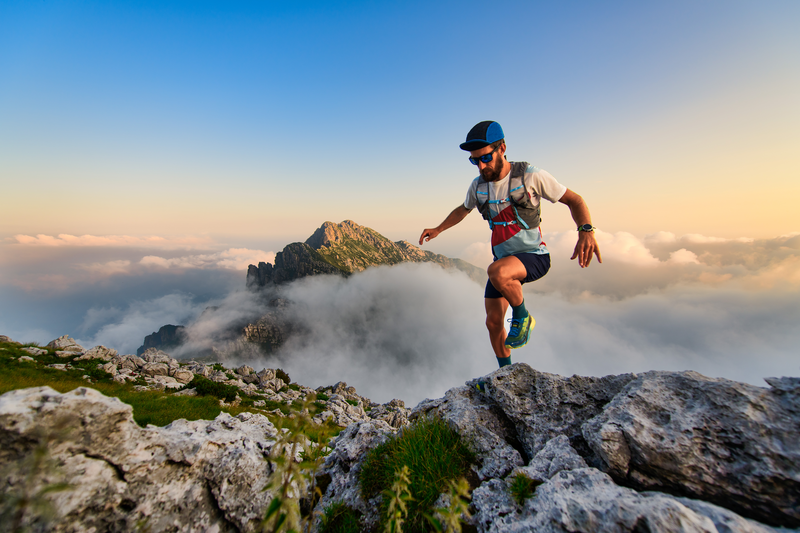
94% of researchers rate our articles as excellent or good
Learn more about the work of our research integrity team to safeguard the quality of each article we publish.
Find out more
ORIGINAL RESEARCH article
Front. Earth Sci. , 03 July 2019
Sec. Atmospheric Science
Volume 7 - 2019 | https://doi.org/10.3389/feart.2019.00142
This article is part of the Research Topic Atmosphere – Cryosphere Interaction in the Arctic, at High Latitudes and Mountains with Focus on Transport, Deposition and Effects of Dust, Black Carbon, and other Aerosols View all 11 articles
In this work we use chemical fingerprints as characteristics ratios of specific crustal elements Ca/Al, Fe/Al, K/Al, Mg/Al, Mn/Al, Ca/Fe, and Mg/Fe to investigate the long-range transport of volcanic aerosols from Iceland. Volcanic dust enters the atmosphere during suspension processes from Icelandic deserts, but mainly from the dust hot spots in remote areas in Iceland, and it is transmitted to the central Balkan area (Belgrade). For this purpose, backward trajectories from Belgrade (φ = 44°48′; λ = 20°28′) in 2012 and 2013, simultaneous with atmospheric aerosols measurements, were calculated by using the Hybrid Single-Particle Lagrangian Integrated Trajectory (HYSPLIT) model. We found that about 17% of air masses passed over Icelandic territory and arrived to Balkan area. In almost all of these episodes ratios of some investigated elements in suburban aerosols of Balkan area corresponded to the ratios of elements investigated in surface soil of the Rangárvellir area – South Iceland in the vicinity of volcanoes. We identified several episodes, such as August 6–8, 2012; June 2–6, 2013; June 26–28, 2013; and September 18–20, 2013; with the characteristic ratios of the highest number of investigated elements in atmospheric aerosol of central Balkan corresponding to ratios from Icelandic soil material. This study provides evidence that Icelandic dust can travel long distances showing the importance of High Latitude Dust sources.
Charles Darwin described the phenomenon he noticed on the sailboat Beagle that the deck and all the equipment were covered with fine dust in the nineteenth century. Also, the sea was covered with a thin layer of dust as long as the sight was visible. This happened when it sailed to the Atlantic coast of West Africa in its famous expedition (Darwin, 1845). Today it is a well-known phenomenon of long-range transport of dust and numerous side effects that have a great impact on the environment. Airborne particles, depending on their aerodynamic radius, can be transported from several hundred to even ten of thousand kilometers (Prospero, 1999; Husar, 2004). This mineral dust mostly comes from natural sources while the anthropogenic effect is far smaller (Mahowald et al., 2004; Tegen et al., 2004a,b). In recent years, increasing attention has been paid to this phenomenon because it has been shown that airborne particles contributing to dust have a major impact on atmospheric and meteorological phenomena through the absorption and scattering of solar radiation, and representing nucleation centers leading to the formation of clouds, rain and ice (Sokolik and Toon, 1999; Arimoto, 2001), while presenting chemical reaction centers for gaseous compounds by changing the mechanisms of chemical reactions in the atmosphere (Andreae and Rosenfeld, 2008). There is also a need to mention the significant degradation of soil from the sources of dust, while the deposition of dust leads to an increase in the nutrients of the soil, and therefore to the increase in the fertility of these areas (Swap et al., 1992). Long-range dust transport affects, to a large extent, animals as well as humans, and it can transmit different pathogens resulting in a direct threat to health (Kellogg and Griffin, 2006). Dust particles with aerodynamic diameter less than 2.5 μm, which mostly contain long-range transport, directly affect human and animal health because they smoothly penetrate the lungs and sensitive alveolar system causing various inflammatory processes, asthma and obstructive pulmonary disease (Prospero et al., 2008; De Longueville et al., 2010). Although this phenomenon of long-range dust transport is generally present, it is necessary to point out that North Africa, more precisely the Sahara, is the largest source of mineral dust, with about 0.8 × 109 tons per year, contributing with 20–70% of global mineral dust (Laurent et al., 2008). In the Sahara region, air currents lead to dust build-ups, which are then transported to the Mediterranean (Rodríguez et al., 2003; Querol et al., 2004) and further north to Arctic regions or west to the Atlantic and there by long-range transport all away across the ocean to the coast of America.
Chemical composition and complex refractive index, chemical and mineralogical particle analysis of airborne dust and size distribution of Sahara dust are well investigated (Kandler et al., 2007, 2009, 2011; Scheuvens et al., 2011, 2013), and the content of numerous mineral species (Illite, Albite, Quartz, Calcite, Dolomite, Halite, Hematite, etc.), as well as the concentration and ratio of cationic and anionic species that represent the “fingerprint” of Saharan dust (Rodríguez et al., 2003; Querol et al., 2004). The same concept is also used to determine the existence of long-range transports from other areas (marine aerosol, large cities, industrial areas, etc.) which can complement the complex picture of transmission and the impact of dust, whether natural or anthropogenic.
Iceland is the largest desert in Europe and Arctic with estimated dust deposition of 31–40 mil tons per year (Arnalds et al., 2014). This can represent about 7% of total global dust emissions and up to 21% of the Saharan dust emissions. About 3% of Icelandic dust is estimated to reach Europe (Groot Zwaaftink et al., 2017). Dust event frequency in Iceland is 34–135 dust days per year on average (Dagsson-Waldhauserova et al., 2014a). Icelandic dust is, however, different to crustal dust such as from Sahara. It is volcanic dust, dark in color, with >75% of volcanic glass with high proportions of FeO, Al2O3, and TiO2 (Dagsson-Waldhauserova et al., 2014b; Arnalds et al., 2016). The identification of Icelandic dust particles transported to Europe, except for volcanic eruptions, is, however, sparse (Ovadnevaite et al., 2009).
Different approaches have been used to identify and characterize source areas of air masses. Backward trajectories are the most commonly calculated type, the HYSPLIT being the most widely used model and particulate matter being the kind of pollutant most frequently investigated (Pérez et al., 2015). The accuracy of trajectory models depends on the source of wind field data, resolution of available meteorological fields, trajectory type, etc (Fleming et al., 2012). Backward trajectories are typically estimated from archived wind field and pressure data and represent the approximate three-dimensional flow path of an air parcel. The HYSPLIT model was used to compute transport, mixing, chemical transformation, and deposition of pollutants and hazardous materials (Stein et al., 2015). It was extensively applied for research of the Fukushima accident and the Eyjafjallajökull volcano eruption, as well as several worldwide investigations of regional to local scale dust and air pollution transport (e.g., McGowan and Clark, 2008; Wang et al., 2010; Cristofanelli et al., 2011; Liu et al., 2013; Draxler et al., 2015; Leelõssy et al., 2017). The HYSPLIT backward trajectories have been frequently used also in long-range dust transportation and Saharan dust intrusions (Hamonou et al., 1999; Varga et al., 2013, 2014).
The main reason why the HYSPLIT model is widely used is that is freely available and prove extremely easy to apply (Pérez et al., 2015). The HYSPLIT model is accessed via web-based Real-time Environmental Applications and Display sYstem (READY) developed by NOAA’s Air Resources Laboratory (ARL) (Draxler et al., 2013).
In this work, we intend to use characteristic ratios of elements as fingerprints for identification of Icelandic volcanic dust particles in central Balkan. In addition, long-range transport of atmospheric aerosols entering the atmosphere by suspension of crustal element material from surface soil and deserts in Iceland is discussed. The large amount of the aerosols samples in suburban central Balkans’ area and surface soil of the Rangárvellir area in Iceland and their analyses done are valuable unique dataset.
Atmospheric aerosols were measured in the suburban area (Figure 1) of Belgrade (φ= 44°48′; λ= 20°28′; 240 m elevation) as 48 h cumulative samples every 6 days, starting at 8 a.m. Low-pressure cascade impactors by Prof. Dr. Berner with vacuum pump with the flow rate of 25 l min–1 was used for sampling (Berner, 1972; Wang and John, 1988). Size segregated aerosols were collected on Tedlar foils with the following stages: PM0.27–0.53, PM0.53–1.06, PM1.06–2.09, PM2.09–4.11, PM4.11–8.11, and PM8.11–16. Samples and blanks were each stored in Petri cups in the freezer (−20°C) until processing.
Figure 1. Map of Europe and sampling sites for atmospheric aerosols: Belgrade ① and the Rangárvellir area ②. https://2.bp.blogspot.com/-LVIaPBN2nvA/ VNECxS7jCPI/AAAAAAAAATc/Whc6_-9OKzc/s1600/Geography%2Bof%2BEurope.jpg.
Surface soil samples were collected in the Rangárvellir area in southern Iceland (63° N; 20° E; 50m elevation) in the vicinity of Mt Hekla, Iceland’s most active volcano and under high erosion processes (Thorarinsdottir and Arnalds, 2012). Soil from this area consists of various materials originating from surrounding volcanoes and lava.
Daily composite means of geopotential heights and wind speed at specific isobaric levels were retrieved from the National Centers for Environmental Prediction/National Center for Atmospheric Research (NCEP/NCAR) reanalysis datasets for the study period (2012–2013).
Samples of atmospheric aerosols were gravimetric measured in a glove box system with controlled nitrogen atmosphere (temperature 20 ± 5 °C and humidity 45 ± 5%). The digestions of collected aerosols were performed using Advanced Microwave Digestion System (ETHOS 1, Milestone, Italy) with HPR-1000/10S high pressure segmented rotor and pressure resistant PTFE vessels. Samples were transfered to the PTFE vessels, using HNO3 (62%, UltraPure, Merck), H2O2 (30%, Sigma-Aldrich) and HF (UltraPure, Merck) and then heated with microwave energy for 50 min. The content of elements in solution samples of atmospheric aerosols was determined by inductively coupled plasma mass spectrometry (ICP-MS), using a Thermo Fisher Scientific iCAP Qc ICP-MS (Bremen, Germany) using EPA Method Standard, Low Level Elements Calibration Stock (10 mg L–1) of elements. Every tenth sample was blank, collected using the same procedure as for the aerosol samples, but without use of the pump to draw air through the filter (Karanasiou et al., 2007; Đuričić-Milanković et al., 2018).
Inductively coupled plasma iCAP-6500 Duo (Thermo Scientific, United Kingdom) with an atomic emission spectrometer was used for determining element concentrations in the extracts of soil from Iceland. Laboratory quality assurance and quality control methods were used in element concentrations determination, including the standard operating procedures, calibration with standards, and analysis of both, reagent blanks and replicates. Elements concentrations are reported in mg kg–1 on a dry weight of soil (Sakan et al., 2016).
The HYSPLIT model is a complete system for computing trajectories complex dispersion and deposition simulations using either puff or particle approaches (Draxler and Hess, 1998). The HYSPLIT continues to be one of the most extensively used atmospheric transport and dispersion models in the atmospheric sciences community (Stein et al., 2015). The HYSPLIT model is widely used to generate backward trajectories in given starting locations (e.g., Rozwadowska et al., 2010; Freitag et al., 2014; Pérez et al., 2015; Su et al., 2015).
Four days backward trajectories were computed to trace the air history by means of the HYSPLIT model. The particle motion is defined as the superposition of a deterministic downwind term (advection) and a stochastic turbulent motion (Draxler and Hess, 1998). The deterministic term is interpolated to each particle position from the wind field provided by an input numerical weather prognostic (NWP) model. Input meteorological data is obtained from analysis of the Global Data Assimilation System (GDAS) data. The GDAS is run operationally 4 times a day (at 00, 06, 12, and 18 UTC) by the NOAA’s National Centers for Environmental Prediction (NCEP). NCEP post-processing of the GDAS converts the data from spectral coefficient form to 1 degree latitude-longitude (360 by 181) grids and from sigma levels to pressure levels. NOAA’s ARL saves the successive analyses and 3-h forecast, four times each day to produce a continuous data archive. The decision to use these data was caused by enhanced data assimilation methods as well as the highest horizontal, vertical and temporal resolution. 4 days backward trajectories were selected because it is sufficient time to determine regional transport pathways. The trajectories were calculated every 1 h from March 14, 2012 to December 26, 2013 for four arrival heights: 500, 1500, 3000, and 5000 m above sea level. The selection of 500 m as the lowest level resulted from the orography around the station. To elucidate the effect of long-range transport, trajectories are analyzed at low and mid tropospheric altitudes, up to 5000 m (e.g., Ogawa et al., 2004; Sangeetha et al., 2018). The trajectories and composite maps are presented for 700 mb level as the most representative HYSPLIT backward trajectory level based on previous analyses of long-range dust transport episodes for Central European and Mediterranean case studies (Hamonou et al., 1999; Varga et al., 2013, 2014).
The contribution of mineral dust from high-latitude sources of the global dust cycle has not been sufficiently investigated yet (Baddock et al., 2017). In the near past there are some works relate to the atmospheric transport of Icelandic volcanic ash during eruption of Eyjafjallajökull (Langmann et al., 2012) as well as volcanic dusts during storm events (Dagsson-Waldhauserova et al., 2015; Taylor et al., 2015; Wilkins et al., 2016) but there are not yet sufficiently investigations relate to volcanic particles in atmospheric aerosols originated from dust storms occurrences in Iceland over continental part of Europe.
In this work we considered next elements Al, Ca, Fe, K, Na, Mg, and Mn and their characteristic ratios as fingerprints for Icelandic volcanic dust in suburban atmospheric aerosols of central Balkan. The contents of elements were shown in Table 1 as distributions of average values with standard deviations, minimum and maximum in suburban aerosol segregated into six Dp fractions for samples measured and collected in Belgrade (Đuričić-Milanković et al., 2018) and for the soil of the Rangárvellir area (Iceland). The main difference between atmospheric aerosols of central Balkan and surface soil of the Rangárvellir area is that dominant mass contribution of Ca is in measured atmospheric aerosol while dominant element in surface soil of investigated area in Iceland is Fe.
Table 1. Average content of elements in atmospheric aerosols of the suburban area of Belgrade (Serbia) and in the soil of the Rangárvellir area (Iceland).
The characteristic ratios that we considered in this work were Ca/Al, Fe/Al, K/Al, Mg/Al, Mn/Al, Ca/Fe, and Mg/Fe. Table 2 presents the averages, standard deviations, minimums and maximums of investigated ratios in atmospheric aerosol of suburban central Balkans’ area and in the surface soil of South Iceland. These ratios measured in PM of atmospheric aerosols vary up to several orders of magnitudes depending on the air mass origin while deviations from the mean values of elements ratios in surface soils of the Rangárvellir area in Iceland are significantly lower indicating the similarity in elements compositions of all investigated samples. The highest average ratios showed Ca/Al in atmospheric aerosols of central Balkan while the highest average ratios in surface soil of the Rangárvellir area in Iceland is for Fe/Al.
Table 2. Average, standard deviation, minimum and maximum of characteristic ratios in atmospheric aerosol of suburban central Balkans’ area and surface soil in South Iceland.
Backward trajectories at altitudes of 500, 1500, 3000, and 5000 m for 101 cases were traced for 96 h using the HYSPLIT model for Belgrade (φ= 44°48′; λ= 20°28′). The trajectories are classified in two groups if they meet the following criteria: their direction of approach and passage over potential source areas (Iceland or Sahara). Each of the two trajectory groups starting at 3000 m is displayed in Figures 2A,B, respectively. We analyzed 101 cases and found that there were 17 (18) cases in which trajectories approached Belgrade from Iceland (the Sahara). Nearly all trajectories from Iceland (Figure 2A) have a north/northwesterly direction, while those from the Sahara (Figure 2B) a south/southwesterly direction. An example of the horizontal and vertical backward air trajectories from Iceland toward Belgrade is shown in Figure 2C. The flow of air parcels in the layer up to 3000 m was from northwestern direction on December 7, 2013. It can be seen a lifting of air parcels at all levels. Selected case for the Saharan dust transport toward Belgrade on April 6, 2012 is presented in Figure 2D. Backward trajectories show a flow of air masses from the south and southwestern direction in the layer above 1500 m. A lowering of air parcels above 1500 m in the first day is followed by lifting and lowering in the last 2 days.
Figure 2. Backward air trajectories approaching Belgrade for the arrival height of 3000 m from: (A) Iceland (17 cases) and (B) Sahara (18 cases) during the period 2012–2013. Selected 4 days backward trajectories ending in Belgrade at 500, 1500, and 3000 m on: (C) December 7, 2013 and (D) April 6, 2012.
The HYSPLIT backward trajectories at different altitudes of 500, 1500, 3000, and 5000 m were counted as individual paths in the present study. The trajectory analyses of all cases indicate that the predominant flow regime is NW and SW. This is in accordance with the results for Belgrade during summer and autumn in 2008 by Mihajlidi-Zelić et al. (2015).
It is expected that each individual trajectory be associated with a synoptic pattern, since trajectories are constructed from wind fields. As a verification, a composite map of all those days when trajectories are assigned to a particular group (cluster) is plotted for the level of 700 hPa (∼3000 m) in Figure 3. Composites are obtained as the average of the geopotential height and wind fields, and meridional wind component of all the 17 events (Figures 3A,C), i.e., 18 events (Figures 3B,D) analyzed for the March 2012–December 2013 period. Figure 3A presents a composite geopotential height and wind flow pattern for the cluster of trajectories presented in Figure 2A, indicating flow to Belgrade from a northwest direction. Low pressure over Northern Europe with a trough over Eastern Europe, and a high pressure system west from Europe produces northwesterlies over Serbia. In these synoptic situations, air from the Atlantic and also from Iceland can approach to Belgrade. The negative meridional wind components are located over Europe (Figure 3C), with the strongest values above the North Sea and France, suggesting dust intrusions from the northwest into central Europe and Serbia. Figure 3B shows the composite geopotential height and wind flow pattern for days of trajectories approaching Belgrade from Africa presented in Figure 2B. A very deep trough exists over Western Europe toward the central Algeria. Following this circulation, the dust was picked up from North Africa, and transported over Mediterranean toward Southeastern Europe, and also to Belgrade in corresponding circulation. The strongest meridional wind flows are located above the southern Adriatic Sea (Figure 3D), suggesting dust intrusions from the south-southwest into the Balkans. Synoptic situations with trajectories approaching Belgrade from Africa usually were characterized by a cyclone in lower troposphere, which developed over northern Italy and extended to the northern part of Africa and the Saharan desert (Vukmirović et al., 2004).
Figure 3. A composite geopotential height (m) and wind flow (m/s) map (A,B), and meridional wind component (C,D) for days of 17 and 18 events analyzed from March 2012 to December 2013 for trajectories represented in Figure 2A (left) and Figure 2B (right), respectively.
In this work we analyzed of characteristic elements ratios corresponding with crustal material of Northern Africa (Kandler et al., 2007, 2009, 2011; Scheuvens et al., 2011, 2013), and with soil of volcanic origin in Iceland in atmospheric aerosols of suburban area of central Balkan of air masses coming from southern and west southern regions as well as air masses coming over Iceland from northwestern directions.
The Ca/Al ratio in soil from Iceland mainly is between 0.7 and 1.0 while in the African dust are varying. This ratio in Atlas region is >1.0, in some regions of Egypt and north Sudan <0.5 but in northern Mali the ratio Ca/Al ≈ 8. Ratio Mg/Al in African dust is >0.3 (Scheuvens et al., 2013) and also in soil of south Iceland Mg/Al > 0.3. In crustal material of Africa 0.1< K/Al < 0.5 and we found the same ratio in aerosols from southern air masses. The ratio Fe/Al in crustal material of Africa were 0.2 and 1.2 while in crustal material from Iceland this ratio is between 1.0 and 3.0. Mn/Al < 0.03 in crustal material of Africa regions (Scheuvens et al., 2013) and also in investigated samples from Iceland this ratio is low and its value is about 0.03 and in some samples of river sediments it was approximately 0.06 while in volcano ash it was near 0.08.
Table 3 shows characteristic elements ratios in suburban atmospheric aerosols of central Balkan which are corresponding with the ratio in crustal material of North Africa in southern and south western air masses episodes.
Table 3. Elements ratio in suburban atmospheric aerosols of central Balkans’ corresponding with their ratios in surface crustal material of Northern Africa (NA) for air masses coming from Northern Africa.
Table 4 contains episodes of north western air masses passing over Iceland with element ratios corresponding with their ratios in surface soil of volcanoes origin in southern Iceland.
Table 4. Element ratios in suburban atmospheric aerosols of central Balkans’ corresponding with volcanic soil in South Iceland.
Table 5 shows dust storm occurrence in Iceland with the corresponding episodes of measured aerosols in central Balkan area.
Backward trajectories have calculated for each taken sample of atmospheric aerosol in central Balkan area – in total 101 backward trajectories that are corresponding with samples of atmospheric aerosols collected in suburban area of central Balkan. We found that 17 of total air masses were coming from the areas around Iceland. In 13 of them we found ratios of some elements that are corresponding to Iceland soil (volcano dust). We have chosen only those (1) which are passing over Iceland, (2) for which the characteristic elements ratios are corresponding to volcanic soil in South Iceland (Table 5), and (3) which are coinciding with dust storm occurrence in Iceland (Figure 4). According to this criterion we found 3 episodes for consideration as contribution of High Latitude Dust natural sources on central Balkan aerosol. Taking into account these criteria we can reliable claim that central Balkan area is under Icelandic dust affects. According to our results at least 3% air masses arriving from Iceland carrying suspended volcanic dust during storm occurrences in Iceland. It is demonstrated that dust storms occurrences on 16th and 17th September 2013 corresponding with backward trajectories of air masses reaching Belgrade calculated for the period from 18th to 20th September 2013 have documented (Beckett et al., 2017).
Figure 4. Satellite images of dust storms in South Iceland on September 16 (A) and 17 (B) 2013 captured in true color by the Moderate Resolution Imaging Spectroradiometer (MODIS) flying on NASA’s Terra satellite (Source: NASA/MODIS).
Most of the dust from the Icelandic desert originates from “dust hot-spots” which are in vicinity of glaciers and along the coastline. Such dust originates from abrasion underneath glaciers and deposited by glacio-fluvial processes. Further from the hot spots, dust can be also deposited on desert areas away from these hotspots and re-suspended again. The primary dust-hotspots contribute larger proportions of dust from Iceland than other areas combined (Arnalds et al., 2016).
Temporal variations of characteristic element ratios with episodes of Icelandic dust (IC) and North Africa dust (NA) have shown on Figure 5. Red arrows denote periods with characteristic elements ratios corresponding to African dust and black arrows indicate characteristic elements ratios with Icelandic volcanic soil measured in atmospheric aerosols in suburban area of Belgrade. Frequencies of air masses from NW and southern segments are almost the same with somewhat higher from NW segments. Nevertheless, a significantly larger number of cases with characteristic elements ratios in atmospheric aerosol of central Balkan corresponding to African crustal material were found in SW and S air masses (Table 3) than those which are corresponding to volcanic dust in air masses from NW segment (Table 4). This difference is consequence of more extensive dust storms on the North Africa than dust storm occurrence in Iceland.
Figure 5. Temporal variations of elements ratios with episodes of Icelandic dust (IC) and North Africa dust (NA).
Characteristic elements ratios corresponding with crustal material of Northern Africa and soil of volcanic origin in Iceland in atmospheric aerosols of suburban area of central Balkan were analyzed. We considered air masses coming from southern and southwestern regions as well as air masses coming over Iceland from northwestern directions. A total of 101 air parcel backward trajectories reaching Belgrade in Serbia were identified by means of a Lagrangian integrated trajectory (HYSPLIT) at four different ending altitudes of 500, 1500, 3000, and 5000 m. Large-scale atmospheric circulation features could be seen to be associated with two clusters of trajectories, from Iceland or Sahara. Air mass trajectories show that emissions from distant sources may cross boundaries and impact remote areas or places where the use of certain substances has been restricted. Three events meeting three set conditions were identified; air masses were passing over Iceland, the characteristic elements ratios corresponded to volcanic soil in South Iceland and these air masses coincide with dust storm occurrence in Iceland. We can conclude that central Balkan area is under influence of Icelandic dust originating from resuspended volcanic particles at least 3% of total air masses affecting central Balkan. This shows the importance of monitoring High Latitude Dust sources, particularly Iceland as the largest European and Arctic desert. Icelandic volcanic dust can contribute to impaired air quality in mainland Europe.
DĐ contributed in organization of measurements, interpretation of the results, and preparing the manuscript. IT contributed in trajectory calculation and preparing the manuscript. SS contributed in analyzing the soil and processing data. SP contributed in aerosol measuring and data processing. JĐ-M contributed in analyzing of the aerosol and data processing. DF contributing in organization of soil sampling and measuring in Iceland. PD-W contributing in dust observation in Iceland and preparing the manuscript.
This study was funded by the Ministry of Education, Science and Technological Development of Serbia (Projects: ON172001, ON176013, and III43007). The preparation of this manuscript was in part funded by the Icelandic Research Fund (Rannis) Grant No. 152248-051 and COST STSM Reference Number: COST-STSM-ES1306-34336 (Grant holder DĐ).
The authors declare that the research was conducted in the absence of any commercial or financial relationships that could be construed as a potential conflict of interest.
The authors gratefully acknowledge the NOAA Air Resources Laboratory (ARL) for the provision of the HYSPLIT transport and dispersion model and READY website (http://ready.arl.noaa.gov), National Centers for Environmental Prediction/National Center for Atmospheric Research for providing the daily composite means used in this publication and to COST Action ES1306 – Connecteur.
Andreae, M. O., and Rosenfeld, D. (2008). Aerosol–cloud–precipitation interactions. Part 1. The nature and sources of cloud-active aerosols. Earth Sci. Rev. 89, 13–41. doi: 10.1016/j.earscirev.2008.03.001
Arimoto, R. (2001). Eolian dust and climate: relationships to sources, tropospheric chemistry, transport and deposition. Earth Sci. Rev. 54, 29–42. doi: 10.1016/s0012-8252(01)00040-x
Arnalds, O., Dagsson-Waldhauserova, P., and Olafsson, H. (2016). The Icelandic volcanic aeolian environment: processes and impacts – a review. Aeolian Res. 20, 176–195. doi: 10.1016/j.aeolia.2016.01.004
Arnalds, O., Olafsson, H., and Dagsson-Waldhauserova, P. (2014). Quantification of iron-rich volcanogenic dust emissions and deposition over ocean from icelandic dust sources. Biogeosci. Discuss 11, 5941–5967. doi: 10.5194/bgd-11-5941-2014
Baddock, M. C., Mockford, T., Bullard, J. E., and Thorsteinsson, T. (2017). Pathways of high-latitude dust in the North Atlantic. Earth Planet. Sci. Lett. 459, 170–182. doi: 10.1016/j.epsl.2016.11.034
Beckett, F., Kylling, A., Sigurđardóttir, G., von Löwis, S., and Witham, C. (2017). Quantifying the mass loading of particles in an ash cloud remobilized from tephra deposits on iceland. Atmos. Chem. Phys. 17, 4401–4418. doi: 10.5194/acp-17-4401-2017
Berner, A. (1972). Praktische erfahrungen mit einem 20-stufen-impactor. Staub Reinhalt. Luft 32:315.
Cristofanelli, P., Calzolari, F., Bonafè, U., Lanconelli, C., Lupi, A., Busetto, M., et al. (2011). Five-year analysis of background carbon dioxide and ozone variations during summer seasons at the mario zucchelli station (Antarctica). Tellus B: Chem.Phys. Meteorol. 63, 831–842. doi: 10.1111/j.1600-0889.2011.00576.x
Dagsson-Waldhauserova, P., Arnalds, O., and Olafsson, H. (2014a). Long-term variability of dust events in iceland. Atmos. Chem. Phys. 14, 13411–13422. doi: 10.5194/acp-14-13411-2014
Dagsson-Waldhauserova, P., Arnalds, O., Olafsson, H., Skrabalova, L., Sigurdardottir, G. M., Branis, M., et al. (2014b). Physical properties of suspended dust during moist and low-wind conditions in iceland. Icel. Agric. Sci. 27, 25–39.
Dagsson-Waldhauserova, P., Arnalds, O., Olafsson, H., Hladil, J., Skala, R., Navratil, T., et al. (2015). Snow–dust storm: unique case study from iceland, March 6–7, 2013. Aeolian Res. 16, 69–74. doi: 10.1016/j.aeolia.2014.11.001
Darwin, C. (1845). Journal of Researches into the Natural History and Geology of the Countries Visited During the Voyage of H.M.S. Beagle Round the World, Under the Command of Capt. Fitz Roy, R.N, 2 Edn. London: John Murray.
De Longueville, F., Hountondji, Y.-C., Henry, S., and Ozer, P. (2010). What do we know about effects of desert dust on air quality and human health in west africa compared to other regions? Sci. Total Environ. 409, 1–8. doi: 10.1016/j.scitotenv.2010.09.025
Draxler, R., Arnold, D., Chino, M., Galmarini, S., Hort, M., Jones, A., et al. (2015). World meteorological organization’s model simulations of the radionuclide dispersion and deposition from the fukushima daiichi nuclear power plant accident. J. Environ. Radioact. 139, 172–184. doi: 10.1016/j.jenvrad.2013.09.014
Draxler, R., Stunder, B., Rolph, G., Stein, A., and Taylor, A. (2013). Hysplit4 User Guide. Available at: http://www.arl.noaa.gov/documents/reports/hysplit_user_guide.pdf (accessed May 21, 2015).
Draxler, R. R., and Hess, G. D. (1998). An overview of the hysplit_4 modelling system for trajectories dispersion, and deposition. Aust. Meteorol. Mag. 47, 295–308.
Đuričić-Milanković, J., Anđelković, I., Pantelić, A., Petrović, S., Gambaro, A., Antonović, D., et al. (2018). Partitioning of particulate matter and elements of suburban continental aerosols between fine and coarse mode. Environ. Sci. Poll. Res. 25, 20841–20853. doi: 10.1007/s11356-018-2037-8
Fleming, Z. L., Monks, P. S., and Manning, A. J. (2012). Review: untangling the influence of air-mass history in interpreting observed atmospheric composition. Atmos. Res. 10, 1–39. doi: 10.1016/j.atmosres.2011.09.009
Freitag, S., Clarke, A. D., Howell, S. G., Kapustin, V. N., Campos, T., Brekhovskikh, V. L., et al. (2014). Combining airborne gas and aerosol measurements with HYSPLIT: a visualization tool for simultaneous evaluation of air mass history and back trajectory consistency. Atmos. Meas. Tech. 7, 107–128. doi: 10.5194/amt-7-107-2014
Groot Zwaaftink, C. D., Arnalds, Ó., Dagsson-Waldhauserova, P., Eckhardt, S., Prospero, J. M., and Stoh, A. (2017). Temporal and spatial variability of icelandic dust emissions and atmospheric transport. Atmos. Chem. Phys. 17, 10865–10878. doi: 10.5194/acp-17-10865-2017
Hamonou, E., Chazette, P., Balis, D., Dulac, F., Schneider, X., Galani, E., et al. (1999). Characterization of the vertical structure of saharan dust export to the mediterranean basin. J. Geophys. Res. 104, 22257–22270. doi: 10.1029/1999jd900257
Husar, B. R. (2004). “Transport of dust: historical and recent observational evidence,” in The Handbook of Environmental Chemistry, eds D. Barceló and A. G. Kostianoy (Berlin: Springer).
Kandler, K., Benker, N., Bundke, U., Cuevas, E., Ebert, M., Knippertz, P., et al. (2007). Chemical composition and complex refractive index of saharan mineral dust at izana, tenerife (spain) derived by electron microscopy. Atmos. Environ. 41, 8058–8074. doi: 10.1016/j.atmosenv.2007.06.047
Kandler, K., Schütz, L., Deutscher, C., Ebert, M., Hofmann, H., JäCKEL, S., et al. (2009). Size distribution, mass concentration, chemical and mineralogical composition and derived optical parameters of the boundary layer aerosol at tinfou, morocco, during SAMUM 2006. Tellus B Chem. Phys. Meteorol. 61, 32–50. doi: 10.1111/j.1600-0889.2008.00385.x
Kandler, K., Schütz, L., Jäckel, S., Lieke, K., Emmel, C., Müller-Ebert, D., et al. (2011). Ground-based off-line aerosol measurements at praia, cape verde, during the saharan mineral dust experiment: microphysical properties and mineralogy. Tellus B Chem. Phys. Meteorol. 63, 459–474. doi: 10.1111/j.1600-0889.2011.00546.x
Karanasiou, A. A., Sitaras, I. E., Siskos, P. A., and Eleftheriadis, K. (2007). Size distribution and sources of trace metals and n-alkanes in the athens urban aerosol during summer. Atmos. Environ. 41, 2368–2381. doi: 10.1016/j.atmosenv.2006.11.006
Kellogg, C. A., and Griffin, D. W. (2006). Aerobiology and the global transport of desert dust. Trends Ecol. Evol. 21, 638–644. doi: 10.1016/j.tree.2006.07.004
Langmann, B., Folch, A., Hensch, M., and Matthias, V. (2012). Volcanic ash over europe during the eruption of eyjafjallajökull on iceland, April–May 2010. Atmos. Environ. 48, 1–8. doi: 10.1016/j.atmosenv.2011.03.054
Laurent, B., Marticorena, B., Bergametti, G., Léon, J. F., and Mahowald, N. M. (2008). Modeling mineral dust emissions from the sahara desert using new surface properties and soil database. J. Geophys. Res. 113:D14218.
Leelõssy, Â., Mészáros, R., Kovács, A., Lagzi, I., and Kovács, T. (2017). Numerical simulations of atmospheric dispersion of iodine-131 by different models. PLoS One 12:e0172312. doi: 10.1371/journal.pone.0172312
Liu, N., Yu, Y., He, J., and Zhao, S. (2013). Integrated modeling of urban–scale pollutant transport: application in a semi–arid urban valley, Northwestern China. Atmos. Poll. Res. 4, 306–314. doi: 10.5094/APR.2013.034
Mahowald, N. M., Rivera Rivera, G. D., and Luo, C. (2004). Comment on “relative importance of climate and land use in determining present and future global soil dust emission” by I. Tegen et al. Geophys. Res. Lett. 31:L24105.
McGowan, H., and Clark, A. (2008). Identification of dust transport pathways from lake eyre, Australia using Hysplit. Atmos. Environ. 42, 6915–6925. doi: 10.1016/j.scitotenv.2018.02.231
Mihajlidi-Zelić, D., Djordjević, D., Relić, D., Tošić, I., Ignjatović, L. J., Stortini, M. A., et al. (2015). Water-soluble inorganic ions in urban aerosols of the continental part of balkans (Belgrade) during the summer – autumn. Open Chem. 13, 245–256.
Ogawa, N., Yoshimura, K., Kikuchi, R., Adzuhata, T., Ozeki, T., and Kajikawa, M. (2004). Effect of long-range transport of air mass on the ionic components in radiation fog in northern Japan. Analyt. Sci. 20, 69–72. doi: 10.2116/analsci.20.69
Ovadnevaite, J., Ceburnis, D., Plauskaite-Sukiene, K., Modini, R., Dupuy, R., Rimselyte, I., et al. (2009). Volcanic sulfate and arctic dust plumes over the North Atlantic Ocean. Atmos. Environ. 43, 4968–4974. doi: 10.1016/j.atmosenv.2009.07.007
Pérez, A., Artuso, F., Mahmud, M., Kulshrestha, U., Luisa Sánchez, M., and Ángeles García, M. (2015). Applications of air mass trajectories. Adv. Meteorol. 2015:284213. doi: 10.1155/2015/284213
Prospero, J. M. (1999). Long-range transport of mineral dust in the global atmosphere: impact of African dust on the environment of the southeastern United States. Proc. Natl. Acad. Sci. U.S.A. 96, 3396–3403. doi: 10.1073/pnas.96.7.3396
Prospero, J. M., Blades, E., Naidu, R., Mathison, G., Thani, H., and Lavoie, M. C. (2008). Relationship between african dust carried in the atlantic trade winds and surges in pediatric asthma attendances in the caribbean. Int. J. Biometeor. 52, 823–832. doi: 10.1007/s00484-008-0176-1
Querol, X., Alastuey, A., Viana, M. M., Rodriguez, S., Artińano, B., Salvador, P., et al. (2004). Speciation and origin of PM10 and PM2.5 in Spain. Aerosol Sci. 35, 1151–1172. doi: 10.1016/j.jaerosci.2004.04.002
Rodríguez, S., Querol, X., Alastuey, A., Viana, M.-M., and Mantilla, E. (2003). Events affecting levels and seasonal evolution of airborne particulate matter concentrations in the western mediterranean. Environ. Sci. Technol. 37, 216–222. doi: 10.1021/es020106p
Rozwadowska, A., Zieliński, T., Petelski, T., and Sobolewski, P. (2010). Cluster analysis of the impact of air back-trajectories on aerosol optical properties at hornsund. Spitsbergen. Atmos. Chem. Phys. 10, 877–893. doi: 10.5194/acp-10-877-2010
Sakan, S., Popović, A., Anđelković, I., and Đorđević, D. (2016). Aquatic sediments pollution estimate using the metal fractionation, secondary phase enrichment factor calculation, and used statistical methods. Environ. Geochem. Health 38, 855–867. doi: 10.1007/s10653-015-9766-0
Sangeetha, S. K., Sivakumar, V., and Gebreslasie, M. (2018). Long-range transport of SO2 over South Africa: a case study of the calbuco volcanic eruption in April 2015. Atmos. Environ. 185, 78–90. doi: 10.1016/j.atmosenv.2018.04.056
Scheuvens, D., Kandler, K., Küpper, M., Lieke, K., Zorn, R., Ebert, M., et al. (2011). Individual-particle analysis of airborne dust samples collected over morocco in 2006 during SAMUM 1. Tellus B Chem. Phys. Meteorol. 63, 512–530. doi: 10.1111/j.1600-0889.2011.00554.x
Scheuvens, D., Schütz, L., Kandler, K., Ebert, M., and Weinbruch, S. (2013). Bulk composition of northern African dust and its source sediments — a compilation. Earth Sci. Rev. 116, 170–194. doi: 10.1016/j.earscirev.2012.08.005
Sokolik, I. N., and Toon, O. B. (1999). Incorporation of mineralogical composition into models of the radiative properties of mineral aerosol from UV to IR wavelengths. J. Geophys. Res. 104, 9423–9444. doi: 10.1029/1998jd200048
Stein, A. F., Draxler, R. R., Rolph, G. D., Stunder, B. J. B., Cohen, M. D., and Ngan, F. (2015). NOAA’s HYSPLIT atmospheric transport and dispersion modeling system. Bull. Am. Meteorol. Soc. 12, 2059–2077. doi: 10.1175/bams-d-14-00110.1
Su, L., Yuan, Z., Fung, J. C., and Lau, A. K. (2015). A comparison of HYSPLIT backward trajectories generated from two GDAS datasets. Sci. Total. Environ. 50, 527–537. doi: 10.1016/j.scitotenv.2014.11.072
Swap, R., Garstang, M., Greco, S., Talbot, R., and Kallberg, P. (1992). Saharan dust in the amazon basin. Tellus 44B, 133–149. doi: 10.1034/j.1600-0889.1992.t01-1-00005.x
Taylor, I., Mackie, S., and Watson, M. (2015). Investigating the use of the saharan dust index as a tool for the detection of volcanic ash in SEVIRI imagery. J. Volcanol. Geothermal Res. 304, 126–141. doi: 10.1016/j.jvolgeores.2015.08.014
Tegen, I., Werner, M., Harrison, S. P., and Kohfeld, K. E. (2004a). Relative importance of climate and land use in determining present and future global soil dust emission. Geophys. Res. Lett. 31:L05105.
Tegen, I., Werner, M., Harrison, S. P., and Kohfeld, K. E. (2004b). Reply to comment by N.M. Mahowald et al. on “Relative importance of climate and land use in determining present and future global soil dust emission”. Geophys. Res. Lett. 31:L24106.
Thorarinsdottir, E. F., and Arnalds, O. (2012). Wind erosion of volcanic materials in the hekla area. South Iceland. Aeol. Res. 4, 39–50. doi: 10.1016/j.aeolia.2011.12.006
Varga, G., Kovács, J., and Újvári, G. (2013). Analysis of saharan dust intrusions into the carpathian basin (Central Europe) over the period of 1979-2011. Glob. Planet. Change 100, 333–342. doi: 10.1016/j.gloplacha.2012.11.007
Varga, G., Újvári, G., and Kovács, J. (2014). Spatiotemporal patterns of Saharan dust outbreaks in the Mediterranean Basin. Aeol. Res. 15, 151–160. doi: 10.1016/j.aeolia.2014.06.005
Vukmirović, Z., Unkašević, M., Lazić, L., Tošić, I., Rajšić, S., and Tasić, M. (2004). Analysis of the saharan dust regional transport. Met. Atmos. Phys. 85, 265–273. doi: 10.1007/s00703-003-0010-6
Wang, F., Chen, D. S., Cheng, S. Y., Li, J. B., Li, M. J., and Ren, Z. H. (2010). Identification of regional atmospheric PM10 transport pathways using HYSPLIT, MM5-CMAQ and synoptic pressure pattern analysis. Environ. Modell. Softw. 25, 927–934. doi: 10.1016/j.envsoft.2010.02.004
Wang, H.-C., and John, W. (1988). Characteristics of the berner impactor for sampling inorganic ions. Aerosol Sci. Technol. 8, 157–172. doi: 10.1080/02786828808959179
Keywords: High Latitude Dust sources, backward trajectories, chemical fingerprints, atmospheric aerosol, long-range transport
Citation: Đorđević D, Tošić I, Sakan S, Petrović S, Đuričić-Milanković J, Finger DC and Dagsson-Waldhauserová P (2019) Can Volcanic Dust Suspended From Surface Soil and Deserts of Iceland Be Transferred to Central Balkan Similarly to African Dust (Sahara)? Front. Earth Sci. 7:142. doi: 10.3389/feart.2019.00142
Received: 30 July 2018; Accepted: 16 May 2019;
Published: 03 July 2019.
Edited by:
Irina Alexandrovna Mironova, Saint Petersburg State University, RussiaReviewed by:
Stefano Federico, Italian National Research Council (CNR), ItalyCopyright © 2019 Đorđević, Tošić, Sakan, Petrović, Đuričić-Milanković, Finger and Dagsson-Waldhauserová. This is an open-access article distributed under the terms of the Creative Commons Attribution License (CC BY). The use, distribution or reproduction in other forums is permitted, provided the original author(s) and the copyright owner(s) are credited and that the original publication in this journal is cited, in accordance with accepted academic practice. No use, distribution or reproduction is permitted which does not comply with these terms.
*Correspondence: Dragana Đorđević, ZHJhZ2FkakBjaGVtLmJnLmFjLnJz
Disclaimer: All claims expressed in this article are solely those of the authors and do not necessarily represent those of their affiliated organizations, or those of the publisher, the editors and the reviewers. Any product that may be evaluated in this article or claim that may be made by its manufacturer is not guaranteed or endorsed by the publisher.
Research integrity at Frontiers
Learn more about the work of our research integrity team to safeguard the quality of each article we publish.