- 1Department of Earth Sciences, Indiana University – Purdue University Indianapolis, Indianapolis, IN, United States
- 2Department of Geology and Geophysics, University of Wyoming, Laramie, WY, United States
Seasonal change in surface melt input and spatial controls on the distribution of subglacial water can cause considerable variability in the aqueous chemistry of subglacial waters. Much of this variability has been interpreted in terms of a single variable: water residence time, with slow flow assumed to correlate with greater mineral dissolution and oxidative weathering. We synthesize data from a range of glacier and ice sheet settings to show that this approach does not adequately describe presently available data. Instead, we propose that two independent variables control spatial and seasonal changes in aqueous chemistry in subglacial settings: atmospheric gas abundance and sediment supply abundance. Where atmospheric gases are abundant, carbonation weathering is responsible for most of the subglacial chemical activity; where they become limited, oxidation weathering becomes more dominant. Where freshly comminuted sediment is abundant, easily dissolved minerals, especially calcite, have proportionally more influence on subglacial hydrochemistry; where sediment supply is limited, silicate minerals, and less reactive carbonate minerals will increase in relative influence. In most settings, simple metrics of the abundance of SO42− and Ca2+ in the subglacial waters can characterize these two variables. In the data we synthesize, neither variable consistently correlates to the inferred water residence time, nor do the variables consistently correlate with each other. Spatial data show that point locations and small catchments on the glacial bed differ substantially from the integrated composition found at glacial outlets. The varied response in the subglacial aqueous chemistry to changing water residence times suggests complex control by a broad range of glaciological factors that affect water routing and subglacial sediment generation.
Introduction
In order the understand the feedbacks between glaciation and chemical weathering, the chemistry of subglacial waters has been characterized in a wide range of glacier and ice sheet settings (e.g., Anderson et al., 1997; Hodson et al., 2000; Wadham et al., 2010; Graly et al., 2017a), both through borehole sampling of subglacial water and by sampling at outlet settings that integrate subglacial flow. In settings where surface melt comprises a large portion of the total runoff, significant seasonal changes in the subglacial chemistry are typically observed with the changing glacial hydrology (Wadham et al., 2000; Yde et al., 2014; Stachnik et al., 2016a). At sites where multiple point locations are characterized, significant differences exist between regions of the glacier bed (Fairchild et al., 1994; Tranter et al., 2002; Graly et al., 2014; Stachnik et al., 2016b). We seek to develop a new conceptual model to understand the causes of these differences. Previous work made a distinction between long residence time waters with high total dissolved solids and short residence time waters with low total dissolved solids (Tranter et al., 1993). Here, we leverage the intervening decades of glacial hydrochemical work to explore the variation in the effect of water residence time on water chemistry in the subglacial environment.
Subglacial environments are comparable to a chemical flow-through reactor in that the output at the terminus reflects the processes that occur over the course of water throughflow. Efficient subglacial weathering requires a supply of atmospheric gases (CO2 and O2), the reactive mineral surfaces of recently comminuted sediment, and sufficient fluid transport to limit reaction saturation (Anderson, 2005). Both gas inflow from surface water and mineral surface production from basal sliding can become less abundant in winter months and in other flow conditions that create long subglacial water residence times (Wadham et al., 2000). We propose that much of the variability in subglacial hydrochemistry can be explained through two independent variables controlling geochemical processes under ice: In circumstances where saturation limitations have not yet occurred, the chemical reactions in the subglacial system can either become limited by the supply of atmospheric gas or limited by the supply of freshly comminuted sediment. Though both limitations can exist, significant changes to one would likely cause diminished effects in the other. That is, weathered sediment is slow to deplete the supply of atmospheric gases, and waters depleted in atmospheric gases are slow to weather subglacial sediments.
This differs from previous conceptual models in that it considers two competing mechanisms by which variable water residence times could affect glacier rock interactions, whereas previous models consider only gas diffusion to be the limiting residence time dependent process. The Tranter et al. (1993) model predicts that complete diffusion of O2 into the weathering system will cause high residence time (delayed flow) waters to have greater sulfate concentrations and higher relative rates of carbonate weathering and that low residence time (quick flow) waters to have a higher proportion of carbonation weathering (though lower pCO2) and a higher proportion of silicate-derived weathering products. Subsequent work showed that sulfide oxidation can occur through Fe reduction even in partially anoxic subglacial settings, but the slow kinetics of these processes associate them with high residence time waters (Bottrell and Tranter, 2002; Tranter et al., 2002). More recent work has recognized that larger glacial systems (such as those in Greenland and Antarctica) will have larger silicate weathering fluxes in long residence time systems (Wadham et al., 2010), but still considers the routing of gas to subglacial sediments to be the primary weathering control.
Atmospheric gases route to the bed through both surface and basal processes. Surface water draws down atmospheric gases through open exchange with air and the turbulent mixing of water and air in moulins (Graly et al., 2017a). Basal melt introduces the air content of the melting glacial ice, which is usually ∼10% air by volume at atmospheric pressure, which although compressed at depth, represents a significant gas source (Berner et al., 1977). In typical ablation zone settings, the rate of net basal melt is 2–3 orders of magnitude lower than surface melt (e.g., MacGregor et al., 2016). So, the primary source of atmospheric gas to the bed is surface melt. Where abundant surface melt provides a high throughflow of air-saturated water to the bed, carbonation weathering dominates the subglacial system (e.g., Anderson et al., 2003). As atmospheric CO2 becomes depleted, oxidation of sulfides has an increasingly large role in the hydrochemistry of subglacial waters. In hypoxic or anoxic conditions, oxidation can only continue through mineral based oxygen sources (such as Fe redox) and eventually conditions may become reducing (Tranter et al., 2002).
Our second variable derives from the decline in production of unweathered sediment with freshly comminuted surfaces where basal water flux is limited. Sediment generation processes such as abrasion and quarrying require a rock substrate that can be only maintained where freshly generated sediment is removed by entrainment in ice or water (Iverson and Semmens, 1995; Hallet, 1996; Alley et al., 1997). Subglacial water must move at flow speeds sufficient to mobilize sand sized particles in order to flush subglacial sediments from the bed (Alley et al., 1997). Sliding rates also decline with low water availability (Joughin et al., 2008), resulting in diminished sediment comminution from abrasion. Comminution during subglacial transport is also reduced or eliminated. In principle, the seasonal or spatial effects of water availability on sediment generation and flushing can work both ways: If the generated sediment is removed in a highly efficient fashion, then there may be too little sediment remaining in the system for much chemical weathering to occur. Alternatively, too little removal of sediment from the system would leave sediments that have already been chemically depleted of reactive accessory minerals and exchangeable cations. When freshly comminuted, unweathered sediment is abundant, carbonate weathering dominates the system even where the bedrock is silicate rich (Raiswell, 1984). High sediment supply regimes can also allow for substantial exchange of cations on fresh silicate mineral surfaces, allowing for large fluxes of Na+ and K+ into the system, with cation exchange continuing well after carbonates are depleted. (Drever and Hurcomb, 1986; Graly et al., 2014). In the most sediment supply limited systems, bulk chemistry will be reflective of the alteration of the underlying bedrock to clay minerals and have a much higher relative abundance of Si (Graly et al., 2014).
Methods and Glaciological Settings
In order to test whether gas limitation and sediment supply limitation act as competing responses to changing subglacial water residence time, we employed two simple metrics to characterize the relative prevalence of each limitation at glacial sites where significant variability in hydrochemistry was documented, either seasonally or between boreholes and outlets. These metrics are meant as first order approximations to compare glacial waters from geologically and glaciologically diverse settings. Therefore, we do not apply site-specific measures such as the correction for sea salt input based on the chemistry of local snow (e.g., Hodson et al., 2000) or balance calculations for calcite dissolution based on the chemistry of local feldspars and amphiboles (e.g., Graly et al., 2016).
Relative gas limitation was assessed by the ratio in equivalents of bicarbonate to bicarbonate and sulfate in the waters. The bicarbonate/(sulfate + bicarbonate) ratio or the slope between sulfate and bicarbonate concentrations has been widely applied to assess the prevalence of the sulfuric acid induced carbonate weathering in previous work on subglacial hydrochemistry (e.g., Sharp et al., 1995; Brown et al., 1996; Wadham et al., 2010; Stachnik et al., 2016a). We interpret a shift toward bicarbonate as suggestive of gas abundance and a shift toward sulfate as suggestive of gas limitation. The use of the bicarbonate/(sulfate + bicarbonate) ratio as a gas limitation metric depends on sulfur oxidation being the primary oxidation pathway in the subglacial system. In settings where carbon-rich sedimentary rocks cause substantial quantities of organic carbon to be oxidized (Graly et al., 2017a), oxidation will produce carbonic rather than sulfuric acid and the gas limitation may not be detectable. Large shifts between carbonate and silicate weathering will also affect bicarbonate production in the system without necessarily changing the relative gas content. Both stable isotopes of carbon (Wadham et al., 2004) and deconvolution of the weathering chemistry (Graly et al., 2017a) can potentially identify the organic and mineral sources of carbon. The systems analyzed here are not expected to have large contributions from organic carbon oxidation (Graly et al., 2017a).
Relative sediment supply limitation was assessed from changes in the abundance in equivalents of Ca2+ among major cations, less Cl- concentration in equivalents. This metric traces relative changes in the contribution of calcite dissolution to the chemistry of the subglacial waters. Since calcite is likely to be the most reactive mineral in the subglacial system (Raiswell, 1984; Tranter et al., 2002; Graly et al., 2016), a relative lack of dissolved calcite suggests that the most reactive mineral surfaces are not present in the system. Ca2+ also sources from feldspar and amphibole weathering, but the abundance of these minerals is unlikely to change with sediment freshness or volume (whereas calcite is often an accessory mineral than can be depleted). Cl- concentration was subtracted so that the effects of seasonally or spatially variable salt fluxes did not affect the relative cation balance. This sediment supply metric is similar to past assessments of the carbonate/silicate weathering prevalence through the ratio of Ca and Mg among cations (e.g., Hodson et al., 2000; Wadham et al., 2010). However, our metric differs in that it excludes Mg, thus allowing for the assessment of the changing relative abundance of calcite and dolomite weathering in carbonate-dominated settings. Though most silicate settings will have primary calcite in the underlying bedrock (White et al., 2005), once calcite is depleted, the sediment supply metric may no longer correctly trace changes in the reactivity of the weathering minerals. Where only silicate minerals are being weathered, Na and K are more readily subject to cation exchange on freshly weathered surfaces (Blum and Stillings, 1995), and a shift toward Ca could indicate increasing sediment limitation. Stable isotopes of Ca can identify these circumstances (Moore et al., 2013), but are not available for the waters analyzed. In settings where insufficient fresh meltwater enters the system, calcite weathering may be limited by saturation and not by sediment supply. The waters analyzed in this study are all below calcite saturation (e.g., Stachnik et al., 2016b), but some may have been at saturation prior to later dilution by meltwater input (Graly et al., 2017a). Where Cl sources from groundwater or the weathering of silicates, the subtraction of Cl from the metric may overstate the salt correction. For both metrics, analysis of spatial variability may reflect the abundance of calcite and sulfides in the underlying bedrock.
We employed these metrics to reanalyze existing data from warm-based or polythermal glaciers in Svalbard, the Alps, and the Greenland Ice Sheet in terms of gas and sediment supply limitation. At most locations, we choose settings where data has been collected over major temporal shifts in the hydrological regime. Where available, this included overwinter flow, either captured directly from proglacial flow or by coring naled ice in the glacial foreland. The naled cores have to be interpreted with caution as the water sources are not always subglacial and chemical fractionation during ice formation is possible (Yde et al., 2012). Early (spring) flow where the first surface meltwater contacts the bed often represents a distinct chemical signature, as long stored waters are flushed from the system (Wadham et al., 2000). Peak (summer) flow is characterized by high inputs of surface meltwater as is often diluted compared to other flow regimes (e.g., Hawkings et al., 2015). Late (autumn) flow represents a return to low flow, but where waning surface meltwater input still exists, and the hydrological regime still can maintain the well-connected flow paths formed during peak melt (e.g., Yde et al., 2014). In the settings we study, there generally aren’t direct measurements of subglacial water residence time (e.g., Chandler et al., 2013), so we infer relatively long residence times in winter and early flow and shorter residence times in peak and late flow.
In Svalbard, we have included data from three sites where net glacial outflow was assessed and compared to naledi that formed from continued subglacial outflow in winter. Werenskioldbreen (Stachnik et al., 2016a,b) and Finterswalderbreen (Wadham et al., 1998, 2000) were sites of year-long hydrochemical monitoring, providing data on early, peak, and late flow from the melt season (as well as winter output). At Werenskioldbreen, several separate glacial outlets and naledi were studied throughout the season, allowing for assessment of spatial variability at that site. At Austre Grønfjordbreen, naled ice was only compared to July subglacial outflow (Yde et al., 2012). The Austre Grønfjordbreen site has strong evidence of chemical fractionation in the lower portions of the naled, with only the topmost layer of ice having a composition resembling subglacial water (Yde et al., 2012). All of the Svalbard glaciers are located in sedimentary and meta-sedimentary terranes rich in carbonates.
Sites in the Alps include Rhone, Oberaar, and Damma Glaciers, which all have outlet stream chemical data from either the entire year or the entire melt season (Hosein et al., 2004; Hindshaw et al., 2011). At Haut D’Arolla, subglacial boreholes were contrasted with average summer outflow at the terminus (Tranter et al., 2002), allowing us to assess spatial variability in the subglacial environment. At Tsanfleuron, several outlets with contrasting interaction with the underlying karst system also demonstrate spatial variability (Fairchild et al., 1994; Zeng et al., 2012). Damma and Rhone Glaciers are located on primarily silicate bedrock, whereas the bedrock under Oberaar, Haut and Tsanfleuron Glaciers is sufficiently carbonate rich that silicate minerals provide only a small contribution to the water chemistry.
Two sites in Greenland have had long-term monitoring of their hydrochemical flux, allowing for assessment of early, peak, and late flow: Leverett Glacier (Hawkings et al., 2015) and Watson River (Yde et al., 2014). The Watson river drains several glacial outlets in central West Greenland; Leverett Glacier is one such outlet, but provides only a small portion of the total flow to Watson River. Subglacial chemistry has been assessed at Isunnguata Sermia (Graly et al., 2014, 2017b), 20 km north of Leverett Glacier. Here, summer outflow and borehole chemistry are available, allowing for assessment of spatial variability. This region of the Greenland Ice Sheet is underlain by a 2.7 Ga accreted terrane that consists predominately of gneisses and amphibolites (van Gool et al., 2002). The structural features of the terrane strike east-west and genetically similar rocks appear at similar latitudes in East Greenland (Kalsbeek et al., 1993), allowing relative confidence that lithology does appreciably change up glacier.
Results
Each of the three locations where winter outflow was assessed at Svalbard indicate some complexity in use of naled ice to assess winter outflow. At Austre Grønfjordbreen, the lower layer of the naled ice contains almost exclusively calcite, whereas the top layer has a chemically complex composition. As newly freezing water is added to the top of the naled (Schohl and Ettema, 1986), the top layer preserves the chemical constituents of the subglacial outflow (Yde et al., 2012). At Werenskioldbreen, the naledi formed from the top down (i.e., newly freezing water was added to the bottom) and the waters below the naled have higher total dissolved solids and a have lower percentage calcite than the ice itself (Stachnik et al., 2016b). At Finsterwalderbreen, only a portion of the naledi were inferred to be of subglacial origin (Wadham et al., 2000). The composition of the naledi from all three sites are significantly enriched in the relative proportion of sulfate compared to early and late ablation-driven flow, suggesting gas limitation overwinter (Figure 1). Werenskioldbreen also has a significant shift toward sediment supply limitation toward winter (as represented by a shift from calcite dissolution to dolomite dissolution), which is not observed at the other Svalbard sites. At Finsterwalderbreen, increasing sediment supply (i.e., calcite dissolution) is indicated in the late flow composition. Spatial variability at Werenskioldbreen suggests that the smaller sub-outlets are more sediment supply limited (with less calcite dissolution) and less gas limited (with less sulfur oxidation) than the bulk outflow. At Austre Grønfjordbreen, the groundwater-influenced proglacial lake contains dissolved calcite products almost exclusively, and therefore lacks the limitation in gas and sediment supply that occur in direct subglacial outflow.
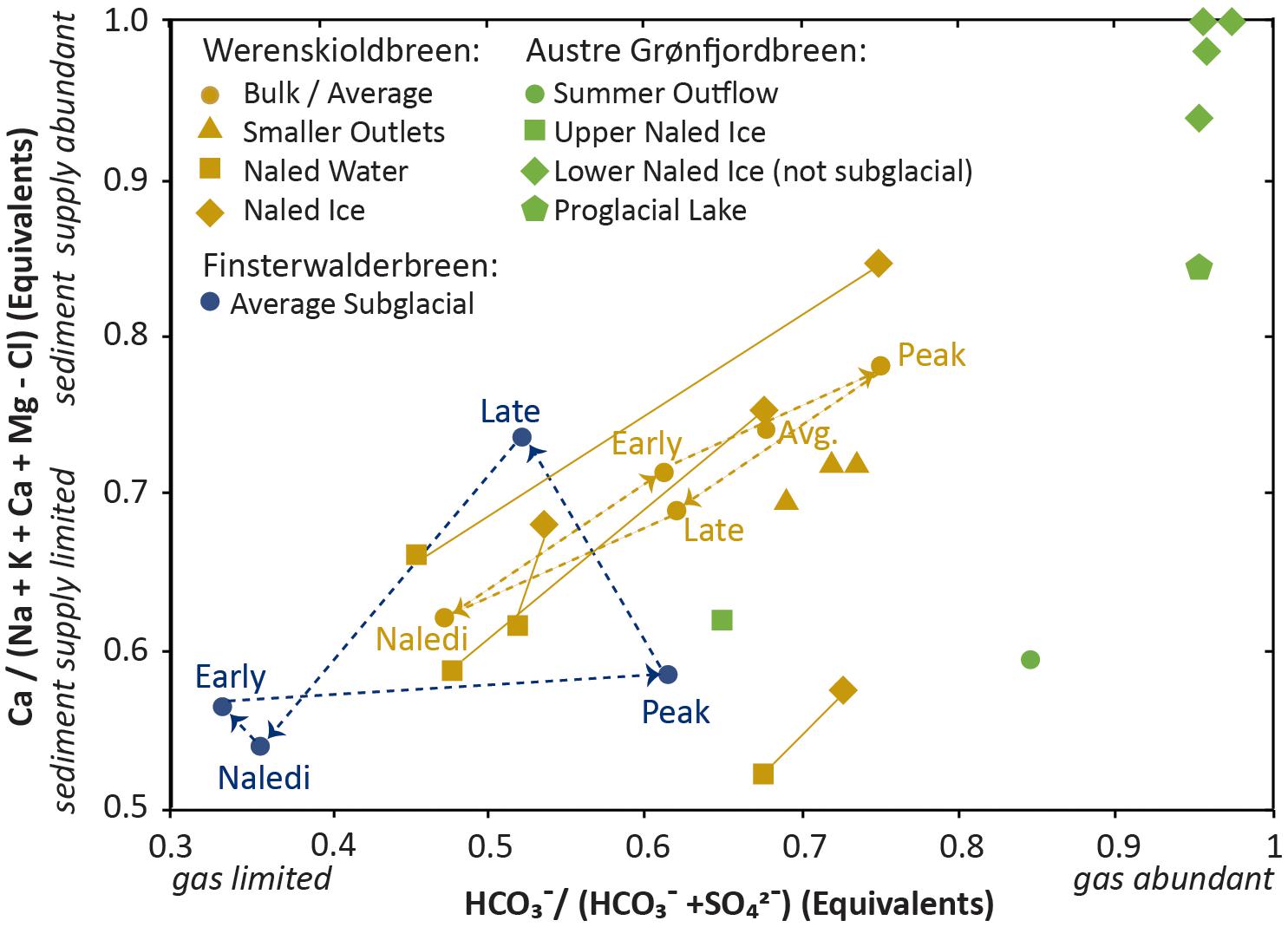
FIGURE 1. Data from Svalbard. The x-axis is reflective of the contribution of carbonation vs. sulfur oxidation to the water chemistry. Oxidation becomes more prevalent in gas-limited systems. The y-axis is reflective of the relative contribution of calcite. As a highly reactive mineral constituent, calcite becomes depleted in sediment limited systems. Seasonal progression at a single outlet is marked with a dashed line. In the Werenskioldbreen data, ice, and water from the same naled are connected with a solid line. The data from Werenskioldbreen and Finsterwalderbreen represents seasonal or multi-sample averages; data from Austre Grønfjordbreen represents single samples.
Among the glaciers studied in the Alps, Damma, and Rhone Glaciers (which are silicate-bedded) have relatively small seasonal shifts (Figure 2). At Damma, outlet waters became increasingly sediment supply limited (with less calcite weathering) as the season progressed and had only slight change in gas limitation. At Rhone, the waters became both sediment supply and gas limited (i.e., sulfate weathering of silicates increased) during peak flow and shifted back toward sediment supply and gas abundance (i.e., carbonation weathering of carbonates) as the melt season waned. This trend is the opposite of what was observed at Werenskioldbreen, which varied similarly, but with summer gas and sediment supply abundance and winter gas and sediment supply limitation. At Oberaar (which is carbonate-based), most of the variability is in gas limitation, with the least gas limitation (i.e., least sulfur oxidation) in early flow and the most gas limitation in winter. At Haut d’Arolla, the three borehole types identified by Tranter et al. (2002) vary inversely with respect to sediment supply and gas limitation. The boreholes with the greatest total dissolved solids have the least sediment supply limitation and the most gas limitation (i.e., sulfate weathering of carbonates). The average of annual outflow during the two melt seasons is less sediment supply limited and/or more gas limited than the trend indicated by the average of borehole types. At Tsanfleuron, the central outlet and the proglacial springs integrate the largest subglacial region and have the greatest interaction with groundwater (Fairchild et al., 1994; Zeng et al., 2012). These are the least gas limited (i.e., least sulfur weathering); smaller lateral outlets show higher proportions of sulfate and therefore greater gas limitation.
At the West Greenland sites where season-long monitoring occurred, the shift in meltwater chemistry between long residence time early flow and short residence time peak flow is substantially different between the various outlet glaciers (Figure 3). At Watson River, early flow from May, 2006 was strongly sediment supply limited and gas abundant (i.e., increased carbonation weathering of silicates) compared to seasonally later flow measured in subsequent seasons (Yde et al., 2014). Early melt waters at Leverett Glacier measured in 2012 showed the opposite trend with gas limitation in early flow but sediment supply limitation in later flow (Hawkings et al., 2016). The two outlets have an almost exactly opposite trend (Figure 3). At Isunnguata Sermia, summer outlet flow was assessed over several days in 2011 and 2013 and from single samples in 2010 and 2012 (Graly et al., 2014, 2017b). These years differ substantially in their degree of sediment supply and gas limitation (Figure 3). The samples which had a high amount of melt early in the season (6–10, 6–12) are much more sediment supply limited and less gas limited than the samples from seasons where the melt season progressed more slowly (6–11, 7–13). The chemistry of subglacial boreholes is variable but is consistently more sediment supply limited and less gas limited (i.e., greater carbonation weathering of silicates) than the terminus results from the low melt seasons. Lateral outlets were opposite of terminal outlets, with generally more sediment supply limitation and less gas limitation than borehole chemistry. The high melt year seasons are more similar to the borehole results than the low melt year results.
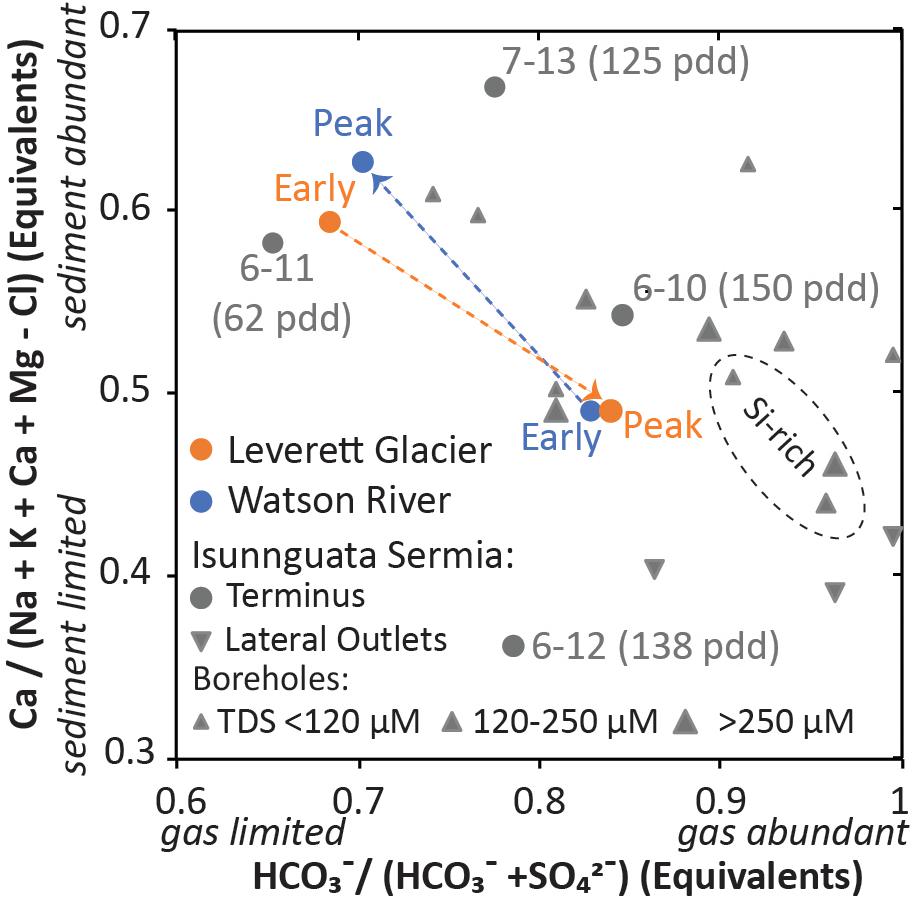
FIGURE 3. Data from Greenland. Data from Leverett Glacier, Watson River, 6–11 and 7–13 represent multi-sample averages. Labels on Isunnguata Sermia terminus samples indicate month and year of collection and the number of positive degree days in the melt season to date. In the boreholes labeled Si-rich, the Ca likely comes from stoichiometric weathering of silicate minerals rather than calcite, and are thus likely more sediment supply limited than the y-axis metric implies.
Discussion
Based on the results from Svalbard, the Alps, and Greenland, a more complex view is needed than previous flow models that predicted a direct relationship between increasing water residence time, total dissolved solids, and the relative abundance of carbonate and sulfide products in subglacial meltwaters (e.g., Tranter et al., 2002). Waters that emerge from glacial outlets during the winter and waters that are flushed out with the early spring melt are assumed to have the longest residence times (e.g., Wadham et al., 2000). In many cases, such as the naledi of Svalbard, the high sulfate content of overwinter waters suggests limitation in the availability of atmospheric gases. However, this effect does not seem to operate in all settings. Particularly, the Watson River in Greenland and the silicate-rich alpine glaciers either lack the gas limitation in winter that elsewhere promotes sulfur weathering or show an opposite effect where summer waters are more sulfate-rich and therefore gas limited. In many cases, long residence times also strongly effect the cation balance, particularly the relative abundance of easily reacted calcite in the waters. Depletion of calcite (and therefore limits in the supply of available sediment) are suggested in long residence time waters from Werenskioldbreen, Finterswalderbreen, and Watson River. However, the opposite effect, with increased calcite dissolution and therefore sediment supply in long residence time waters, is seen at Leverett, Damma, and Rhone Glaciers. Whether gas limitation correlates or anticorrelates to sediment supply limitation also varies between sites. Therefore, there is no one model for the effect of overwinter subglacial water storage. It is likely that the specific glacial hydrologic regime is controlled by glacier scale, sliding rate, basal thermal regime, bedrock geology, glacial surface geometry, and bed geometry, among other possible factors. These factors are likely responsible for the varying chemical consequences of changing flow regimes.
The two-variable model offers a new lens to interpret spatial variability in subglacial waters either known through the borehole sampling of subglacial waters or the parallel sampling of multiple glacial outlets. The classic borehole study at Haut d’Arolla Glacier divided boreholes into three categories based on correspondingly increasing total dissolved solids and sulfur oxidation, which was interpreted as corresponding with water residence time and degree of hydrological isolation (Tranter et al., 2002). The conceptual model presented here shows that the samples with the lowest total dissolved solids and fewest oxidized species are also the most sediment supply limited, in that proportionally less calcite has been dissolved in the waters (Figure 2). This sediment supply limitation, and not necessarily residence time, may be responsible for the relative difference in total dissolved solids between the Haut d’Arolla borehole types (though both factors are likely involved). In the Isunnguata Sermia (Greenland Ice Sheet) boreholes, there is not a consistent relationship between total dissolved solids and sediment supply limitation (Figure 3).
At the sites where multiple boreholes or secondary outlets were compared to a terminus or downstream site that integrated subglacial output, the average of the point locations differed from the integrated whole. Boreholes at Isunnguata Sermia and sub-outlets of Werenskioldbreen tended to be more sediment supply limited (with less calcite dissolution) and gas abundant (with greater carbonation weathering) than their respective glacial outlets. Haut d’Arolla boreholes were either more gas limited or sediment supply limited than the outlet water chemistry. The central Tsanfleuron outlet was less gas limited compared to lateral sites. At both Tsanfleuron and Austre Grønfjordbreen proglacial lakes and springs show almost exclusively calcite dissolution, indicating ample atmospheric CO2 and mineral supply. These data all suggest that point sources on the glacial bed and outlets with more limited spatial integration are not representative of the integrated glacial hydrologic system. This likely reflects the fact that much of the total outflux of the glacial hydrologic system comes from large conduits (Yde et al., 2014). Such conduits are line features on the landscape and have only partial and pressure dependent exchange with the water distributed across the rest of the bed (Meierbachtol et al., 2016; Graly et al., 2017b). At Isunnguata Sermia, the shift in the terminus water composition toward that found in boreholes during high melt years (Figure 3) is consistent with greater flushing of waters from the distributed system. Information about conditions at point locations and not just the integrated result at the terminus is therefore necessary to accurately characterize the chemical conditions prevalent at glacial beds.
The sediment supply and gas limitation conceptual models describe contrasting mechanisms by which the subglacial hydrochemical system affects the underlying landscape and the sort of nutrient output it produces. A sediment supply limited system with only moderate gas limitation is a transport limited system, with transformation of primary rock-derived minerals to secondary minerals such as clays, oxides, and oxyhydroxides controlling nutrient output. In a gas limited system, processes such as anaerobic decay of organic matter and sulfur oxidation or reduction can provide important biochemical fluxes. Where neither limitation occurs, subglacial hydrochemical systems are weathering limited, with solutes only deriving from the carbonation dissolution of calcite, while large fluxes of freshly ground sediments are sent to marine and terrestrial landscapes. Though a complex series of controls exists, long term chemical modification of the landscape under glaciers can be understood as directly controlled by the prevalence of the weathering modes described by these two conceptual models.
Conclusion
A synthesis of spatial and temporal variation in hydrochemical data from several glaciers and glacial outlets in the Alps, Svalbard, and Greenland suggests that a single assessment of residence time is insufficient to capture the chemical differences observed. We provide a simple alternative model that assesses the degree to which either limitations in the availability of atmospheric gases or unaltered fine grain sediment affect chemical processes in the subglacial system. The relative influence of these limitations varies considerably between glaciers and no one relationship between these factors and water residence time exists across multiple sites. Where chemical data is collected alongside detailed glaciological observations, these two conceptual models will aid in understanding which glacial settings cause atmospheric gas to be drawn into the subglacial system and which promote comminution of the underlying bedrock material.
Author Contributions
This paper was written and principally developed by the lead author JG. NH mentored JG during his Ph.D. research, from which this paper was derived. KL is JG’s present post-doctoral mentor and provided suggestions and insights that led to the development of this paper. Both NH and KL reviewed and edited the manuscript.
Funding
This work was made possible by funding from the IUPUI Office of the Vice Chancellor for Research and came out of previous work that was funded through National Science Foundation grant ARC 0909122.
Conflict of Interest Statement
The authors declare that the research was conducted in the absence of any commercial or financial relationships that could be construed as a potential conflict of interest.
Acknowledgments
We would like to thank AH for handling the editorial process and the three reviewers for submitting their comments and improving the quality of the manuscript.
References
Alley, R. B., Cuffey, K. M., Evenson, E. B., Strasser, J. C., Lawson, D. E., and Larson, G. J. (1997). How glaciers entrain and transport basal sediment: physical constraints. Quat. Sci. Rev. 16, 1017–1038. doi: 10.1016/S0277-3791(97)00034-6
Anderson, S. P. (2005). Glaciers show direct linkage between erosion rate and chemical weathering fluxes. Geomorphology 67, 147–157. doi: 10.1016/j.geomorph.2004.07.010
Anderson, S. P., Drever, J. I., and Humphrey, N. F. (1997). Chemical weathering in glacial environments. Geology 25, 399–402. doi: 10.1130/0091-7613(1997)025<0399:CWIGE>2.3.CO;2
Anderson, S. P., Longacre, S. A., and Kraal, E. R. (2003). Patterns of water chemistry and discharge in the glacier-fed Kennicott River, Alaska: evidence of subglacial water storage cycles. Chem. Geol. 202, 297–312. doi: 10.1016/j.chemgeo.2003.01.001
Berner, W., Bucher, P., Oescher, H., and Stauffer, B. (1977). Analysis and Interpretation of Gas Content and Composition in Natural Ice, Vol. 118. London: IAHS, 272–294.
Blum, A. E., and Stillings, L. L. (1995). “Feldspar dissolution kinetics,” in Chemical Weathering Rates of Silicate Minerals, Vol. 31, eds A. F. White and S. L. Brantley (Chantilly, VA: Mineralogical Society of America), 291–351.
Bottrell, S. H., and Tranter, M. (2002). Sulphide oxidation under partially anoxic conditions at the bed of the Haut Glacier d’Arolla, Switzerland. Hydrol. Process. 16, 2363–2368. doi: 10.1002/hyp.1012
Brown, G., Sharp, M., and Tranter, M. (1996). Subglacial chemical erosion: seasonal variations in solute provenance, Haut Glacier d’Arolla, Valais, Switzerland. Ann. Glaciol. 22, 25–31. doi: 10.1017/S0260305500015172
Chandler, D. M., Wadham, J. L., Lis, G. P., Cowton, T., Sole, A., Bartholomew, I., et al. (2013). Evolution of the subglacial drainage system beneath the Greenland Ice Sheet revealed by tracers. Nat. Geosci. 6, 195–198. doi: 10.1038/ngeo1737
Drever, J. I., and Hurcomb, D. R. (1986). Neutralization of atmospheric acidity by chemical weathering in an alpine drainage basin in the North Cascade Mountains. Geology 14, 221–224. doi: 10.1130/0091-7613(1986)14<221:NOAABC>2.0.CO;2
Fairchild, I. J., Bradby, L., Sharp, M., and Tison, J. L. (1994). Hydrochemistry of carbonate terrains in apline glacial settings. Earth Surf. Process. Landf. 19, 33–54. doi: 10.1002/esp.3290190104
Graly, J. A., Drever, J. I., and Humphrey, N. F. (2017a). Calculating the balance between atmospheric CO2 drawdown and organic carbon oxidation in subglacial hydrochemical systems. Glob. Biogeochem. Cycles 31, 709–727. doi: 10.1002/2016GB005425
Graly, J. A., Harrington, J., and Humphrey, N. (2017b). Combined diurnal variations of discharge and hydrochemistry of the Isunnguata Sermia outlet, Greenland Ice Sheet. Cryosphere 11, 1131–1140. doi: 10.5194/tc-11-1131-2017
Graly, J. A., Humphrey, N. F., and Harper, J. T. (2016). Chemical depletion of sediment under the Greenland Ice Sheet. Earth Surf. Process. Landf. 41, 1922–1936. doi: 10.1002/esp.3960
Graly, J. A., Humphrey, N. F., Landowski, C. M., and Harper, J. T. (2014). Chemical weathering under the Greenland Ice Sheet. Geology 42, 551–554. doi: 10.1130/G35370.1
Hallet, B. (1996). Glacial quarrying a simple theoretical model. Ann. Glaciol. 22, 1–8. doi: 10.1017/S0260305500015147
Hawkings, J., Wadham, J., Tranter, M., Lawson, E., Sole, A., Cowton, T., et al. (2015). The effect of warming climate on nutrient and solute export from the Greenland Ice Sheet. Geochem. Perspect. Lett. 1, 94–104. doi: 10.7185/geochemlet.1510
Hawkings, J., Wadham, J., Tranter, M., Telling, J., Bagshaw, E., Beaton, A., et al. (2016). The Greenland Ice Sheet as a hot spot of phosphorus weathering and export in the Arctic. Glob. Biogeochem. Cycles 30, 191–210. doi: 10.1002/2015GB005237
Hindshaw, R. S., Tipper, E. T., Reynolds, B. C., Lemarchand, E., Wiederhold, J. G., Magnusson, J., et al. (2011). Hydrological control of stream water chemistry in a glacial catchment (Damma Glacier, Switzerland). Chem. Geol. 285, 215–230. doi: 10.1016/j.chemgeo.2011.04.012
Hodson, A., Tranter, M., and Vatne, G. (2000). Contemporary rate of chemical denudation and atmospheric CO2 sequestration in glacier basins: an arctic perspective. Earth Surf. Process. Landf. 25, 1447–1471. doi: 10.1002/1096-9837(200012)25:13<1447::AID-ESP156>3.0.CO;2-9
Hosein, R., Arn, K., Steinmann, P., Adatte, T., and Föllmi, K. B. (2004). Carbonate and silicate weathering in two presently glaciated, crystalline catchments in the Swiss Alps. Geochim. Cosmochim. Acta 68, 1021–1033. doi: 10.1016/S0016-7037(03)00445-9
Iverson, N. R., and Semmens, D. J. (1995). Intrusion of ice porous media by regelation: a mechanism of sediment entrainment by glaciers. J. Geophys. Res. 100, 10219–10230. doi: 10.1029/95JB00043
Joughin, I., Das, S. B., King, M. A., Smith, B. E., Howat, I. M., and Moon, T. (2008). Seasonal speedup along the western flank of the Greenland Ice Sheet. Science 320, 781–783. doi: 10.1126/science.1153288
Kalsbeek, F., Austrheim, H., Bridgwater, D., Hansen, B. T., Pedersen, S., and Taylor, P. N. (1993). Geochronology of Archaean and Proterozoic events in the Ammassalik area, South-East Greenland, and comparisons with the Lewisian of Scotland and the Nagssugtoqidian of West Greenland. Precambrian Res. 62, 239–270. doi: 10.1016/0301-9268(93)90024-V
MacGregor, J. A., Fahnestock, M. A., Catania, G. A., Aschwanden, A., Clow, G. D., Colgan, W. T., et al. (2016). A synthesis of the basal thermal state of the Greenland Ice Sheet. J. Geophys. Res. Earth Surf. 121, 1328–1350. doi: 10.1002/2015JF003803
Meierbachtol, T. W., Harper, J. T., Humphrey, N. F., and Wright, P. J. (2016). Mechanical forcing of water pressure in a hydraulically isolated reach beneath Western Greenland’s ablation zone. Ann. Glaciol. 57, 62–70. doi: 10.1017/aog.2016.5
Moore, J., Jacobson, A. D., Holmden, C., and Craw, D. (2013). Tracking the relationship between mountain uplift, silicate weathering, and long-term CO2 consumption with Ca isotopes: Southern Alps, New Zealand. Chem. Geol. 341, 110–127. doi: 10.1016/j.chemgeo.2013.01.005
Raiswell, R. (1984). Chemical models of solute acquisition in glacial melt waters. J. Glaciol. 30, 49–57. doi: 10.1017/S0022143000008480
Schohl, G., and Ettema, R. (1986). Theory and laboratory observations of naled ice growth. J. Glaciol. 32, 168–177. doi: 10.1017/S0022143000015483
Sharp, M., Tranter, M., Brown, G. H., and Skidmore, M. (1995). Rates of chemical denudation and CO2 drawdown in a glacier-covered alpine catchment. Geology 23, 61–64. doi: 10.1130/0091-7613(1995)023<0061:ROCDAC>2.3.CO;2
Stachnik,Ł., Majchrowska, E., Yde, J. C., Nawrot, A. P., Cichała-Kamrowska, K., Ignatiuk, D., et al. (2016a). Chemical denudation and the role of sulfide oxidation at Werenskioldbreen, Svalbard. J. Hydrol. 538, 177–193. doi: 10.1016/j.jhydrol.2016.03.059
Stachnik,Ł., Yde, J. C., Kondracka, M., Ignatiuk, D., and Grzesik, M. (2016b). Glacier naled evolution and relation to the subglacial drainage system based on water chemistry and GPR surveys (Werenskioldbreen, SW Svalbard). Ann. Glaciol. 57, 19–30. doi: 10.1017/aog.2016.9
Tranter, M., Brown, G., Raiswell, R., Sharp, M., and Gurnell, A. (1993). A conceptual model of solute aquisition by Alpine glacial meltwaters. J. Glaciol. 39, 573–581. doi: 10.1017/S0022143000016464
Tranter, M., Sharp, M. J., Lamb, H. R., Brown, G. H., Hubbard, B. P., and Willis, I. C. (2002). Geochemical weathering at the bed of the Haut Glacier d’Arolla, Switzerland - a new model. Hydrol. Process. 16, 959–993. doi: 10.1002/hyp.309
van Gool, J. A. M., Connelly, J. N., Marker, M., and Mengel, F. C. (2002). The Nagssugtoqidian Orogen of West Greenland: tectonic evolution and regional correlations from a West Greenland perspective. Can. J. Earth Sci. 39, 665–686. doi: 10.1139/e02-027
Wadham, J., Hodson, A., Tranter, M., and Dowdeswell, J. (1998). The hydrochemistry of meltwaters draining a polythermal-based, high Arctic glacier, south Svalbard: I. The ablation season. Hydrol. Process. 12, 1825–1849. doi: 10.1002/(SICI)1099-1085(19981015)12:12<1825::AID-HYP669>3.0.CO;2-R
Wadham, J. L., Bottrell, S., Tranter, M., and Raiswell, R. (2004). Stable isotope evidence for microbial sulphate reduction at the bed of a polythermal high Arctic glacier. Earth Planet. Sci. Lett. 219, 341–355. doi: 10.1016/S0012-821X(03)00683-6
Wadham, J. L., Tranter, M., and Dowdeswell, J. A. (2000). Hydrochemistry of meltwaters draining a polythermal-based, high-Arctic glacier, south Svalbard: II. Winter and early Spring. Hydrol. Process. 14, 1767–1786. doi: 10.1002/1099-1085(200007)14:10<1767::AID-HYP103>3.0.CO;2-Q
Wadham, J. L., Tranter, M., Skidmore, M., Hodson, A. J., Priscu, J., Lyons, W. B., et al. (2010). Biogeochemical weathering under ice: size matters. Glob. Biogeochem. Cycles 24:GB3025. doi: 10.1029/2009GB003688
White, A. F., Schulz, M. S., Lowenstern, J. B., Vivit, D. V., and Bullen, T. D. (2005). The ubiquitous nature of accessory calcite in granitoid rocks: implication for weathering, solute evolution, and petrogenesis. Geochim. Cosmochim. Acta 69, 1455–1471. doi: 10.1016/j.gca.2004.09.012
Yde, J. C., Hodson, A. J., Solovjanova, I., Steffensen, J. P., Nørnberg, P., Heinemeier, J., et al. (2012). Chemical and isotopic characteristics of a glacier-derived naled in front of Austre Grønfjordbreen, Svalbard. Polar Res. 31, 1–15. doi: 10.3402/polar.v31i0.17628
Yde, J. C., Knudsen, N. T., Hasholt, B., and Mikkelsen, A. B. (2014). Meltwater chemistry and solute export from a Greenland Ice Sheet catchment, Watson River, West Greenland. J. Hydrol. 519, 2165–2179. doi: 10.1016/j.jhydrol.2014.10.018
Zeng, C., Gremaud, V., Zeng, H., Liu, Z., and Goldscheider, N. (2012). Temperature-driven meltwater production and hydrochemical variations at a glaciated alpine Karst aquifer: implication for the atmospheric CO2 sink under global warming. Eviron. Earth Sci. 65, 2285–2297. doi: 10.1007/s12665-011-1160-3
Keywords: chemical weathering, subglacial hydrology, seasonal variation, glacial processes, hydrochemistry, Greenland ice sheet, svalbard, Alps–northern appennine
Citation: Graly JA, Humphrey NF and Licht KJ (2018) Two Metrics Describing the Causes of Seasonal and Spatial Changes in Subglacial Aqueous Chemistry. Front. Earth Sci. 6:195. doi: 10.3389/feart.2018.00195
Received: 15 May 2018; Accepted: 19 October 2018;
Published: 06 November 2018.
Edited by:
Andrew Jonathan Hodson, University Centre in Svalbard, NorwayReviewed by:
Christian Schröder, University of Stirling, United KingdomFrancois L. L. Muller, National Sun Yat-sen University, Taiwan
Jacob Clement Yde, Western Norway University of Applied Sciences, Norway
Copyright © 2018 Graly, Humphrey and Licht. This is an open-access article distributed under the terms of the Creative Commons Attribution License (CC BY). The use, distribution or reproduction in other forums is permitted, provided the original author(s) and the copyright owner(s) are credited and that the original publication in this journal is cited, in accordance with accepted academic practice. No use, distribution or reproduction is permitted which does not comply with these terms.
*Correspondence: Joseph A. Graly, amdyYWx5QGl1cHVpLmVkdQ==