- 1School of Atmospheric Sciences, Nanjing University of Information Science and Technology, Nanjing, China
- 2Department of Atmospheric Science, School of Environmental Studies, China University of Geosciences, Wuhan, China
- 3Shangqiu Meteorological Bureau, Shangqiu, China
- 4School of Electronic Engineering, Nanjing Xiaozhuang University, Nanjing, China
- 5Key Laboratory of Meteorological Disaster, Ministry of Education/Joint International Research Laboratory of Climate and Environment Change/Collaborative Innovation Center on Forecast and Evaluation of Meteorological Disaster, Nanjing University of Information Science and Technology, Nanjing, China
- 6School of Aeronautics and Astronautics, Shanghai Jiaotong University, Shanghai, China
- 7Reading Academy, Nanjing University of Information Science and Technology, Nanjing, China
We studied the effects of the stationary and transient transport of ozone in the upper troposphere and lower stratosphere (UTLS) on the ozone valley over the Tibetan Plateau (OVTP) in summer using the daily ERA-Interim reanalysis dataset for the time period 1979–2016. We used the Lorenz circulation decomposition method to separate the stationary and transient transport of ozone into terms related to either the mean flow or eddies. The decrease in the total ozone concentration in summer is associated with the transport of ozone, which, in turn, reinforces the OVTP. The zonal (meridional) transport of ozone, which combines stationary and transient transport, strengthens (weakens) the ozone valley. The stationary zonal (meridional) transport of ozone strengthens (weakens) the ozone valley. The transient zonal (meridional) transport of ozone weakens (strengthens) the ozone valley, but this effect is weaker than that of stationary transport. The mean flow has the dominant role, especially in the stationary component. The effect of eddies on the zonal transient transport of ozone is as strong as that of the mean flow. For stationary transport, the zonal deviation of ozone transported by the zonal mean flow in the zonal (meridional) direction
Introduction
Ozone is an important topic in atmospheric research (Cong et al., 2001). The ozone layer in the stratosphere has an important role in the thermal structure of the atmosphere (Andrews et al., 1987) because it absorbs ultraviolet radiation, modifying the thermal and dynamic structure of the atmosphere (Kerr et al., 1993; Lubin and Jensen, 1995; IPCC, 2007; Chipperfield et al., 2015; Xie et al., 2018). This, in turn, can affect aerodynamic, physical and chemical processes in both the troposphere and stratosphere (Farman et al., 1985; Forster and Shine, 1997; Xie et al., 2008; Shi et al., 2010; Wang et al., 2013).
Since the discovery of the Antarctic ozone hole in 1984 (Farman et al., 1985), the depletion of stratospheric ozone and its impact on the Earth’s climate have attracted concern worldwide (Solomon, 1999; Zhou and Zhang, 2005; Crutzen and Oppenheimer, 2008; Tobo et al., 2008; Karpechko et al., 2014; Zhang et al., 2014; Hu et al., 2015; Zhang et al., 2019). The effects of the polar ozone hole on the Earth’s climate have been widely studied. (Hartmann et al., 2000; Pienitz and Vincent, 2000; Ungar, 2000; Perlwitz et al., 2008). Outside the polar regions, strong ozone depletion also occurs over the Tibetan Plateau (Reiter and Gao, 1982; Hingane, 1990). The Tibetan Plateau, also known as the third pole (Xu and Chen, 2005), is the highest plateau on Earth, with an average elevation >4,000 m. The unique topography of the Tibetan Plateau means that the dynamic and thermal properties of ozone in this region are different from those in the surrounding atmosphere, which has a significant impact on the Earth’s climate (Zhang and Chen, 2005; Wang et al., 2006; Wang et al., 2007). Reiter and Gao (1982) found that a center of relatively low total ozone concentrations appears over the Tibetan Plateau when the South Asian High (SAH) moves over the plateau and is maintained in mid-April.
The accuracy of ozone measurements is constantly improving with developments in satellite technology. Stolarski et al. (2013) investigated the seasonal distribution of ozone at mid-latitudes in the northern hemisphere using data from the Total Ozone Mapping Spectrometer (TOMS) onboard NASA’s Earth Probe satellite and found that the trend at 50°N changes from about −0.8% year−1 in winter and early spring to about −0.2% year−1 in summer. Based on more accurate TOMS ozone data, Zhou et al., 1995) found that the total column ozone over the Tibetan Plateau in the half-year of the boreal summer (April–September) is > 5 DU lower than that over eastern China at the same latitude. This center of low ozone concentrations is referred to as the ozone valley over the Tibetan Plateau (OVTP). Zou (1996) confirmed the existence of the OVTP and found that the total column ozone was about 30 DU lower than the zonal mean at the same latitude.
The mechanism of the formation of the summer OVTP was first speculated by Zhou et al. (1995), who suggested that mass transport caused by local ascending motion and the related chemical processes were the main cause of the OVTP. Most subsequent research has suggested that the dynamic transport associated with large-scale circulation patterns plays a major part in the formation of the OVTP in the upper troposphere and lower stratosphere (UTLS), the role of chemical processes is secondary (Guo and Xu, 1986; Su and Wang, 2000; Liu et al., 2003; Liu et al., 2003; Zhou and Chen, 2005; Wan et al., 2007; Tian et al., 2008; Bian et al., 2011; Guo et al., 2012). Zou (1996) found a significant negative correlation between the surface heat flux and the ozone deficit, which implies a combined effect of the chemical and dynamic processes associated with the surface-air heating over the Tibetan Plateau. The absence of about 4 km of the ozone partial column compared with non-mountainous terrains at the same latitude may contribute to the formation of the OVTP (Tian et al., 2009; Bian et al., 2011; Guo et al., 2016).
The dynamic transport processes associated with the atmospheric circulation are important in the global distribution of ozone (WMO, 1985). The Tibetan Plateau is a geographic high and is an elevated heat source associated with thermally forced circulation patterns. Updraft and convective activities provide a favorable circulation background for the emergence of a center of low ozone concentrations (Ye and Xu, 2003). Liu et al. (2003) simulated the OVTP in summer using a three-dimensional chemical transport model (OSLO CTM2). They found that transport processes have a key role in the formation of the OVTP in summer, whereas chemical processes compensate for the reduction in ozone via transport processes. Zhou et al. (2004), using a chemical transport model, showed that horizontal and vertical transport are the main reasons for the center of low ozone concentrations over the Tibetan Plateau in summer and that the effect of horizontal transport in convective transport is more dominant than that of vertical transport. Guo et al. (2012) studied the dynamic effects of the 150–50 hPa SAH on the OVTP by analyzing the meridional, zonal and vertical transport with the WACCM3 model and found that the seasonal variation of the OVTP changes significantly with the SAH. Dynamic processes have an important effect on the OVTP in summer (WMO, 1985; Ye and Xu, 2003; Liu et al., 2003; Zhou et al., 2004; Zhou and Zhang, 2005; Guo et al., 2012; Guo et al., 2016).
Previous studies of the horizontal transport of ozone have mainly focused on stationary transport in the climatological mean state (Zhou et al., 2004; Guo et al., 2012) and little attention has been paid to the effects of transient transport, which is usually used in the study of synoptic processes. The effect of transient transport is therefore underestimated in the studies of the effect of ozone transport on climate. Mak (1978) and Trenberth (1987) considered that the magnitudes of stationary and transient eddies were almost the same. However, Kraucunas and Hartmann (2005) suggested that the contribution of stationary eddies was smaller than that of transient eddies. An analysis of both stationary and transient transport from the point of view of the mean flow and eddies is therefore important in determining the possible mechanisms of changes in ozone concentrations over the Tibetan Plateau. Changes in transient transport can be studied better using data with a high spatial and temporal resolution.
This study focuses on the climate characteristics of the two types of ozone transport over the Tibetan Plateau. Lorenz decomposition of the atmospheric circulation is used to help understand the characteristics of ozone transport over the Tibetan Plateau and to explain its impact on the OVTP. This method has been widely applied to the study of transport characteristics (Starr and White, 1951; Ye and Deng, 1956; Newell et al., 1972; Trenberth, 1987; Yi and Tao, 1997; Wang et al., 2007; Egger and Hoinka, 2011; Yang et al., 2014). However, the decomposition used here is more exhaustive than that used in previous studies and is based on both the mean flow and eddies, which are both important in the better study of ozone transport.
This paper is organized as follows. Materials and Methods Section describes the ERA-Interim reanalysis dataset and Lorenz decomposition method. The analysis of climate characteristics of stationary and transient transport in summer is presented in Results Section. Conclusions and Discussion Section provides our conclusions and discussions.
Materials and Methods
Data
ERA-Interim Reanalysis Dataset
The ERA-Interim reanalysis dataset from the European Center for Medium-Range Weather Forecasts (ECMWF) is produced using a four-dimensional variational approach and has a high horizontal resolution, good background error constraints, modified variances in the satellite radiation data and improved rapid radiative transfer (Dee et al., 2011). Both the pattern and data assimilation systems have improved since the earlier ERA-40 dataset. The time span has been continuously updated since 1979 and there are 14 atmospheric parameters for 37 standard pressure levels ranging from 1,000 to 1 hPa. The horizontal resolution and the data range of ERA-Interim dataset are optional. Previous studies have shown that the ERA-Interim ozone data compare well with ozone observations from satellites and can be used to analyze changes in the ozone flux (Dragani, 2011; Skerlak et al., 2013). The daily ERA-Interim dataset has a higher resolution than the satellite data, which helps the study of transient transport.
We analyzed the daily ERA-Interim reanalysis data for the ozone concentration and horizontal wind fields from 1979 to 2016 in summer (June–August) at a horizontal resolution of (0.75 ° × 0.75 °).
Methods
Lorenz Decomposition of Atmospheric Circulation
There are three decompositions of the atmospheric circulation: 1) in the time domain; 2) in the space domain; and 3) in the space–time domain. The decomposition of a physical quantity A in the time domain is
where
The decomposition in the space domain is
where
Flux is defined as the physical variable “transport” caused by atmospheric motion per unit time through a unit area perpendicular to the direction of motion. For example, if A represents the physical quantity and u represents the eastward component of the wind, then Au is the eastward transport flux of the physical quantity. The eastward flux is positive and the westward flux is negative.
The time decomposition of time-averaged flux Au yields:
where
If we take the ozone zonal transport flux as an example (meridional transport is the same, but for v), we can obtain the following.
Four Items of Stationary Transport
Four Items of Transient Transport
Local changes in the ozone concentration are caused by individual changes in the ozone concentration, horizontal transport and vertical transport. The individual variations in ozone concentration include both dynamic and chemical impacts. The dynamic effects have the most important roles in the formation of the OVTP in the UTLS region (Liu et al., 2003; Tian et al., 2008; Bian et al., 2011; Guo et al., 2012), whereas chemical processes have more impact on the ozone concentration over the Tibetan Plateau in the stratosphere (Wan et al., 2007; Liu et al., 2011; Zhang et al., 2014; Guo et al., 2015). We therefore focused on the dynamic effects regardless of the chemical influences in the UTLS region. The local change in the ozone concentration can be approximated by the continuity equation:
where
where
If we combine Eqs. 6–13, 15, we obtain Eq. 16:
Then we define
where
Results
Characteristics of Ozone Transport in the OVTP
The profiles in Figure 1 show the change of ozone caused by zonal stationary
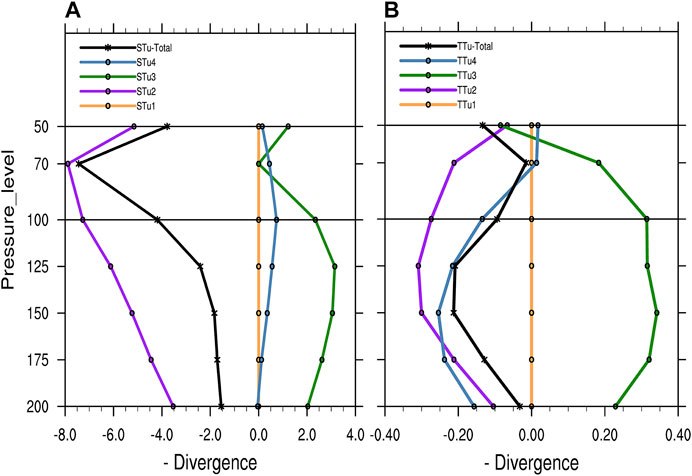
FIGURE 1. Profiles of the change in zonal ozone transport (negative divergence,
Figure 1 shows that the stationary transport of zonal ozone reduces the ozone concentration over the Tibetan Plateau and enhances the OVTP. The transient transport of zonal ozone also reduces the ozone concentration, but the effect in strengthening the OVTP is weaker. The divergence of STu1 and TTu1 in Figure 1 is zero. The stationary transport of the zonal deviation of ozone (Figure 1A) by the zonal mean flow
Both stationary and transient transport strengthen the ozone valley in the zonal direction. The mean flow has the dominant role in zonal transport, especially in the stationary part, whereas the eddies have important roles in transient transport, similar to the mean flow in the zonal direction.
Figure 2 shows the profiles of the change of ozone by
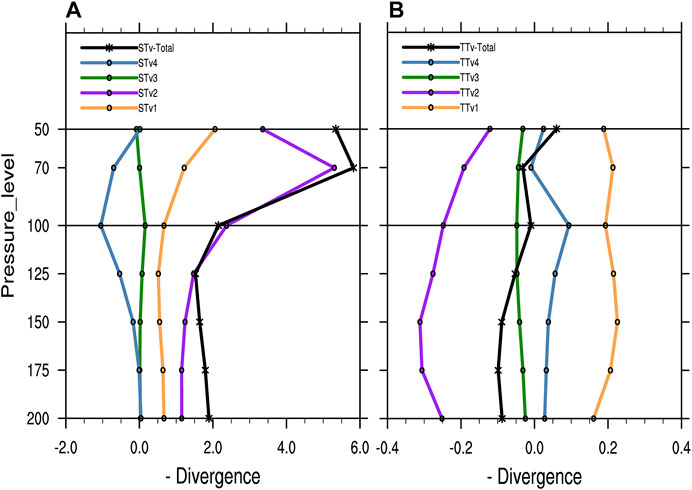
FIGURE 2. Similar to Figure 1, but for meridional ozone transports (v wind).
The effect of stationary transport on the ozone concentration is more significant than that of transient transport, which weakens the ozone valley in the meridional direction. The impact of the mean flow in the meridional direction is as important as that in the zonal transport component. Eddies also contribute to transient transport.
Figure 3 shows the combination of stationary and transient transport in the zonal and meridional directions. The combined effect of all ozone transport in the UTLS region in summer is to reduce the ozone concentration and enhance the ozone valley. The zonal ozone transport (u-Total) strengthens the ozone valley, whereas the meridional ozone transport (v-Total) weakens it; the impact of u-Total is higher than the impact of v-Total (Figure 3). This shows that stationary transport has a major role in altering the ozone valley, whereas the effect of transient transport is relatively weak. The contribution of the mean flow has the key role in both stationary and transient transport. The effect of eddies is important in transient transport, especially in the zonal direction, where it is as strong as the mean flow (Figure 1B).
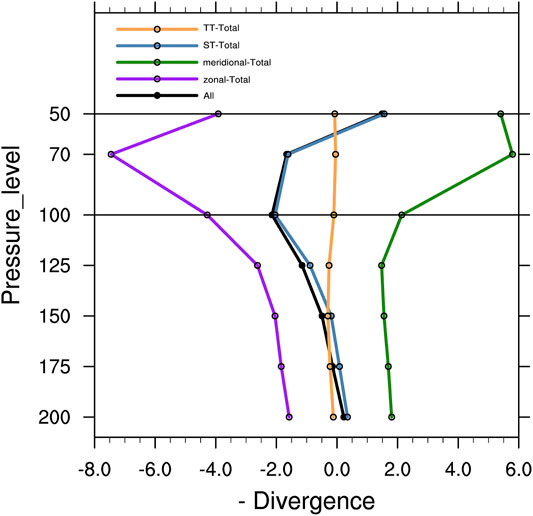
FIGURE 3. Profiles of the change in ozone transport in the UTLS region in summer (units: 1013 m·kg·s−1·kg−1). The yellow, blue, purple and green lines indicate the total transient, stationary, zonal and meridional transport, respectively. The black line indicates the total effect of ozone transports.
Climate Characteristics of Stationary Ozone Transport in Summer
To further study the detailed effects of the different stationary transport components, Figure 4 shows the pressure–latitude cross-section of the change in the zonal ozone stationary transport components
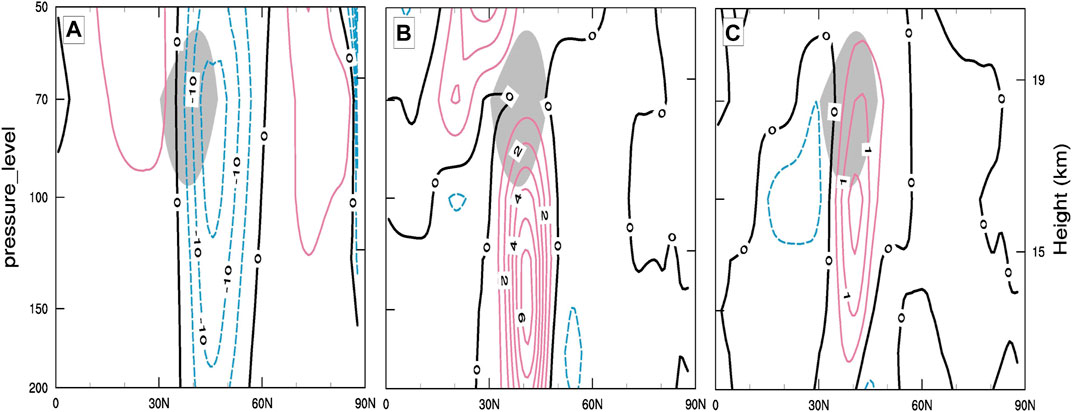
FIGURE 4. Pressure–latitude cross-section of the change in zonal ozone stationary transport by (A)
Figure 4A shows that
Figure 5 shows the change in the meridional ozone stationary transport components
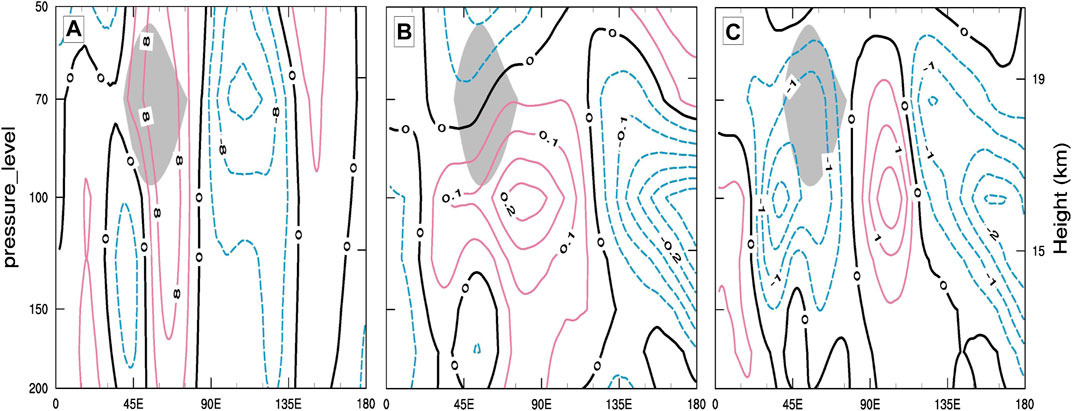
FIGURE 5. Similar to Fig.4, but for the change in meridional ozone stationary transport by (A)
The magnitude of the mean flow components are significantly larger than the other components in stationary transport, which affects the ozone concentration in the center of the OVTP. The zonal component
Climate Characteristics of Transient Ozone Transport in Summer
Figure 6 shows the climate characteristics of the zonal ozone transient transport components
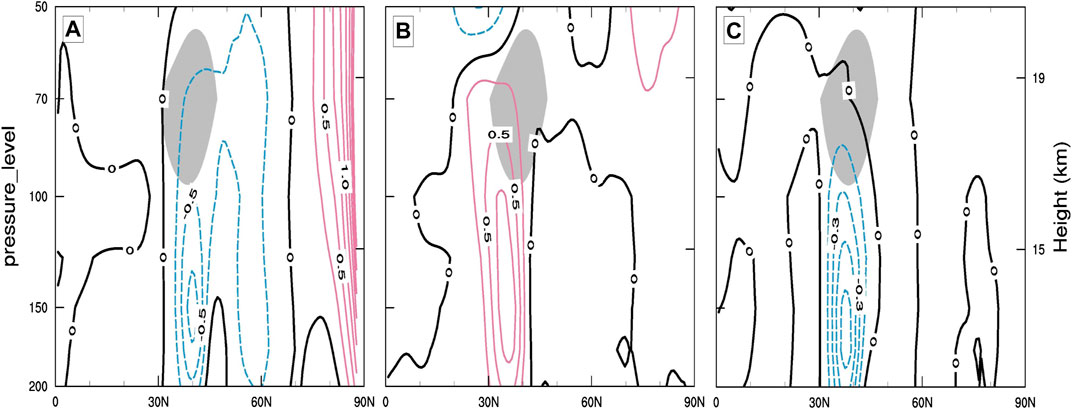
FIGURE 6. Pressure–latitude cross-section of the change in zonal ozone transient transport by (A)
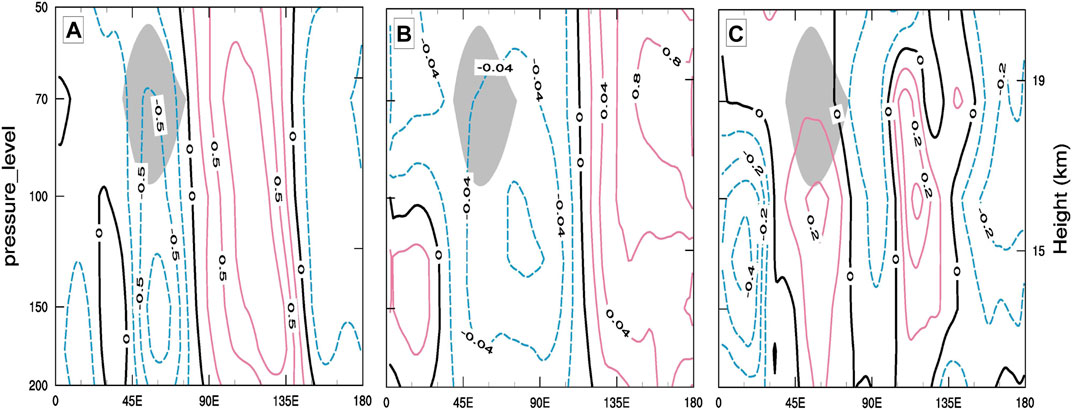
FIGURE 7. Similar to Fig.6, but for the change in meridional ozone transient transport by (A)
The contribution of the zonal deviation of ozone by the mean flow is concordant (Figures 4, 6). Both stationary and transient transport reduce the ozone concentration (Figures 4A, 6A). The effect of eddies on the zonal mean ozone are similar and weaken the OVTP (Figures 4B, 6B). There are a few differences between the
Stationary and transient transport may have different roles in the formation of the ozone valley. They contribute consistently to zonal transport, but inversely to meridional transport, which explains why the effect of the total zonal transport is stronger than the total meridional transport. Different components of transient transport may have different effects—for example, both the zonal and meridional deviations of ozone by the zonal mean flow (
Conclusions and Discussion
Conclusions
This study focused on the climate characteristics of the stationary and transient transport of ozone and their effects on the OVTP in the UTLS region in summer using the ERA-Interim dataset and Lorenz decomposition of the atmospheric circulation. Our main results are as follows.
1) The stationary zonal transport of ozone enhances the ozone valley, whereas the stationary meridional transport weakens it. The transient zonal transport of ozone strengthens the ozone valley and the transient meridional transport also slightly strengthens it. The effect of the total stationary transport (zonal or meridional) is much stronger than that of the total transient transport (zonal or meridional) and the mean flow, especially the stationary part, has the dominant role. The effect of eddies on transient transport is as strong as that of the mean flow in zonal transient transport.
2) The total ozone transport strengthens the ozone valley, with the total stationary transport playing the major part, whereas the total transient transport slightly weakens the ozone valley in the UTLS region in summer. The total zonal ozone transport combining stationary and transient transport enhances the ozone valley; by contrast, the total meridional ozone transport weakens it.
3) Both of the components of the mean flow (
4) The contributions of stationary and transient transport are consistent with the zonal transport, but are opposite to the meridional transport. The impact of transient transport on the formation and maintenance of the OVTP is not negligible.
Discussion
We analyzed the climate characteristics of the stationary and transient transport of ozone and their effects on the OVTP in the UTLS region in summer. The detailed physical mechanisms associated with ozone transport require further study. The stationary and transient transport are separated into terms related to the mean flow or eddies using Lorenz decomposition of the atmospheric circulation. The effect of transient transport is much weaker than stationary transport on the OVTP whereas it is non-negligible, which could have more impacts on the extreme climate. The effects of the mean flow may be caused by the west jet stream. Previous research has shown that the SAH is one of the major factors affecting the OVTP of the dynamic process in summer (Su and Wang, 2000; Liu et al., 2003; Tian et al., 2008; Bian et al., 2011; Guo et al., 2012; Qin et al., 2018). We speculate that the SAH may have the key role in the effect of eddies. The stationary and transient transport of ozone by eddies may be influenced by the climatological and abnormal SAH, respectively. The variation in the distribution of ozone concentrations also influences the ozone transport flux.
Data Availability Statement
The datasets presented in this study can be found in online repositories. The names of the repository/repositories and accession number(s) can be found below: https://apps.ecmwf.int/datasets/.
Author Contributions
DG initiated and coordinated the work. WX provided the calculation and analysis of ozone transport. WX, QS, and YL wrote the manuscript. CS, BS, YS, ZT, and YD gave valuable suggestions for revisions.
Funding
This work was jointly supported by the National Natural Science Foundation of China (41675039, 91837311 and 41875048).
Conflict of Interest
The authors declare that the research was conducted in the absence of any commercial or financial relationships that could be construed as a potential conflict of interest.
Acknowledgments
We thank ECMWF for the meteorological data (available at https://apps.ecmwf.int/datasets/). We are grateful to the High Performance Computing Center of Nanjing University of Information Science and Technology for carrying out the numerical calculations in this paper using its blade cluster system.
References
Andrews, D. G., Holton, J. R., and Leovy, C. B. (1987). Middle atmosphere dynamics. New York, NY: Academic Press, 489.
Bian, J., Yan, R., Chen, H., Lü, D., and Massie, S. T. (2011). Formation of the summertime ozone valley over the Tibetan Plateau: the Asian summer monsoon and air column variations. Adv. Atmos. Sci. 28 (6), 1318–1325. doi:10.1007/s00376-011-0174-9
Chipperfield, M. P., Dhomse, S. S., Feng, W., McKenzie, R. L., Velders, G. J., and Pyle, J. A. (2015). Quantifying the ozone and ultraviolet benefits already achieved by the Montreal Protocol. Nat. Commun. 6, 7233. doi:10.1038/ncomms8233
Cong, C. H., Li, W. L., and Zhou, X. J. (2001). Exchange of atmospheric mass between stratosphere and troposphere over the Tibetan Plateau and its adjacent regions. Acta Meteorol. Sinica. 46 (22), 1914–1918. [in Chinese]. doi:10.3321/j.issn:0023-074X.2001.22.017
Crutzen, P. J., and Oppenheimer, M. (2008). Learning about ozone depletion. Climatic Change 89 (1–2), 143–154. doi:10.1007/s10584-008-9400-6
Dee, D. P., Uppala, S. M., Simmons, A. J., Berrisford, P., Poli, P., Kobayashi, S., et al. (2011). The ERA-Interim reanalysis: configuration and performance of the data assimilation system. Q. J. R. Meteorol. Soc. 137 (656), 553–597. doi:10.1002/qj.828
Dragani, R. (2011). On the quality of the ERA-Interim ozone reanalyses: comparisons with satellite data. Q. J. R. Meteorol. Soc. 137, 1312–1326. doi:10.1002/qj.821
Egger, J., and Hoinka, K.-P. (2011). Global angular momentum fluxes in height coordinates. Mon. Wea. Rev. 139 (8), 2552–2560. doi:10.1175/2010MWR3514.1
Farman, J. C., Gardiner, B. G., and Shanklin, J. D. (1985). Large losses of total ozone in Antarctica reveal seasonal ClOx/NOx interaction. Nature 315 (6016), 207–210. doi:10.1038/315207a0
Forster, P., and Shine, K. (1997). Radiative forcing and temperature trends from stratospheric ozone changes. J. Geophys. Res. 102, 10841–10855. doi:10.1029/96JD03510
Guo, D., Su, Y. C., Zhou, X. J., Xu, J. J., Shi, C. H., Liu, Y., et al. (2016). Evaluation of trend uncertainty of summer ozone valley over Tibetan Plateau in three reanalysis datasets. J. Meteorol. Res. 31 (2), 431–437. doi:10.1007/s13351-017-6058-x
Guo, D., Su, Y., Shi, C., Xu, J., and Powell, A. M. (2015). Double core of ozone valley over the Tibetan Plateau and its possible mechanisms. J. Atmos. Sol. Terr. Phys. 130-131, 127–131. doi:10.1016/j.jastp.2015.05.018
Guo, D., Wang, P., Zhou, X., Liu, Y., and Li, W. (2012). Dynamic effects of the south Asian high on the ozone valley over the Tibetan Plateau. Acta Meteorol. Sin. 26 (2), 216–228. doi:10.1007/s13351-012-0207-2
Guo, S. C., and Xu, Y. H. (1986). The possible contribution of the large scale topographic heating to the discontinuous feature of ozonosphere meridional effect over Asia. J. Yunnan Univ. (Natural Sci. edition) (4), 87–93. [in Chinese].
Hartmann, D. L., Wallace, J. M., Limpasuvan, V., Thompson, D. W., and Holton, J. R. (2000). Can ozone depletion and global warming interact to produce rapid climate change?. Proc. Natl. Acad. Sci. United States 97 (4), 1412–1417. doi:10.1073/pnas.97.4.1412
Hingane, L. S. (1990). ozone valley in the subtropics. J. Atmos. Sci. 47 (14), 1814–1816. doi:10.1175/1520-0469(1990)047<1814:OVITS>2.0.CO;2
Hu, D., Tian, W., Xie, F., Wang, C., and Zhang, J. (2015). Impacts of stratospheric ozone depletion and recovery on wave propagation in the boreal winter stratosphere. J. Geophys. Res. Atmos. 120, 8299–8317. doi:10.1002/2014JD022855
IPCC (2007). Climate Change 2007: a comprehensive report. Cambridge, UK: Cambridge University Press.
Karpechko, A. Y., Perlwitz, J., Manzini, E., and Manzini, E. (2014). A model study of tropospheric impacts of the Arctic ozone depletion 2011. J. Geophys. Res. Atmos. 119, 7999–8014. doi:10.1002/2013JD021350
Kerr, J. B., Mcelroy, C. T., and McElroy, C. T. (1993). Evidence for large upward trends of ultraviolet-B radiation linked to ozone depletion. Science 262, 1032–1034. doi:10.1126/science.262.5136.1032
Kraucunas, I., and Hartmann, D. L. (2005). Equatorial superrotation and the factors controlling the zonal-mean zonal winds in the tropical upper troposphere. J. Atmos. Sci. 62 (2), 371–389. doi:10.1175/JAS-3365.1
Liu, Y., Li, W., Zhou, X., and He, J. (2003). Mechanism of formation of the ozone valley over the Tibetan Plateau in summer- transport and chemical process of ozone. Adv. Atmos. Sci. 20 (1), 103–109. doi:10.1007/BF03342054
Liu, Y., Liu, C., Tie, X., and Gao, S. (2011). Middle stratospheric polar vortex ozone budget during the warming Arctic winter, 2002–2003. Adv. Atmos. Sci. 28 (5), 985–996. doi:10.1007/s00376-010-0045-9
Lorenz, E. N. (1967). The nature and theory of the general circulation of the atmosphere. New York, NY: World Meteorological Organization, 161.
Lubin, D., and Jensen, E. H. (1995). Effects of clouds and stratospheric ozone depletion on ultraviolet radiation trends. Nature 377, 710–713. doi:10.1038/377710a0
Mak, M. (1978). On the observed momenum flux by standing eddies. J. Atmos. 35 (2), 340–348. doi:10.1175/1520-0469(1978)035<0340:OTOMFB>2.0.CO;2
Newell, R. E., Kidson, J. W., Vincent, D. G., and Boer, G. J. (1972). General circulation of the tropical atmosphere and interactions with extratropical latitudes. Cambridge, UK: The Massachusetts Institute of Technology, 258.
Perlwitz, J., Pawson, S., Fogt, R. L., Nielsen, J. E., and Neff, W. D. (2008). Impact of stratospheric ozone hole recovery on Antarctic climate. Geophys. Res. Lett. 35 (8), 135–157. doi:10.1029/2008gl033317
Pienitz, R., and Vincent, W. F. (2000). Effect of climate change relative to ozone depletion on UV exposure in subarctic lakes. Nature 404 (6777), 484–487. doi:10.1038/35006616
Qin, H., Guo, D., Shi, C. H., Li, Z. K., Zhou, S. W., Huang, Y., et al. (2018). The interaction between variations of South Asia high and ozone in the adjacent regions. Chin. J. Atmos. Sci. 42 (2), 421–434. [in Chinese]. doi:10.3878/j.issn.1006-9895.1710.17159
Reiter, E. R., and Gao, D. Y. (1982). Heating of the tibet plateau and movements of the South asian high during spring. Mon. Weather Rev. 110 (11), 1694–1711. doi:10.1175/1520-0493(1982)110<1694:HOTTPA>2.0.CO;2
Shi, C. H., Chen, Y. J., and Zheng, B. (2010). A comparison of the contributions of dynamical transportation and photochemical process to ozone’s seasonal variation in the stratosphere. Chin. J. Atmos. Sci. 59 (4), 310–339. [in Chinese]. doi:10.1158/0008-5472.SABCS11-P5-17-05
Skerlak, B., Sprenger, M., and Wernli, H. (2013). A global climatology of stratosphere-troposphere exchange using the ERAinterim dataset from 1979 to 2011. Atmos. Chem. Phys. Discuss. 13, 11537–11595. doi:10.5194/acp-14-913-2014
Solomon, S. (1999). Stratospheric ozone depletion: a review of concepts and history. Rev. Geophys. 37 (3), 275–316. doi:10.1029/1999RG900008
Starr, V. P., and White, R. M. (1951). A hemispherical study of the atmospheric angular-momentum balance. Q.J R. Met. Soc. 77 (332), 215–225. doi:10.1002/qj.49707733206
Stolarski, R. S., Bloomfield, P., Mcpeters, R. D., and Herman, J. R. (1991). Total ozone trends deduced from nimbus 7 toms data. Geophys. Res. Lett. 18 (6), 1015–1018. doi:10.1029/91GL01302
Su, S. J., and Wang, W. G. (2000). The relation between the total ozone variation in Asia and the South Asian high. J. Yunnan Univ. (Natural Sci. edition) 22 (4), 293–296. doi:10.3321/j.issn:0258-7971.2000.04.013
Tian, W., Chipperfield, M., and Huang, Q. (2008). Effects of the Tibetan Plateau on total column ozone distribution. Tellus B: Chem. Phys. Meteorology 60 (4), 622–635. doi:10.1111/j.1600-0889.2008.00338.x
Tian, W., Chipperfield, M. P., and Lü, D. (2009). Impact of increasing stratospheric water vapor on ozone depletion and temperature change. Adv. Atmos. Sci. 26 (3), 423–437. doi:10.1007/s00376-009-0423-3
Tobo, Y., Iwasaka, Y., Zhang, D., Shi, G., Kim, Y.-S., Tamura, K., et al. (2008). Summertime “ozone valley” over the Tibetan Plateau derived from ozonesondes and EP/TOMS data. Geophys. Res. Lett. 35 (16), 1–5. doi:10.1029/2008GL034341
Trenberth, K. E. (1987). The role of eddies in maintaining the westerlies in the southern hemisphere winter. J. Atmos. Sci. 44 (11), 1498–1508. doi:10.1175/1520-0469(1987)044<1498:TROEIM>2.0.CO;2
Ungar, S. (2000). Knowledge, ignorance and the popular culture: climate change versus the ozone hole. Public Underst. Sci. 9 (3), 297–312. doi:10.1088/0963-6625/9/3/306
Wan, L. F., Guo, D., Liu, R. Q., Shi, C. H., and Su, Y. C. (2007). Evaluation of the WACCM3 performance on simulation of the double core of ozone valley over the Qinghai-Xizang Plateau in summer. Plateau Meteorol. 36 (1), 57–66. doi:10.7522/j.issn.1000-0534.2016.00004
Wang, L., Chen, W., Huang, R. H., and Huang, G. (2007). The climatology and annual cycle of meridional transport of westerly momentum by stationary waves in the Northern Hemisphere. Chin. J. Atmos. Sci. 31 (3), 377–388. [in Chinese]. doi:10.3878/j.issn.1006-9895.2007.03.01
Wang, L., Kushner, P. J., and Waugh, D. W. (2013). Southern hemisphere stationary wave response to changes of ozone and greenhouse gases. J. Clim. 26, 10205–10217. doi:10.1175/JCLI-D-13-00160.1
Wang, W.-G., Fan, W.-X., Wu, J., Xie, Y.-Q., Yuan, M., Chen, X.-M., et al. (2006). A study of spatial-temporal evolvement of the global cross-tropopause ozone mass flux. Chin. J. Geophys. 49 (6), 1451–1466. doi:10.1002/cjg2.972
WMO (1985). Atmosphere ozone 1985. Assessment of our understanding of Process controlling its distribution and change, Global ozone Research and Monitoring Project. Washington, DC: National Aeronautics and Space Administration, 331–343.
Xie, F., Li, J., Sun, C., Ding, R., Xing, N., Yang, Y., et al. (2018). Improved global surface temperature simulation using stratospheric ozone forcing with more accurate variability. Sci. Rep. 8, 14474. doi:10.1038/s41598-018-32656-z
Xie, F., Tian, W., and Chipperfield, M. P. (2008). Radiative effect of ozone change on stratosphere-troposphere exchange. J. Geophys. Res. 113, D00B09. doi:10.1029/2008JD009829
Xu, X. K., and Chen, H. (2005). The spatiotemporal distribution of land surface features in the Tibetan plateau. Clim. Environ. Res. 10 (3), 409–420. doi:10.3969/j.issn.1006-9585.2005.03.014
Yang, W., Wang, P. X., He, J. H., Li, L. P., and Cheng, L. P. (2014). Climatic characteristics of relative atmospheric angular momentum transport and its relationship with jet streams. Chin. J. Atmos. Sci. 38 (2), 363–372. [in Chinese]. doi:10.3878/j.issn.1006-9895.2013.13101
Ye, D. Z., and Deng, G. Y. (1956). Mean meridional circulation and angular momentum balance in the year of 1950. Acta Meteorol. Sin. 27 (4), 307–321. [in Chinese]. doi:10.11676/qxxb1956.022
Ye, Z., and Xu, Y. F. (2003). Climate characteristics of ozone over Tibetan Plateau. J. Geophys. Res. 108. doi:10.1029/2002JD003139
Yi, L., and Tao, S. Y. (1997). Role of the standing and the transient eddies in atmospheric in atmospheric water cycle in the Asian monsoon region. Acta Meteorol. Sin. 55 (5), 532–544. [in Chinese]. doi:10.11676/qxxb1997.053
Zhang, J., Tian, W., Xie, F., Sang, W., Guo, D., Chipperfield, M., et al. (2019). Zonally asymmetric trends of winter total column ozone in the Northern middle latitudes. Clim. Dyn. 52 (7-8), 4483–4500. doi:10.1007/s00382-018-4393-y
Zhang, J., Tian, W., Xie, F., Tian, H., Luo, J., Zhang, J., et al. (2014). Climate warming and decreasing total column ozone over the Tibetan Plateau during winter and spring. Tellus B: Chem. Phys. Meteorology 66 (1), 23415. doi:10.3402/tellusb.v66.23415
Zhou, G. H., Wang, P. X., Shi, N., Li, Q. P., and She, G. J. (2009). An analysis of the anomaly of stationary waves in the Northern Hemisphere 500 hPa height field. Acta Meteorol. Sin. 67 (2), 298–306. [in Chinese]. doi:10.1016/S1003-6326(09)60084-4
Zhou, R. J., and Chen, Y. J. (2005). Ozone variations over the Tibetan and Iranian plateaus and their relationship with the South asia high. J. Univ. Sci. Technol. 35 (6), 899–908. doi:10.1360/biodiv.050028
Zhou, S., and Zhang, R. (2005). Decadal variations of temperature and geopotential height over the Tibetan Plateau and their relations with Tibet ozone depletion. Geophys. Res. Lett. 32 (18), L18705. doi:10.1029/2005GL023496
Zhou, X. J., Li, W. J., Chen, L. X., and Liu, Y. (2004). Study of ozone change over Tibetan Plateau. Acta Meteor. Sin. 62 (5), 513–527. doi:10.11676/qxxb2004.053
Zhou, X. J., Luo, C., Li, W. L., and Shi, E. J. (1995). Total ozone varation in China and ozone low over Tibetan Pleteau. Chin. Sci. Bull. (in Chinese) 40 (15), 1396–1398. doi:10.1360/csb1995-40-15-1396
Keywords: Stationary transport, transient transport, ozone valley over the Tibetan Plateau, Lorenz circulation decomposition method, upper troposphere and lower stratosphere
Citation: Xu W, Song Q, Li Y, Shi C, Sun B, Su Y, Tang Z, Du Y and Guo D (2021) Effects of Stationary and Transient Transport of Ozone on the Ozone Valley Over the Tibetan Plateau in Summer. Front. Earth Sci. 9:608018. doi: 10.3389/feart.2021.608018
Received: 18 September 2020; Accepted: 10 February 2021;
Published: 25 March 2021.
Edited by:
Chaim Garfinkel, Hebrew University of Jerusalem, IsraelReviewed by:
Bijoy Vengasseril Thampi, Science Systems and Applications, Inc., United StatesKe Wei, Institute of Atmospheric Physics, China
Copyright © 2021 Xu, Song, Li, Shi, Sun, Su, Tang, Du and Guo. This is an open-access article distributed under the terms of the Creative Commons Attribution License (CC BY). The use, distribution or reproduction in other forums is permitted, provided the original author(s) and the copyright owner(s) are credited and that the original publication in this journal is cited, in accordance with accepted academic practice. No use, distribution or reproduction is permitted which does not comply with these terms.
*Correspondence: Yajuan Li, yajuanli@njxzc.edu.cn; Dong Guo, dongguo@nuist.edu.cn