- 1Climate and Environmental Physics, Physics Institute, University of Bern, Bern, Switzerland
- 2Oeschger Centre for Climate Change Research, University of Bern, Bern, Switzerland
- 3Institute of Geography, University of Innsbruck, Innsbruck, Austria
- 4Institute of Geological Sciences, University of Bern, Bern, Switzerland
The analysis of the stable isotope of the tree-ring cellulose is an important tool for paleo climatic investigations. Long tree-ring chronologies consist predominantly of oaks and conifers in Europe, including larch trees (Larix decidua) and cembran pines (Pinus cembra) that form very long tree ring chronologies in the Alps and grow at the treeline, where tree growth is mainly determined by temperature variations. We analyzed δ13C, δ18O and δ2H isotopes in the cellulose extracted from tree-rings of wood samples collected at high altitude in the Swiss and Tyrol Alps, covering the whole Holocene period. We found that larch cellulose was remarkably more depleted in deuterium than that of cembran pine, with mean δ2H values of −113.4 ± 9.7‰ for larch and of −65.4 ± 11.3‰ for cembran pine. To verify if these depleted values were specific to larch or a property of the deciduous conifers, we extended the analysis to samples from various living conifer species collected at the Bern Botanical Garden. The results showed that not only the larch, but also all the samples of the deciduous larch family had a cellulose composition that was highly depleted in δ2H with regard to the other evergreen conifers including cembran pine, a difference that we attribute to a faster metabolism of the deciduous conifers. The δ18O values were not statistically different among the species, in agreement with the hypothesis that they are primary signals of the source water. While the δ13C values were slightly more depleted for larch than for cembran pine, likely due to metabolic differences of the two species. We conclude that the deciduous larch conifers have specific metabolic hydrogen fractionations and that the larch unique signature of δ2H is useful to recognize it from other conifers in subfossil wood samples collected for paleoclimatic studies. For climate information the absolute δ2H values of larch should be considered carefully and separate from other species.
Introduction
The stable isotope ratios of carbon, oxygen and hydrogen have been studied in various components of plants, including bulk wood samples, lignin, whole leaves and leaf waxes (Borella et al., 1998; Borella et al., 1999; Loader et al., 2003; Kahmen et al., 2011; Kimak and Leuenberger 2015), and those analyzed in tree ring samples were successfully used for the reconstruction of past climate conditions, thus widening the field of dendroclimatology (Borella et al., 1998; Leuenberger 1998; McCarroll and Loader 2004; Treydte et al., 2007; Frank et al., 2015). Tree ring samples are made of cellulose, hemicelluloses and lignin (Khezami et al., 2005) and cellulose is the most used material for isotope ratio studies (Borella et al., 1998; Borella et al., 1999; Loader et al., 2003; McCarroll and Loader 2004). It is a carbohydrate that contains the stable isotope ratios of carbon (δ13C), oxygen (δ18O) and hydrogen (δD alias δ2H) (Cormier et al., 2018) that carry climate information and can act as climate proxies. In dry alpine sites the δ13C values are controlled mainly by stomatal conductance, which is linked to summer moisture stress and thus to precipitations (Gagen et al., 2004). Early studies noted strong relationships between cellulose δ18O and mean annual temperature and humidity (Libby et al., 1976; Burk and Stuiver 1981). More recently in relatively humid locations tree ring δ18O values have been used to reconstruct precipitation (Brienen et al., 2012; Boysen et al., 2014), whereas in other locations tree-ring δ18O reflected a combination of vapor pressure deficit, relative humidity and sunshine (Roden and Ehleringer 2007; Xu et al., 2011; Boysen et al., 2014; Hartl-Meier et al., 2014; Labuhn et al., 2014). The δ2H values have been measured in tree rings for paleo climatological investigations for forty years (Schiegl 1974), but their interpretation still remains complex. The interest in δ2H is stimulated by recent developments in isotope ratio mass spectrometry combined with equilibrium methods that allow the measurement of carbon, oxygen and hydrogen isotope ratios at the same time (Filot et al., 2006; Loader et al., 2014). This led to results that indicated that δ2H in plant cellulose is determined by 1) the δ2H value of the water source, 2) the water evaporation in the leaf that enriches heavier water isotopes in the liquid phase and 3) the biosynthetic isotopic fractionation between leaf water and the final organic compounds like cellulose, that includes many complex biochemical processes (Cormier et al., 2019). Altogether, the deuterium fractionation is related to both environmental and physiological factors (Augusti 2007).
The oxygen and hydrogen in the plant cellulose originate mainly from meteoric water and the fractionation processes during pre-photosynthetic, photosynthetic and post-carboxylation reactions affects both δ18O and δ2H, but in a different way (Yakir et al., 1990). Leaf evapotranspiration is expected to enrich heavy isotopes in leaf water (Nabeshima et al., 2018), but some enzymes of glucose biosynthesis are selective for the stable H isotopes and imprint a specific Deuterium (2H) abundance in each H group bound to the C of cellulose (Augusti et al., 2006). It should be noted that the analytical approach of cellulose used here (the cellulose pyrolysis) detects all hydrogens, but the exchangeable hydrogens are equilibrated with a water of known hydrogen isotope ratio (Filot et al., 2006). The non-exchangeable hydrogen isotope ratio is then calculated from the measured and the equilibrated non-exchangeable hydrogens. The specific δ2H in each H bound to the C of cellulose documents a distinct biochemical history (Augusti 2007) that showed minor differences between spruce and oak (Augusti et al., 2008). However, the isotopomer variations seem to have a minor effect on total variation of δ2H in cellulose, and no specific studies on larch isotopomers have been reported so far.
In the last decade various studies have been published on deuterium abundance in cellulose from different tree genus, mainly from Quercus, Fagaceae (Kimak et al., 2015), Picea (Gori et al., 2013), Pinus, Pinales (Nabeshima et al., 2018), Larix (Hafner et al., 2011) and Abeis that all use C3 photosynthesis mechanism (Tang et al., 2000; McCarroll and Loader 2004; Szczepanek et al., 2006).
One study found that in the early annual growing season the cellulose of leaves of deciduous oak and beech was enriched (−20‰), while that of the evergreen taxus was more depleted (−100‰) (Kimak et al., 2015). Similar results were found in tree rings cellulose from oak and beech, with higher δ2H values (−10‰) in the early annual growing season than in the late growing season (−50‰), possibly due to the use of stored carbohydrates (Nabeshima et al., 2018). Thus, δ2H values change during the tree growth phases. The heterotrophic metabolism causes a 2H (D) an enrichment of cellulose higher than that of autotropic metabolism, probably due to a higher recycling of compounds in the Calvin and tricarboxylic acid cycles that is associated with a more complete exchange of C-bound H with the surrounding and enriched foliar water (Ziegler 1995; Nabeshima et al., 2018; Cormier et al., 2019). The effect of metabolism on 2H fractionation in cellulose is supported also by the finding that C4 plants are more 2H -enriched than C3 plants (Ziegler et al., 1976). The different photosynthesis systems and anatomies of C3 and C4 plants influence 2H -biological fractionation via C-bound H exchanges with water in the different anatomical compartments (Zhou et al., 2016).
The data reported above indicate that factors other than climate affect the isotopic fractionation signal of tree ring wood, and they should be identified for their robust use in paleo dendroclimatology. To this aim we have measured δ2H, δ18O and δ13C isotopes in the cellulose of 4399 subfossil samples of 120 conifers cembran pine and larch and of one spruce collected at 12 different sites in Switzerland, Austria and Italy. They cover 8930 years and were collected in the project the Eastern Alpine Conifer Chronology for paleoclimatic studies (Nicolussi et al., 2009). With the aim to recognize the non-climatic signals in the dataset we investigated, in this study, site influences and species differences regarding isotope compositions. These investigations were extended to many living conifers sampled at the Bern Botanical Garden.
Materials and Methods
Sampling Sites in the Alps
The wood samples belong to the Eastern Alpine Conifer Chronology Dataset, the collection and preparation of which have been described earlier (Nicolussi et al., 2009). Part of the dataset includes samples from living trees that cover the last 190 years, from 1820–2010 A.D. These tree ring samples were collected at three upper tree-line sites at the proximity of glacier sites at elevations above 1900 m (Ziehmer et al., 2018). These three sites are: Val Roseg (VRR) where samples of four cembran pines and four larch trees were collected, valley d’Hérens (FPCR) four larches and at Grimselsee (UZAR) four cembran pine trees were sampled (Table 1). The other part of the dataset consists of subfossil wood samples from the dominant tree-line species, the deciduous European larch (Larix decidua Mill) and the evergreen cembran pine (Pinus cembra (L.)) with the inclusion of a single tree of the evergreen spruce (Picea abies (L.) H.Karst). They cover the last 9000 years, the whole Holocene, and were collected at 12 different sites in the European Alps which cover a SW-NE transect, at an elevation range of 1,930 to 2,400 m a.s.l. with different aspects. The site locations are shown in Figure 1 and their characteristics are listed in Table 1. Each species and site are represented by four trees. The samples we analyzed were wood blocks of tree-rings covering five consecutive years (Ziehmer et al., 2018).
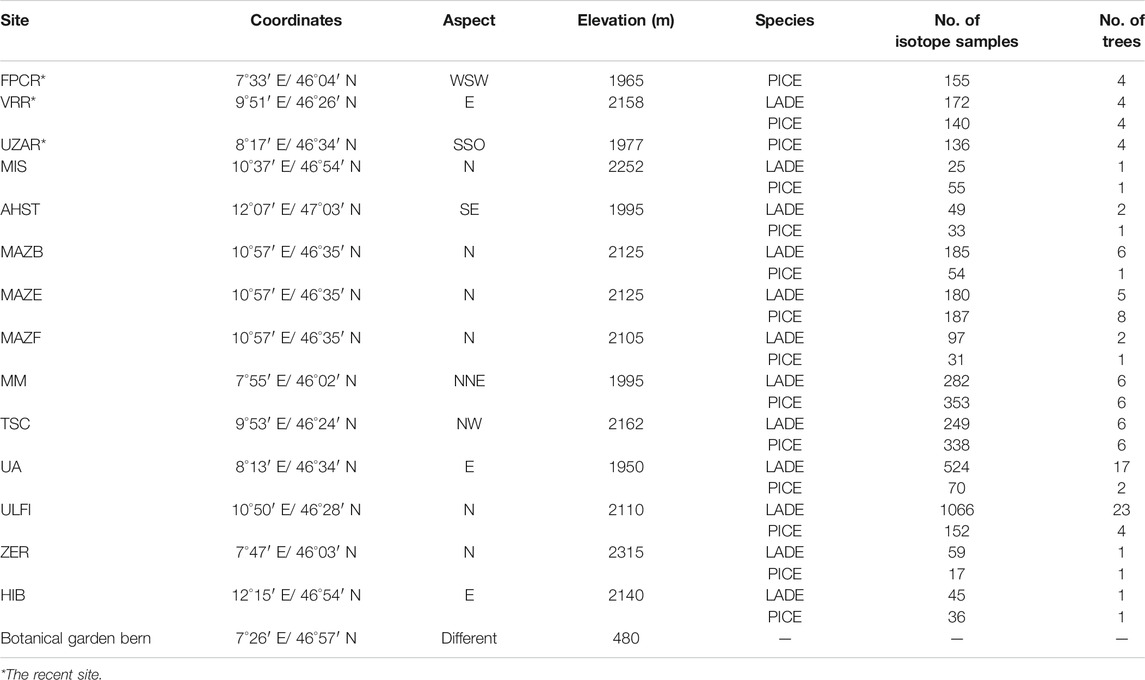
TABLE 1. Characteristics of the 12-sampling sites, number of trees and samples (each sample constitutes of a block of 5 years). Specie code: larch: LADE, cembran pine: PICE, spruce: PCAB.
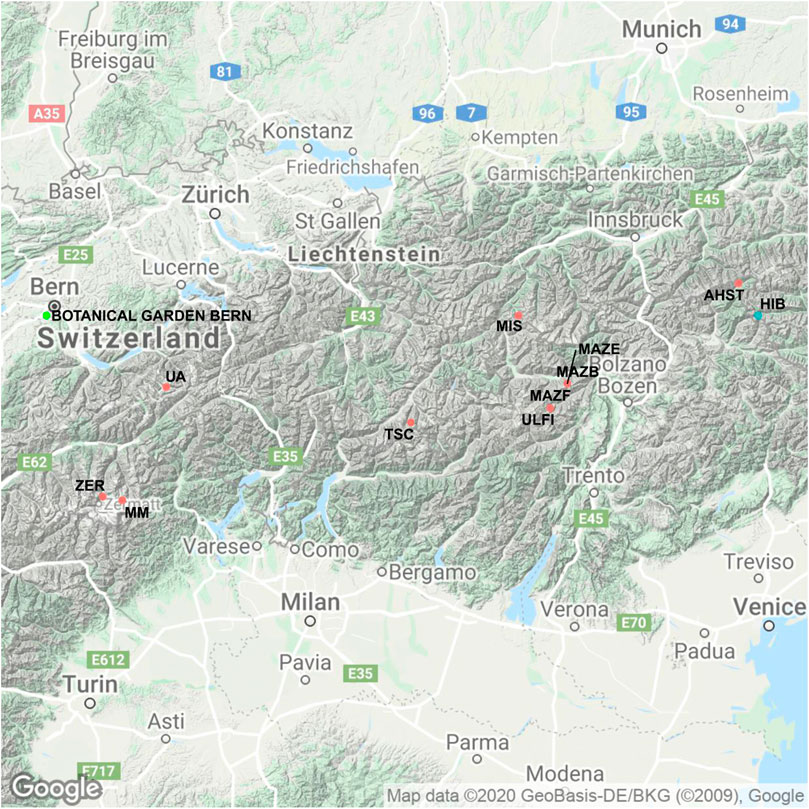
FIGURE 1. Map of the 12 collection sites of the trees described in Figures 2,3. The sites are located in Switzerland and Tyrol and their codes used in Figure 2 are shown. In blue are sites at which larch and cembran pine were collected, in green where cembran pine and spruce were collected, in black the sites of the modern (living) trees (with the exception of VVR, which is as site with both living and subfossil samples), in light-green the location of the Botanical Garden in Bern is illustrated. Details of site characteristics and of sample collection is given in Table 1. (© Google Maps 2020)
Wood Samples From Bern Botanical Garden
We sampled tree branches at the Botanical Garden in Bern, some of which were dead. The bark and the cambium were removed from the wood before preparing the samples for analysis. A small wood amount (about 50 mg) was chosen for the extraction of the cellulose and the isotope ratio analysis was done as described below. Each sample was measured at least twice. The samples included 20 different conifer species and one of the broad leaf gymnosperm Ginkgo biloba. The larch family was represented by six different species: Larix decidua, L. decidua subsp. polonica, L. sibirica, L. laricina and one species of Pseudolarix amabilis. The evergreen conifers included eight different species of pinus (Pinus bungeana, P. nigra, P. sylvestris, P. cembra, P. mugo, P. speuce, P. ponderosa, P. wallichiana). The other evergreen conifers were Cephalotaxus harringtonii, Cedrus atlantica, Cupressus nootkatensis, Taxus baccata and Tsuga Canadensis.
Sample Wood Dating
Calendar-dated tree-ring width series of Holocene wood sections are available at the Institute of Geography of the University of Innsbruck, where also the Eastern Alpine Conifer Chronology (EACC) has been established (Nicolussi et al., 2009). For isotope and cellulose analyses, the calendar-dated discs were cut into five-year blocks.
Stable Isotope Analysis
The isotope measurements were carried out using wood blocks of consecutive five tree rings. The separation of wood samples into five-year blocks was performed at the University of Innsbruck, Austria. The procedure of cellulose extraction and the calculation of the cellulose content (cellulose dry weight/ wood dry weight) (Ziehmer et al., 2018) as well as the triple-isotope analysis were described before (Loader et al., 2015). Briefly, we used conventional Isotope Ratio Mass Spectrometry (Isoprime 100) coupled to a pyrolysis unit (HEKAtech GmbH, Germany), which is similar to the previously used TC/EA (for technical details see (Leuenberger 2007)). This approach was extended to measurements of non-exchangeable hydrogen of alpha-cellulose using the on-line equilibration method (Filot et al., 2006; Loader et al., 2015). The results are reported in per mil (‰) relative to the Vienna Pee Dee Belemnite (VPDB) for carbon and to Vienna Standard Mean Ocean Water (VSMOW) for hydrogen and oxygen (Coplen 1994). We applied two-point calibrations using Merck cellulose and IAEA-CH-6 crystalline sugar for carbon and oxygen isotopes as well as Merck cellulose and IAEA-CH-7 PE polyethylene foil for hydrogen isotopes. Wei Ming 101 cellulose was used as target material. Merck and Wei Ming 101 cellulose are well characterized internal laboratory standard materials. The precision of the measurement was ±3.0‰ for hydrogen, ±0.3‰ for oxygen and ±0.15‰ for carbon (Loader et al., 2015).
Statistical Analysis
Statistical analysis was performed using the R software and was aimed at verifying differences between the groups. The type of data distribution was analyzed with the Shapiro-test (Shapiro and Francia 1972). To verify the equality between the groups we used pairwise comparisons by the Wilcoxon test (Wilcoxon et al., 1970). The density of the data is shown by the violin contours in Figure 2 (Hintze and Nelson 1998).
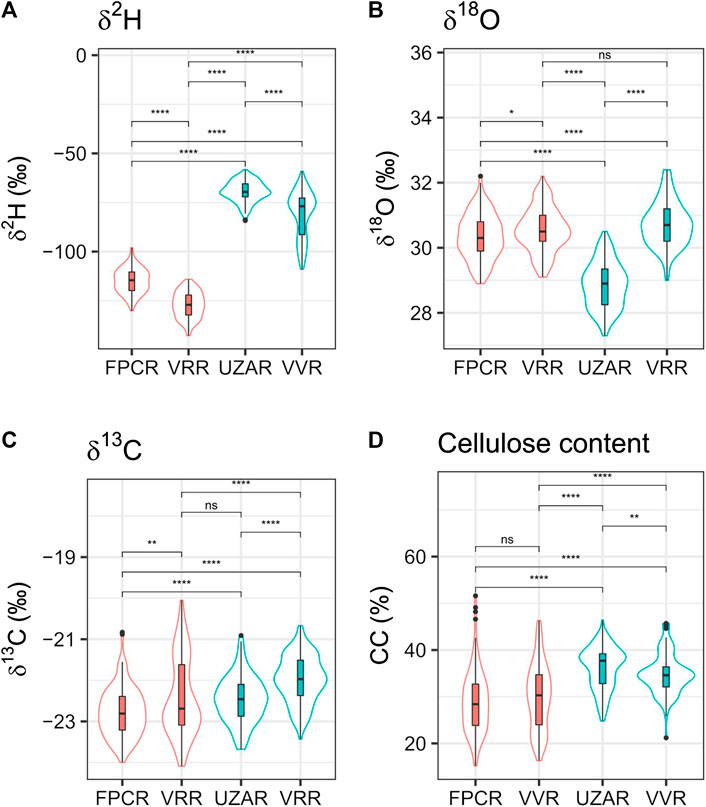
FIGURE 2. Isotopes in the cellulose of living trees from Alpine sites. They are presented as boxplot with median and limits of 90 and 95 percentile, contoured by the violins plots that show the density distribution of the data used (Hintze and Nelson 1998). Mean values of δ2H, δ13O, δ18C and cellulose content of cembran pine and larch trees dated 1820–2010 A.D. and collected at three sites in the Alps: Valley d’Hérens (FPCR), Val Roseg (VRR) and Grimselsee (UZAR). The values of each site are based on four different trees whose wood has been sampled in blocks covering 5 years. At VRR both larch (red) and cembran pine (blue) were collected, while at FPCR and UZAR only one species was collected, respectively larch and cembran pine. The box plots show the median, SD and spread, the surrounding violin plot shows the sample density (Hintze and Nelson 1998). The statistical difference calculated with Wilcoxon test are indicated above the horizontal lines: ns, non-significant: p > 0.05, *p ≤ 0.05, **p ≤ 0.01, ***p ≤ 0.001, ****p ≤ 0.0001. LADE FPCR n samples: 132 n trees: 4LADE VRR: n sample s: 135 n trees: four PICE VRR n sample s: 125 n trees: 4PICE UAZR n sample s: 135 n trees: 4.
Results
Stable Isotopes and Cellulose Content in Living Conifers (1820–2010 A.D)
We first analyzed a subset of the samples of the project the Eastern Alpine Conifer Chronology for paleoclimatic studies (Nicolussi et al., 2009) represented by living trees grown in the last 190 years. They originate from eight larches and eight cembran pines, collected at three sites (VVR, FPCR and UZAR). The results, shown in Figure 2, are separated for species and for collection sites to illustrate and evaluate the effect of these two factors on isotope signatures.
δ2H Data
The δ2H data (Figure 2A) show a major difference between larch and cembran pine irrespective of the collection site. The mean larch value (4 trees at each site) at VRR site was −127.2 ± 6.6‰ and at FPCR site was −114.9 ± 6.3‰, while the mean cembran pine value at the UAZR site was −69.5 ± 11.0‰ and at VRR site −80.9 ± 11.8‰. The VRR cembran pine group shows a tail in the lower part, which is due to a single tree with a mean value of −96.8‰ that is more negative than the other three trees with mean values of −69.1, −73.7, −76.1‰. This made the distribution of the δ2H non-normal in the VRR cembran pine group, while it was normal in the other three groups. The data indicate that the δ2H values follow mainly species type.
δ18O Data
The δ18O data (Figure 2B) show that the density distribution and the means of the larch at the FPCR and at the VRR sites are very similar and are also similar to those of the cembran pine at the VRR site, but significantly different from those of the cembran pine at the UZAR site. A Wilcoxon test indicated a marginally significant difference between two larch groups (p = 0.025), contrarily, the difference between the two cembran pine groups was highly significant (p < 2 × 10–16). The values of the cembran pine group from VRR were not significantly different from those of the larch from the same VRR site (p = 0.06) but were significantly different from the larch values at the FPCR site (p = 6.4 × 10–5). The data indicate that the δ18O values follow mainly the site and not the tree species.
δ13C Data
The δ13C values of larch tended to be more negative than those of cembran pine (Figure 2C). The dispersion of the larch group values at VRR site was larger than that of larch group at FPCR site, but the means are similar with no significant differences. Also, the cembran pine values at the two regions were not significantly different. The only non-significant difference (Wilcoxon test: p = 0.455), although marginal, between the groups was observed for larch at VRR site and cembran pine at UZAR site. These values showed to be affected only to a minor extent by geography and species.
Cellulose Content
The cellulose content values of the four groups are shown in Figure 2D. The value distribution is more dispersed in the larch groups than in the cembran pine groups, independent from the site origin. A non-significant difference is found with the Wilcoxon test between the two larch groups (p = 0.133). The cellulose did not seem to confer major information and was not considered in the following part of the study.
Stable Isotopes of Subfossil Holocene Conifers
In order to verify whether the differences of living trees from Alpine sites shown above are not only due to the specific sites and restricted to the recent period, we extended the analysis to the complete wooden material of the Eastern Alpine Conifer Chronology (Nicolussi et al., 2009) collected at independent sites of the Alps, with the exclusion of the living trees of Figure 1. It consisted of >4000 wood samples from 120 trees (Table 1) and the sites of sample collection as listed in Figure 1.
δ2H Data
The δ2H values of the samples of the two species collected at the 12 sites are shown in Figure 3A as box-plots. It confirms an evident difference between the more negative values of the deciduous larch and the less negative values of the evergreen cembran pine samples, with no overlap for samples collected at the same site. The total mean for larch is −113.4 ± 9.7‰ and that for cembran pine −61.4 ± 11.4‰, values that are very close to those presented in Figure 2. An exception was the subfossil from spruce tree (Picea abies, PCAB) found at the HIB site the identity of which was initially unclear, since it is not easy to distinguish it from larch specimens by microscopic wood anatomy (Schoch et al., 2004). The δ2H values of these samples were in the range of those of the cembran pine (green in Figure 3A) and overlapped with those of the cembran pine that were collected at the same site, with no statistical difference (p = 0.74) between the two. They are trees of different species and different time periods, altogether the differences between larch and cembran pine are always in the range 40 to 50‰, while that at HIB between cembran pine and spruce was close to 0‰.
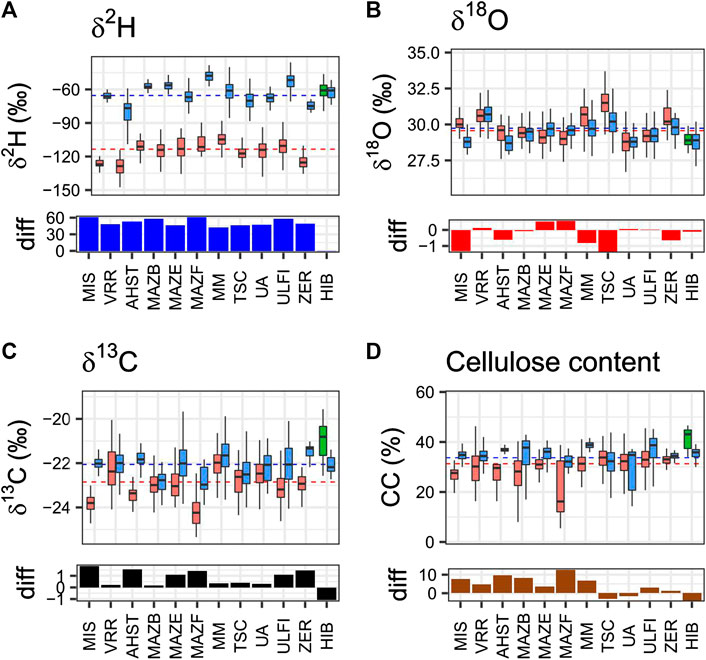
FIGURE 3. Isotopes in the cellulose of sub-fossil Holocene trees. Tree samples were collected at 12 different sites in the Alps. Site abbreviations are indicated below the plots. Samples were dated by dendrochronological tools and the extracted cellulose analyzed for δ2H, δ18O and δ13C isotope ratio variations and for their cellulose content. Each box represents median and limits of 25th and 75th percentile of each specie in each site, and the species are depicted in different colors: larch in red, cembran pine in blue and the single spruce in green. The horizontal black dashed line are the total mean values of cembran pine and larch. The histograms under the box plots show the per mil difference (diff) between the means, larch minus cembran pine (larch minus spruce for the HIB site). AHST: LADE n samples: 49 n trees: 2—PICE: n sample: 33 n trees: 1LADE: MAZB n sample: 185 n trees: 6—PICE n sample: 54 n trees: one LADE: MAZE n sample: 180 n trees: 5—PICE n sample: 187 n trees: eight LADE: MAZF n sample: 97 n trees: 2—PICE n sample: 31 n trees: one LADE: MIS n sample: 25 n trees: 1—PICE n sample: 55 n trees: one LADE: MM n sample: 282 n trees: 6—PICE n sample: 353 n trees: six LADE: TSC n sample: 249 n trees: 6—PICE n sample: 338 n trees: six LADE: UA n sample: 524 n trees: 17—PICE n sample: 70 n trees: two LADE: ULFI n sample: 1066 n trees: 23—PICE n sample: 152 n trees: four LADE: VRR n sample: 172 n trees: 4—PICE n sample: 140 n trees: four LADE: ZER n sample: 59 n trees: 1—PICE n sample: 17 n trees: one PCAB: HIB n sample: 45 n trees: 1—PICE n sample: 36 n trees: 1.
δ18O Data
The δ18O values from each site are shown in Figure 3B. The total mean for larch is 29.6 ± 1.1‰ and that for cembran pine 28.9 ± 0.5‰. A difference between the two species is not evident at sites VRR, HIB, MAZB, UA and ULFI. However, at the site ZER the two species showed a marginally significant difference (difference of the mean values of 0.6‰, p = 0.007), whereas at the sites MIS, AHST, MAZE, MAZF, MM the differences were highly significant (difference of the mean values ok −1 ot 0.6‰ p < 0.001). Boxplots of the 12 sites partially overlap, MIS and TSC are the only sites for which we found a difference of the mean values larger than 1‰. In MAZE, MAZAF, UA, ULFI, VRR sites the mean of larch was smaller than for cembran pine, for the remaining sites it was higher. The differences between the two species are in the range of 0.6 to −1‰ and do not show a common trend. The trees of some sites show values that are rather distant from the mean values and this is probably due to factors linked to the location (elevation, latitude, longitude, aspect) and others that influence the tree metabolism (e.g., soil composition), it can be noted that at sites where δ18O values are below total mean the corresponding δ2H are above or vice versa, suggesting that the two isotopes are subjected to distinct influences and that soil water is not the primary driver.
δ13C Data
The δ13C values of the trees are shown in Figure 3C. The total mean for larch is -22.6 ± 0.8‰ and that for cembran pine −22.2 ± 0.6‰. The difference of the values between the two species is significant at all sites with the exception of MAZB site (p = 0.05) and of UA (p = 0.001). The dotted line indicates the total mean value and most of the cembran pine values (blue boxplots) are above or close to the mean value, while the larch values (red boxplots) are below or close to it. In fact, for the sites where both species are present, the larch is always more depleted than the cembran pine. The difference plot shows that the only negative column is at the site HIB, spruce shows significantly less depleted values than cembran pine, while the oxygen values are similar. This might be due to some characteristics of the site, like the aspect, that are known to affect temperature-controlled changes in assimilation regulated by enzymatic isotopic fractionation processes (δ13C) (Esper et al., 2018).
Stable Isotopes in Contemporary Conifers
The above data showed that larch has δ2H values of cellulose that are much lower than those of cembran pine and spruce (Figures 2,3). In order to verify if this difference is attributed to the metabolism of the evergreen conifers versus that of deciduous larch, we looked for a larger number of conifer species. The Bern Botanic Garden provided us with samples of six different species of larch (deciduous), one pseudo-larch (deciduous), and 13 different species of evergreen conifers including pinus, taxus and cedrus, and the gymnosperm, broadleaf, deciduous Ginkgo Biloba. We processed the samples and analysed their isotope content as describe above. Figure 4 shows the results, in red are the deciduous conifers (larch and pseudo-larch), in green the evergreen conifers and in blue the values of Ginkgo Biloba, the horizontal lines are the mean value of each group and the dashed lines shows the interval of the standard deviation.
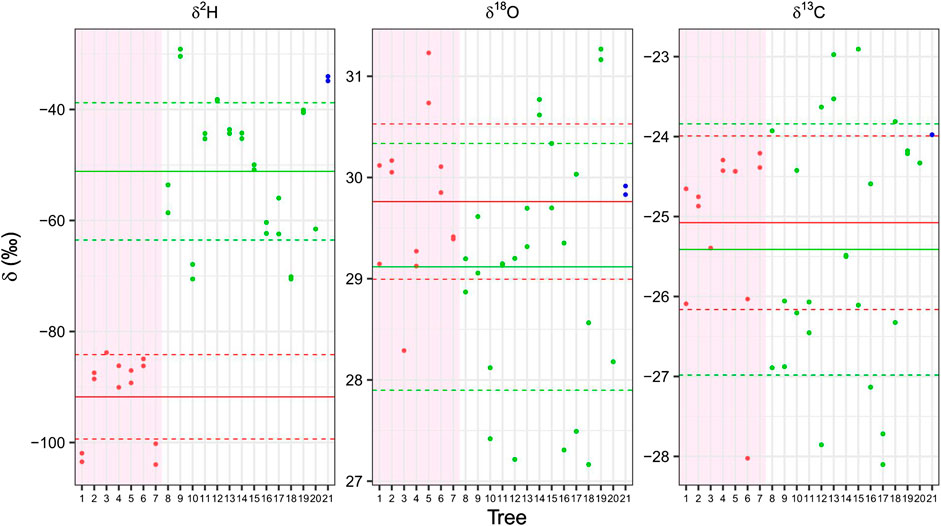
FIGURE 4. Isotopes in cellulose of living trees of 21 different species collected at the Bern Botanical Garden. Each box represents the cellulose isotopes, δ2H δ18O and δ13C. The deciduous conifers (larch and Pseudolarix amabilis) are displayed in red in the reddish back ground (trees 1–7), in green the evergreen conifers (Genus: Pinus, Cipressus, Cedrus, Taxus, Tsuga) (trees 8–20) and in blue the only broadleaf gymnosperm (Ginkgo biloba, tree 21). The means of the deciduous and of the evergreen conifer species are indicated as horizontal red and blue lines, respectively, the dashed lines shows the interval of the standard deviation. A clear distinction between the evergreen and deciduous conifers is present only for δ2H values, even if they come from branches and from different years. 1 = Larix decidua; 2 = Larix decidua polonica; 3 = Larix gmelinii; 4 = Larix kaempferii; 5 = Larix laricina; 6 = Larix sibirica; 7 = Pseudolarix amabilis; 8 = Cedrus atlantica; 9 = Cephalotaxus harringtonia; 10 = Cupressus nootkatensis; 11 = Pinus bungeana; 12 = Pinus cembra; 13 = Pinus mugo; 14 = Pinus nigra; 15 = Pinus nigra 16 = Pinus peuce; 17 = Pinus sylvestris; 18 = Pinus wallichiana; 19 = Taxus baccata; 20 = Tsuga canadensis; 21 = Ginkgo biloba.
δ2H
The δ2H values of the deciduous larch group (mean −91.8 ± 7.6‰) is well separated from that of evergreen conifers (mean −51.1 ± 12.3‰) (Figure 4). This confirms that the deciduous conifer trees are strongly depleted in comparison with the evergreen ones with no overlap between the two groups and a highly significant difference (p = 1.92 e − 05). The values of Pseudolarix amabilis (tree #7 in Figure 4) are in full agreement with those of the other larches (#1–6). While the values of the Cedrus atlantica (#8), Cupressus nootkatensis (#10) and Taxus baccata (#19) are in agreement with those of the Pinus (#11–18), which are all evergreen conifers. Ginkgo biloba (#21) does not belong to the conifer order and is deciduous, but its values are close to those of the evergreen conifers.
δ18O
The difference between the δ18O value of the deciduous conifers (29.8 ± 0.8‰) and that of the evergreen conifers (29.1 ± 1.2‰) was not statistically significant (Figure 4). The distance between the two means was barely significant (p = 0.084). The absolute δ18O and δ2H values of samples from the botanic garden conifers are less depleted from the ones of the of the Alpine trees, this was attributed to higher depletion of the heavy isotopes of hydrogen and oxygen of source water at high elevations (Kern et al., 2014).
δ13C
Mean δ13C values show no statistically significant difference between the deciduous (−25.1 ± 1‰) and the evergreen groups (−25.4 ± 1.5‰) (Figure 4).
Discussion
A major finding of present work is the much higher depletion of deuterium in the cellulose of the deciduous larches compared to the evergreen conifer cembran pine growing at the same site. The δ2H values of larch that we found are in good agreement with the only previous measurement of the hydrogen isotope in larch cellulose (Hafner et al., 2011). The difference between δ2H of larch and cembran pine has been observed in eight trees covering the last 190 years, grown in Val Roseg valley, Grimselstausee and Val d’Herens valley (Figure 2) and the 120 trees covering the whole Holocene (Figure 3)The difference is large, with a δ2H mean of −127‰ for larch and −81‰ for the cembran pine and no overlapping values. This is a non-climatic signal and was not observed in the other two stable isotopes we analyzed, δ13C and δ18O, the values of which were similar in the two conifer species.
All but one of the trees of the Eastern Alpine Conifer Chronology were larch and cembran pine, and thus it was unclear whether the observed differences were specific to the two species or caused by different physiological processes of evergreen and deciduous conifers. The absence of difference between cembran pine and Picea abies in the site HIB is surprising as they are of different species and originate from different time periods, the cembran pine covered the period 5695–5516 B2K, some 1500 years before the spruce tree that covered the 4000–3766 B2K period. Thus, we measured conifer samples from the Bern Botanical Garden and the results showed that the cellulose of all the larch species including the Pseudo-larix amabilis had more depleted δ2H values than that of the evergreen conifers (Figure 4). The deciduous non-conifer Ginkgo biloba resulted to have δ2H value in the range of the evergreen ones. The range of δ2H values of the evergreen conifers (−30 to −70‰) is similar to the ones reported in deciduous broadleaf trees such as oak (−75 and −45‰) (Szczepanek et al., 2006; Etien et al., 2009), and in the conifers Pinus sylvestris (Gori et al., 2013) and spruce (−20 and −50‰) in three regions that cover a vast geographical area with different conditions (Loader et al., 2008). These values are quite distinct from the δ2H values we found in the larch family with a mean of −120‰ and range −150 to −86‰. Thus, all the deciduous conifers (larch and pseudo larches), present δ2H values that are very different from those of the evergreen conifers. This can be used for the identification of larch from spruce wood that have similar wood anatomic characteristics and are therefore very difficult to distinguish in subfossil specimens (Schoch et al., 2004). Moreover, our data show that for paleoclimatic use of isotopic dendrochronology the δ2H values of larch cellulose must not mixed with those of evergreen conifers, but can be used separately or after applying suitable corrections or scaling.
The major δ2H difference indicates that deciduous and evergreen conifer handle hydrogen in a different way, while the similar δ18O values indicate that they handle oxygen in the same way. The soil water is the source of the two elements and their isotopes, and its uptake by the roots does not cause fractionation of both isotope compositions (White et al., 1985; Ehleringer and Dawson 1992; Dawson and Ehleringer 1993; Lin and Sternberg 1993). Evapotranspiration in the leaves causes enrichment in similar extent of the two heavy isotopes (Flanagan et al., 1991; Yakir 1992; McCarroll and Loader 2004; Studer et al., 2015), thus we can conclude that this does not cause the difference of δ2H between the two species but leaving the δ18O in the two species unchanged (Figure 2). The difference will occur in some subsequent biological steps, likely at carboxylation or post-carboxylation levels that might differ in deciduous and evergreen conifers.
Deciduous larches are characterized by a short photosynthesis period that involves a higher photosynthesis capacity to compete with the surrounding evergreen trees (Tranquillini 1962; Tranquillini 1979). Consequently, they have faster rates of photosynthesis and net assimilation and a more efficient carboxylation system than evergreen conifers (Sweet and Wareing 1968; Larcher 1969; Fry and Phillips 1977; Gowin et al., 1980). The biochemical steps that amplify δ2H depletion when photosynthetic activity is high have been identified (Cormier et al., 2018): when the supply of photosynthetic carbohydrates is low, the produced organic compounds are relatively 2H-enriched (Cormier et al., 2018), when the photosynthetic activity is high the produced organic compounds are relatively 2H-depleted (Yakir 1992). Moreover, larch is known to have a lower heterotrophic metabolism than the surrounding evergreen conifers (Vaganov et al., 2009; Rinne et al., 2015).
In cembran pine heterotrophism increase with the altitude (Wieser et al., 2019). The finding that the δ2H difference between larches and evergreen conifers is lower in Bern (∼500 m elevation) than at >2000 m of tree-line (40‰ in Figure 4 vs 52‰ in Figure 3) support the hypothesis that heterotrophism affect δ2H but the difference between the two metabolisms is maintained.
Regarding the δ13C values they were all within the range of −23‰ to −28‰, as previously described in C3 plants (Sternberg and DeNiro 1983; Leuenberger 1998). This value in leaf sugar is controlled mainly by the ratio ci/ca (ci being the CO2 concentration in the intercellular space of leaves and ca the CO2 concentration of the atmosphere), which is related to photosynthetic water-use efficiency, WUE, (also called transpiration efficiency) (Farquhar and Sharkey 1982). In our results δ13C values of larch were consistently slightly more negative than those of cembran pine (Figure 3), in agreement with previous data showing that larch species have more negative carbon values than the evergreen conifers because of their lower water-use efficiency (WUE) (Gower and Richards, 1990; Kloeppel et al., 1998). Altogether the results indicate that neither the species nor geography have major effect on δ13C signature.
Regarding the δ18O values we found no evident difference between the two species, in accordance with the interpretation that these values are more sensitive to the source water signal than to other external variables, such as relative humidity and vapor pressure deficit. We found a δ18O difference between the sites in the Swiss and the Tyrolian Alps, likely due to the source water, the δ18O of which is known to be linked to the altitude and to a continental effect (Rozanski et al., 1993). This indicates that δ18O values of different conifers species can be used together for paleoclimatic studies, after taking into account the geographical effects.
Conclusion
We show that the deciduous larch family of trees presents unique highly negative δ2H values compared to evergreen conifers. We attributed the difference to a higher photosynthetic and metabolic activity of larch that drive a reduced exchange of δ2H sucrose with the surrounding enriched leaf water leading to δ2H depletion of the photosynthetic products, in comparison with cembran pine and more in general with the evergreen conifers. No significant differences of δ18O values were found between trees grown at the same site and under similar conditions, but differences were found among trees grown at different sites. Minor more negative δ13C values of larch were consistently found than those of the cembran pine, but no evident driver of the difference could be identified. We also conclude that for paleoclimatic studies, for δ2H values the evergreen and deciduous conifers should be keep separately or normalized for the tree species, while δ18O does not seem to be affected by tree species, but by the source water of each site. Regarding δ13C we do not have yet a clear driver of the difference in carbon isotope. We suggest the determination of the δ2H values as tool for distinguishing larch samples from those of other conifers, such as spruce, which are difficult to identify in subfossil wood.
Data Availability Statement
The datasets generated for this study are available on request to the corresponding author.
Author Contributions
TA and MZ performed the stable isotope analyses, TA drafted the first version of the manuscript. KN collected the samples and made the cross dating. ML contributed to the evaluation of the results. ML, KN, and CS conceived of the presented idea. All authors provided comments to improve the manuscript.
Funding
The project is funded by the Swiss National Science Foundation (SNSF, 2000212_144255, 200020_172550) as well as by the Austrian Science Fund (FWF, grant I-1183-N19) and is supported by the Oeschger Center for Climate Change Research, University of Bern, Bern, Switzerland (OCCR).
Conflict of Interest
The authors declare that the research was conducted in the absence of any commercial or financial relationships that could be construed as a potential conflict of interest.
Acknowledgments
We are grateful to Peter Nyfeler for his precious assistance during stable isotopes measurements, to Andrea Thurner and Andreas Österreicher for the preparation of the isotope samples from Alpine sites, to Prof. Dr. Markus Fischer and Silvan Glauser of the Botanical Garden of Bern for the kind agreement to collect the conifer samples and the civil service collaborators: Lars Herrmann, dslbGiacomo Ruggia, Jonathan Lamprecht, Yannick Rohrer, Rafael Zuber.
References
Augusti, A., Betson, T. R., and Schleucher, J. (2006). Hydrogen exchange during cellulose synthesis distinguishes climatic and biochemical isotope fractionations in tree rings. New Phytol. 172, 490–499. doi:10.1111/j.1469-8137.2006.01843.x
Augusti, A. (2007). Monitoring climate and plant physiology using deuterium isotopomers of carbohydrates Fysiologisk botanik. PhD thesis. Umea (Sweden): Umea University.
Augusti, A., Betson, T. R., and Schleucher, J. (2008). Deriving correlated climate and physiological signals from deuterium isotopomers in tree rings. Chemical Geology 252 (1–2), 1–8. doi:10.1016/j.chemgeo.2008.01.011
Borella, S., Leuenberger, M., and Saurer, M. (1999). Analysis of δ18O in tree rings: wood-cellulose comparison and method dependent sensitivity. J. Geophys. Res. 104, 19267–19273. doi:10.1029/1999jd900298
Borella, S., Leuenberger, M., Saurer, M., and Siegwolf, R. (1998). Reducing uncertainties in δ13C analysis of tree rings: pooling, milling, and cellulose extraction. J. Geophys. Res. 103, 19519–19526. doi:10.1029/98jd01169
Boysen, B. M., Evans, M. N., and Baker, P. J. (2014). δ18O in the tropical conifer Agathis robusta records ENSO-related precipitation variations. PLoS One 9, e102336. doi:10.1371/journal.pone.0102336
Brienen, R. J. W., Helle, G., Pons, T. L., Guyot, J.-L., and Gloor, M. (2012). Oxygen isotopes in tree rings are a good proxy for amazon precipitation and El Nino-Southern oscillation variability. Proc. Natl. Acad. Sci. 109, 16957–16962. doi:10.1073/pnas.1205977109
Burk, R. L., and Stuiver, M. (1981). Oxygen isotope ratios in trees reflect mean annual temperature and humidity. Science 211, 1417–1419. doi:10.1126/science.211.4489.1417
Coplen, T. B. (1994). Reporting of stable hydrogen, carbon, and oxygen isotopic abundances (technical report). Pure Appl. Chem. 66, 273–276. doi:10.1351/pac199466020273
Cormier, M.-A., Werner, R. A., Leuenberger, M. C., and Kahmen, A. (2019). 2H-enrichment of cellulose and n-alkanes in heterotrophic plants. Oecologia 189, 365–373. doi:10.1007/s00442-019-04338-8
Cormier, M.-A., Werner, R. A., Sauer, P. E., Gröcke, D. R., Leuenberger, M. C., Wieloch, T., et al. (2018). 2H-fractionations during the biosynthesis of carbohydrates and lipids imprint a metabolic signal on the δ2H values of plant organic compounds. New Phytol. 218, 479–491. doi:10.1111/nph.15016
Dawson, T. E., and Ehleringer, J. R. (1993). Isotopic enrichment of water in the “woody” tissues of plants: implications for plant water source, water uptake, and other studies which use the stable isotopic composition of cellulose. Geochimica et Cosmochimica Acta 57, 3487–3492. doi:10.1016/0016-7037(93)90554-a
Ehleringer, J. R., and Dawson, T. E. (1992). Water uptake by plants: perspectives from stable isotope composition. Plant Cell Environ. 15, 1073–1082. doi:10.1111/j.1365-3040.1992.tb01657.x
Esper, J., Holzkämper, S., Büntgen, U., Schöne, B., Keppler, F., Hartl, C., et al. (2018). Site-specific climatic signals in stable isotope records from Swedish pine forests. Trees 32, 855–869. doi:10.1007/s00468-018-1678-z
Etien, N., Daux, V., Masson-Delmotte, V., Mestre, O., Stievenard, M., Guillemin, M. T., et al. (2009). Summer maximum temperature in northern France over the past century: instrumental data versus multiple proxies (tree-ring isotopes, grape harvest dates and forest fires). Climatic Change 94, 429–456. doi:10.1007/s10584-008-9516-8
Farquhar, G. D., and Sharkey, T. D. (1982). Stomatal conductance and photosynthesis. Annu. Rev. Plant. Physiol. 33 (1), 317–345. doi:10.1146/annurev.pp.33.060182.001533
Filot, M. S., Leuenberger, M., Pazdur, A., and Boettger, T. (2006). Rapid online equilibration method to determine the D/H ratios of non-exchangeable hydrogen in cellulose. Rapid Commun. Mass Spectrom. 20, 3337–3344. doi:10.1002/rcm.2743
Flanagan, L. B., Comstock, J. P., and Ehleringer, J. R. (1991). Comparison of modeled and observed environmental influences on the stable oxygen and hydrogen isotope composition of leaf water in Phaseolus vulgaris L. Plant Physiol. 96, 588–596. doi:10.1104/pp.96.2.588
Frank, D., Poulter, B., Saurer, M., Esper, J., Huntingford, C., Helle, G., et al. (2015). Water-use efficiency and transpiration across European forests during the Anthropocene. Nat. Clim. Change 5, 579–583. doi:10.1038/nclimate2614
Fry, D., and Phillips, I. (1977). Photosynthesis of conifers in relation to annual growth cycles and dry matter production: II. Seasonal photosynthetic capacity and mesophyll ultrastructure in Abies grandis, Picea sitchensis, Tsuga heterophylla and Larix leptolepis growing in SW England. Physiol. Plant. 40, 300–306. doi:10.1111/j.1399-3054.1977.tb04077.x
Gagen, M., McCarroll, D., and Edouard, J.-L. (2004). Latewood width, maximum density, and stable carbon isotope ratios of pine as climate indicators in a dry subalpine environment, French Alps. Alpine Research Arctic, Antarctic 36 (2), 166–171. doi:10.1657/1523-0430(2004)036[0166:lwmdas]2.0.co;2
Gori, Y., Wehrens, R., Greule, M., Keppler, F., Ziller, L., La Porta, N., and Camin, F. (2013). Carbon, hydrogen and oxygen stable isotope ratios of whole wood, cellulose and lignin methoxyl groups of Picea abies as climate proxies. Rapid Commun. Mass Spectro. 27 (1), 265–275. doi:10.1002/rcm.6446
Gower, S. T., and Richards, J. H. (1990). Larches: deciduous conifers in an evergreen world. Bioscience 40, 818–826. doi:10.2307/1311484
Gowin, T., Lourtioux, A., and Mousseau, M. (1980). Influence of constant growth temperature upon the productivity and gas exchange of seedlings of Scots pine and European larch. Forest Science 26, 301–309. doi:10.1093/forestscience/26.2.301
Hafner, P., Robertson, I., McCarroll, D., Loader, N. J., Gagen, M., Bale, R. J., et al. (2011). Climate signals in the ring widths and stable carbon, hydrogen and oxygen isotopic composition of Larix decidua growing at the forest limit in the southeastern European Alps. Trees 25 (6), 1141–1154. doi:10.1007/s00468-011-0589-z
Hilasvuori, C., Zang, C., Büntgen, U., Esper, J., Rothe, A., Göttlein, A., et al. (2014). Uniform climate sensitivity in tree-ring stable isotopes across species and sites in a mid-latitude temperate forest. Tree Physiol. 35, 4–15. doi:10.1093/treephys/tpu096
Hintze, J. L., and Nelson, R. D. (1998). Violin plots: a box plot-density trace synergism. Am. Stat. 52, 181–184. doi:10.1080/00031305.1998.10480559
Kahmen, A., Sachse, D., Arndt, S. K., Tu, K. P., Farrington, H., Vitousek, P. M., et al. (2011). Cellulose δ18O is an index of leaf-to-air vapor pressure difference (VPD) in tropical plants. Proc. Natl. Acad. Sci. USA 108, 1981–1986. doi:10.1073/pnas.1018906108
Kern, Z., Kohán, B., and Leuenberger, M. (2014). Precipitation isoscape of high reliefs: interpolation scheme designed and tested for monthly resolved precipitation oxygen isotope records of an Alpine domain. Atmos. Chem. Phys. 14, 1897–1907. doi:10.5194/acp-14-1897-2014
Khezami, L., Chetouani, A., Taouk, B., and Capart, R. (2005). Production and characterisation of activated carbon from wood components in powder: cellulose, lignin, xylan. Powder Technology 157, 48–56. doi:10.1016/j.powtec.2005.05.009
Kimak, A., Kern, Z., and Leuenberger, M. (2015). Qualitative distinction of autotrophic and heterotrophic processes at the leaf level by means of triple stable isotope (C–O–H) patterns. Front. Plant Sci. 6, 1008. doi:10.3389/fpls.2015.01008
Kimak, A., and Leuenberger, M. (2015). Are carbohydrate storage strategies of trees traceable by early-latewood carbon isotope differences?. Trees 29 (3), 859–870. doi:10.1007/s00468-015-1167-6
Kloeppel, B. D., Treichel, I. W., Kharuk, S., and Gower, S. T. (1998). Foliar carbon isotope discrimination in Larix species and sympatric evergreen conifers: a global comparison, Oecologia. 114, 153–159. doi:10.1007/s004420050431
Labuhn, I., Daux, V., Pierre, M., Stievenard, M., Girardclos, O., Féron, A., et al. (2014). Tree age, site and climate controls on tree ring cellulose δ18O: a case study on oak trees from south-western France. Dendrochronologia 32, 78–89. doi:10.1016/j.dendro.2013.11.001
Larcher, W. (1969). Effect of environmental and physiological variables on the carbon dioxide gas exchange of trees. Photosynthetica 3, 167–198.
Leuenberger, M. (1998). Stable isotopes in tree rings as climate and stress indicators. Zürich, Switzerland: Vdf Hochschulverlag AG.
Leuenberger, M. (2007). To what extent can ice core data contribute to the understanding of plant ecological developments of the past?. Terres. Eco. 1, 211–233. doi:10.1016/s1936-7961(07)01014-7
Libby, L. M., Pandolfi, L. J., Payton, P. H., Marshall, J., Becker, B., and Giertz-Sienbenlist, V. (1976). Isotopic tree thermometers. Nature 261, 284. doi:10.1038/261284a0
Lin, G., and Sternberg, L. S. L. (1993). “Hydrogen isotopic fractionation by plant roots during water uptake in coastal wetland plants,” in Stable isotopes and plant carbon-water relations (Cambridge, MA: Academic Press), 497–510. doi:10.1016/b978-0-08-091801-3.50041-6
Loader, N. J., Robertson, I., and McCarroll, D. (2003). Comparison of stable carbon isotope ratios in the whole wood, cellulose and lignin of oak tree-rings. Palaeogeogr. Palaeocl. 196, 395–407. doi:10.1016/s0031-0182(03)00466-8
Loader, N. J., Santillo, P. M., Woodman-Ralph, J. P., Rolfe, J. E., Hall, M. A., Gagen, M., et al. (2008). Multiple stable isotopes from oak trees in southwestern Scotland and the potential for stable isotope dendroclimatology in maritime climatic regions. Chem. Geol. 252, 62–71. doi:10.1016/j.chemgeo.2008.01.006
Loader, N. J., Street-Perrott, F. A., Daley, T. J., Hughes, P. D. M., Kimak, A., Levanič, T., et al. (2014). Simultaneous determination of stable carbon, oxygen, and hydrogen isotopes in cellulose. Anal. Chem. 87, 376–380. doi:10.1021/ac502557x
Loader, N. J., Street-Perrott, F. A., Daley, T. J., Hughes, P. D. M., Kimak, A., Levanič, T., et al. (2015). Simultaneous determination of stable carbon, oxygen, and hydrogen isotopes in cellulose. Anal. Chem. 87, 376–380. doi:10.1021/ac502557x
McCarroll, D., and Loader, N. J. (2004). Stable isotopes in tree rings. Quaternary Science Reviews 23, 771–801. doi:10.1016/j.quascirev.2003.06.017
Nabeshima, E., Nakatsuka, T., Kagawa, A., Hiura, T., and Funada, R. (2018). Seasonal changes of δD and δ18O in tree-ring cellulose of Quercus crispulasuggest a change in post-photosynthetic processes during earlywood growth. Tree Physiol. 38, 1829–1840. doi:10.1093/treephys/tpy068
Nicolussi, K., Kaufmann, M., Melvin, T. M., Van Der Plicht, J., Schießling, P., and Thurner, A. (2009). A 9111 year long conifer tree-ring chronology for the European Alps: a base for environmental and climatic investigations. The Holocene 19, 909–920. doi:10.1177/0959683609336565
Rinne, K. T., Saurer, M., Kirdyanov, A. V., Loader, N. J., Bryukhanova, M. V., Werner, R. A., et al. (2015). The relationship between needle sugar carbon isotope ratios and tree rings of larch in Siberia. Tree Physiol. 35 (11), 1192–205. doi:10.1093/treephys/tpv096
Roden, J. S., and Ehleringer, J. R. (2007). Summer precipitation influences the stable oxygen and carbon isotopic composition of tree-ring cellulose in Pinus ponderosa. Tree Physiol. 27, 491–501. doi:10.1093/treephys/27.4.491
Rozanski, K., Araguás‐Araguás, L., and Gonfiantini, R. (1993). Isotopic patterns in modern global precipitation. Clim. Change Contin. Isoto. Rec. 78, 1–36.
Scheidegger, Y., Saurer, M., Bahn, M., and Siegwolf, R. (2000). Linking stable oxygen and carbon isotopes with stomatal conductance and photosynthetic capacity: a conceptual model. Oecologia 125, 350–357. doi:10.1007/s004420000466
Schiegl, W. E. (1974). Climatic significance of deuterium abundance in growth rings of Picea. Nature 251, 582. doi:10.1038/251582a0
Schoch, W., Heller, I., Schweingruber, F. H., and Kienast, F. (2004). Wood anatomy of central European species. Birmensdorf, Switzerland: Swiss Federal Institute for Forest.
Shapiro, S. S., and Francia, R. S. (1972). An approximate analysis of variance test for normality. J. Am. Stat. Assoc. 67, 215–216. doi:10.1080/01621459.1972.10481232
Sternberg, L., and DeNiro, M. J. (1983). Isotopic composition of cellulose from C3, C4, and CAM plants growing near one another. Science 220, 947–949. doi:10.1126/science.220.4600.947
Studer, M. S., Siegwolf, R. T. W., Leuenberger, M., and Abiven, S. (2015). Multi-isotope labelling of organic matter by diffusion of. Biogeosciences 12, 1865–1879. doi:10.5194/bg-12-1865-2015
Sweet, G. B., and Wareing, P. (1968). A comparison of the seasonal rates of dry matter production of three coniferous species with contrasting patterns of growth. Ann. Bot. 32, 721–734. doi:10.1093/oxfordjournals.aob.a084245
Szczepanek, M., Pazdur, A., PawełCzyk, S., Böttger, T., Haupt, M., Hałas, S., et al. (2006). Hydrogen, carbon and oxygen isotopes in pine and oak tree rings from southern Poland as climatic indicators in years 1900–2003. Geochronometria: Journal on Methods Applications of Absolute Chronology 25, 67–73.
Tang, K., Feng, X., and Ettl, G. J. (2000). The variations in δD of tree rings and the implications for climatic reconstruction. Geochem. Cosmochim. Acta 64, 1663–1673. doi:10.1016/s0016-7037(00)00348-3
Tranquillini, W. (1962). Beitrag zur Kausalanalyse des Wettbewerbs ökologisch verschiedener Holzarten. Ber. Deut. Bot. Ges. 75, 356–364.
Tranquillini, W., Frank, D., Esper, J., Andreu, L., Bednarz, Z., Berninger, F., et al. (1979). Physiological ecology of the alpine timberline. Berlin, Germany: Springer-Verlag.
Treydte, K. (2007). Signal strength and climate calibration of a European tree‐ring isotope network. Geophys. Res. Lett. 34 (24). doi:10.1029/2007GL031106
Vaganov, E. A., Schulze, E. D., Skomarkova, M. V., Knohl, A., Brand, W. A., and Roscher, C. (2009). Intra-annual variability of anatomical structure and δ 13 C values within tree rings of spruce and pine in alpine, temperate and boreal Europe. Oecologia 161 (4), 729–745. doi:10.1007/s00442-009-1421-y
White, J. W., Cook, E. R., and Lawrence, J. R. (1985). The DH ratios of sap in trees: implications for water sources and tree ring DH ratios. Geochem. Cosmochim. Acta 49, 237–246. doi:10.1016/0016-7037(85)90207-8
Wieser, G., Oberhuber, W., and Gruber, A. (2019). Effects of climate change at treeline: Lessons from space-for-time studies, manipulative experiments, and long-term observational records in the Central Austrian Alps. Forests 10 (6), 508. doi:10.3390/f10060508
Wilcoxon, F., Katti, S., and Wilcox, R. A. (1970). “Critical values and probability levels for the Wilcoxon rank sum test and the Wilcoxon signed rank test,” in Selected tables in mathematical statistics. (Providence, RI, American Mathematical Soc.), 1, 171–259.
Xu, C., Sano, M., and Nakatsuka, T. (2011). Tree ring cellulose δ18O of Fokienia hodginsii in northern Laos: a promising proxy to reconstruct ENSO?. J. Geophys. Res.: Atmosphere 116. doi:10.1029/2011jd016694
Yakir, D., DeNiro, M., and Ephrath, J. (1990). Effects of water stress on oxygen, hydrogen and carbon isotope ratios in two species of cotton plants. Plant Cell Environ. 13, 949–955. doi:10.1111/j.1365-3040.1990.tb01985.x
Yakir, D. (1992). Variations in the natural abundance of oxygen‐18 and deuterium in plant carbohydrates. Plant Cell Environ. 15, 1005–1020. doi:10.1111/j.1365-3040.1992.tb01652.x
Zhou, Y., Grice, K., Stuart‐Williams, H., Hocart, C. H., Gessler, A., and Farquhar, G. D. (2016). Hydrogen isotopic differences between C3 and C4 land plant lipids: consequences of compartmentation in C4 photosynthetic chemistry and C3 photorespiration. Plant Cell Environ. 39, 2676–2690. doi:10.1111/pce.12821
Ziegler, H. (1995). “Deuterium content in organic material of hosts and their parasites.” in Ecophysiology of photosynthesis. (Berlin, Germany: Springer), 393–408.
Ziegler, H., Osmond, C., Stichler, W., and Trimborn, P. (1976). Hydrogen isotope discrimination in higher plants: correlations with photosynthetic pathway and environment. Planta 128, 85–92. doi:10.1007/bf00397183
Keywords: stable isotopes, deuterium, larch, conifer, cellulose
Citation: Arosio T, Ziehmer-Wenz MM, Nicolussi K, Schlüchter C and Leuenberger M (2020) Larch Cellulose Shows Significantly Depleted Hydrogen Isotope Values With Respect to Evergreen Conifers in Contrast to Oxygen and Carbon Isotopes. Front. Earth Sci. 8:523073. doi: 10.3389/feart.2020.523073
Received: 12 February 2020; Accepted: 04 November 2020;
Published: 03 December 2020.
Edited by:
Steven L. Forman, Baylor University, United StatesReviewed by:
Nadia Solovieva, University College London, United KingdomKaren L. Bacon, National University of Ireland Galway, Ireland
Copyright © 2020 Arosio, Ziehmer-Wenz, Nicolussi, Schlüchter and Leuenberger. This is an open-access article distributed under the terms of the Creative Commons Attribution License (CC BY). The use, distribution or reproduction in other forums is permitted, provided the original author(s) and the copyright owner(s) are credited and that the original publication in this journal is cited, in accordance with accepted academic practice. No use, distribution or reproduction is permitted which does not comply with these terms.
*Correspondence: Tito Arosio, dGl0by5hcm9zaW9AY2xpbWF0ZS51bmliZS5jaA==