- 1Instituto Dom Luís, Faculdade de Ciências, Universidade de Lisboa, Lisboa, Portugal
- 2Institute of Tectonics and Geophysics, Russian Academy of Sciences, Khabarovsk, Russia
The environmental impact of the Deccan trap volcanism is poorly understood as yet. The paucity of geological markers that can unambiguously be attributed to the Deccan volcanism and the temporal coincidence of the volcanism with an asteroid impact make evaluation of volcanic contribution to the end Cretaceous mass extinction difficult. Here we briefly review environmental proxy records of two reference Cretaceous-Tertiary boundary (KTB) sections, Bidart (France) and Gubbio (Italy). In both sections, a change in color of sediments located just below the KTB is systematically associated with very low values of (low-field) magnetic susceptibility (MS). Rock magnetic characteristics suggest that the decrease in MS values results from the loss (dissolution) of ferrimagnetic mineral in this intervals. In addition to the characteristic change in magnetic assemblage, akaganeite (chlorine-bearing iron oxyhydroxide) is commonly observed under the scanning electron microscope in the low MS intervals at Bidart and Gubbio, but has never been detected in the remaining sedimentary successions. We suggest that the association of granular akaganeite and iron oxides dissolution features can be explained by an ocean acidification and aerosol deposition event linked to the Deccan Phase-2 volcanism.
Introduction
The Deccan traps, the greatest episode of continental flood basalt volcanism in the Phanerozoic, released large volumes of greenhouse gasses into the atmosphere perturbing the Earth's carbon cycle and contributing to the end Cretaceous mass extinction (Courtillot et al., 1986; Keller et al., 2011, 2012). The full appreciation of the climatic effects of the Deccan volcanism has been hampered by difficulties in precise dating of the volcanic episodes and correlating them with biostratigraphically dated marine sections. Recently, three discrete Deccan volcanism phases with variable intensity have been dated based on magnetostratigraphy and 40K-40Ar age (Chenet et al., 2007): Phase-1 (~67.5 Ma, 6% in volume), Phase-2 (~65 Ma; 80% in volume) and Phase-3 (~64.5 Ma; 14% in volume). The timing of the largest Deccan volcanic phase (Phase-2) and the Chicxulub impact are thus not resolvable based on their 40K-40Ar ages. The nearly contemporaneous occurrence of these two catastrophic events limits our ability to evaluate their respective contribution to biotic changes at the end of the Cretaceous.
Environmental changes at the KT boundary are recorded in sedimentary archives. Several carbonate sections from the Bay of Biscay and Tethys realm show peculiar changes in the mineralogy, color and magnetic properties within the narrow stratigraphic interval located just below the KT boundary clays. This interval roughly corresponds to the CF1 and CF2 biozones that show dramatic changes in planktic foraminifera, nanno and macrofossils leading up to the KT boundary extinction and is roughly coincident with the timing of the Deccan Phase-2 eruptions (Thibault and Gardin, 2007; Gertsch et al., 2011; Keller et al., 2012). Conventionally, the changes in sediment properties are attributed to the asteroid impact (e.g., Lowrie et al., 1990). Here we argue instead that these characteristic changes record an ocean acidification event caused by the Deccan Phase-2 eruptions.
Iron Oxide Dissolution and Akaganeite Deposition: Diagenesis or Syn-Depositional Changes Linked to Deccan Phase-2?
The latest Maastrichtian sediments just below the KT boundary at sections from the Bay of Biscay (Bidart) and the Tethys (Gubbio) show significant changes in color, mineralogy and magnetic properties within a narrow stratigraphic interval, thickness of which varies between the sections from several decimeters up to about one meter. The Bidart section consists of hemipelagic to pelagic sediments deposited in a deep basin, and is considered to be one of the most complete KTB sections in Europe (Alegret et al., 2004; Galbrun and Gardin, 2004; Gallala et al., 2009). The KT boundary, easily identifiable by the iridium anomaly (Bonté et al., 1984), is overlain by the typical thin dark clay layer containing the relics of the Chicxulub impact (Apellaniz et al., 1997). The change in color is systematically associated with very low values of magnetic susceptibility (MS) at Bidart and Gubbio (Lowrie et al., 1990; Ellwood et al., 2003; Font et al., 2011). Magnetic susceptibility data, however, is difficult to interpret in a unique way as it includes contributions (in proportion to their abundance) from all—diamagnetic, paramagnetic and ferromagnetic—minerals present in the sediment. Statistical analysis of Isothermal Remanent Magnetization (IRM) acquisition curves (Robertson and France, 1994; Kruiver et al., 2001) provides more detailed information on composition, concentration and grain-size distribution of ferrimagnetic phases. IRM acquisition analyses combined with other rock magnetic techniques identified detrital and biogenic (magnetosomes of magnetotactic bacteria) magnetite, hematite and goethite in the studied sections (Font et al., 2014; Figure 1). When compared to background Cretaceous sediments, the low MS zone is characterized by an absence of biogenic magnetite, a decrease in total ferrimagnetic mineral content, and preferential loss of magnetite with respect to hematite (Galbrun and Gardin, 2004; Font et al., 2011; Abrajevitch et al., 2014). A similar style of ferrimagnetic assemblage modification is commonly observed in marine sediments during reductive diagenesis (Cornell and Schwertmann, 2003; Abrajevitch and Kodama, 2011). Reductive dissolution of detrital iron oxides by downward infiltration of reducing waters had previously been proposed by Lowrie et al. (1990) as an explanation for the low susceptibility of white limestones below the KT boundary at Gubbio. The reducing environment was thought to result from the decomposition of large quantity of organic matter produced by the extinctions after the asteroid impact at the KT boundary.
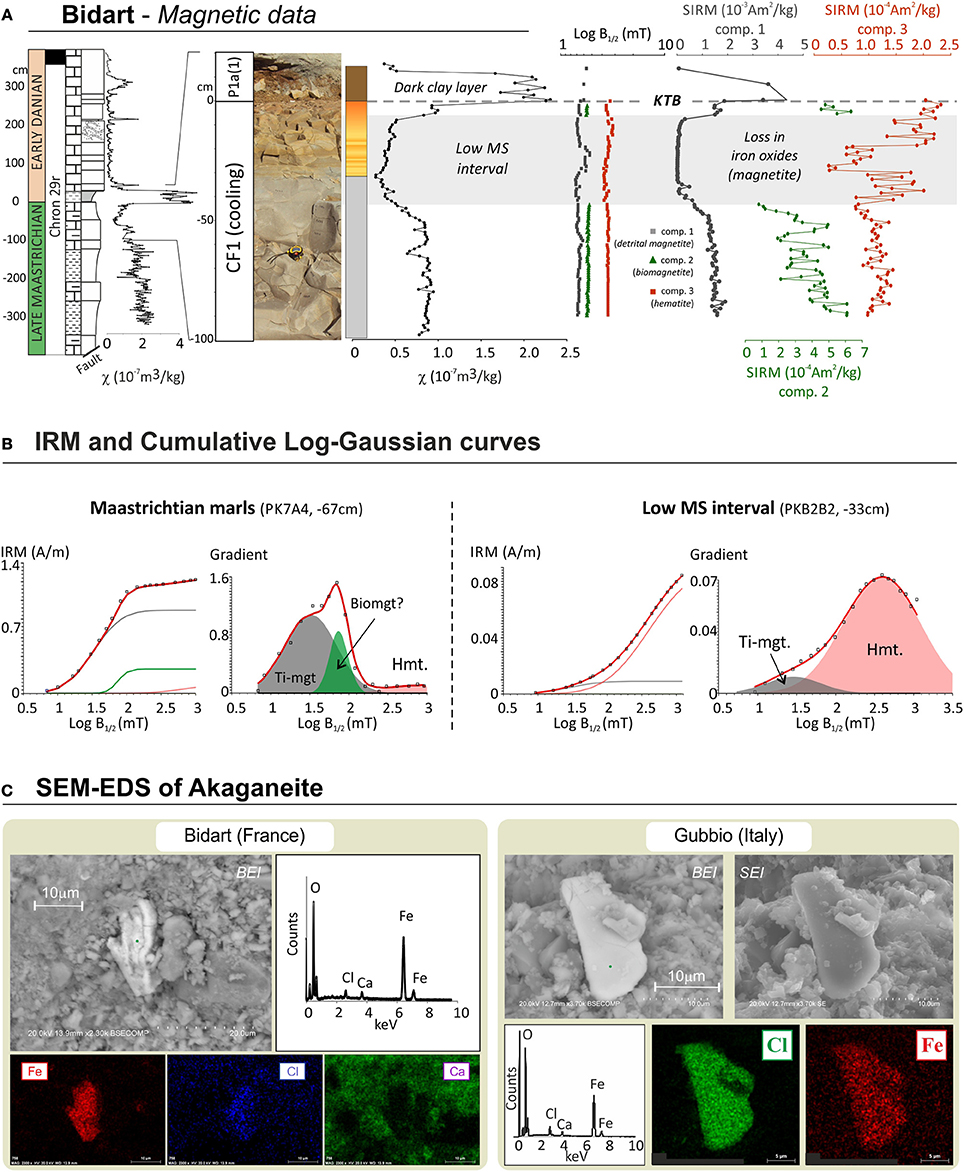
Figure 1. (A) Magnetic data (mass magnetic susceptibility (x) and Isothermal Remanent Magnetization parameters) of the Bidart section [modified from Font et al. (2011, 2014)]. KTB is the Cretaceous-Tertiary boundary. Log B1/2 (mT) is the mean coercivity of each magnetic component. SIRM corresponds to IRM values at saturation. Component 1 and 2 correspond to detrital and biogenic magnetite, respectively, whereas component 3 is probably hematite. The low MS interval is featured by a loss in detrital and biogenic magnetite. (B) Examples of Isothermal Remanent Magnetization (IRM) curves and corresponding Cumulative Log-Gaussian curves of one sample from the lower Maastrichtian marls (PKB7A4) and one sample from the low MS interval (PKB2B2); (C) Scanning Electron Microscopic photographs (SEM) and Energy Dispersive Spectra (EDS) of akaganeite crystals found at Bidart and Gubbio. BEI and SEI correspond to Back-scattered and Secondary Electron Image, respectively. Compositional mapping show that Fe and Cl are associated to the akaganeite crystal, whereas Ca is only observed in the matrix.
However, at Bidart section the presence of biogenic magnetite (which is particularly sensitive to reductive dissolution) in-between the impact clay and the low MS zone (Abrajevitch et al., 2014) and preservation of primary carbon isotopic signature (Font et al., 2014) are incompatible with the downward infiltration model of Lowrie et al. (1990). More likely, the loss of iron oxides in the low MS zone was due to unusual atmospheric and oceanic chemistry, probably related to high influx of CO2 and sulfuric acid aerosols from the Deccan Traps (Chenet et al., 2005; Self et al., 2006). The decrease in the content of detrital magnetic minerals may reflect modification of source sediments due to acidic weathering. Modeling of on-land magnetite dissolution suggests that at rainwater pH value of 4.6 (lower than present day pH of ~5.6) more than 90% of detrital magnetite could have been dissolved during transport from the source to deposition site (Font et al., 2014). The disappearance of biogenic magnetite in the low MS interval at Bidart and Gubbio (Abrajevitch et al., 2014) marks environmental change in marine environment, with the onset of conditions that were unfavorable either to magnetotactic bacteria or to preservation of their fossil magnetosomes.
In addition to the loss of detrital and biogenic magnetite, the low MS interval is characterized by the presence of an unusual mineral akaganeite—a chlorine-bearing iron oxyhyhydroxide (β -Fe2(OH)3Cl) that has hollandite-type (tunnel-like) structure with the Cl ions residing in the tunnels. Akaganeite has been only observed under SEM microscope in two samples located within the low MS interval, but has never been identified in samples from the underlying Maastrichtian marls, the KT boundary nor in the Danian limestones (Font et al., 2011). Akaganeite is rare in nature and is generally found in environments that are rich in Fe(II) and Cl (Reguer et al., 2007; Remazeilles and Refait, 2007, 2008; Yue et al., 2011), such as like hypersaline lakes (Emmerich et al., 2012), iron sulfide-rich environments (Bibi et al., 2011), fumaroles (Johnston, 1977), corroded steel (Li et al., 2008) and weathered meteorites (Bland et al., 1997). Synthetic and natural akaganeite precipitated from aqueous solutions usually forms as bundles of nanometer-scale particles with spindle- or cigar-shaped morphology (Cornell and Schwertmann, 2003; Yue et al., 2011; Zhang and Jia, 2014). In contrast, akaganeite identified by SEM in Bidart and Gubbio sections occurs as large (~5–40 μm) isolated crystals with unusual plate-like, granular and semi-hexagonal morphologies. Chlorine-bearing particles of similar size range (2–20 μm) and morphology are presently observed in aerosols of the Masaya volcano in Nicaragua (Moune et al., 2010). Such similarity suggests that akaganeite particles of Gubbio and Bidart sections are also of volcanic origin, likely formed in the Deccan Traps volcanic plume. In the eruption plume that was expanding vertically into the atmosphere, highly soluble chlorine-rich gasses (NaCl, KCl, FeCl2…) rapidly reacted with iron in the presence of water vapor to form akaganeite according to the following equation: 2Fe2+(aq) + 2Cl−(aq) +3/2H2O+3/4O2 = β-Fe2(OH)3Cl(s) (Remazeilles and Refait, 2007). Volcanic ash, including akaganeite, was then transported through the stratosphere (Kaminski et al., 2011) and become incorporated into marine sediments (Figure 2).
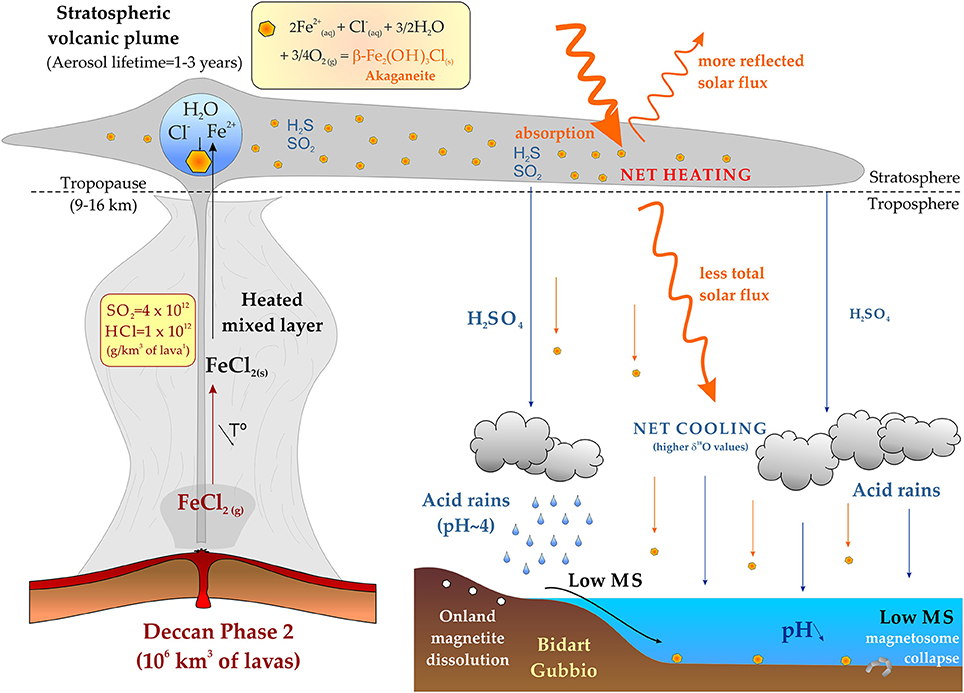
Figure 2. Model of iron oxides dissolution and akaganeite deposition by evoking acid rain and ocean acidification and interactions between volcanic aerosols and the high atmosphere (modified from Font et al., 2014).
Summary
Rock magnetism is an efficient technique for detecting the variations in composition, concentration and grain-size of the mineral magnetic fraction in sedimentary sequences. Distribution patterns of environmentally sensitive biogenic magnetite in the Bidart section indicate that iron oxide dissolution event, identified by the characteristically low MS values of the latest Maastrichtian sediments, predates the deposition of the KT boundary clay, and thus is not causally related to the asteroid impact. The exclusive presence of akaganeite, a mineral known to form in volcanic plumes, within the low MS intervals suggests instead a causal link to the contemporaneous Deccan Phase-2 eruption episode. Acid rains and ocean acidification resulting from the release of large volumes of greenhouse gasses during the eruptions can account for the loss of magnetic phases. We hypothesize that the association of iron oxide dissolution features and the presence of akaganeite in marine sediments might represent valuable indicators of volcanism-related ocean acidification events in geologic records.
Conflict of Interest Statement
The authors declare that the research was conducted in the absence of any commercial or financial relationships that could be construed as a potential conflict of interest.
Acknowledgments
Funding was provided by FCT (ref. PTDC/CTE_GIX/110205/2010). We thank Celia Lee and Ana Sousa for administrative supply, and Fabio Florindo for internal review. We are grateful to Quinsong Liu and Oscar Pueyo Anchuela for their constructive comments.
References
Abrajevitch, A., Font, E., Florindo, F., and Roberts, A. (2014). “The origin of white beds below the cretaceous-tertiary boundary revisited,” in AGU Fall Meeting, (San Francisco, CA).
Abrajevitch, A., and Kodama, K. (2011). Diagenetic sensitivity of paleoenvironmental proxies: A rock magnetic study of Australian continental margin sediments. Geochem. Geophys. Geosyst. 12, 1–18. doi: 10.1029/2010GC003481
Alegret, L., Kaminski, M. A., and Molina, E. (2004). Paleoenvironmental recovery after the Cretaceous/Paleogene boundary crisis: evidence from the marine bidart section (SW France). Palaios 19, 574–586. doi: 10.1669/0883-1351(2004)019<0574:PRATPB>2.0.CO;2
Apellaniz, E., Baceta, J. I., Bernaola-Bilbao, G., Núñez-Betelu, K., Orúe-Etxebarría, X., Payros, A., et al. (1997). Analysis of uppermost cretaceous–lowermost Tertiary hemipelagic successions in the Basque Country (western Pyrenees): evidence for a sudden extinction of more than half planktonic foraminifer species at the K/T boundary. Bull. Soc. Géol. France 168, 783–793.
Bibi, I., Singh, B., and Silvester, E. (2011). Akaganeite (beta-FeOOH) precipitation in inland acid sulfate soils of south-western New South Wales (NSW), Australia. Geochim. Cosmochim. Acta 75, 6429–6438. doi: 10.1016/j.gca.2011.08.019
Bland, P. A., Kelley, S. P., Berry, F. J., Cadogan, J. M., and Pillinger, C. T. (1997). Artificial weathering of the ordinary chondrite Allegan: implications for the presence of Cl- as a structural component in akaganeite. Am. Mineral. 82, 1187–1197.
Bonté, P., Delacotte, O., Renard, M., Laj, C., Boclet, D., Jehanno, C., et al. (1984). An iridium rich layer at the cretaceous tertiary boundary in the bidart section (southern france). Geophys. Res. Lett. 11, 473–476. doi: 10.1029/GL011i005p00473
Chenet, A. L., Fluteau, F., and Courtillot, V. (2005). Modelling massive sulphate aerosol pollution, following the large 1783 Laki basaltic eruption. Earth Planet Sci. Lett. 236, 721–731. doi: 10.1016/j.epsl.2005.04.046
Chenet, A. L., Quidelleur, X., Fluteau, F., Courtillot, V., and Bajpai, S. (2007). K-40-Ar-40 dating of the main Deccan large igneous province: further evidence of KTB age and short duration. Earth Planet Sci. Lett. 263, 1–15. doi: 10.1016/j.epsl.2007.07.011
Cornell, R. M., and Schwertmann, U. (2003). The Iron Oxides: Structure, Properties, Reactions, Occurrences, and Uses. Weinheim: Wiley-VCH. doi: 10.1002/3527602097
Courtillot, V., Besse, J., Vandamme, D., Montigny, R., Jaeger, J. J., and Cappetta, H. (1986). Deccan flood basalts at the cretaceous tertiary boundary. Earth Planet Sci. Lett. 80, 361–374. doi: 10.1016/0012-821X(86)90118-4
Pubmed Abstract | Pubmed Full Text | CrossRef Full Text | Google Scholar
Ellwood, B. B., MacDonald, W. D., Wheeler, C., and Benoist, S. L. (2003). The K-T boundary in oman: identified using magnetic susceptibility field measurements with geochemical confirmation. Earth Planet Sci. Lett. 206, 529–540. doi: 10.1016/S0012-821X(02)01124-X
Emmerich, M., Bhansali, A., Losekann-Behrens, T., Schroder, C., Kappler, A., and Behrens, S. (2012). Abundance, distribution, and activity of fe(II)-oxidizing and fe(III)-reducing microorganisms in hypersaline sediments of lake kasin, southern russia. Appl. Environ. Microb. 78, 4386–4399. doi: 10.1128/AEM.07637-11
Pubmed Abstract | Pubmed Full Text | CrossRef Full Text | Google Scholar
Font, E., Fabre, S., Nédélec, A., Adatte, T., Keller, G., Veiga-Pires, C., et al. (2014). “Atmospheric halogen and acid rains during the main phase of Deccan eruptions: magnetic and mineral evidence,” in Volcanism, Impacts, and Mass Extinctions: Causes and Effects: Geological Society of America Special Paper 505, eds G. Keller and A. C. Kerr, 353–368. doi: 10.1130/2014.2505(18)
Font, E., Nédélec, A., Ellwood, B. B., Mirão, J., and Silva, P. F. (2011). A new sedimentary benchmark for the Deccan Traps volcanism? Geophys. Res. Lett. 38:L24309. doi: 10.1029/2011GL049824
Galbrun, B., and Gardin, S. (2004). New chronostratigraphy of the cretaceous-paleogene boundary interval at bidart (France). Earth Planet Sci. Lett. 224, 19–32. doi: 10.1016/j.epsl.2004.04.043
Gallala, N., Zaghbib-Turki, D., Arenillas, I., Arz, J. A., and Molina, E. (2009). Catastrophic mass extinction and assemblage evolution in planktic foraminifera across the cretaceous/paleogene (K/Pg) boundary at bidart (SW France). Mar. Micropaleontol. 72, 196–209. doi: 10.1016/j.marmicro.2009.05.001
Gertsch, B., Keller, G., Adatte, T., Garg, R., Prasad, V., Berner, Z., et al. (2011). Environmental effects of Deccan volcanism across the cretaceous-tertiary transition in meghalaya, india. Earth Planet Sci. Lett. 310, 272–285. doi: 10.1016/j.epsl.2011.08.015
Johnston, J. H. (1977). Jarosite and akaganeite from white island volcano, new-zealand - an x-ray and mossbauer study. Geochim. Cosmochim. Acta 41, 539–544. doi: 10.1016/0016-7037(77)90291-5
Kaminski, E., Chenet, A. L., Jaupart, C., and Courtillot, V. (2011). Rise of volcanic plumes to the stratosphere aided by penetrative convection above large lava flows. Earth Planet Sci. Lett. 301, 171–178. doi: 10.1016/j.epsl.2010.10.037
Keller, G., Adatte, T., Bhowmick, P. K., Upadhyay, H., Dave, A., Reddy, A. N., et al. (2012). Nature and timing of extinctions in cretaceous-tertiary planktic foraminifera preserved in Deccan intertrappean sediments of the krishna-godavari basin, india. Earth Planet Sci. Lett. 341, 211–221. doi: 10.1016/j.epsl.2012.06.021
Keller, G., Bhowmick, P. K., Upadhyay, H., Dave, A., Reddy, A. N., Jaiprakash, B. C., et al. (2011). Deccan volcanism linked to the cretaceous-tertiary boundary mass extinction: new evidence from ongc wells in the krishna-godavari basin. J. Geol. Soc. India 78, 399–428. doi: 10.1007/s12594-011-0107-3
Kruiver, P. P., Dekkers, M. J., and Heslop, D. (2001). Quantification of magnetic coercivity components by the analysis of acquisition curves of isothermal remanent magnetisation. Earth Planet Sci. Lett. 189, 269–276. doi: 10.1016/S0012-821X(01)00367-3
Li, Q. X., Wang, Z. Y., Han, W., and Han, E. H. (2008). Characterization of the rust formed on weathering steel exposed to qinghai salt lake atmosphere. Corros. Sci. 50, 365–371. doi: 10.1016/j.corsci.2007.06.020
Lowrie, W., Alvarez, W., and Asaro, F. (1990). The origin of the white beds below the cretaceous tertiary boundary in the gubbio section, italy. Earth Planet Sci. Lett. 98, 303–312. doi: 10.1016/0012-821X(90)90032-S
Moune, S., Gauthier, P. J., and Delmelle, P. (2010). Trace elements in the particulate phase of the plume of masaya volcano, nicaragua. J. Volcanol. Geoth. Res. 193, 232–244. doi: 10.1016/j.jvolgeores.2010.04.004
Reguer, S., Dillmann, P., and Mirambet, F. (2007). Buried iron archaeological artefacts: corrosion mechanisms related to the presence of Cl-containing phases. Corros. Sci. 49, 2726–2744. doi: 10.1016/j.corsci.2006.11.009
Remazeilles, C., and Refait, P. (2007). On the formation of beta-FeOOH (akaganeite) in chloride-containing environments. Corros. Sci. 49, 844–857. doi: 10.1016/j.corsci.2006.06.003
Remazeilles, C., and Refait, P. (2008). Formation, fast oxidation and thermodynamic data of Fe(II) hydroxychlorides. Corros. Sci. 50, 856–864. doi: 10.1016/j.corsci.2007.08.017
Robertson, D. J., and France, D. E. (1994). Discrimination of remanence-carrying minerals in mixtures, using isothermal remanent magnetization acquisition curves. Phys. Earth Planet. Int. 82, 223–234. doi: 10.1016/0031-9201(94)90074-4
Self, S., Widdowson, M., Thordarson, T., and Jay, A. E. (2006). Volatile fluxes during flood basalt eruptions and potential effects on the global environment: a Deccan perspective. Earth Planet Sci. Lett. 248, 518–532. doi: 10.1016/j.epsl.2006.05.041
Thibault, N., and Gardin, S. (2007). The late Maastrichtian nannofossil record of climate change in the south atlantic DSDP Hole 525A. Mar. Micropaleontol. 65, 163–184. doi: 10.1016/j.marmicro.2007.07.004
Yue, J., Jiang, X. C., and Yu, A. B. (2011). Experimental and theoretical study on the beta-FeOOH nanorods: growth and conversion. J. Nanopart. Res. 13, 3961–3974. doi: 10.1007/s11051-011-0320-4
Keywords: akaganeite, Deccan volcanism, mass extinction, acidification, rock magnetism
Citation: Font E and Abrajevitch A (2014) Paleoenvironmental signature of the Deccan Phase-2 eruptions. Front. Earth Sci. 2:23. doi: 10.3389/feart.2014.00023
Received: 18 August 2014; Paper pending published: 05 September 2014;
Accepted: 09 September 2014; Published online: 25 September 2014.
Edited by:
Kenneth Philip Kodama, Lehigh University, USAReviewed by:
Oscar Pueyo Anchuela, Universidad de Zaragoza, SpainQingsong Liu, Chinese Academy of Sciences, China
Copyright © 2014 Font and Abrajevitch. This is an open-access article distributed under the terms of the Creative Commons Attribution License (CC BY). The use, distribution or reproduction in other forums is permitted, provided the original author(s) or licensor are credited and that the original publication in this journal is cited, in accordance with accepted academic practice. No use, distribution or reproduction is permitted which does not comply with these terms.
*Correspondence: Eric Font, IDL-FCUL, Instituto Dom Luís, Faculdade de Ciências, Universidade de Lisboa, Edifício C8-8.3.22, Campo Grande, 1749-016 Lisboa, Portugal e-mail: font_eric@hotmail.com