- Department of Earth Sciences, Natural History Museum of Geneva, Geneva, Switzerland
The Mesozoic sedimentary cover of the Aiguilles Rouges Massif straddling the Swiss-French border has yielded several archosaur footprint sites dated to the Early or Middle Triassic and composed of mostly poorly preserved footprints lacking any orderly arrangement and resting on a megatracksite level. Here we describe two short archosaur trackways attributed to Isochirotherium herculis and located at ca. 2400 m asl in two distinct small valleys separated from each other by a linear distance of 6.4 km. Projection of both trackways onto the same plane showed that they were aligned with a deviation angle of only 3°. These aligned trackways are interpreted as remnants of a straight and narrow walking route taken by a single trackmaker species. It is possible that both trackway segments were made by the same individual. In the present landscape, the Triassic outcrops are small and scattered along a roughly straight NE-SW line. The orientation of the trackways agrees with the general orientation of the outcrops, which is very unlikely to be caused by chance only. We explain this apparent coincidence as resulting from the structural inheritance of a general NE-SW Paleozoic shear zone that controlled the orientation of the Vindelician High on which the archosaurs walked, then that defined the axis of the much later Massif uplift, and eventually affected the general orientation of the erosion that uncovered the trackways.
Introduction
The last phase of the Alpine orogeny in the Western Alps was marked by the uplift of several massifs made of pre-Mesozoic crystalline basement, together with their Early Mesozoic sedimentary covers. The Aiguilles Rouges Massif was uplifted during the late Miocene (e.g., Valla et al., 2012). Its autochthonous Triassic cover, which crops out in several spots above the basement and under the thrust sheet “Nappe de Morcles,” is called the Vieux Emosson Formation. It consists of a basal unit composed of conglomerates and sandstones, and an upper unit composed of sandstones and mudstones, with thin dolomite beds near the top. Facies analyses indicate deposition in a continental fluvial lacustrine system, with the lower unit interpreted as having been deposited in braided streams and the upper unit as mainly representing deposition in a terminal splay – playa environment (Wizevich et al., 2019a). The sandstones have yielded assemblages of archosaur footprints scattered along a 28 km long and circa 100 m wide irregular band of sediments oriented NE-SW (Demathieu and Weidmann, 1982; Avanzini and Cavin, 2009; Cavin et al., 2012; Klein et al., 2016; Wizevich et al., 2019a, b; Figure 1). Most of these sites consist of trampled surfaces forming a single large megatracksite (Meyer and Thüring, 2003; Klein et al., 2016).
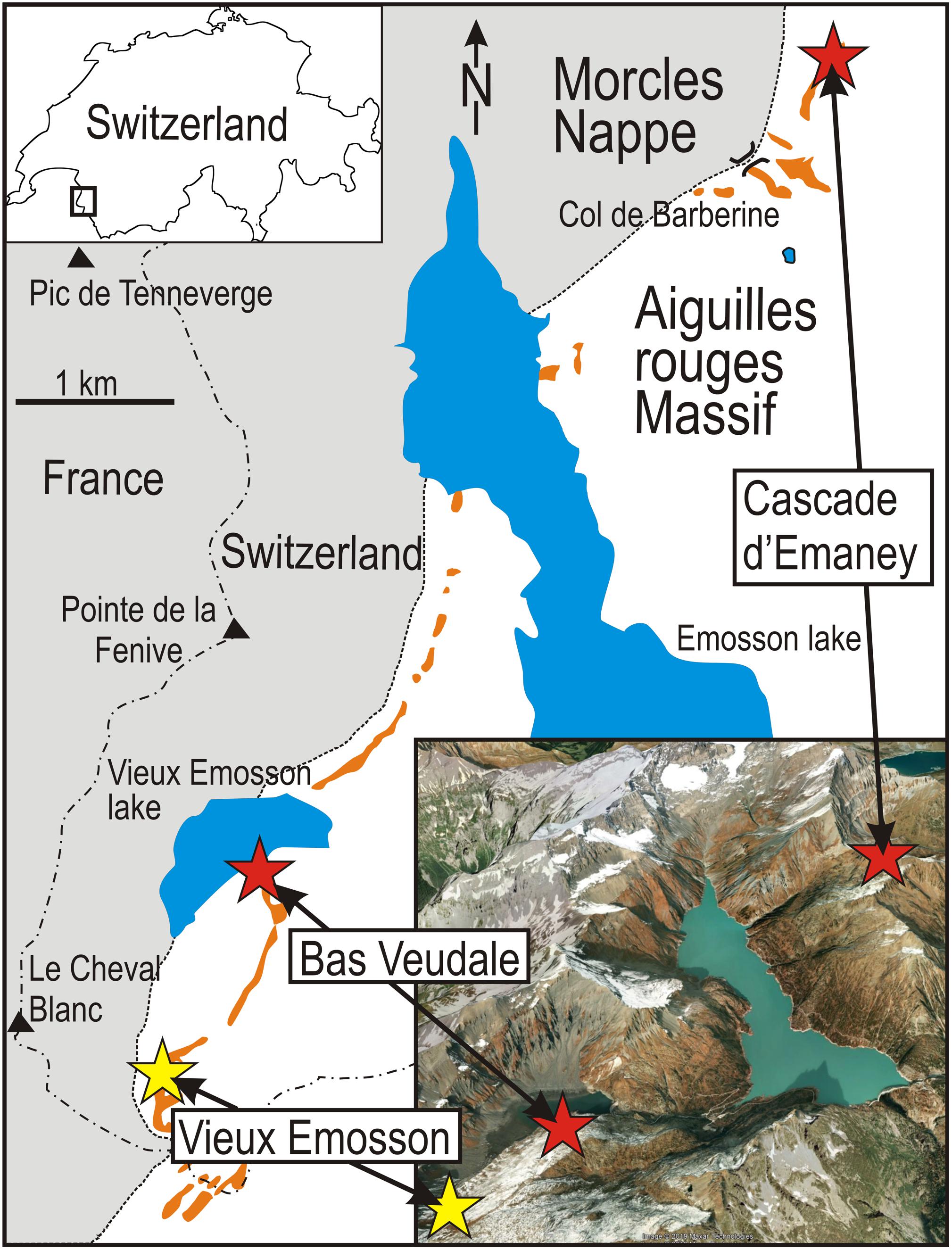
Figure 1. Geographic and geological settings of the study area, with the location of the area within Switzerland (upper left box) and an inclined aerial view (Google Earth) showing the locations of the sites in topographic context (lower right box). Orange patches represent the sandstone outcrops of the Triassic. Studied sites are indicated by red stars and the historical site of Vieux Emosson is indicated by a yellow star.
Footprints are abundant but generally poorly preserved and lacking morphological details. Their original impression in wet and soft sediment, and the effects of recent erosion, make it difficult to identify the footprints taxonomically and to recognize trackways [scored 1 and 2 on the preservation scale of Marchetti et al. (2019)]. Only a few footprints show diagnostic features that allow ichnotaxonomic assignment (see Avanzini and Cavin, 2009; Cavin et al., 2012; Klein et al., 2016). In most places, the majority of footprints consist of oval to circular concave epirelief features on a sandstone bedding surface often showing ripple marks. The presence of outer rims in some cases suggests that footprints represent true tracks rather than undertracks.
Nine ichnotaxa including several dinosaurs were initially recognized in the ichnoassemblage (Demathieu and Weidmann, 1982), but recent reinterpretations (Klein et al., 2016) restricted the assemblage to chirotheriids (Chirotherium barthii, ?Chirotherium sickleri, Isochirotherium herculis, Isochirotherium isp.). The assemblage is characteristic of the Early Middle Triassic (Olenekian-Anisian) (Avanzini and Cavin, 2009; Klein et al., 2016). Because of the warm summers of recent decades, formerly long-lasting snow fields have melted and two short trackways have been discovered near two previously studied sites separated by 6.4 km in a straight line. Both trackways are preserved at a level situated slightly below the main megatracksite (Figures 1, 2).
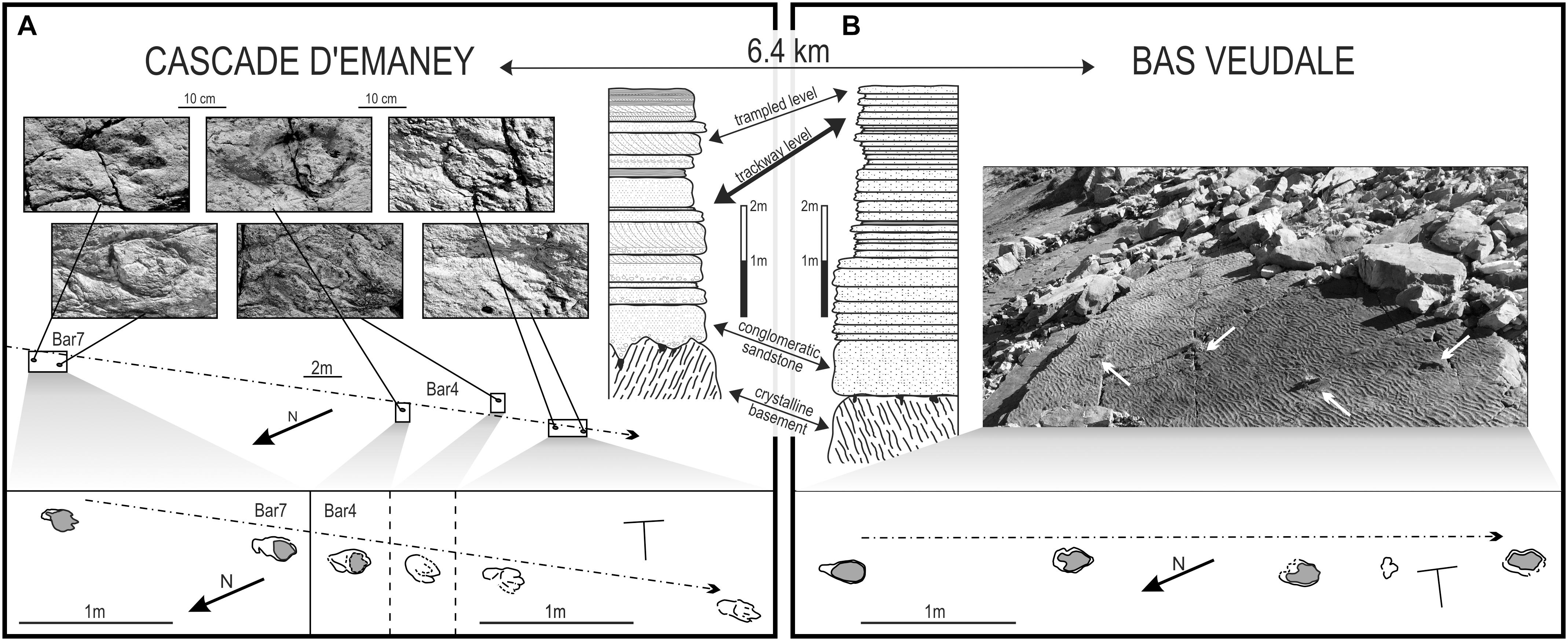
Figure 2. Comparison of the Cascade d’Emaney and Bas Veudale trackway segments, with trackway settings and sedimentological settings. The Cascade d’Emaney trackway (A) is composed of two sections (Bar 4 and Bar 7) separated by a gap of 18 m, with six footprints preserved; the Bas Veudale trackway (B) is composed of a single section composed of four footprints. Details of the trackways are depicted in the boxes at the bottom of the figure. Stratigraphic relationships between both sites: the pre-alpine basement (crystalline basement) is made up of various metamorphic rocks, whereas the autochtonous Triassic sediments include conglomeratic sandstones at the base followed by various sandstones with cross stratifications, ripple-marks, and dessication cracks; clayey mudstones interrupted by thin quartzitic sandstone layers, both with ripple-marks; and dolomitic limestones, dolomites and cargneules. The trackway level studied here (bold double-headed arrow) is located below a trampled level visible some tens of meters from both studied trackways.
Materials and Methods
Material
Cascade d’Emaney: The new trackway [Bar 4 (“Bar” corresponds to “Barberine,” the name of the mountain pass nearby)] was found in 2012 near the Cascade d’Emaney ichnosite of Cavin et al. (2012) (coordinates: N46°06′26.900′′; E6°56′19.800′′) (Figure 1). It is located about 8 m to the east of a couple of subparallel tracks assigned to an indeterminate chirotheriid, and consists of four preserved pes imprints that belong to a trackway that probably originally contained seven steps judging from the interpreted stride length (Figure 2A). Bar 4 is 10 m in total length and is precisely aligned with a pair of footprints (Bar 7) located 18 m toward the NE. The good alignment between Bar 4 and Bar 7, and the similarity in the length/width parameters of the pes imprints in both cases (Table 1), suggests that all six preserved pes imprints are remnants of a single, substantially longer trackway.
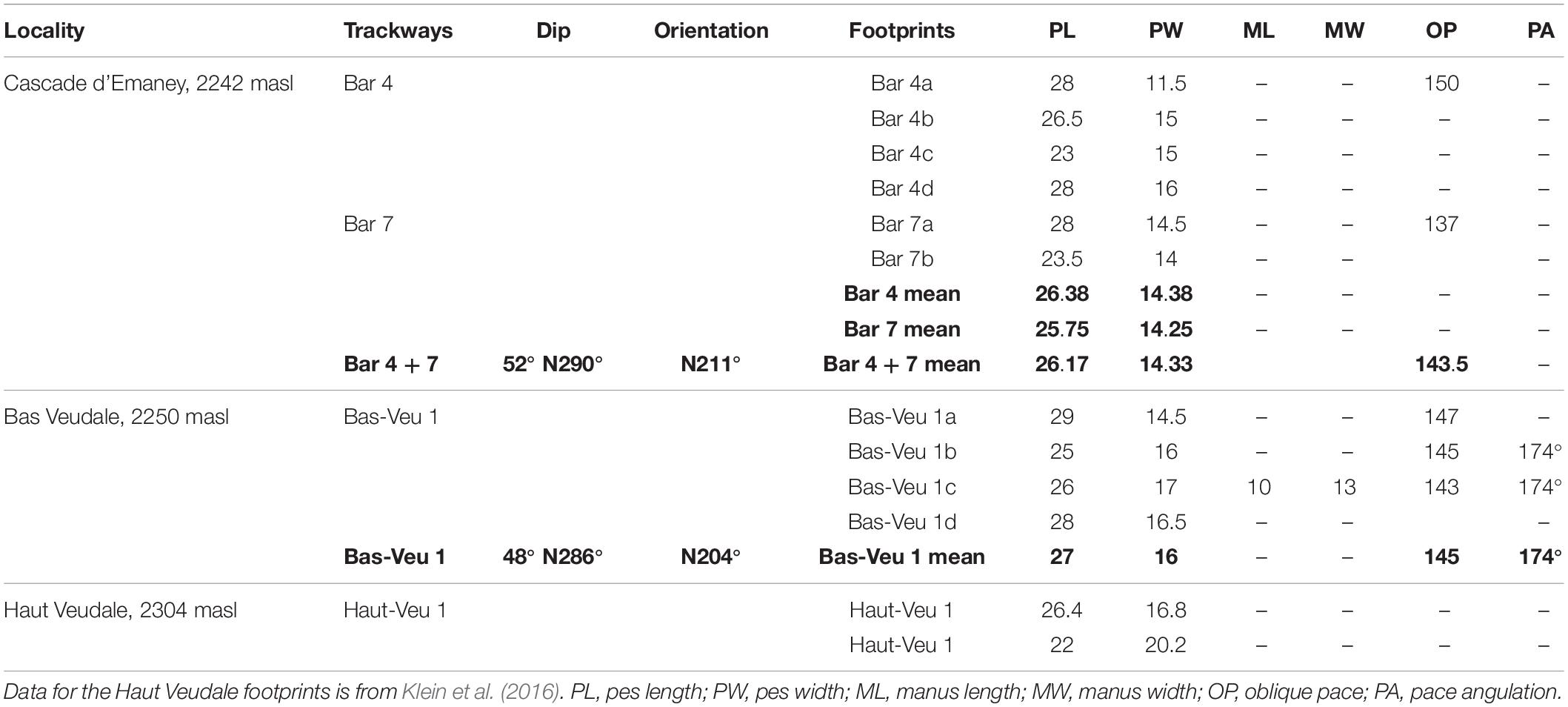
Table 1. Measurements (in centimeters) of Isochirotherium herculis footprints from three Triassic localities in SW Switzerland.
Bas Veudale: A new trackway named Bas Veudale was found in September 2015 by LC circa 20 m to the NNW (coordinates: N46°03′36′′; E6°53′34′′) (Figure 1) of a site previously studied by Klein et al. (2016) and called here Haut Veudale. The trackway (Figure 2B) is 4.6 m long and contains four pes and a single faint and uncertain manus imprint. The pace angulation characteristics are summed up in Table 1.
Methods
The trackway direction on the bedding plane was measured by plotting a horizontal line through the footprints (Figure 3A). The geometry of the study area is illustrated in Figure 3B. For both trackway-bearing surfaces, the dip magnitude, dip direction and trackway direction were all measured. The uniformity of dip throughout the study area (e.g., Amberger, 1960) allows the orientations of both trackways to be compared in the present plane of dip.
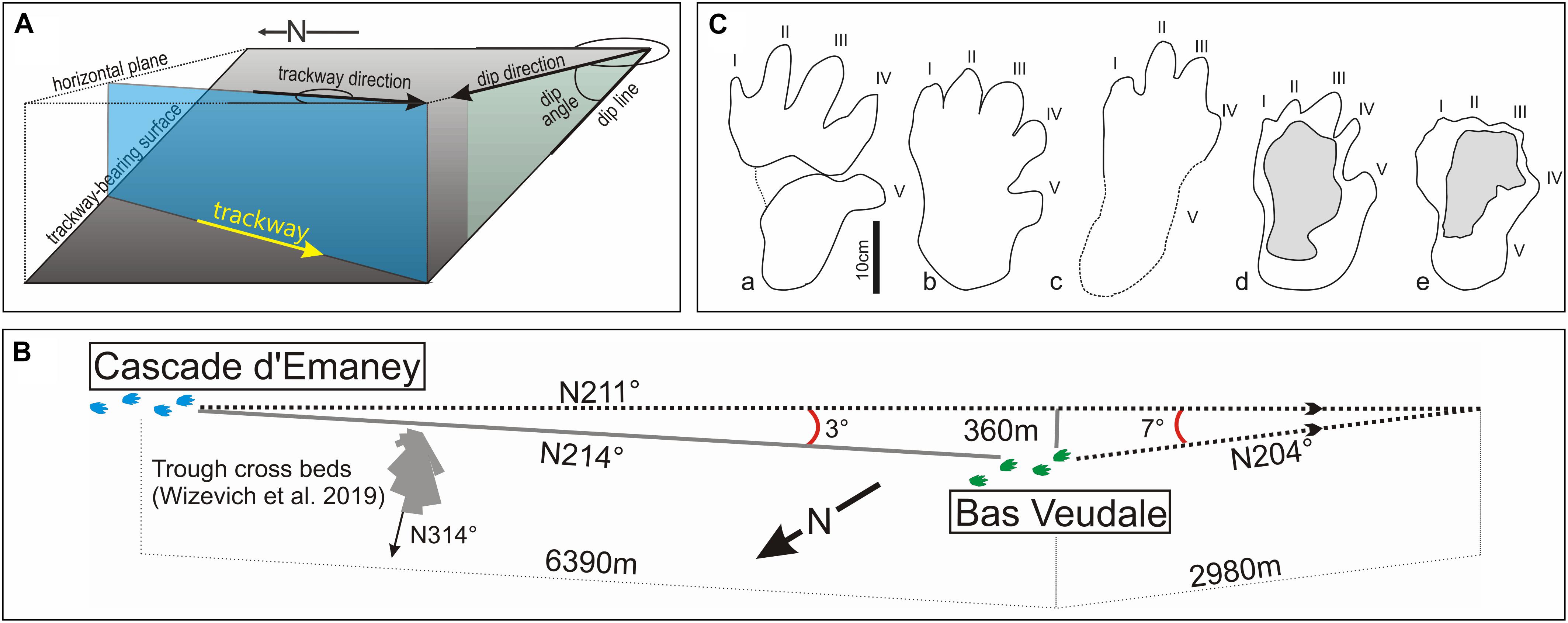
Figure 3. Trackways geometrical settings and morphology of the footprints. (A) Measurement of the trackway and dip direction; (B) geometry of trackways; (C) outlines of I. herculis pes from various localities. a, Lower Triassic (Olenekian) of Germany (redrawn from Klein and Haubold, 2003, Figure 2o); b, Lower Triassic (Aegean) of Germany (redrawn from Diedrich, 2012, Figure 6c); c, Cascade d’Emaney outcrop (bar 7b, this work); d, Bas Veudale outcrop (Bas-Veu 1d, this work); e, Haut Veudale outcrop (redrawn from Klein et al., 2016, Figure 11b).
The new trackways were mapped by tracing the outlines of the footprints on acetate sheets, and a 3D photogrammetric reconstruction of the Bas Veudale trackway was produced. Because of the poor preservation of the footprints, we were not able to apply the standard protocol recently proposed for fossil ichnological studies (Falkingham et al., 2018). Comparisons between important parameters for the new trackways from both sites were done using Mann–Whitney tests for each parameter known from more than four measurements.
Results
Stratigraphy
Klein et al. (2016) pointed out that most of the sites located along a 28 km transect in the Triassic cover of the Aiguilles Rouges Massif correspond to a single terrestrial megatracksite composed mainly of trampled areas of poorly preserved chirotheriid footprints. They noticed that most of the 18 outcrops they examined contained a single footprint level at the top of the basal sandstone conglomerate unit, although five of them contained two or three distinct track levels located 1 m or less above the top of the basal sandstone (Klein et al., 2016; Wizevich et al., 2019a). The new Bas Veudale track is probably situated some tens of centimeters below the Haut Veudale trampled level, although faults, and falling rocks make it impossible to observe the precise stratigraphic relationship between these two surfaces (Figure 2). We consider this lower level to correspond to the track-bearing level at the Cascade d’Emaney site for the following reasons: (1) The trampled level is situated a few decimeters above the newly identified track-bearing level in both cases; (2) readily identifiable tracks occur at both sites, but are absent on the trampled level and elsewhere in the section; and (3) C. herculis tracks from both sites are characterized by similar parameters. The new trackways thus indicate the presence of a track-bearing layer located under the previously recognized megatracksite, and extending over several square kilometers. This new level is still poorly documented because it is located within the sandstone series, making it less prone to exposure by erosion.
Trackways
Morphometric parameters for the new trackways and for each footprint are given in Table 1, and a photogrammetric rendering of the Bas Veudal trackway is presented in Supplementary Information (Supplementary Video S1). The distance between the two trackways is 6.4 km and a straight line between the two outcrops is oriented at 214° from the north (Figure 3B). Trigonometric calculations indicate that a deviation of 3° of the direction of the Emaney trackway would cause this trackway, if it continued for a distance of 6.4 km, to intersect the trackway at Bas Veudale. Three degrees is considered to be within the error range of the measurement of the trackways directions.
At the Cascade d’Emaney site, Bar 4 and Bar 7 cannot be compared statistically to each other because Bar 7 contains only two footprints. However, the similar mean pes lengths (25.75 cm and 26.38 cm) and the mean pes widths (14.25 cm and 14.38 cm) of footprints from both trackway segments, together with their good spatial alignment, suggest that these trackway segments could have been made by the same animal despite being separated by a gap of 8 m. Comparison between the Cascade d’Emaney trackway (Bars 4 and 7) and the Bas Veudale trackway shows no significant difference in pes length or pes width (Mann–Whitney test, p > 0.05). Only two oblique paces (OP) can be measured in the Cascade d’Emaney trackway, and consequently they cannot be statistically compared to the three OP in the Bas Veudale trackway. It is worth noting, however, that the average OP length values for both trackways are very close (143.5 cm, N = 2 for Cascade d’Emaney, and 145 cm, N = 3 for Bas Veudale).
Both trackways are referred with caution to the ichnospecies Isochirotherium herculis. Two footprints from the trampled megatracksite surface located nearby Bas Veudale and studied by Klein et al. (2016) were previously referred to this taxon (Klein et al., 2016). These two footprints are morphometrically similar to those from both trackways described here (Table 1). Although the pes outlines is poorly preserved in most footprints forming both trackways, the relative digits lengths, when they can be assessed, are similar to those seen in the two previously described isolated footprints: the digits II and III are longest and subequal in length, or digit III is slightly longer (Figure 3C). The pace angulation is only measurable for two strides from the Bas Veudale site, but lies within the range of pace angulations measured by Diedrich (2015) for trackways of Isochirotherium herculis from the Middle Triassic of the Germanic Basin, respectively, 174° in the Swiss site and 169°–178° in the German site. However, referral of the footprints to Isochirotherium herculis is uncertain because of their poor quality. However, the main concern of the present study is the comparing the two trackway segments rather establishing their ichnosystematic identification.
Discussion
A Rectilinear Route
The orientations of the I. herculis trackway segments from Cascade d’Emaney and Bas Veudale differ by only 3° (Figure 3B). So far, these two track segments are the only ones recognized in this layer, or in any nearby one, and it is likely that their alignment indicates that they belong to a single rectilinear main route. Within the same stratigraphic level at the Cascade d’Emaney site, two subparallel trackways 7 and 5 m long (Bar 1 and Bar 2 in Cavin et al., 2012) were previously referred to indeterminate chirotheriids by Cavin et al. (2012) and to C. barthii by Klein et al. (2016). Both show a general SW orientation similar to that of the new trackway (with a slight westward shift, i.e., N230° for Bar 1 and Bar 2 contra N211° for the new trackway), thus supporting the interpretation that the trackmakers had a preferred direction.
The ripple marks visible in the Bas Veudale outcrop (Figure 2B, and Supplementary Video S1) are the result of wave-induced currents on fine sand in a shallow body of water located in a proximal terminal splay (or an abandoned channel). The current direction inferred from these wave ripples is parallel to some paleocurrent measurements of Wizevich et al. (2019a), who calculated paleocurrents from trough cross-beds (average N314°) and ripple marks (average N327°). The overall transport direction is northwest (Wizevich et al., 2019a), implying flow from the Vindelician High toward the Germanic basin (and not toward the Tethyan realm as previously thought). This current orientation is approximately perpendicular to the route followed by I. herculis (Figures 3B, 4A).
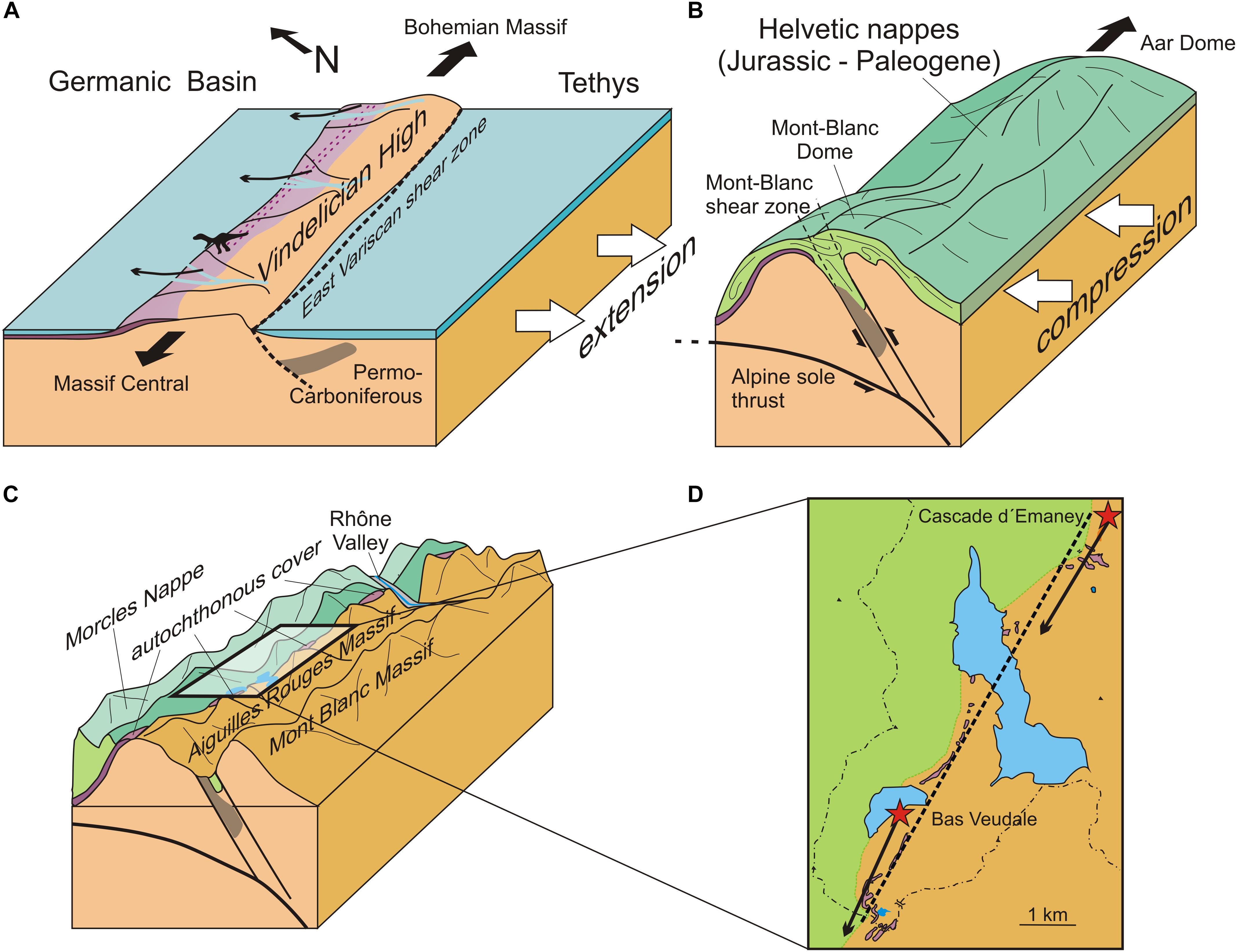
Figure 4. Highly schematic representation of the paleogeographic and tectonic evolution of the study area, illustrating the basis of the correspondence between the alignment of the Triassic outcrops and the direction of both trackways (not to scale). (A) Triassic, the archosaurs move along the Vindelician High that separated the Tethys Ocean from the Germanic Basin during the beginning of the Tethyan rifting; (B) Miocene, the trackway bearing layers are uplifted and tilted during the Alpine orogeny; (C) Recent, erosion exposes the levels with the trackways; (D) Recent, simplified geological map. The dotted line indicates the alignment of the outcrops and the arrows indicate the orientation of the trackways. Adapted from Ballèvre et al. (2018) (A); von Tscharner et al. (2016) (B); Leloup et al. (2005) (B,C).
Chirotheriid tracks are generally surmised to have been made by carnivorous archosaurs. Today, most carnivorous mammals rarely move in a straight line or for long distances. Empirical observations indicate that the daily movement distance (the sum of straight-line distances between consecutive locations during continuous 24 h radio tracking) is always three to ten times longer than the straight line distance (shortest distance between locations at the beginning and end of a 24 h tracking period) for the Iriomote cat (Prionailurus bengalensis iriomotensis) (Schmidt et al., 2003), the wolf (Canis lupus) (Jędrzejewski et al., 2001), and for the lynx (Lynx lynx) (Jędrzejewski et al., 2002). Such behaviors are inconsistent with the straight kilometers-long route inferred here. However, migrations along straight routes are well known in some large ungulates. The longest African migration, occurring in Namibia and Botswana, is that of the Burchell’s zebra (Equus quagga), with some individuals traveling 50 km per day along highly linear and unidirectional routes (Naidoo et al., 2016). One particular population of North American pronghorn (Antilocapra americana) moves nearly 50 km per day along a corridor averaging only 2 km wide, and has apparently been using this route for at least 6000 years (Berger et al., 2006). The use by mammals of an invariant corridor that is topographically constrained, particularly by the need to pass through bottlenecks, might be a good analog for the straight movement of archosaurs along the probably relatively narrow Vindelician High. The ichnofossil record also shows evidence of long and roughly unidirectional movement, although much shorter than the route conjectured to exist in this study, especially among Jurassic theropods, and sauropods. The longest measured theropod trackway worldwide is a Megalosauripus trackway from the Late Jurassic of Turkmenistan that extends for 311 m and forms a slightly undulating straight line (Lockley et al., 1996; Fanti et al., 2013), while a Lower Cretaceous site in the Jiaguan Formation, SW China, yielded a straight trackway of cf. Eubrontes with a length of 69 m (Xing et al., 2015). The site of Plagne, France, has yielded a rather rectilinear sauropod trackway 155 m long (Mazin et al., 2017). In the Middle Jurassic of Oxfordshire, UK, the Ardley Quarry has yielded several theropod and sauropod straight trackways that reach 180 and 200 m in length, respectively (Day et al., 2004). The Cenozoic record is poorer, but mention can be made of a late Miocene trackway site in the United Arab Emirates showing the straight movement of a herd of proboscideans over approximately 200 m (Bibi et al., 2012).
Parallel Orientation of the Trackways and the Series of Outcrops
Beyond the alignment of two trackway segments separated by several kilometers, another striking feature of these sites is that the orientation of the inferred route matches the alignment in the present landscape of the Triassic outcrops along a 28 km long and 100 m wide band (Figure 4D). There is no a priori reason why the orientation of the straight route should mirror the alignment of outcrops shaped by orogenic and erosional processes entirely independent of the movements of the archosaurs. This situation, however, can be explained by examining the geological processes that shaped the Triassic topography and contributed to establishing the layout of the modern trackways-bearing outcrops.
In the Middle Triassic, the “Germanic Trias” to the north and the “Alpine Trias” to the south, were separated by the Vindelician High, an exposed low-elevation area oriented roughly ENE – WSW (Ballèvre et al., 2018) along which the tracemakers moved (Figure 4A). The WSW – ENE orientation of the Vindelician High was dictated by the orientation of the remnant of the Permian East-Variscan shear zone (Ballèvre et al., 2018). Structural inheritance plays a major role in the fact that the Helvetic zone and the East-Variscan shear zone roughly conform to the SW – NE orientation of alpine paleogeographic domain boundaries. Moreover, tectonic faults that occurred during the Late Carboniferous and the Permian were reactivated during Triassic-Jurassic rifting as well as during the Alpine shortening (Leloup et al., 2005; Ballèvre et al., 2018). During the Tethyan rifting, the southern part of the Germanic Basin was controlled by the reactivation of these Variscan faults transmitted from the Tethyan rift system to its northern periphery (Szulc, 1999, 2000; Stollhofen et al., 2008). The uplift and tilting of the Aiguilles Rouges and Mont-Blanc massifs occurred along an axis that followed the East-Variscan shear zone, and consequently also the axis of Vindelician High, and produced the Mont-Blanc and the Aar domes extending to the northeast (Figure 4B). Pleistocene glacial erosion affected the boundary between the basement and the Morcles nappe along this SW – NE axis, and unearthed the Triassic cover along the original Vindelician High, whose orientation corresponds to that of the trackway (Figures 4C,D).
Although poorly preserved, both trackway segments are similar enough with respect to footprint parameters and track orientation to suggest that they form parts of a single route, at least 6.4 km long, left by trackmakers belonging to the same species, and possibly even made by the same individual. The alignment of the Triassic fossiliferous outcrops corresponds to the general direction of the trackways. This surprising concordance is explained by the Triassic topography, whose orientation corresponds to that of a major tectonic structure that was reactivated during Alpine uplift and influenced the orientation of later erosion. Hence, a 240-million-years-old fleeting animal behavior has been preserved on a large scale even through a major orogenic event.
Data Availability Statement
All datasets generated for this study are included in the article/Supplementary Material.
Author Contributions
LC and AP contributed equally to the analysis of the data and to the writing of the manuscript.
Funding
The Etat du Valais and the Commune de Finhaut contributed to funding the fieldwork.
Conflict of Interest
The authors declare that the research was conducted in the absence of any commercial or financial relationships that could be construed as a potential conflict of interest.
Acknowledgments
We thank Philippe Wagneur for the photogrammetry work, Edwin Gnos for his comments on a previous version of the manuscript, as well as colleagues and volunteers for their help during fieldwork and discussion: Pierre-Alain Proz, Emilie Läng, Jean-Luc Chavan, Christophe Ferrante, Johnathan Pesaresi, Uthumporn Deesri, Alexandre Muller, Christian A. Meyer, Michael C. Wizevich, Basil Thüring, Daniel Marty, Hendrik Klein, Peter Falkingham, Marco Avanzini, Elias Samakassou, Jean-Luc Epard, Cédric Schnyder, Jean Boissonnas, and the Vouilloz family. Data interpretation is the responsibility of the authors only. We are thankful to CS, for his thorough editorial work and to the two reviewers for their constructive comments.
Supplementary Material
The Supplementary Material for this article can be found online at: https://www.frontiersin.org/articles/10.3389/feart.2020.00004/full#supplementary-material
VIDEO S1 | Photogrammetric rendering of the Bas Veudale trackway.
References
Amberger, G.-F. (1960). L’autochtone de la Partie Nord-Ouest du Massif des Aiguilles Rouges: Haute-Savoie et Valais, Ph.D. thesis. Genève: Université de Genève, 103.
Avanzini, M., and Cavin, L. (2009). A new Isochirotherium trackway from the Triassic of vieux emosson, SW Switzerland: stratigraphic implications. Swiss J. Geosci. 102, 353–361. doi: 10.1007/s00015-009-1322-4
Ballèvre, M., Manzotti, P., and Dal Piaz, G. V. (2018). Pre-alpine (Variscan) inheritance: a key for the location of the future valaisan basin (Western Alps). Tectonics 37, 786–817. doi: 10.1002/2017tc004633
Berger, J., Cain, S. L., and Berger, K. M. (2006). Connecting the dots: an invariant migration corridor links the Holocene to the present. Biol. Lett. 2, 528–531. doi: 10.1098/rsbl.2006.0508
Bibi, F., Kraatz, B., Craig, N., Beech, M., Schuster, M., and Hill, A. (2012). Early evidence for complex social structure in Proboscidea from a late miocene trackway site in the United Arab Emirates. Biol. Lett. 8, 670–673. doi: 10.1098/rsbl.2011.1185
Cavin, L., Avanzini, M., Bernardi, M., Piuz, A., Proz, P.-A., Meister, C., et al. (2012). New vertebrate trackways from the autochthonous cover of the aiguilles rouges massif and reevaluation of the dinosaur record in the Valais, SW Switzerland. Swiss J. Palaeontol. 131, 317–324. doi: 10.1007/s13358-012-0040-0
Day, J. J., Norman, D. B., Gale, A. S., Upchurch, P., and Powell, H. P. (2004). A middle jurassic dinosaur trackway site from Oxfordshire, UK. Palaeontology 47, 319–348. doi: 10.1111/j.0031-0239.2004.00366.x
Demathieu, G. R., and Weidmann, M. (1982). Les empreintes de pas de reptiles dans le trias du vieux emosson [Finhaut, Valais, Suisse]. Eclogae Geologicae Helvetiae 75, 721–757.
Diedrich, C. (2015). Isochirotherium trackways, their possible trackmakers (? Arizonasaurus): intercontinental giant archosaur migrations in the middle Triassic tsunami-influenced carbonate intertidal mud flats of the European Germanic Basin. Carbonates Evaporites 30, 229–252. doi: 10.1007/s13146-014-0228-z
Diedrich, C. G. (2012). Middle Triassic chirotherid trackways on earthquake influenced intertidal limulid reproduction flats of the European Germanic Basin coasts. Cent. Eur. J. Geosci. 4, 495–529.
Falkingham, P. L., Bates, K. T., Avanzini, M., Bennett, M., Bordy, E. M., Breithaupt, B. H., et al. (2018). A standard protocol for documenting modern and fossil ichnological data. Palaeontology 61, 469–480. doi: 10.1111/pala.12373
Fanti, F., Contessi, M., Nigarov, A., and Esenov, P. (2013). New data on two large dinosaur tracksites from the upper Jurassic of Eastern Turkmenistan (Central Asia). Ichnos 20, 54–71. doi: 10.1080/10420940.2013.778845
Jędrzejewski, W., Schmidt, K., Okarma, H., and Kowalczyk, R. (2002). Movement pattern and home range use by the Eurasian lynx in Białowieża Primeval Forest (Poland). Proc. Ann. Zool. Fennici 39, 29–41.
Jędrzejewski, W., Schmidt, K., Theuerkauf, J., Jedrzejewska, B., and Okarma, H. (2001). Daily movements and territory use by radio-collared wolves (Canis lupus) in Bialowieza Primeval Forest in Poland. Can. J. Zool. 79, 1993–2004. doi: 10.1139/cjz-79-11-1993
Klein, H., and Haubold, H. (2003). Differenzierung von ausgewählten Chirotherien der Trias mittels Landmarkanalyse. Hallesches Jahrbuch für Geowissenschaften B 25, 21–36.
Klein, H., Wizevich, M. C., Thüring, B., Marty, D., Thüring, S., Falkingham, P., et al. (2016). Triassic chirotheriid footprints from the Swiss Alps: ichnotaxonomy and depositional environment (Cantons Wallis & Glarus). Swiss J. Palaeontol. 135, 295–314. doi: 10.1007/s13358-016-0119-0
Leloup, P.-H., Arnaud, N., Sobel, E. R., and Lacassin, R. (2005). Alpine thermal and structural evolution of the highest external crystalline massif: the Mont Blanc. Tectonics 24, 1–26.
Lockley, M. G., Meyer, A. C., Schultz-Pittman, R., and Forney, G. (1996). “Late Jurassic dinosaur tracksites from Central Asia: a preliminary report on the world’s longest trackways,” in The Continental Jurassic, ed. M. Morales (Flagstaff, AZ: Museum of Northern Arizona Bulletin), 60.
Marchetti, L., Belvedere, M., Voigt, S., Klein, H., Castanera, D., Díaz-Martínez, I., et al. (2019). Defining the morphological quality of fossil footprints. Problems and principles of preservation in tetrapod ichnology with examples from the Palaeozoic to the present. Earth Sci. Rev. 193, 109–145. doi: 10.1016/j.earscirev.2019.04.008
Mazin, J.-M., Hantzpergue, P., and Olivier, N. (2017). The dinosaur tracksite of Plagne (early Tithonian, Late Jurassic; Jura Mountains, France): the longest known sauropod trackway. Geobios 50, 279–301. doi: 10.1016/j.geobios.2017.06.004
Meyer, C. A., and Thüring, B. (2003). Dinosaurs of Switzerland. C. R. Palevol 2, 103–117. doi: 10.1016/s1631-0683(03)00005-8
Naidoo, R., Chase, M., Beytell, P., Du Preez, P., Landen, K., Stuart-Hill, G., et al. (2016). A newly discovered wildlife migration in Namibia and Botswana is the longest in Africa. Oryx 50, 138–146. doi: 10.1017/s0030605314000222
Schmidt, K., Nakanishi, N., Okamura, M., Doi, T., and Izawa, M. (2003). Movements and use of home range in the Iriomote cat (Prionailurus bengalensis iriomotensis). J. Zool. 261, 273–283. doi: 10.1017/s0952836903004205
Stollhofen, H., Bachmann, G., Barnasch, J., Bayer, U., Beutler, G., Franz, M., et al. (2008). “Upper rotliegend to early cretaceous basin development,” in Dynamics of Complex Intracontinental Basins. The Central European Basin System, eds R. Littke, U. Bayer, D. Gajewski, and S. Nelskamp (Berlin: Springer), 181–210.
Szulc, J. (1999). Anisian-Carnian evolution of the Germanic basin and its eustatic, tectonic and climatic controls. Zentralbl. Geol. Paläontol. Teil I 1, 813–852.
Szulc, J. (2000). Middle Triassic evolution of the northern Peri-Tethys area as influenced by early opening of the Tethys Ocean. Proceedings Annales Societatis Geologorum Poloniae. 70, 1–48.
Valla, P. G., van der Beek, P. A., Shuster, D. L., Braun, J., Herman, F., Tassan-Got, L., et al. (2012). Late Neogene exhumation and relief development of the Aar and Aiguilles Rouges massifs (Swiss Alps) from low-temperature thermochronology modeling and 4He/3He thermochronometry. J. Geophys. Res. 117, 1–23.
von Tscharner, M., Schmalholz, S. M., and Epard, J.-L. (2016). 3-D numerical models of viscous flow applied to fold nappes and the Rawil depression in the Helvetic nappe system (western Switzerland). J. Struct. Geol. 86, 32–46. doi: 10.1016/j.jsg.2016.02.007
Wizevich, M. C., Ahern, J., and Meyer, C. A. (2019a). The Triassic of southwestern Switzerland–Marine or non-marine, that is the question! Palaeogeogr. Palaeoclimatol. Palaeoecol. 514, 577–592. doi: 10.1016/j.palaeo.2018.11.016
Wizevich, M. C., Meyer, C. A., Linnemann, U., Gärtner, A., Sonntag, B.-L., and Hofmann, M. (2019b). U–Pb zircon provenance of Triassic sandstones, western Swiss Alps: implications for geotectonic history. Swiss J. Geosci. 112, 1–16.
Xing, L., Lockley, M. G., Zhang, J., Klein, H., Marty, D., Peng, G., et al. (2015). The longest theropod trackway from East Asia, and a diverse sauropod-, theropod-, and ornithopod-track assemblage from the lower Cretaceous Jiaguan Formation, southwest China. Cretac. Res. 56, 345–362. doi: 10.1016/j.cretres.2015.05.008
Keywords: paleoichnology, ethology, orogeny, Triassic, Chirotheriidae, footprint
Citation: Cavin L and Piuz A (2020) A Several-Kilometer-Long Archosaur Route in the Triassic of the Swiss Alps. Front. Earth Sci. 8:4. doi: 10.3389/feart.2020.00004
Received: 30 October 2019; Accepted: 14 January 2020;
Published: 04 February 2020.
Edited by:
Corwin Sullivan, University of Alberta, CanadaReviewed by:
David Hone, Queen Mary University of London, United KingdomGrzegorz Pienkowski, Polish Geological Institute, Poland
Copyright © 2020 Cavin and Piuz. This is an open-access article distributed under the terms of the Creative Commons Attribution License (CC BY). The use, distribution or reproduction in other forums is permitted, provided the original author(s) and the copyright owner(s) are credited and that the original publication in this journal is cited, in accordance with accepted academic practice. No use, distribution or reproduction is permitted which does not comply with these terms.
*Correspondence: Lionel Cavin, lionel.cavin@ville-ge.ch