- 1International Centre of Future Science, Dinosaur Evolution Research Centre, Jilin University, Changchun, China
- 2Robert Gillespie Academic Skills Centre and Department of Biology, University of Toronto Mississauga, Mississauga, ON, Canada
- 3Australian Centre for Neutron Scattering, Australian Nuclear Science and Technology Organization, Lucas Heights, NSW, Australia
- 4Department of Biology, University of Toronto Mississauga, Mississauga, ON, Canada
The early Permian cave system in the Dolese Brothers Limestone Quarry near Richards Spur, Oklahoma represents a unique depositional environment that has been interpreted as preserving an upland biota. The quarry and the region around it represent Paleozoic cave systems that underwent periods of flooding not unlike present-day conditions that are commonly associated with monsoonal episodes. The Richards Spur locality is particularly rich in captorhinid eureptiles which represent one of the earliest reptilian clades to have evolved a specialized dentition. Although the multiple-tooth rowed Captorhinus aguti is the most abundant captorhinid at Richards Spur, at least one other species has been described (Captorhinus magnus) and assigned to the same genus, but five other captorhinid taxa have also been found. We describe a new member of the genus Captorhinus (Captorhinus kierani) and explore details of the dental anatomy against the two other members of the genus at Richards Spur, C. aguti and C. magnus, as well as with a member of the genus not presently known from Richards Spur (Captorhinus laticeps). Findings suggest that the nature of the ogival dentition described previously as a synapomorphy uniting C. aguti with C. magnus is not supported and we propose a more informative method for differentiating among dental characters within the clade. The discovery of a new species of Captorhinus provides additional evidence for captorhinid taxic diversity at Richards Spur and is supportive of niche partitioning, which is likely associated with reducing intra-specific competition within the clade. In addition, we argue that the captorhinid fossils at Richards Spur likely includes one additional, currently undescribed multiple-tooth rowed form, that renders the current practice of assigning disarticulated cranial remains, specifically dental fragments, to the species C. aguti problematic. Finally, we offer a method for a comprehensive examination of the dental characteristics, which can then be applied to explore taxic diversity at Richards Spur and examine one of the earliest examples of niche specialization. As a consequence of this research, additional insight into exploring biological interactions between Paleozoic taxa can be examined, with an opportunity to shed light on what might have driven these evolutionary processes.
Introduction
The genus Captorhinus and the more inclusive Captorhinomorpha presents one of the most widely recognized Paleozoic amniote clades. The entire clade is well known and contains many thousands of fossils found throughout Permian aged sediments of North America and around the world. One particular location, Richards Spur, is unique in its preservational environment (Lucas, 2018) and the composition of its fauna (Modesto et al., 2007, 2018) and provides an excellent opportunity to explore the ecological interplay between closely related species. Here, we examine a previously unknown member of the Richards Spur fauna and provide a comprehensive analysis of the dental characteristics of three members of the genus Captorhinus that occur at Richards Spur, thus expanding our understanding of how dental specialization might have been a driving force in the niche partitioning at this Paleozoic locality.
Early Permian captorhinids are known from a number of localities across the southwestern United States and represent the first eureptiles to diversify extensively (Modesto et al., 2016, 2018, 2019). Species have been described from New Mexico, Oklahoma and Texas. Of these regions, Oklahoma and specifically the Dolese Brothers Limestone Quarry near Richards Spur has been an exceptionally prolific source for captorhinid material. Six captorhinid taxa have been described from this locality: Baeotherates fortsillensis (May and Cifelli, 1998); Captorhinus aguti ([Ectocynodon aguti] Cope, 1882, 1895); Captorhinus magnus (Kissel et al., 2002); Opisthodontosaurus carrolli (Reisz et al., 2015); Labidosauriscus richardi (Modesto et al., 2018); and an unnamed basal captorhinid (Modesto, 1996). Similar in age to Richards Spur and representing a similar cave deposit, Bally Mountain is known to contain a slightly different faunal assemblage that is rich in the multiple-tooth rowed moradisaurine Captorhinikos valensis (Modesto et al., 2014), but Captorhinus aguti is relatively rare. The age of these localities has been interpreted as being early Artinskian (LeBlanc et al., 2015).
Other, younger captorhinids are known across Oklahoma and Texas and include the single-tooth rowed Labidosaurus hamatus from the lower Permian of Texas (Modesto et al., 2007), which is the largest known captorhinid with a single tooth row as well as the moradisaurine genera (Modesto et al., 2019) Captorhinoides valensis (Olson, 1951 – Middle Vale Formation, Knox County Texas), Captorhinikos chozaensis (Olson, 1954 – Choza Formation, Clear Fork group, Foard County, Texas), Captorhinikos valensis? (Olson, 1954—Upper part of Vale Formation, Clear Fork group, Knox County, Texas), Labidosaurikos meachami (Stovall, 1950—Hennessey Shale, Crescent, Logan County, Oklahoma), and Rothianiscus (Olson, 1965—Chickasha Formation, near Hitchcock, Blaine County. Oklahoma). All are preserved in middle to late Kungurian sediments (Lucas, 2018).
A group of relatively small captorhinids Rhiodenticulatus, Reiszorhinus, Protocaptorhinus, Romeria, Captorhinus laticeps (Clark and Carroll, 1973; Heaton and Reisz, 1980; Berman and Reisz, 1986; Modesto and Smith, 2001; Sumida et al., 2010) are all stratigraphically located in slightly older to significantly older sediments than Richards Spur and are found across the southwest including Texas and New Mexico. Rhiodenticulatus heatoni, for example, is known from the Abo and Cutler Formations of Rio Arriba County, New Mexico, which dates to the Asselian of the lowermost Permian (Berman and Reisz, 1986). There is also evidence of multiple captorhinid taxa across widely scattered localities in northern and central parts of the state. These are distributed in the Cutler, Abo, and Sangre de Cristo Formations, which range in age from the Asselian through to the Kungurian, but are too fragmentary to allow assignment to either existing or new species. Protocaptorhinus pricei (Carroll and Baird, 1972; Clark and Carroll, 1973; Gaffney and McKenna, 1979) is also known from Oklahoma (Noble County—Wellington Formation) and has also been discovered in similar aged strata in Texas (Archer County, Texas). Texas (Mitchell Creek—Waggoner Ranch Formation) is also the location for a single-tooth rowed species, C. laticeps (Williston, 1909), which has also been collected from the Oklahoma site at the McCann Quarry in Kay County, Oklahoma. The basal captorhinid Romeria texana (Price, 1937) and Reiszorhinus olsoni (Sumida et al., 2010) have also been found at Texas localities, although in these instances the entire fauna is much less abundant when compared to Richards Spur. Outside of North America few early Permian remains of captorhinid taxa are known with only one confidently assigned member described from Russia, Riabininus uralensis (Ivakhnenko, 1990).
Captorhinids do extend into the Carboniferous, as evidenced by the late Carboniferous basal captorhinid from Kansas, Euconcordia cunninghami (Müller and Reisz, 2005; Reisz et al., 2016). However, no other Carboniferous material can be referred to the clade. The fossil record of captorhinids also extends into the middle Permian of China and the late Permian of Africa (Modesto et al., 2018, 2019). Overall, there is no doubt that in both abundance and diversity, Richards Spur is the most fossiliferous Permian locality, providing an unprecedented opportunity to study captorhinid diversity.
The conditions at Richards Spur have been studied extensively (Woodhead et al., 2010; MacDougall et al., 2017; Lucas, 2018) and the locality has been shown to represent the remains of a Paleozoic cave system that was exposed to continuous monsoonal events resulting in flooding of the limestone caves over a period of some 4 or 5 million years. What makes this system unique is the presence of an unusually high number of terrestrial vertebrates suggesting that it represents a unique taphonomic event selectively preserving an up-land community. Furthermore, although much of the fossil material collected at Richards Spur during the last century represents disarticulated remains, there are some pockets that were uncovered within the last three decades where reasonably complete and more fully articulated material has been preserved. The nature of this preservation suggests that these more complete remains were less likely to be disturbed after their initial accrual and likely reflect a depositional setting that has been subjected to less reworking. Two such deposits collected in the late 1990’s and in 2005 have yielded exquisitely preserved skull and isolated postcranial remains that contain a sampling of articulated or semi-articulated captorhinomorphs such as Opisthodontosaurus carrolli (Reisz et al., 2015), and Captorhinus aguti, respectively. In addition, the fossiliferous pocket uncovered in 2005 is also the source of the single-tooth rowed form that we describe here. Furthermore, while the abundant captorhinid material discovered at Richards Spur since the 1930s would be suggestive of a contemporaneous fauna, due to the nature of the cave system and the speleothem age being older than the karst fill (Lucas, 2018), an exact age for the fossils found in any specific pocket is difficult to ascertain. Notwithstanding the difficulty in confirming coexistence of these taxa, the 2005 pocket to which this new material belongs contains at least two separate species of the genus Captorhinus (C. aguti and the present find) along with remains attributable to Captorhinus magnus (Reisz personal observation) and those of the basal captorhinid, Opisthodontosaurus, a mycterosaurine varanopid, and at least one trematopid, Acheloma.
Although not presently known from Richards Spur, there is one other species that has been assigned to the genus Captorhinus, which is known from the geologically older McCann Quarry locality in Oklahoma and from the Mitchell Creek locality in Texas. This additional Captorhinus material, as assigned by Heaton (1979), represents yet another single-tooth rowed species, which was part of Heaton’s (1979) systematic study of captorhinids from outside of the Richards Spur locality. However, he used isolated captorhinid skull elements from Richards Spur in order to describe in detail some aspects of internal cranial anatomy. He also synonymized a number of species, all single tooth-rowed forms, into what he interpreted as the more primitive captorhinid taxon, “Eocaptorhinus” laticeps. Gaffney (1990) later synonymized “Eocaptorhinus” with Captorhinus, suggesting that the absence of multiple-tooth rows was insufficient cause to exclude this species from the genus Captorhinus. Dodick and Modesto (1995) upheld Gaffney’s synonymy and more recently, Modesto et al. (2007) continued to support this synonymy while maintaining the species designation as per Heaton. In addition, Modesto et al. (2007) assigned a sister-group relationship that included Captorhinus laticeps as the sister taxon to the less inclusive Captorhinus magnus and Captorhinus aguti clade. This grouping was based on a single autapomorphy associated with the apparent shared characteristic of ogival dentition in the C. magnus-C. aguti clade.
Whereas, most of the other Permian localities, where captorhinomorph material is known, are restricted in their composition to encompassing either basal or advanced forms, the Richards Spur locality, and notably the fossiliferous pocket discovered in 2005, contains a mixed faunal assemblage, which is supportive of a taxonomically diverse locality. The nature of this diversity, which is predominantly due to a heteromorphic dentition across the various taxa, has been speculated to be representative of resource partitioning among Permian captorhinomorphs at Richards Spur (Modesto et al., 2018). Therefore, the new material is especially relevant to the story of the taxic diversity at Richards Spur in that it adds one more taxon (from six to seven) to the list previously articulated by Modesto et al. (2018). It is also important to recognize that this diversity is most obviously manifested in the dental morphology, which contrasts with the rather conservative nature of the gross skull anatomy.
Methods
The type specimen of Captorhinus kierani was scanned at the DINGO thermal-neutron radiography/tomography/imaging station (Garbe et al., 2015) at the Australian Nuclear Science and Technology Organization’s (ANSTO) 20 MW OPAL nuclear research reactor, Sydney, Australia. For this measurement the instrument was equipped with a 2048 × 2048 pixel ANDOR iLon 16-bit CCD camera, 100 mm fixed focal length Carl Zeiss lens and 30 μm thick terbium-doped Gadox scintillator screen (Gd2O2S:Tb, RC Tritec AG) to yield 43.0 × 43.0 × 43.0 μm voxels. To maximize spatial resolution, a collimation ratio (L/D) of 1,000 was used to achieve highest available spatial resolution, where L is the neutron aperture-to-sample length and D is the neutron aperture diameter.
A total of 800 equally-spaced angle shadow-radiographs were obtained every 0.225° as the sample was rotated 180° about its vertical axis. Both dark (closed shutter) and beam profile (open shutter) images were obtained for calibration before initiating shadow-radiograph acquisition. To reduce anomalous noise, a total of three individual radiographs with an exposure length of 14s were acquired at each angle (Mays et al., 2017) for a total scan time of 13.5 h. The individual radiographs were summed in post-acquisition processing using the “Grouped ZProjector” plugin in ImageJ v.1.51h in accordance with our previous measurements (Gee et al., 2019), and tomographic reconstruction of the 16-bit raw data accomplished using Octopus Reconstruction v.8.8.
Individual orthographic slices were used in the production of Figures 8, 11. Stacked orthographic slices were used to form a three-dimensional isomorphic image that is the basis for Figures 10, 12. Data was rendered using ImageJ and Avizo 9.3.0. The unprocessed 16-bit TIFF files are currently part of an ongoing examination of captorhinid anatomy and as such are not yet available publicly. Researchers wishing to access the material should contact the authors directly.
Systematic Paleontology
Eureptilia Olson, 1947
Captorhinomorpha Watson, 1917
Captorhinidae Case, 1911
Captorhinus (Cope, 1895)
C. kierani sp. nov.
Specific Etymology
The new species is named after Kieran Davis who is an amateur collector with undergraduate training in Physical Geography from the University of Coventry and extensive collecting experience, most notably Devonian microfossils of Tennessee.
Diagnosis
A single-tooth rowed small to medium-sized captorhinid characterized by three autapomorphies: 1- presence of well-developed recurved dentition on the apex of parasphenoid plate, at the base the basipterygoid processes, which is distinguishable from an absence of recurved dentition in the same region of the palate in Captorhinus aguti, 2- teeth along the jaw mid-line (tooth position 6–10) possessing a distinct anteromesial descending ridge (carina) nearly reaching the gum-line, and 3- The occiput is unique in the possession of a deep horizontally oriented sulcus extending along the exposed surface of the paired postparietals. There are additional un-polarized character traits that can be used to distinguish Captorhinus kierani from other members of the genus. These include: evidence for 21 maxillary and 18–20 dentary teeth, possession of a nearly horizontal (oriented 70° to the long axis of the skull) suture between the frontal and nasal, which distinguishes this taxon from Captorhinus magnus, where a 45° angle, relative to the skull midline, is formed between the frontal and nasal.
Holotype
Subadult complete skull (OMNH 73281a), preserved next to a large Acheloma skull (OMNH 73281b). Left mandible preserved slightly separated from the skull and extending into the palatal area of Acheloma. It was not possible to extricate the left mandible from the Acheloma skull, hence we decided to assign the same number to both specimens and apply the designation a/b as separators. A single humerus (left), along with additional vertebral fragments are preserved with the original material of Acheloma. Finally, the axial vertebra is preserved in situ in between the braincase and cheek.
Referred Specimen
Isolated, complete right dentary (ROMVP 80229) ~56.5 mm in length with 18 teeth, 16 of which are complete.
Occurrence
Dolese Brothers Limestone Quarry, Richards Spur, Comanche County, Oklahoma, USA; Lower Permian (Leonardian), dated to c. 289 Ma by Woodhead et al. (2010), making the locality lowermost Artinskian according to the current chronostratigraphic system of Cohen et al. (2013).
Description
The holotype was previously figured by LeBlanc and Reisz (2015) and tentatively identified as Captorhinus magnus and erroneously provided with the specimen number BMRP 2005.3.1. Although this original assignment has been shown to be incorrect—notably regarding aspects of the palate and the marginal dentition, it did demonstrate the degree of similarity between all current members of the genus Captorhinus. The present study has benefited from a high-resolution neutron scan, which has permitted an extensive examination of the entire anatomy of the skull. In particular, it was possible to determine the exact configuration of the marginal dentition, of the palatal dentition, and the specific details of the tooth crown morphology. The new material (OMNH73281a) exhibits a suite of anatomical characters that are suggestive of the anatomy of other single-tooth rowed members of the genus, and as such a comparison with the type material of C. magnus and Captorhinus laticeps (FMNH-UC642), as well as one additional and very well-preserved specimen of C. laticeps (OUSM15102), were considered in the comparative study that follows. In addition to the holotype (Figures 1–3), an isolated right dentary (ROMVP 80229—Figure 4) collected from the same fossiliferous pocket at Richards Spur is, on the basis of dental morphology, referred to same species.
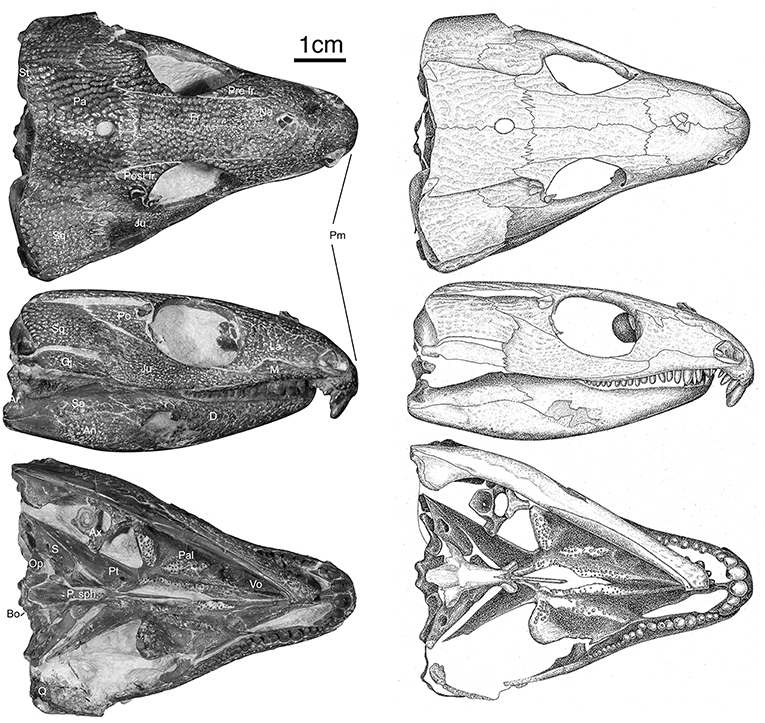
Figure 1. OMNH73281a – Specimen photographs and drawings of Captorhinus kierani. Photograph and illustration by Diane Scott, University of Toronto Mississauga.
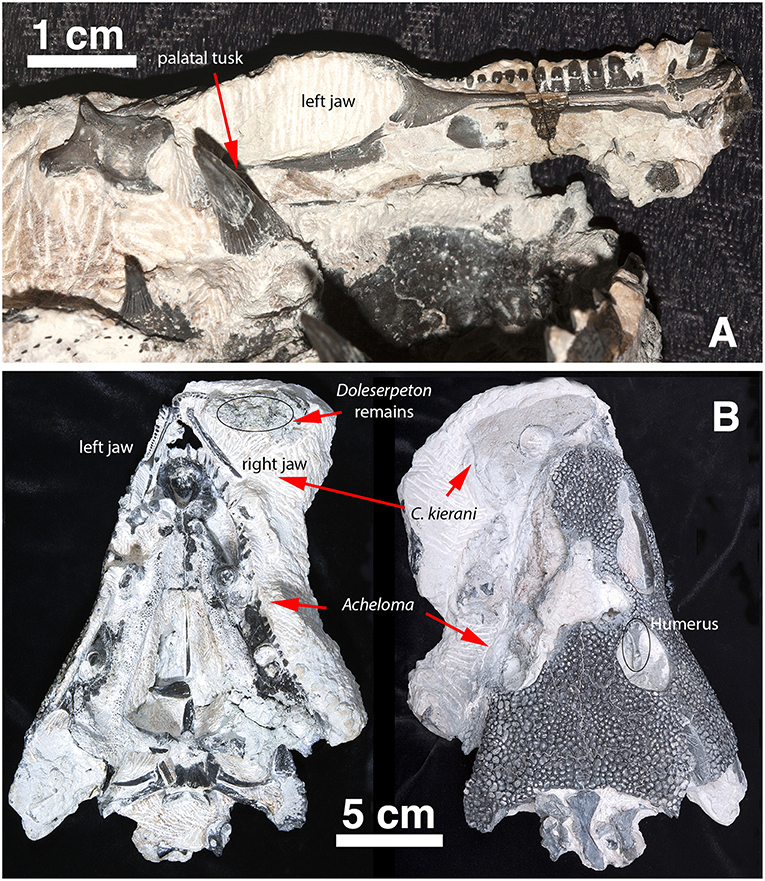
Figure 2. Captorhinus Kierani in association with Acheloma as collected. (A) Left lower jaw of Captorhinus kierani as preserved in association with the anamniote Acheloma. Note the large fang (left foreground) lying mesial to the posterior region of the lower jaw of the holotype. (B) C. kierani and Acheloma in situ in ventral (left) and dorsal (right) aspect. Note the left humerus of C. kierani showing through the orbit of Acheloma in image (B) as viewed dorsally.
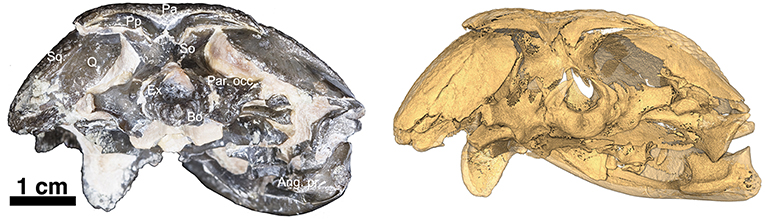
Figure 3. OMNH73281a – Occipital view of Captorhinus kierani. Photograph (Left) of the occiput and isomorphic rendering of neutron scan (Right) showing the occipital elements. Note the incomplete ossification of the opisthotics which support the sub-adult status of the specimen. Also, note how the post-parietals are restricted to the occipital margin of the skull, lack the ornamental sculpting that is found in Captorhinus aguti, and are excavated along their horizontal axis producing an elongate sulcus—a trait diagnostic of the species.
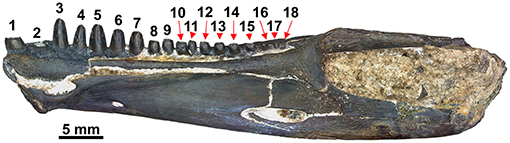
Figure 4. Isolated right dentary of referred specimen (ROMVP 80229) in mesial view. Note the abrupt decline in tooth size between the 7th and 8th tooth.
The holotype is preserved in almost pristine condition and represents an allochthonous deposition of type II characterized by chalky, calcareous deposits containing very fine crystalline inclusions and clastic-rich sediments (MacDougall et al., 2017). This type of preservation is one of three described by MacDougall et al. (2017) and represents the conditions that appear most likely to contain articulated material. The specimen displays no significant crushing in any dimension (Figures 1, 2); it is ~66 mm in length measured from snout tip to occiput and represents a sub-adult as interpreted by the sutural arrangement of the elements of the skull table and the degree of ossification of the braincase. The right mandible is preserved and in articulation with the skull as in life; the left mandible is preserved in association with a large trematopid specimen which is at present difficult to extricate. Indeed, the entire specimen appears as though it was trapped in the jaws of the trematopid. The presence of a large clump of disarticulated material likely belonging to Doleserpeton was found in the area that would comprise the gullet and buccal cavity of the specimen being described. One cannot help but consider the possibility that it represents the regurgitated stomach contents of that specimen. Possible taphonomic interpretations of the events leading to the death and preservation of the material are, however, beyond the scope of the present paper and will not be discussed further.
In addition to the captorhinid cranial material, there were a number of postcranial elements that were initially part of the fossil slab that included a large trematopid and the captorhinid specimen. Preparation of the trematopid resulted in the exposure of a captorhinid scapulocoracoid and humerus in the anatomically appropriate positions while in life. Along with these two appendicular elements, a number of other elements including vertebral elements were contained within the buccal cavity of the large amphibian. At the time, it was decided to pursue the preparation of the Acheloma specimen (Polley and Reisz, 2011), which necessitated sacrificing all of the captorhinid postcranial material save for a single vertebral element, which was preserved in association with the captorhinid skull—notably in the gap between the right quadrate ramus of the pterygoid and the jaw margin. This lone postcranial element of the captorhinid specimen, representing the axis, is sufficiently well preserved to permit a reasonably detailed description.
Skull
The skull table in Captorhinus kierani is not unlike that described for Captorhinus aguti, although the specimen is larger (>60 mm) than mature C. aguti skulls (~45 mm). The difference in size cannot be ignored, as C. aguti material that has been formally described and specimens at our disposal for study show fully grown members, as determined by the ossification of the braincase, to reach a size of ~45 mm. This contrasts with the material described here, where a specimen 66 mm in skull length contains an incompletely ossified braincase. Nevertheless, the general sutural pattern is essentially the same as that which has been described for C. aguti and by extension Captorhinus laticeps, as reconstructed by Heaton (1979), with the possible exception that the post-orbital region of the skull is not as broadly expanded as in C. aguti and more closely approximates the form described for C. laticeps (Figures 5, 6) and Captorhinus magnus. However, while the overall similarity of the skulls of C. magnus and C. kierani may be quite similar, there are some notable differences, as in the relationship between the frontals and nasals, when comparing OMNH73281a to that of C. magnus. In both specimens of C. magnus, where the condition can be determined (OMNH56820 & OMNH 56821), the frontal forms a diagonal interdigitating suture with the nasal at an angle of ~45° to the long axis of the skull (Figure 7A). Contact between the anterior margin of the frontals and the posterior margin of the nasals forms a nearly 90° angle in C. aguti and exceeds 70° in the new captorhinid (OMNH73281a – Figure 7B) as well as both the holotype of C. laticeps (FMNH-UC642) and the McCann quarry specimen (OUSM15102). Given that a nearly right-angle relationship exists in the suture between the frontal and nasal in Rhiodenticulatus and protorothyridids, the condition in the new taxon is evidently primitive for members of the genus Captorhinus, with C. magnus showing the derived condition.
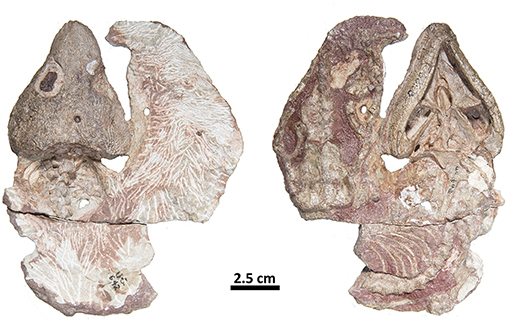
Figure 5. FMNH-UC642. Holotype of Captorhinus laticeps in dorsal (Left) and ventral (Right) views. This specimen contains the skull and post-cranial elements, including shoulder and pelvic girdles. Over the last century additional preparation has resulted in significant damage to most of the exposed surface.
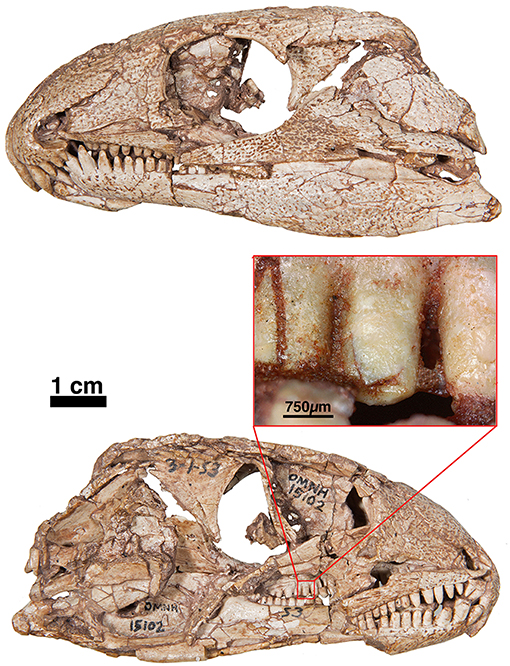
Figure 6. OUSM15102—Captorhinus laticeps in left and right lateral views with an offset close-up view of the marginal dentition in mesial view. Note the peg-like form of the teeth. Unlike the teeth found in Captorhinus kierani, there is no observable descending antero-mesial ridge on any of the teeth. Prominent carinae along the lateral mesiodistal surface also appear to be absent.
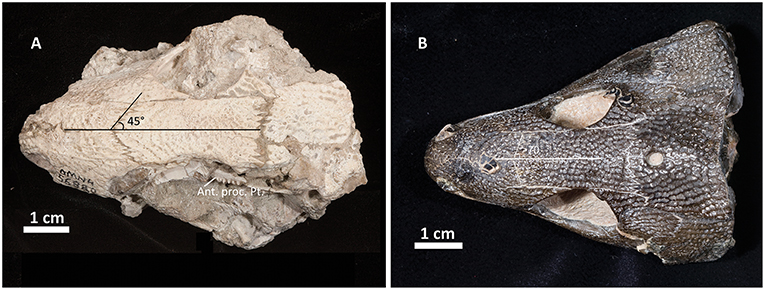
Figure 7. Comparison of the skull table between Captorhinus magnus (A) and Captorhinus kierani (B). Note how the angle formed between the nasals and frontals is much more acute in the former.
At the back end of the skull, Captorhinus kierani presents a more primitive condition, regarding the configuration of the paired postparietals, than that which is expressed by Captorhinus aguti. C. aguti, possesses a pair of narrow, rectangular (longest dimension mesiolaterally) post-parietal elements that contribute dorsally to the skull roof. The region that contributes to the skull roof is ornamented with the same sculpting pattern that is typical of all members of the genus Captorhinus. This anatomy is autapomorphic for C. aguti and distinguishes it from Captorhinus magnus (OMNH56821), and Captorhinus laticeps, including the holotype (FMNH-UC642) and the McCann Quarry specimen (OUSM15102), where the postparietals are restricted to the occipital face and lack any ornamentation. The condition in OMNH73281a, although primitive with respect to the configuration of the post-parietals in C. aguti is nevertheless derived in the presence of an embayed sulcus (Figures 1, 4), which continues as a slender process underneath the supratemporal. In more basal taxa such as Rhiodenticulatus and Euconcordia, the postparietals are also restricted to the occipital face but are much more symmetrical in outline, producing an almost square outline – the apparent primitive condition for the clade.
One final point, regarding the anatomy of the skull, which may help to more fully distinguish members of the genus Captorhinus from more basal forms, is the presence of a distinct bend or lateral deflection of the maxilla near the 9th tooth position. The result is a noticeable kink in the jaw. In Captorhinus magnus, Captorhinus aguti, and Captorhinus kierani, the marginal dentition displays this deflection (LeBlanc and Reisz, 2015). This relationship between the anterior most teeth on the maxilla and their more posterior neighbors can be easily distinguished in the orthographic slice image of the neutron scan of the holotype material (Figure 8). In the material previously identified as Captorhinus laticeps, at least in the case of the holotype (FMNH-UC642), a bend or lateral deflection of the maxilla is not evident but cannot be ruled out.
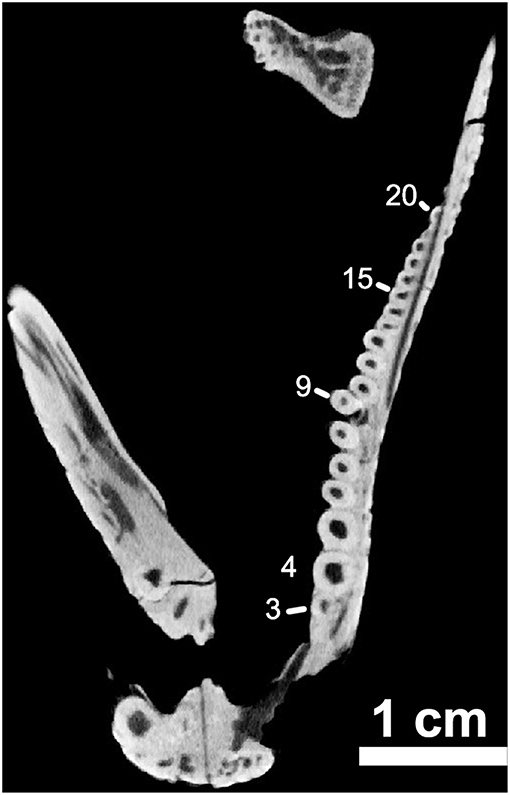
Figure 8. OMNH73281a – orthographic slice of the maxillary dentition in palatal view. Note the distinctive “step” at the ninth tooth position on the left maxilla (Note – 7th visible tooth of section).
Palate
The palate of Captorhinus kierani presents the typical morphology described for single-tooth rowed captorhinids (Heaton, 1979; Modesto, 1998). It is 32 mm across at its widest point (i.e., measured at the transverse flange of the pterygoid) and ~3X as long when measured from the anterior limit of the vomer posteriorly to the posterior limit of the quadrate rami (Figures 1, 10). Three prominent tooth bearing ridges can be found which extend anterolaterally from the region formed by the articulation between the transverse flange of the pterygoid and the basipterygoid articular surface. Two of the ridges, the most lateral of the three and the most medial, are formed exclusively by the pterygoid (including the transverse flange), whereas the central ridge begins on the pterygoid and extends anteriorly onto the palatine.
Vomer
The vomer, in ventral view, is a narrow rectangular element that occupies the anterior portion of the palate. Its posterior margin abuts in an anterolaterally directed interdigitating suture with anterior border of the palatine. Posteromedially, the vomer forms a slightly diagonal suture where it meets the lateral border of the anterior process of the pterygoid. Architecturally, the posterior most end of the vomer demarcates the region of the skull where the snout or maxilla deflects posterolaterally at an angle of ~30° from the midline, resulting in the prototypical expansion of the back end of the skull so characteristic of the heart-shaped skull in captorhinids. Throughout most of its length, the lateral margin of the vomer forms the medial boundary of the internal nares.
The exposed ventral surface is smooth lacking any palatal dentition; it is deeply concave throughout resulting in a prominent sulcus that runs the entire length of the vomer. Unlike the condition in Captorhinus aguti and possibly in Captorhinus laticeps (Heaton, 1979; Modesto, 1998), where the lateral margin of the vomer develops a thin, sharp ridge that tapers both anteriorly, upon contact with the premaxilla, as well as posteriorly, where it contacts the palatine, the vomer of Captorhinus kierani possesses a broader more rounded lateral margin that extends throughout its length (Figures 1, 10). The medial margin contacts the lateral margin of the anterior process of the pterygoid along a ridge that forms the medial boundary to the vomerine sulcus.
Palatine
The palatine presents a broad palatal element which contains one prominent tooth-bearing ridge along its midline. This tooth-bearing region extends posteromedially onto the pterygoid. The palatine is bounded by the anterior ramus of the pterygoid medially and the transverse flange of the pterygoid along its posterior margin. Anteriorly, the palatine is sutured to the posterolateral margin of the vomer through an interdigitating suture. At the point of contact between the palatine and vomer, the former contributes to the posteromedial margin of the internal nares forming the rounded posterior narial boundary. The lateral margin of the palatine is sutured to the maxilla along most of its lateral extent reaching posteriorly to the suborbital foramen. At this point, as in Captorhinus aguti and Captorhinus laticeps, the palatine forms the anteromedial border to the suborbital foramen.
Pterygoid
The pterygoid represents the most prominent palatal element and Captorhinus kierani exhibits an anatomy that is superficially similar to that of Captorhinus laticeps. The nature of the exquisite preservation is such that the precise form of the pterygoid can be determined. Anteriorly, the pterygoid possesses an anteromedially directed ramus which is tapered anteriorly and contributes to the boundary of the interpterygoid vacuity that surrounds the cultriform process of the parasphenoid. This anterior pterygoid process then abuts its partner just anterior to the cultriform process and terminates as a fine point. Along its lateral margin, the anterior process of the pterygoid is sutured to the vomer along a pronounced ridge which is continuous with the vomer. The pterygoid portion of this ridge bears a prominent set of teeth along its entire medial margin, which continues posteriorly until the level of the transverse process.
Unlike the dental configuration exhibited on the exposed surface of the anterior process of the pterygoid in Captorhinus aguti and Captorhinus magnus, where a single row of teeth appears to be present along most of its entirety and certainly in the region nearest the transverse flange, the anterior process of the pterygoid in Captorhinus kierani possesses a varied morphology. The isomorphic rendering of the neutron scan of C. kierani (Figure 10), shows a very clear set of between one and three distinct rows of teeth depending on the region of the pterygoid being examined, starting with two rows nearest the transverse flange, reducing to a single row, for a short distance along the margin of the interpterygoid vacuity, and expanding to three rows at about the mid-length of the interpterygoid vacuity, then decreasing to a single row at approximately the level of the internal nares. Multiple rows of teeth on the anterior process of the pterygoid can be observed in a number of specimens of Captorhinus laticeps figured by Heaton. Unfortunately, the holotype (FMNH-UC642) is damaged and much of the palatal surface is now gone. Williston (1909) described the presence of two rows of teeth along the medial margin of the anterior pterygoid process. However, the surface of the palate has since sustained damage and these features are no longer visible (also see Heaton, 1979). Although this region of the palate displays an array of dental arrangements, this dentigerous platform is certainly more extensive than the single row found in Captorhinus aguti and Captorhinus magnus (MdB—personal observation).
Posterior to the vomerine-pterygoid contact, the pterygoid contacts the palatine along a smooth and a slight posterolaterally directed suture. This sutural contact extends posteriorly until about the region that corresponds with the posterior extent of the maxilla as in Captorhinus aguti. At this point, the pterygoid is expanded laterally, being sutured to the posterior margin of the palatine along a tight interdigitating suture terminating where it makes a sutural contact with the medial margin of the pterygoid process of the jugal. Posterior to the pterygoid-jugal contact, the pterygoid expands slightly laterally resulting in a prominent surface that distinguishes it from the condition in C. aguti, where the lateral most margin of the pterygoid forms a confluent, slightly diagonally inclined margin, extending uninterrupted from the pterygoid-jugal contact to the transverse process of the pterygoid.
Along the lateral border of the palate where the palatine, pterygoid and pterygoid process of the jugal make contact we find the suborbital foramen. This area can be examined through a series of orthographic slices that were the result of a neutron scan of the type of Captorhinus kierani. The image shows (Figure 11) a foramen that is bordered by the pterygoid process of the jugal laterally and the palatine medially. There appears to be no direct contribution to the suborbital foramen by the pterygoid, which is identical to the condition found in Captorhinus aguti. At present the anatomy of this region in Captorhinus magnus is unknown.
The region of the pterygoid associated with the transverse process owns three distinct tooth bearing ridges. The most medial tooth bearing ridge was described above where the anterior process of the pterygoid reaches the vomer and will not be discussed further. The remaining two tooth bearing regions are concentrated in the region that corresponds with the transverse process and do not differ significantly from the condition found in Captorhinus aguti. The medial tooth bearing region radiates diagonally from the transverse process anteriorly and onto the palatine. The last tooth bearing region is found on the transverse process. This region displays a battery of teeth which is typical of the condition found in C. aguti and Captorhinus laticeps.
The transverse processes are strongly deflected ventrally dropping well below the level of the pterygoid body (Figure 12) resulting in a deep expanse, presumably associated with a change in the orientation of the muscle fibers of the m. pterygoideus. It appears that the region associated with the transverse process for the right pterygoid is preserved as it would appear in life while the left side is displaced ventrally yielding a more prominent ventrally directed expansion of the transverse process. Nevertheless, it appears that the degree to which the transverse process of this specimen is directed ventrally is much more extensive than that which we see in Captorhinus aguti.
Posterior to the transverse process, the paired pterygoid elements deflect to the mid-line where they articulate with the basipterygoid processes of the parasphenoid. Immediately posterior to the basicranial articulation the paired pterygoids then deflect laterally, as the quadrate rami, which are reminiscent of the condition found in Captorhinus aguti. The region associated with the pterygoid-basipterygoid contact is fairly similar to the condition found in C. aguti as the basipterygoid tubera articulate with the paired pterygoid elements.
Quadrate
The quadrate is incompletely preserved in OMNH73281a, but it does appear to possess the same basic morphology as found in Captorhinus aguti. The articular surface of the quadrate is represented by an unfinished surface which belies the immature nature of the specimen.
Braincase
The braincase complex is described below with the greatest attention paid to identifying elements that can be directly compared to the other recognized members of the genus Captorhinus. In some cases, due primarily to the incompletely ossified nature of much of the braincase, a complete description is not possible.
Parasphenoid-Basisphenoid Complex
As in Captorhinus laticeps, there does not appear to be any indication of a suture between the parasphenoid and the basisphenoid resulting in a fused basiparasphenoid element (Figures 1, 10). Anteriorly, the basiparasphenoid extends into a long, slender cultriform process as in most other amniotes. Most notably, the basiparasphenoid possesses a small tooth bearing region that is found on a low ridge rising from the region between the basipterygoid processes. At least two teeth and possibly the remnants of a third can be seen to form a single row of teeth that resemble the dentition, in form and size, that are found on other palatal tooth bearing surfaces. Although Fox and Bowman (1966) illustrate parasphenoid teeth in at least one specimen attributed to Captorhinus aguti (Fox and Bowman, 1966: Figure 18, p. 21—specimen identified as KU14749), where around 10 teeth are discernable, none of the other material described by them exhibit any teeth and no other description of parasphenoid teeth in C. aguti is known.
Heaton (1979), described a very similar anatomy for the parasphenoid of Captorhinus laticeps but did not reconstruct teeth in any of the figures of the specimens he examined. The two skulls in our possession, FMNH-UC642 and OUSM15102 do not retain the necessary preservation of that region of the parasphenoid so that we cannot confirm nor refute the presence or absence of teeth in that region.
Ventrally, in Captorhinus kierani, the parasphenoid produces a much more deeply excavated ventral surface when compared to Captorhinus aguti. In both taxa, the basiparasphenoid includes a pair of laterally positioned descending processes. Although this morphology is similar to that found in C. aguti, the condition in OMNH73281a differs in that the ventrally directed processes (crista ventrolateralis) are deeper than those of C. aguti and are deflected ventromedially to the long axis of the basiparasphenoid, forming an incomplete cylindrical “half-pipe” structure (i.e., the vidian sulcus). Posteriorly, the basiparasphenoid expands lateral to the crista ventrolateralis, ultimately forming the boundary of the fenestra ovalis where it contacts the stapedial process of the stapes. This contact is only inferred from a description by Heaton (1979), as exposure of the dorsolateral margins of the basiparasphenoid do not lend themselves to an examination along this plane. Posterior to the basiparasphenoid lies the occipital region of the braincase, which is formed by a single dorsally exposed supraoccipital as well as paired ventrolaterally positioned exoccipitals and a ventrally positioned basioccipital.
Supraoccipital
The supraoccipital is exposed in posterodorsal view (Figure 3). The general shape presents that of a saddle where the dorsally directed process articulates with the paired postparietals while the paired ventrolaterally directed extensions articulate with the exoccipital medially and the opisthotics laterally. As in all amniotes, the supraoccipital forms the dorsal border of the foramen magnum. There is a slight ridge along the midline as described by Heaton (1979) but in general the exposed surface is broader than the morphology exhibited by Captorhinus aguti (Modesto, 1998—Figure 7 p. 30).
Exoccipital and Basioccipital Complex
The basioccipital is exposed in both ventral and occipital aspect and is for all practical purposes identical to the form described for Captorhinus aguti (Fox and Bowman, 1966). The terminal end of the basioccipital includes a smooth and polished articulating surface which served as the occipital condyle for articulation with the atlantal arch. Along the dorsolateral margins of the basioccipital, a sutural contact with the left and right exoccipitals is clearly visible. Ventrolaterally, the basioccipital expands into a pair of basioccipital tubera, which receive the proximal heads of the respective opisthotics.
The paired exoccipitals are exposed in ventral and occipital aspect and form the ventrolateral margins of the foramen magnum where they are sutured to the basioccipital along their ventromedial margins. Dorsally, the paired exoccipitals display a pair of curved wings that form a portion of the dorsolateral margins of the foramen magnum on either side and contact the supraoccipital, which then contributes to the remaining dorsal boundary of the foramen magnum (Figure 3).
Opisthotic
The opisthotic is a poorly ossified element that is loosely sutured to the paroccipital process of the exoccipital dorsomedially and to the supraoccipital dorsolaterally. Distal to these sutural contacts, the opisthotic extends a laterally directed process that tapers to a column-shaped structure terminating in a roughened unfinished surface. This surface would likely have continued as a cartilaginous element that would have contacted the medial face of the occipital flange of the squamosal.
In all of its form, the opisthotic resembles the morphology of Captorhinus aguti and Captorhinus laticeps, except in the nature of its ossification. The limited degree of the ossification of either element reaffirms our conviction that this specimen (OMNH73281a) represents an immature individual—likely a sub-adult. Notwithstanding the poorly ossified nature of the distal most portion of the opisthotic, the portion that is fully ossified displays a deeply grooved surface undoubtedly for insertion of axial musculature (Heaton, 1979, p. 56). Similar in general form to the prominently grooved distal process but located ventromedially, there is a prominent sulcus, which likely accommodated fibers for the insertion of the m. longissimus capitis transversalis cervicus (Heaton, 1979, p. 56).
Stapes
In ventral aspect, the stapes presents a typical reptilian form with a broad footplate that is received by the foramen ovale at an angle of ~20° to the midline of the skull as in Captorhinus laticeps (Heaton, 1979). Distal to the footplate, the stapes tapers sharply terminating in a long element that represents the columella. Near the contact between the stapedial footplate and just before the rod-like columella, there is a prominent stapedial foramen. The general morphology of the stapes presents an element that is very similar to the form seen across captorhinids (Heaton, 1979; Modesto, 1998). However, the region of the footplate appears to be broader than in Captorhinus aguti and more like the condition seen C. laticeps. In addition, and as in C. laticeps, the stapedial foramen is oval rather than the circular form found in C. aguti.
The columella terminates short of the cheek extending laterally to about the same degree as the opisthotic, which overlies the proximal most region of the stapes (i.e., footplate), obscuring it from view. The best-preserved columella is on the right side of the skull and displays a rather poorly ossified structure with significant pitting of the preserved surface that reflects the presence of an extensive cartilaginous extracolumella. The incomplete ossification of this element attends to the general morphology exhibited by the associated palatal elements and supports the authors’ preference in assigning a sub-adult rank to the specimen.
Mandible
The mandibles of the holotype are preserved separately. The left jaw (Figure 2) remains in association with the original slab containing the anamniote Acheloma and the right jaw is in articulation with the rest of the skull of the holotype. The serendipitous nature of the preservation permits a complete examination of the entire mandible in both lateral and mesial aspects.
The mandible of Captorhinus kierani is similar to that of Captorhinus laticeps (Heaton, 1979) and resembles the form of the lower jaw found in other captorhinids, especially as it pertains to the region of the retroarticular process (Figures 9A,D). The dentary of the holotype appears derived in having a reduced number of teeth, 18 tooth positions (Note that the maxilla has 21 tooth positions), when compared to C. laticeps, which has 22 tooth positions in its lower jaw (Heaton, 1979, p. 22). Examination of the McCann Quarry specimen (OUSM 15102) of C. laticeps displays at least 20 teeth with room for an additional 2 or possibly 3 teeth. Given that the holotype of C. kierani and the McCann quarry specimen of C. laticeps (OUSM 15102) are of nearly identical size, it is unlikely that any difference in the number of teeth is related to its ontogenetic stage. Captorhinus magnus has at least 20 teeth in the dentary and a maxillary count of 22 (Kissel et al., 2002). Furthermore, an isolated right dentary (Figure 7), which was found in the same fossiliferous pocket as the new holotype specimen and which is here referred to Captorhinus kierani, presents an essentially complete jaw with 18 teeth, 16 of which are complete. As in most captorhinids, the teeth are largest at the anterior end of the jaw and gradually decline in size posteriorly. The number of teeth in the lower jaw of Captorhinus aguti are more difficult to ascertain as a result of the additional tooth bearing rows, but when examined in lateral aspect, ~16–18 tooth positions can be identified.
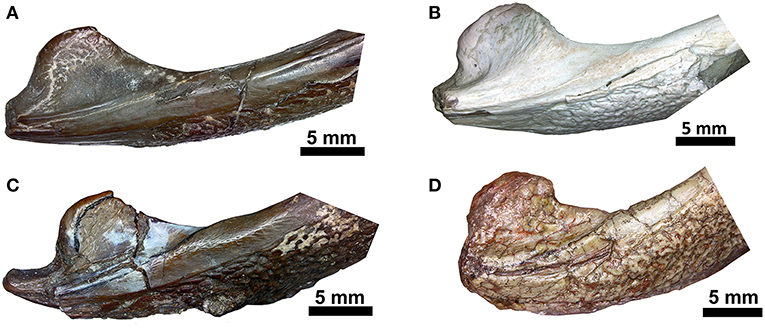
Figure 9. Comparison of the region of the retroarticular process in ventral aspect. (A) Captorhinus kierani (OMNH73281a); (B) Captorhinus magnus (OMNH56821); (C) Captorhinus aguti (OMNH52329); (D) Captorhinus laticeps (FMNH-UC642). Note that to facilitate comparison, images (A,B,D) are flipped horizontally and in life represent the right jaw.
Although the general shape and overall appearance of the lower jaw is conservative in members of the genus Captorhinus, the articular and its associated angular process, as well as the terminal retroarticular process, vary extensively within the group. Heaton (1979) described the primitive condition for the retroarticular process as being short and broad and figured what he described as the derived condition in Captorhinus laticeps, which he ascertained was identical to the condition found in Captorhinus aguti. However, Heaton based his interpretation, for the anatomy of the articular, on the basis of isolated, disarticulated remains and argued that the condition was also evident in OUSM15102 (McCann Quarry specimen). Upon re-examination of the McCann Quarry specimen as well as an examination of the holotype of C. laticeps (FMNH-UC642), we are unable to corroborate Heaton’s conclusion. The McCann Quarry specimen is too badly crushed, in the region of the retroarticular and angular processes, which makes any interpretation spurious. However, although the holotype (FMNH-UC642) has suffered extensive damage since its original description by Williston (1909), the back end of the jaw is in reasonably good condition and while some surface damage is evident, the shape and dimensions of the retroarticular and angular processes remain complete and very much resemble the anatomical condition found in Captorhinus kierani. Given the form of this region of the jaw in basal captorhinomorphs, the shorter, broader retroarticular process and the smooth ventral surface of the angular, which is found in C. laticeps, as interpreted here and contra Heaton (1979), the condition is anatomically identical to that which is found in C. kierani. This morphology is indicative of the primitive condition in these two taxa and contrasts with the anatomy expressed by C. aguti, which possesses a longer, narrower retroarticular process (Figure 9). C. magnus (OMNH56821—Figure 9B) may, however, possess a slightly longer retroarticular process that more closely approximates the configuration found in C. aguti. Nevertheless, the angle formed between the mesially directed angular process of the articular and the posteriorly oriented retroarticular process approaches 90° in both C. aguti (Figure 9C) and C. magnus (Figure 9B) whereas it is much greater (over 120°) in C. kierani (Figure 9A) and C. laticeps (Figure 9D). In addition, the ventral surface of the angular, just anterior to the angular process, is much less rugose in both C. laticeps (FMNH-UC642 and OUSM15102) specimens examined here, as interpreted in this study, C. kierani, and C. magnus, which is in strong contrast to the deeply pitted and rugose surface on the ventral surface of the angular in C. aguti. These differences obviously represent changes in the orientation of muscle fibers and reflect mechanical differences associated with the masticatory apparatuses of these taxa (C. laticeps, C. magnus, & C. kierani) when compared to the anatomy exhibited by C. aguti.
Axial Skeleton
The axial skeleton of captorhinids has been detailed by Dilkes and Reisz (1986) who outlined the general morphology of the entire vertebral series in Captorhinus laticeps. Nevertheless, axial anatomy assigned to specific captorhinid taxa is limited and as such any insight into vertebral anatomy that can be directly attributed to a formally defined member of the genus Captorhinus is relevant. To this end, we describe a solitary cervical vertebra preserved in situ and nestled between the right quadrate ramus of the pterygoid and the right mandible.
The vertebra in question represents the axis (Figures 1, 10), identified by the hatchet shaped neural spine. The centrum is complete and displays an amphicoelous condition with a prominent hole for the notochord. Ventrally, the centrum has a prominent keel which is the result of paired lateral excavations of the axial centrum.
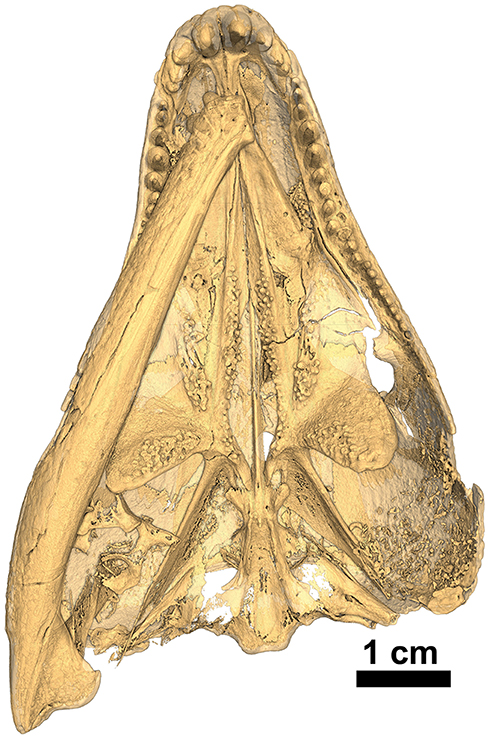
Figure 10. Isomorphic rendering of a neutron scan of the holotype of Captorhinus kierani in palatal view. Note the presence of a pair of teeth on the parasphenoid (derived condition) and the multiple rows of teeth along the medial margin of the anterior process of the pterygoid (primitive condition).
Sutured to the centrum are paired neural arches which together form the lateral margins for the neural canal with the centrum forming the ventral border. The right arch is almost entirely visible in situ where well ossified postzygapophyses can be seen. Directed ventrolaterally, the arch expands on either side into a pair of prominent transverse processes. Each transverse process extends away from the main vertebral body to an extent that is equal to the cross-sectional breadth of the centrum proper. On the left side, the transverse process is not fully exposed as it is embedded into the cheek region. However, what is exposed presents an identical morphology to its right-side partner. In lateral aspect, the transverse processes are broadest along their dorsal margin and taper ventrally, ending at about mid-centrum level as in Captorhinus laticeps (Dilkes and Reisz, 1986, Figure 1). Dorsally, the neural arches terminate in an articular facet that supports the neural spine.
The neural spine is incomplete posterodorsally, but this may reflect the immature status of the specimen. Dilkes and Reisz (1986) describe some variability in neural spine height, but do not describe the axial spine in any detail. The axial outline figured by Dilkes and Reisz (1986, Figure 6, p.1291) shows only a dotted outline for the axial neural spine and although other captorhinid axes are known, it is unclear as to whether the condition seen in this new specimen would display any diagnostically relevant features, as few vertebral ossifications are found in association with captorhinid skulls. There is a triangular concavity on the posterior surface just above the neural canal. This feature appears indistinguishable from the morphology exhibited by Captorhinus laticeps and other captorhinids. Above this concavity there appears to be a ridge as in C. laticeps, which was suggested by Vaughn (1958) as a site of attachment for epaxial musculature. However, given the incomplete nature of the neural spine no additional comment could be made on this point.
Description of Marginal Dentition—Captorhinus kierani
The marginal dentition in Captorhinus kierani is exquisitely preserved and has been prepared to exhibit all faces. There are 21 tooth positions in the upper jaw (4 in the premaxilla and 17 in the maxilla) and 20 in the dentary. Replacement pits are evident—notably at tooth position # 9 (Figure 8) in the maxilla, with the new tooth becoming displaced lingually thus producing a slight asymmetry of the jaw line, a trait that we believe is diagnostic of the genus Captorhinus.
The largest tooth in the upper jaw is represented by the first premaxillary tooth, which is almost circular, with an antero-posterior dimension at its base of 4.35 mm and a mesiolateral dimension of 3.125 mm. The exposed length of the first premaxillary tooth is ~5 mm (Figures 10–12). This tooth is followed by a slightly smaller second premaxillary tooth and two much smaller teeth for a total count of 4 on each premaxilla. The last two teeth on each premaxilla are equivalent in size to the pre-caniniform dentition on the maxilla.
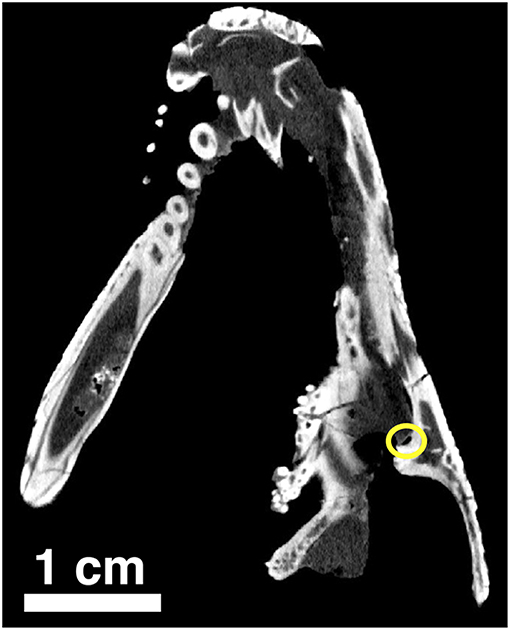
Figure 11. Orthographic slice of the palatal region of Captorhinus kierani as seen through a neutron scan. The tip of the snout is at the top of the image. Note the region of the suborbital foramen surrounded by the yellow circle.
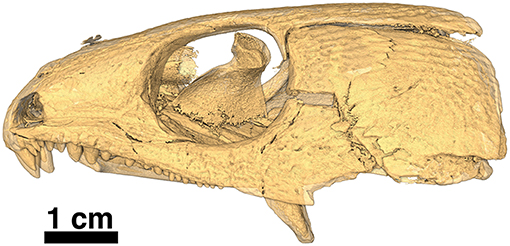
Figure 12. Isomorphic rendering of a neutron scan of the holotype of Captorhinus kierani in left lateral view. Note the absence of the lower jaw, which is part of a separate block containing the anamniote Acheloma (see Figure 2). The transverse process of the pterygoid can be seen extending ventrally, well below the tooth row.
The maxilla contains 17 tooth positions. The first maxillary tooth is small and essentially circular, with a diameter of 1.25 mm. This is in stark contrast to the first premaxillary tooth. The largest maxillary teeth are the 4th and 5th teeth or caniniform teeth, which are also circular in cross-section and have diameters of ~2.5 mm. Posterior to the 5th maxillary tooth, the diameter of the dentition drops to ~1.35 mm. This size is very gradually reduced, throughout the remainder of maxillary tooth row, culminating in the smallest tooth at the back of the tooth row, which has an approximate diameter of 1.2 mm. The diameter of the cheek teeth along the posterior length of the maxilla (i.e., tooth position 14, 15, & 16) is ~80% of the height. The ratios change when moving forward toward the tip of the snout and the cheek teeth there possess a height that is greater relative to the tooth diameter and a ratio approaching 60% is more typical.
The dentition on the lower jaw is reflective of the pattern we see in the upper jaw in as much as dimensions are concerned, but there appears to be room for only 20 teeth, one less than in the upper jaw. However, given the serendipitous nature of the preservation of the left lower jaw, which is isolated from the rest of the skull (Figure 2), additional preparation was possible allowing for a very detailed examination of the crown morphology (Figure 13A). All of the marginal teeth possess a distinct descending antero-mesial ridge, which is particularly evident in the anterior jaw region at tooth positions 6–8 (Figure 13A).
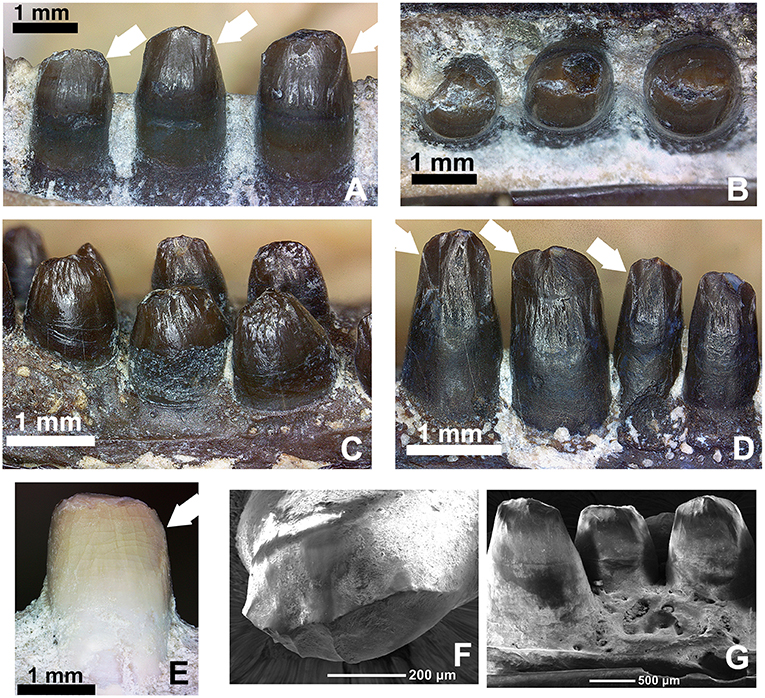
Figure 13. Comparison of the dentition in the mid-dentary region of various members of the genus Captorhinus. (A,B) OMNH73281a—Lingual and occlusal views of the left dentary teeth of Captorhinus kierani. Tooth positions right to left are 6, 7, and 8. White arrows indicate the position of the descending antero-mesial ridge. Note the nearly circular shape of tooth # 6 (far right) when exposed in occlusal aspect. (C,D) Close-up view and comparison of the marginal dentition from two uncatalogued, isolated jaw fragments. The specimen on the left is representative of the generally accepted ogival form that is characteristic of Captorhinus aguti, with short somewhat bulbous teeth. The specimen on the right displays taller teeth, which more closely resemble the dentition seen in C. kierani. It is likely that the teeth shown in the latter image do not represent C. aguti, even with the presence of what appears to be an additional tooth row (refer to Figure 14B for image of entire jaw fragment). Note the presence of the descending antero-mesial ridge on the crown of the specimen—denoted by white arrows, as found on the teeth of C. kierani and to a much lesser degree C. magnus. (E) OMNH56821. Mesial view of 7th dentary tooth of C. magnus. Note the relatively smooth surface with only a very fine ridge along the antero-mesial surface of the crown. This ventrally directed ridge is much more well developed in C. kierani. (F,G) SEM micrographs of C. aguti teeth. LEFT—Occlusal view of dentary tooth (C. aguti) & RIGHT—Lingual view of dentary teeth (C. aguti). Note the enamel boundary separating the exposed tooth crown from the gum-line and the descending ridges along the mesial surface—notably on the tooth seen on the image on the left.
Comparison of Marginal Dentition—Captorhinus spp.
With recent advances in the study of captorhinid dentition (LeBlanc and Reisz, 2015; LeBlanc et al., 2015), captorhinid teeth have been demonstrated to be much more diverse than previously imagined. Modesto et al. (2018) described a basal captorhinid, Labidosauriscus richardi, which was interpreted as retaining a similar undifferentiated marginal dentition as found in Captorhinus laticeps. Specifically, the teeth were described as “chisel-shaped” with slightly recurved anterior marginal teeth, followed by a distinct and enlarged caniniform region. The post-caniniform teeth were peg-like with no noticeable re-curvature but did possess mesially and distally expanded carinae (sensu Modesto et al., 2018), resulting in a convex labial dental surface. Heaton (1979) described a similar morphology for the teeth in C. laticeps, but examination of the holotype (FMNH-UC642) and the McCann Quarry specimen (OUSM15102) is unable to fully corroborate the degree to which the teeth of C. laticeps (Figure 5) and Labidosauriscus richardi resemble one another. The tooth count in captorhinids has been described as constituting a range from 4 to 5 premaxillary teeth, followed by 17 to 22 maxillary teeth and a dentary containing between 20 and 27 teeth (Heaton, 1979). It is important to note that Heaton based these counts on many isolated remains, including material from Texas and Oklahoma, and it is possible that these varied counts may be diagnostic at a less inclusive level.
While, the holotype of Captorhinus laticeps is too badly damaged to properly compare the marginal dentition, OUSM15102 retains slightly better-preserved teeth. Both the maxillary and the dentary tooth rows can be fully examined and present a general anatomy that is clearly distinct from that which is displayed by Captorhinus aguti. The marginal dentition in C. laticeps (OUSM15102) is noticeably taller and slender when compared to the teeth from the same region of the jaw in C. aguti. However, the surface details, such as whether there is a descending antero-mesial ridge on the marginal dentition of C. laticeps, is impossible to determine. The morphology exhibited by Captorhinus kierani produces an appearance that outwardly resembles the dentition described for C. laticeps, where the marginal dentition is tall and generally slender (Figure 5), but otherwise, the form in C. kierani is not unlike the condition found in additional, isolated dentary fragments (Figure 14B).
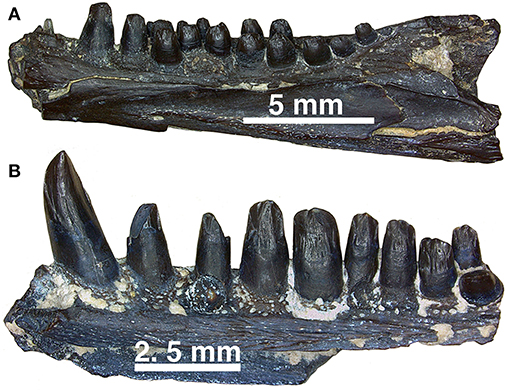
Figure 14. (A,B) Dentary fragments (uncatalogued material) currently assigned to Captorhinus aguti in mesial view. Note the difference in the relative size of the dentition between the more typical C. aguti multiple-tooth rowed form and the taller dentition expressed by the isolated fragment of another multiple-tooth rowed form, which may reflect the remains of yet another Richards Spur captorhinid. Note that while a pair of teeth are present providing support of a multiple-tooth rowed form, the nature of the crown morphology is distinctively different from remains that have been confidently assigned to C. aguti.
The caniniform region in Captorhinus kierani is much less pronounced than that which is found in Labidosauriscus richardi. In this way, C. kierani is a closer match to Captorhinus laticeps. However, the detailed surface anatomy of the marginal teeth in C. kierani, is much more closely approximated by Captorhinus magnus (Figure 13E) and a possible variant of Captorhinus aguti (Figure 13D). This similarity is especially noteworthy when comparing the mesial surface of the marginal dentition in C. kierani (Figure 13A) to an isolated multiple-tooth rowed dentary fragment of the C. aguti variant (Figure 13D). Captorhinus magnus possesses an antero-mesial descending ridge on its dentary teeth in the same region of the jaw as C. kierani, albeit much less well-developed (Figure 13E). It is noteworthy that while the marginal dentition of C. kierani and C. magnus differ in their morphology, the size of the marginal dentition is quite similar, while simultaneously being somewhat larger than teeth in the same region of the jaw in Captorhinus aguti.
Attempts have been made to try and more fully quantify the size of teeth belonging to Captorhinus aguti (Kissel et al., 2002), but these measurements have proven to be problematic as in one specimen of C. aguti, the dentition can range from circular in cross-section to linguo-labially expanded to virtually any ratio in between, with the most mesial and hence largest teeth tending to broader more fully expanded bases. The differences in ratios between tooth diameter and crown height are also problematic as the posterior-most teeth in Captorhinus kierani match the values calculated for the dentary and maxillary teeth in C. aguti (Modesto, 1996, 1998) but not in their anterior-most cheek teeth (i.e., tooth position 7 & 8—Figure 13A), which possess dimensions more typical of those calculated for Captorhinus laticeps. We suggest that these measures are spurious at best and consider a more detailed tooth crown morphology as a better indicator of taxonomic identity. The height of the teeth relative to their basal diameter is also quite variable, and although both Modesto (1998) and Kissel et al. (2002) provide an accurate representation of these dimensions, we are uncertain as to the diagnostic implications of these attributes.
The crowns of the cheek teeth in Captorhinus aguti, when examined under SEM (Figures 13F,G) and compared to those of Captorhinus magnus (Figure 13E) and Captorhinus kierani (Figures 13A,B) display a unique morphology. The nature of the derived condition, as previously articulated by Modesto (1998) and as applied in a phylogenetic construct by Modesto et al. (2018) provides us with an opportunity to explore species specific differences amongst the recognized members of the genus. A typical C. aguti tooth, located between position 7 and 15 along the marginal row (Figure 13C), presents a bulbous tooth with convex lingual and labial surfaces. These surfaces join apically, producing a raised ridge which results in the ogival condition; the result is a ridge that extends anteroposteriorly along the occlusal surface of the crown, but which does not extend onto either the anterior or posterior edges of the teeth (Figure 13C). However, there are some isolated remains from the Richards Spur cave system that have generally been accepted as belonging to C. aguti, on the basis of the presence of an additional tooth bearing row (Figure 14B), which are not quite as bulbous and possess a descending antero-mesial cutting surface or ridge that is quite similar to that which is depicted by C. kierani (Figure 13A). Although isolated remains of tooth bearing elements that have been assigned to C. magnus (i.e., collected from the same pocket and expressed as white bone) appear to exhibit an anatomy that is virtually indistinguishable from the material presently being studied, we question the affinity of this material and cannot rule out the possibility that this material used by Modesto et al. (2018) and Kissel et al. (2002) to assess morphological differences amongst Richards Spur captorhinids can be confidently assigned to C. magnus. Further preparation of the mesial surface of a C. magnus specimen (OMNH56821, Figure 13E) depicts a dentition that lacks the antero-mesial descending ridge that is described for C. kierani (Figure 13A) and which is also found on the isolated dentigerous fragments (Figures 13D, 14B).
Examining isolated jaw fragments of Captorhinus aguti displays a rather notable range in the dimensions and ratios of the marginal dentition (Figures 13C,D, 14A,B). Whether these teeth represent a variant of the same species (C. aguti) or are indicative of two separate, multiple-tooth rowed forms must await further study. In addition, although all multiple-tooth rowed forms are generally accepted as belonging to C. aguti, it is noteworthy to point out how the dentition exhibited in Figure 13D more closely approximates the dental anatomy expressed by Captorhinus kierani (Figures 13A,B). Furthermore, comparison with the dental morphology found in Captorhinus magnus (Figure 13E), which was previously described as ogival and used as a synapomorphy uniting it with C. aguti (Kissel et al., 2002), exhibits instead what might be described as an intermediate form.
Therefore, it appears that among the known members of the genus Captorhinus, the general morphology of the dentition expressed by Captorhinus kierani is most like that of Captorhinus laticeps, at least as far as proportions are concerned. However, details of the crown anatomy in C. laticeps are, unfortunately impossible to fully survey as both the holotype (FMNH-UC642) and the McCann Quarry specimen (OUSM15102), which were available for study, lack the necessary preservation for rendering a confident interpretation. As a result, this undermines our ability to fully assess the relevance of the differences in the dental morphology between what is phylogenetically the most basal member of the genus Captorhinus (i.e., C. laticeps) and more derived members of the clade such as C. kierani, Captorhinus magnus and Captorhinus aguti. Furthermore, although we concur with Kissel et al. (2002), that the dentition in C. magnus represents a derived state, relative to C. laticeps, with proportions that approach those of the ogival condition found in C. aguti, we disagree with their conclusion that the dental configuration as seen in C. magnus (Figure 13E) is anatomically identical to the condition in C. aguti (Figures 13C,F,G, 14A). Indeed, the nature of ogival dentition associated with the cheek teeth of C. aguti and described in detail by Modesto (1998) presents a unique morphology that is diagnostic at the species level and hence is informative only when distinguishing between C. aguti and other members of the genus Captorhinus.
Discussion
The genus Captorhinus has drawn a great deal of attention in the last decade (Modesto et al., 2007, 2018, 2019; LeBlanc and Reisz, 2015), notably due to a renewed interest in studying the dental morphology within the clade. This description has also pointed out differences associated with the skull table and notably the relationship of the front-nasal suture. However, aside from the difference in the angle formed by the frontals, there are no other notable differences between Captorhinus aguti, Captorhinus magnus and Captorhinus kierani that can be associated with the skull table. This is not surprising as the general surface anatomy of the skull is quite conservative across all smaller members of the clade in general, and the genus Captorhinus in particular. However, notwithstanding the number of tooth rows, the detailed morphology of the dentition is much more informative, especially when examined relative to other tetrapods.
Captorhinids are distinguished from other contemporary tetrapods by their unique dental morphology, specifically the ogival condition described by Modesto (1998) and Kissel et al. (2002), who included this trait as a diagnostic feature of Captorhinus magnus. Later, Modesto et al. (2007) used Kissel et al.’s study to support the sister-group relationship between C. magnus and Captorhinus aguti. The ogival morphology described for C. aguti, however, is quite distinct from the morphology exhibited by C. magnus and we question the value of the ogival trait as a synapomorphy uniting the two taxa. Unlike C. aguti, C. magnus retains an antero-mesial descending ridge (carina) on the marginal dentition associated with the dentary, albeit much less conspicuous than that which is found in the new material; the latter possesses a more pronounced ridge that extends ventrally to the level of the gum-line. Comparison of the dental morphology of the new material with that of C. laticeps is not possible as the holotype (FMNH-UC642) is too poorly preserved to permit a detailed comparison, and while the McCann quarry specimen (OUSM15102) is better preserved, the surface detail of the dentition is insufficient to determine its specific morphology relative to that which is displayed by Captorhinus kierani.
At the outset of this investigation, three discrete species were recognized: Captorhinus aguti, Captorhinus magnus and Captorhinus laticeps. However, the discovery of a new specimen (OMNH73281a), described here, provides us with an opportunity to re-examine the current composition of the genus, and dental variation within it. Of greater relevance is the nature of the Richards Spur locality and its ability to shed light on the taxic diversity that marked this Permian locality. Much of the focus on exploring the taxic diversity has been propagated by the discovery of numerous fossil remains comprising a wide range of Paleozoic tetrapods, including the abundance of Captorhinus spp. remains. However, given the nature of the preservation, which results in primarily disarticulated remains, it is the contention of the authors that assignment of fragmentary remains to particular species remains problematic at this time.
Furthermore, there has been a generally accepted view that all multiple-tooth rowed members of the genus Captorhinus are assignable to the species Captorhinus aguti. This assumption is based on the absence of any other notable characteristics that can be considered as diagnostic at the species level. However, as we have discussed here, there are at least two separate species of single-tooth rowed forms (i.e., Captorhinus magnus and Captorhinus kierani) at Richards Spur. The diagnoses are based primarily on dental differences, as iterated here, and it would not be too surprising if additional multiple-tooth rowed forms (Figure 14) also occur. Variability in dental anatomy undoubtedly evolved to minimize intra-specific competition (see Modesto et al., 2018) and with the material now available for study, combined with the unique taphonomic character of the Richards Spur locality, it is essential that we consider potential causes for variability much more critically.
Woodhead et al. (2010) evaluated the nature of the Richards Spur Cave system on the basis of speleothem samples that were determined to be indicative of a tightly controlled chronology dating to 289 ± 0.68 million years. Based on all currently available ages for the entire system, an age range between 286 and 289 Ma is possible (MacDougall et al., 2017). While the material collected from a fossiliferous pocket in 2005 cannot be precisely dated to the speleothem sample described here and hence cannot be assumed to closely bracket the 289 Ma age, the fact that it represents articulated, well preserved remains is suggestive of burial within the cave system immediately or very shortly after death. The pristine nature of the preservation of the new material, combined with its direct association with the large trematopid Acheloma (see description), along with at least two other species of Captorhinus and a number of varanopid remains, within the same pocket, is at least supportive of a more fully constrained preservational environment. However, as described by Lucas (2018), the nature of the preservation makes it difficult to ascertain a precise age for the fossil material as the speleothem age pre-dates the karst fill. Modesto et al. (2018) have previously considered the possibility that at least some of the Richards Spur fauna was contemporaneous and argued for morphological differentiation, notably in the dentition of Richards Spur captorhinids, as indicative of taxic diversity. However, while specialized niche partitioning may be the explanation for the taxic diversity, what could have been the factors that contributed to this pattern?
The speleothem data is supportive of an environment that reflects a tropical lowland habitat that would have occupied the western most region of Pangea at or very near the equator (Woodhead et al., 2010). It is likely that this region was subjected to monsoonal events, which would have rendered it subject to periods of extensive rainfall. Modesto et al. (2018) explored the relevance of the speleothem data and considered how this interpretation of the environment impacted on the taxic diversity at Richards Spur. One rather significant conclusion was that it was suggestive of a precocious fauna that was “pre-adapted” (Modesto et al.’s terminology) to more arid climatic conditions that were to become more widespread later in the early Permian and specifically in the Kungurian. Given that the Richards Spur locality is chronostratigraphically associated with the Artinskian, this would imply that Richards Spur experienced the climatic change that would come to dominate the Permian earlier than most of the other contemporaneous fossil localities that are known in Texas, New Mexico, and Oklahoma (Tabor et al., 2013). Whether these climatic events contributed to the increased dental diversity among captorhinids is compelling, but uncorroborated.
Although the diagnostic value of captorhinid dentition has been considered for over 50 years (Fox and Bowman, 1966; Bolt and DeMar, 1975; and de Ricqlès and Bolt, 1983), most of the attention focused on the presence or absence of multiple tooth rows. Nevertheless, the dental anatomy exhibited by Captorhinus kierani is, therefore, representative of yet another morphological difference in the Richards Spur fauna. It is tempting to infer that the dental anatomy of C. kierani is part of a phylogenetic morphocline, beginning with the taller, rectangular teeth of Captorhinus laticeps, leading to the slightly shorter and more bulbous teeth of C. kierani, with a distinctive antero-mesial ridge, to the even shorter teeth of Captorhinus magnus, lacking a distinct ridge, and finally to the ogival form expressed by Captorhinus aguti. However, we do not offer such an explicit phylogenetic interpretation, but instead present the diverse anatomy as an example of the niche partitioning that is supported by these differences and which is known among closely related extant lizards (Lappin and Jones, 2014).
In general, details of the dental anatomy have, in our estimation, been over-simplified, rendering their diagnostic efficacy limited. The results of this study have provided a more discrete set of character state interpretations, notably of the dentition, that should help to more fully diagnose the members of the genus Captorhinus at Richards Spur. Previously Modesto (1998) articulated a fairly complete review of the dental morphology and more recently he and a number of colleagues (Modesto et al., 2007) reiterated the importance of the nature of the specialized crown anatomy, which could be used to more fully distinguish between the various captorhinid species. We agree with Modesto (1998) and Modesto et al. (2007) in assessing the ogival nature of the marginal dentition for Captorhinus aguti and describe some additional variation in the marginal dental morphology (refer to sections 9.6 & 9.7) of OMNH73281a.
LeBlanc and Reisz (2015) and LeBlanc et al. (2015) described in detail the pattern associated with the growth and replacement patterns attributed to captorhinids and undertook an extensive histological examination of the differences between replacement patterns exhibited between single-tooth rowed members of the clade and multiple-toothed forms. Their conclusions were that there is a pronounced asymmetry in jaw growth where the mesial side of the jaw outpaces the growth rate of the labial side. Typically, new teeth must resorb the roots of older teeth and grow into the existing tooth bearing region. However, with the differential rate of growth, newly erupted teeth, along the mesial surface of the dental lamina, grow into an unoccupied area, as the region housing the older tooth migrates distally onto the labial surface. In effect, this form of replacement allows for the addition of new teeth as well as the retention of older teeth, thus producing the characteristic multiple-tooth rows. While this method of tooth replacement is well documented (Bolt and DeMar, 1975; de Ricqlès and Bolt, 1983), LeBlanc and Reisz (2015) showed that this pronounced asymmetry can also be detected in single-tooth forms (LeBlanc and Reisz, 2015—Figure 9B), where the single-tooth rowed Captorhinus magnus demonstrates a “stepped” pattern that is not unlike the condition described for the multiple-tooth rowed Captorhinus aguti. This distinctive step or more precisely bend or lateral deflection suggests an asymmetry in the growth of the jaw in both Captorhinus magnus and Captorhinus aguti and it is precisely this asymmetry that we have described for Captorhinus kierani. The impact of this developmental pattern is informative as to the evolution of the genus Captorhinus as it is suggestive of a plausible evolutionary scenario.
From a phylogenetic perspective, only members of the genus Captorhinus exhibit this distinctive step. Although other captorhinomorphs such as moradisaurines exhibit multiple tooth rows, they do now present the “stepped” pattern and likely evolved an alternative developmental pathway (LeBlanc et al., 2015; Modesto et al., 2019). Within the genus Captorhinus, the “stepped” pattern does not appear to be present in Captorhinus laticeps and at present the known material is too poorly preserved to render a verdict. It is possible that currently assigned members of C. laticeps may prove to represent separate species, but a decision on this claim must await further study. What we do know is that the most basal captorhinids such as Euconcordia, lack this step and what has been demonstrated is that the stepped pattern is an apparent precursor to the development of multiple-tooth rows in the genus Captorhinus. What we still do not know is whether the incipient step found in both Captorhinus magnus and Captorhinus kierani are an intermediate step leading toward the derived multiple-tooth rowed Captorhinus aguti, or whether it simply represents a separate developmental pattern. A definitive conclusion on this topic must await additional study. However, it is not too difficult to speculate on how altering the growth rate of the jaw in C. magnus and C. kierani could be coupled with increasing size.
When examining the known captorhinid material from Richards Spur, Captorhinus aguti is a relatively small representative for the genus with fully grown skulls, as determined by the ontogenetic stage and degree of maturity of the braincase, reaching ~45 mm in total length. On the other hand, specimens of Captorhinus magnus and Captorhinus kierani both exceed 60 mm, while containing an incompletely ossified braincase at a size that is already larger than the commonly known specimens of C. aguti. Could the increase in overall body size be responsible for a change in the differential growth rate between the mesial and distal margins of the jaw that is so critical to the development of the multiple tooth rows found in the apparently smaller C. aguti? If so, then developmental timing (heterochrony) may be responsible for altering feeding behavior across various species of contemporaneous taxa. While we cannot be certain that all of the Richards Spur fauna associated with the 2005 fossiliferous pocket represents co-existence, the discovery of at least three separate species of Captorhinus (C. aguti, C. magnus, & C. kierani), along with trematopid and varanopid remains is at least suggestive of the possibility of a contemporaneous existence.
Conclusion
What we have, therefore, is a new species of Captorhinus at Richards Spur, one that now increases the taxic diversity of the locality to three discrete species: C. aguti, C. magnus, & C. kierani (Figure 15), with a very distinct possibility of yet another multiple-tooth rowed species (i.e., as exemplified by the variable [refer to Figures 13C,D and Figures 14A,B] dental morphology of isolated jaw fragments exhibiting multiple-tooth rows but distinct from known C. aguti material) as yet undescribed. In addition to these three or possibly four species of the genus Captorhinus, there are an additional four recognized captorhinomorphs from this locality (Modesto et al., 2018). Given the conservative nature of the overall skeletal anatomy (i.e., no obvious means of distinguishing between members of the species using postcranial material—for an alternative interpretation see Kissel et al., 2002), the variation of the dental morphology is suggestive of resource partitioning (Modesto et al., 2018) and is, therefore, supportive of a taxically diverse ecology in what has been interpreted as an upland locality (MacDougall et al., 2017). Our findings are supportive of a Paleozoic environment that is predisposed to supporting an abundance of closely related species, where intra-specific competition is mitigated by dental specialization. Richards Spur, therefore, provides us with a unique window into the study of species specific interactions, which are seldom possible for extinct clades.
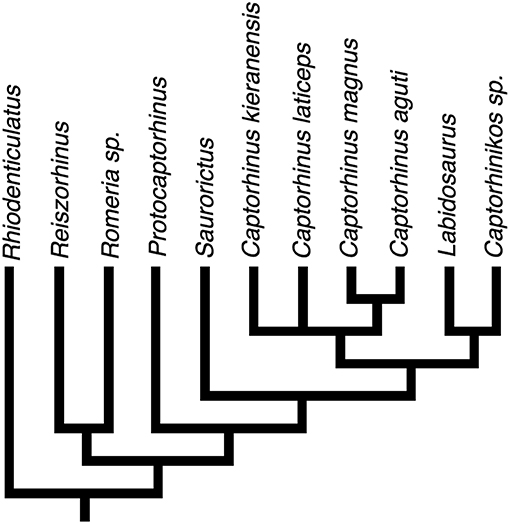
Figure 15. Overview of relationships for representative captorhinids. Note that this phylogeny does not reflect a formal analysis but is included simply as a general overview. For specific character diagnostics please refer to Modesto et al. (2019).
Data Availability
Data used to generate the orthographic and isometric figures is currently not available for public access as the scanned material is being applied in another study that is currently underway. Researchers wishing to access the raw data should contact MdB or RR.
Author Contributions
MdB drafted the manuscript and prepared all figures. RR contributed specimens and edited the final draft of the manuscript. JJB scanned the type specimen.
Conflict of Interest Statement
The authors declare that the research was conducted in the absence of any commercial or financial relationships that could be construed as a potential conflict of interest.
Acknowledgments
We thank Diane Scott for contributing to the preparation of the type material and for assistance with some of the photography. We also thank Nicola Horsman for preparing and illustrating the type specimen. We are grateful to Adrienne Stroup and Bill Simpson of the Field Museum of Natural History in Chicago for the loan of the holotype and to Richard Cifelli of the Oklahoma Museum of Natural History for the loaning of the McCann Quarry specimen. We would also like to thank the editor, Dr. Michel Laurin, for his valuable suggestions in drafting the manuscript. This research was partly funded by an NSERC Discovery Grant to RR and The International Center of Future Science, Dinosaur Evolution Research Center, Jilin University, Changchun, Jilin Province, China, and the University of Toronto.
References
Berman, D. S., and Reisz, R. R. (1986). Captorhinid reptiles from the early permian of New Mexico, with description of a new genus and species. Ann. Car. Mus. Natural His. 55, 1–28.
Bolt, J. R., and DeMar, R. (1975). An explanatory model of the evolution of multiple rows of teeth in Captorhinus aguti. J. Paleontol. 49, 814–832.
Carroll, R. L., and Baird, D. (1972). Carboniferous stem-reptiles of the family romeriidae. Bull. Mus. Compar. Zoology 143, 321–364.
Clark, J., and Carroll, R. L. (1973). Romeriid reptiles from the lower permian. Bull. Mus. Compar. Zoology 144, 353–407.
Cohen, K. M., Finney, S. C., Gibbard, P. L., and Fan, J.-X. (2013). The ICS international chronostratigraphic chart. Episodes 36, 199–204.
Cope, E. D. (1882). Third contribution to the history of the vertebrata of the permian formation of Texas. Proc. Am. Philos. Soc. 20: 447–461.
de Ricqlès, A., and Bolt, J. R. (1983). Jaw growth and tooth replacement in Captorhinus aguti (Reptilia: Captorhinomorpha): a morphological and histological analysis. J. Vertebrate Paleontol. 3 7–24.
Dilkes, D. W., and Reisz, R. R. (1986). The axial skeleton of the early permian reptile Eocaptorhinus laticeps (Williston). Can. J. Earth Sci. 23, 1288–1296.
Dodick, J. T., and Modesto, S. P. (1995). The cranial anatomy of the captorhinid reptile Labidosaurikos meachami from the lower permian of Oklahoma. Palaeontol. 38, 687–711.
Fox, R. C., and Bowman, M. C. (1966). Osteology and relationships of Captorhinus aguti (Cope) (Reptilia: Captorhinomorpha). The University of Kansas Paleontological Contributions Vertebrata 11:79
Gaffney, E. S. (1990). The comparative osteology of the triassic turtle Proganochelys. Bull. Am. Mus. Natural His. 194:263
Gaffney, E. S., and McKenna, M. C. (1979). A late permian captorhinid from Rhodesia. Am. Mus. Novitat. 2688, 1–15.
Garbe, U., Randall, T., Hughes, C., Davidson, G., Pangelis, S., and Kennedy, S. J. (2015). A new neutron radiography/tomography/imaging station DINGO at OPAL. Phys. Procedia 69, 27–32. doi: 10.1016/j.phpro.2015.07.003
Gee, B. M., Bevitt, J. J., Garbe, U., and Reisz, R. R. (2019). New material of the microsaur Llistrofus from the cave deposits of richards spur, Oklahoma and the paleoecology of the hapsidopareiidae. Peer.J. 7:e6327. doi: 10.7717/peerj.6327
Heaton, M. (1979). Cranial anatomy of primitive captorhinid reptiles from the late pennsylvanian and early permian Oklahoma and Texas. Oklahoma Geolog. Survey 127:84.
Heaton, M., and Reisz, R. R. (1980). A skeletal reconstruction of the early permian captorhinid reptile Eocaptorhinus laticeps (Williston). J. Paleontol. 54, 136–143.
Ivakhnenko, M. F. (1990). Rannepermskie elementy faunicheskikh kompleksov tetrapod vostochnoy evropy. Paleontologicheskii Zhurnal 2, 102–111.
Kissel, R. A., Dilkes, D. W., and Reisz, R. R. (2002). Captorhinus magnus, a new captorhinid (Amniota: Eureptilia) from the lower permian of Oklahoma, with new evidence on the homology of the astragalus. Can. J. Earth Sci. 39, 1363–1372. doi: 10.1139/E02-040
Lappin, A. K., and Jones, M. E. H. (2014). Reliable quantification of bite-force performance requires use of appropriate biting substrate and standardization of bite out-lever. J. Experiment. Biol. 217, 4303–4312. doi: 10.1242/jeb.106385
LeBlanc, A. R. H., Brar, A. K., May, W., and Reisz, R. R. (2015). Multiple tooth-rowed captorhinids from the early permian fissure fills of the bally mountain locality of Oklahoma. Vertebrate Anat. Morphol. Palaeontol. 1, 35–49. doi: 10.18435/B5RP4N
LeBlanc, A. R. H., and Reisz, R. R. (2015). Patterns of tooth development and replacement in captorhinid reptiles: a comparative approach for understanding the origin of multiple tooth rows. J. Vertebrate Paleontol. 35:e919928. doi: 10.1080/02724634.2014.919928
Lucas, S. G. (2018). “Permian tetrapod biochronology, correlation and evolutionary events” in The Permian timescale, eds S. G. Lucas and S. Z. Shen (London: Geological Society of London Special Publications), 405–444.
MacDougall, M. J., Tabor, N. J., Woodhead, J., Daoust, A. R., and Reisz, R. R. (2017). The unique preservational environment of the early permian (Cisuralian) fossiliferous cave deposits of the richards spur locality, Oklahoma. Palaeogeog. Palaeoclimatol. Palaeoecol. 475, 1–11. doi: 10.1016/j.palaeo.2017.02.019
May, W. J., and Cifelli, R. L. (1998). Baeotherates fortsillensis, a new captorhinid reptile from the fort sill fissures, lower permian of Oklahoma. Oklahoma Geol. Notes 58, 128–137.
Mays, C., Bevitt, J., and Stilwell, J. (2017). Pushing the limits of neutron tomography in palaeontology: three-dimensional modelling of in situ resin within fossil plants. Palaeontolica Electronica 20, 1–12. doi: 10.26879/26808
Modesto, S. P. (1996). A basal captorhinid reptile from the fort sill fissures, lower Permian of Oklahoma. Oklahoma Geol. Notes 56, 4–14.
Modesto, S. P. (1998). New information on the skull of the early permian reptile Captorhinus aguti. PaleoBios 18, 21–35.
Modesto, S. P., Flear, V. J., Dilney, M. M., and Reisz, R. R. (2016). A large moradisaurine tooth plate from the lower permian of Texas and its biostratigraphic implications. J. Vertebrate Paleontol. 36:e1221832. doi: 10.1080/02724634.2016.1221832
Modesto, S. P., Lamb, A. J., and Reisz, R. R. (2014). The captorhinid reptile Captorhinikos valensis from the lower permian vale formation of Texas, and the evolution of herbivory in eureptiles. J. Vertebrate Paleontol. 34, 291–302. doi: 10.1080/02724634.2013.809358
Modesto, S. P., Richards, C. D., Ide, O., and Sidor, C. A. (2019). The vertebrate fauna of the Upper Permian of Niger—X. The mandible of the captorhinid reptile Moradisaurus grandis. J. Vertebrate Paleontol. doi: 10.1080/02724634.2018.1531877
Modesto, S. P., Scott, D., and Reisz, R. R. (2018). A new small captorhinid reptile from the lower permian of Oklahoma and resource partitioning among small captorhinids in the richards spur fauna. Papers Palaeontol. 2018, 1–15. doi: 10.1002/spp2.1109
Modesto, S. P., Scott, D. M., Berman, D. S., Müller, J., and Reisz, R. R. (2007). The skull and the palaeoecological significance of Labidosaurus hamatus, a captorhinid reptile from the lower permian of Texas. Zoolog. J. Linnean Soc. 149, 237–262. doi: 10.1111/j.1096-3642.2007.00242.x
Modesto, S. P., and Smith, R. M. H. (2001). A new late permian captorhinid reptile: a first record from the South African Karoo. J. Vertebrate Paleontol. 21, 405–409. doi: 10.1671/0272-4634(2001)021[0405:ANLPCR]2.0.CO;2
Müller, J., and Reisz, R. R. (2005). An early captorhinid reptile (Amniota, Eureptilia) from the upper carboniferous of hamilton, Kansas. J. Vertebrate Paleontol. 25, 561–568. doi: 10.1671/0272-4634(2005)025[0561:AECRAE]2.0.CO;2
Olson, E. C. (1965). New Permian Vertebrates from the chickash formation in Oklahoma. Oklahoma Geolog. Survey 70:70.
Polley, B. P., and Reisz, R. R. (2011). A new lower permian trematopid (Temnospondyli: Dissorophoidea) from richards spur, Oklahoma. Zoolog. J. Linnean Soc. 161, 789–815. doi: 10.1111/j.1096-3642.2010.00668.x
Reisz, R. R., Haridy, Y., and Müller, J. (2016). Euconcordia nom. nov., a replacement name for the captorhinid eureptile Concordia müller and reisz, 2005 (non Kingsley, 1880), with new data on its dentition. Vertebrate Anat. Morphol. Palaeontol. 3, 1–6. doi: 10.18435/B53W22
Reisz, R. R., LeBlanc, A. R. H., Sidor, C. A., Scott, D. M., and May, W. (2015). A new captorhinid reptile from the lower permian of Oklahoma showing remarkable dental and mandibular convergence with microsaurian tetrapods. Sci. Nat. 102:12. doi: 10.1007/s00114-015-1299-y
Sumida, S. S., Dodick, J., Metcalf, A., and Albright, G. (2010). Reiszorhinus olsoni, a new single-tooth-rowed captorhinid reptile from the lower permian of Texas. J. Vertebrate Paleontol. 30, 704–714. doi: 10.1080/02724631003758078
Tabor, N. J., Myers, T. S., Gulbranson, E., Rasmussen, C., and Sheldon, N. D. (2013). Carbon stable isotope composition of modern calcareous soil profiles in California: implications for CO2 reconstructions from calcareous paleosols. SEPM Special Publication 104, 17–34. doi: 10.2110/sepmsp.104.07
Vaughn, P. P. (1958). A specimen of the captorhinid reptile Captorhinikos Chozaensis olson, 1954, from the hennessey formation, lower permian of Oklahoma. J. Geol. 66, 327–332.
Williston, S. W. (1909). New or little known permian vertebrates. Pariotichus. Biolog. Bull. 17, 241–255.
Keywords: Captorhinus, taxic diversity, niche partitioning, dental anatomy, ogival dentition, Richards Spur
Citation: deBraga M, Bevitt JJ and Reisz RR (2019) A New Captorhinid From the Permian Cave System Near Richards Spur, Oklahoma, and the Taxic Diversity of Captorhinus at This Locality. Front. Earth Sci. 7:112. doi: 10.3389/feart.2019.00112
Received: 19 March 2019; Accepted: 29 April 2019;
Published: 15 May 2019.
Edited by:
Michel Laurin, UMR7207 Centre de Recherche sur la Paléobiodiversité et les Paléoenvironnements (CR2P), FranceReviewed by:
Spencer G. Lucas, New Mexico Museum of Natural History and Science, United StatesKenneth Angielczyk, Field Museum of Natural History, United States
Copyright © 2019 deBraga, Bevitt and Reisz. This is an open-access article distributed under the terms of the Creative Commons Attribution License (CC BY). The use, distribution or reproduction in other forums is permitted, provided the original author(s) and the copyright owner(s) are credited and that the original publication in this journal is cited, in accordance with accepted academic practice. No use, distribution or reproduction is permitted which does not comply with these terms.
*Correspondence: Michael deBraga, bWljaGFlbC5kZWJyYWdhQHV0b3JvbnRvLmNh
Robert R. Reisz, cm9iZXJ0LnJlaXN6QHV0b3JvbnRvLmNh