- Research Institute of Petroleum Exploration and Development, PetroChina, Beijing, China
In order to have a better understanding of the geochemical characteristics of gases from deep depths, gases from the clastic sandstone reservoirs in the Dabei and Keshen gas fields in the Kuqa depression, Tarim Basin, and gases from the marine carbonate reservoirs (Ordovician and Cambrian) in the craton area of Tarim Basin and Sichuan Basin (Yuanba, Longgang, Puguang gas fields) are investigated based on the molecular composition, stable carbon and hydrogen isotopes. Deep gas, either from the clastic sandstone reservoirs or from the marine carbonate reservoirs, is dominated by alkane gas. Gases from Kuqa depression and Sichuan Basin are dry gas, with high gas dryness coefficient, 0.976 and 0.999, respectively. Deep gas from the craton area in Tarim Basin includes both dry and wet gases. N2 and CO2 are the common non-hydrocarbon components in the deep gas. Gases from the continental sandstone reservoirs have no H2S, while gases from the marine carbonate reservoirs often have H2S. The relatively high δ13C2 value in the Kuqa depression indicates the gas was generated from humic type III kerogen, while the relatively low δ13C2 value in the craton area of Tarim Basin indicates most of the gas was generated from the marine sapropelic organic matter. Deep gas in Sichuan Basin, which has medium δ13C2 value, was generated from both humic type III and sapropelic type II organic matter. Carbon isotopic anomaly such as partial carbon isotopic reversal or relatively heavy carbon isotope is common in the deep gas, which is caused by secondary alteration. Gases from the Dabei gas field have a mean δ2H1 value of –156‰, while gases from the craton area of Tarim Basin, and Yuanba and Puguang gas fields in Sichuan Basin have relatively heavier δ2H1 value, i.e., average at −130 and −122‰, respectively. The abnormally heavier δ2H1 value in Dabei gas field is due to the high thermal maturity and possible saline depositional environment of the source rocks. This study performed a comprehensive comparison of the geochemical characteristics of the deep gases with different origins, which may provide a hint for future exploration of deep gas in the world.
Introduction
After massive exploitation of conventional oil and gas, exploration has been increasingly difficult and more attention has been paid to the deep and ultra-deep resources, especially in the mature exploration area, deep and ultra-deep area has been a significant target. Debates exist in the definition of exact burial depth of the deep and ultra-deep resources. From a geological perspective, “deep gas” is generally referred to the gas with reservoir depth greater than 4,500 m and “ultra-deep gas” is generally referred to the gas with reservoir depth greater than 6,000 m (He et al., 2016; Li, 2016; Sun et al., 2017; Dai et al., 2018). It is also proposed that definition of the exact reservoir depth of the deep and ultra-deep oil and gas resources should consider the influence of geothermal gradient of the basin (Dai et al., 2004a; Chinese Petroleum Society, 2016). For example, the geothermal gradient is 2.26°C/100 m (Feng et al., 2009) and 2.28°C/100 m (Xu et al., 2011) in the Tarim and Sichuan basins, respectively, so in these two basins, deep gas is referred to the gas with reservoir depth greater than 4,500 m and ultra-deep gas is referred to the gas with reservoir depth greater than 6,000 m (Dai et al., 2018). While in eastern China, due to the relatively high geothermal gradient (i.e., 3.58°C/100 m in the North China Basin) (Chen, 1988), “deep” is referred to reservoir depth greater than 3,500 m and “ultra-deep” is referred to reservoir depth greater than 4,500 m (Chinese Petroleum Society, 2016).
To date, a number of countries have carried out deep gas exploration and great breakthrough has been achieved (Tuo, 2002). During 2008–2018, the global proven oil and gas reserves in the deep and ultra-deep strata with burial depth >4,000 m were around 14 × 109 t and 9.1 × 109 t (oil equivalent), respectively, accounting for 66 and 61% of the total increased oil and gas proven reserves, respectively (Zhao, 2019). In China, most exploration has focused on the shallow-middle depths and few exploration has been carried out on the deep oil and gas resources, so the exploration degree of deep resources is low. However, the deep oil and gas resources in China have great potential. The geological oil and gas reserves of China’s deep and ultra-deep depths are 26.6 × 109 t and 49.7 × 1012 m3, respectively, which account for 21 and 55% of the total oil and gas geological reserves in China, respectively (Strategic Research Center of Oil and Gas Resources, 2017). The exploration degree is only 13 and 10% for the deep and ultra-deep oil and gas, respectively (Strategic Research Center of Oil and Gas Resources, 2017). Recently, significant discoveries have been made in the exploration of deep gas in the Tarim and Sichuan basins in China. For example, a number of marine carbonate oil/gas zones have been discovered, such as Lunnan-Tahe, Tazhong, and Halahatang in the Tarim Basin and Puguang, Longgang and Yuanba in the Sichuan Basin, and terrestrial clastic gas fields such as Dabei and Keshen in the Kuqa depression in Tarim Basin (Sun et al., 2013). In China, the deepest gas field is the Keshen gas field in Tarim Basin with reservoir depth over 8,000 m (Well Keshen 902, 8,038 m).
With increasing improvements in the technological development and theoretical understanding, exploration of deep gas will become more and more practical. Moreover, natural gas may form in the deep parts of the sedimentary basin and migrate upward and accumulate in the shallow layers. A full understanding of the origin of deep gas will shed light for the research of natural gas in both shallow and deep basins. However, knowledge about the deep gas in many sedimentary basins is still poor and geochemical information about the deep gas is limited. Previous study has indicated that a number of factors contribute to the origin of deep gas such as the type of organic matter, thermal maturity, oil stability, the influences of minerals, water, non-hydrocarbon gas, etc. (Dyman et al., 2003). This makes the study of the origin and source of deep gas very difficult. Dai et al. (2018) carried out a geochemical study of the ultra-deep gas in the Sichuan Basin and proposed that most of these deep gases were sourced from humic organic matter. In order to have a better understanding of the origin and distribution of deep gas, this study performed a comprehensive comparative investigation of the geochemical characteristics of the deep gases from different types of reservoirs in China. First of all, gases from the clastic sandstone reservoirs in the Dabei gas field in Kuqa depression, Tarim Basin were emphatically investigated (Figure 1A). Dabei gas field is one of the 15 large tight gas fields in China, which is also the only large tight gas field in Tarim Basin (Dai et al., 2014). It is also the one with largest burial depth and most complex structures among the continental large gas fields that have ever been found. The sandstone reservoir under the salts in the Dabei gas field generally has depth more than 5,300 m, porosity of 1∼8%, and permeability of 0.01 × 10–15∼1.0 × 10–15 m2, which belongs to typical deep tight gas field (Dai et al., 2014). For comparison, literature data of deep gases from the sandstone reservoir in Keshen gas field in the same depression, and deep gases from the marine carbonate reservoirs in the craton area of Tarim Basin (Figure 1B) and Sichuan Basin (Yuanba, Longgang, Puguang gas fields) (Figure 2) are also investigated. A detailed comparative geochemical research about the deep gases with different origins from different basins may deepen our understanding of the origin and accumulation of deep gas and shed a light on the future exploration of deep gas in the world.
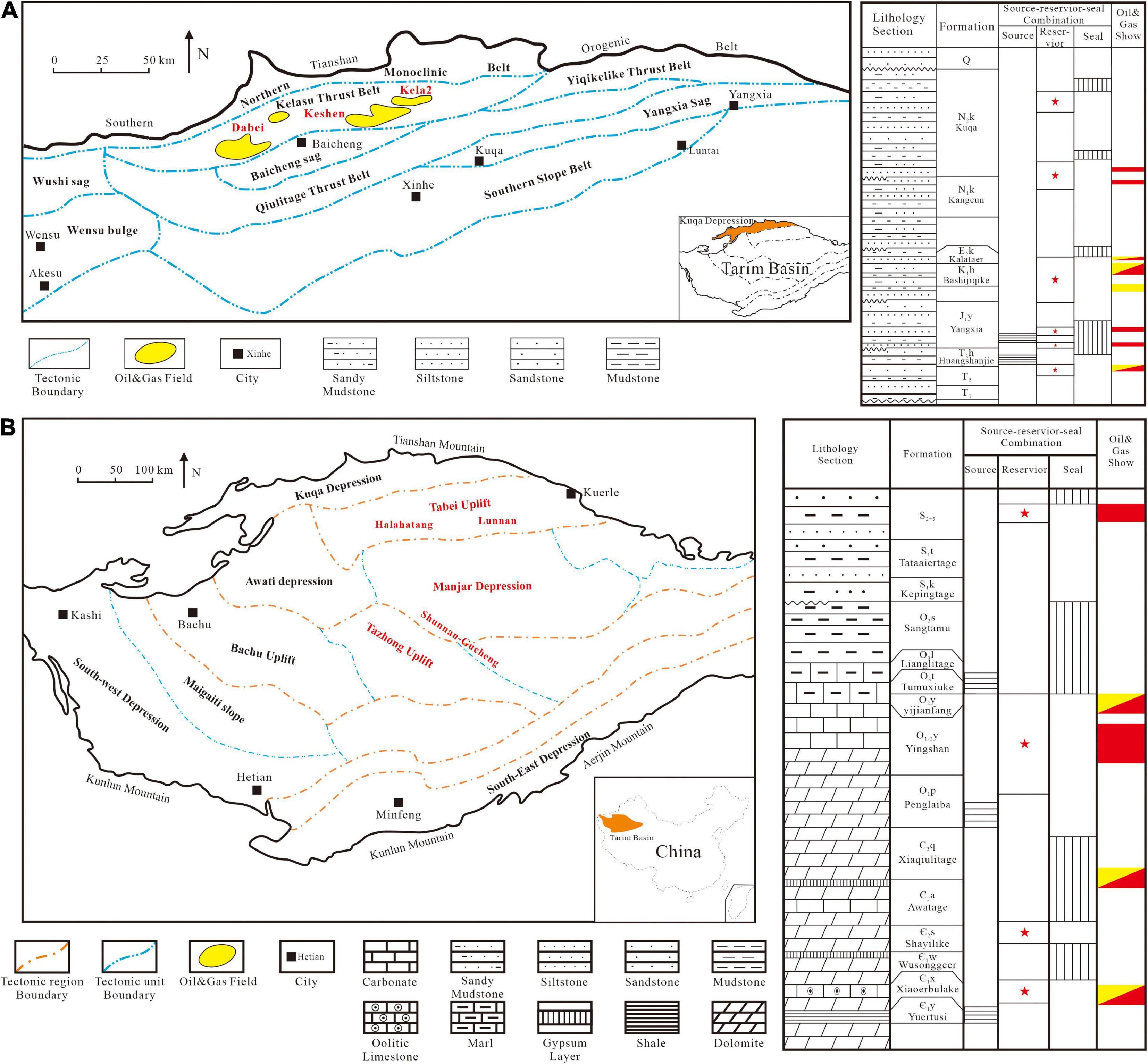
Figure 1. (A) Location map of the Dabei, Keshen, and Kela2 gas fields in the Kuqa depression, Tarim Basin and the stratigraphic column of the Kuqa depression (Wang J. et al., 2018; Zhao et al., 2018). (B) Location map of the cratonic Tarim basin and the corresponding stratigraphic column (Ni et al., 2013; Zhu et al., 2019b).
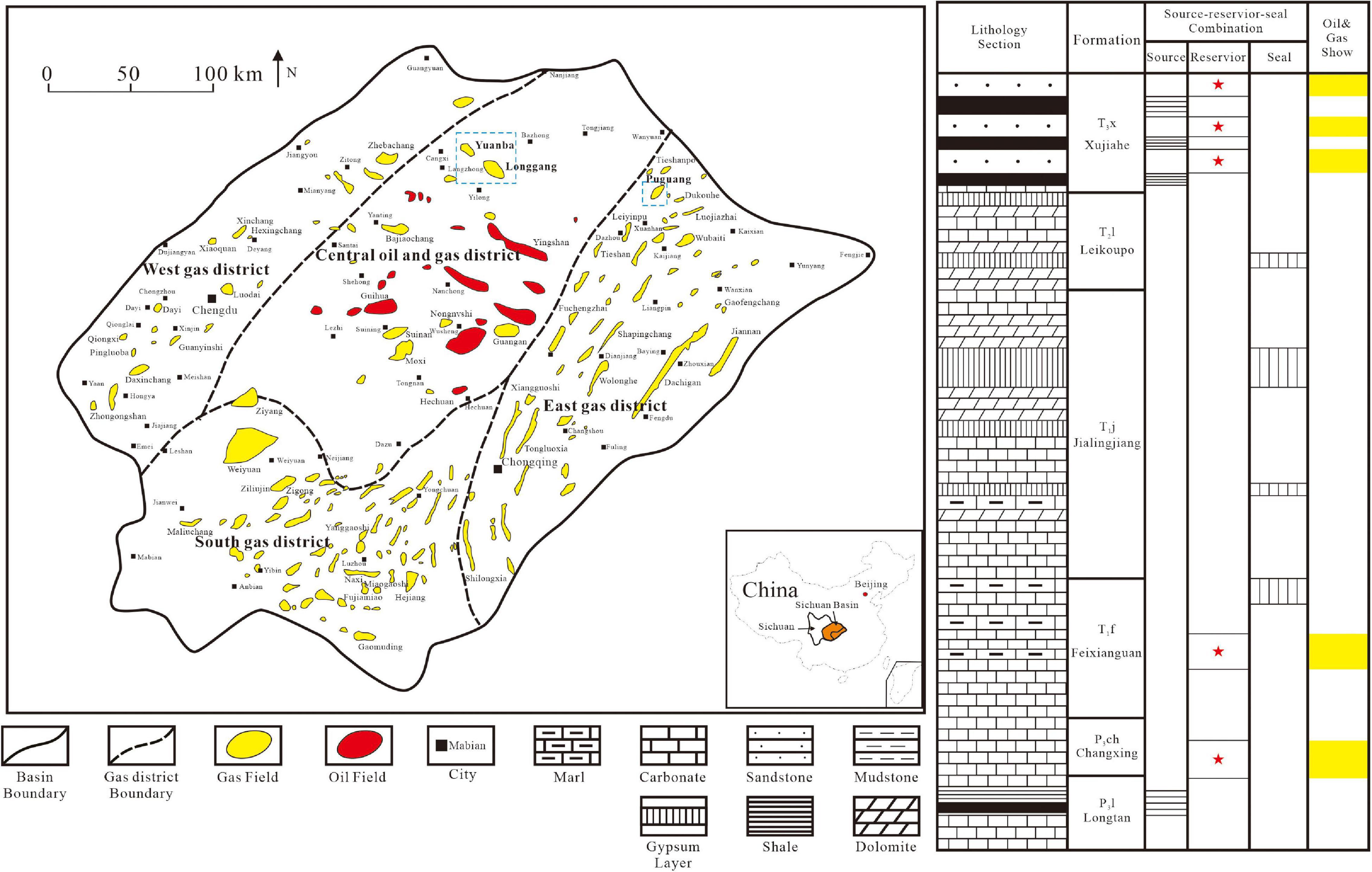
Figure 2. Location map of the Puguang, Yuanba and Longgang gas fields in the Sichuan Basin, the stratigraphic column and source-reservoir-seal combination (Dai et al., 2012a; Qin et al., 2016). Reservoir layer is marked as “*”.
Geochemistry of Source Rocks
Tarim Basin
Tarim Basin is located in northwest China and has an area of 560,000 km2. A series of giant gas fields have been discovered in this basin, which made it one of the most important gas basins in China. Tarim Basin is a large superimposed and composite basin, and it consists of a Paleozoic cratonic basin in the south (Figure 1B) and a Mesozoic–Cenozoic foreland basin in the north (Figure 1A). The basin was deposited with sediments from Sinian to Quaternary with maximum thickness up to 15,000 m. The main gas-bearing reservoirs include the strata of Ordovician, Carboniferous, Triassic, Jurassic, Cretaceous, and Tertiary. Potential source rocks include the Cambrian–Ordovician highly mature–over mature marine carbonates, Carboniferous–Permian marine–terrestrial transitional highly mature–middle mature carbonates and mudstones, and the Triassic–Jurassic low mature–middle mature lacustrine mudstones and lacustrine-swamp coal measures.
In the Kuqa depression, the widely distributed Middle-Lower Jurassic coal measures deposited in the lacustrine-swamp environment have been regarded as the main source rocks for the coal-derived gases (Liang et al., 2003b; Qin et al., 2007). The thickness of coal bed usually ranges from 6 to 29 m. The Middle-Lower Jurassic source rocks are dominated by type III kerogen and have δ13C values −22∼−26‰ (Liang et al., 2003a; Wang et al., 2005). Thermal maturity of the source rocks in the depression is generally greater than 0.6%, and in the Baicheng sag, thermal maturity of the source rocks is very high, i.e., 1.5∼3.5% (Dai, 2016).
The Cambrian-Lower Ordovician carbonates have been considered as the main source rocks of the oil-derived gases in the Paleozoic cratonic Tarim Basin (Liang et al., 2002). The Cambrian-Lower Ordovician source rocks are deposited in a sub-compensation and evaporating lagoon environment and the water medium is saline water. It is dominated by type I kerogen and it has total organic carbon of 0.27∼5.52%. The carbon isotope value of kerogen is −32.5∼−27.0‰ (Chen et al., 2000, 2001). The source rocks are at high to over mature stage and the vitrinite reflectance value (Ro%) is 1.8∼3.0% (Wang et al., 2002; Zhang Z. et al., 2006).
Sichuan Basin
The Sichuan Basin is located in eastern Sichuan and has an area of 18 × 104 km2 (Figure 2). A number of giant gas fields have been discovered in this basin and recent exploration success of the deep gases with reservoir depth more than 4,500 m has made Sichuan Basin one of the most important gas producing basins in China. The Sichuan Basin is a tectonic superimposed basin, where the late Mesozoic–Cenozoic foreland basin overlying on a Sinian–Middle Mesozoic passive margin (Ma et al., 2007). It has deposited super-thick Sinian-Middle Triassic marine carbonates (4∼7 km), the early stage of Late Triassic marine-continental transitional deposition (300∼400 m) and the middle stage of Late Triassic-Eocene terrestrial clastic rocks (2∼5 km) (Ma et al., 2010).
In the Sichuan Basin, Permian is an important strata developing two sets of source rocks including Middle Permian carbonates and Upper Permian coal measures with Upper Permian Dalong Formation marine mudstone source rocks in local areas (Chen et al., 2018b). Among them, the Upper Permian Longtan Formation has been considered to make main contribution to the gas source of Puguang, Yuanba and Longgang gas fields (Ma, 2008; Ma et al., 2008; Wu et al., 2015a; Qin et al., 2016; Guo, 2011; Zhao et al., 2011). The marine-continental transitional coal-bearing deposits of the Upper Permian Longtan Formation include mudstone, carbonaceous mudstone, coal and carbonates. The Longtan mudstone source rocks are widely distributed in the whole basin and the coal bed has thickness of 2∼5 m in the northeastern Sichuan Basin, 5∼15 m in the central and southeastern Sichuan Basin, and less than 1 m in the north and southwestern Sichuan Basin (Chen et al., 2018b). According to the analysis of 372 samples, the TOC of Longtan source rocks has an average value of 2.83% (Chen et al., 2018a). In the southwestern part of eastern Sichuan Basin, the Longtan coal measure source rocks have TOC up to 7.47% with an average of 2.91%, δ13C of kerogen of −24.0∼−23.5‰, thermal maturity (Ro%) of 1.89∼2.63% (Yang et al., 2002).
Samples and Methods
276 gas samples with reservoir depth more than 4,500 m from Sichuan and Tarim basins were analyzed in this study, including 11 gas samples collected from the Dabei gas field in the Kuqa depression, Tarim Basin and 265 samples from published literature (see Table 1 for the natural gas from Dabei gas field). All the gases were called as deep gas, which includes both deep gas and ultra-deep gas. Among the 276 samples, 53 samples were from the Kelasu thrust belt in the Kuqa depression, i.e., 47 samples from the Dabei gas field (Li et al., 2005; Zhang et al., 2011; Dai, 2016; Wei et al., 2019; Zhu et al., 2019a), and 6 samples from the Keshen gas field (Wei et al., 2019), marked as Kuqa-Dabei and Kuqa-Keshen, respectively in all figures; 153 gas samples were from the Tabei and Tazhong uplifts in the Tarim Basin (Wang, 2005; Ni et al., 2013; Wang et al., 2014; Wu et al., 2014; Zhu et al., 2014, 2015; Wang Y. et al., 2018; Zhou et al., 2019), marked as Tarim-craton in all figures; and 70 samples were from Longgang, Yuanba, and Puguang gas fields in Sichuan Basin (Li et al., 2015, 2016; Wang et al., 2015; Wu et al., 2015b; Qin et al., 2016; Dai et al., 2018), marked as Sichuan in all figures.
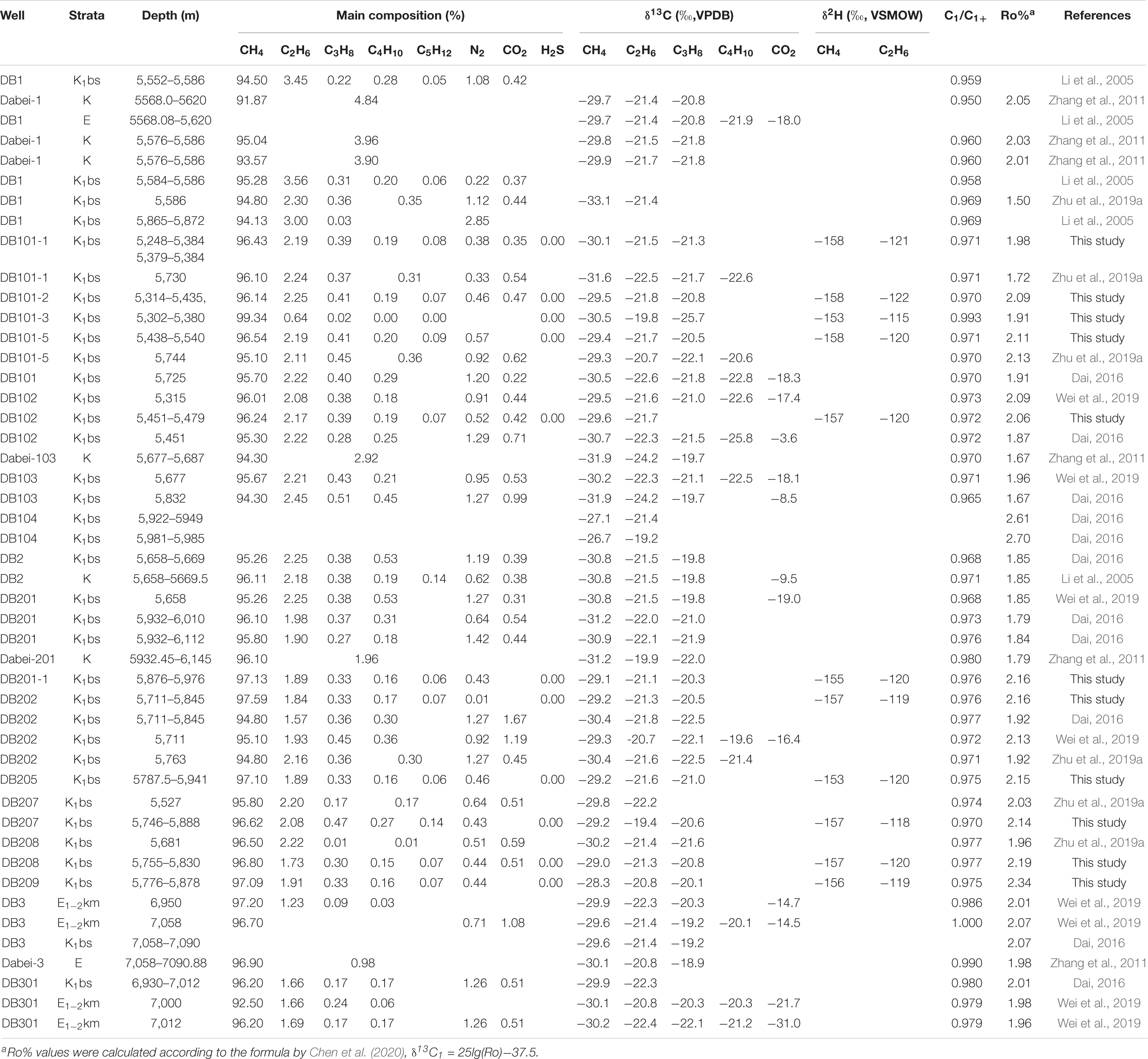
Table 1. Molecular composition and stable carbon and hydrogen isotopic values of deep gas from Dabei gas field, Kuqa depression, Tarim basin.
The 11 gas samples were analysed in the PetroChina Research Institute of Petroleum Exploration and Development (RIPED). Gas molecular composition was conducted on a two-channel Wasson-Agilent 7890 gas chromatograph, equipped with one auxiliary oven, two capillary and six packed analytical columns, one flame ionization detector (FID) and two thermal conductivity detectors (TCD). The carrier gas was high-pure N2 for FID and He for TCD. GC oven temperature was initially set at 68°C for 7 min, and then increased to 90°C at 10°C/min, held at 90°C for 1.5 min, ramped to 175°C at 15°C/min and held at this temperature for 5 min.
Compound specific stable carbon isotopic composition was determined on a Thermo Delta V mass spectrometer interfaced with a Thermo Trace GC ultra-gas chromatograph (GC). Individual hydrocarbon gas components (C1-C5) and CO2 were separated by using a fused silica capillary column (PLOT Q 27.5 m × 0.32 mm × 10 μm) on a gas chromatograph with He as the carrier gas, then converted into CO2 in a combustion interface and introduced into the mass spectrometer. The GC oven temperature was increased from 33 to 80°C at 8°C/min, then to 250°C at 5°C/min, and maintained at this temperature for 10 min. All analyses were analysed in triplicate, and the stable carbon isotopic values were reported in the δ-notation in per mil (‰) relative to VPDB. The analytical precision was estimated to be ± 0.3‰.
Compound specific stable hydrogen isotopic composition was measured on a MAT253 isotope ratio mass spectrometer interfaced with a Trace GC ultra-gas chromatograph (GC) and a micropyrolysis furnace (1,450°C). Gas components were separated on a HP-PLOT Q column (30 m × 0.32 mm × 20 μm). The carrier gas was helium with flow rate of 1.2 ml/min. A split injection sample introduction mode with split ratio of 1:10 was used for methane and a non-split injection mode was used for ethane and propane. The GC oven temperature program was the same as that for the carbon isotope. Natural gas was separated into individual gas components via the gas chromatograph and then transformed into C and H2 in the micropyrolysis furnace at 1,450°C. H2 went into the mass spectrometer and was determined for δ2H. The stable hydrogen isotope value (δ2H) was reported in the δ-notation in per mil (‰) relative to VSMOW and the analytical precision was expected to be ± 3‰.
The laboratory working standard was a coal-derived hydrocarbon gas which was obtained from the Ordos basin in China. An international laboratory standard comparison has been conducted for the working standard, for which two-point calibrations were performed with international measurement standards for both carbon isotope ratios (NBS19 and L-SVEC CO2) and hydrogen isotope ratios (VSMOW and SLAP) (Dai et al., 2012b).
Geochemical Characteristics of Deep Gases
Molecular Composition
Methane is the dominant gas component of the gases, 1.39∼99.34% with an average of 83.80% (n = 269) (Figure 3). Among them, 236 gas samples have methane content more than 70%, and 110 gas samples have methane more than 90%, accounting for 87.73 and 40.89% of the total, respectively. C2+ heavy hydrocarbons range between 0.01 and 36.26% with an average of 4.06% (n = 266). 209 out of 266 samples have C2+ heavy hydrocarbons less than 5%, accounting for 78.57%. In general, samples from the Kelasu thrust belt in the Kuqa depression have relatively high content of methane (average 95.76%, n = 49), with average gas dryness coefficient of 0.976 (n = 49), indicative of dry gas. While samples from the craton area of Tarim Basin have average methane content of 81.98% (n = 153) and C2+ heavy hydrocarbons of 6.24% (n = 153). The gas dryness coefficient varies greatly, except for sample from Well LN44 in the Tabei uplift which has gas dryness coefficient of 0.238, the gas dryness coefficient mainly ranges between 0.594 and 0.999, indicating the existence of both dry gas and wet gas. Among the gases from the craton area of Tarim Basin, gas in the Shunnan-Gucheng area has relatively very low C2+ heavy hydrocarbon (average 0.69%, n = 19) and high gas dryness coefficient (average 0.992, n = 19), belonging to dry gas; while gas from Halahatang area has relatively very high content of C2+ heavy hydrocarbons (average 25.50%, n = 9) and low gas dryness coefficient (average 0.713, n = 9), belonging to wet gas. Gases from Puguang, Yuanba and Longgang gas fields in the Sichuan Basin have very low C2+ heavy hydrocarbons (0.01∼0.78% with average value of 0.11%, n = 66) and high gas dryness coefficient (average 0.999, n = 66).
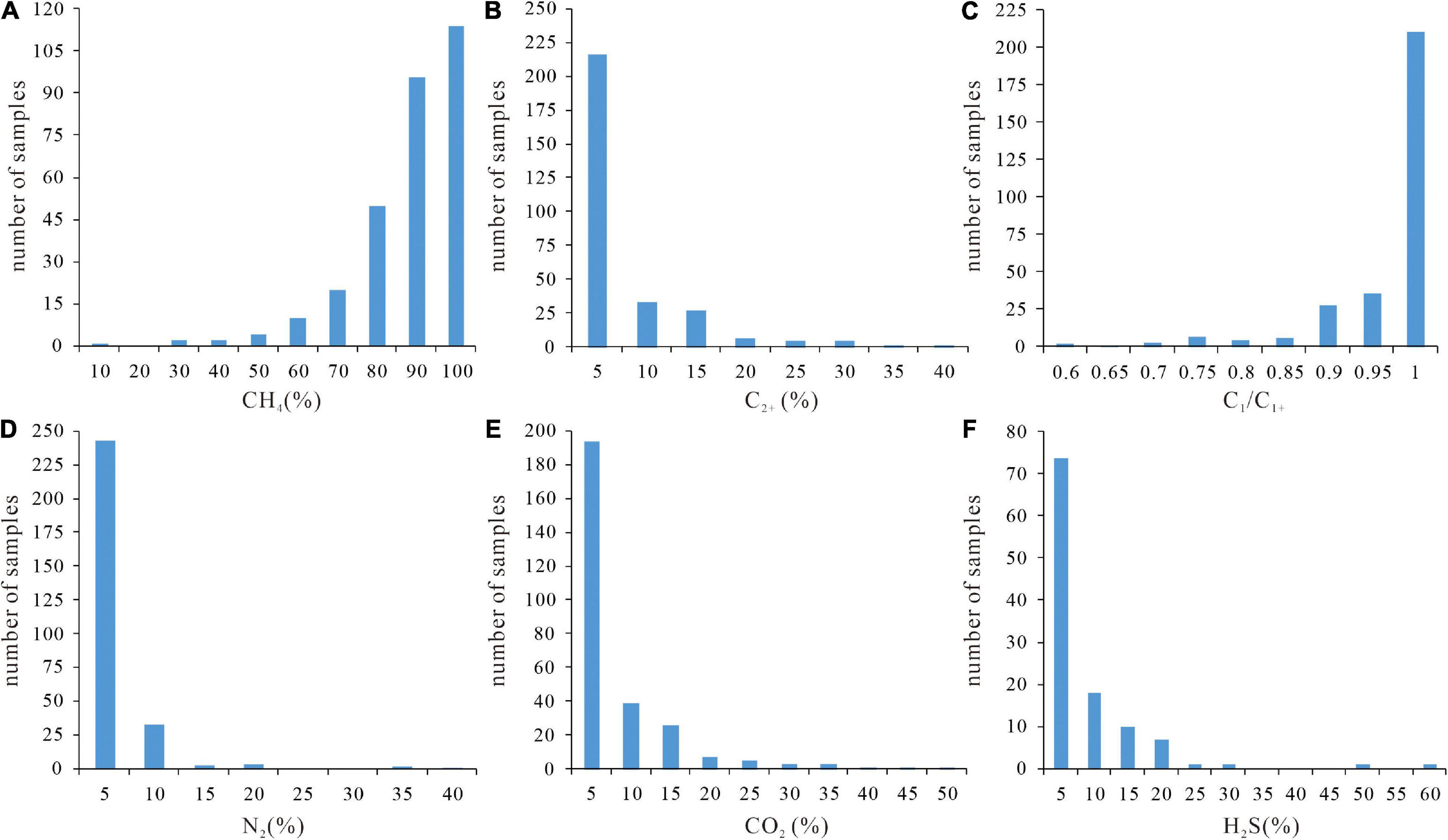
Figure 3. Histogram of the content of CH4 (A), C2+ (B), C1/C1+ (C), N2 (D), CO2 (E), and H2S (F) of the deep gases from Tarim and Sichuan basins. Data source see section “Samples and Methods” in the text.
Non-hydrocarbon gas has an average content of 11.56% (n = 260). 162 out of 260 samples have non-hydrocarbon gas less than 10%, 82 out of 260 samples have non-hydrocarbon gas of 10∼30%, and only 16 samples have non-hydrocarbon gas more than 30%. N2 and CO2 are common non-hydrocarbon gas, and nearly exist in all samples. 215 out of 258 samples have N2 less than 5%, 31 out of 258 samples have N2 of 5∼10%; 171 out of 252 samples have CO2 less than 5%, 61 out of 252 samples have CO2 of 5∼15% and 20 out of 252 samples have CO2 more than 15% (Figure 3). H2S only presents in the gases with marine carbonates as reservoirs, such as gases from Sichuan Basin and the craton area of Tarim Basin. In those gases from Kelasu thrust belt which has sandstone as reservoirs, there is no sign of the presence of H2S. 76 out of 115 samples have H2S less than 5%, 28 out of 115 samples have H2S of 5∼15%, 9 out of 115 samples have H2S of 15∼30% and two samples from Puguang gas field in the Sichuan Basin have H2S even more than 45%. In general, gases from the Dabei gas field have the lowest content of non-hydrocarbon gas, i.e., 0.01∼2.94% with an average value of 1.37% (n = 34). Followed by the gas from Keshen gas field, which has non-hydrocarbon gas of 3.50% (average, n = 6). Then followed by the gas from the craton area of Tarim Basin (average 11.17%, n = 153) and Sichuan Basin (average 18.39%, n = 67).
Carbon Isotope
The stable carbon isotopes of methane, ethane and propane vary greatly for gases from different areas. In total, δ13C1 varies from –26.5 to –54.9‰ with an average of –35.4‰ (n = 253), and δ13C2 varies from –16.1 to –41.1‰ with an average of –29.7‰ (n = 226). Among them, gas from Dabei and Keshen gas fields in the Kuqa depression has δ13C1 of –26.5∼–33.1‰ (average –29.7‰, n = 50), δ13C2 of –16.1∼–24.2‰ (average –21.1‰, n = 49) and δ13C3 of –18.9∼–25.7‰ (average –21.0‰, n = 38). A small number of samples from the Dabei gas field have slight carbon isotopic reversal between ethane and propane. Except one sample has carbon isotopic reversal magnitude up to 5.9‰, most samples have reversal magnitude less than 2.2‰. While most gases from the Keshen gas field have carbon isotopic reversal between ethane and propane and the reversal magnitude can be up to 3.6‰. Gas from the craton area of Tarim Basin has much lower carbon isotopes, i.e., δ13C1 of −30.2∼−54.9‰ (average −40.5‰, n = 133), and δ13C2 of −26.2∼−41.1‰ (average −34.2‰, n = 125). Most samples have normal carbon isotopic distribution among C1∼C3 alkane gases, i.e., methane and its homologues become more and more enriched in 13C with increasing carbon number (δ13C1 < δ13C2 < δ13C3). Samples from Sichuan Basin have δ13C1 of −27.4∼−33.7‰ (average −29.9‰, n = 70), and δ13C2 of −21.0∼−32.2‰ (average −27.0‰, n = 52). The average C2+ heavy hydrocarbon content is only 0.11% (n = 66), so only 4 samples have δ13C3 values with average of −28.0‰.
The δ13C of CO2 varies greatly, i.e., −2.8∼−31.0‰ with an average of −6.0‰ (n = 110). Gas from the Dabei and Keshen gas fields has δ13CCO2 values of −3.6∼−31.0‰ (average −15.1‰, n = 19). Among them, one sample has δ13CCO2 value of −3.6‰, 3 samples have δ13CCO2 values of −8.5∼−9.7‰, and all other 15 samples have δ13CCO2 values lower than −10‰. Gas from the craton area of Tarim Basin has δ13CCO2 values of −25.0∼0.6‰ (average −7.1‰, n = 41). Among them, 27 samples have δ13CCO2 values greater than −8‰, 5 samples have δ13CCO2 values of −8.8∼−9.8‰ and 9 samples have δ13CCO2 values lower than −10‰.
Hydrogen Isotope
The stable hydrogen isotopic values vary between −111∼−158‰ with an average of −134‰ (n = 44) for methane, between −94∼−145‰ with an average of −124‰ (n = 29) for ethane and between −91∼−130‰ with an average of −111‰ (n = 18) for propane. Gas from the Dabei gas field has relatively very high δ2H value, δ2H value ranges −153 to −158‰ with an average of −156‰ (n = 11) for methane and from −115 to −122‰ with an average of −119‰ (n = 11) for ethane. Gas from the craton area of Tarim Basin has δ2H1 value of −121∼−140‰ (average −130‰, n = 18) and δ2H2 value of −94∼−145‰ (average −126, n = 18). Due to the relatively small amount of C2+ heavy hydrocarbons in the samples from Sichuan Basin but relatively large required sample amount for the hydrogen isotopic analysis, gas from Sichuan Basin only has hydrogen isotopic values for methane, which ranges from −111 to −156‰ with an average of −122% (n = 15). All the samples have normal hydrogen isotopic distribution pattern among C1∼C3 alkane gases, i.e., methane and its homologues become more enriched in D with increasing carbon numbers (δ2H1 < δ2H2 < δ2H3).
Origin of Deep Gases
Type and Origin of Deep Gas
Natural gas in nature can be divided into biotic and abiotic origin, and the biotic gas can be divided into microbial gas and thermogenic gas. According to the type of organic matter, thermogenic gas can be further divided into oil-related gas in sapropelic origin (including oil-associated gas and oil-cracked gas) and coal-derived gas in humic origin. The oil-related gas is produced by the thermal decomposition of sapropelic kerogen or the thermal cracking of oil which was generated from sapropelic kerogen. The coal-derived gas is generated from the thermal degradation of humic kerogen or the thermal cracking of heavy hydrocarbons generated from humic organic matter (Dai et al., 1992). Since natural gas is composed of a few simple small molecules, gas origin studies are mainly dependent on its molecular composition, stable carbon and hydrogen isotopes. A number of studies using molecular composition, carbon and hydrogen isotopes have been performed to investigate the gas origin (Bernard et al., 1976; James, 1983; Clayton, 1991; Dai, 1993; Prinzhofer and Huc, 1995; Lorant et al., 1998; Whiticar, 1999; Tang et al., 2000; Dai et al., 2005a, 2012a, 2016a; Milkov and Etiope, 2018).
The C1/(C2 + C3) vs. δ13C1 diagram has been widely used to investigate the gas origin (Bernard et al., 1976; Whiticar, 1999; Milkov and Etiope, 2018). In this diagram (Figure 4), all the deep gas samples fall in the field of thermogenic gas and far away from the field of primary microbial gas. This indicates there is no mixing with primary microbial gas. Some gas samples from the craton area of Tarim Basin fall in the oil-associated gas field, but most of the samples are more toward the field of late mature thermogenic gas, especially the gas from Sichuan Basin, which shows particularly low content of C2+ heavy hydrocarbons and the C1/(C2 + C3) ratio can be up to 4689 (Well Y123 in Yuanba gas field). Though there is significant overlap of thermogenic, primary microbial and abiotic gases in the δ13C1 vs. δ13CCO2 diagram (Figure 5), this diagram is very useful for distinguishing secondary microbial gas. As seen in Figure 5, all gas samples are away from the field of secondary microbial gas. This further indicates that all the deep gas has no mixing with neither primary microbial gas or secondary microbial gas. This agrees well with the geological conditions. The burial depth of the produced layer of all gas samples was more than 4,500 m, microbial activities are unlikely to be active at such depth.
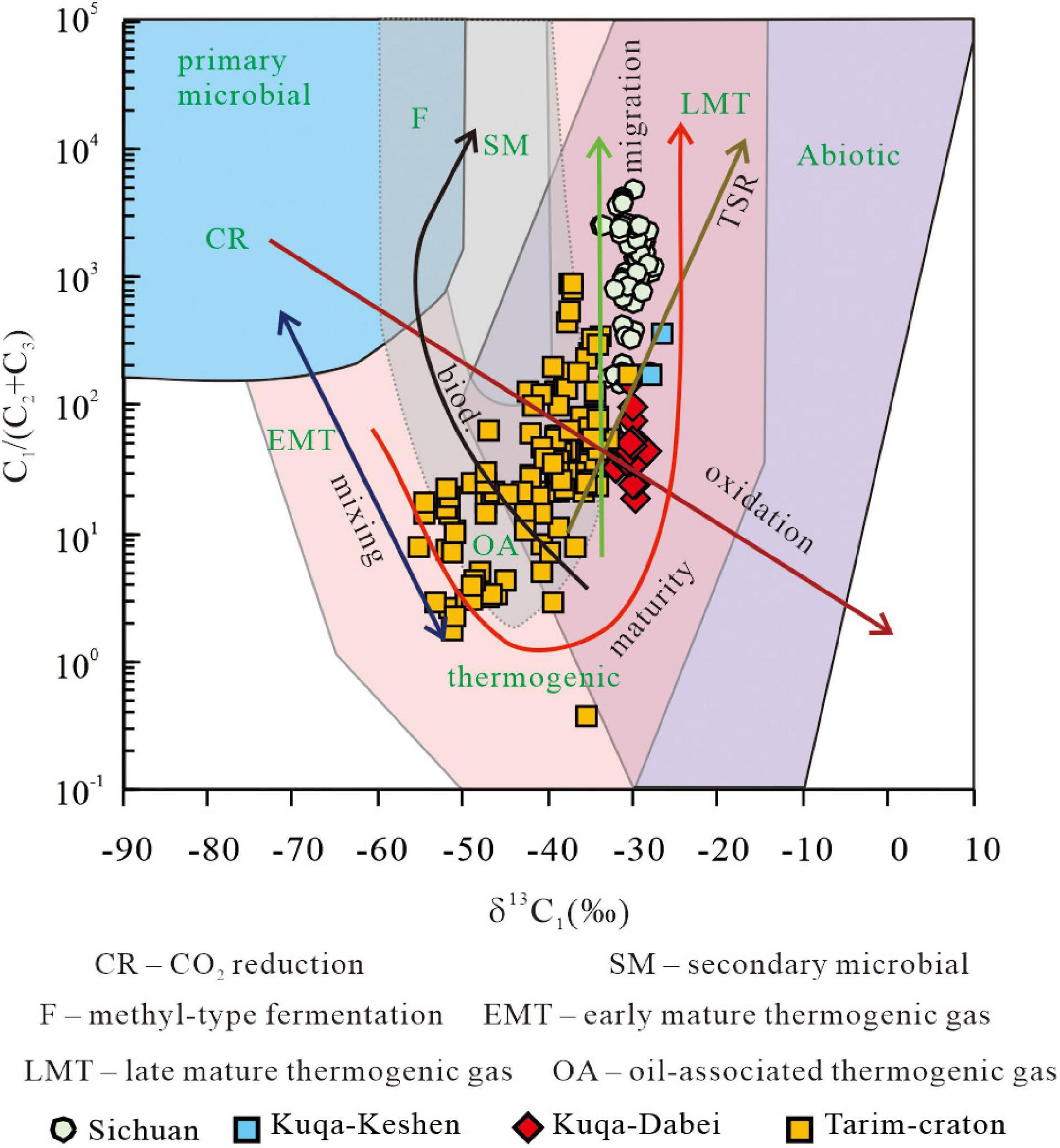
Figure 4. Genetic diagram of C1/(C2 + C3) vs. δ13C1 of gases from Dabei and Keshen gas fields in the Kuqa depression, craton area of Tarim Basin and Sichuan Basin, China (Milkov and Etiope, 2018). Data source see section “Samples and Methods” in the text.
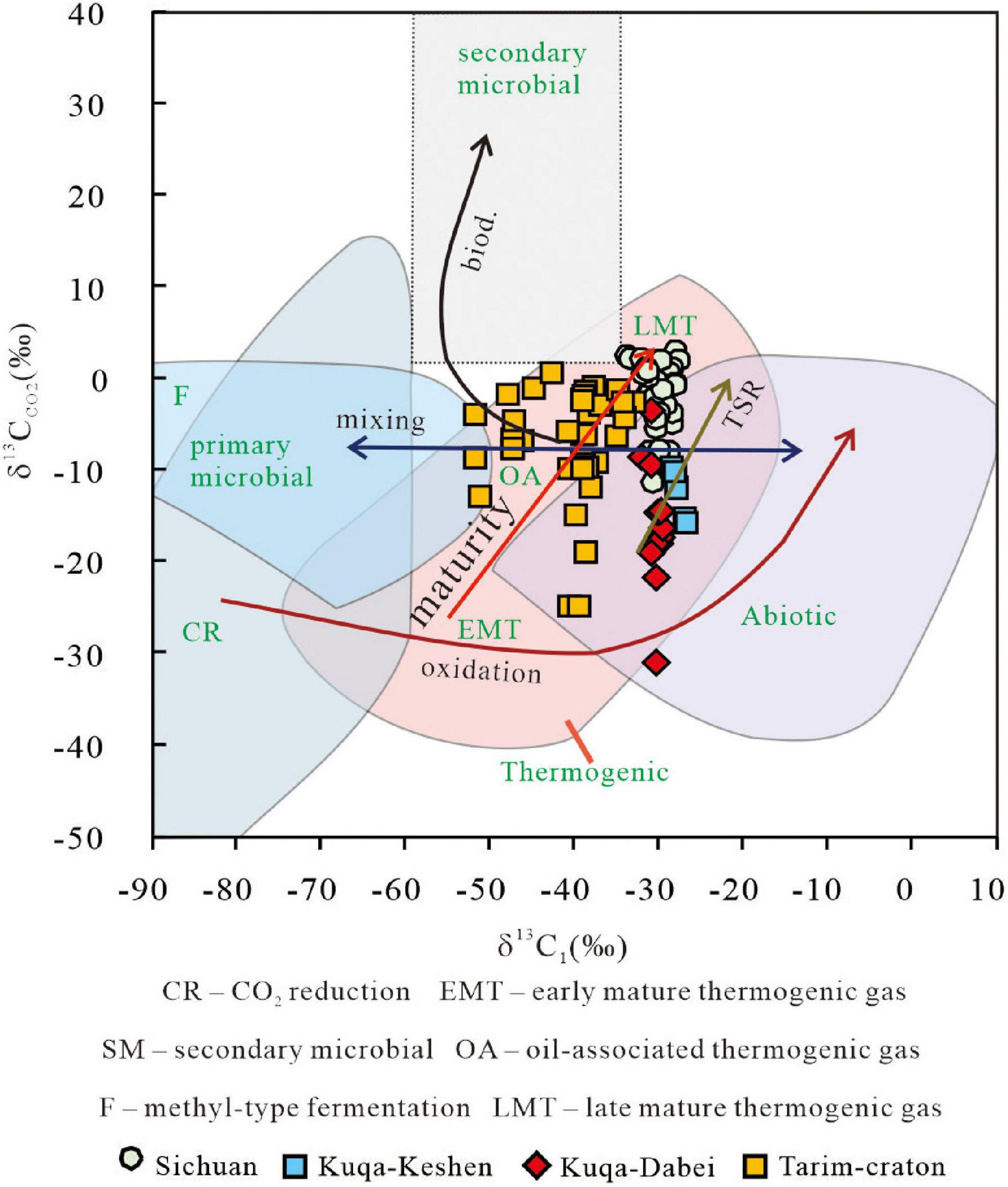
Figure 5. Genetic diagram of δ13CCO2 vs. δ13C1 of gases from Dabei and Keshen gas fields in the Kuqa depression, craton area of Tarim Basin and Sichuan Basin, China (Milkov and Etiope, 2018). Data source see section “Samples and Methods” in the text.
With the increase of thermal evolution degree of the organic matter, natural gas become more and more enriched in 13C, plot of δ13C1 vs. δ13C2 has been widely used as a gas maturity trend and classification diagram for natural gas origin (Jenden et al., 1988; Dai and Qi, 1989; Rooney et al., 1995; Berner and Faber, 1996; Huang et al., 1996; Tang et al., 2000). A theoretical slope value of 2.4 was calculated for the correlation line between δ13C1 and δ13C2 (Xia and Gao, 2017). Different source rocks, secondary alteration or different gas generation mechanism may cause deviation from the theoretical linear trend. As shown in Figure 6, gases from the continental sandstone reservoirs (Dabei and Keshen gas fields) in the Kuqa depression have δ13C2 values far greater than −28‰, and all fall in the up-left area in the δ13C1 vs. δ13C2 diagram for gases generated from type III kerogen (Jenden et al., 1988; Dai and Qi, 1989; Rooney et al., 1995; Berner and Faber, 1996). Compared to the gases generated from type III kerogen, gases from Dabei gas field were the most similar to the gases generated from type III kerogen in Niger Delta (Rooney et al., 1995), but still much heavier carbon isotope of ethane, which result in the fact that only two gas samples from the Dabei gas field obey the evolution trend of gas generated from type III kerogen in Niger Delta and all other gas samples fall above the gas maturity trend (Figure 6). Gases from the Keshen gas field have even heavier carbon isotope of ethane (up to −16.1‰ of δ13C2) and fall far above the evolution trend of gas generated from type III kerogen in Niger Delta of type III kerogen (Rooney et al., 1995). Gases with reservoir depth shallower than 4,500 m from Kela2 gas field in Kuqa depression were also shown for comparison. In general, gases from Kela2 gas field have the heaviest carbon isotope of methane (−25.1∼−30.8‰, average −27.4‰, n = 24), gases from the Keshen gas field have the heaviest carbon isotope of ethane (−16.1∼−17.8‰, average −17.0‰, n = 5) and gases from the Dabei gas field have the lightest carbon isotope of methane and ethane. All these characteristics indicate that gases from the continental sandstone reservoirs (Dabei and Keshen gas fields) in the Kuqa depression were generated from higher plant-derived type III kerogen. Gases from Sichuan Basin can be divided into two groups, the first group falls in the region of gases generated from type III kerogen (Dai and Qi, 1989; Rooney et al., 1995; Berner and Faber, 1996), and the second group falls in the region of gases generated from type II kerogen (Huang et al., 1996). Gases in the first group were generated from the humic type III kerogen and gases in the second group were produced from the sapropelic organic matter. Most gas samples from the craton area of Tarim Basin have δ13C2 values lower than −28‰ and match better with the evolution trend of gas generated from type II kerogen (Rooney et al., 1995; Berner and Faber, 1996; Huang et al., 1996; Tilley and Muehlenbachs, 2006), and only several samples have δ13C2 values greater than −28‰ and follow the evolution trend of gases generated from the type III kerogen (Jenden et al., 1988; Rooney et al., 1995; Figure 6). This indicates that most gases from the craton area of Tarim Basin were generated from the sapropelic organic matter. Compared to the deep gases from the continental sandstone reservoirs in the Kuqa depression and marine carbonate reservoirs in the craton area of Tarim Basin, gases from Sichuan Basin have relatively medium values of carbon isotope of ethane. For the comparison of gases generated from type III kerogen, several samples from the Longgang gas field in Sichuan Basin have δ13C2 values greater than −25‰, which is close to the δ13C2 values of gases from Dabei gas field (−19.2∼−24.2‰, average −21.6‰, n = 47). Except these gases, all other coal-derived gases from the marine carbonate reservoirs in Sichuan Basin have lower δ13C2 values than that of the gases from the continental sandstone reservoirs. However, for the comparison of gases generated from type II kerogen, most gases from Sichuan Basin have greater δ13C2 values than that of the gases from the craton area of Tarim Basin.
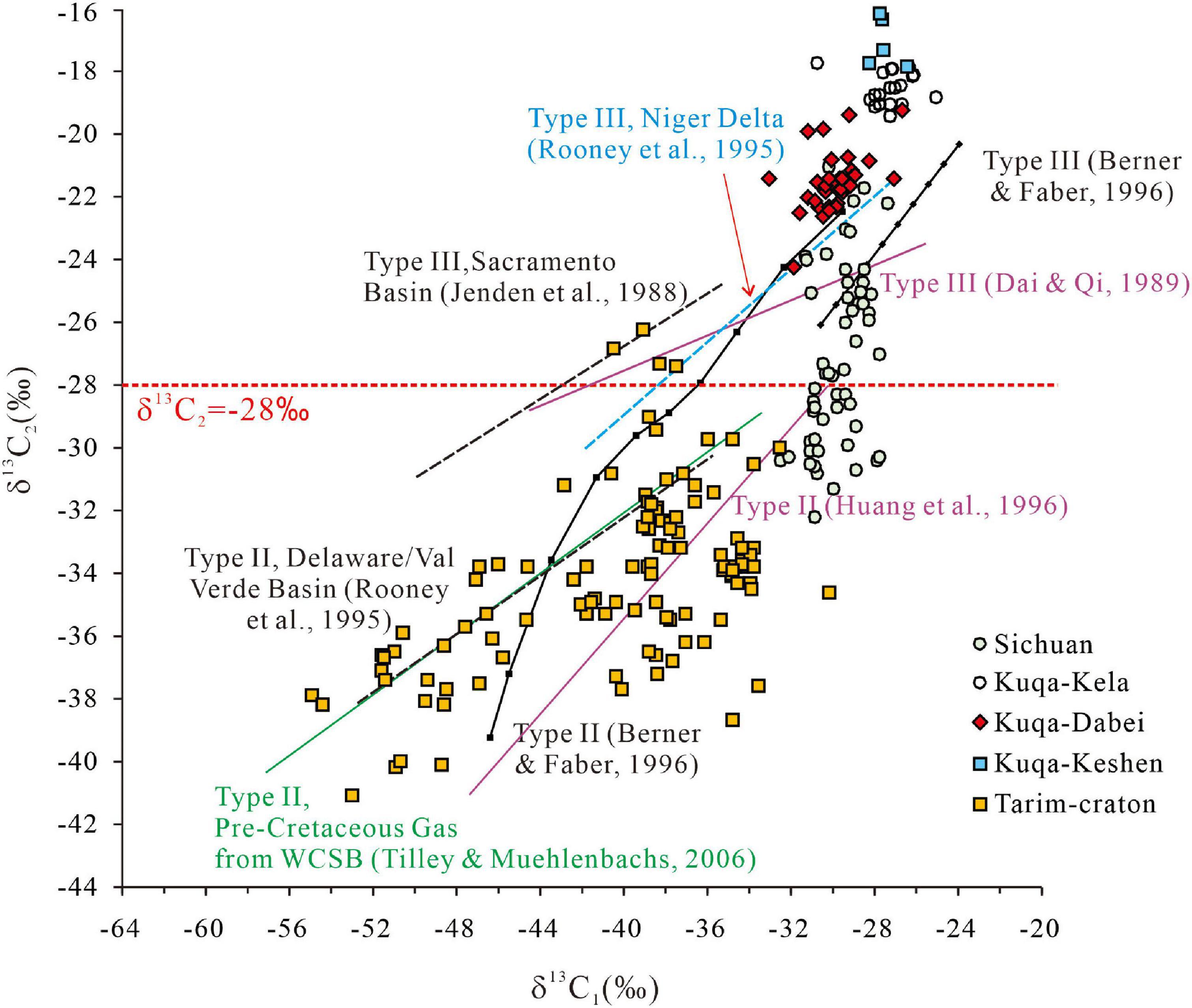
Figure 6. δ13C1 vs. δ13C2 cross-plot of gases from Dabei, Keshen and Kela2 gas fields in the Kuqa depression, craton area of Tarim Basin, and Sichuan Basin. Also shown are published maturity trends of type II kerogen (Rooney et al., 1995; Huang et al., 1996; Tilley and Muehlenbachs, 2006) and type III kerogen (Jenden et al., 1988; Dai and Qi, 1989; Rooney et al., 1995; Berner and Faber, 1996). Data source see section “Samples and Methods” in the text.
Humic organic matter is dominated by aromatic structures which is enriched in 13C, while sapropelic organic matter is dominated by aliphatic structures which is enriched in 12C. During the decomposition process of kerogen, carbon isotope of methane is more inclined to the influences of thermal maturity while carbon isotope of ethane can better reflect the features of its precursor. Therefore, gas sourced from humic organic matter generally has heavier carbon isotope of ethane compared to that from sapropelic organic matter at similar thermal maturity. A number of cut-off values of δ13C2 have been proposed for the distinguishing of gas origin in sapropelic or humic according to numerous empirical studies in the Chinese sedimentary basins (Wang, 1994; Gang et al., 1997; Liang et al., 2002; Dai et al., 2005b; Xiao et al., 2008). According to the detailed gas study in the Kuqa depression by Liang et al. (2002), a cut-off value of −28‰ of δ13C for ethane was proposed to distinguish the gas sources (sapropelic vs. humic). Based on the study of the Sinian-Jurassic gases in the Sichuan Basin, Wang (1994) suggested a cut-off value of −29‰ of δ13C for ethane to distinguish the gas origin. Dai et al. (2005b) proposed a δ13C2 >−27.5‰ for gases sourced from humic organic matter and a δ13C2 <−29‰ for gases sourced from sapropelic organic matter according to a comprehensive study on the natural gas in China, and Dai et al. (2012a) suggested a cut-off value of δ13C2 >−28‰ for gases sourced from humic organic matter in the Sichuan Basin. Taking −28‰ of δ13C2 as a cut-off value, combined with the δ13C1 vs. δ13C2 diagram (Figure 6), gas from the continental sandstone reservoirs in the Dabei and Keshen gas fields in the Kelasu thrust belt in the Kuqa depression were generated from the type III kerogen and belong to coal-derived gas (Zhang et al., 2011; Dai et al., 2014; Wang, 2014; Wei et al., 2019), gases from the marine carbonate reservoirs (Ordovician and Cambrian) in the craton area in the Tarim Basin are mostly sourced from the sapropelic organic matter and belong to oil-related gas (Wang et al., 2014; Zhu et al., 2014; Chen F. et al., 2018). Gases from the marine carbonate reservoirs (Permian Changxing Formation and Triassic Feixianguan Formation) from the Longgang, Yuanba and Puguang gas fields in Sichuan Basin were complicated (Yang et al., 2002; Zhu et al., 2005; Hao et al., 2008; Ma, 2008; Zhao et al., 2011; Hu et al., 2014; Qin et al., 2016; Dai et al., 2018). Gases from the Longgang gas field were mainly generated from the coal measure of Permian Longtan Formation (Zhao et al., 2011; Qin et al., 2016; Dai et al., 2018), or with minor contribution of oil cracked gas derived from the Upper Permian carbonaceous source rocks (Deng et al., 2018). Different opinions exist for the origin of gases from Yuanba gas field. Some scholars proposed that gases from Yuanba gas field were mainly coal-derived gas or with minor contribution from oil-related gas, which were sourced from the coal measure of Permian Longtan Formation and/or organic matter in Permian Dalong Formation (Hu et al., 2014; Dai, 2016; Dai et al., 2018). However, some scholars proposed that gases from Yuanba gas field were mainly oil cracked gas from oil cracking which was generated from the Permian source rocks (Guo, 2011; Guo et al., 2014; Li et al., 2015; Wu et al., 2015b). Gases from Yuanba gas field were also interpreted as oil cracked gas sourced from the sapropelic organic matter in Sinian/Lower Cambrian Qiongzhusi Formation (Wu et al., 2017). Gases from the Puguang gas field were mainly sourced from the marine sapropelic organic matter with minor contribution from the humic organic matter in Permian Longtan Formation (Hao et al., 2008; Ma, 2008). There is also study indicating that gases in the Puguang gas field were generated from multiple sources such as Permian, Silurian and Cambrian (Zhao et al., 2011). The gas origin distinguishing can be further evidenced in the δ13C1 ∼δ13C2 ∼δ13C3 genetic diagram (Figure 7). In Figure 7, gas from the Keshen gas field has very heavy carbon isotope of ethane, which is also clearly reflected in Figure 6. Gas from the craton area of Tarim Basin has relatively heavy carbon isotope of propane and some samples even fall in the area of coal-derived origin.
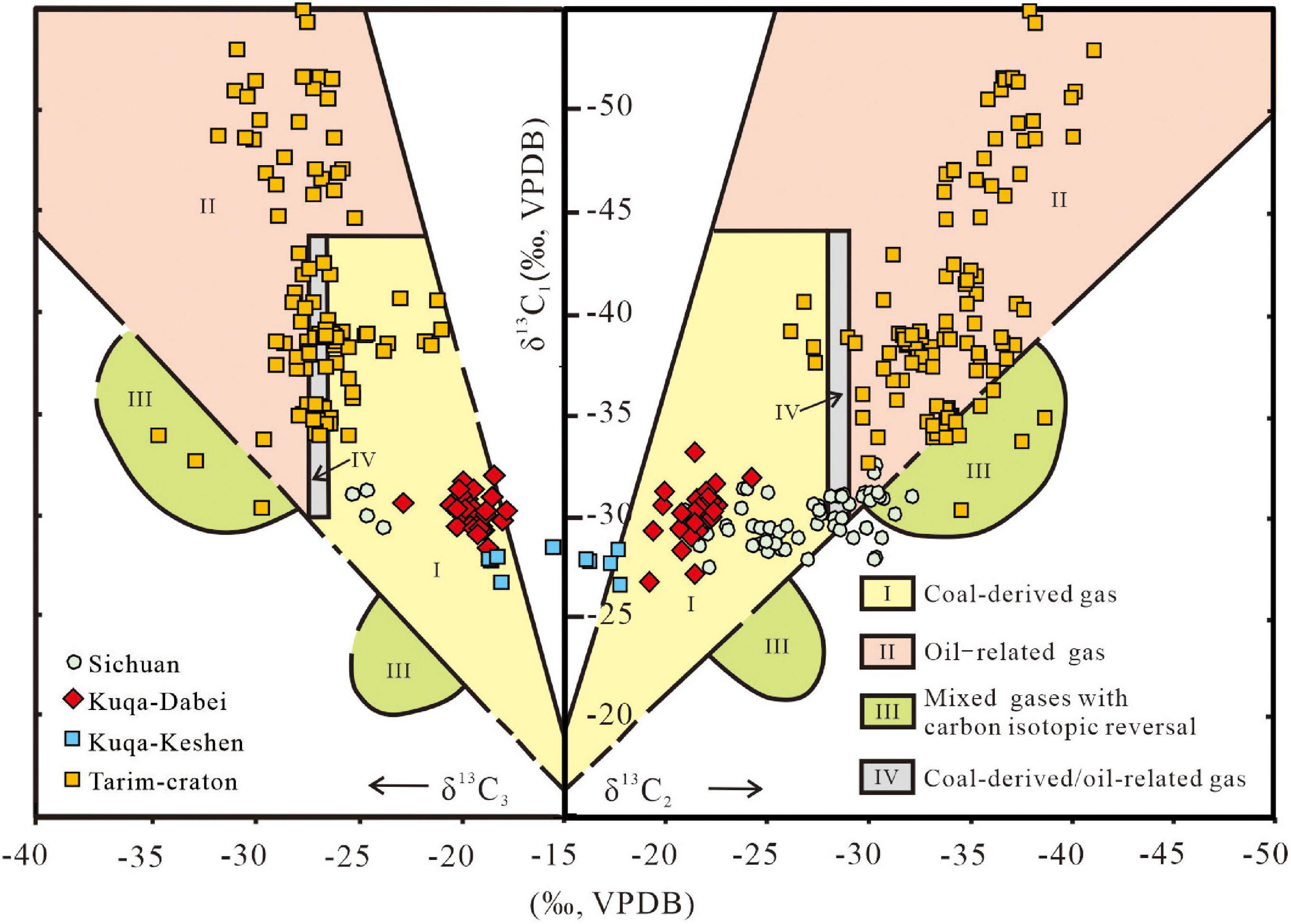
Figure 7. Plot of δ13C1 vs. δ13C2 vs. δ13C3 of gases from Dabei and Keshen gas fields in the Kuqa depression, craton area in the Tarim Basin, and Sichuan Basin (Dai et al., 1992). Data source see section “Samples and Methods” in the text.
Carbon Isotope Anomaly of the Deep Gas
Partial carbon isotopic reversal is common in the deep gas. The coal-derived tight gas from the Kuqa depression shows partial carbon isotopic reversal between ethane and propane, especially gas from the Keshen gas field, where 4 out of 5 samples have partial carbon isotopic reversal between ethane and propane (δ13C1 < δ13C2 > δ13C3). A few samples from the marine carbonate reservoirs either in the craton area of Tarim Basin (δ13C1 < δ13C2 > δ13C3) or Sichuan Basin (δ13C1 > δ13C2) also have partial carbon isotopic reversal.
Partial carbon isotopic reversal among C1∼C3 alkane gases are usually interpreted as a result from the mixing of gases generated from different types of organic matter or at different thermal maturity (biotic vs. abiotic, thermogenic vs. microbial, humic vs. sapropelic, gases with different maturities), bacterial oxidation, high thermal maturity, thermochemical sulfate reduction (Dai et al., 2004b; Hao et al., 2008). Some gas samples from the Keshen and Dabei gas fields have partial carbon isotopic reversal between ethane and propane, i.e., δ13C1 < δ13C2 > δ13C3. Due to the limited amount for C3+ heavy hydrocarbon gas, hydrogen isotopic distribution pattern between ethane and propane is not available. Such partial carbon isotopic reversal has also been reported for gases from other wells with burial depth either more than 4,500 m or less than 4,500 m in the Kelasu-Yiqikelike thrust belt and South Slope Belt (Liu et al., 2007). The partial carbon isotopic reversal between ethane and propane of gases from the Kuqa depression has been explained as abiotic gas (Zhou, 1998; Zhang, 2002). The δ13C1 vs. δ2H1 diagram (Figure 8) can better interpret the abiotic methane. As shown in Figure 8, gas from the craton area of Tarim Basin and Sichuan Basin falls in the area of thermogenic gas, while gas from the Dabei gas field falls in the overlapped area of abiotic gas and thermogenic gas. However, counterview existed according to the helium isotope. Researches found that natural gas in the Kuqa depression has R/Ra values of 0.03∼0.108 with an average of 0.05 (Qin, 1999) or 3He/4He values of n × 10–8 (Liu et al., 2007), indicating the absence of mantle-derived helium, hence proposed that the partial carbon isotopic reversal was not caused by the mixing between biotic and abiotic gases. Mixing of gases generated from different sources or generated at different thermal maturity levels have been advocated to interpret the partial carbon isotopic reversal. Zhang et al. (2011) proposed that the partial carbon isotopic reversal of gases from Dabei gas field were caused by the mixing between coal-derived gas sourced from the Jurassic coal measures and oil-related gas that was sourced from the Triassic lacustrine source rocks. Dai et al. (2014) indicated that the partial carbon isotopic reversal of gases from Dabei gas field was due to the mixing of gases with the same origin but different maturity levels. Isotopic fractionation caused by gas leakage has also been proposed to interpret the partial carbon isotopic reversal of gases from the Kuqa depression (Qin, 1999). Except these single factor, multi-factor analysis has also been carried out. For example, Liu et al. (2007) proposed that the partial carbon isotopic reversal of gases from the Kuqa depression was caused by the mixing with gas of the same origin but different maturity or resulting from the high temperature, high pressure, multistage hydrocarbon accumulation and diffusion. A recent study proposed that the partial carbon isotopic reversal was due to the mixing between gases of the same origin but different sources or mixing between oil-related and coal-derived gases or high temperature and high pressure (Wei et al., 2019).
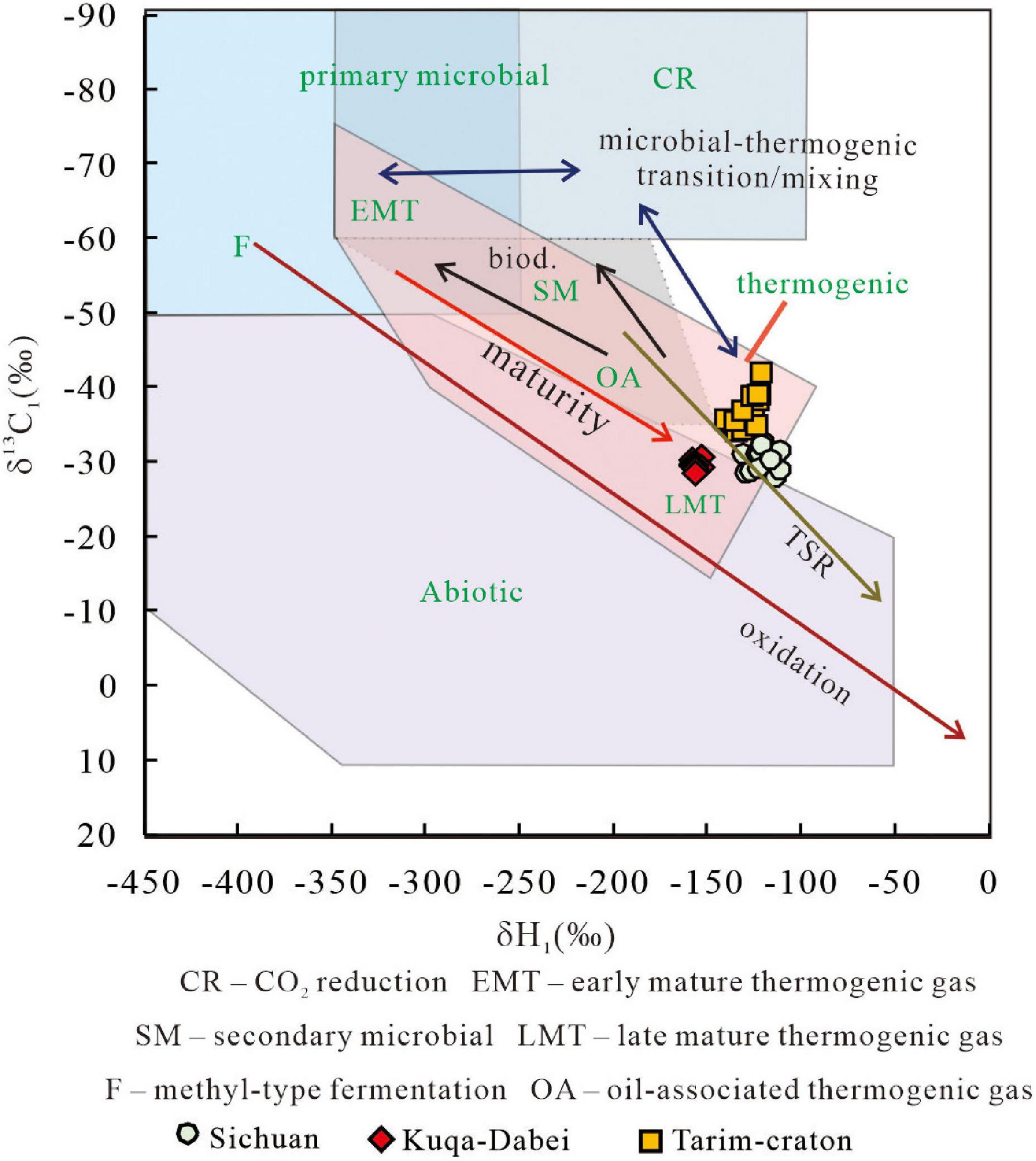
Figure 8. Plot of δ2H1 vs. δ13C1 of gases from Dabei gas field in the Kuqa depression, craton area of Tarim basin and Sichuan basin (Milkov and Etiope, 2018). Data source see section “Samples and Methods” in the text.
Partial carbon isotopic reversal between methane and ethane also exists in the deep gases from marine carbonate reservoirs in the Sichuan Basin (Wang, 1994; Zhu et al., 2006; Hao et al., 2008). Mixing of gases from different sources/maturity levels has been advocated to interpret the partial carbon isotopic reversal found in the gases from marine carbonate reservoirs (Wang, 1994; Hao et al., 2008; Ma, 2008; Deng et al., 2018). For example, partial carbon isotopic reversal between methane and ethane was found in the gases from Puguang gas field, which has been interpreted as mixing of oil cracked gases with different sources, mixing between secondary-cracking and primary cracking gases, in-reservoir mixing of oil cracked gases with different thermal maturities (Hao et al., 2008), or mixing of gases generated from different sources (Wang, 1994; Ma, 2008). In the Longgang gas field, partial carbon isotopic reversal between methane and ethane has been interpreted as a result from the isotopic fractionation during the migration of coal-derived gas (Dai et al., 2018), or mixing of gases generated from humic organic matter and gases generated from the cracking of oil (Deng et al., 2018).
One biggest difference between the gases from continental sandstone reservoirs and marine carbonate reservoirs is the content of H2S. Gases from the continental sandstone reservoirs have no H2S, while gases from marine carbonate reservoirs have varying content of H2S. In the Puguang gas field in Sichuan Basin, the content of H2S can be up to 58.34%. In most cases, the high content of H2S in deeply buried gas reservoirs are due to the thermochemical sulfate reduction (TSR). A number of studies have demonstrated the existence of TSR in gases from the craton area of Tarim Basin and northeastern Sichuan Basin (Cai et al., 2001, 2007, 2009, 2013; Zhu et al., 2005, 2006, 2019b; Hao et al., 2008; Liu et al., 2013). Previous studies found that TSR may affect the carbon isotopic composition of hydrocarbon gases (Krouse et al., 1988; Worden and Smalley, 1996; Cai et al., 2004; Hao et al., 2008; Pan et al., 2006; Zhu et al., 2006). After the liquid hydrocarbon are exhausted, C2∼C5 heavy hydrocarbon gases become the dominant reactants for TSR, then there will be a significant increase of CO2 and H2S content (Hao et al., 2008). At this stage, due to the kinetic isotopic fractionation, 12C-12C bond will break faster than 12C-13C bond, resulting in the preferentially loss of C2+ heavy hydrocarbon gases enriched in 12C (Worden and Smalley, 1996; Krouse et al., 1988). Methane is the least reactive compound and its involvement in TSR as a reductive reactant has long been in dispute. Therefore, during the stage with C2∼C5 heavy hydrocarbon gases as the dominant reactants for TSR, the increasing extent of TSR will cause the increase of δ13C2 values but not affect the δ13C1 values, which will change the partial carbon isotopic reversal between methane and ethane into normal trend (Hao et al., 2008). A gas souring index [GSI = H2S/(H2S + ΣCn)] has been used to show the extent of TSR (Worden et al., 1995). During this stage, CO2 should positively correlate with H2S, GSI should positively correlate with gas dryness coefficient (C1/C1+), δ13C2 values correlate negatively with δ13C1-δ13C2 values. As seen in Figure 9, the relatively high content of CO2 and H2S in the gases from Sichuan Basin (H2S: 9.78%, n = 51; CO2: 8.93%, n = 67) and the craton area of Tarim Basin (H2S: 2.2%, n = 64; CO2: 5.38%, n = 152) indicates the existence of TSR. In general, high H2S content accompanied with high CO2 content, however, such correlation between H2S and CO2 is not very strong (Figure 9). The correlation between GSI and C1/C1+, GSI and δ13C2, GSI and δ13C1−δ13C2 values is also not very apparent. This has been explained as that TSR has not affected the hydrocarbon gas (Puguang gas field) (Hu et al., 2010), or differences in the depositional environment and thermal maturity of source rocks in different gas fields (Wu et al., 2015a). Previous study in the Puguang gas field proposed that hydrocarbon gas was generated from the oil cracking and altered by TSR, and the transformation from a C2+ heavy hydrocarbon dominated TSR stage to a methane dominated stage caused different effects on the δ13C of different hydrocarbon gas (Hao et al., 2008). Considering the geological background, extremely high thermal maturity of source rocks might play a key role. The present burial depth of these marine carbonates is of 4,775∼7,150 m and the present thermal maturity is between 2.0 and 3.0% (Hao et al., 2008; Qin et al., 2016). However, the compression from the movement of the Pacific plate resulted in the entire uplift of Sichuan Basin during the Himalayan orogeny and the uplift amplitude reached 2,400 m (Liu et al., 2008; Deng et al., 2009). Before the uplift, the marine carbonate reservoir (Changxing and Feixianguan formations) in the Puguang gas field reached a maximum burial depth of 7,000 m with temperature up to 220°C (Hao et al., 2008). The maximum burial depth in the Longgang gas field is also estimated to be over 7,000 m and the present thermal maturity is 2.81% (Ro%) for the reservoir bitumen in the Changxing Formation with burial depth of 6118.81∼6119.89 m (Qin et al., 2016). The deep gases from Sichuan Basin all show extremely high gas dryness coefficient (average 0.999, n = 67) and the average content of C2+ heavy hydrocarbon is only 0.11%. Both molecular and isotopic compositions of hydrocarbon gases are affected by the thermal evolution degree of source rocks, especially with such high gas dryness coefficient, it is hard to determine the effects from thermal maturity or TSR.
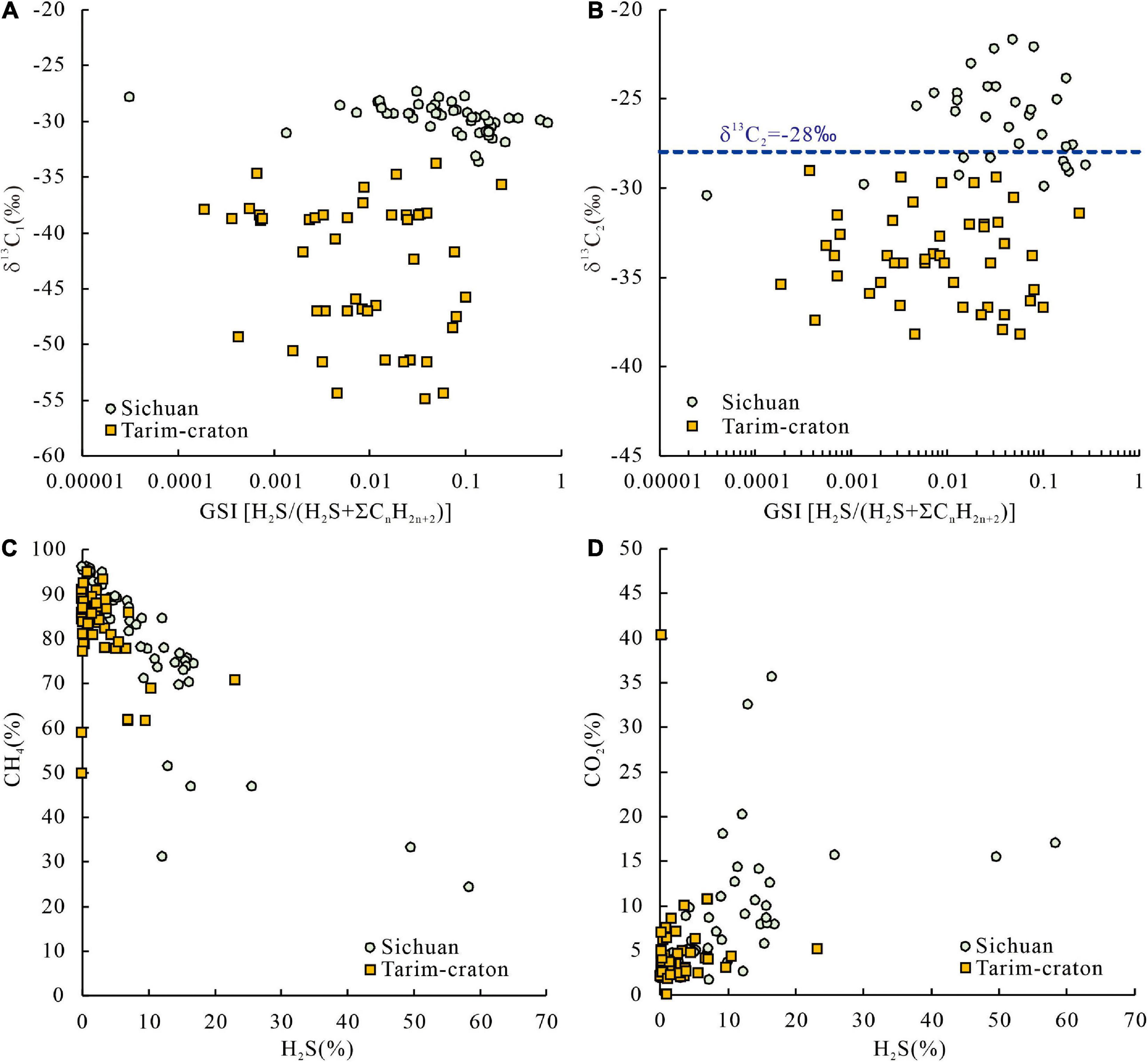
Figure 9. Plot of δ13C1 vs. GSI [H2S/(H2S + ΣCnH2n+2)] (A), δ13C2 vs. GSI [H2S/(H2S + ΣCnH2n+2)] (B), CH4 vs. H2S (C), and CO2 vs. H2S (D) of gases from the craton area of Tarim Basin and Sichuan Basin. Data source see section “Samples and Methods” in the text.
TSR will cause the increase of carbon isotope of hydrocarbon gases, which may contribute to the relatively heavy carbon isotopic values of ethane in the gases from Sichuan Basin (Figure 6). As methane can be the dominant reducing reactant for TSR after most C2+ heavy hydrocarbons are exhausted at elevated temperature (Hao et al., 2008), in this case, TSR will result in the increase of carbon isotope of methane. However, great care has to be paid to the explanation of the carbon isotope of methane since other processes also exert influences on the δ13C1 values. For example, the relatively heavy carbon isotope of methane in the Longgang gas field in Sichuan Basin was interpreted as a result of high thermal maturity of the source rocks and the mixing of gas degassed from water (Qin et al., 2016).
Origin of Non-hydrocarbon Gas CO2
Milkov and Etiope (2018) found that there is significant overlap of δ13CCO2 values for the primary microbial gas, thermogenic gas and abiotic gas in the δ13CCO2−CO2 plot (Figure 5). According to the carbon isotopic studies of 390 CO2 gas samples from different sedimentary basins in China, Dai et al. (1996) suggested a distinguishing criterion for CO2, i.e., CO2 of biotic origin (including thermogenic and microbial): CO2 content <15% and δ13CCO2 <−10‰; CO2 of abiotic origin: CO2 content >60% or δ13CCO2 >−8‰ (Figure 10). CO2 in the deep gas has wide variation of carbon isotope (Figures 5, 10). It is clear that contributions from microbial effects can be ignored (Figure 5). In general, samples from the continental sandstone reservoirs have relatively light carbon isotope of CO2 (average −15.1‰, n = 19), indicative of the thermogenic origin (Figure 10). Gases from the Sichuan Basin have δ13C value of −1.77‰ (n = 50) for CO2, indicating that most CO2 gases are of abiotic origin. As shown in Figure 10, most deep gases from Sichuan Basin fall in the region of abiotic CO2. The content of CO2 in the deep gas from Sichuan Basin has a peak range of 5∼10%. The δ13C values of CO2 have an average of −1.8‰ (n = 50) and a peak range of −5‰∼ + 3‰, which is close to the δ13C value of marine carbonate reservoir (0.9∼3.7‰) (Hu et al., 2010). A number of studies have demonstrated the abiotic CO2 was generated from the dissolution of marine carbonates through acid fluid (Hao et al., 2008, 2015; Huang et al., 2010; Liu et al., 2014). Dai et al. (2018) proposed that the CO2 was generated from the decomposition of marine carbonates during the over mature thermal evolution stage.
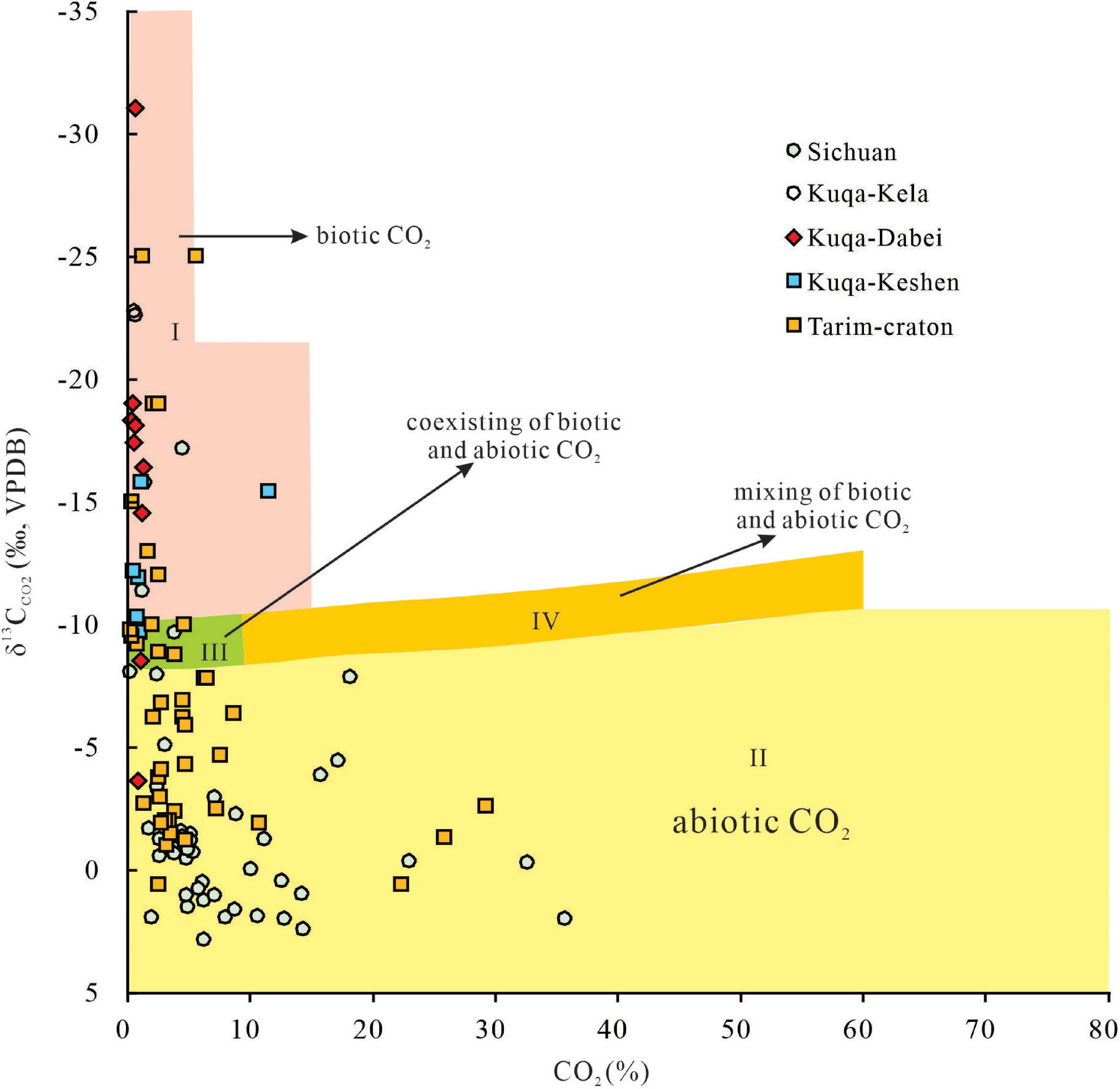
Figure 10. Plot of δ13CCO2 vs. CO2 of gases from Dabei, Keshen and Kela2 gas fields in the Kuqa depression, craton area of Tarim Basin and Sichuan Basin, distinguishing the origin of CO2 (Dai et al., 1992). Data source see section “Samples and Methods” in the text.
Thermal Maturity of Deep Gases
Gas Dryness and Gas Wetness
Due to the decomposition of heavy hydrocarbons at elevated temperature, with increasing thermal maturity of source rocks, the relative content of methane of hydrocarbon gases will increase and the content of C2+ heavy hydrocarbon gases decrease. Gas dryness coefficient [D = C1/(C1-C5)] or gas wetness coefficient [W = (C2-C5)/(C1-C5)] has been used to evaluate the gas maturity. According to compositional study of the natural gases from Ordos, Sichuan, Bohaibay, Qiongdongnan, Junggar, and Turpan-Hami basins in China, a negative correlation between gas wetness and gas maturity was established for the coal-derived gases sourced from the humic organic matter (Ro% = −0.419lnW + 1.908) (Dai et al., 2016b). In general, gases with gas dryness coefficient (D) greater than 0.95 is defined to be dry gas and gases with gas dryness coefficient (D) lower than 0.95 is wet gas. Gas generation during the thermal stage with vitrinite reflectance greater than 2.0% is usually dominated by methane and the produced gas is commonly dry gas. According to the delineation of dry gas via gas dryness coefficient, deep gases from Dabei and Keshen in the Kelasu thrust belt in Kuqa depression, Longgang, Yuanba and Puguang gas fields in Sichuan Basin are dry gas, while gases from the craton area of Tarim Basin are composed of both dry gas and wet gas. For example, gases from Shunnan-Gucheng area are mostly dry gas, while gases from Halahatang are mostly wet gas.
Theoretically, with increasing burial depth of source rocks, the geothermal temperature will increase and the thermal maturity of source rocks will increase as well. Therefore, gas dryness coefficient commonly increases with increasing burial depth. However, processes such as migration, leakage and TSR may affect the molecular composition of natural gases, thus result in the changes of gas dryness coefficient. Therefore, great care has to be paid to the researches of natural gases with gas dryness coefficient. Figure 11A shows the changes of gas dryness coefficient with burial depth of the coal-derived deep gases from Dabei and Keshen gas fields in the Kuqa depression. Gases with different burial depth of reservoirs from Dawanqi oil field (Zhou and Bao, 2011; Wang et al., 2012) and Kela2 gas field (Qin et al., 2007) in the Kelasu thrust belt were shown for comparison. According to previous studies, due to the barrier of the gypsum-salt layer with massive thickness between the Jurassic-Triassic source rocks and the Neogene Kangcun Formation reservoirs, gases generated from the Jurassic-Triassic source rocks could migrate into the Kangcun Formation reservoirs through the Tuzimaza large fault and form the Dawanqi oil field, while it formed the highly mature-over mature Dabei gas field under the gypsum-salt layer (Liu et al., 2005). Because of the influences of fault and erosion of cap layer, leakage of hydrocarbon gases occurred for the shallow gas, which result in the gas distribution with wet gas in the top and dry gas in the bottom vertically (Tian et al., 2001; Zhou and Bao, 2011). As shown in Figure 11, gases from the Dawanqi gas field have relatively low C1/(C1-C5) ratios and shallower burial depth, while with increasing burial depth, gases from Kela2, Dabei and Keshen gas fields have much higher C1/(C1-C5) ratios. Actually, gases from Dawanqi oil field have relatively heavy carbon isotope of methane (−28∼−33‰) and the δ13C1 gets lighter with increasing burial depth (Zhao, 2003), so the apparently low dryness coefficient of gases from Dawanqi oil field is mainly caused by the leakage of light composition in the shallow layers. The deep gases from Dabei and Keshen gas fields demonstrate a slight increasing trend of gas dryness coefficient with increasing burial depth, indicating the influences from thermal maturity.
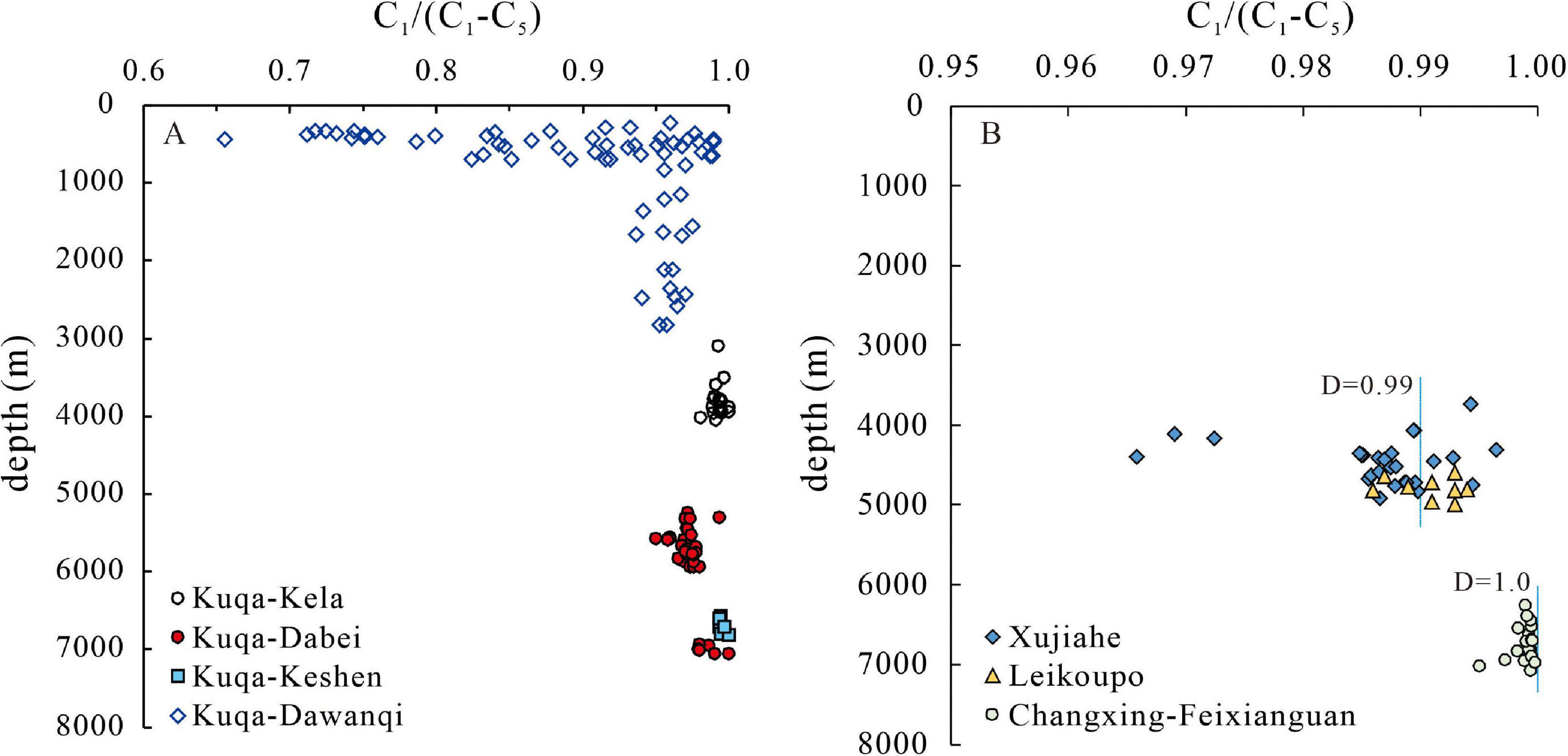
Figure 11. (A) Plot of C1/(C1-C5) vs. burial depth of natural gases from the Dabei, Keshen, Kela2 and Dawanqi gas fields in the Kuqa Depression, Tarim Basin. (B) Plot of C1/(C1-C5) vs. burial depth of natural gases from the Xujiahe, Leikoupo and Changxing-Feixianguan formations of Yuanba gas field in Sichuan Basin. Data of Kela2 gas field were from Qin et al. (2007), data of Dawanqi oil field were from Zhou and Bao (2011) and Wang et al. (2012), data of Yuanba gas field were from Dai et al. (2013), Yin et al. (2013), Huang (2014), Wu et al. (2015b) and Li et al. (2016).
TSR commonly occurred in the northeastern Sichuan Basin (Cai et al., 2003, 2013; Zhu et al., 2005, 2006; Hao et al., 2008). Since TSR consumed liquid hydrocarbon in the first, followed by C2+ heavy hydrocarbon and then methane (Hao et al., 2008), the extremely high gas dryness coefficient in the Longgang, Yuanba and Puguang gas fields not only reflects the high thermal maturity of source rocks to some extent. Figure 11B shows the natural gases from Xujiahe, Leikoupo, and Changxing-Feixianguan formations in the Yuanba gas field in Sichuan Basin. In general, all gases in the Yuanba gas field belong to dry gas and have very high dryness coefficient, indicating the high thermal maturity. Gases from Changxing-Feixianguan formations have relatively higher dryness coefficient values (nearly 1) than those from Xujiahe and Leikoupo formations, however, the difference is usually not more than 0.02. Therefore, it is hard to tell the exact contribution of thermal maturity or TSR to the high dryness coefficient of gases from Changxing-Feixianguan formations.
Logarithmic Linear Correlation Between δ13C1 and Ro%
Due to the kinetic isotopic fractionation effect during the hydrocarbon generation process, a logarithmic linear relation exists between δ13C1 and Ro% (Stahl and Carey, 1975; Schoell, 1980). A 12C-12C bond is easier to break than a 12C-13C bond, which makes the residual precursors and late gaseous products more enriched in 13C (Berner and Faber, 1996; Cramer et al., 1998; Tang et al., 2000). Different δ13C1–Ro% models have been developed for gases derived from different types of organic matters (Stahl and Carey, 1975; Schoell, 1980; Dai and Qi, 1989). So based on the relation between carbon isotope of methane and the thermal maturity of gas source rocks, one can obtain the thermal maturity level of gas source rocks by using the measured δ13C values of methane. Recently new equations of δ13C1–Ro% [δ13C1 = 25lg(Ro)−37.5 for gases sourced from the humic organic matter; δ13C1 = 25lg(Ro)−42.5 for gases sourced from the sapropelic organic matter] have been established for primary gases which have not undergone secondary alteration according to abundant measured carbon isotopic values of methane in China (Chen et al., 2020). According to the δ13C1–Ro% equation for gases from humic organic matter, deep gas from the Kuqa depression was calculated based on its carbon isotopic values of methane. The gas maturity is estimated to be 1.50∼2.75% with an average of 2.06%. This is consistent with the local geological background. In the Kuqa depression, thermal maturity is higher in the east than that in the west, and in the deposition centre such as Baicheng sag, thermal maturity of source rocks (Ro%) is 1.5∼3.5% (Dai, 2016). The gas dryness coefficient of 0.974 also implies the relatively high thermal maturation.
According to the calculation with the above δ13C1–Ro% equations, deep gases from the Yuanba, Longgang and Puguang gas fields in the Sichuan Basin also have high gas maturity around 2.0% Ro%. However, methane in these gas fields might be altered by secondary processes such as TSR, water dissolution, resulting in the changes of δ13C values of methane. Therefore, errors may exist for the gas maturity calculation based on the δ13C values of methane for these gases.
Hydrogen Isotopes
Carbon isotope of natural gas is mainly affected by the type of organic matter and the level of thermal maturity of source rocks. However, unlike the carbon isotope, the gas hydrogen isotope is highly dependent on the thermal maturity of source rocks and salinity of the water medium of the formation environment of the source organic matters (Schoell, 1980; Dai, 1993; Ni et al., 2015; Wang et al., 2015). Influences from the type of organic matter on the gas hydrogen isotope is weak, which will be easily masked by the influences from the water salinity of the depositional environment of the source organic matter (Ni et al., 2019).
In the Tarim Basin, a boundary δ2H1 value of −150‰ has been found between the gases sourced from the Middle-Lower Jurassic humic organic matter in the Kuqa depression (<−150‰) and the oil-cracked gases sourced from Cambrian-Lower Ordovician marine sapropelic organic matter in the Lunnan low uplift and the Ordovician oil-cracked gases in the Tazhong low uplift (>−150‰) (Ni et al., 2013). As shown in Figure 12, such boundary value also exists between the deep gases from the Dabei gas field in the Kuqa depression and those from the craton area of Tarim Basin. The deep gases from the Dabei gas field have δ2H value of methane of −153∼−158‰ with an average value of –156‰, while the deep gases from the craton area of Tarim Basin have relatively heavier hydrogen isotope of methane, with an average value of −130‰. Deep gases from the Yuanba and Puguang gas fields have even heavier δ2H1 value, with an average of −122‰. According to previous studies, bacterial methane sourced from the marine and saline-water lacustrine organic matter (δ2H > −190 or −200‰) has heavier hydrogen isotope compared to those from continental freshwater source rocks (δ2H < −190 or −200‰) (Schoell, 1980; Shen, 1995). Apparently deep gases from the Dabei gas field have δ2H1 value much heavier than this determining value for gases sourced from the continental freshwater organic matter.
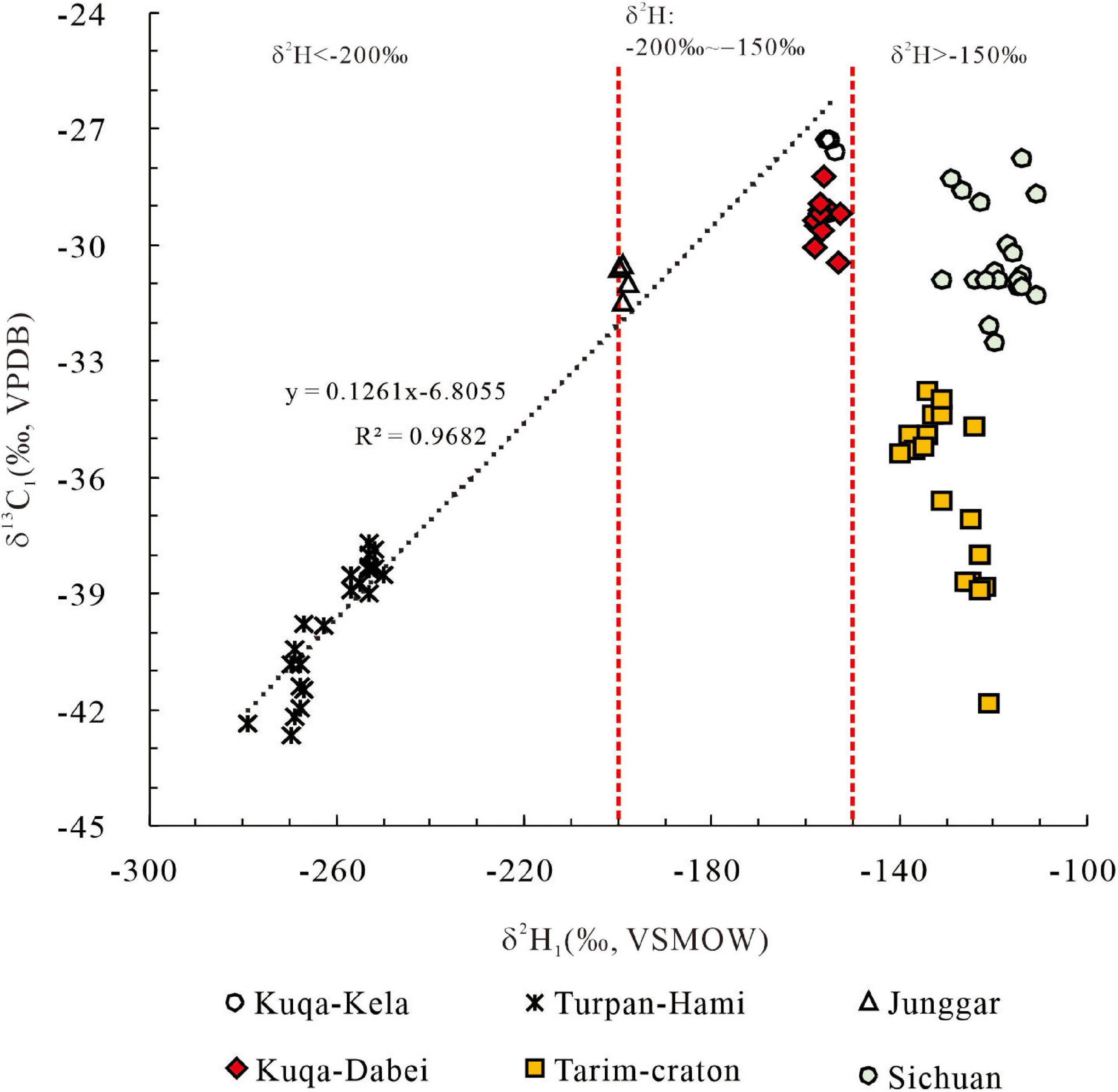
Figure 12. Plot of δ13C1 vs. δ2H1 of gases from Dabei and Kela2 gas fields in the Kuqa depression, craton area of Tarim Basin, Turpan-Harmi and Junggar basins in China. Gas data of the Dabei and craton area of Tarim Basin see section “Samples and Methods” in text, gas data of Kela2 gas field were from Qin et al. (2007), gas data of the Turpan-Hami basin were from Ni et al. (2015) and gas data of Junggar basin were from Wang et al. (2013).
The deuterium concentration in methane was found to increase with increasing thermal maturity (Schoell, 1980; Ni et al., 2011), methane in the Dabei gas field was expected to have relatively higher δ2H values due to the high thermal maturity of their source rocks (Ro%: 1.5∼3.5%). Because gas hydrogen isotope was mainly dependent on the thermal maturity and the water salinity of depositional environment of source organic matters, if one wants to investigate any influences from the thermal maturity or the water medium of depositional environment, a comparison has to be made with one fixed influencing factor. So gas samples with different thermal maturity but similar depositional environment from various locations in China were compared here (Figure 12).
Deep gases in the Dabei gas field are considered mainly sourced from the Middle-Lower Jurassic coal measures deposited in the lacustrine-swamp environment with freshwater medium (Zhang et al., 2011; Guo et al., 2016). Coal resources are widely distributed in the northwestern China and it is mainly accumulated in the Middle-Lower Jurassic formations. Actually in China the Early Middle Jurassic was one of the four major coal forming periods among the 14 coal forming periods in the geological history. The Middle-Lower Jurassic coal-bearing strata which was formed during the Early Middle coal forming period were widely distributed in the Tarim, Junggar, Turpan-Hami, Qaidam basins in northwestern China with similar depositional environment. Gases from the Turpan-Hami Basin (Ni et al., 2015), Junggar Basin (Wang et al., 2013) and Kela 2 gas field in the Kuqa depression in Tarim basin (Dai, 2016) are coal-derived gas sourced from the Jurassic coal measures. Under similar depositional environment, the differences in deuterium concentration of gases from Kela 2 gas field, Junggar and Turpan-Hami basins are mainly due to the differences in the level of thermal maturity of their source organic matters. There is a very good correlation between hydrogen and carbon isotopic compositions of methane (y = 0.1261×−6.8055, R2 = 0.9682). This may indicate a possible influence of thermal maturity on gas isotopes since organic matter 2H/1H and 13C/12C ratios increase systematically throughout the thermal maturation (Schoell, 1980; Dai, 1993; Li et al., 2001).
Compared to the gases from Kela 2 gas field, Turpan-Hami and Junggar basins, considering the influences from thermal maturity of source rocks, the Dabei gases are relatively much more enriched in D at similar thermal maturity level and show remarkable offset toward the maturity trend (Figure 12). However, gases from these four areas demonstrate consistently quite good correlation between δ13C1 and δ13C2 (R2 = 0.9007), δ2H1 and δ2H2 (R2 = 0.9975) (Figure 13). This implies that the carbon isotope is affected by one type of factor while the hydrogen isotope is affected by another type of factor. Therefore, under one type of influencing factor, the correlation can be linear between methane and ethane for both carbon and hydrogen isotopes. If the carbon isotope was plotted vs. hydrogen isotope, then offset will occur (Figure 12).
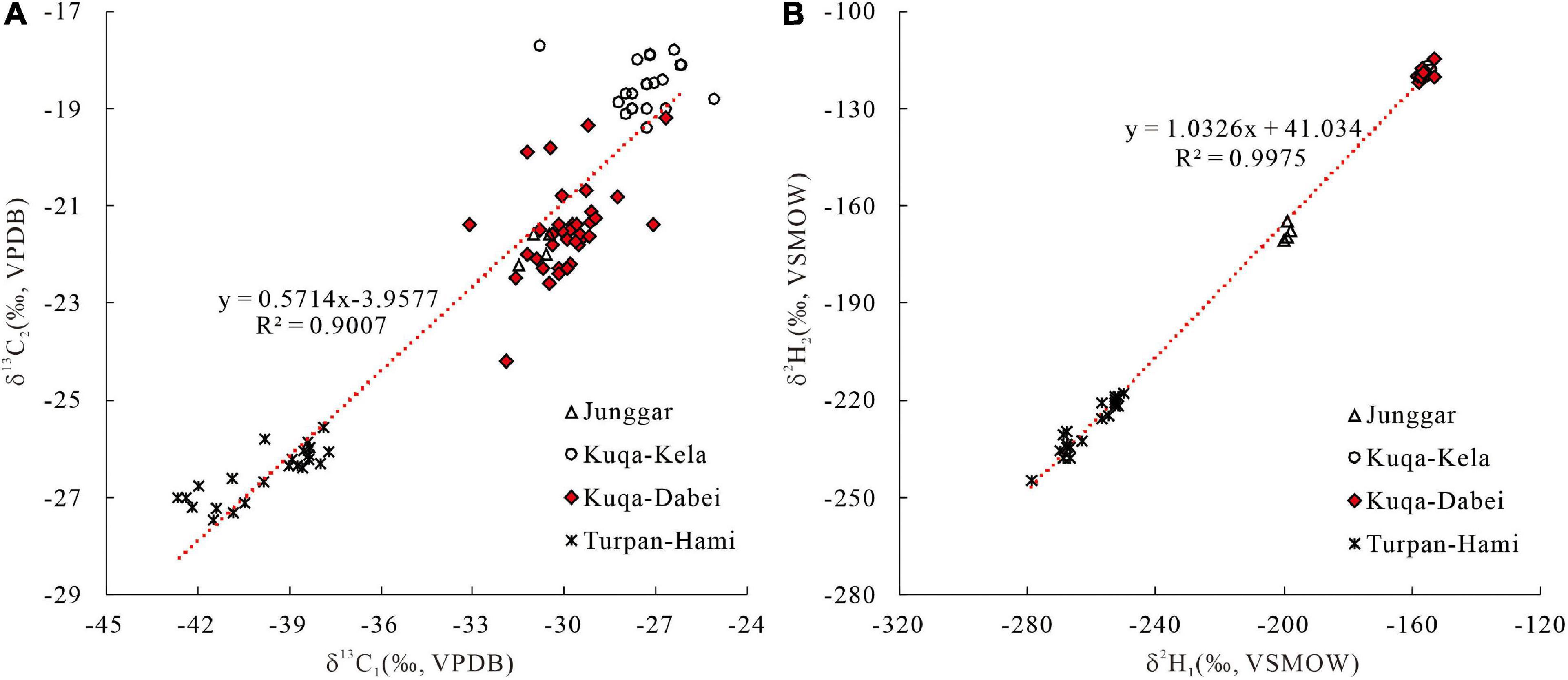
Figure 13. Plots of δ13C1 vs. δ13C2 (A) and δ2H1 vs. δ2H2 (B) of gases from Dabei and Kela2 gas fields in the Kuqa depression in Tarim Basin, also from the Turpan-Harmi and Junggar basins in China. Gas data of Kela2 gas field were from Qin et al. (2007), gas data of the Turpan-Hami Basin were from Ni et al. (2015) and gas data of Junggar Basin were from Wang et al. (2013).
Considering the heavy carbon isotopes of methane (average: –30.0‰) and ethane (average: –21.7‰), it is unlikely that the heavy hydrogen isotope is due to the mixing with oil-cracked gases. Gas derived from marine source rocks normally has much heavier hydrogen isotope due to the saline water depositional environment, for example, gases from the Lunnan and Tazhong areas in the Tarim basin are gases sourced from the Cambrian-Lower Ordovician marine sapropelic organic matter with saline water depositional environment, so they are more enriched in D but depleted in 13C (Figure 12; Ni et al., 2013). Oil-cracked gas normally has much heavier carbon isotope of ethane compared to the oil-associated gas but normally still less than –28‰, and it also contains trace amount of ethane, i.e., the oil-cracked gas from the Sinian strata in the Weiyuan gas field (Dai et al., 2003). The carbon isotopic distribution pattern among methane and its homologues is normal in general and only a few samples have carbon isotopic reversal between ethane and propane of the deep gases from Dabei gas field (Table 1). To some extent, mixing of gases with different origins often causes a partial reversed carbon isotopic distribution pattern among methane and its homologues (Dai et al., 2004b). However, mixing with gases from sapropelic organic matter from deeper strata is unlikely. Though potential mixing with gases from the marine sapropelic organic matter will cause the enrichment of D, but it will also cause the depletion of 13C, especially for ethane, which is not consistent with the heavy carbon isotope of the deep gases in the Dabei gas field.
Except the thermal maturity, one other potential cause for the relatively heavy hydrogen isotope is the saline depositional environment. The Kela 2 gases are on the maturity trend, while the gases from Dabei gas field have a big offset (Figure 12), which indicates that differences exist in the depositional environment of their source rocks. Based on the studies of paleontological fossil, mineralogy and petrology, and geochemistry (biomarkers, trace elements and carbon/hydrogen isotopes), a number of studies have demonstrated that marine transgressive happened several times during the Triassic-Jurassic period in the Kuqa depression (Chen, 1995; Zhou et al., 1999; Zhang B. et al., 2006). For example, based on the research of paleontological fossils and trace elements, Zhang B. et al. (2006) proposed that there were three big transgressive during the Triassic-Jurassic period in the Kuqa depression. According to the research based on stromatolite and trace elements, Chen (1995) thought that marine transgressive existed during the Middle Jurassic in the Tarim Basin. The author calculated the paleosalinity to be 33.4‰ according to the boron content, which is very similar to the modern seawater salinity. Extensive transgressive-regressive cycles during the Jurassic have been reported in the worldwide since Jurassic sea-level curves were first proposed several decades ago (Hallam, 1978, 2001). Major episodes of eustatic rise occurred in the Early Hettangian, Early Sinemurian, Early Pliensbachian, Early Toarcian, Early and Late Bajocian, Middle Callovian and Late Oxfordian to Kimmeridgian, among them, the Early Bajocian transgessive/deepening event can be recognised widely across the world (Hallam, 2001). Therefore, the relatively heavy hydrogen isotope in the Dabei gases may result from a possible saline depositional environment. Compared to the gases from Kela 2 gas field, the deep gases from Dabei gas field are more inclined to the influences from the transgressive, which caused the apparent enrichment in D in the deep gases in the Dabei gas field.
Conclusion
With increasing improvements in technological development and theoretical understanding, deep and ultra-deep areas have become more and more important, especially in the mature exploration area. A comprehensive knowledge about the geochemical characteristics of deep gas from continental sandstone reservoirs and marine carbonate reservoirs may improve our understanding of the origin and accumulation of deep gas. This study carried out a detailed comparison of the molecular composition, stable carbon and hydrogen isotopes of deep gases from the clastic sandstone reservoirs in the Dabei and Keshen gas fields in the Kuqa depression, Tarim basin, and deep gases from the marine carbonate reservoirs in the craton area of Tarim Basin and Sichuan Basin (Yuanba, Longgang, Puguang gas fields). The following conclusions were obtained.
(1) Deep gas, either from the continental sandstone reservoirs of Dabei and Keshen gas fields in the Kuqa depression, Tarim Basin or from the marine carbonate reservoirs of the craton area of Tarim Basin and Puguang, Yuanba and Longgang gas fields in the Sichuan Basin, is dominated by alkane gas. The average content of methane is about 83.80%. Deep gas from the Kuqa depression in Tarim Basin has gas dryness coefficient of 0.976, while gas from the Sichuan Basin has even higher gas dryness coefficient of 0.999, indicative of dry gas. Deep gas from the craton area of Tarim Basin has various gas dryness coefficient, mainly 0.594∼0.999, indicating the existence of both dry gas and wet gas. N2 and CO2 are the common non-hydrocarbon gases in the deep gas. One important difference between the deep gas from sandstone reservoirs and that from carbonate reservoirs is the content of H2S. Deep gas from the continental sandstone reservoirs have no H2S, while deep gases from the marine carbonate reservoirs often have H2S, which can be even higher than 45%.
(2) Deep gas from the continental sandstone reservoirs in Dabei and Keshen gas fields in the Kuqa depression has δ13C2 values much higher than −28‰, and has δ13C1∼δ13C2 maturity trend similar to the gases generated from type III kerogen, indicative of coal-derived origin. Most gas samples from the craton area of Tarim Basin have δ13C2 values lower than −28‰ and match better with the evolution trend of gas generated from type II kerogen. Deep gases from the Sichuan Basin include two types: gases generated from humic type III kerogen, and gases generated from sapropelic type II kerogen. Carbon isotopic anomaly such as relatively heavy carbon isotope of methane or/and ethane and carbon isotopic reversal is common in the deep gas. Carbon isotopic reversal between ethane and propane exists in the deep gas from Kuqa depression, and carbon isotopic reversal between methane and ethane is often found in the deep gas from Sichuan Basin. Secondary alteration such as mixing, TSR, diffusion, high temperature may have important contribution to such carbon isotopic anomaly.
(3) A boundary δ2H1 value of −150‰ is found between the deep gases from the continental sandstone reservoirs in the Kuqa depression (<−150‰) and the deep gases from the marine carbonate reservoirs in the craton area of Tarim Basin and Sichuan Basin (>−150‰). The deep gas from Kuqa depression has average δ2H value of methane of –156‰, while the deep gas from the craton area of Tarim Basin has average δ2H value of methane of −130‰ and the deep gas from Yuanba and Puguang gas fields has even heavier δ2H1 value of −122‰. The extremely high hydrogen isotope of methane in Dabei gas field is due to the high thermal maturity and possible saline depositional environment of the source rocks.
Data Availability Statement
The original contributions presented in the study are included in the article/supplementary material, further inquiries can be directed to the corresponding author/s.
Author Contributions
YN: manuscript writing. LY, FL, and JC: manuscript discussion. CY: experimental analyses. GZ: assistance in sample collection. All authors contributed to the article and approved the submitted version.
Funding
This work was funded by Petrochina Project (2017D-5008-08 and 2019A-0209) and China National Major S&T Projects (2016ZX05007-01).
Conflict of Interest
All authors are affiliated with the company institution Research Institute of Petroleum Exploration and Development, PetroChina.
Acknowledgments
We thank Prof. Jinxing Dai from the PetroChina Research Institute of Petroleum Exploration and Development for long-standing helpful and open discussion on an earlier version of this study.
References
Bernard, B. B., Brooks, J. M., and Sackett, W. M. (1976). Natural gas seepage in the Gulf of Mexico. Earth Planet. Sci. Lett. 31, 48–54. doi: 10.1016/0012-821x(76)90095-9
Berner, U., and Faber, E. (1996). Empirical carbon isotope/maturity relationships for gases from algal kerogens and terrigenous organic matter, based on dry, open-system pyrolysis. Org. Geochem. 24, 947–955. doi: 10.1016/s0146-6380(96)00090-3
Cai, C., Hu, W., and Worden, R. H. (2001). Thermochemical sulphate reduction in Cambro–Ordovician carbonates in Central Tarim. Mar. Petrol. Geol. 18, 729–741. doi: 10.1016/s0264-8172(01)00028-9
Cai, C., Worden, R. H., Bottrell, S. H., Wang, L., and Yang, C. (2003). Thermochemical sulphate reduction and the generation of hydrogen sulphide and thiols (mercaptans)in Triassic carbonate reservoirs from the Sichuan Basin. China Chem. Geol. 202, 39–57. doi: 10.1016/s0009-2541(03)00209-2
Cai, C., Wu, G., Li, K., Chen, L., Li, M., and Li, H. (2007). Thermochemical sulfate reduction and origin of sulfur in crude oils in Palaeozoic carbonates. Bull. Min. Petrol. Geochem. 26, 44–48.
Cai, C., Xie, Z., Worden, R. H., Hu, G., Wang, L., and He, H. (2004). Methane-dominated thermochemical sulphate reduction in the Triassic Feixianguan Formation East Sichuan Basin, China: towards prediction of fatal H2S concentrations. Mar. Petrol. Geol. 21, 1265–1279. doi: 10.1016/j.marpetgeo.2004.09.003
Cai, C., Zhang, C., Cai, L., Wu, G., Jiang, L., Xu, Z., et al. (2009). Origins of Palaeozoic oils in the tarim basin: evidence from sulfur isotopes and biomarkers. Chem. Geol. 268, 197–210. doi: 10.1016/j.chemgeo.2009.08.012
Cai, C., Zhang, C., He, H., and Tang, Y. (2013). Carbon isotope fractionation during methane-dominated TSR in East Sichuan Basin gasfields, China: a review. Mar. Petrol. Geol. 48, 100–110. doi: 10.1016/j.marpetgeo.2013.08.006
Chen, F., Zhang, Y., Zhu, G., Zhang, B., Lu, Y., and Zhang, Z. (2018). Geochemistry and accumulation process of deep natural gas in the platform-basin region, Tarim Basin. Nat. Gas. Geosci. 29, 880–891.
Chen, J., Li, W., Ni, Y., Dai, X., Liang, D., Deng, C., et al. (2018a). The Permian source rocks in the Sichuan Basin and its natural gas exploration potential (Part 2): geochemical characteristics of source rocks and latent capacity of natural gas resources. Nat. Gas. Ind. 38, 33–45.
Chen, J., Li, W., Ni, Y., Liang, D., Deng, C., and Bian, L. (2018b). The Permian source rocks in the Sichuan Basin and its natural gas exploration potential (Part 1): spatial distribution of source rocks. Nat. Gas. Ind. 38, 1–16.
Chen, J., Wang, X., Chen, J., Ni, Y., Xiang, B., He, W., et al. (2020). New formula to decipher the relationship between carbon isotope composition of methane and maturity of natural gas source rocks. Sci. China 64, 470–493.
Chen, J., Xu, Y., and Huang, D. (2000). Geochemical characteristics and origin of natural gas in Tarim basin, China. AAPG Bull. 84, 591–606.
Chen, J., Xu, Y., and Huang, D. (2001). Geochemical characteristics and origin of natural gas in east portion of Tarim basin (II). Acta Sedimentol. Sin. 19, 141–144.
Chen, R. (1995). Once more discussion on the Middle Jurassic marine-flooding event deposition of the Tarim Basin. Exp. Petrol. Geol. 17, 311–315.
Chinese Petroleum Society (2016). Report on Advances in Deep Petroleum Geology Discipline. Beijing: Science and Technology of China Press.
Clayton, C. (1991). Carbon isotope fractionation during natural gas generation from kerogen. Mar. Petrol. Geol. 8, 232–240. doi: 10.1016/0264-8172(91)90010-x
Cramer, B., Krooss, B. M., and Littke, R. (1998). Modelling isotope fractionation during primary cracking of natural gas: a reaction kinetic approach. Chem. Geol. 149, 235–250. doi: 10.1016/s0009-2541(98)00042-4
Dai, J. (1993). Characteristics of carbon and hydrogen isotopes of natural gases and their discriminations. Nat. Gas. Geosci. 4, 1–40.
Dai, J. (2016). Giant Coal-Derived Gas Fields and Their Gas Sources in China. Beijing: Science Press.
Dai, J., Chen, J., Zhong, N., Pang, X., and Qin, S. (2003). Giant Gas Fields and Its Sources in China. Beijing: Science Press.
Dai, J., Ding, W., Hou, L., and Mi, J. (2004a). “Deep gas exploration and research in Songliao Basin,” in Proceedings of Symposium on Deep Gas Exploration in Songliao Basin, ed. C. Jia (Beijing: Petroleum Industry Press), 27–44.
Dai, J., Li, J., Luo, X., Zhang, W., Hu, G., Ma, C., et al. (2005a). Stable carbon isotope compositions and source rock geochemistry of the giant gas accumulations in the Ordos Basin, China. Org. Geochem. 36, 1617–1635. doi: 10.1016/j.orggeochem.2005.08.017
Dai, J., Liao, F., and Ni, Y. (2013). Discussions on the gas source of the Triassic Xujiahe Formation tight sandstone gas reservoirs in Yuanba and Tongnanba, Sichuan Basin: an answer to Yinfeng et al. Petrol. Explor. Develop. 40, 250–256.
Dai, J., Ni, Y., and Zou, C. (2012a). Stable carbon and hydrogen isotopes of natural gases sourced from the Xujiahe Formation in the Sichuan Basin, China. Org. Geochem. 43, 103–111. doi: 10.1016/j.orggeochem.2011.10.006
Dai, J., Ni, Y., Hu, G., Huang, S., Liao, F., Yu, C., et al. (2014). Stable carbon and hydrogen isotopes of gases from the large tight gas fields in China. Sci. China 44, 563–578.
Dai, J., Ni, Y., Huang, S., Gong, D., Liu, D., Feng, Z., et al. (2016a). Origins of secondary negative carbon isotopic series in natural gas. Nat. Gas. Geosci. 27, 1–7. doi: 10.1016/j.jnggs.2016.02.002
Dai, J., Ni, Y., Qin, S., Huang, S., Peng, W., and Han, W. (2018). Geochemical characteristics of ultra-deep natural gas in the Sichuan Basin, SW China. Petrol. Explor. Develop. 45, 619–628. doi: 10.1016/s1876-3804(18)30067-3
Dai, J., Ni, Y., Zhang, W., Huang, S., Gong, D., Liu, D., et al. (2016b). Relationships between wetness and maturity of coal-derived gas in China. Petrol. Explor. Develop. 43, 737–740. doi: 10.1016/s1876-3804(16)30088-x
Dai, J., Pei, X., and Qi, H. (1992). Natural Gas Geology in China (Volume I). Beijing: Petroleum Industry Press.
Dai, J., and Qi, H. (1989). Relationship of δ13C-Ro of coal-derived gas in China. Chin. Sci. Bull. 34, 690–692.
Dai, J., Qin, S., Tao, S., Zhu, G., and Mi, J. (2005b). Development trends of natural gas industry and the significant progress on natural gas geological theories in China. Nat. Gas. Geosci. 16, 127–142.
Dai, J., Song, Y., Dai, C., and Wang, D. (1996). Geochemistry and accumulation of carbon dioxide gases in China. AAPG Bull. 80, 1615–1626.
Dai, J., Xia, X., Li, Z., Coleman, D. D., Dias, R. F., Gao, L., et al. (2012b). Inter-laboratory calibration of natural gas round robins for δ2H and δ13C using off-line and on-line techniques. Chem. Geol. 31, 49–55. doi: 10.1016/j.chemgeo.2012.03.008
Dai, J., Xia, X., Qin, S., and Zhao, J. (2004b). Origins of partially reversed alkane δ13C values for biogenic gases in China. Org. Geochem. 35, 405–411. doi: 10.1016/j.orggeochem.2004.01.006
Deng, B., Liu, S., Liu, S., Li, Z., and Zhao, J. (2009). Restoration of exhumation thickness and its significance in Sichuan basin, China. J. Chengdu Univ. Technol. 36, 675–686.
Deng, Y., Hu, G., and Zhao, C. (2018). Geochemical characteristics and origin of natural gas in Changxing-Feixianguan formations from Longgang gasfield in the Sichuan basin, China. Nat. Gas. Geosci. 29, 892–907.
Dyman, T. S., Wyman, R. E., Kuuskraa, V. A., Lewan, M. D., and Cook, T. A. (2003). Deep natural gas resources. Nat. Resour. Res. 12, 41–56.
Feng, C., Liu, S., Wang, L., and Li, C. (2009). Present-day geothermal regime in Tarim Basin, northwest China. Chin. J. Geophys. 52, 2752–2762.
Gang, W., Gao, G., Hao, S., Huang, Z., and Zhu, L. (1997). Carbon isotope of ethane applied in the analyses of genetic types of natural gas. Exp. Petrol. Geol. 19, 164–167.
Guo, T. (2011). Basic characteristics of deep reef bank reservoirs and major controlling factors of gas pools in the Yuanba gas field. Nat. Gas. Ind. 31, 12–16.
Guo, X., Huang, R., Fu, X., and Duan, J. (2014). Gas accumulation and exploration direction of the Permian and Triassic reservoirs of reef-bank facies in Sichuan Basin. Oil Gas Geol. 35, 295–302.
Guo, X., Liu, K., Jia, C., Song, Y., Zhao, M., Zhuo, Q., et al. (2016). Fluid evolution in the Dabei Gas Field of the Kuqa Depression, Tarim Basin, NW China: implications for fault-related fluid flow. Mar. Petrol. Geol. 78, 1–16. doi: 10.1016/j.marpetgeo.2016.08.024
Hallam, A. (1978). Eustatic cycles in the Jurassic. Palaeogeogr. Palaeoclimatol. Palaeoecol. 23, 1–32. doi: 10.1016/0031-0182(78)90079-2
Hallam, A. (2001). A review of the broad pattern of Jurassic sea-level changes and their possible causes in the light of current knowledge. Palaeogeogr. Palaeoclimatol. Palaeoecol. 167, 23–37. doi: 10.1016/s0031-0182(00)00229-7
Hao, F., Guo, T., Zhu, Y., Cai, X., Zou, H., and Li, P. (2008). Evidence for multiple stages of oil cracking and thermochemical sulfate reduction in the Puguang gas field, Sichuan Basin, China. AAPG Bull. 92, 611–637. doi: 10.1306/01210807090
Hao, F., Zhang, X., Wang, C., Li, P., Guo, T., Zou, H., et al. (2015). The fate of CO2 derived from thermochemical sulfate reduction (TSR) and effect of TSR on carbonate porosity and permeability, Sichuan Basin, China. Earth-Sci. Rev. 141, 154–177. doi: 10.1016/j.earscirev.2014.12.001
He, Z., Jin, X., Wo, Y., Li, H., Bai, Z., Jiao, C., et al. (2016). Hydrocarbon accumulation characteristics and exploration domains of ultra-deep marine carbonates in China. China Petrol. Explor. 21, 3–14.
Hu, A., Li, M., Yang, C., Dai, J., Ma, Y., and Guo, T. (2010). Characteristics of reservoir bitumen in Puguang and Maoba gas fields with high H2S content in north-eastern Sichuan basin. Acta Petrol. Sin. 31, 231–236.
Hu, G., Yu, C., Gong, D., and Tian, X. (2014). The origin of natural gas and influence on hydrogen isotope of methane by TSR in the Upper Permian Changxing and the Lower Triassic Feixianguan formations in northern Sichuan Basin, SW China. Energ. Explor. Exploit. 32, 139–158. doi: 10.1260/0144-5987.32.1.139
Huang, D., Liu, B., Wang, T., Xu, Y., Chen, S., and Zhao, M. (1996). Genetic types of natural gases and their maturity discrimination in the east of Tarim Basin. Sci. China 39, 101–111.
Huang, R. (2014). Source and Accumulation of Natural Gas in Leikoupo Formation, Yuanba Area, Eastern-Northern Sichuan Basin. Geoscience 28, 412–418.
Huang, S., Huang, K., Tong, H., Liu, L., Sun, W., and Zhong, Q. (2010). Origin of CO2 in natural gas from the Triassic Feixianguan Formation of Northeast Sichuan Basin. Sci. China 53, 642–648. doi: 10.1007/s11430-010-0046-7
James, A. T. (1983). Correlation of natural gas by use of carbon isotopic distribution between hydrocarbon components. AAPG Bull. 67, 1176–1191.
Jenden, P. D., Newell, K. D., Kaplan, I. R., and Watney, W. L. (1988). Composition of stable-isotope geochemistry of natural gases from Kansas, mid-continent, USA. Chem. Geol. 71, 117–147. doi: 10.1016/0009-2541(88)90110-6
Krouse, H. R., Viau, C. A., Eliuk, L. S., Ueda, A., and Halas, S. (1988). Chemical and isotopic evidence of thermochemical sulphate reduction by light hydrocarbon gases in deep carbonate reservoirs. Nature 333, 415–419. doi: 10.1038/333415a0
Li, M., Huang, Y., Obermajer, M., Jiang, C., Snowdon, L. R., and Fowler, M. G. (2001). Hydrogen isotopic compositions of individual alkanes as a new approach to petroleum correlation: case studies from the Western Canada Sedimentary Basin. Org. Geochem. 32, 1387–1399. doi: 10.1016/s0146-6380(01)00116-4
Li, P., Hao, F., Guo, X., Zou, H., Yu, X., and Wang, G. (2015). Processes involved in the origin and accumulation of hydrocarbon gases in the Yuanba gas field, Sichuan Basin, southwest China. Mar. Petrol. Geol. 59, 150–165. doi: 10.1016/j.marpetgeo.2014.08.003
Li, P., Hao, F., Guo, X., Zou, H., Zhu, Y., Yu, X., et al. (2016). Origin and distribution of hydrogen sulfide in the Yuanba gas field, Sichuan Basin, Southwest China. Mar. Petrol. Geol. 75, 220–239. doi: 10.1016/j.marpetgeo.2016.04.021
Li, X., Xiao, Z., Hu, G., Tian, H., and Zhou, Q. (2005). Origin and geochemistry of natural gas in Kuqa depression, Tarim Basin. Xinjiang Petrol. Geol. 26, 489–492.
Li, Z. (2016). Research frontiers of fluid-rock interaction and oil-gas formation in deep-buried basins. Bull. Min. Petrol. Geochem. 35, 807–816.
Liang, D., Chen, J., Zhang, B., Zhang, S., Wang, F., and Zhao, M. (2003a). Terrestrial Oil and Gas Generation in the Kuqa Depression, Tarim Basin. Beijing: Oil Industry Press.
Liang, D., Zhang, S., Chen, J., Wang, F., and Wang, P. (2003b). Organic geochemistry of oil and gas in the Kuqa depression, Tarim Basin, NW China. Org. Geochem. 34, 873–888. doi: 10.1016/s0146-6380(03)00029-9
Liang, D., Zhang, S., Zhao, M., and Wang, F. (2002). Hydrocarbon sources and stages of reservoir formation in Kuqa depression, Tarim basin. Chin. Sci. Bull. Suppl. 1, 62–70. doi: 10.1007/bf02902820
Liu, Q., Jin, Z., Wu, X., Liu, W., Gao, B., Zhang, D., et al. (2014). Origin and carbon isotope fractionation of CO2 in marine sour gas reservoirs in the Eastern Sichuan Basin. Org. Geochem. 74, 22–32. doi: 10.1016/j.orggeochem.2014.01.012
Liu, Q., Qin, S., Li, J., Liu, W., Zhang, D., Zhou, Q., et al. (2007). Natural gas geochemistry and its origins in Kuqa depression. Sci. China 51, 174–182. doi: 10.1007/s11430-008-5003-3
Liu, Q. Y., Worden, R. H., Jin, Z. J., Liu, W. H., Li, J., Gao, B., et al. (2013). TSR versus non-TSR processes and their impact on gas geochemistry and carbon stable isotopes in Carboniferous, Permian and Lower Triassic marine carbonate gas reservoirs in the Eastern Sichuan Basin, China. Geochim. Cosmochim. Acta 100, 96–115. doi: 10.1016/j.gca.2012.09.039
Liu, S., Sun, W., Li, Z., Deng, B., and Liu, S. (2008). Tectonic uplifting and gas pool formation since Late Cretaceous epoch, Sichuan basin. Nat. Gas. Geosci. 19, 293–300.
Liu, W., Zhang, D., Zheng, J., Chen, M., Wang, X., and Gao, B. (2005). A preliminary discussion on geochemical dynamic tracing of oil/gas reservoiring process. Oil Gas Geol. 26, 717–723.
Lorant, F., Prinzhofer, A., Behar, F., and Huc, A. (1998). Carbon isotopic and molecular constraints on the formation and the expulsion of thermogenic hydrocarbon gases. Chem. Geol. 147, 249–264. doi: 10.1016/s0009-2541(98)00017-5
Ma, Y. (2008). Geochemical characteristics and origin of natural gases from Puguang gas field on eastern Sichuan basin. Nat. Gas. Geosci. 19, 1–7. doi: 10.1016/j.coal.2016.12.003
Ma, Y., Cai, X., Zhao, P., Luo, Y., and Zhang, X. (2010). Distribution and further exploration of the large-medium sized gas fields in Sichuan Basin. Acta Petrol. Sin. 31, 347–354.
Ma, Y., Guo, X., Guo, T., Huang, R., Cai, X., and Li, G. (2007). The Puguang gas field: new giant discovery in the mature Sichuan Basin, southwest China. AAPG Bull. 91, 627–643. doi: 10.1306/11030606062
Ma, Y., Zhang, S., Guo, T., Zhu, G., Cai, X., and Li, M. (2008). Petroleum geology of the Puguang sour gas field in the Sichuan Basin, SW China. Mar. Petrol. Geol. 25, 371–386. doi: 10.1016/j.marpetgeo.2008.01.009
Milkov, A. V., and Etiope, G. (2018). Revised genetic diagrams for natural gases based on a global dataset of >20,000 samples. Org. Geochem. 125, 109–120. doi: 10.1016/j.orggeochem.2018.09.002
Ni, Y., Dai, J., Zhu, G., Zhang, S., Zhang, D., Su, J., et al. (2013). Stable hydrogen and carbon isotopic ratios of coal-derived and oil-derived gases: a case study in the Tarim basin, NW China. Int. J. Coal Geol. 116–117, 302–313. doi: 10.1016/j.coal.2013.06.006
Ni, Y., Liao, F., Gao, J., Chen, J., Yao, L., and Zhang, D. (2019). Hydrogen isotopes of hydrocarbon gases from different organic facies of the Zhongba gas field, Sichuan Basin, China. J. Pet. Sci. Eng. 179, 776–786. doi: 10.1016/j.petrol.2019.04.102
Ni, Y., Ma, Q., Ellis, G. S., Dai, J., Katz, B., Zhang, S., et al. (2011). Fundamental studies on kinetic isotope effect (KIE) of hydrogen isotope fractionation in natural gas systems. Geochim. Cosmochim. Acta 75, 2696–2707. doi: 10.1016/j.gca.2011.02.016
Ni, Y., Zhang, D., Liao, F., Gong, D., Xue, P., Yu, F., et al. (2015). Stable hydrogen and carbon isotopic ratios of coal-derived gases from the Turpan-Hami Basin, NW China. Int. J. Coal Geol. 152, 144–155. doi: 10.1016/j.coal.2015.07.003
Pan, C. C., Yu, L. P., Liu, J. Z., and Fu, J. M. (2006). Chemical and carbon isotopic fractionations of gaseous hydrocarbons during abiogenic oxidation. Earth Planet. Sci. Lett. 246, 70–89. doi: 10.1016/j.epsl.2006.04.013
Prinzhofer, A., and Huc, A. (1995). Genetic and post-genetic molecular and isotopic fractinations in natural gases. Chem. Geol. 126, 281–290. doi: 10.1016/0009-2541(95)00123-9
Qin, S. (1999). Origin of abnormal natural gas in Kuqa Depression of Tarim Basin. Petrol. Exploration. 4, 21–23.
Qin, S., Dai, J., and Liu, X. (2007). The controlling factors of oil and gas generation from coal in the Kuqa Depression of Tarim Basin, China. Int. J. Coal Geol. 70, 255–263. doi: 10.1016/j.coal.2006.04.011
Qin, S., Yang, Y., Lv, F., Zhou, H., and Li, Y. (2016). The origin of gas in the Changxing–Feixianguan gas pools in the Longgang gas field in the Sichuan Basin, China. J. Nat. Gas. Geosci. 1, 327–334. doi: 10.1016/j.jnggs.2016.11.002
Rooney, M. A., Claypool, G. E., and Chung, H. M. (1995). Modeling thermogenic gas generation using carbon isotope ratios of natural gas hydrocarbons. Chem. Geol. 126, 219–232. doi: 10.1016/0009-2541(95)00119-0
Schoell, M. (1980). The hydrogen and carbon isotopic composition of methane from natural gases of various origins Geochim. Cosmochim. Acta 44, 649–661. doi: 10.1016/0016-7037(80)90155-6
Shen, P. (1995). Characteristics of carbon and hydrogen isotopic compositions of light hydrocarbons. Sci. China 38, 211–220.
Stahl, W. J., and Carey, B. D. (1975). Source-rock identification by isotope analyses of natural gases from fields in the Val Verde and Delaware basins, West Texas. Chem. Geol. 16, 257–267. doi: 10.1016/0009-2541(75)90065-0
Strategic Research Center of Oil and Gas Resources (2017). National Petroleum Resource Assessment. Beijing: China Land Press.
Sun, L., Zou, C., Zhu, R., Zhang, Y., Zhang, S., Zhang, B., et al. (2013). Formation, distribution and potential of deep hydrocarbon resources in China. Petrol. Explor. Dev. 40, 687–695. doi: 10.1016/s1876-3804(13)60093-2
Sun, W., Liu, S., Cao, J., Deng, B., Song, J., Wang, G., et al. (2017). Analysis on the formation conditions of large-scale marine deep and super-deep strata gas fields in the middle-nothern segments of western Sichuan Superimposed Basin, China. Acta Petrol. Sin. 33, 1171–1188.
Tang, Y., Perry, J. K., Jenden, P. D., and Schoell, M. (2000). Mathematical modeling of stable carbon isotope ratios in natural gases. Geochim. Cosmochim. Acta 64, 2673–2687. doi: 10.1016/s0016-7037(00)00377-x
Tian, Z., Zhang, G., Zou, H., Li, Q., and Pi, X. (2001). The major controlling factors and pool-forming pattern of oil and gas reservoirs in Kuqa petroleum system, Tarim basin. Petrol. Explor. Dev. 28, 12–16.
Tilley, B., and Muehlenbachs, K. (2006). Gas maturity and alteration systematics across the estern Canada Sedimentary Basin from four mud gas isotope depth profiles. Org. Geochem. 37, 1857–1868. doi: 10.1016/j.orggeochem.2006.08.010
Tuo, J. (2002). Research status and advances in deep oil and gas exploration. Adv. Earth Sci. 17, 565–571.
Wang, F., Liu, C., Zhu, L., Zhang, S., Zhang, B., and Liang, D. (2002). Thermal maturity of the Cambrian source rocks in the platform in Tarim basin Xinjiang Petrol. Geology 23, 372–375.
Wang, G. (2005). Analysis of Natural Gas Accumulation Conditions in the Cratonic Area of Tarim Basin. Ph.D thesis, Southwest Petroleum University, Chengdu.
Wang, J., Zhang, H., Zhang, R., Yang, X., Zeng, Q., Chen, X., et al. (2018). Enhancement of ultra-deep tight sandstone reservoir quality by fractures: a case study of Keshen gas field in Kuqa depression, Tarim Basin. Oil Gas Geol. 39, 77–88.
Wang, Y., Chen, J., Pang, X., Zhang, B., Chen, Z., Zhang, G., et al. (2018). Origin of deep sour natural gas in the Ordovician carbonate reservoir of the Tazhong Uplift, Tarim Basin, northwest China: insights from gas geochemistry and formation water. Mar. Petrol. Geol. 91, 532–549. doi: 10.1016/j.marpetgeo.2018.01.029
Wang, Q., Bao, J., Xie, Z., and Wang, S. (2012). Geochemical characteristics and origin of the natural gas in the Dabei-Dawanqi. J. Yangtze Univ. Sci. & Eng. 9, 28–31. doi: 10.1016/s0140-6701(02)80284-3
Wang, S. (1994). Geochemical characteristics of natural gases from the Jurassic-Sinian formations in the Sichuan basin. Nat. Gas. Ind. 14, 1–5.
Wang, T., Song, D., Li, M., Yang, C., Ni, Z., Li, H., et al. (2014). Natural gas source and deep gas exploration potential of the Ordovician yingshan formation in the shunnan-gucheng region, tarim basin. Oil Gas Geol. 35:602.
Wang, X., Liu, W., Shi, B., Zhang, Z., Xu, Y., and Zheng, J. (2015). Hydrogen isotope characteristics of thermogenic methane in Chinese sedimentary basins. Org. Geochem. 8, 178–189. doi: 10.1016/j.orggeochem.2015.03.010
Wang, X., Zhi, D., Wang, Y., Chen, J., Qin, Z., Liu, D., et al. (2013). Source Rocks and Oil-Gas Geochemistry in Junggar Basin. Beijing: Oil Industry Press.
Wang, Z. (2014). Formation mechanism and enrichment regularities of Kelasu subsalt deep large gas field in Kuqa depression, Tarim basin. Nat. Gas. Geosci. 25, 153–166.
Wang, Z., Wang, G., Xiao, Z., Li, X., Lu, Y., and Zhang, Q. (2005). Kinetics on Natural Gas Generation in the Kuqa Foreland Basin. Beijing: Science Press.
Wei, Q., Li, X., Liang, W., Sun, K., Xie, Z., Li, J., et al. (2019). Geochemical characteristics and genesis of deep tight sandstone gas in the Dabei-Keshen Zone, Kuqa Depression. Bull. Min. Petrol. Geochem. 38, 418–427.
Whiticar, M. J. (1999). Carbon and hydrogen isotope systematics of bacterial formation and oxidation of methane. Chem. Geol. 161, 291–314. doi: 10.1016/s0009-2541(99)00092-3
Worden, R. H., and Smalley, P. C. (1996). H2S-producing reactions in deep carbonate gas reservoirs: Khuff Formation, Abu Dhabi. Chem. Geol. 133, 157–171. doi: 10.1016/s0009-2541(96)00074-5
Worden, R. H., Smalley, P. C., and Oxtoby, N. H. (1995). Gas Souring by Thermochemical Sulfate Reduction at 140oC. New York, NY: Office of Scientific and Technical Information.
Wu, X., Liu, G., Liu, Q., Liu, J., and Yuan, X. (2015a). Geochemical characteristics and genetic types of natural gas in the Changxing-Feixianguan formations from Yuanba gasfield in the Sichuan basin. Nat. Gas. Geosci. 26, 2155–2165.
Wu, X., Liu, G., Liu, Q., Liu, J., and Yuan, X. (2015b). Geochemical characteristics and genetic types of natural gas in the Changxing-Feixianguan Formations from the Yuanba Gas Field in the Sichuan Basin, China. J. Nat. Gas. Geosci. 1, 267–275. doi: 10.1016/j.jnggs.2016.09.003
Wu, X., Tao, X., and Liu, J. (2014). Geochemical characteristics and genetic types of natural gas from Lunnan area in Tarim Basin. Nat. Gas. Geosci. 25, 53–61.
Wu, Z., Liu, X., Li, X., Wang, X., and Zheng, J. (2017). The application of noble gas isotope in gas-source correlation of Yuanba reservoir, Sichuan basin. Nat. Gas. Geosci. 28, 1072–1077.
Xia, X., and Gao, Y. (2017). Mechanism of linear covariations between isotopic compositions of natural gaseous hydrocarbons. Org. Geochem. 113, 115–123. doi: 10.1016/j.orggeochem.2017.07.003
Xiao, Z., Xie, Z., Li, Z., and Ma, C. (2008). Isotopic characteristics of natural gas of Xujiahe Formation in southern and middle of Sichuan basin. Geochimica 37, 245–250.
Xu, M., Zhu, C., Tian, Y., Rao, S., and Hu, S. (2011). Borehole temperature logging and characteristics of subsurface temperature in the Sichuan Basin. Chin. J. Geophys. 54, 1052–1060.
Yang, J., Wang, Y., Wang, L., Wen, Y., Liu, H., and Zhou, G. (2002). The origin of natural gases and geochemistry characters of Changxing reef and Feixianguan oolitic beach gas reservoirs in eastern Sichuan Basin. Acta Sedimentol. Sin. 20, 349–353.
Yin, F., Liu, R., Wang, W., Zhang, Y., and Pan, L. (2013). Geochemical characters of the tight sandstone gas from Xujiahe Formation in Yuanba gas field and its gas source. Nat. Gas. Geosci. 24, 621–627.
Zhang, B., Chen, J., Bian, L., and Zhang, S. (2006). Rediscussion on the sea-slooding events during Triassic to Jurassic of Kuqa Depression in Tarim Basin. Acta Geol. Sin. 80, 236–313.
Zhang, S., Zhang, B., Zhu, G., Wang, H., and Li, Z. (2011). Geochemical evidence for coal-derived hydrocarbons and their charge history in the Dabei Gas Field, Kuqa Thrust Belt, Tarim Basin, NW China. Mar. Petrol. Geol. 28, 1364–1375. doi: 10.1016/j.marpetgeo.2011.02.006
Zhang, Z., Liu, W., Zheng, J., Wang, Q., and Chen, G. (2006). Carbon isotopic reversed distribution of the soluble organic components for the Cambrian and Ordovician carbonate rocks in Tabei and Tazhong areas. J. Mineral. Petrol. 26, 69–74.
Zhao, J. (2003). Theory and Application of Natural Gas Accumulation in Foreland Basin. Beijing: Oil Industry Press.
Zhao, L., Zhang, T., Yang, X., Guo, X., and Rao, H. (2018). Gas-water distribution characteristics and formation mechanism in deep tight sandstone gas reservoirs of Keshen block, Kuqa Depression, Tarim Basin. Nat. Gas. Geosci. 29, 500–509.
Zhao, W., Xu, C., Wang, T., Wang, H., Wang, Z., Bian, C., et al. (2011). Comparative study of gas accumulations in the Permian Changxing reefs and Triassic Feixianguan oolitic reservoirs between Longgang and Luojiazhai-Puguang in the Sichuan Basin. Chin. Sci. Bull. 56, 3310–3320.
Zhao, Z. (2019). Analysis of global oil and gas exploration characteristics during the past decade. Oil Forum 38, 58–64.
Zhou, S., Li, Y., Zhang, Z., Luo, B., Yuan, J., and Duan, Y. (1999). Influence on organic matter cauced by Mesozoic transgressive events in the Kuqa Depression, Tarim Basin. Acta Sedimentol. 17, 106–111.
Zhou, X. (1998). Traces of plutonic inorganic gas were found in the Tarim Basin. Nat. Gas. Geosci. 9, 40–41.
Zhou, X., and Bao, J. (2011). Geochemical characteristics and genesis type of natural gas in the Kuqa Depression Petrol. Geol. Eng. 25, 9–12.
Zhou, X., Lü, X., Zhu, G., Cao, Y., Yan, L., and Zhang, Z. (2019). Origin and formation of deep and superdeep strata gas from Gucheng-Shunnan block of the Tarim Basin, NW China. J. Pet. Sci. Eng. 177, 361–373. doi: 10.1016/j.petrol.2019.02.059
Zhu, G., Chi, L., Zhang, Z., Li, T., Yang, H., Chen, W., et al. (2019a). Composition and origin of molecular compounds in the condensate oils of the Dabei gas field, Tarim Basin, NW China. Petrol. Explor. Dev. 46, 482–495.
Zhu, G., Zhang, Z., Zhou, X., Li, T., Han, J., and Sun, C. (2019b). The complexity, secondary geochemical process, genetic mechanism and distribution prediction of deep marine oil and gas in the Tarim Basin, China. Earth Sci. Rev. 198:102930. doi: 10.1016/j.earscirev.2019.102930
Zhu, G., Huang, H., and Wang, H. (2015). Geochemical significance of discovery in cambrian reservoirs at Well ZS1 of the Tarim Basin, Northwest China. Energy Fuels 29, 1332–1344. doi: 10.1021/ef502345n
Zhu, G., Zhang, B., Yang, H., Su, J., and Han, J. (2014). Origin of deep strata gas of Tazhong in Tarim Basin, China. Org. Geochem. 74, 85–97. doi: 10.1016/j.orggeochem.2014.03.003
Zhu, G., Zhang, S., Liang, Y., Dai, J., and Li, J. (2005). Isotopic evidence of TSR origin for natural gas bearing high H2S contents within the Feixianguan Formation of the northeastern Sichuan Basin, southwestern China. Sci. China 48, 1960–1971. doi: 10.1360/082004-147
Keywords: deep gas, ultra-deep gas, tight gas, hydrogen isotope, carbon isotope, Sichuan Basin, Tarim Basin
Citation: Ni Y, Yao L, Liao F, Chen J, Yu C and Zhu G (2021) Geochemical Comparison of the Deep Gases From the Sichuan and Tarim Basins, China. Front. Earth Sci. 9:634921. doi: 10.3389/feart.2021.634921
Received: 29 November 2020; Accepted: 03 March 2021;
Published: 15 April 2021.
Edited by:
Yunpeng Wang, Chinese Academy of Sciences, ChinaReviewed by:
Se Gong, Commonwealth Scientific and Industrial Research Organisation (CSIRO), AustraliaXiangchun Chang, Shandong University of Science and Technology, China
Copyright © 2021 Ni, Yao, Liao, Chen, Yu and Zhu. This is an open-access article distributed under the terms of the Creative Commons Attribution License (CC BY). The use, distribution or reproduction in other forums is permitted, provided the original author(s) and the copyright owner(s) are credited and that the original publication in this journal is cited, in accordance with accepted academic practice. No use, distribution or reproduction is permitted which does not comply with these terms.
*Correspondence: Yunyan Ni, bml5eUBwZXRyb2NoaW5hLmNvbS5jbg==