- 1Faculty of Geology, University of Science, Vietnam National University, Hanoi, Hanoi, Vietnam
- 2Department of Earth and Atmospheric Sciences, Indiana University Bloomington, Bloomington, IN, United States
Thoron (220Rn), an isotope of radon with a strong α-decay energy, and its short-lived metallic progeny can pose an elevated lung cancer hazard in room air when unfired-soil derived building materials are used in earthen dwellings. Changes in moisture content and density influencing the thoron exhalation rate from earthen materials into room air were studied in the laboratory with terra rossa from a village on the Ðồng Văn Karst Plateau Geopark, Việt Nam, where ethnic minorities construct traditional dwellings with unfired terra rossa walls and floors. Our results show that the thoron exhalation rate from mud surfaces depends on (i) the content of radioactive parental nuclides in mineral components; (ii) the moisture content of mud where ∼5–10 weight % water maximizes the 220Rn exhalation rate; and (iii) the density of dry mud as primarily controlled by internal macroscopic voids, fractures, and porosity. Additional time-series of 220Rn exhalation data from an interior mud wall of a terra rossa-built house under different seasonal and weather conditions show that the temperature is influencing thoron exhalation via the water vapor pressure deficit (VPD) in air and the associated amount of atmospheric moisture adsorbed onto indoor mud surfaces. Our data suggest that occupants of “mud house” earthen dwellings in northern Việt Nam are exposed to an increased thoron geohazard during cooler weather, low VPD, and high relative humidity in air. Detailed studies are needed to evaluate the thoron geohazard for inhabitants of mud-built dwellings in other climates and geological terrains.
Introduction
Most radioactivity in room air of buildings is typically caused by radon isotopes and their radioactive metallic progeny after exhalation of radon isotopes from building materials, soil, sediment, and rock containing parental radionuclides. Over half of the natural environmental radiation dosage in room air originates from radon-emitting building materials (Meisenberg and Tschiersch, 2010; Cevik et al., 2011; Trevisi et al., 2013). An abundance of studies is available about the radiation geohazard from the radon isotope 222Rn with a comparatively long half-life of 3.84 days (Samuelsson and Petterson, 1984; Sahoo et al., 2007). In fact, the name of the element radon is often used uncritically and synonymously for the isotope 222Rn (Faheem and Matiullah, 2008; Chen et al., 2010; Sakoda et al., 2010; Hassan et al., 2011; Bavarnegin et al., 2013; Yang et al., 2019), whereas few studies are concerned with the exhalation of the radon isotope 220Rn called thoron with a half-life of only 55.6 s (Tuccimei et al., 2006; Hosoda et al., 2007; Ujić et al., 2008, 2010; Kanse et al., 2013). The relatively short half-life of 220Rn and its limited transport distance via diffusion and convection from solid sources into room air often results in the common misconception that thoron exhalation and its radiation geohazard from building materials are negligible. As a consequence, thoron and its progeny have not been considered in official safety thresholds of indoor radiation exposure (Nazaroff and Nero, 1988; Urosević et al., 2008; Meisenberg and Tschiersch, 2010; Ujić et al., 2010). Moreover, thoron is rarely measured individually because the detection of short-lived thoron requires specialized equipment that can discriminate between different α-decay energies, thus often side-lining thoron quantification in radon studies (e.g., Steinhäusler, 1996). In light of the World Health Organization (WHO) (2009) efforts to reduce radiation from radon, proper recognition of the thoron radiation geohazard is important, especially for inhabitants of unfired soil-derived dwellings with significant concentrations of thoron in room air (Meisenberg and Tschiersch, 2011; Meisenberg et al., 2017).
Exhalation rates of radon isotopes from minerals and their subsequent transport into room air depend on the concentration and distribution of parental radionuclides in soil/rock-derived building materials, on the size and shape of grains, porosity, moisture content, temperature, and meteorological conditions (Balek and Beckman, 2005; Hassan et al., 2009; Sakoda et al., 2010, 2011; International Atomic Energy Agency (IAEA), 2013). This study addresses the thoron geohazard from terra rossa that is typically utilized by economically disadvantaged ethnic minorities for the construction of traditional earthen dwellings (i.e., mud houses) in mountainous regions of northern Việt Nam. Such mud houses are constructed by compacting moist, local terra rossa to gradually build up walls (Figures 1A–C) with a thickness of ∼60 cm. A typical family of six to eight people, encompassing three generations, lives in a mud house with bare earthen walls and floor. Surveys of indoor air proved that the radiation geohazard of 220Rn substantially exceeds the average environmental background thoron concentration in outdoor air, especially at close distances to earthen walls and floor (Nguyễn-Thùy et al., 2019), while thoron and its progeny account for > 80 % of the combined average effective dose and represent the predominant radioactive inhalation hazard for residents of mud houses (Nguyễn-Văn et al., 2020).
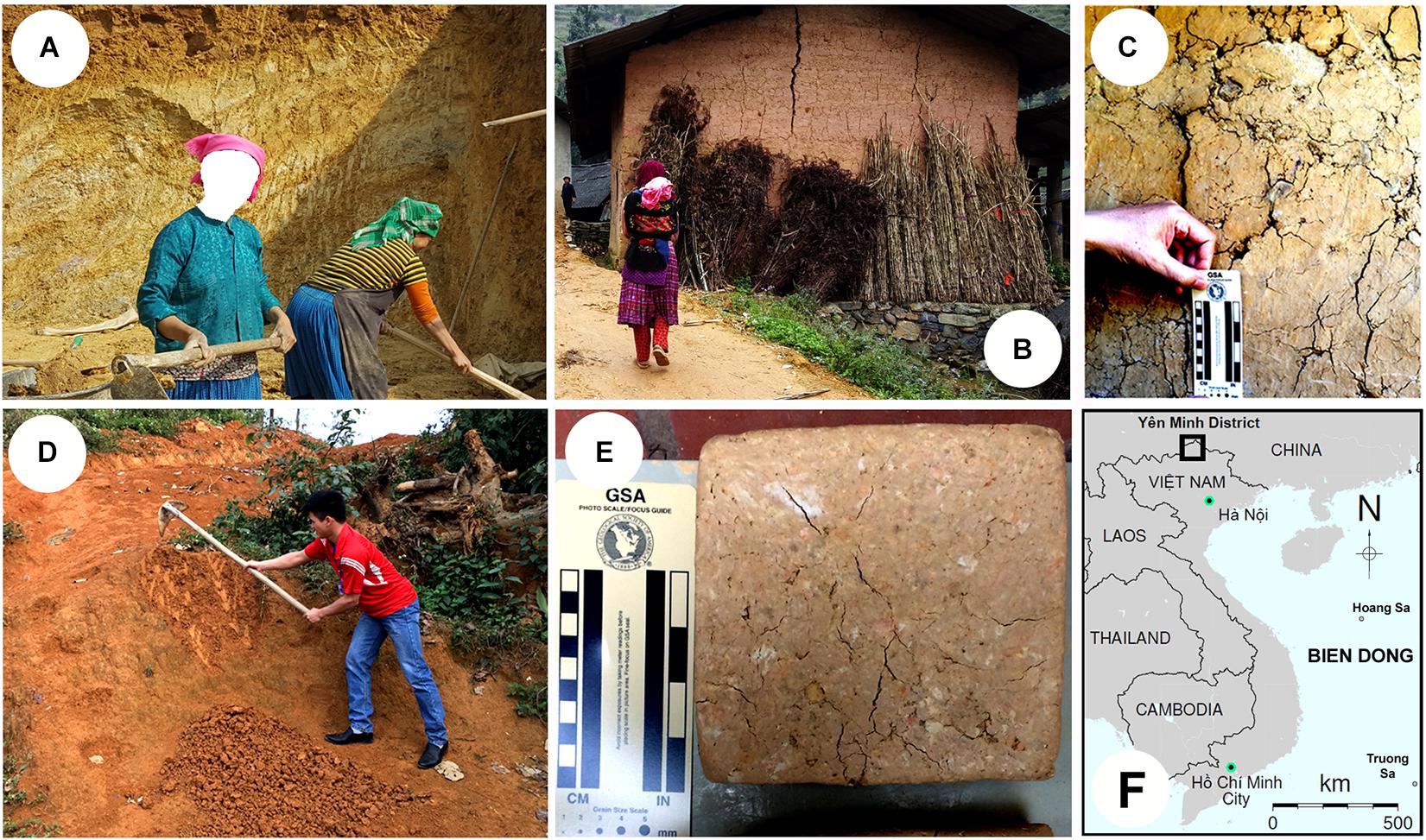
Figure 1. (A) Local mining of terra rossa for the construction of (B) Mud houses in the Ðồng Văn Karst Plateau Geopark, Hà Giang Province, northern Việt Nam. (C) Closeup view of the exterior wall of a mud house. The scale on the left is in cm. (D) Collecting terra rossa in Yên Minh District for manufacturing of (E) Mud bricks in the laboratory to measure 220Rn exhalation rates. (F) The black rectangle on the map of Việt Nam indicates the location of the Yên Minh District where terra rossa was mined for mud houses and mud bricks. Pictures of quarry (A) and mud house (B) courtesy of Jian Liu, Indiana University.
Gypsum-based plaster covering on mud walls and ceramic tiles on floors can provide effective diffusion barriers for radon, yet such modern home improvements are not affordable for most inhabitants of mud houses. Bare earthen walls and floors are able to exchange moisture with ambient room air that is seasonally providing high levels of humidity. The objective of this study is to reproducibly determine and compare the 220Rn exhalation rates from unfired terra rossa building material (i.e., representing typical mud walls in traditional earthen dwellings) under various weather conditions. The results of this study are guiding us toward devising economically affordable and culturally acceptable surface treatment options in mud houses to remediate the thoron geohazard in room air.
Materials and Methods
Origin of Mud, and Preparation of Experimental Mud Bricks
Clay-rich terra rossa was collected in December 2016 in the Ðồng Văn Karst Plateau Geopark, Hà Giang Province (23°11′N, 105°03′ E) at a location where villagers traditionally collect material for the construction of their earthen dwellings (i.e., mud houses) Figures 1A,D,F). After removing surface litter, ∼100 kg of moist terra rossa was transferred from a depth interval of ∼20 to ∼100 cm into polyethylene bags and transported to Việt Nam National University (VNU) in Hà Nội where the terra rossa was air–dried for 1 month, powdered, and sieved to a grain size of ∼2 mm to eliminate pebbles, leaves, and roots.
Kilogram aliquots of powdered terra rossa were moistened by addition of 0.2 L of water, kneaded to achieve homogeneity, and manually pressed into a square wooden mold with an interior dimension of 11 × 11 × 7.5 cm to produce 22 standard–sized mud bricks (Figure 1E) varying in wet weight from 1.2 to 2.0 kg. The variance was mainly caused by the inclusion of air bubbles when limited pressure was applied by hand during compaction. After their release from the mold, the mud bricks were air–dried for 1 week and re–weighed. Further moisture and volume losses were monitored from first stage measurements on January 9, 2017, until final measurements on December 6, 2017, to document the bricks’ surface area, weight, and bulk density. We started with 22 original mud bricks in the first stage, but that number decreased for later stages because some of the original bricks received various surface coverings to evaluate the effect on exhalation of radon isotopes. The water content of mud bricks during the first stage of measurements was determined for ∼2–g brick fragments (i.e., <0.1 wt. % of a total brick) in wt. % via their weight loss after 24 h of heating to 105°C, whereas the moisture contents of bricks during the second and third stages of measurements were determined gravimetrically for whole bricks after prolonged drying at room temperature between measurement stages.
Grain Size
Three randomly chosen samples of collected terra rossa were used for analyses of their grain size distribution. Prior to laser diffraction of wet samples with a Horiba LA 950 Grain Size Analyzer (HORIBA, Ltd., Kyoto, Japan) at VNU, organic matter was removed according to Mikutta et al. (2005). Three milliliters of 6 wt. % aqueous hydrogen peroxide (H2O2) was used to treat organic matter in approximately 10 g of terra rossa over 1 h at room temperature, followed by fivefold rinsing with deionized water, centrifugation, and disposal of the supernatant water.
Metallic Radionuclides in Terra Rossa
The metallic radionuclide occurrence in terra rossa was evaluated by randomly selecting two samples of dried terra rossa from the total collected material. Additional sieving yielded ∼15-g aliquots (equivalent to a volume of ∼10 cm3) of the 1-mm grain size fractions. After oven-drying at 105°C for 12 h, the two samples were analyzed for activities of metallic radionuclides 226Ra, 232Th, and 40K at the Analytical Center for Multi-Elemental and Isotope Research, Siberian Branch of the Russian Academy of Sciences, Novosibirsk, Russia (SB, RAS) via high-resolution semiconductor γ-spectrometry (Melgunov et al., 2003; Gavshin et al., 2005). Low concentrations of radionuclides required an extended counting time from 12 to 48 h. The accuracy and reproducibility of the analyses were controlled by parallel measurements of Russian National Geological Reference Materials, including samples of SG-1A, SG-3, SG-2, DVG, DVT, ZUK-1, BIL-1, and ST-1A (Govindaraju, 1994). The overall statistical error in the determination due to the random process of decay was < 5 % for all analytical γ lines. Detection limits for 226Ra and 232Th in the measured samples were ∼0.04 Bq, while the limit for 40K was 0.3 Bq.
The combined activity concentrations of radionuclides 226Ra, 232Th, and 40K in terra rossa samples are expressed as the radium equivalent activity (Raeq) that is calculated via the algebraic expression in Eq. 1 (OECD Nuclear Energy Agency (NEA-OECD), 1979; United Nations Scientific Committee on the Effects of Atomic Radiation (UNSCEAR), 1982, 2000; Tufail, 2012):
where Raeq is the radium equivalent γ-activity (Bq kg–1), and C226–Ra, C232–Th, and C40–K are the activity concentrations of 226Ra, 232Th, and 40K in Bq kg–1, respectively.
Exhalation Rate of 220Rn From Mud Bricks
The thoron exhalation rate from mud bricks was repeatedly measured in 2017 under standard conditions in turbulent air from a chamber (Figure 2) in three stages on January 9, January 18, and December 6 with increasing dryness of the mud bricks. A single mud brick was placed into an airtight high-density polyethylene chamber where all brick surfaces were exposed to turbulent air. Forced convection was achieved by a small fan (Figure 2). The interior dimension of the chamber measured 28 × 18 × 14 cm with a wall thickness of 2 mm. Air from the chamber rapidly streamed at a flow rate of 1 L min–1 in closed-circuit mode through laboratory vinyl tubing, a 1.0-μm air filter, and a SARAD® RTM 2200 α-spectrometer (SARAD GmbH, Dresden, Germany) with an internal membrane pump. The concentration of 220Rn was measured in 10-min intervals for at least 0.5 h. The 220Rn exhalation rate is based on Eq. 2:
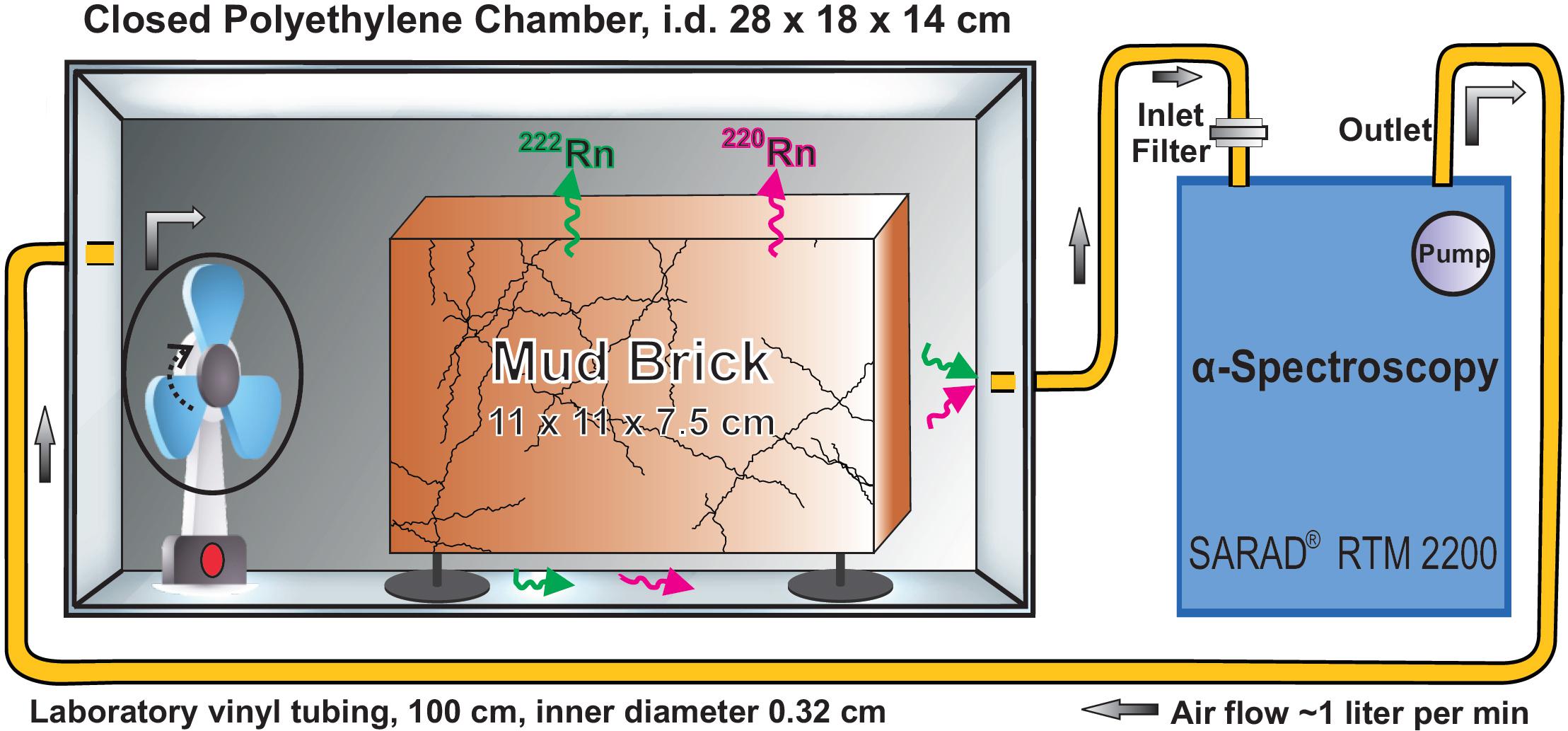
Figure 2. Schematic for the reproducible measurement of 220Rn exhalation rates from mud bricks. A mud brick is placed into a high-density polyethylene chamber exposing all brick surfaces to turbulent air. Air from the chamber rapidly circulates through tubing and an α-spectrometer. Image of fan courtesy of Freepik.
where ETn is the 220Rn exhalation rate (Bq m–2 h–1; Tuccimei et al., 2006; Kanse et al., 2013); CTn is the equilibrium concentration of 220Rn in the air of the chamber (Bq m–3); V is the volume of air surrounding the brick in the chamber (m3); A is the geometric surface area of the mud brick (m2); and λTn is the 220Rn decay constant (0.0126 s–1).
220Rn Exhalation in a Mud House
A full-sized mud house was constructed near Hà Nội (Figure 3A) with original moist terra rossa building material from Hà Giang Province, northern Việt Nam, to monitor the long-term 220Rn exhalation from mud walls throughout drying and under changing weather conditions. After completion of the construction in November 2017, a portable SARAD® Thoron Scout (SARAD GmbH, Dresden, Germany) was placed at a distance of 20 cm from an interior mud wall and 50 cm above the mud floor (Figures 3C–E). A computer fan drew air from the space between the mud wall and a wooden board through a central hole in the board into a perforated polyethylene enclosure that contained the SARAD® Thoron Scout. Although the Thoron Scout is a passive thoron detection device without an internal pump, the active air flow from an external fan makes its thoron data compatible with those from the SARAD® RTM 2200 with an internal pump (Schimmelmann et al., 2018). Hourly average 220Rn isotope concentrations in room air were automatically logged by the Thoron Scout from November 8, 2017 until January 14, 2018, with a small hiatus in early January. Measurements resumed from June 2 until August 18, 2018.
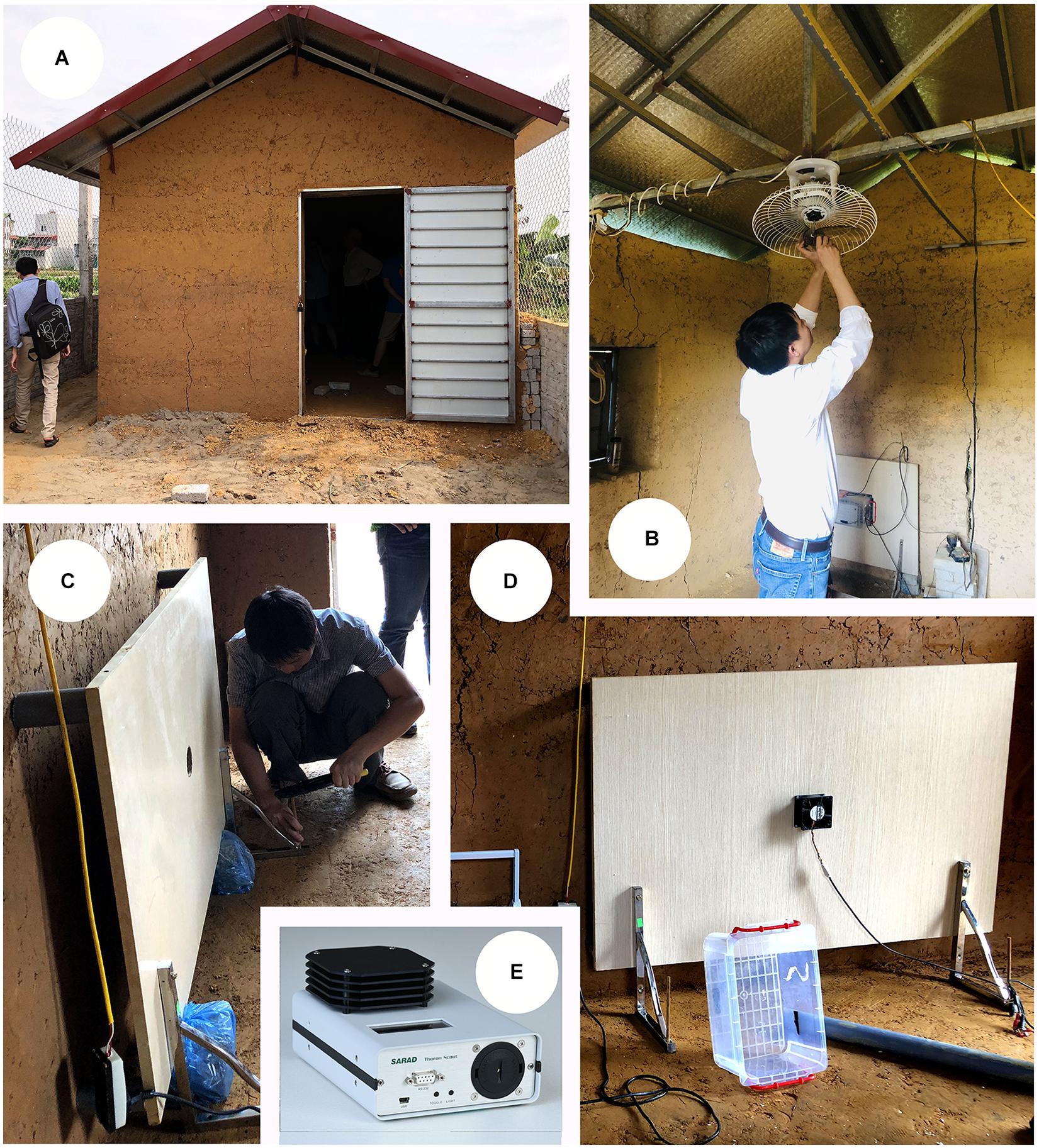
Figure 3. (A) Mud house near Hà Nội that was constructed with terra rossa from Hà Giang Province and was completed in November 2017. (B) An oscillatory ceiling fan provides optional turbulence for room air. The background shows the SARAD® Thoron Scout assembly in position ∼50 cm above the floor. (C) White board with central hole to draw air from close to the mud wall. (D) White board with fan attached. The perforated translucent polyethylene container will house the (E) Thoron Scout when attached against the white board.
Meteorological time-series of daily mean air temperature and relative humidity were recorded at the Hà Nội–Nội Bài International Airport ∼40 km north of the mud house location (Weather Underground1). A regional precipitation time-series covering the entire 2018 monsoon season in Hà Nội was obtained from Meteoblue®2. The water vapor pressure deficit (VPD) corresponds to the difference between the actual amount of water vapor in the air and the theoretical amount of water vapor at 100 % relative humidity. VPD is primarily dependent on temperature, and to a lesser extent on barometric pressure. A higher VPD indicates more intense drying of moist surfaces. To determine the VPD for outdoor air, we first calculated the actual vapor pressure at dew point temperature and the saturation vapor pressure at air temperature using Tetens’ formula (Monteith and Unsworth, 2013). Dew point temperature was calculated with coefficients A = 237.3 and B = 17.269 (Weiss, 1977). In addition to 220Rn concentrations, the SARAD® Thoron Scout instrument recorded temperature, barometric pressure, and relative humidity of the air inside the mud house as input for VPD calculation. Outdoor temperature and humidity data next to the mud house near Hà Nội were recorded in 30-min intervals by an Elitech GSP-6 temperature and relative humidity logger (Elitech, London, United Kingdom).
Adsorption of Atmospheric Moisture on Terra Rossa Mud
The initial moisture contents of five terra rossa fragments (∼1.5 g each) of a brick and seven fragments (10–70 g each) from the interior wall of a mud house (Figure 3A) were gravimetrically determined via weight loss in weight % (wt. %) after heating at 105°C for 24 h. Three dried fragments from brick and seven dried fragments from the mud wall were placed next to water-soaked sponges in a hermetically closed chamber 40 × 60 × 6 cm, without any direct contact between liquid water and terra rossa samples. The chamber with close to 100 % relative humidity in air was kept at 25 °C in the laboratory. The fragments were re-weighed daily for 14 days to monitor any weight changes owing to adsorption of moisture.
Results
Physical Properties of Terra Rossa Samples
Terra rossa represents a silty clay loam according to the classification of the U.S. Department of Agriculture (Garcìa-Gaines and Frankenstein, 2015). The sand, silt, and clay size fractions within terra rossa’s grain size range from 0.1 to 150 μm are 12.8, 36.9, and 50.2 wt. % on a dry basis, respectively.
The surface area and density of mud bricks were calculated according to measured changes in brick size and weight along slow drying (Table 1). The water content of bricks decreased through the three stages of measurements, whereas the bulk density and surface area decreased slightly (Table 1).
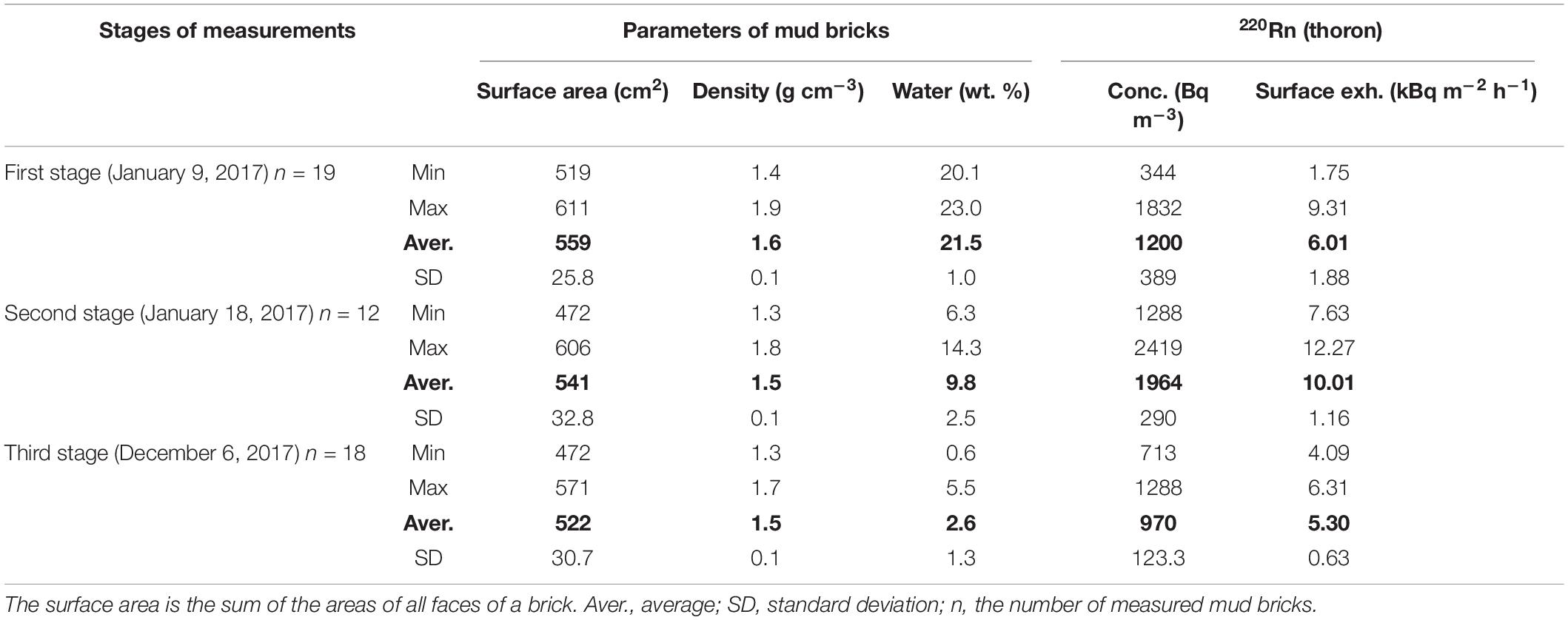
Table 1. Surface area (cm2), bulk density (g cm–3), and water content (wt. %) of cuboid-shaped mud bricks after air-drying through three experimental stages, and 220Rn (thoron) concentrations (Conc.) and surface exhalation rates (Surface exh.) of mud bricks in the polyethylene chamber.
Adsorption of Atmospheric Moisture on Terra Rossa
Fragments of terra rossa from the interior side of the ∼60-cm thick wall of a mud house near Hà Nội 3 years after its construction (consisting of similar terra rossa as used for the manufacture of mud bricks) averaged 4.2 (±1.1) wt. % moisture. In the 5 days prior to sampling of fragments during October 2020, the outdoor temperature and outdoor relative humidity in the mud house averaged ∼24°C and ∼75 %, respectively. After exposure of the dried fragments for about 2 weeks to laboratory air at 25 °C and about 100 % relative humidity, the terra rossa samples had gained on average more than 5 wt. % of moisture (Figure 4).
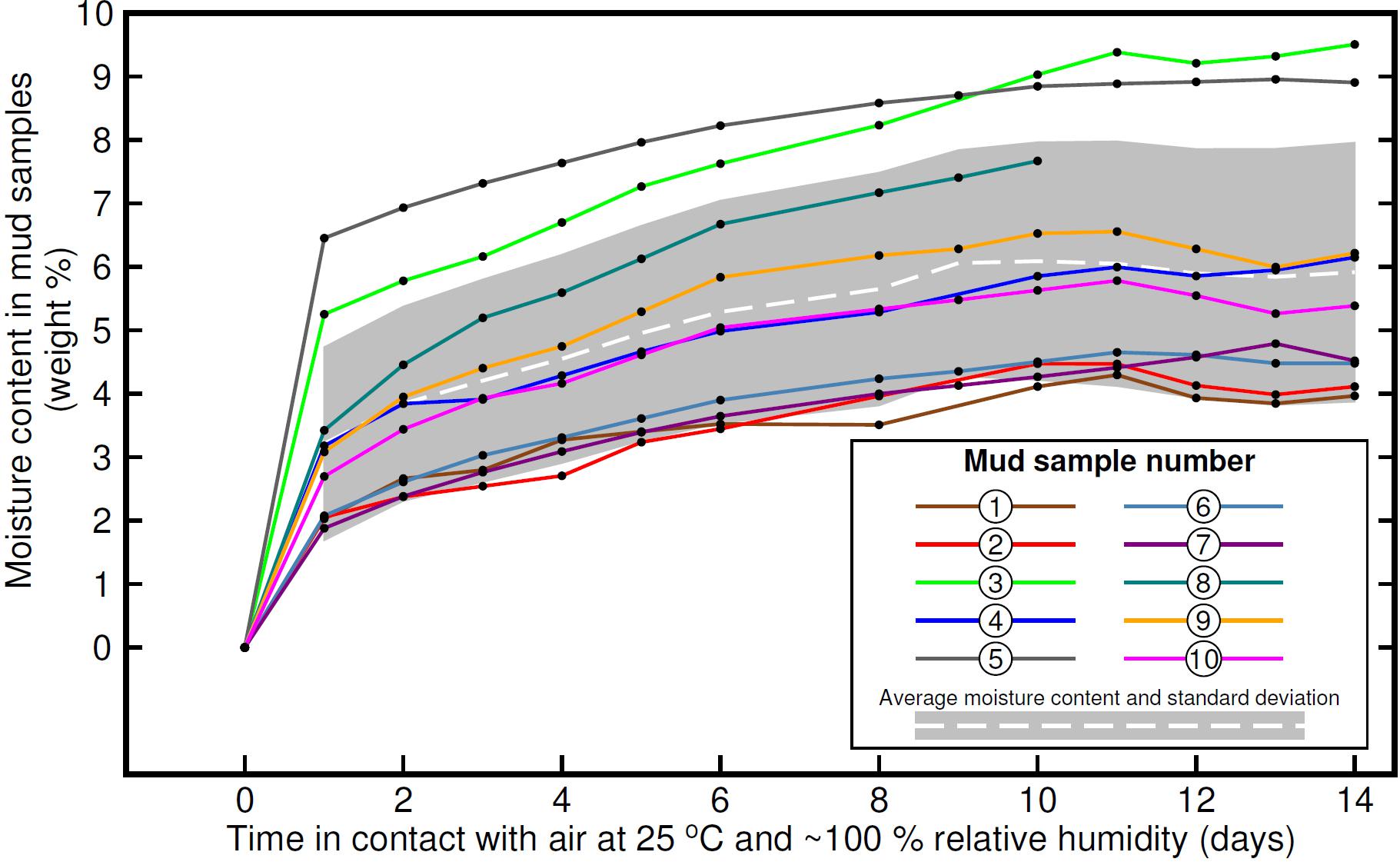
Figure 4. Time-series of adsorption of water to pre-dried (at 105°C) mud fragments from the inner wall of a mud house while exposed to air with ∼100 % relative humidity at 25 °C. The running average and its standard deviation are shown by a white dashed line and a gray band, respectively.
Concentrations of 226Ra, 232Th, 40K, and Their Radium Equivalent in Terra Rossa
The concentrations of metal radionuclides 226Ra, 232Th, and 40K in two terra rossa samples DYM and SYM were measured by semiconductor γ-spectrometry (Table 2). The two terra rossa samples show similar respective concentrations with averages of 52.0 Bq kg–1 for 226Ra, 68.5 (±2.1) Bq kg–1 for 232Th, and 259.0 (±1.0) Bq kg–1 for 40K.
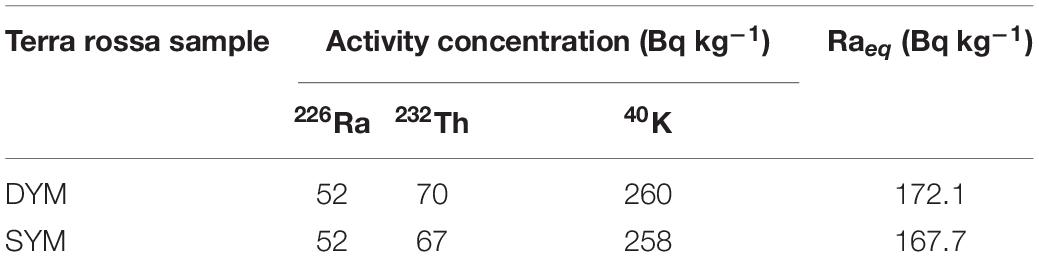
Table 2. Activity concentrations of 226Ra, 232Th, and 40K (Bq kg–1) together with their radium equivalent activity (Raeq, Bq kg–1) in two terra rossa samples.
220Rn Exhalation Rate From Experimental Mud Bricks
Measured concentrations of 220Rn in chamber air surrounding mud bricks and calculated 220Rn exhalation rates (Table 1) encompassed large ranges during the first stage of measurements when bricks still contained ≥ 20 wt. % moisture. Values became more stable in the second and third stages (Figure 5). Maximum 220Rn exhalation rates were observed during the second stage with a mean value of 10.01 (±1.16) kBq m–2 h–1 in comparison to the first stage at 6.01 (±1.88) kBq m–2 h–1 and the third stage at 5.30 (±0.63) kBq m–2 h–1.
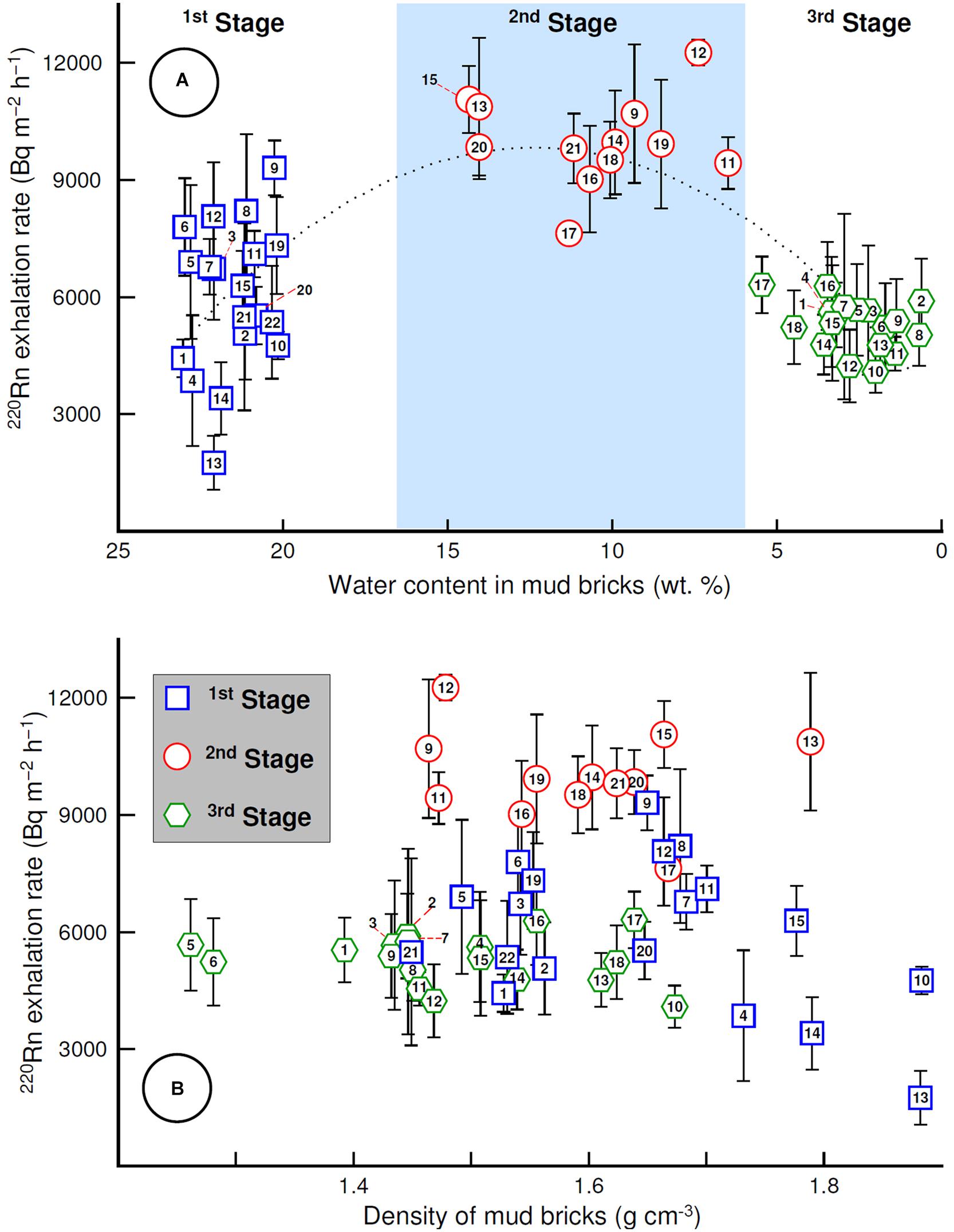
Figure 5. (A) 220Rn exhalation rates from mud bricks (numbered from 1 to 22) with different moisture contents along three consecutive measurement stages (on January 9, January 18, and December 6, 2017) along slow drying. The dotted line was drawn to guide the eye. (B) 220Rn exhalation rates from mud bricks versus bulk density. Not all bricks were measured during all stages of measurements.
220Rn Exhalation Rate From Interior Mud Wall in Mud House
Time-series of the 220Rn concentration in air close to the inside wall of a mud house near Hà Nội are compared to meteorological time-series of outdoor Hà Nội air in Figure 6. The 220Rn concentration started low in November of 2017 when the ∼60-cm thick mud walls were still glistening wet from recent construction. Over the following months, the mud walls gradually dried and the 220Rn exhalation increased until June of 2018. Drying was most intense by the end of June when high summer temperatures increased the outdoor air VPD. Continued drying during falling temperatures in the later part of the monsoon season witnessed decreasing 220Rn concentrations.
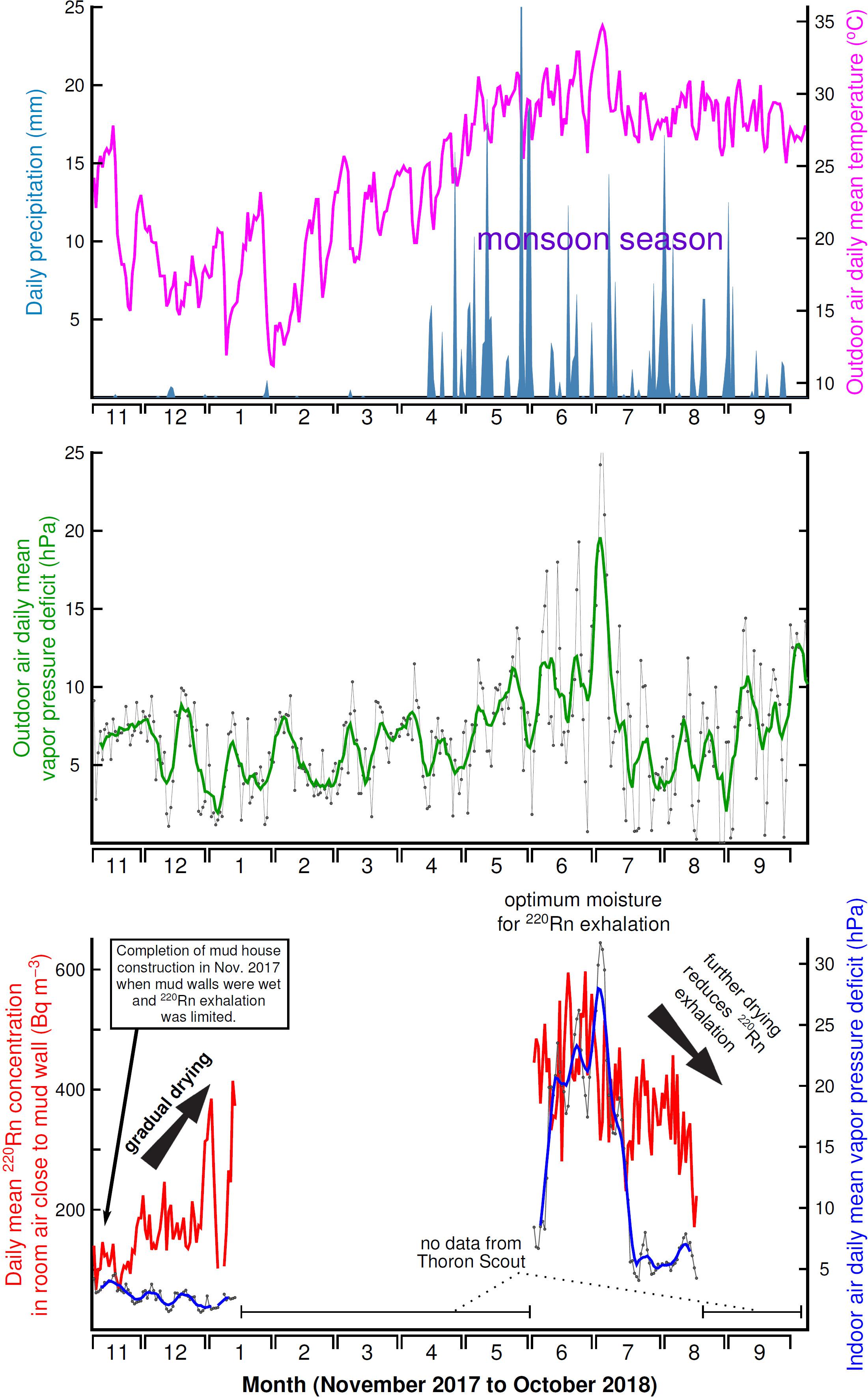
Figure 6. Time-series of daily averages of 220Rn concentration in room air of a mud house near Hà Nội based on measurements with a SARAD® Thoron Scout, in comparison with meteorological time-series of Hà Nội outdoor air (measured at Hà Nội–Nội Bài International Airport) and mud house indoor air (measured by Thoron Scout).
A 9-day time-series of 220Rn concentration in indoor air of the mud house near Hà Nội with ventilation controlled by an optional oscillating ceiling fan (Figure 3B) in October 2020 is represented in Figure 7 together with temperature and VPD of indoor and outdoor air. The large variability of hourly mean 220Rn concentrations suggests dramatic changes in air flow and air residence time between the white board and the mud wall in the single-room mud house (Figures 3B–D). No discernible correlation with temperature or VPD is apparent over the 9 days of measurements when the moisture content of the thick mud wall was essentially constant. Air turbulence introduced in the first part of the time-series by the oscillatory fan limited the thoron concentration in air that was admitted to the Thoron Scout by the computer fan blowing air from behind the white wall into the perforated polyethylene enclosure. Turning the ceiling fan off during the fifth day of measurements almost doubled the average thoron concentration in the polyethylene enclosure from 202 to 382 Bq m–3 (Figure 7).
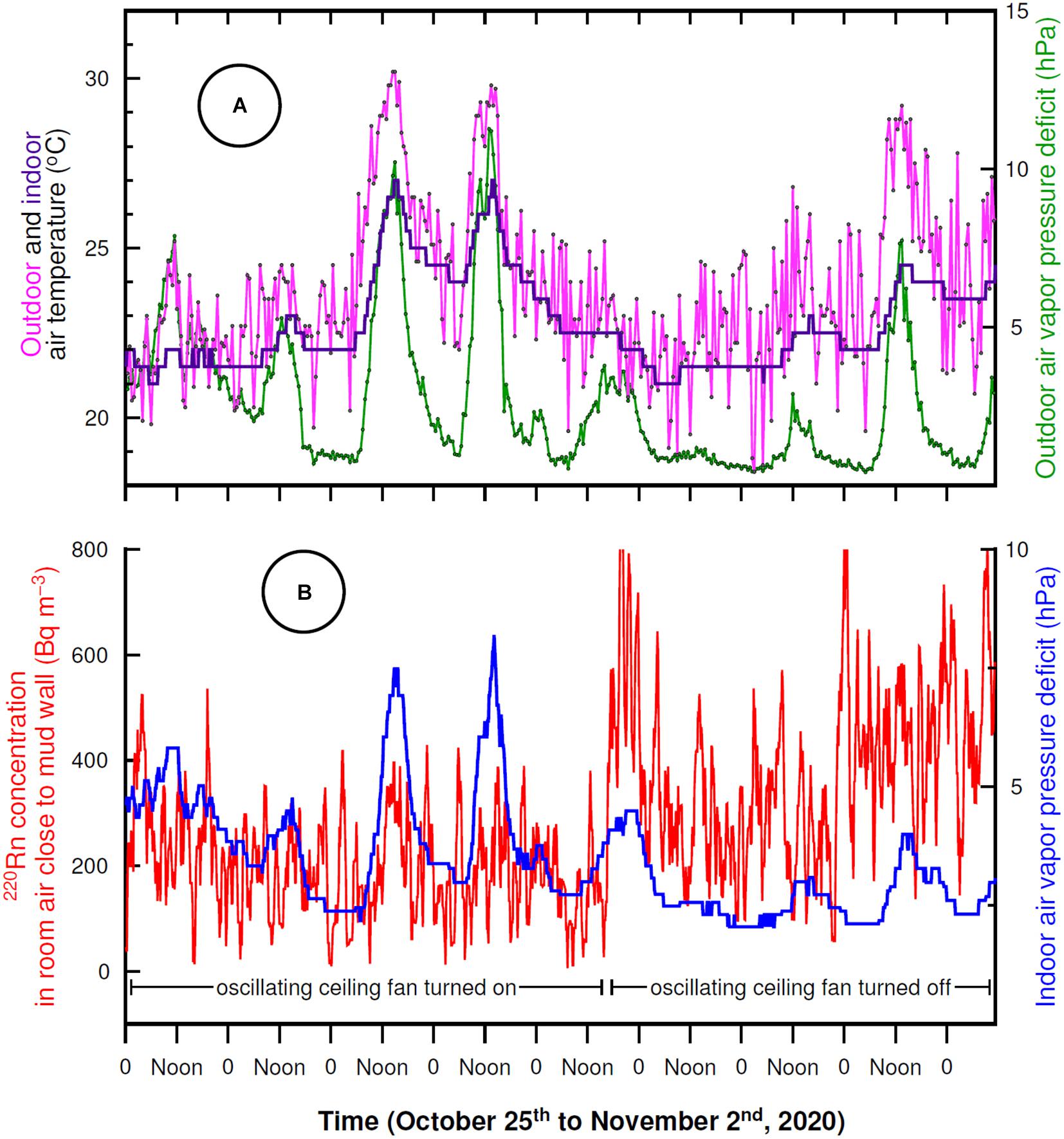
Figure 7. Time-series from a mud house near Hà Nội over 9 days documenting hourly moving average values of (A) outdoor and indoor air temperatures, water vapor pressure deficit (VPD) in outdoor air and (B) indoor VPD, and 220Rn concentrations in room air near the surface of a mud wall (Figure 3) based on data recorded in 10-min intervals by a SARAD® Thoron Scout in October 2020.
Discussion
Sources of Radioactivity in Terra Rossa
Radioactive nuclides like 238U of uranium, 226Ra of radium, 232Th of thorium, and 40K of potassium occur naturally in bedrock and soils, as well as in mineral-containing construction materials. While 222Rn is a product from the decay of 238U via 226Ra, 220Rn (thoron) is produced along the 232Th decay chain. The measured concentrations for 226Ra (mean value of 52 Bq kg–1) and 232Th (mean value of 68.5 Bq kg–1) in terra rossa samples are above their corresponding worldwide average concentrations in natural soils (i.e., 35 Bq kg–1 for 226Ra and 30 Bq kg–1 for 232Th), whereas the concentrations of 40K in terra rossa samples fall below the worldwide average concentration of 400 Bq kg–1 for 40K in soils (United Nations Scientific Committee on the Effects of Atomic Radiation (UNSCEAR), 1982, 2000). Elevated activities of 226Ra and 232Th in terra rossa foster the exhalation of radon isotopes from exposed mud surfaces into indoor air.
The radiological hazard from 226Ra, 232Th, and 40K activities in terra rossa was calculated via the radium equivalent activity, Raeq (OECD Nuclear Energy Agency (NEA-OECD), 1979; United Nations Scientific Committee on the Effects of Atomic Radiation (UNSCEAR), 1982, 2000). The calculated average radium equivalent activity of the two terra rossa samples DYM and SYM of 169.9 (±3.1) Bq kg–1 is lower than the criterion limit of 370 Bq kg–1 (OECD Nuclear Energy Agency (NEA-OECD), 1979; Ravisankar et al., 2014), which makes an annual dose of 1.5 mGy equal to the maximum permissible annual effective dose of 1 mSv for the general public as recommended by the International Commission on Radiological Protection (ICRP) (1991).
With regard to Raeq alone, terra rossa would be a safe building material for mud houses in the studied area. However, it would be misleading to only take γ-radiation into account and ignore α-radiation. Thoron is an intermittent nuclide along the thorium decay chain, which originates from primordial 232Th. Thoron’s transport distance in indoor air is limited owing to the short half-life of 55.6 s. As a consequence, thoron’s spatial distribution in room air is inhomogeneous (Meisenberg and Tschiersch, 2011). Building materials are recognized as one of the main sources of radon isotopes in indoor air. The concentration of thoron in indoor air declines with increasing distance from mud walls and floors toward the center of the room. High concentrations of α-emitting thoron and progenies in indoor air of mud houses in the center of rooms (>100 Bq m–3) and especially near bare mud walls (>350 Bq m–3) are responsible for > 80 % of the average effective dosage affecting inhabitants (Nguyễn-Thùy et al., 2019; Nguyễn-Văn et al., 2020). Severely elevated concentrations of thoron up to 1500 Bq m–3 close to bare mud walls of traditional dwellings were also found in China (Shang et al., 2008) and in an experimental room constructed with unfired mud bricks in a laboratory at the Helmholtz Zentrum in Munich, Germany (Meisenberg and Tschiersch, 2011), although in both cases 232Th in the mud building material only produced γ-radiation with an average activity concentration of 40–50 Bq kg–1. Thoron concentrations in room air adjacent to surfaces of unfired mud are directly linked to the content of 232Th in the building material. Thus, the combined α- and γ-radiation geohazards of the studied terra rossa call into question the safety of unfired terra rossa as a building material.
Influence of Terra Rossa Bulk Density
The bulk density of experimental mud bricks was primarily influenced by the inclusion of air bubbles when pressing wet terra rossa into a mold to shape bricks, and later decreased via drying as water in pore spaces was replaced by air (Table 1). During the first stage of measurements, when the mud bricks were still relatively wet with ≥ 20 wt. % water, the high bulk density from 1.5 to 1.9 g cm–3 primarily meant fewer air-filled bubbles and partially water-filled pores in the bricks. Scarcity of air-filled passages to outside air slowed the diffusion and suppressed the exhalation of 220Rn. High bulk density in the presence of liquid water decreased the exhalation rate of 220Rn because radon atoms recoiling out of the mineral grains were predominantly retained in water-filled pore space (Rogers and Nielson, 1991; Faheem and Matiullah, 2008) (Figure 5B).
Shrinkage of drying mud bricks caused the formation of open cracks that likely connected internal voids in the bricks to outside air. During the second stage of measurements with reduced moisture content, the strongly turbulent air in the chamber also forced convection and air flow into macroscopic cracks, and thus assisted in the exhalation of 220Rn (Figure 5B). During the third stage of measurements with low moisture contents of < 5 wt. %, the bulk density had strongly decreased in comparison with the first and second stages (Table 1). The decreased moisture content in mud bricks filled pore spaces and cracks almost completely with air and provided diffusion pathways for radon (Faheem and Matiullah, 2008), but fewer recoiling 220Rn atoms slowed down enough to remain in open pore space and become available for exhalation.
The Role of Residual Moisture for Thoron Exhalation From Terra Rossa
Our results from progressive drying of mud bricks across three stages of measurements emphasize the importance of moisture content (Figure 5A and Table 1) and indicate highest 220Rn exhalation rates of up to 12.27 kBq m–2 h–1 with a mean of 10.01 (±1.16) kBq m–2 h–1 during the second stage at an intermediate moisture content of 9.8 (±2.5) wt. %. The observed residual moisture content in mud bricks during the second stage falls into the range of typical values of 8–13 wt. % moisture in soil that was reported to maximize radon exhalation (Hosoda et al., 2007; Faheem and Matiullah, 2008; Yang et al., 2019).
The principle of maximized exhalation of 220Rn from mud surfaces with an intermediate moisture content of between 5 and 10 wt. % was confirmed by time-series of measurements in the room of a research mud house near Hà Nội (Figure 6) where the mean moisture content on the inside of the ∼60-cm thick wall of the mud house was still 4.2 (±1.1) wt. % almost 3 years after construction, at the tail end of the summer monsoon season in mid-October of 2020. Laboratory experiments with fragments of terra rossa samples from the interior wall of the mud house indicated a weight gain commensurate with a moisture content between ∼4 and ∼9 wt. % upon prolonged exposure to air with high humidity (Figure 4). A dry interior wall or mud floor of a mud house will adsorb moisture from the air until it reaches a dynamic equilibrium between adsorption and drying. The ∼60 cm thick walls of traditional mud houses can store a substantial amount of moisture that acts as a reservoir and not only prevents rapid drying over hours to days, but also buffers against fast accumulation of adsorbed moisture close to interior mud surfaces on days when the relative humidity in air is high and the water VPD is low. In effect, diurnal and short-term weather changes cannot rapidly affect the thoron exhalation from interior mud surfaces (Figure 7). However, longer-term to seasonal changes in VPD significantly influence the residual moisture in terra rossa and the thoron exhalation.
Practical Considerations for Mud House Inhabitants
The moisture loading in northern Việt Nam’s (sub)tropical ambient air is highly variable throughout the year, which is mostly due to the large temperature difference between summer and winter (Figure 6). The temperature range across seasons at higher altitude in Hà Giang Province is even larger than in Hà Nội. Low temperatures in mountainous Hà Giang Province combined with low clouds, fog, and high relative humidity in winter decrease VPD in air, increase adsorption of moisture into exposed mud to possibly reach ∼10 wt. %, and temporarily increase the exhalation of radon isotopes from mud into indoor air of mud houses. Occupied mud houses traditionally use open wood fires for cooking and have no chimney. Over time, ash from cooking fires, fingerprints, animal droppings, and aerosols may deposit hygroscopic salts on interior mud surfaces increasing the potential for adsorption of moisture and enhanced 220Rn exhalation.
Paradoxically, although the moisture loading in air is higher during the warm summer monsoon months, the higher summer temperatures greatly increase VPD, which dries mud surfaces inside of mud houses and limits the exhalation of 220Rn from mud into room air (Figure 6). The observed high concentrations of 220Rn in the mud house in June can be explained by residual moisture that derived from the compaction of wet terra rossa earlier in November 2017 (Figure 6) when the house was newly constructed.
Forced turbulence of room air in a mud house by an oscillating ceiling fan reduced the residence time of 210Rn-laden air close to mud surfaces, mixed the air from near walls and floors with overall room air on the order of seconds, and thus effectively diluted the 210Rn near mud surfaces (Figure 7). Without forced turbulence, the air flow close to mud surfaces tends to be laminar and better retains exhaled 220Rn near mud surfaces. The large short-term variability of 210Rn concentrations near a mud wall that was observed regardless of forced turbulence may be due to the convective flow of room air as influenced by meteorological parameters, such as temperature gradients between mud surfaces and outdoor and/or indoor air, as well as wind forcing air through cracks into the room. Forced turbulence of room air by electrical fans is not useful for remediation of the thoron geohazard in room air because (i) it merely dilutes thoron in air near mud surfaces with overall room air and (ii) it does not diminish the health hazard from inhaling metallic radioactive progeny of radon isotopes from room air. Exhalation of radon isotopes from porous earthen substrates can only be stopped by impermeable surface treatments that need to be economically and socially acceptable. Additional research is needed for different types of earthen dwellings in different climates to devise suitable mitigation strategies.
Conclusion
Thoron exhalation rates from terra rossa bricks with different densities and moisture contents in conjunction with concentrations of prominent γ-emitting radionuclides call into question the radiological safety of terra rossa and possibly other unfired earthen materials for uses as building material. The average 226Ra and 232Th activities of the studied terra rossa samples are 52.0 and 68.5 Bq kg–1, respectively, which are above the average values of 35 Bq kg–1 for 226Ra and 30 Bq kg–1 for 232Th in average soil around the world (United Nations Scientific Committee on the Effects of Atomic Radiation (UNSCEAR), 1982, 2000). The relatively high activities of 226Ra and 232Th radionuclides in terra rossa explain the significant exhalation of radon from terra rossa and the associated α-radiation health hazard in indoor air when terra rossa is used for the construction of earthen dwellings. Exhalation of radon isotopes from interior mud walls and mud floors into room air of mud houses is controlled by multiple characteristics of earthen materials and meteorological parameters, i.e., the mineralogical composition of mud, residual moisture, porosity, permeability, temperature, and water VPD in room air. Especially the moisture content of terra rossa mud is strongly affecting 220Rn exhalation rates. Starting from a low exhalation rate in waterlogged terra rossa from northern Việt Nam, subsequent drying gradually increases the 220Rn exhalation rate until peaking at ∼10 kBq m–2h–1 at an intermediate moisture content of ∼5–10 wt. %, followed by a decline of the exhalation rate toward dryer mud surfaces. Lowered density and associated macroporosity as a result of cracks in drying bricks effectively add surface area for exhalation and increase the thoron exhalation rate. Seasonal variations of temperature and moisture content in ambient air influence the VPD of air and the potential of mud surfaces to adsorb/desorb moisture. Adsorption of moisture into interior mud walls and floors from air at lower temperature and higher relative humidity can temporarily increase the thoron exhalation rate. The present knowledge of the physical and meteorological parameters affecting the exposure of inhabitants of earthen buildings in northern Việt Nam to radiation makes it unlikely that mud houses can be structurally modified to eliminate the radiation geohazard. Our ongoing efforts focus on affordable treatment of mud surfaces to at least limit the transport of exhaled 220Rn from mud walls into room air.
Data Availability Statement
The raw data supporting the conclusions of this article will be made available by the authors, without undue reservation.
Author Contributions
NN: investigation, design of the study, data curation, and writing. DN-T and HN-V: conceptualization, investigation, design of the study, data curation, writing, review, and editing. NN-H: data curation and writing. AS: conceptualization, investigation, design of the study, writing, review, and editing. All authors contributed to the article and approved the submitted version.
Conflict of Interest
The authors declare that the research was conducted in the absence of any commercial or financial relationships that could be construed as a potential conflict of interest.
Funding
This work was funded by Vingroup Joint Stock Company and supported by the Domestic Master/Ph.D. Scholarship Program of Vingroup Innovation Foundation (VINIF), Vingroup Big Data Institute (VINBIGDATA) grant number VINIF.2020.TS.64 (NN). This contribution received support from the Indiana University Open Access Article Publishing Fund. Measurements with the SARAD® RTM 2200 in Vietnam were made possible by the U.S. Department of Energy, Office of Science, Office of Basic Energy Sciences, Chemical Sciences, Geosciences, and Biosciences Division under Award Number DE-SC0006978.
Acknowledgments
We are grateful to M. S. Melgunov, Director of the Analytical Center for Multi-Elemental and Isotope Research SB RAS, for analyzing the natural γ-radiation of radionuclides in terra rossa. Nùng Văn Minh collected terra rossa samples. Jian Liu from Indiana University contributed images from field work. Minh Ngọc Schimmelmann served as a cultural liaison and facilitated logistics of research. We thank the reviewers for their constructive comments and suggestions.
Footnotes
References
Balek, V., and Beckman, I. N. (2005). Theory of emanation thermal analysis XII. Modelling of radon diffusion release from disordered solids on heating. J. Therm. Anal. Calorim. 82, 755–759. doi: 10.1007/s10973-005-0960-7
Bavarnegin, E., Fathabadi, N., Moghaddam, M. V., Farahani, M. V., Moradi, M., and Babakhni, A. (2013). Radon exhalation rate and natural radionuclide content in building materials of high background areas of Ramsar, Iran. J. Environ. Radioact. 117, 36–40. doi: 10.1016/j.jenvrad.2011.12.022
Cevik, U., Kara, A., Celik, N., Karabidak, M., and Celik, A. (2011). Radon survey and exposure assessment in Karaca and Çal Caves, Turkey. Water Air Soil Pollut. 214, 461–469. doi: 10.1007/s11270-010-0437-6
Chen, J., Rahman, N. M., and Atiya, I. A. (2010). Radon exhalation from building materials for decorative use. J. Environ. Radioact. 101, 317–322. doi: 10.1016/j.jenvrad.2010.01.005
Faheem, M., and Matiullah, M. (2008). Radon exhalation and its dependence on moisture content from samples of soil and building materials. Radiat. Meas. 43, 1458–1462. doi: 10.1016/j.radmeas.2008.02.023
Garcìa-Gaines, R. A., and Frankenstein, S. (2015). USCS and the USDA Soil Classification System: Development of a Mapping Scheme. Vicksburg, MS: U.S. Army Engineer Research and Development Center, Cold Regions Research and Engineering Laboratory.
Gavshin, V. M., Melgunov, M. S., Sukhorukov, F. V., Bobrov, V. A., Kalugin, I. A., and Klerkx, J. (2005). Disequilibrium between uranium and its progeny in the lake Issyk-Kul system (Kyrgyzstan) under a combined effect of natural and manmade processes. J. Environ. Radioact. 83, 61–74. doi: 10.1016/j.jenvrad.2005.02.012
Govindaraju, K. (1994). Compilation of working values and sample description for 383 geostandards. Geostand. Newslett. 18, 1–158. doi: 10.1046/j.1365-2494.1998.53202081.x-i1
Hassan, N. M., Hosoda, M., Ishikawa, T., Sorimachi, A., Sahoo, S. K., Tokonami, S., et al. (2009). Radon migration process and its influence factors; review. Jpn. J. Health Phys. 44, 218–231. doi: 10.5453/jhps.44.218
Hassan, N. M., Ishikawa, T., Hosoda, M., Iwaoka, K., Sorimachi, A., Sahoo, S. K., et al. (2011). The effect of water content on the radon emanation coefficient for some building materials used in Japan. Radiat. Meas. 46, 232–237. doi: 10.1016/j.radmeas.2010.11.006
Hosoda, M., Shimo, M., Sugino, M., Furukawa, M., and Fukushi, M. (2007). Effect of soil moisture content on radon and thoron exhalation. J. Nucl. Sci. Technol. 44, 664–672. doi: 10.1080/18811248.2007.9711855
International Atomic Energy Agency (IAEA) (2013). Measurement and Calculation of Radon Releases from NORM Residues. Technical Reports Series No. 474. Vienna: International Atomic Energy Agency (IAEA).
International Commission on Radiological Protection (ICRP) (1991). 1990 Recommendations of the International Commission on Radiological Protection, Vol. 21. Stockholm: International Commission on Radiological Protection.
Kanse, S. D., Sahoo, B. K., Sapra, B. K., Gawarea, J. J., and Mayya, Y. S. (2013). Powder sandwich technique: a novel method for determining the thoron emanation potential of powders bearing high 224Ra content. Radiat. Meas. 48, 82–87. doi: 10.1016/j.radmeas.2012.10.014
Meisenberg, O., Mishra, R., Joshi, M., Gierl, S., Rout, R., Guo, L., et al. (2017). Radon and thoron inhalation doses in dwellings with earthen architecture: comparison of measurement methods. Sci. Total Environ. 579, 1855–1862. doi: 10.1016/j.scitotenv.2016.11.170
Meisenberg, O., and Tschiersch, J. (2010). Specific properties of a model of thoron and its decay products in indoor atmospheres. Nukleonika 55, 463–469.
Meisenberg, O., and Tschiersch, J. (2011). Thoron in indoor air: modeling for a better exposure estimate. Indoor Air 21, 240–252. doi: 10.1111/j.1600-0668.2010.00697.x
Melgunov, M. S., Gavshin, V. M., Sukhorukov, F. V., Kalugin, I. A., Bobrov, V. A., and Klerkx, J. (2003). Anomalies of radioactivity on the southern bank of the Ysyk-Köl Lake (Kyrgyzstan). Chem. Sustain. Dev. 11, 859–870.
Mikutta, R., Kleber, M., Kaiser, K., and Jahn, R. (2005). Review: organic matter removal from soils using hydrogen peroxide, sodium hypochlorite, and disodium peroxodisulfate. Soil Sci. Soc. Am. J. 69, 120–135. doi: 10.2136/sssaj2005.0120
Monteith, J., and Unsworth, M. (2013). “Chapter 17 – Micrometeorology: (ii) interpretation of flux measurements,” in Principles of Environmental Physics, eds J. Monteith and M. Unsworth (Amsterdam: Academic Press), 321–349. doi: 10.1016/B978-0-12-386910-4.00017-2
Nazaroff, W. W., and Nero, A. V. J. (eds) (1988). “Radon and its decay products in indoor air,” in Environmental Science and Technology: A Wiley-Interscience Series of Texts and Monographs, (New York, NY: John Wiley and Sons, Inc). Available online at: https://www.amazon.com/Products-Indoor-Environmental-Science-Technology/dp/0471628107
Nguyễn-Thùy, D., Nguyễn-Văn, H., Schimmelmann, J. P., Nguyễn, T. A. N., Doiron, K., and Schimmelmann, A. (2019). 220Rn (thoron) geohazard in room air of earthen dwellings in Vietnam. Geofluids 4, 1–11. doi: 10.1155/2019/7202616
Nguyễn-Văn, H., Nguyễn-Thùy, D., Nguyễn, N. T. A., Streil, T., Schimmelmann, J., Doiron, K., et al. (2020). Excessive radon-based radiation in indoor air caused by soil building materials in traditional homes on Ðồng Văn karst plateau, northern Vietnam. Chemosphere 257:127119. doi: 10.1016/j.chemosphere.2020.127119
OECD Nuclear Energy Agency (NEA-OECD) (1979). Exposure to Radiation from Natural Radioactivity in Building Materials. Paris: OECD.
Ravisankar, R., Vanasundari, K., Suganya, M., Raghu, Y., Rajalakshmi, A., Chandrasekaran, A., et al. (2014). Multivariate statistical analysis of radiological data of building materials used in Tiruvannamalai, Tamilnadu, India. Appl. Radiat. Isot. 85, 114–127. doi: 10.1016/j.apradiso.2013.12.005
Rogers, V. C., and Nielson, K. K. (1991). Multiphase radon generation and transport in porous materials. Health Phys. 60, 807–815. doi: 10.1097/00004032-199106000-00006
Sahoo, B. K., Nathwani, D., Eappen, K. P., Ramachandran, T. V., Gaware, J. J., and Mayya, Y. S. (2007). Estimation of radon emanation factor in Indian building materials. Radiat. Meas. 42, 1422–1425. doi: 10.1016/j.radmeas.2007.04.002
Sakoda, A., Ishimori, Y., Hanamoto, K., Kataoka, T., Kawabe, A., and Yamaoka, K. (2010). Experimental and modeling studies of grain size and moisture content effects on radon emanation. Radiat. Meas. 45, 204–210. doi: 10.1016/j.radmeas.2010.01.010
Sakoda, A., Ishimori, Y., and Yamaoka, K. (2011). A comprehensive review of radon emanation measurements for mineral, rock, soil, mill tailing and fly ash. Appl. Radiat. Isot. 69, 1422–1435. doi: 10.1016/j.apradiso.2011.06.009
Samuelsson, C., and Petterson, H. (1984). Exhalation of 222Rn from porous materials. Radiat. Prot. Dosim. 7, 95–100. doi: 10.1093/oxfordjournals.rpd.a082971
Schimmelmann, A., Fernandez Cortes, A., Cuezva, S., Streil, T., and Lennon, J. T. (2018). Radiolysis via radioactivity is not responsible for rapid methane oxidation in subterranean air. PLoS One 13:e0206506. doi: 10.1371/journal.pone.0206506
Shang, B., Tschiersch, J., Cui, H., and Xia, Y. (2008). Radon survey in dwellings of Gansu, China: the influence of thoron and an attempt for correction. Radiat. Environ. Biophys. 47, 367–373. doi: 10.1007/s00411-008-0163-2
Steinhäusler, F. (1996). Environmental 220Rn: a review. Environ. Int. 22, 1111–1123. doi: 10.1016/S0160-4120(96)00227-9
Trevisi, R., Nuccetelli, C., and Risica, S. (2013). Screening tools to limit the use of building materials with enhanced/elevated levels of natural radioactivity: analysis and application of index criteria. Constr. Build Mater. 49, 448–454. doi: 10.1016/j.conbuildmat.2013.08.059
Tuccimei, P., Moroni, M., and Norcia, D. (2006). Simultaneous determination of 222Rn and 220Rn exhalation rates from building materials used in Central Italy with accumulation chambers and a continuous solid state alpha detector: influence of particle size, humidity and precursors concentration. Appl. Radiat. Isot. 64, 254–263. doi: 10.1016/j.apradiso.2005.07.016
Tufail, M. (2012). Radium equivalent activity in the light of UNSCEAR report. Environ. Monit. Assess. 184, 5663–5667. doi: 10.1007/s10661-011-2370-6
Ujić, P., Čeliković, I., Kandić, A., Vukanac, I., Ðurašević, M., Dragosavac, D., et al. (2010). Internal exposure from building materials exhaling 222Rn and 220Rn as compared to external exposure due to their natural radioactivity content. Appl. Radiat. Isot. 68, 201–206. doi: 10.1016/j.apradiso.2009.10.003
Ujić, P., Čeliković, I., Kandić, A., and Žunić, Z. (2008). Standardization and difficulties of the thoron exhalation rate measurements using an accumulation chamber. Radiat. Meas. 43, 1396–1401. doi: 10.1016/j.radmeas.2008.03.003
United Nations Scientific Committee on the Effects of Atomic Radiation (UNSCEAR) (1982). Ionizing Radiation: Sources and Biological Effects. New York, NY: United Nations.
United Nations Scientific Committee on the Effects of Atomic Radiation (UNSCEAR) (2000). Sources, Effects and Risks of Ionizing Radiation. New York, NY: United Nations.
Urosević, V., Nikezić, D., and Vulović, S. (2008). A theoretical approach to indoor radon and thoron distribution. J. Environ. Radioact. 99, 1829–1833. doi: 10.1016/j.jenvrad.2008.07.010
Weiss, A. (1977). Algorithms for the calculation of moist air properties on hands calculator. Trans. ASAE 20, 1133–1136. doi: 10.13031/2013.35716
World Health Organization (WHO) (2009). WHO Handbook on Indoor Radon: A Public Health Perspective. Geneva: WHO.
Keywords: earthen dwelling, inhalation hazard, radioactivity, mud house, radon isotope, room air, soil brick, thoron
Citation: Nguyễn NTA, Nguyễn-Thùy D, Nguyễn-Văn H, Nguyễn-Hải N and Schimmelmann A (2021) Radioactive Thoron 220Rn Exhalation From Unfired Mud Building Material Into Room Air of Earthen Dwellings. Front. Earth Sci. 9:629241. doi: 10.3389/feart.2021.629241
Received: 13 November 2020; Accepted: 16 March 2021;
Published: 30 April 2021.
Edited by:
Francesco Italiano, National Institute of Geophysics and Volcanology, Section of Palermo, ItalyReviewed by:
Rakesh C. Ramola, Hemwati Nandan Bahuguna Garhwal University, IndiaGiovanni Martinelli, National Institute of Geophysics and Volcanology, Section of Palermo, Italy
Copyright © 2021 Nguyễn, Nguyễn-Thùy, Nguyễn-Văn, Nguyễn-Hải and Schimmelmann. This is an open-access article distributed under the terms of the Creative Commons Attribution License (CC BY). The use, distribution or reproduction in other forums is permitted, provided the original author(s) and the copyright owner(s) are credited and that the original publication in this journal is cited, in accordance with accepted academic practice. No use, distribution or reproduction is permitted which does not comply with these terms.
*Correspondence: Hu’ó’ng Nguyễn-Vãn, aHVvbmd0ZWN0b25pY3NAdm51LmVkdS52bg==
†ORCID: Nguyệt Thị Ánh Nguyễn, orcid.org/0000-0002-3627-1692; Du’o’ng Nguyễn-Thùy, orcid.org/0000-0001-8899-6233; Hu’ó’ng Nguyễn-Văn, orcid.org/0000-0003-1523-5921; Nam Nguyễn-Hải, orcid.org/0000-0002-1705-0106; Arndt Schimmelmann, orcid.org/0000-0003-4648-5253