geochemistry: anoverview of indicators of organic matter sources and diagensis in lakesediments. Organ. Geochem. 20, 867–900. doi: 10.1016/0146-6380(93)90100-P
Mischke, S., Aichner, B., Diekmann, B., Herzschuh, U., Plessen, B., Wünnemann, B., et al. (2010). Ostracods and stable isotopes of a late glacial and Holocene lake record from the NE Tibetan Plateau. Chem. Geol. 276, 95–103. doi: 10.1016/j.chemgeo.2010.06.003
Mischke, S., Weynell, M., Zhang, C., and Wiechert, U. (2013). Spatial variability of 14C reservoir effects in Tibetan Plateau lakes. Quat. Int. 313-314, 147–155. doi: 10.1016/j.quaint.2013.01.030
Morrill, C., Overpeck, J. T., Cole, J. E., Liu, K., Shen, C., and Tang, L. (2006). Holocene variations in the Asian monsoon inferred from the geochemistry of lake sediments in central Tibet. Quat. Res. 65, 232–243. doi: 10.1016/j.yqres.2005.02.014
Murray, A. S., and Wintle, A. G. (2000). Luminescence dating of quartz using and improved single-aliquot regenerative protocol. Radiat. Meas. 32, 571–577. doi: 10.1016/S1350-4487(99)00253-X
Murray, A. S., and Wintle, A. G. (2003). The single aliquot regenerative dose protocol: potential for improvements in reliability. Radiat. Meas. 37, 377–381. doi: 10.1016/S1350-4487(03)00053-2
Olsson, I. U. (2009). Radiocarbon dating history: early days, questions, and problems met. Radiocarbon 51, 1–43. doi: 10.2458/azu_js_rc.51.3477
Peng, J., Dong, Z. B., Han, F. Q., Long, H., and Liu, X. J. (2013). R package numOSL: numeric routines for optically stimulated luminescence dating. Ancient TL 31, 41–48.
Prescott, J. R., and Hutton, J. T. (1994). Cosmic ray contributions to dose rates for luminescence and ESR dating: large depths and long-term time variations. Radiat. Meas. 23, 497–500. doi: 10.1016/1350-4487(94)90086-8
Reimer, P. J., Austin, W. E. N., Bard, E., Bayliss, A., Blackwell, P. G., Ramsey, C. B., et al. (2020). The IntCal20 Northern hemisphere radiocarbon age calibration curve (0–55 cal kBP). Radiocarbon 62, 725–757. doi: 10.1017/RDC.2020.41
Roberts, H. M. (2007). Assessing the effectiveness of the double-SAR protocol in isolating a luminescence signal dominated by quartz. Radiat. Meas. 42, 1627–1636. doi: 10.1016/j.radmeas.2007.09.010
Shen, J., Liu, X. Q., Wang, S. M., and Matsumoto, R. (2005). Palaeoclimatic changes in the Qinghai lake area during the last 18,000 years. Quat. Int 136, 131–140. doi: 10.1016/j.quaint.2004.11.014
Shen, Z. X., Bloemendal, J., Mauz, B., Chiverrell, R. C., Dearing, J. A., Lang, A., et al. (2008). Holocene environmental reconstruction of sediment-source linkages at Crummock Water, English Lake District, based on magnetic measurements. Holocene 18, 129–140. doi: 10.1177/0959683607085604
Shi, X. H., Kirby, E., Furlong, K. P., Meng, K., Robinson, R., Lu, H. J., et al. (2017). Rapid and punctuated Late Holocene recession of Siling Co, central Tibet. Quat. Sci. Rev. 172, 15–31. doi: 10.1016/j.quascirev.2017.07.017
Singarayer, J. S., and Bailey, R. M. (2003). Further investigations of the quartz optically stimulated luminescence components using linear modulation. Radiat. Meas. 37, 451–458. doi: 10.1016/S1350-4487(03)00062-3
Song, Y. G., Lai, Z. P., Li, Y., Chen, T., and Wang, Y. X. (2015). Comparison between luminescence and radiocarbon dating of late Quaternary loess from the Ili Basin in Central Asia. Quat. Geochronol. 30, 405–410. doi: 10.1016/j.quageo.2015.01.012
Song, Y. G., Luo, D., Du, J. H., Kang, S. G., Cheng, P., Fu, C. F., et al. (2017). Radiometric dating of late Quaternary loess in the northern piedmont of South Tianshan Mountains: implications for reliable dating. Geol. J. 53:8. doi: 10.1002/gj.3129
Stuiver, M., and Braziunas, T. F. (1993). Modeling atmospheric 14C influences and 14C ages of marine samples to 10,000 BC. Radiocarbon 35, 137–189. doi: 10.1017/S0033822200013874
Stuiver, M., Reimer, P. J., and Reimer, R. W. (2021). CALIB 8.1.0 [WWW program]. Available online at: http://calib.org (accessed 01 January 2021).
Wang, H., Cui, P., Liu, D. Z., Liu, W. M., Bazai, N. A., Wang, J., et al. (2019). Evolution of a landslide-dammed lake on the southeastern Tibetan Plateau and its influence on river longitudinal profiles. Geomorphology 343, 15–32. doi: 10.1016/j.geomorph.2019.06.023
Wang, Y., Shen, J., Wu, J., Liu, X. Q., Zhang, E. L., and Liu, E. F. (2007). Hard-water effect correction of lacustrine sediment ages using the relationship between 14C levels in lake waters and in the atmosphere: the case of Lake Qinghai. J. Lake Sci. 19, 504–508. doi: 10.18307/2007.0502
Wang, Y. X., Chen, T. Y., Chongyi, E., An, F. Y., Lai, Z. P., Zhao, L., et al. (2018). Quartz OSL and K-feldspar post-IR IRSL dating of loess in the Huangshui river valley, northeastern Tibetan plateau. Aeolian Res. 33, 23–32. doi: 10.1016/j.aeolia.2018.04.002
Wang, Y. X., Chen, T. Y., Wu, C., Lai, Z. P., Guo, S. D., and Cong, L. (2019). Formation and evolution of the Xitaijinair Salt Lake in Qaidam Basin revealed by chronology. Arid Land Geogr. 42, 876–884. doi: 10.12118/j.issn.1000-6060.2019.04.19
Wang, Z. L., Zhao, H., Dong, G. H., Zhou, A. F., Liu, J. B., and Zhang, D. J. (2014). Reliability of radiocarbon dating on various fractions of loess-soil sequence for Dadiwan section in the western Chinese Loess Plateau. Front. Earth Sci. 8, 540–560. doi: 10.1007/s11707-014-0431-1
Wu, Y. H., Wang, S. M., Zhou, L. P., and Sun, Z. B. (2007). Modern reservoir age for 14C dating in DaiHai Lake. Qua. Sci. 4, 507–510. doi: 10.3321/j.issn:1001-7410.2007.04.005
Xu, H., Ai, L., Tan, L. C., and An, Z. S. (2006). Stable isotopes in bulk carbonates and organic matter in recent sediments of Lake Qinghai and their climatic implications. Chem. Geol. 235, 262–275. doi: 10.1016/j.chemgeo.2006.07.005
Yao, T. D., Thompson, L., Yang, W., Yu, W. S., Gao, Y., Guo, X. J., et al. (2012). Different glacier status with atmospheric circulations in Tibetan Plateau and surroundings. Nat. Clim. Change 2, 663–667. doi: 10.1038/nclimate1580
Yu, J. J., Zheng, M. P., Wu, Q., Wang, Y. S., Nie, Z., and Bu, L. Z. (2016). Natural evaporation and crystallization of Bangor salt lake water in Tibet. Sci. Technol. Rev. 34, 60–66. doi: 10.3981/j.issn.1000-7857.2016.05.006
Yu, S. Y., Shen, J., and Colman, S. M. (2007). Modeling the radiocarbon reservoir effect in lacustrine systems. Radiocarbon 49, 1241–1254. doi: 10.2458/azu_js_rc.49.3016
Zhang, J. F., Zhou, L. P., Yao, S. C., Xue, B., and Wang, X. L. (2007). Radiocarbon and optical dating of lacustrine sediments—a case study in Lake Gucheng. Quat. Sci. 4, 522–528. doi: 10.1016/S1872-5791(07)60044-X
Zhang, S., Zhang, J. F., Zhao, H., Liu, X. J., and Chen, F. H. (2020). Spatiotemporal complexity of the “Greatest lake Period” in the Tibetan Plateau. Sci. Bull. 65:16. doi: 10.1016/j.scib.2020.05.004
Zhong, Q., Fang, H., Liu, C. W., Li, X. C., Lu, J. Q., and Gao, B. T. (2010). The Paleozoic carbonate rocks depression of Coqen basin: evidence from geophysics on the Qinghai-Tibet Plateau, China. Geol. Bull. China 29, 870–879. doi: 10.3969/j.issn.1671-2552.2010.06.009
Zhou, A. F., Chen, F. H., Wang, Z. L., Yang, M. L., Qiang, M. R., and Zhang, J. W. (2011). Temporal change of radiocarbon reservoir effect in Sugan Lake, Northwest China during the Late-Holocene. Radiocarbon 51, 529–535. doi: 10.2458/azu_js_rc.51.3514
Zhou, K. E., Xu, H., Lan, J. H., Tan, D. N., Shen, E. G., Yu, K. K., et al. (2020). Variable Late Holocene 14C Reservoir Ages in Lake Bosten, Northwestern China. Front. Earth Sci. 7:601503. doi: 10.3389/feart.2020.601503
Zhu, D. G., Meng, X. G., Zhao, X. T., Shao, Z. G., Xu, Z. F., Yang, C. B., et al. (2004). Evolution of ancient large Lake in the Southeast of the Northern Tibetan Plateau. Acta Geol. Sin. 78, 982–992. doi: 10.1111/j.1755-6724.2004.tb00220.x
Keywords: lake reservoir effect, lacustrine sediments, optically stimulated luminescence, radiocarbon dating, Tibetan Plateau
Citation: Cong L, Wang Y, Zhang X, Chen T, Gao D and An F (2021) Radiocarbon and Luminescence Dating of Lacustrine Sediments in Zhari Namco, Southern Tibetan Plateau. Front. Earth Sci. 9:640172. doi: 10.3389/feart.2021.640172
Received: 10 December 2020; Accepted: 20 April 2021;
Published: 28 May 2021.
Edited by:
Feng Cheng, University of Nevada, Reno, United StatesReviewed by:
Yougui Song, Institute of Earth Environment, Chinese Academy of Sciences, ChinaCopyright © 2021 Cong, Wang, Zhang, Chen, Gao and An. This is an open-access article distributed under the terms of the Creative Commons Attribution License (CC BY). The use, distribution or reproduction in other forums is permitted, provided the original author(s) and the copyright owner(s) are credited and that the original publication in this journal is cited, in accordance with accepted academic practice. No use, distribution or reproduction is permitted which does not comply with these terms.
*Correspondence: Yixuan Wang, eWl4dWFud2FuZ0Bpc2wuYWMuY24=
Disclaimer: All claims expressed in this article are solely those of the authors and do not necessarily represent those of their affiliated organizations, or those of the publisher, the editors and the reviewers. Any product that may be evaluated in this article or claim that may be made by its manufacturer is not guaranteed or endorsed by the publisher.
Research integrity at Frontiers
Learn more about the work of our research integrity team to safeguard the quality of each article we publish.