- 1Water Science and Environmental Engineering Research Center, College of Chemical and Environmental Engineering, Shenzhen University, Shenzhen, China
- 2Center for Agricultural Water Research in China, China Agricultural University, Beijing, China
- 3State Key Laboratory of Hydroscience and Engineering, Tsinghua University, Beijing, China
- 4School of Water Resources and Electric Power, Qinghai University, Xining, China
China, with its fragile environment and ecosystems, is vulnerable to climate change. Continuous changes in climatic conditions have altered precipitation patterns in most regions of China. Droughts become more frequent and severe in the Xi River basin in South China. It is expected that rapid urbanization and climate change will continue to aggravate water stress in this region. There is an urgent need to develop sustainable water management strategies in face of growing water demand and changing water availability. Projection of future climate change impacts on drought conditions has thus become imperative to support improved decision-making in sustainable water management. In this study, we assessed the risk of extreme droughts under future climate projections in the Xi River basin. The variable infiltration capacity (VIC) model was applied to simulate the hydrological processes of the basin under a multitude of future climate scenarios from CMIP5. Based on the precipitation and runoff series obtained from the VIC model, a comprehensive analysis with respect to the major characteristics of meteorological and hydrological droughts had been carried out. This study is of practical and theoretical importance to both policymakers and scholars. First, this study may be a readily available reference work for policymakers when taking consideration of building drought mitigation plans into future water management practices. Second, the findings in this study may provide some valuable insights into the inherent connection between climatic and hydrological changes under a changing climate. Recognition of the connection and interrelation may contribute to the improvement of climatic and hydrological models in practices.
Introduction
Both climate change and global warming have been at the center of arguments for decades, but almost all scientists and scholars now have come to an agreement that the average temperature of our planet has risen by around 1°C since the last century and the rise is expected to continue in the future (IPCC, 2007). Rising global temperatures unfreeze polar ice caps resulting in sea level rise and regional climate change. Extreme weather events become more intense and frequent, e.g., heatwaves, floods, and droughts. As regional climate patterns change, their impacts from one location to another can be erratic (Zhou and Zhang, 2014; Wanders and Wada, 2015). While regional climate change has increased precipitation in some areas, it has also led to droughts in other regions. As a result, both short-term and long-term regional impact studies under climate change are needed to improve policymakers’ understanding of the changing climate and environment, and to highlight the urgency and necessity of developing regional climate mitigation and adaptation plans.
China, with its fragile environment and ecosystems, is vulnerable to the negative effects of climate change (Xu et al., 2015). In recent years, China has experienced more frequent extreme weather events, e.g., heavy rains and droughts (Wang and Li, 2005; Chen and Sun, 2015; Tan et al., 2016). In fact, continuous changes in climatic conditions have altered precipitation patterns in most regions of China, especially, in the southern portions of China. Droughts become more frequent and severe in many regions of southern China in recent decades (Huang et al., 2010; Li et al., 2012). It is expected that rapid urbanization and climate change will continue to aggravate water stress in southern China. There is an urgent need to develop sustainable water management strategies in face of growing water demand and changing water availability. Therefore, projection of future climate change impacts on drought conditions has become imperative to support improved decision-making in sustainable water management.
The Xi River is the longest river in South China, with a total drainage area of 353,000 km2, accounting for 78% of the total area of the Pearl River basin. The Xi River basin stretches from eastern Yunnan province into southern Guangdong province, and has a humid subtropical or tropical monsoon climate. In terms of mean annual runoff, the Xi River ranks 2nd behind the Yangtze River in China, and is almost 4.5 times that of the Rhine River in Europe. The mean annual precipitation in Xi River basin is approximately 1,447 mm (Niu and Chen, 2010). The Xi River basin is resource-rich, with fertile agricultural soils, abundant mineral resources, water and energy intensive industries, and supports fifty million people in South China. It has been positioned as a new national economic growth zone thanks to the economic development initiative of Xi River Economic Belt.
Although Xi River basin has abundant freshwater resources, there are many regions that are subject to periodic water scarcity due to maldistribution of water resources (Cui et al., 2007). About 80% of the annual precipitation falls during the wet season between April and September, while only 8% of that falls during the winter season between December and February (Duan et al., 2017). As a result of maldistribution of water resources, the Xi River basin has experienced recurring droughts and water stress (Fischer et al., 2013). An intense period of droughts occurred from 1962 to 1963 and about 5% of the basin land was affected, with an area of 16,200 km2 (Niu et al., 2015). A continuous period of droughts lasting 9 years occurred from 1984 to 1992 (Lin et al., 2017). A frequent period of droughts occurred from 2003 to 2015 and the basin had seen widespread droughts almost every year during this period (Wu et al., 2016). In addition, the Xi River basin has encountered great economic losses because of severe droughts in recent years (Xiao et al., 2012; Chen et al., 2013; Niu et al., 2015). Increasingly frequent droughts have caused significant socioeconomic impacts and necessitate urgent actions from government and stakeholders. Improving understanding of historical variations in precipitation and future trends in climate are needed so as to aid policymakers in building drought mitigation plans into local water management practices.
The Xi River basin has long been of interest to scientist and scholars because of its social, economic and cultural importance. Most studies across the Xi River basin were focused on the assessment of the hydrological changes through trend analysis, and only a few studies aimed at investigating climate change impacts on hydrological processes (He et al., 2009; Zhang and Lu, 2009). However, few studies had been carried out with a glimpse into the future. Moreover, with respect to future hydrological changes, most studies were focused on using General Circulation Models (GCMs) and hydrological models to analyze the long-term mean values of variations in hydrological conditions (Yuan et al., 2017). There were few studies focusing on the connection between climate change and hydrometeorological extremes at a regional scale, despite the fact that large river basins with so-called abundant freshwater resources and dense population may be particularly vulnerable to extreme weather incidents (Teng et al., 2012; Li et al., 2016).
To address the aforementioned issues, the objectives of this study are: (1) to project future streamflow in the Xi River basin and evaluate future drought conditions including meteorological and hydrological droughts by using two types of drought indexes, i.e., the Standardized Precipitation Index (SPI) and the Standardized Runoff Index (SRI); (2) to investigate climate change impacts on the variations in the mean and extreme values of drought characteristics by using three parameters, i.e., frequency, duration and severity; and (3) to reveal the inherent connection between climatic and hydrological changes under a changing climate.
The remainder of this paper is organized as follows. Section “Materials and Methods” describes the data and methods. Section “Results” presents the results of the relative changes of river flows and drought characteristics, as well as a relationship analysis among precipitation, temperature and runoff. Section “Discussion” gives a discussion, while the final section contains conclusions.
Materials and Methods
Study Area
The Xi River basin is located in South China between 21–27.00°N latitude and 102.00–114.00°E longitude. The river rises in Yunnan province, flows through Guizhou, Guangxi and Guangdong provinces, and finally reaches the Pearl River Delta on the South China Sea. The total length of the river is about 2,214 km, including several main tributaries, e.g., Nanpan River, Hongshui River, Yu River, Liu River, etc. The location of the Xi River basin is shown in Figure 1.
Climate Change Scenarios
In this study, we simulated the terrestrial hydrological processes over the Xi River basin with CMIP5 multi model ensembles (MMEs). The downscaled GCMs were obtained from “Downscaled CMIP3 and CMIP5 Climate and Hydrology Projections”1. Then, we chose 16 GCMs based on selection criteria including data integrity of long-time series, usage frequency of numerical modeling, and variability of the downscaled data. The Bias Correction Spatial Disaggregation (BCSD) climatic data at a 0.5° × 0.5° spatial resolution and a monthly time step under the RCP4.5 scenario from 1960 to 2099 were used in this study. The summary of the selected GCMs is provided in Table 1.
To obtain local meteorological forcing data from the GCMs, which are the prerequisites of simulation of the Variable Infiltration Capacity (VIC) model, a stochastic weather generation method was applied to temporally disaggregate the monthly climatic data, including precipitation and temperature, into the daily meteorological forcing datasets (Wood et al., 2004). In addition, we chose RCP4.5 to study the potential impacts of future climate change. The reason is that a variety of mitigation actions have been taken in China to reduce greenhouse gas emissions; thus RCP4.5 may be an appropriate emissions scenario that can better reflect the future climate conditions in the Xi River basin (Riahi and Nakicenovic, 2007; Thomson et al., 2011; Gao et al., 2014). Therefore, the results obtained from this study may be more meaningful in terms of supporting decision-making in building future drought mitigation plans into local water management practices.
Macro-Scale Hydrological Model
The future terrestrial hydrological scenarios in the Xi River basin were derived from simulation of the VIC model. The historical meteorological forcing data required by the VIC model, such as temperature and wind speed, were obtained from the work of Feng et al. (2004). The soil and vegetation datasets required for the VIC model were obtained from the work of Nijssen et al. (2001). Previous studies demonstrated that the VIC model was capable of producing acceptable results for the purpose of runoff simulation in the Xi River basin by using the aforementioned meteorological parameters (Niu and Chen, 2010). Next, by using the BCSD climatic data as the input of the VIC model, the daily time series of the major hydrological parameters for the period 1960–2099 were obtained.
Drought Indices
The SPI and the SRI were used to analyze the meteorological and hydrological droughts, respectively. The SPI is calculated based on accumulated precipitation, which describes the degree of deviation of accumulated precipitation from climatological averages. The SPI is applicable at either monthly or multi-monthly temporal scales (e.g., 3-month) over different spatial scales so that it has been widely used to investigate the evolution of meteorological droughts (McKee, 1997). The procedure for calculating the SPI is outlined below. Firstly, an appropriate probability distribution is chosen to fit the accumulated precipitation. Then, the cumulative probability is calculated based on the accumulated precipitation according to the selected distribution. Finally, the SPI, i.e., the standard normal deviation with mean zero and standard deviation one, can be obtained from the cumulative probability. In this study, the log-normal distribution was selected to fit the accumulated precipitation over the Xi River basin (Niu et al., 2015). Thus, the SPI can be expressed as:
where x is accumulated precipitation, y = ln(x); is sample variance; is sample mean. More information about calculation of the SPI can be found in Lloyd-Hughes and Saunders (2002). The procedure for calculating the SRI is similar to that of the SPI except a key point that the SRI is calculated based on accumulated runoff. The time series of the two drought indexes were calculated by using the monthly series for the whole-basin area as well as the grid cells in the Xi River basin for every GCM. The selected threshold for drought identification is zero, and thus a drought event is defined as a consecutive and uninterrupted period, with an SPI/SRI value lower than this level (Vicente-Serrano et al., 2017). Based on this definition, three different parameters can be obtained to characterize droughts: (1) frequency—the number of drought events in a given period; (2) duration—the length of an identified drought event; (3) severity—the accumulated deficit volume of an identified drought event.
Results
The projected results show that the Xi River basin would experience a temperature increase of about 1.3–1.7°C in the near future (2030–2059) and a temperature increase of about 1.9–2.4°C in the distant future (2070–2099), in comparison with the historical period (1971–2000). As shown in Figure 2, the largest increase would occur in the northern part of the Xi River basin, whereas the smallest increase would occur in southeastern part in the near future. Unlike the moderate temperature increase in the near-future scenario, the northern part of the Xi River basin would experience an obvious increase in temperature up to 2.4°C in the distant future. These results indicate that temperature would increase continuously across the Xi River basin in the future regardless of spatial and temporal considerations. With respect to the changes in the precipitation pattern, the results also show an upward trend in annual precipitation, increasing up to 4.5% in the near future (see Figure 2C) and up to 7% in the distant future over the basin (see Figure 2D). Overall, it is expected that both average temperature and precipitation in the Xi River basin would increase in the future.
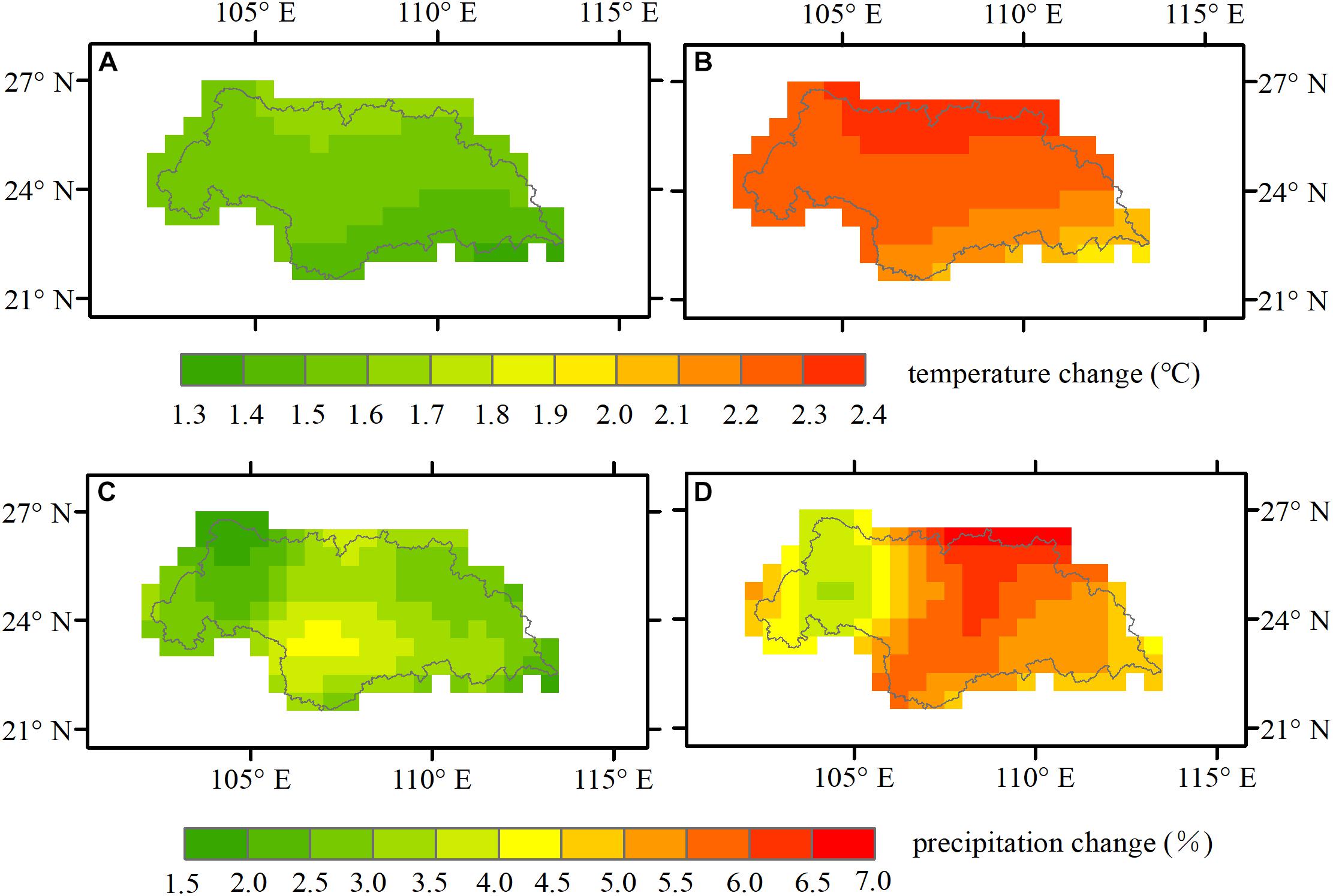
Figure 2. Changes in temperature (°C) between the future periods and the historical period 1971–2000: (A) 2030–2059 and (B) 2070–2099, and changes in precipitation (%) between the future periods and the historical period 1971–2000: (C) 2030–2059 and (D) 2079–2099.
Spatial Changes in River Flows
The relative changes in extreme river flow and average river flow between the future periods (i.e., 2030–2059 and 2070–2099) and the historical period (i.e., 1971–2000) are shown in Figure 3. The 95th percentile (Q95) and the 10th percentile (Q10) of the monthly flow were used to describe the extreme conditions (i.e., the low flow and the high flow) in this study. The results from MMEs shown in Figure 3 indicate that climate change would have a significant impact on the hydrological patterns in the Xi River basin. For the near future (2030–2059), the low flow would probably decrease across the Xi River basin except several areas in the midstream region. In addition, the Xi River basin would probably see a more significant reduction in the low flow in the near future rather than in the distant future (2070–2099). For example, in the central basin, some grids show a significant decrease in the low flow by about 40–60%, while such a decrease climbs to around 20–40% in the distant future. A modest decrease in the mean flow is found across most areas of the Xi River basin. In the near future, the mean flow would probably reduce between 4 and 49%. There are only a few grids in the midstream showing an increase ranging from 2 to 13%. Similar implications could be obtained from observing the mean flow in the distant future, but the degree of reduction with respect to the mean flow would be alleviated in some regions of the central basin. The high flow would decrease in the midstream region under RCP4.5 in the near future. The changes in the high flow in the distant future would be very similar to that case, but the magnitude of reduction could be different. In addition, more grids show an increase in the high flow in the midstream and downstream regions during 2070–2099.
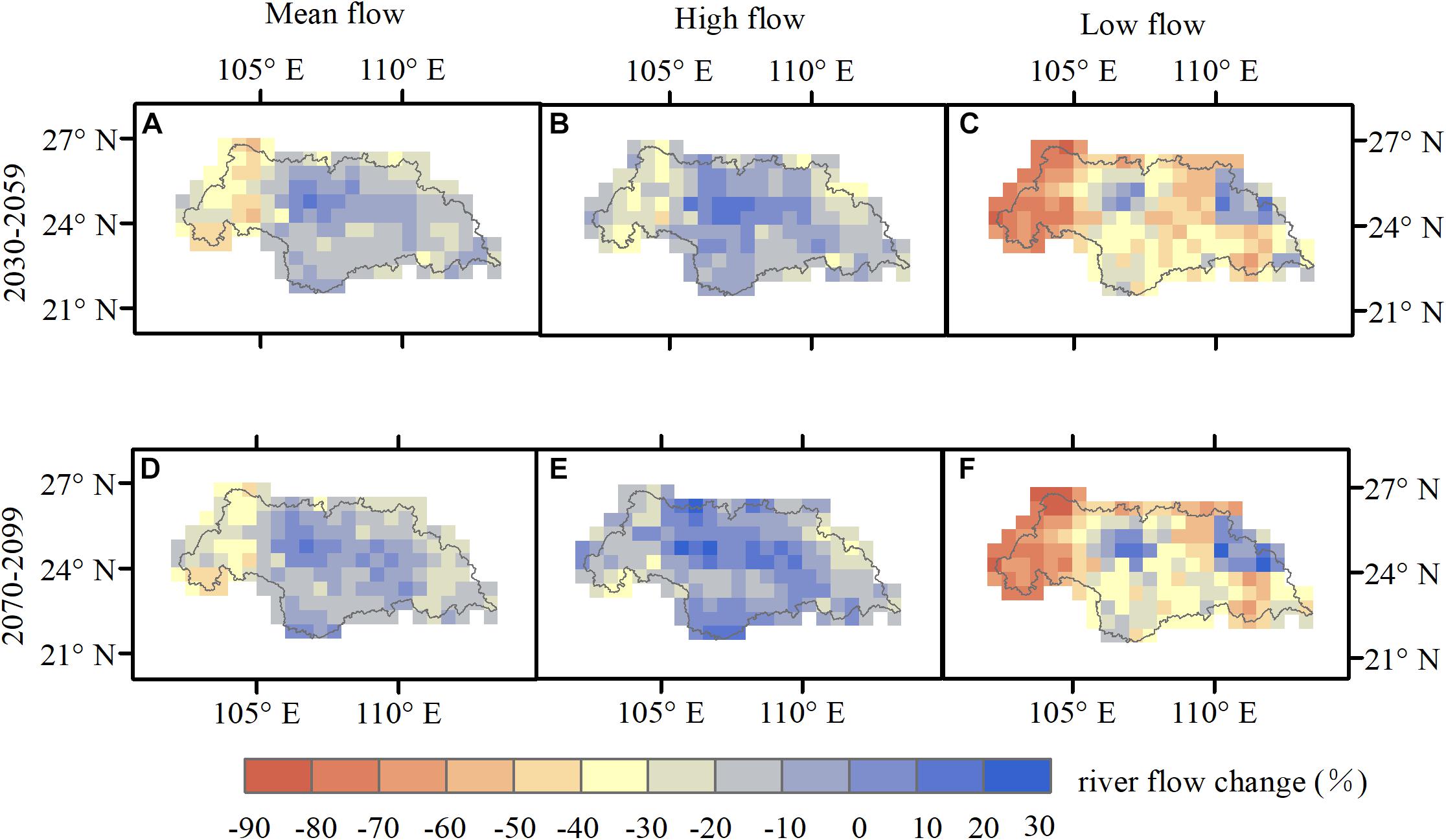
Figure 3. Changes in river flows between the future period 2030–2059 and the historical period 1971–2000: (A) mean flow, (B) high flow (Q95), and (C) low flow (Q10), and changes in river flows between the future period 2070–2099 and the historical period 1971–2000: (D) mean flow, (E) high flow (Q95), and (F) low flow (Q10) over the Xi River basin.
Spatial Changes in Drought Characteristics
Mean Droughts
Figure 4 illustrates the relative changes projected by MMEs with respect to mean drought characteristics, i.e., frequency, duration and severity, between two future periods (2030–2059 and 2070–2099) and the historical period (1971–2000). Meteorological droughts described by the SPI would decrease in major areas of the Xi River basin, except the southeastern and northern parts of the Xi River basin. Meanwhile, the mean duration and severity of meteorological droughts would probably increase across most areas of the Xi River basin. However, the northern and eastern Xi River basin would experience a decrease in the mean duration. Our results indicate that global warming would result in less meteorological drought events but longer dry episodes across the Xi River basin. With respect to hydrological droughts described by the SRI, the drought frequency would increase over major parts of the Xi River basin. However, the northern and southeastern Xi River basin would experience a decrease in the mean duration and severity of hydrological droughts. Meanwhile, the increase in the mean duration and severity described by the SRI in the near future would be less than that of the SPI in the northern and southeastern Xi River basin. The reason could be attributed to the buffer processes of the land surface, leading to smaller changes in the mean duration of hydrological droughts in contrast to meteorological droughts.
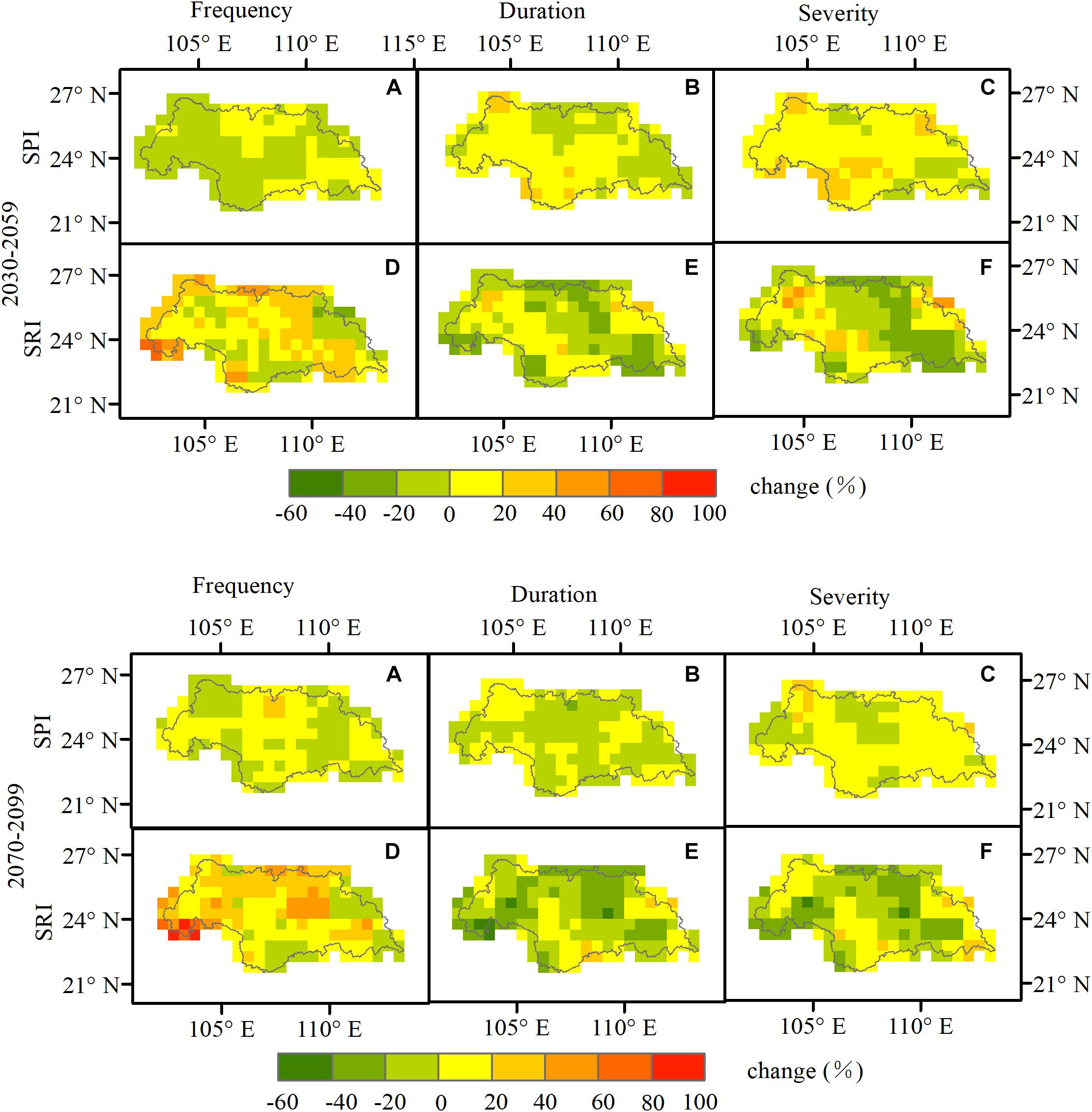
Figure 4. Changes in the characteristics of meteorological droughts for the SPI between the future periods (2030–2059 and 2070–2099) and the historical period (1971–2000): (A) frequency, (B) duration, (C) severity, and changes in the characteristics of hydrological droughts for the SRI between the future periods (2030–2059 and 2070–2099) and the historical period (1971–2000): (D) frequency, (E) duration, and (F) severity.
The relative changes in frequency, duration and severity for the distant future, i.e., 2070–2099, is shown in the bottom panel of Figure 4. More grids of the Xi River basin would see a significant increase in the frequency of meteorological droughts in the distant future than in the near future. However, the mean duration of meteorological droughts would decrease significantly in most parts of the Xi River basin. The changes in the spatial pattern of hydrological droughts in the distant future would be consistent with the changes described in period 2030–2059. For example, it is expected that the drought frequency would increase in most areas of the Xi River basin, especially in the western areas, while the mean duration and severity would probably decrease in major parts of the Xi River basin.
Extreme Droughts
Figure 5 describes the relative changes in extreme drought conditions between the future and historical periods. The longest duration of meteorological droughts would decrease in most areas of the Xi River basin, while the western and central areas of the basin would experience a notable increase in the longest duration of meteorological droughts. The highest severity of meteorological droughts would probably increase over most areas of the central Xi River basin. The relative changes in extreme hydrological droughts would show more intensive variations compared with that of extreme meteorological droughts. A decrease in the longest duration of hydrological droughts is observed affecting about 60% of the total grid cells across the Xi River basin. In the lower central part of the basin, the highest severity of hydrological droughts would be expected to increase, which is similar to the changes in meteorological droughts. The change patterns in extreme drought conditions show a multitude of changes as compared with that in mean drought conditions (Figure 4). For example, the mean duration of meteorological droughts would tend to increase in the lower central region of the Xi River basin, while the longest duration would tend to decrease in the same region. Moreover, with respect to both meteorological and hydrological droughts in the central Xi River basin, the increment in the longest duration and highest severity would be much greater than that in the mean duration and severity. These results describe worsening extreme drought conditions over the Xi River basin in the near future.
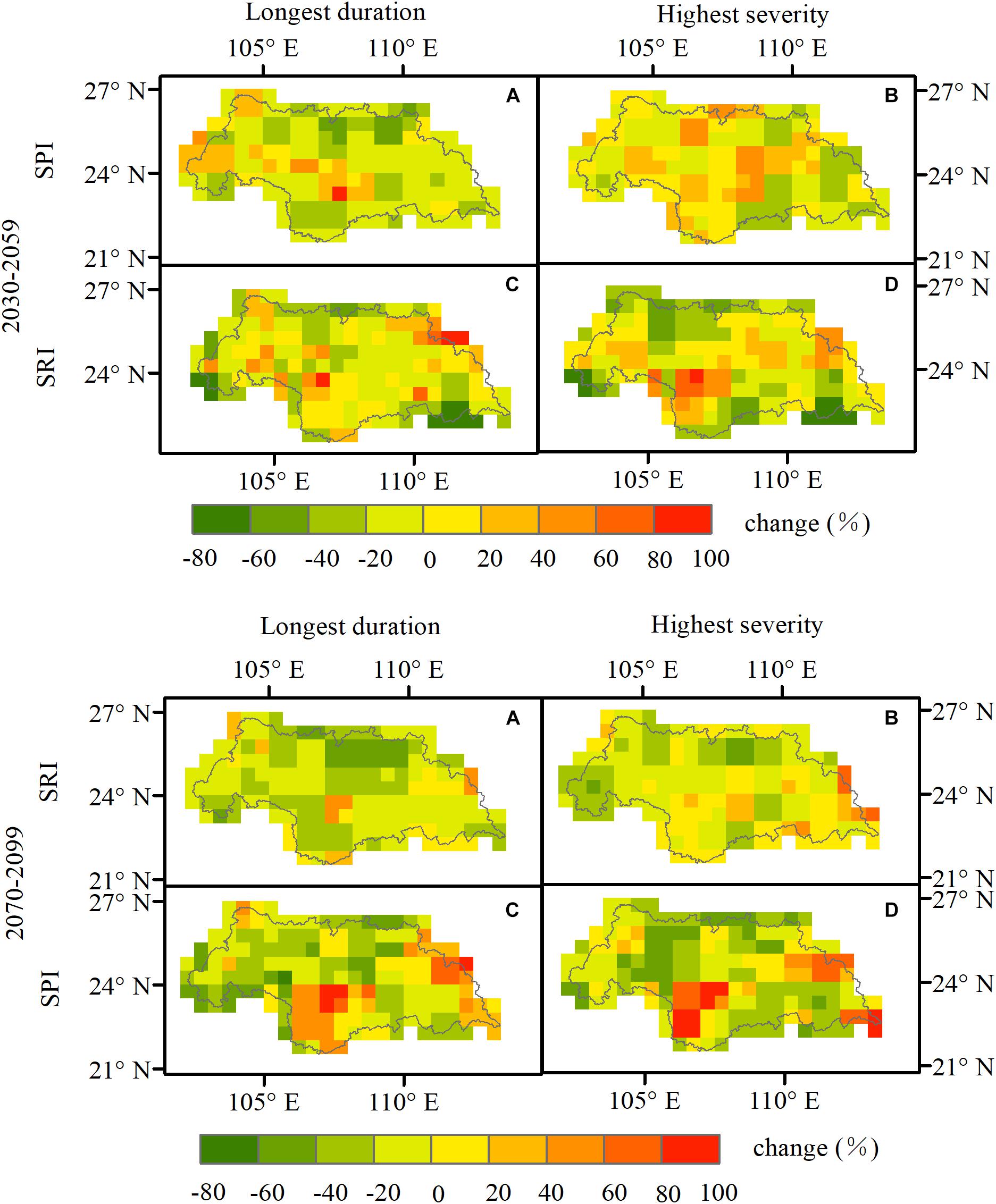
Figure 5. Changes in the characteristics of extreme meteorological droughts for the SPI between the future periods (2030–2059 and 2070–2099) and the historical period (1971–2000): (A) longest duration and (B) highest severity, and changes in the characteristics of extreme hydrological droughts for the SRI between the future periods (2030–2059 and 2070–2099) and the historical period (1971–2000): (C) longest duration, and (D) highest severity.
Similar implications could be reached for the future period 2070–2099. As shown in Figure 5, more areas of the Xi River basin in the distant future would experience a decrease in the longest duration and highest severity of meteorological droughts. For hydrological droughts, the maximum increment in the longest duration would be around 85% in the central basin. Except the southern part of the central basin and the eastern part of the Xi River basin, the longest duration would decrease with similar patterns as the highest severity.
Response of Runoff to Climate Change in the Future
Our study indicates that climate change could affect the timing and magnitude of average and extreme river flows as well as mean and extreme drought conditions in the Xi River basin. According to the comparison results from Figures 2, 3, the changes of streamflow do not always follow the change patterns of precipitation, which indicates that both precipitation and temperature (evapotranspiration) could affect the hydrological processes. Thus, to assess the climate change impacts on streamflow, the annual percentage deviation for streamflow as a function of the annual percentage deviations for precipitation and temperature is described using the contour plot (Figure 6). It is observed that the percentage changes in annual streamflow are positively related to the annual precipitation changes, but negatively related to the annual temperature changes. In addition, streamflow seems to be more sensitive to the changes in precipitation than that in temperature. For example, a 30% increase in annual precipitation could result in a 60% increase in annual streamflow when temperature stays unchanged, whereas a 1.2°C decrease in temperature would lead to a relatively small increase in annual streamflow with unchanged annual precipitation (see Figure 6).
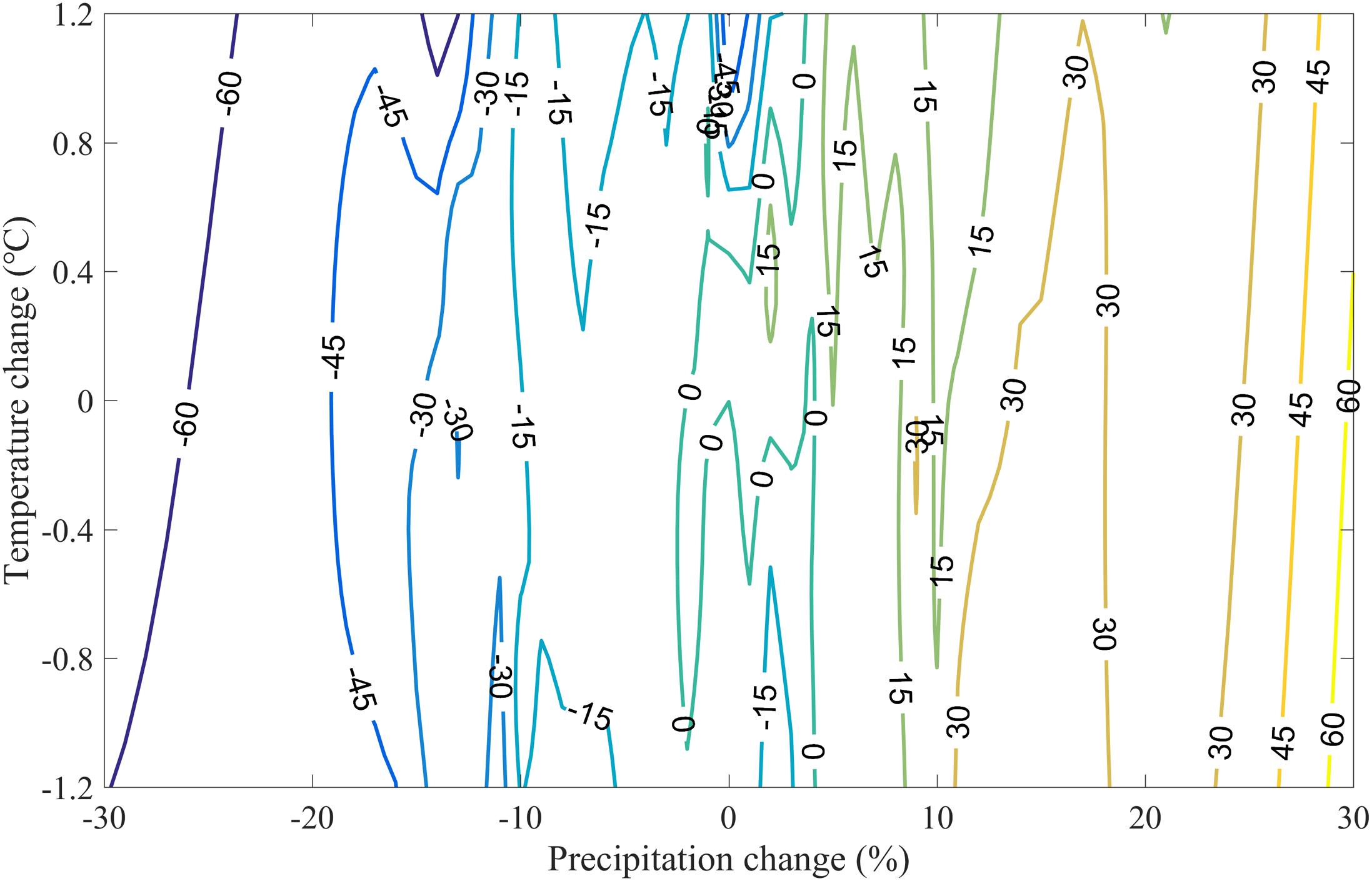
Figure 6. Contour plot of annual streamflow change (%) as a function of annual precipitation and temperature changes for the Xi River basin in the future.
Figure 7 is derived from several cross sections of Figure 6. Streamflow changes subject to temperature changes under different precipitation scenarios across the Xi River basin are shown in Figure 7A. For precipitation-increase scenarios, streamflow and temperature are almost linearly related, with different changing slopes, but this relationship may turn into dissimilar non-linear patterns under the precipitation-decrease scenarios. For example, the slope of the streamflow vs. temperature line is about 3% per °C for the 20% precipitation-increase scenario, indicating that a 1°C increase in temperature would result in a decrease of 3.0% in streamflow. For the 10% precipitation-increase scenario, a 1°C increase in temperature would lead to a 2.5% decrease in streamflow. However, for the precipitation-decrease scenarios, e.g., the 20% precipitation-decrease scenario, limited changes are found in streamflow as temperature changes. Figure 7B shows streamflow changes subject to precipitation changes under different temperature scenarios for the Xi River basin. It is observed that streamflow and precipitation are almost linearly related under a fixed temperature scenario. However, some fluctuations appear, especially in the cases with precipitation changes less than 10%, which might be attributed to numerical instability (Fu et al., 2007). For the scenario with a temperature increase of 1°C, the slope of the streamflow vs. precipitation line is about 2%, indicating that a 10% increase in annual precipitation would result in an increase in annual streamflow by 20%. Moreover, the change slopes for the streamflow vs. precipitation lines under different temperature scenarios are particularly similar. In general, annual streamflow is more sensitive to the changes in precipitation than that in temperature. Moreover, the sensitivity of streamflow with respect to the changes in temperature rises along with increasing precipitation.
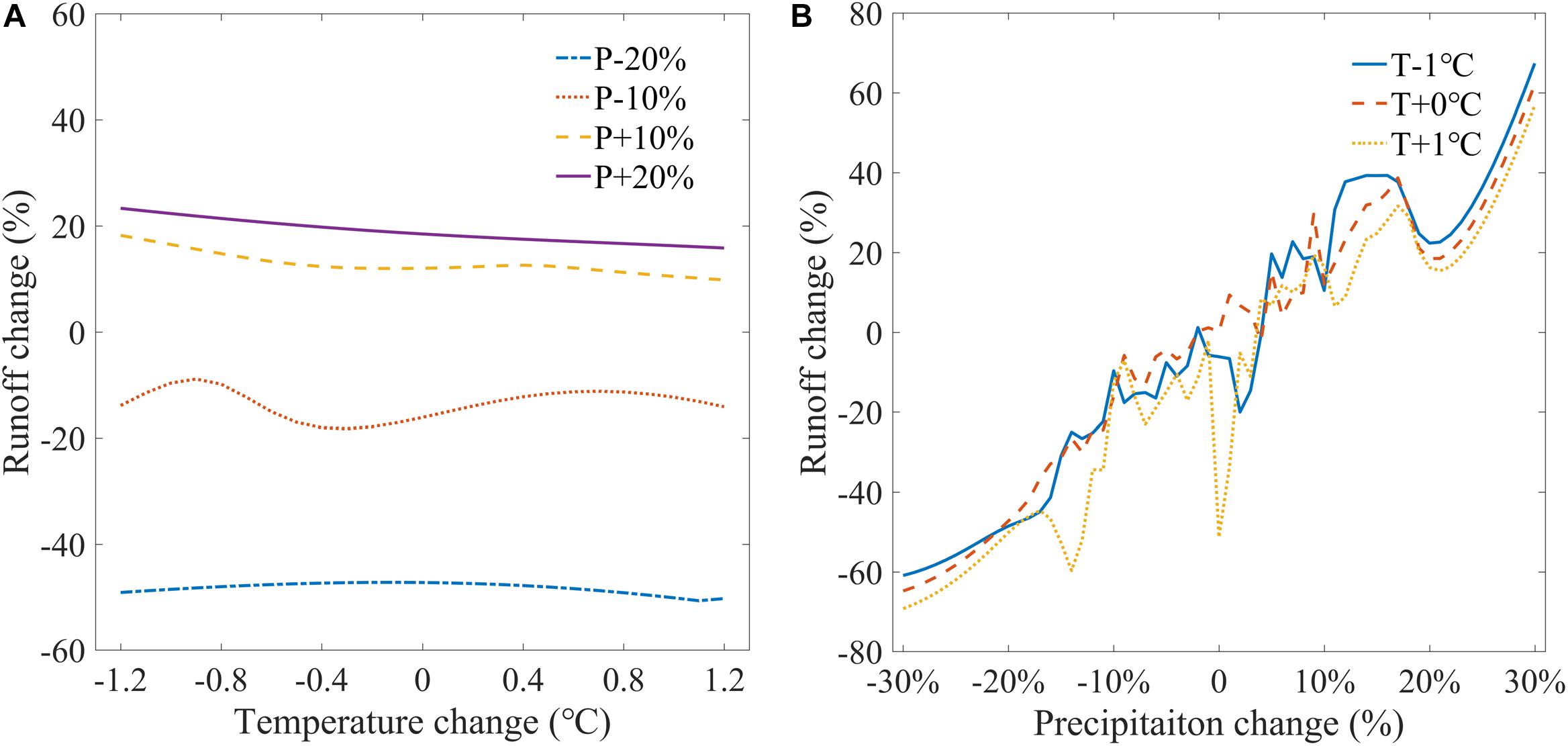
Figure 7. Streamflow changes as function of precipitation changes (A) and temperature changes (B) at different scenarios for the Xi River basin.
Discussion
Uncertainties Arising From the Future Projections
In terms of drought risk analysis under climate change, projection of the variations of terrestrial hydrological processes is the first and most important procedure. However, these processes may be subject to a multitude of uncertainties owing to the selection of emission scenarios, GCMs, downscaling methods as well as impact models. The climate dataset used in this study was obtained from “Downscaled CMIP3 and CMIP5 Climate and Hydrology Projections”—a project meant to provide access to spatial and temporal climate and hydrologic projections for water resource managers and planners based on CMIP3 and CMIP5 (Bureau of Reclamation, 2014). Global and regional studies have demonstrated the superiority of multi-model integration over to a single model (Lambert and Boer, 2001; Ziehmann, 2008; Pierce et al., 2009). The references above also indicate that in terms of selection of the models, with or without a preference for the quality of simulation in study areas, there would be no systematic differences. In this study, the 16 GCMs were statistically downscaled after bias-correlation, making it possible to characterize the potential uncertainties arisen from the GCMs. Moreover, several studies have demonstrated the reliability of the selected GCMs in global and regional climate change studies (Tan et al., 2017; Srinivasa Raju et al., 2017; Sunde et al., 2017; Merabti et al., 2018).
Selection of historical reference data is another potential source of uncertainty. Maurer et al. (2002) conducted a comprehensive verification of the historical reference data used in this study. Moreover, this study is meant to investigate the hydrological response to future climate change. Hence, the analysis had been emphasized on description of the hydrological conditions in the 21st century. Since the climate projections used in this study had been corrected using the BCSD method by other researchers, we do not reassess the reliability of the reference data. In addition, the results in this study are relevant to the specific historical periods. Different researchers used different historical periods as the reference periods for climate change studies, e.g., 1915–2006 (Mantua et al., 2010), 1976–2005 (Prudhomme et al., 2014), 1961–1990 (Kay and Davies, 2008), and 1971–2000 (Dankers et al., 2014; Leng et al., 2015). The near future of 2030–2059 and the distant future period of 2070–2099 were used in our study to provide policymakers with insightful information so as to aid them in building short-term and long-term drought mitigation or adaptation plans into future water management practices.
Due to the inherent characteristics of droughts, e.g., slow onset, as well as the resulting complicated impacts, drought regimes are more ambiguous than that of other water-related incidents (Svoboda et al., 2002). Thus, there is no one universal drought index that can be used to satisfy all needs of researchers; after all, different drought indexes are only suitable for specific drought types. To precisely and visually identify drought occurrence and magnitude, many quantitative definitions of drought indexes have been proposed (Palmer, 1965; Bhalme and Mooley, 1980; McKee et al., 1993). In this study, the SPI and SRI were used to assess the risk of meteorological and hydrological droughts, respectively. It is clear that both the SPI and SRI are only based on monthly data and thus cannot fully reflect actual drought situations. Despite this limitation, both indexes have been widely applied for monitoring specific types of droughts (Hao et al., 2016; Zhang et al., 2017; Merabti et al., 2018).
Comparison Between Our Studies and Previous Studies
Our results show that there exists a substantial increase in precipitation and temperature across the Xi River basin. The high flow is likely to increase in the midstream and downstream region of the basin in the future. The relative increases are larger in the high flow than that in the mean flow, indicating an increase in the risk of floods in the central and southern parts of the basin. Wang and Chen (2014) reported the changes in temperature and precipitation over China under RCP4.5 and RCP8.5 scenarios in the future, and found annual precipitation would increase in most area of China from 2010 to 2099. Sun et al. (2015) concluded that the annual precipitation of the Pearl River basin would increase in the distant future (i.e., after 2030), which is consistent with our findings. Yan et al. (2015) reported that the high flow would increase and the low flow would decrease over the downstream region of the Pearl River basin, and both the high and low flows would decrease in the upstream region of the Pearl River basin under RCP4.5 scenario for 2070–2099. Climate change impacts on droughts were also projected in this study. Our results indicate that the meteorological drought would be more serious in the near future. Zhai et al. (2010) presented the change patterns of dryness/wetness conditions in the Pearl River basin under A1B, A2, and B1 scenarios based on the ECHAM4 model outputs, and reported a finding that future dry periods would occur in the 2020s and 2040s, which is consistent with our findings.
Conclusion
In this study, we assessed extreme drought risk under future climate projections in the Xi River basin. The VIC model was applied to simulate the hydrological processes of the basin under a multitude of future climate scenarios from CMIP5. Based on the precipitation and runoff series obtained from the VIC model, a comprehensive analysis with respect to the major characteristics of meteorological and hydrological droughts, i.e., frequency, duration and severity, had been carried out. The main findings of this study can be summarized as follows:
• A notable declining trend in future flows, including mean flow, high flow and low flows, has been found over the entire basin.
• Significant regional and temporal variations in flow patterns has been found. In the near future, the high flow would be likely to decrease in the midstream region while the low flow would be likely to increase, indicating that this region would face a higher risk of droughts under a changing climate. In addition, most areas of the downstream region would experience an increase in the high flow, indicating a potential risk of floods in this region.
• The changes in drought characteristics imply that meteorological droughts would be more serious and persistent, while hydrological droughts would be more frequent in the near future. In the distant future, both meteorological and hydrological droughts would become more frequent.
• In general, the changes in annual runoff are more sensitive to precipitation than temperature. However, when precipitation increases, temperature may affect runoff generation much more.
Global warming has changed climate patterns in many regions, including the Xi River basin. The changes in regional climatic conditions increase the odds of worsening droughts, and thus place additional pressure on water availability, accessibility, and supply. The Xi River basin’s deteriorating drought conditions have sparked the interest of scientists and researchers and motivated major water policy modifications by providing a glimpse into the basin’s climatic future. In fact, it is difficult to plan for a drought and reduce its impacts when in droughts, and while short-term response plans provide relief support during the event, local authorities can benefit from proactive, longer-term planning that includes risk mitigation strategies. Therefore, projection of future climate change impacts on drought conditions is necessary for improved policy-making in face of future water stress.
This study is of practical and theoretical importance to both policymakers and scholars, despite its limitations in the methodology and model. First, the Xi River basin has been positioned as a new national economic growth zone in South China and this region is facing recurring droughts and worsening water stress in spite of so-called abundant freshwater resources. However, few studies have been carried out for assessing the risk of extreme drought conditions under future climate projections. As a result, this study may be a readily available reference work for policymakers when taking consideration of building drought mitigation plans into future water management practices. Second, the findings in this study may provide some valuable insights into the inherent connection between climatic and hydrological changes under a changing climate. Recognition of the connection and interrelation may contribute to the improvement of climatic and hydrological models in practices.
Data Availability Statement
The datasets generated for this study are available on request to the corresponding author.
Author Contributions
KW, JN, and TL: conceptualization and methodology. KW and JN: data curation and software. KW: formal analysis, validation, and visualization. KW and YZ: writing—original draft and writing—review and editing. YZ: funding acquisition.
Funding
The authors are grateful to the financial support provided by an international cooperation project (No. 2016YFE0201900), National Natural Science Foundation of China (No. 51679233), a science and technology innovation project of Shenzhen (No. 0832-SFCX18SZC025), and the Shenzhen University President’s Fund.
Conflict of Interest
The authors declare that the research was conducted in the absence of any commercial or financial relationships that could be construed as a potential conflict of interest.
Frontiers Media, Ltd. remains neutral with regard to jurisdictional claims in published maps and institutional affiliations.
Footnotes
References
Bhalme, H. N., and Mooley, D. A. (1980). Large-scale droughts/floods and monsoon circulation. Mon. Weather Rev. 108, 1197–1211. doi: 10.1175/1520-0493(1980)108<1197:lsdamc>2.0.co;2
Bureau of Reclamation (2014). “Downscaled CMIP3 and CMIP5 climate and hydrology projections: release of downscaled CMIP5 climate projections,” in Comparison with Preceding Information, and Summary of User Needs US Department of the Interior, Denver, CO.
Chen, H., and Sun, J. (2015). Changes in drought characteristics over china using the standardized precipitation evapotranspiration index. J. Clim. 28:150414125419009.
Chen, Z., Liu, Z., and Huang, Q. (2013). Probability of hydrologic drought duration and intensity of Xijiang River. J. Lake Sci. 25, 576–582. doi: 10.18307/2013.0416
Cui, W., Chen, J., Wu, Y., and Wu, Y. (2007). An overview of water resources management of the Pearl River. Water Supply 7, 101–113. doi: 10.2166/ws.2007.045
Dankers, R., Arnell, N. W., Clark, D. B., Falloon, P. D., Fekete, B. M., Gosling, S. N., et al. (2014). First look at changes in flood hazard in the Inter-Sectoral Impact Model Intercomparison Project ensemble. Proc. Natl. Acad. Sci. U.S. A. 111, 3257–3261. doi: 10.1073/pnas.1302078110
Duan, L., Zheng, J., Li, W., Liu, T., and Luo, Y. (2017). Multivariate properties of extreme precipitation events in the Pearl River basin, China: magnitude, frequency, timing, and related causes. Hydrol. Process. 31, 3662–3671. doi: 10.1002/hyp.11286
Feng, S., Hu, Q., and Qian, W. (2004). Quality control of daily meteorological data in China, 1951-2000: a new dataset. Int. J. Climatol. 24, 853–870. doi: 10.1002/joc.1047
Fischer, T., Gemmer, M., Su, B., and Scholten, T. (2013). Hydrological long-term dry and wet periods in the Xijiang River basin, South China. Hydrol. Earth Syst. Sci. 17, 135–148. doi: 10.5194/hess-17-135-2013
Fu, G., Charles, S. P., and Chiew, F. H. S. (2007). A two-parameter climate elasticity of streamflow index to assess climate change effects on annual streamflow. Water Resour. Res 43:2007
Gao, C., Zhang, Z., Chen, S., and Liu, Q. (2014). The high-resolution simulation of climate change model under RCP4.5 scenarios in the Huaihe River Basin. Geograph. Res. 33, 467–477.
Hao, Z., Hao, F., Singh, V. P., Xia, Y., Ouyang, W., and Shen, X. (2016). A theoretical drought classification method for the multivariate drought index based on distribution properties of standardized drought indices. Adv. Water Resour. 92, 240–247. doi: 10.1016/j.advwatres.2016.04.010
He, H., Lu, H., and Ou, Y. (2009). Flood characteristics of the xijiang river basin in 1959-2008. Adv. Clim. Chang. Res. 5, 14–18.
Huang, W., Yang, X., Li, M., Zhang, X., and Ma, J. (2010). Evolution characteristics of seasonal drought in the south of China during the past 58 years based on standardized precipitation index. Trans. Chinese Soc. Agric. Eng. 26, 50–59.
IPCC (2007). IPCC climate change: the physical science basis. IPCC Clim. Chang. Phys. Sci. Basis 9:996.
Kay, A. L., and Davies, H. N. (2008). Calculating potential evaporation from climate model data: A source of uncertainty for hydrological climate change impacts. J. Hydrol. 358, 221–239. doi: 10.1016/j.jhydrol.2008.06.005
Lambert, S. J., and Boer, G. J. (2001). CMIP1 evaluation and intercomparison of coupled climate models. Clim. Dyn. 17, 83–106. doi: 10.1007/pl00013736
Leng, G. Y., Tang, Q. H., and Rayburg, S. (2015). Climate change impacts on meteorological, agricultural and hydrological droughts in China. Glob. Planet. Chang. 126, 23–34. doi: 10.1016/j.gloplacha.2015.01.003
Li, W., Hou, M., Chen, H., and Chen, X. (2012). Study on drought trend in south china based on standardized precipitation evapotranspiration index. J. Nat. Disasters 21, 84–90. doi: 10.1016/j.scitotenv.2017.10.339
Li, Z., Huang, G., Wang, X., Han, J., and Fan, Y. (2016). Impacts of future climate change on river discharge based on hydrological inference: a case study of the Grand River Watershed in Ontario. Canada. Sci. Total Environ. 54, 198–210. doi: 10.1016/j.scitotenv.2016.01.002
Lin, Q., Wu, Z., Singh, V. P., Sadeghi, S. H. R., He, H., and Lu, G. (2017). Correlation between hydrological drought, climatic factors, reservoir operation, and vegetation cover in the Xijiang Basin. South China. J. Hydrol. 549, 512–524. doi: 10.1016/j.jhydrol.2017.04.020
Lloyd-Hughes, B., and Saunders, M. A. (2002). A drought climatology for Europe. Int. J. Climatol. 22, 1571–1592. doi: 10.1002/joc.846
Mantua, N., Tohver, I., and Hamlet, A. (2010). Climate change impacts on streamflow extremes and summertime stream temperature and their possible consequences for freshwater salmon habitat in Washington State. Clim. Change 102, 187–223. doi: 10.1007/s10584-010-9845-2
Maurer, E., Wood, A., Adam, J., Lettenmaier, D., and Nijssen, B. (2002). A long-term hydrologically-based data set of land surface fluxes and states for the conterminous United States. J. Clim. 15, 3237–3251. doi: 10.1175/1520-0442(2002)015<3237:althbd>2.0.co;2
McKee, D. C. E. B. (1997). Characteristics of 20th century drought in the united states at multiple time scales. Atmos. Sci. Pap. 634, 1–30.
McKee, T., Doesken, N., and Kleist, J. (1993). “The relationship of drought frequency and duration to timescales,” in Paper presented at 8th Conference on Applied Climatology, Anaheim, CA.
Merabti, A., Meddi, M., Martins, D. S., and Pereira, L. S. (2018). Comparing SPI and RDI applied at local scale as influenced by climate. Water Resour. Manag. 32, 1071–1085. doi: 10.1007/s11269-017-1855-1857
Nijssen, B., Schnur, R., and Lettenmaier, D. P. (2001). Global retrospective estimation of soil moisture using the variable infiltration capacity land surface modl, 1980-93. J. Clim. 14, 1790–1808. doi: 10.1175/1520-0442(2001)014<1790:greosm>2.0.co;2
Niu, J., and Chen, J. (2010). Terrestrial hydrological features of the Pearl River basin in South China. J. Hydro Environ. Res. 4, 279–288. doi: 10.1016/j.jher.2010.04.016
Niu, J., Chen, J., and Sun, L. (2015). Exploration of drought evolution using numerical simulations over the Xijiang (West River) basin in South China. J. Hydrol. 526, 68–77. doi: 10.1016/j.jhydrol.2014.11.029
Palmer, W. (1965). Meteorological Drought. Research Paper No.45. Washington, DC: U.S. Department of Commerce.
Pierce, D. W., Barnett, T. P., Santer, B. D., and Gleckler, P. J. (2009). Selecting global climate models for regional climate change studies. Proc. Natl. Acad. Sci. U.S.A. 106, 8441–8446. doi: 10.1073/pnas.0900094106
Prudhomme, C., Giuntoli, I., Robinson, E. L., Clark, D. B., Arnell, N. W., Dankers, R., et al. (2014). Hydrological droughts in the 21st century, hotspots and uncertainties from a global multimodel ensemble experiment. Proc. Natl. Acad. Sci. U.S.A. 111, 3262–3267. doi: 10.1073/pnas.1222473110
Riahi, K., and Nakicenovic, N. (2007). Greenhouse gases-integrated assessment. Technol. Forecast. Soc. Chang. 74, 873–1108.
Srinivasa Raju, K., Sonali, P., and Nagesh Kumar, D. (2017). Ranking of CMIP5-based global climate models for India using compromise programming. Theor. Appl. Climatol. 128, 563–574. doi: 10.1007/s00704-015-1721-1726
Sun, Q., Miao, C., and Duan, Q. (2015). Projected changes in temperature and precipitation in ten river basins over China in 21st century. Int. J. Climatol. 35, 1125–1141. doi: 10.1002/joc.4043
Sunde, M. G., He, H. S., Hubbart, J. A., and Urban, M. A. (2017). Integrating downscaled CMIP5 data with a physically based hydrologic model to estimate potential climate change impacts on streamflow processes in a mixed-use watershed. Hydrol. Process. 31, 1790–1803. doi: 10.1002/hyp.11150
Svoboda, M., LeComte, D., Hayes, M., Heim, R., Gleason, K., Angel, J., et al. (2002). The drought monitor. Bull. Am. Meteorol. Soc. 83, 1181–1190. doi: 10.1175/1520-0477(2002)083<1181:tdm>2.3.co;2
Tan, H., Cai, R., Chen, J., and Huang, R. (2016). Decadal winter drought in Southwest China since the late 1990s and its atmospheric teleconnection: decadal drought in southwest china. Int. J. Climatol 37, 455–467. doi: 10.1002/joc.4718
Tan, M. L., Ibrahim, A. L., Yusop, Z., Chua, V. P., and Chan, N. W. (2017). Climate change impacts under CMIP5 RCP scenarios on water resources of the Kelantan River Basin. Malaysia. Atmos. Res. 189, 1–10. doi: 10.1016/j.atmosres.2017.01.008
Teng, J., Vaze, J., Chiew, F. H. S., Wang, B., and Perraud, J. M. (2012). Estimating the relative uncertainties sourced from GCMs and hydrological models in modeling climate change impact on runoff. J. Hydrometeorol. 13, 122–139. doi: 10.1175/JHM-D-11-058.1
Thomson, A. M., Calvin, K. V., Smith, S. J., Kyle, G. P., Volke, A., Patel, P., et al. (2011). RCP4.5: a pathway for stabilization of radiative forcing by 2100. Clim. Change 109, 77–94. doi: 10.1007/s10584-011-0151-154
Vicente-Serrano, S. M., Zabalza-Martínez, J., Borràs, G., López-Moreno, J. I., Pla, E., Pascual, D., et al. (2017). Extreme hydrological events and the influence of reservoirs in a highly regulated river basin of northeastern Spain. J. Hydrol. Reg. Stud. 12, 13–32. doi: 10.1016/j.ejrh.2017.01.004
Wanders, N., and Wada, Y. (2015). Human and climate impacts on the 21st century hydrological drought. J. Hydrol. 526, 208–220. doi: 10.1016/j.jhydrol.2014.10.047
Wang, L., and Chen, W. (2014). A CMIP5 multimodel projection of future temperature, precipitation, and climatological drought in China. Int. J. Climatol. 34, 2059–2078. doi: 10.1002/joc.3822
Wang, Y., and Li, Z. (2005). Observed trends in extreme precipitation events in China during 1961–2001 and the associated changes in large−scale circulation. Geophys. Res. Lett. 32:2005.
Wood, A. W., Leung, L. R., Sridhar, V., and Lettenmaier, D. P. (2004). Hydrologic implications of dynamical and statistical approaches to downscaling climate model outputs. Clim. Change 62, 189–216. doi: 10.1023/B:CLIM.0000013685.99609.9e
Wu, C., Xian, Z., and Huang, G. (2016). Meteorological drought in the Beijiang River basin, South China: current observations and future projections. Stoch. Environ. Res. Risk Assess. 30, 1821–1834. doi: 10.1007/s00477-015-1157-1157
Xiao, M., Qiang, Z., and Chen, X. (2012). Regionalization and changing properties of drought events along the pearl River Basin. J. Catastrophol. 27, 12–18.
Xu, K., Yang, D., Yang, H., Li, Z., Qin, Y., and Shen, Y. (2015). Spatio-temporal variation of drought in China during 1961-2012: a climatic perspective. J. Hydrol. 526, 253–264. doi: 10.1016/j.jhydrol.2014.09.047
Yan, D., Werners, S. E., Ludwig, F., and Huang, H. Q. (2015). Hydrological response to climate change: the Pearl River, China under different RCP scenarios. J. Hydrol. Reg. Stud. 4, 228–245. doi: 10.1016/j.ejrh.2015.06.006
Yuan, F., Zhao, C., Jiang, Y., Ren, L., Shan, H., Zhang, L., et al. (2017). Evaluation on uncertainty sources in projecting hydrological changes over the Xijiang River basin in South China. J. Hydrol. 554, 434–450. doi: 10.1016/j.jhydrol.2017.08.034
Zhai, J. Q., Liu, B., Hartmann, H., Su, B., Jiang, T., and Fraedrich, K. (2010). Dryness/wetness variations in ten large river basins of China during the first 50 years of the 21st century. Quat. Int. 226, 101–111. doi: 10.1016/j.quaint.2010.01.027
Zhang, S., and Lu, X. X. (2009). Hydrological responses to precipitation variation and diverse human activities in a mountainous tributary of the lower Xijiang. China. Catena 77, 130–142. doi: 10.1016/j.catena.2008.09.001
Zhang, Y., Li, W., Chen, Q., Pu, X., and Xiang, L. (2017). Multi-models for SPI drought forecasting in the north of Haihe River Basin, China. Stoch. Environ. Res. Risk Assess. 31, 2471–2481. doi: 10.1007/s00477-017-1437-1435
Zhou, Y., and Zhang, J. (2014). Application of GIS in downscaling regional climate model results over the province of Ontario. Environ. Syst. Res. 3:8. doi: 10.1186/2193-2697-3-8
Keywords: drought risk analysis, regional climate change, variable infiltration capacity (VIC) model, Xi River basin, hydrological processes
Citation: Wang K, Niu J, Li T and Zhou Y (2020) Facing Water Stress in a Changing Climate: A Case Study of Drought Risk Analysis Under Future Climate Projections in the Xi River Basin, China. Front. Earth Sci. 8:86. doi: 10.3389/feart.2020.00086
Received: 15 January 2020; Accepted: 13 March 2020;
Published: 09 April 2020.
Edited by:
Xander Wang, University of Prince Edward Island, CanadaReviewed by:
Zoe Li, McMaster University, CanadaZoe Courville, Cold Regions Research and Engineering Laboratory, United States
Copyright © 2020 Wang, Niu, Li and Zhou. This is an open-access article distributed under the terms of the Creative Commons Attribution License (CC BY). The use, distribution or reproduction in other forums is permitted, provided the original author(s) and the copyright owner(s) are credited and that the original publication in this journal is cited, in accordance with accepted academic practice. No use, distribution or reproduction is permitted which does not comply with these terms.
*Correspondence: Yang Zhou, yzhou@szu.edu.cn