Leaf Waxes and Hemicelluloses in Topsoils Reflect the δ2H and δ18O Isotopic Composition of Precipitation in Mongolia
- 1Institute of Geography, Friedrich Schiller University Jena, Jena, Germany
- 2Heisenberg Chair of Physical Geography with Focus on Paleoenvironmental Research, Institute of Geography, Technical University of Dresden, Dresden, Germany
- 3Institute of Agronomy and Nutritional Sciences, Soil Biogeochemistry, Martin Luther University Halle-Wittenberg, Halle (Saale), Germany
- 4Institute of Plant and Agricultural Sciences, Mongolian University of Life Sciences, Ulaanbaatar, Mongolia
- 5Institute of General and Experimental Biology, Russian Academy of Science (RAS), Ulan-Ude, Russia
- 6Institute of Soil Science and Soil Geography, University of Bayreuth, Bayreuth, Germany
A Corrigendum on
Leaf Waxes and Hemicelluloses in Topsoils Reflect the δ2H and δ18O Isotopic Composition of Precipitation in Mongolia
by Struck, J., Bliedtner, M., Strobel, P., Bittner, L., Bazarradnaa, E., Andreeva, D., Zech, W., Glaser, B., Zech, M., and Zech, R. (2020). Front. Earth Sci. 8:343. doi: 10.3389/feart.2020.00343
In the original article, the presented δ18Oara values were accidentally not corrected for the oxygen introduced during hydrolysis. The necessary correction results in slightly more positive δ18Oara values.
The authors apologize for this mistake, and state that this does not change the scientific conclusions of the article in any way, particularly the fact that the calculated apparent fractionation (Ɛ18O ara/p) is constant along the Mongolian transect. However, as the apparent fractionation is 44 ± 2‰ (not 41 ± 2‰) and this would affect future data compilations and comparisons, we have corrected all δ18Oara and Ɛ18O ara/p values in the text, the figures, and the supplementary material. We delete our hypothesis stated in the Supplementary Material that a decreasing partial CO2 pressure might cause enhanced 18Oara enrichment, because the correlation between Ɛ18O ara/p and altitude is not significant anymore (R2 = 0.09, p = 0.14). All changes are highlighted in bold.
A correction has been made to the Abstract. The corrected version is as follows:
“The apparent fractionation Ɛapp, i.e., the isotopic difference between precipitation and the investigated compounds, shows no strong correlation with climate along the transect (Ɛ2H n-C29/p = −129 ± 14‰, Ɛ2H n-C31/p = −146 ± 14‰, and Ɛ18O ara/p = +44 ± 2‰). Our results suggest that δ2Hn-alkane and δ18Oara in topsoils from Mongolia reflect the isotopic composition of precipitation and are not strongly modulated by climate. Correlation with the isotopic composition of precipitation has root-mean-square errors of 13.4‰ for δ2Hn-C29, 12.6 for δ2Hn-C31, and 2.2‰ for δ18Oara, so our findings corroborate the great potential of compound-specific δ2Hn-alkane and δ18Oara analyzes for paleohydrological research in Mongolia”.
A correction has been made to the Results. The corrected version is as follows:
“The δ18Oara values range from +31‰ to +41‰ with an average of +35 ± 3‰ (Figure 2B). All compounds show the same trend as the isotopic composition of precipitation (Figures 2E,F), and are significantly more positive in the arid part of the transect (ID: 34–42) compared to the rest (δ2Hn-C29: p = 0.017, δ2Hn-C31: p = 7.08e−4, δ18Oara: p = 2.95e−5).
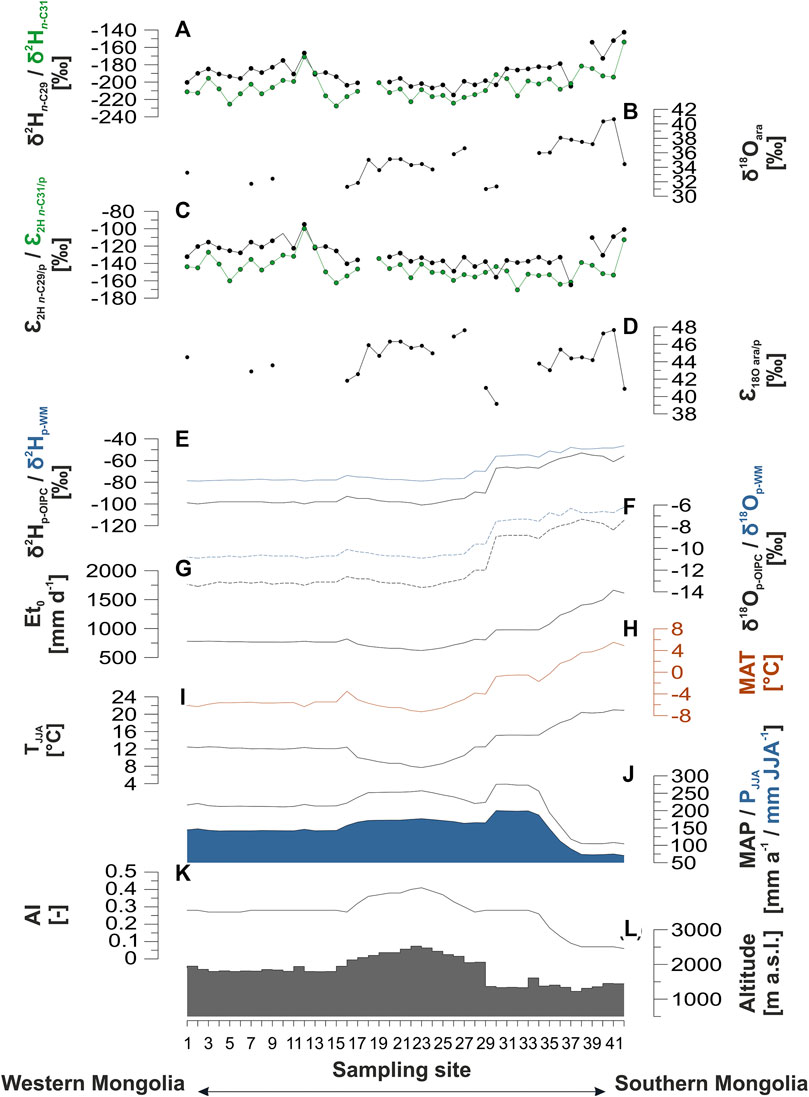
FIGURE 2. δ2H and δ18O signatures of leaf wax-derived n-alkanes and the hemicellulose sugar arabinose as well as their apparent isotope fractionation (Ɛapp), compared to environmental and climatic parameters along the transect (A) Compound-specific δ2H of the leaf wax-derived n-alkane n-C29 (black) and n-C31 (green) (this study) (B) compound-specific δ18Oara (this study) (C) Ɛ2H n-C29/p (black) and Ɛ2H n-C31/p (green) (this study) (D) Ɛ18O ara/p (this study) (E, F) OIPC isotopic composition of precipitation (black line) and amount-weighted isotopic signature of precipitation (blue line): δ2Hp(E), and δ18Op(F), respectively (Bowen et al., 2005; Bowen, 2019; Fick and Hijmans, 2017; IAEA/WMO, 2015) (G) the potential evapotranspiration (Et0) (Trabucco and Zomer, 2019) (H) mean annual temperature (MAP) (Fick and Hijmans, 2017) (I) averaged summer temperature of June, July, and August (TJJA) (J) black line shows the mean annual precipitation (MAP), blue shaded area the summer precipitation amount (PJJA) (Fick and Hijmans, 2017) (K) the aridity index (AI) (Trabucco and Zomer, 2019), and (L) the altitude (Jarvis et al., 2008).
Ɛ18O ara/p ranges from +39‰ to +48‰ with an average of +44 ± 2‰ (Figure 2D). Ɛapp is not statistically different in the arid part of the transect (Ɛ2H n-C29/p: p = 0.791, Ɛ2H n-C31/p: p = 0.554, Ɛ18O ara/p: p = 0.824)”.
A correction has been made to Discussion, δ2Hn-alkaneand δ18OaraAgainst the Isotopic Composition of Precipitation. The corrected version is as follows:
“The δ2Hn-alkane and δ18Oara values correlate significantly with the δ2Hp-WM and δ18Op-WM values (Figure 3, R2 = 0.30, p = 3.22e−4 for δ2Hn-C29; 0.11 and 0.03 for δ2Hn-C31; and 0.36 and 1.60e−3 for δ18Oara).
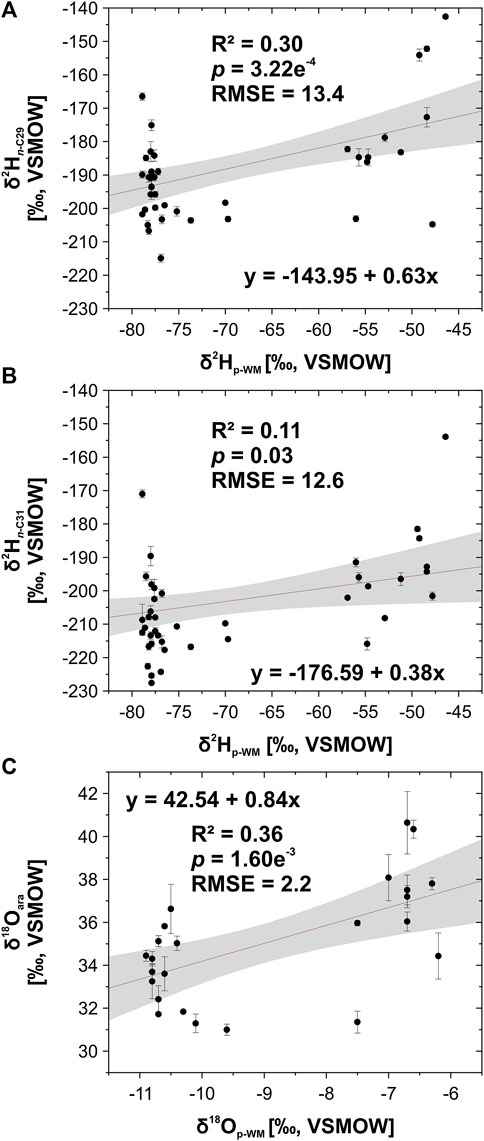
FIGURE 3. δ2H signatures of the leaf wax-derived n-alkanes (A)n-C29 and (B)n-C31, as well as (C) δ18Oara signatures from the topsoil transect through Mongolia plotted against the amount-weighted δ2H and δ18O signature of precipitation (δ2Hp-WM/δ18Op-WM). Red trend lines illustrate linear regressions, gray shaded areas the 95% confidence interval. Bold R2/p-values indicate the level of significance (α = 0.05).
The RMSE is 13.4‰ for δ2Hn-C29, 12.6‰ for δ2Hn-C31 and 2.2‰ for δ18Oara (Figure 3) and thus indicates that the biomarkers accurately record the isotopic composition of precipitation along our transect”.
A correction has been made to Discussion, Apparent Fractionation Against Climate. The corrected version is as follows:
“Thus, this correlation should not be over interpreted. We conclude that Ɛapp is nearly constant with Ɛ2H n-C29/p = −129 ± 14‰, Ɛ2H n-C31/p = −146 ± 14‰, and Ɛ18O ara/p = +44 ± 2‰.
Assuming a constant Ɛbio factor of +27‰ for arabinose (Lehmann et al., 2017; Hepp et al., 2020) evapotranspirative enrichment would be ∼17‰ for δ18Oara”.
A correction has been made to Discussion, Comparison With Other Studies. The corrected version is as follows:
“The Ɛ18O ara/p values for Mongolia (44 ± 2‰) are similar to values reported by Strobel et al. (2020) for relatively arid regions in South Africa. There, the more humid regions have a significantly lower Ɛ18O ara/p (∼37‰), quite similar to the C3 grass sites in Europe (Hepp et al., 2020). The deciduous tree sites in Europe, however, are again characterized by more enriched δ18Osugar values (Ɛ18O sugar/p = ∼43‰). All this indicates that δ18O is more sensitive to evapotranspirative enrichment than δ2H, so that climate can more strongly modulate δ18Osugar, and again that grasses show the signal dampening much more pronounced than dicotyledons”.
A correction has been made to the Conclusion. The corrected version is as follows:
“Leaf wax-derived n-alkanes and the hemicellulose-derived sugar arabinose are significantly more enriched in 2H and 18O in the more arid southern and eastern parts of the transect. This reflects the changes in the isotopic composition of precipitation along the transect, and the correlations with δ2Hp-WM and δ18Op-WM have RMSE of 13.4‰ for δ2Hn-alkane and 2.2‰ for δ18Oara.
The apparent fractionation remains mostly constant at −129 ± 14‰, −146 ± 14‰, and at +44 ± 2‰ for Ɛ2H n-C29/p, Ɛ2H n-C31/p and Ɛ18O ara/p, respectively. There are no significant differences along the transect, nor strong correlations with climate”.
A correction has been made to the Supplementary Material, Apparent Fractionation Against Climate. The corrected version is as follows:
“In addition to climate, we correlated the Ɛapp values against altitude, to test for altitude-controlled evapotranspirative enrichment. In contrast to a previous study by Polissar and Freeman (2010), no impact could be observed for δ2Hn-alkaneand δ18Oara, with Ɛ2H n-C29/p, Ɛ2H n-C31/pand Ɛ18Oara/p being constant along our investigated transect (Supplementary Figure 1)”.
The corrected caption for Supplementary Figure 1 is as follows:
“The corrected δ18Oara values affect the presented data in Figures 2–4, and the Supplementary Figure 1”.
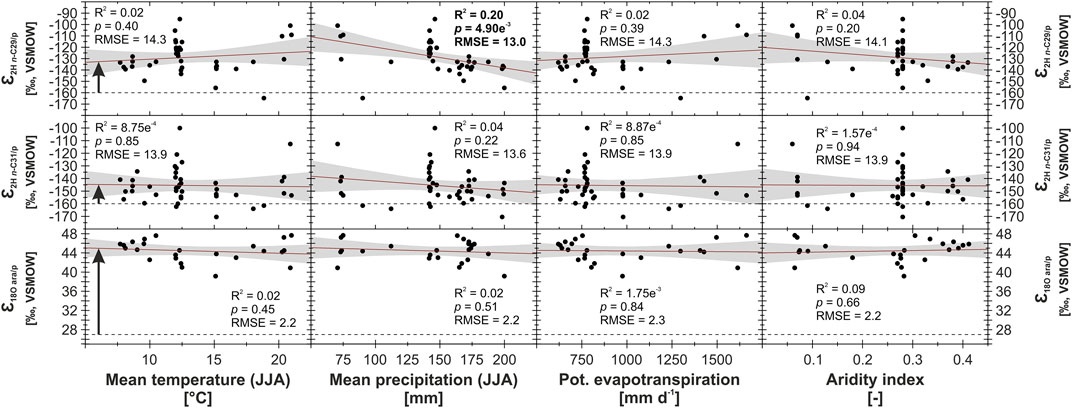
FIGURE 4. Apparent fractionation Ɛ2H n-C29/p, Ɛ2H n-C31/p and Ɛ18O ara/p from the topsoil transect through Mongolia plotted against TJJA, PJJA, Et0, AI (Fick and Hijmans, 2017; Trabucco and Zomer, 2019). Red trend lines illustrate linear regressions, gray shaded areas the 95% confidence interval. Bold R2/p-values indicate the level of significance (α = 0.05). Black dashed lines illustrate values for the biosynthetic fractionation, and black arrows indicate the effect of evapotranspirative enrichment (biosynthetic fractionation factors of −160‰ and +27‰ are assumed for the n-alkanes and arabinose, respectively).
The authors apologize for these errors and state that this does not change the scientific conclusions of the article in any way. The original article has been updated.
Author Contributions
JS, MB, PS, MZ, and RZ designed the study. MB and RZ collected the samples along transect I in 2016. JS and RZ collected the samples along transect II in 2017. JS carried out the major part of the laboratory analyzes in the laboratory of RZ and BG, assisted by LB, PS, and MB. EB, DA, and WZ organized the sample logistics in 2016 and 2017. JS wrote the manuscript with contributions of all coauthors. All authors contributed to the article and approved the submitted version.
Conflict of Interest
The authors declare that the research was conducted in the absence of any commercial or financial relationships that could be construed as a potential conflict of interest.
Supplementary Material
The Supplementary Material for this article can be found online at: https://www.frontiersin.org/articles/10.3389/feart.2020.619100/full#supplementary-material.
References
Bowen, G. J. (2019). Version 3.1. The online isotopes in precipitation calculator. Available at: http://www.waterisotopes.org (Accessed January 31, 2020).
Bowen, G. J., Wassenaar, L. I., and Hobson, K. A. (2005). Global application of stable hydrogen and oxygen isotopes to wildlife forensics. Oecologia 143, 337–348. doi:10.1007/s00442-004-1813-y
Fick, S. E., and Hijmans, R. J. (2017). WorldClim 2: new 1-km spatial resolution climate surfaces for global land areas. Int. J. Climatol. 37, 4302–4315. doi:10.1002/joc.5086
Hepp, J., Schäfer, I. K., Lanny, V., Franke, J., Bliedtner, M., Rozanski, K., et al. (2020). Evaluation of bacterial glycerol dialkyl glycerol tetraether and 2H–18O biomarker proxies along a central European topsoil transect. Biogeosciences 17, 741–756. doi:10.5194/bg-17-741-2020
Jarvis, A., Reuter, H. I., Nelson, A., and Guevara, E. (2008). Hole-filled seamless SRTM data V4, international centre for tropical agriculture (CIAT). Available at: http://srtm.csi.cgiar.org (Accessed January 31, 2020).
Lehmann, M. M., Gamarra, B., Kahmen, A., Siegwolf, R. T. W., and Saurer, M. (2017). Oxygen isotope fractionations across individual leaf carbohydrates in grass and tree species. Plant Cell Environ. 40, 1658–1670. doi:10.1111/pce.12974
Polissar, P. J., and Freeman, K. H. (2010). Effects of aridity and vegetation on plant-wax δD in modern lake sediments. Geochem. Cosmochim. Acta 74, 5785–5797. doi:10.1016/j.gca.2010.06.018
Strobel, P., Haberzettl, T., Bliedtner, M., Struck, J., Glaser, B., Zech, M., et al. (2020). The potential of δ2Hn-alkanes and δ18Osugar for paleoclimate reconstruction—a regional calibration study for South Africa. Sci. Total Environ. 716, 137045. doi:10.1016/j.scitotenv.2020.137045
Trabucco, A., and Zomer, R. (2019). Global aridity index and potential evapotranspiration (ET0) climate database v2. FigshareAvailable at: https://doi.org/10.6084/m9.figshare.7504448.v3 (Accessed January 24, 2019).
Keywords: biomarkers, n‐alkanes, sugars, compound-specific isotopes, apparent fractionation
Citation: Struck J, Bliedtner M, Strobel P, Bittner L, Bazarradnaa E, Andreeva D, Zech W, Glaser B, Zech M and Zech R (2021) Corrigendum: Leaf Waxes and Hemicelluloses in Topsoils Reflect the δ2H and δ18O Isotopic Composition of Precipitation in Mongolia. Front. Earth Sci. 8:619100. doi: 10.3389/feart.2020.619100
Received: 19 October 2020; Accepted: 27 October 2020;
Published: 11 January 2021.
Edited and reviewed by:
Moritz Felix Lehmann, University of Basel, SwitzerlandCopyright © 2021 Struck, Bliedtner, Strobel, Bittner, Bazarradnaa, Andreeva, Zech, Glaser, Zech and Zech. This is an open-access article distributed under the terms of the Creative Commons Attribution License (CC BY). The use, distribution or reproduction in other forums is permitted, provided the original author(s) and the copyright owner(s) are credited and that the original publication in this journal is cited, in accordance with accepted academic practice. No use, distribution or reproduction is permitted which does not comply with these terms.
*Correspondence: Julian Struck, julian.struck@uni-jena.de