- 1Institute for Nature Management, National Academy of Sciences of Belarus, Minsk, Belarus
- 2State Hydrological Institute, Saint Petersburg, Russia
- 3Saint Petersburg State University, Saint Petersburg, Russia
- 4National Centers for Environment Information, North Carolina State University, Asheville, NC, United States
- 5Hydrology Science and Services Corporation, Asheville, NC, United States
The study presents an assessment of the recent and projected changes of the middle and upper Western Dvina River runoff and regional climate during the 20th and 21st centuries. For this assessment, we used historical runoff data, the output of EURO-CORDEX consortium calculations for scenarios RCP4.5 and RCP8.5, and hydrological model “Hydrograph.” Analysis of monthly runoff data for the 1945–2015 period revealed positive trends for each of the five months from December to April. These trends are statistically significant at the 0.05 level. No significant trends were found for other months. Significant negative trends were established for spring flood peak discharges (from −69 to −88 m3 s–1 per 10 years). Usually, maximum discharges are observed during spring floods. Minimum discharges during winter low-water period were increased by 6 m3 s–1 per 10 years. The annual runoff trend was statistically significant only at the Polotsk gauging station (9.5 m3 s−1 per 10 years). To the end of the current century over the study region, estimates of projected meteorological parameters (air temperature and precipitation) show positive tendencies of air temperature (from 2.4°C to 4.7°C depending on scenario) and precipitation (up to 15 to 30 mm). Changes of seasonal and annual temperature and precipitation vary depending on the models and scenarios used. The strongest changes were noticed for the RCP8.5 scenario. The greatest changes within each scenario were revealed for the winter and spring seasons. It is projected that during the 2021–2100 period according to both RCP scenarios, annual discharges will not change in the upper part of the Western Dvina River Basin and increase by 10–12% in its lower part. The maximum spring flood discharges in both RCP scenarios are expected to decrease by 25%. The minimum runoff of winter low-flow period is expected to increase by up to 60 to 90% above the present long-term mean values.
Introduction
The Baltic Sea Basin belongs to regions with a relatively high availability of water resources and possesses a dense hydrographic network. The transboundary location of the major rivers in the Baltic Sea Basin promotes intensive use of the international freshwater sources. Rivers and lakes are used for population and industry water supply, energy production, and shipping. Water resource management requires careful revisions and regular assessments of hydrological regime changes as well as analyses and accounting for the controlling climate factors.
The present research work is a regional case study of climatic and hydrological changes within the eastern part of the Baltic Sea Basin during the recent decades and for the rest of the 21st century. The study object is the upstream part of the Western Dvina (or Zapadnaya Dvina, or Daugava) River Basin, which is located within the boundaries of two countries – Russia and Belarus. We investigated the temporal and spatial variations in hydroclimatic conditions across the Basin having two primary objectives:
(1) to assess annual, seasonal and monthly historical trends in hydrological variables affecting river flows using hydrological instrumental time series for the 1945–2015 time period; and
(2) to assess the projected decadal trends of the hydroclimatic variables in the Basin for the 2021–2100 period.
Several groups of authors have previously documented hydroclimatic changes in the Baltic Sea Basin and particularly in the Western Dvina River Basin. The detailed assessment of climatic change for northern Europe was provided by the BACC Author Team (2008, 2015). The Team studied the past, present, and projected future changes in atmospheric, hydrological and oceanographic conditions over the Baltic Sea Basin and assessed the observed and potential impacts of these changes on natural environment and socio-economic conditions. Hydrological studies within the BACC collaboration provided valuable information for the entire Sea Basin, as well as for individual countries.
The regional peculiarities of streamflow formation in the Baltic Sea Basin during the last decades according to Stahl et al. (2010) consist in positive trends with increasing streamflow in winter months in most catchments of the Basin, while in spring and summer months, strong negative trends were found (decreasing streamflow and shift toward drier conditions).
Regional pattern of changes was provided by Hisdal et al. (2010) and Wilson et al. (2010). They showed the trends toward increased annual, winter and spring streamflow over many rivers of the Sea Basin. The tendency for a decrease in annual discharge in the southern catchments was recognized by Gailiušis et al. (2011) and Hansson et al. (2011). Reihan et al. (2007) have reported a significant increase in winter river discharge and a tendency for decreasing spring floods for the east Baltic States (excluding Russia and Belarus). Kriauciuniene et al. (2012) found no significant change in runoff in 1991–2006 relative to the reference period. An increasing trend in annual mean discharge for the 1961–2000 period was found for Latvian rivers and the increasing trends were statistically significant for many rivers including Daugava (Kıaviņš and Rodinov, 2008; Kıaviņš et al., 2008). Trends in the annual maximum and minimum discharges for the major rivers Daugava, Lielupe, Venta, Gauja, and Salaca indicate a statistically significant decrease in maximum discharge.
Studies of streamflow fluctuations due to climate change within the northwest Russia (Frolova et al., 2017; Nasonova et al., 2018), and Belarus (Polishchuk and Chekan, 2009; Volchek and Gryadinova, 2010; Volchek and Shelest, 2012; Volchek and Parfomuk, 2013; Loginov et al., 2014; Lopukh and Partasenok, 2014) show no significant long-term trends in annual streamflow. Meanwhile, the intra-annual distribution of runoff has changed significantly during the last decades. In particular, runoff during winter low-flow periods increased significantly. This increase was connected with the growing repeatability of thaws, which led to frequent floods occurring during winter low-water period, while spring runoff and snowmelt floods were decreasing due to the exhausted water supply in snow prior to the spring season. However, the general pattern of described changes in water regime varies from year to year due to the increasing of frequency of extreme flow events and of their duration.
The majority of hydrological projections in the Baltic Sea Basin have been done at national levels. The decrease of annual and seasonal streamflow by 2–40% according to the Special Report on Emissions Scenarios (SRES) scenario A1B, A2 and B2 was projected for the rivers in Norway (Beldring et al., 2008), Finland (Veijalainen et al., 2010), Latvia (Apsīte et al., 2011), Lithuania (Kriaučiūnienė et al., 2008) and Poland (Szwed et al., 2010). The annual streamflow increase by 9–34% has been projected for Denmark (Thodsen et al., 2008; Jeppesen et al., 2009). Large uncertainties in the future hydrological regime were reported for Sweden (Yang et al., 2010; Olsson et al., 2011) and projected the streamflow changes within ± 30% (Arheimer and Lindström, 2015). Donnelly et al. (2017) reported the runoff increase in the northern Europe. Roudier et al. (2015) found the relatively strong decrease in flood magnitude in some parts of Finland, NW Russia and north of Sweden. According to Thober et al. (2018), the floods’ intensity decreased by 5–12% in northern Europe. In the eastern part of the Baltic Sea Basin, the current changes in the hydrological regime observed within the Belorussian territory during the past decades (increase of winter and decrease of spring streamflow) are projected to continue for four major river basins of the country (Volchek et al., 2017). The estimates of projected runoff changes over the Russian Northwest show a considerable change of seasonal runoff dynamics for the Volga (Georgievskiy et al., 2018) and the Northern Dvina Basins (Krylenko et al., 2015).
The conducted researches showed the detailed assessment of historical and projected hydroclimatic regime changes in the Baltic Sea Basin. Nevertheless, large-scale studies often describe the general changes without regional details or are presented at the national level and are limited by administrative boundaries cutting the original water system. As a result, there were no regional assessments of possible change in hydrological regime for the entire Western Dvina River Basin. That is why the aim of our study is to estimate current and future changes in climatic and hydrological regimes of this transboundary river basin within at least two countries, to assess their directions and significance, and to analyze possible reasons caused these transformations.
The present study is a contribution to the Baltic Earth Science Plan within the topic “Regional variability of water and energy exchanges in the Baltic Sea region.”
Study Area
The Western Dvina River Basin is about 87900 km2. The basin has common borders with the Lielupe river basins (Latvia), the Neman (Belarus and Lithuania) and the Dnieper (Russia, Belarus and Ukraine) in the south and the Volga River (Russia) in the east. The Western Dvina River Basin’s length is the highest in the east-west direction with a basin’s convexity turned to the south. Basin’s length is about 700 km, width on average is 120 km. The largest part of the catchment is shared almost equally between Russia, Belarus and Latvia. The common length of the Western Dvina River is about 1020 km. The location of the study Basin is presented on the Figure 1.
There are many lakes within the Basin; the lake area percentage is about 3%. The wetland fraction riches 14%, 4% among them are presented by pure bogs and 10% are extra-wet forests, bushes and meadows. In general, about 50% of the Basin’s area is occupied by forests, 4% by bogs, 3% by lakes, and 42% by agricultural lands.
Data and Methods
Data Sources
In this study, we used meteorological and hydrological data of different origin. The first type of data was obtained from the hydrometeorological network of instrumental observations belonging to the State Hydrometeorological Services of Russia and Belarus. It was used to estimate recent changes in climate and runoff of the study region. The dataset consists of:
(1) meteorological data of 18 stations (Figure 2 and Table 1): time series of monthly and annual precipitation and surface air temperature;
(2) hydrological data of 21 stations (Figure 2): time series of monthly and annual streamflow, minimum discharges during winter and summer low-water periods, and maximum discharges during spring high-water periods.
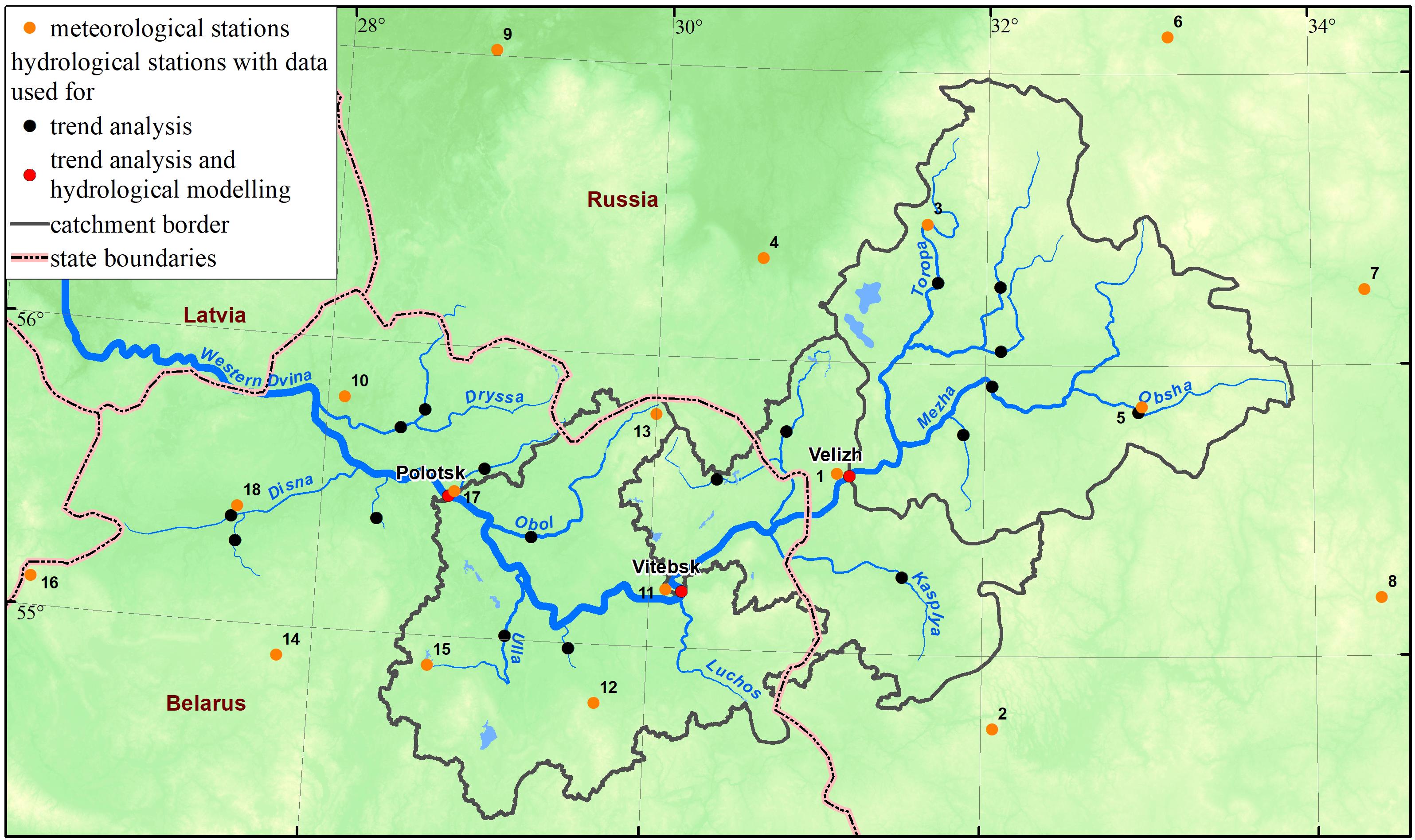
Figure 2. Stations in the Western Dvina River Basin with instrumental observations of meteorological and hydrological characteristics.
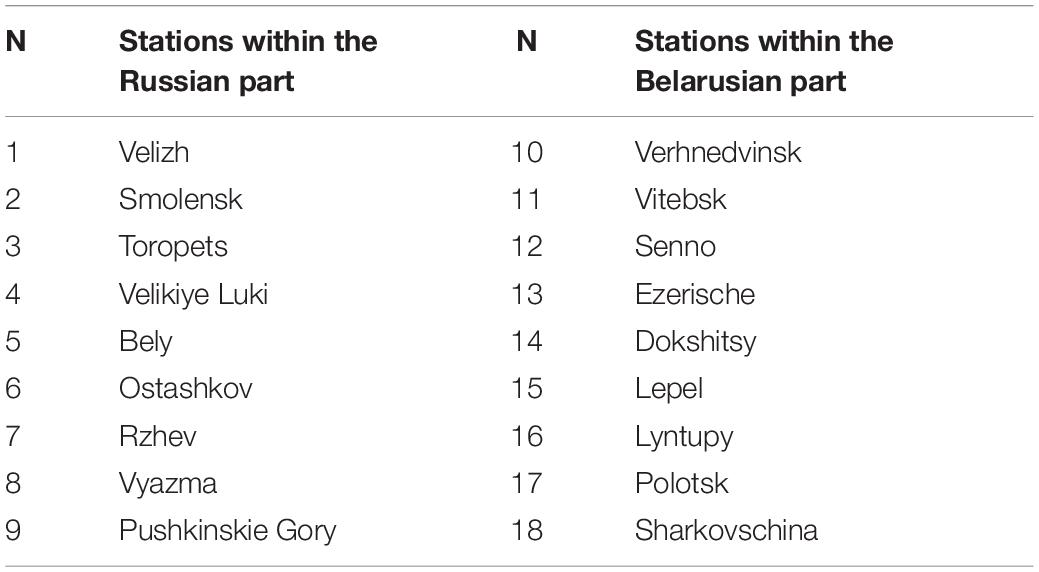
Table 1. Meteorological stations in the Baltic Sea part of Russia and Belarus (numbers in the table correspond to numbers in Figure 2).
The hydrological time series were used to define trends and to analyze the spatial distribution of monthly, minimum and maximum streamflow changes within the studied region as well as for the calibration of the hydrological model described below.
The second type of data was derived from climate models, i.e., from the regional downscaled dataset delivered by EURO-CORDEX consortium. We used daily mean values of air temperature and precipitation and calculated monthly, seasonal, and annual means from 10 to 11 climate models for each characteristic and scenario. The ensemble median was used as generalizing characteristic of projected climatic conditions over the River Basin.
For its projections, the consortium uses the scenario of greenhouse gases concentrations of the RCP family (Representative Concentration Pathways) (Meinshausen et al., 2011) according to the report of the Intergovernmental Panel Climate Change. For the present study, we used two such scenarios of radiative forcing: RCP4.5 (which corresponds to the concentration of 650 ppm) and RCP8.5 (1370 ppm).
For the analysis of climate projections over the Western Dvina River Basin, we selected the 2021–2100 period. The historical period of 1970–2000 was used for comparison with the current climate and the calculation of deviations from meteorological means.
Land use data were derived from the National Atlases of Belarus (Zhmoydyak, 2009), topographic maps, and digital soil map (Sanchez et al., 2009).
Methodology
The assessment of current changes included the calculations of intra-annual distribution, time series of synchronic fluctuations over the study basin, trend analysis, and the study of spatial peculiarities of runoff formation over the region.
The calculation of the intra-annual distribution of runoff was conducted based on monthly discharges time series, which firstly were merged by seasons and thereafter the seasonal values, were used to calculate seasonal streamflow ratio (percentage) to the runoff annual mean values.
Data analysis was conducted on a monthly basis to identify month-to-month variation in the selected hydroclimatic variables and to determine trend consistency within multiple months. However, the results were discussed and spatially analyzed only on a seasonal basis. The winter season was defined as December through February, spring was from March to May, summer was June through September, and autumn from October to November.
The winter season across the study area covers mostly the snow accumulation period up to the spring river ice break-up. Spring includes the river break-up and flooding until the summer and autumn seasons when low-water flow is interrupted time to time by rapid rain-induced floods.
Extreme hydrological characteristics are defined as maximum and minimum discharges. The maximum discharges are sampled as highest values among the two-term daily time series during floods for each year. The maximum discharges are usually observed during the spring season and are associated with snowmelt floods. Minimum discharges defined as the lowest values from two-term daily time series of each year.
The minimum discharges (and in some cases minimum flow levels) were defined for cold and warm periods separately. The cold period lasts from November to April and the warm period lasts from May to October. The definitions of these periods are connected to the climatic conditions of the region and the passing of the air temperature through the 0oC isotherm in spring and autumn respectively.
The specific discharge (SD) from the basin was used to build the spatial distribution of streamflow. The SD is defined as the quantity of water which is derived for a drainage basin unit area in the given time interval. The SD unit is liter s–1 km–2.
A Student’s t-test was used to determine if there is a significant difference between the means of two groups of climatic or hydrological characteristics.
The trend analysis was carried out using the Mann-Kendall (MK) test (Mann, 1945; Kendall, 1975). The test statistically assesses an upward or the downward trend of variable over time. A monotonic upward (downward) trend means that the variable consistently increases (decreases) through time, but the trend may or may not be linear. The MK test was used to test the slope of the estimated linear regression line. The trend slopes were studied using the Sen’s method by choosing the median of the slopes of all lines through the pairs of points (Sen, 1968).
Hydrological Modeling
Runoff modeling was carried out by applying the “Hydrograph” model. The model was developed at the Russian State Hydrological Institute (Vinogradov et al., 2011; Makarieva, 2018).
The model describes the streamflow formation processes in the river basins with different physic-geographical characteristics and uses climate and land-use information as an input data. The climatic input includes the time series of daily air temperature, water vapour pressure deficit (VPD), precipitation and its duration.
Meteorological data for the historical period were obtained from E-OBS archive (Haylock et al., 2008). We used typical intra-annual VPD distribution for wet and dry days, respectively. The output of the model is the continuous hydrograph of runoff in the closing gauge observation point for the defined period. Figure 3 presents the runoff change assessment scheme.
Hydrological projections were made using daily air temperature and precipitation output from the EC-EARTH driving model and SMHI-RCA4 regional climate model. These climate models were selected because they showed the best coherence between observed (E-OBS) and the historical modeled time series of air temperature and precipitation. The air temperature and precipitation bias adjusted model output was used in the study. It was produced according to the Distribution-Based Scaling method (SMHI-DBS45; Yang et al., 2010) developed by EURO-CORDEX members. The calculations were made for RCP4.5 and RCP8.5 scenarios.
The Nash-Sutcliffe model efficiency coefficient (NSE; Nash and Sutcliffe, 1970) and PBIAS criterion (Gupta et al., 1999) were used for model suitability assessment and calculations of expected changes in the streamflow pattern. The PBIAS (percent bias) measures the average tendency of the simulated flows to be larger or smaller than their observed counterparts. The optimal PBIAS value is 0.0. Its positive values indicate a model bias toward the underestimation of the flow, while negative PBIAS values indicate a bias toward the overestimation of the flow. For the period of 1970–2015, we received the following NSE and PBIAS criteria for the Western Dvina River: 0.79 and 9.7% respectively. Modeling results can be considered as satisfactory (Moriasi et al., 2015). The results of historical simulating are presented in Figure 4.
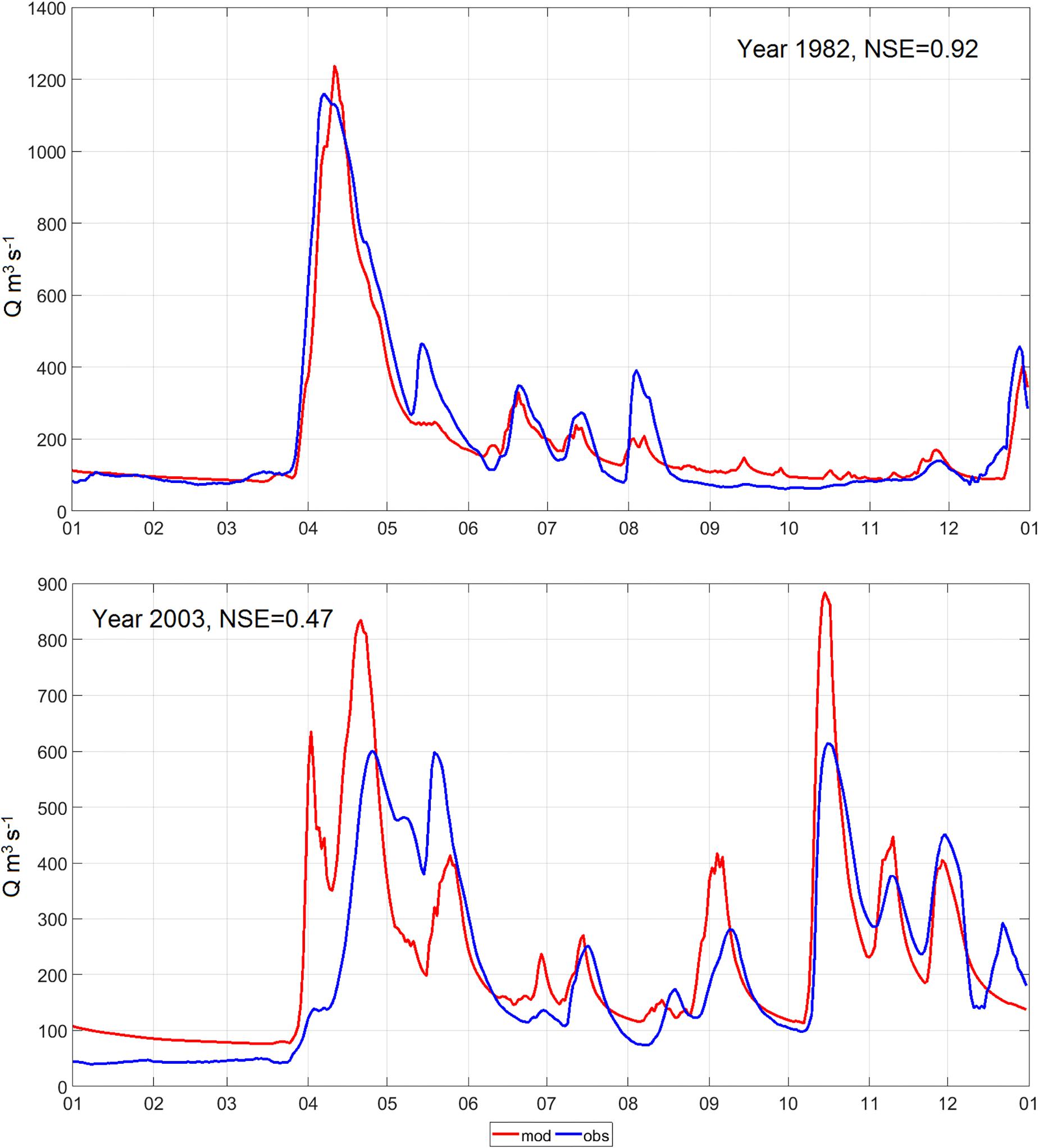
Figure 4. The results of the historical simulating of streamflow on the Western Dvina River at the Vitebsk gauging station. The upper figure presents the best coherence of the observed and modeled streamflow in 1982, the bottom figure presents the worst simulated results for 2003.
Results and Discussion
Climatic Conditions
The climate of the study basin strongly depends on the Atlantic Ocean. The air streams from the Atlantic Ocean bring wet and warm air to the region. As a result, the climate of the Western Dvina River Basin is characterized by mild climate, high humidity, and moderate air temperature. In general, it is possible to earmark three areas in the Western Dvina River Basin, which vary by physiographic factors: the upstream part from the river originating to Vitebsk, the middle part from Vitebsk to Daugavpils, and the near-estuary part. These three areas differ by their climatic conditions, which are formed by the level of the sea and continent interactions.
In the upstream part of the Basin that is located quite far from the influence of the Baltic Sea, the climate is continental. Frequently, this part of the Basin becomes under the influence of wet and cold arctic air masses. The middle part of the Basin in Belarus is located in a transitional zone. Here the weather can be controlled by the prevalence of continental air, the same way as in the upper part of the Basin. However, frequently the sea air masses may arrive here keeping in this part of the Basin mild maritime weather conditions, especially in the transitional periods of spring and autumn.
In the lower part of the Basin, which is the closest to the Baltic Sea, the climate is mild due to the domination of the Baltic Sea air masses that are quite warm in winters and relatively cold in summers.
The annual average temperature across the Basin fluctuates from 4.4°C in the south and west to 5.8°C in the east (Figure 5), with mean January temperatures varying from −5.3°C in the northeast to −4.8°C in the southwest, while mean July temperatures vary from 14°C in the northwest to 15°C in the southeast. The dynamics of the air temperature during the year is smoothed over the entire Basin with annual air temperature amplitudes being about 25°C. Absolute minimum of air temperatures may be about −30 to −40°C (in January); the absolute maximum temperatures can reach from + 31 up to + 37°C (in July to August). During the decades since 1989, the temperature in the region has grown with annual mean values increased by up to + 1oC and the largest increase (by up to + 1.5 – + 2.5°C) was documented in the winter months.
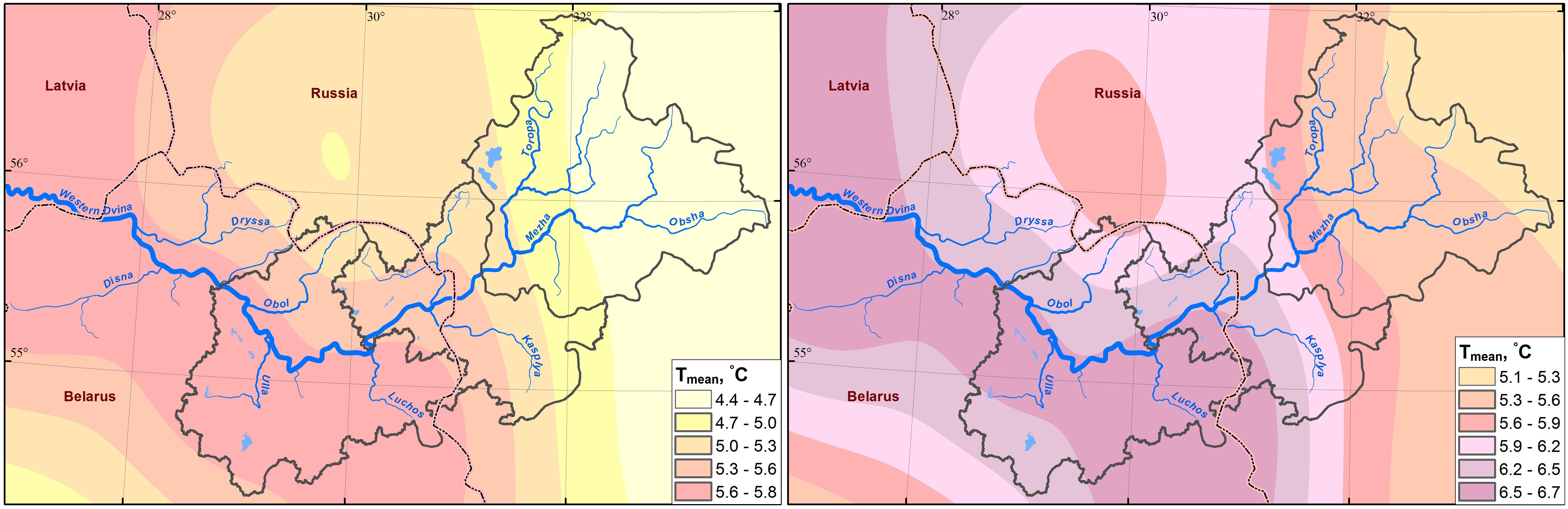
Figure 5. The spatial distribution of the annual temperature mean values over the Western Dvina River Basin for two different periods: 1945 to 2015 (left) and 1989 to 2015 (right).
Within the Western Dvina River Basin, annual precipitation ranges from 650 to 700 mm in the middle part, to 750–800 mm in the upper part of the Basin. About 70% of precipitation falls in liquid form during the warm part of the year. Snow accumulates in winter, with maximum ground snow cover occurring at the end of February. During the last decades (1989–2015), the moistening pattern in the River Basin has changed (Figure 6). Over the entire Basin, the annual precipitation totals increased by about 50 mm.
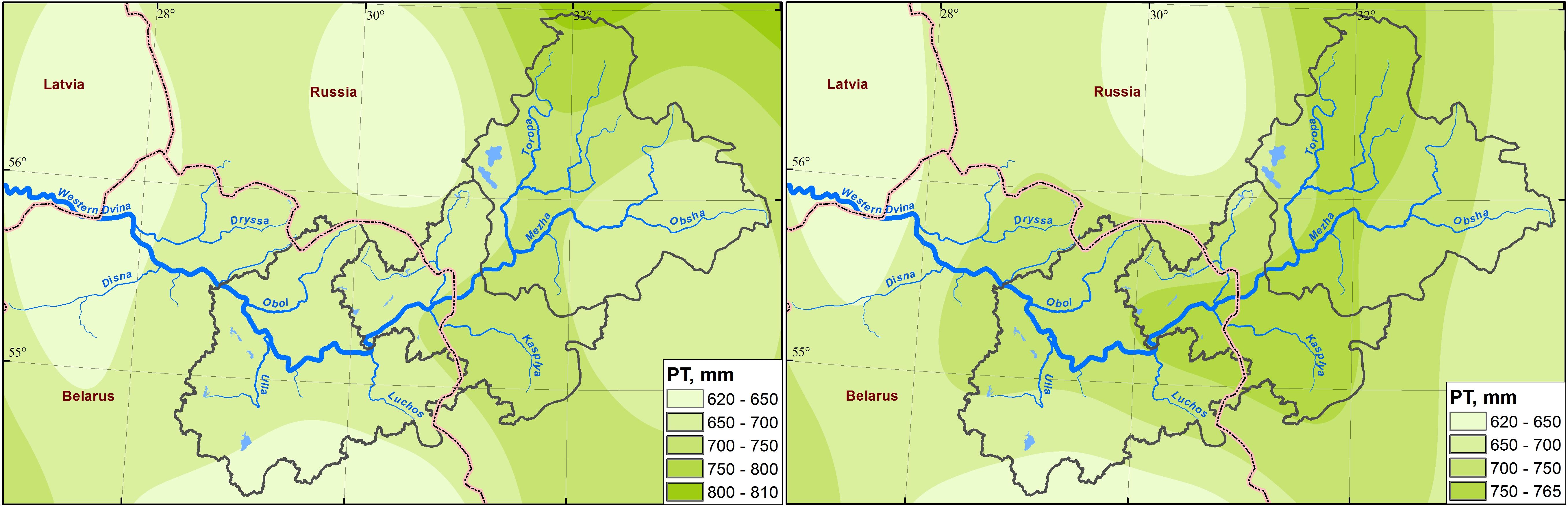
Figure 6. The spatial distribution of annual precipitation totals over the Western Dvina River Basin for two different periods: 1945–2015 (left) and 1989–2015 (right).
Recent Streamflow Pattern
Monthly Streamflow
Monthly and annual discharges in the Western Dvina River gradually increase with the river catchment growth (Table 2).

Table 2. Hydrological characteristics of the Western Dvina River (within Russia and Belarus boundaries).
During the 1945–2015 period, several factors were responsible for changes of monthly and annual discharges of the River. The leading driver of streamflow changes in the study basin is precipitation. Figure 7 represents the decadal changes of monthly discharges in the Western Dvina River near Velizh, Vitebsk and Polotsk gauging stations. The period of 1945–1964 is characterized by the variations of monthly and yearly discharges around the mean values for the 1945–2015 period. The smallest monthly discharges were observed in 1965–1984, except Velizh where the smallest monthly values were observed in 1963–1980. During these decades, the annual discharges were lower than the mean values and low streamflow was observed during all seasons. Discharges were less than the mean values by 3–56% near Velizh, 2–32% near Vitebsk, and 2–37% near Polotsk. The exception was in 1978, when streamflow was higher by 20–23% due to extremely high snowy conditions before the spring snowmelt and, consequently, large floods on many Belarusian rivers.
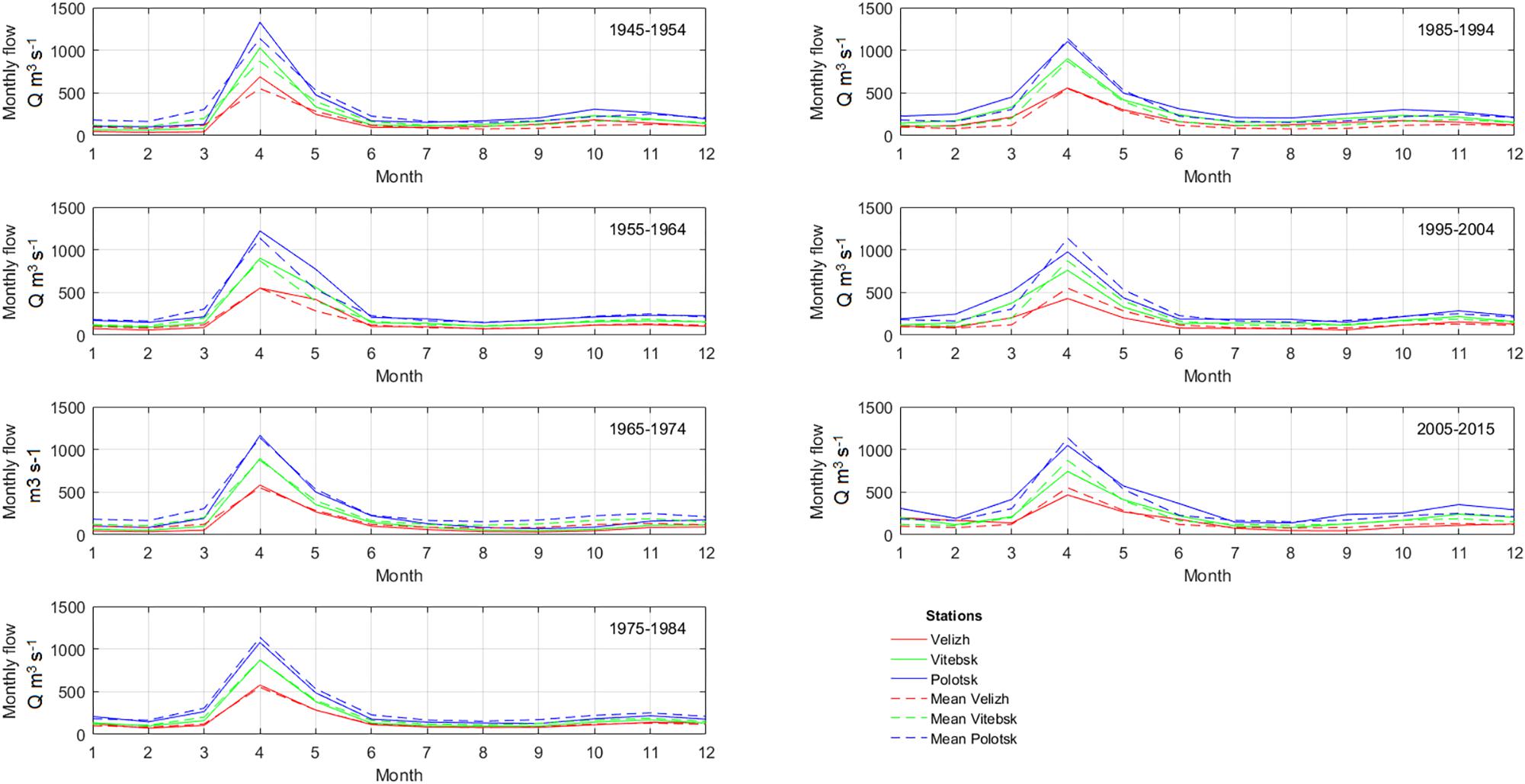
Figure 7. Dynamics of monthly discharges (m3 s–1) in the Western Dvina River at the Velizh, Vitebsk and Polotsk gauging stations.
During the last decades (1985–2015), the discharges again varied around the long-term mean value at the Velizh gauging station, where positive and negative deviations reached up to 60% from the average. In the Belarusian part of the Western Dvina River Basin, the discharges were observed below the long-term mean only in 5 years during 1945–2015. The deviations varied by 2–62% near Vitebsk and 1–48% near Polotsk. The lowest discharge values were documented for 2014 and 2015 and were connected with an outstanding drought, which lasted for several consequence months. The streamflow in 2014 was extremely low with discharge deviations comprised 60% near Velizh and Vitebsk and 46% near Polotsk. The historical extremes of warm low-water period were exceeded in 2014. However, during the 1985–2015 period in 25 years of 30, the annual discharges within the river length were higher than the mean values by 3–60%. The increase of annual discharges during the last decades is connected with the increase of winter streamflow due to frequent thaws and extra water supply in the winter season. At the same time, the spring runoff became lower due to a smaller amount of snowmelt water being available for spring floods.
Intra-Annual Distribution of Streamflow
The intra-annual distribution of streamflow in the Western Dvina River Basin is characterized by low streamflow in winter (December to February), when runoff rate constitutes about 15% of the annual total, spring (March to May) when snowmelt runoff corresponds to 50–55% of this total. During the summer (June to September), the rate of streamflow varied within 15–20% of the annual total and the same streamflow rate is in autumn (October to November). The differences in the rates of intra-annual distribution by the river length are small and varies within 1–3% (Figure 8).
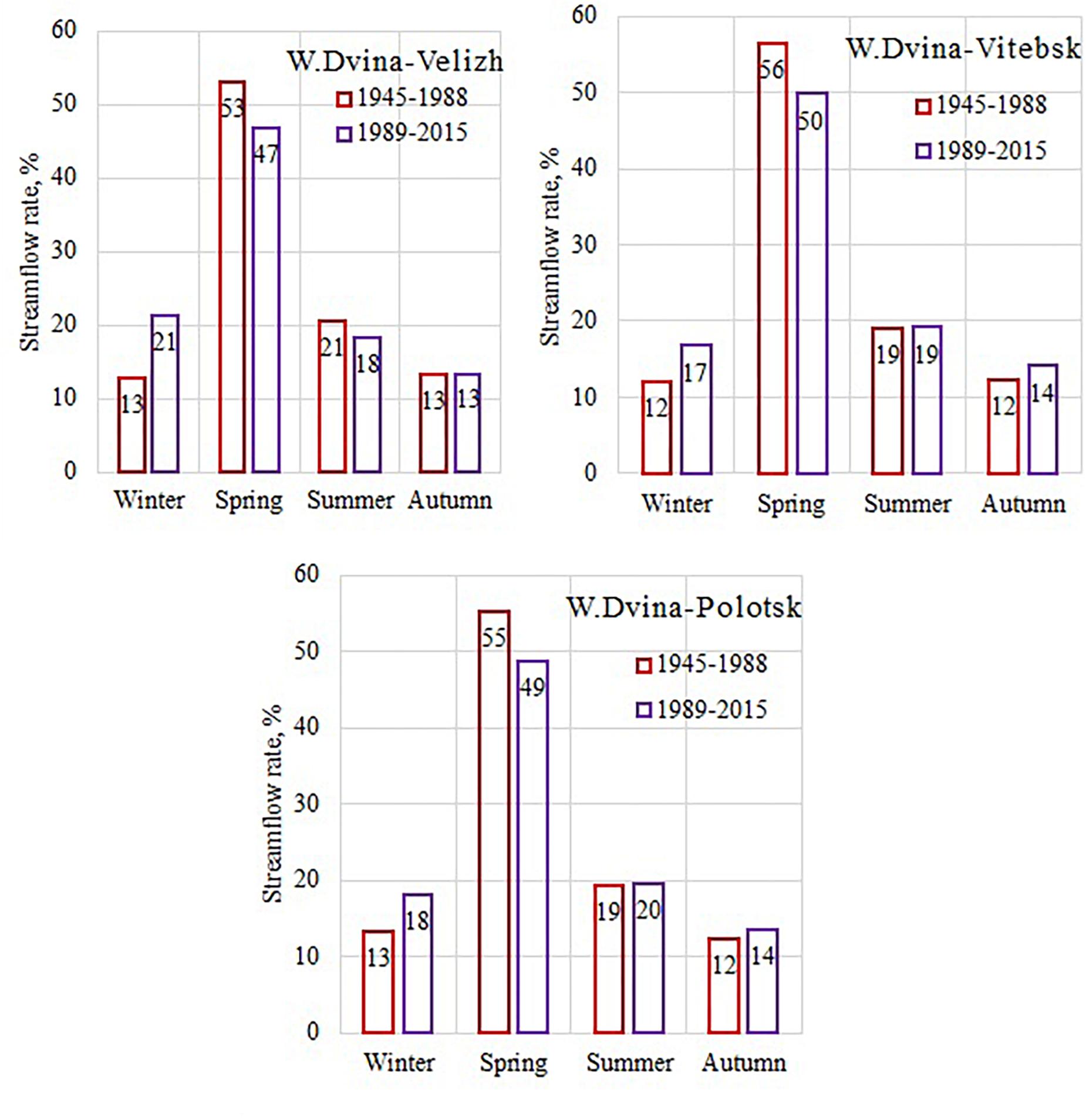
Figure 8. The intra-annual streamflow distribution (percentage) on the Western Dvina River at the Velizh, Vitebsk, and Polotsk gauging stations.
During the last decades, when compared to the previous period, the intra-annual distribution has changed over the entire the Baltic Sea Basin (BACC Author Team, 2008, 2015). There is a large area with similar trends in the intra-annual distribution change in the eastern part of the Baltic Sea Basin, which is observed in the river basins of Western Dvina (Belarus and Russia), Velikaya, Luga, Narva, and Svir‘ (Russia). These changes connected with the growth in the frequency of winter floods and the increase of the values of minimum discharges during the winter low-water periods due to higher air temperatures and an additional inflow of melting water to the rivers (Partasenok et al., 2014; Zhuravlev et al., 2017). Low values or even the absence of snow and ice covers on the rivers and the ground and the low depth of seasonal ground freeze (Nekrasova, 2004) exhaust the water supply before the freshet onset and make conditions for large floods less probable. The differences in the intra-annual distribution at the major gauging stations were tested for significance by t-test, which showed the significant differences (at the significance level values, α = 0.05) in winter and spring flows, while for summer-autumn periods no significant changes were found.
Extreme Characteristics During Spring Flood
The Western Dvina is a typical flat terrain river. The river is supplied mainly by the snow cover melt accumulated during the cold period. This is the reason for relatively high spring flood events observed every year. The flood season usually occurs within just two months: most often, it begins at the end of March (on the main river and in the middle of March on tributaries) and ends in early June when water declines. During the spring snowmelt time, the river level often rises sharply because of the jams.
During the period of remarkable climate change observed in the study region since 1989 up to date, the rise of winter air temperatures and frequent thaws were documented. That is the reason for the earlier flood season start that has shifted to the earlier dates by 4–12 days. At that period, the maximum flood levels in the Belarusian part of the Western Dvina River Basin decreased by 30 to 56 cm (Danilovich et al., 2017). The peaks of spring flood usually observed in the beginning or in the middle of April. The end of spring flood normally occurs in the middle or end of May.
The maximum discharges during spring flood changed during the last 70 years. During two decades, 1945–1954 and 1955–1964, the highest values of spring floods were documented (Figure 6). These decades are characterized by severe winter weather conditions and the formation of sufficient snow-water supply prior to the spring season. This caused an intensive inflow of snowmelt water into the River and its rapid water level rise. In the study river basin, a catastrophic inundation with a 1% probability of occurrence (with frequency once per 100 years) occurred in 1956. This was a highest spring flood in the Western Dvina River Basin and in many other rivers within neighboring regions of Russia and Belarus. The lowest values of maximum discharges during spring flood were observed during the last decades (1985–2015). This is connected with climate warming, which caused the intra-annual distribution change and decrease of the spring runoff rate.
The maximum SDs (a quantity of water which derived from a unit area in a drainage basin in the given time interval, SD) are noticed for small rivers. These rivers are often tributaries of the second order), which are usually located in the right bank of the river or upstream where relief has a higher amplitude of absolute heights and more water is accumulated in the relief depressions (Figure 9).
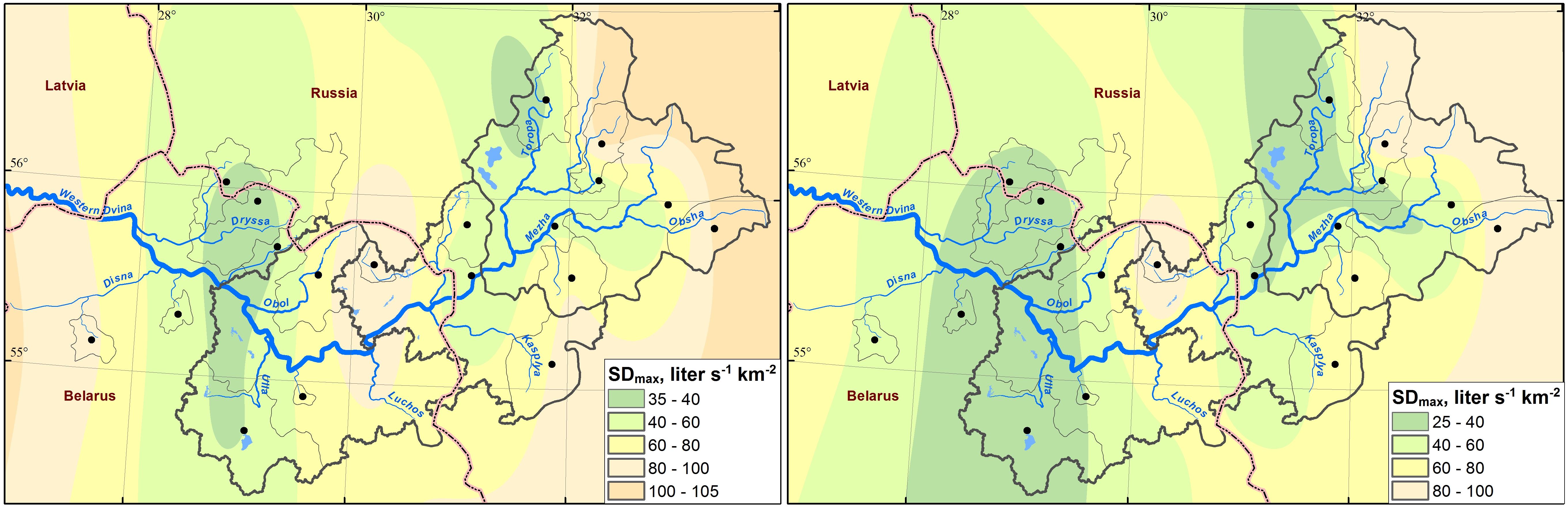
Figure 9. Decadal variability of maximum specific discharges (liter s–1 km–2) on the Western Dvina River and its tributaries.
The maximum SDs were observed on the Western Dvina River near Velizh in 1958 and exceeded the average by 59% (82 liter s–1 km–2). Vitebsk gauging station registered the highest discharge in 1931 (153 liter s–1 km–2), while at Polotsk this extreme was reported in 1956 (97 liter s–1 km–2). At that time, discharges exceeded twofold the average values. There were catastrophic floods in 1931 and 1956 with the 1% probability over large area of the eastern part of the Baltic Sea Basin.
Jams
The formation of jams is a quite frequent event in the Western Dvina River Basin. During the last few decades, the frequency of jams in autumn and winter has increased. For example, according to the data from the Polotsk gauging station on the Western Dvina River, the frequency of jams in the cold season was one time per 17 years during the 1937–1970 period, and one time per 5 years during the 1971–2015 period. The rise of the jam frequencies is connected with a shift of freeze-up dates toward more late dates and with the processes of ice formation which occur inside the water and cause intensive formation of the studge ice and, as a consequence, the jam formation. The duration of jams has increased from 3 days on average during the 1937–1970 period to 20 days every year in the 1971–2015 period.
The spring jams occurrence on the Western Dvina River reduced during the last decades from one time per 2.5 years to one time per 3.5 years. Meanwhile, the highest jam levels during autumn-winter and spring periods has increased.
Low-Flow Periods
The hydrological regime of the Western Dvina River is characterized by low-flow periods in cold (winter) and warm (summer to autumn) seasons. The water supplying during the low-flow period comes from underground water storages. The cold season low-flow period starts with hydrological winter with the ice phenomena formation in November to December (in the last decades, often in January due to the winter temperature rise). This period ends when spring floods start in the end of March and beginning of April. Thereafter, intensive and rapid increases of the river level due to rainfalls occur often during the summer low-flow period.
The dynamic of minimum discharges of cold and warm periods is presented in Figure 10. The dynamic of streamflow in the Western Dvina River Basin in the warm period strongly depends on precipitation and varied about the norm during the study period. The lowest discharges were observed in 1964–1980, 1991–1995, and 2014–2015. The lowest minimum discharge of the cold season depends on precipitation and winter air temperature. The increase of the air temperature in winter and frequent thaws in the Western Dvina River Basin led to immediate snowmelt, extra water supplies into the rivers and formation of winter floods. That is the reason of rising of the minimum discharge during the cold season in the Western Dvina River and its tributaries. The low flow changes in cold period are significant for all rivers in Belarus and northwest of Russia, including the Western Dvina River Basin.
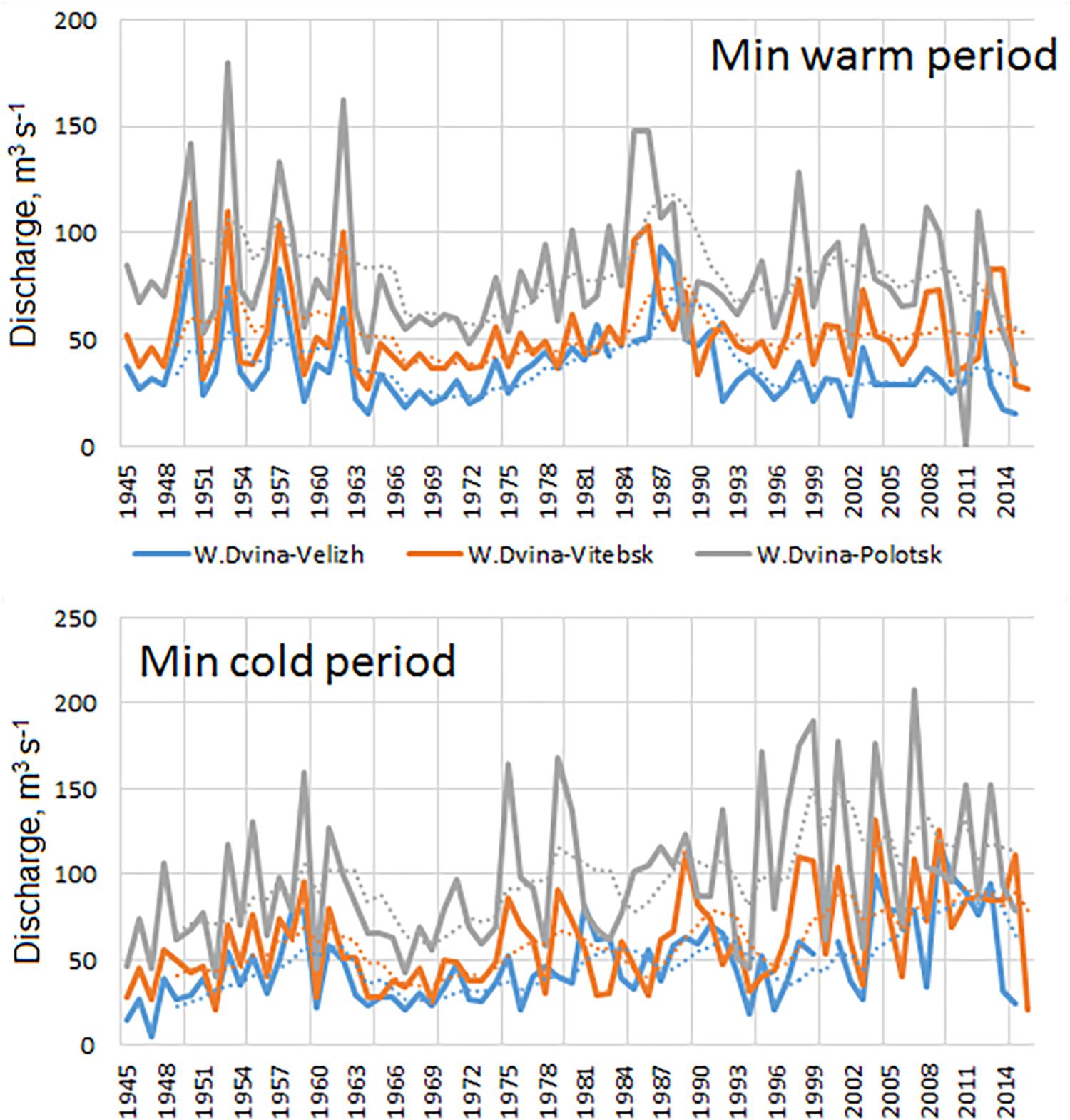
Figure 10. The dynamics of low-flow annual discharges in the Western Dvina River in warm and cold seasons (solid lines) and smoothed by 5-year filter (dotted lines).
The smallest values of minimum discharge were registered in Velizh in 2002 and were equal to 15.1 m3 s–1 in the warm period and in 1947 equal to 4.9 m3 s–1 in the cold period. In Vitebsk, the smallest values of minimum discharge were registered in 1964 (equal to 27 m3 s–1) in the warm season and in 1952 (equal to 21.2 m3 s–1) in the cold season respectively. In Polotsk, lowest discharges were observed in 1952 and 2014 when they were equal to 39.5 m3 s–1 in the warm period.
Usually the winter minimum discharge and level have the lowest values each year. During the last decades in the study area, the frequency of cases when annual minimum level became in the summer season increased strongly. It is connected with growth of winter streamflow rates in the hydrological cycle caused by climate warming and increase of drought frequency in the summer period. Figure 11 represents the dynamics of the cases when summer minimum level exceeded the winter minimum level. The decrease of summer level is stronger in the upper stream of the Western Dvina River (cf., at the Velizh gauging station).
Trends
For the present study, we estimated trends of monthly and annual discharges, maximum discharge during the spring flood period, and minimum discharges during the winter and summer low-water periods.
The analysis of monthly runoff for the 1945–2015 period showed statistically significant trends (at the 0.05 significance level) for January through April at three gauging stations, and in December for Vitebsk and Polotsk stations (Table 3). The trend values are positive in winter months and March and become negative in April. The positive trends in December to March (during the hydrological winter) are related to a general increase of air temperature in the study region, frequent thaws, absence of ice cover and inflow of snowmelt water into the rivers. The negative trends in April might be explained by exhausted snow storage after warm winters that supply rivers with the meltwater. There are no significant trends for other months.
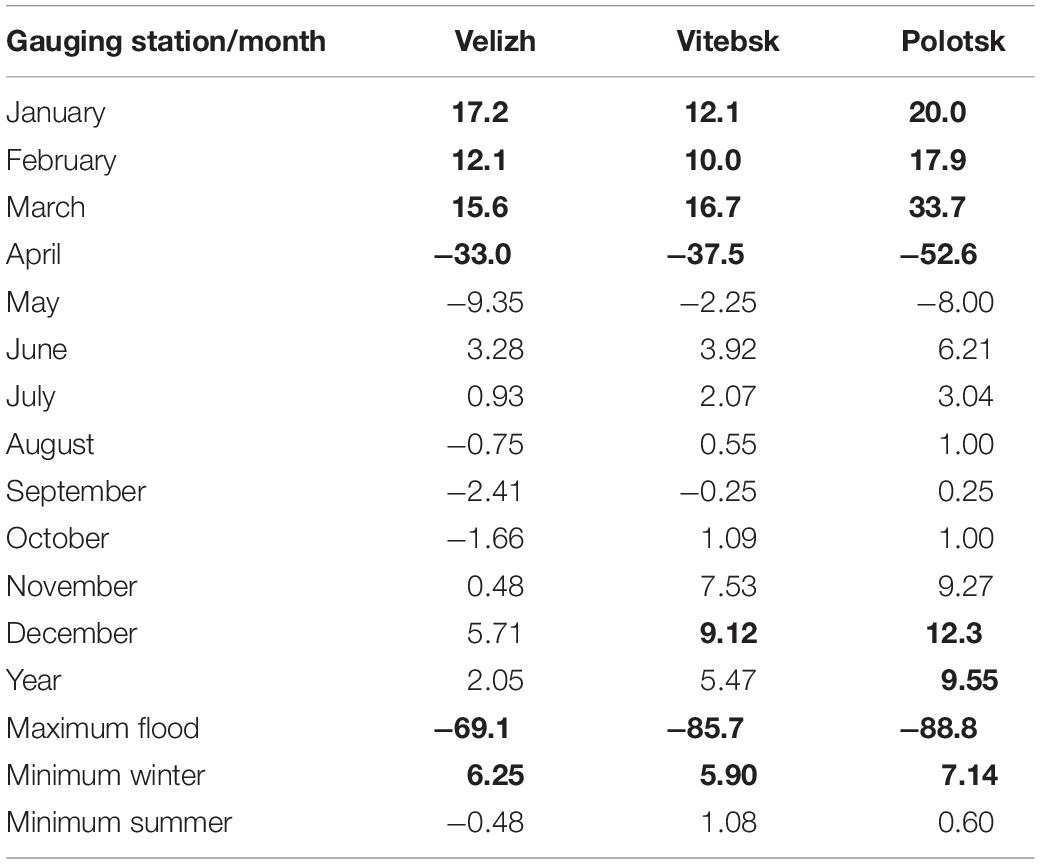
Table 3. The monthly, annual, and extreme streamflow trends (m3 s–1 per 10 years) and their significance (estimates that are significant at the significance levels from 0.001 to 0.05 are shown in bold).
The significant negative trends of the spring flood peak discharges were established for all three major Western Dvina gauging stations: Velizh, Vitebsk and Polotsk. The streamflow change estimates vary within 69–89 m3 s–1 per decade with the largest values in the downstream of the study river basin and the smallest trend values in the upper stream of the Basin.
The minimum discharge trends during the winter low-water period are statistically significant and positive in Velizh, Vitebsk and Polotsk and vary within the 5.9–7.1 m3 s–1 range. There are no significant changes the minimum discharges during the summer low-water period.
The annual discharges trends are significant only at the Polotsk gauging station, where largest changes of monthly and extreme values were observed.
Projected Climate Changes in the Study Region
According to model projections, the average annual mean values of air temperature will gradually increase in the study region. According to scenario RCP4.5 in 2021–2040, deviations of annual air temperature will fluctuate around + 1.4°C. Thereafter by 2060, the deviations will increase up to + 2.7°C. At the end of the 21st century, the greatest value of annual air temperature is expected to increase by 2.8°C. According to scenario RCP8.5, annual deviations of air temperature are expected to be + 1.5°C in the next decade (2021–2030). In the middle of the century (2051–2060), they will reach + 2.5°C, and by the end of the century (2091 to 2100), these deviations will increase up to + 4.7°C. It should be noted that the standard deviation of the air temperature projections remains within the 0.5–1.0°C range that testifies for the coherence of climatic models’ calculations in ensemble.
Intra-seasonal distribution of air temperature deviations during the considered period is characterized by heterogeneity of changes in the cold and warm half of the year for all scenarios of radiative forcing. For scenario RCP4.5 during the winter season, deviations are expected to be positive in all decades, but a noticeable increase is predicted anomaly in the first two decades: up to + 1.5 – + 2.6°C. These anomalies will probably remain at this level up to the middle of the 21st century, and during the 2061–2070 period they will stabilize at 2.5°C. In the next two decades, deviations of annual air temperature will slightly increase by +3.3 to + 3.5°C and to the end of the century will again decrease to +2.8°C. According to scenario RCP8.5, temperature deviations during the winter season in the first half of the 21st century will be probably close to calculated values according to scenario RCP4.5 (+ 1.6 – + 2.5°C), and during several following decades they will be even slightly lower. However, since 2061, an intensive increase in air temperatures is expected in comparison to the previous decades, and these deviations are expected to be within + 4.5 – + 6.1°C.
The spring season is characterized by gradual increase in air temperature; however, the deviation values are expected to be less than in winter. At scenario RCP4.5, deviations of air temperature will probably increase slightly during the 21st century from + 1.0 to + 2.6°C. At scenario RCP8.5, the gradual growth of deviations is very likely occurred in the first half of the century within the 1.4–2.5°C range, and more intensive growth in the second half of century is expected to be within the 3.0–4.5°C range.
The summer season probably will be characterized by similar distribution of deviations by decades; however, their values will be lower than in spring. At scenario RCP4.5, deviations of air temperature will reach + 1.0 – + 2.0°C. At scenario RCP8.5, their values will gradually increase from + 0.8°C (2021 to 2030) to + 4.0°C by the last decade of the current century.
Autumn season are characterized by the smallest values of deviations for scenario RCP4.5. Their values are expected to be below 2.0°C in the first half of the century and then their decrease to + 0.9 – + 1.1°C is expected (2051 to 2091). Finally, for the last decade of the century, an increase up to + 2.2°C is expected.
According to climatic models’ ensemble calculations, decadal future changes of precipitation within the Western Dvina River Basin are highly uncertain. Dynamics of annual precipitation totals under scenario RCP4.5 is also characterized by considerable uncertainty. Negative deviations of annual precipitation totals are noted for periods 2041–2050 and 2081–2090. In other decades, mostly positive deviations of annual precipitation are expected but all of them are small and, probably, range from five up to seven mm. These deviations are statistically insignificant at the 0.05 statistical significance level. Annual precipitation totals anomalies will fluctuate for the most of the 21st century within + 10 to + 15 mm without rapid deviations. The largest values of annual precipitation anomalies are expected to be within + 20 to + 25 mm.
For scenario RCP8.5, the deviations of the annual precipitation totals are more coherent within ensemble and varies from + 3 to + 16 mm by the 2091–2100 period. However, the majority of these precipitation deviations are insignificant at the 0.05 statistical significance level. Deviations of precipitation totals are expected to be within 10 to 30 mm, the greatest within 21–42 mm. At the same time, a gradual increase in precipitation deviation values during the 21st century is noted.
The seasonal distribution of precipitation deviations is characterized by the greatest values during the winter and spring seasons. During the winter season under scenario RCP4.5, precipitation deviations will probably vary within the + 20 to + 28 mm range during the 2031–2100 period. For scenario RCP8.5, seasonal sums of winter precipitation will grow by consecutive decades from 8 mm (2021–2030) to 50 mm at the end of the century. Spring deviations are expected to be practically within the same range. For scenario RCP4.5, precipitation totals will increase by about 15–31 mm and for scenario RCP8.5 precipitation deviations will grow from + 14 to + 50 mm (2081–2090).
Summer precipitation changes according to both scenarios of radiative forcing (RCP4.5 and RCP8.5) is reported with large uncertainties. According to model calculations, it is impossible to define further dynamics of deviations of seasonal summer precipitation. We can only assume that it will be within the variations of seasonal norms.
The autumn season does not express tendencies to increase or decrease. However, autumn precipitation deviations are positive in most cases and probably will be within + 5 to + 24 mm. The differences between deviations for two scenarios are small, especially in the first half of century. The greatest autumn deviations are expected for scenario RCP8.5 for the periods 2041–2050, 2061–2080, and 2091–2100.
Projected Hydrological Changes
Assessments of expected runoff regime of the Western Dvina River were conducted for the 2021–2100 period. The following variables were analyzed: annual flow (Qmean), yearly maximum (which usually corresponds to spring flood peaks, Qmax) and minimum discharges (which usually is observed during the winter or summer low-flow periods, Qmin).
It is projected that during 2021–2100, annual discharges will not change dramatically according to both scenarios – RCP4.5 and RCP8.5 (Figure 12A). There are no significant decadal trend estimates in annual discharges. Thus, the annual streamflow, as well as in the last decades, presumably will vary mostly near its present climatological values (i.e., near “norms”). According to scenario RCP4.5, the decadal streamflow changes will vary within a narrow range and will not exceeded ± 10–12% at the Velizh gauging station mainly with a negative anomaly sign (except the nearest decade, when decrease of annual streamflow up to 21% is expected). At the Vitebsk gauging station, no sizeable decadal changes are expected. However, some increase up to + 12% is expected in the 2071–2080 and 2091–2100 decades. The largest positive changes in projected streamflow were found for the Polotsk gauging station. Here, changes within + 6 to + 11% in the first half the current century and within + 21 to 36% since 2061 are projected up to the end of the study period. According to scenario RCP8.5, decadal streamflow changes at the Velizh gauging station will be less than historical norm by 11–22% with the largest changes projected for last two decades of the 21st century. At the Vitebsk station, decadal changes will not exceed ± 10%. At the Polotsk gauging station, the decadal changes will exceed the historical norm by + 11 to + 26% with maximum increase in the 2041–2070 period.
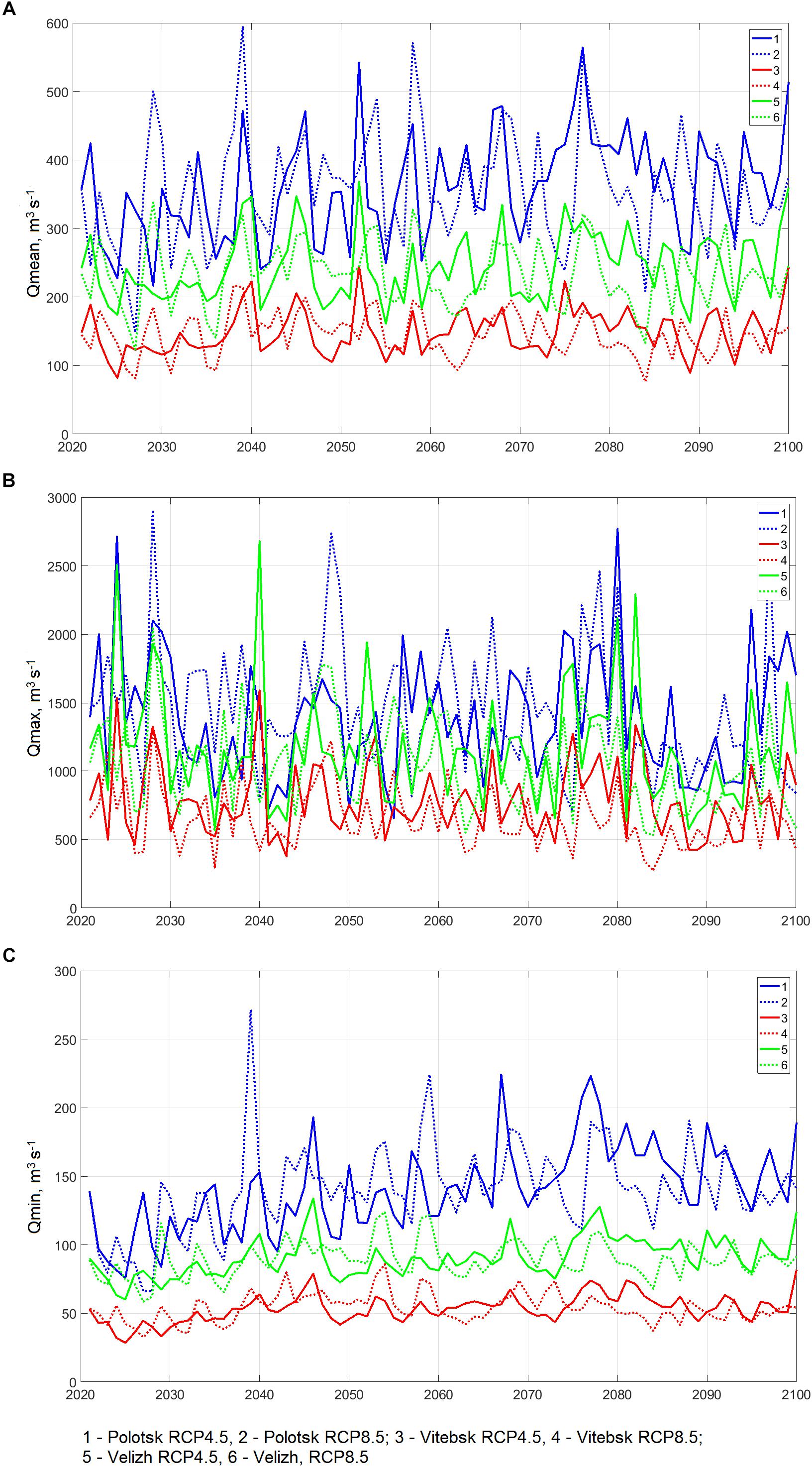
Figure 12. Projected annual (A), maximum (B), and minimum (C) streamflow on the Western Dvina River during the 2021–2100 period.
Projections of maximum streamflow show a general decrease of spring flood discharges (Figure 12B). However, according to RCP4.5 scenario in the nearest decade (2021–2030), the increase of maximum streamflow on 10 to 11% is projected for all three major gauging stations. At the Velizh station during the period of 2021–2100, the maximum streamflow will vary within the norm values without steady positive or negative deviations. At the Vitebsk station, the decadal changes of maximum streamflow will be mostly negative. It will vary within 8–24% with decrease by up to 20–24% in 2041–2050 and 2081–2090. At the Polotsk station, the decadal change will be more notable with decrease less than the historical norm by 11–26%. The smallest flood discharges in are anticipated during the 2031 to 2050 and 2081 to 2090 decades. According to RCP8.5 scenario, the decadal change in spring floods regime will be stronger. The deviations of maximum streamflow are expected to decrease by up to 5–42% for all three major gauging stations. The largest values of decrease will be in 2081–2090.
The minimum streamflow will be changed more remarkable (Figure 12C). The general increase of minimum discharges was projected for both scenarios at all gauging stations. The average increase of minimum discharges is expected to be 60–90%. In Velizh, it will be by 20–78%, in Vitebsk by 66–121%, and in Polotsk by 43–139%. The smallest increase is expected to be in the nearest decade (2021–2030) and the largest in the 2071–2090 decade.
Summary
The current changes of monthly and extreme streamflow in the upper and middle part of the Western Dvina River Basin correspond to a general pattern of hydroclimatic changes in the Baltic Sea Basin occurred during recent decades.
The fluctuations of the streamflow during the period of 1945–2015 are characterized by regional peculiarities of the moistening regime. The smallest monthly discharges were observed in the 1960–1980s of the previous century. During the 1985–2015 period in most of the years, the discharges were higher than the average values by 3–60% along the entire River length. The increase of yearly discharges during the last decades is connected with an increase of winter streamflow due to frequent thaws and extra winter water supply into the River.
During the last decades, the intra-annual distribution in the upper and middle part of the Western Dvina River Basin has changed, the streamflow increased by 6–8% in winter and decreased by 5–6% in spring, in summer-autumn period the changes of streamflow vary within ±3%.
The following seasonal peculiarities have been noticed for the study river basin:
Winter. Presently, due to air temperature growth and frequent thaws, winter floods occurred more often. During the last decades, the frequency of jams in autumn and winter has increased by 17 days per year. Furthermore, the highest jam’s levels during the autumn-winter and spring periods have increased.
Spring. During the period of 1945–1964, the highest values of spring flood were documented, while their smallest values were observed during the 1989–2015 period.
The largest values of maximum SDs are noticed for small rivers (often tributaries of the second order) which are usually located along the right bank of the main river or upstream, where relief has a higher amplitude of absolute heights and more water is accumulated in the relief depressions.
Summer. The lowest discharges were observed in 1964–1980, 1991–1995, and 2014–2015 decades; these are explained by the prevalence of the drought conditions in the Basin.
During the last decades, the frequency of years, when minimum annual discharge was observed in the summer season, significantly increased.
Trends. The analysis of monthly runoff for the 1945–2015 period showed significant positive trends for December through March monthly discharges and minimum winter’s discharges; significant negative trends were found for April monthly discharges and maximum flood discharges. The trends slopes increase from the upper to middle part of the Basin, which can be explained by a closer location of the middle part of the Basin to the Atlantic Ocean and more significant influence here by climate warming.
Projected Changes in Climate. According to both scenarios of radiative forcing, the air temperature will increase gradually in the study region. According to the RCP 4.5 scenario, projected increases of annual air temperature will be by +1.4 to +2.8°C and under the RCP8.5 scenario they will be up to + 4.7°C. According to models’ simulations for all scenarios of radiative forcing, the intra-seasonal distribution of air temperature deviations during the 21st century is characterized by heterogeneity for the cold and warm halves of the year. The largest increase of air temperature is expected in the cold season.
The dynamics of annual precipitation totals under the RCP4.5 scenario is characterized by considerable uncertainty. For scenario RCP8.5, the deviations of annual precipitation totals are more coherent across the model ensemble and vary from + 3 to + 16 mm for the 2091 to 2100 period. At the 0.05 statistical significance level, most of projected precipitation deviations are insignificant.
Projected Changes in Streamflow. Future hydrological regime within the Western Dvina River is expected to change. The projected hydrological changes are stronger for the climate projections under the RCP8.5 scenario. The annual streamflow is not expected to change, with its decadal changes being varied around the “norm” values (except the Polotsk gauging station, where n the second half of the current century an increase of annual streamflow by 21–36% is expected). The spatial pattern of the projections will be characterized by mostly negative runoff changes in the upper stream and positive runoff changes in the lower part of the study river basin. Projected maximum streamflow showed a general decrease with the largest changes in the lower part on the River Basin (Vitebsk and Polotsk; up to 25% of decrease). However, despite a general decrease of spring runoff, slight increases of maximum discharges (by up to + 10%) are projected for the nearest decade (2021–2030).
Projected minimum streamflow showed the largest changes. The minimum discharges are expected to be increased by 60–90% on average for the 2021–2100 period with largest changes being expected in the lower part of the study river basin.
Author Contributions
SZ and ID designed the idea of the study. ID prepared the introduction and methodological part, provided the historical analysis, prepared the simulated climate data to force the hydrological model, and wrote the main part and conclusion. SZ set the hydrological model, prepared the historical hydrological data, calibrated and run the model, and generalized the simulated hydrological data. LK prepared the historical climate and hydrological data, provided the calculations, and prepared the graphs. PG improved the climate data generalization approach, corrected the statistical analyses throughout the manuscript, and edited the text.
Funding
The studies of ID were conducted under the subprogram 1 “The Nature Resources and Ecological Safety” of the State Research Program during 2016–2020 “The Nature Management and Ecology.” The researches of SZ and LK were carried out with the financial support of the RGO-RFBR grant under Project No. 17-05-41118. The support for PG studies was provided by the ARCTIC-ERA Project within the Belmont Forum Initiative.
Conflict of Interest Statement
PG was employed by the company Hydrology Science and Services Corporation, Asheville, NC, United States.
The remaining authors declare that the research was conducted in the absence of any commercial or financial relationships that could be construed as a potential conflict of interest.
Acknowledgments
We acknowledge the E-OBS dataset from the EU-FP6 project ENSEMBLES (http://ensembles-eu.metoffice.com) and the data providers in the ECA&D project (http://www.ecad.eu). We thank the reviewers and the editor for their valuable comments, which helped to improve the manuscript.
References
Apsīte, E., Bakute, A., Elferts, D., Kurpniece, L., and Pallo, I. (2011). Climate change impacts on river runoff in Latvia. Clim. Res. 48, 57–71. doi: 10.3354/cr01004
Arheimer, B., and Lindström, G. (2015). Climate impact on floods: changes in high flows in Sweden in the past and the future (1911–2100). Hydrol. Earth Syst. Sci. 19, 771–784. doi: 10.5194/hess-19-771-2015
BACC Author Team, (2008). Assessment of Climate Change for the Baltic Sea Basin. Berlin: Springer-Verlag 474.
BACC Author Team (2015). Second Assessment of Climate Change for the Baltic Sea Basin. Cham: Springer International Publishing, 501.
Beldring, S., Engen-Skaugen, T., Førland, E. J., and Roald, L. A. (2008). Climate change impacts on hydrological processes in Norway based on two methods for transferring regional climate model results to meteorological station sites. Tellus A Dyn. Meteorol. Oceanogr. 60, 439–450. doi: 10.1111/j.1600-0870.2007.00306.x
Danilovich, I. S., Nahibina, M. E., Zhuravovich, L. N., and Kvach, E. G. (2017). The peculiarities of hydrological regime during last decades within the territory of Belarus. Nat. Re. 2, 5–12. [In Russian]
Döll, P., and Schmied, M. H. (2012). How is the impact of climate change on river flow regimes related to the impact on mean annual runoff? A global-scale analysis. Environ. Res. Lett. 7, 14037–14011. doi: 10.1088/1748-9326/7/1/014037
Donnelly, C., Greuell, W., Andersson, J., Gerten, D., Pisacane, G., Roudier, P., et al. (2017). Impacts of climate change on European hydrology at 1.5, 2 and 3 degrees mean global warming above preindustrial level. Clim. Change 143, 13–26. doi: 10.1007/s10584-017-1971-1977
Frolova, N. L., Agafonova, S. A., Kireeva, M. B., Povalishnikova, E. S., and Pakhomova, O. M. (2017). Recent changes of annual flow distribution of the Volga Basin Rivers. Geogr. Environ. Sustain. 10, 28–39. doi: 10.24057/2071-9388-2017-10-2-28-39
Gailiušis, B., Kriauèiûnienë, J., Jakimavièius, D., and Šarauskienë, D. (2011). The variability of long-term runoff series in the Baltic Sea drainage basin. Baltica 24, 45–54.
Georgievskiy, V. Y., Grek, E. A., Grek, E. N., Lobanova, A. G., and Molchanova, T. G. (2018). Spatiotemporal changes in extreme runoff characteristics for the volga basin rivers. Russ. Meteorol. Hydrol. 43, 633–638. doi: 10.3103/s1068373918100011
Gupta, H. V., Sorooshian, S., and Yapo, P. O. (1999). Status of automatic calibration for hydrologic models: comparison with multilevel expert calibration. J. Hydrol. Eng. 4, 135–143. doi: 10.1061/(ASCE)1084-069919994:2(135)
Hansson, D., Eriksson, C., Omstedt, A., and Chen, D. (2011). Reconstruction of river runoff to the Baltic Sea, AD 1500–1995. Int. J. Climatol. 31, 696–703. doi: 10.1002/joc.2097
Haylock, M. R., Hofstra, N., Klein Tank, A. M. G., Klok, E. J., Jones, P. D., and New, M. (2008). A European daily high-resolution gridded dataset of surface temperature and precipitation. J. Geophys. Res. 113:D20119. doi: 10.1029/2008JD010201
Hisdal, H., Holmqvist, E., Jonsdottir, J. F., Jonsson, P., Kuusisto, E., Lindstrom, G., et al. (2010). Has Streamflow Changed in the Nordic Countries? NVE Report No. 1. Oslo.
Jeppesen, E., Kronvang, B., Meerhoff, M., Sondergaard, M., Hansen, K. M., Andersen, H. E., et al. (2009). Climate change effects on runoff, catchment phosphorus loading and lake ecological state, and potential adaptations. J. Environ. Qual. 38, 1930–1941. doi: 10.2134/jeq2008.0113
Kıaviņš, M., and Rodinov, V. (2008). Long-term changes of river discharge regime in Latvia. Nord. Hydrol. 39, 133–141. doi: 10.1007/s10661-011-2315-0
Kıaviņš, M., Rodinov, V., Timukhin, A., and Kokorîte, I. (2008). Patterns of river discharge: long-term changes in Latvia and the Baltic region. Baltica 21, 41–49. doi: 10.1007/s10661-011-2315-0
Kriauciuniene, J., Meilutyte-Barauskiene, D., Reihan, A., Koltsova, T., Lizuma, L., and Sarauskiene, D. (2012). Variability in temperature, precipitation and river discharge in the Baltic states. Boreal Environ. Res. 17, 150–162.
Kriaučiūnienė, J., Meilutyte-Barauskiene, D., Rimkus, E., Kazys, J., and Vincevicius, A. (2008). Climate change impact on hydrological processes in Lithuanian Nemunas river basin. Baltica 21, 51–61.
Krylenko, I., Motovilov, Y., Antokhina, E., Zhuk, V., and Surkova, G. (2015). Physically-based distributed modelling of river runoff under changing climate conditions. Proc. Int. Assoc. Hydrol. Sci. 368, 156–161. doi: 10.5194/piahs-368-156-2015
Loginov, V., Volchek, A. A., and Volchek, An. A (2014). Spring Floods on the Rivers of Belarus: Spatio-Temporal Fluctuations and Forecast. Minsk: Belarusian Science.
Lopukh, P. S., and Partasenok, I. S. (2014). Influence of Atmospheric Circulation on the Formation of the Hydrological Regime of the Rivers of Belarus. Minsk: Belarus State University.
Makarieva, O. M. (2018). “Certificate of state registration of the computer program No. 2018619084,” in Complex Program of the Distributed Hydrological Model “Hydrograph”, ed. O. M. Makarievacpesnm, (Rospatent: Moscow).
Meinshausen, M., Smith, S. J., Calvin, K., Daniel, J. S., Kainuma, M. L. T., Lamarque, J.-F., et al. (2011). The RCP greenhouse gas concentrations and their extensions from 1765 to 2300. Clim. Change 1, 213–241. doi: 10.1007/s10584-011-0156-z
Moriasi, D. N., Gitau, M. W., Pai, N., and Daggupati, P. (2015). Hydrologic and water quality models: performance measures and evaluation criteria. Am. Soc. Agric. Biol. Eng. 58, 1763–1785. doi: 10.13031/trans.58.10715
Nash, J. E., and Sutcliffe, J. V. (1970). River flow forecasting through conceptual model. Part 1-A discussion of principles. J. Hydrol. 10, 282–290. doi: 10.1016/0022-1694(70)90255-6
Nasonova, O. N., Gusev Ye, M., Volodin, E. M., and Kovalev, E. E. (2018). Application of the land surface model SWAP and global climate model INMCM4.0 for projecting runoff of northern russian rivers. 1. Historical simulations. Water Resour. 45(Suppl. 2):73. doi: 10.1134/S009780781806026X
Nekrasova, L. I. (2004). “Influence of climate warming on hydrological regime of rivers in Belarus during 1988-2002,” in Proceedings of XXIII Nordic Hydrological Conference: Fresh Water Resources Management, Tallinn, 691.
Olsson, J., Yang, W., Graham, L. P., Rosberg, J., and Andreasson, J. (2011). Using an ensemble of climate projections for simulating recent and near-future hydrological change to lake Vanern in Sweden. Tellus A 63, 126–137. doi: 10.1111/j.1600-0870.2010.00476.x
Partasenok, I. S., Groisman, P Ya, Chekan, G. S., and Melnik, V. I. (2014). Winter cyclone frequency and following freshet streamflow formation on the rivers in Belarus. Environ. Res. Lett. 9:095005. doi: 10.1088/1748-9326/9/9/095005
Polishchuk, A. I., and Chekan, G. S. (eds) (2009). Hydrological Monitoring of the Republic of Belarus. Minsk: Knigazbor.
Reihan, A., Koltsova, T., Kriauèiûnienë, J., Lizuma, L., and Meilutytë-Barauskienë, D. (2007). Changes in water discharges of the Baltic States rivers in the 20th century and its relation to climate change. Nord. Hydrol. 38, 401–412. doi: 10.2166/nh.2007.020
Roudier, P. H., Andersson, J., Donnelly, C., Luc, F., Wouter, G., and Fulco, L. (2015). Projections of future floods and hydrological droughts in Europe under a +2°C global warming. Clim. Change 135, 341–355. doi: 10.1007/s10584-015-1570-1574
Sanchez, P. A., Ahamed, S., Carré, F., Hartemink, A. E., Hempe, J., Huising, J., et al. (2009). Digital soil map of the world. Science 325, 680–681. doi: 10.1126/science.1175084
Sen, P. K. (1968). Estimates of the regression coefficient based on Kendall’s tau. J. Am. Stat. Assoc. 63, 1379–1389. doi: 10.1080/01621459.1968.10480934
Stahl, K., Hisdal, H., Hannaford, J., Tallaksen, L. M., van Lanen, H. A. J., Sauquet, E., et al. (2010). Streamflow trends in Europe: evidence from a dataset of near-natural catchments. Hydrol. Earth. Syst. Sci. 14, 2367–2382. doi: 10.5194/hess-14-2367-2010
Szwed, M., Karg, G., Pińskwar, I., Radziejewski, M., Graczyk, D., Kêdziora, A., et al. (2010). Climate change and its effect on agriculture, water resources and human health sectors in Poland. Nat. Hazards Earth Syst. Sci. 10, 1725–1737. doi: 10.5194/nhess-10-1725-2010
Thober, S., Kumar, R., Wanders, N., Marx, A., Pan, M., Rakovec, O., et al. (2018). Multi-model ensemble projections of European river floods and high flows at 1.5, 2, and 3 degrees global warming. Environ. Res. Lett. 13:014003. doi: 10.1088/1748-9326/aa9e35
Thodsen, H., Hasholt, B., and Kjarsgaard, J. H. (2008). The influence of climate change on suspended sediment transport in Danish rivers. Hydrol. Process. 22, 764–774. doi: 10.1002/hyp.6652
Veijalainen, N., Lotsari, E., Alho, P., Vehvilainen, B., and Kayhko, J. (2010). National scale assessment of climate change impacts on flooding in Finland. J. Hydrol. 391, 333–350. doi: 10.1016/j.jhydrol.2010.07.035
Vinogradov, Y. B., Semenova, O. M., and Vinogradova, T. A. (2011). An approach to the scalingproblem in hydrological modelling: the deterministic modelling hydrological system. Hydrol. Process. 25, 1055–1073. doi: 10.1002/hyp.7901
Volchek, A., Korneyev, V., Parfomuk, S., and Bulak, I. (2017). Water Resources and Their Forecast According to the Climate Change in the Territory of Belarus. Brest: Alternativa, 228.
Volchek, A. A., and Gryadinova, O. I. (2010). Minimum River Flow in Belarus. Brest: State University named after A.S. Pushkin.
Volchek, A. A., and Parfomuk, S. I. (2013). “Evaluation and forecast of natural water resources of Belarus,” in Modern Energy- and Resource-Saving, Environmentally Sustainable Technologies and Systems of Agricultural Production: Collection of Research Works by, ed. N. V. Byshovacpesnm, (Ryazan: Brest State University of Technology), 434–440.
Volchek, A. A., and Shelest, T. A. (2012). Spatio-temporal fluctuations of rain floods on the rivers of Belarus. News Russ. Acad. Sci. 3, 76–83.
Wilson, D., Hisdal, H., and Lawrence, D. (2010). Has streamflow changed in the Nordic countries? Recent trends and comparisons to hydrological projections. J. Hydrol. 394, 334–346. doi: 10.1016/j.jhydrol.2010.09.010
Yang, W., Andréasson, J., Graham, L. P., Olsson, J., Rosberg, J., and Wetterhall, F. (2010). Distribution-based scaling to improve usability of regional climate model projections for hydrological climate change impacts studies. Hydrol. Res. 41, 211–229. doi: 10.2166/nh.2010.004
Keywords: climate, streamflow, Western Dvina River, model, trend, change scenarios
Citation: Danilovich I, Zhuravlev S, Kurochkina L and Groisman P (2019) The Past and Future Estimates of Climate and Streamflow Changes in the Western Dvina River Basin. Front. Earth Sci. 7:204. doi: 10.3389/feart.2019.00204
Received: 14 January 2019; Accepted: 25 July 2019;
Published: 13 August 2019.
Edited by:
Markus Meier, Leibniz Institute for Baltic Sea Research (LG), GermanyReviewed by:
Jukka Käyhkö, University of Turku, FinlandXander Wang, University of Prince Edward Island, Canada
Copyright © 2019 Danilovich, Zhuravlev, Kurochkina and Groisman. This is an open-access article distributed under the terms of the Creative Commons Attribution License (CC BY). The use, distribution or reproduction in other forums is permitted, provided the original author(s) and the copyright owner(s) are credited and that the original publication in this journal is cited, in accordance with accepted academic practice. No use, distribution or reproduction is permitted which does not comply with these terms.
*Correspondence: Irina Danilovich, aXJpbmEtZGFuaWxvdmljaEB5YW5kZXgucnU=; Sergey Zhuravlev, aHlkcm9tb2RAZ21haWwuY29t