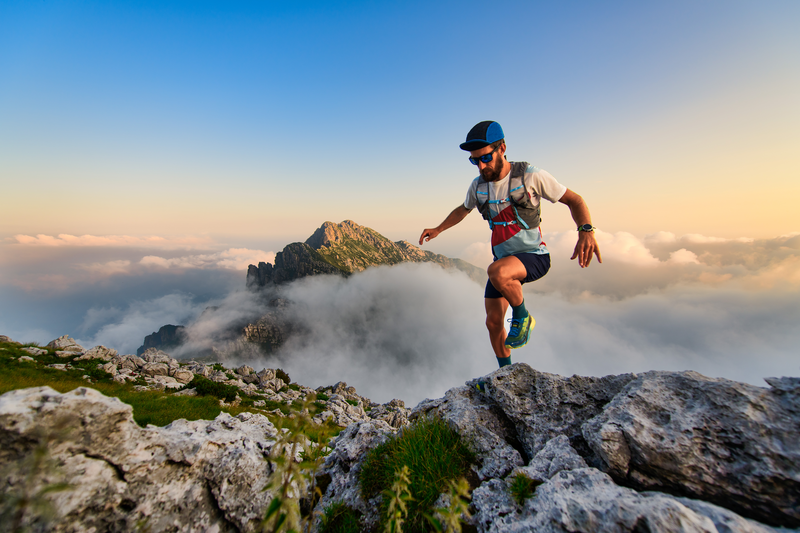
94% of researchers rate our articles as excellent or good
Learn more about the work of our research integrity team to safeguard the quality of each article we publish.
Find out more
ORIGINAL RESEARCH article
Front. Earth Sci. , 05 May 2021
Sec. Paleontology
Volume 9 - 2021 | https://doi.org/10.3389/feart.2021.635179
This article is part of the Research Topic Permian Extinctions View all 18 articles
The end-Permian extinction event (EPEE) considered to have been caused by the eruption of the Siberian Large Igneous Province (SLIP), the age of which is critical for extinction-SLIP model evaluation. The Tunguska Basin flora during this time, in accordance with the EPEE model, supposed to have been killed by the massive injection into the atmosphere of poisonous substances such as methane, sulfates, mercury and massive combastion of coals. In addition, supposed numerous fires presumably devastated the regional flora. However, the diversity of the Tunguska Basin flora drasticly increased at the beginning of Induan or slightly earlier and become diverse at the species level in the Olenekian and Anisian, when the main phase of basalt eruption and associated intrusive activity occurred. The overall magmatic activity during the latest Permian and Early Triassic did not kill the flora, but rather stimulate their diversity. The geomagnetic secular variations from the intrusions revealed the similarity of paleomagnetic directions of the Norilsk group layered intrusions with those of the upper Olenekian and lower Anisian Mokulaev and Kharaelakh volcanic formations and intrusions of the Talnakh group with the Olenekian Moronga-Mokulaev formations. The U-Pb dates and the geomagnetic secular variations data expose the obvious discrepancy between these two datasets. The paleomagnetic data suggest that the Norilsk-1 intrusion is younger than the Talnakn and Kharaelakh intrusions, but the U-Pb dates indicate the opposite. The data from layered intrusions in Norilsk and the other regions suggest their prolonged duration and multi-stadial formation. The U-Pb dates from the intrusions of the Norilsk region roughly constrain the onset of the SLIP and generally postdate the end-Permian extinction.
Recent advances in zircon CA-IDTIMS dating resolve many important geological problems especially those dealing with the rates of different geological and biological processes. One such problem is the end-Permian extinction event (EPEE) that has been widely debated since the recognition of this event (Newell, 1963). Myriad hypotheses regarding the scale and causes of the extinction have been and continue to be suggested e.g. (Hallam and Wignall, 1997; Bond and Grasby, 2017 and references therein). The current commonly accepted model links the EPEE to the magmatic activity that produced the Siberian Large Igneous Province (SLIP), because of the its enormous scale and supposed coincidence of the SLIP magmatism with the extinction in South China (Campbell et al., 1992; Svensen et al., 2009; Shen et al., 2011; Ernst and Youbi, 2017). The model become even more popular when CA-IDTIMS (Chemical Abrasion Isotope Dilution Ionization Mass Spectrometry) dates were obtained from the intrusions and sills in the SLIP region (Burgess and Bowring, 2015). These dates suggested to confirm the coincidence of the SLIP magmatism in the Tunguska Basin with the onset of the marine extinction in South China (Burgess and Bowring, 2015). The latest Permian-Early Triassic magmatism that created the Siberian traps, including the products of the explosion and interaction of the magmas with regional volcanic and sedimentary rocks (coal, evaporite and sulfates), is now considered by many authors to be the main driving force of the EPEE (Hoenisch et al., 2012; Bond and Wignall, 2014; Sobolev et al., 2015; Bond and Grasby, 2017; Burgess et al., 2017; Rothman 2017; Ernst et al., 2021, in press). This extinction model suggests that the large scale of the SLIP volcanic explosions and intrusive/sill emplacement within the Tunguska Basin released large volumes of CO2, but also induced metamorphism of the sedimentary succession surrounding the intrusive and sills, that released sediment-derived hazardous volatiles into the atmosphere through the numerous pipe and vent structures throughout the basin (Svensen et al., 2009; Black et al., 2021, in press). According to recent investigations the intrusive/sills contact metamorphism generated 4.0–9.2 times more CO2 compared with the expected normal degassing of sills (mantle CO2) (Retallack and Jahren, 2008; Svensen et al., 2018). Therefore, the time frame of the SLIP intrusive magmatism relative to the age of the EPEE is very critical for this model evaluation. At the same time the assessment of the impact of the SLIP on the environments and biota mostly focused on the geochemical proxies (Black et al., 2021, in press; Mather and Schmidt 2021, in press). The radioisotopic dates from the Permian-Triassic transition in the Tunguska Basin have never been integrated with the palaeontologic, biostratigraphic, and lithostratigraphic data. The existing sources (published and unpublished) are reviewed and analyzed in this paper to investigate the claims that the Siberian Traps are contemporaneous with the end-Permian extinction. In this paper we are assessing and analyzing paleontological, biostratigraphic, lithostratigraphic and magnetostratigraphic data within a framework of the intrusive/sill emplacement in the entire Tunguska Basin. The floral richness and dynamics of origination and extinction were obtained and analyzed to understand the regional floral evolutionary processes and the relationship of these processes with Siberian Traps magmatism during the Permian-Triassic transition in the Tunguska Basin.
Tunguska Basin occupied the central and western parts of Siberian Platform and consists of Norilsk through, Tunguska syneclise and western part of the Angara anteclise. The basin bounded in east with the Anabar anteclise and with Baikit and Nepsko-Botuoba anteclises in the south. The Tunguska Basin is one of the largest reserves of coal in the world. It is filled with Upper Proterozoic, and Phanerozoic sediments of total thickness 3.5–8.5 km (Kontorovich et al., 1994). The late Paleozoic Siberian coal-bearing deposits unconformably overlie the marine to marginal marine sabkha evaporates, carbonate and siliciclastic sediments of lower-middle Paleozoic and Mississippian age. Most of the Tunguska Basin coal-bearing strata are unconformably overlain by the Triassic volcanics. In some areas, the Triassic rocks variably overlie Ordovician, Pennsylvanian, Lower, Middle and Upper Permian deposits. Only to the northeast and in central parts of the basin (Norilsk and Tura in Nizhnyaya Tunguska areas) the Triassic rocks resting on the Upper Permian with minimal unconformity (Kovrigina, 2000; Cherepovskiy, 2001).
The Norilsk region, where the Permian-Triassic transition is essentially complete (Kazakov, 2002), occurs on the northern edge of the Siberian Platform (Figure 1A). It is bounded on the west and north by the Yenisei–Khatanga troughs. This trough is characterized by the increased mobility throughout the history of development with a deep structure characteristic of riftogenic systems. The trough is separated by mantle faults from the Tunguska and Taymir blocks, which have a common platform structure (Afanasenkov et al., 2016). This individualized tectonic continental crustal block of lower thickness consists of a crystalline basement and sedimentary–volcanic cover (Figure 1B). A series of positive and negative structures and major fault zones dominate in the Norilsk region (Figures 1B,C). Important from the point of view of mineralization are the Norilsk- Kharaelakh and Imangda faults, which both are NNE-trending, and the North Kharaelakh fault, which forms the southern boundary of the Yenisei–Khatanga trough. Seismic evidence indicates that these major faults extend to the base of the crust (Krivolutskaya 2016).
FIGURE 1. Location and position of the Norilsk region within the geologic, stratigraphic and structural content. (1) - Geologic map of the north-western corner of Siberian Platform (from Petrov, 2016). (2) - Cross-section of the northern Siberian Platform along the line Dikson city (A) – Khantai lake (B) - white dashed line on Figure 1A [modified from Afanasenkov et al. (2016)]; (3) - Simplified geologic map of Norilsk region with main layered intrusions, from which Burgess and Bowring (2015) obtained the U-Pb ages [modified from Krivolutskaya (2016), Rad’ko (2016) Dark blue boxes - samples of Latyshev et al., 2020].
The uppermost Permian in the Norilsk region (Ivakinian Regional Stage [RS] of Siberia) is assigned to the Ivakin Formation (Figure 2) (Budnikov et al., 2020). The formation includes titanium-augite trachybasalts, trachy-andesite basalts, labrador porphyrite, tuffs, tuffites, agglomerate tuffs and tuff breccias. Sandstones, gravel conglomerates, and carbonaceous siltstone occur in subordinate quantities within basaltic trachy-andesites. Basalts form several lava flows within the formation. The total thickness of the Ivakin Formation varies from 30 up to 350 m (Kovrigina 2000; Paderin et al., 2016). The plants Todites evenkensis Radczenko, Cordaites insignis (Radczenko) S. Meyen, Javorskia mungatica Radczenko, Сarpolithus candalepensis (Zalessky), S. Meyen, Pecopteria tajmyrensis Schwedov, and Paracalamites sp. suggest the Permian age of this formation (Meyen, 1966; Sadovnikov, 1987b; Mogucheva and Naugolnykh, 2010). The Ivakin Formation correlates with the upper Tailugan and lower Maltsev formations of the Kuznetsk Basin the late Permian ages of which recently were established with CA-IDTIMS dates (Davydov et al., 2021). The Ergalakh intrusive sill complex (Figure 3) has been proposed to be a feeder of the volcanism of the Ivakin Formation (Ryabov et al., 2001; Rad'ko, 2016; Latyshev et al., 2019).
FIGURE 2. Correlation of Permian-Triassic transition of Norilsk region with the International Geologic Time Scale (IGTS) (Henderson et al., 2012). White dashed lines - correlation according to regional flora and fauna. The data on the paleomagnetic (P/M) analysis of the geomagnetic secular variations recorded in the intrusions and their correlation with the Siberian Traps volcanic sequences in Norilsk region is modified from Latyshev et al. (2020); the paleomagnetic data combined with the CA-IDTIMS U-Pb dates obtained from Norilsk group intrusions (Norilsk-1, and Chernogorsky-1) and from Talnakh and Kharaelakh intrusions are from Burgess and Bowring (2015). Distribution of coals from Cherepovskiy (2001); distribution of traps and their thickness and the chroniostratigraphic position of sills and intrusions in left column (1) according to Krivolutskaya (2016), Rad’ko (2016), Ryabov et al. (2014) and in right column (2) by Burgess and Bowring (2015).
FIGURE 3. Chronostratigraphic summary of high-precision U–Pb dating results of zircon from intrusive rocks of the mixed superposition (cross-cutting intrusive layers) at Norilsk-1 intrusion and Neoarchean Stillwater Complex of Montana. (A), distribution of U–Pb ages in well G22, (Distler et al., 1999) Norilsk-1 intrusion [data from Burgess and Bowring (2015)] (B), Stillwater Complex (Wall et al., 2018); (C), the relationship of Norilsk and Ergalakh intrusive complexes in Kuramakit area (modified from Sereda et al., 2020), where the younger Norilsk intrusive complex intruded within and underneath the older Ergalakh complex.
In the Tunguska Basin, the Lower Triassic volcanic and volcaniclastic successions known as Tutonchana Fm in most cases unconformably overlies units of the uppermost Permian Ivakinian RS and the older sediments (Kazakov, 2002; Paderin et al., 2016). The chronostratigraphic Tutonchanian RS, correlates with the Induan of the International Geologic Time Scale (Figure 2). A significant turnover in the biota (flora, bivalves, conchostracans, ostracods, fishes) occurs at the boundary between the Ivakinian and Tutonchanian RS (Ragozin, 1958; Betekhtina et al., 1988; Sadovnikov, 2008; Mogucheva and Naugolnykh, 2010). The wet-dominated cordaites forest disappeared across this boundary and was replaced by dry-dominated conifer-fern flora. The turnover is interpreted as a climate shift from the cool and wet into warmer and drier (Dobruskina, 1994; Meyen, 1997). In the Norilsk region the lower Tutonchanian RS (Syvermin Formation) contains only the fern Cladophlebis sp., but biota in the middle and upper Tutonchanian is more abundant and includes the plantsPecopteris julii Radczenko, Cladophlebis kirjamkensis Prynada, Cladophlebis adnata (Goeppеrt), Cladophlebis denticulata Brongniart, Cladophlebis gorbiatchiana Mogutcheva, Cladophlebis dogaldensis Mogutcheva, Neokoretrophyllites linearis (Prynada), Schizoneura altaica Vladimirovich et Radczenko, Paracalamites triassica Radczenko, Pecopteris pseudotchichatchevii Vladimirovich, Tungussopteris sphenopteroides Vladimirovich, Katasiopteris oblongata Vladimirovich, and Taeniopteris prynadae Mogutchev; the non-marine ostracodsDarwinula regia Mischina, D. postparallela Mischina); the conchostracansRohdendorfium (Bipemphigus) gennisi (Novozhilov), Cyclotunguzites gutta (Lutkevich), Concherisma tomiensis Novojilov, Estherites evenkensis Lutkevich, E. tungussensis Lutkevich, and Lioestheria aequele (Lutkevich); and the non-marine bivalvesUtschamiella tungussica Ragozin, U. babikamensis Ragozin, and U. obrutschevi Ragozin (Ragozin, 1958; Mogucheva, 1973; Kazakov, 2002; Sadovnikov, 2008, Sadovnikov, 2016). Conchostracan Cyclotunguzites gutta is the most diagnostic species in this assemblage as it is found in many regions in the lowermost Triassic, i.e., in the Induan Calvörde Formation, Lower Buntsandstein, Germanic basin, in the lower Vokhmian RS of the East European Paltform (slightly above Induan tetrapod Tupilakosaurus), in the upper Kayitou Formation in South Chin and Sunjiagou Formation in North China (Chu et al., 2019; Davydov et al., 2020; Scholze et al., 2020). This taxon is considered as the index of the Induan Stage in continental facies (Schneider et al., 2019). The Tutonchanian RS in the Central Tunguska Basin and Kuznetsk Basin is characterized by a similar flora and fauna and directly correlates with the Tutonchanian of the Norilsk region (Vladimirovich, 1967; Betekhtina et al., 1986; Dobruskina, 1994; Sadovnikov, 2008; Mogucheva, 2016; Davydov et al., 2021).
We compiled the data on the floral distribution within the Norilsk and Nizhnyaya Tunguska areas from numerous sources, including publications by the specialists on flora working in Siberia (Radchenko and Schwedov, 1940; Schwedov, 1961, Schwedov, 1963; Meyen, 1966; Sadovnikov, 1967; Prinada, 1970; Mogucheva, 1973; Sadovnikov, 1987a, Sadovnikov, 1987b; Porokhovichenko, 2006; Sadovnikov, 2008; Mogucheva and Krugovykh, 2009; Mogucheva and Naugolnykh, 2010; Mogucheva, 2016) and adjust taxonomy towards the recent systematics. Besides, we analyzed and unified the occurrences of flora in different stratigraphic units (formations) with respect to the detailed local stratigraphy and chronostratigraphy. The obtained floral richness in comparison with trap thickness is illustrated in Figure 4. The recent secular variation of paleomagnetic data from the Permian and Triassic for the first time suggested the direct correlation of intrusive and extrusive rocks in the Tunguska Basin (Latyshev et al., 2020).
FIGURE 4. The taxonomic richness and dynamics of the extinction and origination of flora in Norilsk and Kuznetsk Basins calculated using functions implemented in the R-package divDyn (Kocsis et al., 2019). A similar pattern of these parameters is observed in both regions. The floral richness and species origination in the Tunguska and Kuznetsk basins roughly correpsponds with the onset of the mafic magmatism (Late Permian) and climatic optimum in both regions.
Traditionally, the Permian-Triassic boundary in the Tunguska Basin has been placed at the base of the Tutonchanian RS because of the most drastic sedimentologic and biotic change in the Permian-Triassic of Siberia (Vladimirovich, 1967; Prinada, 1970; Betekhtina et al., 1984; Betekhtina et al., 1986; Dobruskina, 1994; Sadovnikov, 2008; Mogucheva, 2016). This position of the boundary was utilized in the all of the official geologic maps in the Tunguska Basin of Russia (Kovrigina, 2000; Permyakov et al., 2012; Paderin et al., 2016; Lipenkov et al., 2018; Varganov et al., 2018).
The CA-IDTIMS U-Pb dates were obtained from the layered intrusions and sills in Tunguska Basin and Taymir (Figures 1–3). Seventeen sill/intrusion samples within the Tunguska Basin yielded dates ranging from 251.813 ± 0.065 to 251.354 ± 0.088 Ma (Burgess and Bowring, 2015). Although Burgess and Bowring (2015) claimed that the eruptions were before and during the mass extinction, most of the obtained ages with the uncertainties postdate the onset of the extinction at 251.941 Ma established in South China (Burgess et al., 2014). The oldest range of the uncertainty from the sample G22-63 from the Norilsk intrusion 251.907 ± 0.067 Ma slightly overlaps the onset of the extinction determined in South China (Burgess 2014). Two U-Pb dates, sample KZ1799-1195, 251.801 ± 0.088 Ma from the Talnakh intrusion and sample G22-65, 251.813 ± 0.065 Ma from the Norilsk intrusion, (Distler et al., 1999) slightly overlap the cessation of the extinction as determined in South China (Burgess et al., 2014). In addition to the lack of the Permian radioisotopic ages for the Tunguska Basin sills and intrusions, the relationship of the intrusive rock with the Triassic volcano-sedimentary succession in the region was assumed but has never been precisely constrained. (Paderin et al., 2016; Rad'ko, 2016). According to the data of Burgess and Bowring (2015), most of the studied sills and intrusions are of Induan (Early Triassic) in age, whereas all traps were placed in the Permian (Figure 2, column 2, see also Figure 3 in Burgess and Bowring, 2015). The latter would mean that an extensive pre-extrusive feeder intrusive-sills system of the of Permian age must be existed in the region, but that one is unknown. Only the small Ergalakh intrusive complex, which is developed around the Norilsk area, has been proposed to be a potential feeder for the latest Permian Ivakin Fm, because of the cross-cutting relationship with the Paleozoic rocks only (Kovrigina, 2000; Ryabov et al., 2001). The scale of the Ergalakh intrusive complex within the entire Tunguska Basin is minimal.
The cross-cutting relationships, petrology, and geochemistry of most of the intrusions in the Norilsk region suggest co-magmatic relationships of the intrusive and extrusive rocks (Zolotukhin et al., 1986; Zolotukhin and Al'Mukhamedov, 1991; Ryabov et al., 2014; Krivolutskaya, 2016). Therefore, according to these data most of the traps are supposed to be Triassic in age. A recent study of the mean geomagnetic directions of the Norilsk group layered intrusions (Norilsk 1, Norilsk 2, and Chernogorsky; Figures 1–3) and Talnakh group of layered intrusions (Talnakh, Oganer, and Zayachiy Creek) disclose their similarity to the geomagnetic signature of the upper Olenekian – lower Anisian Mokulaev (4.3°–5.9°) and Kharaelakh formations (2.9°–4.9°) of Anisian age (Figure 2). At the same time paleomagnetic directions of the intrusions of the Talnakh group of layered intrusions (Talnakh, Oganer, and Zayachiy Creek) reveal the lowest angular differences with the Olenekian Moronga-Mokulaev formations (2.1°–3.5°) (Figure 2) (Latyshev et al., 2020). This difference between formations and intrusions suggest the comagmatic emplacement of these lavas and intrusions as previously suggested by the cross-cutting relationship and the geochemistry (Ryabov et al., 2014; Krivolutskaya, 2016) (Figure 2).
The U-Pb dates from the intrusions (Burgess and Bowring, 2015) integrated with the geomagnetic secular variation data (Figure 2, column 1), manifest the discrepancy between these two datasets especially with regards to the succession of the extrusive rocks and radioisotopically dated intrusives (Davydov, 2021 in press). The geomagnetic secular variation was measured in the open pit (Medvezhyi Creek), the underground Skalistyi and Oktyabrskyi mines and on several natural outcrops (Figure 1C) (Latyshev et al., 2020), whereas U-Pb dates were obtained from the wells at different location (Figure 1C) (Burgess 2014), and the correlation between these two datasets (geomagnetic secular variation and U-Pb dates), except when that they were collected from several multi-layered intrusive bodies, is not possible (Figure 1C).
Radioisotopic U-Pb ages in well G22 in the Norilsk-1 intrusion occur in the opposite direction of the supposed stratigraphy in the well, i.e. the oldest age from sample G22-63-5 occurs near the top and the youngest age from sample G22-105-2 is near the bottom (Davydov, 2021 in press). This suggests a complicated internal structure of the multilayers and their different origin during at least a half-million or more years (Figure 3A). A similar case was reported recently in the Norilsk region, where the Norilsk-type layered intrusive has been intruded into the Ergalakh intrusive complex (considered to be late Permian) and thus the latter is interlayered with the former timewise in an upside-down position (Figure 3C) (Sereda et al., 2020). All this suggests that to recognize the true age of the geomagnetic secular variation, their measurements and the radioisotopic dating must be obtained from the same samples.
The layered intrusions from the other regions are similar in their internal structure, i.e. Precambrian layered intrusions of southern Montana (Figure 3B), where some layers were constructed out of stratigraphic superposition (Wall et al., 2018). This is certainly similar to the 0.3–0.4 Myr case in the Norilsk-1 cross-cut layered intrusive, which was crystallized in multiple phases over a timeframe of about half a million years, and the timing and nature of interaction with the surrounding sedimentary rocks was much more complicated than proposed in recent models (Svensen et al., 2018). A comprehensive radioisotopic calibration in the main multi-layered intrusive complexes in Tunguska Basin is required to prove the link between intrusive magmatism in the region with the end-Permian mass extinction in South China and elsewhere.
Three more CA-ID-TIMS dates from the intrusions in the Taymir Peninsula, northern Siberia, were reported recently. All of them are yielded the Triassic ages: TP-55 - 251.64 ± 0.11; TP-42 - 251.46 ± 0.13 and TP-43 - 250.60 ± 0.22 (Augland et al., 2019). The latter sample reveals a non-overlapping clustering in the age distribution, whereas the older cluster possessing a large uncertainty 251.67 ± 0.41, the maximum age of which slightly overlapped with the end-Permian extinction. This cluster is interpreted to represent initial crystallization in the magma system and probably emplacement of an early pulse of magma. The younger cluster represent the final emplacement and crystallization of the monzonite-diorite horizon within the Dumtalei layered intrusive complex (Augland et al., 2019). These data are quite consistent with the data from the Tunguska Basin (Burgess and Bowring, 2015), but also suggest generally a post-extinction age of the intrusive magmatism in Taymir. Besides, at least two (or more) magmatic crystallization events (magma pulses) are proposed in the Dumtalei layered intrusive complex, like multiple crystallization events in the Norilsk-1 layered intrusion (see discussion regarding G-22 well, Figures 3A,C). The extrusive volcanism in Taymir also appears in the late Changhsingian Syradasai and Shaitan formations with the late Permian flora Cordaites candalepensis (Zalesskyi), Zamiopteris schmalhausenii Schwedov, Nephropsis ingenta Schwedov (Proskurin et al., 2015). This age is consistent with the Changhsingian volcanism in the Ivakin Fm of the Tunguska Basin.
Thus, although the U-Pb dates from the intrusions of the Norilsk and Taymir regions only roughly constrain the onset of the SLIP, what we can conclude at this point is that SLIP generally postdates the end-Permian extinction. Furthermore, inferences about the volcanic, biotic, environmental, and climatic events associated with SLIP need to be reconsidered and better and more directly documented. The layered intrusive rocks in the Tunguska Basin and Taymir regions represent a complex multi-stadial crystallization within an intrusive body and cannot be employed for the evaluation of the sedimentary, and biotic processes during Permian-Triassic transition until their crystallization history can be precisely calibrated with the CA-IDTIMS method. The minimal influence of the intrusive rocks on the surrounding pre-Triassic sedimentary succession and especially on the Carboniferous-Permian coals in the Tunguska Basin is documented in the recent review paper (Davydov, 2021 in press).
The only coal gap in the Earth history lasted from approximately 252.94 (the extinction event in South China) through the late Anisian-Ladinian and is known to be of global scale (Retallack et al., 1996). The very last coal in the Permian of the Norilsk region is documented in the upper Ivakin Fm (Paderin et al., 2016; Rad'ko, 2016). No coals are known or ever been documented in the Tutonchanian, Dvurogian and Putoranian RS in the entire Tunguska Basin (Cherepovskiy, 2001). The first thin, cm-scale coal layers are documented in the volcaniclastic upper Anisian and Ladinian. The coals are getting more frequent in the Upper Triassic (Kazakov, 2002). This coal record in the Tunguska Basin is consistent with the Triassic age of the trap’s succession in the Tunguska Basin.
According to the Burgess and Bowring (2015) model, the pyroclastic eruption started sometime around 255.58 Ma or even earlier, but this hypothesis requires additional confirmation. Only acidic volcanism has been documented in the coal-bearing successions of the Tunguska Basin (Cherepovskiy, 2001; Paderin et al., 2016). Burgess and Bowring (2015) suggested that almost the entire sedimentary-traps succession, except the Samoed Formation in the Norilsk region is Permian in age (Figure 2, column 2, see Figure 3 in Burgess and Bowring, 2015), although none of the Permian plant fossils ever been found in the traps (Radchenko and Schwedov, 1940; Mogucheva, 1973; Gor, 1985; Mogucheva and Krugovykh, 2009).
Flora is one of the most sensitive indicators of environments and hence of climate change (Taylor et al., 2009). The abnormally large Siberian LIP volcanism at the Permian-Triassic transition that caused, as proposed, the most severe of global extinctions (Svensen et al., 2009; Hinojosa et al., 2012; Clapham, 2013; Retallack, 2013; Black et al., 2014; Bond and Wignall 2014; Burgess et al., 2014; Chu et al., 2016; Svensen et al., 2018; Clapham and Renne, 2019; Shen et al., 2019; Feng et al., 2020; Vajda et al., 2020) is supposed to have caused even stronger extinction in the region of this LIP, i.e. in Tunguska Basin (Jones, 2015; Black et al., 2021, in press; Mather and Schmidt 2021, in press). The reality, at least with the flora in the Tunguska Basin, is the opposite of what most people proposed.
The Permian and Triassic flora record in the Tunguska Basin and surrounding regions (Kuznetsk Basin, East Kazakhstan, Taymir, Verkhoyanie) and has been well studied for more than 100 years, because it is the primary correlation tool in these commercially important regions (coal, oil, gas, diamonds) (Zalessky, 1912, Zalessky, 1918; Zalessky and Tchirkova, 1935; Radchenko and Schwedov, 1940; Neiburg, 1948; Andreeva et al., 1956; Neiburg, 1958; Meyen, 1966; Sadovnikov, 1967; Vladimirovich, 1967; Radchenko, 1969; Mogucheva, 1973; Radchenko, 1973; Orlova and Sadovnikov, 1974; Vladimirovich, 1980, Vladimirovich, 1981; Meyen, 1982; Betekhtina et al., 1984; Betekhtina et al., 1986; Sadovnikov, 1987b; Dobruskina, 1994; Dobruskina and Durante, 2004; Sadovnikov, 2008; Mogucheva and Krugovykh, 2009; Mogucheva, 2016; Sadovnikov, 2016).
The biostratigraphic and paleomagnetic data suggests that the Tutonchanian and lower Dvurogian RS belongs to the Induan and that the rest of the Dvurogian and lower Putoranian RS correlates with Olenekian, while the rest of the Putoranian and Uskelterian RS correlates with the Anisian (Figure 2) (Kazakov, 2002; Mogucheva, 2016). This correlation is generally consistent with the Ivakinian-Tutonchanian co-magmatism of the Ergalakh intrusive system and with the Putoranian co-magmatism of the Norilsk, Talnakh, Kharaelakh and most other intrusions in the Norilsk regions (Zolotukhin et al., 1986; Ivanov et al., 2013; Ryabov et al., 2014; Krivolutskaya, 2016; Rad'ko, 2016; Latyshev et al., 2020).
Two provinces are recognized within the Angarian paleofloristic Realm -- Siberian and Sub-Angarian that are divided into several subprovinces: Verkhoyanian, Tunguska-Verkhoyanian and Taymir-Kuznetsk (Figure 5C) (Meyen, 1990; Krassilov, 2003; Dobruskina and Durante, 2004). The Tunguska Basin is a part of the Tunguska-Verkhoyanian province, except for the Norilsk region, which belongs to the Taymir-Kuznetsk subprovince. The distinctive feature of the Tunguska-Verkhoyanie subprovince during the late Permian is the occurrence of the pteridosperms Comia, Callipteris and Compsopteris along with numerous ferns. The Tunguska-Verkhoyanie “cordaites” dominate in almost all localities and horsetails are found in greater numbers. We suggest including those into the Vojnovskyales order as a group of enigmatic gymnosperms. This assumption came from the fact that these “cordaites” are often associated with generative organs of Vojnovskya (Neiburg, 1955; Meyen, 1990).
FIGURE 5. Phytogeographic maps of circumpolar Arctic area during the late Permian and Early Triassic compared with the recent phytogeography. The maps are compiled and modified from Krassilov (2003), Dobruskina and Durante (2004) and Elias (2020). The phytogeographic differentiation and the late Permian climate are quite similar with the current Arctic provincial differentiation and climate (A,C), although the recent provinces are latitudinally narrower than during the late Permian. The phytogeographic differentiation during the climatic optimum in the Early Triassic (B) was strongly reduced, and only Realms with no provinces are recognized at that time.
The flora of the Taymir-Kuznetsk subprovince during this time is characterized by the voinovskian-peltasperms assemblage (Kaierkan and Ambarnaya Fms in Norilsk region), which comprises numerous voinovskians, including Rufloria, and abundant seeds of the genus Tungussocarpus, and the appearance of Samaropsis and Condomajella seeds (Gor, 1965; Gor, 1985). The ferns are rare, poor in diversity and include Pecopteris, Prynadaeopteris или Todites. The occurrence of Psygmophyllum foliage suggests similarity of the late Permian flora of Taymir and Norilsk with that of the Preuralian and Pechora basins (Pukhonto et al., 1998; Naugolnykh, 2007).
The late Permian Euromerian flora in the Northern arid belt along the northern slope of the Variscan orogenic belt (Germany, France, Caucasus) was predominantly xerophytic suggesting relatively warm and dry climate with no coal accumulation (Chumakov and Zharkov, 2003; Roscher and Schneider, 2006; Naugolnykh, 2007). The climate in the Angaran Realm was temperate and very wet with abundant coals that occur up to the top of the middle Changhsingian (Figure 2) (Cherepovskiy, 2001; Dobruskina and Durante, 2004; Davydov, 2021; Davydov et al., 2021). The upper Permian in the Kuznetsk Basin possessed the thickest coals (wet environments) in the entire Siberia (Cherepovskiy, 2001). The occurrence of the number of a late Permian provinces and subprovinces in the Angara Realm, suggests that the climate in the realm was also quite differentiated, similar to the current climate with a comparable differentiation of recent terrestrial biomes (Figure 5) (Foley et al., 2005; Dinerstein et al., 2019). Both the Taymir and Norilsk regions during late Permian – Early Triassic were located approximately at the latitude of the Kuznetsk Basin (Figure 4) and the flora from the Taymir and Norilsk regions directly correlates with the late Permian flora of the Kuznetsk Basin (Gor, 1965; Porokhovichenko, 2006; Mogucheva and Naugolnykh, 2010).
The major climate change occurs in Tunguska and in the Kuznetsk Basin sometimes during the second half of the Changhsingian, around 252.75 Ma (Davydov et al., 2021). At this time, the biomes differentiation is sharply decreased and the Euramerian -- Angaran Realms boundary shifted northward approximately to 15°. No provinces or subprovinces are recognized in the Angaran Realm at this time (Figure 5B). The provinciality decrease is distinguished by the distribution of Early Triassic plant associations known in all paleofloristic realms-provinces. For example, the widely distributed Early Triassic genus Pleuromeia and lycopsids with Annalepis-like scales have been found in both tropical and temperate (Siberia) climatic zones (Dobruskina, 1994; Retallack, 1997; Grauvogel-Stamm and Lugardon, 2001; Grauvogel-Stamm and Ash, 2005). The taxonomic composition of the Triassic conifer-fern flora testifies to the widest migration of plants to the central regions of Angarida both from the peripheral parts of the Angara kingdom and from the tropical belt (Mogucheva, 1996, Mogucheva, 2016). Such a migration, obviously, indicates a sharp weakening of floristic differentiation, and elimination of most paleofloristic barriers between different paleofloristic realms and the provinces-subprovinces that existed in the Late Paleozoic (Dobruskina and Durante, 2004). The latest-Permian - Early Triassic in the Angaran realm is associated with the climatic optimum, i. e. warmer and drier climate that is similar to the mid-Holocene climatic optimum in the region (Monserud et al., 1998). The current global climate warming is also resulting in a shift of the current temperate forest and boreal biomes northwards (D'Orangeville et al., 2016).
Progressive decline of the floral richness is observed in both basins during the late Permian (Figure 4). In the Tunguska Basin, the Wuchiapingian flora is quite diverse (94 species and 41 genera), but drastically reduced in richness in the Changhsingian to 38 species and 24 genera (Figure 4). The chronostratigraphy in the Tunguska Basin is not as precise as in Kuznetsk Basin and the turnover in the region occurs somewhere within the Ivakinian - Early Tutonchanian time (Changhsingian-Early Induan) (Figure 4). The floral richness (over 100 species) that is observed in the middle-late Tutonchanian is highest within the late Permian and Triassic in the region (Figure 4). The origination rate there is quite high, and the extinction rate is very low. Since the early Olenekian the extinction predominates over the origination and floral richness progressively declined towards the end of the Anisian. In the late Anisian only origination is observed in Tunguska Basin (Figure 4).
In the Kuznetsk Basin the floral richness is also declines, but slower and progressively towards the early Changhsingian. The essential taxonomic floral turnover in Kuznetsk Basin occurs in the mid-Changhsingian at approximately 252.75 Ma and ∼800 kyr before the Permian-Triassic boundary in South China (Davydov et al., 2021) (Figure 4). This level coincides with the boundary between the Tailugan and Maltsev formations and this is the former position of the regional Permian-Triassic boundary in Siberia that was utilized for many years and distinguished by the extinction of one small group of the wet-preferrable Vojnovskyales (“cordaites”) and by the appearance of many new taxa of ferns, cicades, pteridosperms, lycopsids, sphenopsids and other (Vladimirovich, 1967; Mogucheva, 1973; Betekhtina et al., 1986), All Paleozoic “cordaitian” (Vojnovskyales) at this time disappear and were replaced by more diverse new conifer-fern flora (46 species and 34 genera). The flora richness and origination in the Kuznetsk Basin in the Triassic progressively increased in Induan and Olenekian and no other extinction observed there (Figure 4). In the Kuznetsk Basin the floral origination in the region strongly predominates over extinction during Early Triassic and util early Anisian. Accordingly, the floral richness in Kuznetsk Basin in Triassic progressively increased and reaches the maximum in the early-middle Anisian, when it is stabilized. The volcanic activity in the Tunguska Basin ceased in the latest Putoranian (Naldrett et al., 1996; Fedorenko et al., 2000; Krivolutskaya, 2016; Paderin et al., 2016). The Late Triassic floral record overall is poor for the Tunguska Basin and in all of Siberia (Mogucheva, 2005).
1) Abundant and rich biota in the Permian-Triassic transition in the Tunguska Basin suggests a Changhsingian and Early-Middle Triassic age of the Tunguska traps.
2) The layered intrusion in the Norilsk region possessed a complicated internal structure and a multi-timing origin of the layers during at least a half-million or more years. The co-magmatism of extrusive and intrusive rocks utilizing geomagnetic secular variation measurements would be reliable when the samples for the latter studies and radioisotopic dating are obtained from the same spots.
3) The discrepancy between paleomagnetic-geochemical and paleontological data from extrusive rocks on one side and radioisotopic U-Pb dates from intrusive ricks on the other, suggests that the problem with the Tunguska Basin magmatism and Permian-Triassic extinction in South China is still unresolved and more complicated than has been considered by many.
4) The very last coal in the Permian of the Norilsk region is documented in the upper Ivakin Fm (Changhsingian). No coals are known or have ever been documented in the Tutonchanian, Dvurogian and Putoranian RS in the entire Tunguska Basin. This interval (Tutonchanian-Putoranian Regional Stages) precisely coincides with coal gap documented in many regions globally.
5) The number of late Permian provinces and subprovinces in the Angara Realm suggests a latitudinally differentiated climate causing the differentiation of terrestrial biomes.
6) The major climate change from cool and wet into warmer and drier occurs in the Tunguska and in Kuznetsk basins sometimes during the second half of the Changhsingian around 252.75 Ma. The differentiation of biomes sharply decreased and the Euramerian -- Angaran Realms boundary shifted northward approximately to 15°. No provinces or subprovinces are recognized in the Angaran Realm during Triassic.
7) The floral richness in the Tunguska and Kuznetsk basins progressively declined starting from the Capitanian towards the mid-Changhsingian. At this level, only wet-dominated Vojnovskyales disappears, whereas the other (conifers, cycades, ferns, lycopsids, pteridosperms and sphenopsids) are diversified. Floral origination is greatly exceeds the extinction in the Tunguska Basin from about the late Changhsingian to Induan. In the Kuznetsk Basin this turnover occurs at the mid-Changhsingian and extended to the end of the Olenekian. A similar pattern of these parameters is observed in both regions.
8) The floral richness and origination in Tunguska and Kuznetsk basins roughly correpsponds with the onset of the mafic magmatism and climatic optimum in both regions.
The raw data supporting the conclusions of this article will be made available by the authors, without undue reservation, to any qualified researcher.
All authors listed have made a substantial, direct, and intellectual contribution to the work and approved it for publication.
This work was supported by the Russian Scientific Foundation, project no. 19-17-00178 and for EK the subsidy allocated to Kazan Federal University for the state assignment #671-2020–0049 during the hard COVID time. The efforts of Prof. Snyder from Boise State University and especially Prof. Spencer Lucas from New Mexico Museum of Natural History & Science resulted in appropriate English. Three anonymous reviewers have made a valuable suggestion that improved the content of the paper.
The authors declare that the research was conducted in the absence of any commercial or financial relationships that could be construed as a potential conflict of interest.
The Supplementary Material for this article can be found online at: https://www.frontiersin.org/articles/10.3389/feart.2021.635179/full#supplementary-material
Afanasenkov, A. P., Nikishin, A. M., Unger, A. V., Bordunov, S. I., Lugovaya, O. V., Chikishev, A. A., et al. (2016). The tectonics and stages of the geological history of the Yenisei–Khatanga Basin and the conjugate Taymir orogen. Geotectonics 50, 161–178. doi:10.1134/S0016852116020023
Andreeva, E. M., Mandelshtam, M. O., Radchenko, G. P., Rotay, A. P., Khalfin, L. L., and Yavorsky, V. I. (1956). Atlas of the key-fossils of fauna and flora from Permian of Kuznets basin. Leningrad: Gosnauchtekhizdat.(In Russian)
Augland, L. E., Ryabov, V. V., Vernikovsky, V. A., Planke, S., Polozov, A. G., Callegaro, S., et al. (2019). The main pulse of the Siberian Traps expanded in size and composition. Sci. Rep. 9, 18723. doi:10.1038/s41598-019-54023-2
Betekhtina, O. A., Mogucheva, N. K., and Dagis, A. S. (1984). K voprosu o vozraste vulkanogennoy tolshchi Tungusskoy sineklizy [The age of the volcanogenic series of the Tunguska Syneclise]. Novosibirsk: Trudy Instituta Geologii i Geofiziki, 4–8. (In Russian)
Betekhtina, O. A., Mogucheva, N. K., Batyaeva, S. K., and Kushnarev, M. P. (1986). “Permian-Triassic boundary in the type section of Mal’tsev formation of Kuzbass,” in Biostratigraphy of the mesozoic of Siberia and far east of Russia. Editors A. L. Yanshin, and A. S. Dagis (Novosibirsk: Transactions of Institute of Geology and Geophysics) 648, 31–38. (In Russian)
Betekhtina, O. A., Gorelova, S. G., Dryagina, L. L., Danilov, V. I., Batyayeva, S. P., and Tokareva, P. A. (1988). Upper Paleozoic of Angarida. Novosibirsk: Nauka (Transactions of Institute of Geology and Geophysics of RAS 707, 265. (In Russian)
Black, B. A., Lamarque, J-F., Shields, C. A., Elkins-Tanton, L. T., and Kiehl, J. T. (2014). Acid rain and ozone depletion from pulsed Siberian Traps magmatism. Geology 42, 67–70. doi:10.1130/G34875.1
Black, B. A., Mittal, T., Lingo, F., Walowski, K., and Hernandez, A. (2021). “Assessing the environmental consequences of the generation and alteration of mafic volcaniclastic deposits during large igneous province emplacement,” in Large igneous provinces: A driver of global environmental and biotic changes. Editors R. E. Ernst, A. J. Dickson, and A. Bekker (New York, Unites States: John Wiley & Sons) 255, 117–132. doi:10.1002/9781119507444
Bond, D. P., and Grasby, S. E. (2017). On the causes of mass extinctions. Palaeogeogr., Palaeoclimatol., Palaeoecol. 478, 3–29. doi:10.1016/j.palaeo.2016.11.005
Bond, D. P., and Wignall, P. B. (2014). “Large igneous provinces and mass extinctions: an update,” in Volcanism, impacts, and mass extinctions: causes and effects. Editors G. Keller, and A. C. Kerr (Boulder, United States: Geological Society of America) 505, 29–55.
Budnikov, I. V., Kutygin, R. V., Shi, G. R., Sivtchikov, V. E., and Krivenko, O. V. (2020). Permian stratigraphy and paleogeography of central Siberia (Angaraland) – a review. J. Asian Earth Sci. 196, 104365. doi:10.1016/j.jseaes.2020.104365
Burgess, S. D., and Bowring, S. A. (2015). High-precision geochronology confirms voluminous magmatism before, during, and after Earth’s most severe extinction. Sci. Adv. 1, e1500470. doi:10.1126/sciadv.1500470
Burgess, S. D., Bowring, S., and Shen, S. (2014). High-precision timeline for Earth’s most severe extinction. Proc. Natl. Acad. Sci. 111, 3316–3321. doi:10.1073/pnas.1317692111
Burgess, S. D., Muirhead, J. D., and Bowring, S. A. (2017). Initial pulse of Siberian traps sills as the trigger of the end-Permian mass extinction. Nat. Commun. 8, 164. doi:10.1038/s41467-017-00083-9
Burgess, S. D. (2014). High-precision U/Pb geochronology of large igneous provinces and mass extinctions: testing coincidence and causation. Cambridge, MA: Massachusetts Institute of Technology.
Campbell, I. H., Czamanske, G. K., Fedorenko, V. A., Hill, R. I., and Stepanov, V. (1992). Synchronism of the Siberian traps and the Permian-Triassic boundary. Science 258, 1760–1763. doi:10.1126/science.258.5089.1760
Cherepovskiy, (2001). Coal reservoirs of Russia. Moscow: Coal Basins of East Siberia 4, 500. (In Russian)
Chu, D., Yu, J., Tong, J., Benton, M. J., Song, H., Huang, Y., et al. (2016). Biostratigraphic correlation and mass extinction during the Permian-Triassic transition in terrestrial-marine siliciclastic settings of south China. Glob. Planet. Change 146, 67–88. doi:10.1016/j.gloplacha.2016.09.009
Chu, D., Tong, J., Benton, M. J., Yu, J., and Huang, Y. (2019). Mixed continental-marine biotas following the Permian-Triassic mass extinction in south and north China. Palaeogeogr., Palaeoclimatol., Palaeoecol. 519, 95–107. doi:10.1016/j.palaeo.2017.10.028
Chumakov, N. M., and Zharkov, M. A. (2003). Climate during the Permian-Triassic biosphere reorganizations; article 2, climate in late Permian and Early Triassic; general inferences. Stratigraphy. Geolog. Correlation 11, 55–70.
Clapham, M. E., and Renne, P. R. (2019). Flood basalts and mass extinctions. Annu. Rev. Earth Planet. Sci. 47, 275–303. doi:10.1146/annurev-earth-053018-060136
Clapham, M. E. (2013). “The end-Permian mass extinction,” in Earth systems and environmental sciences. Amsterdam, Netherlands: Elsevier, 645–652. doi:10.1016/B978-0-12-409548-9.12052-4
D’Orangeville, L., Duchesne, L., Houle, D., Kneeshaw, D., Côté, B., and Pederson, N. (2016). Northeastern North America as a potential refugium for boreal forests in a warming climate. Science 352, 1452–1455. doi:10.1126/science.aaf4951
Davydov, V. I., Karasev, E. V., Nurgalieva, N. G., Schmitz, M. D., Budnikov, I. V., Biakov, A. S., et al. (2021). Climate and biotic evolution during the Permian-Triassic transition in the temperate Northern Hemisphere, Kuznetsk Basin, Siberia, Russia. Palaeogeogr. Palaeoclimatol. Palaeoecol., 110432. doi:10.1016/j.palaeo.2021.110432
Davydov, V. I., Arefiev, M. P., Golubev, V. K., Karasev, E. V., Naumcheva, M. A., Schmitz, M. D., et al. (2020). Radioisotopic and biostratigraphic constraints on the classical middle–upper Permian succession and tetrapod fauna of the Moscow syneclise, Russia. Geology 48, 742–747. doi:10.1130/G47172.1
Davydov, V. I. (2021). Tunguska сoals, Siberian sills and the Permian-Triassic extinction. Earth - Sci. Rev. 212, 103438. doi:10.1016/j.earscirev.2020.103438
Dinerstein, E., Vynne, C., Sala, E., Joshi, A. R., Fernando, S., Lovejoy, T. E., et al. (2019). A global deal for nature: guiding principles, milestones, and targets. Sci. Adv. 5, eaaw2869. doi:10.1126/sciadv.aaw2869
Distler, V. V., Sluzhenikin, S. F., Cabri, L. J., Krivolutskaya, N. A., Turovtsev, D. M., Golovanova, T. A., et al. (1999). Platinum ores of the Norilsk Layered intrusions: magmatic and fluid concentration of noble metals. Geology of Ore Deposits 41, 214–237. (In Russian)
Dobruskina, I. A., and Durante, M. V. (2004). “Restructuring of flora at the Paleophyte and Mesophyte boundary linked to global climate change,” in Plant world in space and time. Editors M. V. Durante, and I. A. Ignatiev (Moscow: GEOS), 197. (In Russian)
Ernst, R. E., and Youbi, N. (2017). How large igneous provinces affect global climate, sometimes cause mass extinctions, and represent natural markers in the geological record. Palaeogeogr., Palaeoclimatol., Palaeoecol. 478, 30–52. doi:10.1016/j.palaeo.2017.03.014
Ernst, R. E., Dickson, A. J., and Bekker, A. (2021). Large igneous provinces: A driver of global environmental and biotic changes. New Jersey, United States: Wiley 255, 592
Fedorenko, V., Czamanske, G., Zen’ko, T., Budahn, J., and Siems, D. (2000). Field and geochemical studies of the melilite-bearing Arydzhangsky suite, and an overall perspective on the Siberian alkaline-ultramafic flood-volcanic rocks. Int. Geology. Rev. 42, 769–804. doi:10.1080/00206810009465111
Feng, Z., Wei, H-B., Guo, Y., He, X-Y., Sui, Q., Zhou, Y., et al. (2020). From rainforest to herbland: new insights into land plant responses to the end-Permian mass extinction. Earth - Sci. Rev. 204, 103153. doi:10.1016/j.earscirev.2020.103153
Foley, J. A., Defries, R., Asner, G. P., Barford, C., Bonan, G., Carpenter, S. R., et al. (2005). Global consequences of land use. Science 309, 570–574. doi:10.1126/science.1111772
Gor, Y. G. (1965). Stratigraphy and flora of the Late Paleozoic coal-bearing deposits of Norilsk region. Leningrad: Nedra, 140. (In Russian)
Gor, Y. G. (1985). “Permian-Triassic boundary in the north of the angarida,” in Stratigraphy and paleontology of the Mesozoic sedimentary basins of the north of USSR. (Petersburg, Russia: Sevmorgeologiya) 125–134. (In Russian)
Grauvogel-Stamm, L., and Ash, S. R. (2005). Recovery of the Triassic land flora from the end-Permian life crisis. Comptes rendus Palevol 4, 593–608. doi:10.1016/j.crpv.2005.07.002
Grauvogel-Stamm, L., and Lugardon, B. (2001). The Triassic lycopsids Pleuromeia and Annalepis: relationships, evolution, and origin: relationships, evolution, and origin. Am. Fern J. 91, 115–149. doi:10.1640/0002-8444(2001)091
Hallam, A., and Wignall, P. B. (1997). Mass extinctions and their aftermath. Oxford: Oxford University Press, 328.
Henderson, C. M., Davydov, V. I., Wardlaw, B. R., Gradstein, F. M., and Hammer, O. (2012). “Chapter 24 - the Permian Period,” in The geologic time scale. Editors F. M. Gradstein, J. G. Ogg, M. D. Schmitz, and G. M. Ogg (Amsterdam,UK: Elsevier Science), 653–679.
Hinojosa, J. L., Brown, S. T., Chen, J., DePaolo, D. J., Paytan, A., Shen, S., et al. (2012). Evidence for end-Permian ocean acidification from calcium isotopes in biogenic apatite. Geology 40, 743–746. doi:10.1130/G33048.1
Hoenisch, B., Ridgwell, A., Schmidt, D. N., Thomas, E., Gibbs, S. J., Sluijs, A., et al. (2012). The geological record of ocean acidification. Science 335, 1058–1063. doi:10.1126/science.1208277
Ivanov, A. v., He, H., Yan, L., Ryabov, V. V., Shevko, A. Y., Palesskii, S. V., et al. (2013). Siberian Traps large igneous province: evidence for two flood basalt pulses around the Permo-Triassic boundary and in the Middle Triassic, and contemporaneous granitic magmatism. Earth Sci. Rev. 122, 58–76. doi:10.1016/j.earscirev.2013.04.001
Jones, M. T. (2015). “The environmental and climatic impacts of volcanic ash deposition,” in Volcanism and global environmental change. Editors A. Schmidt, K. Fristad, and L. T. Elkins-Tanton (Cambridge: Cambridge University Press), 260–273. doi:10.1017/CBO9781107415683
Kazakov, A. M. (2002). Stratigraphy of oil-gas bearing basins of Siberia. Triassic System. (Novosibirsk: GEO Publishing House), 327. (In Russian)
Kocsis, Á. T., Reddin, C. J., Alroy, J., and Kiessling, W. (2019). The R package divDyn for quantifying diversity dynamics using fossil sampling data. Methods Ecol. Evol. 10, 735–743. doi:10.1111/2041-210X.13161
Kontorovich, A. E., Staroseltsev, V. S., Surkov, B. S., Khomenko, A. V., Efimov, A. S., Kaschenko, S. A., et al. (1994). Tunguska basin. Oil-gas bearing bassins and regions of Siberia, Novosibirsk: Siberian branch of Russian Academy of Science5, 91. (In Russian)
Kovrigina, E. K. (2000). State geologic map of Russian federation. 1:1.000.000 scale Norilsk. Sheet R(45) - 47, Norilsk. Explanatory notes. Sankt-Petersburg, Russia: Cartographic Factory, 479. (In Russian)
Krassilov, V. A. (2003). Terrestrial palaeoecology and global change. London, England: Coronet Books Incorporated
Krivolutskaya, N. A. (2016). Siberian traps and Pt-Cu-Ni deposits in the Norilsk area. New York, Unites States: Springer International Publishing
Latyshev, A. V., Ulyakhina, P. S., and Krivolutskaya, N. A. (2019). Signs of the record of geomagnetic reversal in Permian–Triassic trap intrusions of the Ergalakhsky complex, Norilsk region. Izvestiya. Phys. Solid Earth 55, 270–286. doi:10.1134/S1069351319020058
Latyshev, A. V., Rad’ko, V. A., Veselovskiy, R. V., Fetisova, A. M., and Pavlov, V. E. (2020). Correlation of the Permian-Triassic ore-bearing intrusions of the Norilsk region with the volcanic sequence of the Siberian traps based on the paleomagnetic data. Econ. Geology. 115, 1173–1193. doi:10.5382/econgeo.4746
Lipenkov, G., Maschak, M. S., Kirichenko, V. T., Naumov, M. V., Khabarov, A. N., Kovalevskaya, E. O., et al. (2018). “State geologic map of Russian Federation,” in Norilsk series, sheet R-47-kheta. Explanatory notes. Sankt-Petersburg, Russia: Cartographic Factory, 464. (In Russian)
Mather, T. A., and Schmidt, A. (2021). “Environmental effects of volcanic volatile fluxes from subaerial large igneous province,” in Large igneous provinces: A driver of global environmental and biotic changes. Editors R. E. Ernst, A. J. Dickson, and A. Bekker (Hoboken, New Jersey: Wiley 255, 103–116.
Meyen, S. V. (1966). Cordaiteans of the upper Paleozoic of North Eurasia. Moscow, Russia: Nauka.150, 5–184. (In Russian)
Meyen, S. V. (1982). The Carboniferous and Permian floras of Angaraland: a synthesis. Biol. Mem. 7, 109.
Meyen, S. V. (1997). Permian conifers of Western Angaraland. Rev. Palaeobotany Palynology 96, 351–447. doi:10.1016/S0034-6667(96)00046-2
Mogucheva, N. K., and Krugovykh, V. V. (2009). New data on the stratigraphic chart for Triassic deposits in the Tunguska syneclise and Kuznetsk basin. Stratigr. Geol. Correl. 17, 510–518. doi:10.1134/s0869593809050049
Mogucheva, N. K., and Naugolnykh, S. V. (2010). Gagariostrobus cylindricus (Prynada) Mogutcheva and the Permian—Triassic ecosystem flora reorganization in the Tunguska basin. Stratigr. Geol. Correl. 18, 31–41. doi:10.1134/S086959381001003X
Mogucheva, N. K. (1973). Early Triassic flora of Tunguska basin. Moscow, Russia: Nedra Transactions of SNIIGGIMS, 154.(In Russian)
Mogucheva, N. K. (1996). Evolutionary stages of Triassic flora in Siberia (Angarida). The Palaeobotanist 45, 329–333.
Mogucheva, N. K. (2005). “Main stages of reorganisation of early Mesozoic flora of Siberia,” in modern problems of paleofloristics, paleophytogeography, and phytostratigraphy: Transactions of International paleobotany conference. Editors M. A. Akhmetiev, and A. B. German, May 17–18, 2005, Moscow, (Moscow: GEOS), 207–211. (In Russian)
Mogucheva, N. K. (2016). Flora from the Induan stage (Lower Triassic) of middle Siberia. Stratigr. Geol. Correl. 24, 252–266. doi:10.1134/S0869593816020052
Monserud, R. A., Tchebakova, N. M., and Denissenko, O. V. (1998). Reconstruction of the mid-Holocene palaeoclimate of Siberia using a bioclimatic vegetation model. Palaeogeogr., Palaeoclimatol., Palaeoecol. 139, 15–36. doi:10.1016/S0031-0182(97)00127-2
Naldrett, A. J., Fedorenko, V. A., Asif, M., Lin, S., Kunilov, V. E., Stekhin, A. I., et al. (1996). Controls on the composition of Ni-Cu sulfide deposits as illustrated by those at Norilsk, Siberia. Econ. Geology. 91, 751–773. doi:10.2113/gsecongeo.91.4.751
Naugolnykh, S. V. (2007). Permian floras of the Urals. Moscow (Geos). Transact. Geolog. Inst. 524, 322. doi:10.13140/RG.2.2.31875.91688 (In Russian)
Neiburg, M. F. (1948). Upper paleozoic flora of Kuznetsk basin. Moscow, Russia: Academy of sciences of USSR, 12.(In Russian)
Neiburg, M. F. (1955). New representatives of lower Permian flora of Angarida. Doklady Akademii Nauk SSSR 102, 613–616. (In Russian)
Orlova, E. F., and Sadovnikov, G. N. (1974). Stratigrafiya Verkhnepermskikh i Triasovykh otlozheniy yugo-vostochnoy chasti Tungusskoy sineklizy [Stratigraphy of upper Permian and Triassic deposits in the southeastern part of the Tunguska Syneclise]. Izvestiya Akademii Nauk SSSR. Seriya Geologicheskaya 4, 125–133. (In Russian)
Paderin, P. G., Demyanuk, A. F., Nazarov, D. V., Chekanov, V. I., Smelova, L. V., Petrov, V. V., et al. (2016). State geologic map of Russian federation. 1:1.000.000 scale (third generation). Norilsk series. Sheet R45, Norilsk. Explanatory notes. Sankt-Petersburg, Russia: VSEGEI Cartographic Factory, 320. (In Russian)
Permyakov, S. A., Denisenko, E. P., Popova, T. D., Kurakina, A. N., Mironyuk, E. P., Von-der_Flaas, G. S., et al. (2012). State geologic map of Russian federation. Angara-Enisei series. Sheet O-48 - Ust’-Ilimsk. Explanatory notes. Sankt-Petersburg, Russia: VSEGEI Cartographic Factory, 433. (In Russian)
Porokhovichenko, L. G. (2006). Late Paleozoic flora of Norilsk region and its stratigraphic significance. PhD dissertation Tomsk, Russia: Tomsk Federal University. (In Russian)
Proskurin, V. F., Gavrish, A. V., Schneider, G. V., Nagaitsvena, N. N., Mokhov, V. V., Nelyubin, V. V., et al. (2015). State geologic map of Russian federation. 1:1.000.000 scale (third generation) Taymir-Severnaya Zemlya series. Sankt-Petersburg, Russia: VSEGEI Cartographic Factory, 424. (In Russian)
Pukhonto, S. K., Durante, M. V., and Cherepovskiĭ, V. F. (1998). Stratigrafiiã i floristicheskaiã kharakteristika Permskikh otlozheniĭ ugolʹnykh mestorozhdeniĭ Pechorskogo basseĭna. Moskva, Russia: Nauch. mir, 131. (In Russian)
Rad’ko, V. A. (2016). The facies of intrusive and effusive magmatism in the Norilsk region. Saint Petersburg: Cartographic factory of VSEGEI, 226. (In Russian)
Radchenko, G. P., and Schwedov, N. A. (1940). Upper Paleozoic flora of coal-bearing deposits of western part of Nizhnyaya Tunguska River basin. Leningrad, Russia: Glavsevmorput (Transactions of Arctic Geologic Institute), 157. (In Russian)
Radchenko, G. P. (1969). Atlas of late Paleozoic flora of Norilsk region. Leningrad: Scientific exploration Institute of Arctic Geology, 189. (In Russian)
Radchenko, G. P. (1973). “Altai-Sayany folded area,” in Stratigraphy of the USSR. Triassic system. Editors I. I., Gorsky, L. D. Kiparisova, D. V. Nalivkin, and G. P. Radchenko (Moscow: Nedra Publishing House) 7, 223–231. (In Russian).
Ragozin, L. A. (1958). Bivalve non-marine mollusks from the Triassic deposits of Angarida. Bull. Mosc. Soc. Nat. Stud. Geol. section 33, 79–90. (In Russian)
Retallack, G. J., and Jahren, H. A. (2008). Methane release from igneous intrusion of coal during late Permian extinction events. J. Geology. 116, 1–20. doi:10.1086/524120
Retallack, G. J., Veevers, J. J., and Morante, R. (1996). Global coal gap between Permian-Triassic extinction and Middle Triassic recovery of peat-forming plants. Geol. Soc. America Bull 108, 195–207. doi:10.1130/0016-7606
Retallack, G. J. (1997). Earliest Triassic origin of Isoetes and quillwort evolutionary radiation. J. Paleontol. 71, 500–521.
Retallack, G. J. (2013). Permian and Triassic greenhouse crises. Gondwana Res. 24, 90–103. doi:10.1016/j.gr.2012.03.003
Roscher, M., and Schneider, J. W. (2006). Permo-Carboniferous climate: early Pennsylvanian to Late Permian climate development of central Europe in a regional and global context. Geol. Soc. Lond. Spec. Publicat. 265, 95–136. doi:10.1144/GSL.SP.2006.265.01.05
Rothman, D. H. (2017). Thresholds of catastrophe in the Earth system. Sci. Adv. 3, e1700906. doi:10.1126/sciadv.1700906
Ryabov, V. V., Shevko, A., and Gora, M. P. (2001). Magmatic formations in Norilsk region. Novosibirsk: Nonparel Publishers. (In Russian)
Ryabov, V. V., Shevko, A. Y., and Gora, M. P. (2014). Trap magmatism and ore formation in the Siberian Norilsk region. Dordrecht: Springer Netherlands 3, 1682
Sadovnikov, G. N. (1967). Stratigrafiya verkhnepermskikh uglenosnykh otlozheniy basseyna r. Nizhnyaya Tunguska [Stratigraphy of upper Permian coal measures of the Lower Tunguska river basin]. Izvestiya Akademii Nauk SSSR. Seriya Geologicheskaya 3, 105–116. (In Russian)
Sadovnikov, G. N. (1987a). Permian/Triassic non-marine transition beds in the central Angaraland (Siberia). Albertiana 6, 21–23. (In Russian)
Sadovnikov, G. N. (1987b). The stratigraphy of Permian-Triassic sediments of the Tunguska basin. Soviet Geology. 2, 84–89. (In Russian)
Sadovnikov, G. N. (2008). On the global stratotype section and point of the Triassic base. Stratigr. Geol. Correl. 16, 31–46. doi:10.1007/s11506-008-1003-1
Sadovnikov, G. N. (2016). Trap’s volcanism of Siberia and “Permian-Triassic extinction”. Geology. Exploration, 8–14. (In Russian)
Schneider, J. W., Lucas, S. G., Scholze, F., Voigt, S., Marchetti, L., Klein, H., et al. (2019). Late Paleozoic–early Mesozoic continental biostratigraphy — links to the standard global chronostratigraphic scale Palaeoworld 29, 186–238. doi:10.1016/j.palwor.2019.09.001
Scholze, F., Shen, S., Backer, M., Wei, H-B., Hübner, M., Cui, Y-Y., et al. (2020). Reinvestigation of conchostracans (Crustacea: branchiopoda) from the Permian–Triassic transition in southwest China. Palaeoworld 29, 368–390. doi:10.1016/j.palwor.2019.04.007
Schwedov, N. A. (1961). Permian flora of northern Pre-Eniseyi-Lena region. Moscow, Russia: Gosnauchtekhizdat. (In Russian)
Schwedov, N. A. (1963). New early Triassic plants from Norilsk region. Collections Pap. Paleontol. biostratigraphy, 59–67. (In Russian)
Sereda, E., Belyatsky, B., and Krivolutskaya, N. (2020). Geochemistry and geochronology of southern Norilsk intrusions, SW siberian traps. Minerals 10, 165. doi:10.3390/min10020165
Shen, S., Crowley, J. L., Wang, Y., Bowring, S. A., Erwin, D. H., Sadler, P. M., et al. (2011). Calibrating the end-Permian mass extinction. Science 334, 1367–1372. doi:10.1126/science.1213454
Shen, S., Ramezani, R., Chen, J., Cao, C-Q., Erwin, D. H., Zhang, H., et al. (2019). A sudden end-Permian mass extinction in South China:/10.1130/B31909.1;. GSA Bull. 131, 205–223. doi:10.1130/B31909.1
Sobolev, A. V., Arndt, N. T., Krivolutskaya, N. A., Kuzmin, D. V., and Sobolev, S. V. (2015). “The origin of gases that caused the Permian–Triassic extinction,” in Volcanism and global environmental change. Editors A. Schmidt, K. Fristad, and L. T. Elkins-Tanton (Cambridge: Cambridge University Press), 147–163.
Svensen, H., Planke, S., Polozov, A. G., Schmidbauer, N., Corfu, F., Podladchikov, Y. Y., et al. (2009). Siberian gas venting and the end-Permian environmental crisis. Earth Planet. Sci. Lett. 277, 490–500. doi:10.1016/j.epsl.2008.11.015
Svensen, H. H., Frolov, S., Akhmanov, G. G., Polozov, A. G., Jerram, D. A., Shiganova, O. V., et al. (2018). Sills and gas generation in the Siberian traps. Philos. Trans. A. Math. Phys. Eng. Sci. 376, 80. doi:10.1098/rsta.2017.0080
Taylor, T. N., Taylor, E. L., and Krings, M. (2009). Paleobotany: the biology and evolution of fossil plants. 2nd Edn. London: Academic, 490.
Vajda, V., McLoughlin, S., Mays, C., Frank, T. D., Fielding, C. R., Tevyaw, A., et al. (2020). End-Permian (252 Mya) deforestation, wildfires and flooding—an ancient biotic crisis with lessons for the present. Earth Planet. Sci. Lett. 529, 115875. doi:10.1016/j.epsl.2019.115875
Varganov, A. S., Sosnovskaya, O. V., Nazarov, D. V., Chekanov, V. I., Lebedeva, E. A., Gerasicheva, A. V., et al. (2018). State Geologic map of Russian Federation. 1:1.000.000 scale (third generation). Saint-Petersburg: VSEGEI Cartographic Factory, 411. (In Russian)
Vladimirovich, V. P. (1967). “Biostratigraphy of Triassic and Jurassic continental deposits of eastern slope of the Urals, northern Kazakhstan and mountain part of western Siberia,” in Stratigraphy and paleontology of Mesozoic and Paleogene-Neogene continental deposits of the Asiatic part of the USSR. Editor G. G. Martinson (Leningrad: Nauka), 46–55. (In Russian)
Vladimirovich, V. P. (1980). Early Triassic ferns of western Siberia (Kuznetsk basin). Ezhegodnik Vsesoyuznogo Paleontologicheskogo Obshchestva 23, 265–285. (In Russian)
Vladimirovich, V. P. (1981). Ferns of the early Triassic of the western Siberia. Ezhegodnik Vsesoyuznogo Paleontologicheskogo Obshchestva 24, 215–226. (In Russian)
Wall, C. J., Scoates, J. S., Weis, D., Friedman, R. M., Amini, M., and Meurer, W. P. (2018). The Stillwater complex: integrating zircon geochronological and geochemical constraints on the age, emplacement history and crystallization of a large, open-system layered intrusion. J. Petrology. 59 (1), 153–190. doi:10.1093/PETROLOGY/EGY024
Zalessky, M. D., and Tchirkova, H. T. (1935). Observations sur quelques v´eg´etaux fossiles du terrain Permien du bassin de Kousnetzk. Bulletin de l’Acad´emie des Sciences de l’URSS. Classe des sc iences math´ematiques et 2, 312.
Zalessky, M. D. (1912). Cordaites aequalis Goeppert from Siberia and its similarity with Noggerathiopsis hislopi Bunbury from Gondwana. Trans. Geol. Committee Russia, New Ser. 86, 1–43. (In Russian)
Zalessky, M. D. (1918). Paleozoic flora of Angarian series. Trans. Geol. Committee Russia, New Ser. 174, 1–76. (In Russian)
Keywords: Permian-Triassic transition, Norilsk Russia, radioisotopic ages, geomagnetic secular variartion, multi-stadial intrusions, floral diversity and dynamics
Citation: Davydov VI and Karasev EV (2021) The Influence of the Permian-Triassic Magmatism in the Tunguska Basin, Siberia on the Regional Floristic Biota of the Permian-Triassic Transition in the Region. Front. Earth Sci. 9:635179. doi: 10.3389/feart.2021.635179
Received: 29 November 2020; Accepted: 15 February 2021;
Published: 05 May 2021.
Edited by:
Spencer G. Lucas, New Mexico Museum of Natural History and Science, United StatesReviewed by:
Urs Schaltegger, Université de Genève, SwitzerlandCopyright © 2021 Davydov and Karasev. This is an open-access article distributed under the terms of the Creative Commons Attribution License (CC BY). The use, distribution or reproduction in other forums is permitted, provided the original author(s) and the copyright owner(s) are credited and that the original publication in this journal is cited, in accordance with accepted academic practice. No use, distribution or reproduction is permitted which does not comply with these terms.
*Correspondence: V. I. Davydov, dmRhdnlkb3ZAYm9pc2VzdGF0ZS5lZHU=
Disclaimer: All claims expressed in this article are solely those of the authors and do not necessarily represent those of their affiliated organizations, or those of the publisher, the editors and the reviewers. Any product that may be evaluated in this article or claim that may be made by its manufacturer is not guaranteed or endorsed by the publisher.
Research integrity at Frontiers
Learn more about the work of our research integrity team to safeguard the quality of each article we publish.