- 1Key Laboratory of Geoscience Big Data and Deep Resource of Zhejiang Province, School of Earth Sciences, Zhejiang University, Hangzhou, China
- 2Structural Research Center of Oil & Gas Bearing Basin of Ministry of Education, Hangzhou, China
- 3Exploration and Development Research Institute, Tarim Oilfield Company Ltd., Korla, China
- 4Department of Geology and Environmental Earth Science, Miami University, Oxford, OH, United States
We use stratigraphic, sedimentological, and borehole data and seismic profiles from the western Tarim Basin to document its Mesozoic tectonic evolution. A nearly 60-km-wide, Triassic fold-and-thrust belt along the southwestern margin of Tarim Basin is unconformably overlain by a Jurassic-Cretaceous sedimentary sequence along a regional angular unconformity. The Lower-Middle Jurassic strata consist mainly of an upward-fining sequence ranging from terrestrial conglomerates to turbidite deposits, which represent the products of an initial rift stage. Palaeocurrent analyses show that sediments for these rift deposits were derived from the paleo-Kunlun and paleo-Tienshan Mountains to the southwest and northern, respectively. The overlying Upper Jurassic-Cretaceous series consist of coarse-grained, alluvial fan to braided river deposits in the lower stratigraphic member, and lagoonal mudstones and marine carbonates in the upper member. These finer-grained rocks were deposited in a subsiding basin, indicating that a significant change and reorientation in the drainage system should have occurred within the basin during the Early Cretaceous. The western Tarim Basin evolved from a syn-rift stage to a post-rift stage during the Jurassic-Cretaceous. A post-orogenic stretch developed due to the evolution of the Paleo-Tethyan orogenic belt in Central Asia is a likely geodynamic mechanism for this major tectonic switch from a contractional episode in the Triassic to an extensional deformation phase in the Jurassic-Cretaceous.
Introduction
Central Asia comprises an intricate collage of many crustal blocks and discrete geological domains, such as high orogenic plateaus, mountain ranges and sedimentary basins, which are separated by crustal- or lithospheric-scale faults (Figure 1A). This geological collage was built up through progressive accretion of continental fragments and terranes into the south Asian margin throughout the Phanerozoic (Watson et al., 1987; Windley et al., 1990; Allen et al., 1993; Matte et al., 1996; Mattern and Schneider, 2000; Xiao et al., 2005, 2015). The India-Asia collision has shaped the diverse landscape of Central Asia in the Cenozoic, obliterating some of the prior structural relationships while also exhuming previously deeply buried tectonic units (De Grave et al., 2013; Li G.W. et al., 2019). The majority of research on the Central Asian geology has mainly focused on its Paleozoic amalgamation history (Windley et al., 2007; Charvet et al., 2011; Xiao et al., 2015; Han and Zhao, 2018) and the Cenozoic deformation processes related to the growth of the Tibetan Plateau (Molnar and Tapponnier, 1975; Jiang and Li, 2014; Blayney et al., 2016; Sun et al., 2016). Many well-developed Mesozoic sedimentary basins with stratal thicknesses exceeding 5 km attest to widespread tectonic processes during this interval (Hendrix et al., 1992; Graham et al., 1993). However, the definition and origin of these sedimentary basins and their evolution is a topic of debate in the current literature (e.g., Watson et al., 1987; Sobel, 1999; Ritts and Biffi, 2001; Li et al., 2004; Li and Peng, 2010; Yang Y.T. et al., 2017; Cheng F. et al., 2019).
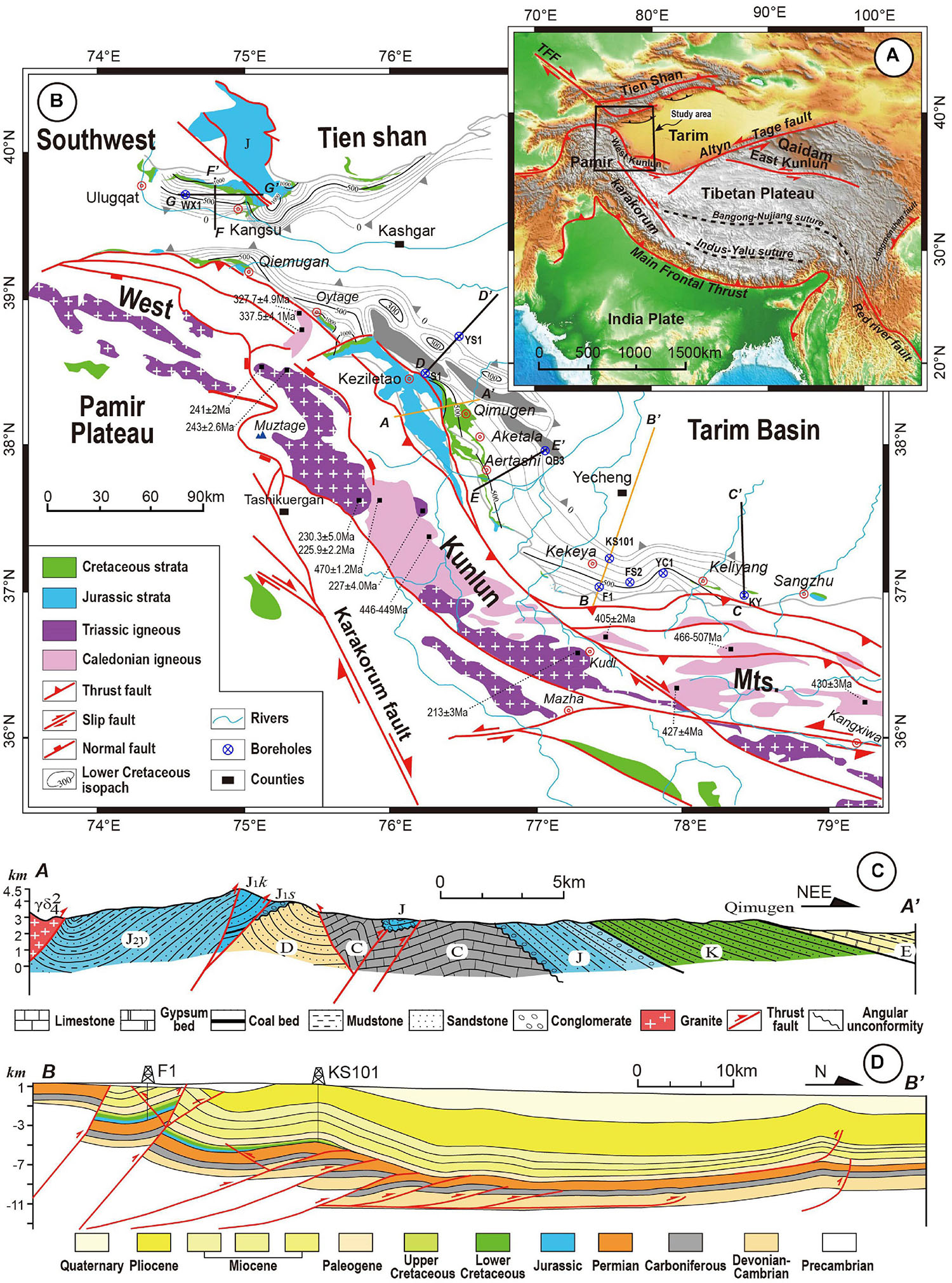
Figure 1. (A) Schematic tectonic map of Northwest China showing the major tectonic units separated by faults and sutures (B) Simplified geological map of the western Tarim Basin and adjacent mountain ranges, presenting distribution and thickness of Lower Cretaceous strata (modified after Cheng X.G. et al., 2019), Paleozoic-Mesozoic magmatic belts and major faults (modified after Cao et al., 2015). Zircon U-Pb geochronological data of the plutons in West Kunlun orogen are from Zhang et al. (2016) and reference therein. Five seismic profiles (C-C’ to G-G’) are shown with black line. (C) E-W trending geological section (A-A’) near Tamuhe river, and another (D) S-N trending geological section (B-B’) near Kekeya are presented below. Section (A-A’) and section (B-B’) show the unconformity between Mesozoic and Paleozoic and the uniform deformation of the Jurassic and Cretaceous formations.
The western Tarim Basin, located to the northern margin of the Paleo-Tethyan orogenic system, is an important geological archive of the long-term tectonism within Asia during the Mesozoic and Cenozoic (Sobel, 1995, 1999; Bershaw et al., 2012; Cao et al., 2015). It has been widely accepted that the early Cimmerian (Triassic) continental collision events produced extensive crustal deformation and magmatism within the Paleo-Tethyan orogenic belt (Figure 1B; Xiao et al., 2002; Zhang et al., 2016; and reference therein). Numerous studies have been carried out to document the petrology, geochemistry and thermochronology of various tectonic units involved in the Triassic collision (Li G.W. et al., 2019). However, tectonic processes of the subsequent post-collisional events have not been investigated in detail, largely due to the incomplete record of sedimentary and magmatic events in the mountain ranges (Sobel, 1999). Although the Jurassic-Cretaceous basinal strata with unusually large sediment thicknesses have been investigated since the 1990s (Hendrix et al., 1992; Graham et al., 1993; Sobel, 1995, 1999), their origin and geological history remain poorly constrained. Several competing models have been proposed (Wu, 2018). Some researchers have suggested successive development of foreland basins in Central Asia as a response to episodic subduction and collision events within the Tethyan domain to the south during this period (Hendrix et al., 1992; Graham et al., 1993; Sobel, 1999; Yang Y.T. et al., 2017). Sobel (1999) argued for an Early-Middle Jurassic transtensional tectonics in the western Tarim Basin that occurred along the Talas-Ferghana fault and a large-scale strike-slip fault along the eastern Pamir. Some other scientists attributed it to an episode of post-Triassic extensional tectonics (Zhang et al., 2000; Chen et al., 2003, 2009, 2018; Li et al., 2013). The validity of these models remains to be tested.
These Jurassic-Cretaceous tectonic deformations occurred after the Paleozoic-Early Mesozoic amalgamation history along the paleo-south Asian margin, and since then they played a first-order role in the localization and evolution of the Cenozoic intracontinental deformation along the northern edge of the Tibetan Plateau (Ritts and Biffi, 2001; Jolivet et al., 2010; Jolivet, 2015; Tong et al., 2020). Therefore, the geological processes of these Mesozoic basins must be clarified in order to understand a coherent history from Paleozoic to Cenozoic. Nowadays the main obstacles limiting our better definition of the pre-Cenozoic tectonic pattern is largely due to the incomplete basin geometry remolded by Cenozoic deformation, and few direct evidence of pre-existed structures are preserved (Figure 1C). Meanwhile, the structures and prototype of the basin that currently covered by thick Cenozoic deposits are lack of detailed studies (Figure 1D).
This paper serves as a study using structural and sedimentological analyses in the field, in combination with new 2D seismic profile interpretation and tectonostratigraphy with published data, to address the tectonic evolution of the western Tarim Basin throughout the Mesozoic. A regional synthesis suggests that the western Tarim Basin suffered extension during the Jurassic-Cretaceous, following the Triassic orogeny along the West Kunlun Mountains (Mts.) and the Southwest Tienshan Mts. This work provides new insights on understanding of tectonic switch from Triassic contractional phase to Jurassic-Cretaceous extensional phase in the western Tarim Basin and establishing a geodynamic relationship between the Paleo-Tethyan orogenic belts and related basins.
Geological Background and Tectonostratigraphy
Geological Background
The Tarim Basin is located in Central Asia, in the Xinjiang Province, northwest China. It is an almond-shaped basin with an area up to 50 × 104 km2 (Figure 1A). The Tarim Basin is the largest intracontinental petroliferous basin in China (Jia and Wei, 2002; Laborde et al., 2019) that is surrounded by the South Tienshan Mountains to the north, the West Kunlun Mountains to the southwest, and the Altyn Mountains to the southeast (Figure 1A). Its stratigraphic record spans the Proterozoic through the Cenozoic and reflects its long Phanerozoic tectonic history (Jia et al., 2004). During the Paleozoic, the northern Tarim margin was separated from the Yili-Middle Tienshan block by the South Tienshan Ocean, which was a seaway in the paleo-Asian Ocean (Xiao et al., 2015; Käßner et al., 2017). The southern margin of the Tarim Basin faced the wide Paleo-Tethyan Ocean, which separated it from the Gondwanaland (Mattern and Schneider, 2000; Stampfli and Borel, 2002). The South Tienshan ocean floor was subducted northward beneath the Yili-Middle Tienshan continental block between the Late Devonian and the Carboniferous, and was finally closed in the latest Carboniferous–earliest Permian (Han and Zhao, 2018). After the Ordovician-Silurian collision with the West Kunlun terrane (Xiao et al., 2005; Zhang C.L. et al., 2018), the southern Tarim experienced the progressive amalgamation with several terranes, which were derived from Gondwanaland, into the active margin of southern Asia, such as Qiangtang-Tianshuihai and Karakoram-Lhasa terranes (Zhu et al., 2013; Robinson, 2015). It is commonly accepted that the Paleo-Tethys Ocean subducted northward under the West Kunlun terrane by the Late Permian-Early Triassic, forming a magmatic arc along the southern margin (Mattern and Schneider, 2000; Xiao et al., 2002). This magmatic arc construction was followed by its collision with the Qiangtang-Tianshuihai terrane along the Kangxiwa suture in the Middle-Late Triassic as revealed by extensive syn-collisional magmatism as well as crustal shortening (Yang et al., 1996; Cao et al., 2015; Zhang et al., 2016). As a result of these tectonic events, the West Kunlun orogenic belt experienced strong basement reactivation and uplift, and crustal deformation propagated toward the northern part of the Tarim Basin, leading to the synchronous uplift of the South Tienshan Mountains during the Triassic (Hendrix et al., 1992; Han et al., 2016). The inferred timing of the amalgamation of the Qiangtang and Lhasa terranes ranges from Middle Jurassic to Late Cretaceous (Fan et al., 2015; Li S. et al., 2019), which makes the Jurassic-Cretaceous tectonic setting of the northern Tibetan Plateau somewhat enigmatic. Finally, the indentation of India into Asia since approximately 65–50 Ma resulted in a northward propagation of deformation across the Tibetan Plateau and forming the large intracontinental foreland basin in the Tarim (Figure 1D; Laborde et al., 2019).
Mesozoic Stratigraphy and Lithofacies
The western Tarim Basin preserved the complete terrestrial sequences spanning the Jurassic through the Cenozoic. Thick Jurassic-Cretaceous sediments are well-exposed in the elongated basins along piedmont of West Kunlun and Southwest TienShan Mts., whereas Triassic deposits are almost absent (Sobel, 1999). The Jurassic record includes the Lower Jurassic Shalitashi (J1s) and Kangsu Formations (J1k), the Middle Jurassic Yangye (J2y) and Taerga (J2t) Formations, and the Upper Jurassic Kuzigongsu (J3k) Formation (Figure 2). The Cretaceous record consists of two groups, the Lower Cretaceous Kezilesu Group (K1kz) and the Upper Cretaceous Yengisar Group, which can be subdivided into the Kukebai (K2k), Wuyitake (K2w), Yigeziya (K2y), and Tuyiluoke (K2t) Formations in a stratigraphically ascending order. The Jurassic-Cretaceous stratigraphy and lithofacies distributions of the western Tarim Basin have been well-studied through field sedimentological observations (e.g., Sobel, 1999; Zhang et al., 2000; Jia et al., 2004). Two tectonostratigraphic units were established as described below.
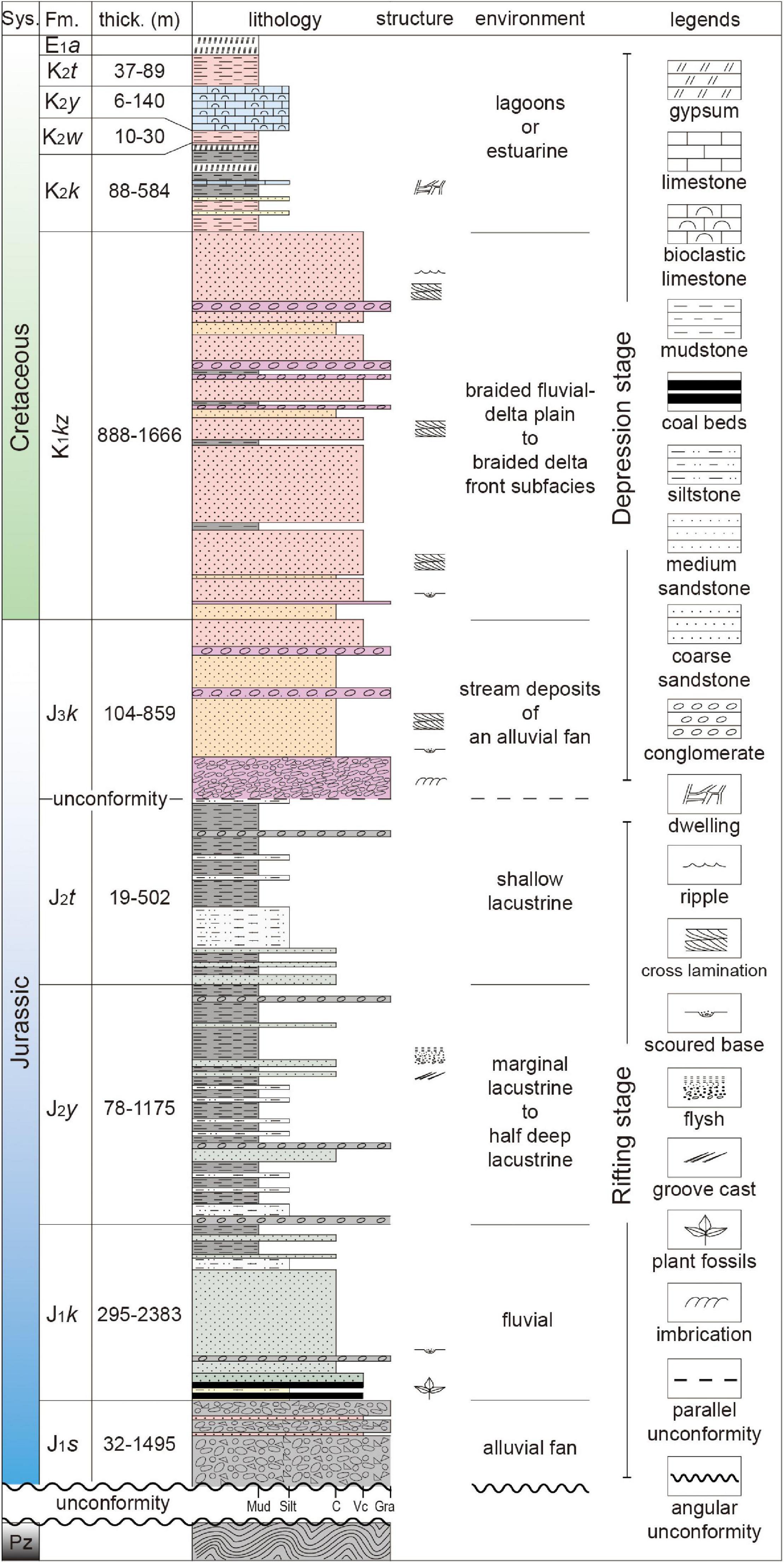
Figure 2. Generalized tectonostratigraphic column of Jurassic to Cretaceous system in the western Tarim Basin. Modified after Sobel (1999) and Zhou et al. (2005).
Lower Tectonostratigraphic Unit: Lower to Middle Jurassic Series
The Lower to Middle Jurassic sequences rest stratigraphically on the Paleozoic basement along an angular unconformity, and start at the bottom with the Shalitashi Formation, which ranges in thickness from 32 m to 1495 m (Zhou et al., 2005). The Shalitashi Formation is composed predominantly of massive and poorly sorted terrigenous conglomerate and polymictic breccia (Figures 3A,B), which are interpreted as proximal gravels representing alluvial fan deposits (Sobel, 1999). The Kangsu Formation mainly consists of stacked gray-greenish sandstones with coal beds containing abundant plant fossils (Figures 3C,D; Liao et al., 2010). Basal conglomerates and erosional basal surfaces represent fluvial deposits (Figure 2). The Middle Jurassic Yangye Formation is composed of continuous gray, black, and gray-green rippled or burrowed siltstone and shale, with disseminated leaf fragments and thin coal beds (Sobel, 1999). In the Keziletao section, this formation is dominated by successive gray-yellow thin sand-shale interbedded series (Figure 3E) with typical Bouma sequences (Figure 3F). Groove casts at subface of sandstone beds (Figure 3G) and soft-sediment deformation of mudstone mass encapsulated by the sandy body (Figure 3H) indicate turbidite deposition. This association is described as marginal lacustrine to half deep lacustrine facies deposits (Zhou et al., 2005). The uppermost part of the unit is Taerga Formation, which comprises gray-yellow shale beds intercalated with siltstones (Figure 3I). These beds are relatively thin and uniform single-layers with thicknesses of centimeter-scale. Thin gray-white argillaceous limestone interlayers and limestone nodules occur the upper part. These laterally well-extended mud-siltstones represent the shallow lacustrine sequences.
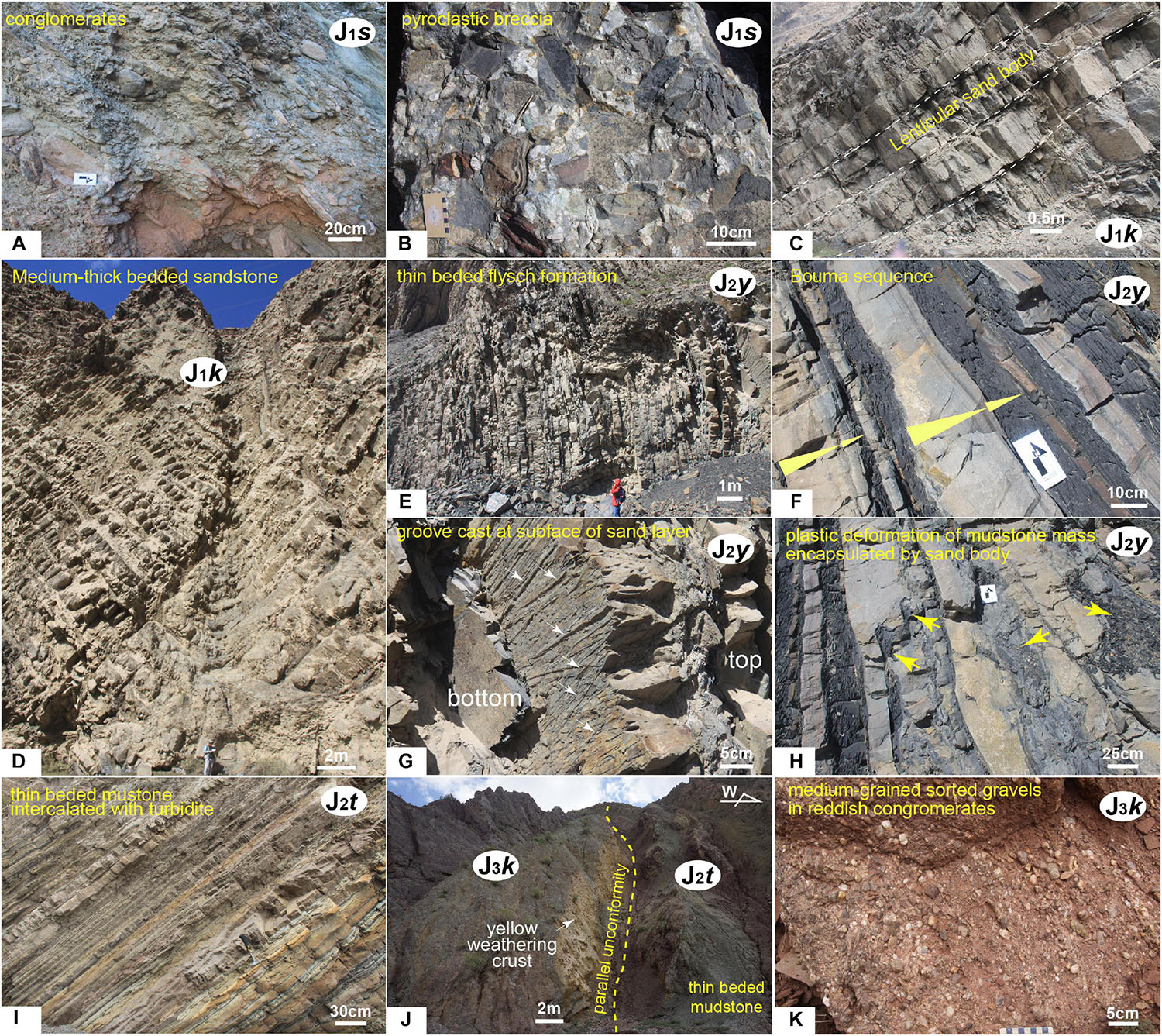
Figure 3. Photographs of Jurassic strata. (A) Conglomerate of Shalitashi Formation in Wulagen section. (B) Pyroclastic breccia of Shalitashi Formation in Keziletao section. (C) Thick-bedded sandstone with a lenticular body of Kangsu Formation in Keziletao section. (D) An isoclinal fold of Kangsu Formation in Keziletao section. (E) Thin-bedded flysch deposits of Yangye Formation in Keziletao section. (F) Bouma sequence in Yangye Formation. (G) Groove cast at the subface of sandy layer in Yangye Formation. (H) Muddy mass with soft-sediment deformation in Yangye Formation. (I) Thin-bedded mudstone with intercalated siltstone of Taerga Formation in Kangsu section. (J) Parallel unconformity and yellow weathering crust between Middle and Upper Jurassic in Oytag section. (K) Medium-grained gravels in reddish conglomerate of Kuzigongsu Formation.
Upper Tectonostratigraphic Unit: Upper Jurassic to Late Cretaceous Series
The stratigraphically upper succession begins with the Upper Jurassic Kuzigongsu Formation, which rests on the Middle Jurassic rocks along a regional parallel unconformity (Figures 2, 3J; Yang Y.T. et al., 2017). The Kuzigongsu Formation comprises reddish thick-bedded conglomerates in the lower member and rhythmic layers of upward-fining pebbly sandstone in the upper member. The medium-grained and well sorted pebbles represent stream deposits in an alluvial fan (Figure 3K). The Kuzigongsu Formation phases upward into pebbly sandstones and mudstones with tabular cross laminations (Figure 4A) and scoured subfaces (Figure 4B) that represent fluvial deposits. The Lower Cretaceous thick-bedded coarse sandstones with muddy interlayers of the Kezilesu Group conformably rest on the Kuzigongsu Formation (Figure 4C). Large cross laminations characterize the Kezilesu Group deposits (Figures 4D–F). The upward-fining sand-mud sequence containing limestone nodules in the upper part of the Kezilesu Group points to a depositional environment transitioning from a braided fluvial-delta plain to a braided delta front subfacies (Sobel, 1999). Since the Late Cretaceous, this region has been predominated by several cycles of mudstone (Figure 4G) and marine carbonate beds (Bosboom et al., 2011; Zhang S.J. et al., 2018). Previous studies have found benthic foraminifers, ostracods and abundant bivalves, which indicate the steady high-energy lagoons or estuarine environments (Xi et al., 2016).
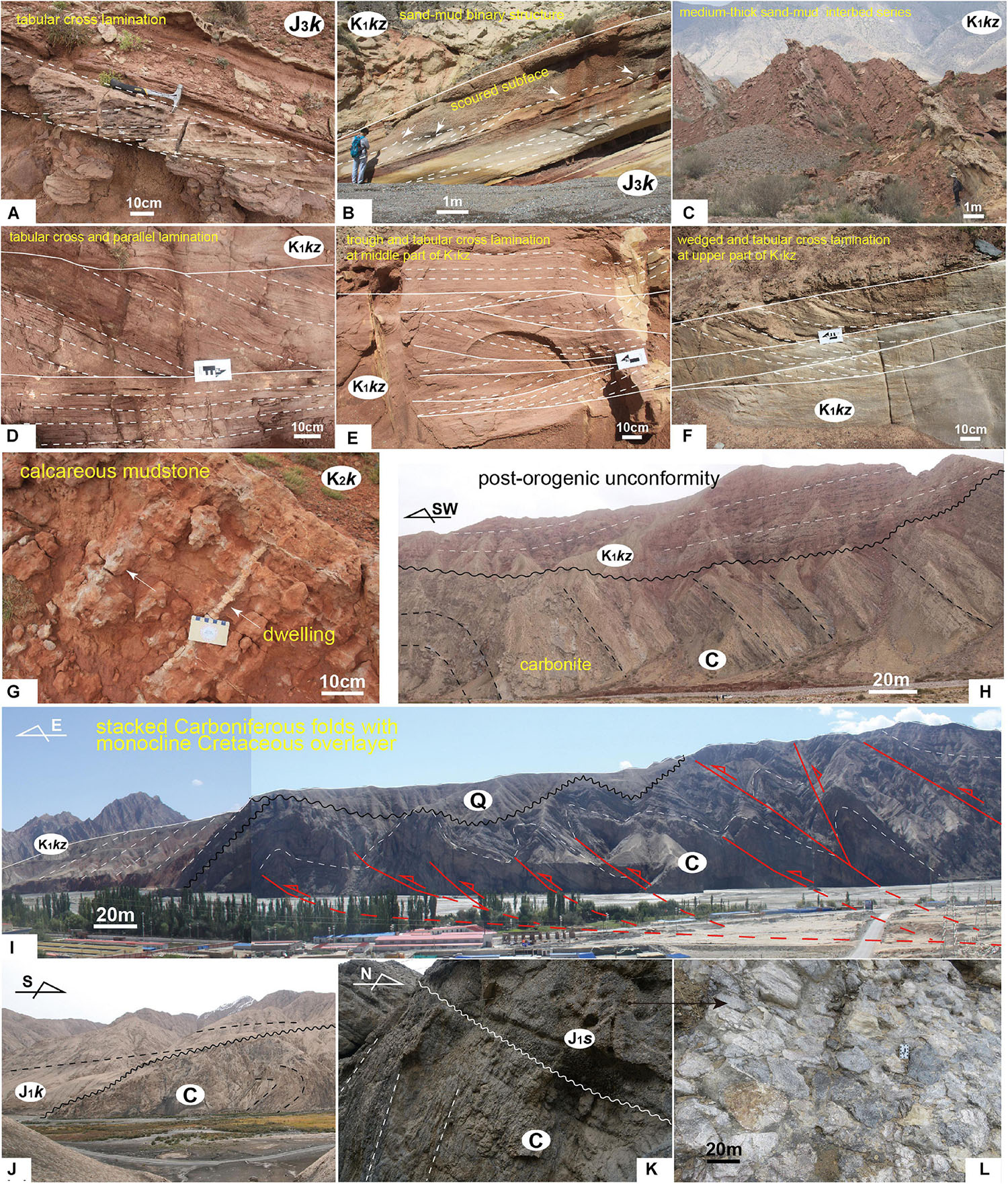
Figure 4. Photographs of Late Jurassic to Cretaceous strata and the underlying deformed Paleozoic strata. (A) Tabular cross lamination in Kuzigongsu Formation. (B) Sand-mud binary structure with scoured subface in Kuzigongsu Formation. (C) Medium- thick sand- mud interbedded series of Kezilesu Group. (D) Tabular cross and parallel sandy layers of Kezilesu Group. (E) Trough and tabular cross lamination at middle part of Kezilesu Group. (F) Wedged and tabular cross lamination at upper part of Kezilesu Group. (G) Reddish calcareous mudstone with dwelling structure in Kukebai Formation. (H) Folded carboniferous and the post- orogenic unconformity (I) Stacked Carboniferous strata with monocline Cretaceous overlayer from Aertashi section. (J) The outcrops from Keziletao show that the recumbent fold occurred within Carboniferous strata, and it was overlaid by the Early Jurassic (Kangsu Fm.) along an angular unconformity. (K) The underlying Carboniferous strata having vertical attitude and the overlying Earliest Jurassic conglomerate in Qimugen section. (L) The conglomerate of Shalitashi Fm. mostly consisted of limestone gravels.
Materials and Methods
To gain information on buried structures and distribution of Mesozoic strata in western Tarim Basin, five available 2D seismic reflection profiles from the Tarim Oilfield Co., Ltd., PetroChina are applied to this study. These seismic profiles are distributed in Keliyang, Qimugen-Aertashi and Kangsu regions from the south to the north (Figure 1B). The synthetic seismic data are time-migrated with the vertical scale in milliseconds two-way travel time, and all of them show relatively clear deep structures. Four drilling wells (WX1, S1, QB3 and KY) are used for calibrating stratigraphic reflector layers on the seismic profiles. The joint use of borehole data and formation occurrence on geologic map is applied to track the strata in the piedmont segments that have low signal-to-noise ratios. The seismic profiles in the basin interior are imaged well so that we could track the reflectors reliably.
On most cross profiles, eight stratigraphic units, including Lower Paleozoic, Carboniferous, Permian, Mesozoic, Paleogene, Miocene, Pliocene and Quaternary units, are determined. Two key lithologic interfaces are identified for their high-amplitude reflection features. One of the lithologic interfaces is the gypsum horizon at the bottom of the Paleogene, and another is the salt layer in the lower Cambrian series. The angular unconformity which separates the Mesozoic strata from the Paleozoic basements, could also be identified by the truncate surface (Figure 5; Cheng et al., 2012).
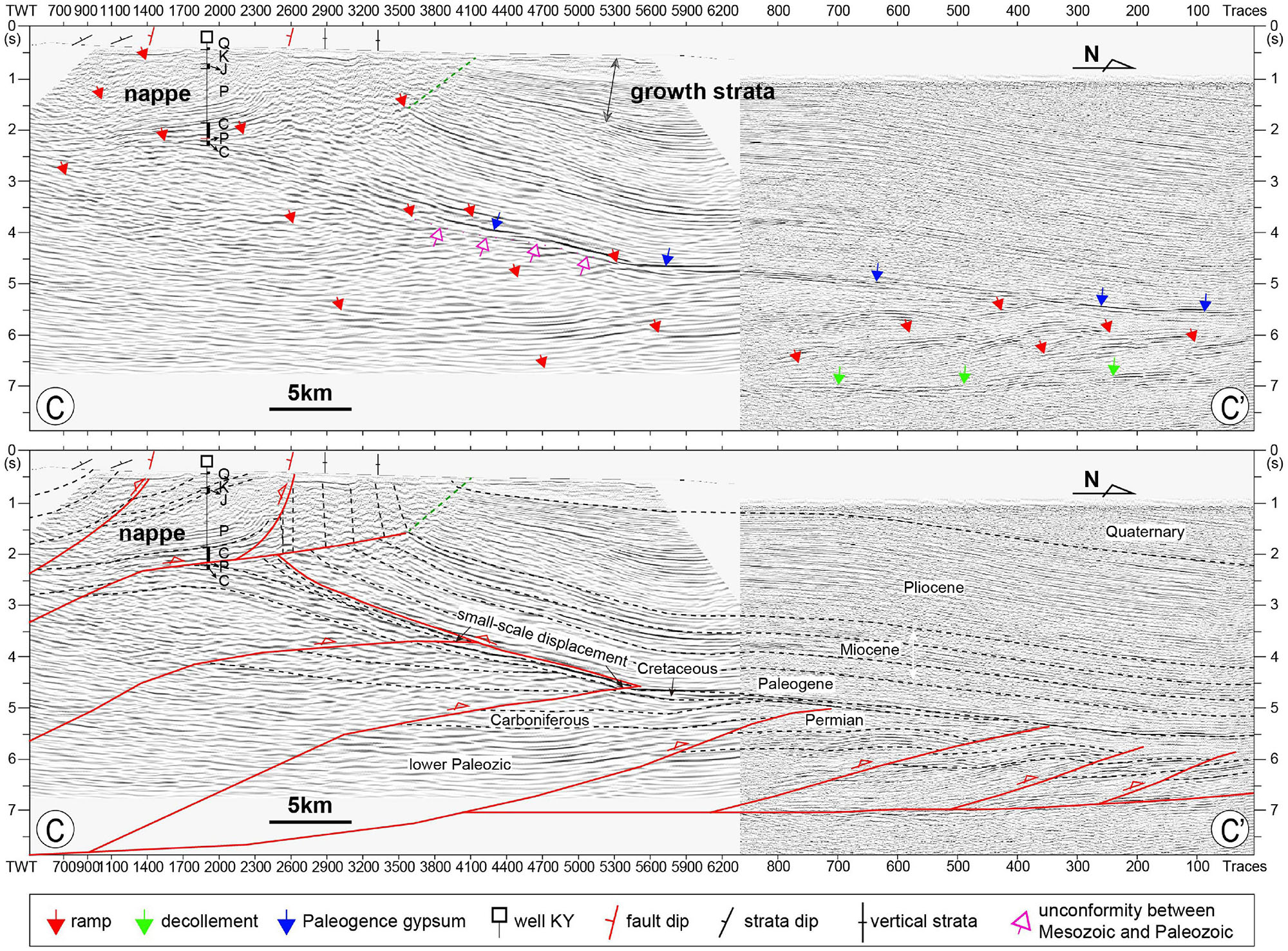
Figure 5. Interpretation of the seismic profile near the Keliyang section, the location is showed in Figure 1B. Strata division of well KY and key surface marks have been indicated on the profile.
Besides, Palaeocurrent data of Jurassic-Cretaceous are collected by measuring dips of cross-lamination and are revised by attitudes of strata in this effort.
Seismic Structures in Western Tarim Basin
Profile C-C’
The seismic line C-C,’ run across the well KY, is almost orthogonal to the West Kunlun piedmont structural belt (Figure 1B). Along this transect, most of the basin surface is covered by Cenozoic sediments. Carboniferous to Permian strata were exhumed by south-dipping basement-involved faults in the southmost of the transect. Well KY reveals that the Carboniferous overlapped on the Permian with gentle attitudes. The forelimb of the asymmetric anticline is almost vertical and exposes Jurassic to Quaternary strata with uniform deformation. We propose a breakthrough fault-propagation fold model to explain this structure (Figure 5). Growth strata reveal that an early anticline has started to generate during Pliocene and the forelimb of the anticline was broke-through in Quaternary (Wang et al., 2016a).
In the basin outback, two regional high-amplitude reflective bands are interpreted as two regional decollement layers corresponding to the Paleocene gypsum reflector and the Lower Cambrian evaporite, respectively (Guilbaud et al., 2017). In Yecheng subbasin, a fold-and-thrust belt with an approximate width of 60 km formed. At the root zone, it was characterized by a series of south-dipping basement-involved faults with a passive-roof back thrust on the top, which is corresponding to the base of Paleocene gypsum, and formed a series of stacked structural wedges (Figure 5). At the front zone, several north-vergent thrust ramps developed and together terminated along the basal detachment. Paleozoic strata were folded by several thrust ramps and formed an array of shear fault-bent anticlines. These anticline’s forelimbs were quite closed relative to their long backlimbs, and the tops of the Permian were partly eroded. The Mesozoic-Cenozoic cover overlying on these wedges showed northward-dipping occurrence on the whole. Comparing with the Paleozoic strata, Jurassic-Cretaceous have obviously endured relatively slight deformation. The angular unconformities between the Jurassic-Cretaceous cover and Paleozoic basements are clearly identified based on seismic reflector discontinuity. Integrated with the evidence of absence of Triassic deposits, smaller-scale displacements between Jurassic-Cretaceous strata contrast to Paleozoic deformation (Figure 5), and the discordant deformation of Cenozoic overlays together indicate that during the Triassic the western Tarim Basin was possibly uplifted and the sector represented fold-and-thrust belt.
Profiles D-D’ and E-E’
The seismic profile D-D’ (Figure 6), which is situated to the north of Qimugen, is a typical section to reveal the topographical controlling of Cretaceous distribution along basinal edge both by reflections and drilling wells (Liao et al., 2010). It shows that the topography low is a catchment with thick Cretaceous deposits, whereas the topography high accumulates thin Cretaceous or none sediments (Cheng et al., 2012). The stacked thrust structures are present in Paleozoic strata with SW-dipping faults, although Late Cenozoic overprints cannot be negligent. We interpreted that most of these SW-vergent faults have been active during Triassic for the following reasons. First, the Cretaceous strata settled on Permian basements unconformably with absence or erosion of Triassic sediments. Secondly, most faults are truncated by Cretaceous subface or have a small displacement in Cretaceous reflections. Finally, the gently stable Cenozoic strata fold between well S1 and YS1 are unable to compensate all the deformation within Paleozoic strata if only Late Cenozoic compressional event was responsible for it. Thus, it urgently calls for the paleo-structures prior to the Cretaceous deposition.
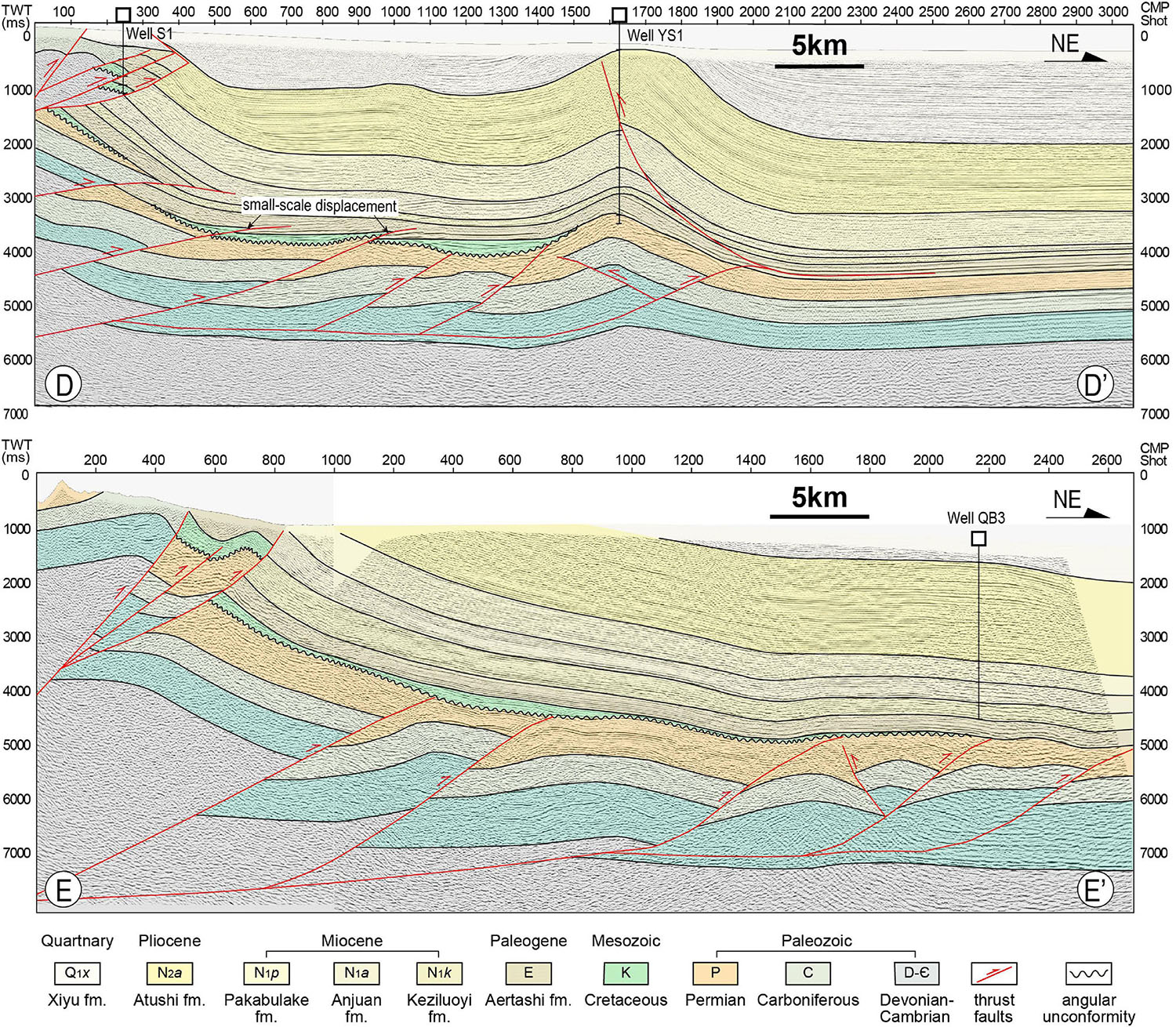
Figure 6. Interpretation of the seismic profiles in Qimugen area. Locations of the profiles are showed in Figure 1B.
Similarly, dual-phases contractional evolution is comprehended along section E-E’ which shares comparable structural style along the Qimugen-Aertashi belt (Figure 6). The whole of the transect shows a simple monocline where the attitudes between Mesozoic and Cenozoic strata are gentle and concordant. It indicates that the Late Cenozoic deformation was mild respect to the complex structures beneath the Cretaceous.
Profiles F-F’ and G-G’
Profiles F-F’ and G-G’ are situated in north Kashgar depression and perpendicular to each other in NS and EW direction, respectively (Figure 1). Kilometer-scaled thick Jurassic-Cretaceous outcrops are exhumed along the north and east of profiles. Different from the above sections, profiles F-F’ and G-G’ lie in the interactional zone between the Pamir and Southwest TienShan Mts. (Figure 1) and thus bare dual load from the south and north (Burtman, 2000; Liu et al., 2017). Complex compressional architecture since Late Miocene erased the trails of paleo-structures which have been deeply buried under the Cenozoic basin (Cheng et al., 2016; Li T. et al., 2019). Nonetheless, graben-shaped structures formed in Jurassic are described via steep reflection axis and thick Jurassic-Cretaceous sequence tracing from outcrops and lateral seismic reflections (Figure 7). On the profile F-F,’ Lower-Middle Jurassic strata were truncated by south-dipping normal fault, whereas the reflections in Upper Jurassic and Cretaceous are continuous. It indicates that the Early-Middle Jurassic sediments were controlled by faults, which show characteristics of syn-rift sequences, while the Late Jurassic and Cretaceous basin was more uncommitted. Although this basin structure requires more details to confirm, the abnormally thick and fast filling of Jurassic sequence on outcrop probably gives support for such normal fault interpretation.
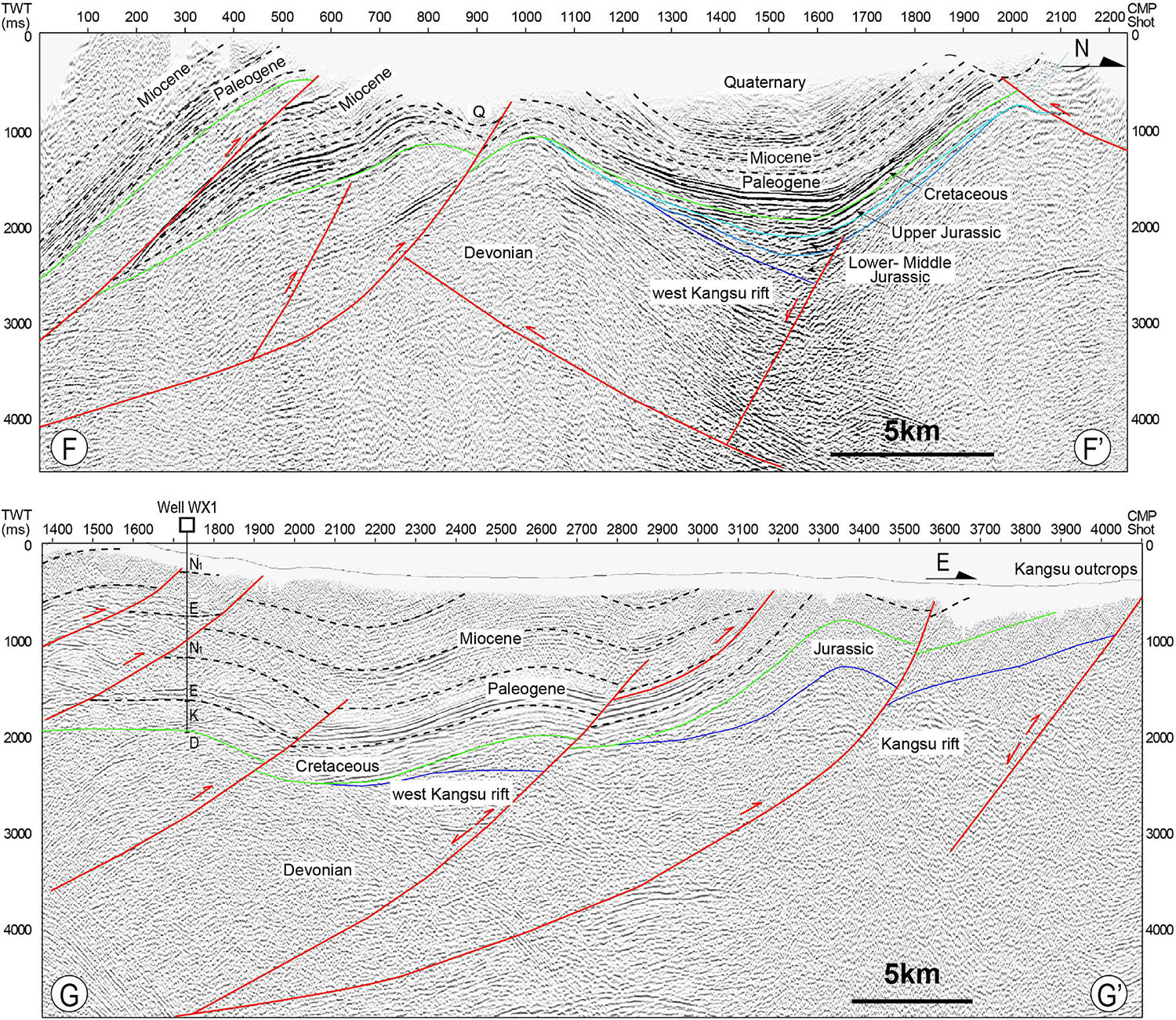
Figure 7. Interpretation of the seismic profiles in Kangsu area. The green and blue lines show the subface of Cretaceous and Jurassic strata, respectively. Locations of the profiles are showed in Figure 1B.
Discussion
Early Cimmerian (Late Triassic) Deformation
It is generally recognized that the regional deformation throughout Central Asia at the end of the Triassic was a result of closure of the Paleo-Tethys Ocean and the ensuing collision with the strips of Cimmerian-terranes drifted from Gondwana (Şengör et al., 1984; Otto, 1997; Wilmsen et al., 2009; Zanchi et al., 2009; Gillespie et al., 2017). In Tibetan Plateau, the Early Cimmerian orogeny was driven by the convergence between Tarim and Qiangtang block (Matte et al., 1996; Yin and Harrison, 2000; Zhu et al., 2013; Song et al., 2015). Previous analyses proposed that the northward subduction of the Paleo-Tethys ocean floor beneath the South Kunlun has initiated by Late Permian-Early Triassic, producing an accretionary prism at the margin of South Asia, and it was followed by the resultant collision in the Late Triassic (Mattern and Schneider, 2000; Xiao et al., 2003). During the collisional phase (ca. 243-227Ma), a mega-magmatic belt of high-K calc-alkaline granitoid belt extending with NW orientation intruded within West Kunlun Mts. (Figure 1B; Liao et al., 2012; Jiang et al., 2013; Wang et al., 2016b; Zhang et al., 2016). And the suture zone along Mazha-Kangxiwa (also called Karakax fault) performed as a ductile shear fault to accommodate the convergence between Qiangtang and Tarim at the end of Triassic (Xu et al., 2007; Ge, 2018). The simultaneous crustal cooling event (ca. 250-200Ma) was recorded by low-temperature thermochronology from these Triassic batholiths that revealed a fast orogenic exhumation progress in West Kunlun Mts. (Cao et al., 2015; Li G.W. et al., 2019). These batholiths thus continued to be the source region for the Tarim Basin to the northeast throughout the Mesozoic (Bershaw et al., 2012; Cao et al., 2015; Han et al., 2016). Together with large scale magmatism, metamorphism and topographic uplift, an intense crustal contraction occurred within the paleo-Kunlun ranges and documented the early Cimmerian orogeny along the middle segment of the Paleo-Tethyan domain (Wu et al., 2016).
Previous studies have revealed that this contractional event in Late Triassic formed a south-verging fold-and-thrust system to the southern West Kunlun Mts., which is called the Tianshuihai back-thrust system (Cowgill et al., 2003). We report here an intense fold-and-thrust deformation generated along the western Tarim Basin that stand by paleo-Kunlun ranges to the south. Structural analysis suggests that the Triassic deformation was mainly controlled by advancing thin-skinned thrust-folds and these north-northeast vergent stacked faults formed the front fold-thrust belt of the paleo-Kunlun wedge, which was probably propelled by the Karakax ductile extrusion from the collisional core zone (Li G.W. et al., 2019). Outcrop structural analyses also give clues about the existence of these paleo-deformations. At Aertashi, along the Yarkant river, Carboniferous strata exposed and formed a series of asymmetric anticlines and synclines that controlled by mountain-ward dipping thrusts (Figure 4I). By contrast, Cretaceous strata just unconformably rest on the upside with a simple east-dipping monoclinic style. At Keziletao, Early Jurassic strata overlay on the Carboniferous recumbent fold through an angular unconformity (Figure 4J). Similarly, it could also be seen that the underlying Carboniferous layers were almost vertical (Figure 4K) and covered by the Earliest Jurassic conglomerate of Shalitashi Fm. from Qimugen section (Figure 4L). Field mapping along transect across western Tarim margin reveals a southeast- strike anticlinoria, which is composed of Devonian-Carboniferous in the core showing closed-form. The Cretaceous cover stays on two limbs with gentle style (Figure 8; Cheng X.G. et al., 2019). The discordant relationships between two sets of strata indicate a two-stage shortening history since the Early Mesozoic: a Triassic stage of southwest tectonic stress making Paleozoic strata thrusted and stacked, followed by a Late Cenozoic stage of tectonic reactivation making Jurassic-Cretaceous strata gently folded. To sum up, the ancient fold-and-thrust belt preserved in the Paleozoic strata, as well as the unconformity between the Paleozoic and Mesozoic strata indicate that the western Tarim Basin was involved into the strongly contractional tectonism during Early Cimmerian event.
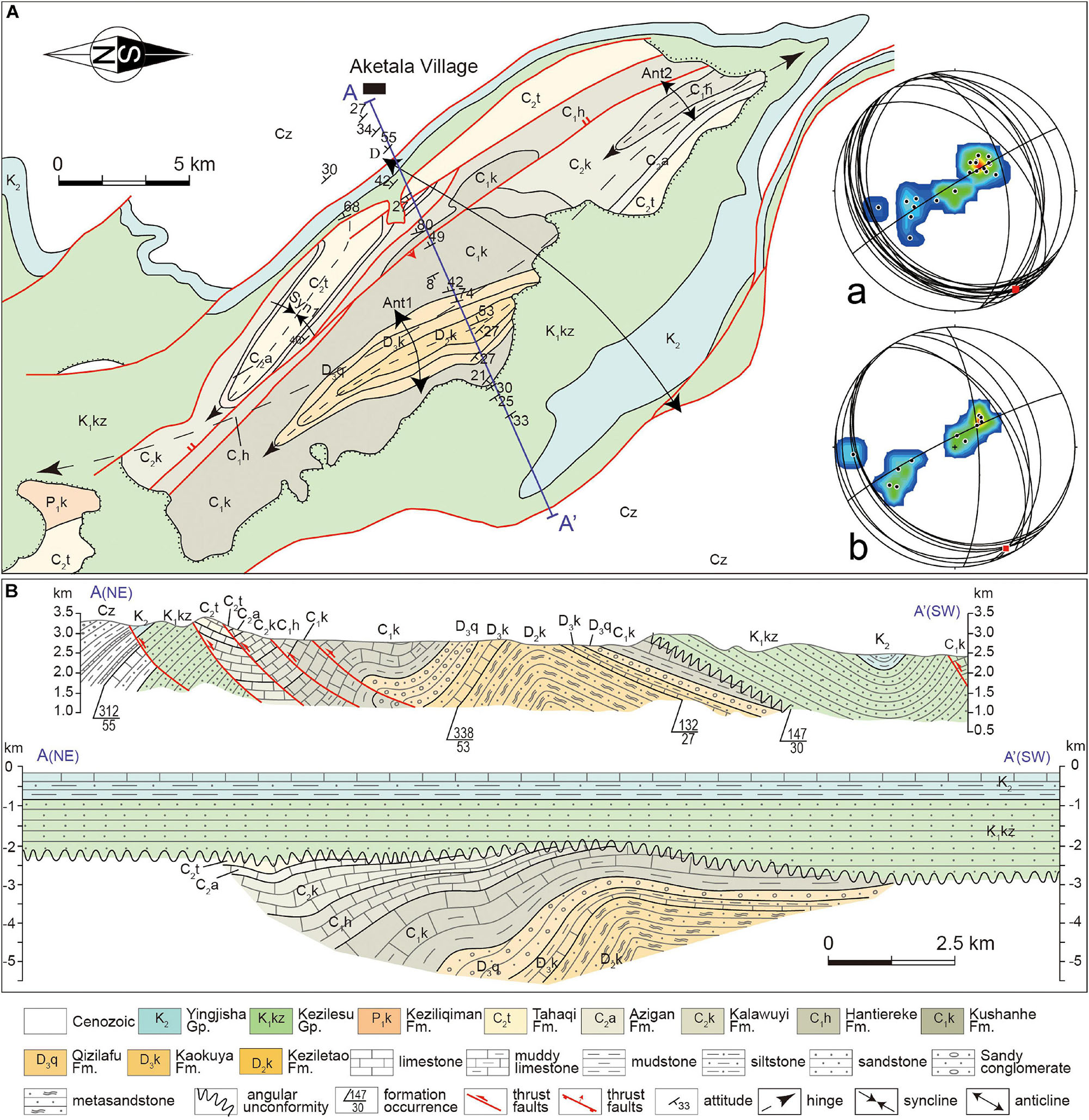
Figure 8. Anticlinoria near Aketala village showing two main phases of deformation (modified after Cheng X.G. et al., 2019). (A) planar geological map and the stereoplots (lower hemisphere- equal area projections) of anticline limbs, data collected from the Cretaceous strata (a) and Paleozoic strata (b). (B) cross section A-A’ and its balanced pattern after restoring the Cenozoic deformation.
Extensional Basin During Jurassic-Cretaceous
The Early Cimmerian orogeny caused the complete absence of Triassic strata in the western Tarim Basin, and the Jurassic-Cretaceous basin overlay on the deformed Paleozoic with a sharply divergent unconformity. The Early Jurassic basin was characteristic by sudden subsidence with thick conglomerate or breccia settled at the bottom (Figures 3A,B). The upward-fining sequence of Lower-Middle Jurassic gradually varied from a set of thick-bedded channel sandstones to deep lacustrine flysch deposits (Figures 3C–H), which imply the fast subsidence phase of the basin. During this stage, there was a significant lateral variation of cumulative stratigraphic thickness along the strike of the banded basin (Figure 9). The maximum subsidence occurred in Qiemugan-Keziletao area in the central regions, where the Jurassic deposits with stratal thicknesses exceeding 4 km accommodate (Figure 9). The moderate and weak subsidence with variable Lower-Middle Jurassic thickness were developed in Kangsu and Kekeya area in the southern and north regions, respectively (Figure 9). These major isolated depocenters were separated by high hinges that reject Jurassic sediments settled on. Palaeocurrent indicators show that the eastward paleo-drainage system had formed along West Kunlun side, but the paleo-drainage system along Southwest Tienshan was dominated by southward channels (Figure 10A). These basins are likely associated with normal faults along the orogenic belt side, as suggested by syn-rifting structures on seismic profiles (Figure 7). The linear geometry of Early-Middle Jurassic basin is also compatible with the trending of paleo-orogens or regional large inherited faults which gave the possibility for reactivation of pre-existing structures during stretching phase (Figure 9; Cheng F. et al., 2019). Although, the transtensional model proposed by Sobel (1999) argue for the Early-Middle Jurassic pull-apart basin that bounded by two postulated large-scale strike-slip faults. One is the Talas-Ferghana fault, and another is possibly the ancestor of Main Pamir thrust. This kinematic model requires the large dextral strike-slip fault cut through the Kashgar depression and extended southward into Yecheng depression. However, seismic profiles from the western Tarim Basin and paleomagnetic constraints do not favor such hypothesis (Li et al., 2007; Huang et al., 2009; Zhang and Sun, 2020). Instead, the southern extension of this zone was a transform-orogen junction with southward motion of the eastern wall accommodated by southward thrusting at the margins of the south Tien Shan and the Tarim Basin (Huang et al., 2009). Therefore, the Early-Middle Jurassic extensional model controlled by range-side normal faults is more comprehensible.
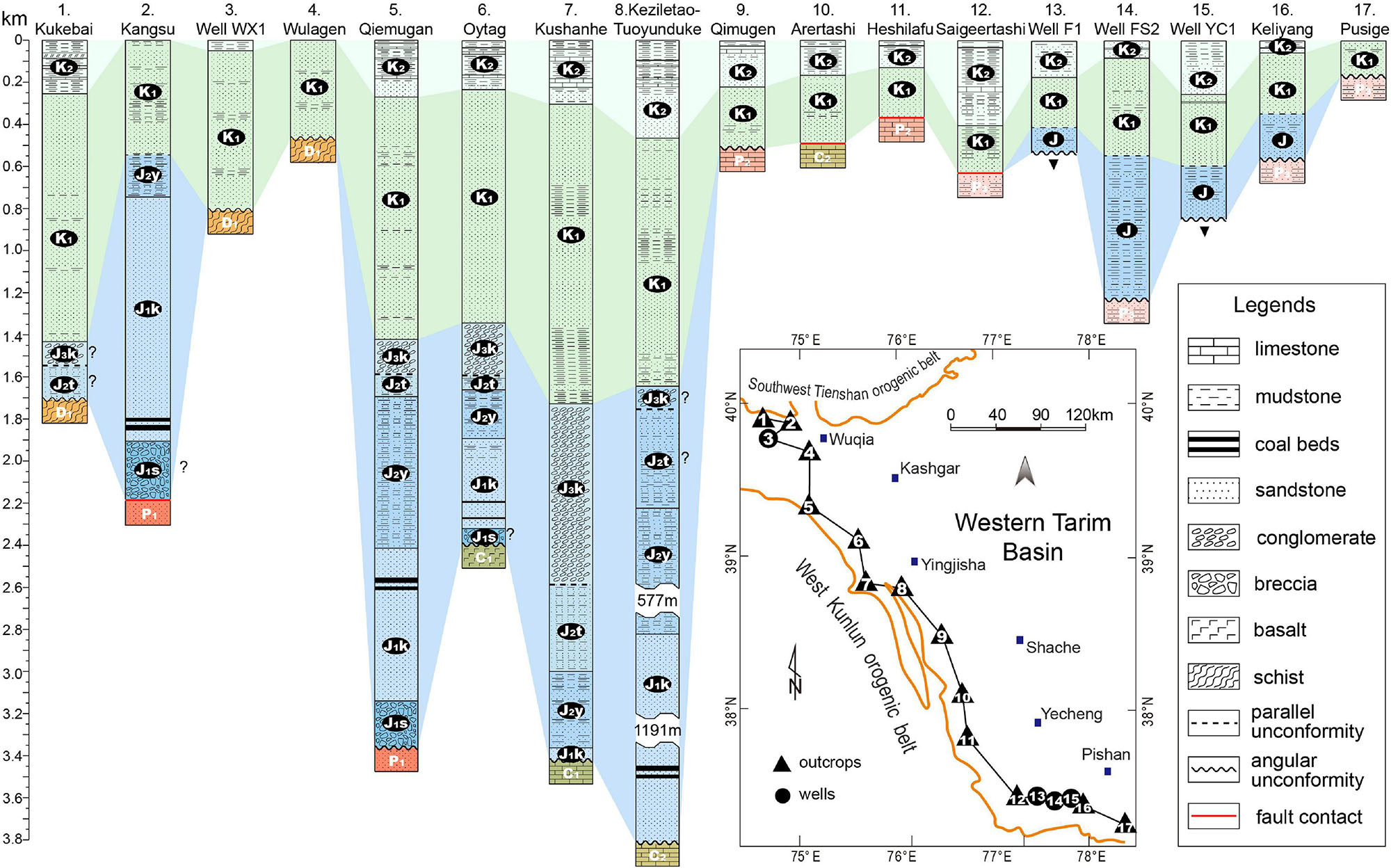
Figure 9. NW- SE correlation of cross-sections shows the distribution of Jurassic and Cretaceous and their stratal thicknesses. The locations of these stratigraphic columns are given below. Thickness data of Cretaceous were measured by PetroChina. Thickness data of Jurassic were from Sobel (1999) and Zhou et al. (2005).
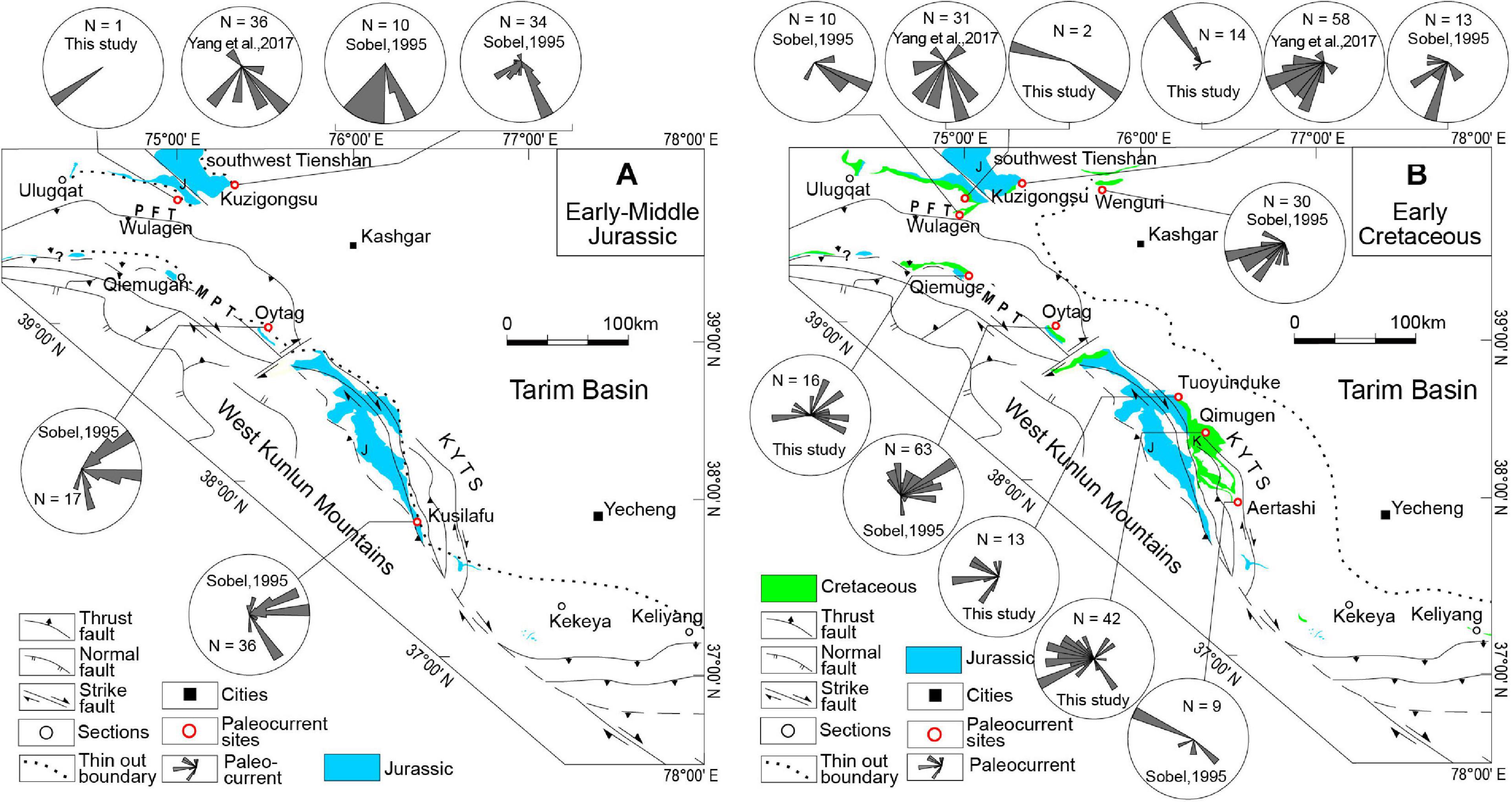
Figure 10. (A) rose diagrams of Early-Middle Jurassic paleocurrent directions. (B) rose diagrams of Early Cretaceous paleocurrent directions. Paleocurrent data were measured in the field and some of them were compiled from Yang W. et al. (2017) and Sobel (1995).
The successive phase of basin developing started with the regional parallel unconformity that separated the upper association from the Lower-Middle Jurassic series. Contrast to the pioneering phase, the sequence of Late Jurassic through Early Cretaceous were characterised by several cycles of coarse clastic deposits with large scale cross laminations that suggest a fluvial to braided delta setting (Figures 4A–F). The Cretaceous basin was obviously more unconfined and not confined by directly faulting effects (Figure 7). Vaster extensiveness and more flat topography during the Early Cretaceous indicate consistent expansion of the basin boundary (Figures 9, 11). Palaeocurrent analyses firstly showed the reversed flow direction from east to west in the middle segment during this time (Figure 10B). It suggests that a new clast source possibly eroded from the basement in outback contributed to the Early Cretaceous basin. Ultimately, the basin was dominated by several recurrent depositions of mudstone-carbonate association in Late Cretaceous (Hao et al., 1987; Bosboom et al., 2014; Xi et al., 2016), showing that the sedimentary environment was chiefly controlled by several transgressive-regressive cycles (Zhang S.J. et al., 2018).
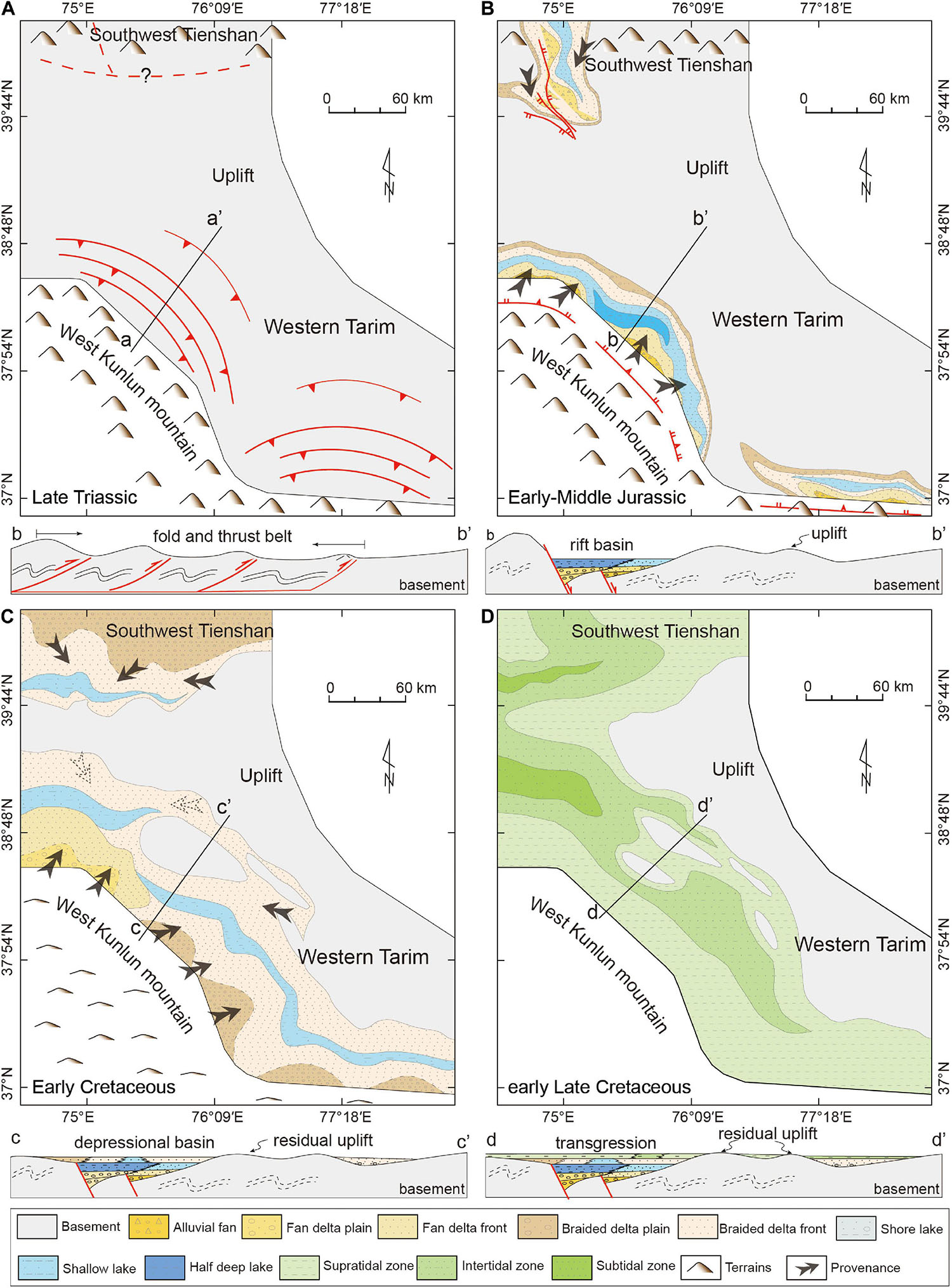
Figure 11. Paleogeographic evolution of the western Tarim Basin during the Late Triassic to Late Cretaceous. The basin boundary is retreated to the original position by restoring the structural deformation of balanced sections from Cheng et al. (2012, 2016). (A) Late Triassic paleogeography. (B) Early-Middle Jurassic paleogeography. (C) Early Cretaceous paleogeography. (D) Early Late Cretaceous paleogeography.
Syn-rift basins are usually associated with the rapid stretching of continental lithosphere and form a series of block normal faults that create discrete sunken space for sediments (Jackson et al., 2006; Allen and Allen, 2013). If crustal thinning is substantial, rift basins are commonly accompanied by inherited post-rift (depression) phase depends on thermal subsidence compensation (McKenzie, 1978; Ziegler and Cloetingh, 2004; Shi et al., 2017). As the basin structurally reorganizes, a transitional unconformity usually develops (Bell et al., 2014; Zhang et al., 2019). Meanwhile, the transition from isolated to large depocenters is expressed by a progressive change in the sequence stratigraphy, from coarse, alluvial fan and fluvial facies into lacustrine or marine facies (Gawthorpe and Leeder, 2000).
A similar process occurred in the western Tarim Basin during the Jurassic to Cretaceous. Fault-controlled, fast and thick accumulation of clastic sediments resulted in the formation of the Lower- Middle Jurassic system in the western Tarim Basin, and the coeval and lithologically analog sequences represent syn-rift phase in sedimentary basin evolution. Comparing to the syn-rift type, extensional faulting gradually became inactive showing on seismic interpretation (Figure 7) and the sediments covered the rift shoulder since Late Jurassic. Simultaneously, a transitional unconformity between Middle and Upper Jurassic occurred and the basin came into depressional phase. This growing unconformity ultimately affected the basin outback with the expansion of Cretaceous deposition. As a result, the unconformity between the Cretaceous and Paleozoic were widely preserved in the western Tarim Basin (Figure 4H). The lopsidedness of Cretaceous thickness tends to decrease, and sediments deposited in a single and relatively unconfined basin. Nonetheless, the thickness of Lower Cretaceous still varies according to the terrains where they get accommodation. In particular, stratigraphic columns show obvious continuity and inheritance of thickness between the Lower Cretaceous members and Lower-Middle Jurassic members (Figure 9). In other words, the Cretaceous inherited the Jurassic basin depocenters and further expanded on the formed basis (Figure 11). Cretaceous deltaic and ensuing epeiric sea deposits overlapping on the top along a parallel unconformity represent the post-rift accumulation. The relatively persistent depositional sequences accompanied by subsequent transgressions imply that the western Tarim region was seldom disturbed by additional structural activity. In this regard, a complete Late Mesozoic extensional basin mode overprinting on the fossil fold-and-thrust belts has been established.
Geodynamic Switch for Paleo-Tethyan Evolution
The drivers for regional extension have been linked to subduction retreat (Uyeda and Kanamori, 1979; Zheng and Dai, 2018), mantle plume and related continental rift (White and McKenzie, 1989; Li et al., 2008), and post-orogenic stretch that caused by lithosphere thinning (Kay and Kay, 1993; Krystopowicz and Currie, 2013). Subduction retreat and mantle plume event commonly produce massive volcanism or intrusions in the uppermost crust (Bryan and Ferrari, 2013; Ernst et al., 2019). However, the western Tarim Basin and its periphery are relatively inactive and without magmatic material inputting from surrounding orogens during Jurassic-Cretaceous (Chapman et al., 2018).
Geological observation indicates that mega-collisional orogenic belts usually undergo late or post-orogenic thinning (or extension) around the world, such as the well-known examples of the eastern Alps and Tibetan Plateau (Gaudemer et al., 1988; Ratschbacher et al., 1989). Conventionally, a complete orogenic evolution comprises three major stages orderly: collision and crustal thickening, to metamorphism and orogenic root delamination, and finally lithospheric collapse and extension (Leech, 2001). Crustal thickening and topography elevating along Paleo-Tethyan orogenic belt have been demonstrated based on structural, petrological, geochemical and thermochronological evidence discussed above (Xu et al., 2007; Cao et al., 2015; Liu et al., 2015; Zhang et al., 2016; Li G.W. et al., 2019). Some of Latest Triassic post-collisional magmatism and bimodal magmatism have been reported in West Kunlun and South Tienshan Mts. in recent years (Liao et al., 2012; Jiang et al., 2013; Tang et al., 2017). It indicates the post-orogenic delamination could have occurred. However, the post-Triassic extensional basin was always ignored (Leith, 1985; Brookfield and Hashmat, 2001). Structural and tectonostratigraphic analyses in western Tarim Basin provide new insights into the evolution between the Early Cimmerian orogeny and the subsequent Jurassic-Cretaceous extension along the Paleo-Tethyan orogenic belt. Chronologically, the Early Jurassic rift succession unconformably rested on the Paleozoic strata that deformed in Triassic orogeny. Such rapid basin subsidence and sedimentation following Triassic collision are most like post-orogeny pattern that usually makes an abrupt change from positive relief to negative landform (Peron-Pinvidic and Osmundsen, 2020). Spatially, the distributions of Jurassic-Cretaceous strata are mainly constrained by the residual landscape within the scope of Triassic fold-and-thrust belts (Figure 11). The depocenters are situated close to orogenic belt side, whereas the far outback of basin has no Jurassic-Cretaceous record. Close tempo-spatial coupling implies that the paleo- fold-and-thrust belt could play potential roles in controlling the subsequent subsidence and deposition, although the detail manners of how the ancient contractional structures reverse and reorganize are required to confirm. Taking all of these considerations, we propose that the post-orogenic dynamics of Paleo-Tethyan orogenic belt is likely to dominate the tectonic switch from Triassic contraction to Jurassic-Early Cretaceous extension along the South Asian margin.
Conclusion
A better understanding of the Mesozoic western Tarim Basin provides significant insights into the tectonism prior to Cenozoic for the northwest margin of the plateau. A synthetical analysis based on seismic interpretation, tectonostratigraphic and sedimentological studies leads us to draw the following main conclusions:
(1) New seismic profile interpretations provide constrains on the basin architecture. An approximate 60 km wide fold-and-thrust deformation belt formed along the western Tarim Basin margin during Triassic. These range-side dipping stacked faults belong to the north front thrusts of the paleo-Kunlun wedge, which was caused by contractional deformation during the Early Cimmerian orogeny. This contractional event was the primary cause that leading to the topography uplift and the absence of the Triassic system in the western Tarim Basin.
(2) The Jurassic-Cretaceous system rests on the folded Paleozoic strata along a post-orogenic unconformity. The Jurassic-Cretaceous stratigraphy is divided into two tectono-sequences, separating by the regional parallel unconformity between Middle Jurassic and Upper Jurassic series. The fault-controlled Early-Middle Jurassic basin accumulated thick sediments, evolving from alluvial fan to deep lacustrine deposits. The Late Jurassic-Cretaceous basin underwent a significant expansion, characterised by braided fluvial-delta and epeiric sea facies. Based on the inherited depocenters of the Jurassic and Cretaceous basin and the distinct tectonostratigraphic evolution history, we propose that the western Tarim basin evolved from a syn-rift stage to a post-rift stage during the Jurassic-Cretaceous.
(3) We discuss the close tempo-spatial relation between the Triassic fold-and-thrust belt and the ensuing Jurassic-Cretaceous extensional basin. And we propose that the post-orogenic stretch is a likely geodynamic mechanism for this major extension. This event highlights a significant tectonic switch from a contractional episode in the Triassic to an extensional deformation phase in the Jurassic-Cretaceous, and it could be associated with the coherent evolution of the Paleo-Tethyan orogenic belt in Central Asia.
Data Availability Statement
The original contributions presented in the study are included in the article/supplementary material, further inquiries can be directed to the corresponding author/s.
Author Contributions
HW performed conceptualization, investigation, methodology, and writing original draft. XC performed investigation and methodology. HC performed funding acquisition. CC, JS, and CZ performed project administration. YD performed final writing and edit. CL and WZ performed writing review and editing. YZ performed investigation. XL and FZ performed conceptualization, investigation, writing review and editing, and supervision. All authors contributed to the article and approved the submitted version.
Funding
This work was funded jointly by the Second Tibetan Plateau Scientific Expedition and Research of China (Grant No. 2019QZKK0708), the National Natural Science Foundation of China (Grant Nos. 41720104003, 41972217, 41972218, 42072233, and 41772205), the Major Science and Technology Projects of CNPC (Grant No. ZD2019-183-001), and the Fundamental Research Funds for the Central Universities of China (Grant Nos. 2019FZA3008 and 2019QNA3013).
Conflict of Interest
CC, JS, and CZ were employed by the Tarim Oilfield Company.
The remaining authors declare that the research was conducted in the absence of any commercial or financial relationships that could be construed as a potential conflict of interest.
Acknowledgments
We appreciate field cooperation with experts from Hangzhou Institute of Geology, PetroChina Exploration and Development Research Institute.
References
Allen, M. B., Windley, B. F., and Zhang, C. (1993). Palaeozoic collisional tectonics and magmatism of the Chinese Tienshan, central Asia. Tectonophysics 220, 89–115. doi: 10.1016/0040-1951(93)90225-9
Allen, P. A., and Allen, J. R. (2013). Basin Analysis: Principles and Application to Petroleum Play Assessment. New Jersey: Wiley-Blackwell.
Bell, R. E., Jackson, C. A. L., Elliott, G. M., Gawthorpe, R. L., Sharp, I. R., and Michelsen, L. (2014). Insights into the development of major rift-related unconformities from geologically constrained subsidence modelling: Halten Terrace, offshore mid Norway. Basin Res. 26, 203–224. doi: 10.1111/bre.12049
Bershaw, J., Garzione, C. N., Schoenbohm, L., Gehrels, G., and Tao, L. (2012). Cenozoic evolution of the Pamir plateau based on stratigraphy, zircon provenance, and stable isotopes of foreland basin sediments at Oytag (Wuyitake) in the Tarim Basin (west China). J. Asian Earth Sci. 44, 136–148. doi: 10.1016/j.jseaes.2011.04.020
Blayney, T., Najman, Y., Dupont-Nivet, G., Carter, A., Millar, I., Garzanti, E., et al. (2016). Indentation of the Pamirs with respect to the northern margin of Tibet: constraints from the Tarim basin sedimentary record. Tectonics 35, 2345–2369. doi: 10.1002/2016TC004222
Bosboom, R., Dupont-Nivet, G., Grothe, A., Brinkhuis, H., Villa, G., Mandic, O., et al. (2014). Linking Tarim Basin sea retreat (west China) and Asian aridification in the late Eocene. Basin Res. 26, 621–640. doi: 10.1111/bre.12054
Bosboom, R. E., Dupont-Nivet, G., Houben, A. J. P., Brinkhuis, H., Villa, G., Mandic, O., et al. (2011). Late eocene sea retreat from the Tarim Basin (west China) and concomitant Asian paleoenvironmental change. Palaeogeogr. Palaeoclimatol. Palaeoecol. 299, 385–398. doi: 10.1016/j.palaeo.2010.11.019
Brookfield, M. E., and Hashmat, A. (2001). The geology and petroleum potential of the North Afghan platform and adjacent areas (northern Afghanistan, with parts of southern Turkmenistan, Uzbekistan and Tajikistan). Earth Sci. Rev. 55, 41–71. doi: 10.1016/S0012-8252(01)00036-8
Bryan, S. E., and Ferrari, L. (2013). Large igneous provinces and silicic large igneous provinces: progress in our understanding over the last 25 years. Geol. Soc. Am. Bull. 125, 1053–1078. doi: 10.1130/B30820.1
Burtman, V. S. (2000). Cenozoic crustal shortening between the Pamir and Tien Shan and a reconstruction of the Pamir-Tien Shan transition zone for the Cretaceous and Palaeogene. Tectonophysics 319, 69–92. doi: 10.1016/S0040-1951(00)00022-6
Cao, K., Wang, G. C., Bernet, M., van der Beek, P., and Zhang, K. X. (2015). Exhumation history of the West Kunlun Mountains, northwestern Tibet: evidence for a long-lived, rejuvenated orogen. Earth Planet. Sci. Lett. 432, 391–403. doi: 10.1016/j.epsl.2015.10.033
Chapman, J. B., Scoggin, S. H., Kapp, P., Carrapa, B., Ducea, M. N., Worthington, J., et al. (2018). Mesozoic to Cenozoic magmatic history of the Pamir. Earth Planet. Sci. Lett. 482, 181–192. doi: 10.1016/j.epsl.2017.10.041
Charvet, J., Shu, L. S., Laurent-Charvet, S., Wang, B., Faure, M., Cluzel, D., et al. (2011). Palaeozoic tectonic evolution of the Tianshan belt, NW China. Sci. China Earth Sci. 54, 166–184. doi: 10.1007/s11430-010-4138-1
Chen, H. L., Luo, J. C., Guo, Q. Y., Liao, L., Xiao, Z. Y., Cheng, X. G., et al. (2009). Deformation history and tectonic evolution of southeastern Tarim Basin in Mesozoic and Cenozoic. Geotecton. Metallogen. 33, 38–45. doi: 10.16539/j.ddgzyckx.2009.01.009
Chen, X. H., Yin, A., Gehrels, G. E., Cowgill, E. S., Grove, M., Harrison, T. M., et al. (2003). Two phases of Mesozoic north-south extension in the eastern Altyn Tagh range, northern Tibetan Plateau. Tectonics 22:1053. doi: 10.1029/2001TC001336
Chen, Y. G., Wu, H. X., Zhang, L., Cheng, X. G., Chen, C., Zhang, Y. Q., et al. (2018). Characteristics of the late triassic paleo-structure in the mountain front region of western Kunlun and its control of Jurassic-Cretaceous. Chin. J. Geol. 53, 1405–1418. doi: 10.12017/dzkx.2018.082
Cheng, F., Jolivet, M., Guo, Z. J., Lu, H. Y., Zhang, B., Li, X. Z., et al. (2019). Jurassic-Early Cenozoic tectonic inversion in the Qilian Shan and Qaidam Basin, North Tibet: new insight from seismic reflection, isopach mapping, and drill core data. J. Geophys. Res. Solid Earth 124, 12077–12098. doi: 10.1029/2019JB018086
Cheng, X. G., Chen, H. L., Lin, X. B., Yang, S. F., Chen, S. Q., Zhang, F. F., et al. (2016). Deformation geometry and timing of the Wupoer thrust belt in the NE Pamir and its tectonic implications. Front. Earth Sci. 10, 751–760. doi: 10.1007/s11707-016-0606-z
Cheng, X. G., Chen, H. L., Shi, J., Liao, L., Du, Z. L., and Huang, Z. B. (2012). Distribution characteristics and controlling factors of Jurassic-Cretaceous in the front of West Kunlun mountains. Earth Sci. J. China Univers. Geosci. 37, 635–644. doi: 10.3799/dqkx.2012.073
Cheng, X. G., Wu, H. X., Li, Y., Chen, H. L., Zhang, F. Q., and Shi, J. (2019). Influences of Indosinian structures on later structural deformation and sedimentation in piedmont of Western Kunlun mountains. Xinjiang Petrol. Geol. 40, 27–33. doi: 10.7657/XJPG20190104
Cowgill, E., Yin, A., Harrison, T. M., and Wang, X. F. (2003). Reconstruction of the Altyn Tagh fault based on U-Pb geochronology: role of back thrusts, mantle sutures, and heterogeneous crustal strength in forming the Tibetan Plateau. J. Geophys. Res. Solid Earth 108:2346. doi: 10.1029/2002JB002080
De Grave, J., Glorie, S., Buslov, M. M., Stockli, D. F., McWilliams, M. O., Batalev, V. Y., et al. (2013). Thermo-tectonic history of the Issyk-Kul basement (Kyrgyz Northern Tien Shan, Central Asia). Gondwana Res. 23, 998–1020. doi: 10.1016/j.gr.2012.06.014
Ernst, R. E., Liikane, D. A., Jowitt, S. M., Buchan, K. L., and Blanchard, J. A. (2019). A new plumbing system framework for mantle plume-related continental Large Igneous Provinces and their mafic-ultramafic intrusions. J. Volcanol. Geotherm. Res. 384, 75–84. doi: 10.1016/j.jvolgeores.2019.07.007
Fan, J. J., Li, C., Xie, C. M., Wang, M., and Chen, J. W. (2015). The evolution of the Bangong-Nujiang Neo-Tethys ocean: evidence from zircon U-Pb and Lu-Hf isotopic analyses of Early Cretaceous oceanic islands and ophiolites. Tectonophysics 655, 27–40. doi: 10.1016/j.tecto.2015.04.019
Gaudemer, Y., Jaupart, C., and Tapponnier, P. (1988). Thermal control on post-orogenic extension in collision belts. Earth Planet. Sci. Lett. 89, 48–62. doi: 10.1016/0012-821X(88)90032-5
Gawthorpe, R. L., and Leeder, M. R. (2000). Tectono-sedimentary evolution of active extensional basins. Basin Res. 12, 195–218. doi: 10.1111/j.1365-2117.2000.00121.x
Ge, C. L. (2018). The Characteristics of the Western Extension of the Karakax Fault in West Kunlun and its Tectonic Implication. Master’s thesis, China University of Geosciences, Beijing.
Gillespie, J., Glorie, S., Xiao, W., Zhang, Z., Collins, A. S., Evans, N., et al. (2017). Mesozoic reactivation of the Beishan, southern Central Asian Orogenic Belt: Insights from low-temperature thermochronology. Gondwana Res. 43, 107–122. doi: 10.1016/j.gr.2015.10.004
Graham, S. A., Hendrix, M. S., Wang, L. B., and Carroll, A. R. (1993). Collisional successor basins of western China: impact of tectonic inheritance on sand composition. Geol. Soc. Am. Bull. 105, 323–344. doi: 10.1130/0016-7606
Guilbaud, C., Simoes, M., Barrier, L., Laborde, A., Van der Woerd, J., Li, H., et al. (2017). Kinematics of active deformation across the western Kunlun Mountain Range (Xinjiang, China) and potential seismic hazards within the southern Tarim Basin. J. Geophys. Res. Solid Earth 122, 10398–10426. doi: 10.1002/2017JB014069
Han, Y. G., and Zhao, G. C. (2018). Final amalgamation of the Tianshan and Junggar orogenic collage in the southwestern Central Asian Orogenic Belt: Constraints on the closure of the Paleo-Asian Ocean. Earth Sci. Rev. 186, 129–152. doi: 10.1016/j.earscirev.2017.09.012
Han, Y. G., Zhao, G. C., Sun, M., Eizenhofer, P. R., Hou, W. Z., Zhang, X. R., et al. (2016). Detrital zircon provenance constraints on the initial uplift and denudation of the Chinese western Tianshan after the assembly of the southwestern Central Asian Orogenic Belt. Sediment. Geol. 339, 1–12. doi: 10.1016/j.sedgeo.2016.03.028
Hao, Y. C., Zeng, X. L., and Guo, X. P. (1987). The marine cretaceous in the western part of the Tarim Basin of Xinjiang and its depositional environments. Acta Geol. Sin. 3, 205–217.
Hendrix, M. S., Graham, S. A., Carroll, A. R., Sobel, E. R., McKnight, C. L., Schulein, B. J., et al. (1992). Sedimentary record and climatic implications of recurrent deformation in the Tian Shan Evidence from Mesozoic strata of the north Tarim, south Junggar, and Turpan basins, northwest China. Geol. Soc. Am. Bull. 104, 53–79.
Huang, B. C., Piper, J. A., and Zhu, R. X. (2009). Paleomagnetic constraints on neotectonic deformation in the Kashi depression of the western Tarim Basin, NW China. Int. J. Earth Sci. 98, 1469–1488.
Jackson, C. A. L., Gawthorpe, R. L., Leppard, C. W., and Sharp, I. R. (2006). Rift-initiation development of normal fault blocks: insights from the Hammam Faraun fault block, Suez Rift, Egypt. J. Geol. Soc. 163, 165–183. doi: 10.1144/0016-764904-164
Jia, C. Z., and Wei, G. Q. (2002). Structural characteristics and petroliferous features of Tarim Basin. Chin. Sci. Bull. 47, 1–11. doi: 10.1007/BF02902812
Jia, C. Z., Zhang, S. B., and Wu, S. Z. (2004). Stratigraphy of the Tarim Basin and Adjacent Areas. Beijing: China Science Publishing House.
Jiang, X. D., and Li, Z. X. (2014). Seismic reflection data support episodic and simultaneous growth of the Tibetan Plateau since 25 Myr. Nat. Commun. 5:5453. doi: 10.1038/ncomms6453
Jiang, Y. H., Jia, R. Y., Liu, Z., Liao, S. Y., Zhao, P., and Zhou, Q. (2013). Origin of Middle Triassic high-K calc-alkaline granitoids and their potassic microgranular enclaves from the western Kunlun orogen, northwest China: a record of the closure of Paleo-Tethys. Lithos 156, 13–30. doi: 10.1016/j.lithos.2012.10.004
Jolivet, M. (2015). Mesozoic tectonic and topographic evolution of Central Asia and Tibet: a preliminary synthesis. Geol. Soc. 29:427. doi: 10.1144/SP427.2
Jolivet, M., Dominguez, S., Charreau, J., Chen, Y., Li, Y., and Wang, Q. (2010). Mesozoic and Cenozoic tectonic history of the central Chinese Tian Shan: Reactivated tectonic structures and active deformation. Tectonics 29:TC6019. doi: 10.1029/2010TC002712
Kay, R. W., and Kay, S. M. (1993). Delamination and delamination magmatism. Tectonophysics 219, 177–189. doi: 10.1016/0040-1951(93)90295-U
Käßner, A., Ratschbacher, L., Pfänder, J. A., Hacker, B. R., Zack, G., Sonntag, B. L., et al. (2017). Proterozoic-Mesozoic history of the Central Asian orogenic belt in the Tajik and southwestern Kyrgyz Tian Shan: U-Pb, Ar-40/Ar-39, and fission-track geochronology and geochemistry of granitoids. Geol. Soc. Am. Bull. 129, 281–303. doi: 10.1130/B31466.1
Krystopowicz, N. J., and Currie, C. A. (2013). Crustal eclogitization and lithosphere delamination in orogens. Earth Planet. Sci. Lett. 361, 195–207. doi: 10.1016/j.epsl.2012.09.056
Laborde, A., Barrier, L., Simoes, M., Li, H., Coudroy, T., Van der Woerd, J., et al. (2019). Cenozoic deformation of the Tarim Basin and surrounding ranges (Xinjiang: China): a regional overview. Earth Sci. Rev. 197:102891. doi: 10.1016/j.earscirev.2019.102891
Leech, M. L. (2001). Arrested orogenic development: eclogitization, delamination, and tectonic collapse. Earth Planet. Sci. Lett. 185, 149–159. doi: 10.1016/S0012-821X(00)00374-5
Leith, W. (1985). A mid-Mesozoic extension across Central Asia. Nature 313, 567–570. doi: 10.1038/313567a0
Li, G. W., Sandiford, M., Fang, A. M., Kohn, B., Sandiford, D., Fu, B. H., et al. (2019). Multi-stage exhumation history of the West Kunlun orogen and the amalgamation of the Tibetan Plateau. Earth Planet. Sci. Lett. 528:115833. doi: 10.1016/j.epsl.2019.115833
Li, J. H., Cai, Z. Z., Luo, C. S., and Geng, Y. H. (2007). The structural transfer at the southern end of Talas-Ferghana fault and its regional tectonic response in the Cenozoic. Acta Geol. Sin. 81, 23–31. doi: 10.3321/j.issn:0001-5717.2007.01.004
Li, S., Yin, C., Guilmette, C., Ding, L., and Zhang, J. (2019). Birth and demise of the Bangong-Nujiang Tethyan Ocean: a review from the Gerze area of central Tibet. Earth Sci. Rev. 198:102907. doi: 10.1016/j.earscirev.2019.102907
Li, T., Chen, Z. X., Chen, J., Thompson Jobe, J. A., Burbank, D. W., Li, Z. G., et al. (2019). Along-strike and downdip segmentation of the Pamir Frontal Thrust and its association with the 1985 M-w 6.9 Wuqia earthquake. J. Geophys. Res. Solid Earth 124, 9890–9919. doi: 10.1029/2019JB017319
Li, Y. J., Zhao, Y., Sun, L. D., Song, W. J., Zheng, D. M., Liu, Y. L., et al. (2013). Meso-Cenozoic extensional structures in the Northern Tarim Basin, NW China. Intern. J. Earth Sci. 102, 1029–1043. doi: 10.1007/s00531-012-0847-3
Li, Z., and Peng, S. (2010). Detrital zircon geochronology and its provenance implications: responses to Jurassic through Neogene basin-range interactions along northern margin of the Tarim Basin, Northwest China. Basin Res. 22, 126–138. doi: 10.1111/j.1365-2117.2009.00440.x
Li, Z., Song, W. J., Peng, S. T., Wang, D. X., and Zhang, Z. P. (2004). Mesozoic-Cenozoic tectonic relationships between the Kuqa subbasin and Tian Shan, northwest China: constraints from depositional records. Sediment. Geol. 172, 223–249. doi: 10.1016/j.sedgeo.2004.09.002
Li, Z. X., Bogdanova, S. V., Collins, A. S., Davidson, A., De Waele, B., Ernst, R. E., et al. (2008). Assembly, configuration, and break-up history of Rodinia: a synthesis. Precambr. Res. 160, 179–210. doi: 10.1016/j.precamres.2007.04.021
Liao, L., Cheng, X., Wang, B., Chen, H., Luo, J., and Shi, J. (2010). Reconstruction of Mesozoic sedimentary paleoenvironment in the southwestern Tarim Basin, Northwestern China. Acta Geol. Sin. 84, 1195–1207.
Liao, S. Y., Jiang, Y. H., Zhou, Q., Yang, W. Z., Jin, G. D., and Zhao, P. (2012). Geochemistry and geodynamic implications of the Triassic bimodal magmatism from Western Kunlun Orogen, northwest China. Intern. J. Earth Sci. 101, 555–577. doi: 10.1007/s00531-011-0686-7
Liu, D. L., Li, H. B., Sun, Z. M., Cao, Y., Wang, L. Z., Pan, J. W., et al. (2017). Cenozoic episodic uplift and kinematic evolution between the Pamir and Southwestern Tien Shan. Tectonophysics 712, 438–454. doi: 10.1016/j.tecto.2017.06.009
Liu, Z., Jiang, Y. H., Jia, R. Y., Zhao, P., and Zhou, Q. (2015). Origin of Late Triassic high-K calc-alkaline granitoids and their potassic microgranular enclaves from the western Tibet Plateau, northwest China: implications for Paleo-Tethys evolution. Gondwana Res. 27, 326–341. doi: 10.1016/j.gr.2013.09.022
Matte, P., Tapponnier, P., Arnaud, N., Bourjot, L., Avouac, J. P., Vidal, P., et al. (1996). Tectonics of Western Tibet, between the Tarim and the Indus. Earth Planet. Sci. Lett. 142, 311–330. doi: 10.1016/0012-821X(96)00086-6
Mattern, F., and Schneider, W. (2000). Suturing of the Proto- and Paleo-Tethys oceans in the western Kunlun (Xinjiang, China). J. Asian Earth Sci. 18, 637–650. doi: 10.1016/S1367-9120(00)00011-0
McKenzie, D. (1978). Some remarks on development of sedimentary basins. Earth Planet. Sci. Lett. 40, 25–32. doi: 10.1016/0012-821X(78)90071-7
Molnar, P., and Tapponnier, P. (1975). Cenozoic tectonics of Asia: effects of a continental collision. Science 189, 419–426. doi: 10.1126/science.189.4201.419
Otto, S. C. (1997). Mesozoic-Cenozoic history of deformation and petroleum systems in sedimentary basins of Central Asia: implications of collisions on the Eurasian margin. Petrol. Geosci. 3, 327–341. doi: 10.1144/petgeo.3.4.327
Peron-Pinvidic, G., and Osmundsen, P. T. (2020). From orogeny to rifting: insights from the Norwegian ‘reactivation phase’. Sci. Rep. 10:14860. doi: 10.1038/s41598-020-71893-z
Ratschbacher, L., Frisch, W., Neubauer, F., Schmid, S. M., and Neugebauer, J. (1989). Extension in compressional orogenic belts - the eastern Alps. Geology 17, 404–407. doi: 10.1130/0091-761319890172.3.CO;2
Ritts, B. D., and Biffi, U. (2001). Mesozoic northeast Qaidam basin: response to contractional reactivation of the Qilian Shan, and implications for the extent of Mesozoic intracontinental deformation in central Asia. Memoirs Geol. Soc. Am. 194, 293–316. doi: 10.1130/0-8137-1194-0.293
Robinson, A. C. (2015). Mesozoic tectonics of the Gondwanan terranes of the Pamir plateau. J. Asian Earth Sci. 102, 170–179. doi: 10.1016/j.jseaes.2014.09.012
Şengör, A. M. C., Yilmaz, Y., and Sungurlu, O. (1984). Tectonics of the Mediterranean Cimmerides: nature and evolution of the western termination of Palaeo-Tethys. Geol. Soc. Spec. Public. 17, 77–112. doi: 10.1144/GSL.SP.1984.017.01.04
Shi, X., Jiang, H., Yang, J., Yang, X., and Xu, H. (2017). Models of the rapid post-rift subsidence in the eastern Qiongdongnan Basin, South China Sea: implications for the development of the deep thermal anomaly. Basin Res. 29, 340–362. doi: 10.1111/bre.12179
Sobel, E. R. (1995). Basin Analysis and Apatite Thermochronology of the Jurassic-Paleogene Western Tarim basin. Thesis, Stanford University, Stanford.
Sobel, E. R. (1999). Basin analysis of the Jurassic-Lower Cretaceous southwest Tarim basin, northwest China. Geol. Soc. Am. Bull. 111, 709–724. doi: 10.1130/0016-76061999111<0709:BAOTJL>2.3.CO;2
Song, P., Ding, L., Li, Z., Lippert, P. C., Yang, T., Zhao, X., et al. (2015). Late Triassic paleolatitude of the Qiangtang block: implications for the closure of the Paleo-Tethys Ocean. Earth Planet. Sci. Lett. 424, 69–83. doi: 10.1016/j.epsl.2015.05.020
Stampfli, G. M., and Borel, G. D. (2002). A plate tectonic model for the paleozoic and Mesozoic constrained by dynamic plate boundaries and restored synthetic oceanic isochrons. Earth Planet. Sci. Lett. 196, 17–33. doi: 10.1016/S0012-821X(01)00588-X
Sun, J., Xiao, W., Windley, B. F., Ji, W., Fu, B., Wang, J., et al. (2016). Provenance change of sediment input in the northeastern foreland of Pamir related to collision of the Indian Plate with the Kohistan-Ladakh arc at around 47Ma. Tectonics 35, 315–338. doi: 10.1002/2015TC003974
Tang, G. J., Cawood, P. A., Wyman, D. A., Wang, Q., and Zhao, Z. H. (2017). Evolving mantle sources in postcollisional Early Permian-Triassic magmatic rocks in the heart of Tianshan Orogen (Western China). Geochem. Geophys. Geosyst. 18, 4110–4122. doi: 10.1002/2017GC006977
Tong, K., Li, Z., Zhu, L., Tao, G., Zhang, Y., and Yang, W. (2020). Fold-and-thrust deformation of the hinterland of Qilian Shan, northeastern Tibetan Plateau since Mesozoic with implications for the plateau growth. J. Asian Earth Sci. 198:104131. doi: 10.1016/j.jseaes.2019.104131
Uyeda, S., and Kanamori, H. (1979). Back-arc opening and the mode of subduction. J. Geophys. Res. 84, 1049–1061. doi: 10.1029/JB084iB03p01049
Wang, C., Cheng, X. G., Chen, H. L., Li, K., Fan, X. G., and Wang, C. Y. (2016a). From folding to transpressional faulting: the Cenozoic Fusha structural belt in front of the Western Kunlun Orogen, northwestern Tibetan Plateau. Intern. J. Earth Sci. 105, 1621–1636. doi: 10.1007/s00531-016-1305-4
Wang, C., Liu, L., Korhonen, F., Yang, W. Q., Cao, Y. T., He, S. P., et al. (2016b). Origins of Early Mesozoic granitoids and their enclaves from West Kunlun, NW China: implications for evolving magmatism related to closure of the Paleo-Tethys ocean. Intern. J. Earth Sci. 105, 941–964. doi: 10.1007/s00531-015-1220-0
Watson, M. P., Hayward, A. B., Parkinson, D. N., and Zhang, Z. M. (1987). Plate tectonic history, basin development and petroleum source rock deposition onshore china. Mar. Petrol. Geol. 4, 205–225. doi: 10.1016/0264-8172(87)90045-6
White, R., and McKenzie, D. (1989). Magmatism at rift zones: the generation of volcanic continental margins and flood basalts. J. Geophys. Res. 94, 7685–7729. doi: 10.1029/JB094iB06p07685
Wilmsen, M., Fürsich, F. T., Seyed-Emami, K., and Majidifard, M. R. (2009). An overview of the stratigraphy and facies development of the Jurassic System on the Tabas Block, east-central Iran. Geol. Soc. Lond. Spec. Public. 312:323. doi: 10.1144/SP312.15
Windley, B. F., Alexeiev, D., Xiao, W. J., Kröner, A., and Badarch, G. (2007). Tectonic models for accretion of the Central Asian Orogenic Belt. J. Geol. Soc. Lond. 164, 31–47. doi: 10.1144/0016-76492006-022
Windley, B. F., Allen, M. B., Zhang, C., Zhao, Z. Y., and Wang, G. R. (1990). Paleozoic accretion and Cenozoic redeformation of the Chinese Tien Shan Range, central Asia. Geology 18, 128–131.
Wu, C., Yin, A., Zuza, A. V., Zhang, J., Liu, W., and Ding, L. (2016). Pre-Cenozoic geologic history of the central and northern Tibetan Plateau and the role of Wilson cycles in constructing the Tethyan orogenic system. Lithosphere 8, 254–292. doi: 10.1130/L494.1
Wu, H. X. (2018). Characteristics of Paleostructure in the Mountain Front Region of Southwestern Tarim Basin and its Control of Jurassic-Cretaceous Deposition. Master’s thesis, Zhejiang University, Hangzhou.
Xi, D., Cao, W., Cheng, Y., Jiang, T., Jia, J., Li, Y., et al. (2016). Late Cretaceous biostratigraphy and sea-level change in the southwest Tarim Basin. Palaeogeogr. Palaeoclimatol. Palaeoecol. 441, 516–527. doi: 10.1016/j.palaeo.2015.09.045
Xiao, W. J., Han, F. L., Windley, B. F., Yuan, C., Zhou, H., and Li, J. L. (2003). Multiple accretionary orogenesis and episodic growth of continents: Insights from the Western Kunlun Range, central Asia. Intern. Geol. Rev. 45, 303–328. doi: 10.2747/0020-6814.45.4.303
Xiao, W. J., Windley, B. F., Chen, H. L., Zhang, G. C., and Li, J. L. (2002). Carboniferous-Triassic subduction and accretion in the western Kunlun, China: Implications for the collisional and accretionary tectonics of the northern Tibetan Plateau. Geology 30, 295–298.
Xiao, W. J., Windley, B. F., Liu, D. Y., Jian, P., Liu, C. Z., and Yuan, C. (2005). Accretionary tectonics of the Western Kunlun Orogen, China: a Paleozoic-early Mesozoic, long-lived active continental margin with implications for the growth of southern Eurasia. J. Geol. 113, 687–705. doi: 10.1086/449326
Xiao, W. J., Windley, B. F., Sun, S., Li, J. L., Huang, B. C., Han, C. M., et al. (2015). A Tale of amalgamation of three Permo-Triassic collage systems in central Asia: Oroclines, sutures, and terminal accretion. Annu. Rev. Earth Planet. Sci. 43, 477–507. doi: 10.1146/annurev-earth-060614-105254
Xu, Z. Q., Qi, X. X., Yang, J. S., Ji, S. C., Li, H. B., and Chen, F. Y. (2007). Senses and timings of two kinds of shear in the Kangxiwar strike- slip shear zone, West Kunlun, and their tectonic significance. Geol. Bull. China 26, 1252–1261. doi: 10.1016/S1872-5791(07)60044-X
Yang, J. S., Robinson, P. T., Jiang, C. F., and Xu, Z. Q. (1996). Ophiolites of the Kunlun mountains, China and their tectonic implications. Tectonophysics 258, 215–231. doi: 10.1016/0040-1951(95)00199-9
Yang, W., Guo, Z. J., Jiang, Z. X., Gloria, H., Zhang, C., and Wang, S. W. (2017). Jurassic-Cretaceous basin-range pattern in the southwestern Tian Shan foreland basin: evidence from detrital U-Pb zircon geochronology. Geotecton. Metallogen. 41, 533–550. doi: 10.16539/j.ddgzyckx.2017.03.008
Yang, Y. T., Guo, Z. X., and Luo, Y. J. (2017). Middle-Late Jurassic tectonostratigraphic evolution of Central Asia, implications for the collision of the Karakoram-Lhasa Block with Asia. Earth Sci. Rev. 166, 83–110. doi: 10.1016/j.earscirev.2017.01.005
Yin, A., and Harrison, T. M. (2000). Geologic evolution of the Himalayan-Tibetan orogen. Annu. Rev. Earth Planet. Sci. Lett. 28, 211–280. doi: 10.1146/annurev.earth.28.1.211
Zanchi, A., Zanchetta, S., Berra, F., Mattei, M., Garzanti, E., Molyneux, S., et al. (2009). The Eo-Cimmerian (Late? Triassic) orogeny in North Iran. Geol. Soc. 312:31. doi: 10.1144/SP312.3
Zhang, C. L., Zou, H. B., Ye, X. T., and Chen, X. Y. (2018). Timing of subduction initiation in the Proto-Tethys ocean: evidence from the Cambrian gabbros from the NE Pamir Plateau. Lithos 31, 40–51. doi: 10.1016/j.lithos.2018.05.021
Zhang, C. S., Xiao, A. C., Li, J. Y., and Shi, D. (2000). Depositional feature Jurassic fault basin in Southwest Tarim depression. J. Mineral. Petrol. 20, 41–45. doi: 10.19719/j.cnki.1001-6872.2000.03.008
Zhang, F. Q., Dilek, Y., Cheng, X. G., Wu, H. X., Lin, X. B., and Chen, H. L. (2019). Late Neoproterozoic-early Paleozoic seismic structure-stratigraphy of the SW Tarim Block (China), its passive margin evolution and the Tarim-Rodinia breakup. Precambr. Res. 334:105456. doi: 10.1016/j.precamres.2019.105456
Zhang, S. J., Hu, X. M., Han, Z., Li, J., and Garzanti, E. (2018). Climatic and tectonic controls on Cretaceous-Palaeogene sea-level changes recorded in the Tarim epicontinental sea. Palaeogeogr. Palaeoclimatol. Palaeoecol. 501, 92–110. doi: 10.1016/j.palaeo.2018.04.008
Zhang, Y., Niu, Y. L., Hu, Y., Liu, J. J., Ye, L., Kong, J. J., et al. (2016). The syncollisional granitoid magmatism and continental crust growth in the West Kunlun Orogen, China - Evidence from geochronology and geochemistry of the Arkarz pluton. Lithos 245, 191–204. doi: 10.1016/j.lithos.2015.05.007
Zhang, Z. L., and Sun, J. M. (2020). Cenozoic tectonic rotations in different parts of the NE Pamir: implications for the evolution of the arcuate orogeny. Intern. J. Earth Sci. 109, 1921–1939. doi: 10.1007/s00531-020-01880-2
Zheng, J., and Dai, H. (2018). Subduction and retreating of the western Pacific plate resulted in lithospheric mantle replacement and coupled basin-mountain respond in the North China Craton. Sci. China Earth Sci. 61, 406–424. doi: 10.1007/s11430-017-9166-8
Zhou, X. Y., Luo, J. H., and Mai, G. R. (2005). Structural Features and Petroleum Geology of Kashi Sag and its Adjacent Area in Western Tarim Basin. Beijing: Petroleum Industry Press.
Zhu, D. C., Zhao, Z. D., Niu, Y., Dilek, Y., Hou, Z. Q., and Mo, X. X. (2013). The origin and pre-Cenozoic evolution of the Tibetan Plateau. Gondwana Res. 23, 1429–1454. doi: 10.1016/j.gr.2012.02.002
Keywords: western Tarim Basin, Triassic thrust, Early-Middle Jurassic rift, Cretaceous depression, tectonic switch, Paleo-Tethys
Citation: Wu H, Cheng X, Chen H, Chen C, Dilek Y, Shi J, Zeng C, Li C, Zhang W, Zhang Y, Lin X and Zhang F (2021) Tectonic Switch From Triassic Contraction to Jurassic-Cretaceous Extension in the Western Tarim Basin, Northwest China: New Insights Into the Evolution of the Paleo-Tethyan Orogenic Belt. Front. Earth Sci. 9:636383. doi: 10.3389/feart.2021.636383
Received: 01 December 2020; Accepted: 23 February 2021;
Published: 16 March 2021.
Edited by:
Renqi Lu, Institute of Geology, China Earthquake Administration, ChinaReviewed by:
Matias Ghiglione, University of Buenos Aires, ArgentinaDi Li, China University of Geosciences, China
Copyright © 2021 Wu, Cheng, Chen, Chen, Dilek, Shi, Zeng, Li, Zhang, Zhang, Lin and Zhang. This is an open-access article distributed under the terms of the Creative Commons Attribution License (CC BY). The use, distribution or reproduction in other forums is permitted, provided the original author(s) and the copyright owner(s) are credited and that the original publication in this journal is cited, in accordance with accepted academic practice. No use, distribution or reproduction is permitted which does not comply with these terms.
*Correspondence: Xiubin Lin, xiubin_lin@zju.edu.cn; linxiubin1984@163.com; Fengqi Zhang, zhangfq78@zju.edu.cn