- 1Ecology and Biodiversity, Department Biology, Vrije Universiteit Brussel, Brussels, Belgium
- 2Department of Aquatic Ecology, Netherlands Institute of Ecology (NIOO-KNAW), Wageningen, Netherlands
- 3Department of Terrestrial Ecology, Netherlands Institute of Ecology (NIOO-KNAW), Wageningen, Netherlands
- 4Faculty of Science and Engineering, Groningen Institute for Evolutionary Life Sciences, University of Groningen, Groningen, Netherlands
- 5Department of Biology, Miami University, Oxford, OH, USA
It is widely recognized that the release of nutrients by herbivores via their waste products strongly impacts nutrient availability for autotrophs. The ratios of nitrogen (N) and phosphorus (P) recycled through herbivore release (i.e., waste N:P) are mainly determined by the stoichiometric composition of the herbivore's food (food N:P) and its body nutrient content (body N:P). Waste N:P can in turn impact autotroph nutrient limitation and productivity. Herbivore-driven nutrient recycling based on stoichiometric principles is dominated by theoretical and experimental research in freshwater systems, in particular interactions between algae and invertebrate herbivores. In terrestrial ecosystems, the impact of herbivores on nutrient cycling and availability is often limited to studying carbon (C):N and C:P ratios, while the role of terrestrial herbivores in mediating N:P ratios is also likely to influence herbivore-driven nutrient recycling. In this review, we use rules and predictions on the stoichiometry of nutrient release originating from algal-based aquatic systems to identify the factors that determine the stoichiometry of nutrient release by herbivores. We then explore how these rules can be used to understand the stoichiometry of nutrient release by terrestrial herbivores, ranging from invertebrates to mammals, and its impact on plant nutrient limitation and productivity. Future studies should focus on measuring both N and P when investigating herbivore-driven nutrient recycling in terrestrial ecosystems, while also taking the form of waste product (urine or feces) and other pathways by which herbivores change nutrients into account, to be able to quantify the impact of waste stoichiometry on plant communities.
Introduction
Herbivores are a major component of most ecosystems, ranging in size from zooplankton to elephants. All herbivores consume and digest autotroph biomass, and release nutrients, e.g., nitrogen (N) and phosphorus (P), in wastes through excretion (urine) or egestion (feces). Nutrient release by herbivores can strongly impact nutrient availability for autotrophs in terrestrial, marine, and freshwater ecosystems (Pastor et al., 1993; McNaughton et al., 1997; Covich et al., 1999; Sirotnak and Huntly, 2000; Hunter, 2001; Vanni, 2002; Bardgett and Wardle, 2003; McIntyre et al., 2007; Cech et al., 2008; Roman and McCarthy, 2010; Metcalfe et al., 2014; Turner, 2015; Doughty et al., 2016). The ratio of N to P released (i.e., waste N:P) may be crucial for mediating ecosystem impacts of herbivore-driven nutrient recycling (Sterner, 1990; Urabe et al., 1995; Elser and Urabe, 1999). Two basic “stoichiometric rules” have been formulated, one based on how food and consumer body N:P determine waste N:P (rule 1), and the other on how waste N:P affects autotroph nutrient limitation and productivity (rule 2). Both rules allow for explicit predictions about the N:P stoichiometry of nutrient release and its ecosystem consequence (Table 1).
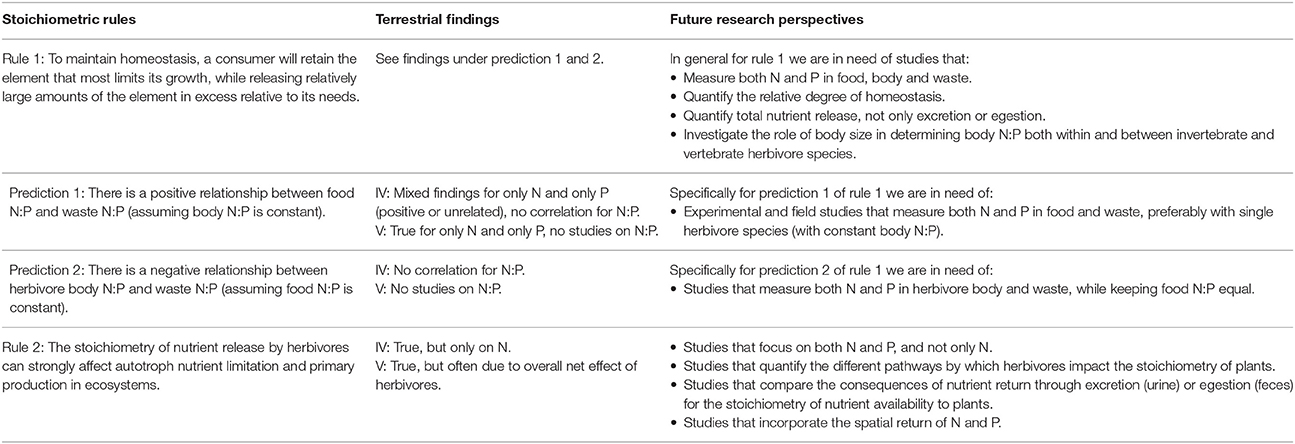
Table 1. Overview of the findings of the two stoichiometric rules, derived from interactions between algae and invertebrate herbivores in freshwater ecosystems, applied to terrestrial invertebrate (IV) and vertebrate (V) herbivores and ecosystems, including future research perspectives on each rule/prediction.
Thus far, evidence for these rules is mainly restricted to interactions between freshwater (pelagic) algae and invertebrate herbivores (Elser et al., 1988; Sterner, 1990; Sterner et al., 1992; Elser and Urabe, 1999; Sterner and Elser, 2002; Vanni, 2002), and to a lesser extent herbivorous fish (Schindler and Eby, 1997; Hood et al., 2005). However, herbivore-driven nutrient recycling also likely plays a major role in terrestrial ecosystems (Pastor et al., 1993; McNaughton et al., 1997; Hunter, 2001; Wardle et al., 2004; Metcalfe et al., 2014; Doughty et al., 2016). Indeed, the ratio of carbon (C) to nutrient (N and/or P) in plant tissues has long been recognized as an important determinant of herbivore feeding selectivity and subsequent nutrient cycling and availability in terrestrial ecosystems (Ritchie et al., 1998; Pastor et al., 2006; Bakker et al., 2009b). However, compared to aquatic systems, the role of terrestrial herbivores in mediating N:P ratios has received little attention so far. Because the ratio of N:P availability influences the type of growth limitation and the functional composition of terrestrial plant communities (Elser et al., 2007; Fujita et al., 2014), we hypothesize that the impact of terrestrial herbivores on this ratio has potentially strong ecosystem consequences.
In this review, we explore how we can apply these stoichiometric rules to terrestrial ecosystems, focusing on N:P ratios. We first explain the two rules derived from algae-invertebrate interactions in more detail. We then synthesize studies that applied these rules to terrestrial herbivores and ecosystems, identify research gaps, and suggest perspectives for future research.
Rule 1 (Individual Consumer Level)—Relationships between Food, Body and Waste N:P
Rule 1 is based on mass balance and the assumption that consumers maintain elemental homeostasis in their tissues by differential release of N and P. Stoichiometry theory predicts a positive relationship between food N:P and waste N:P, assuming constant consumer body N:P (Sterner and Elser, 2002). Second, waste N:P is predicted to be negatively related to body N:P, if food N:P is constant (Figure 1). These predictions have been supported in lab studies using aquatic invertebrate herbivores (Daphnia) feeding on phytoplankton (Elser and Urabe, 1999; Sterner and Elser, 2002). However, support for these predictions from field data is mixed. For example, a strong negative correlation between consumer body N:P and waste N:P was found in systems with large variation among animal species in body N:P (e.g., Vanni et al., 2002; McManamay et al., 2011), while in other systems food N:P was more important in predicting waste N:P (e.g., Urabe, 1993; Torres and Vanni, 2007). Recent syntheses suggest that body size and temperature have much more influence than body nutrients on excretion rates and ratios (Allgeier et al., 2015; Vanni and McIntyre, 2016).
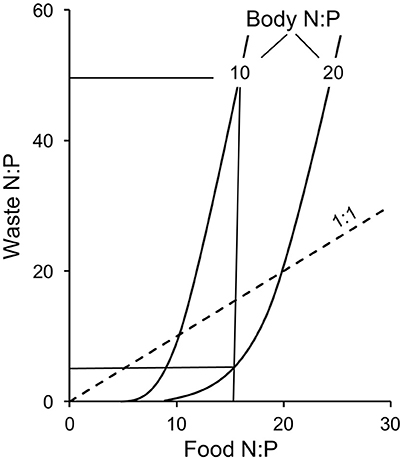
Figure 1. Predicted relationships for the N:P of the consumer's waste products as a function of its food N:P and body N:P. Two curves for herbivores with body N:P values of 10 and 20 are shown. First, the predicted relationship between waste N:P and food N:P is positive; linear when food N:P > body N:P and curvilinear when food N:P < body N:P. This means that if the herbivore with a body N:P of 20 consumes food with an N:P of 15, it will release wastes with an N:P < 15. In contrast, if the same herbivore ingests food with an N:P of 25, it will release wastes that have an N:P > 25. Secondly, for any given food different consumers will recycle nutrients at different ratios depending on their body N:P ratio. If both herbivores feed on plants with an N:P ratio of 15, the herbivore with the low N:P (10) needs to sequester relatively more P, and will thus release wastes at a much higher N:P, than the herbivore with high body N:P. Redrawn from Sterner and Elser (2002) with permission of authors.
Rule 2 (Ecosystem Level)—Impact of N:P Release by Herbivores on Plants
Rule 2 states that the stoichiometry of nutrient release by herbivores strongly affects autotroph nutrient limitation and primary production (Elser and Urabe, 1999). For example, if a consumer feeding on N-limited plants (low plant N:P) releases waste products with an even lower N:P than that in plant tissue (following rule 1), the herbivore could render the plant community even more N-limited, which can then impact competitive interactions between plants and plant community composition (Sterner, 1990; Fujita et al., 2014). However, the impact on plant communities will depend on the proportion of nutrient demand met by consumer-driven recycling. In freshwater systems, for instance, it sustains anywhere from <5 to >80% of algal nutrient uptake (Taylor et al., 2015). So far, field evidence that nutrient recycling by herbivores can shift autotroph assemblages between N- and P-limitation is scant (e.g., Sterner et al., 1992; Knoll et al., 2009), and hence a general underpinning of rule 2 is still lacking.
Even though tests of these rules are still scarce, especially under field conditions, according to mass-balance principles the relative ratios of nutrient release by herbivores should be influenced by stoichiometric balance. In the following sections, we take on the challenge of applying this stoichiometric view to herbivore-driven nutrient recycling in terrestrial ecosystems.
Applying Rule 1 to Terrestrial Herbivores
Although rule 1 predicts a positive relationship between food N:P and waste N:P (Figure 1, Table 1), most studies with terrestrial herbivores focused on single nutrients. Mixed results are found for invertebrate herbivores; food N and waste N can be positively related (lepidopterans; Kagata and Ohgushi, 2012), or unrelated (grasshopper; Zhang et al., 2014). Similarly, food P and waste P were unrelated for caterpillars (Meehan and Lindroth, 2009), but positively related for a grasshopper species (Zhang et al., 2014). The latter study also investigated the N:P ratio of food and waste simultaneously (the only terrestrial study we know of), and the ratios were not correlated. The authors suggest that the lack of relationship between food and waste nutrients is likely because mechanisms other than excretion maintain N:P homeostasis, such as pre-ingestive regulation of the nutrient balance through food selection (Zhang et al., 2014) or compensatory feeding (Meehan and Lindroth, 2009). Work on large vertebrate herbivores is much more extensive—but again, measurements were often done on either N or P and not both—and generally finds positive correlations between food and waste nutrient contents. For example, diet N and fecal N were positively correlated for rabbits (Gil-Jimenez et al., 2015), roe deer (Verheyden et al., 2011), white-tailed deer (Osborn and Ginnett, 2001), blackbuck antelope (Jhala, 1997), and several African herbivores (Wrench et al., 1997). This positive relationship was also found for P for cattle (Zhang et al., 2016) and several African herbivores (Wrench et al., 1997). Furthermore, urinary N (excretion) of large ungulates increases with plant N concentration (Hobbs, 1996). These relationships seem so reliable that fecal N and P contents are used to predict food N and P contents (Wrench et al., 1997; Verheyden et al., 2011; Gil-Jimenez et al., 2015), although corrections for the presence of indigestible forms of N, e.g., tannins, are needed (Verheyden et al., 2011; Steuer et al., 2014).
The second prediction derived from rule 1 is a negative relationship between consumer body N:P and waste N:P (Table 1). The only published study of terrestrial herbivores found no clear relationship between body and waste N:P for an invertebrate herbivore (grasshopper; Zhang et al., 2014); we found no studies on vertebrate herbivores.
This synthesis reveals the lack of studies examining relationships between food, body, and waste N:P (and not just N or P) in terrestrial herbivores, making it impossible to draw any general conclusions. Future work should include simultaneous measurements of N and P in food, bodies, and waste (Table 1). Controlled experiments where single herbivore species (constant body N:P) feed on food sources varying in N:P will provide a good test of the first prediction. Additionally, field studies on single species experiencing seasonal changes in food quality (food N:P) will be important, and again waste products and food sources of these herbivores need to be analyzed for both N and P. To test the second prediction, studies are needed where herbivores with a range of body N:P are fed a constant food N:P, and waste N:P is measured. Importantly, the predicted stoichiometric relationships between food, body and waste N:P might be impacted by several mitigating factors. These include the degree to which animals maintain homeostasis, which is variable and perhaps related to growth rate (Hood and Sterner, 2010; Downs et al., 2016), and which mechanisms they use to regulate this homeostasis (i.e., pre- or post-ingestive). Furthermore, the type of waste product (excretion or egestion; Halvorson et al., 2015) and the relationship between body size and body N:P are important mitigating factors, which should be taken into account and discussed below.
Animals that produce two types of wastes (feces and urine) can regulate their body composition pre-assimilation (by preferentially assimilating elements in short supply and releasing excess nutrients in feces) or post-assimilation (by excreting excess metabolic nutrients in urine before they reach toxic levels in the blood). Interestingly, the N:P stoichiometry of these two forms of nutrient release differ for terrestrial vertebrate herbivores; i.e., urine contains hardly any P but a high concentration of N in soluble form, while feces contain most of the P and some N (Morse et al., 1992; Hobbs, 1996). Relative concentrations of N in urine and feces depend on forage N, whereby herbivores that consume plants of high N (e.g., ungulate grazers consuming green grasses) return N to the soil mainly in the form of urine, while herbivores consuming plants of low N (e.g., ungulate browsers consuming tree twigs) need to extract as much N as possible and mainly produce feces of very low N (Pastor et al., 2006). However, very few studies quantify total nutrient release, instead of only excretion (often the case for aquatic animals) or egestion (often the case for terrestrial vertebrates) to test predictions of stoichiometry theory. This needs to be addressed in future studies, as the theory is based on mass-balance, and tests must therefore include all fluxes mediated by animal physiology (Table 1).
Generally, differences in body N:P are driven by patterns of investment in P-rich materials such as RNA and bone (Gillooly et al., 2005). Investments in RNA decrease significantly with body size (small organisms generally have higher growth rates), suggesting an increase in body N:P with increasing body size for invertebrates (Sterner and Elser, 2002; Back and King, 2013). However, more P is sequestered into supportive tissue like bones, suggesting that for vertebrates body N:P decreases with body size (Sterner and Elser, 2002) since skeleton mass scales allometrically (more than proportionally) with body mass (Anderson et al., 1979; Prange et al., 1979). This uncovers an important difference between aquatic and terrestrial systems, where terrestrial herbivores, especially larger individuals, need to invest more in P-rich structural tissue to counterbalance gravity. Hence, more studies investigating the role of body size in determining body N:P both within and between invertebrate and vertebrate herbivore species are needed (Table 1).
Applying Rule 2 to Terrestrial Ecosystems
Rule 2 states that the stoichiometry of nutrient release by herbivores can strongly affect autotroph nutrient limitation and primary production (Elser and Urabe, 1999). However, in terrestrial ecosystems it is hard to isolate the effect of herbivore-driven nutrient recycling, as the “net effect” of herbivores on nutrient cycling depends not only on direct effects of nutrient release through waste products, but also on indirect effects through modification of plant litter quantity and quality, and in the case of vertebrates, by alteration of soil physical properties through trampling (Ritchie et al., 1998; Belovsky and Slade, 2000; Hunter, 2001; Bardgett and Wardle, 2003; Schrama et al., 2013). Therefore, most empirical studies addressing how terrestrial herbivores shift plant assemblages between N- or P-limitation examined the “net effect” of herbivores (e.g., Carline et al., 2005; Frank, 2008; Zhang et al., 2011; Bai et al., 2012; Nitschke et al., 2015; Sitters et al., 2017) and not on the effects of nutrient release per se.
Many studies on herbivore-driven nutrient recycling in terrestrial ecosystems have focused on N, both for invertebrates (e.g., Seastedt and Crossley, 1984; Lovett and Ruesink, 1995; Belovsky and Slade, 2000; Reynolds and Hunter, 2001; Hunter et al., 2003; Metcalfe et al., 2014) and vertebrates (e.g., Pastor et al., 1988, 1993, 2006; McNaughton et al., 1988; Hobbs et al., 1991; Frank and McNaughton, 1993; Frank and Evans, 1997; McNaughton et al., 1997; Ritchie et al., 1998; Sirotnak and Huntly, 2000; Olofsson et al., 2001; Stark et al., 2003; Fornara and Du Toit, 2008). For invertebrate herbivores, the general view is that they speed up nutrient cycling in terrestrial systems by changing litter quantity and quality, modifying the nutrient content of throughfall, and releasing easily-available nutrients in frass and cadavers (Hunter, 2001). The direction of the impact of vertebrate herbivores on N cycling has traditionally been considered to depend on system fertility and corresponding plant N content; vertebrates have a positive effect on N availability and primary production in systems of high fertility, and a negative effect in low fertility systems (Hobbs, 1996; Bardgett and Wardle, 2003; Pastor et al., 2006). This is partly based on the proportion of N released, which is higher and mainly through urine when the nutrient content of plants is higher (Hobbs, 1996), but also on changes in plant litter quality, as herbivores feeding in systems with low plant N facilitate a shift toward litter of low N by selectively consuming high-N plant tissue (Bardgett and Wardle, 2003).
Stoichiometry theory has challenged the traditional views for impacts of herbivores on nutrient cycling. Modeling results demonstrate that if herbivores promote microbial C-limitation through vegetation consumption and respiration, they will have a positive effect on N availability in sites with low plant N by decreasing microbial immobilization rates, and a positive effect in sites with high plant N by decreasing mineralization rates (Cherif and Loreau, 2013). For vertebrate herbivores these results are party supported by field data (Bakker et al., 2009a; Sitters et al., 2017). Also, for invertebrates, labile C in excreta can result in N immobilization and lower availability (Lovett and Ruesink, 1995). These studies again show the need for a more integrative framework to understand and quantify the different pathways by which vertebrate (Sitters and Olde Venterink, 2015) and invertebrate herbivores (Hunter, 2001) impact nutrient cycling and availability to plants.
To predict how the stoichiometry of nutrient release by herbivores affects autotroph nutrient limitation and primary production in terrestrial systems, we must expand studies on the role of P, because N- and P-limitation are both prevalent in terrestrial ecosystems (Elser et al., 2007). The effect of herbivores on N- and P-limitation depends first on the form in which N and P are returned to the soil (urine or feces). N in urine is soluble and directly available to plants, while feces contain a substantial amount of organic matter, which needs to be decomposed and mineralized to render the N and P available to plants (Hobbs, 1996). Furthermore, N in feces and urine is subject to a significant loss from the system via ammonia volatilization and leaching (Ruess and McNaughton, 1988; Frank and Zhang, 1997; Augustine, 2003), suggesting that terrestrial herbivores (that produce both types of waste products) may drive ecosystems to N-limitation through nutrient release (e.g., Cech et al., 2008). Very little data however exist, comparing the consequences of nutrient return through urine or feces for the stoichiometry of nutrient availability to plants.
Additionally, nutrient release by herbivores strongly increases the spatial heterogeneity of N:P availability across the landscape. Terrestrial herbivores typically do not graze and excrete randomly, but are attracted to landscape features, such as nutrient-rich areas with high food quality, resulting in a net import of nutrients and creating nutrient hotspots in the landscape. At the same time, large parts of the landscape with poorer quality vegetation experience a net removal of nutrients (McNaughton et al., 1997; Augustine et al., 2003; van der Waal et al., 2011). Water bodies may also induce spatial patterns; semi-aquatic herbivores such as hippopotamus, beaver or water birds can transport nutrients across ecosystem boundaries and thus strongly impact nutrient redistribution (Sitters et al., 2015; Subalusky et al., 2015; Bakker et al., 2016). Furthermore, social behavior may affect nutrient release. When herbivores defecate in common latrines, they concentrate nutrients in the landscape (e.g., rhinos, rabbits, horses), which function as hotspots of nutrients, possibly with a low N:P ratio, though data are scarce and not fully consistent regarding the effect on soil P (see Edwards and Hollis, 1982; Willott et al., 2000; Jewell et al., 2007). In this respect, there are similarities and differences between terrestrial and aquatic habitats. In both, animals can mediate a net translocation of nutrients across habitats and ecosystems (Vanni et al., 2001; Flecker et al., 2010; Ebel et al., 2015; Sitters et al., 2015). However, in aquatic habitats excreted nutrients may easily mix in the water, whereas in terrestrial habitats patches of released nutrients are much more spatially disconnected; this suggests that terrestrial animals may induce spatial variation in nutrient supply and stoichiometry more so than aquatic animals.
Concluding Remarks
The stoichiometric view of herbivore-driven nutrient recycling in terrestrial ecosystems has not yet received the attention it deserves. We were unable to find firm evidence for rule 1, as most studies investigating relationships between food, herbivore bodies, and wastes focused on single nutrients. At the same time, many studies consider the “net” effect of herbivores on nutrient cycling, making it impossible to determine the impact of waste stoichiometry on plant communities per se (rule 2). We therefore suggest several perspectives on future research, to increase our understanding of the stoichiometry of nutrient release by terrestrial herbivores, ranging from invertebrates to mammals, and its impact on ecosystem stoichiometry, plant nutrient limitation, and productivity (Table 1).
Author Contributions
All authors listed, have made substantial, direct and intellectual contribution to the work, and approved it for publication.
Funding
JS was financially supported by a grant of the Research Foundation Flanders (FWO), grant 12N2615N. MPV has been financially supported by the AfricanBioServices project which received funding from the European Union's Horizon 2020 research and innovation programme under grant agreement No 641918. MJV was supported by US National Science Foundation grants 0918993 and 1255159. Additional funding was provided by Strategic Resources of the Netherlands Institute of Ecology (NIOO-KNAW); this is publication number 6272.
Conflict of Interest Statement
The authors declare that the research was conducted in the absence of any commercial or financial relationships that could be construed as a potential conflict of interest.
References
Allgeier, J. E., Wenger, S. J., Rosemond, A. D., Schindler, D. E., and Layman, C. A. (2015). Metabolic theory and taxonomic identity predict nutrient recycling in a diverse food web. Proc. Natl. Acad. Sci. U.S.A. 112, 2640–2647. doi: 10.1073/pnas.1420819112
Anderson, J. F., Rahn, H., and Prange, H. D. (1979). Scaling of supportive tissue mass. Q. Rev. Biol. 54, 139–148. doi: 10.1086/411153
Augustine, D. J. (2003). Long-term, livestock-mediated redistribution of nitrogen and phosphorus in an East African savanna. J. Appl. Ecol. 40, 137–149. doi: 10.1046/j.1365-2664.2003.00778.x
Augustine, D. J., McNaughton, S. J., and Frank, D. A. (2003). Feedbacks between soil nutrients and large herbivores in a managed savanna ecosystem. Ecol. Appl. 13, 1325–1337. doi: 10.1890/02-5283
Back, J. A., and King, R. S. (2013). Sex and size matter: ontogenetic patterns of nutrient content of aquatic insects. Freshwater Science 32, 837–848. doi: 10.1899/12-181.1
Bai, Y. F., Wu, J. G., Clark, C. M., Pan, Q. M., Zhang, L. X., Chen, S. P., et al. (2012). Grazing alters ecosystem functioning and C:N:P stoichiometry of grasslands along a regional precipitation gradient. J. Appl. Ecol. 49, 1204–1215. doi: 10.1111/j.1365-2664.2012.02205.x
Bakker, E. S., Knops, J. M. H., Milchunas, D. G., Ritchie, M. E., and Olff, H. (2009a). Cross-site comparison of herbivore impact on nitrogen availability in grasslands: the role of plant nitrogen concentration. Oikos 118, 1613–1622. doi: 10.1111/j.1600-0706.2009.17199.x
Bakker, E. S., Olff, H., and Gleichman, J. M. (2009b). Contrasting effects of large herbivore grazing on smaller herbivores. Basic Appl. Ecol. 10, 141–150. doi: 10.1016/j.baae.2007.10.009
Bakker, E. S., Pages, J. F., Arthur, R., and Alcoverro, T. (2016). Assessing the role of large herbivores in the structuring and functioning of freshwater and marine angiosperm ecosystems. Ecography 39, 162–179. doi: 10.1111/ecog.01651
Bardgett, R. D., and Wardle, D. A. (2003). Herbivore-mediated linkages between aboveground and belowground communities. Ecology 84, 2258–2268. doi: 10.1890/02-0274
Belovsky, G. E., and Slade, J. B. (2000). Insect herbivory accelerates nutrient cycling and increases plant production. Proc. Natl. Acad. Sci. U.S.A. 97, 14412–14417. doi: 10.1073/pnas.250483797
Carline, K. A., Jones, H. E., and Bardgett, R. D. (2005). Large herbivores affect the stoichiometry of nutrients in a regenerating woodland ecosystem. Oikos 110, 453–460. doi: 10.1111/j.0030-1299.2005.13550.x
Cech, P. G., Kuster, T., Edwards, P. J., and Olde Venterink, H. (2008). Effects of herbivory, fire and N2-fixation on nutrient limitation in a humid African savanna. Ecosystems 11, 991–1004. doi: 10.1007/s10021-008-9175-7
Cherif, M., and Loreau, M. (2013). Plant–herbivore–decomposer stoichiometric mismatches and nutrient cycling in ecosystems. Proc. R. Soc. B. Biol. Sci. 280:20122453. doi: 10.1098/rspb.2012.2453
Covich, A. P., Palmer, M. A., and Crowl, T. A. (1999). The role of benthic invertebrate species in freshwater ecosystems - Zoobenthic species influence energy flows and nutrient cycling. Bioscience 49, 119–127. doi: 10.2307/1313537
Doughty, C. E., Roman, J., Faurby, S., Wolf, A., Haque, A., Bakker, E. S., et al. (2016). Global nutrient transport in a world of giants. Proc. Natl. Acad. Sci. U.S.A. 113, 868–873. doi: 10.1073/pnas.1502549112
Downs, K. N., Hayes, N. M., Rock, A. M., Vanni, M. J., and Gonzalez, M. J. (2016). Light and nutrient supply mediate intraspecific variation in the nutrient stoichiometry of juvenile fish. Ecosphere 7:e01452. doi: 10.1002/ecs2.1452
Ebel, J. D., Leroux, S. J., Robertson, M. J., and Dempson, J. B. (2015). Ontogenetic differences in Atlantic salmon phosphorus concentration and its implications for cross ecosystem fluxes. Ecosphere 6, 1–18. doi: 10.1890/es14-00516.1
Edwards, P. J., and Hollis, S. (1982). The distribution of excreta on new forest grassland used by cattle, ponies and deer. J. Appl. Ecol. 19, 953–964.
Elser, J. J., Bracken, M. E. S., Cleland, E. E., Gruner, D. S., Harpole, W. S., Hillebrand, H., et al. (2007). Global analysis of nitrogen and phosphorus limitation of primary producers in freshwater, marine and terrestrial ecosystems. Ecol. Lett. 10, 1135–1142. doi: 10.1111/j.1461-0248.2007.01113.x
Elser, J. J., Elser, M. M., Mackay, N. A., and Carpenter, S. R. (1988). Zooplankton-mediated transitions between N-limited and P-limited algal growth. Limnol. Oceanogr. 33, 1–14.
Elser, J. J., and Urabe, J. (1999). The stoichiometry of consumer-driven nutrient recycling: theory, observations, and consequences. Ecology 80, 735–751. doi: 10.1890/0012-9658(1999)080[0735:tsocdn]2.0.co;2
Flecker, A. S., McIntyre, P. B., Moore, J. W., Anderson, J. T., Taylor, B. W., and Hall, R. O. (2010). Migratory fishes as material and process subsidies in riverine ecosystems. Am. Fish. Soc. Symp. 73, 559–592.
Fornara, D. A., and Du Toit, J. T. (2008). Browsing-induced effects on leaf litter quality and decomposition in a southern african savanna. Ecosystems 11, 238–249. doi: 10.1007/s10021-007-9119-7
Frank, D. A. (2008). Ungulate and topographic control of nitrogen: phosphorus stoichiometry in a temperate grassland; soils, plants and mineralization rates. Oikos 117, 591–601. doi: 10.1111/j.2007.0030-1299.16220.x
Frank, D. A., and Evans, R. D. (1997). Effects of native grazers on grassland N cycling in Yellowstone National Park. Ecology 78, 2238–2248. doi: 10.1890/0012-9658(1997)078[2238:eongog]2.0.co;2
Frank, D. A., and McNaughton, S. J. (1993). Evidence for the promotion of aboveground grassland production by native large herbivores in Yellowstone National Park. Oecologia 96, 157–161.
Frank, D. A., and Zhang, Y. M. (1997). Ammonia volatilization from a seasonally and spatially variable grazed grassland: Yellowstone National Park. Biogeochemistry 36, 189–203. doi: 10.1023/a:1005705121160
Fujita, Y., Olde Venterink, H., van Bodegom, P. M., Douma, J. C., Heil, G. W., Holzel, N., et al. (2014). Low investment in sexual reproduction threatens plants adapted to phosphorus limitation. Nature 505, 82–86. doi: 10.1038/nature12733
Gil-Jimenez, E., Villamuelas, M., Serrano, E., Delibes, M., and Fernandez, N. (2015). Fecal nitrogen concentration as a nutritional quality indicator for european rabbit ecological studies. PLoS ONE 10:e0125190. doi: 10.1371/journal.pone.0125190
Gillooly, J. F., Allen, A. P., Brown, J. H., Elser, J. J., del Rio, C. M., Savage, V. M., et al. (2005). The metabolic basis of whole-organism RNA and phosphorus content. Proc. Natl. Acad. Sci. U.S.A. 102, 11923–11927. doi: 10.1073/pnas.0504756102
Halvorson, H. M., Fuller, C., Entrekin, S. A., and Evans-White, M. A. (2015). Dietary influences on production, stoichiometry and decomposition of particulate wastes from shredders. Freshw. Biol. 60, 466–478. doi: 10.1111/fwb.12462
Hobbs, N. T. (1996). Modification of ecosystems by ungulates. J. Wildl. Manag. 60, 695–713. doi: 10.2307/3802368
Hobbs, N. T., Schimel, D. S., Owensby, C. E., and Ojima, D. S. (1991). Fire and grazing in the tallgrass prairie - contingent effects on nitrogen budgets. Ecology 72, 1374–1382. doi: 10.2307/1941109
Hood, J. M., and Sterner, R. W. (2010). Diet mixing: do animals integrate growth or resources across temporal heterogeneity? Am. Nat. 176, 651–663. doi: 10.1086/656489
Hood, J. M., Vanni, M. J., and Flecker, A. S. (2005). Nutrient recycling by two phosphorus-rich grazing catfish: the potential for phosphorus-limitation of fish growth. Oecologia 146, 247–257. doi: 10.1007/s00442-005-0202-5
Hunter, M. D. (2001). Insect population dynamics meets ecosystem ecology: effects of herbivory on soil nutrient dynamics. Agric. For. Entomol. 3, 77–84. doi: 10.1046/j.1461-9563.2001.00100.x
Hunter, M. D., Linnen, C. R., and Reynolds, B. C. (2003). Effects of endemic densities of canopy herbivores on nutrient dynamics along a gradient in elevation in the southern Appalachians. Pedobiologia 47, 231–244. doi: 10.1078/0031-4056-00187
Jewell, P. L., Kauferle, D., Guesewell, S., Berry, N. R., Kreuzer, M., and Edwards, P. J. (2007). Redistribution of phosphorus by mountain pasture in cattle on a traditional the Alps. Agric. Ecosyst. Environ. 122, 377–386. doi: 10.1016/j.agee.2007.02.012
Jhala, Y. V. (1997). Seasonal effects on the nutritional ecology of blackbuck Antelope cervicapra. J. Appl. Ecol. 34, 1348–1358. doi: 10.2307/2405252
Kagata, H., and Ohgushi, T. (2012). Carbon to nitrogen excretion ratio in lepidopteran larvae: relative importance of ecological stoichiometry and metabolic scaling. Oikos 121, 1869–1877. doi: 10.1111/j.1600-0706.2012.20274.x
Knoll, L. B., McIntyre, P. B., Vanni, M. J., and Flecker, A. S. (2009). Feedbacks of consumer nutrient recycling on producer biomass and stoichiometry: separating direct and indirect effects. Oikos 118, 1732–1742. doi: 10.1111/j.1600-0706.2009.17367.x
Lovett, G. M., and Ruesink, A. E. (1995). Carbon and nitrogen mineralization from decomposing gypsy-moth frass. Oecologia 104, 133–138. doi: 10.1007/bf00328577
McIntyre, P. B., Jones, L. E., Flecker, A. S., and Vanni, M. J. (2007). Fish extinctions alter nutrient recycling in tropical freshwaters. Proc. Natl. Acad. Sci. U.S.A. 104, 4461–4466. doi: 10.1073/pnas.0608148104
McManamay, R. A., Webster, J. R., Valett, H. M., and Dolloff, C. A. (2011). Does diet influence consumer nutrient cycling? Macroinvertebrate and fish excretion in streams. J. North Am. Benthol. Soc. 30, 84–102. doi: 10.1899/09-152.1
McNaughton, S. J., Banyikwa, F. F., and McNaughton, M. M. (1997). Promotion of the cycling of diet-enhancing nutrients by African grazers. Science 278, 1798–1800. doi: 10.1126/science.278.5344.1798
McNaughton, S. J., Ruess, R. W., and Seagle, S. W. (1988). Large mammals and process dynamics in African ecosystems. Bioscience 38, 794–800. doi: 10.2307/1310789
Meehan, T. D., and Lindroth, R. L. (2009). Scaling of individual phosphorus flux by caterpillars of the whitemarked tussock moth, Orygia leucostigma. J. Insect Sci. 9:42. doi: 10.1673/031.009.4201
Metcalfe, D. B., Asner, G. P., Martin, R. E., Espejo, J. E. S., Huasco, W. H., Amezquita, F. F. F., et al. (2014). Herbivory makes major contributions to ecosystem carbon and nutrient cycling in tropical forests. Ecol. Lett. 17, 324–332. doi: 10.1111/ele.12233
Morse, D., Head, H. H., Wilcox, C. J., Vanhorn, H. H., Hissem, C. D., and Harris, B. (1992). Effects of concentration of dietary phosphorus on amount and route of excretion. J. Dairy Sci. 75, 3039–3049.
Nitschke, N., Wiesner, K., Hilke, I., Eisenhauer, N., Oelmann, Y., and Weisser, W. W. (2015). Increase of fast nutrient cycling in grassland microcosms through insect herbivory depends on plant functional composition and species diversity. Oikos 124, 161–173. doi: 10.1111/oik.01476
Olofsson, J., Kitti, H., Rautiainen, P., Stark, S., and Oksanen, L. (2001). Effects of summer grazing by reindeer on composition of vegetation, productivity and nitrogen cycling. Ecography 24, 13–24. doi: 10.1034/j.1600-0587.2001.240103.x
Osborn, R. G., and Ginnett, T. F. (2001). Fecal nitrogen and 2,6-diaminopimelic acid as indices to dietary nitrogen in white-tailed deer. Wildl. Soc. Bull. 29, 1131–1139. doi: 10.2307/3784136
Pastor, J., Cohen, Y., and Hobbs, N. T. (2006). “The role of large herbivores in ecosystem nutrient cycles,” in Large Herbivore Ecology, Ecosystem Dynamics and Conservation, eds R. Dannell, R. Bergstrom, P. Duncan, and J. Pastor (Cambridge: Cambridge University Press), 289–319.
Pastor, J., Dewey, B., Naiman, R. J., McInnes, P. F., and Cohen, Y. (1993). Moose browsing and soil fertility in the boreal forests of Isle Royale National Park. Ecology 74, 467–480. doi: 10.2307/1939308
Pastor, J., Naiman, R. J., Dewey, B., and McInnes, P. (1988). Moose, microbes, and the boreal forest. Bioscience 38, 770–777. doi: 10.2307/1310786
Prange, H. D., Anderson, J. F., and Rahn, H. (1979). Scaling of skeletal mass to body-mass in birds and mammals. Am. Nat. 113, 103–122. doi: 10.1086/283367
Reynolds, B. C., and Hunter, M. D. (2001). Responses of soil respiration, soil nutrients, and litter decomposition to inputs from canopy herbivores. Soil Biol. Biochem. 33, 1641–1652. doi: 10.1016/s0038-0717(01)00085-2
Ritchie, M. E., Tilman, D., and Knops, J. M. H. (1998). Herbivore effects on plant and nitrogen dynamics in oak savanna. Ecology 79, 165–177. doi: 10.2307/176872
Roman, J., and McCarthy, J. J. (2010). The whale pump: marine mammals enhance primary productivity in a Coastal Basin. PLoS ONE 5:e13255. doi: 10.1371/journal.pone.0013255
Ruess, R. W., and McNaughton, S. J. (1988). Ammonia volatilization and the effects of large grazing mammals on nutrient loss from East African grasslands. Oecologia 77, 382–386. doi: 10.1007/bf00378047
Schindler, D. E., and Eby, L. A. (1997). Stoichiometry of fishes and their prey: implications for nutrient recycling. Ecology 78, 1816–1831.
Schrama, M., Veen, G. F., Bakker, E. S., Ruifrok, J. L., Bakker, J. P., and Olff, H. (2013). An integrated perspective to explain nitrogen mineralization in grazed ecosystems. Perspect. Plant Ecol. Evolut. Syst. 15, 32–44. doi: 10.1016/j.ppees.2012.12.001
Seastedt, T. R., and Crossley, D. A. (1984). The influence of arthropods on ecosystems. Bioscience 34, 157–161. doi: 10.2307/1309750
Sirotnak, J. M., and Huntly, N. J. (2000). Direct and indirect effects of herbivores on nitrogen dynamics: voles in riparian areas. Ecology 81, 78–87. doi: 10.1890/0012-9658(2000)081[0078:DAIEOH]2.0.CO;2
Sitters, J., Atkinson, C. L., Guelzow, N., Kelly, P., and Sullivan, L. L. (2015). Spatial stoichiometry: cross-ecosystem material flows and their impact on recipient ecosystems and organisms. Oikos 124, 920–930. doi: 10.1111/oik.02392
Sitters, J., te Beest, M., Cherif, M., Giesler, R., and Olofsson, J. (2017). Interactive effects between reindeer and habitat fertility drive soil nutrient availabilities in arctic tundra. Ecosystems 1–12. doi: 10.1007/s10021-017-0108-1
Sitters, J., and Olde Venterink, H. (2015). The need for a novel integrative theory on feedbacks between herbivores, plants and soil nutrient cycling. Plant Soil 396, 421–426. doi: 10.1007/s11104-015-2679-y
Stark, S., Tuomi, J., Strommer, R., and Helle, T. (2003). Non-parallel changes in soil microbial carbon and nitrogen dynamics due to reindeer grazing in northern boreal forests. Ecography 26, 51–59. doi: 10.1034/j.1600-0587.2003.03336.x
Sterner, R. W. (1990). The ratio of nitrogen to phosphorus resupplied by herbivores–zooplankton and the algal competitive arena. Am. Nat. 136, 209–229. doi: 10.1086/285092
Sterner, R. W., and Elser, J. J. (2002). Ecological Stoichiometry. Princeton, NJ: Princeton University Press.
Sterner, R. W., Elser, J. J., and Hessen, D. O. (1992). Stoichiometric relationships among producers, consumers and nutrient cycling in pelagic ecosystems. Biogeochemistry 17, 49–67.
Steuer, P., Sudekum, K. H., Tutken, T., Muller, D. W. H., Kaandorp, J., Bucher, M., et al. (2014). Does body mass convey a digestive advantage for large herbivores? Funct. Ecol. 28, 1127–1134. doi: 10.1111/1365-2435.12275
Subalusky, A. L., Dutton, C. L., Rosi-Marshall, E. J., and Post, D. M. (2015). The hippopotamus conveyor belt: vectors of carbon and nutrients from terrestrial grasslands to aquatic systems in sub-Saharan Africa. Freshw. Biol. 60, 512–525. doi: 10.1111/fwb.12474
Taylor, J. M., Vanni, M. J., and Flecker, A. S. (2015). “Top-down and bottom-up interactions in freshwater ecosystems: emerging complexities,” in Trophic Ecology: Bottom-Up and Top-Down Interactions Across Aquatic and Terrestrial Systems, eds T. C. Hanley and K. J. La Pierre. (Cambridge: Cambridge University Press), 55–85.
Torres, L. E., and Vanni, M. J. (2007). Stoichiometry of nutrient excretion by fish: interspecific variation in a hypereutrophic lake. Oikos 116, 259–270. doi: 10.1111/j.2006.0030-1299.15268.x
Turner, J. T. (2015). Zooplankton fecal pellets, marine snow, phytodetritus and the ocean's biological pump. Prog. Oceanogr. 130, 205–248. doi: 10.1016/j.pocean.2014.08.005
Urabe, J. (1993). N-cycling and P-cycling coupled by grazer activities–food quality and nutrient release by zooplankton. Ecology 74, 2337–2350. doi: 10.2307/1939586
Urabe, J., Nakanishi, M., and Kawabata, K. (1995). Contribution of metazoan plankton to the cycling of nitrogen and phosphorus in Lake Biwa. Limnol. Oceanogr. 40, 232–241.
van der Waal, C., Kool, A., Meijer, S. S., Kohi, E., Heitkönig, I. M. A., de Boer, W. F., et al. (2011). Large herbivores may alter vegetation structure of semi-arid savannas through soil nutrient mediation. Oecologia 165, 1095–1107. doi: 10.1007/s00442-010-1899-3
Vanni, M. J. (2002). Nutrient cycling by animals in freshwater ecosystems. Annu. Rev. Ecol. Syst. 33, 341–370. doi: 10.1146/annurev.ecolysis.33.010802.150519
Vanni, M. J., Flecker, A. S., Hood, J. M., and Headworth, J. L. (2002). Stoichiometry of nutrient recycling by vertebrates in a tropical stream: linking species identity and ecosystem processes. Ecol. Lett. 5, 285–293. doi: 10.1046/j.1461-0248.2002.00314.x
Vanni, M. J., and McIntyre, P. B. (2016). Predicting nutrient excretion of aquatic animals with metabolic ecology and ecological stoichiometry: a global synthesis. Ecology 97, 3460–3471. doi: 10.1002/ecy.1582
Vanni, M. J., Renwick, W. H., Headworth, J. L., Auch, J. D., and Schaus, M. H. (2001). Dissolved and particulate nutrient flux from three adjacent agricultural watersheds: a five-year study. Biogeochemistry 54, 85–114. doi: 10.1023/a:1010681229460
Verheyden, H., Aubry, L., Merlet, J., Petibon, P., Chauveau-Duriot, B., Guillon, N., et al. (2011). Faecal nitrogen, an index of diet quality in roe deer Capreolus capreolus? Wildlife Biol. 17, 166–175. doi: 10.2981/10-111
Wardle, D. A., Bardgett, R. D., Klironomos, J. N., Setala, H., van der Putten, W. H., and Wall, D. H. (2004). Ecological linkages between aboveground and belowground biota. Science 304, 1629–1633. doi: 10.1126/Science.1094875
Willott, S. J., Miller, A. J., Incoll, L. D., and Compton, S. G. (2000). The contribution of rabbits (Oryctolagus cuniculus L.) to soil fertility in semi-arid Spain. Biol. Fertil. Soils 31, 379–384. doi: 10.1007/s003749900183
Wrench, J. M., Meissner, H. H., and Grant, C. C. (1997). Assessing diet quality of African ungulates from faecal analyses: the effect of forage quality, intake and herbivore species. Koedoe 40, 125–136. doi: 10.4102/koedoe.v40i1.268
Zhang, B., Wang, C., Wei, Z. H., Sun, H. Z., Xu, G. Z., Liu, J. X., et al. (2016). The effects of dietary phosphorus on the growth performance and phosphorus excretion of dairy heifers. Asian-Australas. J. Anim. Sci. 29, 960–964. doi: 10.5713/ajas.15.0548
Zhang, G. M., Han, X. G., and Elser, J. J. (2011). Rapid top-down regulation of plant C:N:P stoichiometry by grasshoppers in an Inner Mongolia grassland ecosystem. Oecologia 166, 253–264. doi: 10.1007/s00442-011-1904-5
Keywords: autotroph productivity, aquatic ecosystems, C:N:P ratios, excretion, feces, herbivore-driven nutrient recycling, nitrogen, phosphorus
Citation: Sitters J, Bakker ES, Veldhuis MP, Veen GF, Olde Venterink H and Vanni MJ (2017) The Stoichiometry of Nutrient Release by Terrestrial Herbivores and Its Ecosystem Consequences. Front. Earth Sci. 5:32. doi: 10.3389/feart.2017.00032
Received: 30 January 2017; Accepted: 07 April 2017;
Published: 25 April 2017.
Edited by:
James Joseph Elser, University of Montana, USAReviewed by:
John Pastor, University of Minnesota, USAJacob Edward Allgeier, University of California, Santa Barbara, USA
Angelica L. Gonzalez, Rutgers University, USA
Copyright © 2017 Sitters, Bakker, Veldhuis, Veen, Olde Venterink and Vanni. This is an open-access article distributed under the terms of the Creative Commons Attribution License (CC BY). The use, distribution or reproduction in other forums is permitted, provided the original author(s) or licensor are credited and that the original publication in this journal is cited, in accordance with accepted academic practice. No use, distribution or reproduction is permitted which does not comply with these terms.
*Correspondence: Judith Sitters, judith.sitters@vub.be