- 1Department of Technology and Safety, UiT—The Arctic University of Norway, Harstad, Norway
- 2International Arctic Research Center, University of Alaska Fairbanks, Fairbanks, AK, United States
- 3Department of Natural Sciences, University of Alaska Southeast, Juneau, AK, United States
- 4Geophysical Institute, University of Alaska Fairbanks, Fairbanks, AK, United States
- 5Department of Geosciences, University of Oslo, Oslo, Norway
Glacial lake outburst floods (GLOFs) significantly affect downstream communities in Alaska. Notably, GLOFs originating from Suicide Basin, adjacent to Mendenhall Glacier, have impacted populated areas in Juneau, Alaska since 2011. On the Kenai Peninsula, records of GLOFs from Snow Glacier date as far back as 1949, affecting downstream communities and infrastructure along the Kenai and Snow river systems. The US National Weather Service, US Geological Survey, and University of Alaska Southeast (for Suicide Basin) provide informational products to aid the public in monitoring both glacial dammed lakes as well as the ensuing GLOFs. This 2 year study (2018–2019) analyzed how communities affected by the aforementioned GLOFs utilize these various products. The participants in this project represented a variety of different sectors and backgrounds to capture a diverse set of perspectives and insights, including those of homeowners, emergency responders, tour operators, and staff at federal and state agencies. In addition, feedback and suggestions were collected from interviewees to facilitate improvements or modifications by the relevant entities to make the informational products more usable. Findings from this study were also used to inform changes to the US National Weather Service monitoring websites for both Suicide Basin and Snow Glacier. This paper’s findings on GLOF information use are relevant for other GLOF-affected communities, from both an information user and information developer perspective.
Introduction
Climate change is directly and immediately impacting the state of the cryosphere in Alaska (USGCRP, 2018; Meredith et al., 2019). Understanding these cryosphere changes in Alaska, particularly from a hazards perspective, is of great scientific and socioeconomic interest (Harrison et al., 2018). Glacier outburst floods are a type of cryospheric hazard, where glacier water is released suddenly (Rounce et al., 2017). Glacier outburst floods vary in terms of their origins, with a few notable examples being moraine-dammed glacial lakes, ice-dammed glacial lakes, and englacial conduits. Glacier outburst floods also vary in terms of their triggers, such as volcanic or seismic activity, icefalls, rockfalls, or avalanches, to name a few. Glacial lake outburst floods (GLOFs) are a specific subset of glacial outburst floods and are one of the most dangerous cryospheric hazards present in Alaska. A GLOF is characterized by the rapid release of water from within or under a glacier or from a moraine-dammed or an ice-dammed lake due to unstable glacial dynamics (Hambrey and Alean, 2004). They can drastically and severely impact downstream ecosystems, human systems, and infrastructure. One of the most deadly contemporary GLOFs occurred in 1941 in Huaraz, Peru (Wegner, 2014). GLOFs occur in many areas around the world, including Alaska, the Alps, and the Himalaya-Hindu Kush area where several large, devastating GLOFs have occurred in recent years (Bajracharya et al., 2020). For example, a GLOF in combination with heavy rainfall in Kedarnath, India in 2013 resulted in more 6,000 fatalities as well as significant road and infrastructure (e.g., power plants) damage (Allen et al., 2016). The impact of GLOFs on human systems and infrastructure is generally acute, sudden, and potentially catastrophic. A GLOF can also affect human systems in less direct ways. Although GLOFs are not frequent events, they can have damaging and long-lasting social and environmental impacts (IPCC, 2012). GLOFs can impact downstream water sources, tourism economies, energy production (particularly in glacierized basins with hydropower infrastructure), as well as livestock, agriculture and other food supplies. GLOFs in Alaska, though not as destructive in terms of life and property as in other parts of the world due to low population density, can nonetheless result in material and non-material damage to downstream homes, infrastructure, riverbank stability, and local economies.
This research contributes to the literature and current understanding on decision support tool development and user engagement in the context of cryospheric hazards (Nel et al., 2016; Bremer and Meisch, 2017; Becker, 2018; Luke et al., 2018; Vincent et al., 2018; Bremer et al., 2019; Huggel et al., 2020). Specifically, we address the gap in knowledge on the use of decision support tools in a GLOF context. We also contribute to the broader flood management literature via our analysis of user engagement and the incorporation of user feedback into revisions of flood information products and GLOF decision support tools.
The purpose of this research was to determine the most effective ways to relay GLOF hazard information to different user groups in two locations in Alaska: the City and Borough of Juneau and the Kenai Peninsula Borough. Specifically, this research investigated (1) how current GLOF informational products are used by different users, (2) what challenges and/or barriers exist regarding their use of current informational products, (3) whether different users have different informational needs, and (4) what are the best formats and communication mechanisms to meet identified user needs. Lastly, this research exemplified successful knowledge co-production via involving both information developers and information users, such that user engagement from both an information developer and information user perspective was used to help refine and develop new GLOF information products.
Background: GLOFs and Alaska Study Sites
GLOFs differ in how they form, depending on the local setting and glacial conditions. Over the course of the melt season, glacier dammed lakes fill up with water until the water releases from the lake (Hambrey and Alean, 2004). An ice-dammed lake can develop when a glacier acts as a dam for a stream draining a side valley or an adjacent lake, formed from melt from an up-valley ice cap or glacier (Cuffey and Paterson, 2010). Ice-dammed lakes typically form in three locations: (1) where ice-free side valleys are blocked by a glacier in the trunk valley; (2) where trunk valleys are blocked by glaciers spilling out from side valleys; or (3) at the junction between two valley glaciers (Benn and Evans, 2014).
GLOF hydrographs differ from precipitation-induced hydrographs. GLOFs tend to start very slowly, as the drainage water connects to the sub-glacial drainage system. However, once this connection is established, friction and melt from the flowing water expands the glacial drainage conduits, leading to a rapid, exponential rise in discharge. After peak flow is achieved, the drainage system is wide open, and the descending limb of the hydrograph is extremely steep such that the GLOFs taper off much more rapidly, within minutes or hours, than floods generated by precipitation events (Hambrey and Alean, 2004).
GLOFs typically occur in summertime or early autumn, when sufficient melt water has accumulated to bring glacial lakes to a critical level and there is increasing summer ablation (Xu et al., 2015). Precipitation-induced floods, on the other hand, do not necessarily have as much seasonal dependence. Glacierized basins in general tend to have distinct seasonal variation in discharge, due to snow accumulation in winter and snow and ice melt in summer, as well as distinct daily variation due to the daily diurnal cycle of meteorological and temperature conditions (Hock et al., 2005).
The impacts of GLOFs often exceed those of other flood events, particularly in the Andes and the Himalayas (Clague and Evans, 2000). This is predominantly due to the sudden release of water which typically result in flood discharges that are substantially larger than those from rain, snow, or glacier melt (Cenderelli and Wohl, 2003). For example, seismic records of a July 2016 glacial outburst flood in the Bhotekoshi/Sunkoshi Rover of Nepal showed that GLOFs can mobilize the large boulders that normally prevent channel erosion, causing larger hydrological impacts than the annual summer monsoon (Cook et al., 2018). Research like this holds implications for human systems and infrastructure in GLOF-prone areas. Though GLOFs are rare in comparison to storm events, they can have much larger and more damaging impacts. There is thus a clear need to think of and communicate glacial outburst floods as unlikely but extreme events.
Study Sites
Juneau, Alaska
The GLOFs originating in the Suicide Basin glacier dammed lake present a unique opportunity to analyze and understand more direct societal and economic impacts of GLOF events on a downstream community, given the close proximity of Suicide Basin to the city of Juneau, the state capital of Alaska (Figure 1). Numerous homes are in the floodplain of Mendenhall River, which the GLOFs drain into, and have been affected in previous GLOF events. Additionally, US Forest Service campsites along Mendenhall Lake are closed during large GLOFs, and tour operators on and around Mendenhall Lake have canceled tours during GLOFs due to unsafe conditions.
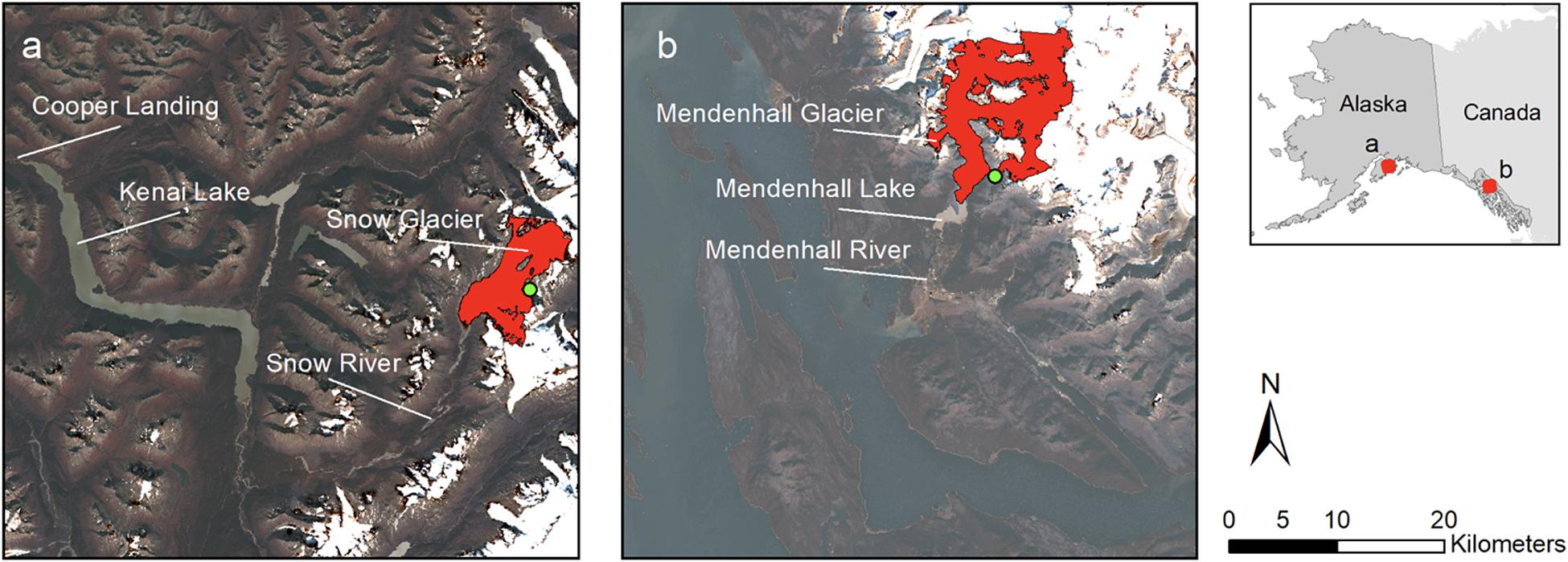
Figure 1. Location of Snow Glacier (a), Kenai Peninsula and Mendenhall Glacier (b), Juneau in Alaska. Green dots show the location of the studied glacial lakes. Background imagery is based on LandsatB images from 2018. The glacier outlines (in red) are based on (Kienholz et al., 2015) JG!ac Inventory (DOI: 10.3189/201SjoG14J230).
Suicide Basin is an approximately 0.7 km2 ice-covered basin that sits roughly ∼3 km up-glacier from the terminus of Mendenhall Glacier in the Mendenhall Valley (Kienholz et al., 2020). The first reported GLOF from Suicide Basin was in 2011. Since then, Suicide Basin has annually released one or more outburst floods into Mendenhall Lake via Mendenhall Glacier, raising water levels in both Mendenhall Lake and Mendenhall River to varying degrees (Morgan et al., 2013; Kienholz et al., 2020). Although the largest GLOF recorded thus far was in 2016, this research (2018–2019) came at an opportune time, given that 2018 was the third largest GLOF on record (National Weather Service Advanced Hydrologic Prediction Service, n.d.). A GLOF occurred in 2019 as well though it was not of record magnitude (National Weather Service Advanced Hydrologic Prediction Service, n.d.). Overall, four of the seven largest floods in the Mendenhall River streamflow record (1966-present) have occurred in the last 8 years as a result of GLOFs (USGS, n.d.).
Kenai Peninsula, Alaska
GLOFs from the Snow Glacier dammed lake, approximately 0.8 km long and ∼140 m deep and located about 40 km northeast from Seward, have occurred approximately every 2–3 years raising water levels in the Snow River on the Kenai Peninsula (Figure 1; Wilcox et al., 2014). The earliest reported GLOF from Snow Glacier was in 1949 (Glacial Dammed Lake Data, n.d.). Though flooding from these GLOF events has not historically caused widespread damage to life or property, communities have experienced, particularly in recent events, nuisance flooding, damage to various transportation-related infrastructure, as well as negative impact on the local economy due to the cancelation of tour operations from heightened river and lake levels. Typical GLOF events from Snow Glacier can raise river water levels several meters in communities like Cooper Landing and Kenai Keys on the Kenai Peninsula. These events can increase debris and sediment in the Kenai River and impact fish, particularly salmon runs, with implications for the sport fishing and tourism sectors on the Kenai Peninsula, both of which play a significant role in the local economy.
Challenges With Forecasting GLOFs
Forecasting GLOF occurrences as well as predicting their respective inundated areas, particularly from a risk assessment perspective, is challenging given the limited amount of quantitative or observational data regarding this type of hazard (Worni et al., 2014). The occurrence and magnitude of a GLOF is dependent on both the climate-driven changes in glacier dynamics as well as year-to-year weather variability, impacting the ability to accurately forecast the timing and magnitude of a GLOF (Li and Sheng, 2012).
Given the uncertainties associated with forecasting GLOFs, and potential compounding effects from other climate change-induced events, which also involve interannual variability (such as increased snowmelt and precipitation), information developers fundamentally want to accurately communicate hazard levels to communities in the surrounding areas. Specifically, information developers are interested in learning how to better communicate the uncertainties associated with their hazard forecasts and thus provide products that are understandable to users. For example, the National Weather Service’s (NWS) Alaska-Pacific River Forecast Center (APRFC) is responsible for issuing all flood-related forecasts in Alaska, which in turn are used by the NWS Weather Forecast Offices (WFO) to issue local forecasts and outlooks to emergency managers, the media, and the public in their jurisdiction.
Beyond the need to create understandable products, creating products that are perceived as useful by target end users is another fundamental goal for information developers, particularly in a hazard context. The APRFC maintains current and predicted hydrographs and time series plots of discharge or streamflow for downstream GLOF-affected lakes and rivers. These hydrographs are utilized by the WFOs and are one of the main resources communities use to understand and prepare for a GLOF. Therefore, feedback on how and if communities utilize these hydrographs is critical for understanding the information needs and use of information users in GLOF-affected communities in Alaska.
Materials and Methods
Research Phases
Case study research was conducted to assess the use of GLOF information products in different, but relatively comparable, communities. The Juneau case study was a longitudinal study conducted over the course of 2 years (2018–2019), to assess whether participants experienced any changes in (1) their perception of the utility of GLOF predictive modeling and (2) their use of GLOF predictive modeling. The Juneau case study underwent Phases I–III discussed below. The Kenai Peninsula case study was conducted over the course of 1 year (2019), to assess the transferability of phase I of the methodology in a different site location. A longitudinal assessment of the Kenai Peninsula case study (e.g., Phases II–III) was not possible for this research due to the fact the Snow Glacier GLOF occurs at a different time scale than the Suicide Basin GLOF (every 2 or 3 years and every year, respectively).
For Phase I (baseline assessment of GLOF familiarity and information product usage), we conducted interviews in Juneau during the 2018 GLOF and on the Kenai Peninsula during the 2019 GLOF. The purpose of Phase I was to gather a baseline assessment of each interviewee’s familiarity with: (a) GLOFs, (b) the current GLOF information products available, and (c) their use, if at all, of these products. In addition, Phase I assessed interviewees’ preferred formats (written, oral, visual) and media (email, radio, social media) for receiving and using information. The information collected during Phase I provided insight into decision-making needs regarding GLOFs and feedback on information gaps or suggested improvements in the current information landscape. Information developers, a subset of the overall interviewees, were asked to answer a few additional questions to help assess their willingness and ability to integrate user feedback into their products as part of this research (Phase II). Please see “Research Participants” section for a detailed description.
We conducted Phase II (sharing user feedback with information developers and new product development by information developers) for the Juneau case study only. Table 1 lists the information developers for the Juneau case study. Phase I findings were shared with the identified information developers in the Juneau case study. After the Phase I findings were shared, information developers were asked to relay their uptake and understanding of the user feedback as well as their plans to adapt their products and tools to better meet user needs. The results from these feedback-relaying sessions helped to inform changes in the public-facing GLOF information products for Juneau.
Phase III (user feedback on new products) was also conducted in the Juneau case study only. A second round of interviews was set up with the 2018 Juneau interviewees during the following year’s GLOF event (2019). Interviewees engaged in user testing of the new informational products developed during Phase II. The interview questions for Phase III were developed and grounded in the literature on user testing and tool development (Cao et al., 2009; Som de Cerff et al., 2018). Interviewees were asked a series of interview questions after the user testing, to assess whether the modified / new tools met their identified needs. Interviewees were also asked for any suggestions for further improvements to the GLOF informational landscape.
Research Participants
An analysis of the two case study communities identified the following interviewees as the most integral community representatives, from the perspective of decision-making regarding GLOFs as well as potential to be affected by GLOFs (Table 1). Interviewees were grouped into five different types of organizations: Homeowner, Federal Agency, Local Agency, Local Business, and State Agency. The same organization groups were identified for both case studies. For the Juneau 2018 interviews, the largest interviewee pool representation was from local agencies (30% of 33 interviewees). However, for the Juneau 2019 interviews, the largest interviewee pool representation was from homeowners, (35% of 20 interviewees). For the Kenai Peninsula case study, the largest interviewee pool representation was from federal agencies (54% of 13 interviewees).
We also distinguished whether an interviewee was an information developer or an affected party regarding the GLOF events for the purposes of Phase II of this research, in order to assess their ability to make amendments to information products. Information developers were otherwise deemed as information users for both Phase I and III of this research. Four information developer organizations were identified (Table 1). However, the University of Alaska Southeast is only an information developer for the Juneau case study because no university researchers are actively involved in the Snow Glacier GLOF monitoring.
We identified interviewees based on a number of different parameters. Information developers (federal and state agencies) were identified via informational meetings with the NWS APRFC, NWS WFOs in Juneau and Anchorage, USGS in Juneau and Anchorage, and the University of Alaska Southeast. Via these meetings, all relevant information developers were identified for both study sites. Affected parties (local agencies, homeowners, and businesses) were identified via these meetings as well, particularly for all the local agencies involved in emergency management as well as homeowners in high-risk areas. Businesses were identified via discussions with the US Forest Service in Juneau, who maintains a list of all active businesses operating on Mendenhall Lake, as well as with the local visitor centers in the Kenai Peninsula, who have records of all active business operations on the Snow and Kenai River. Public notices for further community input were sent out via the NWS Twitter accounts in Juneau and Anchorage and via local newspapers. Lastly, we also utilized a snowball sampling method to identify further interviewees (Handcock and Gile, 2011).
A total of 37 unique individuals were interviewed for the Juneau case study over the 2 year study: 33 individuals in 2018 and four additional individuals in 2019. Of the 33 people interviewed in Juneau in 2018, 16 were re-interviewed in 2019. Of the 17 individuals not interviewed again in 2019, five were no longer in the same position, six did not respond to the re-interview request, and five we were not able to contact. Though there were four new interviewees in 2019, two of these did not provide meaningful responses to all of the interview questions and were therefore not included in the analysis. Thus, the total number of interviews analyzed for the Juneau case study is 35.
Of the 33 Juneau 2018 interviewees, 30% were identified as information developers. All of the information developers were associated with either a federal agency or a state agency. The remaining interviewees, 70%, were identified as an affected party. Although the overall number of Juneau interviewees was different in 2019, the ratio of information developer to affected party (30–70%) remained the same.
A total of 13 unique individuals were interviewed for the Kenai Peninsula case study. Of the 13 Kenai Peninsula 2019 interviewees, 46% of the total were information developers, leaving the remaining interviewees (54%) as an affected party.
Interviews and Coding
Interviewees were asked about five information products for the Juneau case study: (1) NWS APRFC Mendenhall Lake hydrograph, (2) NWS flood advisory and flood watch statements, (3) NWS inundation map of Juneau, (4) US Geological Survey (USGS) time-lapse camera images of Suicide Basin, and (5) USGS stream gage at Suicide Basin (see Supplementary Appendix A for examples of the analyzed information products). In addition, as part of phase II and III for the Juneau case study, interviewees were asked about a newly developed product (June 2019), a webpage hosted by the NWS Juneau Forecast Office that has all the aforementioned materials compiled on one page.
For the Kenai Peninsula case study, we inquired about three information products that are directly comparable to those analyzed in the Juneau case study: (1) NWS APRFC Snow and Kenai river systems hydrographs, (2) NWS flood advisory and flood watch statements, and (3) USGS stream gages along the Snow and Kenai Rivers, as well as a fourth product, (4) Civil Air Patrol photos, which provided a similar, but not exact, comparison to the USGS time-lapse camera imagery from the Juneau case study (see Supplementary Appendix A for examples of the analyzed information products). At the time of this research, USGS time-lapse camera imagery was not available to be analyzed nor was there a comparable inundation map for the Snow Glacier watershed to be used in the Kenai Peninsula case study.
For Phase I, a structured interview protocol (see Supplementary Appendices B,C for the interview questions for the Juneau and Kenai Peninsula case studies, respectively) was used to ask interviewees about: (1) their experiences and familiarity with GLOF events, (2) their familiarity and use of the aforementioned, respective GLOF informational products, (3) their preferred method of information provision, and (4) their preferred mediums to receive information.
A structured interview protocol was also used for Phase II (see Supplementary Appendix B), to share interviewee feedback from Phase I on the existing GLOF information products with information developers for the Juneau case study. Findings from Phase II helped to inform the development of public-facing graphs and time-lapse animations, created by University of Alaska Southeast researchers, as well as the new Suicide Basin monitoring website, hosted by the NWS Juneau WFO. Lastly, for Phase III, a structured interview protocol (see Supplementary Appendix A) was also utilized to collect feedback from Juneau interviewees regarding the new GLOF information product that was developed.
All interviews were recorded and transcribed with the consent of the interviewees. Given that the interview protocol was structured, interview analysis consisted of coding the transcriptions so that the information could be synthesized and analyzed systematically. Interviews were coded twice by one coder to ensure the information was accurately reported and relayed. Interviews were also analyzed using qualitative content analysis for themes related to: challenges of using GLOF informational products, the use of GLOF informational products in decision-making, challenges to data use in general, and lessons learned and recommendations for future GLOF events (Mayring, 2004).
Theory
Decision Support Tools
Decision support tools are resources that help support a variety of decision tasks including information gathering and analysis, model building, and decision implementation (Bhargava et al., 2007). Decision support tools do not necessarily have to be computer-based, or web-based. However, most literature defines them as being at least computer-based (Welp, 2001). The information technology literature, where the concept of decision support tools first emerged in the early 1970s, argues that the two most widely utilized methods to implement a decision support tool are via data-driven and model-driven approaches (Morton, 1971). Data-driven approaches support managers in utilizing a large amount of pertinent information while model-driven approaches help managers in using analytical and/or predictive models (Bhargava et al., 2007).
Decision support tools hold a different meaning in the natural resource management literature, where their use is focused less on analytical simulation and more on communication, training, forecasting, and/or experimentation (Van de Ven et al., 1998). Natural resource management decision support tools also differ from traditional information technology tools in that they can integrate ordinary knowledge, e.g., qualitative information, into modeling efforts. Although such information can provide richer and more robust meaning to a model’s outputs, it can also complicate modeling efforts. Thus, it is important to develop decision support tools in a participatory manner, as early as possible, with applicable stakeholders and decision-makers so that these nuances can be accounted for, tested, and iterated upon in a meaningful manner (Welp, 2001). For this research, we utilized a combined definition of decision support tool, based on (a) the model-driven system definition from the information technology literature and (b) the natural resources management definition of decision support systems, which emphasizes stakeholder engagement.
Usability of Decision Support Tools in Flood Management
The existing literature on evaluating the use and usability of decision-support tools in natural hazard planning and response encompasses a variety of hazard scenarios. Flood management lends itself well to use of decision support tools given that completely predicting and controlling the impact of a flood is seldom, if ever, possible. Ahmad and Simonovic (2006) describe the flood management process as a three-phase process that consists of: (1) pre-flood planning, (2) flood emergency management, and (3) post-flood recovery. In this context, an effective decision support tool should provide usable information for the different decisions that need to be made at each of the aforementioned phases.
Ahmad and Simonovic (2006) assessed the applicability of the Decision Support for Management of Floods (DESMOF) tool via a case study application in the Red River basin in Manitoba, Canada. Their research involved assessing the tool’s features with flood managers. Although their research focused on engaging stakeholders and decision-makers and eliciting their feedback, they did not utilize this feedback to revise or change their tool. Rather, their research focused on assessing whether such a tool could be helpful to decision-makers. Todini (1999) conducted a similar review of a flood decision support system (FLOODSS) in Italy, to see how it could better meet the needs of its users and found that in the context of flood management, users are generally meteorologists, operational managers, and civil response managers for whom effective decision support tools were shown to integrate both technical and social components of floods. Despite Todini’s (1999) focus on the importance of addressing the social subsystem in a decision support tool, his research did not include specific components of stakeholder engagement nor iterative development based off stakeholder feedback.
Levy (2005) addressed how multiple criteria decision-making can support the transfer of knowledge about flood processes as well as the facilitation of coordination between flood decision-makers and affected citizens. Levy (2005) also did not include any stakeholder engagement or feedback incorporation in his research design. However, he stresses the need for human-computer interface analyses to better facilitate knowledge transfer, increase decision-making transparency, improve communication, as well as make linkages and assumptions more explicit and understandable to flood information users (Levy, 2005).
Although there are few studies that have explicitly explored the use of decision support tools in the GLOF context, there is a growing body of literature on the potential socioeconomic benefits of using GLOF monitoring for hazard assessments (Huggel et al., 2002; McKillop and Clague, 2007; Shrestha et al., 2010; Wang et al., 2011; Klimeš et al., 2014; Rounce et al., 2016; Allen et al., 2019; Thompson et al., 2020).
Results
Phase I Results: Baseline Assessment of GLOF Familiarity and Information Product Usage
Previous Experience With GLOF Event
In both case studies, the majority of interviewees had experienced a GLOF event prior to the interview. In the Juneau case study, there were only four out of 35 interviewees who had not previously experienced a GLOF. In the Kenai Peninsula case study, five out of the 13 interviewees had not previously experienced a GLOF.
It is worth noting the difference in the interviewees’ self-expressed understanding of the concept of a GLOF between the two different case studies. Interviewees were asked to quantify their understanding of the concept of a GLOF on a scale of 1–5, with 1 being the least familiar and 5 being the most familiar (Table 2). For the Juneau case study, there was a higher self-reported understanding of the concept compared to the Kenai Peninsula case study. This difference can possibly be due to fewer Kenai Peninsula interviewees having experienced a GLOF compared to the Juneau interviewees.
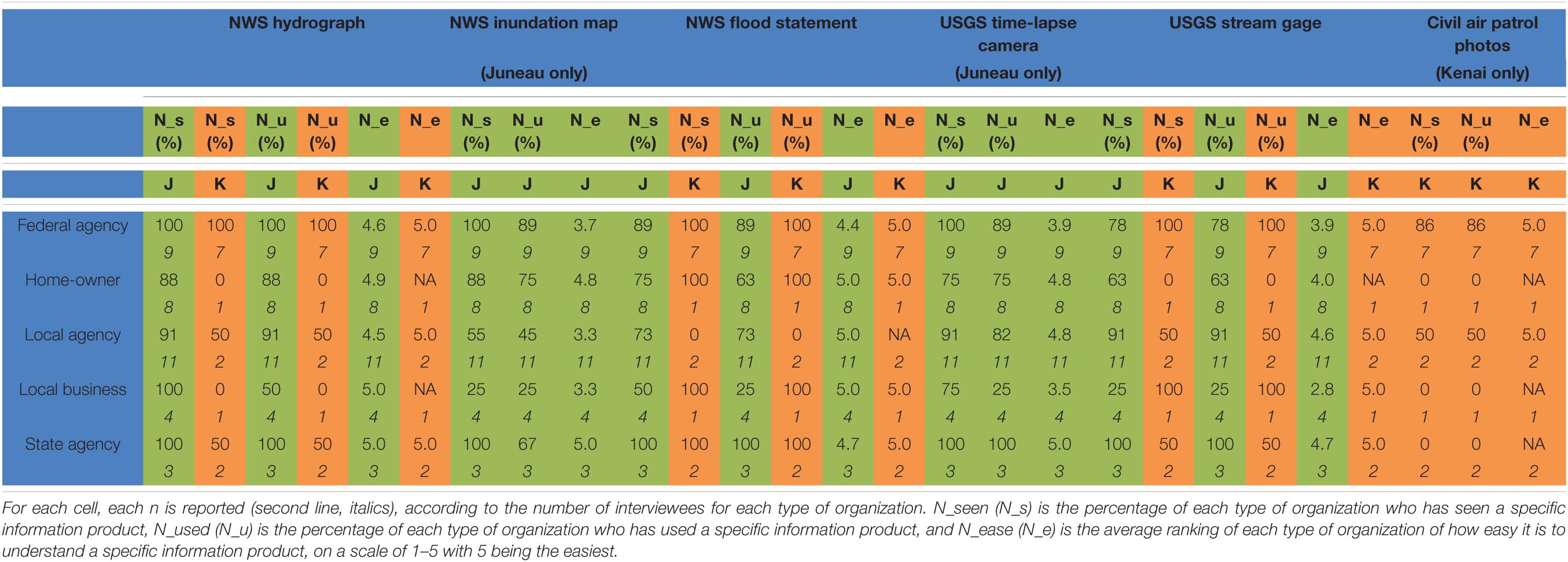
Table 2. Interviewee use and understanding of information products, by type of organization for the cumulative Juneau (n = 35, orange shading) and Kenai Peninsula (n = 13, green shading) case studies.
Use of GLOF Information Products
The NWS hydrograph was the most understood and used information product for the Juneau case study: 98% of interviewees reported having seen and used the NWS hydrograph (Table 2). The NWS hydrograph and the USGS stream gage were the only two information products that did not have any difference between whether a user had seen and used the product. Homeowners did not report a difference in seeing and using an information product except for the NWS inundation map. All homeowners in Juneau reported they had seen the NWS inundation map but only 86% of them had actually used it (Table 2).
In the Kenai Peninsula case study, there was no reported difference between seeing and using any of the information products. Compared to the Juneau case study, the NWS flood statement was the most understood and used information product: 100% of the Kenai Peninsula interviewees reported having seen and used the NWS flood statement. There was generally less usage of the various information products in the Kenai Peninsula case study compared to the Juneau case study. This was seen across the various different information products and across organization groups.
Preferred Media and Formats to Receive Information
For the Juneau case study (Figure 2A), the top three media through which interviewees preferred to receive information in were: e-mail (77% of 35 interviewees), text message (71%), and social media (46%). E-mail was the preferred medium among federal agencies, while homeowners preferred text messages. Local agencies, local businesses, and state agencies were tied between email and text message as their preferred method to receive information. No organization group had social media as their top preferred method.
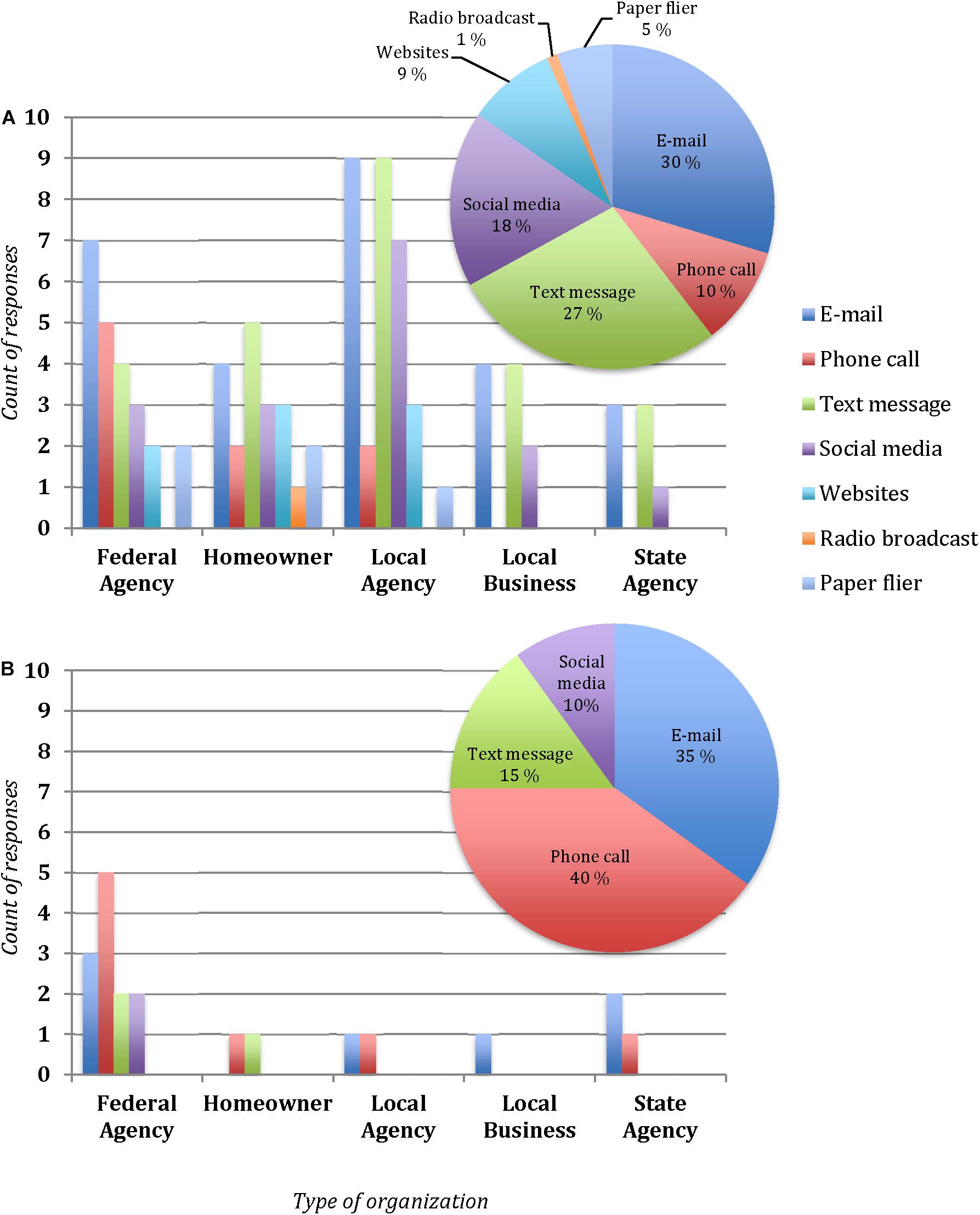
Figure 2. Preferred media to receive GLOF information by type of organization for (A) Juneau interviewees (n = 35) and (B) Kenai Peninsula interviewees (n = 13). Interviewees could list multiple preferred media, thus number of responses exceed number of interviewees. The pie chart is an aggregated representation of all interviewee responses in each case, respectively.
For the Kenai Peninsula case study (Figure 2B), phone calls were the preferred medium to receive information (62% of 13 respondents), followed by e-mail (54% of 13 respondents) and text messages (23% of 13 respondents). State agencies and local businesses reported e-mail as their preferred medium while federal agencies reported phone calls. Homeowners were tied between phone calls and text messages and local agencies between phone calls and emails as their preferred medium. Finally, local businesses preferred email. The main difference between the preferred media in both case studies was the difference in phone calls and social media, where phone calls played a larger role in the Kenai Peninsula case study and social media for the Juneau case study.
The preferred format for receiving information in the Juneau case study was via graphs and charts, followed by written narratives and face-to-face communication (Figure 3A). Graphs and charts were the preferred format for all organization groups apart from homeowners, where written narratives were identified as the preferred format.
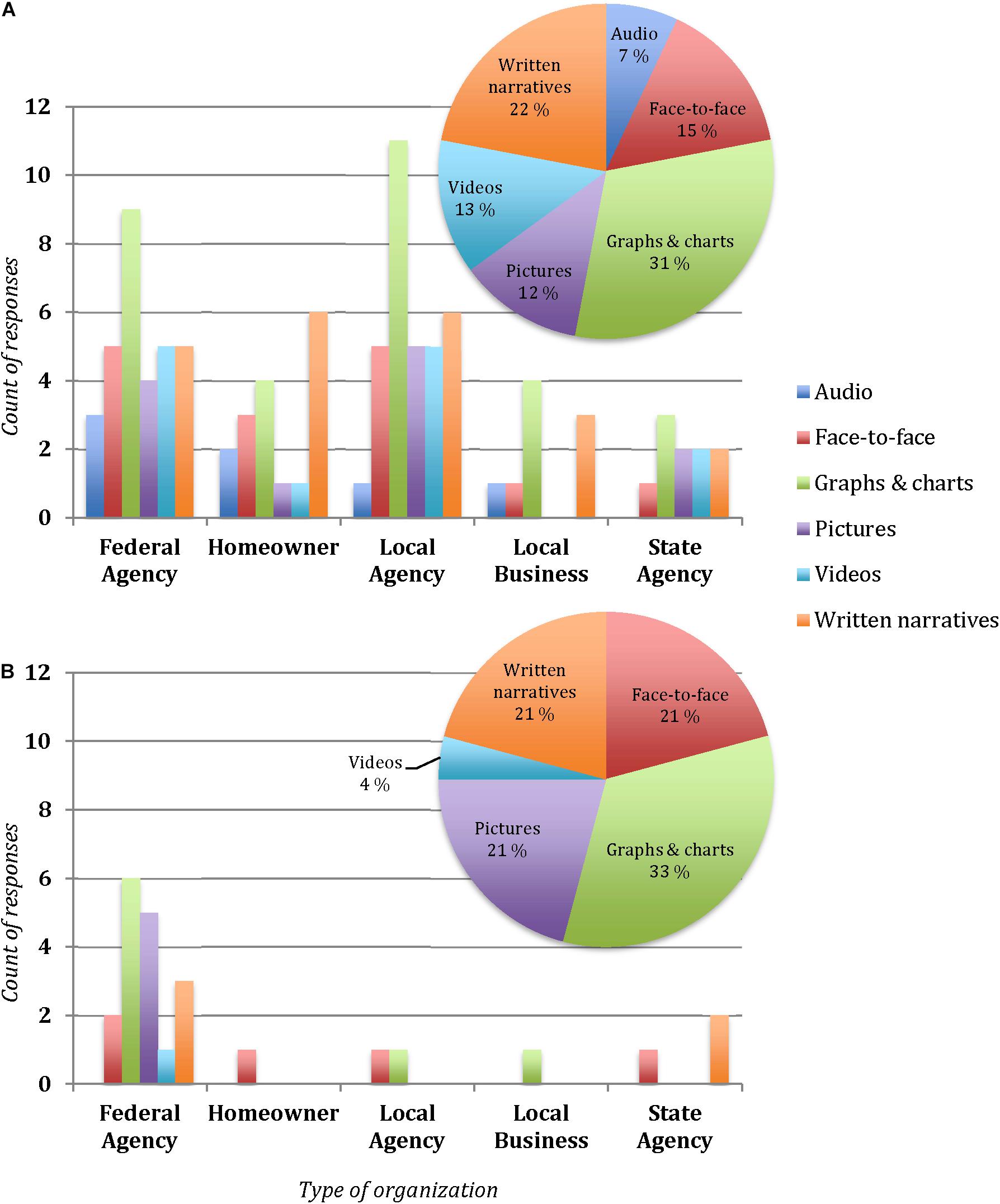
Figure 3. Preferred formats to receive GLOF information by type of organization for (A) Juneau interviewees (n = 35) and (B) Kenai Peninsula interviewees (n = 13). Interviewees could list multiple preferred formats, thus number of responses exceed number of interviewees. The pie chart is an aggregated representation of all interviewee responses in each case, respectively.
In comparison, graphs and charts were also identified as the overall preferred format by interviewees in Kenai Peninsula case study (Figure 3B). However, there was a three-way tie between face-to-face communication, pictures, and written narratives for the second preferred format. Federal agencies (n = 7) and local business representatives (n = 1) indicated graphs and charts were their preferred format and homeowners indicated written narratives, which is similar to the Juneau case study. However, local agencies (n = 2) were tied between face-to-face communication and graphs and charts as their preferred format meanwhile state agencies (n = 2) preferred written narratives.
Phase II Results: Sharing Feedback With Information Developers and New Product Development
This research identified preferred informational needs for different user groups regarding GLOF events and whether current informational products are meeting those needs. Collecting this user information helped inform the development of new information products in Juneau to better meet the main identified user need for having information regarding GLOFs displayed on a single webpage, as well inform modifications to existing informational products for the Kenai Peninsula. In particular, user feedback from the Juneau case study led directly to the development of a new GLOF monitoring website for Suicide Basin. The website was hosted by the NWS and compiled with information regarding the status of the Suicide Basin GLOF that was collected by the NWS, USGS, and the University of Alaska. Importantly, this new website served as a “one-stop shop” for information users, a request that came through via multiple interviews. Users previously had visit to at least three different web pages to find information about Suicide Basin. By putting all the information in one place, it was also much easier to link information from different products, helping users better understand the overall hazard situation associated with the Suicide Basin GLOF.
Following the success of the new Suicide Basin monitoring website over the 2019 GLOF season, a similar site was also set-up by the NWS, compiled with information collected from the NWS and USGS, in time for the Snow Glacier GLOF later that summer. Although the 2019 Kenai Peninsula interviews were conducted prior to the new website going live, informal feedback collected thereafter showed that the new website was well received by users.
Phase III Results: User Feedback on New Product
Interviewees during Phase I, II, and III had different preferences for how they wanted to see information and how they wanted to receive information; however, it was clear across all interviewees for Phase III, that they appreciated the new one-stop-shop Suicide Basin monitoring page, as it helped them to easily and readily access information on GLOF events. Importantly, all interviewees indicated they were very pleased that the information developers worked to meet their needs. Many interviewees indicated they have a need to feel “connected” to someone knowledgeable about GLOF events and participating in this study helped to meet that need. In addition, seeing the new product go online and that their feedback was utilized by information developers as part of that process was also assuring.
Nonetheless, nearly all interviewees expressed a desire to have face-to-face meetings, receive texts, or participate in community meetings to educate themselves about the GLOFs. The hazardous potential of GLOFs along with the fascination that people have living alongside these active glaciers increases interviewees’ desire to learn more about the process of GLOFs as well as their future outlook and impact on their communities. As one interviewee (homeowner) mentioned,
“I have questions about the long-term outlook of the glacier. It kind of connects us to it in a way that might not be there for everyone. It impacts decisions about our home that we make. Until I know for certain that the GLOFs are not going to get past a certain level into our house, I don’t want put new hardwoods in only to have them get ruined.”
Interviewees in the Juneau case study expressed an interest in having more engagement with scientists studying GLOFs to learn more about both the processes at play behind these events as well as their projected impacts and changes. Several interviewees expressed an interest in having community forums where they can discuss and document the impacts they have experienced from GLOFs, so that there is a formalized record of what is happening on a local level, and to also serve as a liaison for scientists and agencies wanting to know more how these events impact communities in Alaska.
Discussion
Novel Approach to Assessing Use of GLOF Information Products
This research served as a prototype for GLOF information developers on how to integrate user-driven feedback into their product offerings and communication strategies; several studies have highlighted that social science-based research like this has not been previously done for GLOF-impacted communities (Sherpa et al., 2019; Shreevastav, 2019; Welling et al., 2019). Given the unique nature of GLOFs, particularly the scientific challenge in forecasting GLOF occurrences as well as the magnitude of, and risks associated with, a GLOF event, special attention is warranted on understanding how to communicate GLOF-related information with affected stakeholders. Specifically, this research created a methodology and blueprint for how to elicit user input for GLOF-related information development and communication and how to integrate this input back into existing information products. This research builds on the existing decision support tool literature providing a reproducible, transparent, and relevant process structure for future user elicitation and integration endeavors. In particular, it is important for information and service providers, such as the NWS, to clearly understand user concerns so that tools and communication products target actual, vs. perceived, user needs. This research also helped support information developers, such as the NWS, in fulfilling their strategic mandates to create more tailored products for specific end users.
Three main findings emerged from our analysis. Firstly, there is a difference in information product understanding and use by interviewees. An interviewee’s use of information products varied with their familiarity with GLOFs as well as their previous experiences. As one interviewee (homeowner) mentioned, “I’m not concerned anymore since I’ve seen this happen for a long time so unless it’s expected to be higher than what I’ve already seen, I’m not too worried.” This suggests that some interviewees, particularly those that have had previous, direct experiences with a GLOF, do not use the information products since they feel comfortable with their acquired knowledge of GLOFs and their impacts. Though this speaks highly to the amount of local knowledge that exists regarding these events, there is potential concern that some of these interviewees could be at-risk for serious impacts if they are not monitoring early warning information and an unprecedentedly large GLOF event occurs (Sellnow and Seeger, 2001).
Secondly, there are differences in the understanding of GLOF-related information by interviewees in the two different case studies. In comparison, the Kenai Peninsula interviewees were less familiar than the Juneau interviewees with the concept of GLOFs. This can be due to a number of reasons. Snow Glacier is further away from populated areas when compared to Suicide Basin. However, there is more reported monetary damage to infrastructure and property in the Snow Glacier watershed due to GLOFs compared to Suicide Basin. This initially seemed like a surprising finding—many interviewees were not familiar with the Snow Glacier GLOFs but they were aware of their impacts. One interviewee (local agency) illuminated this point during our discussion, “we get a lot of high water events here, whether it’s coming from rain, snowmelt, or a glacier, it doesn’t really matter to us.” Similarly, although there is a wealth of local knowledge and familiarity regarding high water events on the Kenai Peninsula, GLOFs can have catastrophically large impacts, particularly if they are compounded with a high rainfall event or snowmelt season. The Kenai Peninsula is at-risk for such a compounded GLOF event, given the area’s predisposition for high water events and increased reliance on past, local knowledge. This is a similar finding to other flood literature, where assessments of severe floods (1998, 2000) in the United Kingdom found that groups that were at-risk during these events was not due to their lack of awareness; instead, they underestimated the magnitude of these unprecedented events due to their reliance on local knowledge acquired from previous flood events (Burningham et al., 2008). Therefore, there is clear benefit and need to communicate the potential risk from an unprecedented GLOF on surrounding communities, and to ensure that equally robust monitoring efforts take place in the Snow Glacier drainage system, as is currently taking place via the University of Alaska Southeast for the Juneau area (Sellnow and Seeger, 2001).
Lastly, despite the differences in how interviewees want to see information and ultimately use it, the feedback from the Juneau case study Phase I results indicated clearly that all interviewees wanted information compiled in a centralized location such as a single website. Many interviewees indicated in a hazard context, they want to be able to access GLOF information in one place, so that they can make quick and informed judgment. This piece of feedback was well-received by information developers in Phase II and via the NWS, USGS, and the University of Alaska Southeast, a one-stop-shop webpage to monitor information on Suicide Basin was developed prior to the 2019 flood season. Findings from the Phase III interviews, in which this new informational product was assessed, showed that unanimously, all interviewees approved of and appreciated the new informational product. Over the course of 2 years, interviewees in Juneau indicated they appreciated seeing information developers taking their feedback seriously and being part of a research project that provided actionable and meaningful results to their community.
While community-specific, these findings speak to the success and importance of engaging information users in an iterative process in decision-support tool development (Cao et al., 2009; Som de Cerff et al., 2018). The information developers we interviewed found this research to be very beneficial as it enabled them to better understand and therefore more effectively meet user needs. As such, our work supports the importance as well as the utility of boundary spanning—the providing of a bridge between the information developer and information user worlds—in a cryospheric hazard context. This is consistent with previous research emphasizing the significance of boundary spanning in the context of climate change and flood hazards (Guston, 2001; Morss et al., 2005; Briley et al., 2015; Hering, 2016; Kettle et al., 2017).
Limitations
The methodology created and utilized in this research identified good practices for how to elicit and integrate user feedback into decision support tools that are created to help inform and support decision-making regarding GLOF hazards. Lessons learned from the Juneau case study were used to assess the transferability of the developed methodology regarding user needs in GLOF forecasting to another community (the Kenai Peninsula). However, there were differences in interviewee pool composition and overall sample size that should be considered when comparing results. Furthermore, due to limitations in predicting GLOFs and the different timescales of the GLOF events for both case studies (annually for the Juneau case study, and every 2–3 years for the Kenai Peninsula case study), a longitudinal study was not possible for the Kenai Peninsula case study. However, the way this research was structured, in which the second year interviews for the Juneau case study looked at the user feedback for the newly developed GLOF monitoring product, comparing the two case studies on the basis of Phase I of this research was nonetheless possible.
In addition, there were statistical limitations, due to the small and different, sample sizes between the two case studies. Particularly due to the small sample size for the Kenai Peninsula case study (n = 13). As a result, we did not test for statistical significance. The information presented in this paper is relayed in a qualitative manner since the results are limited in their ability to provide broader generalizations and implications, such as regarding how GLOF information products can be presented in other GLOF-prone communities as well as how to engage and strengthen relationships between GLOF information developers and information users in order to enhance GLOF informational products. Lastly, more interviews would be needed to assess whether results between the two study sites and differences in within each case study are statistically significant.
Future Research and Recommendations
It would be ideal to collect user feedback on the newly developed GLOF monitoring product for the Kenai Peninsula case study during the next GLOF (∼2021 or 2022) so that the findings can be compared with the feedback collected during the 2019 Juneau interviews. In addition, it would be worthwhile to compare the findings from this research with case studies from GLOF communities in other parts of the world, to see if the findings are specific to the Alaska context or transferrable to other areas.
Conclusion
Although this research was focused on decision-making under uncertainty regarding cryospheric hazards–an under-researched hazard in current user engagement literature, as discussed in the “Theory” section. This research sought to also create generalizable principles and lessons learned for potential application in other hazard scenarios. Namely, we demonstrated that there is benefit from conducting a formalized user assessment to understand user needs as well as user comprehension of decision support tools, particularly those that are utilized in a GLOF hazard context. The feedback gathered from users also helped informed the development of a new GLOF-related information website for Suicide Basin, providing information developers an opportunity to understand how their products are being used and what are areas for further expansion and enhancement.
Lessons learned from this research can benefit those working in the GLOF sector but also other entities working to streamline and integrate community and user feedback into their products and resources, particularly those focused on community-based hazards. Taking the time to talk to users to understand their needs and concerns is important for information developers (Lemos and Morehouse, 2005). It helps to build trust within the community, but also opens the avenue to understand what is important to information users, such that information products can be better tailored to real needs. Furthermore, information users in a hazard context also have their own supply of local knowledge, given their past experiences with hazard events and their own successful adaptation strategies (McEwen et al., 2012; McEwen and Jones, 2012). It is in the interest of communities as a whole to find ways to utilize this type of local knowledge, particularly in remote areas where observational data may be scarce. However, it is important to note the potential limitations of the transferability of some the recommendations from this study regarding how GLOF information can be presented to stakeholders in other contexts. Given that many GLOF-prone communities are in remote locations, with limited access to WiFi and Internet connectivity in general, the ability to utilize web-based platforms as a means to communicate GLOF-related information may not possible nor appropriate. Therefore, further investigation regarding the best possible information communication and dissemination channels is important to take into consideration regarding each GLOF-prone community context.
In this project, community members in both case studies expressed a desire to be able to contribute to the dialogue and information provision on GLOF events. The willingness of interviewees to participate and provide meaningful feedback, as well as the willingness and ability of information developers to create new information products to address this feedback was deemed as a major success of this iterative, community-based research. This highlights that co-production, boundary spanning, and community-based knowledge development can help empower and strengthen the relationship between communities and information developers and offer new avenues for engaging in meaningful two-way dialogue and collaboration (Shaw et al., 2013; Meadow et al., 2015; Nel et al., 2016; Bednarek et al., 2018; Posner and Cvitanovic, 2019). This case-based research demonstrates the benefit of engaging information developers and affected community members with each other in order to better understand and prepare for hazardous events.
Data Availability Statement
The raw data supporting the conclusions of this article can be made available by the authors, without undue reservation.
Ethics Statement
The studies involving human participants were reviewed and approved by the Institutional Review Board. Written informed consent for participation was not required for this study in accordance with the national legislation and the institutional requirements.
Author Contributions
DA-F designed the project, led the data collection in 2018 and 2019, analyzed all the data, and wrote the manuscript. ST, EH, RH, and CK provided feedback on the project design and commented on the manuscript. All authors contributed to the article and approved the submitted version.
Funding
This work was supported by the Alaska Center for Climate Assessment and Policy NOAA Award NA16OAR4310162, USDA National Institute of Food and Agriculture Hatch project 1018914, and the State of Alaska.
Conflict of Interest
The authors declare that the research was conducted in the absence of any commercial or financial relationships that could be construed as a potential conflict of interest.
Acknowledgments
We thank the National Weather Service Alaska-Pacific Forecast Center, the National Weather Service Juneau and Anchorage Forecast Offices, the City and Bureau of Juneau, and the U.S. Geological Survey Field Office in Juneau for their support and comments throughout the research design that helped to strengthen this research. We also thank the communities and interviewees in Juneau and the Kenai Peninsula for providing their insights and time, without which, this paper would not have been possible.
Supplementary Material
The Supplementary Material for this article can be found online at: https://www.frontiersin.org/articles/10.3389/feart.2021.635163/full#supplementary-material
References
Ahmad, S., and Simonovic, S. P. (2006). An intelligent decision support system for management of floods. Water Resour. Manag. 20, 391–410. doi: 10.1007/s11269-006-0326-3
Allen, S. K., Rastner, P., Arora, M., Huggel, C., and Stoffel, M. (2016). Lake outburst and debris flow disaster at Kedarnath, June 2013: hydrometeorological triggering and topographic predisposition. Landslides 13, 1479–1491. doi: 10.1007/s10346-015-0584-3
Allen, S. K., Zhang, G., Wang, W., Yao, T., and Bolch, T. (2019). Potentially dangerous glacial lakes across the Tibetan Plateau revealed using a large-scale automated assessment approach. Sci. Bull. 64, 435–445. doi: 10.1016/j.scib.2019.03.011
Bajracharya, S. R., Maharjan, S. B., Shrestha, F., Sherpa, T. C., Wagle, N., and Shrestha, A. B. (2020). “Inventory of glacial lakes and identification of potentially dangerous glacial lakes in the Koshi, Gandaki, and Karnali River Basins of Nepal, the Tibet Autonomous Region of China,” in International Centre for Integrated Mountain Development GPO Box 3226, Kathmandu, (Kathmandu, Nepal: Nepal and United Nations Development Programme (UNDP) GPO Box 107).
Becker, M. (2018). “The role of intermediaries in flood risk management,” in Oxford Research Encyclopedia of Natural Hazard Science, (Oxford: Oxford University Press).
Bednarek, A. T., Wyborn, C., Cvitanovic, C., Meyer, R., Colvin, R. M., Addison, P. F. E., et al. (2018). Boundary spanning at the science–policy interface: the practitioners’ perspectives. Sustain. Sci. 13, 1175–1183. doi: 10.1007/s11625-018-0550-9
Bhargava, H. K., Power, D. J., and Sun, D. (2007). Progress in Web-based decision support technologies. Decis. Support Syst. 43, 1083–1095. doi: 10.1016/j.dss.2005.07.002
Bremer, S., and Meisch, S. (2017). Co-production in climate change research: reviewing different perspectives. Wiley Interdiscip. Rev. 8:e482. doi: 10.1002/wcc.482
Bremer, S., Wardekker, A., Dessai, S., Sobolowski, S., Slaattelid, R., and van der Sluijs, J. (2019). Toward a multi-faceted conception of co-production of climate services. Clim. Serv. 13, 42–50. doi: 10.1016/j.cliser.2019.01.003
Briley, L., Brown, D., and Kalafatis, S. E. (2015). Overcoming barriers during the co-production of climate information for decision-making. Clim. Risk Manag. 9, 41–49. doi: 10.1016/j.crm.2015.04.004
Burningham, K., Fielding, J., and Thrush, D. (2008). “It’ll never happen to me”: understanding public awareness of local flood risk. Disasters 32, 216–238. doi: 10.1111/j.1467-7717.2007.01036.x
Cao, L., Mohan, K., Xu, P., and Ramesh, B. (2009). A framework for adapting agile development methodologies. Eur. J. Inform. Syst. 18, 332–343. doi: 10.1057/ejis.2009.26
Cenderelli, D. A., and Wohl, E. E. (2003). Flow hydraulics and geomorphic effects of glacial-lake outburst floods in the Mount Everest region, Nepal. Earth Surf. Processes Landf. 28, 385–407. doi: 10.1002/esp.448
Clague, J. J., and Evans, S. G. (2000). A review of catastrophic drainage of moraine-dammed lakes in British Columbia. Quat. Sci. Rev. 19, 1763–1783. doi: 10.1016/S0277-3791(00)00090-1
Cook, K. L., Andermann, C., Gimbert, F., Adhikari, B. R., and Hovius, N. (2018). Glacial lake outburst floods as drivers of fluvial erosion in the Himalaya. Science (New York, N.Y.) 362, 53–57. doi: 10.1126/science.aat4981
Cuffey, K. M., and Paterson, W. S. B. (2010). The Physics of Glaciers. Cambridge, MA: Academic Press.
Glacial Dammed Lake Data (n.d.). Available online at: https://www.weather.gov/aprfc/gdlData?10 (accessed January 14, 2020).
Guston, D. H. (2001). Boundary organizations in environmental policy and science: an introduction. Sci. Technol. Hum. Values 26, 399–408. doi: 10.1177/016224390102600401
Handcock, M. S., and Gile, K. J. (2011). Comment: on the concept of snowball sampling. Sociol. Methodol. 41, 367–371. doi: 10.1111/j.1467-9531.2011.01243.x
Harrison, S., Kargel, J. S., Huggel, C., Reynolds, J., Shugar, D. H., Betts, R. A., et al. (2018). Climate change and the global pattern of moraine-dammed glacial lake outburst floods. Cryosphere 12, 1195–1209. doi: 10.5194/tc-12-1195-2018
Hering, J. G. (2016). Do we need “more research” or better implementation through knowledge brokering? Sustain. Sci. 11, 363–369. doi: 10.1007/s11625-015-0314-8
Hock, R., Jansson, P., and Braun, L. N. (2005). “Modelling the response of mountain glacier discharge to climate warming,” in Global Change and Mountain Regions, eds H. K. M. Bugmann, M. A. Reasoner, and U. M. Huber (Cham: Springer), 243–252. doi: 10.1007/1-4020-3508-x_25
Huggel, C., Allen, S., von Dach, S. W., Dimri, A. P., Mal, S., Linbauer, A., et al. (2020). “An integrative and joint approach to climate impacts, hydrological risks and adaptation in the indian himalayan region,” in Himalayan Weather and Climate and their Impact on the Environment, eds A. P. Dimri, B. Bookhagen, M. Stoffel, and T. Yasunari (Cham: Springer), 553–573. doi: 10.1007/978-3-030-29684-1_26
Huggel, C., Kääb, A., Haeberli, W., Teysseire, P., and Paul, F. (2002). Remote sensing based assessment of hazards from glacier lake outbursts: a case study in the Swiss Alps. Can. Geotech. J. 39, 316–330. doi: 10.1139/t01-099
IPCC (2012). Managing the Risks of Extreme Events and Disasters to Advance Climate Change Adaptation. A Special Report of Working Groups I and II of the Intergovernmental Panel on Climate Change, eds C. B. Field, V. Barros, T. F. Stocker, D. Qin, D. J. Dokken, K. L. Ebi, et al. (Cambridge: Cambridge University Press), 582.
Kettle, N. P., Trainor, S. F., and Loring, P. A. (2017). Conceptualizing the science-practice interface: lessons from a collaborative network on the front-line of climate change. Front. Environ. Sci. 5:33. doi: 10.3389/fenvs.2017.00033
Kienholz, C., Herreid, S., Rich, J. L., Arendt, A. A., Hock, R., and Burgess, E. W. (2015). Derivation and analysis of a complete modern-date glacier inventory for Alaska and northwest Canada. J. Glaciol. 61, 403–420. doi: 10.3189/2015JoG14J230
Kienholz, C., Pierce, J., Hood, E., Amundson, J. M., Wolken, G., Jacobs, A., et al. (2020). Evolution of an ice-covered marginal basin and implications for outburst floods, Mendenhall Glacier, Alaska. Front. Earth Sci. 8:137. doi: 10.3389/feart.2020.00137
Klimeš, J., Benešová, M., Vilímek, V., Bouška, P., and Rapre, A. C. (2014). The reconstruction of a glacial lake outburst flood using HEC-RAS and its significance for future hazard assessments: an example from Lake 513 in the Cordillera Blanca, Peru. Nat. Hazards 71, 1617–1638. doi: 10.1007/s11069-013-0968-4
Lemos, M. C., and Morehouse, B. J. (2005). The co-production of science and policy in integrated climate assessments. Glob. Environ. Change 15, 57–68. doi: 10.1016/j.gloenvcha.2004.09.004
Levy, J. K. (2005). Multiple criteria decision making and decision support systems for flood risk management. Stoch. Environ. Res. Risk Assess. 19, 438–447. doi: 10.1007/s00477-005-0009-2
Li, J., and Sheng, Y. (2012). An automated scheme for glacial lake dynamics mapping using Landsat imagery and digital elevation models: a case study in the Himalayas. Int. J. Remote Sens. 33, 5194–5213. doi: 10.1080/01431161.2012.657370
Luke, A., Sanders, B. F., Goodrich, K. A., Feldman, D. L., Boudreau, D., Eguiarte, A., et al. (2018). Going beyond the flood insurance rate map: insights from flood hazard map co-production. Nat. Hazards Earth Syst. Sci. 18, 1097–1120. doi: 10.5194/nhess-18-1097-2018
McEwen, L., and Jones, O. (2012). Building local/lay flood knowledges into community flood resilience planning after the July 2007 floods, Gloucestershire, UK. Hydrol. Res. 43, 675–688. doi: 10.2166/nh.2012.022
McEwen, L. J., Krause, F., Jones, O., and Garde Hansen, J. (2012). Sustainable flood memories, informal knowledge and the development of community resilience to future flood risk. WIT Trans. Ecol. Environ. 159, 253–264. doi: 10.2495/FRIAR120211
McKillop, R. J., and Clague, J. J. (2007). A procedure for making objective preliminary assessments of outburst flood hazard from moraine-dammed lakes in southwestern British Columbia. Nat. Hazards 41, 131–157. doi: 10.1007/s11069-006-9028-7
Meadow, A. M., Ferguson, D. B., Guido, Z., Horangic, A., Owen, G., and Wall, T. (2015). Moving toward the deliberate coproduction of climate science knowledge. Weather Clim. Soc. 7, 179–191. doi: 10.1175/WCAS-D-14-00050.1
Meredith, M., Sommerkorn, M., Cassotta, S., Derksen, C., Ekaykin, A., Hollowed, A., et al. (2019). “Polar Regions,” in IPCC Special Report on the Ocean and Cryosphere in a Changing Climate, eds H. O. Pörtner, D. C. Roberts, V. Masson-Delmotte, P. Zhai, M. Tignor, E. Poloczanska, et al. https://www.ipcc.ch/srocc/cite-report/ in press
Morgan, P. M., Walter, J. I., Peng, Z., Amundson, J. M., and Meng, X. (2013). “Mendenhall Glacier (Juneau, Alaska) icequake seismicity and its relationship to the 2012 outburst flood and other environmental forcing,” in Proceedings of the AGU Fall Meeting Abstracts, San Francisco, CA.
Morss, R. E., Wilhelmi, O. V., Downton, M. W., and Gruntfest, E. (2005). Flood risk, uncertainty, and scientific information for decision making: lessons from an interdisciplinary project. Bull. Am. Meteorol. Soc. 86, 1593–1602. doi: 10.1175/bams-86-11-1593
Morton, M. S. S. (1971). Management Decision Systems: Computer-Based Support for Decision Making. Cambridge, MA: Division of Research, Graduate School of Business Administration, Harvard University.
National Weather Service Advanced Hydrologic Prediction Service (n.d.). Available online at: https://water.weather.gov/ahps2/hydrograph.php?wfo=PAJK&gage=mnda2 (accessed January 13, 2020).
Nel, J. L., Roux, D. J., Driver, A., Hill, L., Maherry, A. C., Snaddon, K., et al. (2016). Knowledge co-production and boundary work to promote implementation of conservation plans. Conserv. Biol. 30, 176–188. doi: 10.1111/cobi.12560
Posner, S. M., and Cvitanovic, C. (2019). Evaluating the impacts of boundary-spanning activities at the interface of environmental science and policy: a review of progress and future research needs. Environ. Sci. Policy 92, 141–151. doi: 10.1016/j.envsci.2018.11.006
Rounce, D. R., Byers, A. C., Byers, E. A., and McKinney, D. C. (2017). Brief communication: observations of a glacier outburst flood from Lhotse Glacier, Everest area, Nepal. Cryosphere. 11, 443–449. doi: 10.5194/tc-11-443-2017
Rounce, D. R., McKinney, D. C., Lala, J. M., Byers, A. C., and Watson, C. S. (2016). A new remote hazard and risk assessment framework for glacial lakes in the Nepal Himalaya. Hydrol. Earth Syst. Sci. 20:3455. doi: 10.5194/hess-20-3455-2016
Sellnow, T. L., and Seeger, M. (2001). Exploring the boundaries of crisis communication: the case of the 1997 red river valley flood. Commun. Stud. 52, 153–167. doi: 10.1080/10510970109388549
Shaw, J., Danese, C., and Stocker, L. (2013). Spanning the boundary between climate science and coastal communities: opportunities and challenges. Ocean Coast. Manag. 86, 80–87. doi: 10.1016/j.ocecoaman.2012.11.008
Sherpa, S. F., Shrestha, M., Eakin, H., and Boone, C. G. (2019). Cryospheric hazards and risk perceptions in the Sagarmatha (Mt. Everest) National Park and Buffer Zone, Nepal. Nat. Hazards 96, 607–626. doi: 10.1007/s11069-018-3560-0
Shreevastav, B. B. (2019). Flood scenario and its risk management, policy, practices in Nepal. Int. J. Sci. Eng. Res. 10, 571–581. doi: 10.14299/ijser.2019.04.07
Shrestha, A. B., Eriksson, M., Mool, P., Ghimire, P., Mishra, B., and Khanal, N. R. (2010). Glacial lake outburst flood risk assessment of Sun Koshi basin, Nepal. Geomat. Nat. Hazards Risk 1, 157–169. doi: 10.1080/19475701003668968
Som de Cerff, W., van de Vegte, J., Boers, R., Brandsma, T., de Haij, M., van Moosel, W., et al. (2018). Agile development in meteorological R&D: achieving a minimum viable product in a scrum work setting. Bull. Am. Meteorol. Soc. 99, 2507–2518. doi: 10.1175/BAMS-D-17-0273.1
Thompson, I., Shrestha, M., Chhetri, N., and Agusdinata, D. B. (2020). An institutional analysis of glacial floods and disaster risk management in the Nepal Himalaya. Int. J. Disaster Risk Reduct. 47:101567. doi: 10.1016/j.ijdrr.2020.101567
Todini, E. (1999). An operational decision support system for flood risk mapping, forecasting and management. Urban Water 1, 131–143. doi: 10.1016/s1462-0758(00)00010-8
USGS (n.d.). Available online at: https://waterdata.usgs.gov/nwis/inventory/?site_no=15052500 (March 17, 2020).
Van de Ven, F. H. M., Van Haperen, H., and Ubbels, A. (1998). “New ways for decision making in water management and their effects on decision support systems,” in Proceedings of the International Symposium on Lowland Technology, Saga.
Vincent, K., Daly, M., Scannell, C., and Leathes, B. (2018). What can climate services learn from theory and practice of co-production? Clim. Serv. 12, 48–58. doi: 10.1016/j.cliser.2018.11.001
Wang, W., Yao, T., Gao, Y., Yang, X., and Kattel, D. B. (2011). A first-order method to identify potentially dangerous glacial lakes in a region of the southeastern Tibetan Plateau. Mt. Res. Dev. 31, 122–130. doi: 10.1659/mrd-journal-d-10-00059.1
Wegner, S. A. (2014). Lo Que El Agua Se Llevó: Consecuencias Y Lecciones Del Aluvión De Huaraz De 1941. Lima: MINAM.
Welling, J., Ólafsdóttir, R., Árnason, þ, and Guðmundsson, S. (2019). Participatory planning under scenarios of glacier retreat and tourism growth in southeast iceland. Mt. Res. Dev. 39, D1–D13. doi: 10.1659/mrd-journal-d-18-00090.1
Welp, M. (2001). The use of decision support tools in participatory river basin management. Phys. Chem. Earth Part B 26, 535–539. doi: 10.1016/s1464-1909(01)00046-6
Wilcox, A. C., Wade, A. A., and Evans, E. G. (2014). Drainage events from a glacier-dammed lake, Bear Glacier, Alaska: remote sensing and field observations. Geomorphology 220, 41–49. doi: 10.1016/j.geomorph.2014.05.025
Worni, R., Huggel, C., Clague, J. J., Schaub, Y., and Stoffel, M. (2014). Coupling glacial lake impact, dam breach, and flood processes: a modeling perspective. Geomorphology 224, 161–176. doi: 10.1016/j.geomorph.2014.06.031
Keywords: glacial lake outburst flood, user engagement, decision support tools, Juneau, Kenai Peninsula, Alaska
Citation: Abdel-Fattah D, Trainor S, Hood E, Hock R and Kienholz C (2021) User Engagement in Developing Use-Inspired Glacial Lake Outburst Flood Decision Support Tools in Juneau and the Kenai Peninsula, Alaska. Front. Earth Sci. 9:635163. doi: 10.3389/feart.2021.635163
Received: 29 November 2020; Accepted: 17 March 2021;
Published: 15 April 2021.
Edited by:
Adam Emmer, University of Graz, AustriaReviewed by:
Alton Byers, University of Colorado Boulder, CO, United StatesJan Klimes, Institute of Rock Structure and Mechanics (ASCR), Czechia
Copyright © 2021 Abdel-Fattah, Trainor, Hood, Hock and Kienholz. This is an open-access article distributed under the terms of the Creative Commons Attribution License (CC BY). The use, distribution or reproduction in other forums is permitted, provided the original author(s) and the copyright owner(s) are credited and that the original publication in this journal is cited, in accordance with accepted academic practice. No use, distribution or reproduction is permitted which does not comply with these terms.
*Correspondence: Dina Abdel-Fattah, dina.abdel-fattah@uit.no