- 1State Key Laboratory of Continental Dynamics, Department of Geology, Northwest University, Xi’an, China
- 2Organic Geochemistry Unit, School of Earth Sciences, School of Chemistry, Cabot Institute for the Environment, University of Bristol, Bristol, United Kingdom
- 3School of Environmental and Chemical Engineering, Xi’an Polytechnic University, Xi’an, China
- 4State Key Laboratory of Loess and Quaternary Geology, Institute of Earth Environment, Chinese Academy of Sciences, Xi’an, China
Unusual “H-Shaped” branched and isoprenoidal glycerol monoalkyl glycerol tetraethers (GMGTs, or H-GDGTs) have been found in peat, lake, and ocean sediments. A survey of recent samples from a modern global peat database suggested that there is a relationship between the abundance of H-GDGTs relative to regular GDGTs and temperature. However, this relationship has not been widely explored, including in a historical, stratigraphic or geological context. Here, we report the abundance and distribution of H-iso- and H-brGDGTs in two (Hani and Gushantun) peat cores from northeastern China through the late Quaternary (last 15 kyr) to examine whether the relationship between temperature and the relative abundance of H-GDGTs is preserved downcore. The results indicate that high relative abundances of H-brGDGTs are associated with high (or increasing) reconstructed mean annual air temperature (MAATpeat), albeit with considerable divergence in some intervals. On the other hand, high relative abundances of H-isoGDGTs are generally associated with low (or decreasing) MAATpeat. These findings are partly inconsistent with the observations from the modern database of globally distributed peats, which showed that the abundance of both (br- and iso-) H-GDGTs is positively correlated with temperature. The deviation in the relationship between H-isoGDGTs and temperature suggests that additional factors, for example pH and shifts in archaeal community related to hydrology, exert an influence on the abundance of H-isoGDGTs on the long-term.
Introduction
Branched and isoprenoid glycerol dialkyl glycerol tetraethers (brGDGTs and isoGDGTs) are commonly found in diverse environments, such as the water column, pests and soils as well as (mesophilic) marine and lacustrine sediments (see review by Schouten et al., 2013). IsoGDGTs are produced by a wide range of archaea and can have between 0 and 8 cyclopentane moieties (see Schouten et al. (2013) for structures), with more rings at higher growth temperature (De Rosa et al., 1980). BrGDGTs were first discovered in peat (Sinninghe Damsté et al., 2000) and subsequently found to be ubiquitous in mineral soils, peat, marine sediments, and lake sediments (e.g., Schouten et al., 2000; Hopmans et al., 2004; Weijers et al., 2006; Weijers et al., 2007; Sinninghe Damsté et al., 2008; Huguet et al., 2010; Loomis et al., 2011; Naafs et al., 2017; Zheng et al., 2017). Although the exact biological source(s) of brGDGTs are still ambiguous, they are believed to be produced by bacteria (Weijers et al., 2006; Sinninghe Damsté et al., 2014; Chen et al., 2018; Sinninghe Damsté et al. 2018). Both the isoGDGTs and brGDGTs are widely used as paleoclimatic proxies to reconstruct temperature and pH in natural archives, such as marine sediments, peat, and mineral soils (Schouten et al., 2002; Weijers et al., 2007; Naafs et al., 2017; Wang et al., 2020). In addition to these relatively well-studied isoGDGTs and brGDGTs, a series of unusual “H-shaped” glycerol dialkyl glycerol tetraethers (H-GDGTs) have been detected in cultures and natural samples (Morii et al., 1998; Schouten et al., 2000; Schouten et al., 2008a; Sugai et al., 2004; Liu et al., 2012a; Liu et al., 2012b; Liu et al., 2012c; Zhu et al., 2014; Bauersachs et al., 2015; Becker et al., 2016; Naafs et al., 2018). Isoprenoidal H-GDGTs (H-isoGDGTs) with 0–4 cyclopentane moieties were initially found in the hyperthermophilic euryarchaeon Methanothermus fervidus (Morii et al., 1998). However, these compounds have also been found in mesophilic marine and lacustrine sediments (Schouten et al., 2008a; Liu et al., 2012a) as well as peat (Naafs et al., 2018). H-shaped branched GDGTs (H-brGDGTs) have only rarely been reported in environmental settings. H-1020 was initially identified in marine sediments (Liu et al., 2012a). More recently, Naafs et al. (2018) reported H-1020, and two new H-brGDGTs (H-1034 and H-1048), in peat, the first known occurrence of H-brGDGTs in the terrestrial realm. Based on a global database of modern peat samples, these authors demonstrated that the relative abundance of H-brGDGTs, and H-isoGDGTs over regular br- and isoGDGTs is correlated with mean annual air temperature, with highest abundance in tropical peats (Naafs et al., 2018). This suggests that the formation of the extra covalent bond in H-GDGTs plays a role in maintaining membrane stability and helps microbes adapt to the higher temperature (Schouten et al., 2008b; Knappy et al., 2011; Naafs et al., 2018).
This work offered a novel insight into the temperature adaption of GDGT producing organisms, however, also raised questions about the nature of these relationships, their fidelity, and their utility in palaeoclimate investigations. One way to probe these questions is via downcore variations in their abundance, comparing them to changes in environmental conditions (i.e., across redox boundaries), climate variations and other climate proxies. Here, we report the downcore abundance of H-GDGTs from two peat cores in Northeastern China. In order to better understand temperature controls on H-GDGTs and to compare the results with the global peat dataset from Naafs et al. (2018), we focus on peat intervals and exclude the lacustrine layers at the bottom. These cores span the Holocene and part of the deglaciation, a period of relatively large variations in temperature (∼1–12°C) (Zheng et al., 2017; Zheng et al., 2018). Thus, they offer the opportunity to investigate the relationships between environmental parameters (mainly be temperature) and the abundance and distribution of H-GDGTs in a relatively cold geological setting.
Materials and Methods
The two peat cores were collected from Hani and Gushantun peatland in Northeastern China (Figure 1). The Gushantun peat deposit (42°18′N, 126°17′E) is situated in Huinan County in Jilin Province at an elevation of 500 m on the western flank of the Changbai Mountains. The samples are from a 735 cm long core collected from near the center of the Gushantun peat. The core consists of 655 cm of brown to dark brown peat containing a large amount of non-degraded plant residue, spanning the last 12,000 years. Below 655 cm depth, the sediment is grayish-green to dark brown mud, representing lacustrine depositional conditions (See the Supplementary Material for more details).
The Hani peat deposit (42°13′N, 126°31′E) is situated in Liuhe County in Jilin Province at an elevation of 910 m on the western flank of the Changbai Mountains. The samples are from an 885 cm core collected from near the center of the Hani mire. The core consists of 574 cm of brown to dark brown peat, underlain by 11 cm of brown peat with sand and then 262 cm of dark brown peat, in total spanning the last 15,400 years. Below 847 cm depth, the sediment is grayish-green mud, representing the original lacustrine depositional conditions (See the Supplementary Material for more details).
The study sites, cores, chronostratigraphy of the peat sequences based on Accelerator Mass Spectrometry (AMS) 14C dates, and the procedures for lipid extraction and analysis were described in previous papers (See the Supplementary Material for the details; Zheng et al., 2017; Zheng et al., 2018). Briefly, the freeze-dried, homogenized samples were extracted ultrasonically with dichloromethane (DCM, 3×), DCM/methanol (1:1, v/v, 3×), and methanol (2×). The total lipid extract was then base hydrolyzed in 1 M KOH/methanol (5% H2O in volume) at 80°C for 2 h. The solution was extracted at least six times with n-hexane, and the combined extracts were dried under a stream of N2 gas. These neutral fraction extracts were then separated into a saturated hydrocarbon and a polar fraction on a short silica gel column using n-hexane and methanol as eluents, respectively. Half of the polar fraction was filtered through 0.45 μm PTFE syringe filters, dried under nitrogen gas, and used to analyze GDGTs.
The GDGTs were analyzed using an Agilent 1,200 series liquid chromatography and triple quadruple mass spectrometry (LC–MS2) system, equipped with an autosampler and ChemStation manager software. Samples were re-dissolved in 300 μL n-hexane/isopropanol (99:1, v/v) and injected. Separation of the GDGTs was achieved using two silica columns in tandem (150 mm × 2.1 mm, 1.9 μm, Thermo Finnigan, United States) maintained at 40°C, following Yang et al. (2015). The GDGTs were eluted isocratically for the first 5 min with 84% A and 16% B, where A = n-hexane and B = EtOAc. The following elution gradient was used: 84/16 A/B to 82/18 A/B from 5 to 65 min and then to 100% B in 21 min, followed by 100% B for 4 min to wash the column and then back to 84/16 A/B to equilibrate the column for 30 min. We used a constant flow rate of 0.2 ml/min. The GDGTs were ionized in an atmospheric pressure chemical ionization (APCI) chamber with single ion monitoring at m/z 1,050, 1,048, 1,046, 1,036, 1,034, 1,032, 1,022, 1,020, and 1,018 for the (H-) brGDGTs and m/z 1,302, 1,300, 1,298, 1,296, 1,294, and 1,292 for the (H-) isoGDGT. The GDGTs were quantified from integrated peak areas of the [M+H]+ ions. H-isoGDGTs and H-brGDGTs were identified based on published relative retention times and mass spectra (See Naafs et al. (2018) for the structures of H-GDGTs; Jia et al., 2014; Liu et al., 2012a; Schouten et al., 2008b).
In order to compare the results with those of previous studies, we calculated the relative abundance of H-GDGTs using the following equations used by Naafs et al. (2018):
Results and Discussion
“H-Shaped” Glycerol Dialkyl Glycerol Tetraethers Distribution and Abundance
H-GDGTs were detected in all samples in Hani and Gushantun, including those at shallow depth. H-isoGDGT-0 and -1 were present in almost all of the peat samples. H-isoGDGT-0 was the most abundant, comprising ∼24–100% (mostly > 70%) of total H-isoGDGTs in Hani and ∼84–100% in Gushantun. H-brGDGTs, including H-1020, H-1034, and H-1048, were also detected in all samples. The relative abundance of H-1048, H-1034 and H-1020 is ca. 23–53%, 19–55%, and 14–41% in Gushantun and ca. 18–57%, 21–46%, and 18–49% in Hani, respectively.
The abundance of total H-GDGTs (both br and iso) is positively correlated with the abundance of their regular counterparts (r = 0.76–0.91, p < 0.001; data not shown), which is similar to that seen in the global peat database (Naafs et al., 2018). These positive linear correlations suggest that both regular GDGTs and H-GDGTs are derived from similar microbial sources and/or that their controlling factors (e.g., preservation) in peat are similar. It is also possible that a precursor-product relationship exists as proposed by Knappy et al. (2011), who speculated that H-isoGDGTs are biosynthetically derived from regular isoGDGTs.
In Hani peat, the relative abundance of both H-isoGDGTs and H-brGDGTs over regular GDGTs (H-isoGDGT%-HN and H-brGDGT%-HN, Eqs 1 and 2) varies from ca. 1.8–6.9% and from ca. 1.1–6.8% (Figure 2), respectively. In Gushantun peat, the relative abundance of H-brGDGTs and H-isoGDGTs (H-brGDGT%-GST and H-isoGDGT%-GST) varies between 0.9 and 5.9% and 1.2 and 5.1%, respectively (Figure 3). These values are similar to those reported in marine/lacustrine sediments and global peat database (Schouten et al., 2008a; Naafs et al., 2018).
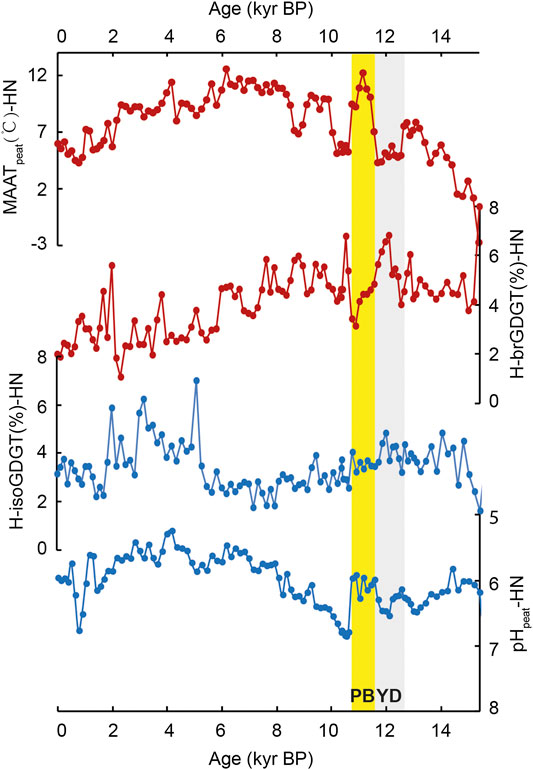
FIGURE 2. Comparison of the abundance of both H-isoGDGTs and H-brGDGTs (Eqs 1 and 2, respectively), mean annual air temperature (MAATpeat) and pHpeat in Hani peat. YD and PB are known as the Younger Dryas (YD) and the Preboreal, highlighted by the gray and yellow box, respectively (the depicted period is approximate).
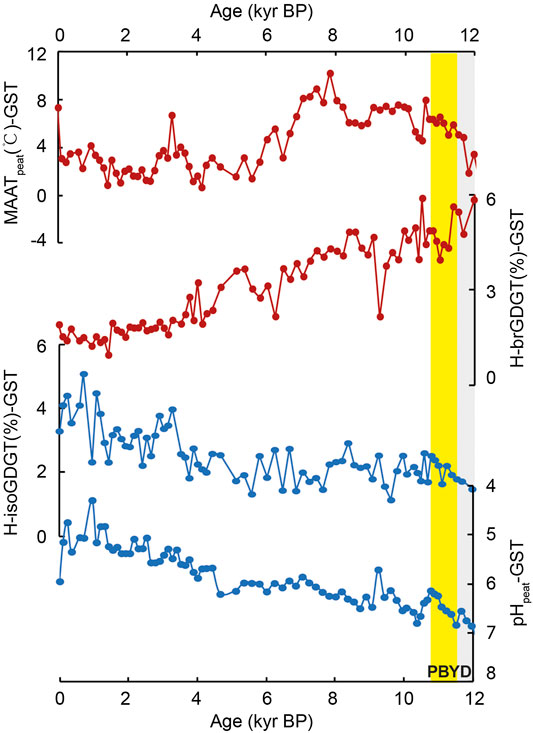
FIGURE 3. Comparison of the abundance of H-isoGDGTs and H-brGDGTs (Eqs 1 and 2, respectively), mean annual air temperature (MAATpeat) and pHpeat in Gushantun peat. YD and PB are known as the Younger Dryas (YD) and the Preboreal, highlighted by the gray and yellow box, respectively (the depicted period is approximate).
Interestingly, the relative abundances of H-brGDGTs and H-isoGDGTs exhibit opposite trends across the Holocene in both peat cores (Figures 2 and 3). In both Hani and Gushantun, H-brGDGT (%) is high during the last deglaciation and early Holocene, followed by a decline in the late Holocene (∼last 6 ka, as showed in Figure 2 and 3). On the other hand, H-isoGDGT (%) is low during the last deglaciation and early Holocene, and high during the late Holocene. These results at least indicate that i) The relative abundance of H-GDGTs is not solely related to temperature and ii) archaeal-produced H-isoGDGTs respond differently to environmental/paleoclimatic conditions (e.g., temperature and hydrology etc.) than bacterial-produced H-brGDGTs.
Relationships Between Temperature and “H-Shaped” Glycerol Dialkyl Glycerol Tetraethers
In the modern global peat database, the relative abundances of H-brGDGTs and H-isoGDGTs are both positively correlated with temperature (Naafs et al., 2018). Sollich et al. (2017) observed a similar relationship in marine sediments. Therefore, we expect the depth profiles of H-brGDGTs (%) and H-isoGDGTs (%) to be similar to each other and to the trend in MAATpeat-based temperatures. That is only partially observed here. In the Hani peat, the depth profile of H-brGDGT (%) shares some features with temperature reconstructed using the distribution of brGDGTs and the peat-specific calibration (MAATpeat; Naafs et al., 2017), but the profiles are not identical. H-brGDGT (%) is highest at the base of the peat and remains high while MAATpeat increases from ca. 15 to 11 ka (Figure 2). After ca. 6 ka, MAATpeat declines and H-brGDGT (%) decreases. Similarly, in the Gushantun peat H-brGDGT (%) is high in the early Holocene, just like MAATpeat (Figure 3), but the two parameters are partly decoupled at the base of the peat and latest Holocene. The strongest divergence between H-brGDGT (%) and MAATpeat occurs for the post-deglaciation millennial events, with the former failing to capture abrupt cold/warm events such as Younger Dryas (YD), Preboreal (PB) and ca.8.7 cold event recorded in the MAATpeat record of Hani. In fact, H-brGDGTs (%)-HN is high (ca. 5–6%) during the cold YD and ca.8.7 event and low (ca. 3–4%) at the warm PB period.
In the modern global peat dataset from Naafs et al. (2018) there is scatter in the relationship between temperature and the relative abundance of H-brGDGTs. For example, some modern peats from Siberia (MAAT of – 8°C) have a higher abundance of H-brGDGTs (ca. 4%), than peats from Germany (MAAT of 9°C with <1% H-brGDGTs). As such, the Hani and Gushantun peat profiles share some similarities with the modern dataset–a first order relationship between H-brGDGT distributions and temperature–but also divergences.
In contrast, H-isoGDGT (%) appears to exhibit a completely opposite relationship with temperature than expected from the modern database. High relative abundances of H-isoGDGTs are associated with low reconstructed temperature, and vice versa, in both peat cores (Figures 2 and 3). In fact, in many intervals, H-isoGDGT (%) and MAATpeat exhibit opposite depth profiles. Such a relationship with temperature is contrary to that observed by Naafs et al. (2018) and Sollich et al. (2017). However, in terrestrial hot springs (e.g., Tibet and Yellowstone Naitonal Park; Jia et al., 2014), there is no apparent correlation between the relative abundance of H-isoGDGTs and temperature.
We do not observe the relative abundance of H-GDGTs decreases with depth in two peat cores, thus there appears to be little downcore degradation of either regular or H-GDGTs, suggesting that this is not responsible for the different trends. In any case, it is difficult to envision a mechanism by which H-isoGDGTs were preferentially degraded relative to regular isoGDGTs (and in fact, H-isoGDGTs appear to be well preserved; Schouten et al., 2008b; Knappy et al., 2011).
Instead, the lack of correlation between H-isoGDGT (%) and MAATpeat could be due to changes in peat hydrology and acidity. In both peats, high H-isoGDGT (%) is associated with the wet late Holocene period, and low H-isoGDGT (%) is associated with the dry early Holocene (Zheng et al., 2018). Culture studies suggest that the archaea that biosynthesize H-isoGDGTs are strict anaerobes, including some (extremophilic) members of the Euryarchaeota and Crenarchaea (Morii et al., 1998; Sugai et al., 2004; Schouten et al., 2008b; Knappy et al., 2011; Liu et al., 2012a). Thus, wet conditions during the late Holocene, associated with shoaling of the water table and anoxic conditions, could shift the archaeal community to one that produces a greater proportion of H-isoGDGTs, resulting in relative high H-isoGDGT (%) (ca.0.2–4 kyr in GST and 2–5 kyr in HN, as the yellow boxes showed in Figures 2 and 3).
Additionally, in both peats some intervals characterized by a high abundance of H-isoGDGTs are also associated with low pH. This is inconsistent with Naafs et al. (2018) who found no relationship between H-isoGDGT (%) and pH. However, it is consistent with results from terrestrial hot springs (e.g., Yellowstone National Park), in which the relative abundance of H-isoGDGTs is negatively correlated with pH but has no relationship with temperature. H-isoGDGTs have been identified in some archaea growing at neutral pH, but only at high temperature (>80°C), while at more acidic pH they occur at lower temperature (Morii et al., 1998; Schouten et al., 2008b). This indicates that Archaea do produce H-isoGDGTs under acidic conditions, including at low temperature. Thus, we propose that the high abundance of H-isoGDGTs in Hani and Gushantun is driven primarily by elevated temperatures and only secondarily by low pH (acidic conditions). This is consistent with Jia et al. (2014), who suggested that inclusion of H-isoGDGTs in archaeal lipid membranes is to increase acid tolerance.
The lack of a strong positive relationship between temperature and H-brGDGT (%) and H-isoGDGT (%) (in fact, an apparent negative relationship for the latter) appears to conflict with the global dataset compiled in Naafs et al. (2018). However, that work focused on peats spanning a broad temperature range; moreover, in that dataset, temperature only appeared to exert a control on H-GDGT abundance at relatively high values. Both the Hani and the Gushantun peat were deposited at relatively low temperature, such that other factors including pH or anoxia exert a stronger influence. In fact, H-isoGDGT (%) in the global dataset is relatively insensitive to temperature in the range explored in these peats (up to 14°C).
Another finding of this work is the apparent opposite behavior of H-brGDGT (%) and H-isoGDGT (%). Divergent relationships with environmental parameters have also been observed for regular brGDGTs and isoGDGTs. For example, isoGDGT cyclization is strongly associated with high temperature (Schouten et al., 2013); in contrast, a clear relationship between brGDGT cyclization and temperature is lacking in mineral soil and peat (Weijers et al., 2007; Naafs et al., 2017). Similarly, decreasing pH is typically associated with a decrease in the degree of cyclization in brGDGTs but an increase in isoGDGTs. Considering the distinguishing microbial source of the two H-GDGTs, these differences could reflect an ecological control. However, an intriguing alternative is that the differences between these membrane lipid classes (isoprenoidal vs alkyl chains; glycerol stereochemistry; Schouten et al., 2008b; Knappy et al., 2011; Sollich et al., 2017) cause cyclization and cross-linking to have different impacts on overall membrane proton permeability and resulting in different adaption mechanisms for archaea and bacteria.
Clearly, future research is needed to explore these lipids’ biological source(s) and the environmental controls on their abundance and distribution. Nonetheless, our results indicate that the proxy potential for H-brGDGT (%) and H-isoGDGT (%) in peat is currently limited. We suggest that H-brGDGT (%) has greater utility as a temperature proxy than H-isoGDGT (%), perhaps due to a more limited range of biological sources in peatlands, but it appears that both parameters are influenced by additional factors.
Conclusion
H-isoGDGTs and H-brGDGTs were detected in two peat cores from NE China. This is the first report of downcore variations of H-GDGTs across the late Quaternary in a terrestrial setting. We found that high relative abundances of H-brGDGTs are generally associated with high MAATpeat at Gushantun and Hani, consistent with previous work (Naafs et al., 2018). However and inconsistent with previous work, high relative abundances of H-isoGDGTs are generally associated with low MAATpeat. These results are partly inconsistent with the previous hypothesis that the relative abundance of H-GDGTs in peat is related to temperatures alone; in fact, pH appears to be a control on H-isoGDGTs. These observations suggest that although environmental factors (e.g., temperature, pH) can have an impact on the relative abundance of H-GDGTs, this effect differs in both H-iso- and brGDGTs in Hani and Gushantun settings. This difference has likely been exacerbated because of the low temperature of the peats studied here; it is possible that a stronger temperature control will occur in peat profiles formed at higher temperatures.
Data Availability Statement
The datasets generated for this study are available on request to the corresponding author.
Author Contributions
All authors contributed to manuscript revision, read and approved the submitted version.
Funding
This work was supported by the National Natural Science Foundation of China Grants (41872031, 41372033), the Outstanding Youth Foundation of Shaanxi Province (2018JC-021), the Key Scientific and Technological Team Project in Shaanxi Province, a Marie Curie International Incoming Fellowship within the 7th European Community Framework Program, funds from the State Key Laboratory of Loess and Quatenary Geology (SKLLQG1731), and the MOST Special Fund from the State Key Laboratory of Continental Dynamics, Northwest University. R.D. Pancost and B.D.A. Naafs were funded through the advanced ERC grant “the Greenhouse Earth System” (T-GRES, project reference 340923). B.D.A. Naafs received additional funding through a Royal Society Tata University Research fellowship.
Conflict of Interest
The authors declare that the research was conducted in the absence of any commercial or financial relationships that could be construed as a potential conflict of interest.
Supplementary Material
The Supplementary Material for this article can be found online at: https://www.frontiersin.org/articles/10.3389/feart.2020.541685/full#supplementary-material
References
Bauersachs, T., Weidenbach, K., Schmitz, R. A., and Schwark, L. (2015). Distribution of glycerol ether lipids in halophilic, methanogenic and hyperthermophilic archaea. Org. Geochem. 83-84, 101–108. doi:10.1016/j.orggeochem.2015.03.009
Becker, K. W., Elling, F. J., Yoshinaga, M. Y., Söllinger, A., Urich, T., and Hinrichs, K.-U. (2016). Unusual Butane- and Pentanetriol-based tetraether lipids in Methanomassiliicoccus luminyensis, a representative of the seventh order of Methanogens. Appl. Environ. Microbiol. 82, 4505–4516. doi:10.1128/aem.00772-16
Chen, Y., Zheng, F., Chen, S., Liu, H., Phelps, T. J., and Zhang, C. (2018). Branched GDGT production at elevated temperatures in anaerobic soil microcosm incubations. Org. Geochem. 117, 12–21. doi:10.1016/j.orggeochem.2017.11.015
De Rosa, M., Esposito, E., Gambacorta, A., Nicolaus, B., and Bu’Lock, J. D. (1980). Effects of temperature on ether lipid composition of Caldariella acidophila. Phytochemistry 19, 827–831. doi:10.1016/0031-9422(80)85120-x
Hopmans, E. C., Weijers, J. W. H., Schefuss, E., Herfort, L., Sinninghe Damsté, J. S., and Schouten, S. (2004). A novel proxy for terrestrial organic matter in sediments based on branched and isoprenoid tetraether lipids. Earth Planet Sci. Lett. 224, 107–116. doi:10.1016/j.epsl.2004.05.012
Huguet, A., Fosse, C., Laggoun-Défarge, F., Toussaint, M.-L., and Derenne, S. (2010). Occurrence and distribution of glycerol dialkyl glycerol tetraethers in a French peat bog. Org. Geochem. 41, 559–572. doi:10.1016/j.orggeochem.2010.02.015
Jia, C., Zhang, C. L., Xie, W., Wang, J.-X., Li, F., Wang, S., et al. (2014). Differential temperature and pH controls on the abundance and composition of H-GDGTs in terrestrial hot springs. Org. Geochem. 75, 109–121. doi:10.1016/j.orggeochem.2014.06.009
Knappy, C. S., Nunn, C. E. M., Morgan, H. W., and Keely, B. J. (2011). The major lipid cores of the archaeon Ignisphaera aggregans: implications for the phylogeny and biosynthesis of glycerol monoalkyl glycerol tetraether isoprenoid lipids. Extremophiles 15 (4), 517–528. doi:10.1007/s00792-011-0382-3
Liu, X. L., Summons, R. E., and Hinrichs, K.-U. (2012a). Extending the known range of glycerol ether lipids in the environment: structural assignments based on tandem mass spectral fragmentation patterns. Rapid Commun. Mass Spectrom. 26 (19), 2295–2302. doi:10.1002/rcm.6355
Liu, X. L., Lipp, J. S., Simpson, J. H., Lin, Y.-S., Summons, R. E., and Hinrichs, K.-U. (2012b). Mono- and dihydroxyl glycerol dibiphytanyl glycerol tetraethers in marine sediments: identification of both core and intact polar lipid forms. Geochem. Cosmochim. Acta 89, 102–115. doi:10.1016/j.gca.2012.04.053
Liu, X. L., Lipp, J. S., Schröder, J. M., Summons, R. E., and Hinrichs, K.-U. (2012c). Isoprenoid glycerol dialkanol diethers: a series of novel archaeal lipids in marine sediments. Org. Geochem. 43, 50–55. doi:10.1016/j.orggeochem.2011.11.002
Loomis, S. E., Russell, J. M., and Sinninghe Damsté, J. S. (2011). Distributions of branched GDGTs in soils and lake sediments from western Uganda: implications for a lacustrine paleothermometer. Org. Geochem. 42, 739–751. doi:10.1016/j.orggeochem.2011.06.004
Morii, H., Eguchi, T., Nishihara, M., Kakinuma, K., König, H., and Koga, Y. (1998). A novel ether core lipid with H-shaped C80-isoprenoid hydrocarbon chain from the hyperthermophilic methanogen Methanothermus fervidus. Biochim. Biophys. Acta Lipids Lipid. Metabol. 1390 (3), 339–345. doi:10.1016/s0005-2760(97)00183-5
Naafs, B. D. A., Inglis, G. N., Zheng, Y., Amesbury, M. J., Biester, H., Bindler, R., et al. (2017). Introducing global peat-specific temperature and pH calibrations based on brGDGT bacterial lipids. Geochem. Cosmochim. Acta 208, 285–301. doi:10.1016/j.gca.2017.01.038
Naafs, B. D. A., McCormick, D., Inglis, G. N., and Pancost, R. D.; T-Gres peat database collaborators (2018). Archaeal and bacterial H-GDGTs are abundant in peat and their relative abundance is positively correlated with temperature. Geochem. Cosmochim. Acta 227, 156–170. doi:10.1016/j.gca.2018.02.025
Schouten, S., Baas, M., Hopmans, E. C., and Sinninghe Damsté, J. S. (2008a). An unusual isoprenoid tetraether lipid in marine and lacustrine sediments. Org. Geochem. 39 (8), 1033–1038. doi:10.1016/j.orggeochem.2008.01.019
Schouten, S., Baas, M., Hopmans, E. C., Reysenbach, A.-L., and Sinninghe Damsté, J. S. (2008b). Tetraether membrane lipids of Candidatus “Aciduliprofundum boonei”, a cultivated obligate thermoacidophilic euryarchaeote from deep-sea hydrothermal vents. Extremophiles 12 (1), 119–124. doi:10.1007/s00792-007-0111-0
Schouten, S., Hopmans, E. C., Enno, S., and Sinninghe Damste, J. S. (2002). Distributional variations in marine crenarchaeotal membrane lipids: a new tool for reconstructing ancient sea water temperatures? Earth Planet Sci. Lett. 204 (1-2), 265–274. doi:10.1016/s0012-821x(02)00979-2
Schouten, S., Hopmans, E. C., Pancost, R. D., and Damste, J. S. S. (2000). Widespread occurrence of structurally diverse tetraether membrane lipids: evidence for the ubiquitous presence of low-temperature relatives of hyperthermophiles. Proc. Natl. Acad. Sci. U.S.A. 97, 14421–14426. doi:10.1073/pnas.97.26.14421
Schouten, S., Hopmans, E. C., and Sinninghe Damsté, J. S. (2013). The organic geochemistry of glycerol dialkyl glycerol tetraether lipids: a review. Org. Geochem. 54, 19–61. doi:10.1016/j.orggeochem.2012.09.006
Sinninghe Damsté, J. S., Hopmans, E. C., Pancost, R. D., Schouten, S., and Geenevasen, J. A. J. (2000). Newly discovered non-isoprenoid glycerol dialkyl glycerol tetraether lipids in sediments. Chem. Commun. 17, 1683–1684. doi:10.1039/b004517i
Sinninghe Damsté, J. S., Ossebaar, J., Schouten, S., and Verschuren, D. (2008). Altitudinal shifts in the branched tetraether lipid distribution in soil from Mt. Kilimanjaro (Tanzania): implications for the MBT/CBT continental palaeothermometer. Org. Geochem. 39, 1072–1076. doi:10.1016/j.orggeochem.2007.11.011
Sinninghe Damsté, J. S., Rijpstra, W. I. C., Foesel, B. U., Huber, K. J., Overmann, J., Nakagawa, S., et al. (2018). An overview of the occurrence of ether- and ester-linked iso-diabolic acid membrane lipids in microbial cultures of the Acidobacteria: implications for brGDGT paleoproxies for temperature and pH. Org. Geochem. 124, 63–76. doi:10.1016/j.orggeochem.2018.07.006
Sinninghe Damsté, J. S., Rijpstra, W. I. C., Hopmans, E. C., Foesel, B. U., Wüst, P. K., Overmann, J., et al. (2014). Ether- and ester-boundiso-diabolic acid and other lipids in members of acidobacteria subdivision 4. Appl. Environ. Microbiol. 80, 5207–5218. doi:10.1128/aem.01066-14
Sollich, M., Yoshinaga, M. Y., Häusler, S., Price, R. E., Hinrichs, K. U., and Bühring, S. I. (2017). Heat stress dictates microbial lipid composition along a thermal gradient in marine sediments. Front. Microbiol. 8, 1550. doi:10.3389/fmicb.2017.01550
Sugai, A., Uda, I., Itoh, Y. H., and Itoh, T. (2004). The core lipid composition of the 17 strains of hyperthermophilic archaea, thermococcales. J. Oleo Sci. 53 (1), 41–44. doi:10.5650/jos.53.41
Wang, M., Tian, Q., Li, X., Liang, J., and Hou, J. (2020). TEX86 as a potential proxy of lake water pH in the Tibetan Plateau. Palaeogeogr. Palaeoclimatol. Palaeoecol. 538, 109381. doi:10.1016/j.palaeo.2019.109381
Weijers, J. W. H., Schouten, S., Hopmans, E. C., Geenevasen, J. A. J., David, O. R. P., Coleman, J. M., et al. (2006). Membrane lipids of mesophilic anaerobic bacteria thriving in peats have typical archaeal traits. Environ. Microbiol. 8, 648–657. doi:10.1111/j.1462-2920.2005.00941.x
Weijers, J. W. H., Schouten, S., van den Donker, J. C., Hopmans, E. C., and Sinninghe Damsté, J. S. (2007). Environmental controls on bacterial tetraether membrane lipid distribution in soils. Geochem. Cosmochim. Acta 71, 703–713. doi:10.1016/j.gca.2006.10.003
Yang, H., Lü, X. X., Ding, W. H., Lei, Y. Y., Dang, X. Y., and Xie, S. C. (2015). The 6-methyl branched tetraethers significantly affect the performance of the methylation index (MBT′) in soils from an altitudinal transect at Mount Shennongjia. Org. Geochem. 82, 42–53. doi:10.1016/j.orggeochem.2015.02.003
Zheng, Y., Pancost, R. D., Liu, X., Wang, Z., Naafs, B. D. A., Xie, X., et al. (2017). Atmospheric connections with the North Atlantic enhanced the deglacial warming in northeast China. Geology 45, 1031–1034. doi:10.1130/g39401.1
Zheng, Y., Pancost, R. D., Naafs, B. D. A., Li, Q., Liu, Z., and Yang, H. (2018). Transition from a warm and dry to a cold and wet climate in NE China across the Holocene. Earth Planet Sci. Lett. 493, 36–46. doi:10.1016/j.epsl.2018.04.019
Keywords: branched glycerol dialkyl glycerol tetraethers, isoprenoid glycerol dialkyl glycerol tetraethers, temperature, “H-shaped” glycerol dialkyl glycerol tetraethers, holocene, peat
Citation: Tang X, Naafs BDA, Pancost RD, Liu Z, Fan T and Zheng Y (2021) Exploring the Influences of Temperature on “H-Shaped” Glycerol Dialkyl Glycerol Tetraethers in a Stratigraphic Context: Evidence From Two Peat Cores Across the Late Quaternary. Front. Earth Sci. 8:541685. doi: 10.3389/feart.2020.541685
Received: 10 March 2020; Accepted: 25 September 2020;
Published: 02 February 2021.
Edited by:
Steven L. Forman, Baylor University, United StatesCopyright © 2021 Tang, Naafs, Pancost, Liu, Fan and Zheng. This is an open-access article distributed under the terms of the Creative Commons Attribution License (CC BY). The use, distribution or reproduction in other forums is permitted, provided the original author(s) and the copyright owner(s) are credited and that the original publication in this journal is cited, in accordance with accepted academic practice. No use, distribution or reproduction is permitted which does not comply with these terms.
*Correspondence: Yanhong Zheng, emhlbmdud3VAMTYzLmNvbQ==