- 1Geological Survey of Slovenia, Ljubljana, Slovenia
- 2Istituto Nazionale di Geofisica e Vulcanologia, Sezione di Sismologia e Tettonofisica, L’Aquila, Italy
We present the compilation of a new database of active faults in Slovenia, aiming at introducing geological data for the first time as input for a new national seismic hazard model. The area at the junction of the Alps, the Dinarides, and the Pannonian Basin is moderately seismically active. About a dozen Mw > 5.5 earthquakes have occurred across the national territory in the last millennium, four of which in the instrumental era. The relative paucity of major earthquakes and low to moderate fault slip rates necessitate the use of geologic input for a more representative assessment of seismic hazard. Active fault identification is complicated by complex regional structural setting due to overprinting of different tectonic phases. Additionally, overall high rates of erosion, denudation and slope mass movement processes with rates up to several orders of magnitude larger than fault slip rates obscure the surface definition of faults and traces of activity, making fault parametrization difficult. The presented database includes active, probably active and potentially active faults with trace lengths >5 km, systematically compiled and cataloged from a vast and highly heterogeneous dataset. Input data was mined from published papers, reports, studies, maps, unpublished reports and data from the Geological Survey of Slovenia archives and dedicated studies. All faults in the database are fully parametrized with spatial, geometric, kinematic and activity data with parameter descriptors including data origin and data quality for full traceability of input data. The input dataset was compiled through an extended questionnaire and a set of criteria into a homogenous database. The final database includes 96 faults with 240 segments and is optimized for maximum compatibility with other current maps of active faults at national and EU levels. It is by far the most detailed and advanced map of active faults in Slovenia.
Introduction
Modern seismic hazard assessment studies strive in incorporating state-of-the-art knowledge from its various input datasets. We report on the new map and database of active, probably active and potentially active faults in Slovenia through the lens of seismic hazard input model. Slovenia and its immediate vicinity is a seismically moderately active region with a number of damaging to very destructive historic earthquakes, including some of the strongest events in the broader Alpine area. Several historic earthquakes with moment magnitudes in mid to upper 6s and maximum intensities up to Imax = X have occurred in the past (e.g. 1348, 1511). Recent damaging earthquakes include the 1976 Ms 6.5, Ms 6.1 and Ms 6.0 Friuli earthquakes, 1998 Ms 5.7 Krn-Bovec earthquake and the 2004 Mw 5.2 Bovec earthquake (Aoudia et al., 2000; Bajc et al., 2001; Kastelic, 2008; Gosar, 2012; Gosar, 2019a; Gosar, 2019b) (Figure 1). The near-continuous tectonic activity in Mesozoic and Cenozoic spans rifting phases of Meliata and Vardar oceans and the complex Alpine orogeny phase, beginning in Cretaceous and still ongoing (Schmid et al., 2020, and references therein). The long and varied tectonic history has resulted in strong structural footprints and overprints.
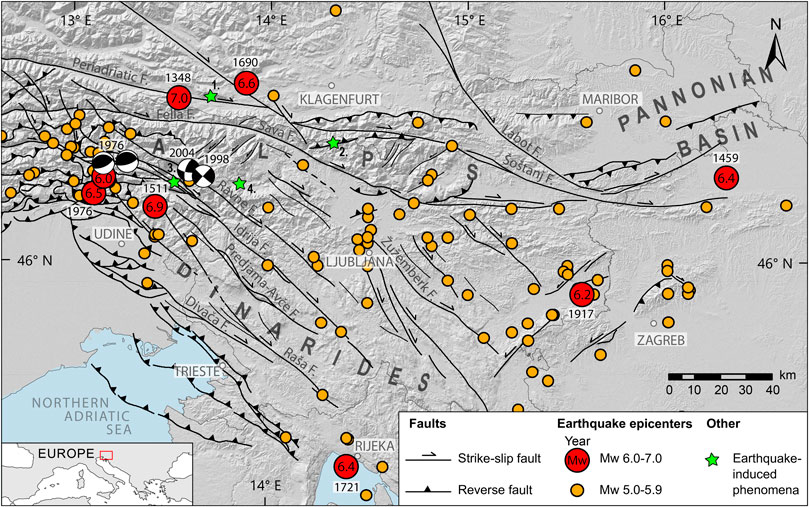
FIGURE 1. Generalized seismotectonic map of the junction between the Alps, the Dinarides and the Pannonian Basin tectonic domains with plotted significant seismicity (Mw ≥ 5.0). Faults west of Slovenia are summarized after Poli and Zanferrari (2018). Earthquakes are from SHEEC catalogs 1000–1899 (Stucchi et al., 2012) and 1900–2006 (Grünthal and Wahlstrom, 2012). Focal mechanisms for Mw > 5.0 events (Bajc et al., 2001; Pondrelli et al., 2006; Kastelic, 2008). Earthquake induced phenomena: 1 - Dobratsch, 2 - Veliki vrh, 3 - Srpenica, 4 - Lake Bohinj.
Slovenia is located in a relatively quiescent part of the broad Eurasia-Africa collision zone. While the most active part of the collision zone is located further south in the central and eastern Mediterranean region, with historic earthquakes with Mw > 8 (e.g. Papadopoulos et al., 2007; Shaw et al., 2008; Stiros, 2010), the part at the junction of the Alps, the Dinarides and the Pannonian basin is nevertheless an area of noteworthy tectonic and seismic activity. The largest historic earthquakes in the region produced major damage even by modern standards. The 1348 Villach/Carinthia/Friuli earthquake may have resulted in as many as 5000–10 000 fatalities according to contemporary sources, although it may well be that part of the figure was the result of the devastating Black death epidemic of bubonic plague at the time (Hammerl, 1994). The same event resulted in two large landslides: the landslide/collapse of Mt. Dobratsch (∼1 km3) and most likely the Veliki vrh landslide (20–100 million m3) (Zorn, 2002; Merchel et al., 2014) (Figure 1). The 1511 Idrija/Friuli earthquake caused up to 12 000 fatalities and resulted in major damage in parts of Slovenia and northeast Italy (Ribarič, 1979; Cecić, 2011). These figures are large by modern standards and extremely large taking into account the much smaller medieval and early renaissance populations in the region. It should be noted that the epicenters of both, and in particular the 1348 event are comparatively poorly constrained and have been variously placed in Slovenia, northeast Italy or south Austria. Paleoseismic evidence strongly supports an epicenter of the 1511 in Slovenia (Bavec et al., 2013).
The damaging 1895 M 5.9-6.1 Ljubljana earthquake (Živčič, 2009; Stucchi et al., 2012). hit the urban, logistical and governmental center of the country, clearly demonstrating the earthquake hazard to the modern population (e.g. Lapajne, 1989; Vidrih and Godec, 1995). The Ljubljana skyscraper, built in 1933, used state-of-the-art earthquake-resistant construction. As part of former Yugoslavia, Slovenia enforced a new, strict earthquake-resistant building code following the destructive 1963 Mw 6.0 Skopje earthquake (e.g. Georgescu et al., 2013). Past seismic activity is rather extensively documented in several earthquake catalogs (Ribarič, 1982; Zivčić, 2009; Stucchi et al., 2012; Grünthal et al., 2013). The most recent and currently used earthquake hazard map was compiled and published nearly two decades ago (Lapajne, 2001). The map is mostly based on instrumental and historic seismicity, resulting in strong concentration of earthquake hazard in three areas affected by strong historic events: the extreme NW Slovenia, bordering on the Friuli region in Italy, central Slovenia, resulting from the 1895 event and the extreme SE part of the country, resulting from earthquakes in 1917, 1924 and 1928.
The contribution of fault earthquake sources had thus far not been systematically included in the national earthquake hazard map. The presence of active major regional strike-slip faults has been recognized for some time (Poljak et al., 2000, 2010; Vrabec & Fodor, 2006; Placer, 2008). The Idrija fault has long been tentatively linked with the 1511 earthquake (Ribarič, 1979; Fitzko et al., 2005). Active faults had never before been systematically mapped and cataloged on a national level. The last structural map of Slovenia that included active and inactive faults, the ‘Structural-tectonic map of Slovenia’ was published two decades ago. It used now obsolete concepts and methods and a rather limited data set based on the general geologic map of Slovenia, which was compiled from the 1960s to the 1990s (Poljak, 2000). Parts of Slovenian territory are included in other national or broader European databases. The western half of Slovenia is included in the Italian DISS database of seismogenc faults (DISS Working Group, 2018), while the majority of the country is part of the SHARE EDSF database of seismogenic faults, which covers a large part of Europe (Basili et al., 2013). Following the call by the Ministry of Environment and Spatial Planning for a new earthquake hazard map, the Office of Seismology and Geology of the Slovenian Environment Agency contracted the Geological Survey of Slovenia to produce a new active fault map as a geologic input into the new national earthquake hazard map. The goals in compiling a new active fault map and database included: compatibility with existing active fault and fault earthquake source maps and databases (e.g. SHARE, DISS), future expandability and adaptability through the use of state-of-the-art concepts and methods and full traceability of all input data, reasoning and argumentation for each spatial, geometric, kinematic and activity parameter. The project also coincides with compilation of the new European earthquake hazard map (ESHM20), for which this map and database is also being used as an indirect input (Basili et al., 2020). Our database is built upon the experience and well-established knowledge on active fault studies and data base compilations (e.g. Wallace, 1986; Yeats, 2012; Styron and Pagani 2020, and references therein) and reflects the current state of knowledge on active faults in Slovenia.
Regional Geologic Characteristics
General Structure and Regional Tectonic Subdivision
The regional tectonic subdivision follows a structural imprint formed over a complex history of Variscan orogenic cycle followed by Mesozoic rifting and the Alpine orogenic phase which has been ongoing since the late Mesozoic. The study region is characterized by a triple junction involving three distinct lithospheric domains: the European plate, the Adria microplate and the Pannonian domain (Brückl et al., 2010) (Figure 2). The Adria microplate, a fragment of the African plate, detached in the lower Jurassic is in long-term collision with the European plate (Anderson and Jackson, 1987). Its current GNSS data-derived motion is northwards at approximately 2-4 mm/yr, combined with counterclockwise rotation of approximately 0.30°/Myr an angular velocity around a pivot point underneath the western Alps (Weber et al., 2006, 2010; Serpelloni et al., 2016). The Pannonian domain consists of several structural blocks of different continental affinity, mainly the ALCAPA and Tisza crustal blocks/mega-units merged into one soft plate (Brückl et al., 2010; Schmid et al., 2020). The Pannonian domain underwent thinning due to crustal extension and thermal collapse in its Pannonian basin part (e.g. Handy et al., 2014; Horváth et al., 2015) that occupies the NE-most area of our study zone. The boundary between the European plate and the Pannonian domain is represented by strike-slip, with local transtensional and transpressional regimes and a general GNSS data-derived eastward motion of the Pannonian domain at approximately 1 mm/yr (Brückl et al., 2010; Serpelloni et al., 2016). The contact between the Adria microplate and the Pannonian domain is of transpressional nature (Brückl et al., 2010).
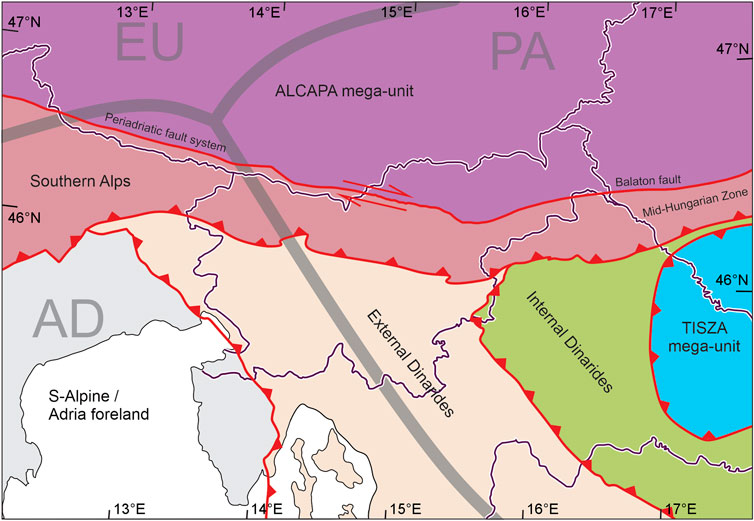
FIGURE 2. General structure and regional tectonic subdivision of Slovenia and its vicinity. The thick gray lines represent the boundary between the European plate (EU), the Adria microplate (AD) and the Pannonian Domain (PA) (from Brückl et al., 2010). Higher order units are compiled and simplified from Schmid et al. (2020).
The collisional triple junction has resulted in a complex regional tectonic structure comprised of higher-order Adria-derived units (Southern Alps, the Dinarides, ALCAPA mega-unit) and the complex Tisza mega-unit (Schmid et al., 2020). The Southern Alps are a thrust belt formed in the Oligocene to Early Miocene top-to-S thrusting on generally E-W striking thrust faults, extending from northern Italy across its NE territories into NW Slovenia and further across the central and eastern Slovenia where it is predominantly buried under thick Neogene sediments of Paratethyan origin of the Pannonian basin (Schmid et al., 2020, and references therein). Part of the thrust belt in the northern and northeastern Italy is active, accommodating the ongoing CCW rotation of the Adria microplate (e.g., Galadini et al., 2005; Monegato and Poli, 2015; Poli et al., 2018).
The Dinarides are an Adria passive margin-derived unit comprising a stack of top-to-SW thrusts and nappes, formed during latest Cretaceous to Eocene. The external Dinarides are composed of a very thick sequence of Paleo-Mesozoic platform carbonates of the Adriatic carbonate platform, while the Internal Dinarides are composed of composite nappes, derived from distal Adriatic margin and obducted Western Vardar ophiolites (Schmid et al., 2020). After Middle-Late Eocene the northward motion of the Adria microplate resulted in the formation of a narrow belt of steep reverse faults west of the External Dinarides, forming the boundary with the undeformed Adria foreland (Placer et al., 2001, 2010; Vrabec and Fodor, 2006). The extreme NW part of the External Dinarides and neighboring part of the Southern Alps are cross cut by the subvertical faults of the Dinaric dextral strike-slip system, formed in the Late Miocene, absorbing the NNW-SSE shortening due the CCW rotation of the Adria microplate and accommodating the eastward motion of the Pannonian domain (Poljak et al., 2000, 2010; Grenerczy et al., 2005; Vrabec and Fodor, 2006; Caporali et al., 2008; Kastelic et al., 2008; Weber et al., 2010).
The northeastern part of Slovenia is part of the ALCAPA mega-unit, divided from the Southern Alps by the Periadriatic fault system. The ALCAPA mega-unit comprises the Austroalpine unit of the Alps (Schmid et al., 2020, and references therein). The dextral strike-slip Periadriatic fault system has accommodated the still-ongoing left-lateral extrusion of the ALCAPA mega-unit since 21–23 Ma (e.g. Schmid et al., 2013). The structure and activity in the eastern part of Slovenia are mostly controlled by the interaction between the ALCAPA and Tisza mega-units. Tisza is an accreted tectonic block/mega-unit composed of composite terranes of Eurasian origin formed in the Mesozoic and finally emplaced in present-day configuration in Paleogene-Miocene (Csontos et al., 1998; Handy et al., 2014; Schmid et al., 2020). The boundary between the ALCAPA and Tisza mega-units is the Miocene-formed Mid-Hungarian Zone (MHZ) of which only the north-westernmost part is located in Slovenia. The northern edge of the MHZ, also referred to as the Balaton fault/line is the eastward continuation of the Periadriatic fault system. The MHZ is zone of repeated tectonic inversions (Csontos et al., 1998), currently in its south-westernmost part a sinistral strike-slip zone resulting from the Tisza eastward motion outpacing the eastward motion of ALCAPA (Serpelloni et al., 2016).
Geodetic Evidence
The N-S horizontal shortening rate across the territory of Slovenia has been estimated at 2–4 mm/yr, based on GNSS measurements (Weber et al., 2010; Serpelloni et al., 2016). Strong motion vector divergence is observed from west to east, with motion vectors in W Slovenia deviating counterclockwise from north and motion vectors in E Slovenia deviating clockwise from north, with E-W velocity components <1 mm/yr (e.g. Serpelloni et al., 2016). GNSS measurements have also been used to constrain slip rates on several faults (Pavlovčič Prešeren et al., 2005; Caporali et al., 2013). In the past decade some data on fault slip rates has also been obtained by PSInSAR. The method has mostly been used to detect and observe slope mass movement processes, however, motion along faults in NW Slovenia has been observed as well, with derived horizontal and vertical motion components on the order of 0.5–1 mm/yr (Milanič, 2010). Vertical motion has also been reported with leveling line polygons, in western and central Slovenia, which returned cumulative values of uplift up to 7 mm/yr (Rižnar et al., 2005; Rižnar et al., 2007).
Seismicity
Slovenia is considered a region of moderate seismicity. The historic record begins in 792 AD (Ribarič, 1982), however, reliable records are considered to begin in the 14th century (Živčić, 2009). Major historic earthquakes with epicenters in Slovenia or the immediate vicinity include the 1348 M 6.4–7.1 (Imax = IX-X) Villach earthquake, 1511 Mw 6.9 (Imax = X) Idrija earthquake, the 1689 Mw 5.6 (Imax = VIII) Šentvid pri Stični earthquake, the 1690 Mw 6.6 (Imax = VIII-IX) Carinthia earthquake, the 1699 Mw 5.6 (Imax = VIII) Metlika earthquake and the 1895 M 5.9–6.1 (Imax = VIII-IX) Ljubljana earthquake and more recently the 1917 M 5.7-6.2 (Imax = VIII) Brežice earthquake, the 1926 Mw 5.8 (Imax = VII-VIII) Cerknica earthquake and the 1998 M 5.4 (Imax = VII-VIII) Krn-Bovec earthquake (Ribarič, 1982; Postpischl, 1985; CPTI Working Group, 2004; Guidoboni et al., 2007; Živčić, 2009; BRGM-EDF-IRSN/SisFrance, 2010; Rovida et al., 2011; Grünthal et al., 2013; Stucchi et al., 2012; Tiberi et al., 2018). Evidence of prehistoric earthquakes is relatively sparse, with paleoearthquakes so far attributed to two known active faults, the Idrija fault and Vodice fault (Bavec et al., 2013; Jamšek Rupnik et al., 2015). No older events have been constrained beyond preliminary detection. Additional evidence of Late Pleistocene and Holocene earthquakes has been found in lacustrine sediments of past and current lakes in the Julian Alps of NW Slovenia. Rapuc et al. (2018) found in Lake Bohinj evidence of up to 29 events in the past 6600 years, including the large events in 1348, 1511 and 1690 (Figure 1). Two seismite layers dating to 12790 ± 85 BP have been found in lacustrine sediments in Srpenica, Julian Alps (Marjanac et al., 2001).
Compilation of the Fault Database
Objective
The objective was to compile a new map and database of active faults with the potential to generate Mw > 5.5 earthquakes, which translates into >5 km length assuming full-length rupture (Wells and Coppersmith, 1994). The primary use of this database is to provide geological input on active faulting for a new design ground acceleration map of Slovenia and the database format, parameter choice and constraints are determined by this use. Additionally, the aim was to produce a standalone database of active faults in Slovenia, at a level of systematic mapping and parametrization that had never been done in the past, and to provide a homogenous and uniform basis for research of individual faults and fault systems, including future structural, geodetic and paleoseismological investigations. The database is designed for use on a national level, such as input for the national seismic hazard map. It may serve as a starting point for more specific applications, such as fault displacement hazard analysis, however, only taking into account limitations of the database and considering that it was not mapped on a scale and with such detail to be directly compliant with seismic hazard models for the critical facilities (e.g. IAEA-SSG-9 guidelines; IAEA, 2010) neither does it include the distributed rupture data that are needed for fault displacement analyses (e.g. Youngs et al., 2003). In this aspect the presented active fault database can serve as a reference model.
Database Criteria, Format and Workflow
In setting up the criteria for the active fault database, we studied several regional/national compilations in order to comply with the accepted definitions and standards (e.g. Haller et al., 2011; Styron and Pagani, 2020, and references therein). Each fault is an individual database entry that includes all relevant spatial, geometric, kinematic and activity data. This includes full traceability of all geometric and seismotectonic parameters, including origin of parameter or input data, with references, and full history of changes. Given our regional setting and the fact our database is included in the new version of the European database of seismogenic faults (Basili et al., 2020), the fault geologic and seismotectonic parametrization is such that it supports direct translation into fault seismic sources in a format compatible with existing European databases such as SHARE (Basili et al., 2013) and DISS (Basili et al., 2008; DISS Working Group, 2018) by using the composite seismic source approach.
The workflow included extensive data mining, compilation and critical synthesis of available data, systematical identification and parametrization of faults, followed by preparation of the database (Figure 3). Data was systematically mined for geologic, paleoseismic, geodynamic, geophysical, geodetic and seismological data. Data sources included publishes papers, reports, internal (unpublished) reports in the archive of the Geological survey of Slovenia. Input data also includes published and unpublished geologic maps, field observations, shallow (high-resolution seismic reflection, seismic refraction tomography, electrical resistivity tomography, ground-penetrating radar) and deep geophysical (seismic data) and DEMs at various resolutions, most notably the national 5 m DEM (Public Information of Slovenia, the Surveying and Mapping Authority of the Republic of Slovenia, DEM 5, 2006) and to some extend the national LiDAR DEM (Ministry of the Environment and Spatial Planning, Slovenian Environment Agency, 2011). We evaluated older published data on faults by comparison with the latest data sets, including geomorphic analysis of shaded relief. We systematically identified faults with surface trace lengths of 5 km or longer and constrained their geometry using all available data. Each fault was mapped for individual segments. We constrained seismotectonic parameters for all faults and their segments.
The final database is composed of three components: the fault map (.shp file), parametrization table (MS Excel spreadsheet, linked to the .shp file) and an explanatory report (240 + pages, currently only in Slovene). The .shp file includes all fault and segment traces. The parametrization table includes all geometric, kinematic and activity parameters for each individual fault and segment. It is accompanied by a .txt log of all changes in any parameter in the parametrization table, with each change fully documented, with argumentation. The explanatory report contains definitions and argumentation for the database format, parameter choice and definitions, as well as detailed discussion of all available data for each individual fault, separated into two sections–fault trace description with a fault trace map, geometric, kinematic and activity parameter discussion and definition. Parametrization, including the discussion of all available data and argumentation for the determined parameter values is thus fully traceable.
Criteria, Definitions, Parameters, Data Origin, Quality Designators
We defined all faults and fault segments according to a predetermined set of criteria, definitions and parameters.
Fault Activity
We classified faults by activity into three categories: active, probably active and potentially active. A fault is considered:
• Active, if there is direct evidence for its activity in Quaternary (2.6 Ma). This includes: paleoseismic evidence of activity, offset Quaternary geomorphic indicators, geophysical evidence of offset Quaternary strata, seismicity correlated with the fault and geodetic evidence of fault activity. Similar criteria were applied for southern alpine thrusts in north Italy (e.g. Galadini et al., 2012).
• Probably active, if there is indirect evidence for its activity in Quaternary. This includes: the presence of Quaternary sedimentary basins structurally related with the fault, effects of the fault on drainage systems (offsets, deflections, changes in stream behavior such as for example a change from incision to aggradation, etc.), offsets of geologic markers impossible to explain with preceding tectonic phases (assuming an inherited/reactivated fault).
• Potentially active, if it is in a favourable structural relationship with a known active fault or if it is in a favourable geometry for activity within the current stress field.
Direct evidence of Quaternary activity includes: results of geodetic observations (GNSS, leveling data, geodetic control/reference networks), geomorphic and age dating analysis (offsets of Quaternary surfaces), geophysical and age dating analysis (offsets of Quaternary strata), paleoseismological evidence and seismicity data. Any fault with Quaternary activity confirmed by one or several of these methods is considered active. Due to a general lack of Quaternary sediments overlaying the faults, small number of active faulting studies, and generally insufficient age constraints on Quaternary surfaces, evidence of activity during any time over the Quaternary period is considered as evidence of fault activity. Indirect evidence of activity includes: Quaternary sedimentary basins in structural relationship with the fault, with no quantitative data, and offsets of Neogene strata. Such faults are considered probably active. In potentially active faults, a favourable structural relationship with a confirmed active fault is considered such, that activity on the active fault can induce activity on the potentially active fault. Additionally, faults with no evidence of activity (but not evidence of inactivity) within an active fault system is considered potentially active. Also, a fault is considered potentially active if it is in a favourable geometry with respect to the current stress field.
Similar temporal constraints (e.g. Quaternary activity) have been used in a number of similar active fault databases, such as for example the active fault map of Turkey (Emre et al., 2018), the Quaternary active fault map of Iberia (García-Mayordomo et al., 2012), the Quaternary fault and fold database of the United States (U.S. Geological Survey et al., 2020) and to a lesser extent Greece (Ganas et al., 2013), where active faults show activity in Middle Pleistocene to Holocene, and Neogene syn-rift basins are also considered as evidence of very likely present activity. In areas where slip rates are higher, and/or more age data for Quaternary surfaces is available, generally shorter time spans are used (e.g. Langridge et al., 2016) or additional activity classes are added (e.g. Emre et al., 2018; U.S. Geological Survey et al., 2020).
Fault Segmentation
A fault segment is defined as part of the fault that is distinct from other parts in geologic criteria and is not necessarily an earthquake segment, e.g. not defined by the limits of historic coseismic surface ruptures. Fault segments were divided into five classes: earthquake, behavioral, structural, geologic and geometric, according to the classification by McCalpin (2009), Supplementary Datasheet S9. Where the fault trace was not evident on the surface and was presumably covered, we defined the segment as inferred.
Seismotectonic Parametrization
Seismotectonic fault parametrization was based on critical synthesis of all available geologic, geodynamic, geodetic, geophysical, geomorphic and seismicity data. We assigned each individual fault and fault segment full seismotectonic parameters (Table 1).
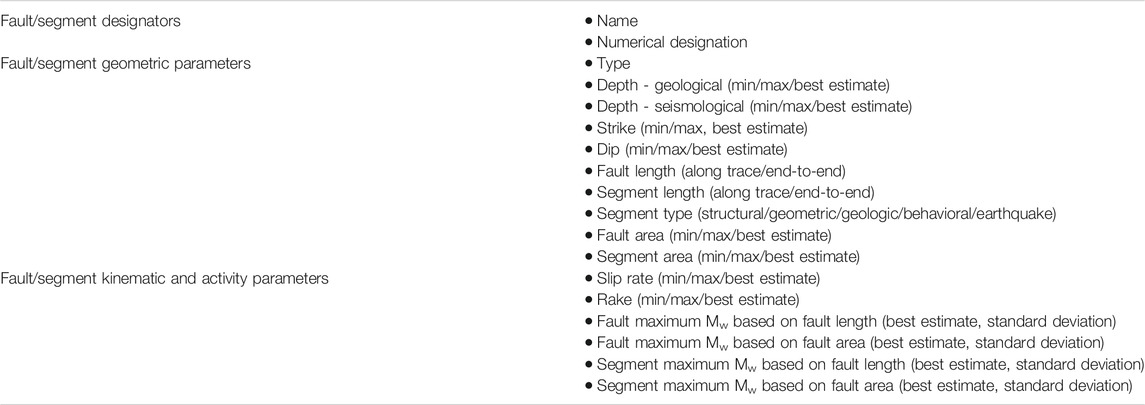
TABLE 1. List of designators and seismotectonic parameters for each fault and fault segment in the database.
Every parameter is described with full argumentation for all faults (in the parametrization table and explanatory report). Where appropriate, parameters are defined with minimum, maximum and best estimate values. The best estimate of average slip rate is based on the evaluation of the amount and quality of direct and indirect evidence and available data. Where such argumentation is not possible due to a lack of data, the average was taken as the best estimate. An example of fault parametrization attributes is presented for the Idrija fault (Table 2).
Data Origin and Quality Designators
Quality of estimated parameters, which include fault depth, strike, dip, rake, slip rate and maximum magnitude was adapted from the SHARE database (Basili et al., 2013) and is marked by the following designators: LD - Literature Data, OD - Original Data, ER - Empirical Relationship, EJ - Expert Judgement (Table 2). These quality designators make assessment of quality of each parameter easier.
Results
Fault Database
As of mid-2020 the first full version of the map and database is considered complete and is available online at: tectonics.geo-zs.si. It includes 96 individual faults and 240 individual fault segments. Of the 96 faults in the database, 18 are confirmed active and 20 probably active (Figure 4). The remaining 58 are potentially active and, by definition, do not show direct or indirect evidence of activity. These may include incipient faults or older, exhumed faults from past tectonic phases. Incipient faults may not have accumulated sufficient offset to clearly show activity, while old, exhumed faults may produce distinct geomorphic expression, but no known direct or indirect evidence of activity.
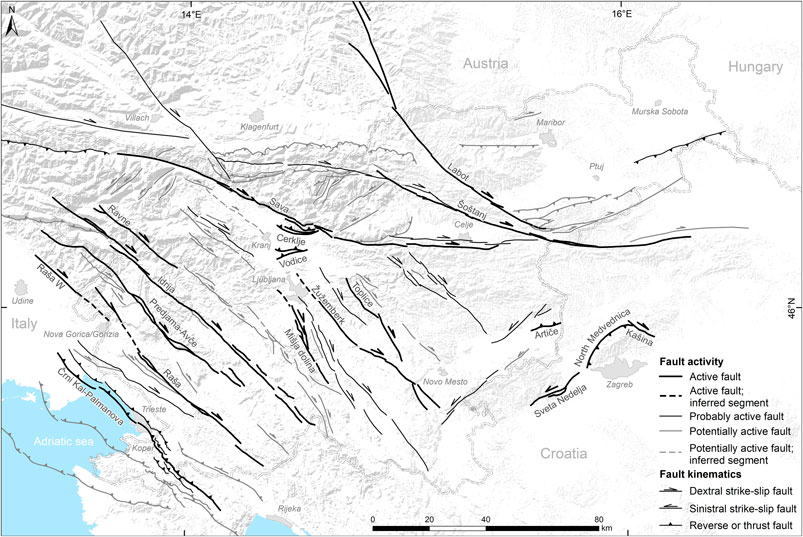
FIGURE 4. Map of active, probably active and potentially active faults in Slovenia and the immediate vicinity.
We consider the database to be highly complete for active, probably active and potentially active faults with surface traces 5 km long or longer in areas with hard bedrock (carbonates, flysch, metamorphic and igneous rocks), including most of western, northern and central Slovenia. In these areas, fault traces are distinct and readily identifiable through geologic mapping and geomorphic analysis, even in the relatively limited areas of clastic rocks.
Areas with deep infill of Neogene clastic sediments and sedimentary rocks, mostly including northeastern Slovenia and to a lesser extent parts of central and southeastern Slovenia are considered less complete. Neogene sediment thickness locally exceeds 2 km in SE Slovenia and 6 km in NE Slovenia. While all available data has been included, large amounts of deep petroleum geophysical data remain publicly unavailable and once included may result in future changes in the fault map and database. Many faults in this environment are blind (e.g. Artiče fault, Ljutomer fault). While blind faults with relatively shallow fault tips (<1 km) may readily be detected at the surface by their narrow fault propagation folds, blind faults with deeper fault tips may produce very gentle fault propagation folds at the surface and without high-quality deep geophysical data may escape detection.
Challenges
The area of Slovenia is tectonically moderately active, and there are numerous factors that make systematic identification, mapping and parametrization of active faults comparatively difficult. The main compounding factors are high slope mass movement, erosion and denudation rates, and the very complex tectonic history of the region and resulting structural overprinting.
Slope Mass Movement, Erosion, Denudation
Large parts of Slovenia are highly susceptible to slope mass movement processes, which are orders of magnitude larger than expected tectonic movement (e.g. Komac and Ribičič, 2006; Komac, 2012; Žibret et al., 2012; Komac and Hribernik, 2015). Slope mass movement processes and erosion are likely to obscure surface fault traces, particularly any relatively recent geomorphic markers; this is particularly true in the clastic rocks in parts of western, central and eastern Slovenia.
Slip Rates–Data Set Heterogeneity
The slip rate data set is highly heterogeneous. Various methods, covering vastly different time spans are used to determine the slip rate on individual faults. Time spans range from several years (GNSS, PSInSAR, extensometer), to several decades (leveling data), to thousands and tens of thousands of years (paleoseismic, geomorphic, geologic) and even several million years (geologic). Table 3 presents available data for the Idrija fault, for which the largest dataset for slip rate evaluation was available.
Data heterogeneity is the result of unharmonized data obtained in various projects and works with vastly different methods and goals. In general, a strongly harmonized effort, simultaneously encompassing all faults will be required for a more homogenous data set. This may involve short-term geodetic slip rate determination, longer-term slip rate from age dating of displaced surfaces or combination of both. While a significant uncertainty in slip rates is acceptable from a general scientific viewpoint, large uncertainties are problematic when data is used for fault activity parametrization in seismic hazard assessment.
Structural Overprinting
Structural overprinting during successive tectonic phases in Mesozoic and Cenozoic have led to the presence of numerous partly overlapping and reactivated faults and fault systems. Reverse faults in southeastern Slovenia have likely been active in the lower-Neogene and post-Neogene (e.g. Tomljenović and Csontos, 2001). Fortunately, some deep geophysical data is available in the area, indicating a very small to negligible contribution of the lower-Neogene phase (c.f. Gosar and Božiček, 2006; Gosar, 2008), allowing good constraints on the activity of these fault. Reverse faults in northeastern Slovenia are in most cases reactivated lower-Neogene normal faults, connected to inverted sedimentary basins (Fodor et al., 2002). Another area of very strong multiple structural overprints is the Sava folds, with at least two major folding phases and likely more. Paleostress analysis of faults in NW External Dinarides of Slovenia indicates four successive tectonic phases in which the faults were active (Žibret and Vrabec, 2016).
We may conclude from the surface data that a mapped fault is favorably oriented for activity in the current stress field, however, there is typically little data on the extent of the fault at depth. Geophysical data is typically lacking in all areas except in the Trieste gulf, Krško basin and in NE Slovenia. A fault with a clear surface trace may be exhumed and/or truncated or offset at depth by more recent faulting and thus inactive. Each fault requires detailed geologic mapping, geomorphic analysis and where possible also paleoseismic investigations. While in some cases, particularly with late-Neogene and post-Neogene reverse faults in thick clastic sediment successions, separation of individual tectonic phases and constraining recent activity is fairly straightforward, faults in zones with little or no young clastic sediments are much less well constrained. Poorly constrained fault histories (kinematic evolution through successive tectonic phases) reduce the confidence and validity of long-term slip rates estimated from offset geologic markers. It is likely that most potentially active faults have comparatively little contribution to the seismic hazard, due to their implied low slip rate (e.g. if slip rates were higher, the probability of detection of fault’s probable or confirmed activity would be higher). This is a major future challenge that will require extensive and detailed work on individual faults.
Fault Systems
Adriatic Foreland Fault System
The Adriatic foreland fault system is characterized by NW-SE striking thrusts and reverse faults, extending from northern Istria and the Trieste gulf (NE Adriatic Sea) into the western Karst region (Figure 5). The system includes one active (Palmanova-Črni Kal thrust), three probably active (Kubed thrust, Hrastovlje thrust, Socerb thrust) and five potentially active faults (Buje fault, Buzet thrust, Sočerga-Lupoglav thrust, Skadanščina fault). The longest faults are the Buje fault (73 km), the Buzet thrust (75 km) and the Palmanova-Črni Kal thrust (81 km), all of which run across the Istria peninsula into the Trieste gulf and potentially into the southernmost part of the Friuli plain, NE Italy. The longest faults are segmented by perpendicular, NE-SW running subvertical strike-slip faults. The comparatively short (<5 km) Sistiana and Monte Spaccato strike-slip faults are probably active, as indicated by offset late Quaternary strata on the Monte Spaccato fault (Carulli, 2011). These faults are interpreted as faults and imply activity of thrust and reverse faults.
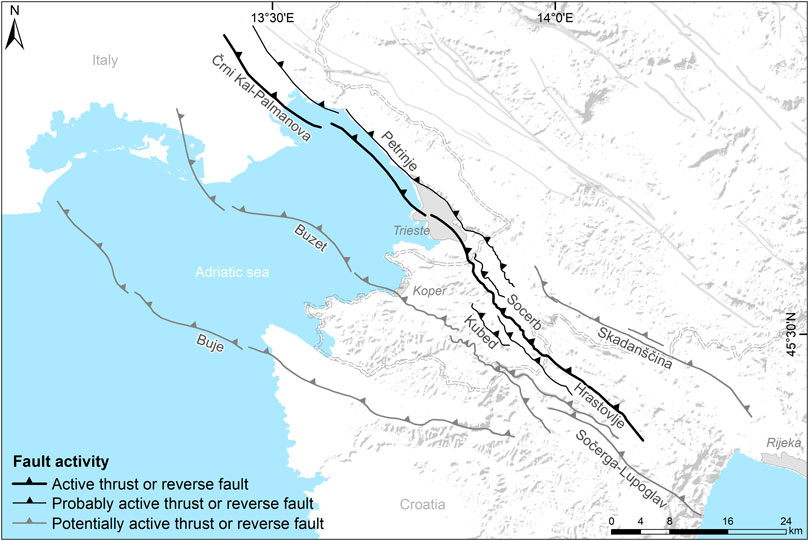
FIGURE 5. Adriatic foreland fault system. The system comprises a thrust belt approximately 30 km wide. The dominant active fault is the Črni Kal–Palmanova thrust.
The westernmost faults (Buje fault, Buzet thrust) are very shallow angle thrusts (approx. 15°), and the dip increases toward the northeast. The easternmost fault in the system, the Skadanščina fault is a steeply (60°) dipping, SW-vergent reverse fault. The maximum depth of the fault system can be tentatively estimated from geophysical data, as deep seismic reflection data within the Trieste gulf suggest the faults are listric, with a maximum depth of approximately 7 km (Busetti et al., 2010; Carulli, 2011).
Assessment and quantification of fault activity within the system is much more difficult. Evidence of recent activity includes offset late Quaternary marine sediments along the Palmanova-Črni Kal fault in the Trieste gulf (Busetti et al., 2010). There is little additional quantitative data on slip rates within the fault system. Rižnar et al. (2007) published an analysis of leveling data across the fault system, which indicates large ongoing vertical displacement rates totaling 2–3 mm/yr across the system. We consider these values exceedingly high, with implied total slip rates up to 5–8 mm/yr and thus not reliable. There is little significant historic seismicity associated with this fault system. The largest historic earthquake located in the SE part of the system in the Croatian part of Istria is the Mw 5.6 1574 Lupoglav earthquake (CPTI Working Group, 2004; Rovida et al., 2011).
Dinaric Fault System
The Dinaric fault system (DFS), characterized by dominant, long NW-SE striking dextral strike-slip faults, extends from the Karst region in SW Slovenia across much of western into central Slovenia (Figures 6, 7). Within the DFS we have identified a total of seven active faults (Raša fault, Raša West fault, Predjama-Avče fault, Idrija fault, Ravne fault, Mišja dolina fault, Žužemberk fault), four probably active faults (Divača fault, Dražgoše fault, Ortnek fault, Dobrepolje fault) and 21 potentially active faults (Brestovica fault, Tomačevica fault, Kobjeglava fault, Vipava fault, Bate fault, Golek fault, Banjšice fault, Jesenovec fault, Zala fault, Podplanina fault, Čeplez fault, Vrhinka fault, Borovnica fault, Rakitna fault, Možice fault, Sorica fault, Ratitovec fault, Želimlje fault, Pokljuka fault, Zasip fault, Kranj fault). The DFS is dominated by two fault sets, centered on several large, active regional faults: the western and the eastern fault sets.
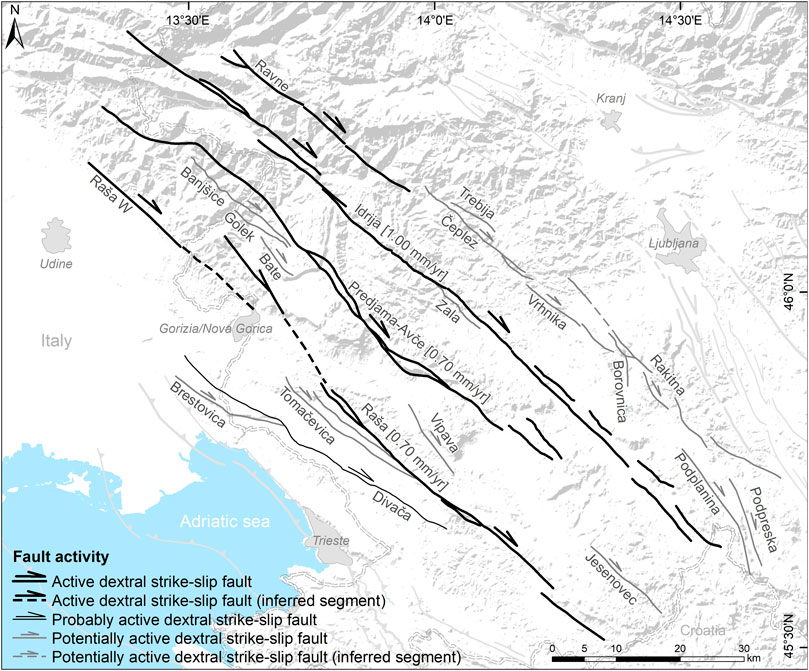
FIGURE 6. Western fault set of the Dinaric fault system. The dominant faults are the Raša fault, the Predjama-Avče fault and the Idrija fault.
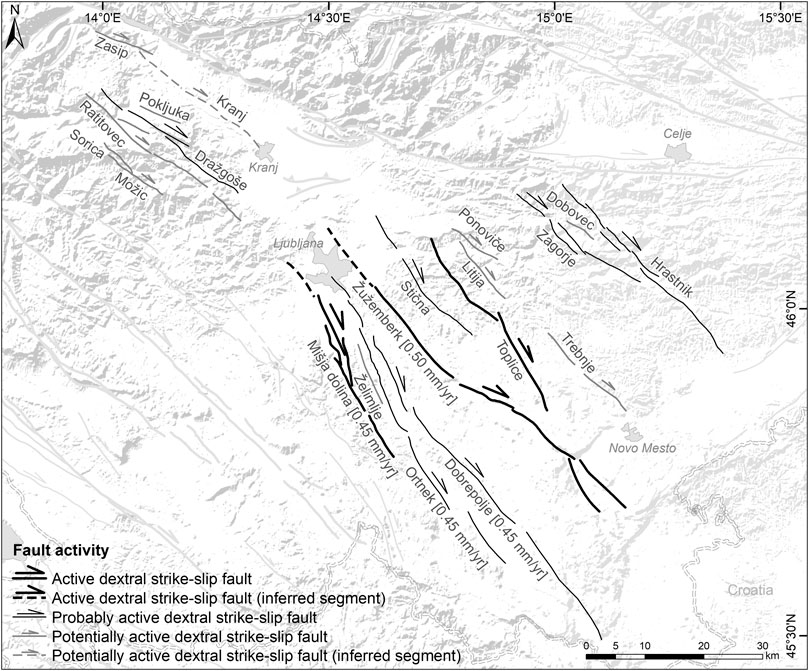
FIGURE 7. Eastern fault set of the Dinaric fault system and the dextral strike-slip faults of the Sava compressive wedge. The dominant faults are the Mišja dolina fault, the Ortnek fault, the Dobrepolje fault and the Žužemberk fault.
The western fault set (Figure 6) is centered on three major regional active faults: the Raša fault (87 km), the Predjama-Avče fault (75 km) and the Idrija fault (124 km). The Raša fault is a subvertical dextral strike-slip fault with an average estimated slip rate of 0.7 mm/yr. The Predjama-Avče fault is a steeply NE-dipping dextral transpressive fault, with an average estimated slip rate of 0.7 mm/yr. The Idrija fault is the longest fault of the system, a subvertical dextral strike-slip fault with an average estimated slip rate of 1.0 mm/yr. Slip rates on all three faults were determined through age dating of displaced surfaces (Moulin et al., 2014, 2016) and long-term displacement of geologic markers (Čar, 2010). Paleoseismic evidence has linked the Idrija fault to the 1511 Mw 6.9 earthquake (Bavec et al., 2013). Additionally, the Idrija fault is also the likely source of the 1926 Mw 5.8 Cerknica earthquake (Ribarič, 1982). Significant events within the western fault set also include the 1998 Ms 5.7 Krn-Bovec earthquake and the 2004 Mw 5.2 Bovec earthquake on the Ravne fault (Bajc et al., 2001; Kastelic, 2008). The Raša and Predjama-Avče faults have not produced any significant historic earthquakes, although the 1956 ML 5.1 Ilirska Bistrica earthquake (Grünthal et al., 2013) might have been caused by the Raša fault (Ribarič, 1982). There is evidence of the presence of another active fault in this fault set, the Selce fault, located south of the termination of the Predjama-Avče fault (Vičič et al., 2019); at this time the total reliably known surface fault length is < 5 km (Gospodarič, 1989; Šebela, 2005) precluding inclusion into the database, however, the fault and surrounding area needs to be further investigated in the future.
The eastern fault set (Figure 7) encompasses a number of long active and probably active faults, including: the Mišja dolina fault (52 km), the Ortnek fault (57 km), the Dobrepolje fault (65 km) and the Žužemberk fault (79 km). It is noteworthy that the surface traces of the first three faults are contained within a corridor only 6 km wide. Average estimated slip rates across the four faults are 0.45 mm/yr, 0.45 mm/yr, 0.45 mm/yr, and 0.50 mm/yr, respectively. The slip rate on the Mišja dolina fault is estimated from offset Quaternary markers in geophysical data (Atanackov, 2013), while the slip rate on the Žužemberk fault is estimated from GNSS data (Vrabec et al., 2011). Slip rates on the Ortnek and Dobrepolje faults are estimated from the faults’ similarity to the other two faults. No major historic seismicity has been conclusively attributed to this fault set, however, the 1895 Mw 5.9–6.1 Ljubljana earthquake has recently been tentatively linked to the Želimlje or Ortnek faults (Tiberi et al., 2018).
The western fault set appears to define a corridor across which a significant slip rate occurs, with approximately 2.5 mm/yr of dextral strike slip across the 25 km wide zone encompassed by the three major faults. The eastern fault set appears to define another corridor, approximately 15 km wide, across which approximately 1–2 mm/yr of dextral strike slip occurs. The rest of the DFS, both to the west, in the central part between the two major fault sets and to the east includes faults with low estimated fault rates (Figure 8).
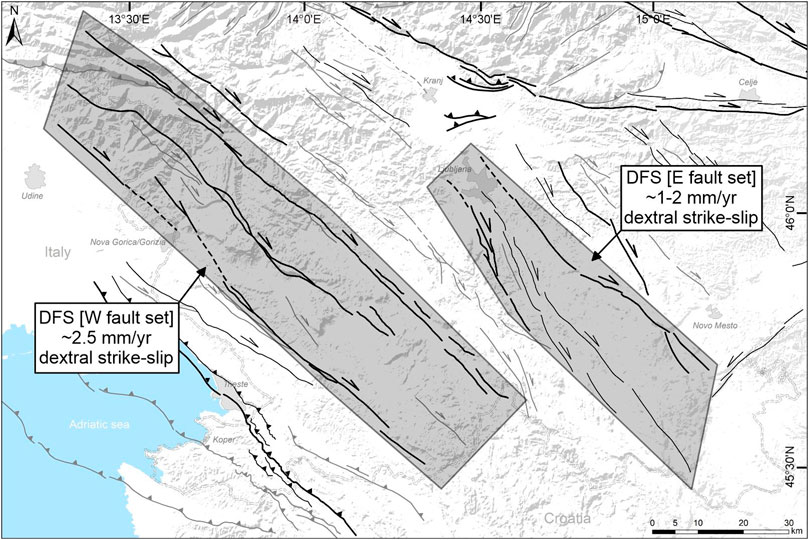
FIGURE 8. Dinaric fault system western and eastern sets indicating two corridors of comparatively high slip rates. The western fault set accommodates up to ˜ 2.5 mm/yr of dextral strike slip, while the eastern fault set accommodates up to ˜ 1–2 mm/yr of dextral strike slip. Slip rates on other faults within the system are estimated to be an order of magnitude lower.
We currently consider the Raša West fault an individual fault, distinct from the Raša fault due to the unclear potential connection between the two faults. The NW part of the Raša fault is relatively poorly constrained. The fault is well defined in the carbonates of the Karst plateau, where it splays with the main splay branches being the Kobjeglava and Tomačevica faults (Jurkovšek, 2008). The fault then likely progresses into the flysch and Quaternary lacustrine, alluvial and fluvial sediments of the Vipava valley. The Raša West and Raša faults are less than 4 km apart at their closest points and will likely be merged into a single fault pending further constraints on their geometries in the Vipava valley.
In its NW part the DFS transitions into the generally E-W striking reverse faults and thrusts of the Friuli region (Vrabec and Fodor, 2006; Poli and Zanferrari, 2017; Poli and Zanferrari, 2018). The Gemona-Kobarid fault is the only large reverse fault that pushes significantly into the area covered by the fault database. It terminates against the Idrija Fault in NW Slovenia (Figure 4). The fault is considered potentially active. The entire transitional area indicates areas of distinct uplift (Milanič, 2010). The uplift is currently not attributed to a specific structure or set of structures. It may potentially indicate larger-scale reverse faulting or minor pop-up structures within the transpressional transition area. Vertical displacement rate data from PSInSAR does not rule out local reactivation of structures in the South Alpine Thrust Front (Milanič, 2010).
Periadriatic Fault System
The Periadriatic fault system (PAFS) is characterized by dominant long, generally WNW-ESE striking dextral strike-slip faults, relatively minor E-W striking thrusts and reverse faults and SW-NE striking minor sinistral strike-slip faults (Figure 9). In the PAFS we identified five active (Sava fault, Vodice fault, Cerklje fault, Šoštanj fault, Labot (Lavantal) fault), four probably active (Stol (Hochstuhl) fault, Periadriatic fault, Celje fault, Velenje fault) and 12 potentially active faults (Srednji vrh fault, Košuta fault, Zelenica fault, Gornji grad fault, Logarska dolina fault, Menina fault, Olševa fault, Matkov kot fault, Podolševa fault, Savinja fault, Vransko fault, Northern Karavanke fault).
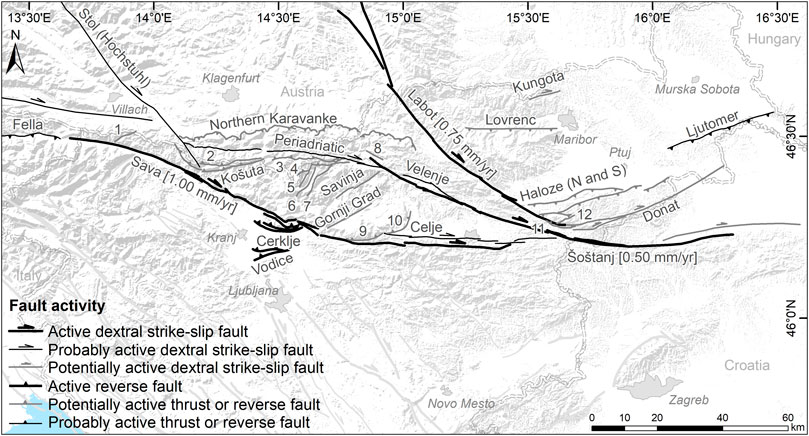
FIGURE 9. Faults of the Periadriatic fault system (PAFS), the Mid-Hungarian fault system (MHFS) and the Raba extensional fault system. Numbered faults include: 1 – Srednji vrh fault, 2 – Zelenica fault, 3 – Olševa fault, 4 – Podolševa fault, 5 – Matkov Kot fault, 6 – Logarska dolina fault, 7 – Studenec fault, 8 – Javornik fault, 9 – Menina fault, 10 – Vransko fault, 11 – Dravinja fault, 12 – Mlinišče fault.
The dominant faults of the PAFS are the Periadriatic fault, the Sava fault, the Šoštanj fault and the Labot fault (Figure 9). The dextral strike-slip Sava fault is confirmed active, 150 km long, with a geomorphic marker and age dating average estimated slip rate of 1 mm/yr (Vrabec et al., 2006; Jamšek Rupnik et al., 2012; Jamšek Rupnik, 2013). The dextral strike-slip Šoštanj fault is also confirmed active by offsets of Quaternary strata along the fault (Brezigar, 1985), with an estimated average slip rate of 0.5 mm/yr. GNSS data on the Šoštanj fault is so far inconclusive (Pavlovčič et al., 2005). The dextral strike-slip Labot (Lavantal) fault is 201 km long, confirmed active by GNSS measurements, with an average estimated slip rate of 0.75 mm/yr (Pavlovčič Prešeren et al., 2005). These major faults form a dextral-strike slip system/corridor with an average slip rate of ∼1–2 mm/yr.
Two minor reverse faults within the system have produced fault scarps within the Quaternary landforms: the Cerklje and Vodice faults (Verbič, 2006; Jamšek Rupnik et al., 2012, Jamšek Rupnik et al., 2013). The Cerklje fault is related to a restraining bend of the Sava fault (Vrabec, 2001), while the Vodice fault occurs at the transition from PAFS to the Sava folds structural style due east. Genetically, the Vodice fault is probably linked to the Sava folds (Jamšek Rupnik, 2013), although its position south of the Sava fault restraining bend and the Cerklje fault as part of it may suggest a linkage with the PAFS as well.
No major historic earthquakes have been attributed to the PAFS with certainty. The largest event to have been potentially produced by the PAFS is the 1348 M 6.4–7.1 Villach/Carinthia/Friuli earthquake (Stucchi et al., 2012). The earthquake caused significant damage in western Slovenia, southern Austria (Carinthia) and northeastern Italy (Friuli), including the two large landslides. The 1690 Mw 6.5-6.6 Carinthia earthquake was also potentially produced by a fault in the PAFS (e.g. Eisinger & Gutdeutsch, 1994; CPTI Working Group, 2004; Rovida et al., 2011). The 1857 Mw 5.1-5.2 Rosegg earthquake and the 1877 Mw 4.9–5.0 Valbruna earthquake (CPTI Working Group, 2004; Rovida et al., 2011) may also have been caused by the PAFS.
Mid-Hungarian Fault System
The Mid-Hungarian fault system (MHFS) includes regional dextral strike-slip and reverse faults, encompassing the northern part of eastern Slovenia. The MHFS is the northwesternmost part of the Mid-Hungarian Zone, extending to its transition into the PAFS. The MHFS includes one probably active fault (Ljutomer fault) and six potentially active faults (Haloze North and South faults, Dravinja fault, Cerovec fault, Mlinišče fault, and Donat fault) (Figure 9). All faults displace thick sequences of Neogene and Quaternary marine and fluvial clastic sedimentary rocks and sediments. Thus, geologic mapping returns comparatively limited data and more emphasis is put on geomorphic analysis and the very limited available 2D seismic reflection profiles. Consequently, there is more uncertainty in positional and kinematic parameters than with faults in western and central Slovenia.
The northernmost faults in the MHFS are relatively steeply dipping, north-vergent reverse faults, including: the probably active Ljutomer fault and the potentially active Haloze North and Haloze South faults. These faults have been interpreted as reverse faulting along the northern edge of the broader transpressive Donat fault zone (e.g. Fodor et al., 1998). The most distinct of the three reverse faults is the Ljutomer fault, a steeply (70°) southward dipping reverse fault on the northern limb of the large Ormož-Selnica anticline. It is considered probably active, with an estimated slip rate of 0.25 mm/yr. Its probable activity is implied by the dog leg offset of the Mura river. The northwestern part of the MHFS is defined by the potentially active Haloze North and Haloze South reverse faults, dipping southward at 50° and 65–75°, respectively. Estimated slip rates are low, at 0.05 mm/yr for each fault, respectively. The Boč mountain is interpreted as a pop-up structure at the restraining bend between the Labot fault (PAFS) and the Donat fault (MHFS).
The dominant fault in the (Slovenian part of) MHFS is the Donat faut. The fault is dextral strike-slip to dextral transpressive as evidenced by kinematic indicators (Fodor et al., 1998). It is considered potentially active, with the slip rate estimated at 0.65 mm/yr, tentatively based on the assumed slip rate continuity from the Labot and Šoštanj faults.
Historic seismicity in the MHFS is very limited. The 1838 Mw 4.5 Ormož Kog and 1839 Mw 4.8 Ormož Zavrč earthquakes are tentatively attributed to the MHFS, however, the presumed epicentral uncertainty is very large, precluding conclusive attribution (Stucchi et al., 2012).
Sava Compressive Wedge and Balaton Fault System
The Sava compressive wedge (SCW) and Balaton fault system (BFS) are part of a structurally complex zone, comprising diffuse structural transitions from neighboring fault systems and tectonic zones. Motion vectors diverge across the wedge, turning counterclockwise from the north in the western part of the zone, and clockwise from the north in the eastern part of the zone (Serpelloni et al., 2016). The main deformation mechanism in the zone is N-S shortening with several accommodating systems of faults and folds (Figure 10). The SCW and the part of the BFS in Slovenia corresponds to the Sava folds. The Sava folds comprise generally E-W to ENE-WSE trending folds and associated thrusts and reverse faults (Placer, 1998).
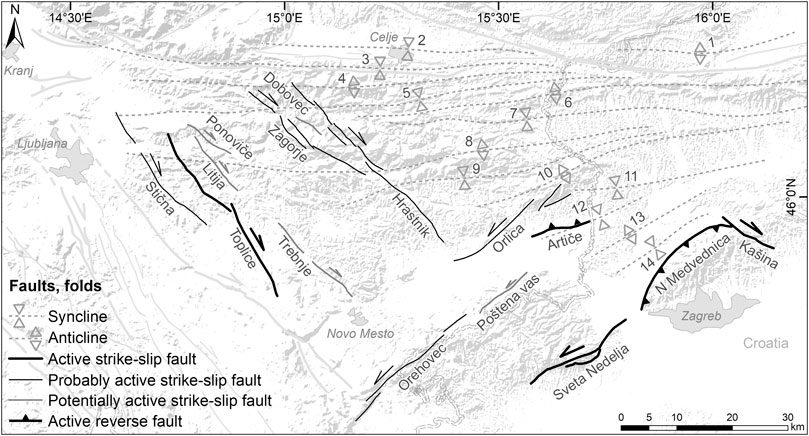
FIGURE 10. Dextral strike-slip faults within the Sava compressive wedge (SCW), faults of the Balaton fault system (BFS) and the Sava folds (from Placer, 1998): 1 - Pletovarje-Macelj anticline, 2 - Celje syncline, 3 - Motnik syncline, 4 - Trojane anticline, 5 - Laško syncline, 6 - Rudnica-Ivančica anticline, 7 - Planina-Desinice syncline, 8 - Litija anticline, 9 - Senovo syncline, 10 - Orlica anticline, 11 - Bizeljsko-Zagorje syncline, 12 - Brezina syncline, 13 - Marija Gorica anticline, 14 - Brdovec syncline.
Folding is confirmed to be ongoing in the SE part of the Sava folds system (Tomljenović and Csontos, 2001). The major Krško syncline is active, with an inferred long-term post-Pontian vertical displacement rate of approximately 0.4 mm/yr. Many studies were carried out on the structure of the Krško basin (e.g. Verbič, 2005; GeoZS, 2010; Cline et al., 2016; Jamšek Rupnik et al., 2016; Poljak, 2017; Atanackov et al., 2018; Bavec et al., 2018). The active 11-km long Artiče blind reverse fault is associated with folding of the E part of the north limb of the Krško syncline. Based on available data and to the best of our knowledge the Artiče and North Medvednica boundary faults are the only currently confirmed Quaternary active reverse faults in the system (the North Medvednica boundary fault is located in Croatia (Matoš et al., 2014)). No definitive evidence of active folding or reverse faulting has been found in the northern part of the Sava folds. This may well be due to the fact that large parts of the area are characterized by a thick succession of Neogene clastics, highly susceptible to weathering, erosion and slope mass movement processes, making identification of faults and their recent activity difficult. We have so far identified a number of short (<5 km) reverse fault and thrust fault segments, however, further work is ongoing to identify potentially related and additional structures. It is likely that extensive work, including geomorphic analysis, geophysical surveys and field mapping will be required to provide additional data.
While reverse faulting and folding is active in the southeastern part of the SCW with BFS (Matoš et al., 2014; Markušić et al., 2020), other parts of the system are overprinted by geometrically and kinematically different faults. In the northern part the structural trends from the SCW transition into the southern part of the PAFS. The nominal boundary is Celje fault, which we attribute to the PAFS mainly for historic reasons.
In the western part of the SCW there is an apparent continuation of dextral strike-slip faults from the DFS, which we consider to be diffusely delimited in the east by the Žužemberk fault. This fault set includes a total of eight dextral strike-slip faults (Figure 10): one active (Toplice fault), three probably (Stična fault, Zagorje fault, Hrastnik fault) and four potentially active faults (Litija fault, Trebnje fault, Ponoviče fault, Dobovec fault). The two longest faults in this fault set are the Toplice fault (37 km) and the Hrastnik fault (49 km). Inferred slip rates are very low on all faults, well below 0.1 mm/yr. Unlike the faults of the DFS, these faults are generally shorter and highly segmented. While these faults may be the diffuse tapering out of the DFS to the east, they may also be at least in part fortuitously aligned tear faults along presumed dominant E-W reverse faults and folds of the Sava folds, as interpreted in the E part of the Sava folds (Tomljenović & Csontos, 2001). Further work is needed on this.
The southeastern part of the SCW is characterized by faults of the BFS: WSW-ENE striking sinistral strike-slip and generally E-W striking reverse faults (Figure 10). The most active part of the system is in Croatia, comprising the major Sveta Nedelja fault and North Medvednica boundary fault. The Sveta Nedelja is a large, 28 km long, deep sinistral strike-slip fault with an estimated average slip rate of 0.22 mm/yr (Herak et al., 2009; Šumanovac et al., 2009). The North Medvednica boundary fault is a major reverse fault (24 km, active, 0.20 mm/yr) (Tomljenović et al., 2008; Basili et al., 2013; Matoš et al., 2014; van Gelder et al., 2015). To the north, in SE Slovenia, the BFS comprises 4 faults: one active (Artiče fault), two probably active (Orehovec fault, Orlica fault) and one potentially active (Poštena vas fault). Slip rates on all four faults are <0.1 mm/yr.
The SCW including BFS is characterized by significant historic seismicity concentrated in its SE part (Ivančić et al., 2006; Herak et al., 2009). The most recent earthquake in the region is the March 22, 2020 Mw 5.3 Zagreb earthquake, most likely produced by the North Medvednica boundary fault (Markušić et al., 2020). The largest event in the region, likely produced by the North Medvednica boundary fault is the 1880 MW 6.0 Zagreb earthquake (Herak et al., 2009; Stucchi et al., 2012). Further significant (M>∼5) events in the vicinity include the earthquakes in 1775, 1837, 1880, 1889 and 1893 (Stucchi et al., 2012). In SE Slovenia the following significant events have occurred: 1917 M 5.7 (Imax = VIII) Brežice earthquake, 1924 M 5.0 and 1928 M 4.8 Brežice earthquakes and the 2015 M 4.2 (Imax = VII) Kostanjevica ob Krki earthquake (ARSO, 2020). The NE part of SCW is characterized by lesser seismicity; the largest historic earthquake is the 1974 M 4.8 Kozjansko event (ARSO, 2020). None of these events have been attributed a causative fault.
Raba Extensional Fault System
The Raba extensional fault system comprises a WSW-ENE system of normal faults (Márton et al., 2002; Fodor et al., 2011). The fault system formed during the early Neogene rifting phase and some faults may have locally been reactivated. Two faults in the database have broadly been attributed to this system–the Lovrenc and Kungota faults in NE Slovenia (Figure 9), however, both are considered potentially active with no firm evidence of recent activity.
Slip Rates and Rate Distribution, Comparison With Regional GNSS Data
We compare slip rate distribution in our fault database to the most complete and most recent published analysis of regional GNSS motion vectors (Serpelloni et al., 2016). There are caveats to be addressed prior to comparison. While GNSS motion vectors are derived from recent and ongoing motion, most fault slip rates are obtained from longer-term data, including offset geomorphic and geological markers. Three distinct zones of ongoing displacement are evident in fault data. The DFS accommodates a total of ∼3.5–4.5 mm/yr dextral strike slip over a NW-SE oriented zone approximately 65 km wide (Figure 11). The PAFS accommodates approximately 1–2 mm/yr dextral strike slip, while the SCW including BFS is tentatively interpreted to accommodate approximately ∼0.5 mm/yr sinistral strike slip. Reverse faults in the SE part of the SCW and BFS and potentially over the entire system likely accommodate significant N-S shortening, however, data is too sparse for a reliable estimate.
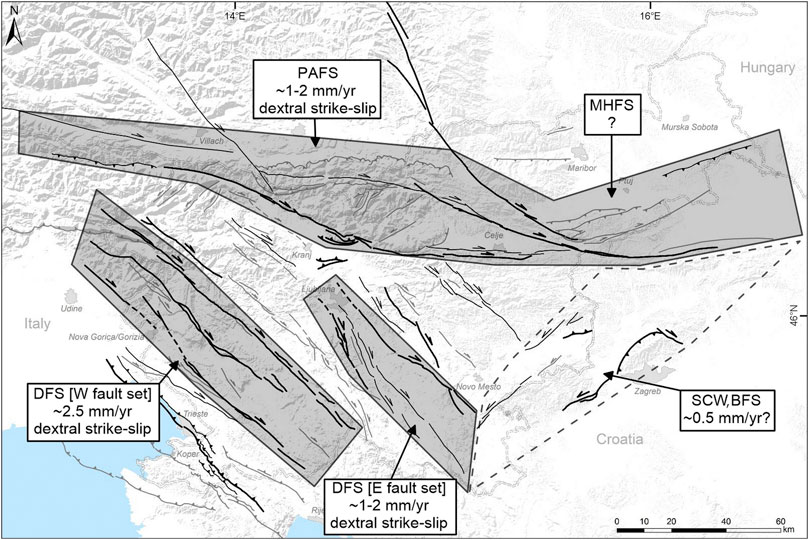
FIGURE 11. Approximate cumulative horizontal displacement/slip rates across the most active fault systems in Slovenia. The Dinaric fault system contains two spatially well-constrained fault sets which concentrate most of the displacement.
GNSS-derived motion horizontal vectors across the DFS (in a fixed Eurasian reference frame) are generally directed northward, with some minor deviations both to the east and west (Serpelloni et al., 2016). The horizontal N-S motion velocity diminishes from approximately 2.5 to 1.5 mm/yr across the DFS western fault set, for an approximate shortening rate of 1.0 mm/yr. This compares to a total northward component of approximately 1.7 mm/yr that we derive from the estimated slip rates across the DFS western fault set. The slip rates across the three major faults of the DFS western fault set are based on long-term offsets of geologic and geomorphic markers and therefore indicate a longer-term average (over several hundred kyr to several Myr). On the other hand, the number of GNSS vectors across the entire DFS is quite limited. A similar consideration for the DFS eastern fault set produces a total northward component of approximately 0.7–1.4 mm/yr from the fault database. This compares to an approximate 0.5–1.0 mm/yr N-S shortening across the fault set indicated by GNSS data.
The GNSS-derived eastward speed increases from approximately 0.0–0.2 mm/yr to approximately 0.8 mm/yr across the PAFS, for a total dextral strike-slip rate of 0.6–0.8 mm/yr. This compares to 1–2 mm/yr derived from estimated fault slip rates. Part of the SCW that includes BFS is characterized by sinistral transpressive movement, with faults showing pure sinistral strike slip (e.g. Sveta Nedelja fault) and reverse motion (North Medvednica boundary fault). It appears the most active faults are at the southern margin of the system, including the Sveta Nedelja fault and the North Medvednica boundary fault. Slip rates appear to diminish toward the north, however, data for these faults is sparse.
Conclusions
We have compiled a new, state-of-the-art fault database for Slovenia. For the first time we have systematically mapped, characterized and parametrized all known active, probably active and potentially active faults in Slovenia, with surface fault traces >5 km. We have attributed individual faults to well-defined fault systems, including the Adriatic foreland fault system, the Dinaric fault system, the Periadriatic fault system, the Mid-Hungarian fault system, the Sava compressive wedge and Balaton fault system and the Raba extensional fault system. All faults are fully geometrically and seismotectonically parametrized. The format and parameters were optimized for translation into a database of composite fault sesimogenic sources. The current version of the fault database contains 96 faults and 241 segments and was compiled for the preparation of the new seismic hazard map in Slovenia. Of the 96 faults in the database, 18 are classified as active, 20 as probably active and 58 as potentially active.
The largest and most active faults in Slovenia the regional dextral-strike slip faults of the Dinaric fault system and the Periadriatic fault system. The longest faults include the Idrija fault (DFS, 124 km), the Sava fault (PAFS, 150 km), the Šoštanj fault (PAFS, 89 km) and the Labot (Lavanttal) fault (PAFS, 180 km; strongly segmented). The two fault systems also contain faults with the highest slip rates, on the order of 0.5–1.0 mm/yr. Reverse faults formed through inversion of Neogene and Quaternary sedimentary basins and therefore typically occur in conjunction with major folding. The systems in which reverse faults occur include the Periadriatic fault system, the Mid-Hungarian fault system, and the Sava compressive wedge and Balaton fault system. Their occurrence in clastic sediments and poorly lithified rock, which are highly susceptible to weathering and erosion, results in obscuration of fault traces and formation of blind faults. The only significant and consistent exception to this are the thrusts and reverse faults in the Adriatic foreland fault system and the Vodice and Cerklje faults in the Ljubljana basin.
We have identified ‘corridors’ where large amounts of deformation occur. The Periadriatic fault system accommodates approximately 1–2 mm/yr of dextral strike slip. The Dinaric fault system contains two distinct fault sets that likely accommodate over 75–95% of the total of approximately 4.5–5.0 mm/yr of dextral strike slip across the fault system. The western fault set accommodates approximately 2.5 mm/yr, about 50–55% of the total dextral strike slip across the fault system. The eastern fault set accommodates approximately 1–2 mm/yr of dextral strike slip, about 20–40% of the total dextral strike slip across the fault system. Lesser, less well constrained activity is noted across other fault systems. The Adriatic foreland fault system is particularly poorly constrained, with little available data. The Mid-Hungarian fault system, the Sava compressive wedge and Balaton fault system and the Raba extensional fault system also lack good data on slip rates, however, total slip rates on the order of ∼0.5–1.0 mm/yr are inferred with some certainty.
We have identified several areas where currently unknown active faults possibly or likely exist, however, their surface expressions are exceedingly vague or the faults are blind. These regions include the Sava folds, the region to the south of the termination of the Avče-Predjama fault, the northern part of the Ljubljana basin, southern Julian Alps and parts of southern Slovenia. The Sava folds require additional extensive, in-depth structural analysis to further constrain the presence, geometry and potential activity of reverse faults and thrusts associated with the formation of the folds. We expect that additional faults from these zones may be added in future versions of the database.
Reconnaissance geomorphic work on the national LiDAR dataset has indicated a number of potential paleoseismological investigation sites on multiple faults. At this time paleoseismological data is available for only a handful of faults and individual locations, insufficient to constrain past behavior of investigated faults. A side product of the fault database is a set of potential future paleoseismological investigation sites.
While the data for seismotectonic parameters has been compiled using a constant set of criteria, there is significant remaining heterogeneity. Slip rates are a particularly highly heterogeneous dataset, with values determined over widely ranging time spans. A significant discrepancy between estimated slip rate values and values based on modeling has been found (e.g. Kastelic and Carafa, 2012) and will need to be addressed in the future. Systematic and methodologically homogeneous nation-wide geodetic investigations will be required to further homogenize the fault slip rate data set.
The fault database has been translated into a database of fault seismogenic sources using the composite source approach, to provide one of geologic inputs into the new seismic hazard map of Slovenia. Seismicity data was extensively used to constrain geometries, kinematic and activity parameters of fault seismic sources. The methodology and results will be the subject of a future paper. Extensive cross-border harmonization of composite seismic sources has been done where possible, however, the fault map requires further cross-border harmonization. The database is being updated regularly with newly published data and results of ongoing research activities. The latest version of the fault database is accessible online available at: tectonics.geo-zs.si.
Data Availability Statement
The paper is fully based on the database of active faults in Slovenia. The database of active faults is part of the ongoing work on a new national map of seismic hazard for Slovenia and is linked to the database of (composite) fault seismic sources. With ongoing acquisition of new data and availability of new publications, including cross-border harmonization, the database will be updated accordingly. The main arguments and conclusions of this paper are state of the art in knowledge on active faults. Future updates to fault parameters are expected. The online version (data repository), with original contributions presented in the study, is available at: tectonics.geo-zs.si. The authors’ main motivation for an online version of the database is to provide users with an always up to date, full database.
Author Contributions
JA-Project Administration, Supervision, Conceptualization, Investigation, Methodology, Writing-original draft, Writing-review & editing; PJR-Project Administration, Conceptualization, Methodology, Investigation, Visualization, Writing-original draft, Writing-review & editing; JJ-Methodology, Investigation, Writing-original draft; BC-Investigation, Writing-original draft; MN-Investigation, Writing-original draft; BM-Investigation, Visualization, Writing-original draft; AM-Investigation, Visualization, Writing-original draft; MB- Investigation, Writing-original draft; VK-Conceptualization, Methodology, Writing-original draft, Writing-review & editing.
Funding
Environment Agency of Slovenia (Project “Elaboration of Seismotectonic maps”™), and Slovenian Research Agency (Program Regional Geology P1-0011).
Conflict of Interest
The authors declare that the research was conducted in the absence of any commercial or financial relationships that could be construed as a potential conflict of interest.
Acknowledgments
VK acknowledges the support from the Seismology and Earthquake Engineering Research Infrastructure Alliance for Europe (SERA) project (European Union Horizon 2020 research and innovation program grant agreement No.730900). The authors thank I. Rižnar, M. Poljak and L. Placer for valuable discussions and reality checks. A. Gosar, P. Zupančič, M. Živčić, B. Šket-Motnikar and M. Čarman from the Environment Agency of Slovenia are greatly acknowledged for many fruitful discussions during elaboration of the database and for yearly reviews of the database. Two reviewers and the editor are acknowledged for their comments that helped improve the paper.
References
Anderson, H., and Jackson, J. (1987). Active tectonics of the Adriatic region. Geophys. J. Int. 91 (3), 937–983. doi:10.1111/j.1365-246x.1987.tb01675.x
Aoudia, A., Saraó, A., Bukchin, B., and Suhadolc, P. (2000). The 1976 Friuli (NE Italy) thrust faulting earthquake: a reappraisal 23 years later. Geophys. Res. Lett. 27 (4), 573–576. doi:10.1029/1999gl011071
ARSO (2020). Močni potresi v preteklosti (Strong earthquakes in the past). Available at: http://www.arso.gov.si/potresi/potresna%20aktivnost/Mo%c4%8dni_potresi_v_preteklosti.pdf (in Slovenian, with English summary). (Accessed April 20, 2020).
Atanackov, J., Jamšek Rupnik, P., Rižnar, I., Bavec, M., Petronio, L., Cline, K., et al. (2018). “High-resolution seismic reflection surveys in the Krško basin,” in Rman, N., 5. slovenski geološki kongres, Zbornik povzetkov, Velenje, 3–5 oktober 2018. Editor M. Novak (Ljubljana, Slovenia: Geological Survey of Slovenia).
Atanackov, J. (2013). Uporaba in optimizacija visokoločljive refleksijske seizmične metode v raziskavah neotektonike. = Application and optimisation of high-resolution seismic reflection method for investigations in neotectonics. PhD thesis. Ljubljana (Slovenia): University of Ljubljana, 261.
Bajc, J., Aoudia, A., Saraò, A., and Suhadolc, P. (2001). The 1998 Bovec-Krn Mountain (Slovenia) earthquake sequence. Geophys. Res. Lett. 28 (9), 1839–1842. doi:10.1029/2000gl011973
Basili, R., Valensise, G., Vannoli, P., Burrato, P., Fracassi, U., Mariano, S., et al. (2008). The Database of Individual Seismogenic Sources (DISS), version 3: summarizing 20 years of research on Italy's earthquake geology. Tectonophysics 453 (1–4), 20–43. doi:10.1016/j.tecto.2007.04.014
Basili, R., Kastelic, V., Demircioglu, M. B., Garcia Moreno, D., Nemser, E. S., Petricca, P., et al. (2013). The European database of seismogenic faults (EDSF). Compiled Framework Project SHARE. doi:10.6092/INGV.IT-SHARE-EDSF
Basili, R., Danciu, L., Carafa, M. M. C., Kastelic, V., Maesano, F. E., Tiberti, M. M., et al. (2020). “Insights on the European fault-source model (EFSM20) as input to the 2020 update of the European seismic hazard model (ESHM20),” in EGU general assembly 2020, Lund, Sweden, 4–8 May 2020, 7008.
Bavec, M., Atanackov, J., Celarc, B., Hajdas, I., Jamšek Rupnik, P., Jež, J., et al. (2013). “Evidence of Idrija fault seismogenic activity during the Late Holocene including the 1511 Mm 6.8 earthquake,” in Seismic hazard, critical facilities and slow active faults: proceedings of the 4th international INQUA meeting on paleoseismology, active tectonics and archeoseismology (PATA days), 9–15 october 2013, Aachen, Germany. Editor C. Grützner (Aachen, Germany: Grützner & Reicherter Geosolutions, Aachen UG), 23-26.
Bavec, M., Cline, K., Atanackov, J., Jamšek Rupnik, P., Cline, M., et al. (2018). “Recent geological and seismotectonic investigations in Krško basin with respect to planning complex infrastructure,” in Rman, N., 5. slovenski geološki kongres, Zbornik povzetkov, Velenje, 3–5 oktober 2018. Editor M. Novak (Ljubljana, Slovenia: Geological Survey of Slovenia).
Brezigar, A. (1985). Premogova plast Rudnika lignita Velenje = Coal seam of the Velenje coal mine. Geologija 28–29, 319–336.
BRGM-EDF-IRSN/SisFrance (2010). Histoire et caractéristiques des séismes ressentis en France. Available at: http://www.sisfrance.net/.
Brückl, E., Behm, M., Decker, K., Grad, M., Guterch, A., Keller, G. R., et al. (2010). Crustal structure and active tectonics in the Eastern Alps. Tectonics 29 1–17. doi:10.1029/2009TC002491
Buser, S., Grad, K., and Pleničar, M. (1967). Osnovna geološka karta SFRJ 1:100.000. List Postojna. Beograd, Serbia: Zvezni geološki zavod, L33–77.
Busetti, M., Volopi, V., Nicolich, R., Barison, E., Romeo, R., Baradello, L., et al. (2010). Dinaric tectonic features in the gulf of Trieste (northern Adriatic Sea). Bolletino di Geofisica Teorica ed. Applicata 51 (2–3), 117–128.
Caporali, A., Aichhorn, C., Becker, M., Fejes, I., Gerhatova, L., Ghitau, D., et al. (2008). Geokinematics of central Europe: new insights from the CERGOP-2/environment project. J. Geodyn. 45, 246–256. doi:10.1016/j.jog.2008.01.004
Caporali, A., Neubauer, F., Ostini, L., Stangl, G., and Zuliani, D. (2013). Modeling surface GPS velocities in the Southern and Eastern Alps by finite dislocations at crustal depths. Tectonophysics 590, 136–150. doi:10.1016/j.tecto.2013.01.016
Čar, J. (2010). Geological structure of the Idrija – Cerkno hills between Stopnik and Rovte 1: 25.000, explanatory book. Ljubljana, Slovenia: Geological survey of Slovenija.
Carulli, G. B. (2011). Structural model of the Trieste gulf: a proposal. J. Geodyn. 51 (1), 156–165. doi:10.1016/j.jog.2010.05.004
Cline, M. L., Cline, K., M., Jamšek Rupnik, P., Atanackov, J., Bavec, M., and Lowick, S. E. (2016). “Tectonic geomorphology and geochronology supporting a probablistic seismic hazard analysis in the Krško Basin, Slovenia: implications for a critical infrastructure,” in 7th international INQUA meeting on paleoseismology, active tectonics and archeoseismology (PATA days), Editors J. McCalpin, and C. Gruetzner (Crestone, CO: Crestone Science Center), 63–66.
CPTI Working Group (2004). Catalogo Parametrico dei Terremoti Italiani, versione 2004 (CPTI04). Ist. Naz. Geofis. Vulcanologia (INGV). doi:10.6092/INGV.IT-CPTI04
Csontos, L., Nagymarosy, A., and Nagymarosy, A. (1998). The Mid-Hungarian line: a zone of repeated tectonic inversions. Tectonophysics 297 (1), 51–71. doi:10.1016/s0040-1951(98)00163-2
DISS Working Group (2018). Database Individual Seismogenic Source (DISS), Data from: version 3.2.1: a compilation of potential sources for earthquakes larger than M 5.5 in Italy and surrounding areas. Available at: http://diss.rm.ingv.it/diss/.
Eisinger, U., and Gutdeutsch, R. (1994). “The Villach earthquake of December 4th, 1690 in the German sources,” in Materials of the CEC project "review of historical seismicity in Europe. Editor P. A. Albinie Moroni (Milano, Italy: CNR), 133–137.
Emre, Ö., Duman, T. Y., Özalp, S., Şaroğlu, F., Olgun, Ş., Elmacı, H., et al. (2018). Active fault database of Turkey. Bull. Earthquake Eng. 16, 3229–3275. doi:10.1007/s10518-016-0041-2
Fitzko, F., Suhadolc, P., Aoudia, A., and Panza, G. F. (2005). Constraints on the location and mechanism of the 1511 Western-Slovenia earthquake from active tectonics and modeling of macroseismic data. Tectonophysics 404 (1–2), 77–90. doi:10.1016/j.tecto.2005.05.003
Fodor, L, Jelen, B, Márton, E, Rifelj, H, Kraljić, M, and Kevrić, R (2002). Miocene to Quaternary deformation, stratigraphy and paleogeography in Northeastern Slovenia and Southwestern Hungary = Deformacije, stratigrafija in paleogeografija severovzhodne Slovenije in jugozahodne Madžarske od miocena do kvartarja. Geologija 45 (1), 103–114.
Fodor, L., Jelen, B., Márton, E., Skaberne, D., Čar, J., and Vrabec, M. (1998). Miocene-Pliocene tectonic evolution of the Slovenian Periadriatic fault: implications for Alpine-Carpathian extrusion models. Tectonics 17 (5), 690–709. doi:10.1029/98tc01605
Fodor, L., Uhrin, A., Palotás, K., Selmeczi, I., Nádor, A., Tóth-Makk, Á., et al. (2011). Geological conceptual model within the framework of project T-JAM. Ljubljana, Budapest, Geological Survey of Slovenia, Magyar Állami Földtani Intézet. Available at: https://issuu.com/t-jam/docs/geological_model (Accessed December 7, 2020).
Galadini, F., Falcucci, E., Galli, P., Giaccio, B., Gori, S., Messina, P., et al. (2012). Time intervals to assess active and capable faults for engineering practices in Italy. Eng. Geol. 139–140, 50–65. doi:10.1016/j.enggeo.2012.03.012
Galadini, F., Poli, M. E., and Zanferrari, A. (2005). Seismogenic sources potentially responsible for earthquakes withM≥ 6 in the eastern Southern Alps (Thiene-Udine sector, NE Italy). Geophys. J. Int. 161, 739–762. doi:10.1111/j.1365-246x.2005.02571.x
Ganas, A., Oikonomou, I. A., and Tsimi, C. (2013). NOAfaults: a digital database for active faults in Greece. Bull. Geol. Soc. Greece 47, 518. doi:10.12681/bgsg.11079
García-Mayordomo, J., Insua-Arévalo, J. M., Martínez-Díaz, J. J., Jiménez-Díaz, A., Martín-Banda, R., Martín-Alfageme, S., et al. QAFI Compilers Working Group (2012). The Quaternary faults database of Iberia (QAFI v.2.0). J. Iberian Geol. 38 (1), 285–302. doi:10.5209/rev_jige.2012.v38.n1.39219
Georgescu, E. S., Borcia, I. S., Matei, C. L., Craifaleanu, I. G., Dragomir, C. S., Dobre, D., et al. (2013). “The Skopje, Macedonia, earthquake of 1963 vs. Vrancea, Romania, earthquake of 1977. Long-run impacts in earthquake engineering,” in International Conference on Earthquake Engineering. 50 years from the Skopje catastrophic earthquake, Skopje, Macedonia, July 1963.
GeoZS – Geološki zavod Slovenije (2010). Geotechnical, geological, and seismological (GG&S) evaluations for the new nuclear power plant at the Krško site (NPP Krško II): Geology – phase 1, rev. 1. Ljubljana, Slovenia: Geological Survey of Slovenia, 178.
Gosar, A. (2012). Application of environmental seismic intensity scale (ESI 2007) to Krn Mountains 1998 M w= 5.6 earthquake (NW Slovenia) with emphasis on rockfalls. Nat. Hazards Earth Syst. Sci. 12 (5) 1659–1670. doi:10.5194/nhess-12-1659-2012
Gosar, A., and Božiček, B. (2006). Structural maps of seismic horizons in the Krško basin = Strukturne karte seizmičnih horizontov v Krški kotlini. RMZ - Mater. Geoenviron. 53 (3), 339–352.
Gosar, A. (2008). Gravity modelling along seismic reflection profiles in the Krsko basin (SE Slovenia). Geologica Carpathica 59 (2), 147–158.
Gosar, A. (2019a). Review of geological and seismotectonic investigations related to 1998 Mw5.6 and 2004 Mw5.2 earthquakes in Krn Mountains. Geologija 62 (1), 61–73. doi:10.5474/geologija.2019.002
Gosar, A. (2019b). Review of geological and seismotectonic investigations related to 1998 Mw5.6 and 2004 Mw5.2 earthquakes in Krn Mountains. Geologija 62 (1), 61–73. doi:10.5474/geologija.2019.002
Gosar, A., Šebela, S., Košták, B., and Stemberk, J. (2011). On the state of the TM 71 extensometer monitoring in Slovenia: seven years of micro-tectonic displacement measurements. Acta Geodyn. Geomater. 8 (4), 389–402.
Gospodarič, R. (1989). Prispevek k vodnogospodarskim osnovam Pivke. = the contribution to the water economy bases of Pivka. Acta Carsologica 18, 21–38.
Gosar, A., Šebela, S., Košták, B., and Stemberk, J. (2009). Surface versus underground measurements of active tectonic displacements detected with TM 71 extensometers in Western Slovenia. Acta Carsologica 38 (2–3), 213–226. doi:10.3986/ac.v38i2-3.123
Grenerczy, G., Sella, G., Stein, S., and Kenyeres, A. (2005). Tectonic implications of the GPS velocity field in the northern Adriatic region. Geophys. Res. Lett. 32 (16), L16311. doi:10.1029/2005GL022947
Grünthal, G., Wahlström, R., and Stromeyer, D. (2013). The SHARE European earthquake catalogue (SHEEC) for the time period 1900-2006 and its comparison to the European-Mediterranean Earthquake Catalogue (EMEC). J. Seismol 17, 1339–1344. doi:10.1007/s10950-013-9379-y
Grünthal, G., and Wahlström, R. (2012). The European-Mediterranean Earthquake Catalogue (EMEC) for the last millennium. J. Seismol 16 (3), 535–570. doi:10.1007/s10950-012-9302-y
Guidoboni, E., Ferrari, G., Mariotti, D., Comastri, A., Tarabusi, G., and Valensise, G. (2007). CFTI4Med, catalogue of strong earthquakes in Italy (461 B.C.-1997) and Mediterranean area (760 B.C.-1500). INGV-SGA Available at: http://storing.ingv.it/cfti4med/ (Accessed August 2007).
Haller, K. M., Machette, M. N., Dart, R. L., and Rhea, B. S. (2011). U.S. Quaternary fault and fold database released. Eos Trans. AGU 85 (22), 218. doi:10.1029/2004eo220004
Hammerl, C. (1994). “The earthquake of January 25th, 1348: discussion of source,” in Historical investigations of European Earthquakes Materials of CEC project ‘review of historical seismicity in Europe’ and further contributions. Editors P. Albini, and A. Moroni (Pisa, Italy: Instituto di Ricerca sul Rischio Sismico), Vol. 2, 225–240.
Handy, M. R., Ustaszewski, K., and Kissling, E. (2014). Reconstructing the Alps-Carpathians-Dinarides as a key to understanding switches in subduction polarity, slab gaps and surface motion. Int. J. Earth Sci. 104, 1. doi:10.1007/s00531-014-1060-3
Heidbach, O., Tingay, M., Barth, A., Reinecker, J., Kurfeß, D., and Müller, B. (2008). The world stress map database release 2008. Deutsches GeoForschungsZentrum GFZ. doi:10.1594/GFZ.WSM.Rel2008
Herak, D., Herak, M., and Tomljenović, B. (2009). Seismicity and earthquake focal mechanisms in North-Western Croatia. Tectonophysics 465, 212–220. doi:10.1016/j.tecto.2008.12.005
Horváth, F., Musitz, B., Balázs, A., Végh, A., Uhrin, A., Nádor, A., et al. (2015). Evolution of the Pannonian basin and its geothermal resources. Geothermics 53, 328–352. doi:10.1016/j.geothermics.2014.07.009
Ivančić, I., Herak, D., Markušić, S., Sović, I., and Herak, M. (2006). Seismicity in Croatia in the period 2002-2005. Geofizika 23 (2), 88–103.
Jamšek Rupnik, P., Benedetti, L., Bavec, M., and Vrabec, M. (2012). Geomorphic indicators of Quaternary activity of the Sava fault between Golnik and Preddvor. RMZ – Mater. Geoenviron. 59 (2–3), 299–314.
Jamšek Rupnik, P. (2013). Geomorphological evidence of active tectonics in the Ljubljana Basin. Doctoral Dissertation. Ljubljana, Slovenia: University of Ljubljana, 214.
Jamšek Rupnik, P., Benedetti, L., Preusser, F., Bavec, M., and Vrabec, M. (2013). Geomorphic evidence of recent activity along the Vodice thrust fault in the Ljubljana Basin (Slovenia) – a preliminary study. Ann. Geophys. Spec. Issue 56 (6) 1–10. doi:10.4401/ag-6252
Jamšek Rupnik, P., Atanackov, J., Skaberne, D., Jež, J., Milanič, B., Novak, M., et al. (2015). “Paleoseismic evidence of the Vodice fault capability (Ljubljana basin, Slovenia),” in Proceedings of the 6th International INQUA Meeting on Paleoseismology, Active Tectonics and Archaeoseismology, 19–24 April, 2015, Pescina, Italy: Istituto nazionale di geofisica e vulcanologia, 423–426.
Jamšek Rupnik, P., Atanackov, J., Cline, M., Cline, K., M., Rižnar, I., et al. (2016). “Fault characterization field campaign related to probabilistic seismic hazard assessment for nuclear infrastructure in the Krško Basin, Slovenia,” in International INQUA workshop on paleoseismology, active tectonics and archaeoseismology (PATA days), 30 May–3 June 2016. Editors J. McCalpin, and C. Gruetzner (Crestone, CO): Crestone Science Center. 128–131.
Jurkovšek, B. (2008). Geological map of the northern part of the Trieste-Komen plateau 1:25.000. Ljubljana, Slovenia: Geological Survey of Slovenia.
Kastelic, V., and Carafa, M. M. C. (2012). Fault slip rates for the active External Dinarides thrust-and-fold belt. Tectonics 31 1–18. doi:10.1029/2011TC003022
Kastelic, V. (2008). Seismotectonic study of Ravne fault and 1998 and 2004 Upper Posočje earthquakes. Ph.D. Thesis. Ljubljana, Slovenia: University of Ljubljana, 112.
Kastelic, V., Vrabec, M., Cunningham, D., and Gosar, A. (2008). Neo-Alpine structural evolution and present-day tectonic activity of the eastern Southern Alps: the case of the Ravne Fault, NW Slovenia. J. Struct. Geol. 30 (8), 963–975. doi:10.1016/j.jsg.2008.03.009
Komac, M., and Hribernik, K. (2015). Slovenian national landslide database as a basis for statistical assessment of landslide phenomena in Slovenia. Geomorphology 249, 94–102. doi:10.1016/j.geomorph.2015.02.005
Komac, M. (2012). Regional landslide susceptibility model using the Monte Carlo approach - the case of Slovenia. Geol. Q. 56 (1), 41–54.
Komac, M., and Ribičič, M. (2006). Landslide susceptibility map of Slovenia at scale 1:250,000. Geologija 49 (2), 295–309. doi:10.5474/geologija.2006.022
Langridge, R., Ries, W., Litchfield, N., Villamor, P., Van Dissen, R., Barrell, D., et al. (2016). The New Zealand active faults database. N. Z. J. Geol. Geophys. 59, 86–96. doi:10.1080/00288306.2015.1112818
Lapajne, J., Šket Motnikar, B., and Zupančič, P. (2001). Potresna nevarnost Slovenije: projektni pospešek tal 1:500.000. Ljubljana, Slovenia: Ministrstvo za okolje in prostor, Uprava Republike Slovenije za geofiziko.
Lapajne, J. (1989). Veliki potresi na slovenskem - III: potres v Ljubljani leta 1895 = great earthquakes in Slovenia - Part III: the Ljubljana earthquake of 1895. Ujma 3, 55–61.
Marjanac, T., Marjanac, L., Poljak, M., Živčič, M., and Bavec, M. (2001). Srpenica seismites - indicators of paleoseismicity in the Upper Soča valley, NW Slovenia. Geologija 44 (2), 341–350. doi:10.5474/geologija.2001.026
Markušić, S., Stanko, D., Korbar, T., Belić, N., Penava, D., and Kordić, B. (2020). The Zagreb (Croatia) M5.5 earthquake on 22 March 2020. Geosciences 10 (7), 252. doi:10.3390/geosciences10070252
Márton, E., Fodor, L., Jelen, B., Márton, P., Rifelj, H., and Kevrić, R. (2002). Miocene to quaternary deformation in NE Slovenia. J. Geodyn. 34 (5), 627–651. doi:10.1016/s0264-3707(02)00036-4
Matoš, B., Tomljenović, B., and Trenc, N. (2014). Identification of tectonically active areas using DEM: a quantitative morphometric analysis of Mt. Medvednica, NW Croatia. Geol. Q. 58 (1), 51–70. doi:10.7306/gq.1130
Merchel, S., Mrak, I., Braucher, R., Benedetti, L., Repe, B., Bourlès, D. L., et al. (2014). Surface exposure dating of the Veliki vrh rock avalanche in Slovenia associated with the 1348 earthquake. Quat Geochronol. 22, 33–42. doi:10.1016/j.quageo.2014.02.002
McCalpin, J. P. (2009). Paleoseismology. International geophysics series 95. 2nd Edn (Burlington, MA: Academic Press, Elsevier).
Milanič, B. (2010). Ocena recentnih tektonskih premikov v severozahodni Sloveniji na podlagi primerjave PSInSAR podatkov in nivelmanske izmere = Estimate of recent tectonic movement in northwest Slovenia based on the comparison of PSInSAR data and levelling data. Master’s thesis. Ljubljana (Slovenia): University of Ljubljana.
Monegato, G., and Poli, M. E. (2015). Tectonic and climatic inferences from the terrace staircase in the Meduna valley, eastern Southern Alps, NE Italy. Quat. Res. 83, 229–242. doi:10.1016/j.yqres.2014.10.001
Moulin, A., Benedetti, L., Gosar, A., Rupnik, P. J., Rizza, M., Bourlès, D., et al. (2014). Determining the present-day kinematics of the Idrija fault (Slovenia) from airborne LiDAR topography. Tectonophysics 628, 188–205. doi:10.1016/j.tecto.2014.04.043
Moulin, A., Benedetti, L., Rizza, M., Jamšek Rupnik, P., Gosar, A., Bourlès, D., et al. (2016). The Dinaric fault system: large-scale structure, rates of slip, and Plio-Pleistocene evolution of the transpressive northeastern boundary of the Adria microplate. Tectonics 35 (10), 2258–2292. doi:10.1002/2016TC004188
Papadopoulos, G. A., Daskalaki, E., Fokaefs, A., and Giraleas, N. (2007). Tsunami hazards in the Eastern Mediterranean: strong earthquakes and tsunamis in the East Hellenic Arc and Trench system. Nat. Hazards Earth Syst. Sci. 7, 57–64. doi:10.5194/nhess-7-57-2007
Pavlovčič Prešeren, P., Stopar, B., and Vrabec, M. (2005). Hitrost premikov ob prelomih v vzhodni Sloveniji: opazovanja iz let 1996, 1999 in 2002. = Displacement rates along the faults in NE Slovenia: campaigns from 1996, 1999 and 2002. Geodetski vestnik 49, 407–415.
Placer, L. (1982). Tektonski razvoj idrijskega rudišča = Structural history of the Idrija mercury deposit. Geologija 25 (1), 7–94.
Placer, L. (1998). Structural meaning of the Sava folds. Geologija 41, 191–221. doi:10.5474/geologija.1998.012
Placer, L. (2008). Principles of the tectonic subdivision of Slovenia. Geologija 51 (2), 205–217. doi:10.5474/geologija.2008.021
Placer, L., Živčić, M., and Vrabec, M. (2001). “Recent deformation and seismic activity at the Adria microplate boundary in Western Slovenia and Croatia,” in Quantitative neotectonics and seismic hazard assessment: new integrated approaches for environmental management. Editor G. Bada (Balaton, Hungary: Malév Air Tours), 74–75.
Placer, L., Vrabec, M., and Celarc, B. (2010). The bases for understanding of the NW Dinarides and Istria Peninsula tectonics. Geologija 53 (1), 55–86. doi:10.5474/geologija.2010.005
Poli, M. E., and Zanferrari, A. (2017). A new seismotectonic model for the Friuli area. GNGTS 2017. Available at: http://www3.ogs.trieste.it/gngts/files/2017/S12/Riassunti/Poli.pdf (Accessed April 20, 2020).
Poli, M. E., and Zanferrari, A. (2018). The seismogenic sources of the 1976 Friuli earthquakes: a new seismotectonic model for the Friuli area. Boll. Geofis. Teorica Ed. Appl. 59 (4), 463–480. doi:10.4430/bgta0209
Poljak, M. (2017). Geological map of the eastern part of the Krško basin 1:25,000. Explanatory booklet. Ljubljana: Geological Survey of Slovenia, 108.
Poljak, M., Gosar, A., and Živčić, M. (2010). “Active tectonics in Slovenia” in geology of the Adriatic area,” in International Geological Congress on the Adriatic Area (ADRIA 2006), Urbino, 19-20 June 2006 (Bologna, Italy, University of Bologna, Department of Earth and Geological-Environmental Sciences), 15–24.
Poljak, M., Živčić, M., and Zupančič, P. (2000). The seismotectonic characteristics of Slovenia. Pure Appl. Geophys. 157 (1–2), 37–55. doi:10.1007/pl00001099
Pondrelli, S., Salimbeni, S., Ekström, G., Morelli, A., Gasperini, P., and Vannucci, G. (2006). The Italian CMT dataset from 1977 to the present. Phys. Earth Planet. Interiors 159 (3-4), 286–303. doi:10.1016/j.pepi.2006.07.008
Postpischl, D. (1985). Catalogo dei terremoti italiani dall’anno 1000 al 1980. Consiglio Nazionale delle Ricerche, Progetto Finalizzato Geodinamica (CNR-PFG). Quad. Ric. Sci. 1145 (2), 239.
Rapuc, W., Sabatier, P., Andrič, M., Crouzet, C., Arnaud, F., Chapron, E., et al. (2018). 6600 years of earthquake record in the Julian Alps (Lake Bohinj, Slovenia). Sedimentology 65, 1777–1799. doi:10.1111/sed.12446
Rovida, A., Camassi, R., Gasperini, P., and Stucchi, M. (2011). CPTI11, the 2011 version of the parametric catalogue of Italian earthquakes. Milano, Bologna: Istituto Nazionale di Geofisica e Vulcanologia (INGV). doi:10.6092/INGV.IT-CPTI11
Ribarič, V. (1979). The Idrija earthquake of March 26, 1511 - a reconstruction of some seismological parameters. Tectonophysics 53 (3-4), 315–324. doi:10.1016/0040-1951(79)90076-3
Ribarič, V. (1982). Seismicity of Slovenia. Katalogue of earthquakes (792 A.D.–1981). Ljubljana, Slovenije: Seizmološki zavod SR Slovenije, 649.
Rižnar, I., Koler, B., and Bavec, M. (2005). Identification of potentially active structures along the Sava River using topographic, and leveling line data. Geologija 48 (1), 107–116. doi:10.5474/geologija.2005.010
Rižnar, I., Koler, B., and Bavec, M. (2007). Recentna aktivnost regionalnih geoloških struktur v zahodni Sloveniji. = Recent activity of the regional geologic structures in western Slovenia. Geologija 50 (1), 111–120. doi:10.5474/geologija.2007.009
Savić, D., and Dozet, S. (1985). Osnovna geološka karta SFRJ, list Delnice, 1:100.000. Beograd, Serbia: Zvezni geološki zavod.
Schmid, S. M., Scharf, A., Handy, M. R., and Rosenberg, C. L. (2013). The Tauern Window (Eastern Alps, Austria): a new tectonic map, with cross-sections and a tectonometamorphic synthesis. Swiss J. Geosci. 106, 1. doi:10.1007/s00015-013-0123-y
Schmid, S. M., Fügenschuh, B., Kounov, A., Maţenco, L., Nievergelt, P., Oberhänsli, R., et al. (2020). Tectonic units of the Alpine collision zone between Eastern Alps and western Turkey. Gondwana Res. 78, 308–374. doi:10.1016/j.gr.2019.07.005
Šebela, S. (2005). Tektonske zanimivosti Pivške kotline. = Tectonic sights of the Pivka basin. Acta Carsologica 34 (3), 566–581.
Serpelloni, E., Vannucci, G., Anderlini, L., and Bennett, R. A. (2016). Kinematics, seismotectonics and seismic potential of the eastern sector of the European Alps from GPS and seismic deformation data. Tectonophysics 688, 157–181. doi:10.1016/j.tecto.2016.09.026
Shaw, B., Ambraseys, N. N., England, P. C., Floyd, M. A., Gorman, G. J., Higham, T. F. G., et al. (2008). Eastern Mediterranean tectonics and tsunami hazard inferred from the AD 365 earthquake. Nat. Geosci. 1, 268–276. doi:10.1038/ngeo151
Stiros, S. C. (2010). The 8.5+ magnitude, AD365 earthquake in Crete: coastal uplift, topography changes, archaeological and historical signature. Quat. Int. 216 (1–2), 54–63. doi:10.1016/j.quaint.2009.05.005
Stucchi, M., Rovida, A., Rovida, A., Gomez Capera, A. A., Alexandre, P., Camelbeeck, T., et al. (2012). The SHARE European earthquake catalogue (SHEEC) 1000-1899. J. Seismol 17 (2), 523–544. doi:10.1007/s10950-012-9335-2
Styron, R., and Pagani, M. (2020). The GEM global active faults database. Earthquake Spectra 36 (1), 160–180. doi:10.1177/8755293020944182
Šumanovac, F., and Orešković, J. (2009). The ALP 2002 Working GroupCrustal structure at the contact of the Dinarides and Pannonian basin based on 2-D seismic and gravity interpretations of the Alp07 profile in the ALP 2002 experiment, Geophys. J. Int. 179, 615–633.
Tiberi, L., Costa, G., Jamšek Rupnik, P., Cecić, I., and Suhadolc, P. (2018). The 1895 Ljubljana earthquake: can the intensity data points discriminate which one of the nearby faults was the causative one?. J. Seismol 22, 927–941. doi:10.1007/s10950-018-9743-z
Tomljenović, B., Csontos, L., Marton, E., and Marton, P. (2008). Tectonic evolution of the northwestern Internal Dinarides as constrained by structures and rotation of Medvednica mountains, north Croatia. Geol. Soc. Lond. Spec. Publications 298, 145–167.
Tomljenović, B., and Csontos, L. (2001). Neogene–Quaternary structures in the border zone between Alps, Dinarides and Pannonian Basin (Hrvatsko zagorje and Karlovac Basins, Croatia). Int. J. Earth Sci. 90 (3), 560–578. doi:10.1007/s005310000176
U.S. Geological SurveyAlaska Department of Natural ResourcesArizona Geological SurveyCalifornia Geological SurveyColorado Geological SurveyIdaho Geological SurveyIllinois State Geological SurveyLouisiana Geological SurveyMontana Bureau of Mines and GeologyNevada Bureau of Mines and GeologyNew Mexico Bureau of Mines and Mineral ResourcesTexas Bureau of Economic GeologyUtah Geological Survey (2020). Quaternary fault and fold database for the United States. Available at: https://www.usgs.gov/natural-hazards/earthquake-hazards/faults (Accessed December 10, 2020).
van Gelder, I. E., Matenco, L., Willingshofer, E., Tomljenović, B., Andriessen, P. A. M., Ducea, M. N., et al. (2015). The tectonic evolution of a critical segment of the Dinarides‐Alps connection: kinematic and geochronological inferences from the Medvednica Mountains, NE Croatia. Tectonics 34 (9), 1952–1978. doi:10.1002/2015tc003937
Verbič, T. (2005). Quaternary stratigraphy and neotectonics of the eastern Krško basin. Part 2: neotectonics. Razprave 46 (1), 171–216.
Verbič, T. (2006). Quaternary-active reverse faults between Ljubljana and Kranj, central Slovenia. Razprave 47 (2), 101–142.
Vičič, B., Aoudia, A., Javed, F., Foroutan, M., and Costa, G. (2019). Geometry and mechanics of the active fault system in western Slovenia. Geophys. J. Int. 217, 1755–1766. doi:10.1093/gji/ggz118
Vidrih, R., and Godec, M. (1995). Ljubljanski potres leta 1895 in njegov vpliv na razvoj gradbeno-tehničnih predpisov = the 1895 Ljubljana Earthquake and its Influence on the development of Technical-construction regulations. Ujma 9, 231–237.
Vrabec, M. (2001). Strukturna analiza cone Savskega preloma med Trstenikom in Stahovico. PhD thesis. Ljubljana (Slovenia): University of Ljubljana, 94.
Vrabec, M., and Fodor, L. (2006). “Late Cenozoic tectonics of Slovenia: structural styles at the Northeastern corner of the Adriatic microplate,” in The Adria microplate: GNSS geodesy, tectonics and hazards (NATO science series IV, Earth and environmental Sciences 61). Editors N. Pinter, N. Grenerczy, J. Weber, S. Stein, and D. Medak (Dordrecht, Netherlands: Springer), 151–168.
Vrabec, M., Pavlovčič Prešeren, P., and Stopar, B. (2006). GNSS study (1996–2002) of active deformation along the Periadriatic fault system in northeastern Slovenia: tectonic model. Geologica Carpathica 57 (1), 57–65.
Vrabec, M., Sterle, O., Weber, J., and Stopar, B. (2011). Adria microplate motion and active deformation in the surrounding Dinaric - Alpine transition from GPS. Geophys. Res. Abstr. 13, 13021.
Wallace, R. E. (1986). Active tectonics: studies in geophysics (Washington, DC: National Academy Press), 266.
Weber, J., Vrabec, M., Pavlovčič-Prešeren, P., Dixon, T., Jiang, Y., and Stopar, B. (2010). GPS-derived motion of the Adriatic microplate from Istria Peninsula and Po Plain sites, and geodynamic implications. Tectonophysics 483, 214–222. doi:10.1016/j.tecto.2009.09.001
Weber, J., Vrabec, M., Stopar, B., Pavlovcic-Preseren, P., and Dixon, T. (2006). “The Pivo-2003 experiment: a gaps study of Istria peninsula and Adria microplate motion and active tectonics in Slovenia,” in The Adria microplate: GNSS geodesy, tectonics and hazards (NATO science series IV, Earth and environmental Sciences 61). Editors N. Pinter, N. Grenerczy, J. Weber, S. Stein, and D. Medak (Dordrecht, Netherlands: Springer), 305–320.
Wells, D., and Coppersmith, K. (1994). New empirical relationships among magnitude, rupture length, rupture width, rupture area, and surface displacement. BSSA 84 (4), 974–1002.
Yeats, R. (2012). Active faults of the world. Cambridge, United Kingdom: Cambridge University Press.
Youngs, R. R., Arabasz, W. J., Anderson, R. E., Ramelli, A. R., Ake, J. P., Slemmons, D. B., et al. (2003). A methodology for probabilistic fault displacement hazard analysis (PFDHA). Earthquake Spectra 19, 191–219. doi:10.1193/1.1542891
Žibret, G., Komac, M., and Jemec, M. (2012). PSInSAR displacements related to soil creep and rainfall intensities in the Alpineforeland of western Slovenia. Geomorphology 175–176, 107–114. doi:10.1016/j.geomorph.2012.07.002
Žibret, L., and Vrabec, M. (2016). Paleostress and kinematic evolution of the orogen-parallel NW-SE striking faults in the NW External Dinarides of Slovenia unraveled by mesoscale fault-slip data analysis. Geol. Croatica, 69 (3), 295–305. doi:10.4154/gc.2016.30
Živčić, M. (2009). Katalog potresov v Sloveniji. Internal documentation. Ljubljana, Slovenia: Ministry of the Environment and Spatial Planning, Slovenian Environment Agency, Seismology and Geology Office.
Keywords: Southern Alps, Eastern Alps, active faults, seismic hazard, geologic data input, Dinarides, Pannonian Basin
Citation: Atanackov J, Jamšek Rupnik P, Jež J, Celarc B, Novak M, Milanič B, Markelj A, Bavec M and Kastelic V (2021) Database of Active Faults in Slovenia: Compiling a New Active Fault Database at the Junction Between the Alps, the Dinarides and the Pannonian Basin Tectonic Domains. Front. Earth Sci. 9:604388. doi: 10.3389/feart.2021.604388
Received: 09 September 2020; Accepted: 19 February 2021;
Published: 10 May 2021.
Edited by:
Federica Ferrarini, G. d’Annunzio University of Chieti and Pescara, ItalyReviewed by:
Andrey Kozhurin, Institute of Volcanology and Seismology, Far Eastern Branch (RAS), RussiaAlessandro Maria Michetti, University of Insubria, Italy
Copyright © 2021 Atanackov, Jamšek Rupnik, Jež, Celarc, Novak, Milanič, Markelj, Bavec and Kastelic. This is an open-access article distributed under the terms of the Creative Commons Attribution License (CC BY). The use, distribution or reproduction in other forums is permitted, provided the original author(s) and the copyright owner(s) are credited and that the original publication in this journal is cited, in accordance with accepted academic practice. No use, distribution or reproduction is permitted which does not comply with these terms.
*Correspondence: Jure Atanackov, jure.atanackov@geo-zs.si