- 1State Key Laboratory of Eco-hydraulics in Northwest Arid Region of China, Xi’an University of Technology, Xi’an, China
- 2Ningxia Capital Sponge City Construction & Development Company Limited, Guyuan, China
- 3Key Laboratory of National Forestry Administration on Ecological Hydrology and Disaster Prevention in Arid Regions, Xi’an University of Technology, Xi’an, China
Changes in soil moisture following the establishment of sponge cities play a key role in the regulation of the relationship between surface runoff and rain resources in arid and semi-arid regions of the Loess Plateau. Based on soil moisture and rainfall monitoring data obtained at the fine-scale (per 10-cm depth and hourly), temporal and spatial variation in soil moisture under different sponge measures and their responses to rain events were analyzed, in addition to water quality changes were investigated by water sampling. The results showed, from 2000 to 2018, the area of farmland greatly decreased from 56.88 to 5.02% in Guyuan, while the area of construction land increased from 29.24 to 45.96%. The area of changes in Guyuan accounted for 63.68% of the total area. Precipitation in July and August was large, and accounted for 19.11 and 23.24% of the multi-year average precipitation, respectively. The grasslands and sunken green spaces exhibited good water retention effects, with average soil moisture of 14.40 and 13.77% during the study period, and 18.48 and 15.52% during the rain event, respectively. During the rain event, the total nitrogen (TN), ammonia nitrogen (NH3-N), available phosphorus (AP), and total phosphorus (TP) can be effectively reduced by the sunken green spaces, with average concentrations of 0.79, 0.28, 0.03, and 0.05 mg/L, respectively. The development of sponge measures could improve the urban ecological environment and hydrological conditions in the Loess Plateau, and increase the potential utilization of urban rainwater resources.
Introduction
With the implementation of the reform and opening up policy in China, remarkable social and economic developments have been observed, and urbanization rate increased from 17.55% in 1977 to 57.35% in 2016 (Chen, 2007; Liu and Luk, 2009; Wang et al., 2017a). Urbanization has altered urban hydrological conditions and floods in urban spaces have become widespread in China (Zheng et al., 2013; Hu et al., 2015, Lyu et al., 2017, 2018a,b; Hu et al., 2018). Heavy rains and floods hit about 200 cities annually in China (Yin et al., 2015; Sang and Yang, 2017; Lyu et al., 2018c). On July 21 2012, in Beijing, 79 people died following heavy rains (Yu and Liu, 2015). Wuhan in China often has substantial losses following inundation in the rainy season (Lyu et al., 2018a). Therefore, the construction of a sponge city was proposed in 2012, and the concept of low impact development was adhered to in the development of sponge cities in China (Xia et al., 2017; Yuan et al., 2017; Wang et al., 2017b). Regulating urban hydrological processes and increasing the storage capacity of urban rainwater are key goals in the development of sponge cities, and since 2015, a pilot sponge city is being constructed in China (Sang and Yang, 2017; Xia et al., 2017).
Sponge city measures include planting grasslands, establishing grass ditches and sunken green spaces, and other measures (Shafique and Kim, 2015). By adopting sponge measures, sources of flood runoff could be controlled, and the hydrological characteristic of the city surface was changed by regulating soil moisture using different sponge measures (Wang et al., 2009, 2017a). Water could also be accumulated and redistributed, which was as a way of regulating urban hydrological processes, to reduce floods and to improve urban water cycling. Therefore, it is critical to investigate the changes in soil hydrological characteristics that occur following the construction of sponge cities.
The water collected and stored in the soil is the result of the combined effects of precipitation infiltration, redistribution, evaporation, and water uptake by plant roots (Heathman et al., 2009; Chaney et al., 2015; Hou et al., 2015; Xu et al., 2017; Xiao et al., 2019). Soil water content influences penetration strongly, and the depth of penetrating decreases dramatically with increasing of water content (Njoku and Rague, 1996). At present, in order to explore the impact of low-impact development measures on hydrological process, numerous studies have been carried out to analyze the changing characteristics of soil moisture and to explore the impact of low-impact development strategies on water quality. Xu et al. (2016) have shown that soil depth influences the spatial and temporal distribution of soil water content significantly. Studies by Davis et al. (2009) have shown that bioretention measures such as rainwater gardens reduce runoff and flood peaks considerably, while storing rain. De Busk and Wynn (2011) observed that bioretention could reduce 97 to 99% of surface runoff, and Ahiablame and Shakya (2016) observed that different sponge measures reduced urban flooding significantly. In addition, Liu et al. (2016) and others have shown that the stable infiltration rate of a sunken greens spaces ranged between 0.5 and 2.3 mm/min. Furthermore, Wang et al. (2012) showed the runoff that can be accommodated by the water grass ditches is 2.16 times that of the hard road. The above studies have reported some effects of sponge measures on runoff and hydrological processes. However, only few studies have explored spatial and temporal variation of soil moisture and water quality under different sponge measures, particularly in the course of rain events. Therefore, it is necessary to study the hydrological characteristics of soil water and water quality under different sponge measures, and the responses during rain events.
As the largest and deepest loess deposit in the world, the Loess Plateau covers an area of 640,000 km2 (Fu et al., 2017). Most of the plateau is located in semi-arid zone with low precipitation. The Loess Plateau is an area associated with soil erosion and drought, and its ecological environment is very fragile. In recent years, urbanization activities in the Loess Plateau have increased, accompanied by the frequent extreme precipitation events. Due to the collapsible characteristics of the Loess Plateau, the damage caused by urban flood is more severe than in other areas. In April 2016, Guyuan city located in the western part of China’s Loess Plateau became the second pilot city among the 16 declared sponge cities in China. Assessing the impact of sponge city construction on soil moisture and water quality in cities in the Loess Plateau could reveal some rules that could facilitate the improvement of the ecological environment in the Loess Plateau.
Therefore, the present study analyzed the effects of sponge city measures in Guyuan city on the Loess Plateau, on soil moisture changes and water quality changes. The purpose of the present study is to: (1) analyze land-use change processes in the course of urbanization in Guyuan city; (2) quantitatively analyze the temporal and spatial changes of soil water content in the soil profile under different sponge measures and the response to rain events; and (3) evaluate impacts of different sponge measures during a rain event on water quality.
Materials and Methods
Study Area
Guyuan city is located on the bank of Qingshui River to the north of Liupan Mountain on the Loess Plateau (Figure 1). The terrain is high in the south and low in the north. The coordinates are 36°00′N∼106°16′E and the altitude is 1753 m. It falls under the warm temperate semi-arid climate zone. It has drought and little precipitation, and the climate is a typical mid-temperate continental climate. The multi-year average temperature is 5°C and the multi-year average precipitation is 438.5 mm. The annual precipitation varied greatly among years, and mainly concentrated in June to September, accounting for 79.37% of the multi-year average precipitation. The rain events are short, water resources are scarce. The soil in the Loess Plateau is collapsible. The vegetation is dominated by Larix principis-rupprechtii and Hippophae salicifolia. The study site is located in the Rose Garden Community in the western part of Yuanzhou district of Guyuan city. The sponges in the study area are covered with water grass ditches, sunken green spaces, and grasslands.
Rainfall and Soil Moisture Monitoring
Three soil moisture monitoring tubes (ET100-Pro, Insentek, Beijing, China) were installed in the middle of the three different sponge measures, including water grass ditches, grasslands, and sunken green spaces, to monitor changes in soil moisture content. Volumetric soil moisture was obtained at 10-cm intervals to a depth of 60 cm using each moisture monitoring tube from August 30, 2018, to August 29, 2019. The soil layer from the top to the bottom was divided into six layers at equal intervals, and soil moisture content at each layer was measured every hour.
Since the precipitation in Guyuan was concentrated in July and August, the present study monitored the rain events from 00:00 on July 16, 2019, to 24:00 on August 29, 2019. In addition, rain event was monitored from 10:00 on July 21, 2019, to 22:00 on July 21, 2019, and the rainfall data were recorded every 5 min. Rainfall data were obtained from the Guyuan weather station.
Water Quality Analysis
The total nitrogen (TN) content, ammonia nitrogen (NH3-N) content, nitrate-nitrogen (NN) content, total phosphorus (TP) content, and available phosphorus (AP) content of the water samples were determined using an automatic Kjeldahl apparatus (Kjeltec 8400, FOSS Analytical, Hillerød, Denmark).
Water samples were collected from the three sponge measures at 21 time points during a rain event from 10:00 on July 21, 2019, to 22:00 on July 21, 2019 (Figure 2). The time distribution of sampling points is relatively uniform, and changes in water quality during rain event can be captured. The first sample was obtained at 12:45 on July 21, 2019, and the last sample at 18:30 on July 21, 2019. During the sample collection, three replicates were collected for each sample, and water quality indicators such as TN, TP, NH3-H, NN, and AP were tested in each sample.
Results
Land-Use Change
In 2000, 2010, and 2018, there were mainly seven land-use in Guyuan city, including road, construction land, forestland, farmland, grassland, water system land, and unutilized land, as shown in Figures 3A–C. Construction land, farmland, and forestland were the main types of land-use in Guyuan in 2000, 2010, and 2018. The proportion of construction land in Guyuan increased from 29.24% in 2000 to 45.96% in 2018, increasing by 0.93% per year. The proportion of farmland decreased from 56.88% in 2000 to 5.02% in 2018, which was mainly observed in the central and northern parts of Guyuan city. Similarly, the area of water system land decreased slightly, and the areas of the other land-use exhibited increasing trends. The land use change area accounts for 63.68% of the total area, mainly in the central and northern regions from 2000 to 2018 (Figure 3D).
Variation in Precipitation and Rainfall
The monthly changes in precipitation in Guyuan city from 1985 to 2015 were shown in Figure 4. From 1985 to 2015, maximum annual precipitation and minimum annual precipitation are 703.2 mm and 313.9 mm, respectively. And the average annual precipitation was 438.5 mm. The coefficient of variation of annual precipitation reaches 0.21, and the inter-annual variability of annual precipitation was weak. Rainfall is the main form of precipitation during the flood season from June to October. Maximum monthly rainfall and minimum monthly rainfall are 285 mm and 0 mm. And the proportion of rainfall in August and July of the total annual precipitation were the greatest, in the following proportion: 23.24 and 19.11%.
The hourly rainfall from 00:00 on July 16, 2019, to August 29, 2019 in Guyuan was shown in Figure 5A. The total rainfall was 133.87 mm, accounting for 30.53% of the multi-year average precipitation. The rain event from 10:00 on July 21, 2019 to 22:00 on July 21, 2019 were shown in Figure 5B. The rain event began falling at 10:15 on July 21, 2019, and ended at 18:45. It lasted for 510 min and lasted nearly 9 h. The total rainfall was 13.63 mm and the average rain intensity was 0.03 mm/min.
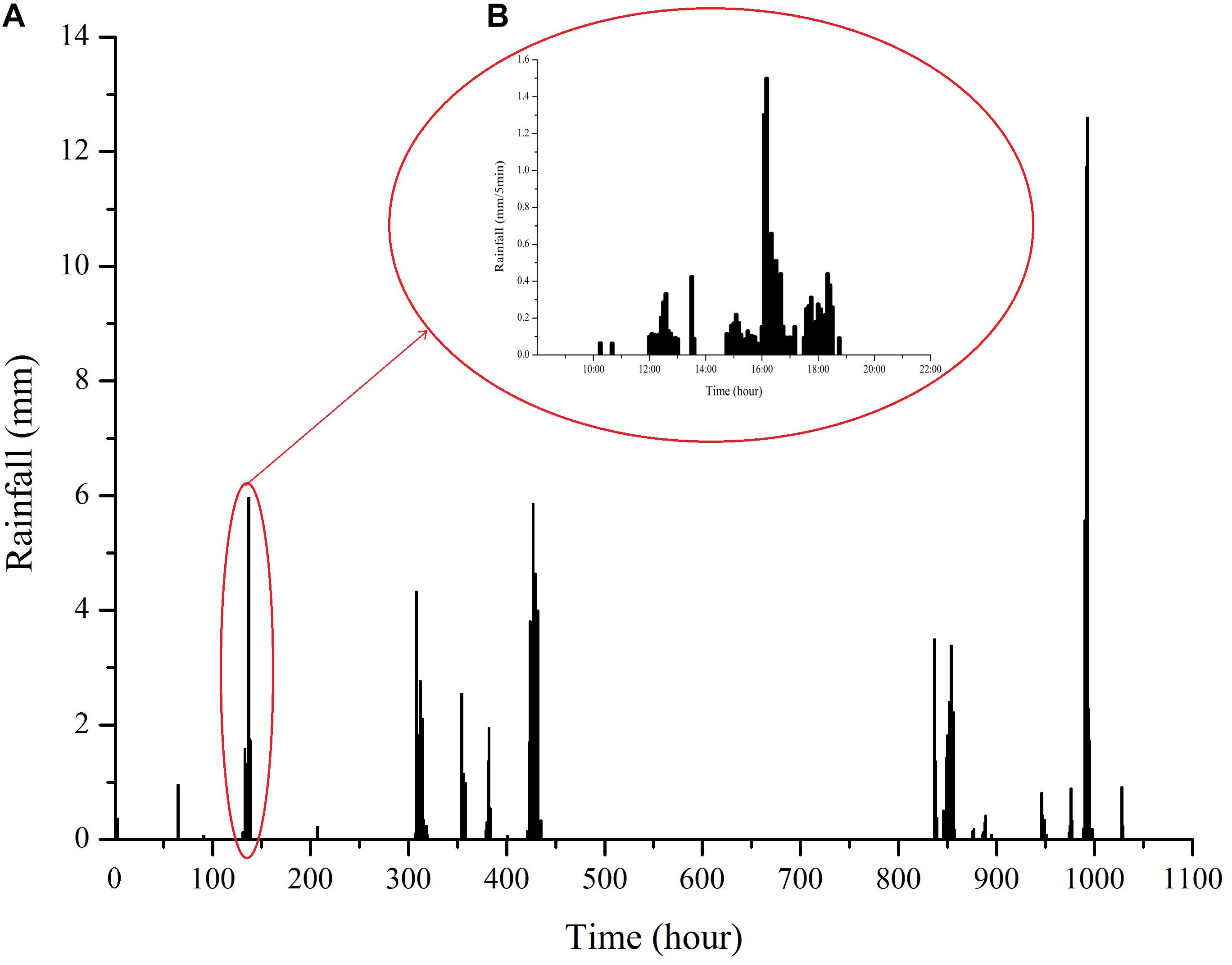
Figure 5. Hourly rainfall from 1:00 on July 16, 2019 to 24:00 on August 29, 2019 in Guyuan City (A); From 10:00 to 22:00 on July 21, 2019, every 5 min rainfall of the rain event in Guyuan City (B).
Temporal and Spatial Changes in Soil Moisture Under Different Sponge Measures
The changes in average soil moisture at different soil depths under three types of sponge measures, such as grasslands, sunken green spaces, and water grass ditches in Guyuan, as shown in Figure 6. During the monitoring period, the average soil moisture in water grass ditches varied with no clear pattern with an increase in soil depth, and the average value was stable at 4.80%. The average soil moisture in the grasslands and the sunken green spaces increased with an increase in soil depth, with average values of 14.40 and 13.77%, respectively.
The monthly average soil moisture under the three sponge measures over the 12-month monitoring period was shown in Figure 7. The average monthly soil moisture under the three sponge measures were 14.35, 4.78, and 13.74%, respectively. The monthly average soil moisture in the grasslands and sunken green spaces increased significantly from April to December. Compared with water grass ditches, average soil moisture in the grasslands and the sunken green spaces varied more greatly.
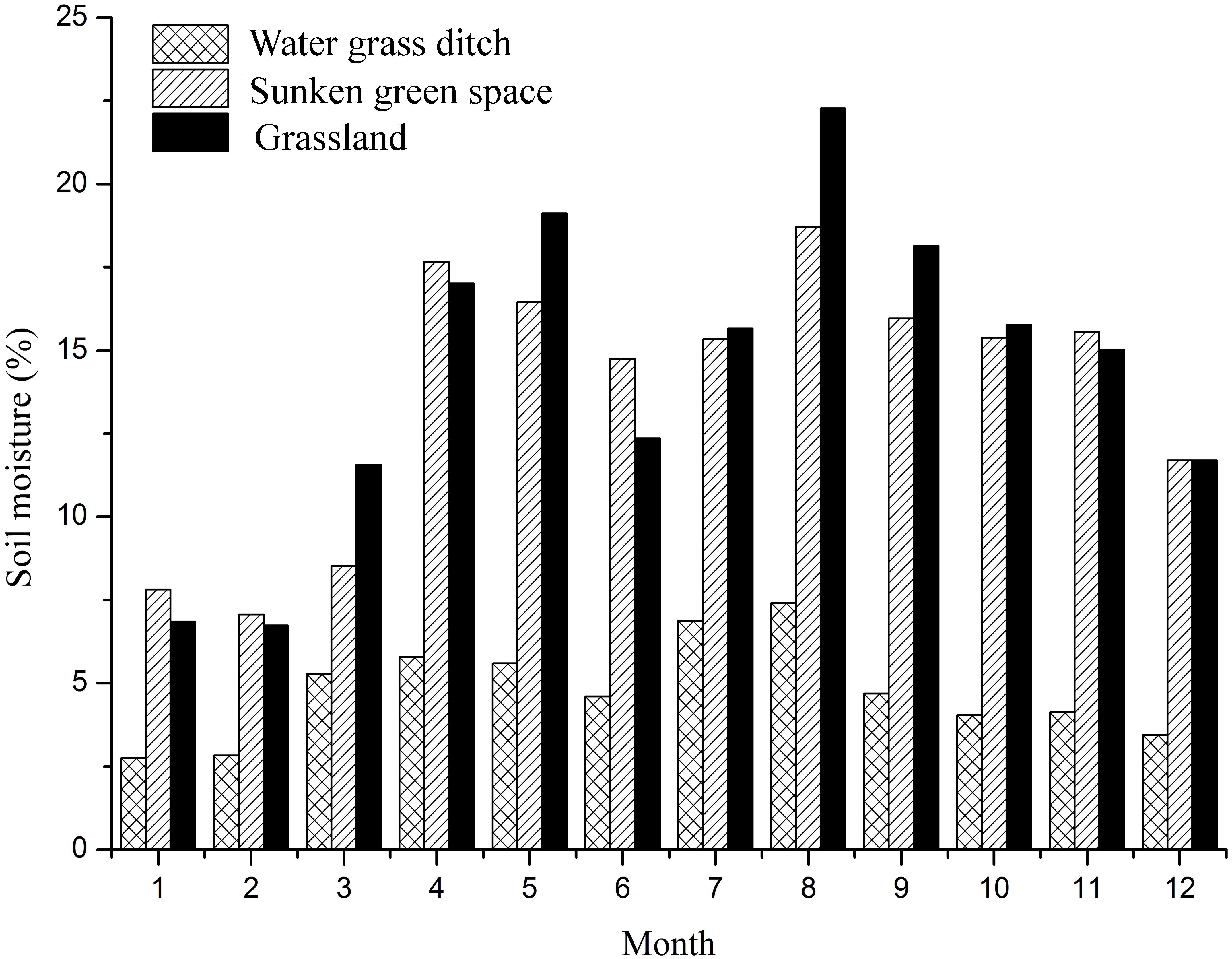
Figure 7. Changes of average soil moisture content in different months under different sponge measures.
Figure 8 showed the variations in average soil moisture in grasslands, water grass ditches, and sunken green spaces on the process of the rain event. The average soil moisture under the three sponge measures exhibited increasing trends with an increase in rainfall duration. The rainfall peaked at the 7th hour, and the average soil moisture in the water grass ditches also increased significantly. However, the average soil moisture in the grassland and the sunken green space increased at the 8th hour, and the response to rainfall was delayed. When the rain event was end, the average soil moisture in the water grass ditches began to decrease, but the average soil moisture in the grassland and the sunken green space continued to increase. During the entire duration of rain event, the average soil water in the grassland, water grass ditches, and the sunken green space were 18.48, 11.43, and 15.52%, respectively.
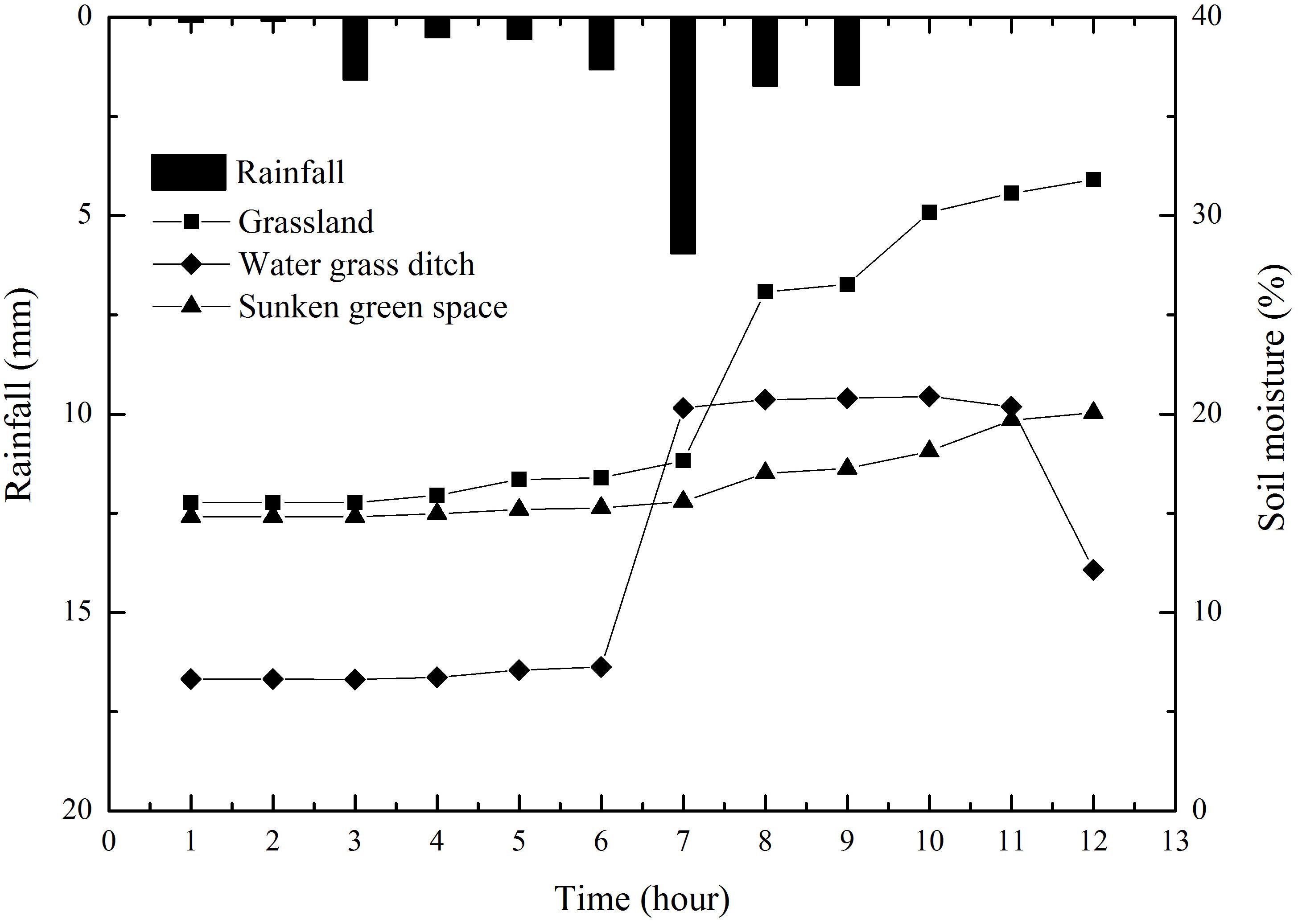
Figure 8. Changes of average soil moisture content under different sponge measures during the rain event.
Water Quality Change Under Different Sponge Measures
During the rain event, there were obvious differences in the five water quality indicators among the three sponge measures (Figure 9). The average TN concentration in the water was the highest under three sponge measures, such as grassland, water grass ditch and sunken green space, reaching 1.07, 0.93, and 0.79 mg/L, respectively. Except for TN, compared with other three water quality indicators, the average concentration of NH3-H was also higher. The average concentration of TN and NH3-H in the water under the grassland were the highest, compared with the water grass ditch and sunken green space. Among the five water quality indicators, the average concentration of NN in the water under grassland was the lowest, and the average concentration of NN in the water under the water grass ditch and sunken green space was much higher than that of grassland. However, the average concentration of AP in the water was the lowest under the water grass ditch and sunken green space, but the average concentration of AP in the water in the grassland was higher. Compared with water grass ditch and grassland, the average concentration of TN, NH3-H, AP, and TP in the water under the sunken green space was the lowest, with concentrations of 0.79, 0.28, 0.03, and 0.05 mg/L, respectively.
Discussion
Temporal and Spatial Differences in Soil Moisture Content Under Different Sponge Measures
Increasing use of impermeable land-uses, such as building land and rural roads, would affect the spatial and temporal changes of soil hydrological processes. The construction of sponge measures can regulate soil hydrological processes. There were differences in soil moisture under different sponge measures (Rosenbaum et al., 2012). Soil moisture was higher in the three sponge measures in summer and autumn compared to spring and winter (Figure 10), and this may be affected by rainfall, which was similar to the results of previous studies (Liu and Shao, 2014; Wang et al., 2015; Liao et al., 2017; Xu et al., 2017). The soil moisture content in the water grassland ditches in the four seasons was not high and fluctuated with an increase in soil depth. The cause of the phenomenon was probably due to the better drainage of the water grassland ditches. Soil moisture in the sunken green spaces and grassland increased with an increase in soil depth, which was more pronounced in fall, which may be mainly because time changes in soil moisture were affected by climatic factors (such as precipitation and temperature; Entin et al., 2000; Koster et al., 2004).
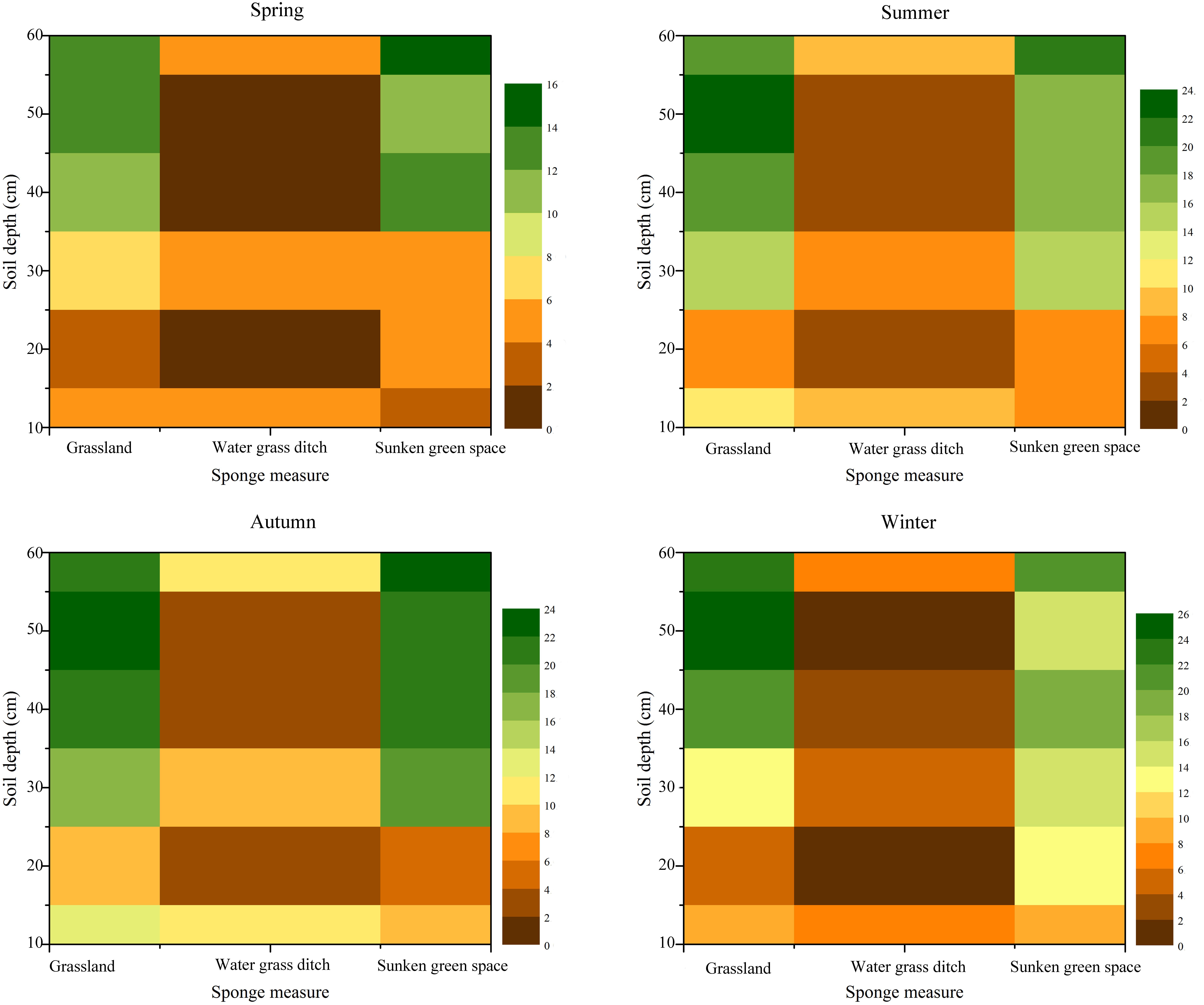
Figure 10. Seasonal differences in soil moisture under different soil depths under different sponge measures.
At a depth of 10 cm, the soil moisture content under all the three sponge measures increased gradually with an increase in the duration of rain event (Figure 11A). Soil moisture was strongly affected by topographic factors (Lin et al., 2006). Root distribution had a strong influence on soil moisture of the top soil profile (Jia et al., 2017; Xu et al., 2017). When the rainfall peaked at the 7th hour, the soil moisture increased significantly under all the three sponge measures, and the changes in soil moisture in the grasslands were the most notable. This may be related to the vegetation cover of the grassland, because the grassland vegetation there was sparse and not high. At 30, 40, 50, and 60 cm soil depth, the soil moisture in the grasslands and sunken green spaces were very high, which may be because the deep soil moisture was relatively stable (He et al., 2012), which was also related to the magnitude of rain events, as shown in Figures 11C–F (Heisler-White et al., 2008).
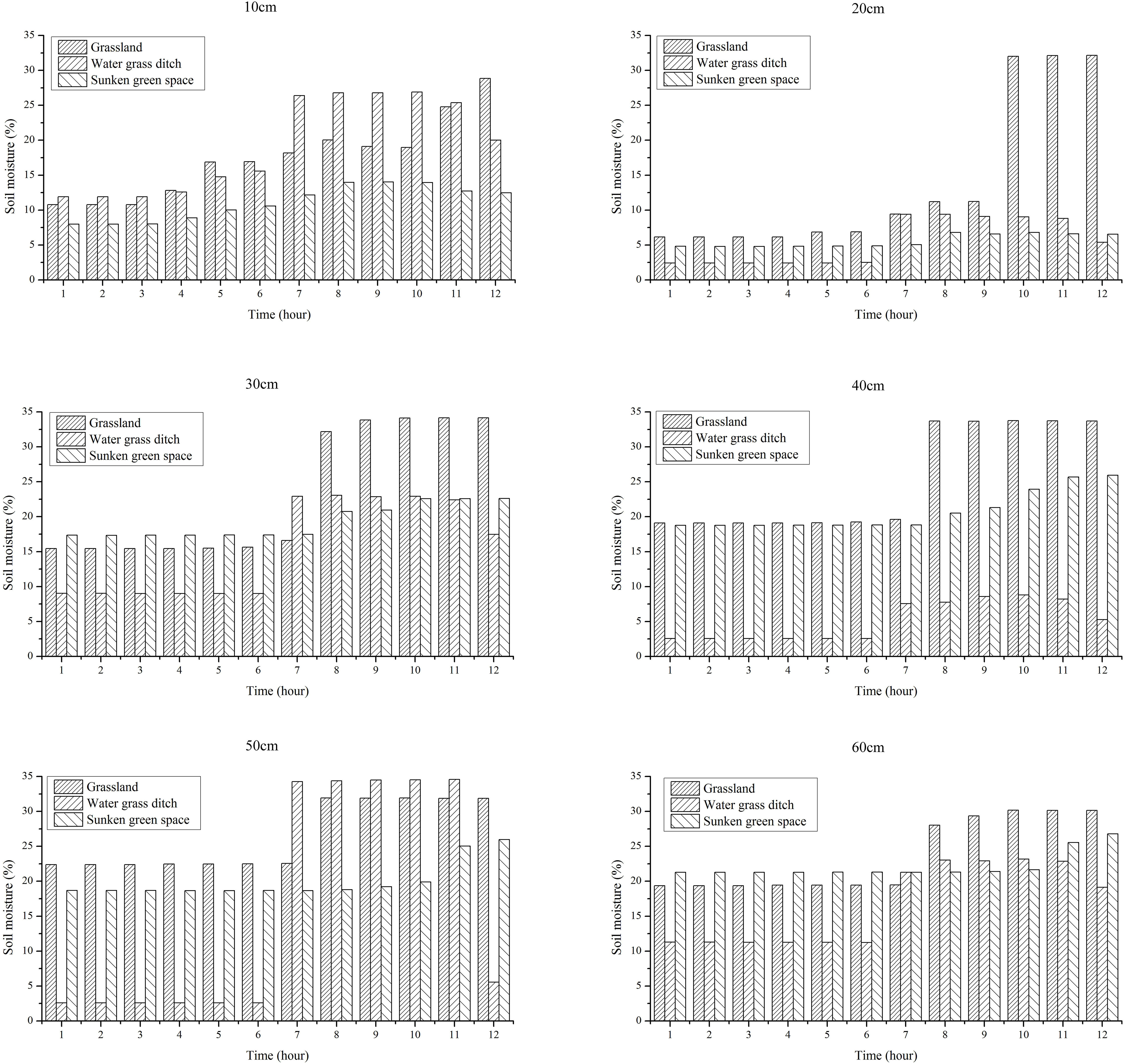
Figure 11. Soil moisture at different soil depths under different sponge measures during the rain event.
Potential Uses of Rain Resources Following the Construction of a Sponge City in Guyuan
One of the main purposes of sponge city development was to control water quality and water recycling, to increase the potential of rainwater resources, and to make better use of rain resources (Matteo et al., 2019; Nguyen et al., 2019). During the construction of the sponge city in Guyuan, the implementation of different sponge measures had affected urban water resources considerably, particularly with regard to soil moisture and water quality in time and space. The implementation of different sponge measures had also influenced the distribution of soil moisture in space and time. The water-holding capacity of the sunken green spaces and the grasslands was significantly higher than that of the water grass ditches. The grassland was very sensitive to rainfall. Similarly, water quality was affected variably. The sunken green spaces reduced the TP and AP in the water significantly, as shown in Figure 9. The changes observed after the implementation of the sponge measures imply that the rainwater resources had different potential uses. A portion of the recovered rain resources could be used to alleviate water shortages in cities and to improve urban ecology (Su et al., 2019). Figures 12A–C showed the changes in water quality before maximum rainfall intensity, at maximum rainfall intensity, and after maximum rainfall intensity. At different stages of the rainfall event, the sunken green spaces could always decrease the TP and AP concentrations. Therefore, water purification could be achieved by establishing sunken green spaces in the city. In addition, the establishment of grasslands and sunken green spaces may facilitate water conservation, surface runoff reduction, and flood risk mitigation in case of heavy rain (Mehrabadi et al., 2013; Mahmoud et al., 2014; Karim et al., 2015).
Conclusion
With the increase in urbanization in Guyuan, the permeable surfaces in the form of farmland had been decreasing, and the construction land had been increasing. Due to the unique climatic conditions of the Loess Plateau of China, the precipitation during the year was concentrated in July and August, and the soil moisture in the soil layer increased significantly during the July-August rainy season. Grasslands, water grass ditches, and sunken green spaces had been established during the development of the sponge city. When rain events occurred, the grasslands and the sunken green spaces could conserve water, simultaneously, the sunken green spaces could purify the water. The development of sponge cities in the Loess Plateau in China could increase the value of rainwater resources and their potential applications.
Data Availability Statement
All datasets generated for this study are included in the article/supplementary material.
Author Contributions
LJ designed experiments, analyzed the results, and wrote manuscripts. GX, ZL, and PL providing experimental funding and equipment. MH, ZZ, BW, YZ, JZ, and YC conducted experiments.
Funding
This research was supported by the National Key Research and Development Program (2016YFC0402404), the National Natural Science Foundations of China (No. 51979219), Shaanxi Province Innovation Talent Promotion Plan Project Technology Innovation Team (No. 2018TD-037), and the Research Program for Key Technologies of Sponge City Construction and Management in Guyuan City (Grant NO. SCHM-2018-0103).
Conflict of Interest
MH was employed by the company Ningxia Capital Sponge City Construction & Development Company Limited, Guyuan, China.
The remaining authors declare that the research was conducted in the absence of any commercial or financial relationships that could be construed as a potential conflict of interest.
Acknowledgments
In addition, we thank the reviewers for their useful comments and suggestions.
References
Ahiablame, L., and Shakya, R. (2016). Modeling flood reduction effects of low impact development at a watershed scale. J. Environ. Manage. 171, 81–91. doi: 10.1016/j.jenvman.2016.01.036
Chaney, N. W., Roundy, J. K., Herrera-Estrada, J. E., and Wood, E. F. (2015). High-resolution modeling of the spatial heterogeneity of soil moisture: applications in network design. Water Resour. Res. 51, 619–638. doi: 10.1002/2013wr014964
Chen, J. (2007). 2008 Economic Blue Book on China’s Economic Situation Analysis and Prediction. Beijing: Social Sciences Documents Publishing House.
Davis, A. P., Hunt, W. F., Traver, R. G., and Clar, M. (2009). Bioretention technology: overview of current practice and future needs. J. Environ. Eng. 135, 109–117. doi: 10.1061/(asce)0733-9372(2009)135:3(109)
De Busk, K., and Wynn, T. (2011). Storm-water bioretention for runoff quality and quantity mitigation[J]. J. Environ. Eng. 137, 800–808. doi: 10.1061/(asce)ee.1943-7870.0000388
Entin, J. K., Robock, A., Vinnikov, K. Y., Hollinger, S. E., Liu, S., and Namkai, A. (2000). Temporal and spatial scales of observed soil moisture variations in the extratropics. J. Geophys. Res. 105, 865–877.
Fu, B. J., Wang, S., Liu, Y., Liu, J. B., Liang, W., and Miao, C. Y. (2017). Hydrogeomorphic ecosystem responses to natural and anthropogenic changes in the Loess Plateau of China. Annu. Rev. Earth Planet. Sci. 45, 223–243. doi: 10.1146/annurev-earth-063016-020552
He, B. S., Huang, X. L., Ma, M. H., Chang, Q. R., Tu, Y., Li, Q., et al. (2018). Analysis of flash flood disaster characteristics in China from 2011 to 2015. Nat. Hazards 90, 407–420. doi: 10.1007/s11069-017-3052-7
He, Z., Zhao, W., Liu, H., and Chang, X. (2012). The response of soil moisture to rainfall event size in subalpine grassland and meadows in a semi-arid mountain range: a case study in northwestern china’s qilian mountains. J. Hydrol. 183–190. doi: 10.1016/j.jhydrol.2011.11.056
Heathman, G. C., Larose, M., Cosh, M. H., and Bindlish, R. (2009). Surface and profile soil moisture spatio-temporal analysis during an excessive rainfall period in the Southern Great Plains, USA. Catena 78, 159–169. doi: 10.1016/j.catena.2009.04.002
Heisler-White, J. L., Knapp, A. K., and Kelly, E. F. (2008). Increasing precipitation event size increases aboveground net primary productivity in a semi-arid grassland. Oecologia 158, 129–140. doi: 10.1007/s00442-008-1116-9
Hou, J., Liang, Q., Zhang, H., and Hinkelmann, R. (2015). An efficient unstructured MUSCL scheme for solving shallow water equations. Environ. Model. Softw. 66, 131–152. doi: 10.1016/j.envsoft.2014.12.007
Hu, S. L., Han, C. F., and Meng, L. P. (2015). A scenario planning approach for propositioning rescue centers for urban waterlog disasters. Comput. Ind. Eng. 87, 425–435. doi: 10.1016/j.cie.2015.05.036
Jia, X. X., Shao, M. A., Zhu, Y. J., and Luo, Y. (2017). Soil moisture decline due to afforestation across the Loess Plateau, China. J. Hydrol. 546, 113–122. doi: 10.1016/j.jhydrol.2017.01.011
Karim, M. R., Bashar, M. Z. I., and Imteaz, M. A. (2015). Reliability and economic analysis of urban rainwater harvesting in a megacity in Bangladesh. Resour. Conserv. Recycle 104(Part A), 61–67. doi: 10.1016/j.resconrec.2015.09.010
Koster, R. D., Dirmeyer, P. A., Guo, Z., Bonan, G., Chan, E., and Cox, P. (2004). Regions of strong coupling between soil moisture and precipitation. Science 305, 1138–1140. doi: 10.1126/science.1100217
Liao, K., Lai, X., Zhou, Z., and Zhu, Q. (2017). Applying fractal analysis to detect spatio-temporal variability of soil moisture content on two contrasting land use hillslopes. CATENA 157, 163–172. doi: 10.1016/j.catena.2017.05.022
Lin, H. S., Kogelmann, W., Walker, C., and Brunset, M. A. (2006). Soil moisture patterns in a forested catchment: a hydropedological perspective[J]. Geoderma 131, 345–368. doi: 10.1016/j.geoderma.2005.03.013
Liu, B. X., and Shao, M. A. (2014). Estimation of soil water storage using temporal stability in four land uses over 10 years on the Loess Plateau, China. J. Hydrol. 517, 974–984. doi: 10.1016/j.jhydrol.2014.06.003
Liu, C., Zhang, Y., Wang, Z., Wang, Y., and Bai, P. (2016). The LID pattern for maintaining virtuouswater cycle in urbanized area: a preliminary study of planning and techniques for sponge City. J. Nat. Resour. 31, 719–731.
Liu, W. M., and Luk, M. K. R. (2009). Reform and opening up: way to the sustainable and harmonious development of air transport in china. Transp. Policy 16, 220–223.
Lyu, H. M., Sun, W. J., Shen, S. L., and Arulrajah, A. (2018a). Flood risk assessment in metrosystems of mega-cities using a GIS-based modeling approach. Sci. Total Environ. 626, 1012–1025. doi: 10.1016/j.scitotenv.2018.01.138
Lyu, H. M., Xu, Y. S., Cheng, W. C., and Arulrajah, A. (2018b). Flooding hazards across southern China and prospective sustainability measures. Sustainability 10:1682. doi: 10.3390/su10051682
Lyu, H. M., Shen, S. L., and Arulrajah, A. (2018c). Assessment of geohazards and preventative countermeasures using AHP incorporated with GIS in Lanzhou China. Sustainability 10:304. doi: 10.3390/su10020304
Lyu, H. M., Wang, G. F., Cheng, W. C., and Shen, S. L. (2017). Tornado hazards on June 23rd in Jiangsu Province, China: preliminary investigation and analysis. Nat. Hazards 85, 597–604. doi: 10.1007/s11069-016-2588-2
Mahmoud, W. H., Elagib, N. A., Gaese, H., and Heinrich, J. (2014). Rainfall conditions and rainwater harvesting potential in the urban area of Khartoum. Resour. Conserv. Recycle 91, 89–99. doi: 10.1016/j.resconrec.2014.07.014
Matteo, R., Andrew, N., Yong, P., Jian-min, Z., Craig, L., Yan-peng, C., et al. (2019). Urban and river flooding: comparison of flood risk management approaches in the UK and China and an assessment of future knowledge needs. Water Sci. Eng. 12, 274–283. doi: 10.1016/j.wse.2019.12.004
Mehrabadi, M. H. R., Saghafian, B., and Fashi, F. H. (2013). Assessment of residential rainwater harvesting efficiency formeeting non-potablewater demands in three climate conditions. Resour. Conserv. Recycle 73, 86–93. doi: 10.1016/j.resconrec.2013.01.015
Nguyen, T. T., Ngo, H. H., Guo, W. S., Wang, X. C., Ren, N. Q., Li, G. B., et al. (2019). Implementation of a specific urban water management: sponge City. Sci. Total Environ. 652, 147–162. doi: 10.1016/j.scitotenv.2018.10.168
Njoku, E. G., and Rague, B. W. (1996). Spatial and Temporal Trends in Land Surface Moisture and Temperature Observable using Data from the Nimbus-7 Microwave Radiometer. IGARSS 96 Remote Sensing for a Sustainable Future. Pasadena, CA: CALTECH.
Rosenbaum, U., Bogena, H. R., Herbst, M., Huisman, J. A., and Vereecken, H. (2012). Seasonal and event dynamics of spatial soil moisture patterns at the small catchment scale. Water Resour. Res. 48, 3472–3476.
Sang, Y. F., and Yang, M. (2017). Urban waterlogs control in China: more effective strategies and actions are needed. Nat. Hazards 85, 1291–1294. doi: 10.1007/s11069-016-2614-4
Shafique, M., and Kim, R. (2015). Low impact development practices: a review of current research and recommendations for future directions. Nephron Clin. Pract. 22, 543–563. doi: 10.1515/eces-2015-0032
Su, D., Zhang, Q. H., Ngo, H. H., Dzakpasu, M., Guo, W. S., and Wang, X. C. (2019). Development of a water cycle management approach to Sponge City construction in Xi’an, China. Sci. Total Environ. 685, 490–496. doi: 10.1016/j.scitotenv.2019.05.382
Wang, H., Mei, C., and Liu, J. (2017a). Systematic construction pattern of the sponge city [J]. J. Hydraul. Eng. 49, 1009–1014.
Wang, Y. T., Sun, M. X., and Song, B. M. (2017b). Public perceptions of and willingness to pay for sponge city initiatives in China. Resour. Conserv. Recycl. 122, 11–20. doi: 10.1016/j.resconrec.2017.02.002
Wang, J.-L., Che, W., and Yi, H.-X. (2009). Low impact development for urban stormwater and flood control and utilization [J]. China Water Wastewater 25, 6–10.
Wang, Y., Zhang, X., Cheng, F., and Wang, X. (2012). Study on regulation effect of grass filter strip on city rainstorm runoff. China Water Wastewater 28, 61–63.
Wang, Y. Q., Hu, W., Zhu, Y. J., Shao, M. A., Xiao, S., and Zhang, C. C. (2015). Vertical distribution and temporal stability of soil water in 21-m profiles under different land uses on the Loess Plateau in China. J. Hydrol. 527, 543–554. doi: 10.1016/j.jhydrol.2015.05.010
Xia, J., Zhang, Y. Y., Xiong, L. H., He, S., Wang, L. F., and Yu, Z. B. (2017). Opportunities and challenges of Sponge City construction related to urban water issues in China. Sci. China Earth Sci. 60, 652–658. doi: 10.1007/s11430-016-0111-8
Xiao, L., Zhang, Y., Li, P., Xu, G. C., Shi, P., and Zhang, Y. (2019). Effects of freeze-thaw cycles on aggregate-associated organic carbon and glomalin-related soil protein in natural-succession grassland and Chinese pine forest on the Loess Plateau. Geoderma 334, 1–8. doi: 10.1016/j.geoderma.2018.07.043
Xu, G., Ren, Z., Li, P., Li, Z., Yuan, S., Zhang, H., et al. (2016). Temporal persistence and stability of soil water storage after rainfall on terrace land. Environ. Earth Sci. 75:966.
Xu, G. C., Li, Z. B., Li, P., Zhang, T. G., Chang, E. H., Wang, F. C., et al. (2017). The spatial pattern and temporal stability of the soil water content of sloped forestland on the Loess Plateau, China. Soil Sci. Soc. Am. J. 81, 902–914. doi: 10.2136/sssaj2016.10.0331
Yin, J., Ye, M., Yin, Z., and Xu, S. (2015). A review of advances in urban flood risk analysis over China. Stoch. Environ. Res. Risk Assess. 29, 1063–1070. doi: 10.1007/s00477-014-0939-7
Yu, M., and Liu, Y. M. (2015). The possible impact of urbanization on a heavy rainfall event in Beijing. J. Geophys. Res. Atmos. 120, 8132–8143. doi: 10.1002/2015jd023336
Yuan, Y., Xu, Y. S., and Arulrajah, A. (2017). Sustainable measures for mitigation of flooding hazards: a case study in Shanghai, China. Water 9:310. doi: 10.3390/w9050310
Keywords: soil moisture, water quality, rain event, sponge city, the Loess Plateau
Citation: Jia L, Xu G, Huang M, Li Z, Li P, Zhang Z, Wang B, Zhang Y, Zhang J and Cheng Y (2020) Effects of Sponge City Development on Soil Moisture and Water Quality in a Typical City in the Loess Plateau in China. Front. Earth Sci. 8:125. doi: 10.3389/feart.2020.00125
Received: 05 October 2019; Accepted: 01 April 2020;
Published: 24 April 2020.
Edited by:
Qiuhua Liang, Loughborough University, United KingdomReviewed by:
Jiahong Liu, China Institute of Water Resources and Hydropower Research, ChinaKaibo Wang, Institute of Earth Environment (CAS), China
Zhengchao Zhou, Shaanxi Normal University, China
Ming Xiao Zhang, China Institute of Water Resources and Hydropower Research, China
Copyright © 2020 Jia, Xu, Huang, Li, Li, Zhang, Wang, Zhang, Zhang and Cheng. This is an open-access article distributed under the terms of the Creative Commons Attribution License (CC BY). The use, distribution or reproduction in other forums is permitted, provided the original author(s) and the copyright owner(s) are credited and that the original publication in this journal is cited, in accordance with accepted academic practice. No use, distribution or reproduction is permitted which does not comply with these terms.
*Correspondence: Guoce Xu, xuguoce_x@163.com