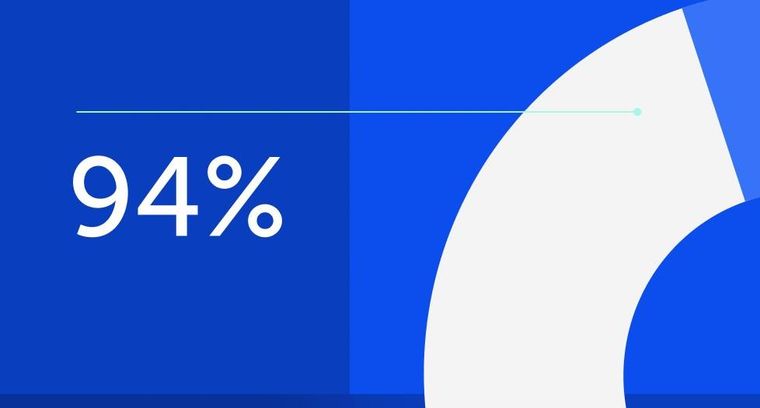
94% of researchers rate our articles as excellent or good
Learn more about the work of our research integrity team to safeguard the quality of each article we publish.
Find out more
ORIGINAL RESEARCH article
Front. Earth Sci., 13 April 2021
Sec. Paleontology
Volume 9 - 2021 | https://doi.org/10.3389/feart.2021.617124
This article is part of the Research TopicEarly Avian EvolutionView all 14 articles
As key components of the tetrapod pectoral girdle, the scapula and coracoid have played a significant role in the evolution of forelimb locomotion among terrestrial vertebrates. The transition from a rigid fused scapulocoracoid in ancestral non-avian theropods to a presumably more flexible separated scapula-coracoid in early birds is considered to be one of the key morphological transitions related to the rapid refinement of flight. In most Mesozoic birds (e.g., Enantiornithes and Ornithuromorpha) and crown birds the scapula and coracoid are separate (unfused), with few exceptions (e.g., flightless paleognaths). In contrast, in Confuciusornis, a basal pygostylian from the Early Cretaceous Jehol Biota known from thousands of specimens, the scapula and coracoid remain plesiomorphically fused. This raises questions regarding the influence of shoulder girdle architecture on the early evolution and refinement of avian flight. The paravian scapula-coracoid joint has never previously been investigated using histology, and thus joint morphology has only been inferred superficially. In order to better understand the evolution of this joint in Mesozoic birds, we make the first histological study of the scapulocoracoid glenoid joint in Confuciusornis. The results demonstrate that the scapula and coracoid both consist of cancellous and compact bone, with both fibrolamellar and parallel-fibered structure. A thin layer of calcified cartilage is present on the glenoid fossa surface, representing remnants of the articular surface for the humerus. Both histology and computed tomography reveal that the scapulocoracoid of Confuciusornis is fully fused, forming a synostosis. Humeral histology suggests the studied individual was nearing completion of its first year of growth, suggesting the Confuciusornis scapulocoracoid fused before skeletal maturity was achieved, as in flightless paleognaths, whereas in the plesiomorphic condition fusion occurs late in ontogeny. We hypothesize the fused scapulocoracoid of Confuciusornis is secondarily evolved and suggest the primary factor responsible for this morphology may have been a decrease in mechanical stimulation at the glenoid of Confuciusornis relative to other volant birds, linked to the unique flight style of this taxon. Further investigation into the histology of the glenoid joint in other Mesozoic paravians and extant birds will help to clarify the morphological transition of the scapula-coracoid joint in early avian evolution.
Structural modifications of the scapula and coracoid during the early evolution of Aves are often described as being among the key morphological changes in the early evolution of birds (Chatterjee, 1997; Chiappe and Witmer, 2002; O’Connor et al., 2011). In Archaeopteryx the scapula and coracoid are generally regarded as synostosed elements that form a single immobile unit called the scapulocoracoid, a morphology inherited from non-avian dinosaurs (Chiappe and Witmer, 2002). However, these two bones became distinct at an early stage of avian evolution, being already separate in the long boney tailed bird Jeholornis, a taxon commonly resolved in cladistic analyses as only more derived than Archaeopteryx (Figure 1; Zhou and Zhang, 2002a; O’Connor and Zhou, 2019). The scapula and coracoid to form the glenoid surface for the articulation of the humerus in all tetrapods (Benton, 2014). These elements are key components of the pectoral girdle and have played an important role during the evolution of locomotion in terrestrial vertebrates (Benton, 2014). Therefore, it follows that the change early in avian evolution from fusion between the scapula and coracoid to complete separation of these elements most likely represents a flight adaptation, presumably facilitating greater mobility and more advanced forms of powered flight.
Figure 1. A simplified cladogram of Paraves indicating the condition of the joint state between the scapula and coracoid, along with ontogenetic stage. Modified from O’Connor and Zhou (2019).
However, as new specimens of Archaeopteryx have recently become available, this dichotomy between fused and separate has been shaken, as in most specimens the scapula and coracoid appear to be only sutured [e.g., the Eichstätt specimen (the smallest), Solnhofen specimen (the largest), Munich specimen (Elzanowski, 2001), Daiting specimen (Kundrát et al., 2019), Thermopolis specimen (Mayr et al., 2005), and 12th specimen (Rauhut et al., 2018)]. Synchrotron radiation based computed tomography (CT) scans of the Daiting Archaeopteryx, a relatively small specimen, revealed that the scapula and coracoid were closely connected but not fully fused, and the two bones were interpreted as forming a sutural contact (Kundrát et al., 2019). The scapulocoracoid is described as fused (or apparently co-ossified) in the London, Berlin, and Maxberg specimens (Ostrom, 1976), suggesting complete fusion occurred late in the ontogeny of Archaeopteryx (Chiappe and Witmer, 2002). Although the specific joint morphology apparently changes with ontogeny, these two bones are firmly articulated and immobile in Archaeopteryx, forming a scapulocoracoid complex (Chiappe and Witmer, 2002).
Paraves is the clade of maniraptoran theropods that includes Aves and its closest relatives, the Troodontidae and Dromaeosauridae (Brusatte et al., 2014). In these non-avian paravian clades the morphology of the scapulocoracoid joint appears similar to that observed in Archaeopteryx, in which fusion of the scapulocoracoid unit is only found in adult individuals. In the probable troodontid Anchiornis, sub-adult specimens (e.g., BMNHC PH822 and IVPP V14378) have an unfused scapula and coracoid (Xu et al., 2009; Pei et al., 2017), whereas these elements are apparently fused in other, presumably adult specimens (LPM-B00169) (Hu et al., 2009). The only known specimen of the probable troodontid Xiaotingia (STM 27-2), a presumed adult, has a fused scapulocoracoid (Xu et al., 2011) and this condition is also reported in the troodontid Jinfengopteryx (CAGS-IG-04-0801) (Ji et al., 2005).
Among the Dromaeosauridae, subadult to adult specimens of Deinonychus (MOR 1178) (Parsons and Parsons, 2009), Velociraptor (IGM 100/986; Parsons and Parsons, 2009), and Microraptor (CAGS 20-7-004 and CAGS 20-8-001) (Hwang et al., 2002) reportedly possess fused scapulocoracoids, whereas the two elements are only sutured in obvious juveniles (Deinonychus MCZ 8791; Parsons and Parsons, 2015). However, the scapula and coracoid remain connected by a suture in putatively adult specimens of some taxa, including in Buitreraptor (MPCA-245) (Makovicky et al., 2005) and Sinornithosaurus (IVPP V12811) (Xu et al., 1999). This may suggest that some specimens identified as adults, such as Deinonychus (YPM 5236; Parsons and Parsons, 2015), are in fact not fully mature, or that the scapula and coracoid did not become fused in all lineages of dromaeosaurids. It is impossible to differentiate between these hypotheses without relevant histological or CT data, combined with the careful use of suture patterns to assess maturity in these specimens (Bailleul et al., 2016).
Among basal (non-ornithothoracine) lineages of Mesozoic birds, the scapula and coracoid are completely separate in the holotype of Jeholornis (Zhou and Zhang, 2002a, b), but in some specimens these bones are preserved firmly articulated (e.g., STM2-19) (O’Connor et al., 2018b) or appear sutured (YFGP-yb2) (Lefèvre et al., 2014). Similarly, in Sapeornis (Figure 2) the two elements are tightly joined in the holotype IVPP V12698 (Zhou and Zhang, 2002b, 2003a), but are separate in the subadult specimens DNHMD3078 (Gao et al., 2012), IVPP V13396 (Provini et al., 2008), and HGM-41HIII0405 (Pu et al., 2013).
Figure 2. Scapulae and coracoids of basal avialans. (A) Archaeopteryx Munich specimen; (B) Jeholornis IVPP V13886 (unpublished specimen); (C) Sapeornis IVPP V13698 (holotype); (D) Confuciusornis IVPP V14385; (E) Chongmingia STM9-9; (F) Jinguofortis IVPP V24194. Scale bar 1 cm.
Confuciusornis is the most common bird in the Jehol Biota, with thousands of specimens reported, mostly from the Yixian Formation (Wang et al., 2019c). Specimens range considerably in size although no clear juveniles are known (humerus length ranging from 41.01 to 78.5 mm; Chiappe et al., 1999, 2008; Zhang et al., 2009; Wang and O’Connor, 2017; Wang et al., 2019c). In all reported specimens of the basal pygostylian Confuciusornis the scapulocoracoid is described as fused (Hou, 1997; Chiappe et al., 1999; Ji et al., 1999; Hou et al., 2002; Dalsätt et al., 2006; Zhang et al., 2009; Wang and Zhou, 2018; Wang et al., 2019c), in contrast to the condition in other non-ornithothoracine avians. In the subadult holotype of Eoconfuciusornis zhengi IVPP V11977, the oldest and basal-most member of Confuciusornithidae from the Huajiying Formation, the scapula and coracoid are reportedly only sutured (Zhang et al., 2008a). However, in BMNHC-PH870, an osteologically mature specimen also from the Huajiying Formation, the scapula and coracoid are reportedly fused (Navalón et al., 2017). This and other potentially ontogenetic differences make it impossible to determine if BMNHC-PH870 is referable to Eoconfuciusornis or if it represents another currently unnamed taxon (Navalón et al., 2017).
Despite the enormous number of specimens of Confuciusornis, the fact that none reveal an earlier stage in which the scapula and coracoid are sutured or separate appears to represent a distinct departure from other early paravians, in which these elements are only fused in mature, adult specimens. In contrast to other non-ornithuromorpha paravians, which grew so slowly that they required several years to reach skeletal maturity (Erickson et al., 2009; Zheng et al., 2014; Prondvai et al., 2018; Shen et al., 2019), Confuciusornis had elevated growth rates, reaching near-adult size in the first year of uninterrupted growth (de Ricqlès et al., 2003) after which they continue to grow more slowly for several years forming an outer circumferential layer (OCL) marked by several lines of arrested growth (LAGs) (Chinsamy et al., 2019). This developmental strategy may partly explain the fact the scapulocoracoid is reportedly fused in all known specimens of Confuciusornis, a morphology which is otherwise only found in the most mature specimens of taxa with more protracted growth rates (e.g., Anchiornis, Microraptor, and Archaeopteryx). A rapid growth strategy has previously been cited as an explanation for the fusion of the scapulocoracoid in the Jinguofortisidae, a clade of basal pygostylians consisting of two taxa (Chongmingia and Jinguofortis) each known from a single specimen (Wang et al., 2016, 2018). Histology indicates these two specimens are mature adults (Wang et al., 2016, 2018) making it impossible to determine if the fusion is due to rapid growth, skeletal maturity, or both.
Among neornithines, the scapula and coracoid are typically separate although they are fused into a single scapulocoracoid in some extant flightless taxa including all flightless members of the Paleognathae (the so-called “ratites”), e.g., Struthio camelus, Apteryx australis (McGowan, 1982), and Dromaius novaehollandiae (Maxwell and Larsson, 2007), and some members of the recently extinct flightless clades the Aepyornithidae, Gastrornithidae, and Dinornithiformes (Worthy et al., 2017). Among extant paleognaths, only the volant tinamous (Tinamidae) have separate scapulae and coracoids (Bertelli et al., 2014). The fossil record clearly demonstrates that these two elements were separate in early paleognaths, such as the Paleocene–Middle Eocene Lithornithidae (Torres et al., 2019), indicating that the fused scapulocoracoid of some paleognaths is a derived feature that probably evolved multiple times in this clade along with flightlessness (Faux and Field, 2017). As the wings and sternal carina of Paleocene and Eocene paleognaths were well-developed (Houde, 1986), flightlessness in extant paleognaths is also a derived condition (McNab, 1994; Torres et al., 2019). This suggests that fusion of the scapulocoracoid in neornithines evolved as a consequence of pectoral reduction related to the loss of flight. Notably, in contrast to observations from extinct pennaraptorans, the scapula and coracoid of the ostrich fuse very early on, not via bone but via unmineralized cartilage during embryonic development (day 21) before any extensive ossification has begun (day 22; Maxwell and Larsson, 2009).
Previous studies of neornithines (crown birds) have revealed the developmental trajectory, ossification sequence, regulatory genes, and growth factors of the scapula in embryonic and early post-hatching growth (Williams, 2003; Sawad et al., 2009; Young et al., 2019). Despite the importance of the scapula-coracoid complex in the pectoral girdle of birds (and in fact in all tetrapods) (Benton, 2014), few histological studies have been conducted on pectoral girdle elements (Russell and Joffe, 1985; Wang et al., 2019a), and the histology of the scapula-coracoid joint has never been reported in any paravian. As a result, the histology of the scapulocoracoid joint is poorly understood even in modern birds. The joint between the separate scapula and coracoid has been reported in some birds as a syndesmosis (bound together by the Lig. coracoscapulare interosseum consisting of elastic cartilage), and in others as an elaborate joint (e.g., Branta, Pelecanus), in which the articulation is partially synovial and partially syndesmotic (Baumel and Witmer, 1993). In such a configuration the synovial parts are more mobile than the syndesmoses, as would be expected (Baumel and Witmer, 1993). However, these reports were not accompanied by supporting illustrations.
In extinct organisms descriptions of fusion between elements should be supported with microanatomical evidence, such as histological data, or–at the least–CT data. Surface morphology may suggest a fused joint (i.e., a bony synostosis) even in specimens whose internal anatomy instead reveal the joint to be incompletly fused, retaining either a fibrous suture or some cartilaginous remnants (Bailleul and Horner, 2016). To date, no microanatomical investigation of an apparently fused scapulocoracoid has been conducted on any extinct paravian. Therefore, previous descriptions of purportedly fused scapulocoracoids require re-examination in order to better assess patterns of fusion between these elements. In cases where the two bones are tightly articulated, in particular, it is difficult to determine whether the bones remain separated or if they are actually sutured without investigation of the internal anatomy, as demonstrated in one specimen of Jeholornis in which X-rays revealed the presence of a suture (Lefèvre et al., 2014). Here we take a step in this direction by examining the osteohistology of the scapulocoracoid joint morphology in Confuciusornis. This joint has never been studied through osteohistology in an extinct theropod until now. We compare the histology to that observed in extant birds in which the scapula and coracoid are fused and unfused, and discuss the underlying factors that may affect fusion of pectoral girdle elements in birds.
Institutional abbreviations. BMNHC, Beijing Museum of Natural History, Beijing, China; CAGS, Chinese Academy of Geological Sciences, Beijing, China; DNHM, Dalian Natural History Museum, Dalian, China; GMV, Geological Museum of China, Beijing, China; HGM, Henan Geological Museum, China; IGM, Mongolian Institute of Geology, Mongolia; IVPP, Institute of Vertebrate Paleontology and Paleoanthropology, Chinese Academy of Sciences, Beijing, China; LPM, Liaoning Paleontological Museum, Liaoning Province, China; MCZ, Museum of Comparative Zoology, Harvard University, Cambridge, MA, United States; MOR, Museum of the Rockies, Bozeman, MT, United States; MPCA, Museo Provincial de Ciencias Naturales, General Roca, Río Negro, Argentina; STM, Shandong Tianyu Museum, Shandong Province, China; YFGP, Yizhou Fossil and Geology Park, Yixian, China; YPM, Yale/Peabody Museum of Natural History, New Haven, CT, United States.
We sectioned the scapulocoracoid through the glenoid of Confuciusornis IVPP V11521, a partial skeleton retaining the sternum, ribs, vertebrae, pectoral girdle, pelvis, femora, and tail (Hou et al., 1999). Although this specimen was originally described as the paratype of Confuciusornis dui (Hou et al., 1999), it is conservatively referred to Confuciusornis indet. After reexamination in the latest analysis concerning the furcula of this same specimen (Wu et al., 2021). The scapulocoracoid is mediolaterally crushed (Figure 3A) and the distal part of the scapula is missing. All elements were previously prepared free from the matrix. The humerus measures 66 mm, which falls within the larger half of the known size range of Confuciusornis specimens (humeral length: 41.01–78.5 mm; Chiappe et al., 1999, 2008; Zhang et al., 2009; Wang and O’Connor, 2017; Wang et al., 2019c). As the scapula is incomplete in this specimen, the length of the scapula was not measured.
Figure 3. Histology (ground-sections) of Confuciusornis (IVPP V11521). (A) Gross morphology of the scapulocoracoid; (B) CT slice of pectoral girdle indicated by the black line in panel (A); (C) Associated histological section indicated by the black line in panel (A), magnified region showing the compact bone connecting the scapula and coracoid is in Supplementary Figure 1A; (D) histological section of the humerus; (E) polarized light image of panel (D). cm, compact bone; cn, cancellous bone; cr, coracoid; ec, erosion cavity; gf, glenoid fossa; icl, inner circumferential layer; ocl, outer circumferential layer; sc, scapula; tb, trabecula; tl, tide line.
An adult Spilopelia chinensis (Spotted dove) and an 8-day old (post-hatching) S. camelus (Common ostrich) were sampled to allow comparison with the scapula-coracoid joint histology in extant birds. The scapulocoracoid of a more mature Common ostrich specimen IVPP OV586 was CT scanned to provide additional comparison. Based on skull length (18 cm), OV586 is estimated to be approximately 6 months old (Castanet et al., 2000; Cuff et al., 2015).
Prior to cutting, the scapulocoracoid of Confuciusornis IVPP V11521 was scanned at Yinghua Testing Co., Ltd., Shanghai, China, using high-resolution micro-computed tomography (μCT) scanning (Phoenix v | x m) with a detector resolution of 6 μm per pixel, and three-dimensional reconstructions were created with the software Avizo (version 8.1).
The ostrich scapulocoracoid IVPP OV586 was CT scanned the at the Key Laboratory of Vertebrate Evolution and Human Origins at the Institute of Vertebrate Paleontology and Paleoanthropology, Chinese Academy of Sciences, using an industrial CT (mi-CT 450ICT, developed by the Institute of High Energy Physics, Chinese Academy of Sciences) with a detector resolution of 160 μm per pixel, and three-dimensional reconstructions were created with the software VG studio (version 2.2).
The specimen was then embedded in EXAKT Technovit 7200 one-component resin and allowed to dry for 12 h, cut into slices through the glenoid fossa using the CT scans as a guide, and polished until the desired optical contrast was reached (slice thickness ∼70 μm). In total, five ground sections were made (also see Wu et al., 2021), observed under natural and polarized light using a Nikon eclipse LV100NPOL, and photographed with a DS-Fi3 camera and the software NIS-Element v4.60. The “photomerge” tool in Adobe Photoshop CS6 was used to reconstruct each section.
The shoulder joints of the ostrich and dove were extracted from their thawed carcasses with razor blades and fixed in 10% neutral buffered formalin (NBF) for at least 48 h, then demineralized in HCl and EDTA (JYBL-II, Cat DD0017, Leagene). After demineralization, the samples were embedded following the standard protocol for paraffin sections (Bailleul et al., 2017), dehydrated with a graded series of ethanol solutions (in 70, 80, 90, 95, and 100% EtOH for ∼1 h each, and two additional 100% EtOH for ∼1 h each), cleared in xylene (three changes for 30 min each), infiltrated in melted paraffin wax, and embedded manually (Paraplast Plus EMS Cat#19216). Samples were sectioned at 5 μm on a rotary microtome (Leica Biosystems RM2265) and mounted on charged slides (Superfrost Plus, Fisher Scientific).
The selected slices were stained using a modified Masson’s trichrome protocol (Witten and Hall, 2003) as follows: deparaffinized with xylene (2 changes for 5 min each), dehydrated with a graded series of ethanol solution (100, 95, 90, 80, 70, and 50% for 1 min each), rinsed in deionized water for 2 min, stained for 10 min with Mayer’s acid hematoxylin (Ruitaibio), rinsed in deionized water for 1 min, stained with Xylidine Ponceau/Acid Fuchsin for 2 min (equal volumes of 0.5% xylidine ponceau 2R CI no. 16150 in 1% acetic acid and 0.5% Acid Fuchsin CI no. 42685 in 1% acetic acid), rinsed for 1 min in deionized water, stained for 4 min with 1% phosphomolybdic acid, rinsed for 1 min in deionized water, stained with light green for 90 s (2% light green CI 42095 in 2% citric acid, diluted 1:10 with deionized water prior to use) and rinsed in deionized water for 1 min. Sections were then dipped twice in 100% ethanol for 10 s, cleared in xylene for 4 min, mounted in Permount and coverslipped.
Histological terminology is following Padian and Lamm (2013) and ontogenetic terminology is following Prondvai et al. (2014).
The scapulocoracoid of IVPP V11521 is crushed, collapsing the medullary cavity. Although distorted by compaction, the medullary cavity can still be identified in the bodies of the scapula and coracoid, both from the remnants of the space itself and from the presence of some cancellous tissue between the walls of cortical bone (Figure 3).
The scapulocoracoid consists of both compact and cancellous bone. The compact bone is mainly distributed in the bodies of the scapula and coracoid, while the cancellous bone is primarily located in the glenoid region (Figures 3B,C). A layer of compact bone connects the bodies of the scapula and coracoid opposite the glenoid on the medial (costal) side of the scapulocoracoid junction (Figures 3B,C and Supplementary Figure 1). The cancellous bone consists of erosion cavities of various sizes separated by trabeculae, similar to the epiphyseal structures and metaphyseal regions of limb bones (de Ricqlès et al., 2003). These features are also visible in the CT scans (Figure 3B).
The cancellous bone in the Confuciusornis scapulocoracoid consists of incipient fibrolamellar bone (FLB), and contains reticular to obliquely oriented vascular canals with frequent anastomoses (Figure 4A and Supplementary Figure 2). Densely arranged, plump, oval, haphazardly aligned osteocyte lacunae predominate, although some flatter osteocyte lacunae are aligned parallel to the margins of the erosion cavities (Figure 4B). The compact bone of the scapula and coracoid bodies comprises two layers: a thick inner layer of incipient FLB and a much thinner outer layer of parallel-fibered bone (PFB) (Figures 4C,D and Supplementary Figure 3). There is no clear boundary between the FLB and PFB tissue (Figures 4C,D and Supplementary Figure 3), but polarized light reveals obvious differences in collagen fiber orientation between them (Figure 4D and Supplementary Figure 3). The vascular canals in the FLB are primarily obliquely oriented, and anastomoses can be observed between several canals (Figure 4C). The inner osteocyte lacunae are plump, but the lacunae become progressively flatter and increasingly more arranged in parallel to each other toward the periosteum (Figure 4C and Supplementary Figure 4). The PFB is nearly avascular, with a low density of flat osteocyte lacunae arranged parallel to the periosteal surface (Figure 4C and Supplementary Figure 4). Because the specimen is broken and not a typical long bone mid-shaft section, it is difficult to identify primary osteons in either the cancellous or the compact bone of the scapulocoracoid, but the absence of secondary osteons is clear.
Figure 4. Close up histology (ground-section) of Confuciusornis IVPP V11521. (A) Osteohistology beneath the glenoid fossa, showing that the cancellous bone consists of fibrolamellar tissue and the compact bone consists of parallel-fibered tissue; (B) Osteohistology around the trabeculae and erosion cavities; (C) osteohistology of the scapula blade; (D) osteohistology of the scapular blade under polarized light; (E) brace indicates dark layer without celluar structures, comprising diagenetically altered calcified cartilage, with potential remnants of hyaline cartilage mixed with sediment, location of the section is indicated in Supplementary Figure 2B; (F) highly diagenetically altered calcified cartilage under polarized light; (G) osteohistology of the humerus; (H) osteohistology of the humerus under polarized light. cl, chondrocyte lacunae showing a cell-doublet organization; cn, cancellous bone; cr, coracoid; ec, erosion cavity; flb, fibrolamellar bone; gf, glenoid fossa; ol, osteocyte lacunae; pfb, parallel-fibered bone; po, primary osteon; sc, scapula; vc, vascular canals; va, vascular canals anastomose.
The humerus of Confuciusornis IVPP V11521 is also crushed. The midshaft of the humerus consists of compact bone (Figure 3D). An inner circumferential layer (ICL) lines the medullary cavity, consisting of PFB. The ICL is separated from the thicker FLB layer by a tide line (Figures 3D,E). The majority of the compacta consists of FLB, which gradually transitions into PFB toward the periosteum as the vascular canals and osteocyte lacunae decrease in numbers. As in the scapulocoracoid, no clear boundary between the FLB and PFB layers exists (Figure 3D). The PFB of the ICL is avascular, and the flat osteocyte lacunae and collagen fibers are oriented parallel to the endosteum. The FLB layer is richly vascularized by longitudinal canals, among which are numerous anastomoses (Figure 4G). The outer PFB layer is avascular, and is interpreted as the OCL. This layer is more continuous than in the scapulocoracoid (Figure 3D). LAGs are absent in the OCL (Figures 4G,H) and the FLB mid-cortical bone. In the OCL the osteocyte lacunae are flat and the dense collagen fibers are arranged parallel to the periosteum (Figure 4H), as in the ICL. Primary osteons are abundant in the FLB (Figure 4G and Supplementary Figure 5), but no secondary osteons are visible.
In general, the bone microstructure of the scapulocoracoid and humerus is consistent with observations from previous histological studies of Confuciusornis, with both FLB and PFB present (Zhang et al., 1998; de Ricqlès et al., 2003; Erickson et al., 2009; Chinsamy et al., 2019). de Ricqlès et al. (2003) reported that in adult Confuciusornis the weight bearing femur undergoes the most remodeling, exhibiting numerous secondary osteons, followed by the humerus in which just a few secondary osteons are present, while the non-load-bearing pygostyle and fibula only have primary osteons (de Ricqlès et al., 2003). However, a recent osteohistology analysis of 14 Confuciusornis specimens by Chinsamy et al. (2019) did not report secondary osteons in any element, even in individuals with three to four LAGs and humeral lengths over 65 mm. According to Chinsamy et al. (2019), Confuciusornis grew rapidly from early ontogeny until almost adult size, then experienced at least three to four years slow and episodic growth to reach skeletal maturity, indicated by the LAGs in the OCL. In contrast to long bones, no noticeable ICL is visible in the ground sections of the scapulocoracoid. The presence of an OCL in the humerus suggests growth had slowed down by the time of death in this individual, but the absence of LAGs in the OCL indicates that IVPP V11521 had not finished its first year of growth at the time of death.
In the adult Spotted dove, the subchondral bone of the scapula and coracoid is composed of densely arranged secondary osteons (Figure 5C). The collagen fibers are oriented in parallel around the central canal of the Haversian system and along the erosion cavities. In some regions the PFB is nearly avascular (Figure 5D). The ossified scapula and coracoid are connected by fibrocartilage (Figures 5C,D), and covered by a single thick layer of articular cartilage, much thicker than that on the humeral head, together forming the glenoid fossa (Figure 5A). The articular cartilage is also more fibrous than the humeral articular cartilage (Figure 5B), with larger collagen fibers (as indicated by the faintly redder stain; Figure 5D).
Figure 5. Paraffin thin section showing the histology of the pectoral girdle of an adult Spilopelia chinensis (Spotted dove). (A) Thin-section of shoulder joint in Spotted dove, indicated by the white line in panel (E). It shows a synovial joint between the humerus and the scapula and coracoid, the latter two technically “separated” and only linked together by some unmineralized fibrocartilage; (B) Close-up of left black box in panel (A), showing the histology of the humeral head and its articular cartilage, with its multiple zones; (C) Close-up of middle black box in panel (A); (D) Close-up of right black box in panel (A), showing the synchondrosis joint formed by fibrocartilage and calcified cartilage between the scapula and coracoid; (E) 3D reconstruction of shoulder joint of the Spotted dove. cc, calcified cartilage; cr, coracoid; fb, fibrocartilage; hu, humerus; md, middle zone; pfb, parallel-fibered bone; sb, subchondral bone; sc, scapula; sn, synovial cavity; so, secondary osteon; st, superficial tangential zone; te, tear. This slide was stained with a modified Masson’s trichrome.
The humerus and scapulocoracoid are not fully ossified in the 8-day old (post-hatching) ostrich we sampled. These bones are composed mostly of hyaline cartilage with a few cartilage canals in the bodies of the elements, and newly formed bone tissue along the perichondrium (Figure 6). The glenoid fossa is almost completely cartilage at this ontogenetic stage. Invasive cartilage canals indicate the individual is at an early stage of ossification (Figure 6C).
Figure 6. Paraffin thin-section showing the histology of pectoral girdle of an 8-day-old Struthio camelus (Common ostrich). (A) Thin-section of the shoulder joint. It shows a synovial joint between the humerus and the already fused (but still mostly unmineralized) cartilaginous scapulocoracoid; (B) Close-up of black box in panel (A), showing the synovial cavity and fibrous capsule of the synovial joint between the humerus and scapulocoracoid, and the hyaline cartilage of the humerals head; (C) Close-up of right black box in panel (A), showing the fibroid surface of the articular surface, hyaline cartilage of the un-ossified scapulocoracoid body, and invasive cartilage canals; (D) Close-up of left black box in panel (A), showing hypertrophic chondrocytes. ac, articular cartilage; ca, cartilage canals; fb, fibrocartilage; fc, fibrous capsule; hc, hyaline cartilage; hp, hypertrophic chondrocytes; sn, synovial cavity. Slide stained with a modified Masson’s trichrome.
The scapulocoracoid of IVPP OV586 measures 22 cm in length. Superficially the scapula and coracoid appear fully fused at the level of the glenoid with the acromion process of the scapula also fused to the sternolateral margin of the coracoid (Supplementary Figure 6A). In the CT scans traversing the glenoid fossa, the joint between the scapula and coracoid is composed of an outer layer of compact bone and an inner layer of cancellous bone. Within the cancellous bone the bony trabeculae have a uniform arrangement, with no trace of any gap, osteoid bridge, rugose digitation or remnant of bone wall that would indicate the presence of a suture or cartilage (Supplementary Figure 6B). In contrast, clear remnants of the bony wall are present between the lateral corner of the sternal margin of the coracoid and the acromion process of scapula indicating fusion between these two parts of the scapula and coracoid was not complete at the time of death (Supplementary Figure 6C). This CT data indicates that the scapula and coracoid at the level of the glenoid fused early in the Common ostrich, well within the first year of postnatal growth, and prior to fusion between the acromion process of scapula and the sternal margin of the coracoid.
Although broken, the FLB and PFB matrix of the cancellous bone and compact bone tissue under the glenoid fossa of Confuciusornis is continuous and uniform in all the CT scan images and ground sections (Figures 3B,C). There is no evidence of a gap to indicate the presence of a suture (Bailleul et al., 2016). Our preliminary data on the Spotted dove show that the two bones in this taxon are linked together by fibrocartilage, making this joint a synchondrosis (Figure 5). No trace of a cartilaginous connection that would indicate a synchondrosis like that in the Spotted dove can be identified in IVPP V11521. The presence of an ongoing fusion, as would be indicated by the existence of a less mineralized osteoid bridge (Funston et al., 2019) or rugose digitation (Brochu, 1995; Parsons and Parsons, 2015), is also not supported by the evidence. Therefore, we confirm that the scapula and coracoid in Confuciusornis IVPP V11521 are completely fused into a bony scapulocoracoid, forming a true synostosis.
A thin amorphous brown layer is preserved along the articular surface of the glenoid fossa (Figure 4 and Supplementary Figure 2B). Viewed under transmitted light, this layer is clearly distinct from the bone tissue beneath it (Figure 4E). Under polarized light, this layer is uniformly dark, with no observable cellular structures (Figure 4F). Near this layer, there are some large globular lacunae, distinct from the small fusiform to oval osteocyte lacunae in the bone tissue. A cell-doublet confirms these are chondrocyte lacunae (Figure 4F), which in turn supports identification of this layer as calcified cartilage, most likely representing a remnant of the articular cartilage of the glenoid fossa. Calcified fibrocartilage was also found on the other side of the glenoid cavity of the same specimen, where it articulates with the furcula (Wu et al., 2021). Calcified cartilage tissue has been previously reported in two specimens of Confuciusornis and one of Yanornis, also from the Jehol Biota (de Ricqlès et al., 2003; Jiang et al., 2017; Bailleul et al., 2019a). The tissue of the darker layer has been deeply altered histologically by diagenetic processes, and may have been invaded by bacteria post-mortem. In the Spotted dove, the fibrocartilaginous component of the articular cartilage occupies the same position above the calcified cartilage (Figure 5). Compared to calcified cartilage, fossilized hyaline cartilage and fibrocartilage are very rare (Schwarz et al., 2007; Schweitzer, 2011). Further investigation is required (e.g., using SEM and EDS analyses) to determine if any remnants of the hyaline cartilage are also fossilized. Preservation of remnants of fossilized fibrocartilage reported in another specimen of Confuciusornis was suggested to be related to pyroclastic flows (Jiang et al., 2014, 2017).
Osteohistology has become widely applied in vertebrate paleontology during the last three decades (e.g., see review by Ray et al., 2010; Padian and Lamm, 2013; Bailleul et al., 2019b). Studying the microstructure of bone can help scientists better understand not only growth-related questions but also functional aspects of the skeleton such as joint morphology and mobility (Bailleul et al., 2019a), and much more (Bailleul et al., 2019b). Over the past three decades, abundant fossil birds have been unearthed from deposits yielding the Early Cretaceous Jehol Biota. As the number of taxa has increased, so has the number of histological studies conducted on these specimens (e.g., de Ricqlès et al., 2003; O’Connor et al., 2014, 2018a; Jiang et al., 2017; Chinsamy et al., 2019). Until recently these histological analyses have focused almost entirely on limb bones in growth related studies. Here we utilize histology to shed light on the morphology of the joint between the scapula and coracoid in Confuciusornis. This study represents the first traditional, non-digital osteohistological analysis of the glenoid joint in a Mesozoic theropod, although the histology of this joint has been viewed through virtual thin-sections in the Daiting Archaeopteryx (Kundrát et al., 2019) and the scapular corpus has been studied through ground sections in the enantiornithine Mirusavis IVPP V18692 (Wang et al., 2019a).
In Confuciusornis, a separate scapula and coracoid have not been described in any published specimen, nor can this condition be identified from published descriptions or figures (e.g., holotype IVPP V10918, V10923, V10928, V11308, V13313, V14412, GMV2132-33, DNHM D2454) (Hou et al., 1995; Hou, 1997; Chiappe et al., 1999; Dalsätt et al., 2006; Zhang et al., 2009; Wang et al., 2019c), even in one purportedly immature specimen (IVPP V13172; Wang et al., 2019c). We conducted a survey of 132 unpublished Confuciusornis specimens at the Shandong Tianyu Museum of Nature and five unpublished specimens at the IVPP (IVPP V11305, V11548, V11552, V11795, and V13338), and found no specimens that clearly preserved a separate or sutured scapula and coracoid. In contrast, unfused scapulae and coracoids are readily identified in specimens of numerous paravian taxa in which it is evident that these bones only became fused later in ontogeny (e.g., Anchiornis, Microraptor, Archaeopteryx, and Sapeornis).
Both CT data and histological analysis of Confuciusornis IVPP V11521 confirm the presence of a synostosis joint between the scapula and coracoid (the elements are fully fused)–no traces of a gap, fibrous connection, intervening cartilage, osteoid bridge, or rugose digitation, features which would indicate a suture, synchondrosis or syndesmosis joint, can be identified in the ground sections (Figures 3,4). This study demonstrates that in a large but young specimen of Confuciusornis, apparently within the first year of its growth, fusion of the scapulocoracoid was already complete. This indicates that the scapulocoracoid fused relatively early in the ontogeny of Confuciusornis, prior to skeletal maturity. Although at this stage interpretations are preliminary, since only a single specimen has been studied, the combined facts that Confuciusornis IVPP V11521 preserves no LAGs in the humerus, indicating it is most likely in its first year of growth, yet records no vestiges of ongoing fusion at the scapulocoracoid juncture, strongly suggests that in contrast to other Mesozoic paravians fusion between these elements began early in ontogeny and was completed within the first year, before somatic maturity.
At this time, it is uncertain if the relative early fusion of scapula and coracoid in Confuciusornis is due to differences in growth strategy between Confuciusornis and most other non-ornithuromorpha paravians, or if there exists some other underlying functional explanation, and how fusion patterns are affected by developmental plasticity. In stark contrast to other non-ornithothoracine paravians, Confuciusornis attained the majority of its body mass in the first year, after which it continued to grow at a minimal rate for three to four years, depositing several LAGs in the OCL (Chinsamy et al., 2019). In contrast, other non-ornithuromorpha paravians took several years to reach the same relative body mass (Erickson et al., 2009; Zheng et al., 2014; Prondvai et al., 2018; Shen et al., 2019), and their fossil record is dominated by subadults. A large size range is observed in Confuciusornis, but size does not strictly correlate with the number of visible LAGs, an inconsistency interpreted as the result of developmental plasticity (Chinsamy et al., 2019). Similar developmental plasticity has also been documented in other paravians with protracted growth, in that size and osteohistological maturity are not strongly correlated in such taxa (e.g., Sapeornis, Anchiornis) (Zheng et al., 2014). Despite the abundance of specimens, morphological variation in development has yet to be quantified, but developmental plasticity clearly was present in non-neornithine avians and thus extended well into at least some lineages of the Avetheropoda, contra Griffin and Nesbitt, 2016. Confuciusornis IVPP V11521 may be relatively young, but is fairly large (humeral length 66 mm, in the higher end of the documented size range of 41.01–78.5 mm; Chiappe et al., 1999, 2008; Zhang et al., 2009; Wang and O’Connor, 2017; Wang et al., 2019c). Since developmental plasticity can affect character states as well as size (Griffin and Nesbitt, 2016), further sampling both within Confuciusornis and across a broader range of paravians is required to shed light on the potential effects of development plasticity on fusion events in this taxon. However, given that fusion is entirely complete between these two elements and no sutured specimens have ever been identified, developmental plasticity is unlikely to strongly affect the conclusion that fusion of the scapulocoracoid occurs early in Confuciusornis.
The fused scapulocoracoid in the Jinguofortisidae is hypothesized to reflect the accelerated osteogenesis in this clade, relative to other non-ornithuromorpha birds (Wang et al., 2018). This may also at least partially explain the ubiquitous presence of scapulocoracoid fusion in Confuciusornis since it is known this taxon grew more rapidly than other non-ornithuromorpha paravians (Chinsamy et al., 2019). The histology of the limb bones in the only two known jinguofortisid specimens indicates they are mature, so without further data it cannot be determined if fusion in this calde is simply due to ontogenetic maturity. In addition, the exact morphology of the scapulocoracoid joint in jinguofortisids is unknown, as it has never been investigated using high resolution scans or histology. However, the fact that the scapulocoracoid joint is completely fused in Confuciusornis IVPP V11521 within the first year of its growth and thus prior to skeletal maturity still represents a departure from the pattern observed in most other paravians, in which fusion only occurs with skeletal maturity, and thus rapid growth rates in Confuciusornis do not fully explain the differences in fusion pattern observed.
The fully synostosed morphology observed in Confuciusornis IVPP V11521, together with the absence of any known specimen preserving a suture, suggests the scapula and coracoid fused early in ontogeny, potentially first via cartilage during embryology in a pattern similar to that seen in the Common ostrich (Figure 6). In the ostrich, however, the bones ossify post-hatching from different centers, even though cartilage forms a single unit embryonically (Maxwell and Larsson, 2009). If fossilized, a hatchling ostrich would appear to have separate scapulae and coracoids, despite the unmineralized cartilaginous connection in embryonic life. Morphological observations and CT scans indicate the scapulocoracoid at the level of the glenoid is fully fused in the ostrich specimen IVPP OV586. Although the exact age of this ostrich specimen is unknown, we estimate it to have been a juvenile approximately 6 months old, based on the skull length (18 cm), and the small size of the scapulocoracoid (length 22 cm) compared to that of an adult ostrich specimen (IVPP OV1026, scapulocoracoid length 25 cm). Unfortunately histology cannot be conducted on this specimen to corroborate this estimate. This specimen demonstrates that like Confuciusornis the ostrich scapulocoracoid fuses early during postnatal ontogeny, during the first year of growth, well before skeletal maturity is achieved. In order to fully compare the pattern in the Common ostrich with that in Confuciusornis, detailed knowledge of the post-hatching ossification pattern in the scapulocoracoid would be required for both taxa. This is further complicated by the fact that fusion of compact bones is heterochronic and may vary among individuals and between species (Brochu, 1995). For example, in crocodilians, the other clade of extant archosaurs, closure of the synchondrosis between the scapula and coracoid begins only after hatching. Closure in immature specimens is reported in Caiman crocodilus, Melanosuchus niger, and Paleosuchus palpebrosus, whereas in Alligator closure is considered to be a gerontic character, associated with old age (Brochu, 1995). Without fossils capturing the early ontogenetic stages of Confuciusornis, the pattern of fusion in this taxon may never be fully understood.
In the Spotted dove, a volant neornithine, the separated scapula and coracoid are connected by fibrocartilage, forming a rigid synchondrosis joint (Figure 5). This makes it unclear what the functional difference is between a fused and unfused scapula-coracoid complex in birds, since in both cases the joint is immobile. Another major challenge in understanding the early avian transition from sutured or fused (Archaeopteryx, Jinguofortisidae, and Confuciusornis) to connected by a synchondrosis or completely separated (Ornithothoraces) is that both morphologies occur in volant taxa, both within Aves and among volant non-avian dinosaurs. The scapulocoracoid is fused in the volant dromaeosaurid Microraptor, whereas all known specimens of the volant Scansoriopterygidae have separate scapulae and coracoids (although the very limited record of this clade is dominated by immature individuals) (Zhang et al., 2008b; Xu et al., 2015; Wang et al., 2019b).
The early fusion of the scapulocoracoid in Confuciusornis, unlike in most other Cretaceous non-ornithuromorph paravians, and the presence of a sutured scapula and coracoid in the holotype of the basal confuciusornithid Eoconfuciusornis, may collectively suggest that fusion of the scapulocoracoid in Confuciusornis is secondarily derived, as in flightless paleognaths. This hypothesis is somewhat consistent with differences in the morphology of the scapulocoracoid between Archaeopteryx and Sapeornis, in which the coracoid retains the plesiomorphic axe-shape, and Jeholornis, Confuciusornis, and ornithothoracines, in which the coracoid is strut-like (O’Connor et al., 2011). An alternative but not mutually exclusive hypothesis is that separation of the scapulocoracoid evolved more than once in early avians, appearing evolving independently in the Jeholornis lineage and then again in the lineage including Sapeornis and Ornithothoraces. The high degree of homoplasy at this early point in avian evolution, and the absence of early ontogenetic stages in the non-enantiornithine Mesozoic avian fossil record, hinder interpretations. Microanatomical studies of the scapula-coracoid joint in Sapeornis and Jeholornis will help to elucidate this issue in the future.
Scapulocoracoid fusion in Neornithes is not common. The scapula and coracoid are separate in most neognaths (Figure 5), including several species from different lineages which have secondarily lost flight, such as Thambetochen chauliodus (Anatidae; Olson and Wetmore, 1976) and Strigops habroptilus (Psittaciformes; Livezey, 1992), and flightless lineages such as the Phorusrhacidae (Alvarenga and Höfling, 2003). A quarter of all extant species in the Rallidae are flightless (Olson, 1973) and in these taxa the scapula and coracoid remain separate although they demarcate an obtuse angle (acute in volant birds), and processes for muscle attachment are reduced (Olson, 1973). This may suggest that following the loss of flight reduction of the scapula-coracoid joint precedes fusion of these elements in neornithines or that fusion of the scapulocoracoid in neornithines is not related to flightlessness as is commonly suggested (e.g., Mayr, 2007; Mayr, 2011). This morphology is primarily found in large flightless taxa (e.g., Dinornithidae, Gastornitihidae, “ratites”) and thus may potentially be related to body size as well.
Several factors affect skeletal fusion. Age and diet can result in fusion among pectoral girdle elements in some living species. Older adult Hoatzin often lay down new bone within connective tissues, resulting in the fusion of parts of the skeleton, probably because of the high calcium content of their vegetarian diet (Kaiser, 2007). In the Alligator closure of the synchondrosis between the scapula and coracoid is associated with senescence (Brochu, 1995). This type of effect may explain rare fusion of these elements in taxa like Archaeopteryx, but does not explain the condition in Confuciusornis.
Soaring frigatebirds and pelicans are among the few volant crown birds that have fused elements in the pectoral girdle. The furcula and sternum are fused in the Spot-billed pelican (Pelecanus philippensis; Sathyamoorthy et al., 2012) and in frigatebirds the furcula and coracoid are fused to each other and to the sternum (Kaiser, 2007), but the scapula and coracoid remain separate (Olson, 1977; F. Seranno pers. comm.). Understanding the function of these fused elements may help to elucidate the evolutionary changes in the fusion of the shoulder joint in Mesozoic paravians. Fusion of the axial elements provides rigidity necessary for flight, while fusion of other bones reduces weight while providing strength, all of which are considered advantageous for flight (Kaiser, 2007; Lovette and Fitzpatrick, 2016; Gill et al., 2019). Pectoral fusion may evolve for a number of reasons. For example, fusion of the sternum and furcula in pelicans may be related to the expansion of these elements, which is purportedly a feature that aids in homeostasis (Kaiser, 2007). Frigatebirds are extremely agile and have the lowest wing-loading among all birds (Brooke, 2018). Pectoral fusion in this group may serve to reduce body mass, helping to lower wing-loading while retaining strength, thus reducing the cost of flight (Weimerskirch et al., 2003). This is unlikely the underlying cause of scapulocoracoid fusion in Confuciusornis, the skeleton of which is generally robust.
Mechanical stimuli (e.g., weight bearing, movement, and tension) promote chondrogenesis and result in delayed ossification and may also affect joint structure. The asymmetric, enlarged ischiopubic synchondrosis in children forms via delayed ossification, due to mechanical forces being applied asymmetrically by the weight-bearing non-dominant limb (Herneth et al., 2004). In mice, tensile stress will promote the expression of core-binding factor α1 (Cbfa1) and vascular endothelial growth factor (VEGF) in the spheno-occipital synchondrosis (Lei et al., 2008). Even in tendons, tension is required to initiate chondrogenesis (Hall, 2005). In contrast, absence of mechanical stimuli can result in joint fusion. Lack of in ovo movement results in fusion of the normally synovial ankle joint in paralyzed chicken embryos (Persson, 1983). In rabbits, intervertebral discs degenerate and are replaced by bone after the transverse processes of the lumbar vertebrae are fixed through surgery (Phillips et al., 2002). Skeletogenesis is sensitive to mechanical stimulation; this reactivity has a possible role in evolution as a source of phenotypic plasticity and the formation of de novo skeletal elements from responsive tissues (Müller, 2003). This may suggest that, during the evolution of the confuciusornithiform lineage, these birds experienced less mechanical stimulation of the glenoid than members of avian lineages in which the scapula and coracoid are unfused or fuse only in senescence. This in turn would suggest the existence of important mechanical differences in the flight stroke, or in the forces exerted on the glenoid, between forms in which the scapulocoracoid is sutured and/or fused (at least in mature individuals) (e.g., Archaeopteryx and Confuciusornis) and taxa in which the two elements are fully separate (e.g., ornithothoracines). Because fusion occurs in both volant (e.g., Confuciusornis) and non-volant taxa (e.g., Struthionidae), however, flight mechanics cannot be the only factor affecting the occurence of fusion in this joint.
Although the underlying pressures responsible for changes in the joint architecture of the scapulocoracoid are not understood at this time, studies of extant birds hint at the genes that may have been active in producing the two phenotypes. In the chicken, for example, abnormal expression of the Hoxc6 gene may result in fusion of the scapula and coracoid, accompanied by thickening of the scapular head, reduction of the coracoid (resulting in reduction or loss of the supracoracoid process and a shorter, thicker coracoid), loss of the acrocoracoid process, and other malformations of the pectoral girdle elements (Oliver et al., 1990; Williams, 2003). Differences in expression of this gene may have been involved in the transition within Paraves from fusion to separation of the scapula-coracoid complex.
The fusion of the scapulocoracoid in Confuciusornis is likely the result of a complex interplay of genetic, mechanical and epigenetic factors and cannot be fully understood from the currently available data.
Among early paravians in which the scapulocoracoid is fused, Confuciusornis is unique in that a synotosis is present in all available and published specimens. In contrast, these bones are fused only in the most mature individuals of other paravians. However, previous descriptions of joint morphology have not utilized microanatomical tools capable of determining the type of joint present with high accuracy. In this first osteohistological study of the scapulocoracoid glenoid joint in a Cretaceous paravian, we demonstrate that the scapulocoracoid is completely fused in Confuciusornis IVPP V11521. Humeral histology suggests this specimen was nearing completion of its first year of growth. The bodies of the scapula and coracoid consist of compact bone with an internal FLB layer and outer PFB layer. The bone along the glenoid fossa consists of cancellous FLB. Calcified cartilage is preserved on the articular surface of the glenoid. Both CT data and histology confirm the presence of a synostosed joint and a fully fused scapulocoracoid in IVPP V11521. This evidence suggests the Confuciusornis scapulocoracoid fused early in development, before skeletal maturity was achieved. However, further sampling of Confuciusornis is required to understand how developmental plasticity may affect the timing of this fusion event. Fusion of the scapulocoracoid in Confuciusornis most likely results from a complex interplay of genetic, mechanical and epigenetic factors; we hypothesize the primary factor may be a relative decrease in the amount of mechanical stimulation received by the glenoid of Confuciusornis relative to those of other volant birds, as a unique flight style has already been suggested for this taxon. Further investigation into the architecture of the glenoid joint in other Mesozoic paravians, and the timing and pattern of scapulocoracoid fusion in selected extant birds, will help to elucidate the transition from sutured/fused to unfused and separate that occurs early in avian evolution, and may shed light on flight dynamics in early birds.
The original contributions presented in the study are included in the article/Supplementary Material, further inquiries can be directed to the corresponding author/s.
Ethical review and approval was not required for the animal study because the dove specimen is cadaveric when we collect, and the ostrich is collected legally from a commercial ostrich farm.
All authors designed the research, performed the research, and analyzed the data. QW and AB collected the data. QW, AB, JO’C, and ZL wrote the manuscript.
This work has been supported by the National Natural Science Foundation of China (41688103). AB also thanks the CAS-PIFI[cpsenter]Program.
The authors declare that the research was conducted in the absence of any commercial or financial relationships that could be construed as a potential conflict of interest.
We thank Gao Wei for photographing for the specimen, Zhang Shukang for preparing the ground sections, Zhang Limin for facilitating lab access, and Georg Janßen and Oliver Rauhut for providing the photo of the Munich Archaeopteryx (Figure 2A), and Holly Woodward for discussing the histology of the Confuciusornis specimen, and F. Seranno for discussing the pectoral girdle characters of frigatebirds. We also thank two reviewers for their comments that improved the manuscript.
The Supplementary Material for this article can be found online at: https://www.frontiersin.org/articles/10.3389/feart.2021.617124/full#supplementary-material
Alvarenga, H. M., and Höfling, E. (2003). Systematic revision of the phorusrhacidae (aves: ralliformes). Papéis Avulsos de Zool. 43, 55–91. doi: 10.1590/S0031-10492003000400001
Bailleul, A. M., and Horner, J. R. (2016). Comparative histology of some craniofacial sutures and skull-base synchondroses in non-avian dinosaurs and their extant phylogenetic bracket. J. Anat. 229, 252–285.
Bailleul, A. M., Li, Z., O’Connor, J. K., and Zhou, Z. (2019a). Origin of the avian predentary and evidence of a unique form of cranial kinesis in Cretaceous ornithuromorphs. Proc. Natl. Acad. Sci. 116, 24696–24706. doi: 10.1073/pnas.1911820116
Bailleul, A. M., O’Connor, J. K., and Schweitzer, M. H. (2019b). Dinosaur paleohistology: review, trends and new avenues of investigation. PeerJ 2019, 1–45. doi: 10.7717/peerj.7764
Bailleul, A. M., Scannella, J. B., Horner, J. R., and Evans, D. C. (2016). Fusion patterns in the skulls of modern archosaurs reveal that sutures are ambiguous maturity indicators for the dinosauria. PLoS One 11:0147687. doi: 10.1371/journal.pone.0147687
Bailleul, A. M., Witmer, L. M., and Holliday, C. M. (2017). Cranial joint histology in the mallard duck (Anas platyrhynchos): new insights on avian cranial kinesis. J. Anat. 230, 444–460. doi: 10.1111/joa.12562
Baumel, J. J., and Witmer, L. M. (1993). “Osteologia,” in Handbook of avian anatomy: nomina anatomica avium, Second Edn, eds J. J. Baumel, A. S. King, J. E. Breazile, H. E. Evans, and J. C. Berge (Cambridge: Nuttall Ornithological Club), 45–132.
Bertelli, S., Chiappe, L. M., and Mayr, G. (2014). Phylogenetic interrelationships of living and extinct Tinamidae, volant palaeognathous birds from the new world. Zool. J. Linn. Soc. 172, 145–184. doi: 10.1111/zoj12156
Brochu, C. A. (1995). Heterochrony in the crocodylian scapulocoracoid. J. Herpetol. 29:464. doi: 10.2307/1565002
Brooke, M. (2018). Far From Land: The Mysterious Lives of Seabirds. Princeton: Princeton University Press.
Brusatte, S. L., Lloyd, G. T., Wang, S. C., and Norell, M. A. (2014). Gradual assembly of avian body plan culminated in rapid rates of evolution across the dinosaur-bird transition. Curr. Biol. 24, 2386–2392. doi: 10.1016/j.cub.2014.08.034
Castanet, J., Curry Rogers, K., Cubo, J., and Jacques-Boisard, J. (2000). Periosteal bone growth rates in extant ratites (ostriche and emu). Implications for assessing growth in dinosaurs. Comptes Rendus de l’Académie des Sci.-Ser. III-Sci. de la Vie 323, 543–550. doi: 10.1016/S0764-4469(00)00181-5
Chiappe, L. M., Ji, S., Ji, Q., and Norell, M. A. (1999). Anatomy and systematics of the confuciusornithidae (theropoda: aves) from the late mesozoic of northeastern china. Bull. Am. Museum Nat. His. 242:89.
Chiappe, L. M., Marugán-Lobón, J., Ji, S., and Zhou, Z. (2008). Life history of a basal bird: morphometrics of the early cretaceous Confuciusornis. Biol. Lett. 4, 719–723. doi: 10.1098/rsbl.2008.0409
Chiappe, L. M., and Witmer, L. M. (2002). Mesozoic birds: above the heads of dinosaurs. California: University of California Press.
Chinsamy, A., Marugán-Lobón, J., Serrano, F. J., and Chiappe, L. (2019). Osteohistology and life history of the basal pygostylian, Confuciusornis sanctus. Anat. Rec. 303, 949–962. doi: 10.1002/ar.24282
Cuff, A. R., Bright, J. A., and Rayfield, E. J. (2015). Validation experiments on finite element models of an ostrich (Struthio camelus) cranium. PeerJ 3:e1294. doi: 10.7717/peerj.1294
Dalsätt, J., Zhou, Z., Zhang, F., and Ericson, P. G. P. (2006). Food remains in Confuciusornis sanctus suggest a fish diet. Naturwissenschaften 93, 444–446. doi: 10.1007/s00114-006-0125-y
de Ricqlès, A., Padian, K., Horner, J. R., Lamm, E. T., and Myhrvold, N. (2003). Osteohistology of Confuciusornis sanctus (theropoda: aves). J. Vertebrate Paleontol. 23, 373–386.
Elzanowski, A. (2001). A new genus and species for the largest specimen of Archaeopteryx. Acta Palaeontologica Polonica 46, 519–532.
Erickson, G. M., Rauhut, O. W. M., Zhou, Z., Turner, A. H., Inouye, B. D., Hu, D., et al. (2009). Was dinosaurian physiology inherited by birds? reconciling slow growth in Archaeopteryx. PLoS One 4:e7390. doi: 10.1371/journal.pone.0007390
Faux, C., and Field, D. J. (2017). Distinct developmental pathways underlie independent losses of flight in ratites. Biol. Lett. 13:20170234. doi: 10.1098/rsbl.2017.0234
Funston, G. F., Currie, P. J., Ryan, M. J., and Dong, Z. M. (2019). Birdlike growth and mixed-age flocks in avimimids (theropoda, oviraptorosauria). Sci. Rep. 9, 1–21. doi: 10.1038/s41598-019-55038-5
Gao, C., Chiappe, L. M., Zhang, F., Pomeroy, D. L., Shen, C., Chinsamy, A., et al. (2012). A subadult specimen of the early cretaceous bird Sapeornis chaoyangensis and a taxonomic reassessment of sapeornithids. J. Vertebrate Paleontol. 32, 1103–1112. doi: 10.1080/02724634.2012.693865
Gill, F. B., Prum, R. O., and Robinson, S. K. (2019). Ornithology, Fourth Edn. New York: W.H. Freeman and Macmillan Learning.
Griffin, C. T., and Nesbitt, S. J. (2016). Anomalously high variation in postnatal development is ancestral for dinosaurs but lost in birds. PNAS 113, 14757–14762. doi: 10.1073/pnas.1613813113
Herneth, A. M., Philipp, M. O., Pretterklieber, M. L., Balassy, C., Winkelbauer, F. W., and Beaulieu, C. F. (2004). Asymmetric closure of ischiopubic synchondrosis in pediatric patients: correlation with foot dominance. Am. J. Roentgenol. 182, 361–365. doi: 10.2214/ajr.182.2.1820361
Hou, L., Martin, L. D., Zhou, Z., Feduccia, A., and Zhang, F. (1999). A diapsid skull in a new species of the primitive bird Confuciusornis. Nature 399, 679–682.
Hou, L., Zhou, Z., Gu, Y., and Zhang, H. (1995). Confuciusornis sanctus, a new late Jurassic sauriurine bird from china. Chinese Sci. Bull. 40, 1545–1551.
Hou, L., Zhou, Z., Zhang, F., and Gu, Y. (2002). Mesozoic birds from western Liaoning in China. Shenyang: Liaoning Science and Technology Publishing House.
Houde, P. (1986). Ostrich ancestors found in the northern hemisphere suggest new hypothesis of ratite origins. Nature 324, 563–565. doi: 10.1038/324563a0
Hu, D., Hou, L., Zhang, L., and Xu, X. (2009). A pre-Archaeopteryx troodontid theropod from china with long feathers on the metatarsus. Nature 461, 640–643. doi: 10.1038/nature08322
Hwang, S. H., Norell, M. A., Ji, Q., and Gao, K. (2002). New specimens of Microraptor zhaoianus (theropoda: dromaeosauridae) from northeastern china. Am. Museum Novitates 3381, 1–44. doi: 10.1206/0003-00822002381<0001:NSOMZT<2.0.CO;2
Ji, Q., Chiappe, L. M., and Ji, S. (1999). A new late mesozoic confuciusornithid bird from china. J. Vertebrate Paleontol. 19, 1–7. doi: 10.1080/02724634.1999.10011117
Ji, Q., Ji, S., Lü, J., You, H., Chen, W., Liu, Y., et al. (2005). First avialian bird from china (Jinfengopteryx elegans gen. et sp. nov.). Geol. Bull. China 24, 197–205.
Jiang, B., Harlow, G. E., Wohletz, K., Zhou, Z., and Meng, J. (2014). New evidence suggests pyroclastic flows are responsible for the remarkable preservation of the Jehol biota. Nat. Commun. 5:3151. doi: 10.1038/ncomms4151
Jiang, B., Zhao, T., Regnault, S., Edwards, N. P., Kohn, S. C., Li, Z., et al. (2017). Cellular preservation of musculoskeletal specializations in the cretaceous bird Confuciusornis. Nat. Commun. 8, 1–10. doi: 10.1038/ncomms14779
Kundrát, M., Nudds, J., Kear, B. P., Lü, J., and Ahlberg, P. (2019). The first specimen of Archaeopteryx from the upper jurassic mörnsheim formation of germany. His. Biol. 31, 3–63. doi: 10.1080/08912963.2018.1518443
Lefèvre, U., Hu, D., Escuillié, F., Dyke, G., and Godefroit, P. (2014). A new long-tailed basal bird from the lower cretaceous of north-eastern china. Biol. J. Linn. Soc. 113, 790–804. doi: 10.1111/bij.12343
Lei, W. Y., Wong, R. W. K., and Rabie, A. B. M. (2008). Factors regulating endochondral ossification in the spheno-occipital synchondrosis. Angle Orthod. 78, 215–220. doi: 10.2319/020707-59.1
Livezey, B. C. (1992). Morphological corollaries and ecological implications of flightlessness in the kakapo (psittaciformes: Strigops habroptilus). J. Morphol. 213, 105–145. doi: 10.1002/jmor.1052130108
Makovicky, P. J., Apesteguía, S., and Agnolín, F. L. (2005). The earliest dromaeosaurid theropod from south america. Nature 437, 1007–1011. doi: 10.1038/nature03996
Maxwell, E. E., and Larsson, H. C. E. (2007). Osteology and myology of the wing of the Emu (Dromaius novaehollandiae), and its bearing on the evolution of vestigial structures. J. Morphol. 268, 423–441.
Maxwell, E. E., and Larsson, H. C. E. (2009). Comparative ossification sequence and skeletal development of the postcranium of palaeognathous birds (aves: palaeognathae). Zool. J. Linn. Soc. 157, 169–196. doi: 10.1111/j.1096-3642.2009.00533.x
Mayr, G. (2007). The birds from the paleocene fissure filling of walbeck (germany). J. Vertebr. Paleontol. 27, 394–408.
Mayr, G. (2011). Metaves, mirandornithes, strisores and other novelties - a critical review of the higher-level phylogeny of neornithine birds: higher-level phylogeny of birds. J. Zool. Syst. Evol. Res. 49, 58–76. doi: 10.1111/j.1439-0469.2010.00586.x
Mayr, G., Pohl, B., and Peters, D. S. (2005). A well-preserved Archaeopteryx specimen with theropod features. Science 310, 1483–1486. doi: 10.1126/science.1120331
McGowan, C. (1982). The wing musculature of the Brown kiwi Apteryx australis mantelli and its bearing on ratite affinities. J. Zool. 197, 173–219. doi: 10.1111/jzo.1982.197.2.173
McNab, B. K. (1994). Energy conservation and the evolution of flightlessness in birds. Am. Nat. 144, 628–642. doi: 10.1086/285697
Müller, G. B. (2003). Embryonic motility: environmental influences and evolutionary innovation. Evol. Dev. 5, 56–60. doi: 10.1046/j.1525-142x.2003.03009.x
Navalón, G., Meng, Q., Marugán-Lobón, J., Zhang, Y., Wang, B., Xing, H., et al. (2017). Diversity and evolution of the confuciusornithidae: evidence from a new 131-million-year-old specimen from the huajiying formation in NE china. J. Asian Earth Sci. 152, 12–22. doi: 10.1016/j.jseaes.2017.11.005
O’Connor, J. K., Chiappe, L. M., and Bell, A. (2011). “Pre-modern birds: avian divergences in the Mesozoic,” in Living Dinosaurs: the Evolutionary History of Birds, eds G. D. Dyke and G. Kaiser (Hoboken, NJ: Wiley & Sons), 39–114.
O’Connor, J. K., Erickson, G. M., Norell, M. A., Bailleul, A. M., Hu, H., and Zhou, Z. (2018a). Medullary bone in an early cretaceous enantiornithine bird and discussion regarding its identification in fossils. Nat. Commun. 9:5169. doi: 10.1038/s41467-018-07621-z
O’Connor, J. K., Wang, X.-L., Sullivan, C., Wang, Y., Zheng, X.-T., Hu, H., et al. (2018b). First report of gastroliths in the early cretaceous basal bird Jeholornis. Cretaceous Res. 84, 200–208.
O’Connor, J. K., Wang, M., Zheng, X., Wang, X., and Zhou, Z. (2014). The histology of two female early cretaceous birds. Vertebrata PalAsiatica 52, 112–128.
O’Connor, J. K., and Zhou, Z. (2019). The evolution of the modern avian digestive system: insights from paravian fossils from the yanliao and jehol biotas. Palaeontology 63, 13–27. doi: 10.1111/pala.12453
Oliver, G., De Robertis, E. M., Wolpert, L., and Tickle, C. (1990). Expression of a homeobox gene in the chick wing bud following application of retinoic acid and grafts of polarizing region tissue. EMBO J. 9, 3093–3099. doi: 10.1002/j.1460-2075.1990.tb07506.x
Olson, S. L. (1973). Evolution of the rails of the south atlantic islands (aves: rallidae). Smithsonian Contrib. Zool. 86, 484–485. doi: 10.5479/si.00810282.152
Olson, S. L. (1977). A lower eocene frigatebird from the green river formation of wyoming (pelecaniformes: fregatidae). Smithson. Contrib. Paleobiol. 1–33. doi: 10.5479/si.00810266.35.1 ∗∗v,
Olson, S. L., and Wetmore, A. (1976). Preliminary diagnoses of two extraordinary new genera of birds from pleistocene deposits in the hawaiian islands. Proc. Biol. Soc. Wash. 89, 247–258.
Padian, K., and Lamm, E.-T. (2013). Bone histology of fossil tetrapods: advancing methods, analysis, and interpretation. Berkeley: University of California Press.
Parsons, W. L., and Parsons, K. M. (2009). Further descriptions of the osteology of Deinonychus antirrhopus (saurischia, theropoda). Bull. Buffalo Soc. Nat. Sci. 38, 43–54.
Parsons, W. L., and Parsons, K. M. (2015). Morphological variations within the ontogeny of Deinonychus antirrhopus (theropoda, dromaeosauridae). PLoS One 10:0121476. doi: 10.1371/journal.pone.0121476
Pei, R., Li, Q., Meng, Q., Norell, M. A., and Gao, K.-Q. (2017). New specimens of Anchiornis huxleyi (theropoda: paraves) from the late jurassic of northeastern china. Bull. Am. Museum Nat. His. 2017, 1–67.
Persson, M. (1983). The role of movements in the development of sutural and diarthrodial joints tested by long-term paralysis of chick embryos. J. Anat. 137, 591–599.
Phillips, F. M., Reuben, J., and Wetzel, F. T. (2002). Intervertebral disc degeneration adjacent to a lumbar fusion. J. Bone Joint Sur. 84:6. doi: 10.1302/0301-620X.84B2.0840289
Prondvai, E., Godefroit, P., Adriaens, D., and Hu, D. (2018). Intraskeletal histovariability, allometric growth patterns, and their functional implications in bird-like dinosaurs. Sci. Rep. 8:258. doi: 10.1038/s41598-017-18218-9
Prondvai, E., Stein, K. H. W., de Ricqlès, A., and Cubo, J. (2014). Development-based revision of bone tissue classification: the importance of semantics for science. Biol. J. Linn. Soc. Lond. 112, 799–816. doi: 10.1111/bij.12323
Provini, P., Zhou, Z. H., and Zhang, F. (2008). A new species of the basal bird Sapeornis from the early cretaceous of liaoning, china. Vertebrata PalAsiatica 14, ∗∗,Google Scholar
Pu, H.-Y., Chang, H.-L., Lü, J.-C., Wu, Y.-H., Xu, L., Zhang, J.-M., et al. (2013). A new juvenile specimen of Sapeornis (pygostylia: aves) from the lower cretaceous of northeast china and allometric scaling of this basal bird. Paleontol. Res. 17, 27–38.
Rauhut, O. W. M., Foth, C., and Tischlinger, H. (2018). The oldest Archaeopteryx (theropoda: avialiae): a new specimen from the kimmeridgian/tithonian boundary of schamhaupten, bavaria. PeerJ 6:e4191. doi: 10.7717/peerj.4191
Ray, S., Bandyopadhyay, S., and Appana, R. (2010). “Bone Histology of a Kannemeyeriid Dicynodont Wadiasaurus: Palaeobiological Implications,” in New Aspects of Mesozoic Biodiversity Lecture Notes in Earth Sciences, ed. S. Bandyopadhyay (Berlin, Heidelberg: Springer), 73–89.
Russell, A. P., and Joffe, D. J. (1985). The early development of the quail (Coturnix c. japonica) furcula reconsidered. J. Zool. 206, 69–81.
Sathyamoorthy, O. R., Thirumurugan, R., Senthil Kumar, K., and Jayathangaraj, M. G. (2012). Gross anatomical studies on the sternum and clavicle of spot-billed pelican (Pelecanus philippensis). TN J. Vet. Anim. Sci. 8, 166–170.
Sawad, A. A., Hana, B. A., and Al-Silawi, A. N. (2009). Morphological study of the skeleton development in chick embryo (Gallus domesticus). Int.J. Poul. Sci. 8, 710–714. doi: 10.3923/ijps.2009.710.714
Schwarz, D., Wings, O., and Meyer, C. A. (2007). Super sizing the giants: first cartilage preservation at a sauropod dinosaur limb joint. J. Geol. Soc. 164, 61–65. doi: 10.1144/0016-76492006-019
Schweitzer, M. H. (2011). Soft tissue preservation in terrestrial mesozoic vertebrates. Ann. Rev. Earth Planetary Sci. 39, 187–216. doi: 10.1146/annurev-earth-040610-133502
Shen, C., Lü, J., Gao, C., Hoshino, M., Uesugi, K., and Kundrát, M. (2019). Forearm bone histology of the small theropod Daliansaurus liaoningensis (paraves: troodontidae) from the yixian formation, liaoning, china. His. Biol. 31, 253–261. doi: 10.1080/08912963.2017.1360296
Torres, C. R., Norell, M. A., and Clarke, J. A. (2019). Estimating flight style of early eocene stem palaeognath bird Calciavis grandei (Lithornithidae). Anat. Rec. 303, 1035–1042. doi: 10.1002/ar.24207
Wang, M., O’Connor, J. K., Bailleul, A. M., and Li, Z. (2019a). Evolution and distribution of medullary bone: evidence from a new early cretaceous enantiornithine bird. Natl. Sci. Rev. 7, 1068–1078. doi: 10.1093/nsr/nwz214
Wang, M., O’Connor, J. K., Xu, X., Zhou, Z., O’Connor, J. K., Xu, X., et al. (2019b). A new Jurassic scansoriopterygid and the loss of membranous wings in theropod dinosaurs. Nature 569, 256–259. doi: 10.1038/s41586-019-1137-z
Wang, M., O’Connor, J. K., and Zhou, Z.-H. (2019c). A taxonomical revision of the confuciusornithiformes (aves: pygostylia). Vertebrata PalAsiatica 57, 1–37. doi: 10.19615/j.cnki.1000-3118.180530
Wang, M., Stidham, T. A., and Zhou, Z. (2018). A new clade of basal early cretaceous pygostylian birds and developmental plasticity of the avian shoulder girdle. Proc. Natl. Acad. Sci. 115:201812176. doi: 10.1073/pnas.1812176115
Wang, M., Wang, X., Wang, Y., and Zhou, Z. (2016). A new basal bird from china with implications for morphological diversity in early birds. Sci. Rep. 6, 1–12. doi: 10.1038/srep19700
Wang, M., and Zhou, Z.-H. (2018). A new confuciusornithid (aves: pygostylia) from the early cretaceous increases the morphological disparity of the confuciusornithidae. Zool. J. Linn. Soc. 185, 417–430. doi: 10.1093/zoolinnean/zly045
Wang, W., and O’Connor, J. K. (2017). Morphological coevolution of the pygostyle and tail feathers in early cretaceous birds. Vertebrata PalAsiatica 55, 289–314.
Weimerskirch, H., Chastel, O., Barbraud, C., and Tostain, O. (2003). Frigatebirds ride high on thermals. Nature 421, 333–334. doi: 10.1038/421333a
Williams, M. S. (2003). Developmental anomalies of the scapula the most forgotten bone. Am. J. Med. Genet. 120A, 583–587. doi: 10.1002/ajmg.a.20091
Witten, P. E., and Hall, B. K. (2003). Seasonal changes in the lower jaw skeleton in male atlantic salmon (Salmo salar L.): remodelling and regression of the kype after spawning. J. Anat. 203, 435–450.
Worthy, T. H., Degrange, F. J., Handley, W. D., and Lee, M. S. Y. Y. (2017). The evolution of giant flightless birds and novel phylogenetic relationships for extinct fowl (aves, galloanseres). R. Soc. Open Sci. 4:170975. doi: 10.1098/rsos.170975
Wu, Q., O’Connor, J., Li, Z.-H., and Bailleul, A. M. (2021). Cartilage on the furculae of living birds and the extinct bird Confuciusornis: a preliminary analysis and implications for flight style inferences in Mesozoic birds? Vertebr. Palasiat. 59, 106–124. doi: 10.19615/j.cnki.1000-3118.201222
Xu, X., Wang, X., and Wu, X. (1999). A dromaeosaurid dinosaur with a filamentous integument from the yixian formation of china. Nature 401, 262–266. doi: 10.1038/45769
Xu, X., You, H., Du, K., and Han, F. (2011). An Archaeopteryx-like theropod from China and the origin of Avialae. Nature 475, 465–470. doi: 10.1038/nature10288
Xu, X., Zhao, Q., Norell, M., Sullivan, C., Hone, D., Erickson, G., et al. (2009). A new feathered maniraptoran dinosaur fossil that fills a morphological gap in avian origin. Chinese Sci. Bull. 54, 430–435. doi: 10.1007/s11434-009-0009-6
Xu, X., Zheng, X., Sullivan, C., Wang, X., Xing, L., Wang, Y., et al. (2015). A bizarre Jurassic maniraptoran theropod with preserved evidence of membranous wings. Nature 521, 70–73. doi: 10.1038/nature14423
Young, M., Selleri, L., and Capellini, T. D. (2019). “Genetics of scapula and pelvis development: An evolutionary perspective,” in Current Topics in Developmental Biology Organ Development, ed. D. M. Wellik (Cambridge: Academic Press), 311–349.
Zhang, F., Hou, L., and Ouyang, L. (1998). Osteological microstructure of Confuciusornis: preliminary report. Vertebrata PalAsiatica 36, 126–135.
Zhang, F., Zhou, Z., and Benton, M. J. (2008a). A primitive confuciusornithid bird from china and its implications for early avian flight. Sci. China Ser. D Earth Sci. 51, 625–639. doi: 10.1007/s11430-008-0050-3
Zhang, F., Zhou, Z., Xu, X., Wang, X., Sullivan, C., Zhou, Z., et al. (2008b). A bizarre Jurassic maniraptoran from china with elongate ribbon-like feathers. Nature 455, 1105–1108. doi: 10.1038/nature07447
Zhang, Z., Gao, C., Meng, Q., Liu, J., Hou, L., and Zheng, G. (2009). Diversification in an early cretaceous avian genus: evidence from a new species of Confuciusornis from china. J. Ornithol. 150, 783–790. doi: 10.1007/s10336-009-0399-x
Zheng, X., O’Connor, J. K., Wang, X., Wang, M., Zhang, X., and Zhou, Z. (2014). On the absence of sternal elements in Anchiornis (paraves) and Sapeornis (aves) and the complex early evolution of the avian sternum. Proc. Natl. Acad. Sci. 111, 13900–13905. doi: 10.1073/pnas.1411070111
Zhou, Z., and Zhang, F. (2002a). A long-tailed, seed-eating bird from the early cretaceous of china. Nature 418, 405–409. doi: 10.1038/nature00930
Zhou, Z., and Zhang, F. (2002b). Largest bird from the early cretaceous and its implications for the earliest avian ecological diversification. Naturwissenschaften 89, 34–38. doi: 10.1007/s00114-001-0276-9
Zhou, Z., and Zhang, F. (2003a). Anatomy of the primitive bird Sapeornis chaoyangensis from the early cretaceous of liaoning, china. Can. J. Earth Sci. 40, 731–747. doi: 10.1139/e03-011
Keywords: Confuciusornis, histology, scapulocoracoid, pectoral girdle, cretaceous birds
Citation: Wu Q, Bailleul AM, Li Z, O’Connor J and Zhou Z (2021) Osteohistology of the Scapulocoracoid of Confuciusornis and Preliminary Analysis of the Shoulder Joint in Aves. Front. Earth Sci. 9:617124. doi: 10.3389/feart.2021.617124
Received: 14 October 2020; Accepted: 25 March 2021;
Published: 13 April 2021.
Edited by:
Haijun Song, China University of Geosciences, ChinaReviewed by:
Francisco José Serrano, Natural History Museum of Los Angeles County, United StatesCopyright © 2021 Wu, Bailleul, Li, O’Connor and Zhou. This is an open-access article distributed under the terms of the Creative Commons Attribution License (CC BY). The use, distribution or reproduction in other forums is permitted, provided the original author(s) and the copyright owner(s) are credited and that the original publication in this journal is cited, in accordance with accepted academic practice. No use, distribution or reproduction is permitted which does not comply with these terms.
*Correspondence: Qian Wu, d3VxaWFuQGl2cHAuYWMuY24=; Jingmai O’Connor, amluZ21haS5vY29ubm9yQGdtYWlsLmNvbQ==
Disclaimer: All claims expressed in this article are solely those of the authors and do not necessarily represent those of their affiliated organizations, or those of the publisher, the editors and the reviewers. Any product that may be evaluated in this article or claim that may be made by its manufacturer is not guaranteed or endorsed by the publisher.
Research integrity at Frontiers
Learn more about the work of our research integrity team to safeguard the quality of each article we publish.