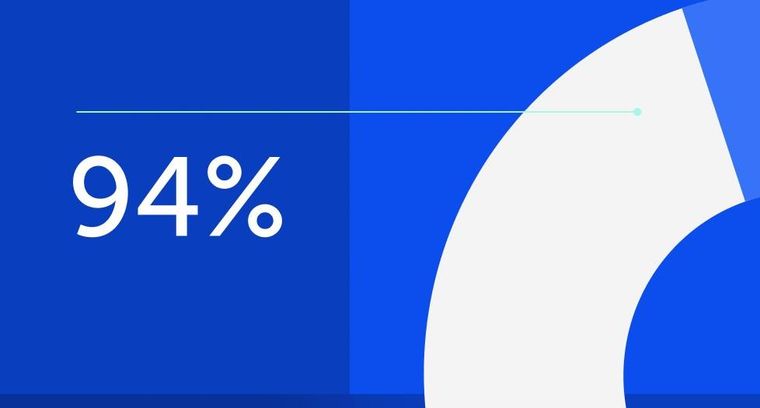
94% of researchers rate our articles as excellent or good
Learn more about the work of our research integrity team to safeguard the quality of each article we publish.
Find out more
ORIGINAL RESEARCH article
Front. Earth Sci., 28 May 2021
Sec. Solid Earth Geophysics
Volume 9 - 2021 | https://doi.org/10.3389/feart.2021.601530
This article is part of the Research Topic3D Printing in Geology and Geophysics: A New World of Opportunities in Research, Outreach, and EducationView all 7 articles
3D printing developed as a prototyping method in the early 1980s, yet it is considered as a 21st century technology for transforming digital models into tangible objects. 3D printing has recently become a critical tool in the geoscience research, education, and technical communication due to the expansion of the market for 3D printers and materials. 3D printing changes the perception of how we interact with our data and how we explain our science to non-experts, researchers, educators, and stakeholders. Hence, a one-day short course was designed and delivered to a group of professors, students, postdoctoral fellows, and technical staff to present the application of 3D printing in teaching and communication concepts in the geoscience. This case study was aimed at evaluating how a diverse group of participants with geoscience and engineering background and no prior experience with computer-aided modeling (CAD) or 3D printing could understand the principles of different 3D printing techniques and apply these methods in their respective disciplines. In addition, the course evaluation questionnaire allowed us to assess human perception of tangible and digital models and to demonstrate the effectiveness of 3D printing in data communication. The course involved five modules: 1) an introduction lecture on the 3D printing methods and materials; 2) an individual CAD modeling exercise; 3) a tour to 3D printing facilities with hands-on experience on model processing; 4) a tour to experimentation facilities where 3D-printed models were tested; and 5) group activities based on the examples of how to apply 3D printing in the current or future geoscience research and teaching. The participants had a unique opportunity to create a digital design at the beginning of the course using CAD software, analyze it and 3D print the final model at the end of the course. While this course helped the students understand how rendering algorithms could be used as a learning aid, educators gained experience in rapid preparation of visual aids for teaching, and researchers gained skills on the integration of the digital datasets with 3D-printed models to support societal and technical objectives.
3D printing is a 21st century technology for transforming digital models into physical objects. This technology is rapidly evolving, with more access to 3D printing machines and materials (Wohlers Report, 2019). This is an innovative tool in medical (Baden et al., 2015) and biomedical sciences (Hoy, 2013), engineering (Meyers et al., 2016; Boyajian et al., 2020), and communication (Baden et al., 2015; Malmström et al., 2020). 3D printing revolutionizes how we interact with our data and how we explain our science to non-experts (Horowitz and Schultz, 2014). Creating repeatable, tangible models is emerging in the geoscience education and research as well as in the related industries, such as petroleum recovery, groundwater storage, and carbon dioxide sequestration (Ishutov et al., 2018). One of the biggest advantages of 3D printing is that all the processes involved in the creation of a 3D object, from generating the design to obtaining the printed part, facilitate the learning of concepts and tools, which also develops creativity and communication skills. Earth science data are often modeled in 3D, and 3D printers can provide this 3D visualization and tangible aspect of digital data (Figure 1).
FIGURE 1. Major benefits of using 3D printing in geosciences. It is useful for developing creativity and design skills through 3D modeling. 3D printing is a convenient tool for rapid manufacture of learning and teaching aids. Any 2D or 3D model can be replicated for a better communication, especially among non-specialists. Any digital data can be reproduced with 3D printing, even if the physical sample does not exist anymore. Research ideas and concepts can be repeatedly tested on the 3D-printed samples. All data can be retrieved or repeated from the digital repositories, which include files of 3D-printed models.
3D printing or so-called additive manufacturing of an object involves deposition of a material layer by layer (Squelch, 2017). Therefore, this technology enables manufacturing models in various sizes and proportions (e.g., small objects can be printed large, so that more details are visible or large objects can be scaled down, so that one can hold the planet in the hand). Sustainable learning through a tangible approach is critical for understanding of complex geologic ideas, where learners can collect, gather and evaluate information about the exterior of the model and internal structures (Szulżyk-Cieplak et al., 2014). Moreover, the same model can be used to communicate these ideas to others, including non-experts in a technical subject (Dadi et al., 2014). 3D printing is essential for commination with impaired people, especially students who require special needs for education (Kostakis et al., 2015; Jo et al., 2016; Pantazis and Priavolou, 2017; Koehler et al., 2018). In the Earth science curriculum, those students can learn common topics such as volcanoes or plate tectonics by using 3D-printed models in the classroom or at home. Buehler et al. (2016) demonstrates an example of a short course for students with intellectual disabilities in an inclusive context that results in enhancing digital literacy skills and reducing stigmas about these individuals at a community level.
Application of 3D printing in high-school education has already shown enhanced haptic perception of the learning material. Elrod (2016) emphasized that if 3D printing would be used in the K-12 environment, students could be better prepared for careers in emerging fields of technology [e.g., science, technology, engineering, and mathematics (STEM disciplines)]. Schelly et al. (2015) demonstrated that even a 3-day short course for middle- and high-school teachers from a variety of disciplines (sciences, engineering, and arts) gained a high interest in utilizing this technology in their classrooms. Chiu et al. (2015) presented a successful model for learning, self-learning, and mastery learning approaches for freshman students with different levels of technological literacy using 3D printers. Reggia et al. (2015) suggested that providing engineering students with an opportunity to perform a project-based design course using 3D printing was an essential curricular element in many engineering programs. Chien and Chu (2018) proposed that 3D printing could enable high-school students to improve their ability to transform from STEM to STEAM (science, technology, engineering, arts, and mathematics) using 3D printers and to create a bridging curriculum with respect to high-school and college students.
Roy and Brine (2017) developed a coursework model to build intellectual capital for the next generation who would vastly depend on 3D printing, because they would shape a smart community in both developing and developed economy context. Martin et al. (2014) explained an idea of “think globally, produce locally,” where 3D printing would become more affordable with the versatility of machines and the ability to engage students with many different STEM-based activities. Gatto et al. (2015) showed that engineering education is on the course of adapting to the social and industrial revolution brought by additive manufacturing, because the latter allowed for sharing digital data in repositories and repeatedly reproducing the data to test ideas and concepts (Figure 1).
For the geoscience education, not many examples are found in the literature for using 3D printing in any full-time curriculum or short courses. Ford and Minshall (2019) demonstrate how teaching models of terrains, fossils, and mineral crystals can complement digital models for a better perception of 3D features. 3D printing is currently used in four geoscience areas, primarily for research and communication: paleontology, geomorphology, porous rocks, geomechanics (Figure 2). These 3D-printed models help organizing a full description, classification, and preservation of geologic specimens. Resolution of 3D printers determines the accuracy of internal and external features of 3D-printed models and hence affects the repeatability of the digital design in different materials (Figure 2). These characteristics are critical not only for creating teaching aids in the Earth Science curriculum, but also for conducting experimental research with 3D-pritned specimens (Ishutov et al., 2018). 3D printing also has value for communication of geoscience to non-specialist audiences to convey technical information, to support legal arguments, and to provide general knowledge of the nature. Currently, there is no universal short course that can provide fast, but positive learning experience of digital modeling and 3D printing to understand and explain geologic concepts among both experts and generalists.
FIGURE 2. Applications of 3D printing in the geoscience research areas: (A) paleontology, (B) geomorphology, (C) porous rocks, and (D) geomechanics. A blue chart indicates the characteristics of 3D-printed models that are critical for each of the geoscience areas. Materials used in a specific application have different physical and chemical properties, which affect the resolution of a 3D-printed model. 3D printer’s hardware and post-processing of 3D-printed models determine the accuracy of external and internal features. A combination of the three previous characteristics affects the repeatability of a digital design 3D-printed in multiple copies.
This course was developed to test how a group of participants from STEM disciplines, but with various academic backgrounds could perceive the fundamentals of available 3D printing techniques and materials and their relative merits. With little or no prior knowledge of CAD modeling and 3D printing, participants learnt about applications of 3D printing in studies of reservoir rocks (Squelch 2017), fossils (Rahman et al., 2012), geomechanics (Hodder et al., 2018), geomorphology (Hasiuk and Harding, 2016), and porous media (Ishutov, 2019). This one-day short course was divided into five modules and involved students, postdoctoral fellows, technicians, and professors interested in current advances of 3D printing in research and teaching. In addition, participants explored the application of 3D printing in a technical communication. The objectives of the study included: 1) to evaluate if learners with versatile educational and cultural backgrounds could perceive the basic concepts of 3D printing techniques and material properties to provide an assessment of 3D-printed models for research in their respective discipline; 2) to test if fast learning of CAD modeling and 3D printing could help the participants utilize 3D-printed models to explain geologic concepts to generalist audiences; and 3) to prove that 3D-printed models were effective tools for the geoscience education.
The short course was designed for the participants without prior experience of CAD modeling or 3D printing. In addition, the course was open for students, professors, postdoctoral fellows, technicians, and research associates from the geoscience and engineering disciplines. The short course took place at the University of Alberta, Edmonton, Canada and involved 50 participants. The course learning outcomes were: 1) to understand capabilities and limitations of different 3D printing techniques; 2) to demonstrate how to digitally design 3D-printable models using CAD software, web platforms, and computed tomography data; 3) to provide the assessment of digital models and their relative replicas 3D-printed from real data; and 4) to characterize how 3D printing can increase the effectiveness of teaching and data communication.
The short course was organized in five modules: 1) an introduction lecture on the 3D printing methods and materials; 2) an individual CAD modeling exercise; 3) a tour to 3D printing facilities with hands-on experience on model processing; 4) a tour to experimentation facilities where 3D-printed models are tested; and 5) group activities based on the examples of how to apply 3D printing in current or future geoscience research and teaching (Table 1). Each module was taught by one of the four instructors, and facility tours were led by four instructors, two instructors per facility. All instructions on how to complete each module were organized in a digital e-book (pdf).
Module 1 included a lecture on the history of “rapid prototyping” and how 3D printing evolved as a tool for engineering industries. In addition, the workflow of creating a digital model and transferring it into a tangible object was covered. The model preparation for 3D printing was explained with examples of using printing specifications, such as the thickness of each layer, the vertical and horizontal dimensions, and the print speed. The lecture also contained post-processing methods, such as ultraviolet (UV) light curing or removal of support material that held the internal porous structure and external elements during printing to avoid deformation or damage of intricate designs. Instructors discussed 3D printing methods that differed by power source, resolution, precision, accuracy, build volume, materials, and price. The importance and applications of 3D-printed models were covered briefly for the areas of geoscience and engineering. At the end of the lecture, participants had a discussion session with instructors (Figure 3A).
FIGURE 3. Photographs of the short course modules. (A) Module 1 “Overview of the 3D printing technology.” Course instructors presented a lecture on common additive manufacturing methods and materials and showed examples of 3D-printed models. (B) Module 2 “The art of making 3D-printable models.” Participants learned basic skills of CAD modeling using TinkerCAD. (C) Module 3 “Elko Garage Tour.” Live 3D printing process was shown to participants. (D) Module 4 “GeoPrint Tour.” Participants were shown industrial scale printing and experimental program performed with 3D-printed models. (E) Module 5 “Application of 3D printing in the geoscience.” Discussion of specific applications of geoscience models in edication and research.
Module 2 involved an individual CAD modeling exercise using an online platform on laptops or tablets (Figure 3B). The scale of 3D-printed models varied over the orders of magnitude: from nanometer-size features to the size of the 3D printer’s build volume. This activity was aimed at teaching the participants to create complex geological models (like rocks and fossils) using common shapes (e.g., cylinders, cubes) or multi-scale elements, which were then translated for 3D printing. At the end of this exercise, participants were able to export their model of choice for 3D printing and receive at the end of the course.
Module 3 represented a tour to the Elko Engineering Garage (University of Alberta, Edmonton, Canada) that introduced the participants to the activities associated with creating and 3D printing digital designs as well as post-processing of 3D-printed models (Figure 3C). Participants were exposed a variety of 3D printers and post-processing tools, as well as they had an opportunity to investigate a 3D laser scanner. Instructors made connections of the material covered in the lecture, such as material properties, 3D printing resolution, and model dimensions with the real applications in workspace. Participants were able to observe the 3D printing process of the digital models that they designed in module 2 and had a hands-on experience on post-processing their models to make give them a smooth, finished look.
Module 4 involved a visit to the GeoPRINT facility (University of Alberta, Edmonton, Canada), where an industrial-grade sand printer and a high-resolution stereolithography printer were located (Figure 3D). This tour introduced participants to two specific 3D printers used for geomechanical and flow research at Reservoir Geomechanics Research Group. Participants explored about the differences in material preparation, printing, and post-processing between these two technologies.
Module 5 included a group exercise on the comparison of CAD models for porous rocks, fossils and geomorphic features with their 3D-printed counterparts (Figure 3E). Participants assessed the differences in material finishes, accuracy of external and internal elements, and scales of 3D printing (using criteria in Figure 2). In addition, there was a discussion of potential application of 3D-printed models in the geoscience experiments to validate numerical simulations and complement existing laboratory tests. Instructors facilitated the discussion of 3D-printing techniques that participants have seen in modules 3 and 4 and how they could be applied to fundamental research in the areas of multi-phase fluid flow and reactive transport, discrete fracture networks, geomorphology, and paleontology (Figure 3E).
Out of seven ASTM categories of 3D printing, four methods were shown in this short course: stereolithography, binder jetting, material extrusion, and material jetting. All 3D printers belonging to these categories were demonstrated in Modules 3 and 4. Materials used for demonstration of 3D printing techniques included polymers, plastics, sand, and resins.
The software used in module 2 for CAD modeling exercises was Autodesk TinkerCAD (https://www.tinkercad.com). It is a free online platform that requires only registration with email. The software used for processing of digital designs before 3D printing was Autodesk Meshmixer (http://www.meshmixer.com). It is a freeware that can be installed on most operating systems.
The course survey is proved to be one of the effective forms of analysis of the short course efficiency (Chiu et al., 2015; Schelly et al., 2015; Meyers et al., 2016; Pantazis and Priavolou, 2017; Ford and Minshall, 2019; Assante et al., 2020). The surveys are usually conducted before and after the course to assess how learning objectives are fulfilled. In each module, the following criteria were used to build the course evaluation survey:
• fundamentals of 3D printing and its basic operating principles;
• advantages and disadvantages of 3D printing technologies;
• performance and functional constraints of 3D printing for specific applications.
• complete 3D-printing sequence of designing, fabricating, and measuring models;
• source of mismatch between digital and 3D-printed models.
• causes of errors and irregularities in 3D-printed models;
• hands-on experience of 3D printing in class for improved student understanding of subject matter.
• important 3D printing research challenges;
• resources to support experiments for teaching and classroom projects.
• understanding if humans learn better when using 3D-printed models;
• current and future 3D printing applications.
At the end of the course, instructors distributed an electronic evaluation form to all participants and asked them to complete it within 1 h. The questions in the survey were composed in a Google Docs form to allow for anonymous and individual response from each participant, who was required to indicate only their academic level. The post-course questionnaire was segmented into sections: 1) overall recommendation for the short course; 2) assessment of course materials (e-booklet, lecture slides, exercise instructions; 3) course content (cohesiveness of modules, ease of learning the material, laboratory tours, and visual aids); 4) time spent on each module; and 5) evaluation of instructors’ teaching abilities; 6) effectiveness of course learning outcomes. Section 1 responses were based on Yes/No scale. Responses in sections 2, 3, 5 were collected using the following scheme: strongly disagree, disagree, neutral, agree, and strongly agree. Responses in section 4 were registered using the following scheme: not enough, adequate, too much, no opinion. The last section was evaluated using Likert scale out of 5, where a higher value is a more positive response.
The short course involved 50 participants from geosciences and engineering (Figure 4A); it was expected to receive mixed comments about the course contents and organization of modules. Nonetheless, 97% of all participants responded that the course would be recommended to others (Figure 4B). In this case, others were referred to peer students, colleagues, and other academic staff. This outcome was positive to propose the course to various professional organizations as a customized workshop, e.g., for industry professionals interested in the use of 3D printing in research and technical communication. The instructors observed that despite the differences in age and academic background, the participants communicated with each other in a friendly manner. Based on the results of the post-course questionnaire, the short course outcomes were assessed for the adequacy and organization of the course materials, structure, and coherence of the course modules, and efficiency of the course instructors and learning objectives.
FIGURE 4. Demographics of the short course participants. (A) Indication of the academic level and/or position. (B) Responses of participants from (A) to the question: “Will you recommend this short course to others?”
An e-book contained a set of short, descriptive instructions with images and figures about each module (Figure 5) that was useful to most participants. Course objectives were clear, so that the short course agenda was understood by learners with different backgrounds (24 positive responses out of 32 responses in total). In addition, the survey showed that the e-book was a valuable component of the course as it helped navigating through activities and exercises (27 positive responses out of 33 responses in total). On the other hand, not all participants found the e-book visually appealing and suggested adding pseudo 3D cartoons that would visually simplify and outline different 3D printing processes (20 positive responses out of 33 responses in total; Figure 6). Other comments pointed out on the use of bolded text, underlining or different colors to highlight the key information in the e-book. Also, more than half of the class noted that activities were clearly defined by the instructors and suggested to include more details about the operation of software as numbered bullet points so there would be a step-by-step tutorial (21 positive responses out of 35 responses in total; Figure 6). A few additional notes were that the introductory lecture slides in module 1 were cohesive and well organized. For the next run of the course, instructors will prepare a short workflow with bullet points for each activity and exercise and will place them in the e-book as a support material. More images and snapshots will be added for each activity to allow the participants to navigate between the exercises.
FIGURE 5. An example of the module instructions from the course e-book. The full version of the e-book was available for participants a day before the course. Each module contained synopsis and a set of exercises.
FIGURE 6. Responses of participants for evaluation of the course materials, such as e-booklet and slides. All the course activities were described in the e-booklet provided on the short course day.
The course content was developed using several approaches: lecture slides, individual exercises, group exercises, and facility tours. The majority of the class responded that modules were cohesive (29 positive responses out of 33 responses in total; Figure 7). Participants were mostly engaged during the visits to the Elko Garage and GeoPrint facilities (modules 3 and 4), because these tours improved their understanding of the 3D printing process (30 positive responses out of 32 responses in total). Observing the printing methods and interaction with 3D-printed models provided a motivation for the learners to incorporate this technology in their research, teaching, or other activities (29 positive responses out of 34 responses in total; Figure 7). In addition, the majority of participants could understand all aspects of digital design, processing, and post-processing of 3D-printed models via the CAD modeling exercise (module 2) (31 positive responses out of 34 responses it total). Instructors observed that even those participants who did not have any experience with digital modeling of simple shapes could learn it fast, because at the end of the exercise everyone was on the same level.
FIGURE 7. Responses of participants for evaluation of the course content. Participants assessed each activity at the end of the short course. *A question about the advanced 3D printing course is whether participants would like to have a short course on the applications of 3D printing in their respective discipline (not geoscience).
The group exercise involving comparison of digital models with their 3D-printed counterparts and the discussion of applications in the geosciences (Module 5) was expected to be challenging, because the participants were divided into mixed groups of 10 people to avoid accumulating representatives of the same department and academic level in one group. E.g., one group might have consisted of two undergraduate students from civil engineering and geology, three professors from electrical engineering, computer engineering and geophysics, three postdoctoral fellows from mechanical engineering, and petroleum engineering, and two research associates from atmospheric science and computer science, respectively. Most of the class responded positively to such combination of groups, because it allowed them to share a broader spectrum of ideas given the versatility of backgrounds (32 positive responses out of 35 responses in total; Figure 7). Some participants responded that they would prefer to classify the groups by the department, so that they would share the same interest in 3D printing and might make the group work more cohesive. This model could be another option for the group activity, where the groups could be formed by the department only, but the course contents would need to be more general, rather than focusing on the geoscience and engineering applications.
Participants would also asked to have more group activities to share the knowledge learnt, which confirmed that this intentional split into mixed groups worked well for leaning the unknown concepts. A few people were not interested in the geoscience applications and would have liked to participate in the content related to their discipline only or in a more generic content. This was a viable comment, and more than half of the class responded that they would like to have an advanced 3D printing course to explore the applications in their relative subjects of interest (26 positive responses out of 30 responses in total; Figure 7). Perhaps a separate short course covering specific applications of 3D printing in STEM disciplines might be developed to satisfy this interest. The most expected comment was that participants were thinking of getting their own 3D printer to manufacture models for research, teaching, and communication.
Each module had a different time period for completion, because it depended on the speed of the instructor’s delivery and the pace of participants (Figure 8). It was designed to spend more time on individual and group exercises (Modules 2 and 5), so that the pace between the participants could be averaged as some people needed more time to learn new tools. In general, almost all learners (29 out of 33) agreed that the 50-min lecture in module 1 was sufficient to grasp the main concepts. Some participants (12 out of 33) noted that they would need more time to go through the functionalities of the software in Module 2 to complete the CAD exercises. In future, this module could be timed in a different way, where the participants would have an extensive, detailed introduction into the software and then they would be given a set of exercises to complete. Also, for those who could complete a mandatory set of exercises faster, additional activities would be provided. For the group exercises (module 5), about half of the class completed their assignments on time, while a quarter of the class felt that the time could be reduced (Figure 8). To adjust this module, more exercises would be provided, specifically a small section discussing case studies in the geoscience.
FIGURE 8. Responses of participants for evaluation of the time spent on each module of the short course.
The next set of questions in the survey was aimed at revealing any flaws in the style and structure of the instruction. It was found that the majority of the class was satisfied with the teaching style and delivery of the modules by instructors (28 positive responses out of 33 responses in total; Figure 9). One participant noted that it would be useful to have solutions for each exercise, mainly for the ones related to the group activity. The answers could not be compiled for each activity as they varied by the group and the amount of material covered in each case. A few participants would like to have more one-to-one communications with instructors, but it might not always possible, given the size of the class and time allocated for each activity. It is foreseen that the class size will be reduced to have more time assisting each participant in all activities, even though the majority of participants (31 out of 33; Figure 9) felt supported during the course.
FIGURE 9. Responses of participants for evaluation of the instructors’ delivery of the short course.
The survey showed that instructors were knowledgeable (32 positive responses out of 33 responses in total) and well-prepared (30 positive responses out of 34 responses in total) for the course, which fulfilled the course objective of sustainable learning and communication through tangible models. It is confirmed that 3D printing promoted the curiosity among the learners and facilitated an interest in creation of a model simultaneously with the instructor. Developing creative potential entailed improving a problem-based approach to demonstrate theoretical concepts that could be accessible by different groups of participants. This short course demonstrated that diverse groups were able to assimilate, apply, and describe new knowledge more effectively, including collaborative and individual learning. There is a need in studying how these methods can complement traditional instruction in terms of retention of material and motivating learners to study and develop their communication and problem-solving skills.
The course learning objectives were evaluated during interactive exercises of the course as well as post-course questionnaire. After completion of each module, participants were asked to complete the same set of three questions based on the course objectives. Their responses were averaged using Likert scale, where more positive responses were approaching 5 and less positive responses were approaching 1 (Table 2). Participants were scoring how each of the three objectives was fulfilled when they completed modules subsequently. It was evident that more confidence was gained toward the end of the short course when all three course objectives were assessed (increasing scores from Module 1 to Module 5 in Table 2). While not all participants had geoscience background, collaborative learning is proven to be effective in enhancing creativity and hence enabling a large class to adopt the new technology. Post-course questionaries demonstrated that faculty, students, research fellows, and technicians could effectively work in teams to understand basic concepts of 3D printing techniques and material properties. They used this information to provide an assessment of 3D-printed models and to generate ideas for research in their respective disciplines.
Individual CAD modeling exercise (module 2) helped the participants understand how geological and engineering models could be designed and utilized to explain ideas and concepts to generalist audiences. In module 5, instructors provided an example of 3D-printed porous rock created from a digital model (Figure 10). All participants were asked to use this workflow to characterize how the rock porosity could have been formed and to explain why the rock grains had angular or rounded geometry and how they were transported to form a larger formation. Participants with a geoscience background were assessing responses of participants that did not have any background in the geoscience. It was noted that comparison of images, 3D digital models, and 3D-printed samples altogether provided better understanding of the rock properties rather than each model separately. Also. participants with good technical background in CAD within the team could help teaching other teammates, providing additional peer learning element in the process.
FIGURE 10. Workflow for generation of 3D-printed samples from digital models. Source data are either optical or CT images of natural rocks (e.g., Berea sandstone). Images are segmented into pores and grains; the grain volume is transferred to 3D printing software as a CAD model. Selected 3D printer creates a tangible model layer-by-layer (polymer in this example). Pore space is filled with support material (soft polymer) that is removed by post-processing.
Module 5 was very useful for synthesizing previous modules and providing exercises linking CAD modeling from module 2 with 3D printing methods presented in module 1 and materials observed in modules 3 and 4. Participants were asked to choose one model for which both CAD and 3D-printed models were available (Figure 11). Their task was to prepare a 1-min presentation of the model intended for general audience. The exercise was aimed at evaluating if 3D-printed models could improve geoscience learning for non-specialists. This collaborative learning approach demonstrated that expertise from students with different backgrounds could contribute to the cognitive process. Instead of learning under the instructions of tutors, participants collaboratively worked and learnt together. Participants noted that those teammates without geoscience background provided more intuitive and comprehensive description of selected models. It might be due to the fact that specialists could not often formulate higher-level explanation of concepts and phenomena.
FIGURE 11. Examples of 3D-printed models used in course exercises. (A) Fossil and rock specimens. (B) Geomorphology and porous models.
Post-course questionnaire showed that 3D printing was an efficient tool in teaching and communication geological data and hypotheses to many types of diverse audiences. This study proved that non-specialists could learn, understand, and explain scientific concepts without prior knowledge about them. This finding is important because 3D printing can be used in many university curricula where students with any background can learn sciences in any environment. In particular, tangible aspect of 3D-printed models is vital for the geoscience education where most of the data are in a 3D format. Future development of the short course will involve several examples of non-geoscience data (e.g., engineering, medicine) to challenge participants in interpretation of concepts that are far beyond their expertise. This approach will help identifying if 3D-printed models are useful in communicating more complex phenomena to non-specialist audience.
3D printing is an emerging technology in the geoscience that provides additional teaching support, enhances technical communication using visual aids, and enables repeatable experimentation in research. While the process of incorporating this technology into the regular curriculum in academic institutions may take years, short courses can help this process by improving student and faculty engagement and by developing skills for a more qualitative knowledge acquisition. The short course presented in this study was useful for a diverse group of participants including professors, students, postdoctoral fellows, and technicians from the geoscience and engineering disciplines, because it allowed them to communicate geological concepts using digital models and their tangible counterparts. Participants demonstrated that this technology allowed them having the capacity for modification and sharing digital data and supporting educators who wanted to produce teaching models without prior expertise and in a rapid manner.
While this one-day short course had five modules, participants acknowledged that the time spent on each module was adequate as the modules contained the right amount of instructions and activities. It was designed in a way that participants would create their digital model, learn about different 3D printing techniques, observe how these techniques worked live and how 3D-printed models were experimented with in the laboratory, and finally 3D print their own model and discuss its properties. It was noted by the participants that course materials, such as e-booklet and slides with instructions, helped them digesting technical information in a cohesive way.
The main objectives of the short course was fulfilled, because the majority of participants responded that they would start using 3D printing for their research, teaching, or communication. Moreover, many participants had an interest in taking an advanced short course on the applications of this technology in their respective disciplines and to recommend this short course to others. Each module can certainly be modified and adjusted according to the background of the audience. This short course can be a primer for educators willing to introduce creative modeling in their teaching schedule and prepare students for problem-solving skills using tangible models. Making testable analogs of natural phenomena for the geoscience researchers is critical and can be achieved through acquiring CAD modeling skills in this course. Besides creating visual and teaching aids, this technology is a powerful tool in communication, as shown in the short course, because the participants with diverse academic backgrounds could discuss ideas and concepts without prior knowledge about them, only using 3D-printed models.
The raw data supporting the conclusions of this article will be made available by the authors, without undue reservation.
Written informed consent was obtained from the relevant individuals for the publication of any potentially identifiable images or data included in this article.
SI was the primary designer of the short course contents and the paper outline. He presented a poster at 2019 American Geophysical Union Conference on that study. SI developed exercises for the short course and prepared introduction and methods sections. KH developed presentation slides for the short course and wrote sections on results and discussion. RC was responsible for the introduction and conclusions. Figures were collected and analyzed by all authors. GZ-N was responsible for the lab tours.
The course was partially funded by MIP-CONACYT-280097 Grant, Mexico and NSERC 549236, Natural Sciences and Engineering Research Council of Canada. The funds covered the costs of 3D-printed models for participants of the short course.
The authors declare that the research was conducted in the absence of any commercial or financial relationships that could be construed as a potential conflict of interest.
We would like to thank the University of Alberta and Faculty of Engineering for the opportunity to host this short course on campus. Our special gratitude is to the Elko Engineering Garage for providing a demonstration tour and 3D printing the short course models. We are grateful to the Reservoir Geomechanics Research Group [RG]2 for support in preparation of this course. We also thank NSERC for support in continuous running of GeoPRINT GeoInnovation Environment at the Department of Civil and Environmental Engineering.
Assante, D., Cennamo, G. M., and Placidi, L. (2020). “3D Printing in Education: an European Prospective,” in Proceedings from the IEEE Global Engineering Education Conference (EDUCON). Porto: Portugal, 1133–1138.
Baden, T., Chagas, A. M., Gage, G., Marzullo, T., Prieto-Godino, L. L., and Euler, T. (2015). Open Labware: 3-D Printing Your Own Lab Equipment. Plos Biol. 13, e1002086. doi:10.1371/journal.pbio.1002086
Boyajian, M. K., Lubner, R. J., Roussel, L. O., Crozier, J. W., Ryder, B. A., and Woo, A. S. (2020). A 3D Printed Suturing Trainer for Medical Students. Clin. Teach. 17, 1–5. doi:10.1111/tct.13176
Buehler, E., Comrie, N., Hofmann, M., McDonald, S., and Hurst, A. (2016). Investigating the Implications of 3D Printing in Special Education. ACM Trans. Access. Comput. 8, 1–28. doi:10.1145/2870640
Chien, Y.-H., and Chu, P.-Y. (2018). The Different Learning Outcomes of High School and College Students on a 3D-Printing STEAM Engineering Design Curriculum. Int. J. Sci. Math. Educ. 16, 1047–1064. doi:10.1007/s10763-017-9832-4
Chiu, P. H. P., Chiu Lai, K. W., Fan, T. K. F., and Cheng, S. H. (2015). “A Pedagogical Model for Introducing 3D Printing Technology in a Freshman Level Course Based on a Classic Instructional Design Theory,” in Proceedings From the IEEE Frontiers In Education Conference (FIE). El Paso, TX, USA, 1–6. doi:10.1109/FIE.2015.7344287
Dadi, G. B., Goodrum, P. M., Taylor, T. R., and Maloney, W. F. (2014). Effectiveness of Communication of Spatial Engineering Information through 3D CAD and 3D Printed Models. Vis. Eng. 2, 114. doi:10.1186/s40327-014-0009-8
Elrod, R. E. (2016). Classroom Innovation through 3D Printing. Libr. Hi Tech. News, 33 (3), 5–7. doi:10.1108/LHTN-12-2015-0085
Ford, S., and Minshall, T. (2019). Invited Review Article: Where and How 3D Printing Is Used in Teaching and Education. Addit. Manuf. 25, 131–150. doi:10.1016/j.addma.2018.10.028
Gatto, A., Bassoli, E., Denti, L., Iuliano, L., and Minetola, P. (2015). Multi-disciplinary Approach in Engineering Education: Learning with Additive Manufacturing and Reverse Engineering. Rapid Prototyping J. 21, 598–603. doi:10.1108/RPJ-09-2014-0134
Hasiuk, F., and Harding, C. (2016). Touchable Topography: 3D Printing Elevation Data and Structural Models to Overcome the Issue of Scale. Geology. Today. 32, 16–20. doi:10.1111/gto.12125
Hodder, K. J., Nychka, J. A., and Chalaturnyk, R. J. (2018). Process Limitations of 3D Printing Model Rock. Prog. Additive Manufacturing 3, 172–182. doi:10.1007/s40964-018-0042-6
Horowitz, S. S., and Schultz, P. H. (2014). Printing Space: Using 3D Printing of Digital Terrain Models in Geosciences Education and Research. J. Geosci. Education. 62 (1), 138–145. doi:10.5408/13-031.1
Hoy, M. B. (2013). 3D Printing: Making Things at the Library. Med. Ref. Serv. Q. 32 (1), 93–99. doi:10.1080/02763869.2013.749139
Ishutov, S. (2019). Establishing Framework for 3D Printing Porous Rock Models in Curable Resins. Transp Porous Med. 129, 431–448. doi:10.1007/s11242-019-01297-9
Ishutov, S., Jobe, T. D., Zhang, S., Gonzalez, M., Agar, S. M., Hasiuk, F. J., et al. (2018). Three-dimensional Printing for Geoscience: Fundamental Research, Education, and Applications for the Petroleum Industry. Bulletin. 102, 1–26. doi:10.1306/0329171621117056
Jo, W., Hee, I., J., Harianto, R. A., So, J. H., Lee, H., Ju Lee, H., et al. (2016). Instead of Seeing and Hearing, Students Can Use Their Sense of Touch to Recognize the 3D Tactile Aids, Which Might Improve Their Learning and Memory Processes. J. Vis. Impairment Blindness. 110, 115–121. doi:10.1159/000390709
Koehler, K. E., Wild, T. A., and Tikkun, S. (2018). Implications of 3-D Printing for Teaching Geoscience Concepts to Students with Visual Impairments. J. Sci. Educ. Stud. Disabil. 2, 49–81. doi:10.14448/jsesd.10.0004
Kostakis, V., Niaros, V., and Giotitsas, C. (2015). Open Source 3D Printing as a Means of Learning: An Educational experiment in Two High Schools in Greece. Telematics Inform. 32, 118–128. doi:10.1016/j.tele.2014.05.001
Malmström, H., Enger, J., Karlsteen, M., and Weidow, J. (2020). Integrating CAD, 3D-Printing Technology and Oral Communication to Enhance Students' Physics Understanding and Disciplinary Literacy. Eur. J. Phys. 41, 065708. doi:10.1088/1361-6404/aba6bd
Martin, R. K., Bowden, N. S., and Merril, C. (2014). 3D Printing in Technology and Engineering Education. Technol. Eng. Teach. 73, 30–35. doi:10.1163/9789004415133_006
Meyers, K. L., Morgan, A. S., and Conner, B. P. (2016). 3D Printing to Introduce Design in a Cornerstone Project. Glob. J. Eng. Educ. 18, 22–29. doi:10.1007/978-1-4842-0946-2_2
Pantazis, A., and Priavolou, C. (2017). 3D Printing as a Means of Learning and Communication: The 3 Ducation Project Revisited. Telematics Inform. 34, 1465–1476. doi:10.1016/j.tele.2017.06.010
Rahman, I. A., Adcock, K., and Garwood, R. J. (2012). Virtual Fossils: a New Resource for Science Communication in Paleontology. Evo Edu Outreach 5, 635–641. doi:10.1007/s12052-012-0458-2
Reggia, E., Calabro, K., and Albrecht, J. (2015). “A Scalable Instructional Method to Introduce First-Year Engineering Students to Design and Manufacturing Processes by Coupling 3D Printing with CAD Assignments,” in Proceedings of the ASEE Annual Conference & Exposition. Seattle, WA. doi:10.18260/p.23447
Roy, D., and Brine, J. (2017). “3D Printing for Multidisciplinary Education: a Technology with Diverse Potential,” in Proceedings Of the INTED2017 Conference. Valencia, Spain. doi:10.21125/inted.2017.0039
Schelly, C., Anzalone, G., Wijnen, B., and Pearce, J. M. (2015). Open-source 3-D Printing Technologies for Education: Bringing Additive Manufacturing to the Classroom. J. Vis. Languages Comput. 28, 226–237. doi:10.1016/j.jvlc.2015.01.004
Squelch, A. (2017). 3D Printing Rocks for Geo-Educational, Technical, and Hobbyist Pursuits. Geosphere 14, 360–366. doi:10.1130/GES01364.1
Szulżyk-Cieplak, J., Duda, A., and Sidor, B. (2014). 3D Printers—New Possibilities in Education. Adv. Sci. Techn. Res. J. 8, 96–101. doi:10.12913/22998624/57510.12913/22998624/568
Keywords: 3D printing, learning aid, visualization, reservoir, porous rock, geomodeling, fossils, geomorphology
Citation: Ishutov S, Hodder K, Chalaturnyk R and Zambrano-Narvaez G (2021) A 3D printing Short Course: A Case Study for Applications in the Geoscience Teaching and Communication for Specialists and Non-experts. Front. Earth Sci. 9:601530. doi: 10.3389/feart.2021.601530
Received: 01 September 2020; Accepted: 13 May 2021;
Published: 28 May 2021.
Edited by:
Paula Koelemeijer, Royal Holloway, University of London, United KingdomReviewed by:
Raluca Ilie, University of Illinois at Urbana-Champaign, United StatesCopyright © 2021 Ishutov, Hodder, Chalaturnyk and Zambrano-Narvaez. This is an open-access article distributed under the terms of the Creative Commons Attribution License (CC BY). The use, distribution or reproduction in other forums is permitted, provided the original author(s) and the copyright owner(s) are credited and that the original publication in this journal is cited, in accordance with accepted academic practice. No use, distribution or reproduction is permitted which does not comply with these terms.
*Correspondence: Sergey Ishutov, aXNodXRvdkB1YWxiZXJ0YS5jYQ==
Disclaimer: All claims expressed in this article are solely those of the authors and do not necessarily represent those of their affiliated organizations, or those of the publisher, the editors and the reviewers. Any product that may be evaluated in this article or claim that may be made by its manufacturer is not guaranteed or endorsed by the publisher.
Research integrity at Frontiers
Learn more about the work of our research integrity team to safeguard the quality of each article we publish.