- 1Biogeochemistry Program, Japan Agency for Marine-Earth Science and Technology, Yokosuka, Japan
- 2Atmosphere and Ocean Research Institute, The University of Tokyo, Kashiwa, Japan
- 3Japan Synchrotron Radiation Research Institute, SPring-8, Sayo, Japan
- 4Geological Survey of Japan, National Institute of Advanced Industrial Science and Technology, Tsukuba, Japan
- 5Department of Physics and Earth Science, Faculty of Science, University of the Ryukyus, Okinawa, Japan
Because reef-dwelling large benthic foraminifera (LBF) tests, which is composed of high-magnesium calcite, are the common components of modern reef sediments, and ancient reef carbonate rocks, they can provide a continuous record of the paleoenvironment of reef sediments. Evaluation of the responses of the minor element concentrations (Mg, Sr, Na, P, and S) of individual tests to ambient temperature and LBF growth modes was conducted with two cultured species, Calcarina gaudichaudii and Amphisorus kudakajimensis. The elements were assessed as tracers of temperature, salinity, nutrient concentration, and calcification rate. The Mg content of C. gaudichaudii and the Sr content of both species were controlled by temperature. It can be confirmed that the Mg of individual tests of C. gaudichaudii is a useful paleothermometer. There was a large biological modulation of Na, P, and S incorporation. In situ XANES analysis revealed that both inorganic and organic species contributed to the P and S content of LBF tests. We observed five sulfur components with variable oxidation states.
Introduction
The reconstruction of paleoenvironmental conditions is fundamental to understanding the mechanisms of climate change. In tropical and sub-tropical oceans, long-lived clacifiers such as massive corals and giant clams are geologically important producers of biominerals that provide high-resolution records of environmental conditions for up to hundreds of years (e.g., Gagan et al., 2000). Reef-dwelling large benthic foraminifera (LBF, >1 mm in diameter) account for 10% of the inorganic carbon production on seaward reef flats and in protected lagoonal communities (Fujita and Fujimura, 2008). Because LBF tests are common components of modern reef sediments and ancient reef carbonate rocks, they can provide a continuous record of paleoenvironmental changes in reef sediments, such as the Quaternary coral-reef sequences recovered by the Integrated Ocean Drilling Program Expedition 310 and 325 (Fujita et al., 2010; Yokoyama et al., 2011). Environmental proxies of high-Mg calcite LBF tests also have the potential to provide continuous paleoceanographic records during climatically important periods such as the Paleogene (Evans et al., 2013).
The Mg/Ca ratio of foraminifera has been used extensively as a reliable seawater temperature proxy for reconstruction of paleoenvironments (Nürnberg et al., 1996; Toyofuku et al., 2000). Temperature-dependent Mg incorporation is clearly apparent in cultured LBFs (Evans et al., 2013; Maeda et al., 2017), but not in LBFs collected from actual reef beds (Raja et al., 2007).
The Sr/Ca ratio has been a tool for investigating Sr and Ca inventories in paleoceanographic studies (Elderfield et al., 2000). Little is presently known about Sr incorporation into LBFs, and previous studies reported that the Sr/Ca ratios of LBFs are not related to water temperature (Raja et al., 2005, 2007; Maeda et al., 2017). Because salinity is a major component in climate dynamics, reconstruction of past salinity variations in the surface waters have been investigated in the use of Mg/Ca or Sr/Ca paleothermometry with oxygen isotope measurements (Elderfield and Ganssen, 2000; Felis et al., 2009). Recently, it was found that skeletal Na/Ca ratio in low-Mg calcite tests of planktonic and benthic foraminifera is a function of ambient water salinity (Wit et al., 2013; Mezger et al., 2016; Hauzer et al., 2018). On a molar basis, Na is among the most abundant of minor elements in biogenic CaCO3 (Yoshimura et al., 2017). The incorporation of Na into biogenic carbonates is complicated by differences in biogenic osmoregulation and salinity sensitivity (Delaney et al., 1985; Rosenthal and Katz, 1989), thus potential influences of temperature and growth changes and calcification processes on Na incorporation in LBF tests need to be verified.
There has been less examination of anion than cation incorporation into biogenic CaCO3. Phosphorus plays a central role in ocean fertility. Coral-based paleoenvironmental reconstructions have been linked to past changes in reef nutrient cycling caused by changes in river discharge, upwelling activity, and ocean circulation patterns (McCulloch et al., 2003). Recently, a link has been proposed between ocean phosphorus cycling and P/Ca ratios in reef-building and cold-water coral genera (Montagna et al., 2006; LaVigne et al., 2010; Anagnostou et al., 2011). Sulfur is an important component of organic compounds, and S concentrations in biogenic carbonates have been proposed as indicators of skeletal organic materials (Cuif et al., 2008) and structurally substituted sulfate (SSS) in biogenic CaCO3 (Yoshimura et al., 2013; Tamenori et al., 2014). To date, little is known about S and P speciation in foraminifera tests. Because both elements are biological constituents, one key to understanding modes of element incorporation is in situ chemical speciation or chemical mapping (Cuif et al., 2008; Tamenori et al., 2014). Recent advances in synchrotron radiation (SR) X-ray spectroscopy, including improvements in signal stability and sensitivity, have facilitated exploring the chemical environments of minor elements in various materials. This study is the first investigation of the incorporation of phosphorus and sulfur into LBFs.
Culture experiments of foraminifera under controlled laboratory conditions provide a fundamental understanding of element partitioning, isotope fractionation, and proxy development. For this study, we investigated the incorporation of the elements Mg, Sr, Na, S, and P in cultures of typical coral reef LBFs under four temperature conditions. We used high-sensitivity SR-XRF and XANES measurements to assess the influences of temperature and temperature-related growth changes on the incorporation of minor elements into individual tests. By revealing the dominant S- and P-bearing phases in biogenic CaCO3, XANES analyses can refine our understanding of the chemical environments of anions and thereby improve our ability to reconstruct paleoenvironmental changes at Earth’s surface.
Materials and Methods
Culture Experiments
We selected two species of algal symbiont–bearing LBFs, Amphisorus kudakajimensis and Calcarina gaudichaudii, which are common in northwestern Pacific coral reefs. A. kudakajimensis has porcelaneous imperforate test walls, is host to dinoflagellate endosymbionts, and attached to macroalgae in shallow reef areas. C. gaudichaudii precipitates hyaline perforate tests with diatom symbionts. Living adult individuals were collected from the near-shore reef flat in Bise, Okinawa Island on May 3, 2015 and cultured in a University of Ryukyus laboratory until asexual reproduction began. Soon afterward, asexually reproduced individuals were separated and maintained in glass vials with filtered seawater at ∼21°C before culture experiments. We conducted culture experiments with A. kudakajimensis and C. gaudichaudii at the temperatures at 21–30°C (Table 1), and this temperature range is approximately in accordance with that observed around Okinawa Island, which is 21°C–29°C on average from 1993 to 2015 (referenced from the 11th regional Japan coast guard headquarters). The LBFs were maintained in glass vials in water baths at each culture settings within ±0.5°C of the target temperature. The mean daytime photon flux density was ∼22 μmol m-2 s-1 on a 12 h:12 h light:dark cycle. Seawater in the glass vials was changed every 3 weeks. The details of the culture experiment are shown in Maeda et al. (2017).
The weights and lengths of C. gaudichaudii and A. kudakajimensis were measured for each individual test. The dry test weight of all specimens was measured 56 days after culturing began with a Cahn-33 microbalance which has the readability and reproducibility to 1 μg. We measured the major axis of the elliptical tests of A. kudakajimensis. Because the fragile spines of C. gaudichaudii were prone to breakage, we measured the radius of the median plane of C. gaudichaudii. It is difficult to estimate the precipitation rate of foraminifers due to those complicated forms, we used test length and weight for precipitation rate.
Micro-X-Ray Fluorescence and X-Ray Absorption Near-Edge Structure
Whole tests were embedded in epoxy resin under vacuum and ground to expose a cross section across the test wall. The sections parallel to the long axis of the test were then polished. The μ-XRF and μ-XANES measurements were performed at the b-branch of the soft X-ray photochemistry beamline (BL27SU) at the SPring-8 synchrotron radiation facility. A double crystal Si(111) monochromator ensured an energy resolution of 0.35 eV. A silicon drift detector was used to obtain μ-XRF mapping data for each element (Tamenori et al., 2011). The samples were fixed on an aluminum sample holder and installed in a vacuum chamber and fixed on a motorized XYZ stage. We obtained full XRF data at each mapping point and extracted the elemental concentrations in the tests from the XRF datasets. The μ-XRF measurements were taken at photon energies of 3000.0 eV and with acquisition times of 1 s at 12 and 24 μm intervals for the horizontal and vertical axis, respectively. For both species Mg concentrations, which is most sensitive to temperature changes, are variable at the innermost part in the tests (Supplementary Figures S1, S2). There parts are probably corresponds to juvenile tests, thus these measurement points were excluded from calculation. Other parts did not show compositional discontinuity, suggesting most of the data are average of the culture periods.
Results and Discussion
Magnesium Thermometer
Table 1 lists the LBF test element concentrations, and Figure 1 plots them in relation to culture temperature, growth weight, and length. For C. gaudichaudii, the test Mg concentration clearly increased with culture temperature from 30,980 ± 920 to 35,140 ± 920 ppm (Figure 1 and Table 1). The result supports the use of Mg/Ca ratios of individual tests of this species as a robust proxy for water temperature. Although Mg concentrations of both species fell within similar concentration ranges, those of A. kudakajimensis did not show a linear relationship with temperature; at 25°C, the Mg concentration of AMP-25-12 was an outlier. Mg concentration in C. gaudichaudii increased with length but not with test weight, and neither test weight nor length show significant difference with Mg concentrations in A. kudakajimensis (Figure 1). Growth factors do not affect higher Mg of AMP-25-12. The degree to which precipitation rate affects the partitioning of Mg is suggested to be related to solution chemistry, and abiogenic calcite precipitated from solutions with high Mg/Ca ratios (>3 mol/mol) and high saturation states is insensitive to precipitation rate (Saenger and Wang, 2014). The response of LBF growth rates to temperature varied between species, but Mg/Ca ratios of all culture species have the linear relation with temperature between 21°C and 30°C (Maeda et al., 2017, 2018). The lack of a consistent pattern suggests that growth rates had only a minor effect on the Mg partitioning. The 1.9 ± 0.3%/°C (95% confidence interval) increase in Mg/Ca of C. gaudichaudii (Maeda et al., 2017) is comparable to the previously reported values of the 1.9 ± 0.3%/°C in Operculina ammonoides (Evans et al., 2015). However, there is a resolvable difference in the temperature sensitivity between LBF species (3.1 ± 0.5%/°C for A. kudakajimensis, Raja et al., 2005). Therefore species-specific calibration should be needed in paleoceanographic studies.
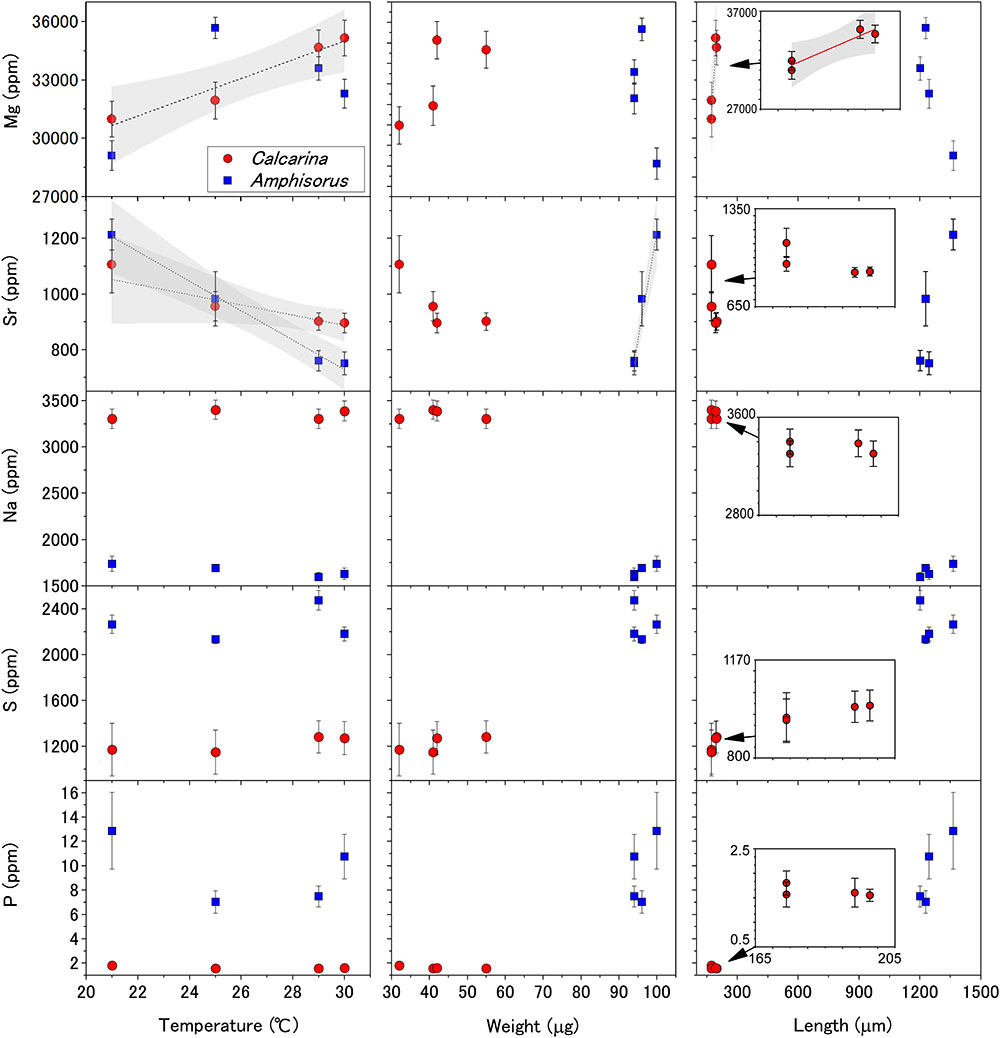
Figure 1. Plots of average test Mg, Sr, Na, S, and P concentrations measured by μ-XRF plotted against culture temperature, test weight, and length. The errors in each X-axis fall within the symbols. Gray areas represent 95% confidence bands of significant correlations (p < 0.05).
The Mg/Ca ratios of benthic foraminifers with high-Mg calcite tests, Planoglabratella opercularis and Quinqueloculina yabei (Toyofuku et al., 2000), O. ammonoides (Evans et al., 2015), and Neorotalia calcar, Baculogypsina sphaerulata (Maeda et al., 2018) were also linearly related to temperature. The Mg/Ca ratios of two LBFs grown in the same culture batch as the samples used in this study were positively correlated with temperature (Maeda et al., 2017). Aside from this general trend, individual differences occurred in A. kudakajimensis. For conventional ICP-AES or ICP-MS analysis, each data comes from analyses of multiple tests with intensive cleaning. Conversely, our μ-XRF samples were embedded in epoxy resin following mild cleaning via brief ultrasonification in ultrapure water and a methanol rinse. Although the analytical protocols differed from previous studies, the present results suggest that the Mg/Ca ratios of the perforate species C. gaudichaudii are more robust proxies for water temperature than the Mg-thermometer of A. kudakajimensis.
Proxies for Seawater Chemistry: Strontium and Sodium
The Sr/Ca in LBFs ranged from 1.8 to 2.4 mmol/mol (Maeda et al., 2017), which is higher than that of planktonic foraminiferal low-Mg calcite (∼1.4 mmol/mol-1, Elderfield et al., 2000; Cléroux et al., 2008). Foraminiferal calcite is a potential recorder of past seawater Sr/Ca ratios, but core-top and culture calibrations suggest that factors such as growth temperature and sensitivity to dissolution affect foraminiferal Sr/Ca ratios to different degrees (Elderfield et al., 2000). Although the Sr/Ca ratios in C. gaudichaudii, A. kudakajimensis, and three other species living on coral reefs are poorly correlated with temperature (Raja et al., 2007), the Sr concentrations of both cultured LBF species was negatively correlated with temperature. Strontium distribution coefficient in abiogenic calcite precipitation experiments increases with increasing calcite precipitation rate (Lorens, 1981). Neither growth weight nor length was correlated with Sr content of C. gaudichaudi, but more Sr incorporated into A. kudakajimensis as more rapid growth at lower temperature (Figure 1). It is possible that temperature is likely to be the predominant control on Sr concentrations in the cultured samples which may be also affected by shell growth rates in a particular species. A previous study of two Globorotalia species, G. inflata and G. truncatulinoides, from the North Atlantic has revealed a positive correlation between sea surface temperature and Sr/Ca ratios, which ranged from ∼1.2 to ∼1.5 mmol mol-1, but G. ruber and G. bulloides, and P. obliquiloculata do not show any temperature dependence (Cléroux et al., 2008). Although interspecific differences in Sr concentration are small, we would expect that Sr incorporation in natural foraminifera tests is affected by other processes, including growth rate and water chemistry (Cléroux et al., 2008).
Because salinity was constant during our experiment, the question we addressed was whether temperature and growth rate control Na concentration. The effect of temperature needs to be solved when applying Na/Ca salinometer (Mezger et al., 2016). The concentrations of Na show considerable interspecific differences. Na concentrations of both species were unaffected by temperature changes. Although test growth was highly variable between individuals, the changes did not appear to be systematically related to changes of Na content in either species (Figure 1). The Na/Ca ratio of benthic and planktonic tests has been proposed as a proxy for ambient salinity (Wit et al., 2013; Mezger et al., 2016), but Na/Ca ratios in cultured planktonic foraminiferal calcite tests do not vary while the Na/Ca ratio of the culture solution changes ∼2.5-fold at a constant Ca concentration (Delaney et al., 1985). The results showed a large biological modulation of Na incorporation into foraminifera tests. Recent culture experiment by Hauzer et al. (2018) demonstrated that the sensitivity of Na/Ca ratios of O. ammonoides to changes in Ca2+ concentrations is greater than salinity control. This fact is very important for understanding Na partitioning if LBFs modify chemical compositions at the site of crystal formation by active transport of Ca2+. Although the type of Ca2+-transporters that are used by foraminifera are still unknown, Ca2+-ATPases, H+/Ca2+ and Na+/Ca2+ antiporters might be responsible for test calcification (de Nooijer et al., 2014).
Amphisorus kudakajimensis and C. gaudichaudii belong to the respective orders miliolida and rotaliida. These orders have different test structures (porcelaneous and perforarte, respectively) which are precipitated via different calcification mechanisms (Figure 2). A. kudakajimensis secrets porcelaneous imperforate tests. Porcelaneous species precipitate needle-shaped micro crystals within cytoplasmic vesicles, then the high-Mg calcite crystals are released to the organic matrices to form a chamber wall (Figure 2A; Erez, 2003; de Nooijer et al., 2009). C. gaudichaudii precipitates hyaline perforate tests. In their calcification, seawater vacuoles are formed by endocytosis, and modified seawater vacuoles are then exocytosed into a delimited biomineralization space and mixed with seawater which is directly transported to the space (Figure 2B; Erez, 2003; de Nooijer et al., 2009). The amount of vesicles at the site of calcification of perforate foraminifera Ammonia beccarii increases during calcification, thus plays a key role in transporting substances for calcification (Nagai et al., 2018). Seawater vacuoles serve as the main ion source not only for the calcification process but also for metabolic processes. Therefore, active uptake of Na+ from seawater vacuoles for cellular processes lowers its concentrations in the calcifying solution. Another possible explanation is the selective Na removal by Na+/Ca2+ antiporter. From these point of views, the lower Na contents of A. kudakajimensis reflects lowered Na/Ca ratios in the vacuoles. On the other hand, the higher Na contents of C. gaudichaudii are considered to be affected by the mixing of exocytosed “modified” seawater vacuoles with directly transported seawater (Figure 2B). Therefore, it is proposed that the Na contents is a function of relative strength of cellular Na+ transport versus mass fluxes of seawater vacuolization, which is related to calcification mechanisms of foraminifera groups.
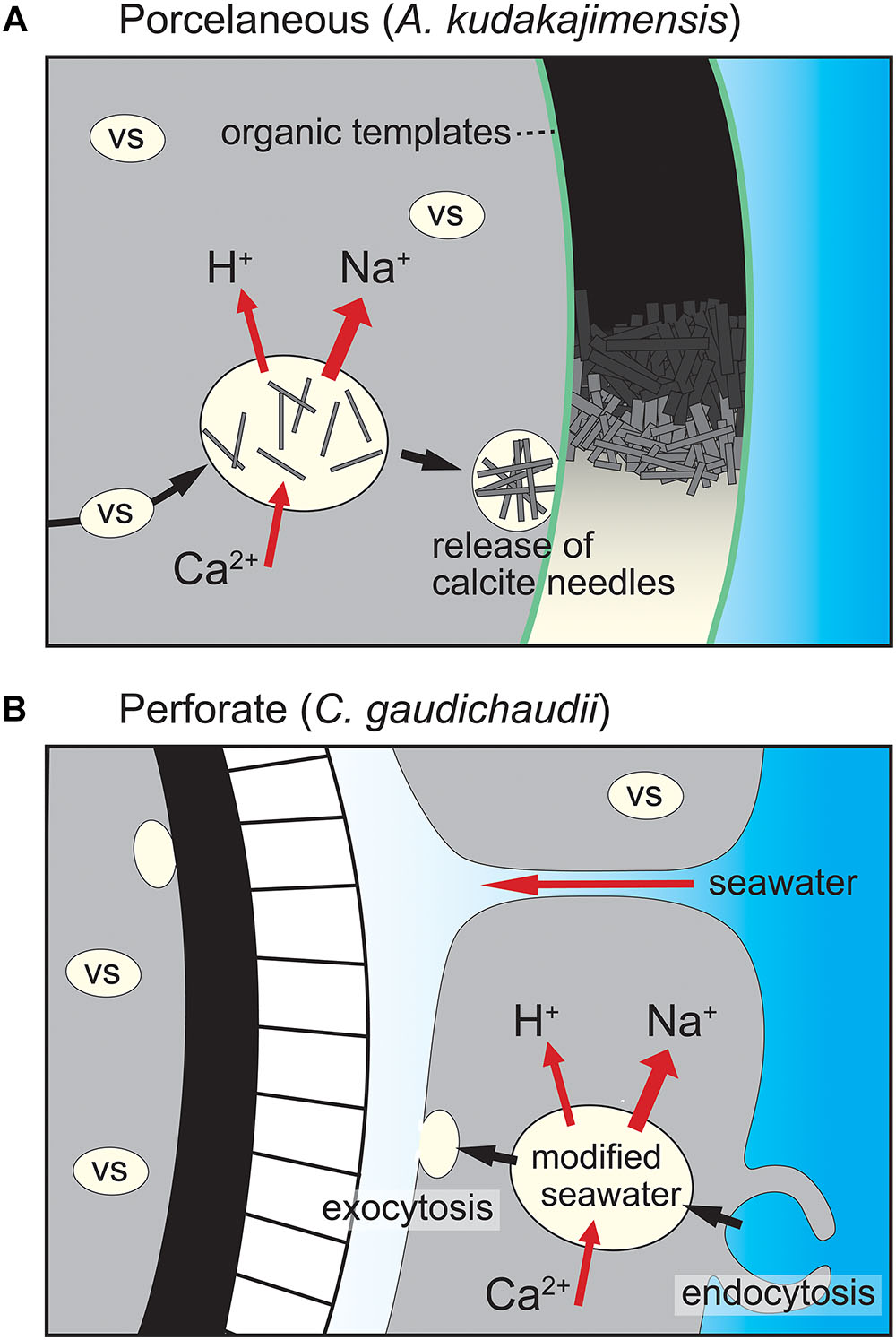
Figure 2. Simplified schemes describing the possible cation transport processes in (A) porcelaneous and (B) perforate foraminifera modified after Erez (2003) and de Nooijer et al. (2009). vs, vacuolized seawater.
Anion Incorporation: Sulfur and Phosphorus
The XANES measurements clarified that both inorganic and organic sulfur coexist in LBF tests. The main peaks of the S K-edge XANES spectra undergo large shifts toward higher energies with increasing oxidation state, from 2473 eV for amino acids to 2482 eV for sulfate (yellow line in Figure 3). The spectrum of protamine sulfate, a model organic sulfate compound, showed a single broad hump at ∼2498 eV, ranging from 2490 to 2510 eV, in the post-edge region, similar to the LBF spectra of 6, 7, and 9 (Figure 3). In contrast, post-edge peaks in the LBF spectra could be confirmed on the high-energy side of the main peak at ∼2484 eV in the inorganic carbonate (Perrin et al., 2017) and CaSO4 spectrum, an indication that inorganic sulfate was also present in the LBF tests (blue line in Figure 3). The present results indicate mixed S speciation, with dominant contributions from both inorganic and organic sulfate and a minor contribution from reduced sulfur and amino acids (Figure 3). A change in relative peak heights of the inorganic sulfate was observed between A. kudakajimensis and C. gaudichaudii, and inorganic sulfate were evident in A. kudakajimensis tests (spectra #1–5 in Figure 3).
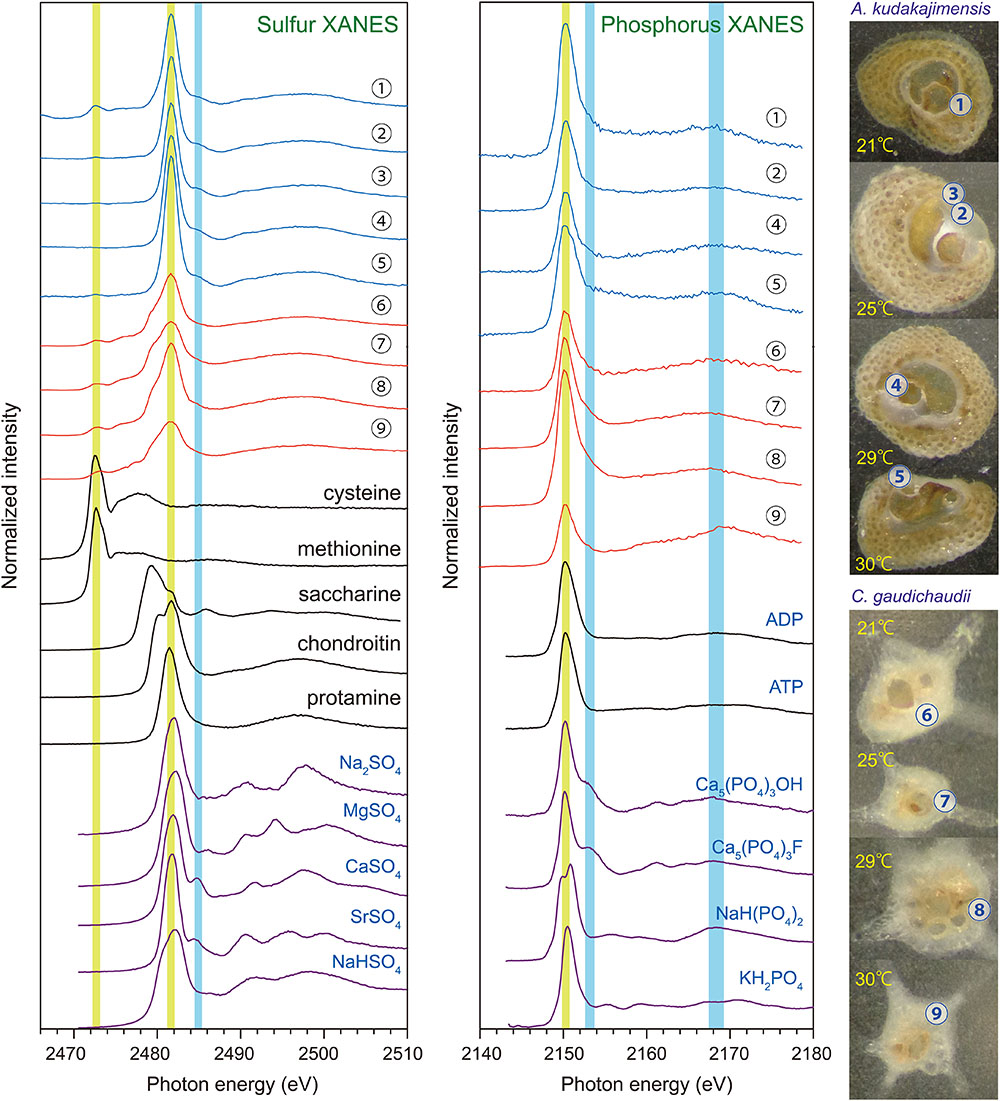
Figure 3. S and P K-edge XANES spectra and photographs with the measurement points of in situ spot XANES analysis of cultured LBF species (A. kudakajimensis: blue, C. gaudichaudii: red). The yellow and blue shades indicate the main peak components in LBF tests and some characteristic peaks of inorganic compounds, respectively. The reference spectra are presented for amino acids (cysteine and methionine) and organic sulfate and phosphate compounds (saccharine, chondroitin, protamine, ADP, and ATP, both shown in black), and inorganic compounds (purple) for fingerprinting identification.
Similar relationships were apparent in the P-XANES. The spectral shapes of most LBF samples were very similar, with a broad peak at ∼2169 eV. Both the shape and the peak are distinguishing features of the organic reference compounds ADP and ATP. Overall, phosphorus is predominantly present as an organic phosphate. Changes in the positions of the post-edge peaks were apparent for P-XANES spectrum #9, and there was a weak shoulder peak on the high-energy side of the main peak in some LBFs and also in inorganic phosphate standards (Figure 3).
In biogenic carbonates, sulfur is present as a major component of S-containing amino acids and sulfated sugars (Dauphin et al., 2013). For example, in situ analysis has revealed heterogeneous S distribution pattern in mollusk shells; intra-prismatic organic matrices and outer membranes are enriched in organic sulfates, whereas S-bearing amino acids are present in low concentrations (Dauphin et al., 2003a,b). Cuif et al. (2008) have revealed sulfate-rich bands in the wall of Globorotalia sp., which is interpreted predominantly as sulfated polysaccharides. However, a low signal-to-noise ratio in the XANES analyses of Globorotalia sp. seems difficult to address the conclusive evidence of whether the average local structural and compositional environment of sulfate are accompanied only by variations in sulfated polysaccharides. On the basis of the spectral profiles confirmed by in situ XANES, we concluded that the sulfur speciation in the LBF tests is more complex and the sulfur is present in oxidized to reduced forms. The main peaks of the sulfur compounds show large shifts toward higher energies with an increasing oxidation state. From the observations on mixed S-species in LBFs, an example of deconvolution of the spectrum of S μ-XANES measurement point #8 is shown in Figure 4. The experimental XANES spectrum was fitted using Gaussian fitting. We observed five peaks at 2471.8, 2474.4, 2477.9, 2479.0, and 2480.6 eV in the near-edge region of the S K-edge spectrum. These correspond to three sulfur components with intermediate oxidation states between the most reduced and oxidized sulfur (Figure 4). This spectral feature is similar to insolvable conchiolin sheets of a freshwater mussel Margaritifera laevis (Tamenori and Yoshimura, 2018). Note that some characteristic peaks were evident at pre- post-edge shoulders and in the post-edge region of each S compound (Yoshimura et al., 2013), thus the deconvoluted peak areas do not simply reflect relative abundance of compounds of different oxidation states.
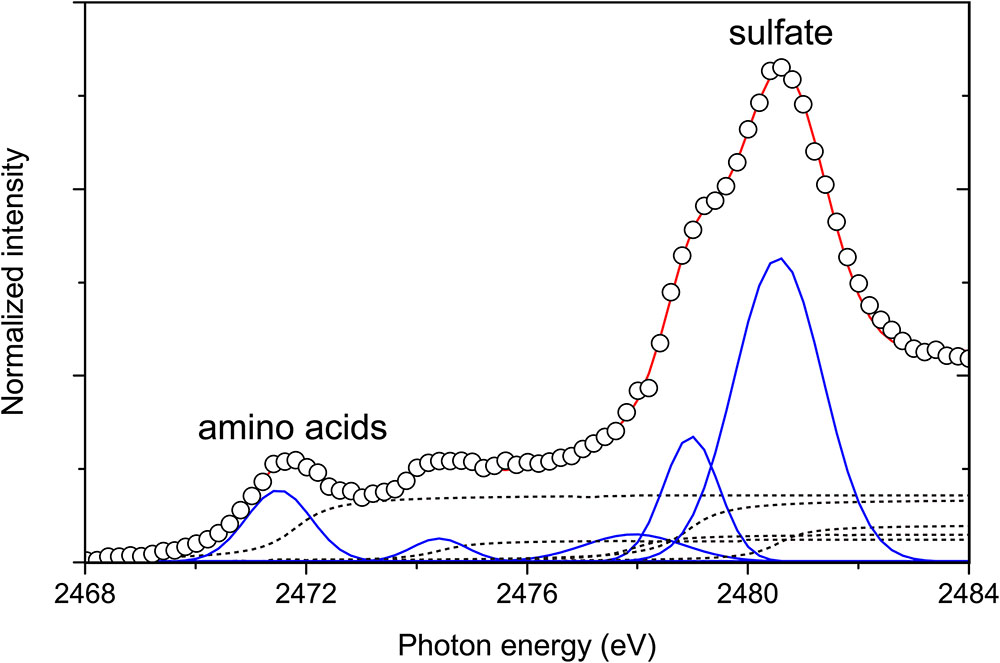
Figure 4. Peak deconvolution of the near-edge region of the sulfur XANES spectrum obtained at the measurement point-8 of Figure 3. The open circles and red line represent measured data and the best-fit line. The blue and dashed lines represent fitted Gaussian peaks and arctangent functions that represent the transition of ejected photoelectrons, respectively.
There was a clear, inter-species difference in anion concentrations. A. kudakajimensis tests were enriched in both S and P. As for the structural SO42- substitution, an increased activity of sulfate ions relative to carbonate ions in the calcifying fluid would have affected sulfate concentrations in the skeletons [the sulfate–carbonate link of Berry (1998) and co-workers]. A growth-related change in the carbonate ion concentration in the calcifying space affects the relative activity of sulfate ions to carbonate ions, which would be recorded as sulfate concentrations in the biogenic carbonates (Yoshimura et al., 2014). The impact of seawater [CO32-] on S/Ca ratios is recently re-evaluated by van Dijk et al. (2017); S incorporation increases with decreasing [CO32-], and high S bands in the test of Amphistegina lessonii coincide with elevated Mg concentrations. There were considerable differences between the two genera in total sulfur contents of individual tests (Figure 1). Neither temperature nor growth rate parameters affected S and P incorporation significantly. Incorporation of S and P in LBFs is apparently insensitive to differences in the conditions under which calcification takes place, i.e., the CaCO3 accumulation rate or calcifying fluid chemistry. Therefore, the organic matrix of the tests is the primary candidate for controlling total S and P contents in modern LBF tests. Extracting reproducible paleoceanographic records, such as carbonate parameters and nutrient cycling, from LBFs will require intensive cleaning of organic matrices or selective measurements of specific ultrastructures at high spatial resolution.
Conclusion
In this study, the Mg, Sr, Na, S, and P concentrations of two LBF species grown under four different temperature conditions are measured. The temperature dependent Mg incorporation is apparent in individual tests of C. gaudichaudii, but that of A. kudakajimensis did not show a linear relationship. The variation of Sr contents in both species showed a clear negative correlation with temperature. Mg and Sr contents of the two species fall in the similar range. In contrast, Na, S and P concentrations of LBF tests highlight considerable interspecific differences, which is critical to consider for cellular ion transport and organic matrices during calcification. The chemical environments of S and P were analyzed using in situ XANES, and they are predominantly present as sulfate and phosphate groups. We observed five sulfur components with variable oxidation states.
Author Contributions
AM, AS, KF, and HK contributed to conception and design of the culture experiments. TY and YT performed the X-ray analysis. TY wrote the first draft of the manuscript. AM wrote the sections of the manuscript. All authors contributed to manuscript revision, read, and approved the submitted version.
Funding
This research was supported by a grant-in-aid for scientific research to YT and TY (16H02950) from the Japan Society for the Promotion of Science. This study was approved by the SPring-8 Proposal Review Committee (Proposals 2015B1525, 2015B1526, and 2016A1462).
Conflict of Interest Statement
The authors declare that the research was conducted in the absence of any commercial or financial relationships that could be construed as a potential conflict of interest.
Acknowledgments
We express our appreciation to Dr. Takashi Toyofuku of JAMSTEC for fruitful discussion.
Supplementary Material
The Supplementary Material for this article can be found online at: https://www.frontiersin.org/articles/10.3389/feart.2019.00124/full#supplementary-material
References
Anagnostou, E., Sherrell, R. M., Gagnon, A., LaVigne, M., Field, M. P., and Mcdonough, W. F. (2011). Seawater nutrient and carbonate ion concentrations recorded as P/Ca, Ba/Ca, and U/Ca in the deep-sea coral Desmophyllum dianthus. Geochim. Cosmochim. Acta 75, 2529–2543. doi: 10.1016/j.gca.2011.02.019
Berry, J. N. (1998). Sulfate in Foraminiferal Calcium Carbonate: Investigating a Potential Proxy for Sea Water Carbonate Ion Concentration. doctor’s thesis. Cambridge: Massachusetts Institute of Technology.
Cléroux, C., Cortijo, E., Anand, P., Labeyrie, L., Bassinot, F., Caillon, N., et al. (2008). Mg/Ca and Sr/Ca ratios in planktonic foraminifera: proxies for upper water column temperature reconstruction. Paleoceanography 23:A3214.
Cuif, J. P., Dauphin, Y., Farre, B., Nehrke, G., Nouet, J., and Salomé, M. (2008). Distribution of sulphated polysaccharides within calcareous biominerals suggests a widely shared two-step crystallization process for the microstructural growth units. Mineral. Mag. 72, 233–237. doi: 10.1180/minmag.2008.072.1.233
Dauphin, Y., Ball, A. D., Castillo-Michel, H., Chevallard, C., Cuif, J. P., Farre, B., et al. (2013). In situ distribution and characterization of the organic content of the oyster shell Crassostrea gigas (Mollusca, Bivalvia). Micron 44, 373–383. doi: 10.1016/j.micron.2012.09.002
Dauphin, Y., Cuif, J. P., Doucet, J., Salomé, M., Susini, J., and Williams, C. T. (2003b). In situ mapping of growth lines in the calcitic prismatic layers of mollusc shells using X-ray absorp- tion near-edge structure (XANES) spectroscopy at the sulphur K-edge. Mar. Biol. 142, 299–304. doi: 10.1007/s00227-002-0950-2
Dauphin, Y., Cuif, J. P., Doucet, J., Salomé, M., Susini, J., and Willams, C. T. (2003a). In situ chemical speciation of sulfur in calcitic biominerals and the simple prism concept. J. Struct. Biol. 142, 272–280. doi: 10.1016/s1047-8477(03)00054-6
de Nooijer, L. J., Hathorne, E. C., Reichart, G. J., Langer, G., and Bijma, J. (2014). Variability in calcitic Mg/Ca and Sr/Ca ratios in clones of the benthic foraminifer Ammonia tepida. Mar. Micropaleontol. 107, 32–43. doi: 10.1016/j.marmicro.2014.02.002
de Nooijer, L. J., Toyofuku, T., and Kitazato, H. (2009). Foraminifera promote calcification by elevating their intracellular pH. Proc. Natl. Acad. Sci. U.S.A. 106, 15374–15378. doi: 10.1073/pnas.0904306106
Delaney, M. L., Bé, A. W., and Boyle, E. A. (1985). Li, Sr, Mg, and Na in foraminiferal calcite shells from laboratory culture, sediment traps, and sediment cores. Geochim. Cosmochim. Acta 49, 1327–1341. doi: 10.1016/0016-7037(85)90284-4
Elderfield, H., Cooper, M., and Ganssen, G. (2000). Sr/Ca in multiple species of planktonic foraminifera: implications for reconstructions of seawater Sr/Ca. Geochem. Geophy. Geosyst. 1:1017.
Elderfield, H., and Ganssen, G. (2000). Past temperature and δ 18 O of surface ocean waters inferred from foraminiferal Mg/Ca ratios. Nature 405, 442–445. doi: 10.1038/35013033
Erez, J. (2003). The source of ions for biomineralization in foraminifera and their implications for paleoceanographic proxies. Rev. Mineral. Geochem. 54, 115–149. doi: 10.2113/0540115
Evans, D., Erez, J., Oron, S., and Müller, W. (2015). Mg/Ca-temperature and seawater-test chemistry relationships in the shallow-dwelling large benthic foraminifera Operculina ammonoides. Geochim. Cosmochim. Acta 148, 325–342. doi: 10.1016/j.gca.2014.09.039
Evans, D., Müller, W., Oron, S., and Renema, W. (2013). Eocene seasonality and seawater alkaline earth reconstruction using shallow-dwelling large benthic foraminifera. Earth Planet. Sci. Lett. 381, 104–115. doi: 10.1016/j.epsl.2013.08.035
Felis, T., Suzuki, A., Kuhnert, H., Dima, M., Lohmann, G., and Kawahata, H. (2009). Subtropical coral reveals abrupt early-twentieth-century freshening in the western North Pacific Ocean. Geology 37, 527–530. doi: 10.1130/g25581a.1
Fujita, K., and Fujimura, H. (2008). Organic and inorganic carbon production by algal symbiont-bearing foraminifera on northwest Pacific coral-reef flats. J. Foraminiferal Res. 38, 117–126. doi: 10.2113/gsjfr.38.2.117
Fujita, K., Omori, A., Yokoyama, Y., Sakai, S., and Iryu, Y. (2010). Sea-level rise during termination II inferred from large benthic foraminifers: IODP expedition 310, Tahiti Sea Level. Mar. Geol. 271, 149–155. doi: 10.1016/j.margeo.2010.01.019
Gagan, M., Ayliffe, L., Beck, J. W., Cole, J., Druffel, E., Dunbar, R. B., et al. (2000). New views of tropical paleoclimates from corals. Quat. Sci. Rev. 19, 45–64. doi: 10.1016/s0277-3791(99)00054-2
Hauzer, H., Evans, D., Müller, W., Rosenthal, Y., and Erez, J. (2018). Calibration of Na partitioning in the calcitic foraminifer Operculina ammonoides under variable Ca concentration: toward reconstructing past seawater composition. Earth Planet. Sci. Lett. 497, 80–91. doi: 10.1016/j.epsl.2018.06.004
LaVigne, M., Matthews, K. A., Grottoli, A. G., Cobb, K. M., Anagnostou, E., Cabioch, G., et al. (2010). Coral skeleton P/Ca proxy for seawater phosphate: multi-colony calibration with a contemporaneous seawater phosphate record. Geochim. Cosmochim. Acta 74, 1282–1293. doi: 10.1016/j.gca.2009.11.002
Lorens, R. B. (1981). Sr, Cd, Mn and Co distribution coefficients in calcite as a function of calcite precipitation rate. Geochim. Cosmochim. Acta 45, 553–561. doi: 10.1016/0016-7037(81)90188-5
Maeda, A., Fujita, K., Horikawa, K., Suzuki, A., Ohno, Y., and Kawahata, H. (2018). Calibration between temperature and Mg/Ca and oxygen isotope ratios in high-magnesium calcite tests of asexually reproduced juveniles of large benthic foraminifers. Mar. Micropaleontol. 143, 63–69. doi: 10.1016/j.marmicro.2018.07.004
Maeda, A., Fujita, K., Horikawa, K., Suzuki, A., Yoshimura, T., Tamenori, Y., et al. (2017). Evaluation of oxygen isotope and Mg/Ca ratios in high-magnesium calcite from benthic foraminifera as a proxy for water temperature. J. Geophys. Res. 122, 185–199. doi: 10.1002/2016jg003587
McCulloch, M., Fallon, S., Wyndham, T., Hendy, E., Lough, J., and Barnes, D. (2003). Coral record of increased sediment flux to the inner Great Barrier Reef since European settlement. Nature 421, 727–730. doi: 10.1038/nature01361
Mezger, E. M., Nooijer, L. J., Boer, W., Brummer, G. J. A., and Reichart, G. J. (2016). Salinity controls on Na incorporation in Red Sea planktonic foraminifera. Paleoceanogr. Paleoclimatol. 31, 1562–1582. doi: 10.1002/2016pa003052
Montagna, P., McCulloch, M., Taviani, M., Mazzoli, C., and Vendrell, B. (2006). Phosphorus in cold-water corals as a proxy for seawater nutrient chemistry. Science 312, 1788–1791. doi: 10.1126/science.1125781
Nagai, Y., Uematsu, K., Wani, R., and Toyofuku, T. (2018). Reading the fine print: ultra-microstructures of foraminiferal calcification revealed using focused ion beam microscopy. Front. Mar. Sci. 5:67. doi: 10.3389/fmars.2018.00067
Nürnberg, D., Bijma, J., and Hemleben, C. (1996). Assessing the reliability of magnesium in foraminiferal calcite as a proxy for water mass temperatures. Geochim. Cosmochim. Acta 60, 803–814. doi: 10.1016/0016-7037(95)00446-7
Perrin, J., Rivard, C., Vielzeuf, D., Laporte, D., Fonquernie, C., Ricolleau, A., et al. (2017). The coordination of sulfur in synthetic and biogenic Mg calcites: the red coral case. Geochim. Cosmochim. Acta 197, 226–244. doi: 10.1016/j.gca.2016.10.017
Raja, R., Saraswati, P. K., and Iwao, K. (2007). A field-based study on variation in Mg/Ca and Sr/Ca in larger benthic foraminifera. Geochem. Geophys. Geosyst. 8:Q10012.
Raja, R., Saraswati, P. K., Rogers, K., and Iwao, K. (2005). Magnesium and strontium compositions of recent symbiont-bearing benthic foraminifera. Mar. Micropaleontol. 58, 31–44. doi: 10.1016/j.marmicro.2005.08.001
Rosenthal, Y., and Katz, A. (1989). The applicability of trace elements in freshwater shells for paleogeochemical studies. Chem. Geol. 78, 65–76. doi: 10.1016/0009-2541(89)90052-1
Saenger, C., and Wang, Z. (2014). Magnesium isotope fractionation in biogenic and abiogenic carbonates: implications for paleoenvironmental proxies. Quat. Sci. Rev. 90, 1–21. doi: 10.1016/j.quascirev.2014.01.014
Tamenori, Y., Morita, M., and Nakamura, T. (2011). Two-dimensional approach to fluorescence yield XANES measurement using a silicon drift detector. J. Synchrotron. Radiat. 18, 747–752. doi: 10.1107/S0909049511027531
Tamenori, Y., and Yoshimura, T. (2018). Sulfur speciation in growth layers of shell cross section of the long-lived bivalve Margaritifera laevis using synchrotron spectromicroscopy analysis. Geochim. Cosmochim. Acta 237, 357–369. doi: 10.1016/j.gca.2018.07.002
Tamenori, Y., Yoshimura, T., Luan, N. T., Hasegawa, H., Suzuki, A., Kawahata, H., et al. (2014). Identification of the chemical form of sulfur compounds in the Japanese pink coral (Corallium elatius) skeleton using mu-XRF/XAS speciation mapping. J. Struct. Biol. 186, 214–223. doi: 10.1016/j.jsb.2014.04.001
Toyofuku, T., Kitazato, H., Kawahata, H., Tsuchiya, M., and Nohara, M. (2000). Evaluation of Mg/Ca thermometry in foraminifera: comparison of experimental results and measurements in nature. Paleoceanography 15, 456–464. doi: 10.1029/1999pa000460
van Dijk, I., de Nooijer, L. J., Boer, W., and Reichart, G. J. (2017). Sulfur in foraminiferal calcite as a potential proxy for seawater carbonate ion concentration. Earth Planet. Sci. Lett. 470, 64–72. doi: 10.1016/j.epsl.2017.04.031
Wit, J. C., De Nooijer, L. J., Wolthers, M., and Reichart, G. J. (2013). A novel salinity proxy based on Na incorporation into foraminiferal calcite. Biogeosciences 10, 6375–6387. doi: 10.5194/bg-10-6375-2013
Yokoyama, Y., Webster, J. M., Cotterill, C., Braga, J. C., Jovane, L., Mills, H., et al. (2011). IODP expedition 325: great barrier reefs reveals past sea-level, climate and environmental changes since the last ice age. Sci. Drill. 12, 32–45. doi: 10.5194/sd-12-32-2011
Yoshimura, T., Tamenori, Y., Kawahata, H., and Suzuki, A. (2014). Fluctuations of sulfate, S-bearing amino acids and magnesium in a giant clam shell. Biogeosciences 11, 3881–3886. doi: 10.5194/bg-11-3881-2014
Yoshimura, T., Tamenori, Y., Suzuki, A., Kawahata, H., Iwasaki, N., Hasegawa, H., et al. (2017). Altervalent substitution of sodium for calcium in biogenic calcite and aragonite. Geochim. Cosmochim. Acta 202, 21–38. doi: 10.1016/j.gca.2016.12.003
Keywords: large benthic foraminifera, proxy, element partitioning, culture experiment, chemical speciation, P and S K-edge XANES
Citation: Yoshimura T, Maeda A, Tamenori Y, Suzuki A, Fujita K and Kawahata H (2019) Partitioning and Chemical Environments of Minor Elements in Individual Large Benthic Foraminifera Cultured in Temperature-Controlled Tanks. Front. Earth Sci. 7:124. doi: 10.3389/feart.2019.00124
Received: 17 August 2018; Accepted: 09 May 2019;
Published: 31 May 2019.
Edited by:
Claire Rollion-Bard, UMR7154 Institut de Physique du Globe de Paris (IPGP), FranceReviewed by:
William Patrick Gilhooly III, Indiana University–Purdue University Indianapolis, United StatesSandrine Le Houedec, L’Université Nantes Angers Le Mans (L’UNAM), France
Copyright © 2019 Yoshimura, Maeda, Tamenori, Suzuki, Fujita and Kawahata. This is an open-access article distributed under the terms of the Creative Commons Attribution License (CC BY). The use, distribution or reproduction in other forums is permitted, provided the original author(s) and the copyright owner(s) are credited and that the original publication in this journal is cited, in accordance with accepted academic practice. No use, distribution or reproduction is permitted which does not comply with these terms.
*Correspondence: Toshihiro Yoshimura, eW9zaGltdXJhdEBqYW1zdGVjLmdvLmpw; Ayumi Maeda, YW1hZWRhQGFvcmkudS10b2t5by5hYy5qcA==
†These authors have contributed equally to this work