- 1Palaeoscience Research Centre, School of Environmental and Rural Science, University of New England, Armidale, NSW, Australia
- 2Museum of Comparative Zoology, Department of Organismic and Evolutionary Biology, Harvard University, Cambridge, MA, United States
Horseshoe crabs are an iconic group of extant chelicerates, with a stunning fossil record that extends to at least the Lower Ordovician (~480 million years ago). As such, the group has retained significant biological and palaeontological interest. The sporadic nature of descriptive and systematic research into fossil horseshoe crabs over the last two centuries has spread information on the group across more than 200 texts dating from the early nineteenth century to the present day. We present the most comprehensive pictorial atlas of horseshoe crabs to date to pool these important data together. This review highlights taxa such as Bellinurus lacoei and Limulus priscus that have never been documented with photography. Furthermore, key morphological features of the true horseshoe crab (Xiphosurida) families—Austrolimulidae, Belinuridae, Limulidae, Paleolimulidae, and Rolfeiidae—are described. The evolutionary history of horseshoe crabs is reviewed and the current issues facing any possible biogeographic work are presented. Four major future directions that should be adopted by horseshoe crab researchers are outlined. We conclude that this review provides the basis for innovative geographic and geometric morphometric studies needed to uncover facets of horseshoe crab evolution.
Introduction
Chelicerates, a group that includes arachnids (spiders, scorpions), eurypterids (sea scorpions), and Xiphosura (the so-called horseshoe crabs) have a stunning and extensive fossil spanning the early Palaeozoic to today and an exceptional modern diversity (Dunlop, 2010). Of these taxa, extant horseshoe crabs have been subject to detailed anatomical (van Der Hoeven, 1838; Owen, 1872; Lankester, 1881; Shuster, 1982; Shultz, 2001; Bicknell et al., 2018b,c,d), biochemical (Kaplan et al., 1977; Botton and Ropes, 1987), physiological (Sokoloff, 1978), morphological (Lee and Morton, 2005; Chatterji and Pati, 2014; Jawahir et al., 2017), and population dynamic (Botton, 1984; Brockmann, 1990; Gerhart, 2007) studies over the past two centuries. Furthermore, the impressive fossil record of this group, and apparent morphological conservatism that allowed survival of all five big mass extinctions, have driven extensive palaeontological interest in the group (Babcock et al., 2000; Rudkin and Young, 2009; Sekiguchi and Shuster, 2009; Krzeminski et al., 2010; Briggs et al., 2012; Dunlop et al., 2012; Lamsdell, 2013; Błazejowski, 2015; Lamsdell and Mckenzie, 2015; Bicknell et al., 2018b,c, 2019b; Bicknell, 2019; Figure 1). Despite this extensive research, numerous avenues for further research remain for horseshoe crabs, and we highlight three here. Firstly, the evolutionary relationship between synziphosurines (the so-called “Synziphosura”) and Xiphosura (Lamsdell, 2013, 2016; Legg et al., 2013; Garwood and Dunlop, 2014). To help clarify this relationship, Lamsdell (2013) removed synziphosurines from Xiphosura and arrayed them within Prosomapoda and Planaterga. Secondly, there are a number of specimens that have been described in open terminology (Haug et al., 2012; Lamsdell et al., 2020) and despite the recent effort to bring taxa into recognized families, and genera, and erect new groups where appropriate (Bicknell, 2019; Bicknell et al., 2019e; Lamsdell et al., 2020), there remain an array of individuals that require taxonomic revision. Lastly, some genera appear to have been extensively over-split (Dunbar, 1923; Størmer, 1972; Fisher, 1984; Anderson, 1994; Haug et al., 2012; Kin and Błazejowski, 2014; Haug and Rötzer, 2018b). We therefore present a pictorial review of horseshoe crabs to aid current and future researchers in (1) the morphology and re-evaluation of taxa, (2) the determination of evolutionary relationships, and (3) the confirmation of species validity (Waterston, 1985; Selden and Siveter, 1987).
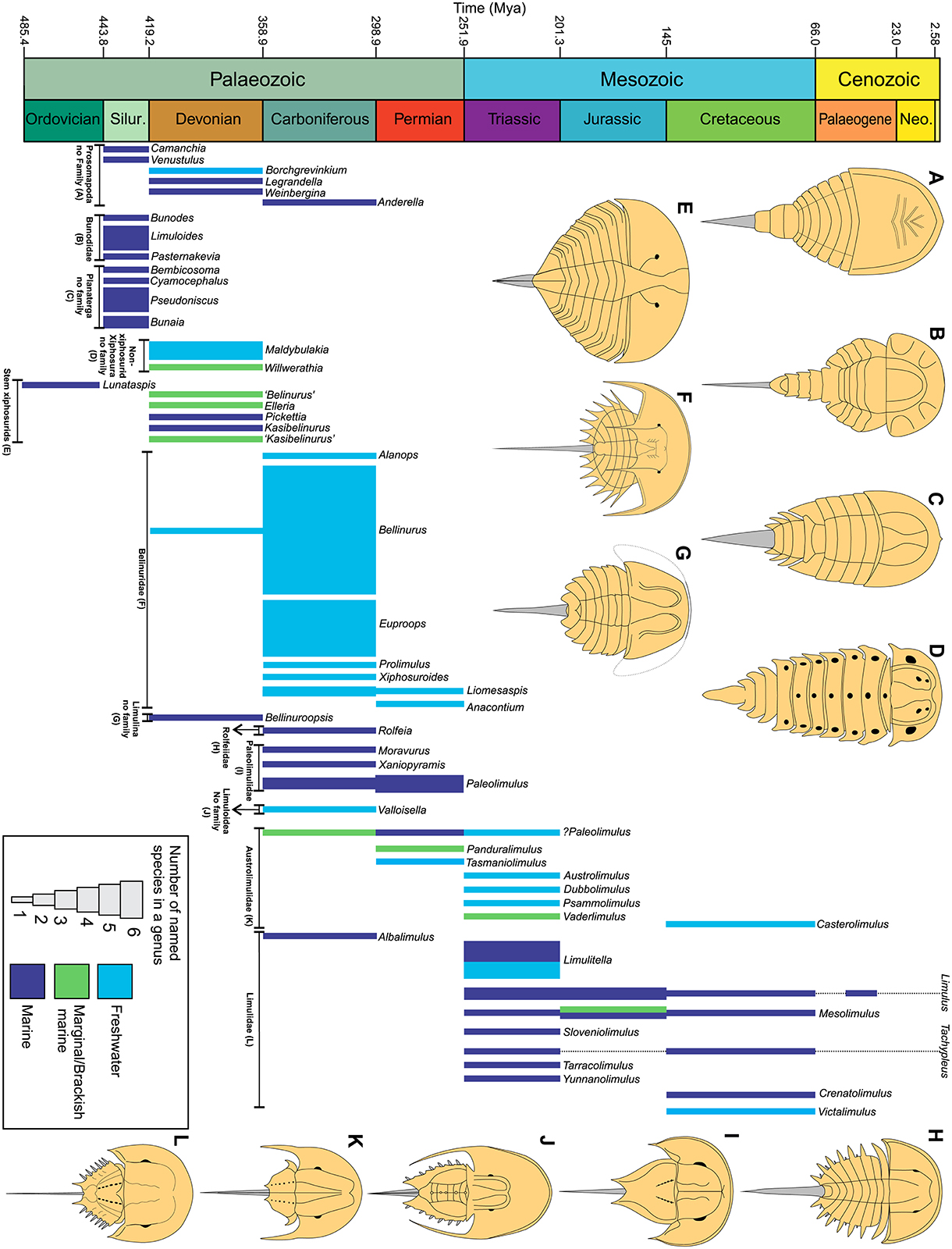
Figure 1. The geological and morphological history of horseshoe crabs across the Phanerozoic. Number of named species is presented as well as suggested palaeoenvironment (Tables 1–7). A major transition to freshwater conditions occurred between the Devonian and Carboniferous. This was concurrent with a decrease in synziphosurine taxa and an increase in xiphosurids. Limulids had a diversification event in the Triassic and there was a transition back to dominantly marine conditions in the Jurassic. Dashed lines represent ghost lineages.
The palaeontological and evolutionary histories, broad taxonomy of families (Størmer, 1955; Novozhilov, 1991), and phylogenetic relationships (Lamsdell, 2013, 2016) of horseshoe crabs has often been reviewed (Bergström, 1975; Selden and Siveter, 1987; Anderson and Selden, 1997; Anderson and Shuster, 2003; Rudkin and Young, 2009). However, a document illustrating all horseshoe crab taxa has not been presented since Woodward (1866, 1867, 1879); Dix and Pringle (1929, 1930); Eller (1938b), and Raymond (1944). We have therefore collated images of all species considered horseshoe crabs (see taxa Dunlop et al., 2019), in a vital step toward understanding the true diversity and extent of Xiphosura (Lamsdell, 2013). We also present taxonomic descriptions of the facets that define members of xiphosurid families and consider of lifestyle and diversity of each group. We have focused on Xiphosurida as there are more taxa in this group than stem xiphosurids and synziphosurines. Nonetheless, synziphosurines and non-xiphosurid xiphosurans (previously considered Kasibelinuridae) are also briefly considered. It is vital to note that a thorough taxonomic revision of all species is beyond the intended scope of this review—namely the depiction and discussion of major horseshoe crab groups—but the images and details here represent the basis for such future work. The ultimate goal of this work is to depict all taxa in an open-access environment for future researchers to use as a reference point to continue research into this somewhat enigmatic group of chelicerates.
Terminology
The following definitions are provided to clarify terminology used in descriptions. See Figure 2 for a depiction of these features.
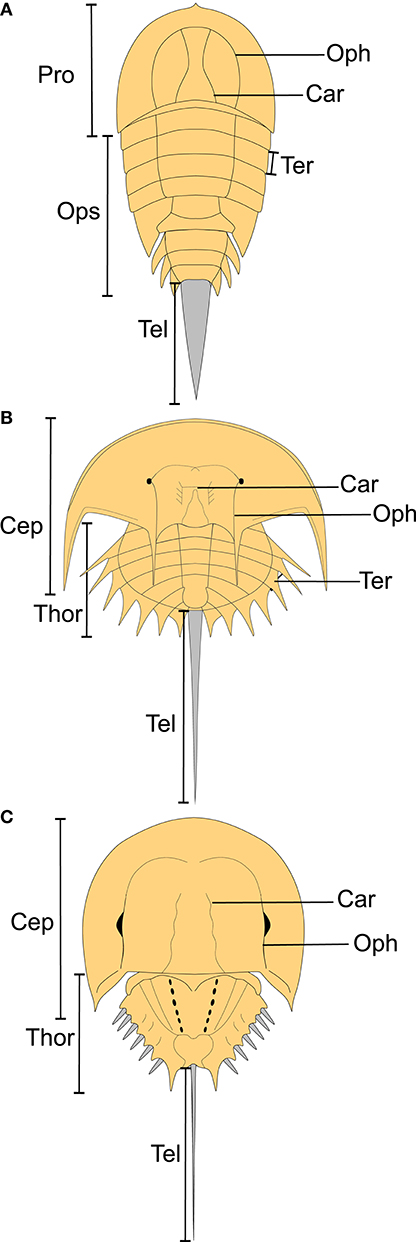
Figure 2. Depiction of horseshoe crab features outlining the key morphological aspects of horseshoe crabs. (A) Reconstruction of Cyamocephalus loganensis showing main morphological features of synziphosurines. (B) Reconstruction of Euproops danae, showing main morphological features of belinurids. (C) Reconstruction of Limulus polyphemus, showing main morphological features of Limulina. Car, cardiac lobe; Cep, cephalothorax; Oph, ophthalmic ridge; Ops, opisthosoma; Pro, prosoma; Tel, telson; Ter, tergite; Thor, thoracetron.
Somite: Fundamental unit or division that construct arthropod bodies (Lamsdell, 2013; Dunlop and Lamsdell, 2017).
Tergite: Physical expression of somites as discrete plates on the dorsal exoskeleton (Lamsdell, 2013; Dunlop and Lamsdell, 2017).
Prosoma: Anterior body section consisting of six somites (Dunlop and Lamsdell, 2017). Prosoma refers to the anterior section of synziphosurines and xiphosurans (Dunlop, 2010; Dunlop and Lamsdell, 2017). The prosoma in Xiphosurida is combined with the two most anterior opisthosomal sections to produce the cephalothorax (Dunlop, 2010; Dunlop and Lamsdell, 2017).
Cephalothorax: Anterior body section of Xiphosurida. Combination of two most anterior opisthosomal segments with prosoma (Dunlop, 2010).
Ophthalmic ridge: Ridge above the lateral compound eye that extends anteriorly and posteriorly relative to the compound eye (Størmer, 1955).
Cardiac lobe: Lobe in the center of the prosoma/cephalothorax that extends into opisthosoma/thoracetron (Størmer, 1955).
Opisthosoma: Posterior section of the arthropod body, consisting of up to 13 tergites (Dunlop and Lamsdell, 2017). Used here for synziphosurines and non-xiphosurid xiphosurans as the group lack a fused opisthosoma (=thoracetron) (Lamsdell, 2013).
Thoracetron: Posterior section of Xiphosurida that is a fused solid plate. Shultz (2001) also suggested the termed tergum for this feature. The section may have expressed tergites.
Telson: Most posterior section of the xiphosuran exoskeleton, styliform and highly mobile (Eagles, 1973). Also called a tailspine.
Institutional Acronyms
AM F: Australian Museum, Sydney, NSW, Australia. AMNH: American Museum of Natural History, New York, USA. B: Geomuseum der WWU Münster, Germany. BGS.GSE: British Geological Survey, Keyworth, England, UK. BMSC: Buffalo Museum, Buffalo, NY, USA. CM: Carnegie Museum of Natural History, Pittsburgh, Pennsylvania, USA. CCMGE: Chernyshev Central Research Geological Exploration Museum, St. Petersburg, Russia. GIN: Geological Institute of the Russian Academy of Sciences, Moscow, Russia. GIUS: Faculty of Earth Sciences, Silesian University, Sosnowiec, Czech Republic. GSC: Geological Survey of Canada, Ottawa, Canada. GZG INV: Geowissenschaftliches Zentrum der Georg-August-Universität Geowissenschaftliches Museum, Göttingen, Germany. ISEA: Museum of the Institute of Systematics and Evolution of Animals, Polish Academy of Sciences, Warsaw, Poland. L, LL: Manchester Museum, University of Manchester, Manchester, England, UK. LPI: Chengdu Geological Center, Chengdu, China. MAN: Muséum-Aquarium de Nancy, Lorraine, France. MAS Pal: Museum am Schölerberg, Osnabrück, Germany. MB.A.: Museum für Naturkunde Leibniz-Insitut, Berlin, Germany. MCZ: Museum of Comparative Zoology, Harvard University, Cambridge, MA, USA. MGSB: Museo Geológico del Seminario de Barcelona, Barcelona, Spain. Specimens ending in MLU, HAU-WIL: Institut für Geologische Wissenschaften und Geiseltalmuseum Martin Luther University Halle-Wittenberg, Halle, Saale, Germany. MM: Manitoba Museum, Winnipeg, Canada. MMF: Geological Survey of New South Wales, Londonderry, NSW, Australia. MMO B: Municipal Museum of Ostrava, Ostrava, Czech Republic. MNHN: Museum National d'Histoire Naturelle of Paris, Paris, France. MNHP: Národní muzeum, Prague, Czech Republic. MSNM: Museo Civico di Storia Naturale di Milano, Milan, Italy. NHMUK PI: Natural History Museum, London, UK. NME: Geologisch-Paläontologischen Sammlung des Naturkundemuseums Erfurt, Germany. NMK D: Wolfgang Munk collection in Naturkundemuseum Kassel, Ottoneum in Kassel, Germany. NMS: National Museums of Scotland, Edinburgh, Scotland. NMW: National Museum of Wales, Cardiff, United Kingdom. NSM: Nova Scotia Museum, Halifax, NS, Canada. NYSM: New York State Museum, Albany, NY, USA. OUMNH: Oxford University Museum of Natural History, Oxford, England, UK. NMV P: Museums Victoria, Carlton, Victoria, Australia. PIN: Paleontological Museum of Yu A Orlov, Moscow, Russia. NHM-UIO: Natural History Museum, University of Oslo, Oslo, Norway. PMSL: Natural History Museum of Slovenia, Ljubljana, Slovenia. SLK: Leunissen private collection. SMF: Forschungsinstitut Senckenberg, Frankfurt am Main, Germany. SMNH: Swedish Museum of Natural History, Stockholm, Sweden. SMNS: State Museum of Natural History Stuttgart, Stuttgart, Germany. SNSB-BSPG: Staatliche Naturwissenschaftliche Sammlungen Bayern – Bayerische Staatssammlung für Paläontologie und Geologie, Munich, Germany. SPW: Poschmann private collection. TMP: The Royal Tyrrell Museum, Drumheller, AB, Canada. TsNIGR: Chernyshev Central Research Geological Museum, St. Petersburg, Russia. UCM: University of Colorado Museum of Natural History, Boulder, CO, USA. UM: Paleontology Center of University of Montana, MT, USA. UMUT PA: The University Museum of the University of Tokyo, Tokyo, Japan. USNM: United States National Museum, Washington, DC, USA. USTL: Laboratoire de paléontologie de l'université de Lille-1, Poitiers, France. UTGD: Geology Department, University of Tasmania, Tasmania, Australia. U.W.: University of Wisconsin Geology Museum, Madison, WI, USA. W.U.: Wichita State University, Wichita, KS, USA. YPM IP: Division of Invertebrate Paleontology in the Yale Peabody Museum, New Haven, CT, USA. YPM IZ: Division of Invertebrate Zoology in the Yale Peabody Museum, New Haven, CT, USA. ZIK: Ukrainian Academy of Sciences, 252.150 Kiev, Ukraine. ZPAL: Institute of Paleobiology, Polish Academy of Science, Warsaw, Poland.
Divisions of Horseshoe Crabs
Synziphosurines
First appearing in at least the early Ordovician of Morocco, synziphosurines went extinct in the Mississippian (Tables 1–4, Figures 3–9) (Anderson and Selden, 1997; Moore et al., 2005b, 2007; Krzeminski et al., 2010; Van Roy et al., 2010; Briggs et al., 2012). There are 13 synziphosurine genera and 20 species. Anderella, Borchgrevinkium, Camanchia, Legrandella, Venustulus, and Weinbergina are currently considered to belong to the clade Prosomapoda (the group that also contains Xiphosura, Figures 4, 5), while Bembicosoma, Bunaia, Bunodes, Cyamocephalus, Limuloides, Pasternakevia, and Pseudoniscus have been placed into Planaterga (Figures 6–9; Lamsdell, 2013). Synziphosurines are characterized by large prosomal shields, unfused opisthosoma with nine to 11 segmented and expressed tergites (Størmer, 1934, 1955; Rudkin et al., 2008; Lamsdell, 2013; Selden et al., 2015). In extreme cases, the three most posterior tergites form a narrow postabdominal (pretelson) section leading to a styliform telson. Lateral compound eyes are known from Legrandella lombardii and Pseudoniscus roosevelti (Eldredge, 1974; Bergström, 1975; Bicknell et al., 2019a). Furthermore, Pasternakevia podolica (Krzeminski et al., 2010) and Weinbergina opitzi (Lehmann, 1956; Stürmer and Bergström, 1981) show evidence for putative ocular features. The remaining taxa lack such ocular features and were possibly blind (Bicknell et al., 2019a). Appendages are known from at least Anderella parva, Venustulus waukeshaensis, and Weinbergina opitzi (Richter and Richter, 1929; Størmer, 1934; Stürmer and Bergström, 1981; Moore et al., 2005a,b, 2007). Synziphosurines inhabited marine to marginal marine environments, and the general lack of thick prosomal margin suggests that the group may not have burrowed, and instead potentially moved above the substrate (Størmer, 1952; Bergström, 1975; Stürmer and Bergström, 1981; Lamsdell et al., 2013). Affinities of synziphosurines are actively debated due to the few useful synapomorphies that have been identified to date (Anderson et al., 1998), which has resulted in an unnatural grouping of assorted stem euchelicerates (Krzeminski et al., 2010; Lamsdell, 2013, 2016; Lamsdell and Mckenzie, 2015; Selden et al., 2015). To build on the phylogenetic work presented in Lamsdell (2013), in which Lamsdell highlighted that synziphosurines comprise both possible stem-horseshoe crabs and stem arachnids, images of all accepted synziphosurines are presented here (Figures 3–9).
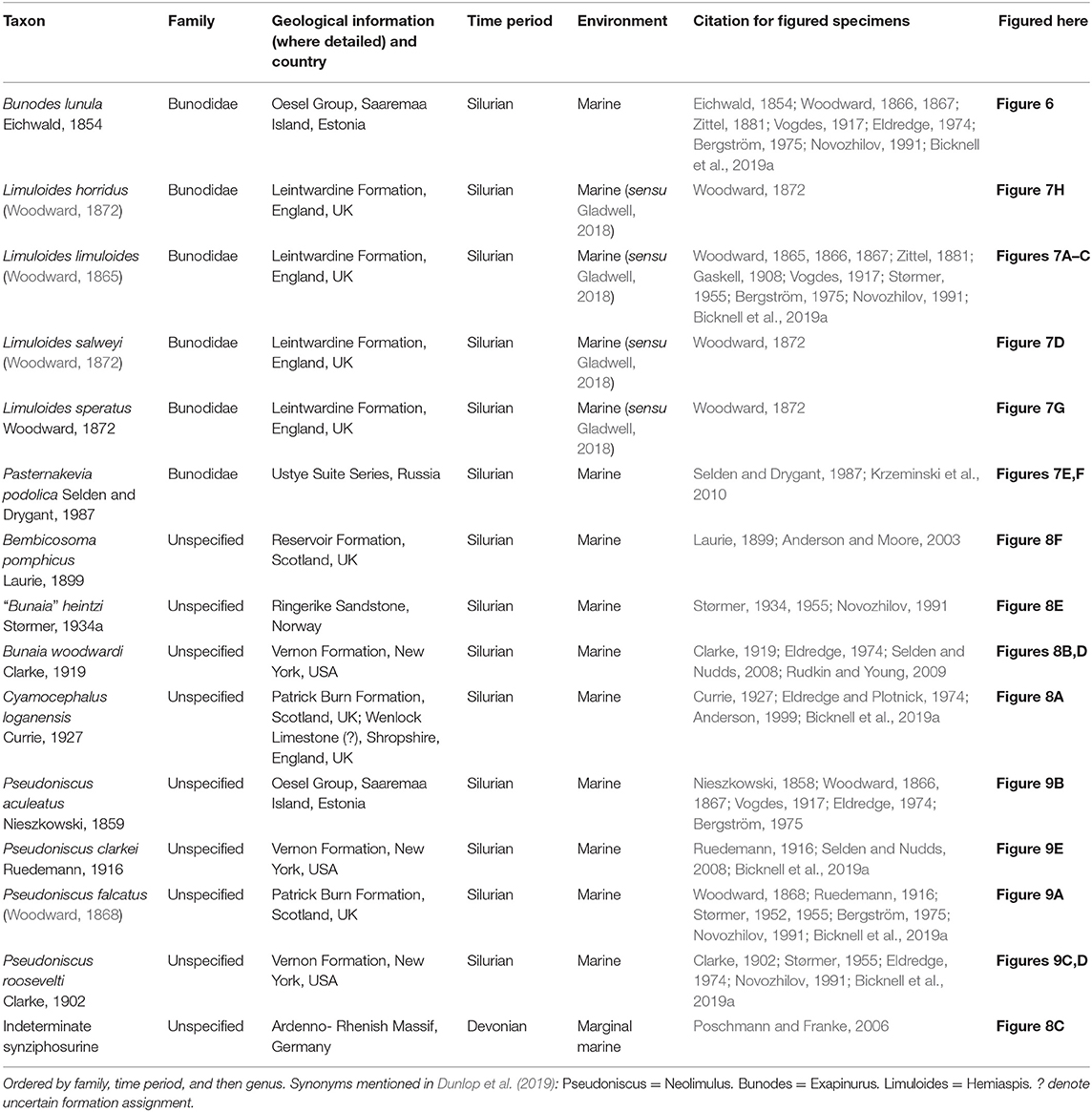
Table 3. Taxa in clade Planaterga, excluding the group Dekatriata, sensu Lamsdell (2013) that traditionally represent synziphosurine groups.
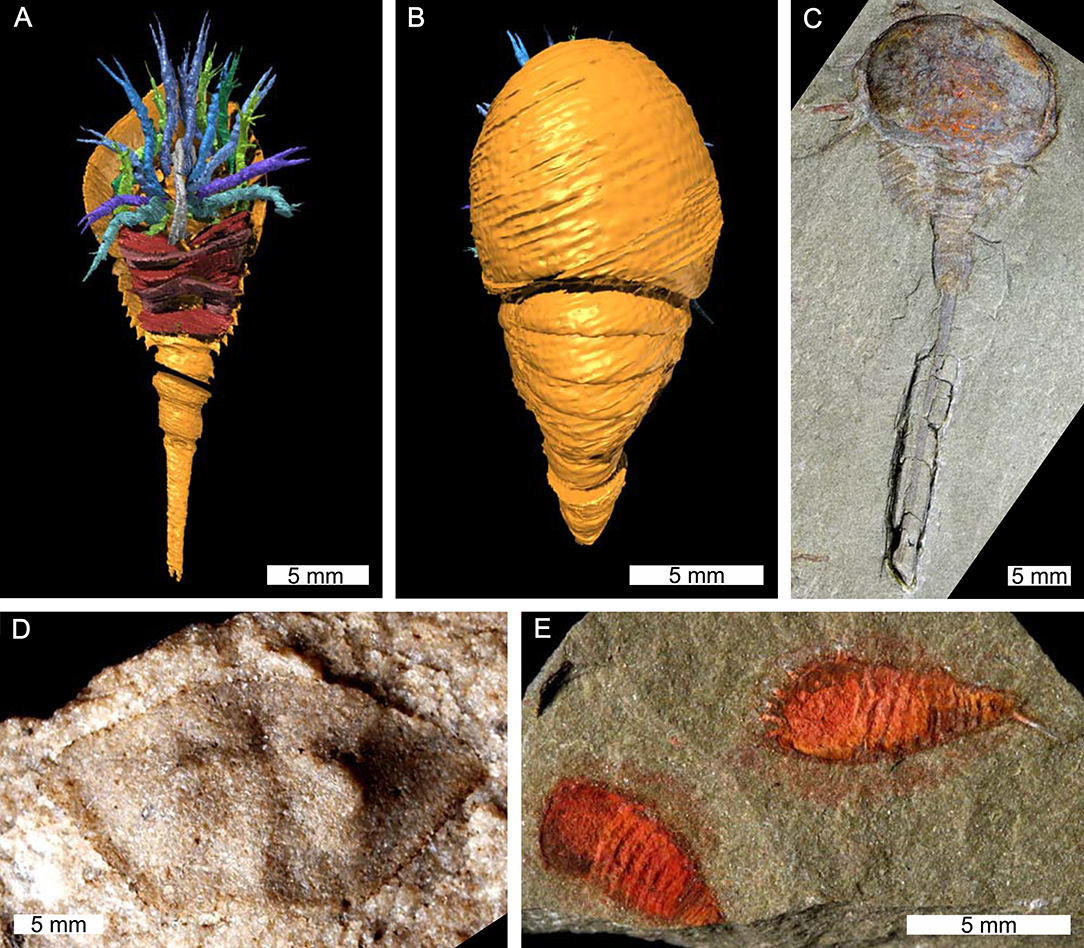
Figure 3. Taxa considered possible horseshoe crabs that currently lack definitive affinities. (A,B) Dibasterium durgae: reconstructed in 3D from the Silurian-aged Herefordshire Konservat-Lagerstätte, England, UK. OUMNH C.29640, holotype (A) Ventral view. (B) Dorsal view. (C) An unnamed xiphosuran from the lower Ordovician-aged Upper Fezouata Formation, Morocco. YPM IP 227586. (D) Drabovaspis complexa from the Ordovician-aged Letná Formation, Czech Republic. MNHP L23577, holotype. This taxon is also considered to have aglaspidid affinities (Dunlop et al., 2019). (E) Two unnamed synziphosurines from the lower Ordovician-aged Lower Fezouata Formation, Morocco. YPM IP 517856. Photo credit: (A,B) Russell Garwood (also see Briggs et al., 2012); (C) Russell Bicknell; (D) Javier Ortega Hernández; (E) Jessica Utrup.
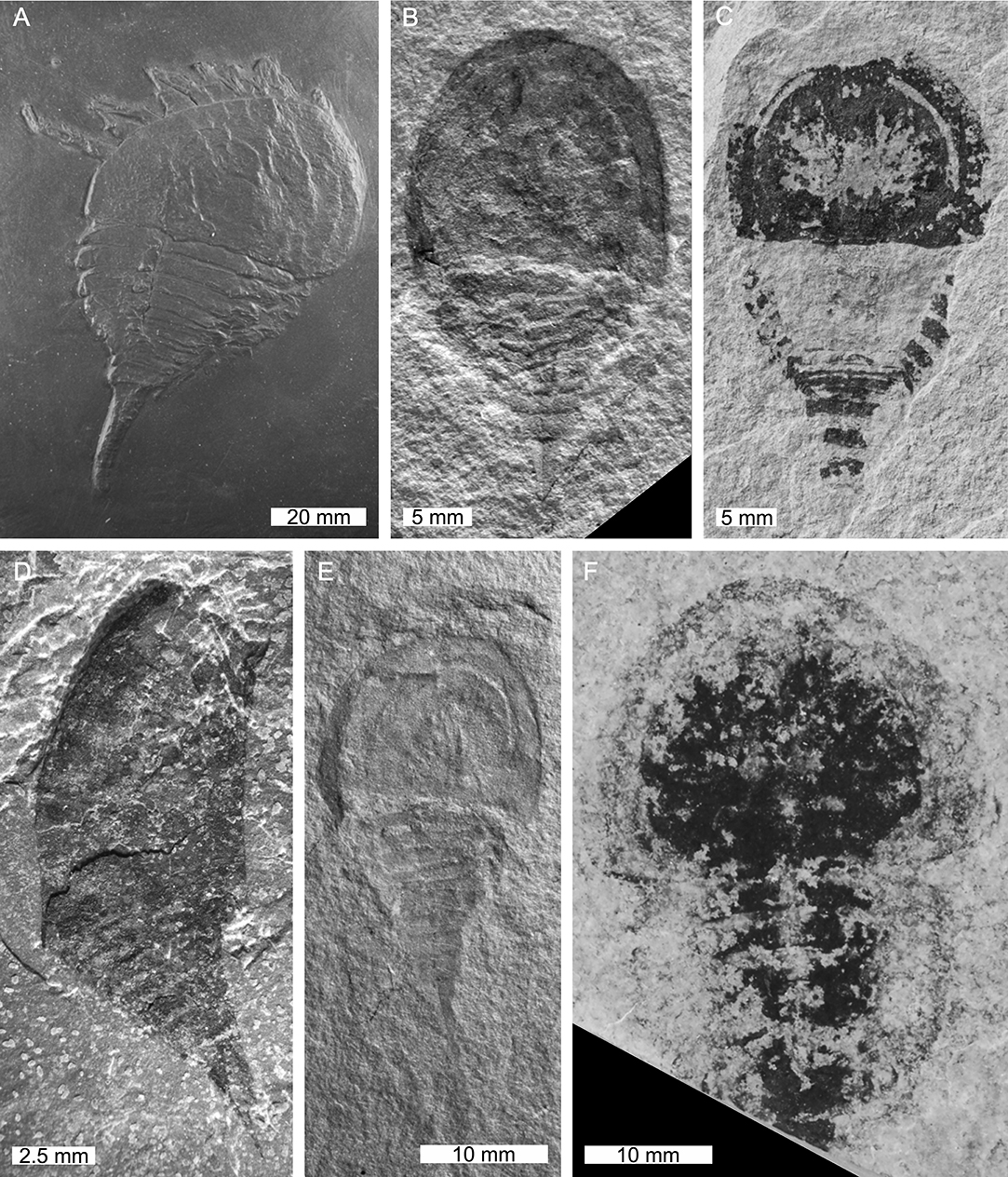
Figure 4. Taxa in Prosomapoda that are not within Planaterga or Xiphosura. (A) Weinbergina opitzi from the Devonian-aged Hunsrück Slate Rheinland, Germany. MB.A.1987. (B,E) Anderella parva from the Carboniferous-aged Bear Gulch Limestone, Montana, USA. (B) CM 54200, holotype. (E) CM 54201, paratype (C) Venustulus waukeshaensis from the Silurian-aged Waukesha Lagerstätte, Wisconsin, USA. YPM IP 204461. (D) Borchgrevinkium taimyrensis from the Devonian-aged Sheshenkarinskoy Suite, Kazakhstan. PIN 12711, holotype. (F) Camanchia grovensis from the Silurian-aged Wenlock Scotch Grove Formation, Iowa, USA. U.W.4018/1a, holotype. Photo credit: (A) Andreas Abele, (B,C,E) Russell Bicknell, (D) Dmitry E. Shcherbakov, (F) Carrie A. Eaton. All converted to gray scale.
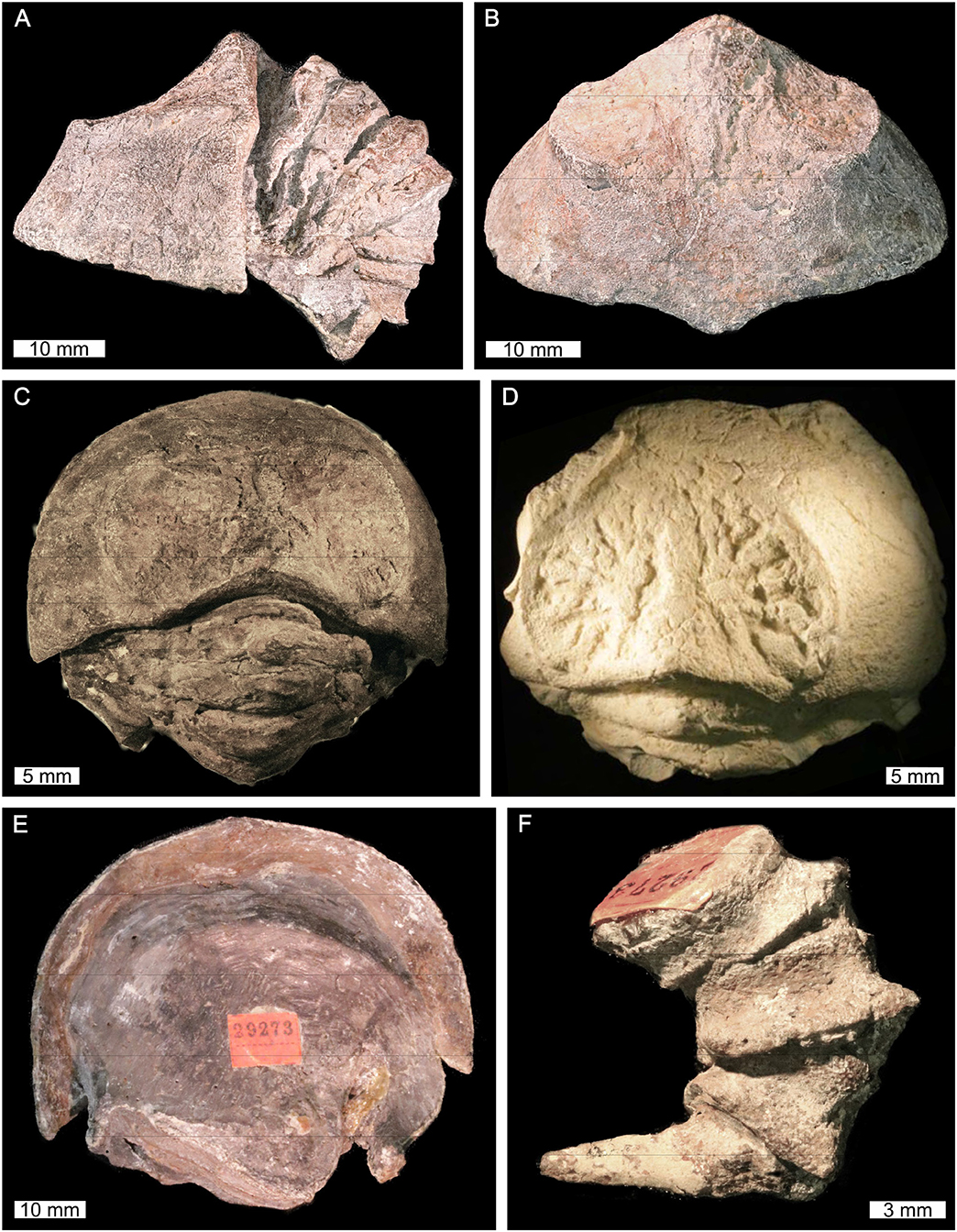
Figure 5. Legrandella lombardii from the Devonian-aged Icla Formation, Bolivia. (A–C,E,F) AMNH 029273, holotype. (A) Lateral view. (B) Anterior view of prosoma. (C) Dorsal view of prosoma. (E) Ventral view of prosoma. (F) Lateral view of telson. (D) AMNH 029274, plastoparatype. Dorsal view of prosoma. Photo credit: Russell Bicknell.
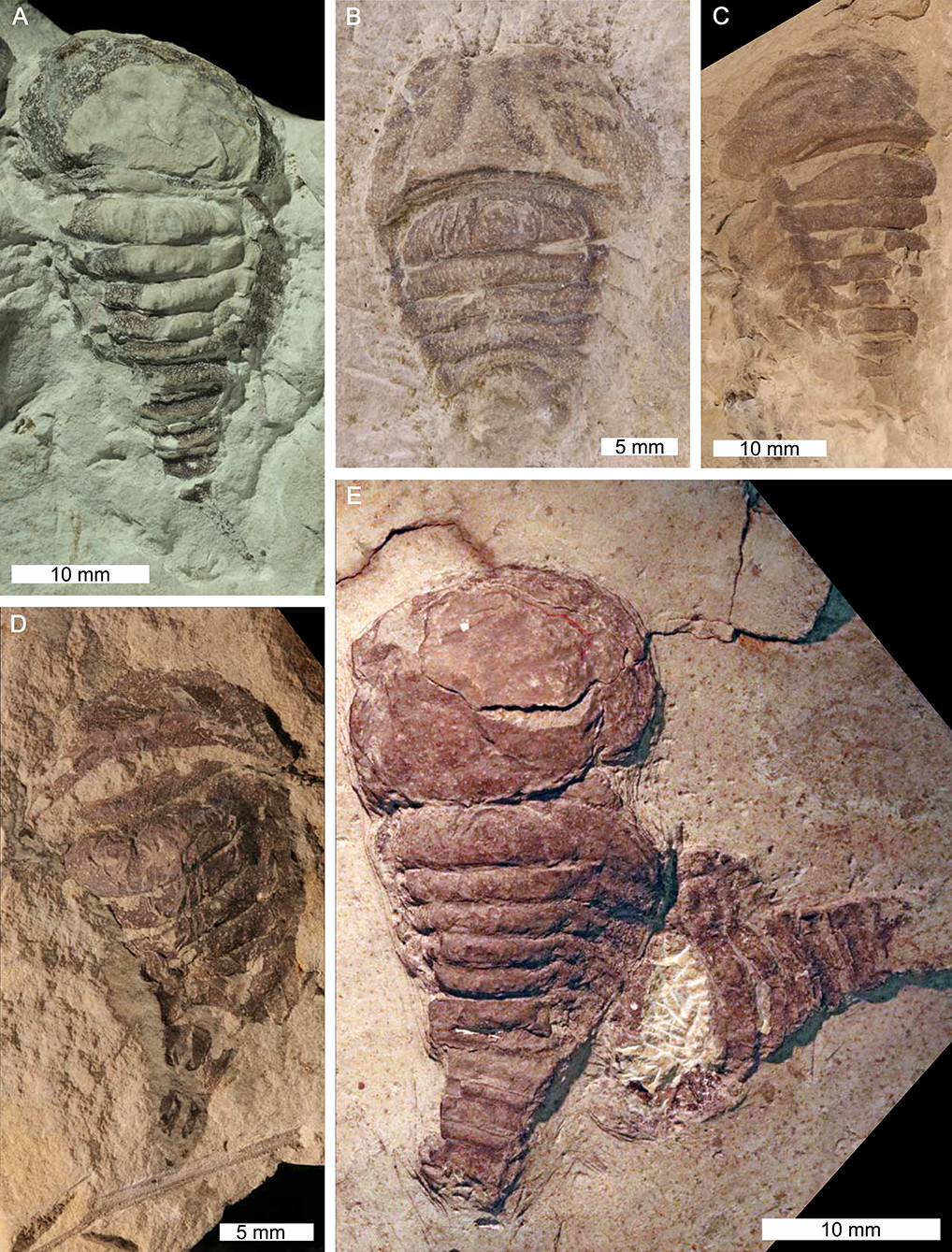
Figure 6. Examples of Bunodes lunula from the Silurian-aged Oesel Group, Saaremaa Island, Estonia. (A) NMS G.2001.10.1. (B) YPM IP 212839. (C) NYSM 19113. (D) NYSM 19114. (E) Slab showing two specimens. AMNH 028734. Photo credit: (A) Bill Crighton; (B–E) Russell Bicknell.
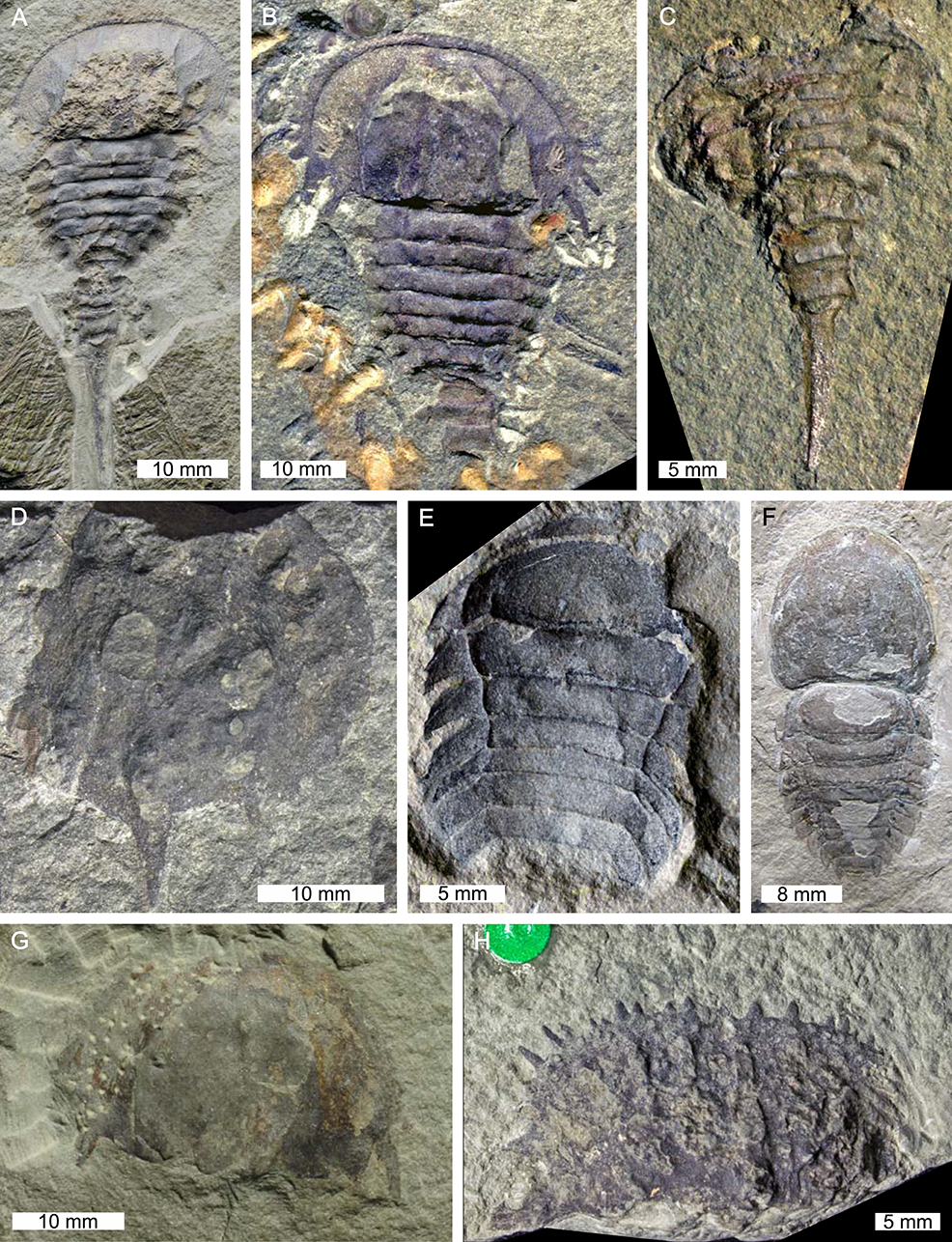
Figure 7. Limuloides and Pasternakevia. (A–C) Limuloides limuloides from the Silurian-aged Leintwardine Formation, England, UK. (A) BGS.GSE 32393. (B) NHMUK PI. In. 60018. (C) NHMUK PI. In. 48422. (D) Limuloides salweyi from the Silurian-aged Leintwardine Formation, England, UK. NHMUK PI. In. 61510, holotype. (E,F) Pasternakevia podolica from the Silurian-aged Ustye Suite Series, Russia. (E) ISEA I–F/MP/3/1499/08. (F) ZIK 35611, holotype. (G) Limuloides speratus from the Silurian-aged Leintwardine Formation. NHMUK PI. I. 1180. (H) Limuloides horridus from the Silurian-aged Leintwardine Formation, England, UK. NHMUK PI. In. 61509, holotype. Photo credit: (A) David Marshall; (B–D,G,H) Stephen Pates; (E) Błażej Błażejowski; (F) Ewa Krzeminska.
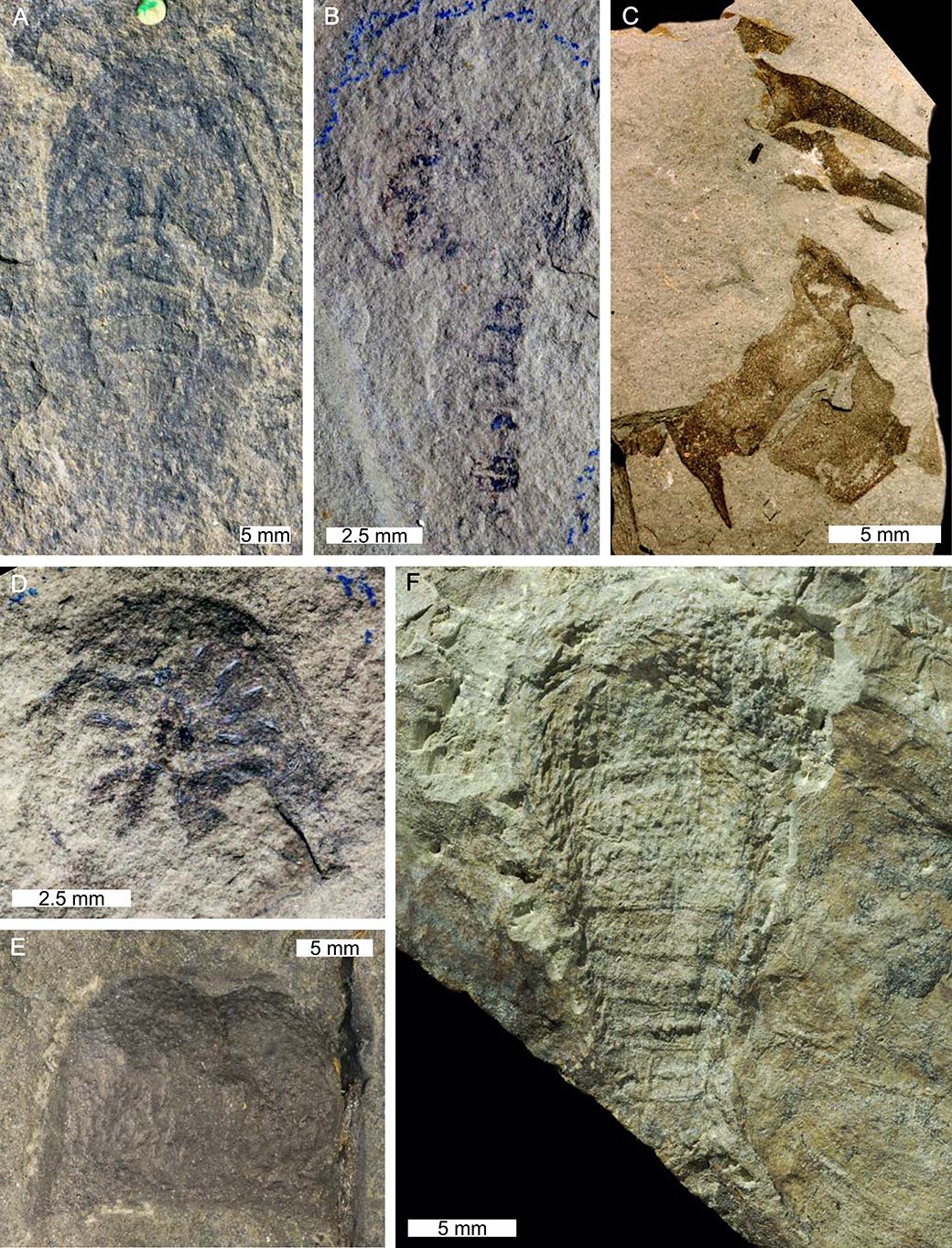
Figure 8. “Synziphosurines” currently lacking a family assignment. (A) Cyamocephalus loganensis from the Silurian-aged Patrick Burn Formation, Scotland, UK. NHMUK PI. I. 16521, holotype. (B,D) Bunaia woodwardi from the Silurian-aged Vernon Shale, New York, USA. (B) NYSM 9911. (D) NYSM 9910. (C) Indeterminate synziphosurine from the Devonian-aged Klerf Formation, Germany. SPW 831-D. (E) “Bunaia” heintzi from the Silurian-aged Ringerike Sandstone, Norway. NHM-UIO PMOA4361, holotype. (F) Bembicosoma pomphicus from the Silurian-aged Reservoir Formation, Scotland, UK. NMS G.1897.32.146, holotype. Photo credit: (A) Javier Ortega Hernández; (B,D) Russell Bicknell; (C) Markus Poschmann; (E) Hans Arne Nakrem; (F) Bill Crighton.
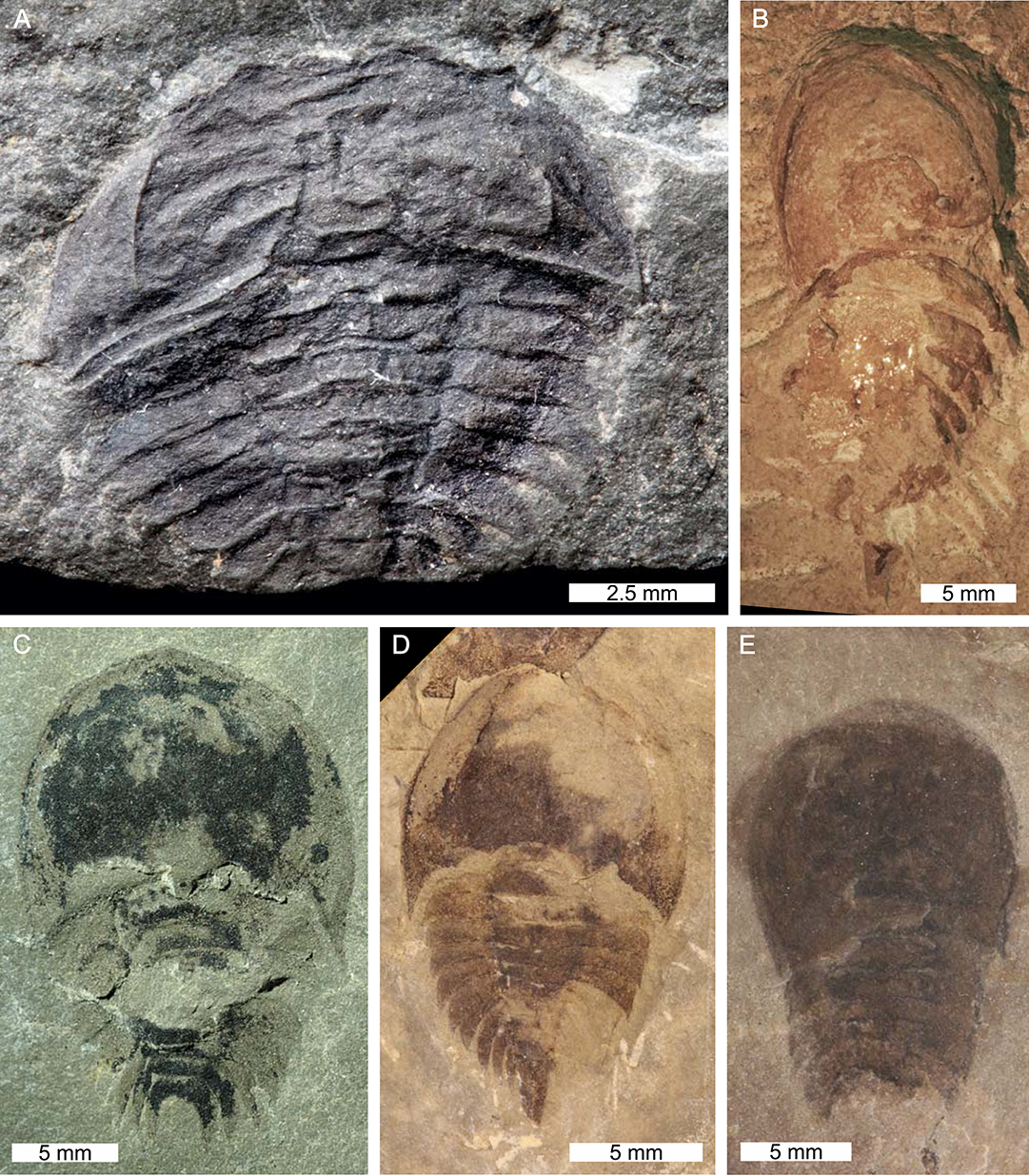
Figure 9. Species within Pseudoniscus. (A) Pseudoniscus falcatus from the Silurian-aged Patrick Burn Formation, Scotland, UK. NHMUK PI. In. 44122, holotype. (B) Pseudoniscus aculeatus from the Silurian-aged Oesel Group, Saaremaa Island, Estonia. AMNH 029281. (C,D) Pseudoniscus roosevelti from the Silurian-aged Vernon Shale, New York, USA. (C) NMS G.2004.45.5a. (D) NYSM 4762. (E) Pseudoniscus clarkei from the Silurian-aged Vernon Shale, New York, USA. NYSM E1030. (D,E) were photographed under ethanol. Photo credit: (A) Lucie Goodayle, NHM, London; (B,D,E) Russell Bicknell; (C) Bill Crighton.
Non-xiphosurid Xiphosura
First appearing in at least the Upper Ordovician of Canada and potentially the Lower Ordovician of Morocco the group contains taxa that have been considered stem-xiphosurids (Tables 1, 4, Figures 10–12; Rudkin and Young, 2009). There are eight genera and 10 species in this group. Two genera—Maldybulakia and Willwerathia—lack a family and the remaining six genera are considered stem-xiphosurids (formerly Kasibelinuridae, although this family was considered unhelpful by Bicknell et al., 2019c as it is a paraphyletic group). Non-xiphosurid xiphosurans are defined as chelicerates with a cardiac lobe extending to the anterior prosomal shield (Lamsdell, 2013). Species of this group can also have ophthalmic ridges, but this is taxon-specific and may be taphonomically controlled. Select taxa have preserved eyes: Kasibelinurus amicorum (Pickett, 1993; Dunlop and Selden, 1998) Lunataspis aurora (Rudkin et al., 2008; Rudkin and Young, 2009), and putatively Willwerathia laticeps (Anderson et al., 1998). Appendages are not known from this group of horseshoe crabs. Similar to synziphosurines, these taxa are mostly marine. Select non-xiphosurid xiphosurans, such as Lu. aurora, show a remarkable morphological similarity to xiphosurids (Rudkin et al., 2008).
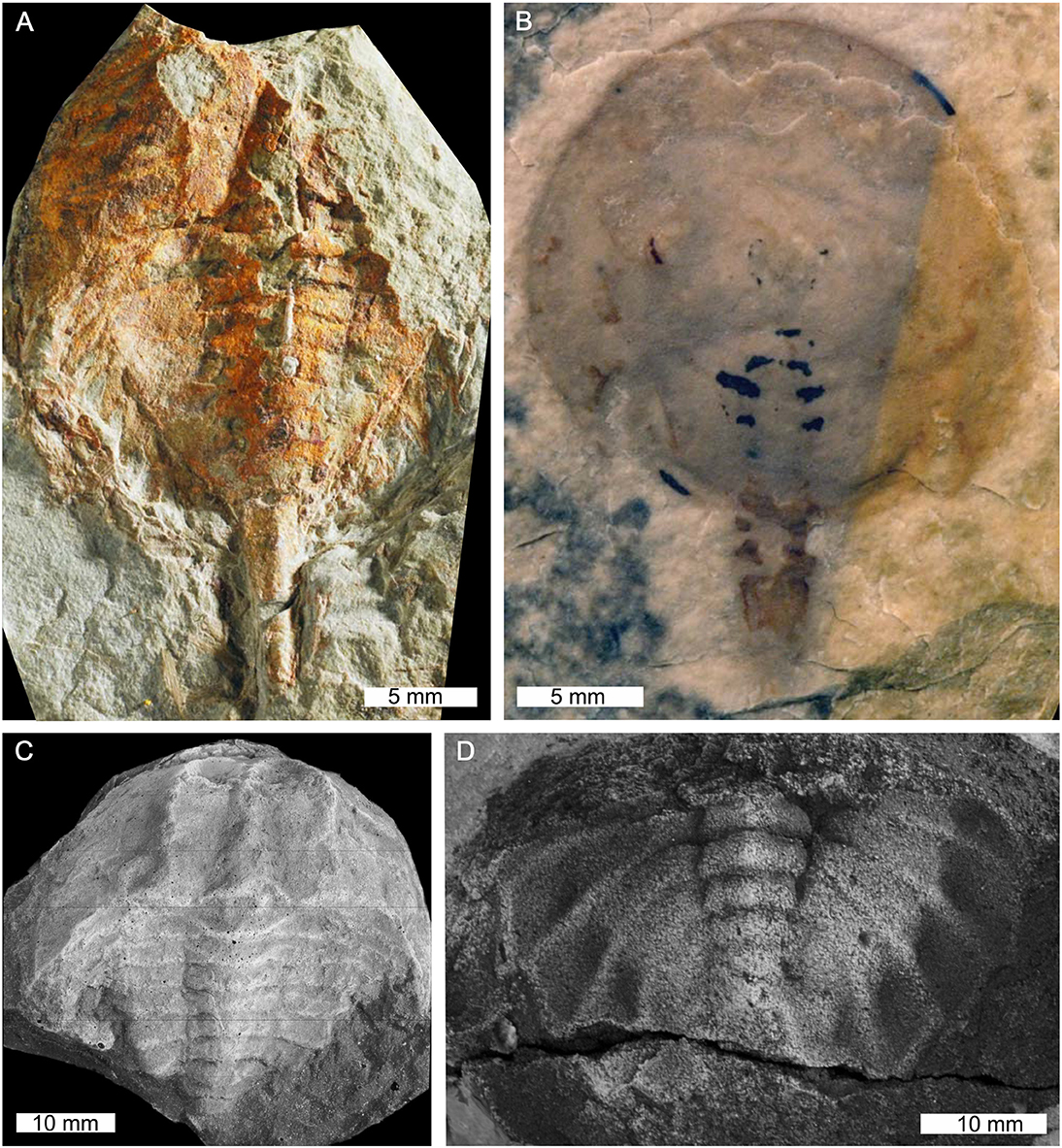
Figure 10. Stem xiphosurids from Canada and the USA. (A) Pickettia carteri from the Devonian-aged Cattaraugus Formation, Pennsylvania, USA. BMSC E 9644, holotype. (B) Lunataspis aurora from the Ordovician-aged Churchill River Group, Canada. MM I-4000A, holotype. (C) “Belinurus” alleghenyensis from the Devonian-aged Chadakoin Formation, New York, USA. Cast of CM11065, holotype. (D) Elleria morani from the Devonian-aged Venango Formation, Pennsylvania, USA. CM11574, holotype. (C,D) were coated with ammonium chloride sublimate. Photo credit: (A) KC Kratt; (B) Permission to reproduce photographs granted by Graham Young and the Manitoba Museum; (C,D) Russell Bicknell.
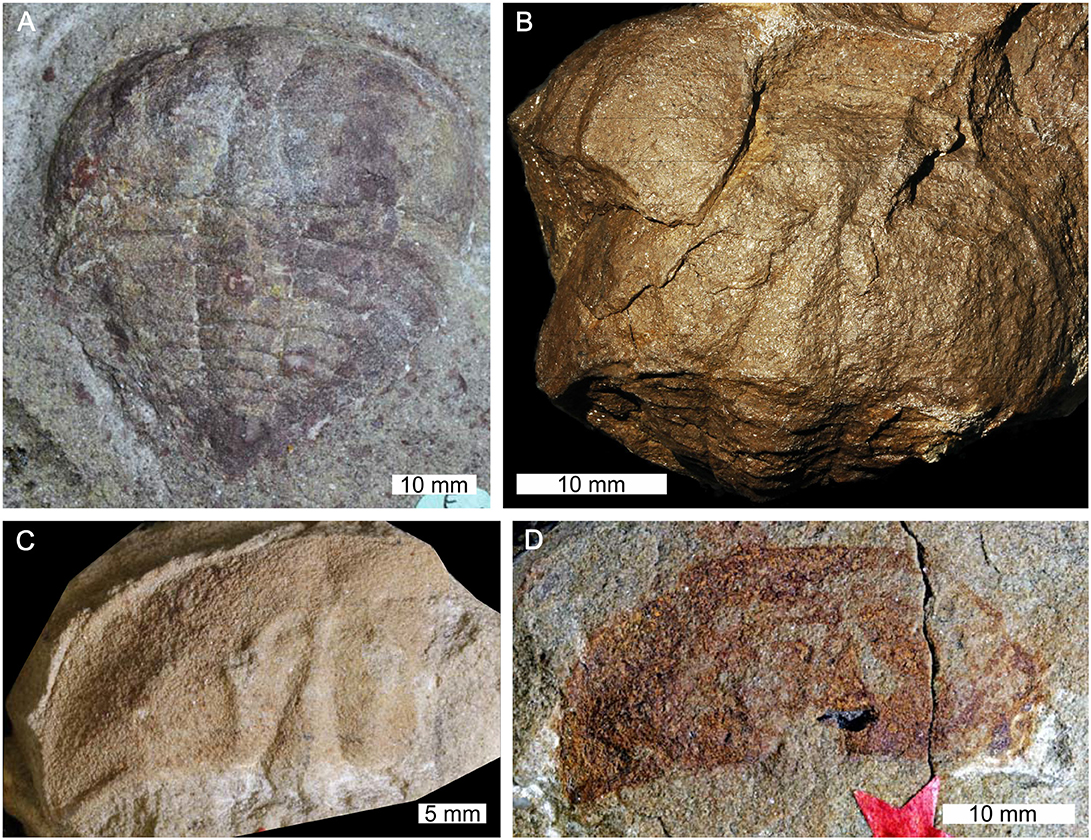
Figure 11. Non-xiphosurid xiphosuran species from Australia and USA. (A) Kasibelinurus amicorum from the Devonian-aged Mandagery Sandstone, Australia. AM F68969, holotype. (B) “Kasibelinurus” randalli from the Devonian-aged Chadakoin Formation, Pennsylvania, USA. (B) USNM PAL 484524. (C,D) “Kasibelinurus” randalli from the Devonian-aged Venango Formation, Pennsylvania, USA. (C) YPM IP 09010, holotype. (D) YPM IP 30656, paratype. Photo credit: (A) Josh White; (B–D) Russell Bicknell.
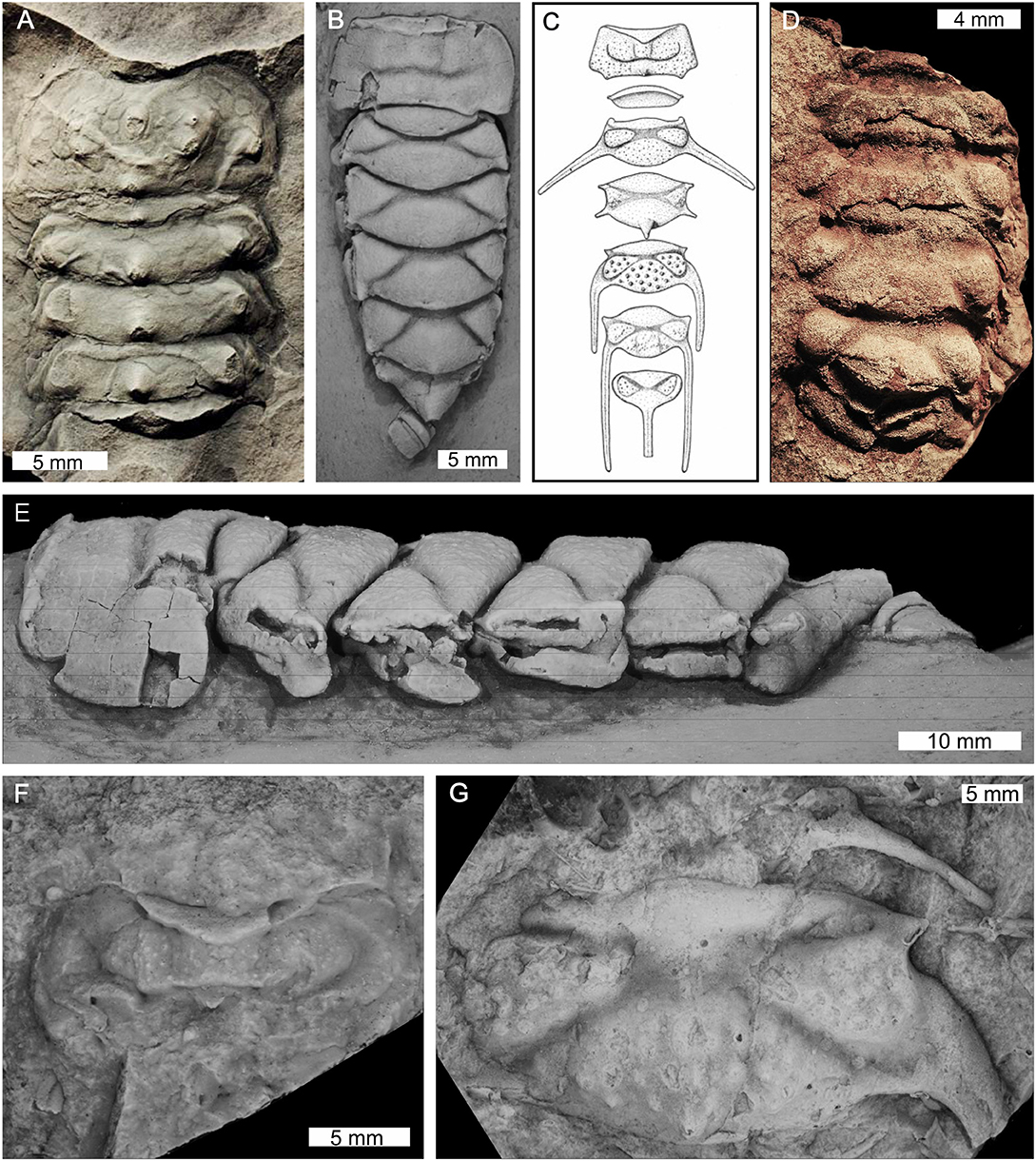
Figure 12. Xiphosuran taxa within genera Maldybulakia and Willwerathia. (A) Willwerathia laticeps from the Devonian-aged Klerf Formation, Germany. Cast of Leunissen collection specimen SLK lb, cast number SPW 1308-D. (B,E) Maldybulakia malcomi from the Devonian-aged Boyd Volcanic Complex, NSW, Australia. AM F102533, holotype. (B) Dorsal view. (E) Lateral view. (C,F,G) Maldybulakia angusi from the Devonian-aged Sugarloaf Creek Formation, NSW, Australia. (C) Reconstruction presented in Edgecombe (1998b, Figure 12). (F) AM F102560. (G) AM F102565, cast of holotype. (D) Maldybulakia mirabilis from the Devonian-aged Sheshenkarinskoy Suite, Kazakhstan. PIN No. 249/1, holotype. (B,E–G) Coated in ammonium chloride sublimate. (B,E–G) Converted to gray scale. Photo credit: (A) Markus Poschmann; (B,E–G) Patrick Smith; (C) Permission to use reconstruction granted by Gregory Edgecombe, (D) Alexander S. Alekseev.
Xiphosurida
True horseshoe crabs are an extant order that first appeared in the Devonian (Figure 1). Key characteristics of true horseshoe crabs are a large, keeled, crescentic cephalothorax with anteriorly located lateral compound eyes, a thoracetron of fused tergites containing one or two sections, and a styliform telson (Anderson and Selden, 1997; Rudkin et al., 2008; Briggs et al., 2012; Lamsdell, 2016). There are 30 genera and at least 82 species in Xiphosurida that are arrayed across the two suborders Belinurina and Limulina (Tables 5–7). Belinurina comprises only the family Belinuridae. Limulina comprises the superfamily Limuloidea, which includes Austrolimulidae, Limulidae, Paleolimulidae, and Rolfeiidae, and the genera Bellinuroopsis and Valloisella (sensu Lamsdell, 2016).
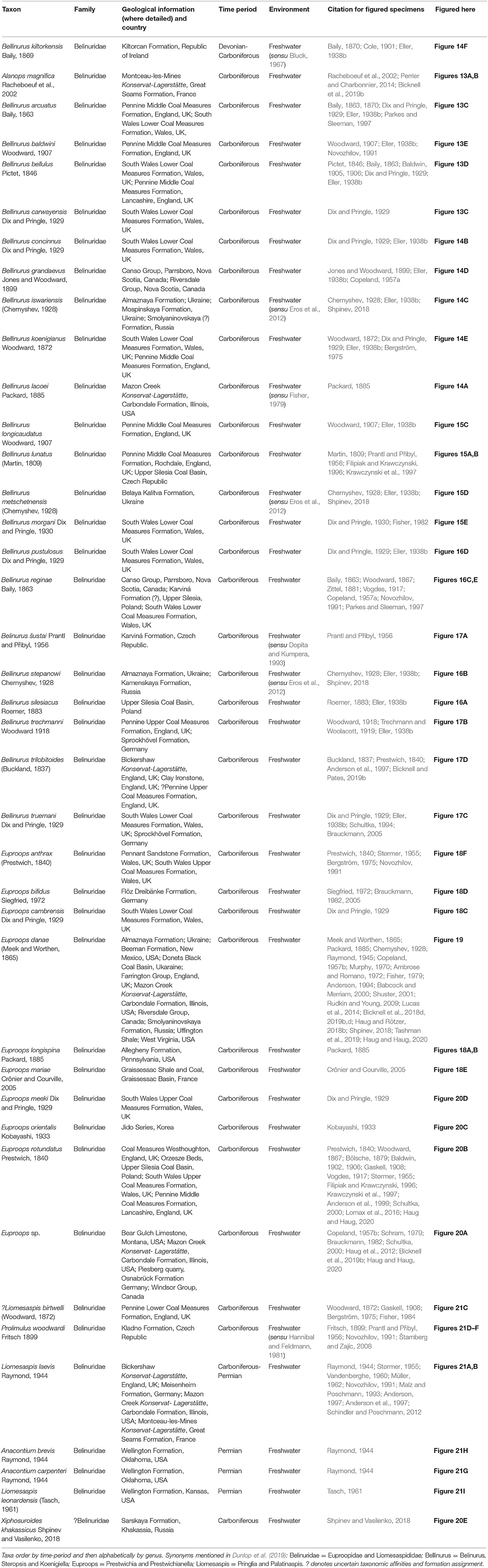
Table 5. Sub-order Belinurina after Dunlop et al. (2019).
Belinurina
All taxa within this sub-order are members of the family Belinuridae. The fossil record of Belinuridae spans possibly from latest Devonian, with the example of Bellinurus kiltorkensis (Eller, 1938b), through to the Carboniferous and the Permian (Figure 1) and this family has the second largest generic diversity in Xiphosurida, with seven genera Alanops, Anacontium, Bellinurus, Euproops, Liomesaspis, Prolimulus, and Xiphosuroides, and 37 named species (Table 5, Figures 13–21). Belinurids have domed cephalothoraxes with flattened margins, genal spines that are either flat, posteriorly extending, or vestigial (Størmer, 1955), and ophthalmic ridges that curve posteriorly from the lateral compound eyes (Størmer, 1955; Fisher, 1977; Haug et al., 2012), which sometimes extend into ophthalmic spines (Fisher, 1977). The thoracetron is fused and ranges between round, trapezoidal, or triangular shapes (Størmer, 1955). Euproops and Bellinurus species have between five and seven articulated and expressed thoracetronic tergites with lateral spines (Størmer, 1955; Bergström, 1975; Fisher, 1977; Haug et al., 2012; Lamsdell, 2016). Anacontium, Liomesaspis, Prolimulus, and Xiphosuroides species have no exposed tergites and no marginal spines (Størmer, 1955; Shpinev and Vasilenko, 2018). Where known, the telson is styliform and elongate for all genera (Bergström, 1975). Appendages are known from select belinurids. Chelicerae and prosomal appendages are known from Euproops danae (Mazon Creek Konservat-Lagerstätte, Carbondale Formation, USA; Schultka, 2000; Haug et al., 2012; Haug and Rötzer, 2018b; Bicknell et al., 2019b) and Alanops magnificus (Montceau-les-Mines Konservat-Lagerstätte, Great Seams Formation, France; Racheboeuf et al., 2002; Bicknell et al., 2019b).
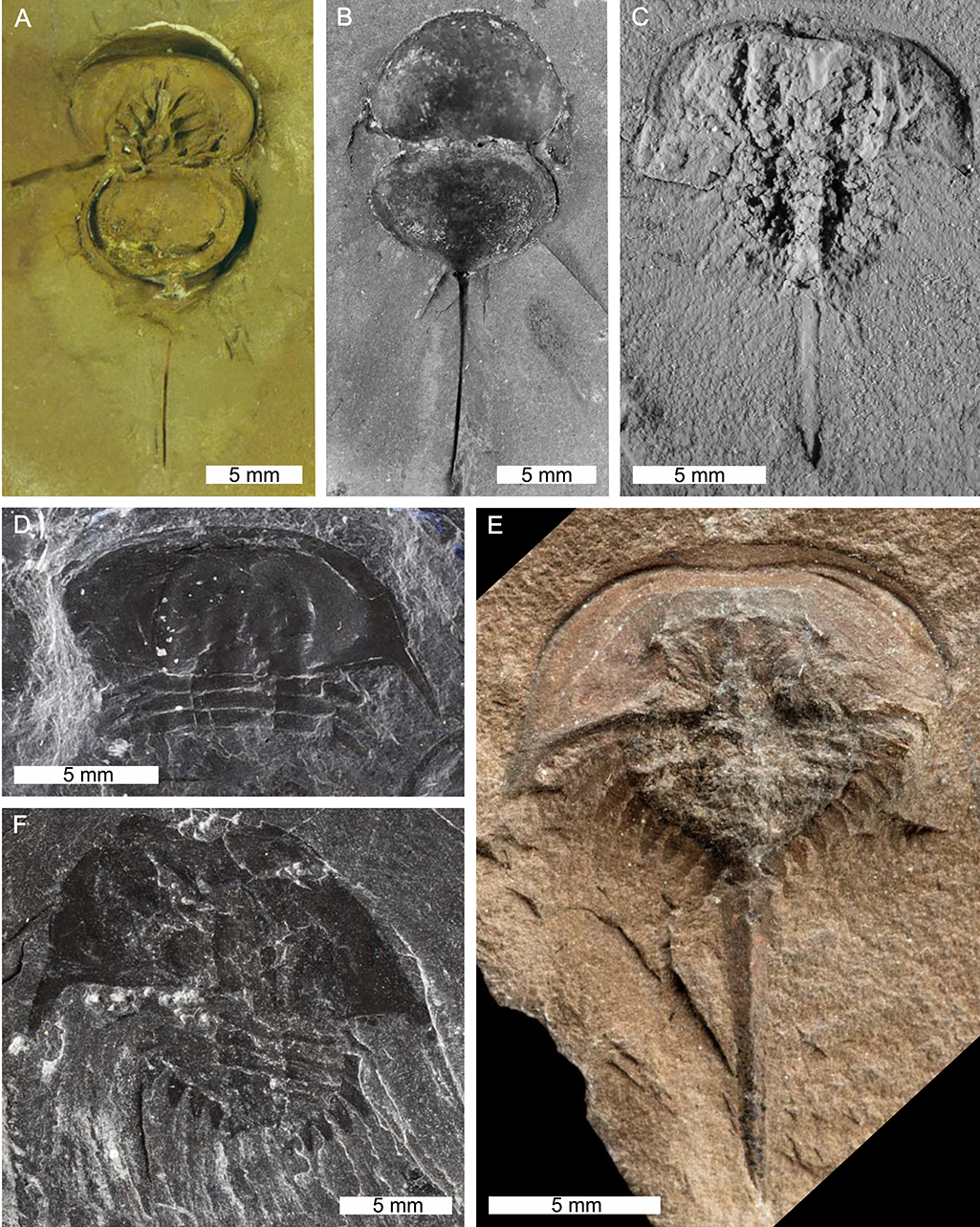
Figure 13. Belinurid species in the genera Alanops and Bellinurus. (A,B) Alanops magnifica from the Carboniferous-aged Montceau-les-Mines Konservat-Lagerstätte, Great Seams Formation, France. (A) MNHN SOT001784, paratype, ventral view. Note appendages. (B) MNHN SOT002154, paratype, dorsal view. (C) Bellinurus arcuatus from the Pennine Middle Coal Measures Formation, England, UK. AM F29886. (D) Bellinurus bellulus from the Carboniferous-aged South Wales Lower Coal Measures Formation, Wales, UK. NMW 70.17. G9. (E) Bellinurus baldwini from the Carboniferous-aged Pennine Middle Coal Measures Formation, England, UK. NHMUK PI. In. 18572, holotype. (F) Bellinurus carwayensis from the Carboniferous-aged South Wales Lower Coal Measures Formation, Wales, UK. NMW 29.197.G3, holotype. (B,C) Converted to gray scale. (C) Coated in ammonium chloride sublimate. Photo credit: (A,B) Dominique Chabard; (C) Patrick Smith, (D,F) Stephen Pates; (E) Lucie Goodayle, NHM, London.
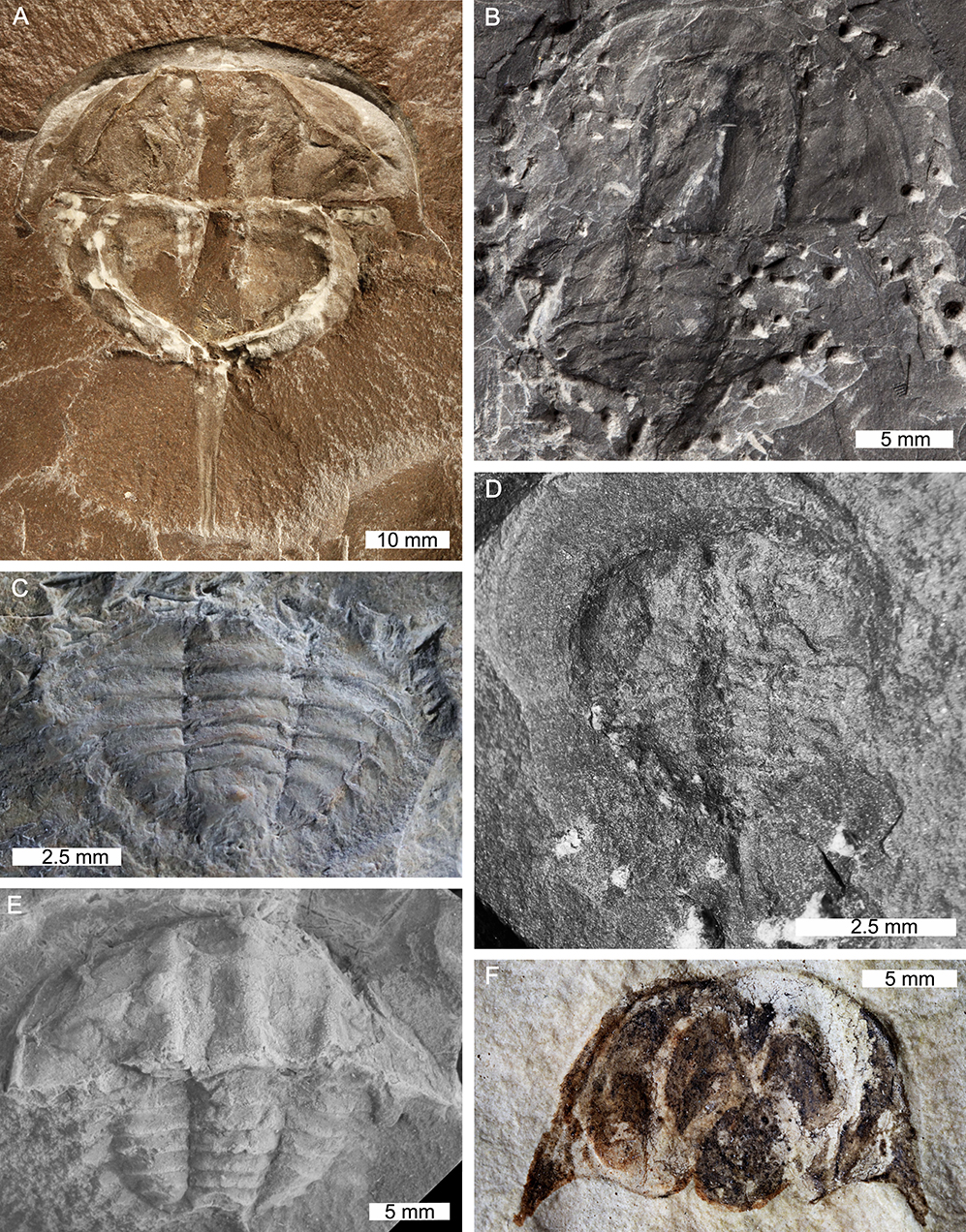
Figure 14. Bellinurus species from Canada, UK, Ukraine, and USA. (A) Bellinurus lacoei from the Carboniferous-aged Mazon Creek Konservat-Lagerstätte, Carbondale Formation, Illinois, USA. USNM 38861, cotype. (B) Bellinurus concinnus from the Carboniferous-aged South Wales Lower Coal Measures Formation, Wales, UK. BGS.GSE 48775, holotype. (C) Bellinurus iswariensis from the Carboniferous-aged Almaznaya Formation, Ukraine. TsNIGR 3/2095. (D) Bellinurus grandaevus from the Carboniferous-aged Canso Group, Nova Scotia, Canada. GSC 12806, hypotype. (E) Bellinurus koenigianus from the Carboniferous-aged Coal Measures Formation, England, UK. CM 11066. (F) Bellinurus kiltorkensis from the Devonian to Carboniferous-aged Kiltorcan Formation, Ireland. NHMUK PI. In. 25931, cast of original specimen. (D,E) Converted to gray scale. Photo credit: (A,C,E) Russell Bicknell; (B) GB3D image, permission given by Mike Howe © 2018 JISC GB3D Type Fossils Online project partners (Amgueddfa Cymru–National Museum Wales); (D) Jodie Francis; (F) Lucie Goodayle, NHM, London.
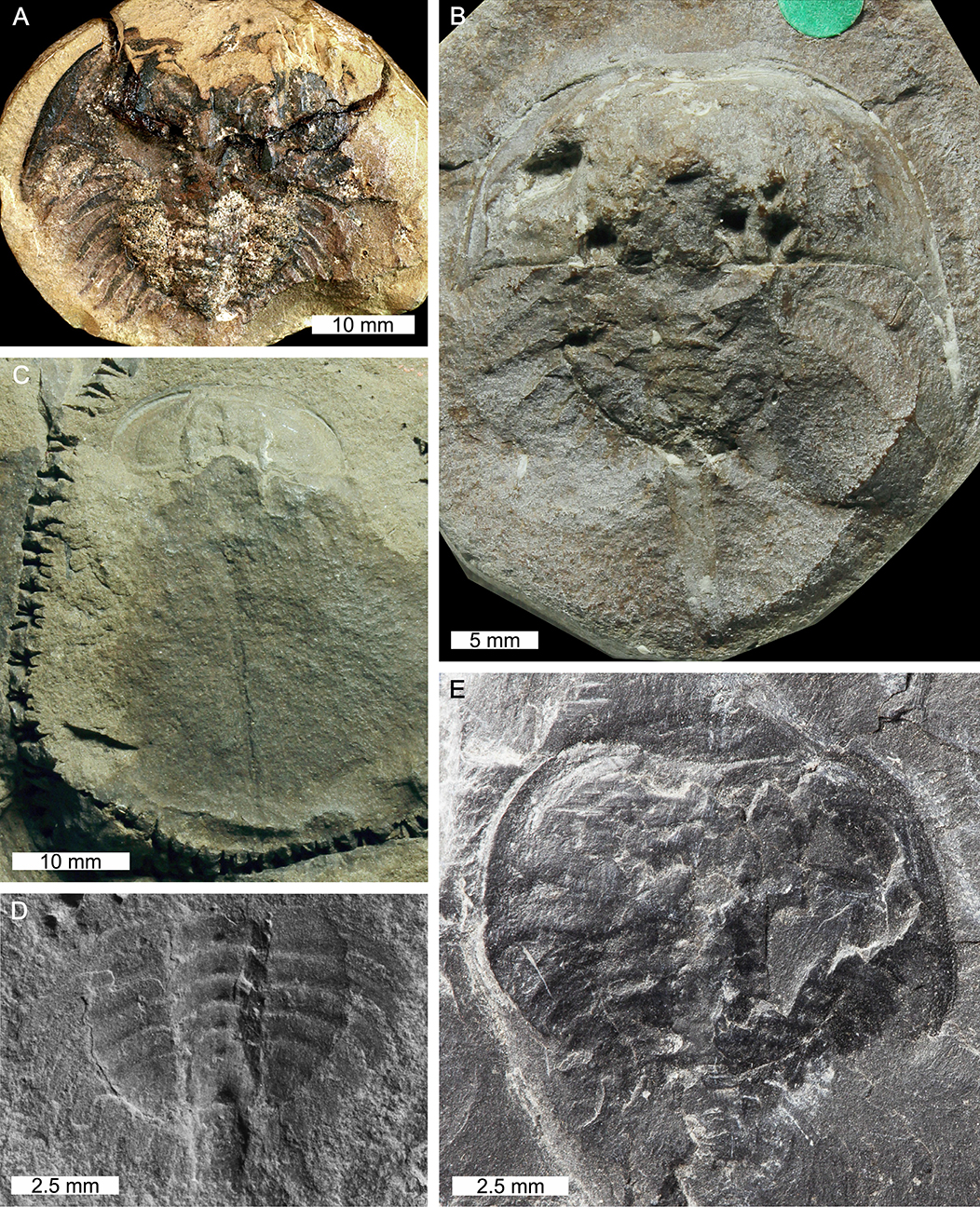
Figure 15. Bellinurus species from the Czech Republic, UK, and Ukraine. (A,B) Bellinurus lunatus. (A) Specimen from Carboniferous-aged Upper Silesia Coal Basin, Czech Republic. GIUS 5-845/7. (B) Specimen from Pennine Middle Coal Measures Formation, England, UK. NHMUK PI. I. 2754. (C) Bellinurus longicaudatus from Carboniferous-aged Pennine Middle Coal Measures Formation, England, UK. NHMUK PI. In. 18563, holotype. (D) Bellinurus metschetnensis from Carboniferous-aged Belaya Kalitva Formation, Ukraine. TsNIGR 8/2095. (E) Bellinurus morgani from Carboniferous-aged South Wales Lower Coal Measures Formation, Wales, UK. BGS.GSE 49362, holotype. (D,E) Converted to gray scale. Photo credit: (A) Błaże Błażejowski; (B,C) Stephen Pates; (D) Russell Bicknell; (E) GB3D image, permission given by Mike Howe © 2018 JISC GB3D Type Fossils Online project partners (Amgueddfa Cymru – National Museum Wales).
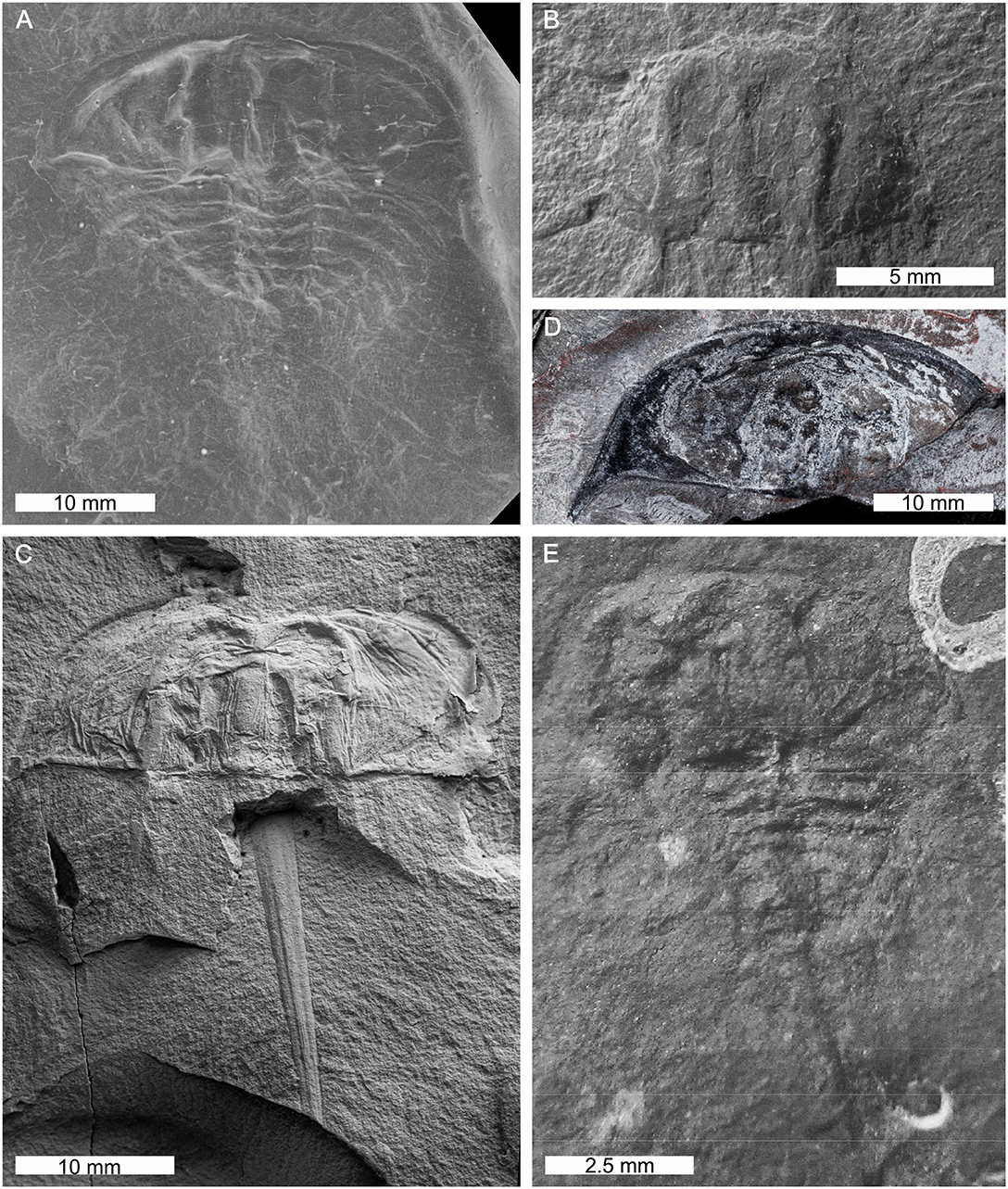
Figure 16. Bellinurus species from Canada, Poland, UK, and Ukraine. (A) Bellinurus silesiacus from the Carboniferous Upper Silesia Coal Basin, Poland. MB.A.1091, cast of original. (B) Bellinurus stepanowi from the Carboniferous-aged Almaznaya Formation, Ukraine. TsNIGR 6/2095. (C,E) Bellinurus reginae. (C) Specimen from Karviná Formation(?), Upper Silesia, Poland. MB.A.1090. (E) Specimen from Carboniferous-aged Canso Group, Nova Scotia, Canada. GSC 12803. (D) Bellinurus pustulosus from Carboniferous-aged South Wales Lower Coal Measures Formation, Wales, UK. NMW 29.197.G2, holotype. ? denotes uncertain formation assignment. (A–C,E) Converted to gray scale. Photo credit: (A) Andreas Abele; (B) Russell Bicknell; (C) Christian Neumann; (D) Stephen Pates; (E) Matt Stimson. (A,B,C,E) Converted to gray scale.
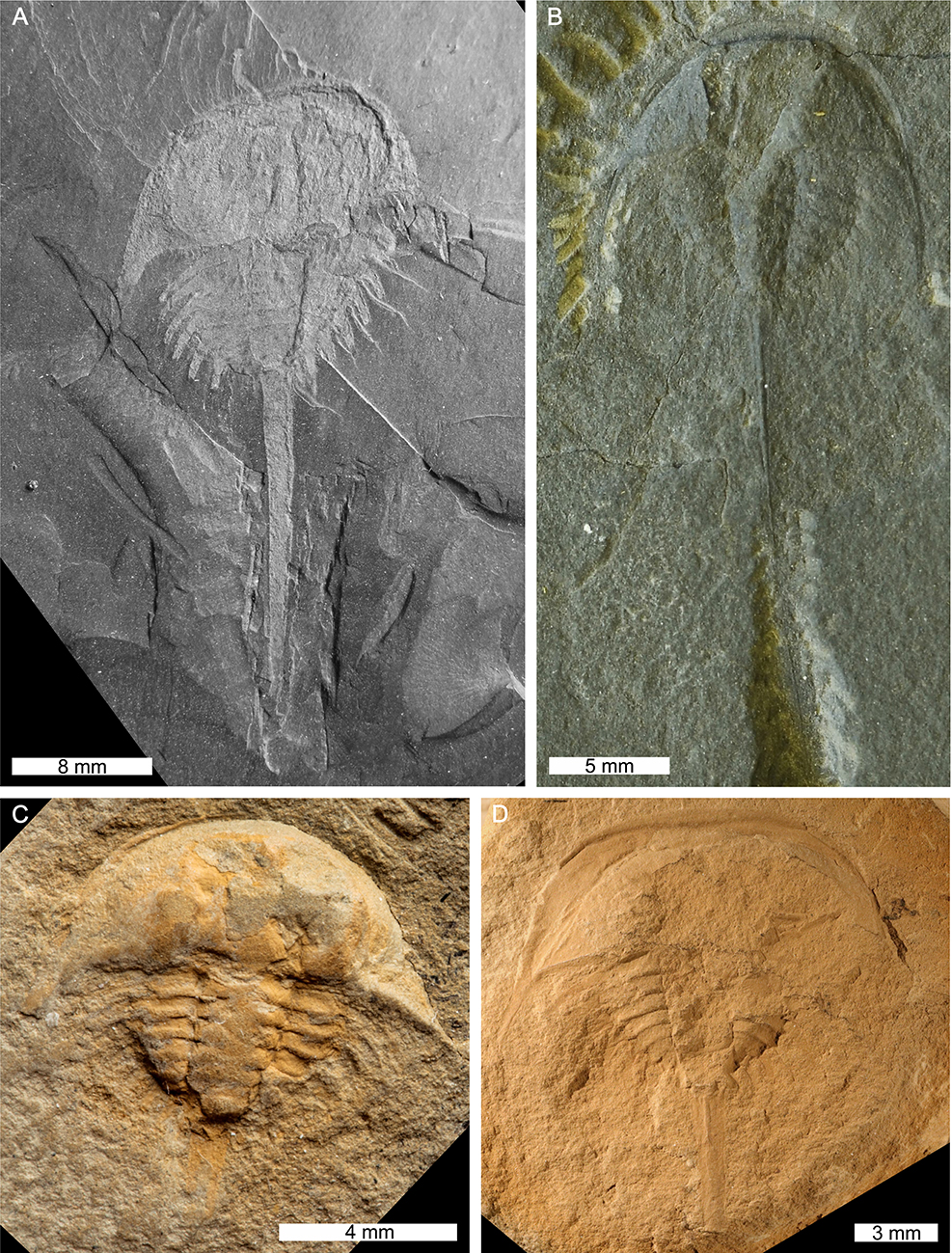
Figure 17. Bellinurus species from the Czech Republic, Germany, and UK. (A) Bellinurus šustai from the Carboniferous-aged Karviná Formation, Czech Republic. MMO B 976, holotype. (B) Bellinurus. cf. truemani from the Carboniferous-aged Sprockhövel Formation, Germany. SMF Viii 314. (C) Bellinurus trechmanni from the Carboniferous-aged Pennine Upper Coal Measures Formation, England, UK. NHMUK PI. In. 18487, holotype. (D) Bellinurus trilobitoides from the Carboniferous-aged ?Pennine Upper Coal Measures Formation, England, UK. LL.111267a. (A) Converted to gray scale. ? denotes uncertain formation assignment. Photo credit: (A) Mertová Eva; (B) Monica Solorzano-Kraemer; (C) Lucie Goodayle; (D) Russell Bicknell.
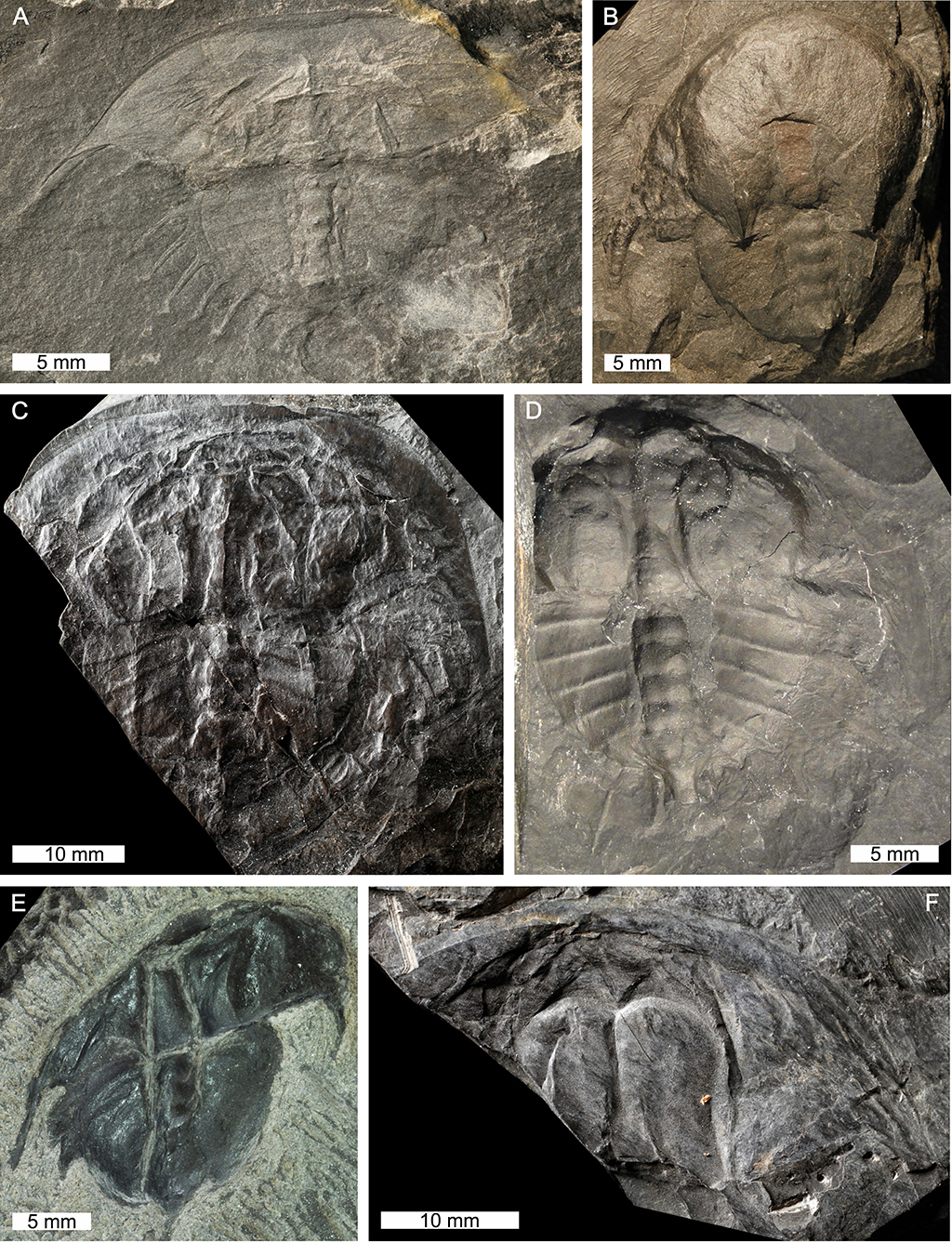
Figure 18. Euproops species from France, Germany, UK, and USA. (A,B) Euproops longispina from the Carboniferous-aged Allegheny Formation, Pennsylvania, USA. (A) USNM 38857, cotype. (B) USNM 38858, cotype. (C) Euproops cambrensis from the Carboniferous-aged South Wales Lower Coal Measures Formation, Wales, UK. NMW 29.198.G1, holotype. (D) Euproops bifidus from the Carboniferous-aged Flöz Dreibänke Formation, Germany. B7.135 holotype. (E) Euproops mariae from the Carboniferous-aged Graissessac Shale and Coal, Graissessac Basin, France. USTL-CC026, holotype. (F) Euproops cf. anthrax from the Carboniferous-aged South Wales Upper Coal Measures Formation, Wales, UK. NMW 27.177.G3. Photo credit: (A,B) Russell Bicknell; (C,F) Stephen Pates; (D) Markus Bertling; (E) Jessie Cuvelier.
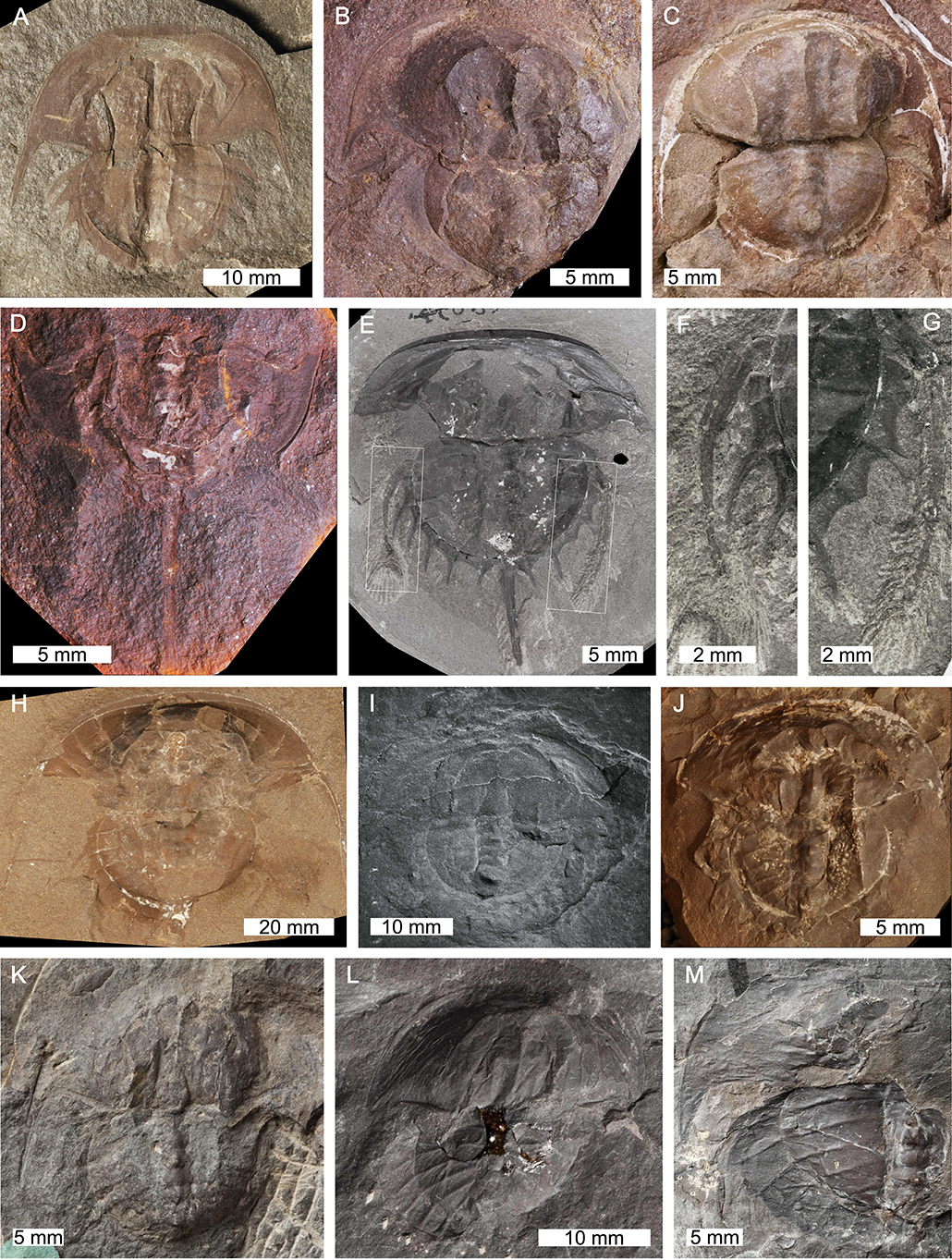
Figure 19. Euproops danae from Carboniferous-aged deposits and select species that have been synonymised with E. danae. (A) Specimen from Carboniferous-aged lower Mercer Shale, Pennsylvania, USA. USNM 697642. (B–H,J) Specimens from the Carboniferous-aged Mazon Creek Konservat-Lagerstätte, Carbondale Formation, Illinois, USA. (B) YPM IP 16912. (C) YPM IP 25590. (D) Specimen that is completely enrolled, ideas mentioned in Fisher (1977) and Anderson (1994) and discussed in Haug et al. (2012). YPM IP 50963. (E–G) Specimen with cephalothoracic appendages preserved. YPM IP 28514 (E) Complete specimen. (F) Close up of left appendage. (G) Close up of right appendage. (H) USNM 38855, hypotype. (J) CM 11061. (M) Specimen from the Carboniferous-aged South Wales Upper Coal Measures Formation, Wales, UK. NMW 70.17.G11 (I) Euproops darrahi=E. danae from the Carboniferous-aged Conemaugh Formation, Pennsylvania, USA. MCZ 109528, holotype. (K) Euproops gwenti=E. danae from the Carboniferous-aged South Wales Upper Coal Measures Formation, Wales, UK. BGS.GSE 48524, holotype. (L) Euproops graigola =E. danae from the Carboniferous-aged South Wales Upper Coal Measures Formation, Wales, UK. BGS.GSE 25424, holotype. (I) Converted to gray scale. (I) Coated with ammonium chloride sublimate. Photo credit (A–H,J) Russell Bicknell; (I,M) Stephen Pates; (K,L) GB3D image, permission given by Mike Howe © 2018 JISC GB3D Type Fossils Online project partners (Amgueddfa Cymru – National Museum Wales).
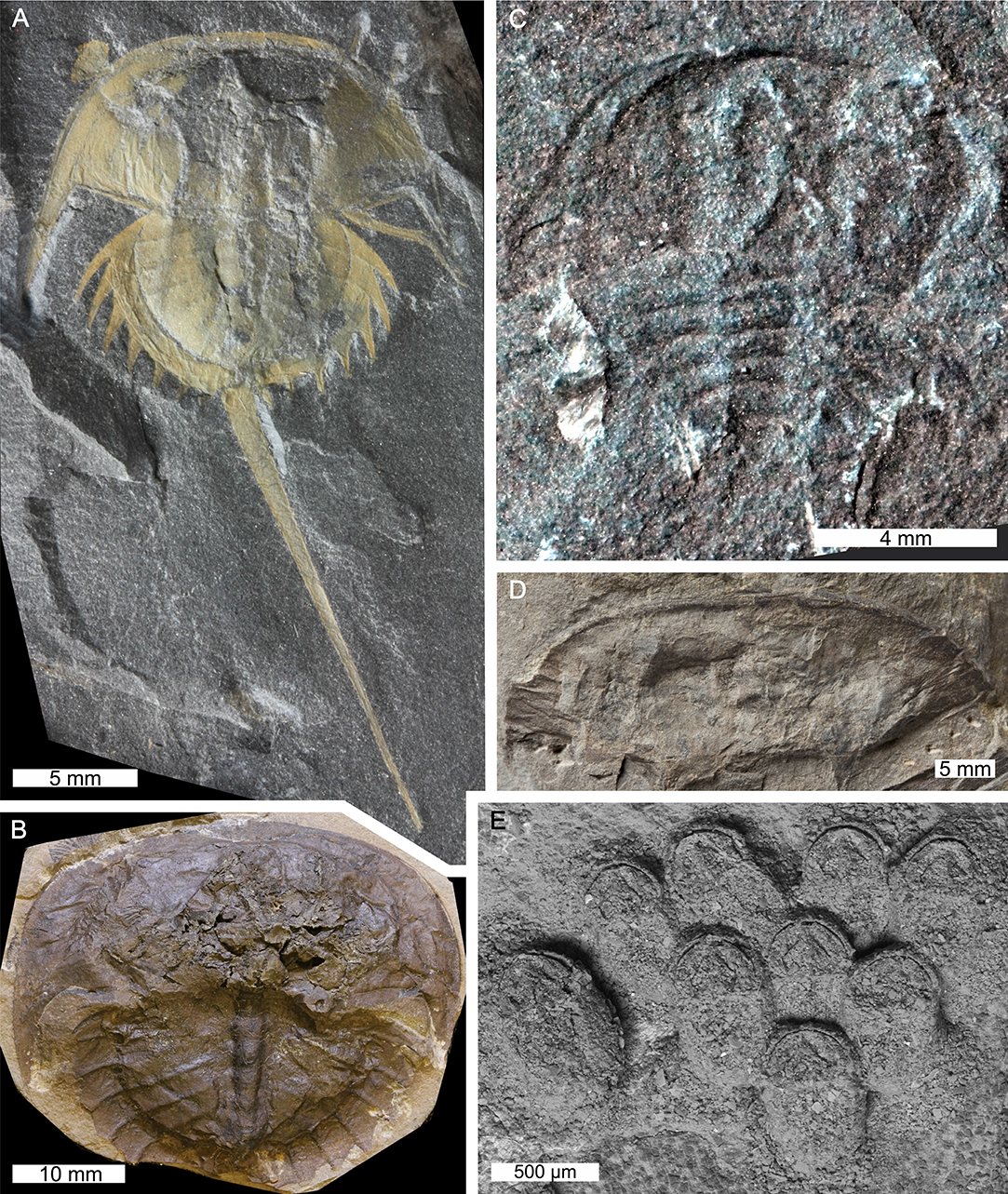
Figure 20. Euproops species from Germany, Korea (formerly the Chōsen region) and UK, and Xiphosuroides. (A) Euproops sp., so call “Piesproops”, from the Carboniferous-aged Osnabrück Formation, Germany. MAS Pal. 1308. (B) Euproops rotundatus specimens from the Carboniferous-aged Pennine Upper Coal Measures Formation (?) England, UK. YPM IP 428963. (C) Euproops orientalis from the Carboniferous-aged Jido Series, Korea. UMUT PA 00433, holotype. (D) Euproops meeki from the Carboniferous-aged South Wales Upper Coal Measures Formation, Wales, UK. BGS.GSE 48529, holotype. (E) Xiphosuroides khakassicus from the Carboniferous-aged Sarskaya Formation, Khakassia, Russia. Scanning electron microscope image. PIN 384/211, holotype. (E) Converted to gray scale. ? denotes uncertain formation assignment. Photo credit (A) Angelika Leipner; (B) Russell Bicknell; (C) Tai Kubo; (D) GB3D image, permission given by Mike Howe © 2018 JISC GB3D Type Fossils Online project partners (Amgueddfa Cymru – National Museum Wales); (E) Constantine Tarásenko.
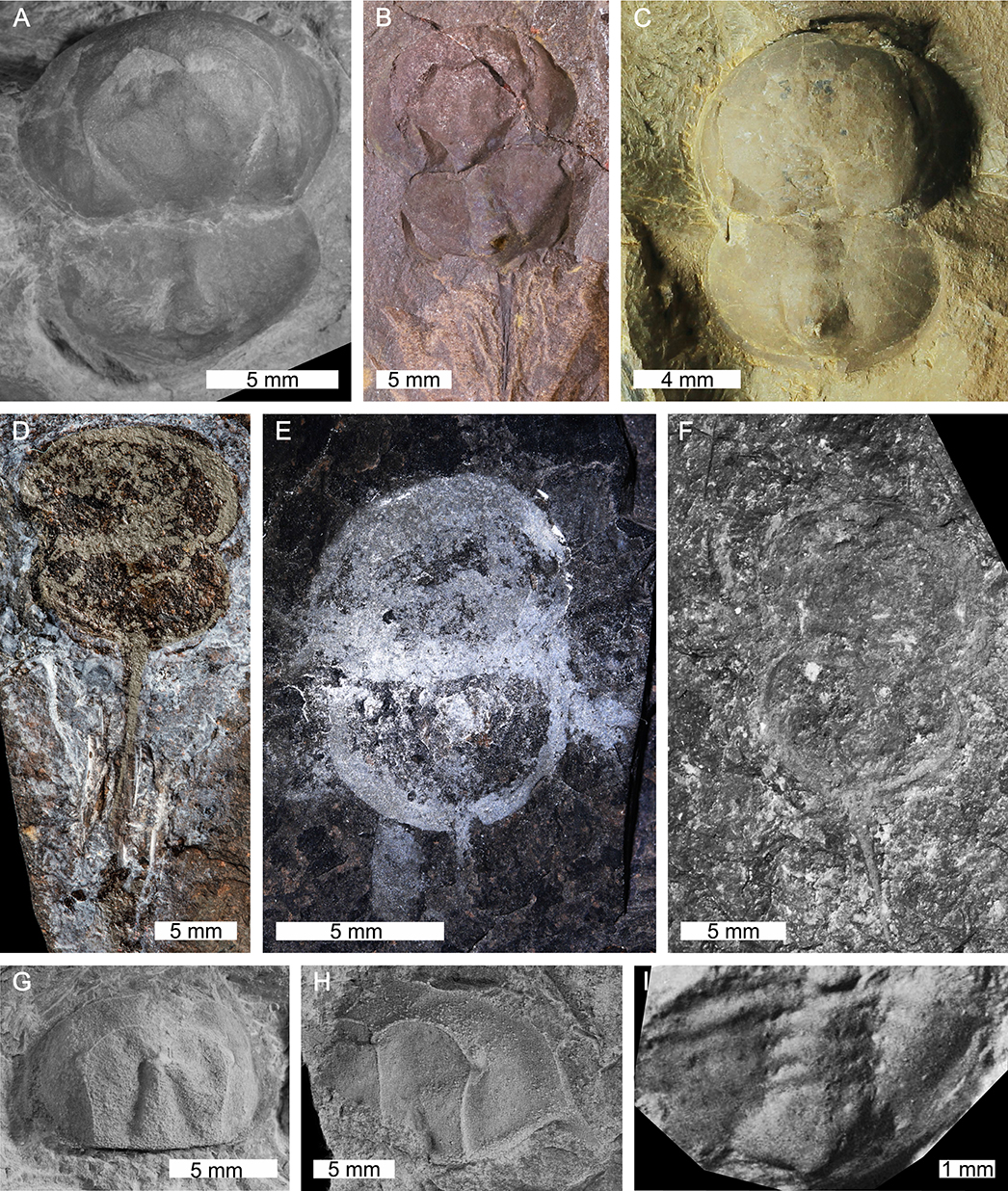
Figure 21. Belinurids in the genera Anacontium, Liomesaspis, and Prolimulus. (A,B) Liomesaspis laevis specimens from the Carboniferous-aged Mazon Creek Lagerstätte, Illinois, USA. (A) MCZ 109536, holotype. (B) YPM IP 16913, paratype. (C) ?Liomesaspis birtwelli from the Carboniferous-aged Pennine Middle Coal Measures Formation, England, UK. NHMUK PI. I. 13882. (D–F) Prolimulus woodwardi from the Carboniferous-aged Kladno Formation, Czech Republic. (D) NHMUK PI. In. 18588, syntype. (E) MCZ 109537, hypotype. (F) MB.A.1989. (G) Anacontium carpenteri from the Wellington Formation, Oklahoma, USA. MCZ 109531, paratype. (H) Anacontium brevis from the Permian-aged Wellington Formation, Oklahoma, USA. MCZ 109533, holotype. (I) Liomesaspis leonardensis from the Permian-aged Wellington Formation, Kansas, USA. Image reproduced from Tasch (1961) as the specimen has been lost (C.D. Burke, pers. comms. 2018). W.U. 200, holotype. (A,F) Converted to gray scale. ? denotes uncertain taxonomic assignment. (G,H) Coated with ammonium chloride sublimate and converted to gray scale. Photo credit: (A,B) Russell Bicknell; (C) Monica (C,E,G,H) Stephen Pates; (D) Lucie Goodayle, NHM, London; (F) Lorenzo Lustri; (G) Mark Renczkowski; (I) Permission to reproduce holotype granted by Kathleen Huber.
Belinurids are an extremely well-studied group of xiphosurids reflecting the expansive literature on the life mode, ontogeny and taxonomy of the group (e.g., Fisher, 1977, 1979; Anderson, 1994; Haug et al., 2012; Haug and Rötzer, 2018a,b; Bicknell et al., 2019d). Belinurids were the most successful horseshoe crab group in exploiting freshwater conditions (Fisher, 1984; Lamsdell, 2016). It has been suggested, that select taxa were likely effective at sub-aerial activity (more so than extant taxa) as cephalothoracic appendages were arranged similarly to extant xiphosurids, permitting more on-land exploration than is observed in extant taxa (Racheboeuf et al., 2002; Haug and Rötzer, 2018b). Euproops danae specifically had morphological characteristics that may have mimicked co-occurring leaves and arachnids (Dunbar, 1923; Fisher, 1979; Todd, 1991; Filipiak and Krawczynski, 1996), although this suggestion remains to be thoroughly explored. The ontogeny of fossil belinurids has been documented using Euproops sp. from the Osnabrück Formation (Pennsylvanian) of Germany (Haug et al., 2012), and E. danae from the Mazon Creek Konservat-Lagerstätte (Pennsylvanian) of the USA (Haug and Rötzer, 2018b). The apparently large belinurid diversity almost definitely reflects over-splitting during the early twentieth century (Anderson, 1997; Lamsdell, 2016) and grouping Euproopidae with Belinuridae (Dunlop et al., 2019). A re-evaluation of the family is therefore needed (Selden and Siveter, 1987) and should build on Anderson (1994); Haug et al. (2012), and Haug and Rötzer (2018b) who synonymised Euproops species after determining that cephalothoracic compression produced variable, supposedly species-diagnostic features (Haug and Rötzer, 2018b; Shpinev, 2018).
Limulina
This sub-order comprises the superfamily Limuloidea, the families Paleolimulidae and Rolfeiidae, and the genus Bellinuroopsis. Limulina has a fossil record ranging from the Devonian to Recent. The diagnostic feature that separates Limuloidea from Belinurina is the fusion of the two most posterior thoracetronic tergites (sensu Lamsdell, 2016).
Paleolimulidae
This family has a fossil record spanning the Carboniferous to Permian (Table 6). Three genera construct Paleolimulidae: Moravurus, Paleolimulus, and Xaniopyramis and there are six species within these three genera (Figure 22). The morphology of paleolimulids broadly resembles that of modern horseshoe crabs, but members of this group are smaller than extant taxa (Størmer, 1955; Shuster, 2001). Paleolimulids have a domed cephalothorax, ophthalmic ridges that converge anteriorly to lateral compound eyes and genal spines that extend posteriorly as far as the fourth thoracic tergite (Lerner et al., 2016). The thoracetron is fused and has an angular axial section with transverse and longitudinal thoracetronic ridges occasionally present (Raymond, 1944; Siveter and Selden, 1987; Novozhilov, 1991), along with a styliform telson (Pickett, 1984; Seegis, 2014). Moveable thoracetronic spines are occasionally preserved (Seegis, 2014). Unique features of select taxa include the additional articulation between the thoracetron and telson known from Paleolimulus signatus and the expressed opercular (VIII) tergite producing a free thoracetronic lobe in Pa. woodae and Xaniopyramis linseyi (Størmer, 1952; Babcock et al., 2000; Lerner et al., 2016). Rare specimens preserve soft-parts. Paleolimulus signatus (Insect Hill Konservat-Lagerstätte, Wellington Formation, USA, Permian) preserves cephalothoracic and thoracetronic appendages (Dunbar, 1923; Raymond, 1944; Størmer, 1952; Babcock and Merriam, 2000; Bicknell et al., 2019b). These appendages are strikingly similar to modern horseshoe crabs (Størmer, 1955; Bicknell et al., 2019b). Xaniopyramis linseyi (Upper Limestone Group, Scotland, Carboniferous) preserves impressions of cephalothoracic appendage muscles (Siveter and Selden, 1987).
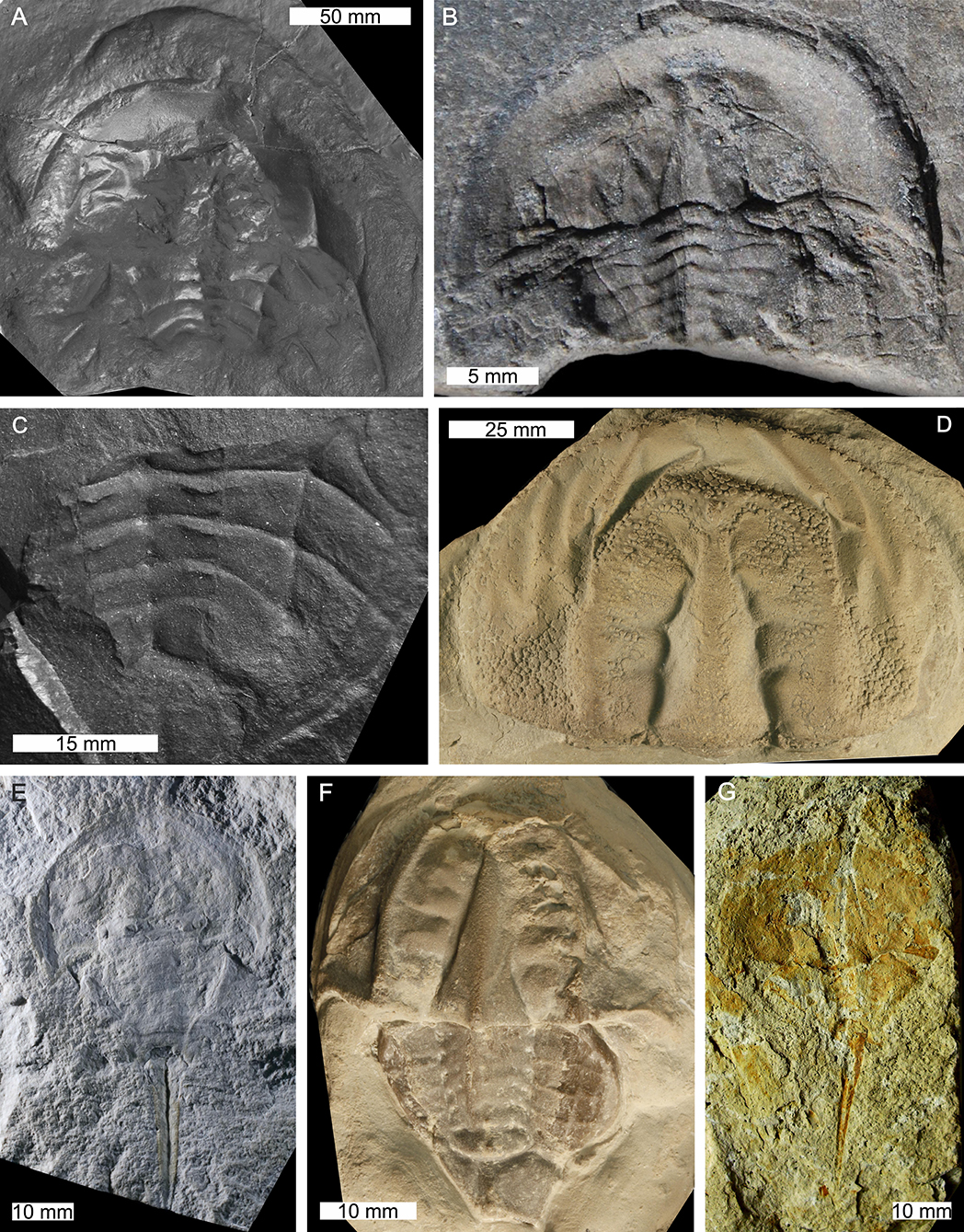
Figure 22. Examples of Carboniferous and Permian paleolimulids. (A) Xaniopyramis linseyi from the Carboniferous-aged Upper Limestone Group, Weardale, England, UK. OUMNH E.03994, rubber cast of holotype. (B) Paleolimulus woodae from the Carboniferous-aged Horton Bluff Formation, Nova Scotia, Canada. NSM005GF045.374, paratype. (C) Moravurus rehori from the Carboniferous-aged Kyjovice Formation, Czech Republic. MMO B 8169, holotype. (D,F) Paleolimulus signatus from the Carboniferous-aged Pony Creek Shale Konservat-Lagerstätte, Wood Siding Formation, Kansas, USA. (D) USNM 484411, hypotype. (F) USNM PAL 484408, hypotype. (E) ?Paleolimulus juresanensis from the Permian-aged Maltchev or Belogor Limestone Beds. CCMGE CM2/3694, holotype. (G) Paleolimulus kunguricus from the Permian-aged Philippovian Formation, Russia. GIN PH-18, holotype. ? denotes uncertain taxonomic assignment. Photo credit: (A) GB3D image, permission given by Mike Howe © 2018 JISC GB3D Type Fossils Online project partners (Amgueddfa Cymru – National Museum Wales); (B) Allan Lerner; (C) Mertová Eva; (D–F) Russell Bicknell; (G) Serge Naugolnykh.
Paleolimulid species were mostly marine taxa and their morphologies, similar to extant horseshoe crabs, reflect this life mode. They may have therefore variably explored swimming and burrowing life modes, with these ecological inferences related to the presence of movable thoracic spines (Siveter and Selden, 1987). Paleolimulus woodae lacked thoracetronic movable spines and may have been capable of swimming, while Xaniopyramis linseyi, adorned with large thoracetronic spines, would have likely burrowed (Siveter and Selden, 1987; Lerner et al., 2016). The diversity of Paleolimulidae has previously been overstated and Paleolimulus is now considered a paraphyletic group (Lamsdell, 2016; Lerner et al., 2017; Bicknell, 2019). Many paleolimulid forms are now considered to be austrolimulids (discussed below), so continued research into these taxa is needed to uncover the true disparity of forms within this family and diversity of both austrolimulids and paleolimulids (Bicknell, 2019).
Rolfeiidae
This monospecific family consists of Rolfeia fouldenensis and is known from the Carboniferous-aged Cementstones Group, Scotland (Table 6, Figure 23). The cephalothorax is domed, exhibiting small genal spines, and a thick cephalothoracic margin. The species has a cardiac lobe narrows anteriorly and ophthalmic ridges that cross the lateral compound eyes, converging at the cardiac lobe (Waterston, 1985). The thoracetron is fused with visible tergal divisions and the opercular tergite is fully expressed. Large fixed and small moveable thoracetronic spines are known from R. fouldenensis (Waterston, 1985; Selden and Siveter, 1987; Lamsdell, 2016) and the telson is styliform. Lamsdell (2016) suggested that transverse cephalothoracic ridge nodes were characteristic of the family; however, as the holotype considered here lack these features, this feature may be treated tentatively. Presently, no appendages are known from this group (Waterston, 1985).
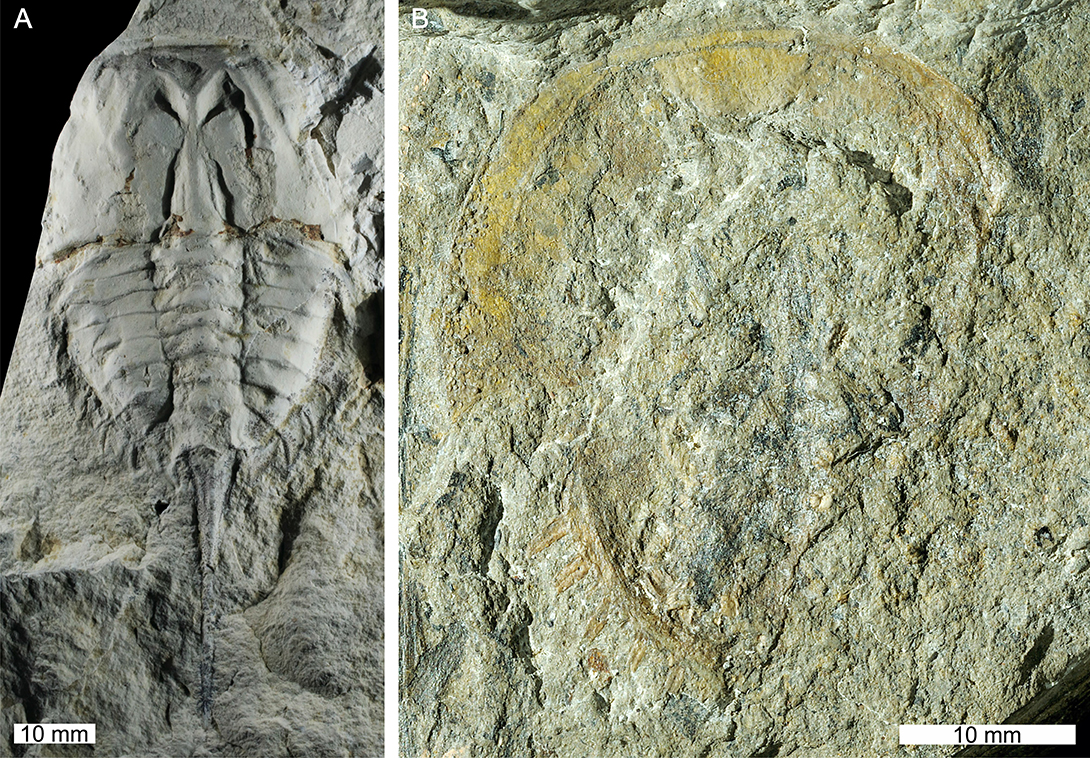
Figure 23. Bellinuroopsis rossicus and Rolfeia fouldenensis. (A) Bellinuroopsis rossicus from the Devonian-aged Lebedjan Formation, Russia. CCMGE CM1/3694, holotype. (B) Rolfeia fouldenensis from the Carboniferous-aged Cementstones Group, Scotland, UK. NMS 1984.67.1A, holotype. Photo credit: (A) Russell Bicknell; (B) Bill Crighton.
Rolfeia fouldenensis is the only species exhibiting large fixed thoracetronic spines extending laterally, coupled with smaller moveable thoracetronic spines (Clarkson, 1985). These spines likely provided the thoracetron with more surface area to prevent individuals from sinking into the substrate (Anderson, 1994) when they were not suspended in water (Siveter and Selden, 1987). Originally thought to be a possible paleolimulid due to tergal expression on the thoracetron (Waterston, 1985), the unique characters of both moveable and overdeveloped fixed spines, coupled with an expressed opercular tergite, were sufficient to erect a new family (Selden and Siveter, 1987; Siveter and Selden, 1987).
Bellinuroopsis
This Devonian-aged, monospecific genus (Bellinuroopsis rossicus) is known from one Russian specimen (Lebedjan Formation, Table 6, Figure 23; Chernyshev, 1933; Moore et al., 2007). The main characteristics that distinguishes Bel. rossicus from other taxa in Limulina are the following: a wedge-shaped cardiac lobe (Størmer, 1955); and an oblong thoracetron with eight, free moving, expressed tergites, tapering slightly to a telson. Furthermore, an expressed opercular (VIII) tergite that is more pronounced than in Rolfeiidae (Størmer, 1955; Novozhilov, 1991). These unique features potentially warrant the erection of a separate family, as suggested by Størmer (1955).
Limuloidea
Taxa in this superfamily are Austrolimulidae, Limulidae, and Valloisella. The diagnostic features of these taxa are a “thoracetron showing no lateral expression of individual tergites” (Lamsdell, 2016, p. 190).
Austrolimulidae
This family ranges from at least the Permian to the Cretaceous (Figure 1). There are at least seven monospecific genera: Austrolimulus, Casterolimulus, Dubbolimulus, Panduralimulus, Psammolimulus, Tasmaniolimulus, and Vaderlimulus (Table 7, Figures 24–26). Austrolimulids have domed cephalothoraxes, with overdeveloped genal spines that terminate as far back as the telson onset. Thoracetrons are mostly fused; occasionally preserve apodemal pits with highly reduced or vestigial moveable spines and styliform telsons (Riek, 1955, 1968; Lerner et al., 2017; Bicknell, 2019). Swallow-tailed thoracetrons are observed in A. fletcheri (Beacon Hill Shale, NSW, Australia, Triassic) and V. tricki (Thaynes Group, Idaho, USA, Triassic; Lerner et al., 2017), but this character is not known from all taxa in the family, including T. patersoni (Jackey Shale, Tasmania, Australia, Permain; Bicknell, 2019). Furthermore, A. fletcheri has a thoracetron with two sections, the posterior section of which has three exposed tergites (Riek, 1955; Pickett, 1984; Novozhilov, 1991; Itow et al., 2003). Lamsdell (2016) described a dorsal thoracetronic keel in Austrolimulidae. This feature is noted in D. peetae (Ballimore Formation, NSW, Australia, Triassic) and T. patersoni, but is not known to the other taxa (Riek, 1955; Pickett, 1984; Allen and Feldmann, 2005; Feldmann et al., 2011; Lerner et al., 2017; Bicknell, 2019). Appendages are known from T. patersoni, in which the distal portions of walking legs are observed (Ewington et al., 1989; Bicknell, 2019), and P. gottingensis (Solling Formation, Germany, Triassic) shows evidence of pushing legs (Meischner, 1962; Bicknell et al., 2019b).
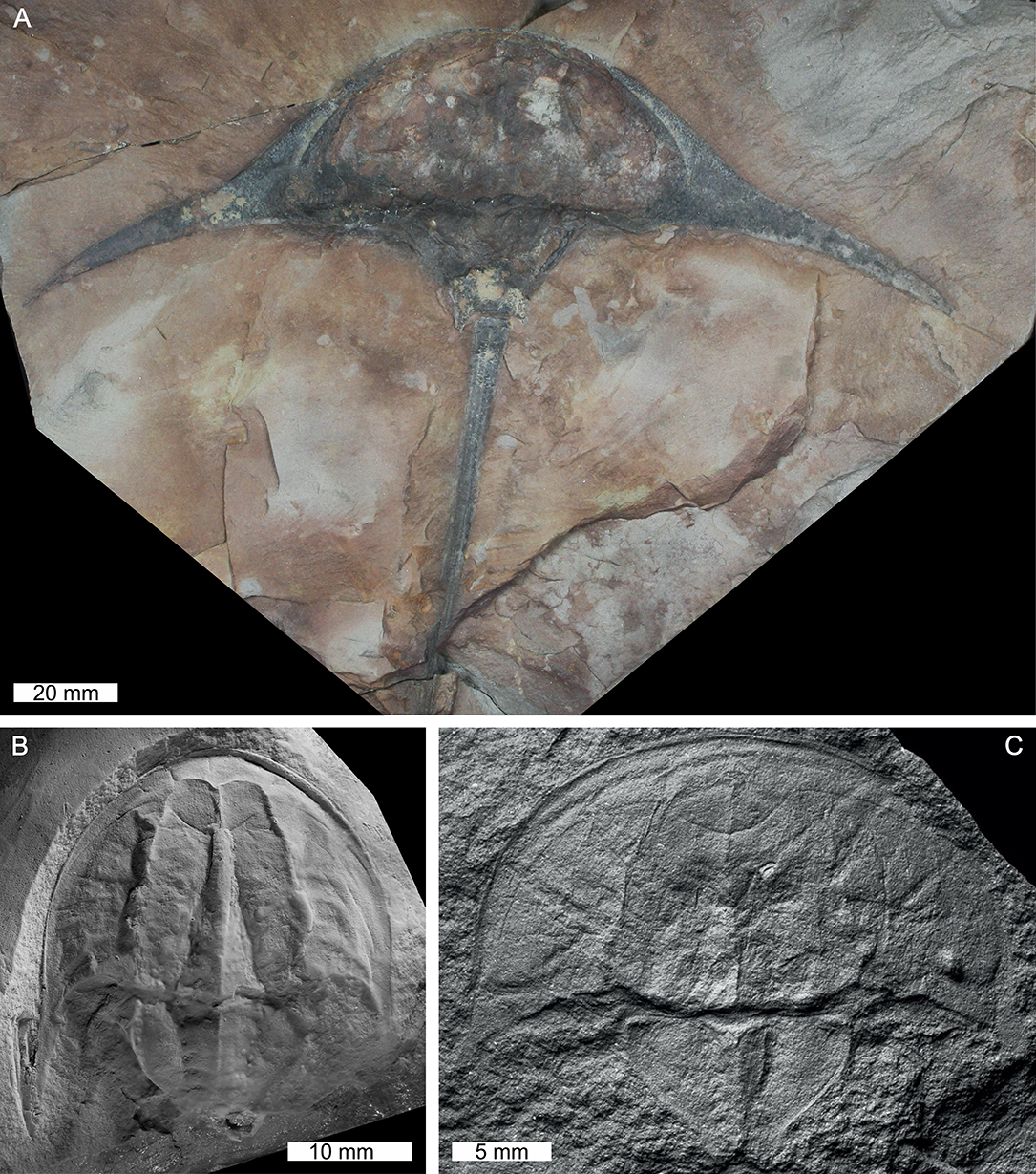
Figure 24. Austrolimulids from Australia. (A) Austrolimulus fletcheri from the Triassic-aged Beacon Hill Shale, NSW, Australia. AM F38274, holotype. (B) Tasmaniolimulus patersoni from the Permian-aged Jackey Shale, Tasmania, Australia. UTGD 123979, holotype. (C) Dubbolimulus peetae from the Triassic-aged Ballimore Formation, NSW, Australia. MMF 27693, holotype. (B,C) Converted to gray scale. Photo credit: (A) Josh White; (B) Russell Bicknell; (C) David Barnes. (B) Coated in ammonium chloride sublimate.
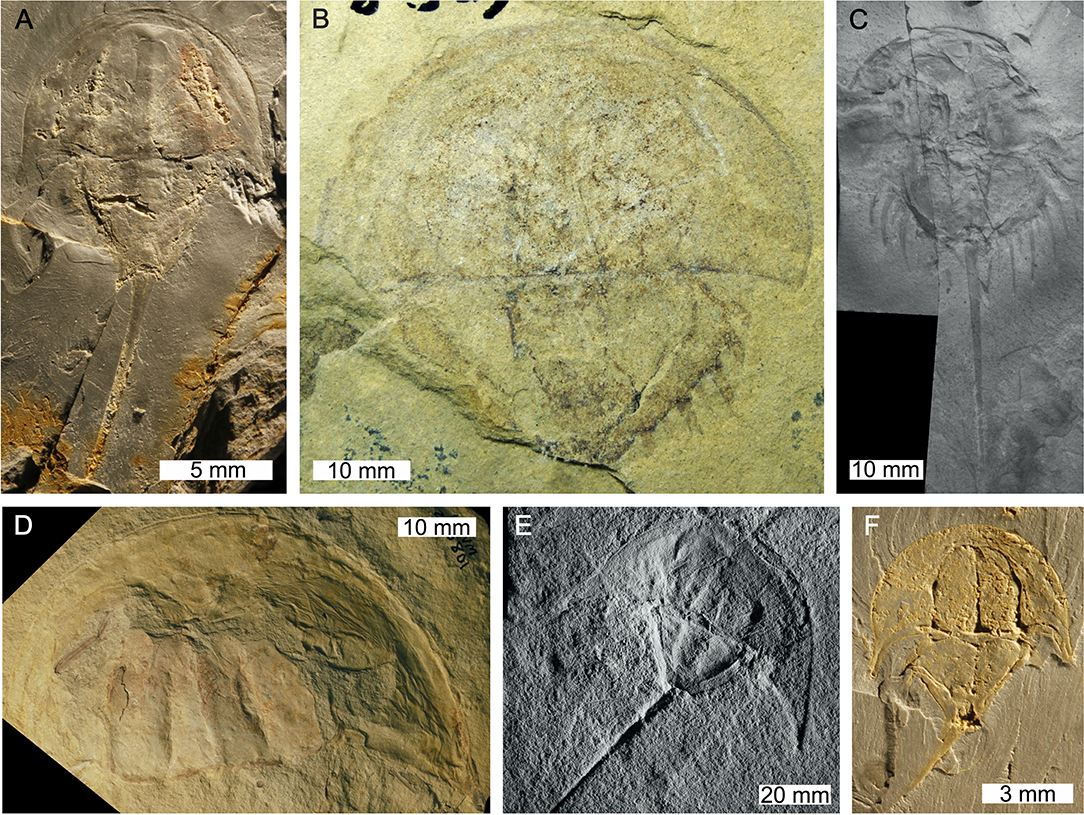
Figure 25. Austrolimulids from the USA. (A,F) Panduralimulus babcocki from the Permian-aged Maybelle Limestone, Texas, USA. (A) USNM 520723, holotype. (F) USNM 520724, paratype. (B,C) Paleolimulus longispinus specimens from the Carboniferous-aged Bear Gulch Limestone, Montana, USA. (B) UM 81-8-5-1. (C) CM 54050. (D) Casterolimulus kletti from the Cretaceous-aged Fox Hills Formation, North Dakota, USA. USNM 206801, holotype. (E) Vaderlimulus tricki from the Triassic-aged Thaynes Group, Idaho, USA. UCM 140.25, holotype. (C) Converted to gray scale. Photo credit: (A,C,D,F) Russell Bicknell; (B) Kallie Moore; (E) Allan Lerner.
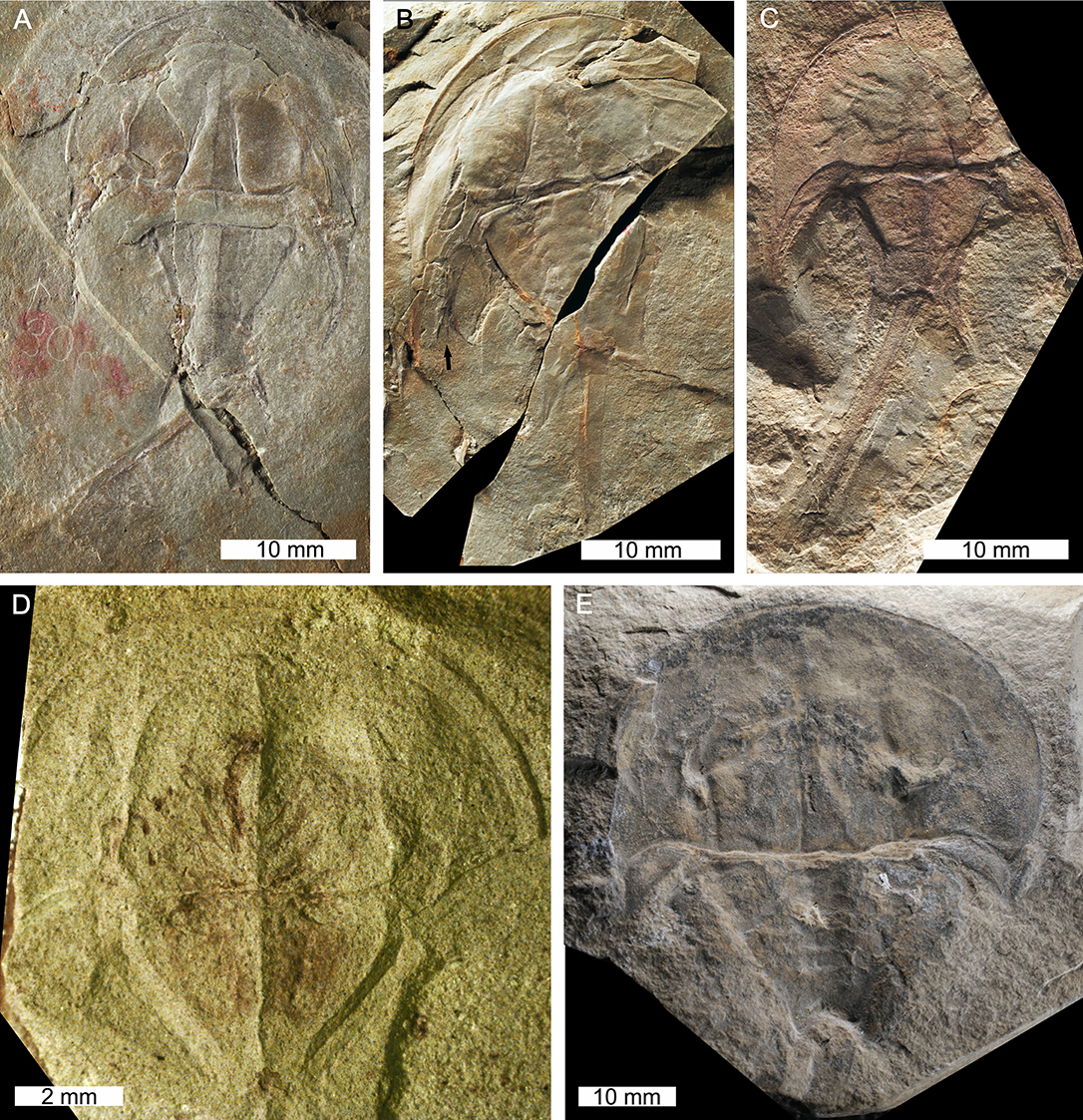
Figure 26. Austrolimulids from Europe. (A–C) Psammolimulus gottingensis from the Triassic-aged Solling Formation, Germany. (A) Complete specimen, GZG INV 15356a. (B) Specimen with pushing leg preserved (black arrow). GZG INV 15376a. (C) Complete specimen with appendage impressions in cephalothorax, GZG.INV.45730a. (D) ?Paleolimulus fuchsbergensis from the Triassic-aged Exter Formation, Germany. SMF VII I 311, holotype. (E) ?Paleolimulus jakovlevi from Permian-aged Araukaritovaya Formation Novoselovka, Ukraine. CCMGE CM1/8886, holotype. ? denotes uncertain taxonomic assignment. Photo credit: (A–C) Gerhart Hundertmark; (D) Norbert Hauschke; (E) Russell Bicknell.
The large genal spine splay and abnormal forms of austrolimulids represent the strangest and most extreme xiphosurid morphologies (they have been considered odd-ball taxa, Eldredge, 1976; Bicknell, 2019). Their morphologies likely reflect the freshwater and marginal conditions that were exploited by the group, and provide evidence against the highly conserved nature of Xiphosurida (Fisher, 1984; Bicknell, 2019). The hypertrophied spines may have permitted more effective motion within unidirectional fluid-flow in rivers (Bicknell, 2019; Bicknell and Pates, 2019b). As discussed above, Lamsdell (2016) and Lerner et al. (2017) suggested that species in Paleolimulus belong in Austrolimulidae (e.g., Pa. fuchsbergensis, Pa. jakovlevi, and Pa. longispinus) using phylogenetic and linear morphometric arguments respectively. These taxa require revision; a direction of research that will begin to uncover the true diversity of these taxa and their interesting morphologies.
Limulidae
This is the most long-lived and most generically diverse xiphosurid family, with a fossil record that spans possibly from the Carboniferous to Recent (Figure 1). There are 10 limulid genera: Albalimulus, Crenatolimulus, Limulitella, Limulus, Mesolimulus, Sloveniolimulus, Tachypleus, Tarracolimulus, Victalimulus, and Yunnanolimulus with 24 species (Table 7, Figures 27–38; Lamsdell, 2016). Limulids have a domed, horseshoe-shaped cephalothoraces with genal spines that can extend posteriorly up to the first third of the thoracetron (Novozhilov, 1991). Ophthalmic ridges are known from all taxa and the lateral compound eyes are located along these ridges (Størmer, 1955; Novozhilov, 1991). Ophthalmic ridges do not converge anteriorly. The thoracetron is completely fused, unsegmented, trapezoidal to sub-hexagonal, often displaying movable spines, with small fixed spines, and a styliform telson (Størmer, 1955; Tiegs and Manton, 1958; Siveter and Selden, 1987; Lamsdell, 2016). Appendages and soft-bodied material are occasionally preserved in fossil limulids. Victalimulus mcqueeni (Latrobe Group, NSW, Australia, Cretaceous), T. syriacus (Haqel and Hadjoula Konservat-Lagerstätten, Lebanon, Cretaceous) and Y. luopingensis (Member II, Guanling Formation, Luoping, China, Triassic) all preserved cephalothoracic and thoracetronic appendages (Riek and Gill, 1971; Hu et al., 2011, 2017; Lamsdell and Mckenzie, 2015; Bicknell et al., 2019b). Limulitella bronnii (Grés á Voltzia Formation, France, Triassic) only preserved cephalothoracic appendages (Wincierz, 1960). Mesolimulus walchi preserved muscle fibers, and cephalothoracic and thoracetronic appendages (Zittel, 1881; Briggs et al., 2005; Bicknell et al., 2019b). Finally, muscle insertions were identified using and augmented laminography on a Limulitella sp. specimen from the Triassic-aged Lower Wellenkalk Member, Muschelkalk, Netherlands (Zuber et al., 2017). Sexual dimorphism has been suggested for select fossil taxa (Bicknell et al., 2019b): Limulus decheni (females have longer cephalothoraces; Hauschke and Wilde, 2004), T. syriacus (females have broader thoracetrons and males have scalloped anterior cephalothoraces; Lamsdell and Mckenzie, 2015) and Y. luopingensis (females have shorter posterior thoracetronic moveable spines and males have modified anterior walking legs; Hu et al., 2017). Most limulids were marine, but V. mcqueeni, Lim. bronnii, and Lim. tejraensis are considered freshwater species, while Lim. liasokeuperinus is considered a marginal marine taxon.
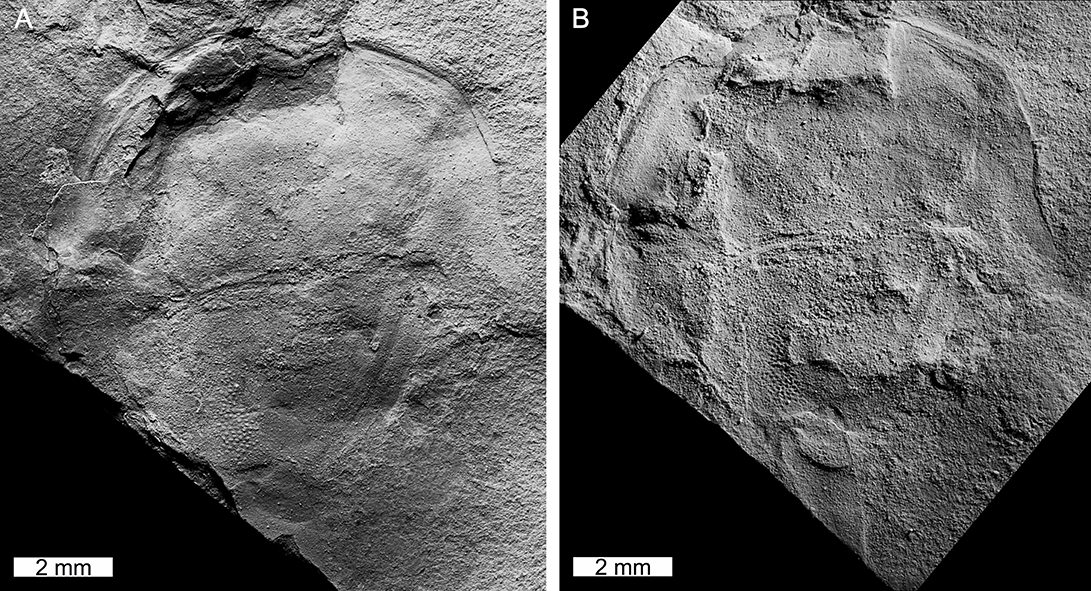
Figure 27. The oldest suggested limulid from the lower Carbonifeous-aged Ballagan Formation, Scotland, UK; Albalimulus bottoni. (A) BSG.GSE2028, holotype, part. (B) BGS.GSE9680, holotype, counter-part. Image mirrored to align with (A) Phylogenetic analyses of Xiphosurida placed this taxon close to the base of Limulidae (Bicknell and Pates, 2019b). Specimens were coated with ammonium chloride sublimate and converted to gray-scale. Photo credit: Russell Bicknell.
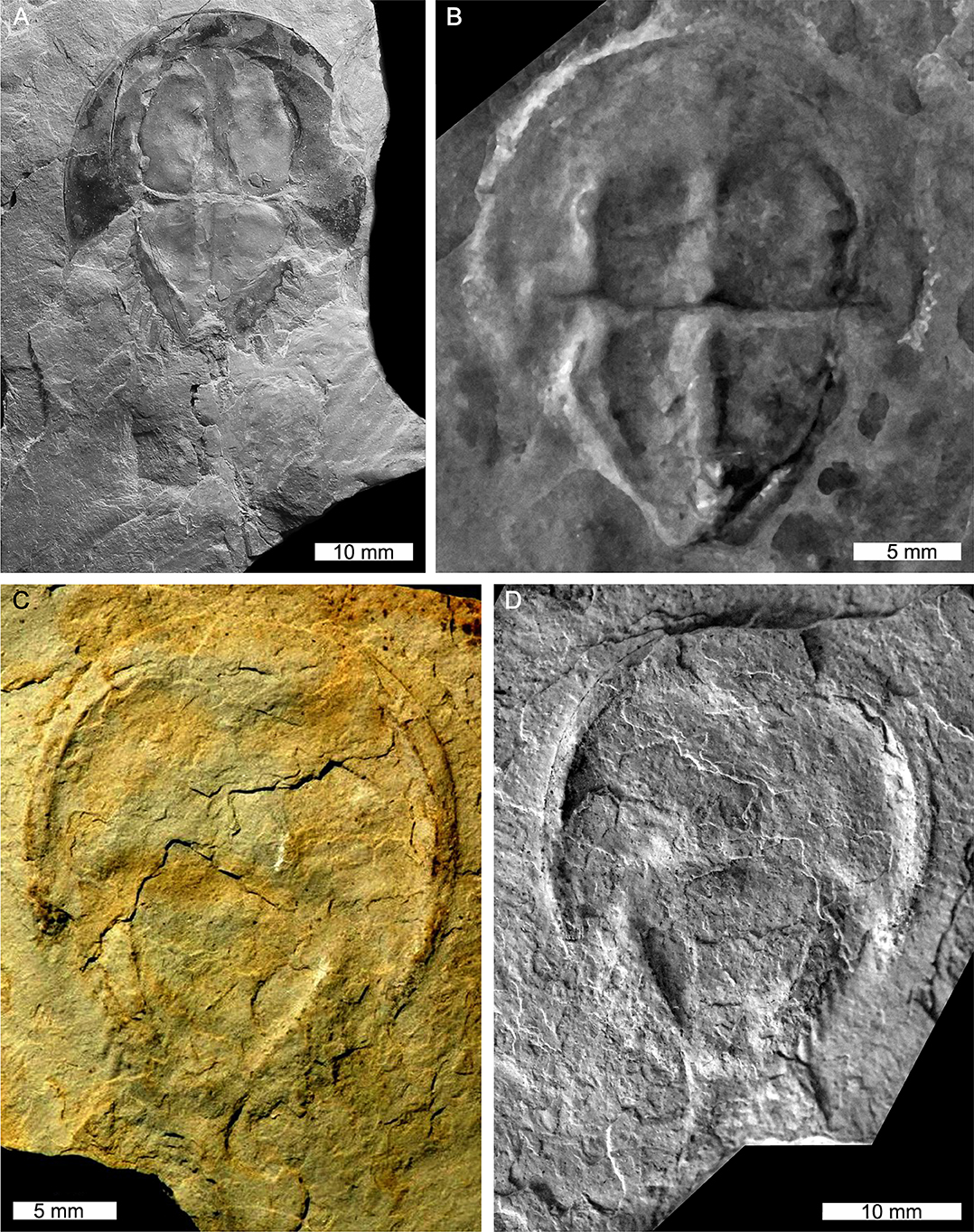
Figure 28. Triassic-aged Limulitella species from France, Germany, and the Netherlands. (A) Limulitella bronnii from the Triassic-aged Grés á Voltzia Formation, France. State Museum of Natural History Stuttgart specimen in Grauvogel collection, LIM 68. (B) Limulitella henkeli from the Triassic-aged Jena Formation, Germany. Slg-TC-4/MLU.Fri1906.VII/5, holotype. (C) Limulitella sp. from the Triassic-aged Lower Wellenkalk Member, Muschelkalk, Netherlands. Specimen within Oosterink private collection. (D) ?Limulitella sp. from the Triassic-aged Lower Muschelkalk, Netherlands, no specimen number. (A,B,D) Converted to gray scale. ? denotes uncertain taxonomic assignment. Photo credit: (A) Dieter Seegis; (B) Norbert Hauschke; (C) Thomas König; (D) Martien Oosterink.
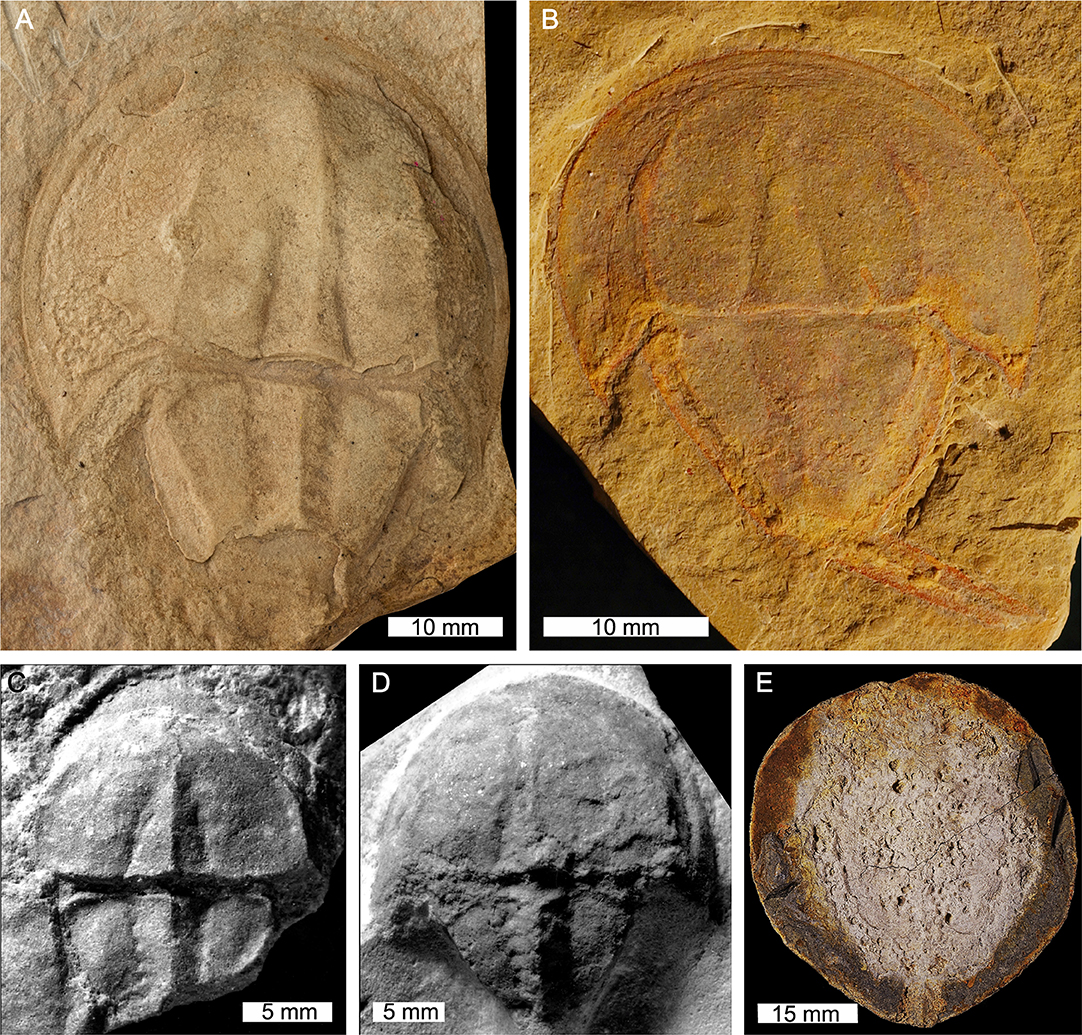
Figure 29. Triassic-aged Limulitella species from France, Germany, Madagascar, and Tunisia. (A) Limulitella vicensis from the Triassic-aged Keuper Formation, France. MAN 8240, holotype. (B) Limulitella tejraensis from the Triassic-aged Ouled Chebbi Formation, Tunisia. ZPAL V. a6/101, holotype. (C,D) ?Limulitella sp. from the Triassic-aged Buntsandstein, Germany. (C) Exemplar 2 figured in Hauschke and Wilde (2008). (D) Exemplar 1 figured in Hauschke and Wilde (2008). (C,D) Geologisch-Paläontologischen Instituts der Ruprecht-Karls-Universität Heidelberg specimens and associated with Ph.D. thesis No. 3R.8.34-4. Specimens are likely lost as they were not found again in the collection. (E) Limulitella sp. from the Triassic-aged Sakamena Group, Madagascar. MSNMi11170, counterpart. ? denotes uncertain taxonomic assignment. Photo credit: (A) Lukáš Laibl; (B) Błażej Błażejowski; (C,D) Permission to reproduce photographs granted by Norbert Hauschke; (E) Giorgio Teruzzi.
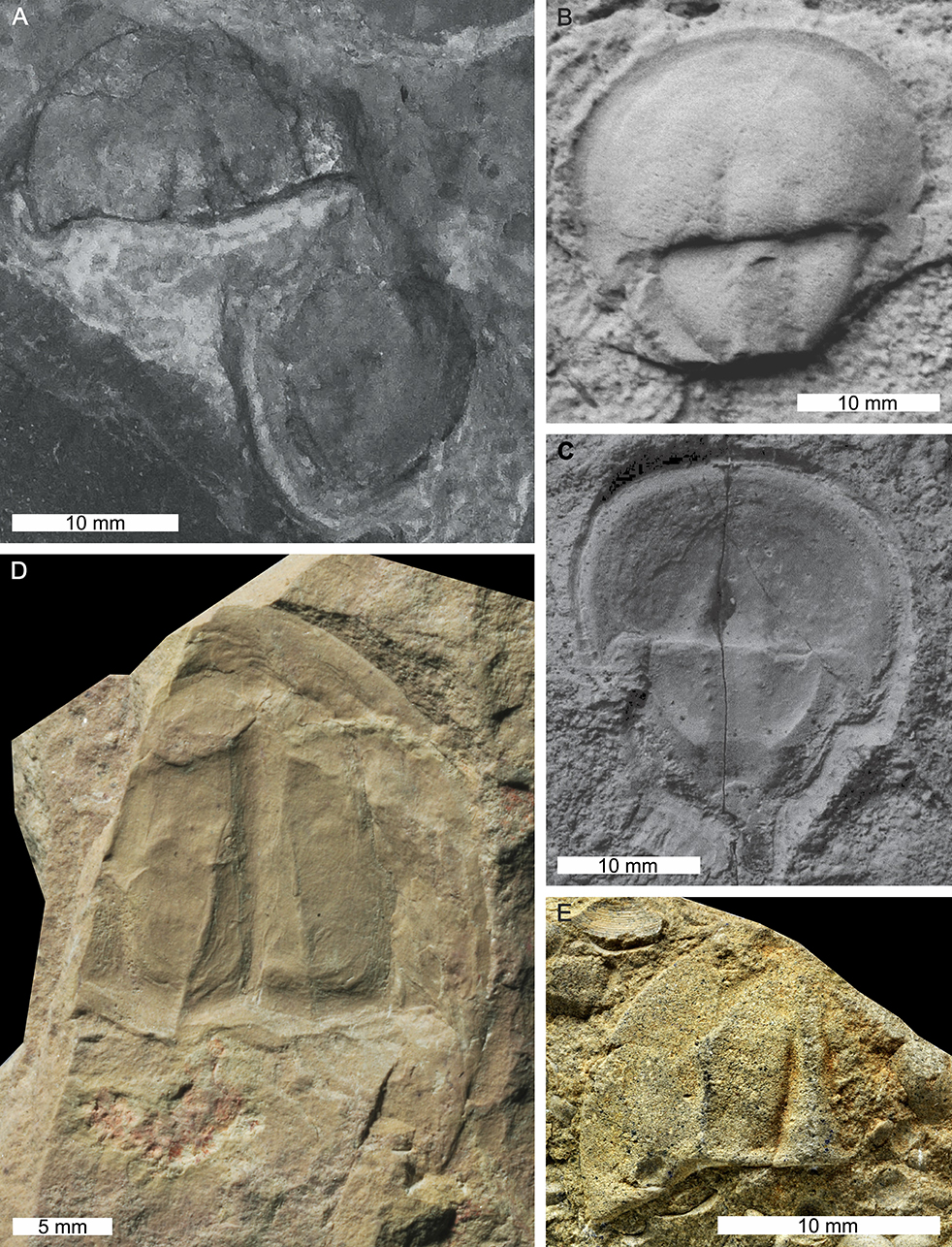
Figure 30. Triassic and Jurassic Limulitella from Germany and Russia. (A) ?Paleolimulus sp., likely Limulitella sp., from the Triassic-aged Bernburg Formation, Germany. HAU-WIL2000. (B,C) Unnamed specimen from the Triassic-aged Trochitenkalk Formation, Germany. (B) Part of specimen. NME 07-56a. (C) Counter-part of specimen. NME 07-56b. (A) may have been lost. (B,C) May be lost (Hartmann pers. comms.). (D) Limulitella cf. liasokeuperinus from the Triassic-aged ?Exter Formation Germany. SNSB-BSPG 1967 XVI 27. Note: holotype lost in World War II. (E) Limulitella volgensis from the Triassic-aged Parshinskaya Formation, Russia. PIN 4048/7. (A–C) Converted to gray scale. ? denotes uncertain taxonomic or formation assignment. Photo credit: (A–C) Permission to reproduce photographs granted by Norbert Hauschke; (D) Mike Reich; (E) Constantine Tarásenko.
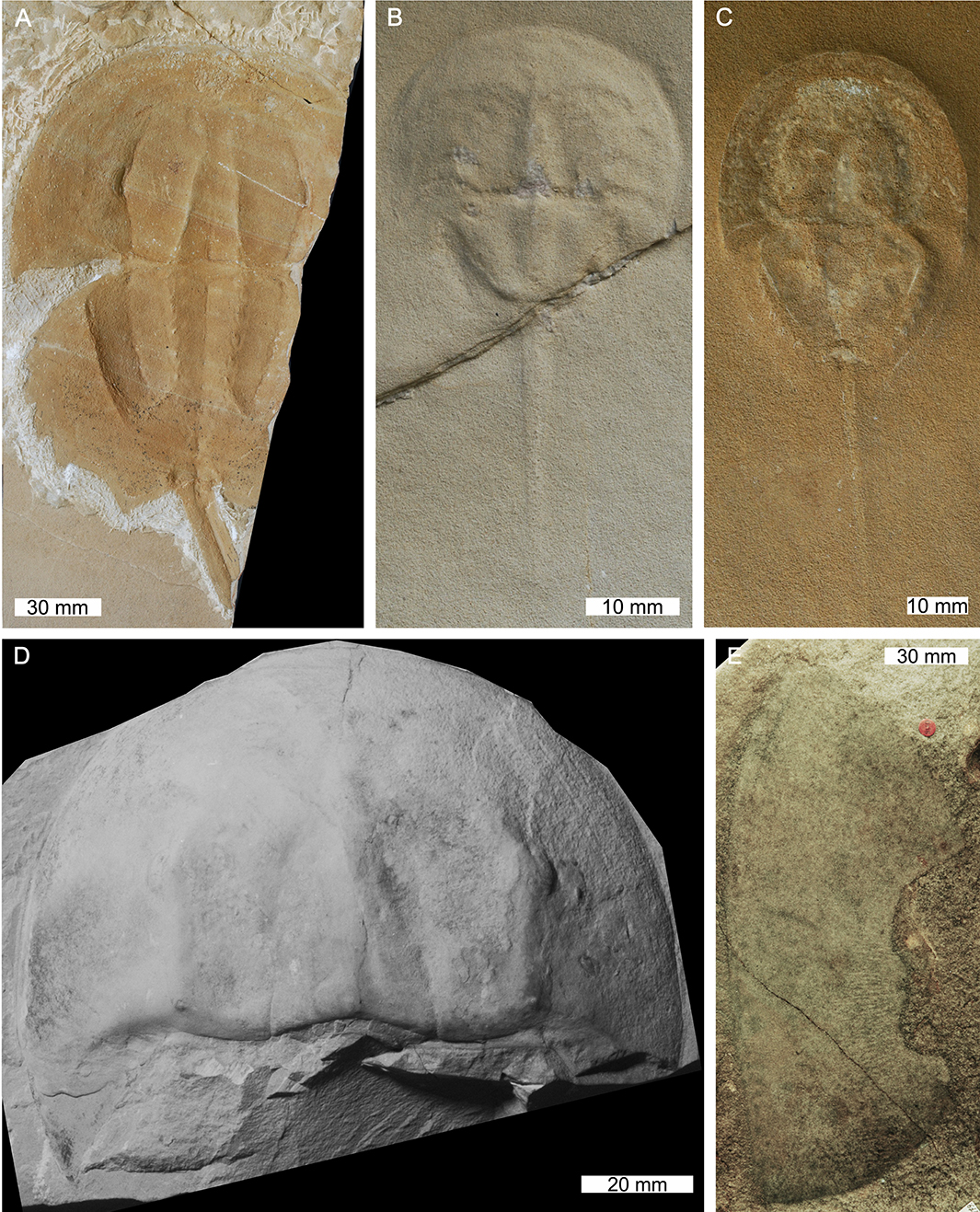
Figure 31. Triassic-aged limulids from Germany, Spain, and Sweden. (A) Tachypleus gadeai from the Triassic-aged Alcover Limestone Formation, Spain. MGSB 19195, holotype. (B) Mesolimulus crespelli from the Triassic-aged Alcover Limestone Formation, Spain. MGSB 35088, holotype. (C) Tarracolimulus rieki from the Triassic-aged Alcover Limestone Formation, Spain. MGSB M 262, holotype. (D) Limulidae gen. et sp. indet, previously Limulus kieri from the Triassic-aged Muschelkalk Limestone, Germany. MB.A.0207. (E) Limulus nathorsti from the Triassic-aged Höör Sandstone, Sweden. SMNH Ar33179, holotype. (D) Converted to gray scale. Photo credit: (A–C) Pedro Adserà; (D) Lorenzo Lustri; (E) Liping Liu.
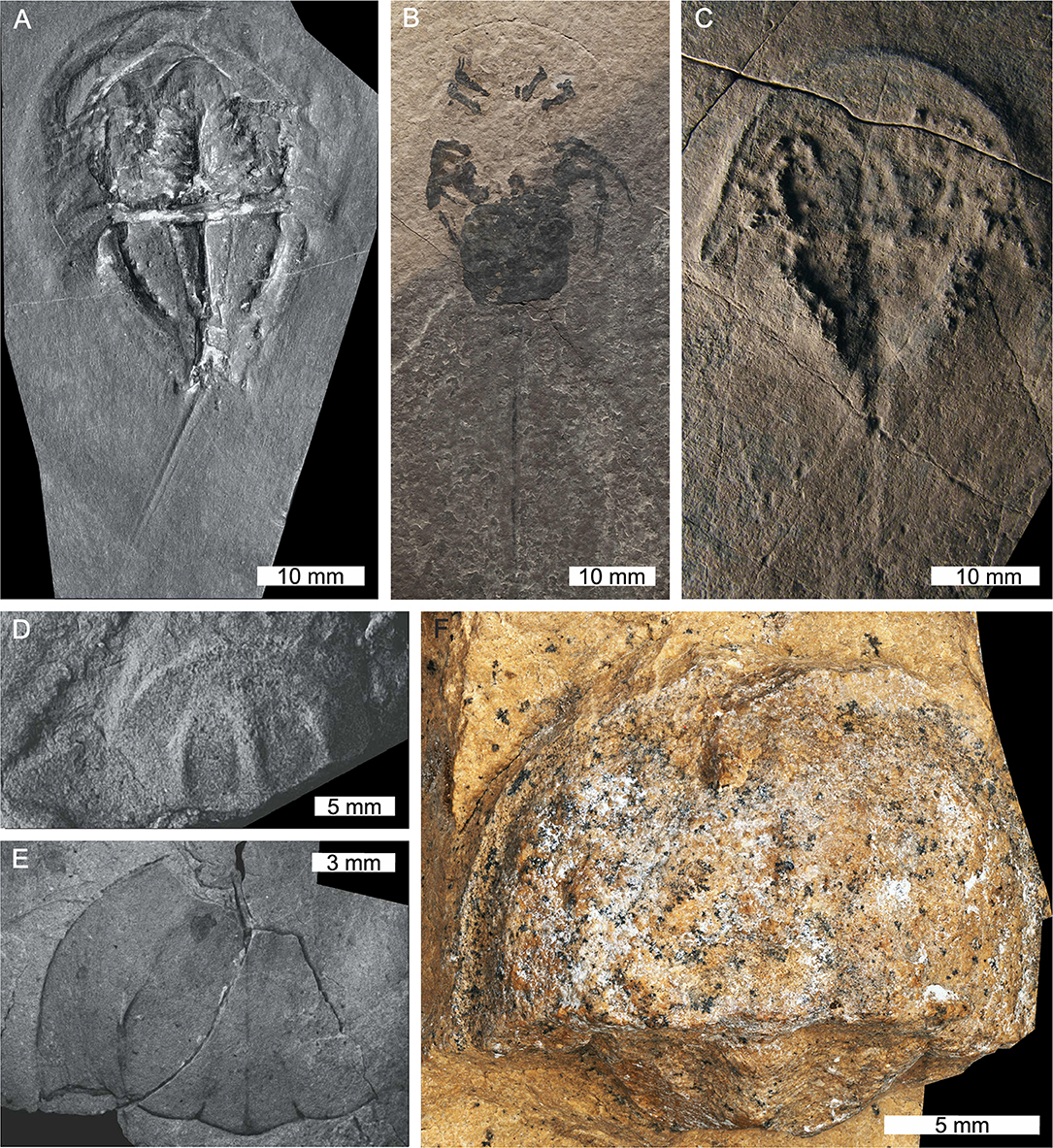
Figure 32. Triassic-aged limulids from China and Europe. (A,B) Yunnanolimulus luopingensis from the Triassic-aged Member II, Guanling Formation, Luoping, China. (A) LPI-61299, holotype. (B) Specimen displaying walking legs and book gills. LPI-61734. (C) Sloveniolimulus rudkini from the Triassic-aged Strelovec Formation, Slovenia. PMSL T-993, holotype. (D,E) Limulidae gen. et sp. indet from the Triassic-aged Volpriehausen Formation, Germany. GPS. MLU 2018.23. (E) Limulidae gen. et sp. indet from the Triassic-aged Bernburg Formation, Germany. GPS. MLU 2018.24. (F) Limulus priscus from the Triassic-aged Muschelkalk Limestone, Germany. SNSB-BSPG AS I 939, holotype. (D,E) Converted to gray scale. Photo credit: (A,B) Shixue Hu; (C) Tomaž Hitij; (D,E) Permission to reproduce photographs granted by Norbert Hauschke; (F) Mike Reich.
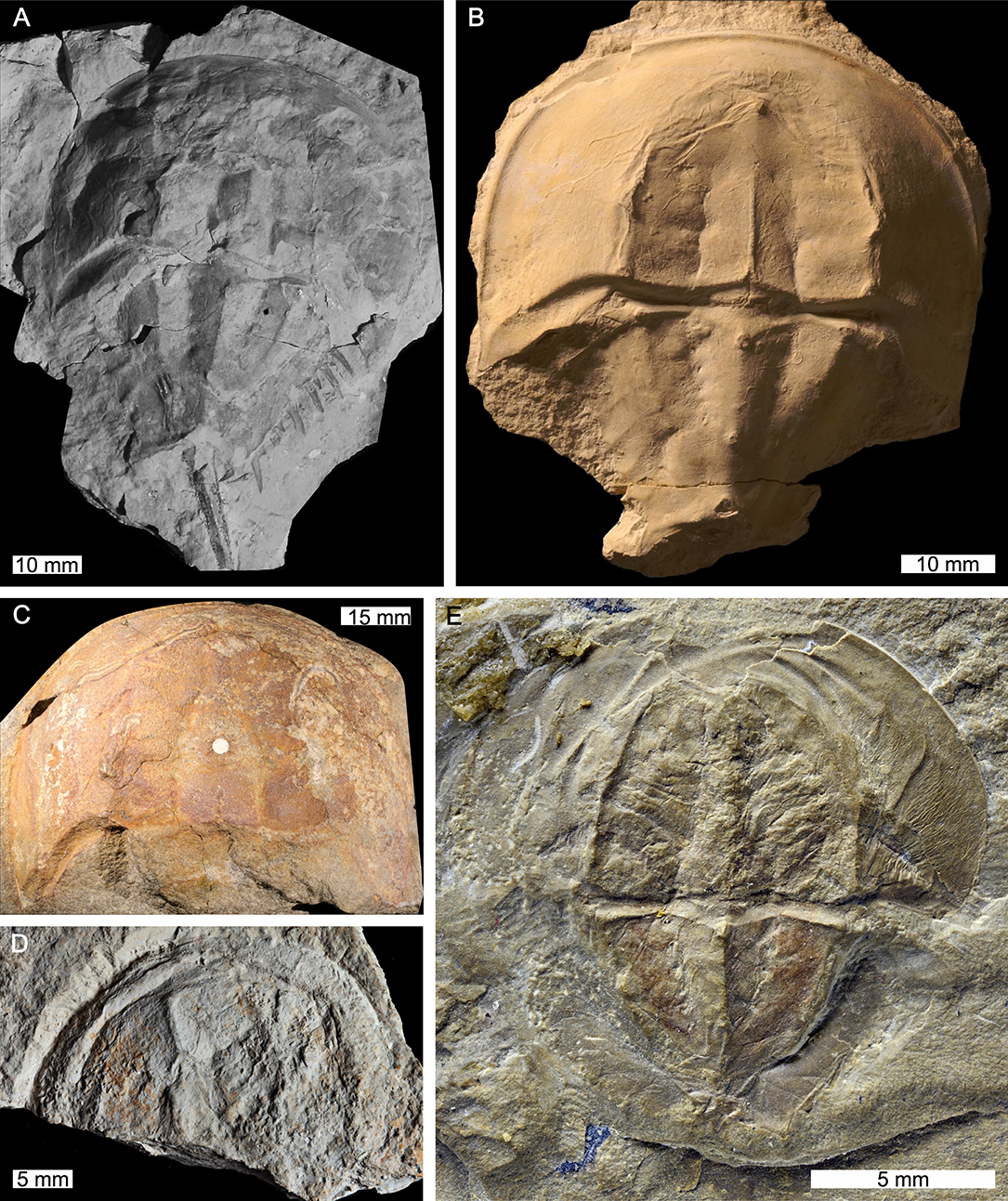
Figure 33. Jurassic-aged limulids from Poland, Russia, and UK. (A) Crenatolimulus sp. from the Jurassic-aged Kcynia Formation, Poland. ZPAL X.1/O-B/XA 13.B. (B) “Limulus” darwini from the Jurassic-aged Kcynia Formation, Poland. ZPAL X.1O-BXA, holotype. (C) Limulus woodwardi from the Jurassic-aged Northampton Sand Formation (?), England, UK. L8627, holotype. (D) Mesolimulus sp. from the Jurassic-aged Purbeck Limestone Group, England, UK. NHMUK PI. I. 3042. (E) Mesolimulus sibiricus from the Jurassic-aged Talynzhansk Formation, Russia. PIN 3290-21, holotype. (A) Converted to gray scale. Photo credit: (A,B) Błażej Błażejowski; (C) Russell Bicknell; (D) Lucie Goodayle, NHM, London; (E) Sergey Bagirov.
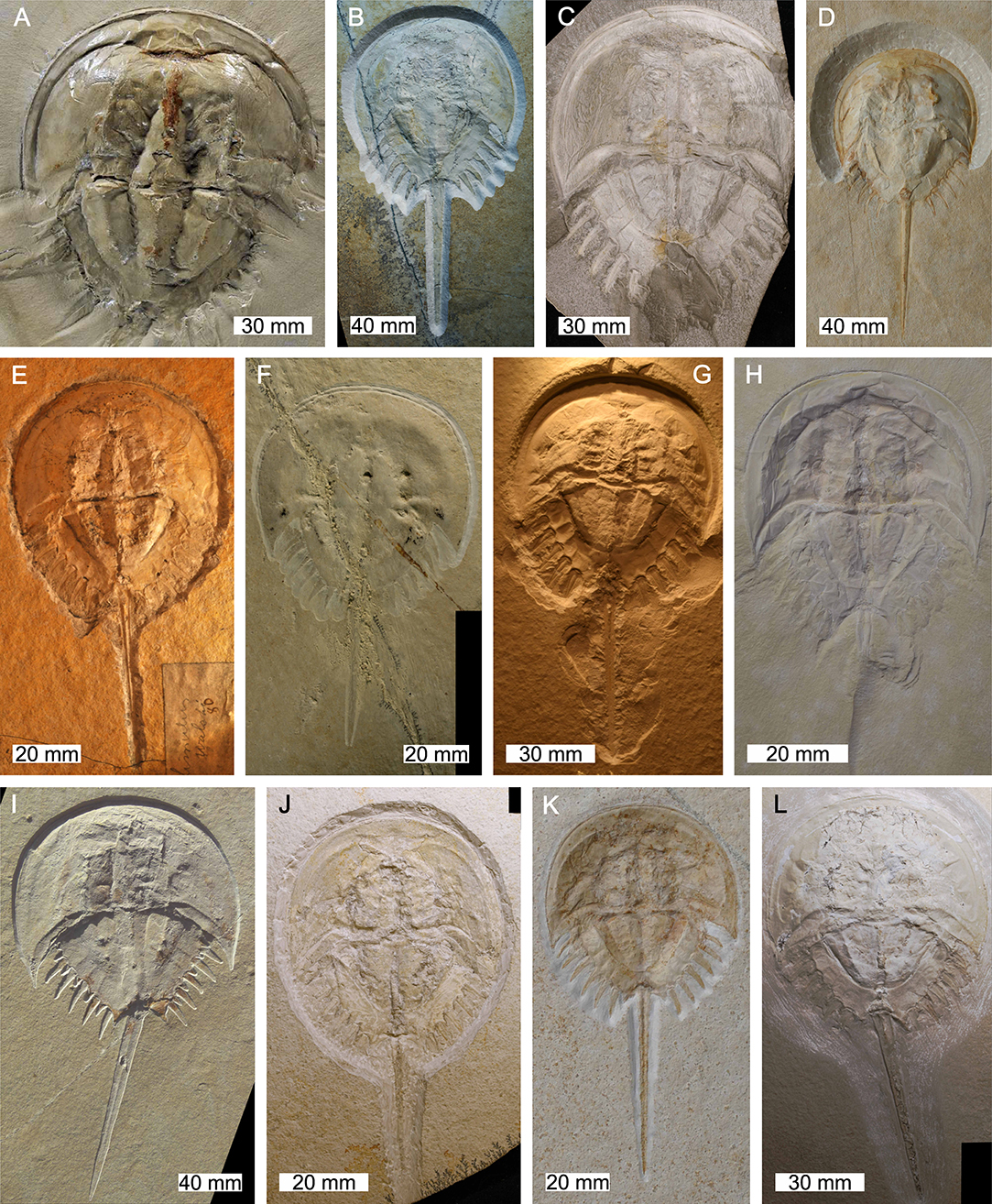
Figure 34. Examples of the iconic Jurassic-aged Mesolimulus walchi from Germany. (A–H, J–L) Specimens from the Solnhofen Limestone, Germany. (A) MNHN.F.A33516. (B) TMP 1984.69.5. (C) YPM IP 9011. (D) SMNS 27585. (E) CM 28515. (F) USNM 706404. (G) MCZ 106368. (H) OUMNH F11569. (J) Specimen preserving gut tract, YPM IP 8975. (K) SMNS 694513. (L) Specimen preserving gut tract, YPM IP 10183. (I) Specimen from the Nusplingen Plattenkalk, Germany, SMNS 70204. Photo credit: (A) Lilian Cazes; (B,C,E–G,J,L) Russell Bicknell; (D,I,K) Guenter Schweigert; (H) Javier Ortega Hernández.
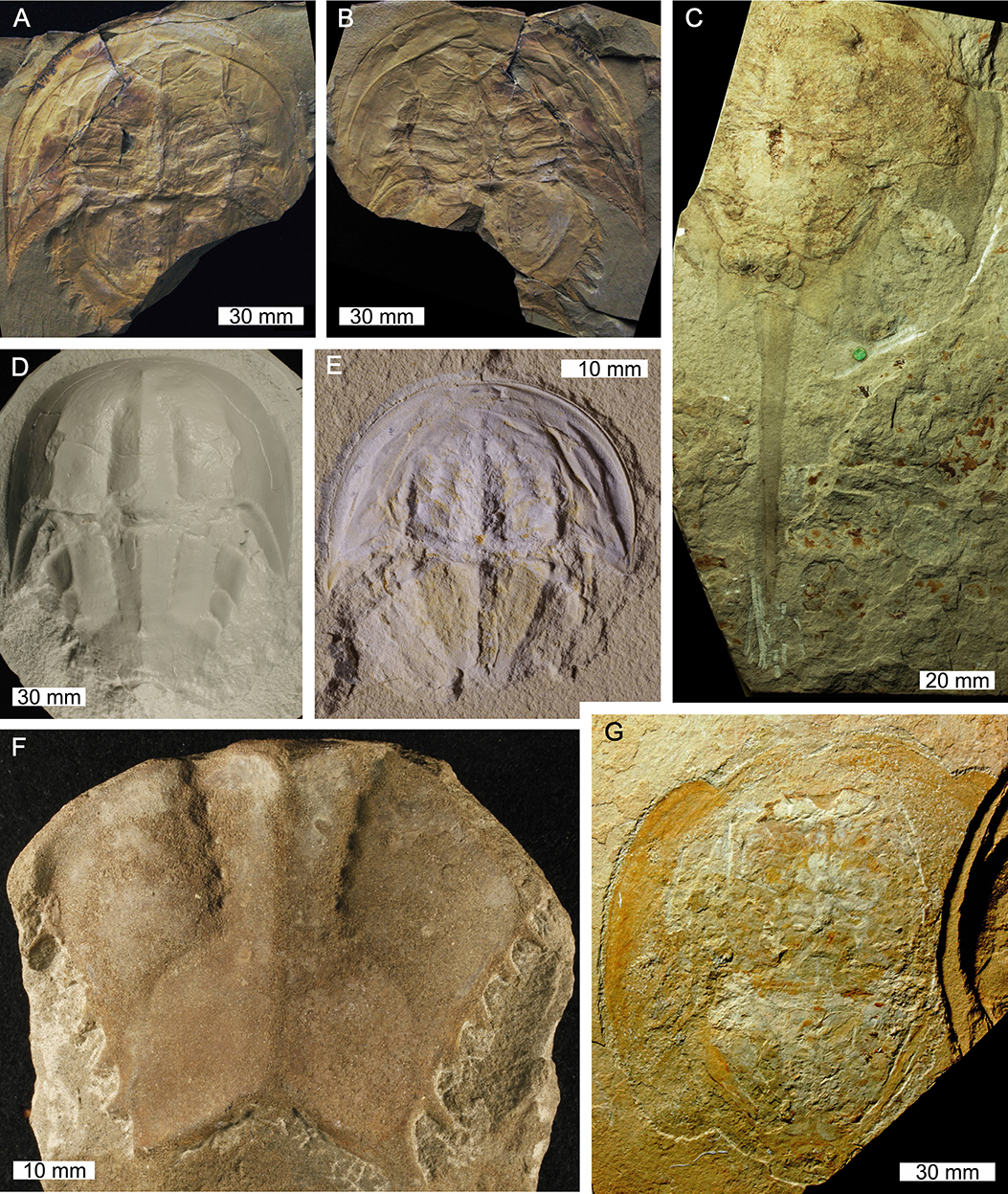
Figure 35. Cretaceous-aged limulids. (A,B) Victalimulus mcqueeni from the Korumburra Group, NSW, Australia. (A) Part, NMV P22410B, holotype. (B) Counter-part showing appendage impressions, NMV P22410A. (C,G) Tachypleus syriacus from the Haqel Konservat-Lagerstätte, Lebanon. (C) NHMUK PI. OR. 59783, holotype. (G) Specimen showing possible sexual dimorphic trait of scalloped anterior cephalothorax, NHMUK PI. OR. 187. (D) Crenatolimulus paluxyensis from the Glen Rose Formation, Texas, USA. (D) USNM 545241, cast of holotype. (E) Mesolimulus tafraoutensis from the Gara Sbaa Lagerstätte, Morocco. MSNM i26844, holotype. (F) Limulus coffini from the Pierre Shale, Colorado, USA, USNM 129043, holotype. Photo credit: (A,B) Frank Holmes; (C,G) Stephen Pates; (D,F) Russell Bicknell; (E) Giorgio Teruzzi.
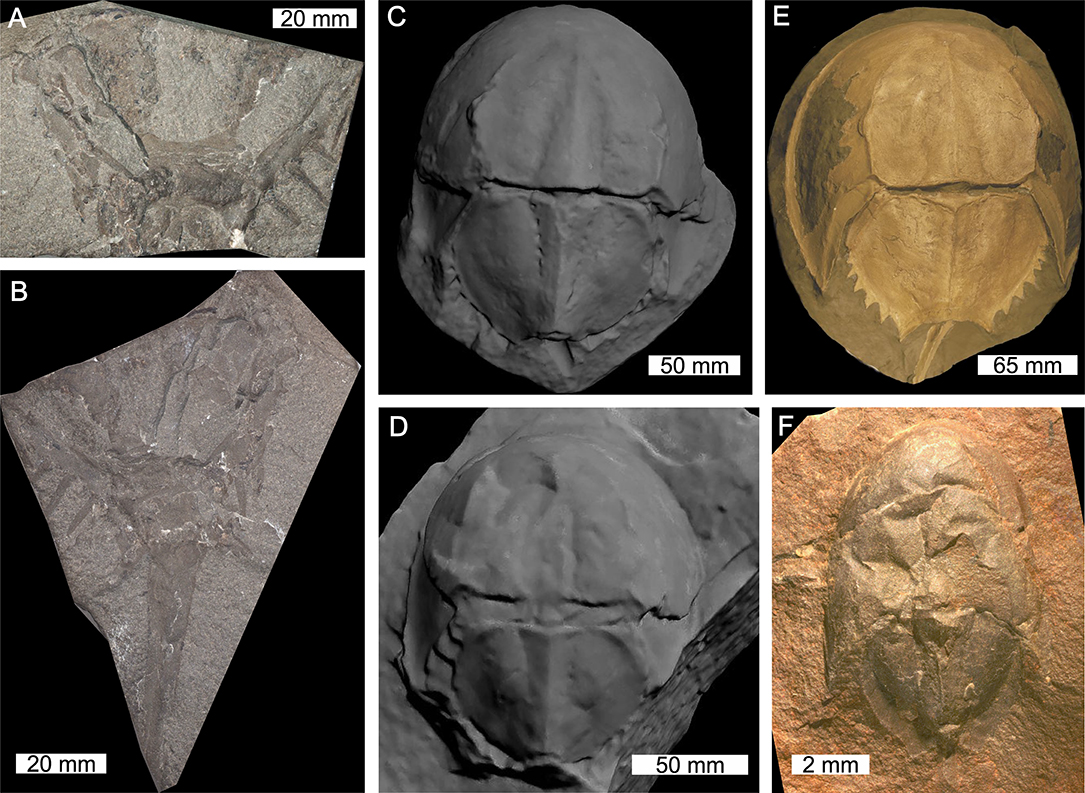
Figure 36. Unnamed Permian xiphosurid, Limulus decheni and Valloisella lievinensis. (A,B) Unnamed xiphosurid from the Permian-aged Zechstein, Germany. (A) Counterpart showing thoracetron, NMK D2.11b. (B) Part showing thoracetron and telson, NMK D2.11a. (C–E) Limulus decheni from the Eocene-aged Domsen Sands, Germany. (C) 3D reconstruction of a surface scan, VET1931.1.MLU. (D) 3D reconstruction of a surface scan, GIE1863.1a.MLU, holotype. (E) Specimen with part of telson preserved, MB.A.1901. (F) Valloisella lievinensis from the Carboniferous-aged Bickershaw Complex, England, UK; LL11133. Photo credit: (A,B) Peter Mansfeld; (C,D) Permission to use 3D reconstructions granted by Lars Schimpf; (E) Andreas Abele; (F) Russell Bicknell.
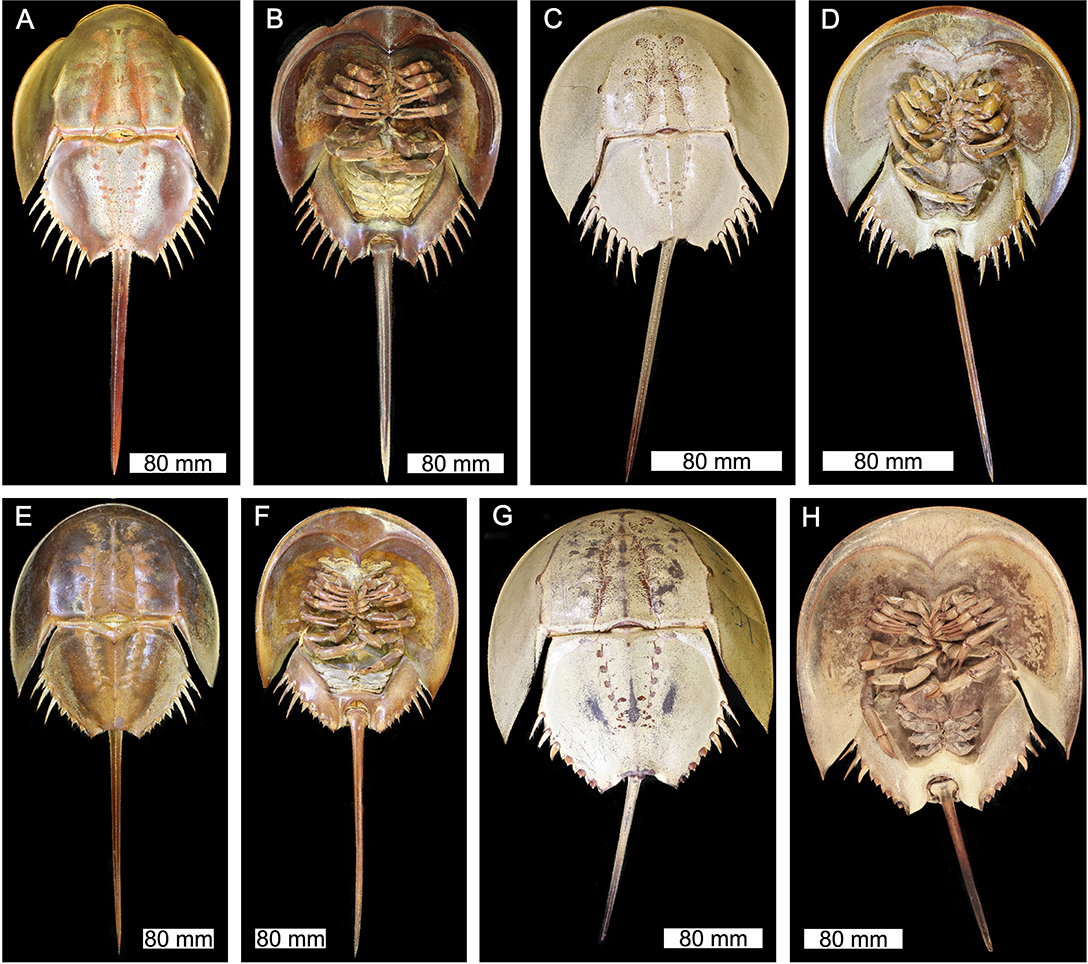
Figure 37. Examples of extant male and female Tachypleus species. (A,B) Male T. tridentatus, YPM IZ 55603. (A) Dorsal view. (B) Ventral view. (C,D) Male T. gigas, YPM IZ 55578. (C) Dorsal view. (D) Ventral view. (E,F) Female T. tridentatus, YPM IZ 55576. (E) Dorsal view. (F) Ventral view. (G,H) Female T. gigas, YPM IZ 103393. (G) Dorsal view. (H) Ventral view. Photo credit: Russell Bicknell.
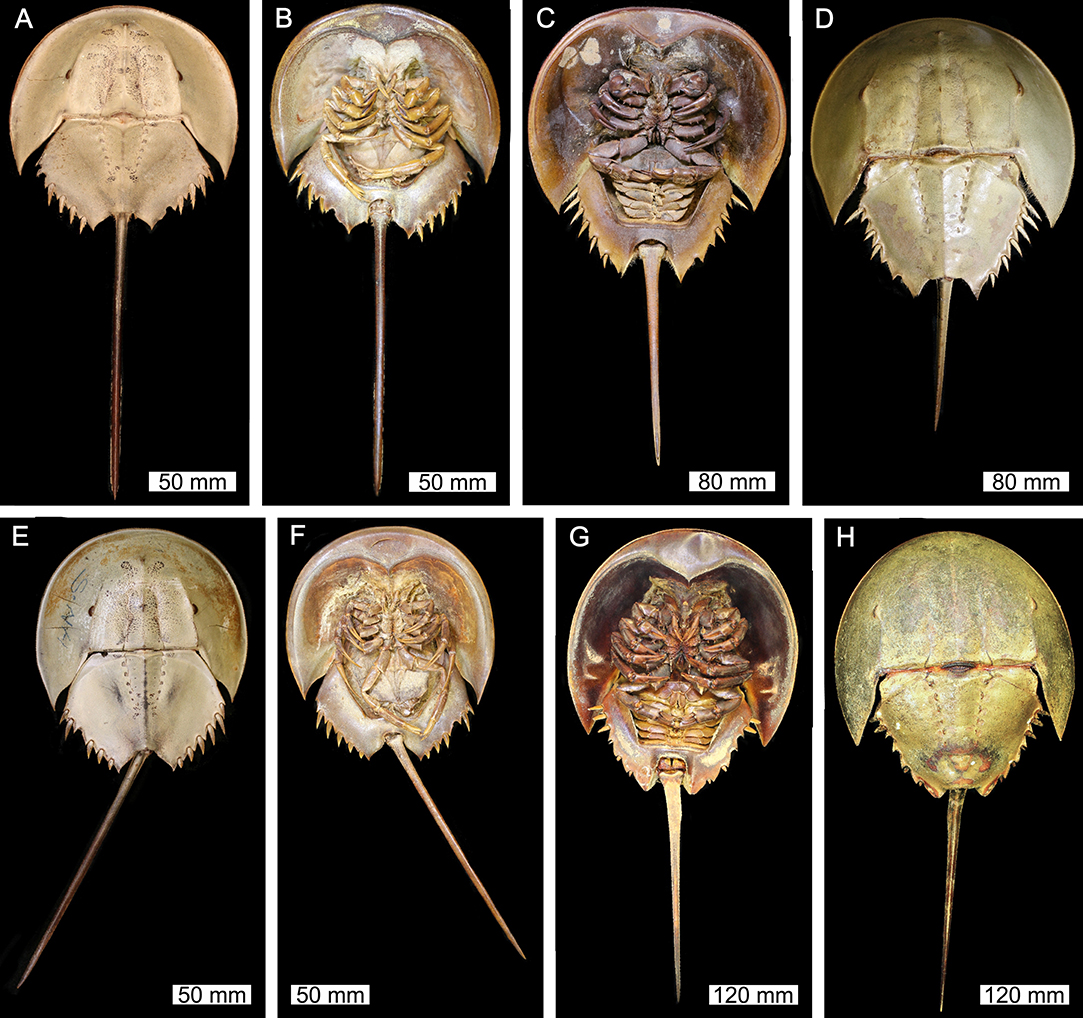
Figure 38. Examples of extant male and female Limulus polyphemus and Carcinoscorpius rotundicauda. (A,B) Male C. rotundicauda, YPM IZ 55595. (A) Dorsal view. (B) Ventral view. (C,D) Male L. polyphemus, YPM IZ 55605. (C) Ventral view. (D) Dorsal view. (E,F) Female C. rotundicauda, YPM IZ 55574. (E) Dorsal view. (F) Ventral view. (G,H) Female L. polyphemus YPM IZ 55601. (G) Ventral view. (H) Dorsal view. Photo credit: Russell Bicknell.
Limulids are thought to represent bradytelic evolution and exhibit strong morphological conservation between extant and fossil taxa. As such, they have been the focus of evolutionary and morphological research (Fisher, 1984; Bicknell and Pates, 2019b; Bicknell et al., 2019b). The limited morphological difference between the 148 Mya Jurassic “Limulus” darwini (Kcynia Formation, Poland) and modern juvenile L. polyphemus has been used to assert stabilomorphism; the “relative morphological stability of organisms in time and spatial distribution, the taxonomic status of which does not exceed genus level” (Błazejowski, 2015, p. 11). The conservation may reflect habitation of similar marine conditions, or convergence on an effective morphology.
Extant limulids have distributions across the east coast of the USA and Asia, with their common names reflecting said distribution (Shuster, 2001; Bicknell and Pates, 2019a): the American, or Atlantic, horseshoe crab, Limulus polyphemus; the Indonesian horseshoe crab, Carcinoscorpius rotundicauda; the Chinese horseshoe crab, Tachypleus gigas; and the Japanese horseshoe crab, T. tridentatus (Figures 35, 36; Itow et al., 2003; Zhou and Morton, 2004; Sekiguchi and Shuster, 2009). The ontogeny and morphology of these taxa has been documented thoroughly across the past two centuries (Shuster, 1982; Haug and Rötzer, 2018a) and the morphological similarities are depicted in Figures 35 and 36. Extant limulids occupy many environmental conditions and can exploit brackish, freshwater, shallow water, and fully-marine conditions (Siveter and Selden, 1987). Limulus polyphemus, T. gigas, and T. tridentatus are mostly shallow marine, bottom-dwelling taxa that spawn on beaches and inhabit a combination of marine sub-habits during ontogeny (Fisher, 1984). Conversely, C. rotundicauda migrates into completely freshwater (Størmer, 1952; Fisher, 1984; Crônier and Courville, 2005; Sekiguchi and Shuster, 2009; Lamsdell, 2016). Despite representing the descendants of a long fossil lineage, they now face an extinction event. Extensive harvesting of specimens for their blood, and as a food source, as well as habitat modification have majorly impacted populations (Botton, 2001; Hsieh and Chen, 2009; Shin et al., 2009; Akbar John et al., 2011; Cartwright-Taylor et al., 2011; Carmichael and Brush, 2012; Nelson et al., 2015; Kwan et al., 2016; Fairuz-Fozi et al., 2018). Measures therefore need to be taken to prevent this group from an extinction event. To this end, L. polyphemus and its kin have now been suggested as world heritage species (Tanacredi et al., 2009) and T. tridentatus was recently listed as an endangered taxon (Laurie et al., 2019) showing that progress is being made in preventing the human-driven extinction of Xiphosurida.
Valloisella
This monospecific genus from the Carboniferous Coal Measures in England and France (Figure 36) was originally considered a belinurid (Anderson and Horrocks, 1995) but has since been placed at the base of Limuloidea by recent phylogenetic analyses (Lamsdell, 2016). The genus is defined by an almond-shaped cephalothorax, genal spines that extend almost to the thoracetron terminus, and a flange located along the thoracetronic margin (Anderson and Horrocks, 1995). The fused thoracetron is trapezoidal with expressed tergal divisions, contrasting most other species in Limuloidea. No appendages are known from this genus.
Horseshoe Crab Evolutionary History and Diversity
Horseshoe crabs experienced three major evolutionary events across the Phanerozoic (Figure 1). The Palaeozoic horseshoe crab record was the most exploratory anatomically and evolutionarily (Błażejowski et al., 2017). The rise of synziphosurines began in the Lower Ordovician (Rudkin et al., 2008; Rudkin and Young, 2009; Dunlop, 2010; Van Roy et al., 2010, 2015). Across the Silurian and Devonian, the marine and marginal marine forms were abundant and represent the first evolutionary radiation of this group, before the diversification of Xiphosurida (Størmer, 1955). Synziphosurine diversity declined heavily, reducing to one taxon in the Carboniferous, when they subsequently went extinct (Selden and Drygant, 1987; Selden and Siveter, 1987; Babcock et al., 1995; Anderson and Selden, 1997; Moore et al., 2007; Lucas et al., 2014). Non-xiphosurid xiphosurans also arose in the Upper Ordovician, potentially even the Lower Ordovician, and are unknown after the Devonian (Bicknell et al., 2019c). Xiphosurida arose in the late Devonian with Bellinuroopsis (Moore et al., 2007). After this, at least four xiphosurid families arose in the Carboniferous: the Belinuridae, Limulidae, Paleolimulidae and Rolfeiidae (Selden and Drygant, 1987; Selden and Siveter, 1987; Babcock et al., 1995; Anderson and Selden, 1997; Lucas et al., 2014; Bicknell, 2019; Bicknell and Pates, 2019b; Bicknell et al., 2019e), with evidence suggesting that Austrolimulidae may also have arisen at this time (Lamsdell, 2016). Carboniferous Coal Measures and Konservat-Lagerstätten record the highest specific diversity and first radiation of Xiphosurida (Anderson, 1997; Moore et al., 2007; Rudkin and Young, 2009). Exploitation of brackish and freshwater conditions by the late Palaeozoic Xiphosurida may reflect adaptation to inconsistent coastlines and fluctuating shallow-marine conditions (Błażejowski et al., 2017). Xiphosurid diversity apparently decreased drastically during the Permian, reflecting the closure of exceptional preservation windows and an increase in xiphosurids inhabiting marginal environments that are poorly preserved in the geological record (Rudkin and Young, 2009). At the end of the Carboniferous, there is no further record of Rolfeiidae, while the first definite austrolimulid species arose in the Permian (Bicknell, 2019). The Permian-Triassic “Great Dying” drove belinurids and paleolimulids to extinction, while austrolimulids and limulids survived into the Mesozoic (Bicknell and Pates, 2019b). The Triassic was a period of extensive exploration in morphology and the second radiation of xiphosurids and the third evolutionary pulse in horseshoe crabs (Bicknell and Pates, 2019b; Bicknell et al., 2019e). An aspect of this radiation was size increase: Mesozoic taxa were much larger (30–60 cm long, including telson) than the Palaeozoic counterparts (3–5 cm) (Størmer, 1955; Bicknell and Pates, 2019b). Austrolimulid diversity peaked in the Triassic (Figure 1) but then decreased into the Cretaceous, during which time the group went extinct. Limulid diversity peaked in the Triassic with 12 species and decreased to five during the Cretaceous (Bicknell et al., 2019e). Only limulids survived into the Tertiary with one named Cenozoic species: the Eocene Limulus decheni (Rudkin and Young, 2009; Schimpf et al., 2017), a suggested “missing link” between extant Asian and American taxa (Hauschke and Wilde, 2004). This evolutionary history is one of generally low generic diversity, such as in the four extant species (Anderson and Selden, 1997; Anderson, 1999; Shuster et al., 2003; Sekiguchi and Shuster, 2009; Dunlop et al., 2012). However, the habitation of marginal environments with poor conditions for exceptional preservation of un-biomineralised exoskeleton cuticle also may have impacted this observed low diversity (Babcock, 1998; Anderson, 1999; Babcock and Merriam, 2000; Lamsdell and Mckenzie, 2015).
Geographical Distribution of Xiphosuran Material
Distribution of horseshoe crab fossils is uneven in space and time; reflecting historical biases in collecting that favored North America and Western Europe. The UK has the highest number of taxa (n = 35), followed by the USA (n = 23) and Germany (n = 22). Other areas with much larger landmasses have far fewer known taxa: South America (n = 1), Australia (n = 7), Asia (n = 5), and Africa (n = 6). This uneven geographical sampling also partly reflects uneven temporal sampling (e.g., 25 UK taxa are Carboniferous, and eight are Silurian and 11 of 22 German taxa are Triassic). Within countries, well-explored horizons or formations also provide apparent diversity peaks. Notably the South Wales Coal Measures formations (South Wales, UK) where six belinurids are known from the South Wales Lower Coal Measures Formation and three belinurids from the South Wales Upper Coal Measures Formation. These nine taxa, within a limited geographic and temporal sample, provide an apparently high Carboniferous diversity skewing the understanding of overall belinurid diversity and geographic spread as well as reflecting an over-splitting of the group. To address these sampling issues (which are by no means limited to horseshoe crabs) further exploration needs to be targeted to under-sampled regions (Africa, Asia, South America) and time periods (Jurassic and younger), as well as reassessing the apparent high diversity of taxa that have not been recently studied. Such efforts, combined with a concerted effort to redescribe and refine horseshoe crab taxonomy will allow ranges of different groups to be compared without the current underlying biases.
Future Directions
Horseshoe crabs are an iconic group of chelicerates and, as depicted here, have been thoroughly, if somewhat sporadically, scientifically explored over the past two centuries. However, in conducting this review we highlighted four main research areas that should be addressed. To conclude this review, potential future directions for horseshoe crab research are presented.
(1) Bicknell (2019), and Bicknell et al. (2019e) highlighted that the traditional views that horseshoe crabs represent evolutionary conservatism, stasis, and bradytelic evolution (Fisher, 1984; Selden and Siveter, 1987; Rudkin et al., 2008) is overstated. In reality, the group experienced three major changes across the Phanerozoic: increased size, thoracetronic fusion, and restriction to marine habitats (Størmer, 1955; Crônier and Courville, 2005; Bicknell and Pates, 2019b). Lamsdell (2016) thoroughly explored the record of habitat change, but the remaining two points should be considered. Thoracetronic fusion has been attributed to a change in ecology, from enrolment to burrowing, but this remains fairly unexplored (Fisher, 1977, 1981, 1982; Waterston, 1985; Lamsdell, 2016; Błażejowski et al., 2017). A study considering when complete fusion developed in the context of palaeoenvironmental and palaeoecological conditions may confirm this hypothesis. Size change is likely associated with exploitation of different niches: smaller Xiphosurida likely preferred freshwater conditions, reflected today in the smallest taxon—Carcinoscorpius rotundicauda (Hauschke and Wilde, 1991; Dunlop et al., 2012). A study considering shape and size change through time would allow this hypothesis to be tested. In addition, modern descriptive and statistical tools, such as multivariate geometric morphometrics, semilandmark, and landmark analyses could be employed to explore this topic in more detail (Bicknell, 2019; Bicknell and Pates, 2019b; Bicknell et al., 2019e).
(2) Rates of morphometric change in horseshoe crabs have not been thoroughly explored (Fisher, 1984). The same morphometric data outlined above could be used to address possible evolutionary rates and quantify whether the group, especially limulids, represent arrested evolution. Time series analyses can also be conducted with these data to study modes and models of evolution (Hunt and Carrano, 2010; Hunt et al., 2015; Bicknell et al., 2018a).
(3) As Tables 6 and 7 outline there are many specimens have been identified as xiphosurids but not formally (re)described in light of recent progress in the field (Lamsdell et al., 2020). Formally describing these specimens would thoroughly aid understanding patterns of horseshoe crab diversity through time. Similarly, new collecting efforts should be focussed on under-represented parts of the globe such as Asia, Africa and South America, as well as Jurassic and younger deposits, where knowledge of this group is hindered by a lack of specimens.
(4) Computer tomography (CT) scanning to document fossil and extant species has become a major tool over the past decade, which has started to positively impact horseshoe crab research. Schimpf et al. (2017) CT scanned Limulus decheni specimens to accelerate digital transfer of important morphological information (Figure 35). Zuber et al. (2017) used CT scans and augmented laminography to document muscle detail in a Limulitella sp. specimen (Figure 24), and Bicknell et al. (2018b) conducted micro-CT scans of iodine stained appendages to show L. polyphemus muscles in situ. Scanning and 3D reconstructions of specimens are still developing and therefore ripe for research, especially for documenting and disseminating information on holotypes.
Conclusions
The atlas presented here is the first comprehensive collation of named taxa and other unnamed specimens considered horseshoe crabs. The work builds on research presented during the early- to middle-twentieth century and, its presentation in an open-access environment will allow all researchers interested in horseshoe crabs access to key anatomical information needed for new taxonomic studies. Brief notes detailing the characteristic features and supposed life modes of families within Xiphosurida are presented, synthesizing other key works on the group. A brief evolutionary history of horseshoe crabs is presented, which outlines diversity changes from the Lower Ordovician to today. Finally, we highlight four major avenues for future research: most notably analyses of morphometric data of horseshoe crabs to mathematically probe the evolutionary history of the group. These same data may represent an important step toward reconciling synziphosurines with true horseshoe crabs.
Author Contributions
RB designed the study and made the figures, with input from SP. RB and SP photographed material and wrote the manuscript.
Funding
This research was supported by funding from an Australian Postgraduate Award (to RB), a University of New England Postdoctoral Research Fellowship (to RB), a Charles Schuchert and Carl O. Dunbar Grants-in-Aid award (to RB), a James R. Welch Scholarship (to RB), and an Alexander Agassiz Postdoctoral Fellowship (to SP). There is no funding for open access publication.
Conflict of Interest
The authors declare that the research was conducted in the absence of any commercial or financial relationships that could be construed as a potential conflict of interest.
Acknowledgments
We thank the following people for providing images of specimens: Alexander S. Alekseev, Allan Lerner, Andreas Abele, Bill Crighton, Błażej Błażejowski, Carrie A. Eaton, Carsten Brauckmann, Christian Neumann, Constantine Tarásenko, David Barnes, David Marshall, Dieter Seegis, Dmitry E. Shcherbakov, Dominique Chabard, Ewa Krzeminska, Frank Holmes, G. Hundertmark, Giorgio Teruzzi, Graham Young, Gregory Edgecombe, Guenter Schweigert, Hans Arne Nakrem, Martien Oosterink, Javier Ortega Hernández, Jessica Utrup, Jessie Cuvelier, Josh White, Kallie Moore, K. C. Kratt, Lars Schimpf, Lilian Caze, Liping Liu, Lorenzo Lustri, Lucie Goodayle, Lukáš Laibl, Mark Renczkowski, Markus Bertling, Markus Poschmann, Matt Stimson, Mertová Eva, Michelle Coyne, Mike Howe, Mike Reich, Monica Solorzano-Kraemer, Norbert Hauschke, Patrick Smith, Paul Selden, Pedro Adserà, Peter Mansfeld, Rodney Feldman, Russell Garwood, Serge Naugolnykh, Sergey Bagirov, Shixue Hu, Tai Kubo, Thomas König, and Tomaž Hitij.
We thank the following people for their help with collections: Albert Kollar, Andreas Abele, Andrew Ross, Angelika Leipner, Brandon Strilisky, Bushra M. Hussaini, Conrad Labandeira, Constantine Tarásenko, Cornelia Kurz, David Gelsthorpe, David Holloway, Hans Arne Nakrem, Isabella von Lichtan, Jessica Cundiff, Jessica Utrup, Jodie Francis, Julien Kimmig, Kacey Page, Lisa Amati, Mark Florence, Matt Riley, Matthew McCurry, Melanie Hopkins, Mertová Eva, Michelle Coyne, Mike Reich, Susan Butts, Sylvain Charbonnier, Takenori Sasaki, Tatiana Tolmacheva, Thomas Servais, and Yong-Yi Zhen.
Finally, we thank Allan Lerner, Błażej Błażejowski, Norbert Hauschke, and Rachel Wade for their support and discussions around this work and the editor PS and two reviewers for their detailed input that thoroughly improved this work.
References
Akbar John, B., Jalal, K. C. A., Zaleha, K., Armstrong, P., and Kmaruzzaman, B. Y. (2011). Effects of blood extraction on the mortality of Malaysian horseshoe crabs (Tachypleus gigas). Mar. Freshw Behav. Physiol. 44, 321–327. doi: 10.1080/10236244.2011.642505
Allen, J. G., and Feldmann, R. M. (2005). Panduralimulus babcocki n. gen. and sp., a new Limulacean horseshoe crab from the Permian of Texas. J. Paleontol. 79, 594–600. doi: 10.1666/0022-3360(2005)079<0594:pbngas>2.0.co;2
Ambrose, T., and Romano, M. (1972). New Upper Carboniferous Chelicerata (Arthropoda) from Somerset, England. Palaeontology 15, 569–578.
Anderson, L. I. (1994). Xiphosurans from the Westphalian D of the Radstock Basin, Somerset Coalfield, the South Wales Coalfield and Mazon Creek, Illinois. Proc. Geol. Assoc. 105, 265–275. doi: 10.1016/S0016-7878(08)80179-4
Anderson, L. I. (1997). The xiphosuran Liomesaspis from the Montceau-les-Mines Konservat-Lagerstätte, Massif Central, France. Neues. Jahrb. Geol. Palaontol. Abh. 204, 415–436. doi: 10.1127/njgpa/204/1997/415
Anderson, L. I. (1999). A new specimen of the Silurian synziphosurine arthropod Cyamocephalus. Proc. Geol. Assoc. 110, 211–216. doi: 10.1016/S0016-7878(99)80071-6
Anderson, L. I., Dunlop, J. A., Eagar, R. M. C., Horrocks, C. A., and Wilson, H. M. (1999). Soft-bodied fossils from the roof shales of the Wigan Four Foot coal seam, Westhoughton, Lancashire, UK. Geol. Mag. 136, 321–329. doi: 10.1017/S0016756899002575
Anderson, L. I., Dunlop, J. A., Horrocks, C. A., Winkelmann, H. M., and Eagar, R. M. C. (1997). Exceptionally preserved fossils from Bickershaw, Lancashire UK (Upper Carboniferous, Westphalian A (Langsettian)). Geol. J. 32, 197–210.
Anderson, L. I., and Horrocks, C. (1995). Valloisella lievinensis Racheboeuf, 1992 (Chelicerata: Xiphosura) from the Westphalian B of England. Neues. Jahrb. Geol. Palaontol. Mh. 11, 647–658. doi: 10.1127/njgpm/1995/1995/647
Anderson, L. I., and Moore, R. A. (2003). Bembicosoma re-examined: a xiphosuran from the Silurian of the North Esk Inlier, Pentland Hills, Scotland. Earth Environ. Sci. Trans. R. Soc. Edinb. 94, 199–206. doi: 10.1017/S0263593300000614
Anderson, L. I., Poschmann, M., and Brauckmann, C. (1998). On the Emsian (Lower Devonian) arthropods of the Rhenish Slate Mountains: 2. The synziphosurine Willwerathia. Paläontol. Z. 72, 325–336. doi: 10.1007/BF02988363
Anderson, L. I., and Selden, P. A. (1997). Opisthosomal fusion and phylogeny of Palaeozic Xiphosura. Lethaia 30, 19–31. doi: 10.1111/j.1502-3931.1997.tb00440.x
Anderson, L. I., and Shuster, C. N. Jr. (2003). “Throughout geologic time: where have they lived,” in The American Horseshoe Crab, eds C. N. Shuster Jr., R. B. Barlow, and H. J. Brockmann (Cambridge: Harvard University Press, 189–223.
Babcock, L. E. (1998). Experimental investigation of the processes of fossilization. J. Geosci. Educ. 46, 252–260. doi: 10.5408/1089-9995-46.3.252
Babcock, L. E., and Merriam, D. F. (2000). Horseshoe crabs (Arthropoda: Xiphosurida) from the Pennsylvanian of Kansas and elsewhere. Trans. Kans. Acad. Sci. 103, 76–94. doi: 10.2307/3627941
Babcock, L. E., Merriam, D. F., and West, R. R. (2000). Paleolimulus, an early limuline (Xiphosurida), from Pennsylvanian-Permian Lagerstätten of Kansas and taphonomic comparison with modern Limulus. Lethaia 33, 129–141. doi: 10.1080/00241160025100017
Babcock, L. E., Wegweiser, M. D., Wegweiser, A. E., Stanley, T. M., and Mckenzie, S. C. (1995). Horseshoe crabs and their trace fossils from the Devonian of Pennsylvania. Penn. Geol. 26, 2–7.
Bábek, O., Mikuláš, R., Zapletal, J., and Lehotský, T. (2004). Combined tectonic-sediment supply-driven cycles in a Lower Carboniferous deep-marine foreland basin, Moravice Formation, Czech Republic. Int. J. Earth Sci. 93, 241–261. doi: 10.1007/s00531-004-0388-5
Baily, W. H. (1863). Remarks on some coal-measure Crustacea belonging to the genus Belinurus, König; with description of two new species from Queen's County, Ireland. Ann. Mag. Nat. Hist. 11, 107–114. doi: 10.1080/00222936308681390
Baily, W. H. (1870). On Fossils Obtained at Kiltorcan Quarry, Co. Kilkenny. Report of the British Association for the Advancement of Science.
Baldwin, W. (1902). On Prestwichia rotundata found in Sparth Bottoms, Rochdale, Lancashire. Trans. Manchester Geol. Soc. 27, 149–155.
Baldwin, W. (1905). Belinurus bellulus, from Sparth, Rochdale. Trans. Manchester Geol. Soc. 28, 198–202.
Baldwin, W. (1906). Prestwichia anthrax and Bellinurus lunatus from Sparth Bottoms, Rochdale. Trans. Manchester Geol. Soc. 29, 124–128.
Beecher, C. E. (1902). Note on a new xiphosuran from the Upper Devonian of Pennsylvania. Am. Geol. 29, 143–146.
Bellmann, H. J. (1997). Die Domsener Sande und die Funde von Limulus decheni zincken bei Teuchern. Hallesches Jahrbuch Geowissensch. 19, 115–119.
Bergström, J. (1968). Eolimulus, a lower Cambrian xiphosurid from Sweden. Geologiska Föreningen Stockholm Förhandlingar 90, 489–503. doi: 10.1080/11035896809454937
Bergström, J. (1975). Functional morphology and evolution of xiphosurids. Fossils Strata 4, 291–305.
Bicknell, R. D. C., Žalohar, J., Miklavc, P., Celarc, B., Križnar, M., and Hitij, T. (2019e). A new limulid genus from the Strelovec Formation (Middle Triassic, Anisian) of northern Slovenia. Geol. Mag. 156, 2017–2030. doi: 10.1017/S0016756819000323
Bicknell, R. D. C. (2019). Xiphosurid from the Upper Permian of Tasmania confirms Palaeozoic origin of Austrolimulidae. Palaeontol. Electron. 22, 1–13. doi: 10.26879/1005
Bicknell, R. D. C., Amati, L., and Ortega Hernández, J. (2019a). New insights into the evolution of lateral compound eyes in Palaeozoic horseshoe crabs. Zool. J. Linn. Soc. 187, 1061–1077. doi: 10.1093/zoolinnean/zlz065
Bicknell, R. D. C., Brougham, T., Charbonnier, S., Sautereau, F., Hitij, T., and Campione, N. E. (2019b). On the appendicular anatomy of the xiphosurid Tachypleus syriacus and the evolution of fossil horseshoe crab appendages. Sci. Nat. 106:38. doi: 10.1007/s00114-019-1629-6
Bicknell, R. D. C., Collins, K. S., Crundwell, M., Hannah, M., Crampton, J. S., and Campione, N. E. (2018a). Evolutionary transition in the late Neogene planktonic foraminiferal genus Truncorotalia. iScience 8, 295–303. doi: 10.1016/j.isci.2018.09.013
Bicknell, R. D. C., Klinkhamer, A. J., Flavel, R. J., Wroe, S., and Paterson, J. R. (2018b). A 3D anatomical atlas of appendage musculature in the chelicerate arthropod Limulus polyphemus. PLoS ONE 13:e0191400. doi: 10.1371/journal.pone.0191400
Bicknell, R. D. C., Lustri, L., and Brougham, T. (2019c). Revision of ‘Bellinurus’ carteri (Chelicerata: Xiphosura) from the late Devonian of Pennsylvania, USA. C. R. Palevol. 18, 967–976. doi: 10.1016/j.crpv.2019.08.002
Bicknell, R. D. C., Paterson, J. R., Caron, J.-B., and Skovsted, C. B. (2018c). The gnathobasic spine microstructure of recent and Silurian chelicerates and the Cambrian artiopodan Sidneyia: functional and evolutionary implications. Arthropod Struct. Dev. 47, 12–24. doi: 10.1016/j.asd.2017.12.001
Bicknell, R. D. C., and Pates, S. (2019a). Abnormal extant xiphosurids in the Yale Peabody Museum Invertebrate Zoology collection. Bull. Peabody Mus. Nat. Hist. 60, 41–53. doi: 10.3374/014.060.0102
Bicknell, R. D. C., and Pates, S. (2019b). Xiphosurid from the Tournaisian (Carboniferous) of Scotland confirms deep origin of Limuloidea. Sci. Rep. 9:17102. doi: 10.1038/s41598-019-53442-5
Bicknell, R. D. C., Pates, S., and Botton, M. L. (2018d). Abnormal xiphosurids, with possible application to Cambrian trilobites. Palaeontol. Electron. 21, 1–17. doi: 10.26879/866
Bicknell, R. D. C., Pates, S., and Botton, M. L. (2019d). Euproops danae (Belinuridae) cluster confirms deep origin of gregarious behaviour in xiphosurids. Arthrop. Sel. 28, 549–555. doi: 10.15298/arthsel.28.4.07
Błażejowski, B., Niedźwiedzki, G., Boukhalfa, K., and Soussi, M. (2017). Limulitella tejraensis, a new species of limulid (Chelicerata, Xiphosura) from the Middle Triassic of southern Tunisia (Saharan Platform). J. Paleontol. 91, 960–967. doi: 10.1017/jpa.2017.29
Błazejowski, B. (2015). “The oldest species of the genus Limulus from the Late Jurassic of Poland,” in Changing Global Perspectives on Horseshoe Crab Biology, Conservation and Management, eds R. H. Carmichael, M. L. Botton, P. K. S. Shin, and S. G. Cheung (Cham: Springer), 3–14. doi: 10.1007/978-3-319-19542-1_1
Błazejowski, B., Gieszcz, P., Brett, C. E., and Binkowski, M. (2015). A moment from before 365 Ma frozen in time and space. Sci. Rep. 5:14191. doi: 10.1038/srep14191
Błazejowski, B., Gieszcz, P., Shinn, A. P., Feldmann, R. M., and Durska, E. (2019). Environment deterioration and related fungal infection of Upper Jurassic horseshoe crabs with remarks on their exceptional preservation. Palaeogeogr. Palaeoclimatol. Palaeoecol. 516, 336–341. doi: 10.1016/j.palaeo.2018.12.015
Błazejowski, B., Gieszcz, P., and Tyborowski, D. (2016). New finds of well-preserved Tithonian (Late Jurassic) fossils from the Owadów-Brzezinki Quarry, Central Poland: a review and perspectives. Volumina Jurassica 14, 123–132. doi: 10.5604/17313708.1222641
Bleicher, M.-G. (1897). Sur la découverte d'une nouvelle espèce de limule dans les marnes irisées de Lorraine. Bull. Soc. Sci. 2, 116–126.
Bluck, B. J. (1967). Deposition of some Upper Old Red Sandstone conglomerates in the Clyde area: a study in the significance of bedding. Scottish J. Geol. 3, 139–167. doi: 10.1144/sjg03020139
Böhm, J. (1908). Über Limulus decheni Zincken. Jahrbuch Königlich Preussischen Geologischen Landesanstalt Bergakademie 26, 240–245.
Bölsche, W. (1879). Über Prestwichia rotundata H. Woodw. sp. aus der Steinkohlenformation des Piesberges bei Osnabrück. Jahresbericht Naturwissensch. Vereins Osnabrück 6, 268–271.
Botton, M. L. (1984). Diet and food preferences of the adult horseshoe crab Limulus polyphemus in Delaware Bay, New Jersey, USA. Mar. Biol. 81, 199–207. doi: 10.1007/BF00393118
Botton, M. L. (2001). “The conservation of horseshoe crabs: what can we learn from the Japanese experience?” in Limulus in the Limelight, ed J. T. Tanacredi (New York, NY: Springer), 41–51. doi: 10.1007/0-306-47590-1_4
Botton, M. L., and Ropes, J. W. (1987). The horseshoe crab, Limulus polyphemus, fishery and resource in the United States. Mar. Fish. Rev. 49, 57–61.
Brauckmann, C. (1982). Der Schwertschwanz Euproops (Xiphosuraida, Limulina, Euproopacea) aus dem Ober-Karbon des Piesbergs bei Osnabrück. Osnabücker Naturwissensch. Mitteilungen 9, 17–26.
Brauckmann, C. (2005). Ausgewahlte Arthropoden: Insecta, Arachnida, Xiphosura, Eurypterida, Myriapoda, Arthropleurida und Trilobita. Courier Forschungsinstitut Senckenberg 254, 87–101.
Braun, K. F. W. (1860). Die Thiere in den Pflanzenschiefern der Gegend von Bayreuth. Jahresbericht von der König. Kreis Landwirtschafts Gewerbschule zu Bayreuth Schuljahr 1859/60.
Briggs, D. E. G., Moore, R. A., Shultz, J. W., and Schweigert, G. (2005). Mineralization of soft-part anatomy and invading microbes in the horseshoe crab Mesolimulus from the upper Jurassic Lagerstätte of Nusplingen, Germany. Proc. R. Soc. Lond. B Biol. Sci. 272, 627–632. doi: 10.1098/rspb.2004.3006
Briggs, D. E. G., Siveter, D. J., Siveter, D. J., Sutton, M. D., Garwood, R. J., and Legg, D. (2012). Silurian horseshoe crab illuminates the evolution of arthropod limbs. Proc. Nat. Acad. Sci. U.S.A. 109, 15702–15705. doi: 10.1073/pnas.1205875109
Briggs, D. E. G., and Wilby, P. R. (1996). The role of the calcium carbonate-calcium phosphate switch in the mineralization of soft-bodied fossils. J. Geol. Soc. 153, 665–668. doi: 10.1144/gsjgs.153.5.0665
Brockmann, H. J. (1990). Mating behavior of horseshoe crabs, Limulus polyphemus. Behaviour 114, 206–220. doi: 10.1163/156853990X00121
Buckland, W. (1837). The Bridgewater Treatises on the Power, Wisdom and Goodness of God as Manifested in the Creation. Treatise IV. Geology and Mineralogy with Reference to Natural Theology. London: William Pickering.
Carmichael, R. H., and Brush, E. (2012). Three decades of horseshoe crab rearing: a review of conditions for captive growth and survival. Rev. Aquacul. 4, 32–43. doi: 10.1111/j.1753-5131.2012.01059.x
Cartwright-Taylor, L., Von Bing, Y., Chi, H. C., and Tee, L. S. (2011). Distribution and abundance of horseshoe crabs Tachypleus gigas and Carcinoscorpius rotundicauda around the main island of Singapore. Aquat. Biol. 13, 127–136. doi: 10.3354/ab00346
Chatterji, A., and Pati, S. (2014). Allometric relationship in the adult population of the Malaysian horseshoe crab (Tachypleus tridentatus; Leach). Int. J. Res. 1, 1378–1385.
Chernyshev, B. I. (1928). Nouvelles donnees sur les Xiphosura du basin Donetz. Bull. Comité Géol. 47, 519–531.
Chernyshev, B. I. (1933). Arthropoda from the Urals and other regions of the USSR. Mater. Centr. Sci. Prospect. Inst. Paleontol. Stratigr. 1, 15–25.
Chlupáč, I. (1963). Report on the merostomes from the Ordovician of Central Bohemia. Věstník Ústredního Ústavu Geol. 38, 399–402.
Chlupáč, I. (1965). Xiphosuran merostomes from the Bohemian Ordovician. Sborník Geol. Paleontol. 5, 7–38.
Chlupáč, I. (1999). Unusual arthropods from the Bohemian Ordovician-a review. Acta Univ. Carol. Geol. 43, 393–396.
Clarke, J. M. (1919). Bunaia Woodwardi, a new merostome from the Silurian waterlimes of New York. Geol. Mag. 6, 531–533. doi: 10.1017/S0016756800202100
Clarkson, E. N. K. (1985). Palaeoecology of the Dinantian of Foulden, Berwickshire, Scotland. Earth Environ. Sci. Trans. R. Soc. Edinb. 76, 97–100. doi: 10.1017/S0263593300010336
Cole, G. A. (1901). On Belinurus kiltorkensis, Baily. Geol. Mag. 8, 52–54. doi: 10.1017/S0016756800174837
Copeland, M. J. (1957a). The arthropod fauna of the Upper Carboniferous rocks of the Maritime Provinces. Geol. Surv. Can. 286, 1–110. doi: 10.4095/101505
Copeland, M. J. (1957b). The Carboniferous genera Palaeocaris and Euproops in the Canadian maritime provinces. J. Paleontol. 31, 595–599.
Crônier, C., and Courville, P. (2005). New xiphosuran merostomata from the Upper Carboniferous of the Graissessac Basin (Massif Central, France). C. R. Palevol. 4, 123–133. doi: 10.1016/j.crpv.2004.11.002
Currie, L. D. (1927). On Cyamocephalus, a new synxiphosuran from the Upper Silurian of Lesmahagow, Lanarkshire. Geol. Mag. 64, 153–157. doi: 10.1017/S0016756800104492
Desmarest, A.-G. (1822). “Les crustacés proprement dits,” in Histoire Naturelle des Crustacés Fossiles, Sous les Rapports Zoologiques et Geologiques, eds A. Brongniart and A.-G. Desmarest (Paris: F.-G. Levrault), 67–142.
Diedrich, C. G. (2011). Middle Triassic horseshoe crab reproduction areas on intertidal flats of Europe with evidence of predation by archosaurs. Biol. J. Linnean Soc. 103, 76–105. doi: 10.1111/j.1095-8312.2011.01635.x
Dix, E., and Jones, S. H. (1932). A note on an arthropod from the South Wales coalfield. Geol. Mag. 69, 275–277. doi: 10.1017/S0016756800097703
Dix, E., and Pringle, J. (1929). On the fossil Xiphosura from the South Wales Coalfield with a note on the myriapod Euphoberia. Summ. Prog. Geol. Surv. 1928, 90–113.
Dix, E., and Pringle, J. (1930). Some coal measure arthropods from the South Wales coalfield. J. Nat. Hist. 6, 136–144. doi: 10.1080/00222933008673194
Dopita, M., and Kumpera, O. (1993). Geology of the Ostrava-Karviná coalfield, Upper Silesian Basin, Czech Republic, and its influence on mining. Int. J. Coal Geol. 23, 291–321. doi: 10.1016/0166-5162(93)90053-D
Dunbar, C. O. (1923). Kansas Permian insects, Part 2, Paleolimulus, a new genus of Paleozoic Xiphosura, with notes on other genera. Am. J. Sci. 5, 443–454. doi: 10.2475/ajs.s5-5.30.443
Dunlop, J. A. (2010). Geological history and phylogeny of Chelicerata. Arthropod Struct. Dev. 39, 124–142. doi: 10.1016/j.asd.2010.01.003
Dunlop, J. A., Compton, M. S., and Friederichs, A. (2012). An annotated catalogue of the horseshoe crabs (Xiphosura) held in the Museum für Naturkunde Berlin. Zoosyst. Evol. 88, 215–222. doi: 10.1002/zoos.201200018
Dunlop, J. A., and Lamsdell, J. C. (2017). Segmentation and tagmosis in Chelicerata. Arthropod Struct. Dev. 46, 396–418. doi: 10.1016/j.asd.2016.05.002
Dunlop, J. A., and Selden, P. A. (1998). “The early history and phylogeny of the chelicerates,” in Arthropod Relationships, eds R. A. Fortey and R. H. Thomas (Dordrecht: Springer), 221–235. doi: 10.1007/978-94-011-4904-4_17
Dunlop, J. A., Penney, D., and Jekel, D. (2019). “A summary list of fossil spiders and their relatives,” in World Spider Catalog, version 20.0. Bern: Natural History Museum Bern.
Eagles, D. A. (1973). Tailspine movement and its motor control in Limulus polyphemus. Comp. Biochem. Physiol. A Mol. Integr. Physiol. 46A, 391–407. doi: 10.1016/0300-9629(73)90428-3
Ebert, M., Kölbl-Ebert, M., and Lane, J. A. (2015). Fauna and predator-prey relationships of Ettling, an actinopterygian fish-dominated Konservat-Lagerstätte from the Late Jurassic of Southern Germany. PLoS ONE 10:e0116140. doi: 10.1371/journal.pone.0116140
Edgecombe, G. D. (1998a). Devonian terrestrial arthropods from Gondwana. Nature 394, 172–175. doi: 10.1038/28156
Edgecombe, G. D. (1998b). Early myriapodous arthropods from Australia: Maldybulakia from the Devonian of New South Wales. Rec. Aust. Mus. 50, 293–314. doi: 10.3853/j.0067-1975.50.1998.1288
Eichwald, E. (1854). Die Grauwackenschichten von Liev-und Esthland. Bull. Soc. Imperiale Nat. Moscou 27, 1–211.
Eldredge, N. (1974). Revision of the suborder Synziphosurina (Chelicerata, Merostomata), with remarks on merostome phylogeny. Am. Mus. Novitates 2543, 1–41.
Eldredge, N. (1976). Differential evolutionary rates. Paleobiology 2, 174–177. doi: 10.1017/S0094837300003456
Eldredge, N., and Plotnick, R. E. (1974). Revision of the pseudoniscine merostome genus Cyamocephalus Currie. Am. Mus. Novitates 2557, 1–10.
Eller, E. R. (1938a). A new xiphosuran, Euproops morani, from the upper Devonian of Pennsylvania. Ann. Carnegie Mus. 27, 152–153.
Eller, E. R. (1938b). A review of the xiphosuran genus Belinurus with the description of a new species, B. alleganyensis. Ann. Carnegie Mus. 27, 129–150.
Eller, E. R. (1940). Belinurus carteri, a new xiphosuran from the upper Devonian of Pennsylvania. Ann. Carnegie Mus. 28, 133–136.
Engelder, T., and Oertel, G. (1985). Correlation between abnormal pore pressure and tectonic jointing in the Devonian Catskill Delta. Geology 13, 863–866.
Eros, J. M., Montañez, I. P., Osleger, D. A., Davydov, V. I., Nemyrovska, T. I., Poletaev, V. I., et al. (2012). Sequence stratigraphy and onlap history of the Donets Basin, Ukraine: insight into Carboniferous icehouse dynamics. Palaeogeogr. Palaeoclimatol. Palaeoecol. 313, 1–25. doi: 10.1016/j.palaeo.2011.08.019
Ewington, D. L., Clarke, M. J., and Banks, M. R. (1989). A Late Permian fossil horseshoe crab (Paleolimulus: Xiphosura) from Poatina, Great Western Tiers, Tasmania. Pap. Proc. R Soc. 123, 127–131. doi: 10.26749/rstpp.123.127
Fairuz-Fozi, N., Satyanarayana, B., Zauki, N. a.M., Muslim, A. M., Husain, M.-L., et al. (2018). Carcinoscorpius rotundicauda (Latreille, 1802) population status and spawning behaviour at Pendas coast, Peninsular Malaysia. Glob. Ecol. Conserv. 15:e00422. doi: 10.1016/j.gecco.2018.e00422
Feldmann, R. M., Schweitzer, C. E., Dattilo, B., and Farlow, J. O. (2011). Remarkable preservation of a new genus and species of limuline horseshoe crab from the Cretaceous of Texas, USA. Palaeontology 54, 1337–1346. doi: 10.1111/j.1475-4983.2011.01103.x
Fiebelkorn, M. (1895). Die Braunkohlenablagerungen zwischen Weissenfels und Zeitz. Zeitschr. Prakt. Geol. 1895, 353–365.
Filipiak, P., and Krawczynski, W. (1996). Westphalian xiphosurans [Chelicerata] from the Upper Silesia Coal Basin of Sosnowiec, Poland. Acta Palaeontol. Pol. 41, 413–425.
Fisher, D. C. (1977). Functional significance of spines in the Pennsylvanian horseshoe crab Euproops danae. Paleobiology 3, 175–195. doi: 10.1017/S009483730000525X
Fisher, D. C. (1979). “Evidence for subaerial activity of Euproops danae (Merostomata, Xiphosurida),” in Mazon Creek Fossils, ed M. H. Nitecki (New York, NY: Elsevier, 379–447.
Fisher, D. C. (1981). The role of functional analysis in phylogenetic inference: examples from the history of the Xiphosura. Am. Zool. 21, 47–62. doi: 10.1093/icb/21.1.47
Fisher, D. C. (1982). Phylogenetic and macroevolutionary patterns within the Xiphosurida. Proc. Third North Am. Paleontol. Convent. 1, 175–180.
Fisher, D. C. (1984). “The Xiphosurida: archetypes of bradytely?” in Living Fossils, eds N. Eldredge and S. M. Stanley (New York, NY: Springer, 196–213.
Fritsch, A. (1899). Preliminary note on Prolimulus Woodwardi, Fritsch, from the Permian Gaskohle at Nyran, Bohemia. Geol. Mag. 6, 57–58. doi: 10.1017/S0016756800141974
Gall, J. C., and Grauvogel-Stamm, L. (1999). “Paläoökologie des des Oberen Buntsandsteins am Westrand des Germanischen Beckens: Der Voltziensandstein im nordöstlichen Frankreich als deltaische Bildung,” in Trias: eine Ganz andere Welt, eds N. Hauschke and V. Wilde (München: Verlag Friedrich Pfeil, 89–120.
Garassino, A., de Angeli, A., and Giovanni, B. P. (2008). New decapod assemblage from the upper Cretaceous (Cenomanian-Turonian) of Gara Sbaa, southeastern Morocco. Atti Soc. Ligust. Sc. Nat. Geogr. 149, 37–67.
Garwood, R. J., and Dunlop, J. (2014). Three-dimensional reconstruction and the phylogeny of extinct chelicerate orders. Peer J 2:e641. doi: 10.7717/peerj.641
Gerhart, S. D. (2007). A Review of the Biology and Management of Horseshoe Crabs, With Emphasis on Florida Populations. Technical Report TR−12. Fish and Wildlife Research Institute, 2.
Giebel, C. (1863). Limulus Decheni Znk im Braunkohlensandstein bei Teuchern. Z. Naturwissensch. 21, 64–68.
Gladwell, D. J. (2018). Asterozoans from the Ludlow Series (Upper Silurian) of Leintwardine, Herefordshire, UK. Pap. Palaeontol. 4, 101–160. doi: 10.1002/spp2.1101
Glushenko, N. V., and Ivanov, V. K. (1961). Paleolimulus from the Lower Permian of the Donetz Basin. Paleontol. Ž. 1961, 128–130.
Hagadorn, J. W. (2002). “Bear Gulch: an exceptional upper Carboniferous plattenkalk,” in Exceptional Fossil Preservation: A Unique View on the Evolution of Marine Life, eds D. J. Bottjer, W. Etter, J. W. Hagadorn, and C. M. Tang (New York, NY: Columbia University Press, 167–183.
Hannibal, J. T., and Feldmann, R. M. (1981). Systematics and functional morphology of oniscomorph millipedes (Arthropoda: Diplopoda) from the Carboniferous of North America. J. Paleontol. 55, 730–746.
Haug, C., and Haug, J. T. (2020). Untangling the Gordian knot—further resolving the super-species complex of 300-million-year-old xiphosurids by reconstructing their ontogeny. Dev. Gen. Evol. 230, 13–26. doi: 10.1007/s00427-020-00648-7
Haug, C., and Rötzer, M. A. I. N. (2018a). The ontogeny of Limulus polyphemus (Xiphosura s. str., Euchelicerata) revised: looking “under the skin”. Dev. Genes Evol. 228, 49–61. doi: 10.1007/s00427-018-0603-1
Haug, C., and Rötzer, M. A. I. N. (2018b). The ontogeny of the 300 million year old xiphosuran Euproops danae (Euchelicerata) and implications for resolving the Euproops species complex. Dev. Genes Evol. 228, 63–74. doi: 10.1007/s00427-018-0604-0
Haug, C., van Roy, P., Leipner, A., Funch, P., Rudkin, D. M., Schöllmann, L., et al. (2012). A holomorph approach to xiphosuran evolution—a case study on the ontogeny of Euproops. Dev. Genes Evol. 222, 253–268. doi: 10.1007/s00427-012-0407-7
Haug, J. T., Haug, C., Waloszek, D., and Schweigert, G. (2011). The importance of lithographic limestones for revealing ontogenies in fossil crustaceans. Swiss J. Geosci. 104, 85–98. doi: 10.1007/s00015-010-0033-1
Hauschke, N., Oosterink, H. W., and Wilde, V. (2009). Erster Nachweis eines Limuliden (Xiphosura, Limulacea) im Muschelkalk von Winterswijk (Niederlande). Der Aufschluss 60, 13–23.
Hauschke, N. (2013). “Die Geologisch-Paläontologischen Sammlungen,” in Die Akademischen Sammlungen und Museen der Martin-Luther-Universität Halle-Wittenberg, ed S. Lehmann (Halle (Saale): Universität Halle-Wittenberg, 80–82.
Hauschke, N., and Mertmann, D. (2015). Ausgewählte Fossilfunde aus den Geologisch-Paläontologischen Sammlungen der Martin-Luther-Universität in Halle (Saale): Sachsen-Anhalt. Der Aufschluss 66, 335–351.
Hauschke, N., and Mertmann, D. (2016). Ausgewählte Fossilfunde aus den Geologisch-Paläontologischen Sammlungen der Martin-Luther-Universität in Halle (Saale): Deutschland. Der Aufschluss 67, 325–353.
Hauschke, N., and Wilde, V. (1984). Limuliden-Reste aus dem unteren Lias Frankens. Mitt. Bayer. Staatssamml. Paläontol. 24, 51–56.
Hauschke, N., and Wilde, V. (1987). Paleolimulus fuchsbergensis n. sp. (Xiphosura, Merostomata) aus der oberen Trias von Nordwestdeutschland, mit einer Übersicht zur Systematik und Verbreitung rezenter Limuliden. Palaontol. Z. 61, 87–108. doi: 10.1007/BF02985944
Hauschke, N., and Wilde, V. (1989). Ein Limulide aus dem Zechstein (Oberes Perm) der Korbacher Bucht (Hessen, Bundesrepublik Deutschland). Geol. Jahrbuch Hessen 117, 17–21.
Hauschke, N., and Wilde, V. (1991). Zur Verbreitung und Ökologie mesozoischer Limuliden. Neues Jahrb. Geol. Palaontol. Abh. 183, 391–411.
Hauschke, N., and Wilde, V. (2000). Limulidenreste aus dem unteren Buntsandstein (Bernburg-Formation) von Beesenlaublingen (Sachesen-Anhalt). Hallesches Jahrbuch Geowissensch. 22, 87–90.
Hauschke, N., and Wilde, V. (2004). Palaeogene limulids (Xiphosura) from Saxony-Anhalt (Germany): systematics and palaeobiogeography. Hallesches Jahrbuch Geowissensch. 18, 161–168.
Hauschke, N., and Wilde, V. (2008). Limuliden aus dem Oberen Buntsandstein von Süddeutschland. Hallesches Jahrbuch Geowissensch. 30, 21–26.
Hauschke, N., Wilde, V., and Brauckmann, C. (2004). Triassic limulids from Madagascar-missing links in the distribution of Mesozoic Limulacea. N. Jb. Geol. Paläontol. Mh. 2, 87–94. doi: 10.1127/njgpm/2004/2004/87
Hauschke, N., Wilde, V., and Pietrzeniuk, E. (1992). Ein Limulide aus dem Muschelkalk (mittlere Trias) von Rüdersdorf bei Berlin. Z. Geol. Wissenschaft 20, 461–466.
Hauschke, N. (2018). “HALLE: The Palaeontological Collection of the Martin Luther University Halle-wittenberg in Halle (Saale),” in Paleontological Collections of Germany, Austria and Switzerland, eds L. A. Beck and U. Joger (Cham: Springer, 281–292.
Hauschke, N. (2014). Conchostraken als Zeitmarken und Faziesanzeiger in kontinentalen Ablagerungen der Trias: Fallbeispiele aus Sachsen-Anhalt und dem östlichen Niedersachsen. Abh. Ber. Nat. 34, 19–55.
Hauschke, N., Shukla, U. K., and Becker, A. (2005). Der Mittlere Buntsandstein (Solling-Formation, Chirotheriensandstein) im Merkelschen Steinbruch in Bernburg an der Saale (Sachsen-Anhalt)-neue Untersuchungen in einem klassischen Aufschluss. Hallesches Jahrbuch Geowissensch. 19, 119–126.
Holland, F. D., Erickson, J. M., and O'brien, D. E. (1975). Casterolimulus: a new late Cretaceous generic link in limulid lineage. Bull. Am. Paleontol. 62, 235–249.
Hsieh, H.-L., and Chen, C.-P. (2009). “Conservation program for the Asian horseshoe crab Tachypleus tridentatus in Taiwan: characterizing the microhabitat of nursery grounds and restoring spawning grounds,” in Biology and Conservation of Horseshoe Crabs, eds J. T. Tanacredi, M. L. Botton, and D. R. Smith (New York, NY: Springer), 417–438. doi: 10.1007/978-0-387-89959-6_26
Hu, S., Zhang, Q., Feldmann, R. M., Benton, M. J., Schweitzer, C. E., Huang, J., et al. (2017). Exceptional appendage and soft-tissue preservation in a Middle Triassic horseshoe crab from SW China. Sci. Rep. 7:14112. doi: 10.1038/s41598-017-13319-x
Hu, S.-X., Zhang, Q.-Y., Chen, Z.-Q., Zhou, C.-Y., Lü, T., Xie, T., et al. (2011). The Luoping biota: exceptional preservation, and new evidence on the Triassic recovery from end-Permian mass extinction. Proc. R. Soc. B 278, 2274–2282. doi: 10.1098/rspb.2010.2235
Hunt, G., and Carrano, M. T. (2010). Models and methods for analyzing phenotypic evolution in lineages and clades. Spec. Pap. Paleontol. Soc. 16, 245–269. doi: 10.1017/S1089332600001893
Hunt, G., Hopkins, M. J., and Lidgard, S. (2015). Simple versus complex models of trait evolution and stasis as a response to environmental change. Proc. Natl. Acad. Sci. U.S.A. 112, 4885–4890. doi: 10.1073/pnas.1403662111
Itow, T., Kato, H., and Kato, K. (2003). Horseshoe crabs in Australia. Bull. Educ. Fac. Shizuoka Univ. Nat. Sci. Ser. 53, 11–22.
Jackson, R. T. (1906). A new species of fossil Limulus from the Jurassic of Sweden. Artiv. Zool. 3, 1–7.
Jansen, U., and Türkay, M. (2010). Palaeontological collections of the Senckenberg Museum (Frankfurt am Main, Germany): new initiatives. Geol. Curator 9, 255–260.
Jawahir, A., Samsur, M., Shabdin, M. L., and Khairul, A. (2017). Morphometric allometry of horseshoe crab, Tachypleus gigas at west part of Sarawak waters, Borneo, East Malaysia. Aquac. Aquar. Conserv. Legisl. 10, 18–24.
Jones, T. R., and Woodward, H. (1899). Contributions to fossil Crustacea. Geol. Mag. 6, 388–395. doi: 10.1017/S0016756800142487
Kaplan, R., Li, S. S. L., and Kehoe, J. M. (1977). Molecular characterization of limulin, a sialic acid binding lectin from the hemolymph of the horseshoe crab, Limulus polyphemus. Biochemistry 16, 4297–4303. doi: 10.1021/bi00638a026
Kin, A., and Błazejowski, B. (2014). The horseshoe crab of the genus Limulus: living fossil or stabilomorph? PLoS ONE 9:e108036. doi: 10.1371/journal.pone.0108036
Kin, A., Gruszczynski, M., Martill, D., Marshall, J. D., and Błazejowski, B. (2013). Palaeoenvironment and taphonomy of a Late Jurassic (Late Tithonian) Lagerstätte from central Poland. Lethaia 46, 71–81. doi: 10.1111/j.1502-3931.2012.00322.x
Klompmaker, A. A. (2019). Marine arthropods from the Middle Triassic of Winterswijk. Staringia 16, 191–194.
Kobayashi, T. (1933). On the occurrence of Xiphosuran remains in Chosen (Korea). Jpn J. Geol. Geogr. 10, 175–182.
Koenig, C. D. E. (1825). Icones Fossilium Sectiles: Centuria Prima. London: GB Sowerby. doi: 10.5962/bhl.title.60262
Krause, T., Hauschke, N., and Wilde, V. (2009). Ein Limulide aus den Gelben Basisschichten des Oberen Muschelkalks von Ohrdruf bei Gotha (Thüringen). Geowissensch. Mitteilungen Thüringen 13, 163–168.
Krawczynski, W., Filipiak, P., and Gwozdziewicz, M. (1997). Zespół skamieniałości z karbonskich sferosyderytów (westfal A) NE cześci górnoślaskiego zagłebia weglowego. Przegl. Geol. 45, 1271–1274.
Križnar, M., and Hitij, T. (2010). Nevretenčarji (invertebrates) Strelovške formacije. Scopolia Supplement, Vol. 5, 91–107.
Krzeminski, W., Krzeminska, E., and Wojciechowski, D. (2010). Silurian synziphosurine horseshoe crab Pasternakevia revisited. Acta Palaeontol. Pol. 55, 133–139. doi: 10.4202/app.2008.0074
Kustatscher, E., Franz, M., Heunisch, C., Reich, M., and Wappler, T. (2014). Floodplain habitats of braided river systems: depositional environment, flora and fauna of the Solling Formation (Buntsandstein, Lower Triassic) from Bremke and Fürstenberg (Germany). Palaeobiol. Palaeoenviron. 94, 237–270. doi: 10.1007/s12549-014-0161-0
Kwan, B. K. Y., Hsieh, H.-L., Cheung, S. G., and Shin, P. K. S. (2016). Present population and habitat status of potentially threatened Asian horseshoe crabs Tachypleus tridentatus and Carcinoscorpius rotundicauda in Hong Kong: a proposal for marine protected areas. Biodivers. Conserv. 25, 673–692. doi: 10.1007/s10531-016-1084-z
Lamsdell, J. C. (2013). Revised systematics of Palaeozoic ‘horseshoe crabs’ and the myth of monophyletic Xiphosura. Zool. J. Linnean Soc. 167, 1–27. doi: 10.1111/j.1096-3642.2012.00874.x
Lamsdell, J. C. (2016). Horseshoe crab phylogeny and independent colonizations of fresh water: ecological invasion as a driver for morphological innovation. Palaeontology 59, 181–194. doi: 10.1111/pala.12220
Lamsdell, J. C., and Mckenzie, S. C. (2015). Tachypleus syriacus (Woodward)—a sexually dimorphic Cretaceous crown limulid reveals underestimated horseshoe crab divergence times. Org. Divers. Evol. 15, 681–693. doi: 10.1007/s13127-015-0229-3
Lamsdell, J. C., Tashman, J. N., Pasini, G., and Garassino, A. (2020). A new limulid (Chelicerata, Xiphosurida) from the late Cretaceous (Cenomanian–Turonian) of Gara Sbaa, southeast Morocco. Cretaceous Res. 106:104230. doi: 10.1016/j.cretres.2019.104230
Lamsdell, J. C., Xue, J., and Selden, P. A. (2013). A horseshoe crab (Arthropoda: Chelicerata: Xiphosura) from the lower Devonian (Lochkovian) of Yunnan, China. Geol. Mag. 150, 367–370. doi: 10.1017/S0016756812000891
Lange, W. (1922). Über neue Fssilfunde aus der Trias von Göttingen. Z. Dtsch. Geol. Ges 74, 162–168.
Laurie, K., Chen, C., Cheung, S., Do, V., Hsieh, H., John, A., et al. (2019). Tachypleus tridentatus (Errata Version Published in 2019). The IUCN Red List of Threatened Species 2019, T21309A149768986.
Laurie, M. (1899). On a Silurian scorpion and some additional eurypterid remains from the Pentland Hills. Trans. R. Soc. Edinburgh 39, 575–590. doi: 10.1017/S0080456800035109
Lee, C. N., and Morton, B. (2005). Experimentally derived estimates of growth by juvenile Tachypleus tridentatus and Carcinoscorpius rotundicauda (Xiphosura) from nursery beaches in Hong Kong. J. Exp. Mar. Biol. Ecol. 318, 39–49. doi: 10.1016/j.jembe.2004.12.010
Lefebvre, B., El Hariri, K., Lerosey-Aubril, R., Servais, T., and van Roy, P. (2016). The Fezouata Shale (Lower Ordovician, Anti-Atlas, Morocco): a historical review. Palaeogeogr. Palaeoclimatol. Palaeoecol. 460, 7–23. doi: 10.1016/j.palaeo.2015.10.048
Legg, D. A., Sutton, M. D., and Edgecombe, G. D. (2013). Arthropod fossil data increase congruence of morphological and molecular phylogenies. Nat. Commun. 4:2485. doi: 10.1038/ncomms3485
Lerner, A. J., Lucas, S. G., and Lockley, M. (2017). First fossil horseshoe crab (Xiphosurida) from the Triassic of North America. Neues Jahrb. Geol. Palaontol. Abh. 286, 289–302. doi: 10.1127/njgpa/2017/0702
Lerner, A. J., Lucas, S. G., and Mansky, C. F. (2016). The earliest paleolimulid and its attributed ichnofossils from the lower Mississippian (Tournaisian) Horton Bluff Formation of Blue Beach, Nova Scotia, Canada. Neues Jahrb. Geol. P-A 280, 193–214. doi: 10.1127/njgpa/2016/0575
Lomax, D. R., Robinson, P., Cleal, C. J., Bowden, A., and Larkin, N. R. (2016). Exceptional preservation of Upper Carboniferous (lower Westphalian) fossils from Edlington, Doncaster, South Yorkshire, UK. Geol. J. 51, 42–50. doi: 10.1002/gj.2602
Lucas, S. G., Lerner, A. J., Dimichele, W. A., Cantrell, A. K., Suazo, T. L., and Chaney, D. S. (2014). “Xiphosurid fossils from the Pennsylvanian Beeman Formation, Otero County, New Mexico,” in New Mexico Geological Society Guide Book, 64th Field Conference, Geology of the Sacramento Mountains Region, Vol. 64, 311–314.
Malz, H. (1964). Kouphichnium walchi, die Geschichte einer Fährte und ihres Tieres. Nat. Mus. 94, 81–97.
Malz, H., and Poschmann, M. (1993). Erste Süßwasser-Limuliden (Arthropoda, Chelicerata) aus dem Rotliegenden der Saar-Nahe-Senke. Osnabrücker Naturwissenschafliche Mitteilungen 19, 21–24.
Martí, J. C. (1993). El Pinetell, un nou jaciment paleontològic de la “Pedra d'Alcover” a la conca de Barberá. Reboll: Butll. Inst. Catalana Hist. Nat. Conca Barberà 6, 33–42.
Martí, J. C. (1994). Noves aportacions paleontològiques al muschelkalk superior de les muntanyes de prades: el cas del Pinetell. Quaderns Vilaniu 25, 67–93.
Martin, E. L. O., Pittet, B., Gutiérrez-Marco, J.-C., Vannier, J., El Hariri, K., Lerosey-Aubril, R., et al. (2016). The Lower Ordovician Fezouata Konservat-Lagerstätte from Morocco: age, environment and evolutionary perspectives. Gondwana Res. 34, 274–283. doi: 10.1016/j.gr.2015.03.009
Martin, W. (1809). Petrificata Derbiensia: Or, Figures and Descriptions of Petrifactions Collected in Derbyshire. Wigan: Kessinger Publishing.
Meek, F. B., and Worthen, A. H. (1865). Notice of some new types of organic remains, from the Coal Measures of Illinois. Proc. Acad. Natl. Sci. U.S.A. 17, 41–48.
Meischner, K.-D. (1962). Neue Funde von Psammolimulus gottingensis (Merostomata, Xiphosura) aus dem Mittleren Buntsandstein von Göttingen. Paläontol. Z. 36, 185–193. doi: 10.1007/BF02987900
Moore, R. A., Briggs, D. E. G., and Bartels, C. (2005a). A new specimen of Weinbergina opitzi (Chelicerata: Xiphosura) from the lower Devonian Hunsrück Slate, Germany. Paläontol. Z. 79, 399–408. doi: 10.1007/BF02991931
Moore, R. A., Briggs, D. E. G., Braddy, S. J., Anderson, L. I., Mikulic, D. G., and Kluessendorf, J. (2005b). A new synziphosurine (Chelicerata: Xiphosura) from the late Llandovery (Silurian) Waukesha Lagerstätte, Wisconsin, USA. J. Paleontol. 79, 242–250. doi: 10.1666/0022-3360(2005)079<0242:ANSCXF>2.0.CO;2
Moore, R. A., Briggs, D. E. G., Braddy, S. J., and Shultz, J. W. (2011). Synziphosurines (Xiphosura: Chelicerata) from the Silurian of Iowa. J. Paleontol. 85, 83–91. doi: 10.1666/10-057.1
Moore, R. A., Mckenzie, S. C., and Lieberman, B. S. (2007). A Carboniferous synziphosurine (Xiphosura) from the Bear Gulch Limestone, Montana, USA. Palaeontology 50, 1013–1019. doi: 10.1111/j.1475-4983.2007.00685.x
Müller, A. H. (1962). Ein weiterer Fund von Pringlia (Merostomata) aus Oberen Karbon Mitteldeutschlands. Ber. Akad. Wiss. Berlin 4, 315–318.
Münster, G. G. (1839). “Die Rhyncholiten des Muschelkalks mit ihrem Fortsätzen,” in Beiträge zur Petrefacten-Kunde, ed G. G. Münster (Bayreuth: In Commission der Buchner'schen Buchhandlung, 48–51.
Murphy, J. L. (1970). Euproops from the “Uffington Shale” of Columbiana County, Ohio. Ann. Carnegie Mus. 41, 281–286.
Naugolnykh, S. V. (2017). Lower Kungurian shallow-water lagoon biota of Middle Cis-Urals, Russia: towards paleoecological reconstruction. Global Geol. 20, 1–13.
Naugolnykh, S. V. (2018). Main biotic and climatic events in early Permian of the Western Urals, Russia, as exemplified by the shallow-water biota of the early Kungurian lagoons. Palaeoworld. doi: 10.1016/j.palwor.2018.10.002
Nelson, B. R., Satyanarayana, B., Zhong, J. M. H., Shaharom, F., Sukumaran, M., and Chatterji, A. (2015). Episodic human activities and seasonal impacts on the Tachypleus gigas (Müller, 1785) population at Tanjung Selangor in Peninsular Malaysia. Estuar. Coast. Shelf. Sci. 164, 313–323. doi: 10.1016/j.ecss.2015.08.003
Nieszkowski, J. (1858). Zusätze zur Monographie der Trilobiten der Ostseeprovinzen: nebst der Beschreibung einiger neuen obersilurischen Crustaceen. Arch. Naturkunde Liv Est Kurland 1, 345–384.
Novitsky, T. J. (2009). “Biomedical applications of Limulus amebocyte lysate,” in Biology and Conservation of Horseshoe Crabs, eds J. T. Tanacredi, M. L. Botton, and D. R. Smith (Dordrecht: Springer), 315–329. doi: 10.1007/978-0-387-89959-6_20
Novojilov, N. J. (1959). Mérostomes du Dévonian inférieur et moyen de Sibérie. Ann. Soc. Géol. Belg. 78, 241–258.
Novozhilov, N. (1991). “Class Merostomata” in Fundamentals of Paleontology. Volume 9: Arthropoda, Tracheata, Chelicerata, eds B. B. Rohdendorf and D. R. Davis (Washington, DC: Smithsonian Institution and National Science Foundation, 591–613.
Ortega Hernández, J., Braddy, S. J., and Rak, Š. (2010). Trilobite and xiphosuran affinities for putative aglaspidid arthropods Caryon and Drabovaspis, Upper Ordovician, Czech Republic. Lethaia 43, 427–431. doi: 10.1111/j.1502-3931.2010.00216.x
Owen, R. (1872). On the anatomy of the American King-crab (Limulus polyphemus, Latr.). Trans. Linnean Soc. London 28, 459–506. doi: 10.1111/j.1096-3642.1873.tb00226.x
Packard, A. S. (1885). On the Carboniferous xiphosurous fauna of North America. Mem. Natl. Acad. Sci. 3, 143–157. doi: 10.5962/bhl.title.14755
Parkes, M. A., and Sleeman, A. G. (1997). Catalogue of the Type, Figured and Cited Fossils in the Geological Survey of Ireland. Dublin: Geological Survey of Ireland Dublin.
Perrier, V., and Charbonnier, S. (2014). The Montceau-les-Mines Lagerstätte (Late Carboniferous, France). C.R. Palevol 13, 353–367. doi: 10.1016/j.crpv.2014.03.002
Pfannenstiel, M. (1928). Eine Jugendform von Limulus Bronni aus dem Plattensandstein Badens. Centralblatt Mineral. Geol. Paläontol. 2, 536–549.
Pickett, J. W. (1984). A new freshwater limuloid from the middle Triassic of New South Wales. Palaeontology 27, 609–621.
Pickett, J. W. (1993). A late Devonian xiphosuran from near Parkes, New South Wales. Mem. Assoc. Austral. Palaeontol. 15, 279–287.
Ponomarenko, A. G. (1985). King crabs and eurypterids from the Permian and Mesozoic of the USSR. Paleontol. J. 19, 100–104.
Poropat, S. F., Martin, S. K., Tosolini, A.-M. P., Wagstaff, B. E., Bean, L. B., Kear, B. P., et al. (2018). Early Cretaceous polar biotas of Victoria, southeastern Australia—An overview of research to date. Alcheringa 42, 157–229. doi: 10.1080/03115518.2018.1453085
Poschmann, M., and Franke, C. (2006). Arthropods and trace fossils from the Lower Devonian (Emsian) of the West Eifel region/Germany and the Grand Duchy of Luxembourg. Ferrantia 46, 97–115.
Prantl, F., and Přibyl, A. (1956). Ostrorepi (Xiphosura) ceskoslovenskeho karbonu. Sborn. Ústredn. Ústavu Geol. 23, 379–424.
Prestwich, J. (1840). On the geology of Coalbrook Dale. Trans. Geol. Soc. Lond. 2, 413–495. doi: 10.1144/transgslb.5.3.413
Přibyl, A. (1967). Moravurus gen. n. eine neue Xiphosurida Gattung aus dem mahrisch-schlesischen Oberkarbon. Cas. Mineral. Geol. 12, 457–460.
Racheboeuf, P. R. (1992). Valloisella lievinensis n. g. n. sp.: nouveau Xiphosure carbonifère du Nord de la France. Neues Jahrb. Geol. Palaontol. Abh. 6, 336–342. doi: 10.1127/njgpm/1992/1992/336
Racheboeuf, P. R., Vannier, J., and Anderson, L. I. (2002). A new three-dimensionally preserved xiphosuran chelicerate from the Montceau-Les-Mines Lagerstätte (Carboniferous, France). Palaeontology 45, 125–147. doi: 10.1111/1475-4983.00230
Raymond, P. E. (1945). Xiphosura in the Langford collection. Coal age fossils from Mazon Creek. State Illinois Sci. Pap. 3, 4–10.
Reeside, J. B., and Harris, D. V. (1952). A Cretaceous horseshoe crab from Colorado. J. Washington Acad. Sci. 42, 174–178.
Richter, R., and Richter, E. (1929). Weinbergina opitzi n. g, n. sp., ein Schwertträger (Merost., Xiphos.) aus dem Devon (Rheinland). Senckenbergiana 11, 193–209.
Riek, E. F. (1955). A new xiphosuran from the Triassic sediments at Brookvale, New South Wales. Rec. Aus. Mus. 23, 281–282. doi: 10.3853/j.0067-1975.23.1955.637
Riek, E. F. (1968). Re-examination of two arthropod species from the Triassic of Brookvale, New South Wales. Rec. Aus. Mus. 27, 313–321. doi: 10.3853/j.0067-1975.27.1968.451
Riek, E. F., and Gill, E. D. (1971). A new xiphosuran genus from Lower Cretaceous freshwater sediments at Koonwarra, Victoria, Australia. Palaeontology 14, 206–210.
Roemer, F. (1883). Über eine Art der limuliden-gattung Belinurus aus dem Steinkohlengebirge Oberschlesiens. Z. Dtsch. Geol. Ges. 35, 429–432.
Röhling, H. G., and Heunisch, C. (2010). Der Buntsandstein. Eine lebensfeindliche Wüste oder doch mehr? BIUZ 40, 268–276. doi: 10.1002/biuz.201010428
Romero, P. A., and Vía Boada, L. (1977). “Tarracolimulus rieke” nuevo Limulido del Triasico de Montral-Alcover (Tarragona). Cuadernos Geol. Iberica 4, 239–246.
Ross, A. J., and Vannier, J. (2002). Crustacea (excluding Ostracoda) and Chelicerata of the Purbeck Limestone Group, southern England: a review. Spec. Pap. Palaeontol. 68, 71–82.
Rudkin, D. M., and Young, G. A. (2009). “Horseshoe crabs–an ancient ancestry revealed,” in Biology and Conservation of Horseshoe Crabs, eds J. T. Tanacredi, M. L. Botton, and D. R. Smith (New York, NY: Springer), 25–44. doi: 10.1007/978-0-387-89959-6_2
Rudkin, D. M., Young, G. A., and Nowlan, G. S. (2008). The oldest horseshoe crab: a new xiphosurid from Late Ordovician Konservat-Lagerstätten, Manitoba, Canada. Palaeontology 51, 1–9. doi: 10.1111/j.1475-4983.2007.00746.x
Ruedemann, R. (1916). Account of some new or little known species of fossils, mostly from the Palaeozoic rocks of New York. N. Y. State Mus. Bull. 189, 7–112.
Rust, J., Bergmann, A., Bartels, C., Schoenemann, B., Sedlmeier, S., and Kühl, G. (2016). The Hunsrück biota: a unique window into the ecology of lower Devonian arthropods. Arthropod Struct. Dev. 45, 140–151. doi: 10.1016/j.asd.2016.01.004
Schimper, W-P. (1853). Palaeontologica alsatica: ou fragments paléontologiques des différents terrains stratifiés qui se rencontrent en Alsace. Mem. Soc. Mus. Hist. Nat. Strasb. 4, 1–10.
Schimpf, L., Isaak, S., Hauschke, N., and Gossel, W. (2017). Computer-generated 3D models and digital storage for use in palaeontological collections, tested for xiphosurans of Eocene age from Saxony-Anhalt, Germany. Hallesches Jahrbuch Geowissensch. 40, 1–16.
Schindler, T., and Poschmann, M. (2012). Das jüngste Vorkommen von Pfeilschwanzkrebsen (Xiphosurida, Euproopidae) im Saar-Nahe-Becken, mit Anmerkungen zur Paläoökologie der Fundschichten (Perm, Südwestdeutschland). Mainzer Geowissensch. Mitteilungen 40, 23–38.
Schram, F. R. (1979). Limulines of the Mississippian Bear Gulch Limestone of Central Montana, USA. Trans. San Diego Soc. Nat. Hist. 19, 67–74.
Schultka, S. (1994). Bellinurus cf. truemanii (Merostomata) aus dem tiefen Oberkarbon (Namur B/C) von Fröndenberg (Nordrhein-Westfalen, Deutschland). Paläontol. Z. 68:339. doi: 10.1007/BF02991347
Schultka, S. (2000). Zur Palökologie der Euproopiden im Nordwestdeutschen Oberkarbon. Mitt. Mus. Nat.kd. Berl. Geowissensch. Reihe 3, 87–98. doi: 10.1002/mmng.4860030105
Seegis, D. (2014). The first fossil limuloid remain from the Stuttgart Formation (Schilfsandstein, Keuper, Karnian, Late Triassic) of Baden-Württemberg, southern Germany. Neu. Jb. Geol. Paläontol. Abh. 274, 229–238. doi: 10.1127/njgpa/2014/0443
Sekiguchi, K., and Shuster, C. N. Jr. (2009). “Limits on the global distribution of horseshoe crabs (Limulacea): lessons learned from two lifetimes of observations: Asia and America,” in Biology and Conservation of Horseshoe Crabs, eds J. T. Tanacredi, M. L. Botton, and D. R. Smith (Dordrecht: Springer), 5–24. doi: 10.1007/978-0-387-89959-6_1
Selden, P., and Nudds, J. (2008). Fossil Ecosystems of North America: A Guide to the Sites and Their Extraordinary Iotas. Boca Raton, FL: CRC Press. doi: 10.1201/b15130
Selden, P. A., and Drygant, D. M. (1987). A new Silurian xiphosuran from Podolia, Ukraine, USSR. Palaeontology 30, 537–542.
Selden, P. A., Lamsdell, J. C., and Qi, L. (2015). An unusual euchelicerate linking horseshoe crabs and eurypterids, from the Lower Devonian (Lochkovian) of Yunnan, China. Zool. Scrip. 44, 645–652. doi: 10.1111/zsc.12124
Selden, P. A., and Siveter, D. J. (1987). The origin of the limuloids. Lethaia 20, 383–392. doi: 10.1111/j.1502-3931.1987.tb00800.x
Shin, P. K. S., Li, H. Y., and Cheung, S. G. (2009). “Horseshoe crabs in Hong Kong: current population status and human exploitation,” in Biology and Conservation of Horseshoe Crabs, eds J. T. Tancredi, M. L. Botton, and D. Smith (Boston, MA: Springer), 347–360. doi: 10.1007/978-0-387-89959-6_22
Shpinev, E. S. (2018). New data on Carboniferous xiphosurans (Xiphosura, Chelicerata) of the Donets Coal Basin. Paleontol. J. 52, 271–283. doi: 10.1134/S0031030118030127
Shpinev, E. S., and Vasilenko, D. (2018). First fossil xiphosuran (Chelicerata, Xiphosura) egg clutch from the Carboniferous of Khakassia. Paleontol. J. 52, 400–404. doi: 10.1134/S0031030118040111
Shultz, J. W. (2001). Gross muscular anatomy of Limulus polyphemus (Xiphosura, Chelicerata) and its bearing on evolution in the Arachnida. J. Arachnol. 29, 283–303. doi: 10.1636/0161-8202(2001)029[0283:GMAOLP]2.0.CO;2
Shuster, C. N. Jr. (1982). A pictorial review of the natural history and ecology of the horseshoe crab Limulus polyphemus, with reference to other Limulidae. Prog. Clin. Biol. Res. 81, 1–52.
Shuster, C. N. Jr. (2001). “Two perspectives: horseshoe crabs during 420 million years, worldwide, and the past 150 years in the Delaware Bay area,” in Limulus in the Limelight, ed J. T. Tanacredi (New York, NY: Springer), 17–40. doi: 10.1007/0-306-47590-1_3
Shuster, C. N. Jr., and Anderson, L. I. (2003). “A history of skeletal structure: clues to relationships among species,” in The American Horseshoe Crab, eds C. N. Shuster Jr., R. B. Barlow, and H. J. Brockmann (Cambridge: Harvard University Press, 154–188.
Shuster, C. N. Jr., Barlow, R. B., and Brockmann, H. J. (2003). The American Horseshoe Crab. Cambridge: Harvard University Press.
Siegfried, P. (1972). Ein Schwertschwanz (Merostomata, Xiphosurida) aus dem Oberkarbon von Ibbenbüren/Westf. Paläontol. Z. 46, 180–185. doi: 10.1007/BF02990151
Siveter, D. J., and Selden, P. A. (1987). A new, giant xiphosurid from the lower Namurian of Weardale, County Durham. Proc. Yorkshire Geol. Soc. 46, 153–168. doi: 10.1144/pygs.46.2.153
Sokoloff, A. (1978). Observations on populations of the horseshoe crab Limulus (= Xiphosura) polyphemus. Res. Popul. Ecol. 19, 222–236. doi: 10.1007/BF02518829
Štamberg, S., and Zajíc, J. (2008). Carboniferous and Permian Faunas and Their Occurence in the Limnic Basins of the Czech Republic. Hradec Králové: Museum of Eastern Bohemia.
Størmer, L. (1934). Downtonian Merostomata from Spitsbergen: with remarks on the suborder Synziphosura. Skrifter utgitt av det Norske Videnskaps-Akademi i Oslo, Matematisk-naturvidenskapelig Klasse 1933, 1–26.
Størmer, L. (1936). Eurypteriden aus dem rheinischen Unterdevon. Abh. Preussischen Geol. Land. Neue Folge 175, 1–74.
Størmer, L. (1955). “Merostomata,” in Treatise on Invertebrate Paleontology, Part P, Arthropoda 2, ed R. C. Moore (Lawrence, KS: University of Kansas; Geological Society of America, 4–41.
Størmer, L. (1972). Arthropods from the Lower Devonian (Lower Emsian) of Alken an der Mosel, Germany. Part 2. Xiphosura. Senck. Leth. 53, 1–29.
Stürmer, W., and Bergström, J. (1981). Weinbergina, a xiphosuran arthropod from the Devonian Hunsrück Slate. Paläontol. Z. 55, 237–255. doi: 10.1007/BF02988142
Sutton, M., Rahman, I., and Garwood, R. (2014). Techniques for Virtual Palaeontology. New York: John Wiley & Sons. doi: 10.1002/9781118591192
Tanacredi, J. T., Botton, M. L., and Smith, D. R. (2009). Biology and Conservation of Horseshoe Crabs. Dordrecht: Springer. doi: 10.1007/978-0-387-89959-6
Tasch, P. (1961). Paleolimnology: part 2: Harvey and Sedgwick Counties, Kansas: stratigraphy and biota. J. Paleontol. 35, 836–865.
Tashman, J. N. (2014). A taxonomic and taphonomic analysis of Late Jurassic horseshoe crabs from a Lagerstätte in central Poland (Masters thesis). Kent, MI: Kent State University.
Tashman, J. N., Feldmann, R. M., and Schweitzer, C. E. (2019). Morphological variation in the Pennsylvanian horseshoe crab Euproops danae (Meek & Worthen, 1865) (Xiphosurida, Euproopidae) from the lower Mercer Shale, Windber, Pennsylvania, USA. J. Crustacean Biol. 39, 396–406. doi: 10.1093/jcbiol/ruz030
Tesakov, A. S., and Alekseev, A. S. (1992). Myriapod-like arthropods from the Lower Devonian of central Kazakhstan. Paleontol. Z. 26, 18–23.
Tiegs, O., and Manton, S. M. (1958). The evolution of the Arthropoda. Biol. Rev. 33, 255–333. doi: 10.1111/j.1469-185X.1958.tb01258.x
Todd, J. A. (1991). A forest-litter animal community from the Upper Carboniferous?: Notes on the association of animal body fossils with plants and lithology in the Westphalian D coal measures at Writhlington, Avon. Proc. Geol. Assoc. 102, 179–184. doi: 10.1016/S0016-7878(08)80215-5
Trechmann, C. T., and Woolacott, D. (1919). On the highest coal-measures or “Zone” of Anthracomya phillipsi in the Durham Coalfield. Geol. Mag. 6, 203–211. doi: 10.1017/S0016756800202689
van Der Hoeven, J. (1838). Recherches Sur L'histoire Naturelle Et L'anatomie Des Limules. Leyden: Luchtmans. doi: 10.5962/bhl.title.120127
Van Roy, P., Briggs, D. E. G., and Gaines, R. R. (2015). The Fezouata fossils of Morocco; an extraordinary record of marine life in the Early Ordovician. J. Geol. Soc. 172, 541–549. doi: 10.1144/jgs2015-017
Van Roy, P., Orr, P. J., Botting, J. P., Muir, L. A., Vinther, J., Lefebvre, B., et al. (2010). Ordovician faunas of Burgess Shale type. Nature 465, 215–218. doi: 10.1038/nature09038
Vandenberghe, A. (1960). Pringlia demaistrei nov. sp., un xiphosure (Chélicérate) du Stéphanien de la Loire. Bull. Soc. Géol. France 7, 687–689. doi: 10.2113/gssgfbull.S7-II.5.690
Vetter, H. (1933). Zum vorkommen von Limulus im mitteldeutschen Braunkohlensandstein. Z. Naturwissensch. 90, 61–75.
Vía Boada, L. (1987a). Artropodos fosiles Triasicos de Alcover-Montral. II. Limulidos. Cuadernos Geol. Ibérica 11, 281–294.
Vía Boada, L. (1987b). Merostoniats fòssils de la península Ibérica. Mem. Real Acad. Cienc. Barcelona 48, 1–79.
Vía Boada, L., and Villalta, J. F. (1966). Hetrolimulus gadeai, nov. gen., nov. sp., représentant d'une nouvelle famille de Limulacés dans le Trias d'Espagne. Com. Rend. Soc.Géol. France 8, 57–59.
Vía Boada, L., Villata, F. J., and Esteban Cerdá, M. (1977). Paleontología y Paleoecolog?a de los yacimientos fosil?feros del Muschelkalk superior entre Alcover y Mont-Ral (Montañas de prades, provincia de Tarragona). J. Iber. Geol. 4, 247–258.
Vogdes, A. W. (1917). Palaeozoic Crustacea: The Publications and Notes on the Genera and Species During the Past Twenty Years, 1895–1917. San Diego, CA: Press of Frye & Smith. doi: 10.5962/bhl.title.10638
von Fritsch, K. W. G. (1906). Beitrag zur Kenntnis der Tierwelt der deutschen Trias. Abh. naturforsch. Ges. Halle, 24, 217–285.
Waterston, C. D. (1985). Chelicerata from the Dinantian of Foulden, Berwickshire, Scotland. Earth Environ. Sci. Trans. R Soc. 76, 25–33. doi: 10.1017/S0263593300010269
Watson, D. M. S. (1909). Limulus woodwardi, sp. nov., from the lower Oolite of England. Geol. Mag. 6, 14–15. doi: 10.1017/S0016756800120333
Wendruff, A. J. (2016). Paleobiology and Taphonomy of Exceptionally Preserved Organisms From the Brandon Bridge Formation (Silurian), Wisconsin, USA (Ph.D thesis). The Ohio State University, Columbus, USA.
Wilmarth, M. G. (1938). Lexicon of Geologic Names of the United States: Part 2, M-Z. Washington, DC: Government Printing Office.
Wincierz, J. (1960). Ein neuer Limulide aus dem Lias. Paläontol. Z. 34, 207–220. doi: 10.1007/BF02986867
Woodward, H. (1865). On a new genus of Eurypterida from the Lower Ludlow rock of Leintwardine, Shropshire. Quart. J. Geol. Soc. 21, 490–492. doi: 10.1144/GSL.JGS.1865.021.01-02.54
Woodward, H. (1866). A Monograph of the British Fossil Crustacea, Belonging to the Order Merostomata: Pterygotus Anglicus, Agassiz. London: Palaeontographical Society. doi: 10.5962/bhl.title.53733
Woodward, H. (1867). On some points in the structure of the Xiphosura, having reference to their relationship with the Eurypteridae. Q. J. Geol. Soc. 23, 28–40. doi: 10.1144/GSL.JGS.1867.023.01-02.11
Woodward, H. (1868). On a new limuloid crustacean [Neolimulus falcatus] from the Upper Silurian of Lesmahagow, Lanarkshire. Geol. Mag. 5, 1–3. doi: 10.1017/S0016756800207139
Woodward, H. (1872). Notes on some British Palæozoic Crustacea belonging to the order Merostomata. Geol. Mag. 9, 433–441. doi: 10.1017/S0016756800465386
Woodward, H. (1879). Contributions to the knowledge of fossil Crustacea. Q J. Geol. Soc. 35, 549–556. doi: 10.1144/GSL.JGS.1879.035.01-04.37
Woodward, H. (1907). Further notes on the Arthropoda of the British coal-measures. Geol. Mag. 4, 539–549. doi: 10.1017/S0016756800134120
Woodward, H. (1918). Fossil arthropods from the Carboniferous rocks of Cape Breton, Nova Scotia; and from the Upper Coal Measures, Sunderland, England. Geol. Mag. 5, 462–471. doi: 10.1017/S0016756800203944
Young, G. A., Rudkin, D. M., Dobrzanski, E. P., Robson, S. P., Cuggy, M. B., Demski, M. W., et al. (2013). Great Canadian Lagerstätten 3. Late Ordovician Konservat-Lagerstätten in Manitoba. Geosci. Can. 39, 201–213.
Zhang, Q. Y., Hu, S. X., Zhou, C. Y., Lü, T., and Bai, J. K. (2009). First occurrence of horseshoe crab (Arthropoda) fossils from China. Prog. Nat. Sci. 19, 1090–1093.
Zhou, H., and Morton, B. (2004). The diets of juvenile horseshoe crabs, Tachypleus tridentatus and Carcinoscorpius rotundicauda (Xiphosura), from nursery beaches proposed for conservation in Hong Kong. J. Nat. Hist. 38, 1915–1925. doi: 10.1080/0022293031000155377
Zincken, C. (1862). Limulus decheni aus dem Braunkohlensandstein bei Teuchern. Zeitschr. Gesammten Naturwissensch. 19, 329–331.
Zittel, K.a.V. (1881). Handbuch der Palaeontologie. I. Abtheilung, Palaeozoologie. München: R. Oldenbourg. doi: 10.5962/bhl.title.61419
Keywords: Xiphosura, Xiphosurida, synziphosurines, horseshoe crab, pictorial atlas, evolution
Citation: Bicknell RDC and Pates S (2020) Pictorial Atlas of Fossil and Extant Horseshoe Crabs, With Focus on Xiphosurida. Front. Earth Sci. 8:98. doi: 10.3389/feart.2020.00098
Received: 22 January 2019; Accepted: 20 March 2020;
Published: 09 July 2020.
Edited by:
Paul Antony Selden, University of Kansas, United StatesReviewed by:
James Lamsdell, West Virginia University, United StatesJason Dunlop, Museum für Naturkunde Leibniz Institut für Evolutions und Biodiversitätsforschung, Germany
Copyright © 2020 Bicknell and Pates. This is an open-access article distributed under the terms of the Creative Commons Attribution License (CC BY). The use, distribution or reproduction in other forums is permitted, provided the original author(s) and the copyright owner(s) are credited and that the original publication in this journal is cited, in accordance with accepted academic practice. No use, distribution or reproduction is permitted which does not comply with these terms.
*Correspondence: Russell D. C. Bicknell, cmRjYmlja25lbGwmI3gwMDA0MDtnbWFpbC5jb20=