- 1Volcanic Risk Solutions, Massey University, Palmerston North, New Zealand
- 2Department of Geosciences, National Taiwan University, Taipei, Taiwan
- 3Institute of Earth Sciences, Academia Sinica, Taipei, Taiwan
- 4Department of Earth Science, Tohoku University, Sendai, Japan
Subduction zone magmatic budgets and crustal growth processes depend on poorly constrained intrusive:extrusive ratios of arc magmas. Published ambient noise tomography data from northeast Japan reveal upper crustal low-VS bodies, indicating elevated temperatures of plutonic rocks beneath most sites of active arc-front volcanoes, with the exception of Zao volcano. Strikingly, when small eruptions (VEI 1 to VEI 3) from arc-front volcanoes in the last 2 kyr are considered, Zao has erupted most frequently and has produced a more than three times greater tephra volume than any of the other volcanoes. We propose that the frequent low-volume volcanism at Zao is fed by dikes that traverse the crustal section rapidly, fracturing through brittle lithologies. Beneath the other volcanoes, hotter and more ductile plutonic bodies have developed through repeated intrusive activity, hindering magma transport to the surface. This positive feedback mechanism ultimately results in cataclysmic caldera-forming events. We show that the number of late Cenozoic calderas is higher above the imaged low-VS crust, consistent with this interpretation. We propose that generation of plutonic bodies is facilitated by volcaniclastic materials buried in a Miocene rift graben, which dampen fracture propagation and promote repeated sill intrusion. In contrast, transcurrent faulting has moved cold Cretaceous basement of the fore-arc range into the arc-front beneath Zao. These brittle rocks instead provide fracture pathways explored by magmas rapidly rising to the surface. The combined data imply that millions of years of crustal growth and tectonic history have a direct control on present-day volcanic eruption style.
Introduction
The relative proportion of intrusive versus extrusive magmatism in volcanic arcs, and the processes that control this ratio, have long been debated (Crisp, 1984; Wallace, 2001; White et al., 2006). Our understanding of magmatic budgets in subduction zones depends on our ability to quantify the time-integrated volumes of emplacement of subvolcanic plutons and the volcanic outputs. At present, estimated intrusive:extrusive proportions range from 10:1 to 2:1 (White et al., 2006), and this relatively large range is indicative of our current ignorance of this aspect of arc magmatism. At global scale, intrusive:extrusive ratios may depend on magma production rates and the volatile content of the primary melts, which are in turn dependent on subduction parameters and the mantle wedge structure (Turner and Langmuir, 2015a,b; Turner et al., 2016), and on crustal thickness and tectonic stresses in the overriding crust. Intrusive:extrusive ratios may thus significantly vary between different subduction zones (Zellmer, 2008; Zellmer et al., 2015).
This study turns to regional scale variations in intrusive versus extrusive magmatism, and the factors that may influence their relative proportion and associated feedbacks to volcanic activity. The NE Japan arc arguably offers one of the most extensive and detailed datasets with regard to Cenozoic geological history, present day crustal structure, and long-term as well as present-day magmatic activity in the world (Yoshida et al., 2014, and references therein). Combined with the most recent ambient-noise tomographic data of the overriding crust (Chen et al., 2018) and volcanic activity data from the Pliocene (Yoshida et al., 1999, 2014; Acocella et al., 2008) to the present day (Global Volcanism Program, 2013), we show that the processes that control intrusive:extrusive proportions are manifold, and that millions of year of crustal history can have a direct influence on present-day volcanic as well as plutonic activity. This study demonstrates that a good geological and geophysical understanding beyond subduction zone magmatism is critical in elucidating this long-standing research topic.
Geological Background
Yoshida et al. (2014) has provided a detailed review of the evolution of late Cenozoic magmatism and its link to the crust-mantle structure in the NE Japan Arc. We compiled a simplified structural and geological map (Figure 1A) with features relevant to this contribution. The Tohoku region is characterized by a series of Neogene rift basins, which developed simultaneously with the opening of the back-arc basin of the Japan Sea (Sato, 1994; Okamura et al., 1995). Bimodal volcanic activity during the opening of the Japan Sea filled these basins with sediments, including ignimbrites and reworked ignimbrites, many now altered to green tuffs (Yamada and Yoshida, 2011). Miocene to more recent faulting transects these rift basins (Figure 1A).
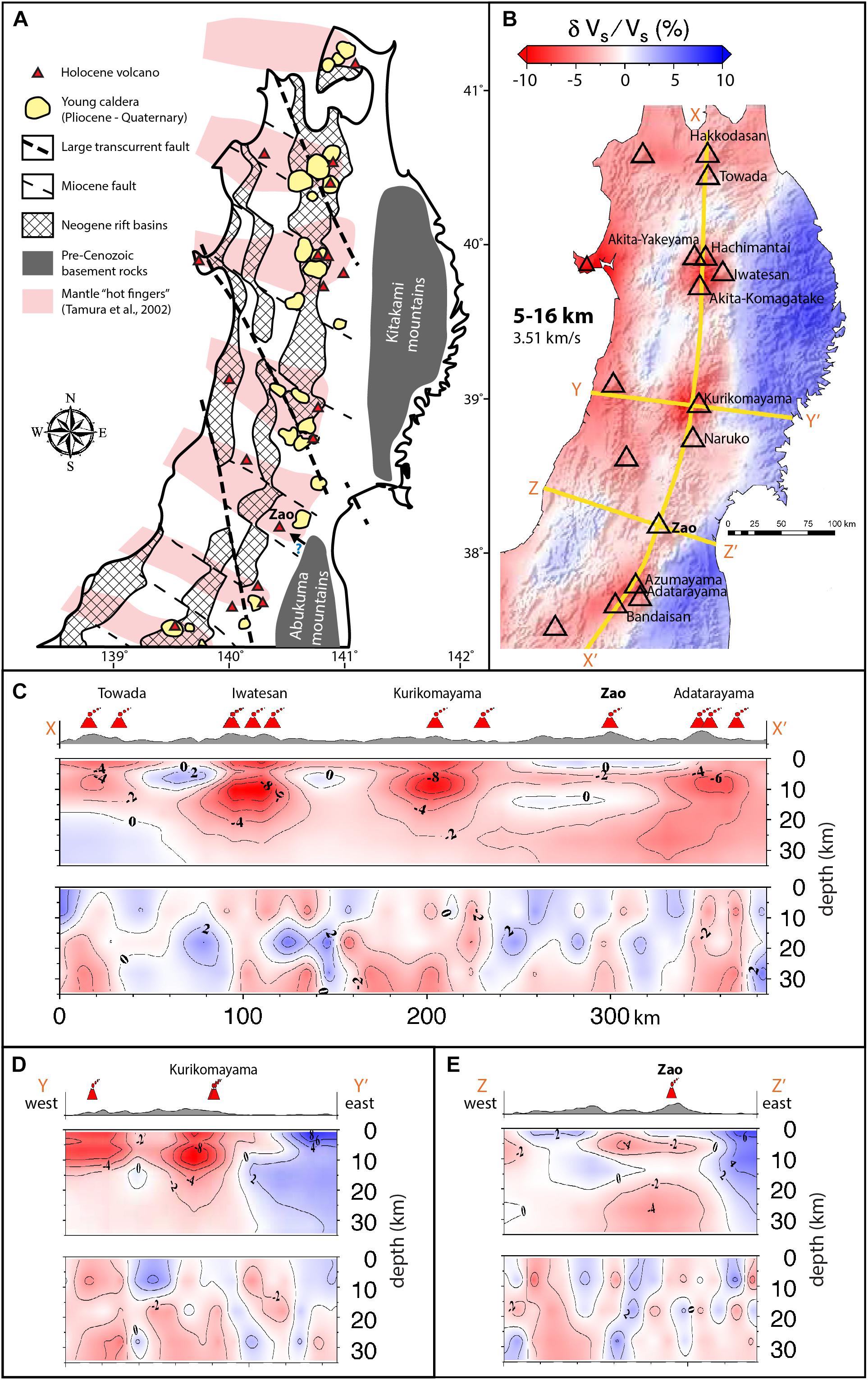
Figure 1. (A) Simplified geological map of the Tohoku region, showing major faults partially offsetting Neogene rift basins (adapted from Yoshida et al., 2014). Mantle low-velocity zones (“hot fingers” of Tamura et al., 2002) are shown, as well as the location of young (Pliocene to Quaternary) calderas (Yoshida et al., 1999; Acocella et al., 2008) and Holocene arc volcanoes (Global Volcanism Program, 2013). At depth, pre-Cenozoic basement rocks of the Abukuma mountains may have displaced (indicated by a small arrow with a blue question mark) into the area of Zao volcano through transcurrent faulting. (B) Average δVS/VS of the recent ambient noise tomography model (Chen et al., 2018) in the Tohoku region, in the depth range of 5–16 km. Note the good correspondence of the greatest arc-front anomalies with the location of young caldera clusters in panel A. Yellow lines indicate cross-sections in panels C–E. (C) Along-arc cross-section of the shear-wave velocity anomaly to a depth of 34 km. Top section shows ambient noise tomography (Chen et al., 2018), as in panel B, with highest sensitivity in the mid- to upper crust. Bottom section shows conventional body-wave tomography model (Niu et al., 2018), using local earthquakes, with higher sensitivity in the lower crust. (D,E) Across arc profiles through Kurikomayama and Zao volcanoes. Data sources and scales as in panel C.
The currently active volcanic arc front is developed on or close to the youngest, easternmost rift system. Magma generation in the mantle wedge is thought to be most productive in so-called “hot fingers” (Tamura et al., 2002), which may be related to small-scale three-dimensional convections within the mantle wedge (Honda and Yoshida, 2005). Most of the young calderas (Yoshida et al., 1999, 2014; Acocella et al., 2008) and all of the Holocene volcanoes have been linked to melt generation in these hot fingers, forming spatially separated clusters of volcanic activity (Figure 1A).
There is a significant body of literature on the crustal composition and structure of NE Japan, and many geophysical (including conventional body-wave tomographic) studies have been conducted to elucidate subvolcanic processes and lithologies (e.g., Hasegawa et al., 2000; Nakajima et al., 2001, 2006; Shibazaki et al., 2008; Zhao et al., 2011; Takada and Fukushima, 2013; Chen et al., 2018; Niu et al., 2018). Beneath the modern volcanic arc, the deep crust is likely composed of hornblende pyroxene gabbros and amphibolites, while the mid- to upper crust beneath the volcanic clusters is thought to consist of pluton complexes representing subvolcanic intrusions (Sato et al., 2002; Nishimoto et al., 2005; Nishimoto et al., 2008; Takada and Fukushima, 2013). Likely, ephemeral magma-mush bodies are also present, as indicated by caldera magmatism (Aizawa et al., 2006), but too small to be resolved by current geophysical techniques. Although Chen et al. (2018) interpreted mid- to shallow crustal low velocity zones as magma reservoirs of the Quaternary magmatic system, the magnitude of the observed shear-wave anomalies (δVS/VS < 10%) is more consistent with high-temperature intrusive rocks than with melt-rich magma chambers.
East of the arc-front, pre-Cenozoic basement rocks are exposed in the Kitakami and Abukuma mountains (Figure 1A). The latter consist exclusively of Cretaceous granitic rocks (Tsuchiya and Kanisawa, 1994; Hirahara et al., 2015, and references therein).
Methods
Yoshida et al. (1999) provides comprehensive age information of all Late Cenozoic calderas of the Tohoku province. Here, we only consider the location of the youngest calderas, formed since the Pliocene (Acocella et al., 2008).
There are two recent tomographic models of the crust beneath the Tohoku region: (i) a conventional body-wave model, with c. 10 km depth resolution and c. 0.1–0.2° lateral resolution (Niu et al., 2018); and (ii) an ambient noise (surface wave) model (Chen et al., 2018), which employs multiple scales of grid in horizontal dimension with 2–5 km thick layers in the upper to mid-crust, i.e., sampling the upper crust with higher resolution than body-wave tomography. The ambient noise approach has no errors in “earthquake” locations, because each station is treated as an earthquake, and station positions are precisely and accurately known. Uneven distribution of earthquakes that often jeopardizes body wave tomography is also avoided. Furthermore, reduced ray-crossing in the shallow crust due to near-vertical ray paths of body waves approaching the surface is remedied using surface waves. The ambient noise model thus provides improved sensitivity in the mid- to upper crust, but resolving power degrades with depth, a natural limitation of surface waves (cf. Huang et al., 2012). It is important to note that a precise, quantitative comparison of spatial resolution between two models requires synthetic modeling with artificial structures for both data sets, which is an unrealistic task. Nevertheless, resolution can be roughly estimated as 20–30 km and 4–5 km in horizontal and vertical directions, respectively, in the upper crust. Brief descriptions of the properties and relative merits of the two recent tomographic models are given in the Supplementary Material.
To investigate processes that control magma transfer through the crust, we employed the results of the ambient noise model, generating a tomographic map of S-wave velocity deviations from an average value 3.51 km s-1 (±0.11 km s-1, 1SD) at a mid- to upper crustal depth range of 5–16 km (Figure 1B). Further, we provide crustal cross-sections of both ambient noise (Chen et al., 2018) and body-wave (Niu et al., 2018) models down to 34 km depth, one along-arc (Figure 1C) and two across-arc (Figures 1D,E). In these cross-sections, velocity anomalies are referenced to the layer averages of the respective models. We then estimated the subvolcanic intrusive volumes beneath each volcano by integrating the tomographic volume with δVS/VS ≤ –2% over a depth range of 5–16 km and radii of 4–13 km in 1 km increments. The –2% velocity criterion was chosen, because it is the common feature of mid- to upper crustal ambient noise tomography among the volcanic groups in the Tohoku area (Chen et al., 2018), suggesting that this corresponds to recent igneous material. The radii to be chosen are less well-defined, but reflect the range of distances (8–26 km) between volcanoes in each volcano group. To avoid potential overlap issues of intrusive volumes beneath individual volcanoes, we plot intrusive volume ratios compared to Zao volcano, with uncertainties (1σ) based on the range in chosen radii.
For the record of volcanic eruptions, we have consulted the Global Volcanism Program (2013). We have taken into account all eruptions since AD 0 of the 12 arc-front volcanoes for which subsurface tomographic data was available. The Volcanic Explosivity Index (VEI, Newhall and Self, 1982) of this dataset ranged from 1 to 5. To investigate magma transfer from depth to the surface, this perspective focuses on the small (VEI 1-3) eruptions, because ≥ VEI 4 events typically tap upper crustal reservoirs, rather than representing smaller melt batches ascending from the deep crust (cf. Zellmer and Annen, 2008). Based on Monte Carlo simulations, the average eruptive volumes and uncertainties (1σ) of these small eruptions were estimated as follows: for VEI 1, 0.00055 ± 0.00026 km3; for VEI 2, 0.0055 ± 0.0026 km3; and for VEI 3, 0.055 ± 0.026 km3.
Results
Tomographic Observations
The recent conventional tomography approach (Niu et al., 2018) supports a model for deep crustal hot zones (Annen et al., 2006) above the hot fingers (Tamura et al., 2002), as seen in the lower panels of Figures 1C–E. In the mid- to upper crust, however, the resolution and accuracy of the ambient noise tomographic inversion (Chen et al., 2018) is better than that of the conventional tomography, as discussed above. Beneath the arc front, the former reveals slower than average seismic S-wave velocities below the clusters of several Holocene volcanoes and young calderas (Figure 1B, and top panels of Figures 1C,D). δVS/VS ratios down to less than –8% are observed. These values are consistent with the existence of hot plutons beneath these clusters (Yoshida et al., 2014). By contrast, the pre-Cenozoic basement rocks east of the arc yield faster than average seismic S-wave velocities (Figures 1B,D,E).
Strikingly, however, there is little tomographic evidence for hot plutons beneath Zao volcano (Figure 1B, and top panels of Figures 1C,E). Zao represents the single Holocene volcano in this area, compared to the clusters of volcanoes in most other areas (Figure 1A). In addition, only one young caldera is found within 20 km of Zao, compared to clusters of young calderas in the other volcanically active arc-front areas. Upper crustal S-wave velocity anomalies of down to c. –4% are only seen in west of Zao, towards the back-arc (Figure 1E).
Volcanological Observations
We have investigated frequency and size of the most recent (since AD 0) volcanic activity in the arc-front volcanoes for which subsurface tomographic data were available. We have restricted this analysis to the last c. 2 kyr in order to (i) have a near-complete eruptive record, including the small VEI 1–3 eruptions, and to (ii) be able to link this volcanic activity to the present-day crustal tomographic observations. Figure 2A is a compilation of the number of VEI 1 to VEI 5 eruptions recorded in the Global Volcanism Program (2013). It is evident that VEI 1 and VEI 2 eruptions dominate in this region, followed by VEI 3 eruptions. Larger eruptions have been rare during the last 2 kyr. On average, all volcanoes within clusters have erupted 4–5 times over this time period. Strikingly, the eruption frequency at Zao volcano is 7 times higher, with more than 30 small eruptions (VEI 1–3) over the last 2 kyr.
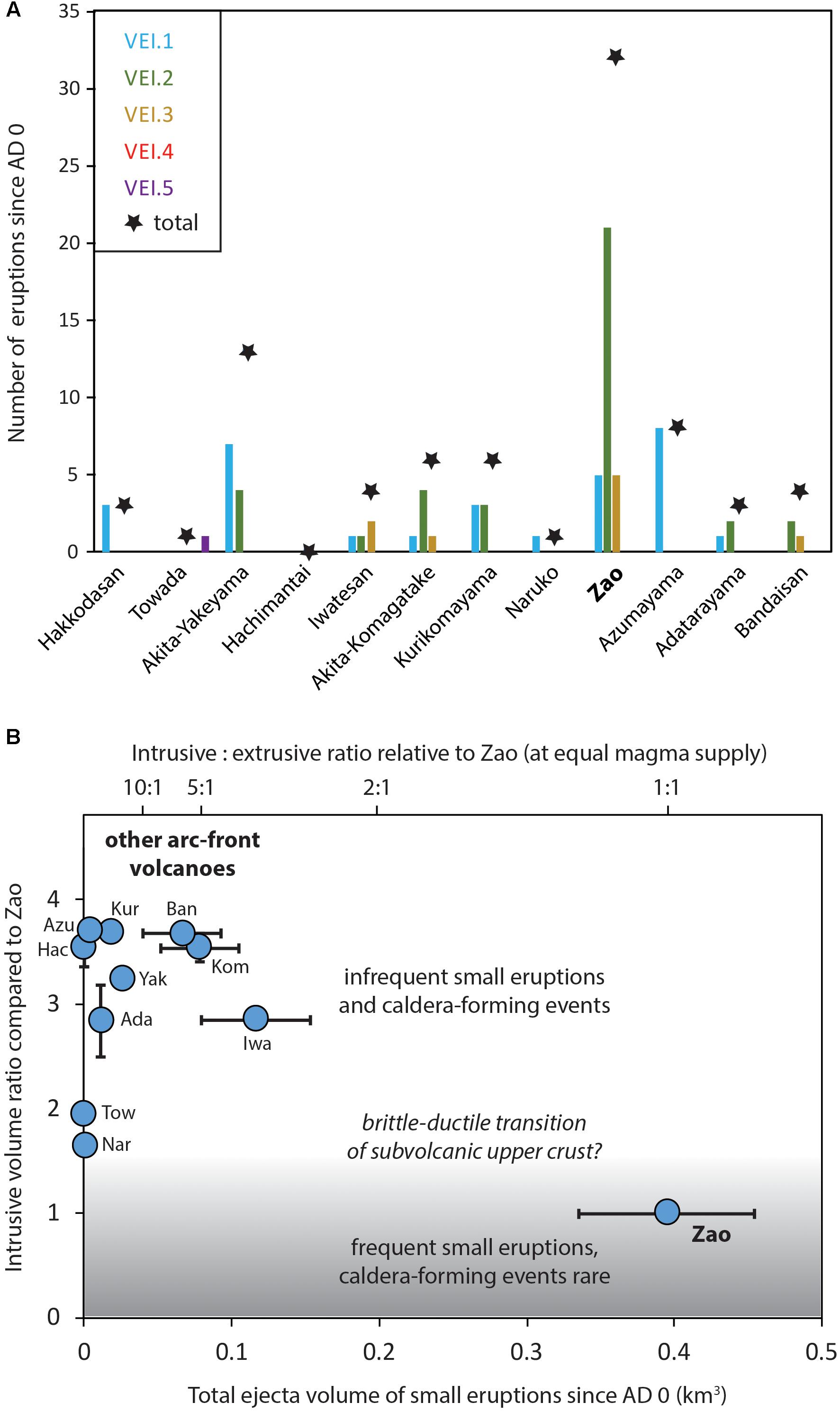
Figure 2. (A) Number of eruptions since AD 0 from each of the arc-front volcanoes listed in Figure 1, as recorded by the Global Volcanism Program (2013), split by Volcanic Explosivity Index (VEI), and summed for each center (star). Note the large number of eruptions at Zao, dominated by VEI 2 eruptions. (B) Subvolcanic intrusive volume ratios (w.r.t. Zao) versus total ejecta volumes of VEI 1 to VEI 3 eruptions since AD 0. See “Methods” section for details. Uncertainties (1σ) in both parameters are indicated when larger than symbol size. Abbreviations: Towada (Tow), Akita-Yakeyama (Yak), Hachimantai (Hac), Iwatesan (Iwa), Akita-Komagatake (Kom), Kurikomayama (Kur), Naruko (Nar), Azumayama (Azu), Adatarayama (Ada), Bandaisan (Ban). Hakkodasan is excluded, as the ambient noise velocity model has too poor constraints in the northernmost and westernmost regions to confidently estimate intrusive volume, due to sparse ray-path coverage in these areas.
Discussion
We have combined the ambient noise tomographic and the volcanological observations in Figure 2B. For each Holocene volcano, we estimated the volume ratios of subvolcanic intrusions in the mid to upper crust compared to Zao (5–16 km depth, 4–13 km radii from vent considered at 1 km intervals, δVS/VS ≤ –2%, see Supplementary Table S1 in the Supplementary Material). For each volcano, we also estimated the total volume generated from small (VEI 1–3) eruptions since AD 0 (see Supplementary Table S2 in the Supplementary Material), to compare the volumes of the most frequent eruptions with the state of the mid- to upper subvolcanic crust. Zao is clearly distinct from the other Holocene arc-front volcanoes on Figure 2B, with ≥ 3 times greater estimated eruptive volumes from VEI 1–3 eruptions, at c. 2–4 times lower intrusive volume.
If the seismically slow regions of the mid- to upper crust shown in Figure 1 indeed represent hot intrusive bodies, the combined data suggest that these may mute frequent small eruptions from the overlying volcanic edifices. Intrusive magmatism is likely pronounced in the Tohoku region due to present-day within-arc compressive stresses, which may impede the opening of magmatic conduits when compared to other arcs (Zellmer, 2008; Chaussard and Amelung, 2014). Then, the intrusions may act as an additional barrier for magma ascent to the surface. Hot plutons and potentially some small ephemeral magma-mushes have too low viscosities to allow melt propagation through tensile fractures (Keller et al., 2013; Galetto et al., 2017), leading to trapping of consecutively ascending melt batches. Heat incubation in these systems is the consequence of a positive feedback mechanism, resulting in frequent intrusions but infrequent eruptions, some of which may be large caldera-forming events that drain upper crustal evolved melts from rapidly formed but ephemeral magma-mush bodies (Michaut and Jaupart, 2006). Most volcanoes in Tohoku appear to operate under this regime.
By contrast, the apparent scarcity of recent mid- to upper crustal intrusives beneath Zao volcano may allow a system of frequent, low-volume eruptive events to be established, directly mirroring the frequent transport of low-volume magma batches through the crustal section (e.g., Zellmer and Annen, 2008), originating from the deep crustal hot zone (Annen et al., 2006) that may have been resolved by the conventional tomographic model (Niu et al., 2018, cf. lower panels of Figures 1C–E). Here, we envisage brittle, high viscosity mid- to upper crustal lithologies that allow magmatic fractures to propagate to the surface. Intrusions and associated caldera-forming events are less frequent in this case. Eruptive products of Zao volcano might be expected to be more primitive than those of the other arc-front volcanoes. Interestingly, however, neither the petrography nor the geochemistry of eruptives from Zao appear to be fundamentally different from those of other arc-front volcanoes in the Tohoku region (Tatsumi et al., 2008; Takahashi et al., 2013). This may indicate that mafic to intermediate arc magmatic evolution dominantly occurs in the deep crust (Annen et al., 2006), prior to magma ascent.
What lithologies does the brittle crust beneath Zao volcano consist of? We see that left-lateral Miocene faults with significant surface offsets of up to c. 25 km exist in southern Tohoku (Figure 1A), related to Miocene back-arc opening with large counter-clockwise movements prior to more recent compressional kinematics (Acocella et al., 2008; Yamada and Yoshida, 2011). The total offset of the most recent Neogene rift grabens indicates a net northwestward movement of crustal rocks above the melt generation zone in the mantle beneath Zao. We postulate that cold and brittle mid-to upper crustal Cretaceous granitoids exposed in the Abukuma mountains east of Zao have been displaced to beneath Zao volcano since the Miocene, allowing fracturing of magmas from the deep crust to the surface. Ambient-noise tomographic data (top panels of Figures 1D,E) indicate that, at mid-crustal levels, the seismically fast fore-arc region extends further toward the arc front than suggested by the surface outcrops of the Kitakami and Abukuma mountain belts (Figure 1A). Further, slightly reduced upper crustal S-wave anomalies in the backarc of Zao (Figure 1E) may represent previous arc-front intrusions, which were also displaced westward since the Miocene.
We note that this idea is in contrast to the notion that old within-arc batholiths may act as barriers that deviate vertical melt ascent, which has recently been suggested as a reason for the lateral offset of Mount St. Helens from the main Cascades arc (Bedrosian et al., 2018). We suggest that the tectonic strike-slip movement of the Cretacious granitoids in the eastern Tohoku region resulted in vertical fracture formation, and these fractures are currently exploited by ascending magmas beneath Zao. It is therefore the combination of cold, brittle lithologies and preexisting fractures that would facilitate magma ascent.
Our knowledge of the volcanic and tectonic history of NE Japan also allows us to discuss the potential reasons for an onset of more pronounced intrusive activity in subduction zones in general. As seen in Figure 1A, most calderas, which are indicative of intrusions (Aizawa et al., 2006), are situated within the Neogene rift basin filled with volcaniclastic and sedimentary successions (Yoshida et al., 2014). Magma ascent through such strata may be hampered by the existence of subhorizontal rheological and density boundaries that promote lateral magma intrusions and the formation of sills (Taisne and Jaupart, 2009; Menand, 2011; Galetto et al., 2017). In contrast, old and structurally isotropic plutonic basement rocks, as postulated to exist in the upper crust beneath Zao, may not constitute suitable wall-rocks for sill intrusions, which typically require layered, anisotropic hosts lithologies (Rocchi and Breitkreuz, 2018).
This work highlights that the concept of a constant intrusive:extrusive proportion of arc magmatism is fundamentally flawed, at least on a regional level. As we only have a present-day geophysical picture of upper crustal structure, we are unable to quantify intrusion histories. However, our data show that intrusive:extrusive ratios can vary significantly between centers of activity within individual volcanic arcs, and suggest that they may vary significantly through time. If the total magma flux per unit area into the subvolcanic crust is taken as equal for each volcano cluster, intrusive:extrusive ratios relative to Zao will scale with ejecta volumes. Then, Figure 2B suggests that intrusive:extrusive ratios at Zao are currently at least 3 times lower than at Iwatesan, and 5 to >20 times lower than at the other Holocene volcanoes in the Tohoku region.
In summary, arc magmatism in the Tohoku area has allowed us to elucidate the long-standing question on the processes that control volcanism versus plutonism in subduction zone environments. Qualitatively, the brittle versus ductile behavior of the subvolcanic crust and variations in the rheology and structural anisotropy of crustal lithologies appear to play a major role in controlling magma transport to the surface. This indicates that typically, millions of years of crustal history and tectonism will exert fundamental controls on the style of subduction zone magmatism, and need to be deciphered before more quantitative progress can be made in assessing the relative proportions of intrusive and extrusive magmatic activity in any volcanically active area.
Author Contributions
GZ conceived the idea, combined all data, and wrote the manuscript. K-XC provided tomographic maps and cross sections, calculated subvolcanic intrusive volumes, and compiled the new data from the Global Volcanism Program. YG and B-YK assisted with the interpretation of the ambient noise tomography results. TY contributed to the discussion of crustal geology and magmatic processes in the Tohoku region.
Funding
This work was supported by Marsden Standard Grant MAU1704 to GZ, Ministry of Science and Technology, Taiwan (MOST) Grants 107-2116-M-001-MY3 and 106-2116-M-002-001 to B-YK and YG, respectively, and Japan Society for the Promotion of Science (JSPS) KAKENHI Grant 26109003 to TY.
Conflict of Interest Statement
The authors declare that the research was conducted in the absence of any commercial or financial relationships that could be construed as a potential conflict of interest.
Acknowledgments
We thank Masao Ban for discussions, and MP for his invitation to contribute to this research topic as well as editorial handling of this perspective.
Supplementary Material
The Supplementary Material for this article can be found online at: https://www.frontiersin.org/articles/10.3389/feart.2019.00040/full#supplementary-material
References
Acocella, V., Yoshida, T., Yamada, R., and Funiciello, F. (2008). Structural control on late miocene to quaternary volcanism in the NE Honshu arc, Japan. Tectonics 27:TC5008. doi: 10.1029/2008TC002296
Aizawa, K., Acocella, V., and Yoshida, T. (2006). “How the development of magma chambers affects collapse calderas: insights from an overview,” in Mechanisms of Activity and Unrest at Large Calderas, Vol. 269, eds C. Troise, G. De Natale, and C. R. J. Kilburn (London: Geological Society, Special Publications), 65–81. doi: 10.1144/GSL.SP.2006.269.01.05
Annen, C., Blundy, J. D., and Sparks, R. S. J. (2006). The genesis of intermediate and silicic magmas in deep crustal hot zones. J. Petrol. 47, 505–539. doi: 10.1093/petrology/egi084
Bedrosian, P. A., Peacock, J. R., Bowles-Martinez, E., Schultz, A., and Hill, G. J. (2018). Crustal inheritance and a top-down control on arc magmatism at Mount St Helens. Nat. Geosci. 11, 865–870. doi: 10.1038/s41561-018-0217-2
Chaussard, E., and Amelung, F. (2014). Regional controls on magma ascent and storage in volcanic arcs. Geochem. Geophys. Geosyst. 15, 1407–1418. doi: 10.1002/2013GC005216
Chen, K.-X., Gung, Y., Kuo, B.-Y., and Huang, T.-Y. (2018). Crustal magmatism and deformation fabrics in northeast Japan revealed by ambient noise tomography. J. Geophys. Res. Solid Earth 123, 8891–8906. doi: 10.1029/2017JB015209
Crisp, J. A. (1984). Rates of magma emplacement and volcanic output. J. Volcanol. Geother. Res. 20, 177–211. doi: 10.1016/0377-0273(84)90039-8
Galetto, F., Acocella, V., and Caricchi, L. (2017). Caldera resurgence driven by magma viscosity contrasts. Nat. Commun. 8:1750. doi: 10.1038/s41467-017-01632-y
Global Volcanism Program (2013). Volcanoes of the World, v. 4.7.4, ed. Venzke, E. (Washington, D.C: Smithsonian Institution). Available at: https://doi.org/10.5479/si.GVP.VOTW4-2013 (accessed September 30, 2018).
Hasegawa, A., Yamamoto, A., Umino, N., Miura, S., Horiuchi, S., Zhao, D., et al. (2000). Seismic activity and deformation process of the overriding plate in the northeastern Japan subduction zone. Tectonophysics 319, 215–239. doi: 10.1016/S0040-1951(99)00296-6
Hirahara, Y., Senda, R., Takahashi, T., Tsuchiya, N., Kagashima, S., Yoshida, T., et al. (2015). Spatial variation of Sr-Nd-Hf isotopic compositions from Cretaceous to Paleogene granitoids in Northeastern Japan Arc. Ganseki Kobutsu Kagaku 44, 91–111. doi: 10.2465/gkk.130830
Honda, H., and Yoshida, T. (2005). Effects of oblique subduction on the 3-D pattern of small-scale convection within the matle wedge. Geophys. Res. Lett. 32:L13307. doi: 10.1029/2005GL023106
Huang, T.-Y., Gung, Y., Liang, W.-T., Chiao, L.-Y., and Teng, L. S. (2012). Broad-band rayleigh wave tomography of Taiwan and its implications on gravity anomalies. Geophys. Res. Lett. 39:L05305. doi: 10.1029/2011GL050727
Keller, T., May, D. A., and Kaus, B. J. P. (2013). Numerical modelling of magma dynamics coupled to tectonic deformation of lithosphere and crust. Geophys. J. Int. 195, 1406–1442. doi: 10.1093/gji/ggt306
Menand, T. (2011). Physical controls and depth of emplacement of igneous bodies: a review. Tectonophysics 500, 11–19. doi: 10.1016/j.tecto.2009.10.016
Michaut, C., and Jaupart, C. (2006). Ultra-rapid formation of large volumes of evolved magma. Earth Planet. Sci. Lett. 250, 38–52. doi: 10.1016/j.epsl.2006.07.019
Nakajima, J., Hasegawa, A., Horiuchi, S., Yoshimoto, K., Yoshida, T., and Umino, N. (2006). Crustal heterogeneity around the Nagamachi–Rifu fault, northeastern Japan, as inferred from travel-time tomography. Earth Planets Space 58, 843–853. doi: 10.1186/BF03351989
Nakajima, J., Matsuzawa, T., Hasegawa, A., and Zhao, D. (2001). Three-dimensional structure of Vp, Vs and Vp/Vs beneath northeastern Japan: implications for arc magmatism and fluids. J. Geophys. Res. 106, 21843–21857. doi: 10.1029/2000JB000008
Newhall, C. G., and Self, S. (1982). The volcanic explosivity index (VEI): an estimate of explosive magnitude for historical volcanism. J. Geophys. Res. Atmos. 82, 1231–1238. doi: 10.1029/JC087iC02p01231
Nishimoto, S., Ishikawa, M., Arima, M., and Yoshida, T. (2005). Laboratory measurement of P-wave velocity in crustal and upper mantle xenoliths from Ichino-megata, NE Japan: ultrabasic hydrous lower crust beneath the NE Honshu arc. Tectonophysics 396, 245–259. doi: 10.1016/j.tecto.2004.12.010
Nishimoto, S., Ishikawa, M., Arima, M., Yoshida, T., and Nakajima, J. (2008). Simultaneous high P–T measurements of ultrasonic compressional and shear wave velocities in Ichino-megata mafic xenoliths: their bearings on seismic velocity perturbations in lower crust of northeast Japan arc. J. Geophys. Res. 113:B12212. doi: 10.1029/2008JB005587
Niu, X., Zhao, D., and Li, J. (2018). Precise relocation of low-frequency earthquakes in Northeast Japan: new insight into arc magma and fluids. Geophys. J. Int. 212, 1183–1200. doi: 10.1093/gji/ggx445
Okamura, Y., Watanabe, M., Morijiri, R., and Satoh, M. (1995). Rifting and basin inversion in the eastern margin of the Japan Sea. Island Arc 4, 166–181. doi: 10.1111/j.1440-1738.1995.tb00141.x
Rocchi, S., and Breitkreuz, C. (2018). “Physical geology of shallow-level magmatic systems - an introduction,” in Physical Geology of Shallow Magmatic Systems. Advances in Volcanology (An Official Book Series of the International Association of Volcanology and Chemistry of the Earth’s Interior), eds S. Rocchi and C. Breitkreuz (New York, NY: Springer), 1–10. doi: 10.1007/11157_2017_32
Sato, H. (1994). The relationship between Late Cenozoic tectonic events and stress field and basin development in northeast Japan. J. Geophys. Res. 99, 22261–22274. doi: 10.1029/94JB00854
Sato, H., Imaizumi, T., and Yoshida, T. (2002). Tectonic evolution and deep to shallow geometry of Nagamachi-Rifu active fault system, NE Japan. Earth Planets Space 54, 1039–1043. doi: 10.1186/BF03353298
Shibazaki, B., Garatani, K., Iwasaki, T., Tanaka, A., and Iio, Y. (2008). Faulting processes controlled by the nonuniform thermal structure of the crust and uppermost mantle beneath the northeastern Japanese island arc. J. Geophys. Res. 113:B08415. doi: 10.1029/2007JB005361
Taisne, B., and Jaupart, C. (2009). Dike propagation through layered rocks. J. Geophys. Res. 114:B09203. doi: 10.1029/2008JB006228
Takada, Y., and Fukushima, Y. (2013). Volcanic subsidence triggered by the 2011 Tohoku earthquake in Japan. Nat. Geosci. 6, 637–641. doi: 10.1038/ngeo1857
Takahashi, T., Hirahara, Y., Miyazaki, T., Senda, R., Chang, Q., Kimura, J.-I., et al. (2013). Primary magmas at the volcanic front of the NE Japan arc: coeval eruption of crustal low-K tholeiitic and mantle-derived medium-K calc-alkaline basalts at Azuma volcano. J. Petrol. 54, 103–148. doi: 10.1093/petrology/egs065
Tamura, Y., Tatsumi, Y., Zhao, D., Kido, Y., and Shukuno, H. (2002). Hot fingers in the mantle wedge: new insights into magma genesis in subduction zones. Earth Planet. Sci. Lett. 197, 105–116. doi: 10.1016/S0012-821X(02)00465-X
Tatsumi, Y., Takahashi, T., Hirahara, Y., Chang, Q., Miyazaki, T., Kimura, J.-I., et al. (2008). New insights into andesite genesis: the role of mantle-derived calc-alcalic and crust-derived tholeiitic melts in magma differentiation beneath Zao volcano, NE Japan. J. Petrol. 49, 1971–2008. doi: 10.1093/petrology/egn055
Tsuchiya, N., and Kanisawa, S. (1994). Early Cretaceous Sr-rich silicic magmatism by slab melting in the Kitakami Mountains, northeast Japan. J. Geophys. Res. 99, 22205–22220. doi: 10.1029/94JB00458
Turner, S. J., and Langmuir, C. H. (2015a). The global chemical systematics of arc front stratovolcanoes: evaluating the role of crustal processes. Earth Planet. Sci. Lett. 422, 182–193. doi: 10.1016/j.epsl.2015.03.056
Turner, S. J., and Langmuir, C. H. (2015b). What processes control the chemical compositions of arc front volcanoes? Geochem. Geophys. Geosyst. 16, 1865–1893. doi: 10.1002/2014GC005633
Turner, S. J., Langmuir, C. H., Katz, R. F., Dungan, M. A., and Escrig, S. (2016). Parental arc magma compositions dominantly controlled by mantle-wedge thermal structure. Nat. Geosci. 9, 772–776. doi: 10.1038/ngeo2788
Wallace, P. J. (2001). Volcanic SO2 emissions and the abundance and distribution of exsolved gas in magmas. J. Volcanol. Geother. Res. 108, 85–106. doi: 10.1016/S0377-0273(00)00279-1
White, S. M., Crisp, J. A., and Spera, F. J. (2006). Long-term volumetric eruption rates and magma budgets. Geochem. Geophys. Geosyst. 7:Q03010. doi: 10.1029/2005GC001002
Yamada, R., and Yoshida, T. (2011). Relationships between Kuroko volcanogenic massive sulfide (VMS) deposits, felsic volcanism, and island arc development in the northeast Honshu arc. Mineralium Deposita 46, 431–448. doi: 10.1007/s00126-011-0362-7
Yoshida, T., Aizawa, K., Nagahashi, Y., Sato, H., Ohguchi, T., Kimura, J., et al. (1999). Geological history of late cenozoic island arc volcanism in Northeast Honshu arc and the formation of caldera swarms. Earth Monthly 27, 123–129.
Yoshida, T., Kimura, J.-I., Yamada, Y., Acocella, V., Sato, H., Zhao, D., et al. (2014). “Evolution of late Cenozoic magmatism and the crust-mantle structure in the NE Japan Arc,” in Orogenic Andesites and Crustal Growth, Vol. 385, eds A. Gómez-Tuena, S. M. Straub, and G. F. Zellmer (London: Geological Society, Special Publications), 335–387. doi: 10.1144/SP385.15
Zellmer, G. F. (2008). “Some first order observations on magma transfer from mantle wedge to upper crust at volcanic arcs,” in Dynamics of Crustal Magma Transfer, Storage and Differentiation, Vol. 304, eds C. Annen and G. F. Zellmer (London: Geological Society, Special Publications), 15–31. doi: 10.1144/SP304.2
Zellmer, G. F., and Annen, C. (2008). “An introduction to magma dynamics,” in Dynamics of Crustal Magma Transfer, Storage and Differentiation, Vol. 304, eds C. Annen and G. F. Zellmer (London: Geological Society, Special Publications), 1–13. doi: 10.1144/SP304.1
Zellmer, G. F., Edmonds, M., and Straub, S. M. (2015). “Volatiles in subduction zone magmatism,” in The Role of Volatiles in the Genesis, Evolution, and Eruption of arc Magmas, Vol. 410, eds G. F. Zellmer, M. Edmonds, and S. M. Straub (London: Geological Society, Special Publications), 1–17. doi: 10.1144/SP410.13
Keywords: heat incubation, crustal magma ascent, crustal growth history, volcanic eruption style, Tohoku volcanism, brittle-ductile transition
Citation: Zellmer GF, Chen K-X, Gung Y, Kuo B-Y and Yoshida T (2019) Magma Transfer Processes in the NE Japan Arc: Insights From Crustal Ambient Noise Tomography Combined With Volcanic Eruption Records. Front. Earth Sci. 7:40. doi: 10.3389/feart.2019.00040
Received: 01 October 2018; Accepted: 20 February 2019;
Published: 15 March 2019.
Edited by:
Mattia Pistone, Université de Lausanne, SwitzerlandReviewed by:
Pablo Samaniego, UMR6524 Laboratoire Magmas et Volcans (LMV), FranceSigrún Hreinsdóttir, GNS Science, New Zealand
Copyright © 2019 Zellmer, Chen, Gung, Kuo and Yoshida. This is an open-access article distributed under the terms of the Creative Commons Attribution License (CC BY). The use, distribution or reproduction in other forums is permitted, provided the original author(s) and the copyright owner(s) are credited and that the original publication in this journal is cited, in accordance with accepted academic practice. No use, distribution or reproduction is permitted which does not comply with these terms.
*Correspondence: Georg F. Zellmer, g.f.zellmer@massey.ac.nz