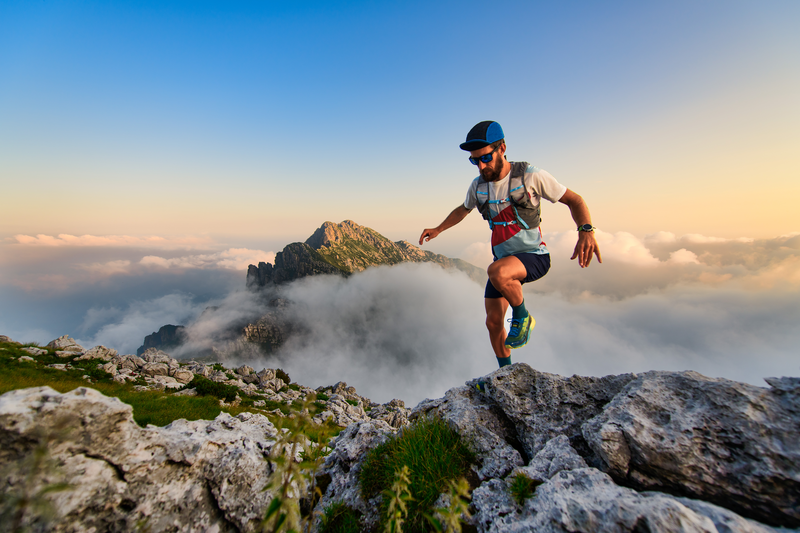
94% of researchers rate our articles as excellent or good
Learn more about the work of our research integrity team to safeguard the quality of each article we publish.
Find out more
ORIGINAL RESEARCH article
Front. Earth Sci. , 02 November 2017
Sec. Paleontology
Volume 5 - 2017 | https://doi.org/10.3389/feart.2017.00088
This article is part of the Research Topic Biology and Evolutionary History of Mesosaurs, the Oldest Known Aquatic Reptiles View all 7 articles
A commentary has been posted on this article:
Commentary: A Reassessment of the Taxonomic Position of Mesosaurs, and a Surprising Phylogeny of Early Amniotes
We reassess the phylogenetic position of mesosaurs by using a data matrix that is updated and slightly expanded from a matrix that the first author published in 1995 with his former thesis advisor. The revised matrix, which incorporates anatomical information published in the last 20 years and observations on several mesosaur specimens (mostly from Uruguay) includes 17 terminal taxa and 129 characters (four more taxa and five more characters than the original matrix from 1995). The new matrix also differs by incorporating more ordered characters (all morphoclines were ordered). Parsimony analyses in PAUP 4 using the branch and bound algorithm show that the new matrix supports a position of mesosaurs at the very base of Sauropsida, as suggested by the first author in 1995. The exclusion of mesosaurs from a less inclusive clade of sauropsids is supported by a Bremer (Decay) index of 4 and a bootstrap frequency of 66%, both of which suggest that this result is moderately robust. The most parsimonious trees include some unexpected results, such as placing the anapsid reptile Paleothyris near the base of diapsids, and all of parareptiles as the sister-group of younginiforms (the most crownward diapsids included in the analyses). Turtles are placed among parareptiles, as the sister-group of pareiasaurs (and in diapsids, given that parareptiles are nested within diapsids). This unexpected result offers a potential solution to the long-lasting controversy about the position of turtles because previous studies viewed a position among diapsids and among parareptiles as mutually exclusive alternatives.
Mesosaurs, a small clade (the three nominal genera and species usually recognized are currently in revision) of Early Permian amniotes known from South America (Brazil and Uruguay) and southern Africa (Namibia and South Africa) are notable in several respects (Piñeiro, 2008). They are the only Early Permian amniotes known from high latitudes. They have long been considered marine, but a recent study of their paleoenvironment suggests that they inhabited a moderately hypersaline sea (Piñeiro et al., 2012c). Likewise, the occasional suggestions that they were piscivorous (e.g., Bakker, 1975) seem unlikely because the few acanthodians and actinopterygians that occur in the same formations as mesosaurs appear to be present in different strata, and the stomacal content of mesosaurs is known to contain only pygocephalomorph crustaceans and possibly, younger mesosaurs, which may represent embryos still in utero (Piñeiro et al., 2012a) or carrion (Silva et al., 2017). Mesosaurs apparently captured their prey with their long snout and sieve-like long, slender teeth. They typically measured <2 m in total length, and apparently did not swim very fast, with an optimal swimming speed estimated at 0.15–0.55 m/s (Villamil et al., 2016).
Mesosaurs were the first amniotes to return to an aquatic lifestyle (Canoville and Laurin, 2010). Even though uncertainty remains about just how terrestrial the first amniotes were, the latest bone microanatomical study suggests that they had become fairly terrestrial (Laurin and de Buffrénil, 2016). Romer (1957, 1958) had initially suggested that the first amniotes were still largely aquatic to amphibious and came onto land mostly to lay eggs. This idea was based largely on the assumed primitively aquatic to amphibious lifestyle of limnoscelids (which remain to be assessed using bone microanatomy) and of the early synapsid Ophiacodon. However, it now appears that Ophiacodon was more terrestrial than initially thought, and the geologically older ophiacodontid Clepsydrops has a typically terrestrial bone microanatomy (Felice and Angielczyk, 2014; Laurin and de Buffrénil, 2016). This leaves the mesosaurs as the first amniotes to have reverted to a clearly aquatic lifestyle.
Mesosaurs also document the first case of extended embryo retention, probably in the form of viviparity (Piñeiro et al., 2012a), given that most of the clues that have been use to suggest viviparity in several Mesozoic marine amniotes also occur in mesosaurs, except for embryos engaged in the birth canals, documented so far only in ichthyosaurs (Motani et al., 2014). These include very small individuals present in the abdominal cavity of much larger ones, though some may represent cannibalism rather than viviparity, as well as parental care of juveniles (Piñeiro et al., 2012a).
Last but not least, mesosaurs have been hypothesized to have been either the sister-group of a large clade that includes parareptiles and eureptiles (Laurin and Reisz, 1995), or the basalmost parareptiles (Modesto, 1999). All this, coupled with the presence of temporal fenestration in mesosaurs (Piñeiro et al., 2012b), makes reassessing the taxonomic affinities of mesosaurs timely.
This study makes no serious attempt at reassessing the origin of turtles, a fascinating but challenging topic that would require a paper of its own. Until the 1990s, turtles were considered to be closely related to captorhinid eureptiles (Clark and Carroll, 1973; Gauthier et al., 1988), which, like all extant turtles, have an anapsid temporal configuration. In the 1990s, the consensus shifted toward an origin of turtles among parareptiles, either as sister-group of procolophonoids (Reisz and Laurin, 1991; Laurin and Reisz, 1995) or of pareiasaurs (Lee, 1993, 1996; Lyson et al., 2010), before shifting swiftly (though not unanimously) thereafter to an origin among diapsids, based on morphological (Rieppel and deBraga, 1996; deBraga and Rieppel, 1997) and molecular data (Hugall et al., 2007; Chiari et al., 2012). A possible stem-turtle, which appears to have upper temporal fenestrae (Schoch and Sues, 2015, 2017), further supports this hypothesis. To complicate things further, some recent studies suggest that the position of turtles is difficult to resolve with confidence because various genes suggest conflicting histories, and that this reflects the “true state of nature” (Lu et al., 2013). Thus, reassessing this question seriously would require adding many additional taxa, and ideally, incorporating molecular as well as morphological data. Clearly, this is an endeavor distinct from assessing the affinities of mesosaurs, which is the goal we pursue in this study. Nevertheless, the taxonomic sample selected here might allow a very limited test of where, among parareptiles, turtles fit, if they fit there at all. Diapsid diversity is great and given that very little of it is sampled here, our study is not designed to try to assess the relative merits of these two competing hypotheses (turtles inside vs. outside diapsids). Given the controversy surrounding the affinities of turtles, in this paper, this vernacular word will refer to crown-turtles, as well as all undoubted stem-turtles with at least a partial carapace, namely, among the taxa discussed below, Proganochelys and Odontochelys.
We started from the matrix of Laurin and Reisz (1995), given that this was the matrix that we knew best, that we had confidence in the accuracy of the anatomical scoring, and that we were confident that we could apply the revised scores in a manner coherent with the original scoring. This last point is particularly important because even if a matrix was scored by a systematist in a perfectly coherent and justified way, another systematist may score additional taxa, or revise the scoring based on new information, in a way that is internally coherent, but incoherent with the original scoring. Using reliably a matrix produced by other authors requires assessing how states were conceived and delimited, and applying the same delimitations in the revised or new scores.
As explained above, our taxon selection is inadequate to test the position of turtles (within vs. outside diapsids). Therefore, some sensitivity analyses were performed to assess the robustness of our conclusions to the inclusion or exclusion of turtles; this allows assessing if the position of turtles (close to mesosaurs, among parareptiles, or much farther, deep inside diapsids) impacts on the assessment of the affinities of mesosaurs, or on the robustness of our conclusions. The only additional taxa, compared to the matrix of Laurin and Reisz (1995) are thus the early parareptile Acleistorhinus, which is now much better known because of the anatomical description given by deBraga and Reisz (1996), the procolophonoid Owenetta kitchingorum, which was thoroughly described by Reisz and Scott (2002), and the stem-turtle Odontochelys (Li et al., 2008). We also split the OTU Testudines, which incorporated Proganochelys in the scoring of Laurin and Reisz (1995), into separate Proganochelys and Chelonii, which we scored based on all turtles crownward of Proganochelys. The source of the scores is documented in the Mesquite Nexus file (SOM 1, on the HAL web site) as notes in individual cells and in the taxon name cell, but in the case of Chelonii, scoring is based on several stem-turtles, such as Australochelys (Gaffney and Kitching, 1995), Condorchelys (Sterli, 2008), Palaeochersis (Sterli et al., 2007), Kayentachelys (Sterli and Joyce, 2007), and Indochelys (Datta et al., 2000), in addition to extant and extinct crown-turtles (Gaffney, 1979; Gaffney et al., 2006). The revised matrix thus has 17 terminal taxa, up from 13 taxa in Laurin and Reisz (1995). We deliberately changed the name of the OTU including extant turtles and part of its stem from Testudines to Chelonii to draw attention to the fact that this OTU has changed somewhat. The choice of the name is further justified simply by the fact that Brongniart (1800) was the first to erect a higher taxon from the class-series (which encompasses orders), rather than family-series that encompassed all or most turtles that were then known, and he named it “Chéloniens,” soon thereafter latinized as “Chelonii” by Latreille (1800; see also Dubois and Bour, 2010). The zoological code does not include rules of priority for class-series nomina, but by analogy to such rules for family and genus-series nomina, Dubois and Bour (2010) suggest using this name, and their suggestion is followed here, given that the name Testudines was clearly intended as a genus- and a family-series nomen. Finally, note that the composition of Chelonii as delimited here does not match Testudines as defined by Joyce et al. (2004), which applies the turtle crown.
We did not add new characters to the matrix (though we split some; see below), but we ordered some characters because they appear to form morphoclines. In this respect, our approach differs from that followed by Laurin and Reisz (1995), which we quote in full because it is highly relevant to what follows. They stated: “A few characters were ordered in this study (Appendix 1 in Supplementary Material). The controversy over whether multi-state characters should be ordered or left unordered is not settled. Some have argued against the use of ordered characters (Mabee, 1989; Hauser and Presch, 1991), while others have argued that characters should be ordered when possible (Mickevich and Lipscomb, 1991; Slowinski, 1993). We have used a mixed approach. All multi-state characters exhibiting what seemed to be a morphocline were mapped on the shortest tree (found with unordered characters only) using MacClade 3.0 (Maddison and Maddison, 1992). When the optimization of the character supported the existence of a morphocline, the character was ordered. Support for the morphocline required that all state transformations for the relevant character be compatible with the morphocline. If a single transformation was ambiguous, the character was not ordered. This procedure allowed us to order six characters (Appendix 1 in Supplementary Material).”
In the more than 20 years that passed after publication of that paper, one of us (ML) has become involved in research on this topic (Grand et al., 2013; Rineau et al., 2015), and this simulation-based work has shown unambiguously that characters that form morphoclines should be ordered because this maximizes resolution power (the ability to recover correct clades) and minimizes false resolutions (artifactual clades). The additional criterion invoked by Laurin and Reisz (1995) consisting in requiring that optimization of each initially unordered character be fully compatible with the ordering scheme now appears invalid, for two main reasons.
First, this assumes that the initially-obtained tree is the correct one, which is never certain in an empirical study, and even less so if ordering scheme of multi-state characters is suboptimal. In this respect, note that in the extreme case of each taxon having a different state, an unordered character has no phylogenetic information content, whereas an ordered character will convey maximal phylogenetic information content.
Second, requiring state optimization to match the presumed morphocline on the tree assumes that all relevant taxa have been included. This is generally not the case, for several reasons: most empirical studies do not include all known extant species of a clade; in some taxa, only a small fraction of the extant biodiversity has been described (Mora et al., 2011); not all extinct taxa (if any) known from the fossil record are typically included, and in any case, the fossil record of most taxa is fragmentary at best (Foote and Sepkoski, 1999; Didier et al., 2017). Thus, this second reason alone would be more than sufficient grounds not to require a priori ordering schemes to be validated through optimization of unordered states onto a tree.
Many more characters (21) were thus ordered. These are (in our numbering; this does not match the numbers in Laurin and Reisz, 1995): 6, 15, 17, 19, 25, 35, 37, 40, 49, 51, 57, 74, 85, 93, 99, 101, 110, 112, 121, 123 (which was binary in Laurin and Reisz, 1995), 128, and 129. In some cases, the states had to be reordered because the initial scheme of Laurin and Reisz (1995) had state 0 as the primitive state; this is not necessarily the case here because the primitive condition may be in the middle of a morphocline. Thus, the states were not necessarily listed by Laurin and Reisz (1995) in an order coherent with a morphocline. This was not problematic for Laurin and Reisz (1995) given that they treated these characters as unordered, but treating them as morphoclines, as done here, requires reordering the states. The only difficult cases are those in which the morphocline seems likely but not absolutely certain. For instance, we ordered character 48 [ectopterygoid: large (0); small (1); absent (2)] because we hypothesize that the ectopterygoid was lost through eduction in size rather than fusion to a neighboring element, a hypothesis supported by the fact that some Permian amniotes, such as, O. kitchingorum (Reisz and Scott, 2002) have a diminutive ectopterygoid, and by the fact that there is no firm evidence that this bone fused to neighboring bones in amniotes or in lissamphibians (Müller et al., 2005). In a broader taxonomic context, this is also consistent with the finding that in temnospondyls, the closest relatives of Doleserpeton, which lacks an ectopterygoid, have a small ectopterygoid, though lepospondyls apparently provide a counter-example (Kimmel et al., 2009). However, if that hypothesis turned out to be false, this ordering would be unwarranted. More information about the characters that were ordered and the exact ordering schemes can be seen in SOM 1 (the matrix in a Mesquite Nexus format).
In the same spirit, we also split some characters that appeared to encompass two or more distinct characters, or merged characters that seem to reflect a single cline. Thus, character 27 (“occipital flange of squamosal”), from Laurin and Reisz (1995), was split into two characters (here, numbers 27 and 28) because we think that the original character can be better considered to consist of two logically independent characters. The initial formulation thus included six states: “Occipital flange of squamosal: in otic notch and overlaps pterygoid (0); gently convex all along the posterior edge of the skull (1); convex above quadrate emargination and concave medial to tympanic ridge (2); absent (3); medial to tympanic ridge, facing posteromedially (4); medial to tympanic ridge, concave, facing posterolaterally or ventrolaterally (5).” These six states were unordered and indeed, it is difficult to see how they could have been ordered, but this rendered this character of little usefulness, given that there were 13 OTUs. We think that it is better to separate the presence or absence of a squamosal contribution to the otic notch or quadrate emargination (in the emargination) and the position of the squamosal, either mostly on the cheek (primitive condition) or largely on the skull table (derived condition). These two binary characters may capture most of the information content of this character. Under both the initial formulation and the new one, accurate scoring requires reasonably undistorted skulls because on severely flattened ones, exact orientation of the squamosal would be difficult to determine.
Conversely, characters 24 and 25 (maxillary region and maxillary tooth) were merged into a single ordered character because these can be conceptualized as increasing differentiation of the tooth row, from a homogeneous tooth row, to the presence of a caniniform region, and finally, the presence of a genuine caniniform tooth (a tooth much larger than the neighboring ones; two positions may be concerned, but normally, only one is occupied by a tooth, because of the continuous replacement pattern).
The scores that have been changed relative to Laurin and Reisz (1995) are highlighted in yellow in SOM 1. These need not all be commented here, but a few highlights can be given. For mesosaurs, some scores that were unknown (?) in the matrix of Laurin and Reisz (1995) have been entered based on personal observations of the authors on several specimens made in the last 5 years, mostly of the collections of the Faculty of Sciences of the University of the Republic (Montevideo). These collections include dozens of Mesosaurus specimens from the Early Permian Mangrullo Formation (Uruguay). To a lesser extent, we also exploited collections in Brazil, Germany and the more limited collection of mesosaurs in Paris. The list of studied Mesosaurus specimens is provided in SOM 2, a table detailing specimen number, geographic provenance, and brief description. In addition, we also checked the literature to revise the entire matrix, occasionally revising the scoring, whenever warranted.
A few of the revisions can be commented here. The foramen orbitonasale (character 10) was not documented in Laurin and Reisz (1995), but our observations suggest that it is absent in mesosaurs. Similarly, a postorbital/supratemporal contact (character 12), scored as being present in mesosaurs by Laurin and Reisz (1995) is now considered to have been absent (Piñeiro et al., 2012b). The postorbital is now also considered to be located far from the occiput (character 13), whereas Laurin and Reisz (1995) had scored it as being close to it (Figures 1, 2).
Figure 1. Skull of Mesosaurus tenuidens (GP-2E-669a) in right lateral view showing the lower temporal fenestra; picture (A) and interpretive labeled line drawing (B). This specimen is an almost complete skull and part of the postcranial skeleton (not shown) housed in the Fossil Vertebrate Collection of Instituto de Geociencias, São Paulo University. Scale bar: 10 mm. a, angular; ax, axis; cev, cervical vertebra; d, dentary; f, frontal; j, jugal; l, lacrimal; ltf, lower temporal fenestra; mx, maxila; n, nasal; p, parietal; pf, postfrontal; po, postorbital; pp, postparietal; prf, prefrontal; q, quadrate; qj, quadratojugal; sa, surangular; sm, septomaxilla; sp, splenial; sq, squamosal; st, supratemporal.
Figure 2. Mesosaur skull reconstruction based on recent evidence. (A), dorsal view; (B), lateral view. Modified from Piñeiro et al. (2012b).
There was a problem with character 22 (maxilla/quadratojugal contact), for which the list of states had been inverted in Appendix 1 in Supplementary Material (list of characters and states) of Laurin and Reisz (1995), though they were stated correctly in the main text and in Appendix 2 in Supplementary Material (the data matrix). The correct coding is that state 0 (the primitive condition) consists in the two bones to be separated in lateral view, and this condition prevails in mesosaurs (Figures 1, 2).
The lower temporal fenestra (character 32), considered to have been absent in Laurin and Reisz (1995) is now considered present (Piñeiro et al., 2012b), as had been correctly assessed by our great predecessor (Huene, 1941). The tabular bone is now considered to have been mid-sized (character 17), a state that was absent from the initial coding.
The jugal was changed from not reaching the anterior orbital rim (as coded in Laurin and Reisz, 1995) to reaching that level. This condition is shown in Uruguayan specimens (Piñeiro et al., 2012b, Figure 1; Figure 1 herein).
Mesosaurs seem to have a low maxillary eminence (Piñeiro et al., 2012b, Figure 1) that even appears to contact the nasal in a short suture between the external naris and the foramen nariale obturatum. However, given that this low eminence reaches its maximal extent anterior and just posterior to the external naris, we consider it not to be homologous with the anterior process found in several other amniotes, such as, Acleistorhinus (deBraga and Reisz, 1996). To clarify this, we have added, in the character formulation, that this process is located posterior to the naris.
Surprisingly, mesosaurs seem to have a slender stapes in all ontogenetic stages in which it is documented (Figure 3). There is no evidence that it was associated with a tympanum (character 69), which would make no sense in aquatic animals like mesosaurs.
Figure 3. Two specimens of Mesosaurus tenuidens with the stapes. Almost complete fetus at an advanced stage of development (FC-DPV 2504) in (A) general view, with a picture (B) and labeled line drawing (C) of the braincase, including occipital elements and stapes, which have been displaced from the rest of the specimen. Picture (D) and labeled line drawing (E) of a second, almost complete but slightly disarticulated adult specimen (FC–DPV 3067) showing the braincase with the right stapes approximately in its anatomical position (though its distal tip has moved anteriorly). In the interpretive drawings (C,E), the stapes is highlighted in yellow. Both specimens are from the Early Permian Mangrullo Formation of Uruguay. Scale for (D,E) 10 mm. Anatomical abbreviations: ask, anterior skull; bo, basioccipital; bpt, basipterygoid; cev, cervical vertebrae; eo, exoccipital; ga, gastralia; op, opistotic; pbs, parabasisphenoid; pro, prootic; psk, posterior skull; qj, quadratojugal; so, supraoccipital; sta, stapes.
The number of coronoid bones is unclear. Some of our specimens might possibly show two, but this interpretation is highly tentative. We don't see strong evidence that there was a single coronoid either. Thus, we have changed the scoring from a single coronoid (in Laurin and Reisz, 1995) to unknown.
We updated the number of scapulocoracoid ossifications from three to only two (Piñeiro, 2004; Modesto, 2010, p. 1387).
Laurin and Reisz (1995) had a character (102) entitled “Ectepicondylar foramen and groove.” Given that the groove may occur without the foramen, and that a foramen may occur without a groove (whenever the foramen leads into a canal that is sharply angled relative to the bone surface), we have decided to split these into two characters. Mesosaurs were scored by Laurin and Reisz (1995) as having either only the groove, or the groove and foramen. Our observations suggest that the foramen is always present, though it is not always easy to observe (Figure 4). Therefore, we have scored both as present.
Figure 4. Specimens of Mesosaurus tenuidens showing how part and counter-part can lead to divergent interpretations about the presence of the ectepicondylar foramen. (A–C) FC-DPV 2042, 2488, and 2103, respectively, photographs of several humeri of adult individuals from the Mangrullo Formation of Uruguay, showing the presence of both ectepicondylar foramen (red arrow) and groove. Scale bars: 3 mm. (D,E). FC-DPV 2385 photographs of a partially articulated mesosaur trunk region preserved as part (D) and counterpart (E). The humerus in (D) suggests that the ectepicondylar foramen is not present, but it can be perfectly seen in (E). Scale bar: 2 mm.
All these changes in scoring, and others not commented here for lack of space, are documented in SOM 1, a Mesquite Nexus file incorporating the data matrix and several trees, which can be accessed in the HAL open archive (https://hal.archives-ouvertes.fr/) through this link: https://hal.archives-ouvertes.fr/hal-01618314. Note that the Nexus format can also be read by MacClade 3.0 (Maddison and Maddison, 1992) and PAUP 4.0 (Swofford, 2003), but the yellow highlighting to mark the changes is visible only in Mesquite.
The data matrix was analyzed using parsimony (with some states ordered, as mentioned above) using the branch and bound algorithm of PAUP 4.0a155 (Swofford, 2003), which guarantees to find all the most parsimonious trees (Hendy and Penny, 1982). Robustness of the results was assessed both by non-parametric bootstrap analysis (Felsenstein, 1985) with 200 replicates and decay (Bremer) index (Bremer, 1988), both using the branch and bound algorithm. Bootstrap frequencies reported below are rounded off to the nearest percent. To establish the number of extra steps required to move mesosaurs to alternative locations in the tree, skeletal topological constraints were enforced. To assess the robustness of our results to taxonomic sampling, we repeated the analyses with some taxa deleted (Mesosauridae, Chelonii, Proganochelys, and Odontochelys).
The search yielded two most parsimonious tree requiring 383 steps, with a CI of 0.5666 and with a retention index of 0.6605 (Figure 5). All lengths reported here were computed in Mesquite 3.1, by distinguishing between partial uncertainty and polymorphism; under the default settings PAUP 4 considers all these as uncertainty, though settings can be changed to interpret these data as in Mesquite. This introduces only a difference in tree lengths between both programs, though the shortest trees in one program remain the shortest ones in the other. In these trees, mesosaurs appear as the sister-group of all other sauropsids, as they were in Laurin and Reisz (1995). However, sauropsid phylogeny differs strongly from the topology recovered by Laurin and Reisz (1995) and most recent analyses because parareptiles appear to be nested within diapsids, as the sister-group of younginiforms (more crownward diapsids are not included in our taxonomic sample). Not surprisingly, this result is not very robust; the smallest clade that includes younginiforms and parareptiles has a Bremer index of 3 and a bootstrap frequency of only 39%. This low bootstrap frequency suggests considerable character conflict. Pareiasaurs appear to be the sister-group of turtles, as previously suggested by Lee (1993, 1996), but procolophonoids appear to be paraphyletic, given that Procolophon is closer to that clade, in the most parsimonious trees, than to O. kitchingorum. In addition, the romeriid Paleothyris appears nested within diapsids, another counter-intuitive result, though this one is the least robust clade (Bremer index of 1; bootstrap frequency of 20%).
Figure 5. Phylogenetic position of mesosaurs among early amniotes and selected related taxa. This cladogram results from a parsimony analysis of a matrix updated from that of Laurin and Reisz (1995) with our observations of mesosaur specimens (mostly from material collected in Uruguay, but also, to a lesser extent, material observed in Brazil, Germany, and France) and with recent literature. Characters that form a cline were ordered; the branch and bound algorithm of PAUP 4 was used. This is a strict consensus of two trees that require 383 steps (in Mesquite). They have a CI (Consistency Index) of 0.56527 and a RI (Retention Index) of 0.65741 (see text for details). Robustness of the results is shown through the bootstrap (based on 200 branch and bound replicates) and Bremer Index (before and after slash, respectively). Note that diapsids do not form a clade under this topology, but to make this more obvious, the smallest clade that includes both diapsid taxa is labeled as “Diapsids”.
The clade that includes all sauropsids except for mesosaurs has a bootstrap frequency of 66%, which is relatively low, but moving mesosaurs within the clade that includes other reptiles, such as placing them at the base of parareptiles, as previously suggested by Gauthier et al. (1988, Figure 4.4) and Modesto (1999, Figure 4A), requires four extra steps. Moving mesosaurs to other phylogenetic positions requires three additional (386) steps. Among the 48 trees of that length, mesosaurs occur in various positions, but always outside the smallest clade that includes all other sauropsids. In four of these trees, mesosaurs are the sister-group of a clade that includes amniotes and diadectomorphs (in which diadectomorphs appear at the base of Synapsida); in three of these trees, mesosaurs are the sister-group of amniotes. In the 41 other trees of that length, mesosaurs appear in their most parsimonious position, as the sister-group of all other sauropsids. The most frequent clade that includes mesosaurs and a subset of the other sauropsids (in this case, all others except for Acleistorhinus) has a low bootstrap frequency (12%).
The characters discussed below were presented in detail by Laurin and Reisz (1995), with very few exceptions. Thus, except for characters not taken from that paper, the discussion of the nature of these characters is kept short, and the emphasis is on their revised taxonomic distribution.
The sauropsid status of mesosaurs is supported by the following four unambiguous synapomorphies, given our data and the shortest trees (the numbers in parentheses following a character number designate the character state):
Character 35(1). Quadrate anterior process short. This process is long in Seymouria, limnoscelids, and Synapsida (ancestrally). In these taxa, this process overlaps at least half of the length of the quadrate ramus of the pterygoid. The derived condition (short process overlapping less than half of quadrate ramus of pterygoid) occurs in mesosaurs (Modesto, 2006), captorhinids, Paleothyris, araeoscelidians, and younginiforms, but parareptiles revert to having a long anterior process.
Character 62(1). Posttemporal fenestra large. In Seymouria, diadectids, and synapsids ancestrally, the posttemporal fenestra is small; it looks almost like a large foramen. Mesosaurs (Modesto, 2006, p. 347) and most other sauropsids, except Acleistorhinus, have a larger posttemporal fenestra. The fenestra was apparently convergently enlarged in limnoscelids.
Character 105(1). Supinator process parallel to shaft. The supinator process was ancestrally sharply angled to the shaft, as seen in Seymouria, diadectomorphs, and early synapsids. All early sauropsids in which this character is documented have a supinator process which is oriented at a much lower angle to the shaft.
Character 123(1). Presence of a single pedal centrale in the adult. Ancestrally in cotylosaurs, two pedal centralia were present, as seen in diadectomorphs and most Permo-Carboniferous synapsids. In synapsids, the situation is somewhat uncertain. Most eupelycosaurs have two centralia, but in Caseasauria, there is no evidence of two centralia; Casea is usually shown with two, but only one is actually preserved (Romer and Price, 1940: Figure 41H), and in Cotylorhynchus only one is preserved, though Stovall et al. (1966, p. 24) indicate that the presence of a second centrale is uncertain. Polymorphism could have been scored for this taxon, but given how poorly known this character is in Caseasauria, we have provisionally kept a scoring that represents the prevailing condition in Eupelycosauria, where it is much better documented (Romer and Price, 1940: Figure 41). Sauropsids have a single pedal centrale in the adult and there is no strong evidence of a second centrale in juveniles, though the ontogeny of most Paleozoic sauropsids (except mesosaurs) is too poorly known to be sure that a second centrale was absent in early ontogeny (state 2). The well-documented ontogeny of mesosaurs shows that in this taxon, fusion occurred fairly late in the ontogeny (state 1; Piñeiro et al., 2016). In this respect, mesosaurs may display an intermediate condition. This character is ordered because it appears to form a cline.
The position of mesosaurs outside the clade that includes all other sauropsids is supported by:
Character 39(2). Intertemporal vacuity long, at least 15% of the skull length. This character is reversed in Procolophon, pareiasaurs, and Odontochelys, which have a shorter interpterygoid vacuity.
Character 49(1). Suborbital foramen present. This is transformed into a fenestra (2) in araeoscelidians, Youngina, Proganochelys, and some crown-turtles. Acleistorhinus lost the foramen (0).
Character 51(1). Absence of parasphenoid wings.
Character 54(1). Presence of supraoccipital anterior crista.
Character 55(2). Supraoccipital plate narrow. The supraoccipital becomes even narrower (3) in Procolophon, pareiasaurs, and turtles, whereas it becomes broader (1) in Acleistorhinus.
The surprising inclusion of parareptiles in the smallest clade that comprises also Araeoscelidians, Youngina and Paleothyris is supported by:
Character 16(2). A reduction in size of the tabular, which is further reduced in the clade that includes Owenetta, Procolophon, pareiasaurs, and turtles. This character is reversed in pareiasaurs, which re-acquire a larger tabular (state 1).
Character 57(1). Paroccipital process contacts tabular distally. This character may characterize a more inclusive clade because it is inapplicable in captorhinids, which lack a tabular, and mesosaurs, in which the situation is uncertain given contradictory information provided by various specimens.
Character 119(1). Carpus and tarsus long and slender (longer globally than wide). This is a weak synapomorphy because among parareptiles, it is documented only in millerettids. This character is reversed in the smallest clade that includes Procolophon, pareiasaurs, and turtles, and it not documented in Acleistorhinus and Owenetta.
Character 126(1). Metapodials overlapping. This is another moderately convincing synapomorphy because among parareptiles, it is documented in Procolophon and in some millerettids (Thommasen and Carroll, 1981). It is also present in turtles, but it is absent in pareiasaurs, and undocumented in Acleistorhinus and Owenetta.
The position of Paleothyris as sister-group of the smallest clade that includes Youngina, parareptiles, and turtles is supported by:
Character 89(1). Posterior trunk (lumbar) neural arches narrow. This is reversed (to swollen; 0) in Owenetta, Procolophon, and pareiasaurs.
Character 90(1). Posterior trunk (lumbar) zygapophyseal buttresses narrow. This refers to the antero-posterior width of the buttresses, not the width of the neural arches, which is typically assessed in anterior or posterior view. This character does not have the same distribution as the previous one as there is no evidence of reversal in the clade.
The equally surprising position of parareptiles as the sister-group of Youngina is weakly supported with a bootstrap frequency of 47% and a Bremer (decay) index of 3. While we view this result with some suspicion and consider it provisory, we provide a list of synapomorphies supporting it. To mention only the characters that unambiguously support this topology, this includes:
Character 9(1). The lacrimal is excluded from the naris and septomaxilla; this is reversed in millerettids and pareiasaurs.
Character 18(1). A high anterodorsal process of the maxilla that reaches the nasal. This is reversed in millerettids (state 0, anterodorsal process absent) and pareiasaurs (state 1, low, anterodorsal process does not reach nasal). Note that there is a strong logical link between both characters (9 and 18), which were both in the matrix of Laurin and Reisz (1995; characters 9 and 18); to solve this problem, we have downweighted both characters to 0.5.
Character 24(1). Caniniform tooth (2) replaced by caniniform region (1). The trend toward less differentiation in tooth size (0) continues within parareptiles, as some millerettids (0 and 1) and all procolophonids and pareiasaurs lack a caniniform region or tooth (0). Mesosaurs are convergent in having a homodont dentition (0), under this topology.
Character 48(1). Absence of ectopterygoid teeth.
Character 70(1). Stapedial dorsal process unossified or absent.
Character 94(1). Transverse processes present on at least 12 caudal vertebrae. This character is undocumented in several parareptiles (Acleistorhinus, millerettids, and Owenetta) and in Odontochelys, so this synapomorphy is only moderately well-established.
Character 101(1). Supraglenoid foramen absent.
Character 110(1). Olecranon process small, with small articular facet facing proximally. This synapomorphy is only moderately satisfactory because it could not be scored for Acleistorhinus, millerettids, and Owenetta, so the condition at the base of parareptiles is poorly documented.
Character 113(1). Iliac blade dorsally expanded and distally flared.
Character 114(1). Large acetabular buttress, overhanging strongly. This synapomorphy is poorly documented because it could not be scored for Acleistorhinus, millerettids, Owenetta, and Odontochelys.
When Mesosauridae is deleted from the matrix, we recover a more conventional phylogeny, in which parareptiles form the sister-group of eureptiles, and in which Paleothyris is excluded from diapsids. The only unorthodox result with this taxonomic sampling is that procolophonoids remain paraphyletic with respect to pareiasaurs and turtles.
Deleting Chelonii from the matrix does not alter the topology of the shortest tree, except that there is no longer a basal trichotomy of turtles. The robustness of the position of mesosaurs outside the smallest clade that includes all other sauropsids is strong, with a Bremer (decay) index of 4, and a bootstrap frequency of 56%. In the bootstrap tree (though not in the most parsimonious tree), Paleothyris is outside Diapsida. However, with that taxonomic sample, the most robust clade (with a Bremer index of 7 and a bootstrap frequency of 97%) includes Pareiasauria, Owenetta, Procolophon, and stem-turtles. This remains one of the most robust clade, with a Bremer index of 6 and a bootstrap frequency of 97%, when Proganochelys is removed (in addition to Chelonii), whereas the position of mesosaurs outside the clade that includes the other sauropsids remains fairly robust, with a Bremer index of 5 and a bootstrap frequency of 68%. Further removing Odontochelys, results in two trees (length of 291 steps in PAUP). Their strict consensus is compatible with the results from the complete taxonomic sample, but much less resolved. The four eureptile taxa and Parareptilia form a large polytomy (Parereptilia remains monophyletic), and two trichotomies are present within Parareptilia (one with Acleistorhinus, Millerettidae, and a clade including pareiasaurs plus both procolophonoids, and a second polytomy including Owenetta, Procolophon, and pareiasaurs).
The position of mesosaurs outside the clade that includes all other sauropsids as suggested by Laurin and Reisz (1995) appears to be a reasonably robust result, though various parareptile clades are more robust. The relatively low bootstrap frequency (58% for Sauropsida; 66% for the largest sauropsid clade that excludes mesosaurs) is not overly convincing, but three additional steps are required to place mesosaurs elsewhere in the tree, and in these alternative trees, mesosaurs fall outside Sauropsida; the position of sister-group of other parareptiles, previously suggested by Gauthier et al. (1988) and Modesto (1999), or other positions within parareptiles imply at least four extra steps and these alternative positions have bootstrap frequencies of 12% or less. Given the mix of primitive and derived features of the mesosaur skeleton, the obtained results are not unexpected. Thus, several characters present in mesosaurs are shared with those present in basalmost amniotes or close relatives of amniotes (see above). This placement of mesosaurs in amniote phylogeny is slightly more robust than in Laurin and Reisz (1995), in which the clade that included all sauropsids except mesosaurs had a Bremer index of only one, but a similar bootstrap frequency of 67%. This moderate robustness improvement (at least as assessed by the Bremer index) benefits from several new anatomical studies on mesosaurs. However, the previous suggestions that mesosaurs are basal parareptiles (Gauthier et al., 1988; Modesto, 1999) are not surprising because mesosaurs share some features with procolophonoids, such as the presence of swollen neural arches and the postorbital not reaching the supratemporal. The relatively low bootstrap frequency (66%) presumably reflects a fair amount of character conflict.
The position of parareptiles in our tree, though unorthodox, offers a possible resolution between two hypotheses about the position of turtles that were previously considered mutually incompatible, namely among parareptiles, as suggested by some paleontological studies (Reisz and Laurin, 1991; Lee, 1993, 1996; Laurin and Reisz, 1995), or among diapsids as suggested by most recent molecular (Hugall et al., 2007; Chiari et al., 2012) and some paleontological phylogenies (Rieppel and deBraga, 1996; deBraga and Rieppel, 1997; Piñeiro, 2004). The possibility that parareptiles are actually diapsids that lost one or both of the fenestrae (the upper fenestra having never been found in the group), which is raised by our results, offers a way out of this controversy, given that turtles can be both parareptiles and diapsids. Under that hypothesis, the upper temporal fenestra closure described by Bever et al. (2015) in the ontogeny of Eunotosaurus might actually characterize parareptiles as a whole. In Eunotosaurus, this closure is achieved by anterior expansion of the supratemporal. The supratemporal is fairly large in most parareptiles (deBraga and Reisz, 1996; Lee, 1997; Reisz and Scott, 2002; Tsuji et al., 2012), so it is possible that they share this mechanism of upper temporal fenestra closure with Eunotosaurus. This possibility could be checked through CT-scanning or mechanical preparation of the internal surface of the skull roof. However, the morphology of the basalmost parareptiles (assuming recent phylogenies are correct) is not consistent with this scenario. Thus, millerettids and the even older and more basal Microleter and Australothyris are among the parareptiles with the smallest supratemporal (Gow, 1972; Tsuji et al., 2010). Clearly, this intriguing by-product of our study on mesosaur affinities will need to be evaluated both with an expanded taxon and character sample and with new anatomical studies of the temporal area of most parareptiles. This is not done here because the purpose of our study was to assess the position of mesosaurs in amniote phylogeny. Assessing the position of parareptiles as a whole, and the controversial issue of turtle origins are much more ambitious goals that our study was not designed to assess.
The position of parareptiles within diapsids obtained here should be tested further because our taxonomic sample of diapsids is sparse, with only two stem-diapsid taxa (araeoscelidians and younginiforms) represented. The fact that this topology is not recovered (with parareptiles forming the sister-group of eureptiles) when Mesosauridae is deleted from the matrix further reinforces this note of caution. When mesosaurs are excluded from the analysis, eureptiles are united by six synapomorphies (character number in parentheses): postorbital/supratemporal contact absent (12); posterolateral corner of skull table formed by parietal and small supratemporal (15); supratemporal small (17); squamosal and posttemporal fenestra in contact (25); quadrate anterior process short (35); and arcuate flange of pterygoid absent (42). The fact that mesosaurs share half of these characters (12, 35, and 42) weakens support for Eureptilia. This topology is new to our knowledge, although Lee (2013) had found, in some of his 12 analyses (eight of a diapsid-focused dataset, and four of a parareptile-focused dataset), that the parareptile Eunotosaurus, which has been claimed to be a close relative of turtles (Lyson et al., 2010; Bever et al., 2015), fits within diapsids. However, our results differ (with the complete taxonomic sample) in placing all parareptiles within diapsids. Still, this new hypothesis is supported by some frequently-discussed characters, such as a temporal emargination, which is present in most parareptiles (Müller and Tsuji, 2007) as well as in crown-diapsids (Laurin, 1991) and turtles. This part of the results is the most surprising though it is possible that morphological support for the taxon Eureptilia (excluding parareptiles) is weaker than commonly realized. For instance, in the analysis of Tsuji et al. (2010), bootstrap frequency for that clade is only 58% (one of the lowest of the tree), and Bayesian posterior probability is 86% (higher, but among the most weakly-supported half of the clades of their tree). Similarly, in the analysis of Laurin and Reisz (1995), Eureptilia had a bootstrap frequency of only 69%, which placed it among the most weakly-supported clades.
Strangely, Procolophonoidea is found here to be paraphyletic with respect to pareiasaurs and turtles, despite the fact that we included one of its most obvious synapomorphies, namely the posterior extension of the orbit (character 37), which Owenetta and Procolophon are the only taxa to display in our matrix. This result is moderately robust (bootstrap frequency of 64% and Bremer index of 5) and it persists with the deletion of Mesosauridae from the analysis. However, we have added Owenetta without adding synapomorphies of Procolophonoidea, so this result may be artifactual.
The position of Paleothyris within diapsids is equally surprising, but this result is not robust, and it disappears when mesosaurs are removed from the analysis. The bootstrap frequency of this clade (in the analysis with all taxa) is barely 20%, and its Bremer (decay) index is only 1, which means that only one more character supports this position for Paleothyris than the next most supported position. This topology reflects partly the fact that the position of mesosaurs at the base of Sauropsida makes the presence of the lower temporal fenestra an amniote synapomorphy (reversed in Captorhinidae, Paleothyris, and most parareptiles and turtles); removing mesosaurs changes the history of this character at the base of amniotes, which in turn supports diapsid monophyly. Furthermore, various studies have de-emphasized the importance of fenestration as a systematic character (Fucik, 1991; Hamley and Thulborn, 1993; Müller, 2003; Cisneros et al., 2004; Modesto et al., 2009; Tsuji et al., 2010; Bever et al., 2015), and temporal morphology may be influenced by several factors (Werneburg, 2012), which complicates interpretation of observed morphology. Thus, the question of diapsid monophyly (aside from the inclusion of turtles) might be worth investigating further.
To sum up, our study suggests that mesosaurs are the basalmost sauropsids; this result appears to be fairly well-supported, at least with our dataset. Moreover, our results raise several problems about the phylogeny of early amniotes, some of which might be worth reinvestigating with an increased sample of taxa and characters, and a fresh look at various specimens. In addition to suggesting yet another hypothesis about the origin of turtles, our results highlight the importance of including mesosaurs in phylogenetic analyses of amniotes, because they can potentially change the topology near the base of Amniota and weaken support for Eureptilia and Diapsida. Including mesosaurs in such analyses is not the established practice (e.g., Reisz et al., 2011; Lyson et al., 2013).
All authors listed have made a substantial, direct and intellectual contribution to the work, and approved it for publication.
This research was funded by the CNRS (recurring grant to the CR2P and PICS project Mesosaur biology and its implications for the evolution of the first amniotes, project number 6326), the French Ministry of Research, the MNHN (ATM Émergences “Mésosaures: émergence des sauropsides, de la viviparité, et premiers retours à l'environnement aquatique”), and the National Geographic Society (grant number 9497-14, “The biology of the earliest known aquatic reptiles”).
The authors declare that the research was conducted in the absence of any commercial or financial relationships that could be construed as a potential conflict of interest.
We wish to thank Michael S. Y. Lee and Juliana Sterli for their very constructive reviews.
The Supplementary Material for this article can be found online at: https://www.frontiersin.org/articles/10.3389/feart.2017.00088/full#supplementary-material
HAL open archive (https://hal.archives-ouvertes.fr/) through this link: https://hal.archives-ouvertes.fr/hal-01618314.
Bakker, R. T. (1975). Dinosaur renaissance. Sci. Am. 232, 58–78. doi: 10.1038/scientificamerican0475-58
Bever, G., Lyson, T. R., Field, D. J., and Bhullar, B.-A. S. (2015). Evolutionary origin of the turtle skull. Nature 525, 239–242. doi: 10.1038/nature14900
Bremer, K. (1988). The limits of amino acid sequence data in angiosperm phylogenetic reconstruction. Evolution 42, 795–803. doi: 10.1111/j.1558-5646.1988.tb02497.x
Brongniart, A. (1800). Essai d'une classification naturelle des Reptiles. 1ère partie. Établissement des ordres. Bull. Sci. Soc. Philom. 2, 81–82.
Canoville, A., and Laurin, M. (2010). Evolution of humeral microanatomy and lifestyle in amniotes, and some comments on paleobiological inferences. Biol. J. Linn. Soc. 100, 384–406. doi: 10.1111/j.1095-8312.2010.01431.x
Chiari, Y., Cahais, V., Galtier, N., and Delsuc, F. (2012). Phylogenomic analyses support the position of turtles as the sister group of birds and crocodiles (Archosauria). BMC Biol. 10:65. doi: 10.1186/1741-7007-10-65
Cisneros, J. C., Damiani, R., Schultz, C., da Rosa, Á., Schwanke, C., Neto, L. W., et al. (2004). A procolophonoid reptile with temporal fenestration from the Middle Triassic of Brazil. Proc. R. Soc. Lond. B 271, 1541–1546. doi: 10.1098/rspb.2004.2748
Clark, J., and Carroll, R. L. (1973). Romeriid reptiles from the Lower Permian. Bull. Mus. Comp. Zool. Harv. 144, 353–407.
Datta, P. M., Manna, P., Ghosh, S. C., and Das, D. P. (2000). The first Jurassic turtle from India. Palaeontology 43, 99–109. doi: 10.1111/1475-4983.00120
deBraga, M., and Reisz, R. R. (1996). The Early Permian reptile Acleistorhinus pteroticus and its phylogenetic position. J. Vertebr. Paleontol. 16, 384–395. doi: 10.1080/02724634.1996.10011328
deBraga, M., and Rieppel, O. (1997). Reptile phylogeny and the interrelationships of turtles. Zool. J. Linn. Soc. 120, 281–354. doi: 10.1111/j.1096-3642.1997.tb01280.x
Didier, G., Fau, M., and Laurin, M. (2017). Likelihood of tree topologies with fossils and diversification rate estimation. Sys. Biol. 66, 964–987. doi: 10.1093/sysbio/syx045
Dubois, A., and Bour, R. (2010). The distinction between family-series and class-series nomina in zoological nomenclature, with emphasis on the nomina created by Batsch (1788, 1789) and on the higher nomenclature of turtles. Bonn Zool. Bull. 57, 149–171.
Felice, R. N., and Angielczyk, K. D. (2014). “Was Ophiacodon (Synapsida, Eupelycosauria) a swimmer? A test using vertebral dimensions,” in Early Evolutionary History of the Synapsida, eds F. Christian Kammerer and D. Kenneth Angielczyk and Jörg Fröbisch (Dordrecht: Springer), 25–51.
Felsenstein, J. (1985). Confidence limits on phylogenies: an approach using the bootstrap. Evolution 39, 783–791. doi: 10.1111/j.1558-5646.1985.tb00420.x
Foote, M., and Sepkoski, J. J. Jr. (1999). Absolute measures of the completeness of the fossil record. Nature 398, 415–417. doi: 10.1038/18872
Fucik, E. (1991). On the value of the orbitotemporal region for the reconstruction of reptilian phylogeny: ontogeny and adult skull analyses of the chelonian skull. Zool. Anzeiger 227, 209–217.
Gaffney, E. S. (1979). Comparative cranial morphology of recent and fossil turtles. Bull. Am. Mus. Nat. Hist. 164, 65–376.
Gaffney, E. S., and Kitching, J. W. (1995). The morphology and relationships of Australochelys, an Early Jurassic turtle from South Africa. Am. Mus. Novit. 3130, 1–29.
Gaffney, E. S., Tong, H., and Meylan, P. A. (2006). Evolution of the side-necked turtles: the families Bothremydidae, Euraxemydidae, and Araripemydidae. Bull. Am. Mus. Nat. Hist. 300, 1–698. doi: 10.1206/0003-0090(2006)300[1:EOTSTT]2.0.CO;2
Gauthier, J., Kluge, A. G., and Rowe, T. (1988). “The early evolution of the Amniota,” in The Phylogeny and Classification of the Tetrapods, Vol. 1: Amphibians, Reptiles, Birds, ed M. J. Benton (Oxford: Clarendon Press), 103–155.
Gow, C. E. (1972). The osteology and relationships of the Millerettidae (Reptilia: Cotylosauria). J. Zool. 167, 219–264. doi: 10.1111/j.1469-7998.1972.tb01731.x
Grand, A., Corvez, A., Duque Velez, L. M., and Laurin, M. (2013). Phylogenetic inference using discrete characters: performance of ordered and unordered parsimony and of three-item statements. Biol. J. Linn. Soc. 110, 914–930. doi: 10.1111/bij.12159
Hamley, T., and Thulborn, T. (1993). “Temporal fenestration in the primitive Triassic reptile Procolophon,” in The nonmarine Triassic, eds S. G. Lucas and M. Morales (Albuquerque: New Mexico Museum of Natural History), 171–174.
Hauser, D. L., and Presch, W. (1991). The effect of ordered characters on phylogenetic reconstruction. Cladistics 7, 243–265. doi: 10.1111/j.1096-0031.1991.tb00037.x
Hendy, M. D., and Penny, D. (1982). Branch and bound algorithms to determine minimal evolutionary trees. Math. Biosci. 59, 277–290. doi: 10.1016/0025-5564(82)90027-X
Huene, H. F. von. (1941). Osteologie und systematische Stellung von Mesosaurus. Palaeontogr. Abt. A 92, 45–58.
Hugall, A. F., Foster, R., and Lee, M. S. Y. (2007). Calibration choice, rate smoothing, and the pattern of tetrapod diversification according to the long nuclear gene RAG-1. Syst. Biol. 56, 543–563. doi: 10.1080/10635150701477825
Joyce, W. G., Parham, J. F., and Gauthier, J. A. (2004). Developing a protocol for the conversion of rank-based taxon names to phylogenetically defined clade name, as exemplified by turtles. J. Paleont. 78, 989–1013. doi: 10.1666/0022-3360(2004)078<0989:DAPFTC>2.0.CO;2
Kimmel, C. B., Sidlauskas, B., and Clack, J. A. (2009). Linked morphological changes during palate evolution in early tetrapods. J. Anat. 215, 91–109. doi: 10.1111/j.1469-7580.2009.01108.x
Latreille, P. A. (1800). Histoire Naturelle des Salamandres de France, Précédée d'un Tableau Méthodique des Autres Reptiles Indigènes. Paris: Imprimerie de Crapelet.
Laurin, M. (1991). The osteology of a Lower Permian eosuchian from Texas and a review of diapsid phylogeny. Zool. J. Linn. Soc. 101, 59–95. doi: 10.1111/j.1096-3642.1991.tb00886.x
Laurin, M., and de Buffrénil, V. (2016). Microstructural features of the femur in early ophiacodontids: a reappraisal of ancestral habitat use and lifestyle of amniotes. C. R. Palevol. 15, 115–127. doi: 10.1016/j.crpv.2015.01.001
Laurin, M., and Reisz, R. R. (1995). A reevaluation of early amniote phylogeny. Zool. J. Linn. Soc. 113, 165–223. doi: 10.1111/j.1096-3642.1995.tb00932.x
Lee, M. S. Y. (1993). The origin of the turtle body plan: bridging a famous morphological gap. Science 261, 1716–1720. doi: 10.1126/science.261.5129.1716
Lee, M. S. Y. (1996). Correlated progression and the origin of turtles. Nature 379, 812–815. doi: 10.1038/379812a0
Lee, M. S. Y. (1997). Pareiasaur phylogeny and the origin of turtles. Zool. J. Linn. Soc. 120, 197–280. doi: 10.1111/j.1096-3642.1997.tb01279.x
Lee, M. S. Y. (2013). Turtle origins: insights from phylogenetic retrofitting and molecular scaffolds. J. Evol. Biol. 26, 2729–2738. doi: 10.1111/jeb.12268
Li, C., Wu, X.-C., Rieppel, O., Wang, L.-T., and Zhao, L.-J. (2008). An ancestral turtle from the Late Triassic of southwestern China. Nature 456, 497–501. doi: 10.1038/nature07533
Lu, B., Yang, W., Dai, Q., and Fu, J. (2013). Using genes as characters and a parsimony analysis to explore the phylogenetic position of turtles. PLoS ONE 8:e79348. doi: 10.1371/journal.pone.0079348
Lyson, T. R., Bever, G. S., Bhullar, B.-A. S., Joyce, W. G., and Gauthier, J. A. (2010). Transitional fossils and the origin of turtles. Biol. Lett. 6, 830–833. doi: 10.1098/rsbl.2010.0371
Lyson, T. R., Bever, G. S., Scheyer, T. M., Hsiang, A. Y., and Gauthier, J. A. (2013). Evolutionary origin of the turtle shell. Curr. Biol. 23, 1113–1119. doi: 10.1016/j.cub.2013.05.003
Mabee, P. M. (1989). Assumptions underlying the use of ontogenetic sequences for determining character state order. Trans. Am. Fish. Soc. 118, 151–158.
Maddison, W. P., and Maddison, D. R. (1992). MacClade: Analysis of Phylogeny and Character Evolution. Version 3.0. Sunderland, MA: Sinauer Associates.
Mickevich, M. F., and Lipscomb, D. (1991). Parsimony and the choice between different transformations for the same character set. Cladistics 7, 111–139. doi: 10.1111/j.1096-0031.1991.tb00028.x
Modesto, S. P. (1999). Observations on the structure of the Early Permian reptile Stereosternum temidum Cope. Palaeont. Afr. 35, 7–19.
Modesto, S. P. (2006). The cranial skeleton of the Early Permian aquatic reptile Mesosaurus tenuidens: implications for relationships and palaeobiology. Zool. J. Linn. Soc. 146, 345–368. doi: 10.1111/j.1096-3642.2006.00205.x
Modesto, S. P. (2010). The postcranial skeleton of the aquatic parareptile Mesosaurus tenuidens from the Gondwanan Permian. J. Vertebr. Paleontol. 30, 1378–1395. doi: 10.1080/02724634.2010.501443
Modesto, S. P., Scott, D. M., and Reisz, R. R. (2009). A new parareptile with temporal fenestration from the Middle Permian of South Africa. Can. J. Earth Sci. 46, 9–20. doi: 10.1139/E09-001
Mora, C., Tittensor, D. P., Adl, S., Simpson, A. G. B., and Worm, B. (2011). How many species are there on Earth and in the ocean? PLoS Biol. 9:e1001127. doi: 10.1371/journal.pbio.1001127
Motani, R., Jiang, D.-Y., Tintori, A., Rieppel, O., and Chen, G.-B. (2014). Terrestrial origin of viviparity in Mesozoic marine reptiles indicated by Early Triassic embryonic fossils. PLoS ONE 9:e88640. doi: 10.1371/journal.pone.0088640
Müller, H., Oommen, O. V., and Bartsch, P. (2005). Skeletal development of the direct-developing caecilian Gegeneophis ramaswamii (Amphibia: Gymnophiona: Caeciliidae). Zoomorphology 124, 171–188. doi: 10.1007/s00435-005-0005-6
Müller, J. (2003). Early loss and multiple return of the lower temporal arcade in diapsid reptiles. Naturwissenschaften 90, 473–476. doi: 10.1007/s00114-003-0461-0
Müller, J., and Tsuji, L. A. (2007). Impedance-matching hearing in Paleozoic reptiles: evidence of advanced sensory perception at an early stage of amniote evolution. PLoS ONE 2:e889. doi: 10.1371/journal.pone.0000889
Piñeiro, G. (2004). Paleofaunas del Pérmico y Permo-Triásico de Uruguay. Bioestratigrafía, Paleobiogeografía y Sistemática. Montevideo: Universidad de la República.
Piñeiro, G. (2008). “Los mesosaurios y otros fósiles de fines del Paleozoico,” in Fósiles de Uruguay, ed D. Perea (Montevideo: DIRAC, Facultad de Ciencias), 179–205.
Piñeiro, G., Ferigolo, J., Meneghel, M., and Laurin, M. (2012a). The oldest known amniotic embryos suggest viviparity in mesosaurs. Hist. Biol. 24, 620–630. doi: 10.1080/08912963.2012.662230
Piñeiro, G., Ferigolo, J., Ramos, A., and Laurin, M. (2012b). Cranial morphology of the Early Permian mesosaurid Mesosaurus tenuidens and the evolution of the lower temporal fenestration reassessed. C. R. Palevol. 11, 379–391. doi: 10.1016/j.crpv.2012.02.001
Piñeiro, G., Ramos, A., Goso, C., Scarabino, F., and Laurin, M. (2012c). Unusual environmental conditions preserve a Permian mesosaur-bearing Konservat-Lagerstätte from Uruguay. Acta Palaeont. Pol. 57, 299–318. doi: 10.4202/app.2010.0113
Piñeiro, G., Demarco, P. N., and Meneghel, M. D. (2016). The ontogenetic transformation of the mesosaurid tarsus: a contribution to the origin of the primitive amniotic astragalus. PeerJ 4:e2036. doi: 10.7717/peerj.2036
Reisz, R. R., and Laurin, M. (1991). Owenetta and the origin of turtles. Nature 349, 324–326. doi: 10.1038/349324a0
Reisz, R. R., and Scott, D. (2002). Owenetta kitchingorum, sp. nov., a small parareptile (Procolophonia: Owenetidae) from the Lower Triassic of South Africa. J. Vertebr. Paleontol. 22, 244–256. doi: 10.1671/0272-4634(2002)022[0244:OKSNAS]2.0.CO;2
Reisz, R. R., Modesto, S. P., and Scott, D. M. (2011). A new Early Permian reptile and its significance in early diapsid evolution. Proc. R. Soc. Lond. B 278, 3731–3737. doi: 10.1098/rspb.2011.0439
Rieppel, O., and deBraga, M. (1996). Turtles as diapsid reptiles. Nature 384, 453–455. doi: 10.1038/384453a0
Rineau, V., Grand, A., Zaragüeta, R., and Laurin, M. (2015). Experimental systematics: sensitivity of cladistic methods to polarization and character ordering schemes. Contrib. Zool. 84, 129–148.
Romer, A. S. (1958). Tetrapod limbs and early tetrapod life. Evolution 12, 365–369. doi: 10.1111/j.1558-5646.1958.tb02966.x
Schoch, R. R., and Sues, H.-D. (2015). A Middle Triassic stem-turtle and the evolution of the turtle body plan. Nature 523, 584–587. doi: 10.1038/nature14472
Schoch, R. R., and Sues, H.-D. (2017). Osteology of the Middle Triassic stem-turtle Pappochelys rosinae and the early evolution of the turtle skeleton. J. Syst. Palaeontol. doi: 10.1080/14772019.2017.1354936. [Epub ahead of print].
Silva, R. R., Ferigolo, J., Bajdek, P., and Piñeiro, G. H. (2017). The feeding habits of Mesosauridae. Front. Earth Sci. 5:23. doi: 10.3389/feart.2017.00023
Slowinski, J. B. (1993). “Unordered” versus “ordered” characters. Syst. Biol. 42, 155–165. doi: 10.1093/sysbio/42.2.155
Sterli, J. (2008). A new, nearly complete stem turtle from the Jurassic of South America with implications for turtle evolution. Biol. Lett. 4, 286–289. doi: 10.1098/rsbl.2008.0022
Sterli, J., and Joyce, W. G. (2007). The cranial anatomy of the Early Jurassic turtle Kayentachelys aprix. Acta Palaeont. Pol. 52, 675–694.
Sterli, J., Rafael, S., De La Fuente, M., and Rougier, G. W. (2007). Anatomy and relationships of Palaeochersis talampayensis, a Late Triassic turtle from Argentina. Palaeontogr. Abt. A 281, 1–61. doi: 10.1127/pala/281/2007/1
Stovall, J. W., Price, L. I., and Romer, A. S. (1966). The postcranial skeleton of the giant Permian pelycosaur Cotylorhynchus romeri. Bull. Mus. Comp. Zool. Harv. 135, 1–30.
Swofford, D. L. (2003). PAUP* Phylogenetic Analysis Using Parsimony (*and other methods). Version 4.0b10. Sinauer Associates.
Thommasen, H., and Carroll, R. L. (1981). Broomia, the oldest known millerettid reptile. Palaeontology 24, 379–390.
Tsuji, L. A., Müller, J., and Reisz, R. R. (2010). Microleter mckinzieorum gen. et sp. nov. from the Lower Permian of Oklahoma: the basalmost parareptile from Laurasia. J. Syst. Palaeontol. 8, 245–255. doi: 10.1080/14772010903461099
Tsuji, L. A., Müller, J., and Reisz, R. R. (2012). Anatomy of Emeroleter levis and the phylogeny of the nycteroleter parareptiles. J. Vertebr. Paleontol. 32, 45–67. doi: 10.1080/02724634.2012.626004
Villamil, J. N., Demarco, P. N., Meneghel, M., Blanco, R. E., Jones, W., Rinderknecht, A., et al. (2016). Optimal swimming speed estimates in the Early Permian mesosaurid Mesosaurus tenuidens (Gervais 1867) from Uruguay. Hist. Biol. 28, 963–971. doi: 10.1080/08912963.2015.1075018
Keywords: Mesosauridae, Sauropsida, Reptilia, Amniota, Permian
Citation: Laurin M and Piñeiro GH (2017) A Reassessment of the Taxonomic Position of Mesosaurs, and a Surprising Phylogeny of Early Amniotes. Front. Earth Sci. 5:88. doi: 10.3389/feart.2017.00088
Received: 12 August 2017; Accepted: 12 October 2017;
Published: 02 November 2017.
Edited by:
Holly Woodward, Oklahoma State University, United StatesReviewed by:
Michael S. Lee, South Australian Museum, AustraliaCopyright © 2017 Laurin and Piñeiro. This is an open-access article distributed under the terms of the Creative Commons Attribution License (CC BY). The use, distribution or reproduction in other forums is permitted, provided the original author(s) or licensor are credited and that the original publication in this journal is cited, in accordance with accepted academic practice. No use, distribution or reproduction is permitted which does not comply with these terms.
*Correspondence: Michel Laurin, bWljaGVsLmxhdXJpbkBtbmhuLmZy
Disclaimer: All claims expressed in this article are solely those of the authors and do not necessarily represent those of their affiliated organizations, or those of the publisher, the editors and the reviewers. Any product that may be evaluated in this article or claim that may be made by its manufacturer is not guaranteed or endorsed by the publisher.
Research integrity at Frontiers
Learn more about the work of our research integrity team to safeguard the quality of each article we publish.