- College of Earth Sciences & Key Laboratory of Mineral Resources in Western China (Gansu Province), Lanzhou University, Lanzhou, China
A 794-cm section was collected from Tengger Nuur in the Inner Mongolian Plateau. Accelerator mass spectrometry 14C data were determined to set an age-depth model after removing about 1920 years of the carbon reservoir effect. Based on the multi-proxies grain size, carbonate-content, total organic carbon-content, ratio of C/N, ratios of Mg/Ca and Sr/Ca, and carbonate carbon and oxygen isotopes, paleoenvironmental changes since the last deglaciation were reconstructed. Tengger Nuur was very shallow during the last deglaciation under a cool and wet climate, especially during the interval of the cold Younger Dryas event. Although, temperature and humidity increased from the early Holocene (∼10,450–8750 cal a BP), low lake levels indicated that the summer monsoon was not sufficiently strong to reach the modern monsoon boundary in Inner Mongolia. High monsoon precipitation caused lake expansion during 8750–5000 cal a BP, but the lake level oscillated in a shallow state under high evaporation. A low lake-level event occurred with the interval of a cold-wet climate during 5450–5100 cal a BP. The summer monsoon receded gradually to maintain a deep lake under high effective humidity during 5000–2000 cal a BP, punctuated by low lake-level events at intervals of 4300–3980 cal a BP and 3700–2750 cal a BP. With the arrival of the cold and dry westerly after 2000 cal a BP, lakes shrank gradually to become salinized or completely desiccated, but their levels oscillated at shallow depths during the four periods of 1900–1800 cal a BP, 1500–1050 cal a BP, 550–400 cal a BP (Little Ice Age), and 100 cal a BP–AD 1985. Therefore, the eastern summer monsoon was weak in the early Holocene, and lake-level oscillation was controlled by effective humidity in arid and semi-arid areas.
Introduction
The region of mid and eastern Mongolia, which lies at the boundary of the modern summer monsoon, is controlled by the winter, summer monsoon, and westerlies. The interplay between the eastern monsoon and westerlies has been significantly affecting the ecological environment of this region since the last deglaciation. Therefore, reconstructions of the paleoenvironmental change have high significance in understanding the evolution and mechanism of the eastern monsoon. The fragile ecological system at the monsoon boundary is sensitive to the monsoon, and thus a large number of lake-filling events triggered by the summer monsoon precipitation record the paleoenvironmental change. Such records based on lake and terrestrial sediments help in understanding the interplay between the climate and environment, and monsoon mechanisms since the last glaciation (Wang, 1992; Chen et al., 2003, 2010; Xiao et al., 2004), and the development of the ecological environment in arid and semi-arid regions in the future.
In the past several decades, several lakes at the modern eastern monsoon boundary of Inner Mongolia, i.e., Daihai Lake (Li et al., 1992; Xiao et al., 2004; Zheng et al., 2010; Zeng et al., 2013), Wulagai Lake (Yu et al., 2014), Baahar Nuur Lake (Guo et al., 2007), Dali Nor Lake (Wang et al., 2004), Haolaihure Paleolake (Liu et al., 2018), Diaojiao Lake (Song et al., 1996; Yang et al., 1997; Yang, 1998), Huangqihai (Li et al., 1992), Hulun Lake (Yang and Wang, 1996; Zhang and Wang, 2000; Wen et al., 2010), Dabusu Lake (Jie et al., 2001), and Angulinao (Zhai et al., 2000), had been used to reconstruct paleoenvironmental changes based on pollen and geochemical proxies. However, although the paleoenvironment records provided much information about environmental changes since last deglaciation, a few these studies did remove the carbon reservoir effect in the age-depth model established for lakes, for example, Xiao et al. (2004); Guo et al. (2007), Wen et al. (2010); Liu et al. (2018), and some of them did not calibrate the tree-ring calendar year. Nevertheless, they provided deep insight into the interplay eastern monsoon and westerlies in this region.
Tengger Nuur, which lies at the modern eastern Asian monsoon boundary in Inner Mongolia, is impacted both by the Asian summer and winter monsoons and westerlies (Figure 1). Studying lake-level fluctuations in Tengger Nuur would provide records of the Asian monsoon and its variability and linkage with the global climate change in the Holocene.
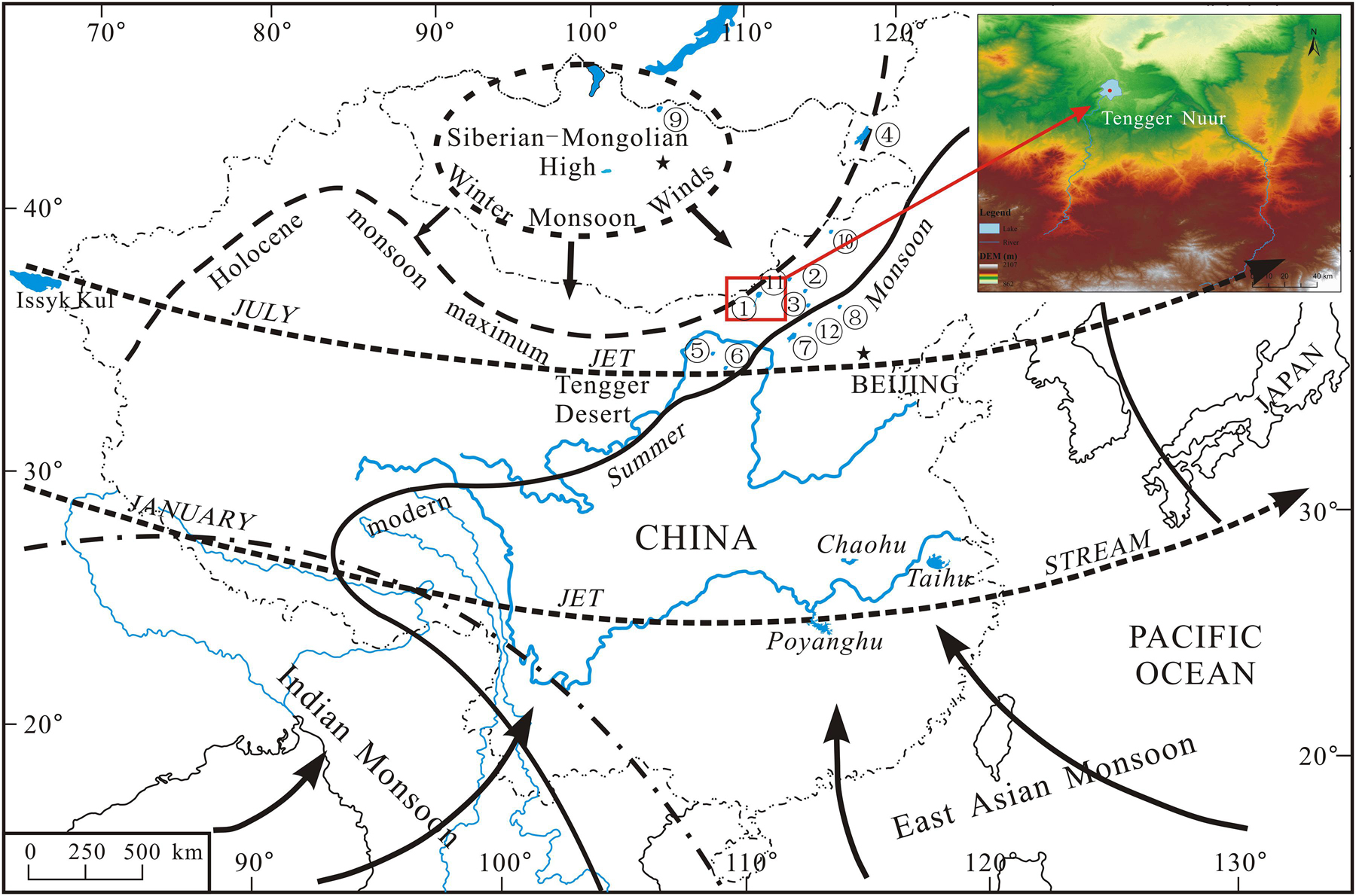
Figure 1. Map of Asian monsoon region showing locations of paleoclimate records used in this study. ➀ Tengger Nuur, ➁ Daojiaohaizi, ➂ Dali Lake, ➃ Hulun Lake, ➄ Yanhaizi, ➅ Baahar Nuur, ➆ Daihai Lake, ➇ Bayanchagan Lake, ➈ Gun Nuur, ➉ Haolaihure, ⑪ Angulinao, ⑫ Huangqihai. Lines indicate modern extent of East Asian and Indian monsoons (dashed lines) and maximum area extent of monsoons during the Holocene (solid line), as mapped by Winkler and Wang (1993).
Geological Setting, Sampling and Lithological Stratigraphy
Tengger Nuur, which means “sky lake” in Mongolian (Nuur means lake or swag) (110°38′–110°42′E, 42°24′–42°28′N, 1077.60 m asl), is located in northern Darhan Muminggan United Banner of Ulanqab League. Aeolian deposition is widely distributed around the lake shoreline. Tengger Nuur, a tectonic fault depression terminal lake, is 8.4 km long and 6.2 km wide, with a mean width of 3.4 km and an area of approximately 28.6 km2. The maximum catchment area has been reported to be about 53 km2 (Wang and Dou, 1998).
The lake area belongs to the middle temperate zone, which falls under a continental arid climate, and experiences a mean annual temperature of 3.4°C. The mean temperature in January is −15.9°C when the extreme minimum temperature can be as low as −41.0°C. In July, the mean temperature is 20.5°C, and the extreme maximum temperature could reach up to 36.6°C. The mean annual sunshine time reaches 2986 h, and the frost-free period is about 106 days. The annual precipitation ranges from 142.6 to 400.3 mm, which mean is about 236.6 mm, chiefly occurring between July and August. The evaporation is about 2360 mm. The lake depends on the supply of rainfall and surface runoff. Currently, seasonal Aibugai River is the only one flowing into the lake. The river is 154 km long, feeding the basin from Daqingshan in the south.
From 1979 onward, the water level of Tengger Nuur has been dropping gradually as the volume of runoff of Aibugai River decreased and wetlands shrunk to become almost completely desiccated in 1985, as historical records suggest. However, the lake began to rise again to reach the recorded highest level in 2018, and the water volume increased to 1 × 108 m3.
A sediment stratum of 794 cm length (TGN-05, 42°27′7.7″N, 110°42′3.6″E, 1052 m asl), including 398 cm digging section and 396 cm core drilled with a UWITEC piston corer “Niederreiter 60”, was obtained from Lake Tengger Nuur in 2005. The groundwater depth is about 398 cm. About 397 samples were collected at intervals of 2 cm. However, sediments in the interval of 346–374 cm were missed in the sampling.
The lithological strata of TGN-05 are as follows: a brown mud layer at 0–389 cm (in this paper, mud indicates sediment grain sizes less than 4 μm); a gray-black mud layer at 389–398 cm; a dark gray mud layer at 398–401 cm; a green-gray silt layer at 401–448 cm; a layer of well-sorted coarse sand with highly rounded fine gravel at 401–448 cm; a dark gray muddy silt layer at 469–521 cm; a blown gray coarse sandy mud layer at 521–540 cm; a layer of well-sorted and highly rounded bluish-gray coarse muddy sand at 540–602 cm; a bluish-gray mud layer 602–609 cm; a layer of highly rounded coarse sandy fine gravel at 609–620 cm; a muddy silt layer at 620–685 cm; a layer of alternating bluish-gray coarse sand and poorly sorted, highly rounded fine gravel at 685–757 cm; a brown silty mud layer at 757–794 cm.
Age Data and Age-Depth Model
A total of 11 samples of bulk organic matter were used for radiocarbon dating of TGN-05 in 14C radioactivity isotope labs, BETA in United States and Beijing University in China. However, the total organic carbon (TOC) contents of the last three samples, TGN-05-828 (828–830 cm), TGN-05-704 (704–706 cm), and TGN-05-792 (792–794 cm), were too low for analysis (Table 1).
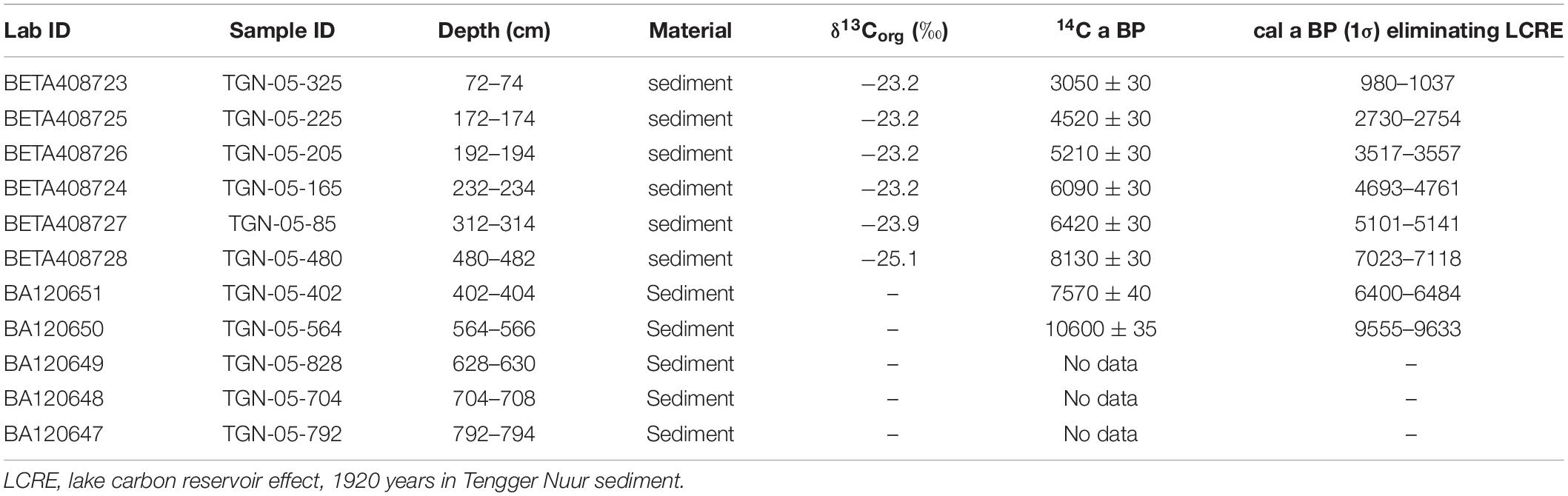
Table 1. Radiocarbon dating results for section TGN-05 determined in the BETA 14C Lab (BETA) and Beijing University 14C Lab (BA).
Recently, several studies found that the lake carbon reservoir effect (LCRE, also “dead carbon” or “old carbon” or “hard water” effect) can result in anomalously old 14C ages of lacustrine sediments. Therefore, LCRE correction should be applied before the construction of an age-to-depth model. Lake carbon reservoir effect has a great spatial and temporal change. For example, that is very small in the Qingtu Lake (Long et al., 2011). Five hundred and seventy year of the age of Lake Bayanchagan in Inner Mongolia could be attributed to LCRE (Jiang et al., 2006), 685 year in Hulun Lake (Wen et al., 2010), 2570 year in Haolaihure Paleolake (Liu et al., 2018), that of Baahar Nuur has been reported to be around 1935 year (Guo et al., 2007), the ca. 360 year can be considered to result from the hard water effect on the radiocarbon dating of Daihai Lake sediments as extrapolated linearly to the core depth of 0 m (Xiao et al., 2004). Chen et al. (2003) regarded that LCRE of Lake Yanhaizi in the Mu Us Desert to be about 879 year according to comprehensively analyzed 14C age of surface and lacustrine core sediments, and pollens. The hard water effect was estimated at 1000–2000 year for lakes in Inner Mongolia (Ren, 1998). Hou et al. (2012) discussed the spatial and temporal pattern of LCREs in Tibetan lakes, and found that they mostly range from 1000 to 3000 14C years.
Lake carbon reservoir effect is generally identified by the difference of macrophyte remains and TOC in bulk lake sediments. However, a large number of analysis results show that macrophyte remains in lacustrine sediments could also be affected by the hard water effect. For instance, Li et al. (2017) used surface macrophyte remains and determined the LCRE of Lake Koucha as 1812 ± 22 year. Therefore, the 14C dating value of organic matter from lacustrine surface sediments could be roughly recognized as the value of LCRE (Chen et al., 2003; Xiao et al., 2004; Jiang et al., 2006; Guo et al., 2007; Li et al., 2017). In addition, 210Pb-137Cs of lacustrine sediments could be occasionally used to calibrate the 14C ages of surface sediments (Zhang et al., 2004). Otherwise, when 14C ages of lacustrine horizons of sediments cannot be measured directly, LCRE data can be obtained through linear extrapolation to the top (Chen et al., 2003; Xiao et al., 2004).
According to the analysis values of the 14C ages of Tengger Nuur (Figure 2), there are measurable differences between the 14C ages of the upper stratum around 200 cm, which are 3050 ± 30 a BP (72–74 cm) and 4520 ± 30 a BP (172–174 cm), and the lower stratum. Meanwhile, the mean grain size of the upper deposits remains at around 6 μm, and the carbonate content is stable at approximately 10% (Figure 2), indicating that the sedimentary environment and the sedimentary rate are relatively consistent. Consequently, the linearly extrapolated 14C age of surface sediments was 1920 a BP, which can also be considered as the LCRE of Tengger Nuur. The Daqingshan Mts, which serve as the water source to Tengger Nuur, also supply water to Diaojiao Lake as the main source. The 14C ages of Diaojiao Lake were estimated at 2380 ± 90 a BP (Song et al., 1996) and 2170 ± 70 a BP (Yang et al., 1997) from shallow lacustrine sediments at 3–5 cm. That of Hulun Lake was 685 ± 21 a BP from sediment at 0–1 cm (Wen et al., 2010).
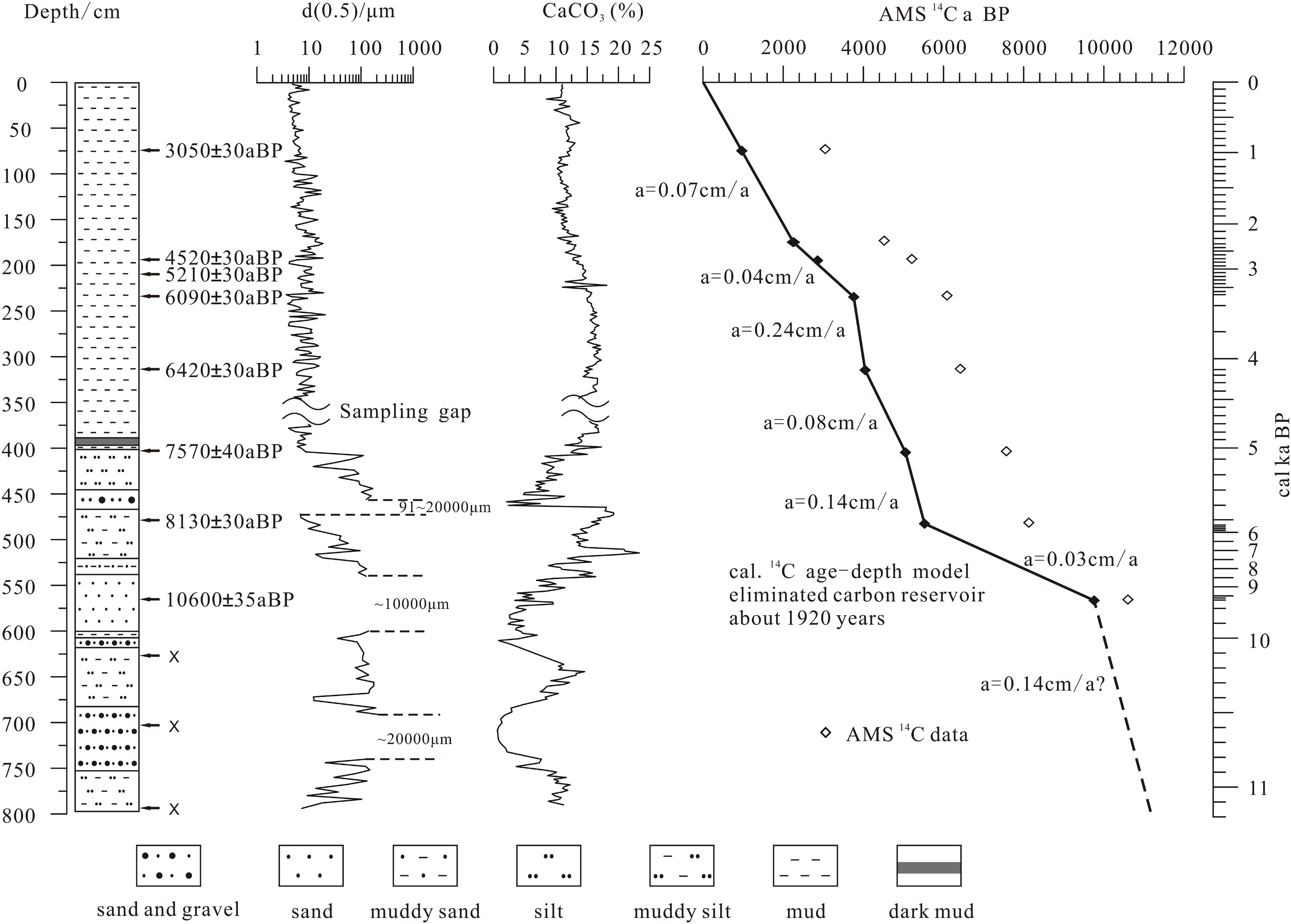
Figure 2. Lithologic section of TGN-05 and the depth-age model based on accelerator mass spectrometry 14C data.
To produce an age-depth model for TGN-05, we first subtracted the reservoir age of 1920 year from all the original 14C ages, assuming that it is constant throughout the stratum, and then performed calibrations on the reservoir-effect-free 14C dates. The conventional ages were converted to calibrated ages using the OxCal 4.2 and IntCal13 calibration curves (Reimer et al., 2013). An age-depth model was then created with the linear relation and sedimentation rate. The sedimentation rate between 400 and 460 cm was about 0.14 cm/a. Because the sediments consist of coarse sand and fine gravel below 550 cm, which is the same as the 400–460 cm interval, we assumed the sedimentation rate of 0.14 cm/a and extrapolated the 14C age-depth model below 550 cm (Figure 2). The sedimentation rate in the offshore lake of the Bojianghaizi Lake near the Tennger Nuur is about 1.62 and 0.92 mm/a to the center (Zhai et al., 2000).
Materials and Methods
Paleoenvironmental proxies, TOC-content, C/N, carbonate-content, carbon and oxygen isotope, element, and grain size were analyzed for sediment samples from TGN-05.
Total organic carbon was determined using the anti-titration method, which uses concentrated sulfuric acid (H2SO4) and potassium dichromate (K2Cr2O7), with an error of less than ±0.5%. Both carbonate and TOC content were determined in the environmental lab of the School of Resources and Environmental Sciences, Lanzhou University. The content of carbon (C), nitrogen (N) in the organic matter were determined by a Vario EL III Elemental Analyzer (at the Chemistry Department of Lanzhou University) after the samples were treated with 1 N HCl.
A common method to analyze carbonate content in soils is the so-called “Scheibler method,” in which samples are treated with hydrochloric acid and the released CO2 is volumetrically determined, and the volume of CO2 is then converted into carbonate concentration (%) (Tatzber et al., 2007). Error is less than ±1%.
For carbonate stable isotopic analyses, all the organic matter were removed by roasting the finer portion (<200 μm) of the samples at about 300°C in a vacuum system before analysis. For carbon and oxygen isotopic composition analyses, 100% phosphoric acid at 90°C was added to the pre-treated samples, and then the acid-produced CO2 gas was purified and transferred into a Finnigan 253 mass spectrometer. The analyses were conducted at the Lanzhou Institute of Geology (Chinese Academy of Sciences) and the isotopic values are reported as the standard δ-per mil notation in V-PDB standard calibrated with NBS-19 (δ18O = −2.20‰, δ13C = + 1.95‰). The sample error is less than 0.3‰.
Metal elements of the bulk lake sediments were determined by the XRF method (PW2403 X, Netherlands) in the Key Laboratory of Western China’s Environmental System (Ministry of Education), Lanzhou University. Then the paleoclimatic indicator Sr/Ca and Mg/Ca ratios (Chivas et al., 1985, 1986; Zhang et al., 2013), a widely used proxy for lake temperature and salinity, were calculated to infer relative environmental change.
The grain sizes of bulk samples were measured using a Malvern Mastersizer 2000 laser granulometer, which was fitted to the limit of grain sizes lower than 2000 μm, and the traditional manual sieve method to determine the weight percentages of three parts, <63–900 μm, and 900–20,000 μm as the fixed hole sizes of sieves 63, 900, and 20,000 μm. After sieving off particles larger than 900 μm, the samples were used for laser granulometer analysis using the following method: (1) adding H2O2 to remove organic matter and soluble salts, (2) using diluted 1N HCl to remove carbonate, and (3) using Na-hexametaphosphate as the dispersing agent to disperse the aggregates. The final results include three part percent concentration, that is <4 μm standing for mud, 4–63 μm for silt, and 63–900 μm for sand, and each part was calibrated with the total weight.
Results and Discussion
Proxies
Grain Size
The grain size of Tengger Nuur sediments (TGN-05) showed obvious changes since the last deglaciation from sand and fine gravel layers in the low part (400–794) (Figure 3) to fine silty mud layer (0–400 cm) in the upper part, punctuated by several coarse particle layers (Figure 4). Gravels are based on quartz grains with high roundness and poor sorting, reflecting off-nearshore shoreline shallow lake deposition.
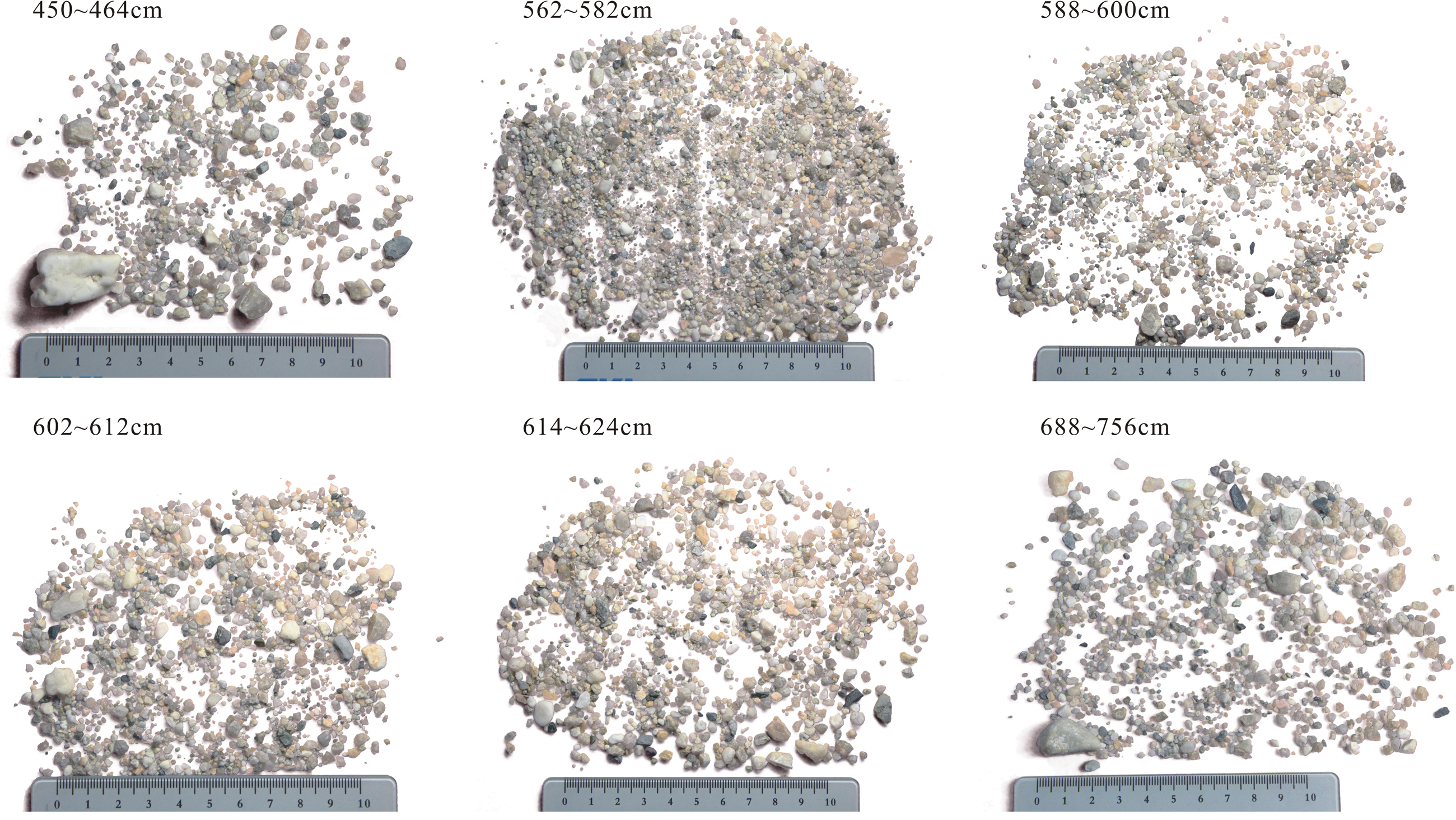
Figure 3. Gravels in the Tengger Nuur lake sediments at depth of 450–756 cm, removed particle parts less than 63 μm size.
Generally, hydrodynamic force controls the distribution of the lake sediment particles. Under ideal condition, gravel and silt to clay size sediments deposit from the shoreline to the center of lake following hydrodynamic weakness. Xiao et al. (2013) and Fan et al. (2016) analyzed the grain size distribution of the modern sediments from Daihai Lake and Dali Lake, respectively, which site near the Tengger Nuur. The results showed that particles of sediments declined gradually from shoreline to the center of lake, and five distinct belts are nearshore suspension fine sand, saltation medium sand, offshore suspension fine silt, medium to coarse silt, and long term suspension clay. The percentage of the nearshore components displays a negative correlation with water depth across the modern lakebed. And, that of the nearshore component increases in the annual precipitation decreasing based on the field observation. Guo et al. (2016) analyzed the modern surface sediments from 68 lakes and reservoirs in the Inner Mongolia Autonomous Region, Gansu Province and Ningxia Hui Autonomous Region, they found that the sediments near lake center mainly contain clay (0.4–1.9 μm), clay-to-fine silt (2.0–12.0 μm) and medium-to-coarse silt (17–58 μm). The sediments in the transitional area (shallow lake) mainly include medium-to-coarse silt (17–58 μm) and fine sand (70–150 μm). The sediments near the shoreline mainly contain medium-to-coarse sands (170–500 μm). The grain sizes of sediments near the regions of river filling are more than 600 μm.
Numerous modern process researches testify that the lake-level decreases with coarse grain sediments increasing, and that deposits under the dry and cold condition. Chen et al. (2003) regarded that coarse sediments were deposited during a shrinkage phase of Lake Yanhaizi in Mongolia under an arid environment, whereas fine sediments were deposited during a period of high lake-level in a humid environment. The lake-level of Qinghai Lake was low and particle was focused on coarse size, and the percents of medium and grain size more than 64 μm were high all during Younger Dryas (YD) event (Liu et al., 2003).
However, aeolian activity can impact the lake near the desert and grain size of sediment loads change with it. For example, a strong storm event can blow coarse particles near the shoreline of lakes into the center of lakes. Fan et al. (2016) analyzed the particles on the surface ice of Dali Lake in the winter, and found that mode grain size of particles is main 17.4 μm or so, and has a small coarse part of mode size 400 μm. Aeolian particles fixed in the sediments of shallow lakes under the cold and dry climate background, but it is difficult to distinct them from the lake sediments in the Tengger Nuur.
Organic Matter
Organic matter as an important fraction of lake sediments originates mainly from the complex mixture non-vascular plants that contain little or no carbon-rich cellulose and lignin, such as phytoplankton, and vascular plants that contain large proportions of these fibrous tissues, such as grasses, shrubs, and trees. So, it can be an effective proxy to interpret the lacustrine paleoenvironments, histories of climate change, and the effects of humans on local and regional ecosystems around the catchments (Meyers and Vergés, 1999). Higher TOC indicates a warm and humid environment, whereas lower TOC indicates a cold and dry environment. The limiting factor of plant growth is effective humidity in semi- and arid areas. Changes in the lake water level have also impacted the organic matter content, and it is low in the shallow water, and the C/N ratios can indicate the type of organic matter delivered to the lake sediments (Meyers and Vergés, 1999; Müller and Ulrike, 1999). Normally, algae typically have atomic C/N ratios between 4 and 10, lacustrine sedimentary C/N ratios of between 10 and 20 represent a mixture of aquatic and higher plant material, whereas C/N ratios >20 indicate higher plant dominance (Meyers, 1994). Plankton has average C/N ratios of ∼6, withmost diatoms varying between 5 and 8 (Lerman, 1979).
As shown in Figure 5, TOC was relatively low (0.1–0.2%) in TGN-05 before 8750 cal a BP (794–540 cm); it was slightly higher at 0.3–0.8% during 8750–2750 cal a BP (540∼200 cm). Since 2750 cal a BP, TOC increased in the range of 0.8–2.2% and reached the maximum value of 2.5–5% under the influence of the marsh environment and human activity.
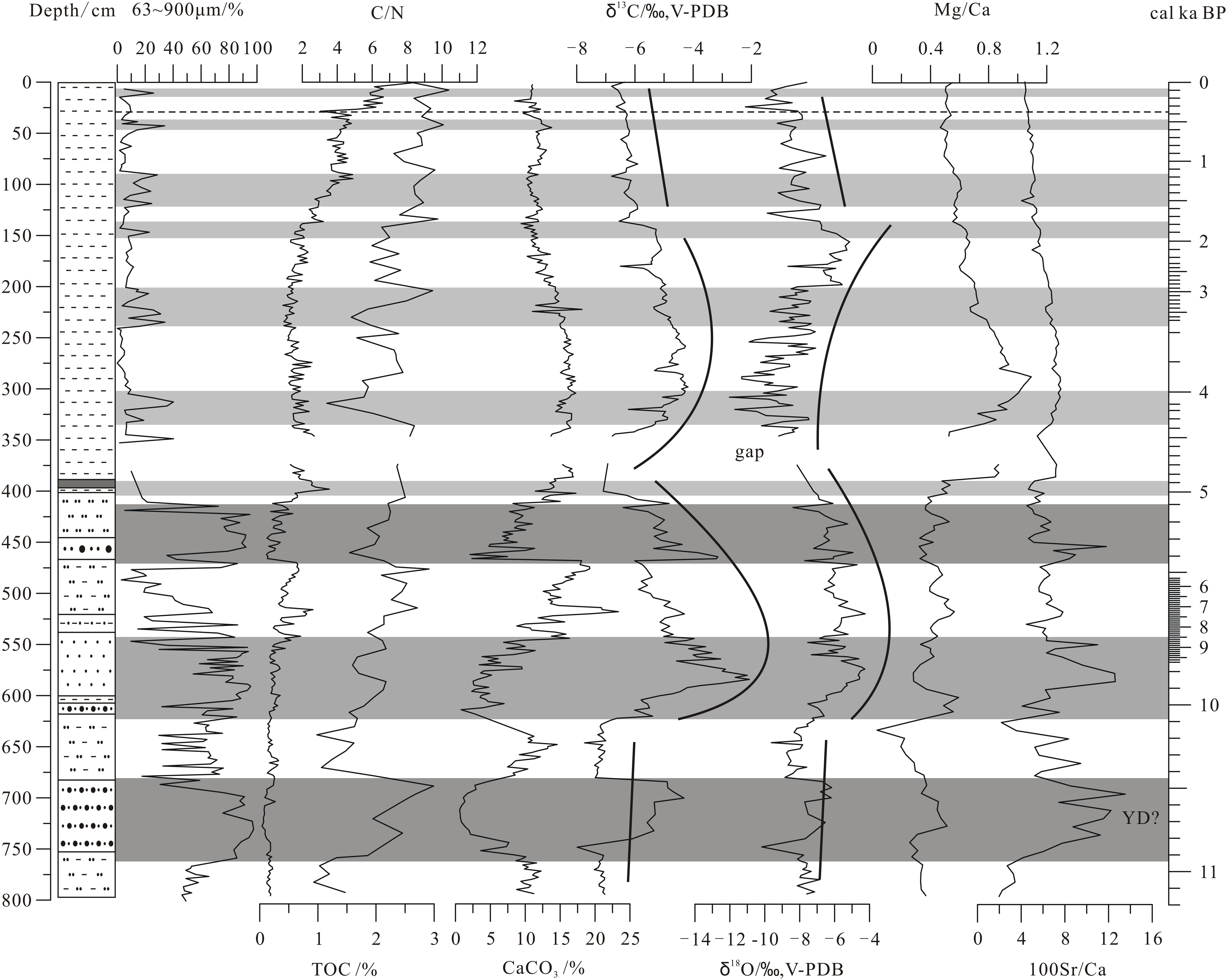
Figure 5. Changes in geochemical proxies in TGN-05 since the last deglaciation. The ratio of Sr/Ca amplifies 100 times to fit for the figure show.
The C/N ratios of Tengger Nuur sediments are at 3–11 and the preponderant value is at 5–6 since the last deglaciation in Figure 5. The ratio is a little higher to 8–11 after about 2000 cal a BP. That inferred the organic matter came from the algae and aquatic plants, but some land higher plants maybe mixed since 2000 cal a BP.
Carbonate
Lacustrine carbonates form mainly in warm and alkaline water bodies. According to the study of lakes in western China, calcite forms in most shallow freshwater environments with low percentages in sediments, and low-Mg calcite and high-Mg calcite to aragonite form with increasing lake depth and salinity (Zhang et al., 2013).
The carbonate content can be divided into several stages, as shown in Figure 5. Carbonate contents were low (1–4%) in the 3 layers formed during 10,920–10,450 cal a BP, 10,050–8750 cal a BP, and 5450–5100 cal a BP with coarse sand to fine gravel. Most of the carbonate was authigenic, forming in western Chinese lakes with fine size, and coarse quartz and feldspar particles in the sediments can dilute the values of carbonate-content such that the determined values become lower than the true values (Zhang et al., 2013). The low content of carbonates during ∼10,920 cal a BP and 10,450–10,050 cal a BP suggests shallow freshwater lake and low-temperature conditions. The high carbonate content (13–8%) during 8750–2750 cal a BP indicates a relatively warm and saline water. Since 2750 cal a BP, carbonate content has been decreasing gradually from 14 to 10% or so, indicating decreases in temperature lowering and salinity.
δ13C and δ18O of Carbonate Bulk Sediments
δ13C and δ18O values are shown in the Figure 5. Lake water chemical conditions and sources can be well traced using carbon and oxygen isotopic signatures (δ13C and δ18O) in lacustrine carbonates. They are controlled by many factors, among which the salinity and temperature of lake water are the leading ones. The controlling factors on δ13C variations in lake sediments are inflow δ13C value, biological productivity, respiration within the lake, exchange of CO2 between the lake and atmosphere, groundwater input, residence time, and changes in the vegetation cover of watersheds (Li and Ku, 1997). Among these, the residence time and biogeochemical features of the lake water are generally considered the most important factors affecting δ13C (Leng and Marshall, 2004). The oxygen isotope compositions of water in lakes (δ18Owater) are dominantly controlled by the local evaporation/precipitation (both rain and snowfall), inflow water (both river and groundwater), and lake water temperature (Talbot, 1990; Menking et al., 1997). The oxygen isotopic composition of the water in a closed lake basin is largely dependent on the ratio of evaporation over precipitation in arid to semi-arid areas. The covariance between δ13C and δ18O can well describe the closed conditions of lakes (Talbot, 1990). Normally, δ13C and δ18O will be synchronously increase with water residence time in lakes (Leng and Marshall, 2004). However, it is also well documented that the two may change asynchronously when extreme temperature or evaporation/precipitation control the isotopic fractionation processes; that is, temperature and evaporation/precipitation have much stronger control over δ18O fractionation than over δ13C fractionation (Wu and Wang, 1997).
Ratio of Mg/Ca in Bulk Sediments
Ratio of the Mg/Ca in bulk sediments is shown in Figure 5. In closed and semi-closed lakes, authi-chemogenic carbonate minerals are formed widely. In general, biogenic and chemogenic carbonates from calcite to low-magnesium calcite, high- magnesium calcite, and aragonite form with increasing Mg/Ca ratio in water (Müller et al., 1972). Mg/Ca has a positive relationship with salinity and can indicate the characteristic of residence time due to the evaporation effect in the closed to semi-closed lakes in northwestern China (Zhang et al., 2013). However, the higher the lake waterbody temperature is, the higher the evaporation is, that leading to higher salinity in closed-lakes. The dominating carbonate mineral in Las Coladas Lake in the south of the San Francisco Basin, Argentina, is aragonite because of the high Mg/Ca ratio of the lake water (Valero-Garces et al., 1995). So, Mg/Ca and Sr/Ca ratio have a positive relationship in lake sediments, indicating residence time and evaporation effect.
Paleoenvironment
The characteristics of TGN-05 sediment particles can be inferred from the lake-level oscillation history of Tengger Nuur since the last deglaciation. Coarse sediments indicate a shallow lake, and fine sediments indicate a stable lake. The results of the grain size analysis of TGN-05 showed that the lake was shallow in the periods of 10,920–8750 cal a BP and 5450–5100 cal a BP, deep and relatively stable during 8750–5450 cal a BP and 5100 cal a BP to present. The chemical characteristics of water differed during different periods owing to the different climate setting (Figure 5).
About >757 cm (∼10,920 cal a BP), fine-grained sediment layer, low Mg/Ca and Sr/Ca ratios indicate a shallow lake with freshwater formed under a cool climate and much higher water supply than evaporation and rudimentary vegetation.
About 757–685 cm (10,920–10,450 cal a BP), coarse sand and fine gravel sediment layer, heavy δ13C and δ18O, high Mg/Ca and higher Sr/Ca ratios represent a shoreline lake with very shallow shoreline brackish-water lake under cold climate and long residence time. This corresponds to the global cold YD event (Hughen et al., 1985; Mikolajewicz et al., 1997), which happened during the Last Glacial Termination recorded the onset of YD was dated to 10,800–10,600 14C years BP and that the end was dated to 10,000–10,050 14C years BP (Ammann and Lotter, 1989; Björck et al., 2003; Muscheler et al., 2008). It was at 11–10 ka BP cooling of Europe and eastern North America (Mathewes, 1993). After calibrating based on the tree-ring chronology, YD was a millennial-scale cold period between approximately 12,900 and 11,500 year BP (Muscheler et al., 2008). The beginning of the YD event recorded at stalagmite of Yamen Cave was nominally at 12,850 ± 50 a BP and the end of the YD dates to 11,500 ± 40 a BP (Yang et al., 2010). And YD event recorded at Hulu Cave from 12,823 ± 60 a BP to 11,473 ± 100 a BP (Wang et al., 2001). However, the YD event recorded in Qinghai Lake was 11.3–10.8 cal ka BP calibrated by the tree-ring (Shen et al., 2005). The Younger Dryas cooling event recorded in the Tibet lakes occuring in 11,000-10,000 a BP, and coldest period was at 10.5–10.7 ka BP (Shen et al., 1996). Cooling wet-type YD event was at 10.80–10.50 ka BP recorded at Inner Mongolian salt lakes (Chen et al., 1996). The duration of the YD is 300 years as recorded at the salt lakes of Inner Mongolian (Chen et al., 1996) to 1350 years at the stalagmites from Hulu Cave and Yamen Cave (Wang et al., 2001; Yang et al., 2010). But till to now, the timing and length of the Younger Dryas remain controversial.
The cold and extremely low lake-level records in Tengger Nuur also had been found in the other lakes around this area. It was a cold desert during 10,950–10,300 a BP, as recorded in Daojiaohaizi sediments; the temperature was 5°C lower and precipitation was 100 mm lower than those today (Yang et al., 1997). The level of Daihai Lake ascended during 13–11 ka BP and declined sharply from 11 to 10 ka BP (Sun et al., 2009). The lake-level of Jalai Nur fell rapidly at the YD event during 10.9–10.6 ka BP, the flaser and lenticular bedding coarse sediment sets suggested the near shore environments (Wang et al., 1994). The aeolian sand layer correlated with the cold and dry YD event at 11.6-11.3 cal ka BP in the Haolaihure Paleolake (Liu et al., 2018). Grain size at 16-64μm in the Qinghai Lake sediments increased obviously during YD event at 11,000–10,400 a BP (Liu et al., 2003). Low lake-level and prevailing aeolian sediment deposition at Gun Nuur under dry conditions were recorded during the earliest Holocene (>10,800–10,300 cal a BP) (Zhang et al., 2012). The 14C age was unequivocal and uncertainty, but we correlated this gravel layer (757–685 cm) to the YD event (10,920–10,450 cal a BP) in Tengger Nuur.
About 685–620 cm (10,450–10,050 cal a BP), sandy silt sediment, light δ13C and δ18O, low Mg/Ca and Sr/Ca ratios indicate gradual increase in lake area to a shallow lake under a low-temperature freshwater environment with evaporation less than water supply.
About 620–540 cm (10,050–8750 cal a BP), silty sand and fine gravel sediment, heavy δ13C and δ18O, and high Mg/Ca and Sr/Ca ratios reflect lake-levels dropped, fluctuating between a shoreline and shallow lake due to a high ratio of evaporation/filling water under increasing temperature. According to the paleoenvironment recorded from Tengger Nuur lake sediments, the Holocene started from 10,050 cal a BP and with the characteristics of temperature increase. However, Tengger Nuur was very shallow with slightly high salinity and high evaporation during 10,050–8750 cal a BP. Several paleoenvironmental records around Tengger Nuur suggest this phenomenon. Hulun Lake was shallow and sand blowing activity strengthened in the early Holocene (10,000–7200 a BP) under cold-dry to warm-dry climate conditions (Zhang and Wang, 2000). According to diatom data, Hulun Lake shrank gradually (Yang et al., 1995). Huangqihai experienced transgressions under a cold and wet climate in the early Holocene (11,000–8000 a BP), and Daihai also had a low level during 12,000–8000 a BP (Li et al., 1992). At the same time, Li et al. (1992) considered Daihai to be brackish water or have slightly high salinity based on ostracod components. They also considered lake level to have dropped to form a river environment under a cold and dry climate during 9000–8000 a BP, and lake level retreated again under a warm climate condition after 8000 a BP. In contrast, Sun et al. (2009) suggested that the lake level of Daihai expanded temporarily as effective precipitation increased under the summer monsoon-related humid climate during ca. 10–9 ka BP.
About 540–469 cm (8750–5450 cal a BP), sandy silt sediment, light δ13C and δ18O, high Mg/Ca and Sr/Ca ratios indicate a gradually increase in lake area to a shallow lake under relatively warm water and evaporation slightly higher than water supply. The temperature increased and vegetable was abundant. However, a low lake-level event interrupted with a cold-wet climate during 5450–5100 cal a BP. Around this area, Lake Baahar Nuur was under a warm and humid climate between ∼7.65 and ∼5.40 ka BP (Guo et al., 2007). Temperature and humidity increased synchronously in the course of 8200–6350 a BP, and then, the climate oscillated from wet to dry from 6350 to 5100 a BP (Song et al., 1996).
The Holocene climatic optimum in the Huangqihai region can be placed between 8000 and 6000 a BP when the temperature was much more than 2–3°C, precipitation was high, and the lake level was also high (Li et al., 1992). Angulinao was deep during 8400–5500 a BP, warming during 8.4–7.3 ka BP, stable during 7.3–6.2 ka BP, and fluctuating during 5.5–5.0 ka BP (Zhai et al., 2000). Effective humidity in the Dali Nor region reached the maximum prior to ∼8000 years BP (Wang et al., 2004). Paleosol thickness reached the maximum in the Mu Us desert during 7000–5500 a BP; and it was in the extreme state in the Ordos Plateau during 6500–5500 a BP (Yang et al., 1997).
About 469–401 cm (5450–5100 cal a BP), coarse sediment layer, heavy δ13C and light δ18O, and low Mg/Ca and Sr/Ca ratios indicate a rapid lake-level decline to form a shoreline lake, and interrupted by a shallow lake during 5300–5100 cal a BP under a low temperature and evaporation environment.
About 401–0 cm (5100 cal a BP ∼AD 1985), silty mud or muddy silt sediments, heavy δ13C and δ18O, high Mg/Ca and Sr/Ca ratios indicate a relatively stable deep lake at the interval 5100–1800 cal a BP. After 1900 cal a BP, lighter δ13C and δ18O, low Mg/Ca and Sr/Ca ratios indicate a freshwater environment under low evaporation with relatively higher effective humidity. Tengger Nuur was completely desiccated in 1985 as history recorded due to a gradual decrease in water supply. It was punctuated by several centennial- to millennial-scale shallow lake events, 330–300 cm (4300–3980 cal a BP), 240–200 cm (3700–2750 cal a BP), 150–140 cm (1900–1800 cal a BP), 120–85 cm (1500–1050 cal a BP), 40–30 cm (550–400 cal a BP), 10–0 cm (100 cal a BP ∼AD 1985). A layer of dark mud in the 389–398 cm interval (4960–4850 cal a BP) suggests a short–stage tidal flat environment as the lake-level started to increase.
Tengger Nuur expanded quickly to reach a high lake level during 5000–2000 cal a BP, under the condition of effective humidity increasing with decreasing temperature in oscillation. This implies that humidity and temperature fluctuated in the opposite directions. Gun Nuur was deep in the mid-Holocene (7000–2500 cal a BP), but three periods of low lake levels and significantly drier conditions were recorded between 7000–5700, 4100–3600, and 3000–2500 cal a BP (Zhang et al., 2012).
Tengger Nuur shrank gradually and became completely desiccated under a cool climate from 2000 cal a BP to 1985 AD, and the period of 1050–550 cal a BP corresponds to the Medieval Warm Period (MWP). Four periods of low lake levels were recorded during 1900–1800 cal a BP, 1500–1050 cal a BP, 550–400 cal a BP (Little Ice Age), and 100 cal a BP–AD 1985. Owing to the retention of a very shallow lake level over a long time, a marsh environment formed around Lake Tengger Nuur and various aquatic plants such as reeds increased TOC of the sediments. The paleoenvironment of most regions in Inner Mongolia indicate a dry climate and gradual shrinking of lakes. For instance, Baahar Nuur Lake was under a dry climate characterized by complete desiccation of the lake after 3700 a BP (Guo et al., 2007). According to paleoenvironmental records of Haolaihure, the regional environment deteriorated after 2.2 cal ka BP as the climate shifted to generally cooler and drier conditions, with a brief return to a warm and wet climate during the MWP (AD 800–1100) (Liu et al., 2018). Daihai and Huangqihai shrank sharply and several periods of retreat have been oscillating since 3000 a BP (Li et al., 1992).
Paleoclimate
The northern region of the East-Asian monsoon transition zone, where Daqingshan is a boundary in the mid-eastern part of Inner Mongolia, is controlled by the westerly and Siberian-Mongolian high winter monsoon winds (WSMHM), and the southern region is controlled by the Eastern Asian Summer monsoon (EASM; Figure 1; Winkler and Wang, 1993). Paleoenvironmental records show that the climate was dry and cold and precipitation was less during the last deglaciation than that today in the WSMHM area of Gun Nuur (Zhang et al., 2012), Daojiaohaizi Lake (Yang et al., 1997), Haolaihure (Liu et al., 2018), feet of the Daqingshan (Cui and Song, 1992), and Baahar Nuur (Guo et al., 2007). The Qingtu paleolake westward of the Tengger Sandy Desert was an aeolian non-lake environment (Zhao et al., 2008). Influenced by EASM Daihai Lake had a low lake-level under the dry and cold climate (Sun et al., 2009). Kutzbach and Street-Perrott (1985) suggested that solar radiation culminated at 11–10 a BP since 18 ka BP in the Northern Hemisphere. Paleoenvironmental records of Tengger Nuur and other lakes testified that the summer monsoon did not strengthen to pass the modern monsoon boundary of Inner Mongolia. However, India’s summer monsoon strengthened since 14–12 ka BP in the India monsoon dominated region of the Tibetan Plateau. Temperature and humidity increased greatly after interrupted by the short YD cold climate event. It became dry after 5 ka BP (He et al., 2004). Selin Co was a deep closed lake that formed stably for a long period with abundant vegetation under a warm and humid climate background from 15.5 to 10.4 cal ka BP (Zhang C. J. et al., 2018). The temperature and humidity increased quickly and lake-levels of Ximenco in eastern Tibetan Plateau (Zhang et al., 2009), Lake Naleng Co (Opitz et al., 2015), Koucha Lake (Mischke et al., 2008), and Luanhaizi in the Qilian Mountain (Mischke et al., 2005), etc., expanded since about 13,000 cal a BP in the interplay of EASM and the Indian monsoon.
Temperature and humidity increased and lakes expanded gradually with the strengthening of solar radiation since the early Holocene according to records, such as in Greenland Ice cores (Greenland Ice-core Project (GRIP) Members, 1993), stalagmites of Dongge cave in the EASM-dominated region (Dykoski et al., 2005), loess sequences (Zhou et al., 2014), and Qinghai Lake (Liu et al., 2007). However, the humidity (Chen et al., 2019) and lake-level oscillation (Guo et al., 2014) in the Arid Central Asia (ACA) the westerly dominated region, Indian Summer Monsoon (ISM)-dominated region, and Eastern Asian Summer Monsoon (EASM)-dominated region had different spatio-temporal characteristics (Figure 6). The lake surface area of Daihai Lake in the southern part of Daqingshan in the modern Eastern Asian monsoon boundary expanded temporarily according to the amelioration of monsoon-related effective precipitation and a relatively humid climate during 10–9.0 ka BP (Sun et al., 2009; Wen et al., 2010). The wettest climate occurred between 10,500 and 6500 cal a BP, during which annual precipitation was up to 30–60% higher than today recorded by Bayanchagan Lake sediments (Jiang et al., 2006). At the same time, in this study, Tengger Nuur in the northern part of Daqingshan was found to have a low lake level during 10,050–8750 cal a BP. Hulun Lake was also had a low lake level and strong aeolian activity under a warm-dry climate in the early Holocene during 10,000–7200 a BP (Yang et al., 1995; Zhang and Wang, 2000; Wen et al., 2010). Haolaihure was under a cool and dry period from 12.2 to 8.7 cal ka BP (Liu et al., 2018). Baahar Nuur Lake (Guo et al., 2007) and Qingtu Lake in the Tengger Sandy Desert (Zhao et al., 2008) had not formed in the early Holocene. Sediments at the feet of Daqingshan recorded a cold climate (Cui and Song, 1992). It was obvious that lake that did not form or low-lake-level sediments were derived from the northern part of the modern summer monsoon boundary and the climate was cold in the early Holocene. Nevertheless, lakes expanded and achieved high levels under a relatively warm-wet climate in the southern part of the modern summer monsoon boundary.
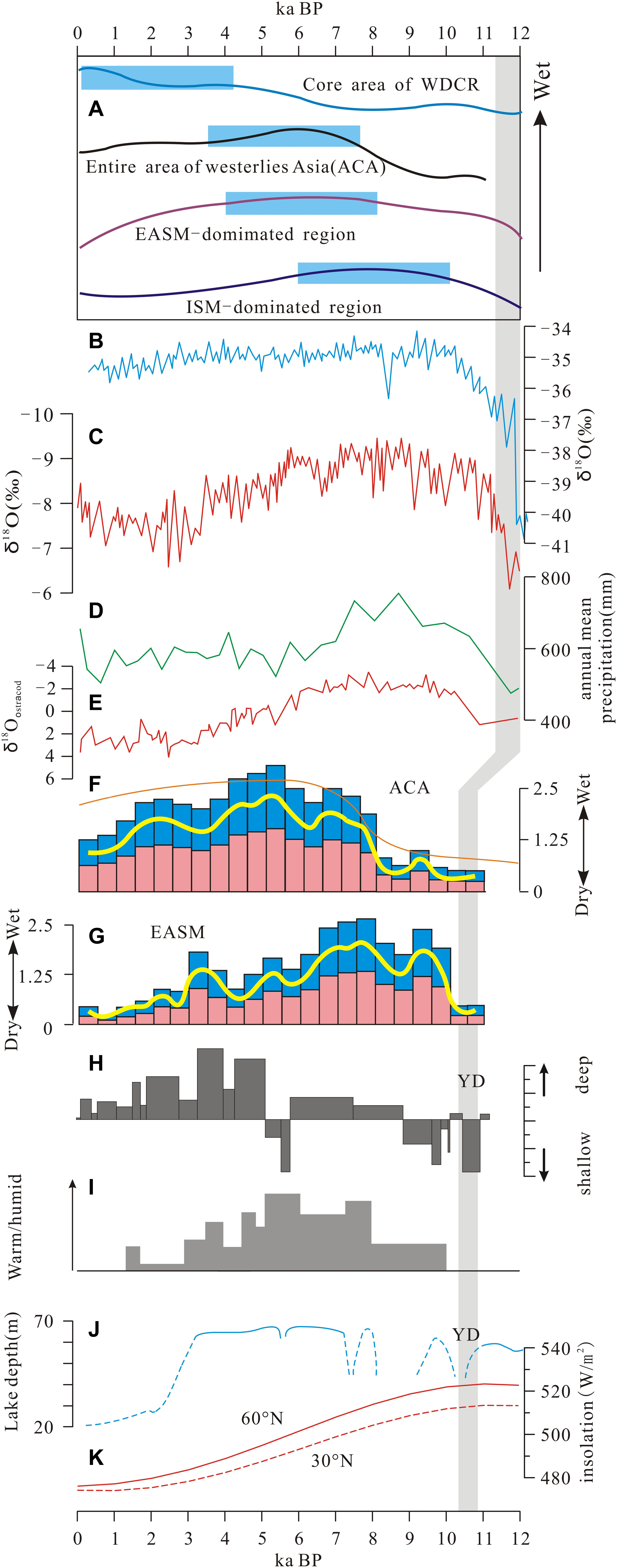
Figure 6. Paleoenvironmental records in different climate regions. (A) westerlies-dominated climatic regime (WDCR), ACA, Eastern Asian Summer Monsoon (EASM), and Indian Summer Monsoon (ISM) (Chen et al., 2019), (B) GRIP (Greenland Ice-core Project (GRIP) Members, 1993), (C) Dongge Cave (Dykoski et al., 2005), (D) Luochuan Loess (Zhou et al., 2014), (E) Qinghai Lake (Liu et al., 2007), (F) Central Asia (ACA) westerly dominated region (Guo et al., 2014), (G) Eastern Asian Summer Monsoon (EASM)-dominated region (Guo et al., 2014), (H) Tengger Nur (this paper), (I) Daihai (Xiao et al., 2004), (J) Daihai (Sun et al., 2009), (K) solar irradiation in the Northern Hemisphere.
An atmospheric general-circulation model simulated a maximum summer solar radiation (7% more than the present value) at interval 11,000–10,000 cal a BP, during which precipitation increased in the Northern Hemisphere, such that lake-levels increased across parts of Africa, Arabia, and India between 10,000 and 5000 a BP (Kutzbach, 1981; Kutzbach and Street-Perrott, 1985). Summer monsoon strengthening was followed by high solar irradiation in the early Holocene, especially in correlation with the lake-level rise in China (Bond et al., 2001). Various paleoclimatic records show that a wetter condition prevailed during the early Holocene since ∼10,000 a BP in China (An, 2000; Chen et al., 2003). However, the low lake-level record in Tengger Nuur showed that the summer monsoon was not sufficiently strong to reach northward of the monsoon boundary, and thus, precipitation was low. A little warm paleoclimate based on pollen and diatom data indicates that solar radiation led to temperature increases in this area in the early Holocene (Chen et al., 2003; Liu et al., 2018).
According to our paleoenvironmental record of Gun Nuur in Mongolia, the lake expanded in the early Holocene (ca. 10.3 cal ka BP), but the significant moisture was probably not related to the northward shift of the present summer monsoon boundary or the moisture delivery from the northern Atlantic through the westerlies. The water source was melting snow, ice and frozen ground or the generation of precipitation from the local recycling of moisture (Zhang et al., 2012). Therefore, solar radiation strengthened in the early Holocene to drive summer monsoon to the modern monsoon boundary in Inner Mongolia, but monsoon precipitation did not influence the northern area of Daqingshan, where the summer monsoon should occur. It is inferred that the summer monsoon was not sufficiently strong to pass the boundary in the early Holocene. Furthermore, this can be deduced that the Daqingshan (height of 2338 m asl at the highest peak) was not covered by ice-snow during the last glacial maximum, and thus, the temperature increase in the early Holocene could not thaw enough ice and snow to fill Tengger Nuur.
Yang and Wang (1996) considered that the westerlies prevailed in the Hulun Lake area at intervals 10.0–7.0 ka BP and 5.0–3.0 ka BP, and eastern monsoon winds reached the northwest region of the summer monsoon boundary at about 7.0–5.0 ka BP. This also shows that the eastern summer monsoon was weak in the early Holocene.
Tengger Nuur started to expand during 8750–5000 cal a BP with high evaporation and a brackish water environment and entered a stable deep state during 5000–2000 cal a BP, punctuated by several millennial- and centennial-scale low lake levels. As researched in the past, the summer monsoon strengthened from the early Holocene to mid-Holocene, the most humid conditions occurred at approximately 5500–2700 cal a BP (Xiao et al., 2002), or 8200–5700 cal a BP in southern Inner Mongolia (Zhou et al., 2001). The summer monsoon exceeded the modern monsoon boundary to the northward region to Mongolia. Abundant precipitation led to the expansion of almost all lakes in the Mongolia Plateau. For example, the boundary of the eastern monsoon winds reached the Hulun Lake region during about 7.0–5.0 ka BP (Yang and Wang, 1996); Angulinao was deep during 8400–5500 a BP and cooled down from 3.0 ka BP to present (Zhai et al., 2000) and aeolian activity strengthened (Zhang and Wang, 2000); Daihai and Huangqihai had high lake levels during 8500–3500 a BP and shrank gradually since 5000 a BP (Li et al., 1992), and grasses and birch forests expanded during 8000–6400 cal year BP, implying a remarkable increase in the monsoon precipitation (Wen et al., 2010). However, the Yanhaizi region was dry between 8.0 and 4.3 ka BP. Chen et al. (2003) regarded that enhanced evaporation over higher monsoon precipitation reduced the effective humidity in the warm climate.
Cold events during 5450–5100 and 4300–3980 cal a BP recorded in Tengger Nuur significantly impacted human activities in northwestern China. Denton and Karlen (1973) defined 5800–4900 a BP as the second neoglaciation and 3300–2400 a BP as the third neoglaciation in the Holocene. Human activity had greatly been set back in the farming-animal husbandry zigzag zone of northern China at this stage of late Yangshao Culture (5500–5000 a BP) (Yang and Suo, 1996). The cold event in northwestern China during 4300–3500 a BP also contributed to the advancement of the Qijia Culture (Zhang H. R. et al., 2018).
After 2000 cal a BP, the Tengger Nuur region was controlled by the westerly current (Chen et al., 2019). The lake shrank gradually with decreasing water supply under the cold and dry climate, and it was completely desiccated in 1985. Moreover, several centennial-scale lake-level oscillations occurred during this stage.
Conclusion
Paleoenvironmental records from Tengger Nuur sediments (TGN-05) showed that solar radiation strengthened to stimulate the strengthening of the eastern monsoon in the Northern Hemisphere since the last deglaciation. However, the eastern summer monsoon in the early Holocene was not sufficiently strong to influence the northern region of the modern monsoon boundary in the Inner Mongolian Plateau. Water from the thawing of ice and snow covering the Daqingshan mountains was not sufficient to fill Tengger Nuur and other lakes in this region. The summer monsoon passed to the northward and northwestward regions of the monsoon boundary during 8750–5000 cal a BP. Lakes expanded rapidly under the associated high monsoon precipitation, but lake levels oscillated in a shallow state owing to high evaporation under high temperatures during this period. The summer monsoon receded gradually during 5000–2000 cal a BP. Nevertheless, lakes entered a stable deep state under high effective humidity with decreasing temperature, punctuated by several centennial-scale low lake-level events. The westerlies influenced this region and the climate was cold and dry after 2000 cal a BP. Lakes changed gradually to become salinized or completely desiccated. The lake state and vegetation cover have a close relationship with effective humidity in the arid Mongolian Plateau.
Data Availability Statement
All datasets presented in this study are included in the article/supplementary material.
Author Contributions
ZC and ZW contributed to fieldwork and manuscript perparation. ZL, TY, LY, WX, ZZ, and SK contributed to laboratory work and helped perparing the experiments. All authors contributed to the article and approved the submitted version.
Funding
We are thankful to the National Natural Science Foundation of China for providing the fund (grant NSFC 41571177) and the Fundamental Research Funds for the Central Universities (grant 2022019zr46).
Conflict of Interest
The authors declare that the research was conducted in the absence of any commercial or financial relationships that could be construed as a potential conflict of interest.
Acknowledgments
We wish to thank LT, HL, and QS for their thorough reviews and many helpful comments.
References
Ammann, B., and Lotter, A. F. (1989). Late-glacial radiocarbon- and palynostratigraphy on the Swiss Plateau. Boreas 1989, 109–126. doi: 10.1111/j.1502-3885.1989.tb00381.x
An, Z. S. (2000). The history and variability of the East Asian paleomonsoon climate. Quat. Sci.Rev. 19, 171–187. doi: 10.1016/s0277-3791(99)00060-8
Björck, S., Koc, N., and Skog, G. (2003). Consistently large marine reservoir ages in the Norwegian Sea during the Last Deglaciation. Quat. Sci. Rev. 22, 429–435. doi: 10.1016/s0277-3791(03)00002-7
Bond, G., Kromer, B., Beer, J., Muscheler, R., Evans, M. N., Showers, W., et al. (2001). Persistent solar influence on North Atlantic climate during the Holocene. Science 294, 2130–2136. doi: 10.1126/science.1065680
Chen, C. T. A., Lan, H. C., Lou, J. Y., and Chen, Y. C. (2003). The Dry holocene megathermal in inner Mongolia. Palaeogeogr. Palaeoclimatol. Palaeoecol. 193, 181–200. doi: 10.1016/s0031-0182(03)00225-6
Chen, F. H., Chen, J. H., Huang, W., Chen, S. Q., Huang, X. Z., Jin, L. Y., et al. (2019). Westerlies Asia and monsoonal Asia: spatiotemporal differences in climate change and possible mechanisms on decadal to sub-orbital timescales. Earth Sci. Rev. 192, 337–354. doi: 10.1016/j.earscirev.2019.03.005
Chen, H. F., Song, S. R., Lee, T. Q., Löwemark, L., Chi, Z. Q., Wang, Y., et al. (2010). A multiproxy lake record from Inner Mongolia displays a late Holocene teleconnection between Central Asian and North Atlantic climates. Quat. Int. 227, 170–182. doi: 10.1016/j.quaint.2010.03.005
Chen, Y. C., Wei, D. Y., Wang, J. J., Guan, S. Z., Qian, Z. H., Yang, Q. T., et al. (1996). Determination and recognization of Younger Dryas and its important significance in the salt lakes sediments of Inner Mongolian. Geol. Chem. Miner. 18, 163–169. (in Chinese).
Chivas, A. R., De Deckker, P., and Shelley, J. M. C. (1985). Strontium content of ostracods indicates lacustrine palaeosalinity. Nature 316, 251–253. doi: 10.1038/316251a0
Chivas, A. R., De Deckker, P., and Shelley, J. M. G. (1986). Magnesium and strontium in non-marine ostracod shells as indicators of palaeosalinity and palaeotemperature. Hydrobiologia 143, 135–142. doi: 10.1007/bf00026656
Cui, Z. J., and Song, C. Q. (1992). Holocene periglacial process es and environmental changes in Daqingshan Mountains, Mongolia, China. Permafr. Periglac. Process. 3, 55–62. (in Chinese), doi: 10.1002/ppp.3430030108
Denton, G. H., and Karlen, W. (1973). Holocene climatic variations- their pattern and possible cause. Quat. Res. 3, 155–205. doi: 10.1016/0033-5894(73)90040-9
Dykoski, C. A., Edwards, R. L., Cheng, H., Yuan, D. X., Cai, Y. J., Zhang, M. L., et al. (2005). A high-resolution, absolute-dated Holocene and deglacial Asian monsoon record from Dongge Cave, China. Earth Planet. Sci. Lett. 233, 71–86. doi: 10.1016/j.epsl.2005.01.036
Fan, J. W., Xiao, J. L., Wen, R. L., Zhai, D. Y., Zhang, S. R., and Qin, X. G. (2016). A model for the linkage between grain size component in the Dali lake sediments and lake level status. Quat. Sci. 36, 612–622. (in Chinese), doi: 10.11928/j.issn.1001-7410.2016.03.11
Greenland Ice-core Project (GRIP) Members (1993). Climate instability during the last interglacial period recorded in the GRIP ice core. Nature 364, 203–207. doi: 10.1038/364203a0
Guo, C., Ma, Y. Z., Hu, C. L., Wu, Y. Q., and Lu, R. J. (2014). Holocene humidity changes in inland China inferred from lake sediments. Prog. Geogr. 33, 786–798. (in Chinese), doi: 10.11820/dlkxjz.2014.06.007
Guo, L. L., Feng, Z. D., Li, X. Q., Liu, L. Y., and Wang, L. X. (2007). Holocene climatic and environmental changes recorded in Baahar Nuur Lake core in the Ordos Plateau, Inner Mongolia of China. Chin. Sci. Bull. 52, 959–966. doi: 10.1007/s11434-007-0132-1
Guo, X. G., Wang, W., Wang, G. L., Liu, L. N., Ma, Y. Z., and He, J. (2016). Within-lake distributions of grain-size components and environmental implications based on the survey of lake surface sediment of Chinese monsoon marginal area. Geogr. Res. 35, 677–691. (in Chinese), doi: 10.11821/dlyj201604007
He, Y., Theakstone, W. H., Zhang, Z. L., Zhang, D., Yao, T. D., Chen, T., et al. (2004). Asynchronous holocene climatic change across China. Quat. Res. 61, 52–63. doi: 10.1016/j.yqres.2003.08.004
Hou, J. Z., D’Andrea, W. J., and Liu, Z. H. (2012). The influence of 14C reservoir age on interpretation of paleolimnological records from the Tibetan Plateau. Quat. Sci. Rev. 48, 67–79. doi: 10.1016/j.quascirev.2012.06.008
Hughen, K. A., Overpeck, J. T., Lehman, S. J., Kashgarian, M., Southon, J., Petersonk, L. C., et al. (1985). Deglacial changes in ocean circulation from an extended radiocarbon calibration. Nature 391, 65–68. doi: 10.1038/34150
Jiang, W. Y., Guo, Z. T., Sun, X. J., Wu, H. B., Chu, G. Q., Yuan, B. Y., et al. (2006). Reconstruction of climate and vegetation changes of Lake Bayanchagan (Inner Mongolia): holocene variability of the East Asian monsoon. Quat. Res. 65, 411–420. doi: 10.1016/j.yqres.2005.10.007
Jie, D. M., Liu, J. F., Li, Z. M., Leng, X. T., Wang, S. Z., and Zhang, G. R. (2001). Carbonate content of sedimentary core and Holocene lake-level fluctuation of Dabusu lake. Mar. Geol. Quat. Geol. 21, 77–82. (in Chinese), doi: 10.2307/2786328
Kutzbach, J. E. (1981). Monsoon climate of the Early Holocene: climate experiment with the Earth’s orbital parameters for 9000 years age. Science 214, 59–61. doi: 10.1126/science.214.4516.59
Kutzbach, J. E., and Street-Perrott, F. A. (1985). Milankovitch forcing of fluctuations in the level of tropical lakes from 18 to 0 kyr BP. Nature 317, 130–134. doi: 10.1038/317130a0
Leng, M. J., and Marshall, J. D. (2004). Palaeoclimate interpretation of stable isotope data from lake sediment archives. Quat. Sci. Rev. 23, 811–831. doi: 10.1016/j.quascirev.2003.06.012
Li, H. C., and Ku, T. L. (1997). δ13C-δ18O covariance as a paleohydrological indicator for closed-basin lakes. Palaeogeogr. Palaeoclimatol. Palaeoecol. 133, 69–80. doi: 10.1016/s0031-0182(96)00153-8
Li, H. Z., Liu, Q. S., and Wang, J. X. (1992). Study of evolution of Huangqihai and Daihai lakes in Holocene in Inner Mongolia Plateau. J. Lake Sci. 4, 31–39. (in Chinese), doi: 10.18307/1992.0104
Li, X. Z., Liu, W. G., Zhou, X., Xu, L. M., and Cheng, P. (2017). A 700-year macrophyte productivity record inferred from isotopes of macrophyte remains and bulk carbonates in Lake Koucha, northeast Qinghai-Tibetan Plateau. Quat. Int. 430, 32–40. doi: 10.1016/j.quaint.2015.11.053
Liu, J., Wang, Y., Wang, Y., Guan, Y. Y., Dong, J., and Li, T. D. (2018). A multi-proxy record of environmental changes during the Holocene from the Haolaihure Paleolake sediments, Inner Mongolia. Quat. Int. 479, 148–159. doi: 10.1016/j.quaint.2016.12.015
Liu, X. Q., Shen, J., Wang, S. M., Wang, Y. B., and Liu, W. G. (2007). Southwest monsoon changes indicated by oxygen isotope of ostracode shells from sediments in Qinghai Lake since the late glacial. Chin. Sci. Bull. 52, 539–544. doi: 10.1007/s11434-007-0086-3
Liu, X. Q., Wang, S. M., and Shen, J. (2003). The grainsize of the core QH-2000 in Qinghai Lake and its implication for paleoclimate and paleoenvironment. J. Lake Sci. 15, 112–117. (in Chinese), doi: 10.18307/2003.0203
Long, H., Lai, Z. P., Wang, N. A., and Zhang, J. R. (2011). A combined luminescence and radiocarbon dating study of Holocene lacustrine sediments from arid northern China. Quat. Geochronol. 6, 1–9. doi: 10.1016/j.quageo.2010.06.001
Mathewes, R. W. (1993). Evidence for younger Dryas-age cooling on the North Pacific coast of America. Quat. Sci. Rev. 12, 321–331. doi: 10.1016/0277-3791(93)90040-s
Menking, K. M., Bischoff, J. L., Fitzpatrick, J. A., Burdette, J. W., and Rye, R. O. (1997). Climate/hydrologic oscillation since ~155000 yr B.P. at Owens lake, reflected in abundance and stable isotope composition of sediment carbonate. Quat. Res. 48, 58–68. doi: 10.1006/qres.1997.1898
Meyers, P. A. (1994). Preservation of elemental and isotopic source identification of sedimentary organic matter. Chem. Geol. 144, 289–302. doi: 10.1016/0009-2541(94)90059-0
Meyers, P. A., and Vergés, E. L. (1999). Lacustrine sedimentary organic matter records of Late Quaternary paleoclimates. J. Paleolimnol. 21, 345–372.
Mikolajewicz, U., Crowley, T. J., Schiller, A., and Voss, R. (1997). Modelling teleconnections between the North Atlantic and North Pacific during the Younger Dryas. Nature 387, 384–387. doi: 10.1038/387384a0
Mischke, S., Herzschuh, U., Zhang, C. J., Jan, B., and Riedel, F. (2005). A late Quaternary lake record from the Qilian Mountains (NW China): lake level and salinity changes inferred from sediment properties and ostracod assemblages. Global Planet. Change 46, 337–359. doi: 10.1016/j.gloplacha.2004.09.023
Mischke, S., Kramer, M., Zhang, C. J., Shang, H. M., Herzschuh, U., and Erzinger, J. (2008). Reduced early Holocene moisture availability in the Bayan Har Mountains, northeastern Tibetan Plateau, inferred from a multi-proxy lake record. Palaeogeogr. Palaeoclimatol. Palaeoecol. 267, 59–76. doi: 10.1016/j.palaeo.2008.06.002
Müller, A., and Ulrike, M. (1999). The palaeoenvironments of coastal lagoons in the southern Baltic sea: the application of sedimentary C/N ratios as source indications of organic matter. Palaeogeogr. Palaeoclimatpl. Palaeoecol. 145, 1–16. doi: 10.1016/s0031-0182(98)00094-7
Müller, G., Iron, G., and Forstner, U. (1972). Formation and diagenesis of inorganic Ca-Mg carbonates in the lacustrine environment. Naturwissenschaften 59, 158–164. doi: 10.1007/bf00637354
Muscheler, R., Kromer, B., Bjorck, S., Svensson, A., Friedrich, M., Kaiser, K. F., et al. (2008). Tree rings and ice cores reveal 14C calibration uncertainties during the Younger Dryas. Nat. Geosci. 4, 263–276.
Opitz, S., Zhang, C. J., Herzschuh, U., and Mischke, S. (2015). Climate variability on the south-eastern Tibetan Plateau since the Lateglacial based on a multiproxy approach from Lake Naleng Co comparing pollen and non-pollen signals. Quat. Sci. Rev. 115, 112–122. doi: 10.1016/j.quascirev.2015.03.011
Reimer, P. J., Bard, E., Bayliss, A., Beck, J. W., Blackwell, P. G., Bronk Ramsey, C., et al. (2013). IntCal13 and Marine13 radiocarbon age calibration curves 0-50,000 years cal BP. Radiocarbon 55, 1869–1887. doi: 10.2458/azu_js_rc.55.16947
Ren, G. Y. (1998). A finding of the influence of hard water on radio carbon dating for lake sediments in inner Mongolia, China. J. Lake Sci. 10, 80–82. (in Chinese), doi: 10.18307/1998.0314
Shen, J., Liu, X. Q., Wang, S. M., and Ryo, M. (2005). Palaeoclimatic changes in the Qinghai Lake area during the last 18,000 years. Quat. Int. 136, 131–140. doi: 10.1016/j.quaint.2004.11.014
Shen, Y. P., Liu, G. X., Shi, Y. F., and Zhang, P. Z. (1996). Climate and environment in the Tibetan Plateau during the younger Dryas cooling event. J. Glaciol. Geocryol. 18, 219–226. (in Chinese).
Song, C. Q., Wang, B. Y., and Sun, X. J. (1996). Implication of paleovegetational changes in Diaojiao lake, Inner Mongolia. Acta Bot. Sin. 38, 568–575. (in Chinese).
Sun, Q. L., Wang, S. M., Zhou, J., Shen, J., Cheng, P., Xie, X. P., et al. (2009). Lake surface fluctuations since the late glaciationat Lake Daihai, North central China: a direct indicator of hydrological process response to East Asian monsoon climate. Quat. Int. 194, 45–54. doi: 10.1016/j.quaint.2008.01.006
Talbot, M. R. (1990). A review of the palaeohydrological interpretation of carbon and oxygen isotopic ratios in primary lacustrine carbonates. Chem. Geol. 80, 261–279. doi: 10.1016/0168-9622(90)90009-2
Tatzber, M., Stemmer, M., Spiegel, H., Katzlberger, C., Haberhauer, G., and Gerzabek, M. N. (2007). An alternative method to measure carbonate in soils by FI-IR spectroscopy. Environ. Chem. Lett. 5, 9–12. doi: 10.1007/s10311-006-0079-5
Valero-Garces, B., Kelts, K., and Ito, E. (1995). Oxygen and carbon isotope trends and sedimentological evolution of a meromictic and saline lacustrine system: the Holocene Medicine Lake Basin, North American Great Plains, USA. Palaeogeogr. Palaeoclimatpl. Palaeoecol. 17, 253–278. doi: 10.1016/0031-0182(94)00136-v
Wang, H. Y., Li, H. Y., Liu, Y. H., and Cui, H. T. (2004). Mineral magnetism of lacustrine sediments and Holocene palaeoenvironmental changes in Dali Nor area, southeast Inner Mongolia Plateau, China. Palaeogeogr. Palaeoclimatol. Palaeoecol. 208, 175–193. doi: 10.1016/j.palaeo.2004.02.026
Wang, P. F. (1992). Preliminry study on the environmental changes of Hulun Buir Sandy Land since the Holocene. J. Desert Res. 12, 13–19. (in Chinese).
Wang, S. M., Ji, L., Yang, X. D., Xue, B., Ma, Y., and Hu, S. Y. (1994). The record of younger Dryas event in lake sediments from Jalai Nur, Inner Mongolia, China. Sci. Bull. 39, 831–835.
Wang, Y. J., Cheng, H., Edwards, R. L., An, Z. S., Wu, J. Y., Shen, C. C., et al. (2001). A high-resolution absolute-dated Late Pleistocene monsoon record from Hulu Cave, China. Science 294, 2345–2348. doi: 10.1126/science.1064618
Wen, R. L., Xiao, J. L., Chan, Z. G., Zhai, D. Y., Xu, Q. H., Li, Y. C., et al. (2010). Holocene climate changes in the mid-high-latitude-monsoon margin reflected by the pollen record from Hulun Lake, northeastern Inner Mongolia. Quat. Res. 73, 293–303. doi: 10.1016/j.yqres.2009.10.006
Winkler, M. G., and Wang, P. K. (1993). “The late Quaternary vegetation and climate of China,” in Global Climates Since the Last Glacial Maximum, ed. H. E. Wright (Minneapolis, MN: University of Minnesota Press), 221–261.
Wu, J. L., and Wang, S. M. (1997). Carbonate δ18O- and δ13C-recorded environmental changes in RM lake core in Zoige of the eastern Tibetan Plateau. Mar. Geol. Quat. Geol. 17, 63–71. (in Chinese).
Xiao, J. L., Fan, J. W., Zhou, L., Zhai, D. Y., Wen, R. L., and Qin, X. G. (2013). A model for linking grain-size component to lake level status of a modern clastic lake. J. Asian Earth Sci. 69, 149–158. doi: 10.1016/j.jseaes.2012.07.003
Xiao, J. L., Nakamura, T., Lu, H. Y., and Zhang, G. Y. (2002). Holocene climate changes over the desert/loess transition of north-central China. Earth Planet. Sci. Lett. 197, 11–18. doi: 10.1016/s0012-821x(02)00463-6
Xiao, J. L., Xu, Q. H., Nakamura, T., Yang, X. L., Liang, W. D., and Inouchi, Y. (2004). Holocene vegetation variation in the Daihai Lake region of north-central China: a direct indication of the Asian monsoon climatic history. Quat. Sci. Rev. 23, 1669–1679. doi: 10.1016/j.quascirev.2004.01.005
Yang, X. D., and Wang, S. M. (1996). The vegetation and climatic-environmental changes in Hulun Lake during Holocene. Oceanol. Limnol. Sin. 27, 67–72. (in Chinese).
Yang, X. D., Wang, S. M., and Xue, B. (1995). Vegetational development and environmental changes in Hulun Lake since Late Pleistocene. Acta Palaeontol. Sin. 34, 647–656. (in Chinese). doi: 10.19800/j.cnki.aps.1995.05.008
Yang, Y., Yuan, D. X., Cheng, H., Zhang, M. L., Qin, J. M., Lin, Y. S., et al. (2010). Precise dating of abrupt shifts in the Asian monsoon during the last deglaciation based on stalagmite data from Yamen Cave, Guizhou Province, China. Sci. China Earth Sci. 53, 633–641. doi: 10.1007/s11430-010-0025-z
Yang, Z. R. (1998). A study on the low temperature fluctuations since the Holocene in Diaojiaohaizi lake area, Daqingsha mountains, Inner Mongolia. Geogr. Res. 17, 138–144. (in Chinese). doi: 10.11821/yj1998020005
Yang, Z. R., Shi, P. J., and Fang, X. Q. (1997). Preliminary study on paleovegetation and environmental changes since 11ka B.P. in Diaojiaohaizi lake area, Daqingmountains, Inner Mongolia. Acta Phytoecol. Sin. 21, 551–563. (in Chinese).
Yang, Z. R., and Suo, X. F. (1996). Preliminary study on relations between human activities and environment in framing-animal husbandry zigzag zone of north China. J. Beijing Norm. Univ. Nat. Sci. 32, 415–420. (in Chinese).
Yu, Z. T., Liu, X. Q., Wang, Y., Chi, Z. Q., Wang, X. J., and Lan, H. Y. (2014). A 48.5-ka climate record from Wulagai Lake in Inner Mongolia, Northeast China. Quat. Int. 333, 13–19. doi: 10.1016/j.quaint.2014.04.006
Zeng, Y., Chen, J., Xiao, J., and Qi, L. (2013). Non-residual Sr of the sediments in Daihai Lake as a good indicator of chemical weathering. Quat. Res. 79, 284–291. doi: 10.1016/j.yqres.2012.11.010
Zhai, Q. M., Qiu, W. L., Li, R. Q., Zhao, Y., and Zheng, L. M. (2000). The middle and late Holocene lacustrine sediments and its climate significance of Angulinao -Bojianghaizi lakes, Inner Mongolia. J. Palaeogeogr. 2, 84–91. (in Chinese).
Zhang, C. J., Cao, J., Lei, Y. B., and Shang, H. M. (2004). The chronological characteristics of bosten lake Holocene sediment environment in Xinjiang, China. Acta Sedimentol. Sin. 22, 494–499. (in Chinese). doi: 10.1007/BF02873097
Zhang, C. J., Dembele, B., Zhang, W. Y., Zhang, J. Y., Wang, H. S., Gang, E., et al. (2018). The low lake-level record according to the Selin Co stratigraphical basis and multi-proxies during the last glacial maximum in the central Tibetan Plateau. Acta Geol. Sin. 92, 2058–2059. doi: 10.1111/1755-6724.13707
Zhang, C. J., Fan, R., Li, J., Mischke, S., Dembele, B., and Hu, X. L. (2013). Carbon and oxygen isotopic compositions: how lacustrine environmental factors respond in Northwestern and Northeastern China. Acta Geol. Sin. 87, 1344–1354. doi: 10.1111/1755-6724.12133
Zhang, C. J., Mischke, S., Zheng, M. P., Prokopenko, A., Guo, F. Q., and Feng, Z. D. (2009). The carbon and oxygen isotopic composition of surface-sediment carbonate in Bosten Lake (Xinjiang, China) and its controlling factors. Acta Geol. Sin. 2, 386–395. doi: 10.1111/j.1755-6724.2009.00029.x
Zhang, C. J., Zhang, W. Y., Feng, Z. D., Mischke, S., Gao, X., Gao, D., et al. (2012). Holocene hydrological and climatic change on the northern Mongolian Plateau based on multi-proxy records from Lake Gun Nuur. Palaeogeogr. Palaeoclimatol. Palaeoecol. 32, 75–86. doi: 10.1016/j.palaeo.2012.01.032
Zhang, H. R., Dembele, B., Zhang, W. Y., Zhang, J. Y., Ma, Y., and Zhang, C. J. (2018). The dry-cold climate of the Qijia archeological civilization in Chankou of the Loess Plateau along the silk road since the Neolithic Period. Acta Geol. Sin. Res. Adv. 92, 2466–2467.
Zhang, Z. K., and Wang, S. M. (2000). Paleoclimate significance of lake level fluctuation, peat development and Eolian Sand-paleosoil series in Hulun Lake Area during Past 13ka. J. Arid Land Resour. Environ. 14, 56–59. (in Chinese). doi: 10.3969/j.issn.1003-7578.2000.03.009
Zhao, Y., Yu, Z. C., Chen, F. H., and Li, J. (2008). Holocene vegetation and climate change from a lake sediment record in the Tengger Sandy Desert, northwest China. J. Arid Environ. 72, 2054–2064. doi: 10.1016/j.jaridenv.2008.06.016
Zheng, Y. E., Zhou, L. P., and Zhang, J. F. (2010). Optical dating of the upper 22m of cored sediments from Daihai Lake, northern China. Quat. Geochronol. 5, 228–232. doi: 10.1016/j.quageo.2009.05.010
Zhou, W. J., Head, M. J., and Deng, L. (2001). Climate changes in northern China since the late Pleistocene and its response to global change. Quat. Int. 83-85, 285–292. doi: 10.1016/s1040-6182(01)00046-5
Keywords: Tengger Nuur, Holocene, stratigraphy, weak summer monsoon, effective humidity
Citation: Chengjun Z, Li Z, Wanyi Z, Yunhan T, Yang L, Xiangling W, Zhen Z and Khomid S (2020) Lake-Level Oscillation Based on Sediment Strata and Geochemical Proxies Since 11,000 Year From Tengger Nuur, Inner Mongolia, China. Front. Earth Sci. 8:314. doi: 10.3389/feart.2020.00314
Received: 24 March 2020; Accepted: 03 July 2020;
Published: 07 August 2020.
Edited by:
Liangcheng Tan, Institute of Earth Environment, Chinese Academy of Sciences, ChinaReviewed by:
Hao Long, Nanjing Institute of Geography and Limnology (CAS), ChinaQianli Sun, East China Normal University, China
Copyright © 2020 Chengjun, Li, Wanyi, Yunhan, Yang, Xiangling, Zhen and Khomid. This is an open-access article distributed under the terms of the Creative Commons Attribution License (CC BY). The use, distribution or reproduction in other forums is permitted, provided the original author(s) and the copyright owner(s) are credited and that the original publication in this journal is cited, in accordance with accepted academic practice. No use, distribution or reproduction is permitted which does not comply with these terms.
*Correspondence: Zhang Chengjun, cjzhang@lzu.edu.cn