- 1Laboratory of Meteorological Disaster, Ministry of Education (KLME)/Joint International Research Laboratory of Climate and Environment Change (ILCEC)/Collaborative Innovation Center on Forecast and Evaluation of Meteorological Disasters (CIC-FEMD), Nanjing University of Information Science and Technology, Nanjing, China
- 2Quzhou Meteorological Bureau, Zhejiang, China
- 3Key Laboratory of Cenozoic Geology and Environment, Institute of Geology and Geophysics, Chinese Academy of Sciences, Beijing, China
- 4CAS Center for Excellence in Life and Paleoenvironment, China University of Chinese Academy of Sciences, Beijing, China
- 5State Key Laboratory of Lake Science and Environment, Nanjing Institute of Geography and Limnology, Chinese Academy of Sciences, Nanjing, China
- 6Department of Geography, The Ohio State University, Columbus, OH, United States
Geological records indicated the termination of the Holocene Thermal Maximum (dramatic drying) occurred progressively later at lower latitudes in both North Africa and East Asia, along with the coherent weakening of local summer monsoon. Here we show that this time-transgressive evolution was dominated by the southward migration of monsoon fringe (shrinking monsoon domain) under monsoon weakening, as illustrated in a transient climate-terrestrial ecosystem model simulation. The monsoon fringe retreating southward during the Holocene, as well as expanding northward during the last deglaciation, occurred synchronously in a belt extending from North Africa to East Asia, which induced a locally humid-arid transition and the subsequent dramatic environment impact. The migration of Afro-Asia monsoon fringe since the Last Glacial Maximum was modulated by the orbital forcing through its impact on land-ocean thermal contrast, aiding by the variation of CO2 concentration.
Introduction
Since the Last Glacial Maximum (LGM), the Afro-Asian monsoon system, including East Asian monsoon, South Asian monsoon and North African monsoon, has experienced a coherent strengthening during the last deglaciation and weakening during the Holocene (Fleitmann et al., 2003; Dykoski et al., 2005; Weldeab et al., 2007; Wang et al., 2008; Shi and Yan, 2019) with the maximum at the Holocene Thermal Maximum (HTM, around 8 ka, kilo years ago before 1950; Haug et al., 2001). Synthesis analysis of monsoon records in North Africa and East Asia illustrated a common asynchronous termination of the HTM, which occurred later in lower latitudes (An et al., 2000; Shanahan et al., 2015). The eco-environmental response to this time-transgressive termination of the HTM is much robust in the northern part of Afro-Asian monsoon domains, as illustrated by the dramatic variation of paleo-lake and vegetation (Street and Grove, 1976; Yu and Harrison, 1996; Lézine et al., 1998; Enzel et al., 1999; Armitage et al., 2015; Goldsmith et al., 2017).
The time-transgressive evolution of paleo-hydrology during the period of the Holocene in North Africa and East Asia was previously attributed to the southward migration of monsoon rainbelt, which was forced by reduced summer insolation (Shanahan et al., 2015), or/and seasonality (An et al., 2000). The mechanism of rainbelt migration may work for East Asia, presented as the anti-phase changes of precipitation within large meridional range of the monsoon (Zhang et al., 2018), but it may not be so robust in North Africa (Doherty et al., 2000). For the uniform time-transgressive evolution of paleo-hydrology occurred in both North Africa and East Asia, is there a common mechanism? The southward shift of the Intertropical Convergence Zone (ITCZ) during this period (Haug et al., 2001) may have contributed to this time-transgressive evolution in the monsoon region, but its physical connection with the environment variation occurred at higher latitudes is not obvious. In addition, why is the environmental response in the northern part of the Afro-Asian monsoon domain so large? Meanwhile, is there any internal relationship between the time-transgressive evolutions of North Africa and East Asia?
To address these questions, the change of monsoon fringe, the limit of the monsoon domain, may be the critical factor. Besides the general monsoon precipitation which widely used to represent the intensity of monsoon (Shi and Yan, 2019), the fringe of Afro-Asian monsoon varied largely as documented in geological records (Winkler and Wang, 1993; Yan and Petit-Maire, 1994; Adams, 1997; Jiang and Liu, 2007; Schneider et al., 2014; Skonieczny et al., 2015; Yang et al., 2015; Goldsmith et al., 2017; Tierney et al., 2017; Sha et al., 2019), and simulated in climate models (Doherty et al., 2000; Jiang et al., 2015a, b). Reconstructions and simulations consistently indicated monsoon fringe swung northward during the last deglaciation, afterward swung southward during the Holocene. On the other hand, the Afro-Asian monsoon is accompanied by an abroad arid region on its northern side (Figure 1A), which is forced by atmospheric meridional circulation and favored by the Tibetan Plateau (Broccoli and Manabe, 1992). Therefore, the migration of northern Afro-Asian monsoon fringe, which corresponds to the expansion or shrink of the monsoon domain, may induce the time-transgressive evolution of local precipitation and the subsequent dramatic response of environment for reasons of possible humid-arid transition. Thus, we hypothesize the recorded time-transgressive evolution of paleo-hydrology in the Afro-Asian monsoon regions and the dramatic environment variation may be dominated by the migration of monsoon fringe.
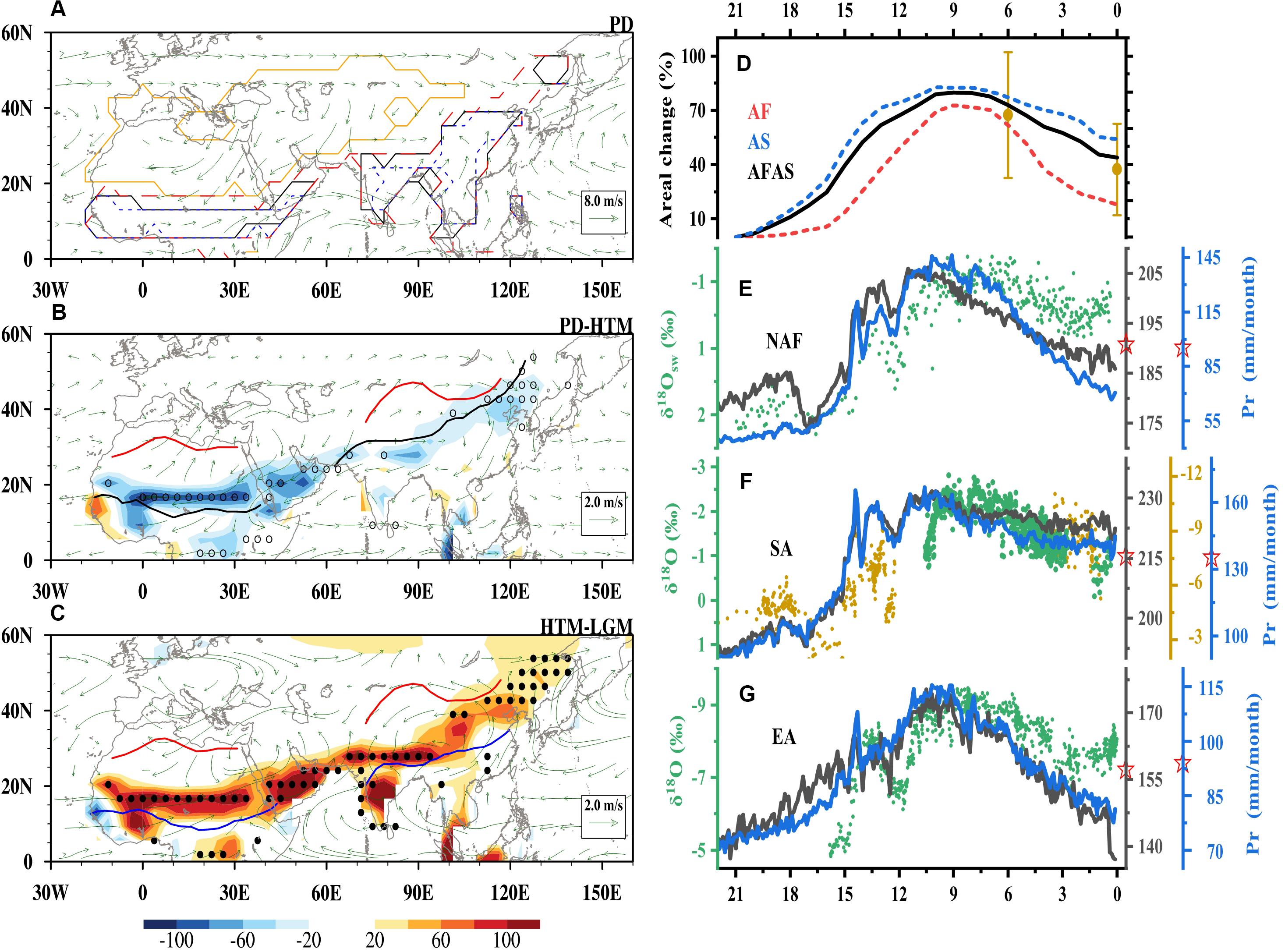
Figure 1. (A) Simulated summer (JJA) 850-hPa wind (UV850; vector, units: m/s), Afro-Asian monsoon domain (black line) and arid domain (orange line) in present day (PD). Monsoon domains at Last Glacial Maximum (LGM) and Holocene Thermal Maximum (HTM) are also indicated by blue dotted and red dashed lines, respectively; (B) Changes of precipitation (Pr; shading, units: mm/month), UV850 (vector, units: m/s), and monsoon domain (black circles) between PD and HTM (PD – HTM); (C) Same as (B), except for changes between HTM and LGM (HTM – LGM); (D) Areal changes (relative to LGM) of monsoon domain over Asia (blue dashed line), North Africa (red dashed line), and Afro-Asian (black solid line) with the unit of %. The multi-model ensemble results of PMIP3 and one intra-model standard deviation range of eight models are plotted in brown dots and bars; and (E–G) Simulated precipitation variation averaged over interior monsoon regions (gray curve; averaged over LGM domain illustrated in (A) and over fringe-migrationing regions (blue curve; averaged over monsoon domain difference between LGM and HTM). Their average values since LGM are labeled in the y-axis (units: mm/month). Records of oxygen isotope of MD03-2707 (Weldeab et al., 2007), Qunf cave (Fleitmann et al., 2003), Dongge cave (Dykoski et al., 2005) and Bittoo cave (Kathayat et al., 2016) are indicated by green/brown dots. The red curve in (B,C) depicts recorded monsoon fringe during HTM (Winkler and Wang, 1993; Adams, 1997; Jiang and Liu, 2007), black curve in (B) PD and blue curve in (C) LGM (Yan and Petit-Maire, 1994).
To test this hypothesis, we investigate the fringe change of the Afro-Asian monsoon and the possible subsequent environment response in a transient climate-terrestrial ecosystem model simulation since the LGM, aided by some other snapshot simulations at the LGM and mid-Holocene. Furthermore, the potential contributions of external forcing to the possible change of monsoon fringe are investigated.
Data and Method
The transient climate-terrestrial ecosystem simulation starting from the LGM used here is TraCE21 (Liu et al., 2009). This simulation was performed using the fully coupled NCAR CCSM3 at a spatial resolution of T31 (3.75°× 3.75°; Collins et al., 2006), and forced by the realistic climatic forcing that consisted of orbital insolation (Orb; Berger, 1978), atmospheric CO2 (Joos and Spahni, 2008), meltwater discharge (McManus et al., 2004), and continental ice sheets (ICE-5G; Peltier, 2004). TraCE21 involved the land processes with a dynamic vegetation component, whose outputs could be used to directly compare with the corresponding proxy records. Four sets of solo-forcing experiments are also performed to investigate their individual contributions to the total climate variation since the LGM (He et al., 2013). In this paper, we employ the full-forcing and two solo-forcing experiments (Orb and CO2) of TraCE21 to study the variations of Afro-Asian monsoon fringe, the subsequent environmental impact and the underling mechanism. The monsoon fringe in model simulation is defined as the edge of monsoon domain, whose general migration represents the expansion or the shrink of monsoon domain. To focus on the long-term evolution of monsoon fringe and local environment, our analyses are all based on the centennial mean data. Aiding to this transient climate simulation, a series of Paleoclimate Modeling Intercomparison Project Phase 3 (PMIP3) simulations during the LGM and mid-Holocene (Supplementary Table 1) are also included to improve the reliability of the results derived from TraCE21.
Comparing to the paleoclimate records, the full-forcing simulation of TraCE21 reasonably reproduced the general evolution of the Afro-Asian monsoon (Otto-Bliesner et al., 2014; Liu et al., 2014; Shanahan et al., 2015; Wen et al., 2016; Cheng et al., 2019; Shi and Yan, 2019). The spatial distribution of the Afro-Asian monsoon and adjacent arid regions are all well simulated (Figure 1A), compared to those derived from observations (Supplementary Figure 1). The simulated variation of monsoon intensity (black curves in Figures 1E–G, averaged precipitation in the monsoon regions) is roughly consistent with the proxies (green dots in Figures 1E–G), strengthening during the last deglaciation and weakening during the Holocene. The migration of northern Afro-Asian monsoon fringe indicated by geological records (Winkler and Wang, 1993; Yan and Petit-Maire, 1994; Adams, 1997; Jiang and Liu, 2007; Yang et al., 2015; Goldsmith et al., 2017) is also qualitatively reproduced by TraCE21, even though the varying magnitude in the simulation is smaller than that of the reconstructions (Figures 1B,C). The reasonable reproduction of TraCE21 for the variation of the Afro-Asian monsoon since the LGM provides the basis to test our hypothesis.
The monsoon domain is the region where the annual precipitation range (local summer minus winter) exceeds 300 mm (2 mm/day) and where summer precipitation exceeds 50% of the annual precipitation. Here, local summer and winter are defined as May through September (MJJAS) and November through next March (NDJFM), respectively, following Wang and Ding (2008). Arid regions are where the local summer precipitation rate is below 1 mm/day (Liu et al., 2012). Here, we focus on the monsoon fringe (the limit of monsoon domain) close to the arid region, whose migration was robust and the subsequent environmental impact was dramatic as illustrated bellow.
Results
Variation of Afro-Asian Monsoon Domain/Fringe
Since the LGM, Afro-Asian monsoon domains robustly co-varied in TraCE21. Along with the weakening of Afro-Asian monsoon during the Holocene (black curves in Figures 1E–G), the shrink of its domain (Figure 1D) was derived by the southward migration of northern monsoon fringe, with the amplitude of about 4 degrees in latitude (Figure 1B). Similar but reversed processes occurred during the last deglaciation (Figure 1C). Notably, referring to the state at the LGM, the varying magnitude of simulated Afro-Asian monsoon domain reached about 80% with the maximum at the HTM period (Figure 1D). In addition, the domain of Afro-Asian monsoon at present day (PD) is large by about 40% than that at the LGM (Figure 1D), expanded in the north, too (see Supplementary Figure 2). The multi-model mean results of PMIP3 indicated similar variation of the Afro-Asian monsoon domain (vertical bars in Figure 1D) and the migration of monsoon fringe (Supplementary Figure 3), with a slight difference in the varying magnitude. Therefore, the gradual variation of the monsoon domain, as indicated in the simulations, could induce the progressively meridional migration of the northern monsoon fringe, and this simulated fluctuation of northern monsoon fringe is consistent with that reconstructed by geological records (Figures 1B,C).
According to the spatial configuration of Afro-Asian monsoon and arid regions (Figure 1A), the southward migration of northern monsoon fringe during the Holocene induced the transition from monsoonal humid climate to arid climate, which was accompanied by the dramatic decline of local precipitation (shading in Figure 1B). A reversed process occurred during the last deglaciation with a larger varying magnitude, which was due to mean state difference between LGM and PD (shading in Figure 1C). Whether the fringe migrated during the last deglaciation or Holocene, the associated precipitation changes all occurred in a belt crossing North Africa to East Asia. Further analysis indicated that the precipitation variation in the fringe-migrationing region (blue curves in Figures 1E–G) is synchronous with that of the interior monsoon (black curves in Figures 1E–G). Climatological precipitation in fringe-migrationing regions was just about half of that in the interior monsoon regions; however, their varying magnitude was nearly twice of that in the interior monsoon regions (Figures 1E–G).
Along with the progressively meridional migration of the northern fringe since the LGM, the associated dramatic precipitation changes are time-transgressive (Figures 2A,B). The southward migration of northern fringe during the Holocene, whether in North Africa or East Asia, induced the decline of precipitation occurred on the north side of the fringe first and then occurred on the interior side later with a larger amplitude. Similar to the precipitation changes, simulated soil moisture indicated a clearer time-transgressive feature (Figures 2C–F), which are roughly consistent to the recorded paleo-hydrological evolution (gray and blue dots in Figure 2; An et al., 2000; Shanahan et al., 2015). Similar but reversed processes occurred during the last deglaciation.
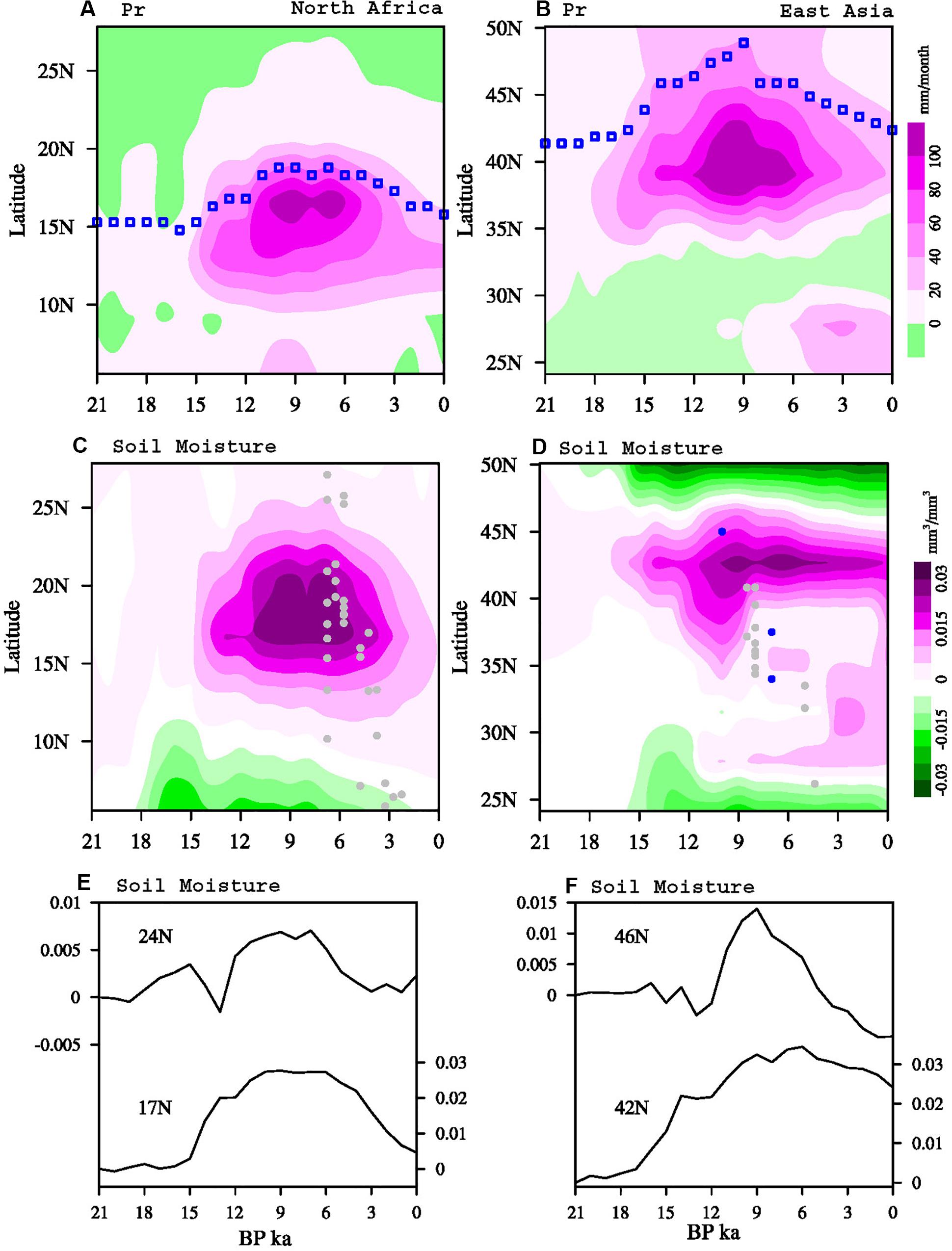
Figure 2. (A) Hovmöller diagram of precipitation anomalies (relative to LGM, units: mm/month) over North Africa (0°–30°E); (B) Same as (A), except for East Asia (110°–120°E). (C,D) Same as (A,B) except for soil moisture (units: mm3/mm3). (E,F) Typical soil moisture series in North Africa and East Asia. Northern monsoon fringe is shown using blue squares in (A,B). Gray and blue dots show ending-time of HTM of individual paleo-hydrological record (An et al., 2000; Shanahan et al., 2015) in (C,D).
Different from the previous explanations which focusing on the migration of monsoon rainbelt (An et al., 2000; Shanahan et al., 2015), here we clearly show that in the model simulation, the Holocene time-transgressive evolution of hydrology in the Afro-Asian monsoon region was caused by the southward migration of the northern monsoon fringe because of shrinking monsoon domain. Along with the migration of the northern monsoon fringe, intensive precipitation changes occurred locally for the reason of humid-arid transition. Furthermore, the model simulation indicated there was a reversed process during the last deglaciation, which is consistent with the finding of previous studies in records and simulations (COHMAP Members, 1988).
The model simulation also clarified the high sensitivity region of climate change associated with the monsoon, which was located around its northern fringe. Our results highlight the integrated changes of the Afro-Asian monsoon, which involved not only monsoon intensity but also the monsoon fringe. Neglecting the changes of monsoon fringe and just focusing on monsoon intensity, the time-transgressive feature and unequal magnitude of climate variation in a broad Afro-Asian monsoon region cannot be well understood.
Eco-Environmental Impact of Monsoon Fringe Migration
As shown above, the intensive precipitation changes, associated with the migration of the northern monsoon fringe, occurred over the humid-arid boundary region. However, in this semi-arid region, the spatial gradient of precipitation is usually large, which induces high sensitivity of local eco-environment to precipitation changes (Huang et al., 2017). Therefore, a dramatic eco-environmental response to the intensive precipitation change in this fringe-migrationing region is expected, and the simulation confirms it.
The intensive precipitation change along the northern fringe of the Afro-Asian monsoon, the dominant variation mode of precipitation over this area (Figure 3A), induced robust variations of local soil moisture (Figure 3B), and vegetation (Figure 3C). With the northward migration of northern monsoon fringe during the last deglaciation, the intensive increasing precipitation over the newly-formed monsoon region increased local soil moisture and then improved local vegetation. After that, a decreasing precipitation in the region monsoon retreated during the Holocene reduced the local moisture and destroyed the local vegetation intensively. The records of paleo-lakes along the northern fringe of Afro-Asian monsoon confirmed the robust change of local soil moisture simulated in TraCE21 (curves in Figure 3E). These lakes only existed during the period of the HTM (triangles in Figure 3E) and disappeared during the LGM and PD, indicating the dramatic variation of local hydrological condition and implying a reasonable simulation of local soil moisture variation in TraCE21. This dramatic hydrological variation around the northern fringe of Afro-Asian monsoon significantly controlled the abundance of local vegetation, grasses in low-latitude North Africa and trees in mid-latitude East Asia, as indicated by TraCE21 (Figure 3C).
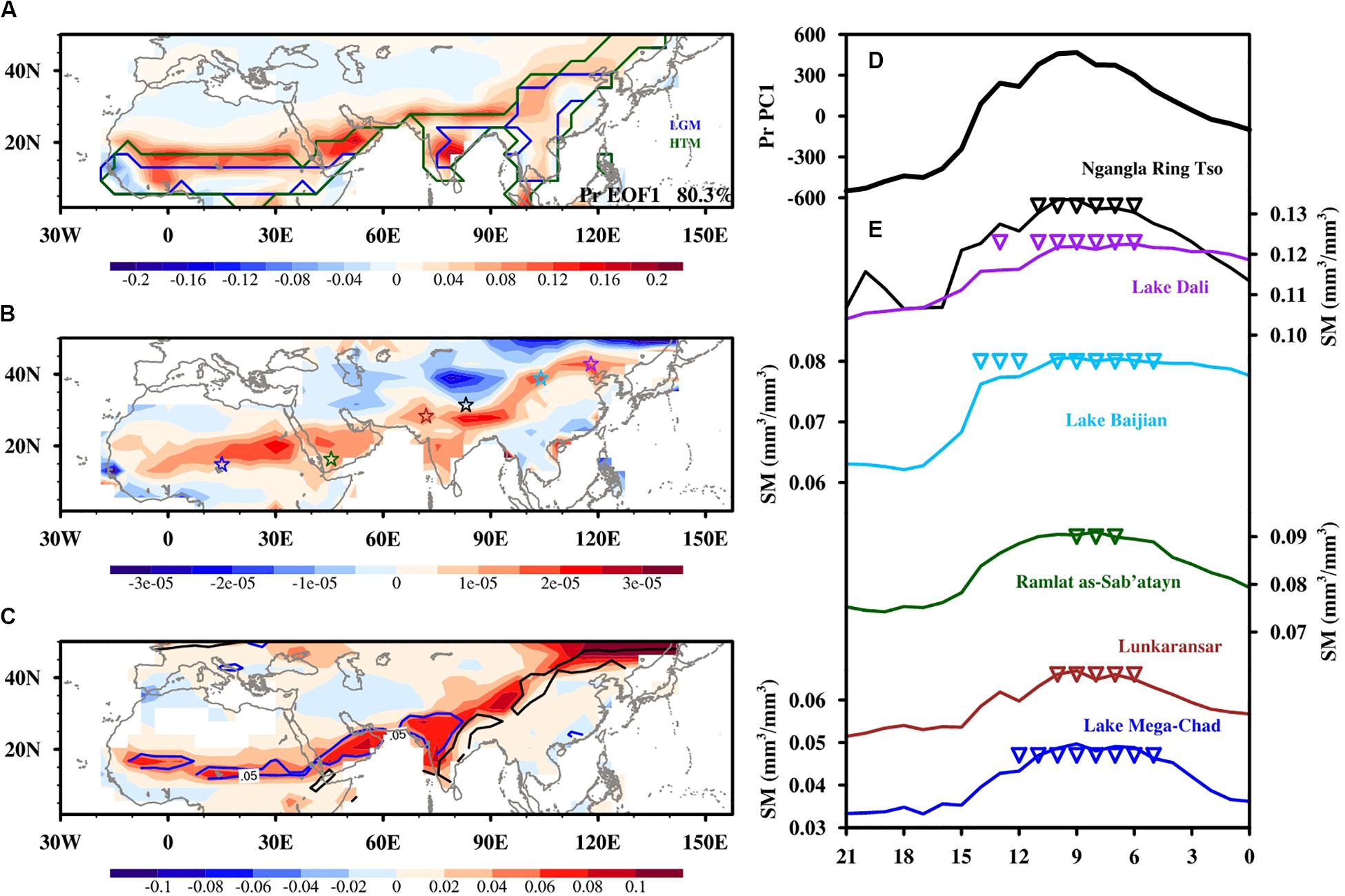
Figure 3. (A) Spatial pattern and (D) corresponding principal component (PC1) of the first empirical orthogonal function (EOF) mode of Afro-Asian JJA precipitation in TraCE21; (B) Regression pattern of soil moisture on precipitation PC1 (units: mm3/mm3/mm/month). Stars indicate locations of Lake phase records (i.e., Ngangla Ring Tso, Hudson et al., 2015; Lake Dali, Goldsmith et al., 2017; Lake Baijian, Long et al., 2012; Ramlat as-Sab’atayn, Lézine et al., 1998; Lunkaransar, Enzel et al., 1999; and Lake Mega-Chad, Armitage et al., 2015); (C) Same as (B), except for total vegetation percentage (units:%/mm/month). Blue curve shows the regression coefficients of C3 grass; and black curve, total tree; (E) Recorded lake phase (triangles) and simulated soil moisture (curves) adjacent to these paleo-lakes. Curves in (A) show simulated Afro-Asian monsoon domains at LGM (blue) ad HTM (green).
Combined the geological records and model simulation, although the simulation is not validated for every process by limited records, we propose the recorded dramatic eco-environmental variation along northern fringe of Afro-Asian monsoon was caused by the migration of the northern monsoon fringe through the associated intensive variation of precipitation since the LGM. Owning to the intensive migration of monsoon fringe since the LGM, the sensitivities of both climate and eco-environment in the abroad region along northern fringe of Afro-Asian monsoon are all high, which provided the insight for the projection of future changes of climate and eco-environment under ongoing global warming.
Cause for Varying Monsoon Domain
The monsoon is a complex climate system. The monsoon circulation is an extension of the cross equatorial Hadley Cell (Bordoni and Schneider, 2008; Merlis et al., 2013; Schneider et al., 2014) in the monsoon season, it is shaped in further by the local large-scale land-ocean thermal contrast (Webster et al., 1998). The moisture transported by the monsoon circulation converges over the monsoon domain, which induces the formation of monsoon precipitation. Thus, the past recorded and the future projected changes of monsoon (Biasutti, 2013; Boos and Korty, 2016; Bischoff et al., 2017; Seth et al., 2019), involving that in the circulation, the precipitation and the domain, could be dominated by the land-ocean thermal contrast (Chou et al., 2001; Bordoni and Schneider, 2008), the meridional temperature gradient (Wang et al., 2001; D’Agostino et al., 2019; Seth et al., 2019), the large scale circulation (Bordoni and Schneider, 2008), the local moist static energy (Neelin and Held, 1987), and the net energy input (Texier et al., 2000; Schneider et al., 2014; Boos and Korty, 2016; D’Agostino et al., 2019). Among all these potential mechanisms, our transient simulations indicate that the changes of land-ocean thermal contrast coincided with the changes of the Afro-Asian monsoon under long-term climate changes since the LGM, which were mainly forced by orbital and CO2 forcing (Cheng et al., 2019).
The solo-forcing experiments of TraCE21 of orbital and CO2 clearly indicate the dominance of land-ocean thermal contrast on the Afro-Asian monsoon domain (Figure 4). Precession-dominated orbital forcing modulates the deglacial expansion and the Holocene shrink of the Afro-Asian monsoon domain through the in-phase change of land-ocean thermal contrast. The increasing CO2 concentration, especially for the period of the last deglaciation, also could expand the domain of the Afro-Asian monsoon through enlarged land-ocean thermal contrast, which basically established the climatological difference between LGM and PD. Similar to the full-forcing experiment of TraCE21 (Figures 1B,C), the migration of the northern monsoon fringe is all dominant for the change of monsoon domain, and could induce the intensive environment impact in the belt shown above (see Supplementary Figure 4). The sum of these two solo-forcing experiments (gray curve in Figures 4B,C) is roughly equal to the full-forcing experiment of TraCE21 (black curve in Figures 4B,C), which illustrates the dominance of these two external forcing during the long-term change of the Afro-Asian monsoon since the LGM. Comparing to the forcing of CO2, orbital forcing is the dominated factor in the simulated migration of monsoon fringe.
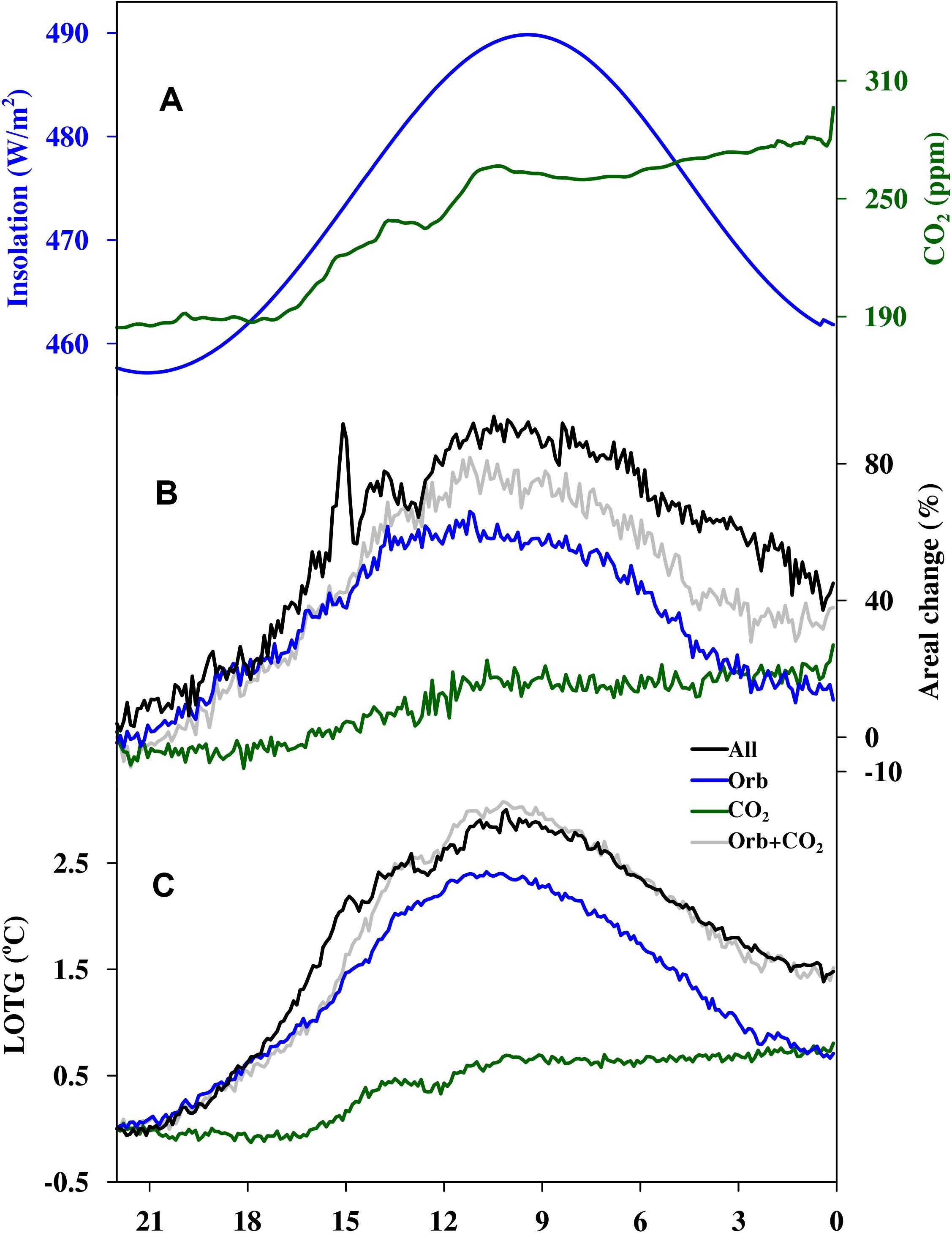
Figure 4. (A) Evolutions of summer-averaged insolation (30°N; blue) and CO2 concentration (green) since LGM; (B) Areal changes (relative to LGM) of Afro-Asian monsoon domain under all-forcing (black curves), orbital forcing (blue), CO2 forcing (green), and the sum of orbital and CO2 forcing (gray); (C) Summer land-ocean thermal contrast (SAT averaged over 0°–60°N, 30°W–180°; land minus ocean) variations under different forcing experiments.
Conclusion and Discussion
Combining model simulation with geological records, we proposed the recorded time-transgressive hydrological evolution around the northern fringe of the Afro-Asian monsoon and the recorded dramatic eco-environmental variation in the same region were caused by the gradual migration of the northern monsoon fringe since the LGM. The migration of the northern monsoon fringe could induce intensive variation of precipitation locally due to the humid-arid transition. Our results clarified the sensitive response of climate and eco-environment of monsoon located around its northern fringe, not the interior. We also propose the LGM-PD difference of climate and eco-environment was mainly attributed to the increasing CO2 concentration, but their intensive long-term fluctuation was caused by orbital forcing through the impact on the land-ocean thermal contrast.
Our analyses clearly illustrated, besides monsoon intensity, we should pay more attention to the accompanying change of monsoon domain (migration of monsoon fringe) under long-term climate change. The clarification about the subsequence of monsoon fringe migration, as illustrated here, is important to understand the diverse evolution of individual geological record in the regions of the Afro-Asian monsoon, which was usually asynchronous in time and unequal in amplitude. The insight about the monsoon fringe, which was derived from its past changes, is important for the projection of future changes of monsoon-related climate and eco-environment under global warming.
Coherent long-term evolution of the Afro-Asian monsoon, as indicated by geological records and model simulation, illustrated the integrity of the Afro-Asian monsoon again, which is consistent with the analyses using the observations. For the long-term variation of the Afro-Asian monsoon, our analyses highlighted the importance of large-scale land-ocean thermal contrast (Webster et al., 1998). Comparing to the meridional scales of North Africa monsoon, it’s large for the East Asia monsoon, which leads to an opposite rainfall changes over the East Asia during the Holocene era as indicated in geological records (An et al., 2000; Xu et al., 2020) and model simulation (Liu et al., 2014). This asynchronous rainfall changes over interior regions of East Asia monsoon was caused by the migration of monsoon rainbelt, which is clearly different to the asynchronous rainfall changes around norther monsoon fringe caused by the migration of monsoon fringe.
Another point should be noticed is that the amplitude of northward migration of the Afro-Asian monsoon fringe and associated environment impact may be small under future warming, even the warming amplitude may be larger than that in the past. As illustrated in Cheng et al. (2019), the varying amplitude of land-ocean thermal contrast relies on the external forcing of temperature change, high under the orbital forcing and small under CO2 forcing. Comparing to the past changes of Afro-Asian monsoon fringe which dominated by orbital forcing, its future changes dominated by CO2 will be relative small.
Data Availability Statement
Publicly available datasets were analyzed in this study. This data can be found here: https://www.earthsystemgrid.org/dataset/ucar.cgd.ccsm3.trace.html.
Author Contributions
JC: conceptualization and writing—original draft preparation. JC and YM: methodology and investigation. YM: formal analysis. HW, HL, and ZL: resources. HW and ZL: writing—review and editing. All authors have read and agreed to the published version of the manuscript.
Funding
This research was supported by the Program of Global Change and Mitigation, Ministry of Science and Technology of the People’s Republic of China (2016YFA0600504), and by the National Natural Science Foundation of China (41776017, 41630527, and 41977381).
Conflict of Interest
The authors declare that the research was conducted in the absence of any commercial or financial relationships that could be construed as a potential conflict of interest.
Supplementary Material
The Supplementary Material for this article can be found online at: https://www.frontiersin.org/articles/10.3389/feart.2020.00322/full#supplementary-material
References
Adams, J. M. (1997). Global Land Environments Since the Last Interglacial. Oak Ridge, TN: Oak Ridge National Laboratory.
An, Z. S., Porter, S. C., Kutzbach, J. E., Xihao, W., Suming, W., and Xiaodong, L. (2000). Asynchronous Holocene optimum of the East Asian monsoon. Quatern. Sci. Rev. 19, 743–762. doi: 10.1016/s0277-3791(99)00031-1
Armitage, S. J., Bristow, C. S., and Drake, N. A. (2015). West African monsoon dynamics inferred from abrupt fluctuations of Lake Mega-Chad. Proc. Natl. Acad. Sci. U.S.A. 112, 8543–8548. doi: 10.1073/pnas.1417655112
Berger, A. (1978). Long-term variations of daily insolation and quaternary climatic changes. J. Atmos. Sci. 35, 2362–2367. doi: 10.1175/1520-0469(1978)035<2362:ltvodi>2.0.co;2
Biasutti, M. (2013). Forced Sahel rainfall trends in the CMIP5 archive. J. Geophys. Res. Atmos. 118, 1613–1623. doi: 10.1002/jgrd.50206
Bischoff, T., Schneider, T., and Meckler, A. N. (2017). A conceptual model for the response of tropical rainfall to orbital variations. J. Clim. 30, 8375–8391. doi: 10.1175/jcli-d-16-0691.1
Boos, W. R., and Korty, R. L. (2016). Regional energy budget control of the intertropical convergence zone and application to mid-Holocene rainfall. Nat. Geosci. 9, 892–897. doi: 10.1038/ngeo2833
Bordoni, S., and Schneider, T. (2008). Monsoons as eddy-mediated regime transitions of the tropical overturning circulation. Nat. Geosci. 1, 515–519. doi: 10.1038/ngeo248
Broccoli, A. J., and Manabe, S. (1992). The effects of orography on Midlatitude Northern Hemisphere dry climates. J. Climate 5, 1181–1201. doi: 10.1175/1520-0442(1992)005<1181:teooom>2.0.co;2
Cheng, J., Ma, W., Liu, Z., and Wu, H. (2019). Varying sensitivity of East Asia summer monsoon circulation to temperature change since Last Glacial Maximum. Geophys. Res. Lett. 46, 9103–9109. doi: 10.1029/2019GL083405
Chou, C., Neelin, J., and Su, H. (2001). Ocean-atmosphere-land feedbacks in an idealized monsoon. Q. J. Roy. Meteor. Soc. 127, 1869–1891. doi: 10.1002/qj.49712757602
Collins, W. D., Bitz, C. M., Blackmon, M. L., Bonan, G. B., Bretherton, C. S., Carton, J. A., et al. (2006). The community climate system model version 3 (CCSM3). J. Clim. 19, 2122–2143. doi: 10.1175/jcli3761.1
D’Agostino, R., Bader, J., Bordoni, S., Ferreira, D., and Jungclaus, J. (2019). Northern Hemisphere monsoon response to mid-holocene orbital forcing and greenhouse gas-induced global warming. Geophys. Res. Lett. 46, 1591–1601. doi: 10.1029/2018gl081589
Doherty, R., Kutzbach, J., Foley, J., and Pollard, D. (2000). Fully coupled climate/dynamical vegetation model simulations over North Africa during the mid-Holocene. Clim. Dyn. 16, 561–573. doi: 10.1007/s003820000065
Dykoski, C. A., Edwards, R. L., Cheng, H., Yuan, D., Cai, Y., Zhang, M., et al. (2005). A highresolution, absolute-dated Holocene and deglacial Asian monsoon record from Dongge Cave, China. Earth Planet. Sci. Lett. 233, 71–86. doi: 10.1016/j.epsl.2005.01.036
Enzel, Y., Ely, L. L., Mishra, S., Ramesh, R., Amit, R., Lazar, B., et al. (1999). High-resolution Holocene environmental changes in the Thar Desert, northwestern India. Science 284, 125–128. doi: 10.1126/science.284.5411.125
Fleitmann, D., Burns, S. J., Mudelsee, M., Neff, U., Kramers, J., Mangini, A., et al. (2003). Holocene forcing of the Indian monsoon recorded in a stalagmite from southern Oman. Science 300, 1737–1739. doi: 10.1126/science.1083130
Goldsmith, Y., Broeckera, W. S., Xu, H., Polissara, P. J., deMenocala, P. B., Poratc, N., et al. (2017). Northward extent of East Asian monsoon covaries with intensity on orbital and millennial timescales. Proc. Natl. Acad. Sci. U.S.A. 114, 1817–1821. doi: 10.1073/pnas.1616708114
Haug, G. H., Hughen, K. A., Sigman, D. M., Peterson, L. C., and Röhl, U. (2001). Southward migration of the intertropical convergence zone through the Holocene. Science 293, 1304–1308. doi: 10.1126/science.1059725
He, F., Shakun, J. D., Clark, P. U., Carlson, A. E., Liu, Z., Otto-Bliesner, B. L., et al. (2013). Northern hemisphere forcing of southern hemisphere climate during the last deglaciation. Nature 494, 81–85. doi: 10.1038/nature11822
Huang, J., Li, Y., Fu, C., Chen, F., Fu, Q., Dai, A., et al. (2017). Dryland climate change: recent progress and challenges. Rev. Geophys. 55, 719–778. doi: 10.1002/2016rg000550
Hudson, A. M., Quade, J., Huth, T. E., Lei, G. L., Cheng, H., Edwards, L. R., et al. (2015). Lake level reconstruction for 12.8–2.3ka of the Ngangla Ring Tso closed-basin lake system, southwest Tibetan Plateau. Quatern. Res. 83, 66–79. doi: 10.1016/j.yqres.2014.07.012
Jiang, D. B., Tian, Z. P., and Lang, X. M. (2015a). Mid-Holocene global monsoon area and precipitation from PMIP simulations. Clim. Dyn. 44, 2493–2512. doi: 10.1007/s00382-014-2175-8
Jiang, D. B., Tian, Z. P., Lang, X. M., Kageyama, M., and Ramstein, G. (2015b). The concept of global monsoon applied to the last glacial maximum: a multi-model analysis. Quat. Sci. Rev. 126, 126–139. doi: 10.1016/j.quascirev.2015.08.033
Jiang, W., and Liu, T. (2007). Timing and spatial distribution of mid-Holocene drying over northern China: response to a southeastward retreat of the East Asian Monsoon. J. Geophys. Res. Atmos. 112, 1984–2012.
Joos, F., and Spahni, R. (2008). Rates of change in natural and anthropogenic radiative forcing over the past 20,000 years. Proc. Natl. Acad. Sci. U.S.A. 105, 1425–1430. doi: 10.1073/pnas.0707386105
Kathayat, G., Cheng, H., Sinha, A., Spötl, C., Edwards, R. L., Zhang, H., et al. (2016). Indian monsoon variability on millennial-orbital timescales. Sci. Rep. 6:24374. doi: 10.1038/srep24374
Lézine, A. M., Saliege, J. F., Robert, C., Wertz, F., and Inizan, M. L. (1998). Holocene lakes from Ramlat as-Sab’atayn (Yemen) illustrate the impact of monsoon activity in Southern Arabia. Quatern. Res. 50, 290–299. doi: 10.1006/qres.1998.1996
Liu, J., Wang, B., Yim, S. Y., Lee, J., Jhun, J., and Ha, K. (2012). What drives the global summer monsoon over the past millennium? Clim. Dyn. 39, 1063–1072. doi: 10.1007/s00382-012-1360-x
Liu, Z., Otto-Bliesner, B. L., He, F., Brady, E. C., Tomas, R., Clark, P. U., et al. (2009). Transient simulation of Last Deglaciation with a new mechanism for Bolling-Allerod warming. Science 325, 310–314. doi: 10.1126/science.1171041
Liu, Z., Wen, X., Brady, E. C., Otto-Bliesner, B., Yu, G., Lu, H., et al. (2014). Chinese cave records and the East Asia Summer Monsoon. Quat. Sci. Rev. 83, 115–128. doi: 10.1016/j.quascirev.2013.10.021
Long, H., Lai, Z. P., Fuchs, M., Zhang, J. R., and Li, Y. (2012). Timing of Late Quaternary palaeolake evolution in Tengger Desert of northern China and its possible forcing mechanisms. Glob. Planet. Change 9, 119–129. doi: 10.1016/j.gloplacha.2012.05.014
McManus, J. F., Francois, R., Gherardi, J. M., Keigwin, L. D., and Brown-Leger, S. (2004). Collapse and rapid resumption of Atlantic meridional circulation linked to deglacial climate changes. Nature 428, 834–837. doi: 10.1038/nature02494
COHMAP Members (1988). Climatic changes of the last 18,000 years: observations and model simulations. Science 241, 1043–1052. doi: 10.1126/science.241.4869.1043
Merlis, T., Schneider, T., Bordoni, S., and Eisenman, I. (2013). Hadley circulation response to orbital precession. Part I: aquaplanets. J. Clim. 26, 754–771. doi: 10.1175/jcli-d-12-00149.1
Neelin, J., and Held, I. (1987). Modeling tropical convergence based on the moist static energy budget. Mon. Weather Rev. 115, 3–12. doi: 10.1175/1520-0493(1987)115<0003:mtcbot>2.0.co;2
Otto-Bliesner, B. L., Russell, J. M., Clark, P. U., Liu, Z., Overpeck, J. T., Konecky, B., et al. (2014). Coherent changes of southeastern equatorial and northern African precipitation during the last deglaciation. Science 346, 1223–1227. doi: 10.1126/science.1259531
Peltier, W. (2004). Global glacial isostasy and the surface of the ice-age earth: the ICE-5G (VM2) model and GRACE. Annu. Rev. Earth Plant. Sci. 20, 111–149. doi: 10.1146/annurev.earth.32.082503.144359
Schneider, T., Bischoff, T., and Haug, G. H. (2014). Migrations and dynamics of the inter-tropical convergence zone. Nature 513, 45–53. doi: 10.1038/nature13636
Seth, A., Giannini, A., Rojas, M., Rauscher, S. A., Bordoni, S., Singh, D., et al. (2019). Monsoon responses to climate changes—connecting past, present and future. Curr. Clim. Change Rep. 5, 63–79. doi: 10.1007/s40641-019-00125-y
Sha, L., Ait Brahim, Y., Wassenburg, J. A., Yin, J., Peros, M., Cruz, F. W., et al. (2019). How far north did the African Monsoon fringe expand during the African Humid Period? Insights from Southwest Moroccan speleothems. Geophys. Res. Lett. 46, 14093–14102. doi: 10.1029/2019GL084879
Shanahan, T., McKay, N., Hughen, K., Overpeck, J., Otto-Bliesner, B., Heil, C., et al. (2015). The time-transgressive termination of the African Humid Period. Nat. Geosci. 8, 140–144. doi: 10.1038/ngeo2329
Shi, J., and Yan, Q. (2019). Evolution of the Asian–African monsoonal precipitation over the last 21 kyr and the associated dynamic mechanisms. J. Clim. 32, 6551–6569. doi: 10.1175/jcli-d-19-0074.1
Skonieczny, C., Paillou, P., Bory, A., Bayon, G., Biscara, L., Crosta, X., et al. (2015). African humid periods triggered the reactivation of a large river system in Western Sahara. Nat. Commun. 6:8751. doi: 10.1038/ncomms9751
Street, F. A., and Grove, A. T. (1976). Environmental and climatic implications of late quaternary lake-level fluctuations in Africa. Nature 261, 385–390. doi: 10.1038/261385a0
Texier, D., de Noblet, N., and Braconnot, P. (2000). Sensitivity of the African and Asian monsoons to mid-holocene insolation and data-inferred surface changes. J. Climate 13, 164–181. doi: 10.1175/1520-0442(2000)013<0164:sotaaa>2.0.co;2
Tierney, J. E., Pausata, F. S. R., and deMenocal, P. B. (2017). Precipitation regimes of the green Sahara. Sci. Adv. 3:e1601503. doi: 10.1126/sciadv.1601503
Wang, B., and Ding, Q. (2008). Global monsoon: dominant mode of annual variation in the tropics. Dyn. Atmos. Oceans 44, 165–183. doi: 10.1016/j.dynatmoce.2007.05.002
Wang, B., Wu, R. G., and Lau, K. M. (2001). Interannual variability of the Asian summer monsoon: contrasts between the India and the western north pacific-east Asian monsoons. J. Clim. 14, 4073–4090. doi: 10.1175/1520-0442(2001)014<4073:ivotas>2.0.co;2
Wang, Y., Cheng, H., Edwards, R. L., Kong, X., Shao, X., Chen, S., et al. (2008). Millennial-and orbital scale changes in the East Asian monsoon over the past 224 000 years. Nature 7182, 1090–1093. doi: 10.1038/nature06692
Webster, P. J., Magana, V., Palmer, T., Shukla, J., Tomas, R., Yanai, M., et al. (1998). Monsoons: processes, predictability and the prospects for prediction. J. Geophys. Res. 1031, 14451–14510. doi: 10.1029/97jc02719
Weldeab, S., Lea, D. W., Schneider, R. R., and Andersen, N. (2007). 155000 years of West African monsoon and ocean thermal evolution. Science 316, 1303–1307. doi: 10.1126/science.1140461
Wen, X., Liu, Z., Wang, S., Cheng, J., and Zhu, J. (2016). Correlation and anti-correlation of the East Asian summer and winter monsoon during the last 21,000 years. Nat. Commun. 7:11999.
Winkler, M. G., and Wang, P. K. (1993). The Late-Quaternary Vegetation and Climate of China, Global Climates Since the Last Glacial Maximum. Minneapolis: University of Minnesota Press, 221–264
Xu, H., Goldsmith, Y., Lan, J., Tan, L., Wang, X., Zhou, X., et al. (2020). Juxtaposition of western Pacific subtropical high on Asian Summer Monsoon shapes subtropical East Asian precipitation. Geophys. Res. Lett. 47:e2019GL084705.
Yan, Z., and Petit-Maire, N. (1994). The last 140 ka in the Afro-Asian arid/semi-arid transitional zone. Palaeogeogr. Palaeocl. 110, 217–233. doi: 10.1016/0031-0182(94)90085-x
Yang, S., Ding, Z., Li, Y., Wang, X., Jiang, W., and Huang, X. (2015). Warming-induced northwestward migration of the East Asian monsoon rain belt from the Last Glacial Maximum to the mid-Holocene. Proc. Natl. Acad. Sci. U.S.A. 112, 13178–13183. doi: 10.1073/pnas.1504688112
Yu, G., and Harrison, S. P. (1996). An evaluation of the simulated water balance of Eurasia and northern Africa at 6000 yr BP using lake status data. Clim. Dyn. 12, 723–735. doi: 10.1007/s003820050139
Keywords: Afro-Asian monsoon, fringe, hydrological evolution, land-ocean thermal contrast, external forcing, vegetation
Citation: Cheng J, Ma Y, Wu H, Long H and Liu Z (2020) Migration of Afro-Asian Monsoon Fringe Since Last Glacial Maximum. Front. Earth Sci. 8:322. doi: 10.3389/feart.2020.00322
Received: 01 June 2020; Accepted: 10 July 2020;
Published: 07 August 2020.
Edited by:
Hai Xu, Tianjin University, ChinaReviewed by:
Zhongshi Zhang, China University of Geosciences, ChinaZhengguo Shi, Institute of Earth Environment (CAS), China
Copyright © 2020 Cheng, Ma, Wu, Long and Liu. This is an open-access article distributed under the terms of the Creative Commons Attribution License (CC BY). The use, distribution or reproduction in other forums is permitted, provided the original author(s) and the copyright owner(s) are credited and that the original publication in this journal is cited, in accordance with accepted academic practice. No use, distribution or reproduction is permitted which does not comply with these terms.
*Correspondence: Jun Cheng, chengjun@nuist.edu.cn