- 1School of Archaeology, University of Oxford, Oxford, United Kingdom
- 2School of Archaeology, Shandong University, Jinan, China
- 3Department of Biology, University of Oxford, Oxford, United Kingdom
- 4Institute of Cultural Heritage, Shandong University, Qingdao, China
- 5Agricultural Technology Centre, Linyi Municipal Bureau of Agriculture and Rural Affairs, Linyi, China
- 6Institute of Genetics and Developmental Biology, Chinese Academy of Sciences, Beijing, China
- 7Archaeology Lab and Preservation Research Institute, China National Centre for Archaeology, Beijing, China
Rice (Oryza sativa L.) is an important cereal crop across East, South and Southeast Asia, from prehistory to today, and grows in a range of ecological conditions, from rainfed upland to deep water. Previous research on early rice in the Lower Yangtze River basin (LYRB) suggested shifts back and forth over time between wet and dry field conditions, and some application of animal dung and/or human feces as fertilizer. In this study, stable carbon and nitrogen isotope analysis was conducted on grains from rice of heritage landraces and modern varieties grown in a glasshouse pot experiment with three watering levels and two manuring levels, and from recent rice fields in China and South Korea including paddy and dry conditions, and with variable manuring histories. The pot experiment results indicate that there is a negative correlation between the δ13C values and watering levels in one heritage accession and three modern accessions; in the remaining two heritage accessions and one modern accession, a similar trend in δ13C values is observed between watering levels. The rice field results show that, similarly, the δ13C values of rice from paddy fields were significantly lower than those from dry fields. The results from the pot experiment also indicate that both watering and manuring tend to increase the δ15N values of rice grains, while the field samples show that intensive manuring in initial growing seasons does not have a consistent effect on rice δ15N values. Overall, stable carbon and nitrogen isotope values of rice grains appear to be useful indicators of water status, with potential in combination to disentangle watering and manuring practices.
1 Introduction
Stable carbon and nitrogen isotope analysis of cereal grains and pulse seeds has been proved a useful technique for indicating crop water status and soil nitrogen composition in modern experiments and farming systems in Europe, western Asia and west Africa (e.g., Araus et al., 1997; Bogaard et al., 2007; Fraser et al., 2011; Wallace et al., 2013; Styring et al., 2016, 2017, 2019a,b; Christensen et al., 2022; Dong et al., 2022). This method can be used to investigate past crop husbandry practices, including water management and manuring (Araus et al., 1997, 1999; Ferrio et al., 2005; Bogaard et al., 2007; Fraser et al., 2011; Aguilera et al., 2012; Bogaard et al., 2013; Araus et al., 2014; Styring et al., 2016, 2017; Stroud et al., 2021; Nayak et al., 2022) and to inform palaeodietary reconstruction (Fraser et al., 2013; Nitsch et al., 2017; Styring et al., 2017; Vaiglova et al., 2021). Most applications so far are to cereal crops other than rice (Oryza sativa L.), such as wheats (e.g., Triticum aestivum L.), barleys (e.g., Hordeum vulgare L.), and millets [Panicum miliaceum L.; Setaria italica (L.) P. Beauv.] (e.g., Dong et al., 2022; Li et al., 2022; Tian et al., 2022).
Rice is an important annual cereal crop cultivated across East, South, and Southeast Asia, from prehistory until today. It adapts to various environmental conditions, from deep water to rainfed upland systems (Fuller et al., 2011). These different regimes have contrasting implications for the ecology of early rice-growing societies (Weisskopf et al., 2015). For example, to cope with various water levels and management, fields developed in size and structure. The analysis of stable carbon and nitrogen isotope values of rice to investigate rice growing practices such as watering and manuring regimes can be traced back to the 1990s (Yoneyama et al., 1990) and has recently been applied to modern and archaeological studies (Kaushal et al., 2016; Yoneda et al., 2019; Shoda et al., 2021; Nayak et al., 2022; Wang et al., 2022). The relationship between rice stable carbon and nitrogen isotope values and growing conditions, however, requires more experimental investigation to assess cause and effect, as well as to compare across different rice varieties. The aim of the present study is to evaluate stable carbon and nitrogen isotope values in modern experimentally grown rice as potential indicators of watering and/or manuring regimes.
1.1 Ecological and archaeological background
There are three wild rice species distributed in modern China: O. rufipogon Griff., O. officinalis Wall. ex Watt and O. meyeriana (Zoll. & Moritzi) Baill. Only O. rufipogon is discussed here as it is the ancestor of O. sativa ssp. japonica, which was domesticated in China (Fuller and Qin, 2009; Fuller et al., 2010; Lv, 2013; Gross and Zhao, 2014; Choi et al., 2017; Ishikawa et al., 2020). O. rufipogon is a perennial that flourishes in wet environments (Pang and Chen, 2002). Sano et al. (1980) found that, when the water level was stable, wild rice populations were predominantly perennials, depending on vegetative propagation from their roots; in temporary swamp habitats, plants relied instead on grains for sexual propagation, following an annual habit. Fuller and Qin (2009) suggested that wild rice stands under more water–stressed conditions produced more grains. Consequently, water availability is a critical factor in wild rice reproductive strategies. The domestication of rice fundamentally changed the life cycle of this plant as domesticated rice is an annual dependent on seed production, while its ancestor is perennial and relies on vegetative or sexual propagation depending on water availability. Overall, assessing the water status of early rice informs our understanding of how human management shaped rice domestication.
The Lower Yangtze River basin (LYRB) (Figure 1) is a center of early rice domestication (Zheng and Jiang, 2007; Fuller et al., 2009). Abundant rice remains have been found at multiple Neolithic sites in this region, ranging from the Shangshan culture (10,000–8,000 BP) to the Guangfulin culture (4,000–3,800 BP) (e.g., Liu et al., 2007; Guo, 2014; Wu et al., 2014; Ma et al., 2016; Zhao, 2018; Zhang et al., 2024). The earliest evidence of irrigation can be traced back to the Early Hemudu culture (around 6,700 BP to 6,500 BP) at the Shi'ao site, with ridges and water inlets/outlets forming paddy fields (Wang et al., 2023) (Supplementary Table 1).
Although early rice remains from the LYRB are generally presumed to represent paddy rice, there is evidence of variation in wetness between and within sites. Phytoliths of rice and associated weeds in rice fields can be classified as fixed types or sensitive types; fixed types appear regardless of the wetness of fields, while sensitive-type phytoliths decrease in proportion when the environment is dry. Thus, the ratio of fixed: sensitive types of phytoliths should reflect the degree of rice field wetness (Weisskopf et al., 2015). Phytolith analysis on Neolithic sites suggested that rice was grown in paddy habitats similar to wild rice at Tianluoshan (6,800–6,500 BP), while drier conditions similar to modern rainfed fields were inferred at Caoxieshan (5,950–5,700 BP), suggesting water control to encourage rice grain production. The drier conditions (drainage to control water level) at Caoxieshan might be realized through small size fields (usually < 10 m2) combined with facilities such as deeply dug reservoirs and channels (Fuller and Qin, 2009). This pattern of paddy fields was commonly found in sites around the Taihu Lake (Ding, 2004, 2010; Qiu et al., 2013, 2014; Gao, 2017; Liu, 2021). At the later site of Maoshan (5,000–4,300 BP), rice again grew under wet conditions in large-scale paddy field systems, which were framed by regular intersecting channels and ridges (Zheng et al., 2014). Alternating wet-dry conditions have been inferred from soil micromorphological analysis at Maoshan (Zhuang et al., 2014).
In addition to watering, manuring regimes of archaeological rice fields have also been considered in previous studies, primarily using geoarchaeological methods. Cao (2008) found that the surface soils of paddy fields from the site of Chuodun had similar values for SOM (soil organic matter), TN (total nitrogen), and TK (total potassium) but higher TP (total phosphorus) and AP (available phosphorus) compared to modern paddy fields, suggesting a long-term application of animal residues as manure. Soil micromorphological analysis provides evidence of animal dung and human feces as fertilizers, as shown at Maoshan (Zhuang et al., 2014). In addition, fungal spores have been interpreted to indicate soil disturbance, erosion and dung decomposition (Zong et al., 2007). Growth experiments can provide novel insights into the impact of factors like watering and manuring on the δ13C and δ15N values of different rice accessions, which directly show the growing conditions of rice plants.
1.2 The carbon cycle in paddy vs. dryland rice fields
Carbon has two stable isotopes, 12C and 13C. The ratio between the two stable isotopes is expressed as δ13C values (Equation 1), where Rsample and Rstandard are the abundance ratios (13C/12C) of the sample and the standard (PDB), respectively (Farquhar et al., 1982). Carbon isotope discrimination (Δ13C) directly expresses the consequence of photosynthesis and is widely used as an indicator for water use efficiency (WUE) of plants (Farquhar and Richards, 1984). Δ13C can be expressed using δ13C values of atmospheric CO2 and of plant (Equation 2) (Farquhar et al., 1982).
In plant physiology, plant growth (the accumulation of dry matter) mainly depends on photosynthesis. The majority of carbon in plants comes from immobilized CO2 from the air (Farquhar et al., 1989; Xiao, 2017). When there is sufficient water supply, 12CO2 is preferentially fixed through photosynthesis over 13CO2, resulting in a low abundance of 13C in the fixed CO2. However, when water availability is limited, the fractionation of 13CO2 is less significant and the δ13C value increases (Farquhar and Richards, 1984; Farquhar et al., 1989; Ehleringer et al., 1993; Wallace et al., 2013; Styring et al., 2016). This is because in a dry environment, stomata in leaves will close to reduce evaporation. Plants then preferentially take up CO2 from inter–cellular space, leading to the remaining dissolved CO2 in cells to become enriched in 13C. In addition, lower stomatal conductance is disadvantageous for external CO2 to enter leaf cells. Thus, paddy rice grown in waterlogged conditions is expected to have lower δ13C values than dry rice, as previously observed in west Asian cereals like wheats and barleys (e.g., Araus et al., 1997, 1999; Wallace et al., 2013, 2015; Bogaard et al., 2016; Styring et al., 2016).
Previous studies have observed a relationship between the stable carbon isotopic composition of rice and water availability (see citations in Supplementary Table 2). For example, Gao et al. (2018) reported pot experiments showing that, under water stress, rice leaves of both japonica landrace Qiuguang and heritage indica landrace Qishanzhan had higher δ13C values than leaves of better watered plants. Furthermore, high water stress was associated with a higher δ13C value compared with low and medium water stress conditions.
Kaushal et al. (2016) analyzed rice grains in India and proposed that, for the same genotype of rice, higher δ13C values were found in dry season rice compared to wet season rice. This could be a result of both a drop in relative humidity and increase in water salinity (as sea water incursion led to the enrichment of the heavier isotope, 13C). Kaushal and Ghosh (2018) also found that δ13C values reflect relative humidity. They tested 105 rice genotypes grown in distinct topographic settings and climatic zones in the southwest monsoon season in India. A significant negative trend was found between δ13C values in rice grains and relative humidity using linear regression. Similar results were observed by Nayak et al. (2022), such that rice grains from the South Deccan (a semi-arid region) had higher δ13C values than those from East India (a monsoon region).
Different rice genotypes have different water use efficiency (WUE), shown by Δ13C values of rice leaves (Impa et al., 2005) or various rice plant organs (such as panicles, though grains were not tested) (Kano-Nakata et al., 2014). Centritto et al. (2009) studied leaves of five upland-adapted cultivars and three lowland cultivars and found greater variations in Δ13C values across genotypes than across water conditions. These findings showed that variations between rice genotypes/accessions also require consideration when interpreting carbon isotopic values of archaeological rice samples. The most direct inferences on water status are accessible from variation within local rice populations in archaeobotanical assemblages.
1.3 The nitrogen cycle in paddy vs. dryland rice fields
Nitrogen has two stable isotopes, 14N and 15N. The ratio between the two stable isotopes is expressed as δ15N values (Equation 3), where Rsample and Rstandard are the abundance ratios (15N/14N) of the sample and the standard (AIR), respectively (Robinson, 2001).
Nitrogen is the most important mineral nutrient for rice. It participates in the production of a series of important substances, such as nucleic acids, amino acids, phospholipids, chlorophyll and endogenous hormones (Hu et al., 2017). Inorganic nitrogen is the main nitrogen source for plants but only accounts for 1%−2% of the total nitrogen in soils. Apart from a small proportion of organic compounds like amino acids, most organic nitrogen is transformed into inorganic nitrogen by microbes in soils before uptake by plants (Hu et al., 2017). Plants mainly take up two forms of inorganic nitrogen: ammonium (), produced by biological N2 fixation (nitrogen-fixing microorganisms convert N2 into ammonia, which reacts with water and forms ammonium) and mineralization/ammonification (breakdown of complex organic matter molecules into monomeric organic nitrogen compounds and ammonium), and nitrate (), produced by nitrification (conversion of ammonium into nitrate by nitrifying bacteria) (Denk et al., 2017).
The transformations of nitrogen influence nitrogen isotope fractionation in soils and thus the nitrogen isotope composition of plants (Hedges and Reynard, 2007). In a comparative study of upland crops and paddy rice, Lim et al. (2015) presented a model in which upland vegetables (cabbage, pepper, onion, and garlic) take up N mainly through ammonification and nitrification processes, with nitrate () as the main N species. Anaerobic waterlogged conditions differentiate the nitrogen cycle of paddy field rice from that of upland crops because they restrict the nitrification process and nitrate production and increase the rate of denitrification. Denitrification converts nitrate to N2, causing the remaining nitrate to be enriched in 15N. Consequently, ammonium becomes the dominant N species in paddy rice, which is directly absorbed by plants. 15N-enriched nitrate can also be utilized by paddy rice as a secondary N source. Lim et al. (2015) suggested that these sources together make the δ15N values of paddy rice (c. +5.6–15.5‰) much higher than those of upland plants (c. +0.3–6.4‰), based on isotopic analysis of homogenized aboveground plant parts. Similarly, Yoneda et al. (2019) found that paddy rice from experimental fields in wetlands had around 2.5‰ higher δ15N values than pot-grown dry rice. The contribution of biological nitrogen fixation (BNF) by diazotrophs such as cyanobacteria and Azolla to contrasting δ15N values in dryland crops and paddy rice requires further investigation, but it is clear that BNF contributes to the productivity of paddy fields (De, 1939; Ley et al., 1959; Yoshida and Ancajas, 1973; Ladha et al., 1983; Ladha and Reddy, 2003).
Another agronomic factor that potentially increases the δ15N values of rice is the application of organic manure. Chung et al. (2017) observed that organic rice had δ15N values above +5‰, while rice grown with synthetic fertilizers had lower values. Yun et al. (2011) found that pig manure significantly increased the δ15N values of paddy rice compared to those grown with synthetic fertilizer and hairy vetch (Vicia villosa Roth.), though the differences in δ15N values decreased over time.
Fabaceae forms an important part of green manure applied to modern rice cultivation, such as Astragalus sinicus L., Vicia faba L., and Vicia sativa L. (e.g., Xiao, 1963; Huang and Shi, 1964; Yuan et al., 2011; Xi, 2014). Legumes usually have δ15N values around 0‰ and the application of such green manure to crops like wheat, barley and potatoes was reported to reduce crops' δ15N values compared to those of the conventional group and the group receiving pig manure (Laursen et al., 2013). Long-term fertilization would not change the δ15N values of legumes unless there was rather high level of organic manure input over long time (Fraser et al., 2011). Another factor that may dramatically increase the δ15N values of legumes is using organic manure with high δ15N values, such as seabird guano (Szpak et al., 2014). In the LYRB during the Neolithic, only a few Fabaceae remains were found at some of the archaeological sites (Qiu, 2015; Gao, 2017) and whether they were used as green manure in rice cultivation is little discussed. The organic manured paddy rice samples in this study include rice grown both with and without green manure of Vicia faba (see below), and can be used as a reference when comparing with archaeological samples in the future.
1.4 Previous work on archaeobotanical rice stable isotopes in East and South Asia
There have been various recent applications of stable carbon and nitrogen isotope analysis to modern rice in agronomic research (see citations in Supplementary Table 2). These isotopic values have been measured to assess the growing environment (Liu et al., 2012; Zhang et al., 2014), to quantify root interaction between rice and weeds (Gealy and Fischer, 2010; Gealy and Gealy, 2011), to evaluate planting methods (Cai and Fu, 1985; Li, 2012; Zhao, 2017), to estimate yields (Laza et al., 2006; Wang, 2016), to select featured landraces (Xiong et al., 2017), to authenticate organic crops (Chung et al., 2017), and/or to differentiate their geographical origin (Korenaga et al., 2010; Chen et al., 2016).
There have as yet been few archaeobotanical studies using stable carbon and nitrogen isotope analysis of rice to infer management (e.g., Kaushal et al., 2019; Shoda et al., 2021; Nayak et al., 2022) or to inform palaeodietary reconstruction (e.g., Wu, 2017). Research in China has mainly focused on the LYRB (Gupta et al., 2013; Wu, 2017) and the Central Plains (Tao et al., 2022a,b; Wang et al., 2022; Tao et al., 2023), two important regions where changes in farming practices and social complexity occurred. In the LYRB, studies have been conducted at the site of Tianluoshan, where the process of rice domestication was ongoing and foraged resources played a major role in human diet (Fuller et al., 2009). Gupta et al. (2013) reported higher δ15N values of bulk samples of rice husks (+7.4‰, n = 1, ca. 4,900 BC) and one charred grain (+4.1‰, 4,700–4,500 BC) at Tianluoshan compared to modern chemical fertilized materials collected from the same province (−3.8‰ and +0.8‰, respectively) and suggested the possible use of human waste as manure. However, a baseline of unmanured modern rice from the local environment was lacking, and the reduction in δ15N values of modern grains due to mineral fertilizer (Yoneyama et al., 1990) was not taken into account. The inference of manuring at Neolithic Tianluoshan therefore requires further investigation.
Wu (2017) analyzed charred rice grains from layer 8 (4,800 BC) to layer 4 (4,500 BC) at Tianluoshan, with three to five single grains analyzed for each layer to complementing the previous approach based mostly on husk remains. It was observed that more than half of the rice grains yielded δ15N values higher than +4‰, as was the case for other plant remains, such as water chestnut, south sour jujube, foxnut, acorn, plum and persimmon. This finding suggested that the commonly used δ15N value of +0–4‰ for plant foods as a baseline in dietary analysis was too low for archaebotanical materials from this region.
Similar challenges of identifying relevant baselines to interpret isotopic data were encountered in studies of rice grains from northern China. These studies (Tao et al., 2022b; Wang et al., 2022; Tao et al., 2023) argued that rice was grown in well-watered, fertile conditions in the Central Plains from around 2,600 BC to 221 BC based on δ13C and δ15N values, but their baselines for watering and manuring levels were derived from experiments with wheat, barley and lentil in western Eurasia (Bogaard et al., 2013; Wallace et al., 2013). Whether or not it is appropriate to transfer thresholds of dryland crops in western Eurasia to rice in East Asia remains open to question. Tao et al. (2023) cited values of rice grains from wetter eastern India and drier South Deccan in Nayak et al. (2022) but used baselines for wheats in Wallace et al. (2013) to interpret δ13C values of rice remains from northern China as well-watered plants. Furthermore, discussion of manuring regimes in these studies needs reconsideration. For paddy rice, the δ15N values cannot be simply explained as the result of manuring effects if paddy fields naturally have relatively high δ15N values caused by denitrification, which may obscure any additional effect of manuring.
Recent work was also conducted on charred rice grains from six sites in the Korean peninsula dating from ca. 1,100 BC to 400 AD (Shoda et al., 2021). The consistency of δ13C and δ15N values through time led the authors to question the prevailing hypothesis that there was a shift from dry to wet cultivation. The δ15N values of rice were consistently higher than those of dry crops during this 1,500–year period. The authors suggested that the elevated δ15N values of rice were due to denitrification in paddy field conditions and therefore that paddy rice was grown throughout this period.
Kaushal et al. (2019) applied their observation that modern dry season rice grains have higher δ13C values than wet season rice grains to interpret the δ13C values of archaeological charred rice grains from seven sites in India belonging to the Harappan and other regional cultures, and inferred changes in climatic (dry/wet) conditions. The authors argued that high δ13C values under low precipitation conditions may also reflect low crop productivity as lower stomatal conductance in dry conditions can be related to a decrease in the influx of CO2 required for photosynthesis. The lower yield of rice could lead to reduced population density and a deurbanized phase in the late Harappan culture.
Nayak et al. (2022) analyzed archaeological charred rice grains from monsoonal East India and the western arid Deccan Plateau (where irrigation channels have been discovered at the site of Paithan) dated from around 1,250 BC to 550 AD. Using modern experimental charred rice grains from both regions as a reference, it was suggested that rice grew in a well-watered environments with varying intensity of manure throughout the study period at both the eastern and the western sites.
1.5 Aims of the study
Whereas rice remains preserved archaeologically are usually charred grains and spikelet bases, or uncharred/waterlogged spikelet bases (e.g., Zheng and Jiang, 2007; Fuller et al., 2009), previous agronomic studies of modern rice isotope values have focused on other plant parts such as roots, shoots and leaves (see citations in Supplementary Table 2). As a result, these modern rice isotope values cannot be used for direct comparison with archaeobotanical values. This study presents a controlled growth experiment to investigate the relationships among rice δ13C and δ15N values and water and manuring treatments. In addition, samples from modern experimental fields complement those from pot experiments. Field experiments ensure realistic growth conditions and access to physiologically mature grains (Christensen et al., 2022) and scope to explore variability of stable isotope values within and between plots under the same treatment.
Three hypotheses are evaluated in this paper. First, we hypothesize that watering will reduce the δ13C values of rice grains. A second hypothesis is that paddy conditions will increase rice δ15N values compared with rice grown under dry conditions. Our third hypothesis is that organic manure will increase the δ15N values of rice compared with unmanured rice.
2 Materials and methods
2.1 Glasshouse pot experiment
A pot experiment was conducted in a glasshouse at the Department of Biology (previously Department of Plant Sciences), University of Oxford, UK. The environmental conditions of the glasshouse were 16 h of daylight and 8 h dark photoperiod, with a 30°C daytime temperature and a 28°C night-time temperature. The irradiance was 800 μmol of photons/m2 per second and the relative humidity was 80%.
The rice grains for this experiment were partly obtained from the collections of the International Rice Research Institute (IRRI), which provided three heritage domestic varieties (O. sativa japonica) from Southeastern Asia (referred to as HER001~HER003, see Table 1). Additional rice grains in this experiment were provided by Dr Xiangdong Fu of the Chinese Academy of Sciences; these will be referred to below as “modern” accessions. Modern rice accessions designated with a “W” refers to the “WuYunjing7” variety (MOD001 and MOD002) (Table 1) and “S” refers to the “Shao314” variety (MOD003 and MOD004) (Table 1). DEP1 (“dense and erect panicle1”) is a genetic locus responsible for determining panicle architecture (density of panicle, grain number per panicle and erectness of panicle), which in turn affects rice grain yields. The mutant DEP1 allele (dep1) (referred to as “dep1” lines), i.e., absence of the DEP1 allele, is a semidwarf form that exhibits increased rice grain yields compared with plants having the DEP1 allele (referred to as “DEP1” lines) (Table 1) (Huang et al., 2009). An initial trial experiment was conducted to determine suitable soil media, growing methods and organic manuring rates (see Supplementary material 1 “Initial trial experiment in 2018” for details).
On 25th May 2018, rice grains of all accessions (Table 1) were placed on moistened paper in petri dishes and moved to a germination cupboard. Germinated grains were planted in pots after 3 days (three grains per pot to allow for unsuccessful growth) and thinned out to one on the 18th of June. Three levels of watering treatment (low, medium/med, high; see Table 2) and two levels of manuring treatment (1% and 5% manuring level, i.e., 1% and 5% organic matter content in the mixed soil, respectively) were set in this experiment (Table 1). The 5% manuring level was only applied to heritage landraces due to the limited amount of grains of modern accessions. For each variety, 3 pots were grown under each watering treatment. Pots were randomized in terms of position within the glasshouse every 2 or 3 days to reduce potential edge effects and to decrease the influence of uneven artificial lighting in the glasshouse. A nutrient solution (see Supplementary material 1 “Initial trial experiment in 2018”) was applied every 2 weeks.
For all of the pots, a 2–3 cm depth water level above the soil was maintained in each tray until the 24th of June, when plants reached the 3-leaf stage. The 3-leaf stage is reached when the rice develops three true leaves (the first–developed leaf has a sheath but no blade, so is not counted), which indicates the end of the seedling stage and the start of the tillering stage (Zhejiang Agronomy University, 1980). Before the 3-leaf stage, rice seedlings grow by consuming the substances stored in the endosperm. Subsequently seedlings became autotrophy through photosynthesis (Shao et al., 2008).
Pots of medium and high watering level were moved to deep plastic trays (each containing 3 liters of water) to replicate paddy conditions (2–3 cm of water above soil). Low watering level pots remained in shallow trays and were kept at 100% soil saturation by observing daily whether there was remaining water in trays; if there was no water left, 170 g of water (per pot) was added to achieve saturation. This amount was the capacity of the growing medium (clay granules) in one pot.
A short-day treatment was introduced on the 25th of June (daylight from 9 am to 6.30 pm) to trigger flowering. Rice plants started flowering in August. By this time pots of medium watering level were moved back to shallow trays for saturated conditions, while high watering level pots remained in deep trays with paddy condition until harvest. The medium watering level was designed with reference to the phytolith analysis by Weisskopf et al. (2015), which suggested that drainage was applied to increase the production of grains in Neolithic fields of the LYRB. Ideally rice plants grown in paddy fields cycle through wet and dry conditions several times during the growth period to provide intermittent air ventilation for roots (Zhejiang Agronomy University, 1980). Rice plants (medium watering level) in this pot experiment were moved from wet to dry conditions once, at the flowering stage.
Rice was harvested from the 17th to 21st of September, when the grains were fully ripe. For heritage landrace HER001 (117264), one of three plants under the 5% manuring level, medium and high watering levels did not survive (Table 1). Growth information such as the length of the growing period, the height of rice replicates, water input and so forth is shown in the Supplementary Figures 1–3. Different from other heritage landraces, HER002 (128438) behaved similarly to modern varieties in terms of growth rate (Supplementary Figures 2A–C) while for plant height, heritage landraces were usually taller than modern varieties (Supplementary Figure 3). In addition, for all accessions, high watering level plants had shortest height while low watering level ones were tallest. Similar findings have been observed by Zhu et al. (2013).
2.2 Field collections
Rice samples were also collected from modern, mostly organic fields in South Korea and China from 2016 to 2020 (Table 3, Figure 1), to complement the glasshouse experiment and further test the hypotheses of this paper. These samples cover different water regimes (both paddy rice and dry rice) as well as various manuring regimes (different levels of organic manure and/or chemical fertilizer).
The South Korean paddy rice samples all derived from the same watering and manuring treatment at the same farm in Buyeo (126°50'29.6”E, 36°10'24.1”N, c. 40–60 m asl). Green manure crops (wheat, barley and hairy vetch) were grown every year from October to May before transplanting the rice seedlings. After rice ears fully emerged, water was drained from the fields and organic manure (“ear manure”) was applied using a tractor. The farm was divided into multiple fields and ten fields were randomly selected for sampling. For each field, several rice panicles were randomly collected by a sickle, which formed one sample.
Paddy rice samples were also collected in 2017 and 2019 at the Anyway farm, Shanghai (121°29'45.4”E, 31°13'34.6”N, c. 2 m asl), China. The Shanghai rice grew under the same paddy watering condition but were treated with five different manuring regimes, including a control group (no organic manure or chemical fertilizer added) and two types of organic manure applied at varying rates (see Table 3). In the same plot, the treatment remained the same between years. Plots averaged 0.33 ha in area and ranged from 0.23 to 0.63 ha. The layout of sampling plots with size and manuring regimes is shown in Supplementary Table 5. In addition, rice was rotated with Vicia faba as green manure each year after harvesting, starting from the winter of 2017. Thus, rice samples harvested in the autumn of 2017 (referred to as “Shanghai 2017 samples” below) can be viewed as an example of organic rice without green manure. For sampling, plots were each divided into a north, middle and south section and one sample (usually a clump of rice plants growing together) were collected from each section.
Paddy and dry rice samples collected from Linyi City (118°21'28.9”E, 35°6'14.4”N, c. 50–60 m asl), Shandong province of North China provided another important part of the field samples in this study. In 2019, dry rice samples were collected from three locations, with location 1 either nothing (control group) or applying chemical fertilizer and location 2 and 3 applying chemical fertilizer and organic manure (Table 3). In 2020, dry rice treated with chemical fertilizer and paddy rice treated with chemical fertilizer and organic manure were grown at the same farm, with two plots around 50 m away from each other. Both plots received chemical fertilizer in 2018. The Shandong 2020 dry rice suffered from heavy rainfall in the summer that year, and the ridge around the field impeded drainage of water and the maintenance of dry conditions. At harvest time, half of the field did not produce plants. Since the remaining field was limited in size, samples were randomly collected from plants which produced panicles. It is reasonable to suggest that these samples grew under conditions more similar to paddy rice than normal dry rice. With the exception of Shandong 2020 dry rice, the paddy and dry rice samples from Shandong followed the same sampling method as Shanghai rice samples.
2.3 Sampling and stable carbon and nitrogen isotope analysis
2.3.1 Pot experiment samples
For each harvested plant, all grains from one side of the panicle were harvested and 15–20 grains randomly sampled using a riffle box. If a plant had two tillers, thus two panicles, an extra sample (15–20 grains) was drawn from the second panicle. This resulted in a total of 79 samples. After sampling, the palea and lemma were removed from the rice grains by hand using a wooden dehusking tool. Grains were weighed before and after dehusking and then homogenized with a Spex 2,760 Freezer/Mill and stored in a refrigerator prior to isotopic analysis. The powdered samples were weighed into tin capsules, then run on a Sercon EA–GSL mass spectrometer at the Research Laboratory for Archaeology and the History of Art, University of Oxford.
δ13C and δ15N values were measured separately due to the relatively low nitrogen content in the samples. An internal ALANINE standard was used to obtain raw data and for drift correction. In each run, additional calibration and check standards were run every 10 samples. IAEA–CH6 and IAEA–CH7 were used for the calibration of δ13C values, and IAEA–P2 and internal ALANINE as check standards. IAEA–N1 and IAEA–N2 are used for the calibration of δ15N values, and IAEA–P2 and internal ALANINE as check standards. The standard uncertainties were calculated based on Szpak et al. (2017) and were 0.17‰ for δ13C values and 0.63‰ for δ15N values (Supplementary Table 6). Samples with unqualified results (e.g., unacceptable drift correction of runs) were reanalysed in 2023, using internal SEAL and ALANINE for calibration standards and IAEA-P2 and Leucine for check standards for both carbon and nitrogen isotope values. The standard uncertainties were calculated based on Szpak et al. (2017) and were 0.18‰ for δ13C values and 0.39‰ for δ15N values.
Plants with two panicles were analyzed separately for stable isotope values and then averaged. The δ13C value of sample ORY010 was around 3‰ higher than that of the other two samples of the same accession and the same treatment. This 3‰ difference is beyond the inter-treatment difference of all the other groups. In addition, the plant from which ORY010 was sampled only developed 15 grains while most of other plants usually have at least 30 grains (see Supplementary material 2). Thus, sample ORY010 was considered as an outlier and excluded for the following discussion. For assessing the effect of watering treatments on stable carbon and nitrogen isotope values, only plants grown with the 1% manuring level were included as they represented the complete low-medium-high watering levels for all accessions. For assessing the effect of manuring treatments on stable nitrogen isotope values, plants under the 1% and 5% manuring levels are included.
2.3.2 Modern field samples
Rice is usually planted in clumps in fields, from dozens of grains directly sowed or sprouts transplanted into a single hole. Rice plants from one clump, containing multiple panicles, are then harvested together. Each sample from the fields refers to one such clump. When selecting grains for stable isotope analysis, the number of panicles of each sample were first counted and the minimum number of panicles among each collection was selected as the number of panicles to sample (Table 3). Grains from one side of the panicles were threshed, mixed and split using a riffle box to obtain a random subset of 50 to 60 grains. These bulk samples were weighed, dehusked and homogenized into powder with a pestle and mortar or using Spex 2,760 Freezer/Mill. The following steps were the same as for pot experiment samples, with differences only in some of the standards used (Supplementary Table 6).
2.3.3 Statistical tests
All of the statistical analyses in this study were performed in R. The IQR method was applied to identify whether there were outliers in the dataset. This method is defined as the difference between the 25th percentile (Q1) and the 75th percentile (Q3) in a dataset. In the IQR analysis, cases more than 1.5 times the IQR from Q3 or < 1.5 times the IQR from Q1 are often classified as outliers (Tukey, 1977), making this analysis more robust to outliers than the standard deviation (Sullivan et al., 2021).
The distribution of the data was first checked using the Shapiro-Wilk test. For pot experiment samples, due to the small sample size and nonparametric distribution of the small datasets, a Spearman's rank-order correlation was applied to assess the relationship between watering levels and δ13C and δ15N values, respectively. To understand the effect of manuring on δ15N values, a Mann-Whitney U test was applied.
For field samples, the Shapiro-Wilk test was applied to check the normality of the data. The δ15N values of Bokashi 5 kg group from the Shanghai 2017 collection did not follow a normal distribution. When testing correlations between δ13C and δ15N values for each organic rice group (including the control group) from Shanghai (2017) and South Korea, the non-parametric Spearman rank correlation test was used while the remaining groups were analyzed using the Pearson correlation test. When combining organic groups together, the non-parametric Mann-Whitney U test was applied to assess the effect of watering and manuring on δ13C and δ15N values.
3 Results
3.1 Pot experiment
The δ13C values of the heritage accessions ranged from −30.2‰ to −27.8‰ at the 1% manuring level (Figure 2A, Table 4, Supplementary material 3). Modern accessions ranged from −30.9‰ to −28.1‰. Modern S-line accessions (MOD003, MOD004) were distributed within the same range of the values as heritage landraces, while W-line accessions (MOD001, MOD002) were around 1‰−1.5‰ lower than heritage accession values. The δ13C values of pot experiment samples showed greater variation between accessions than within accessions.
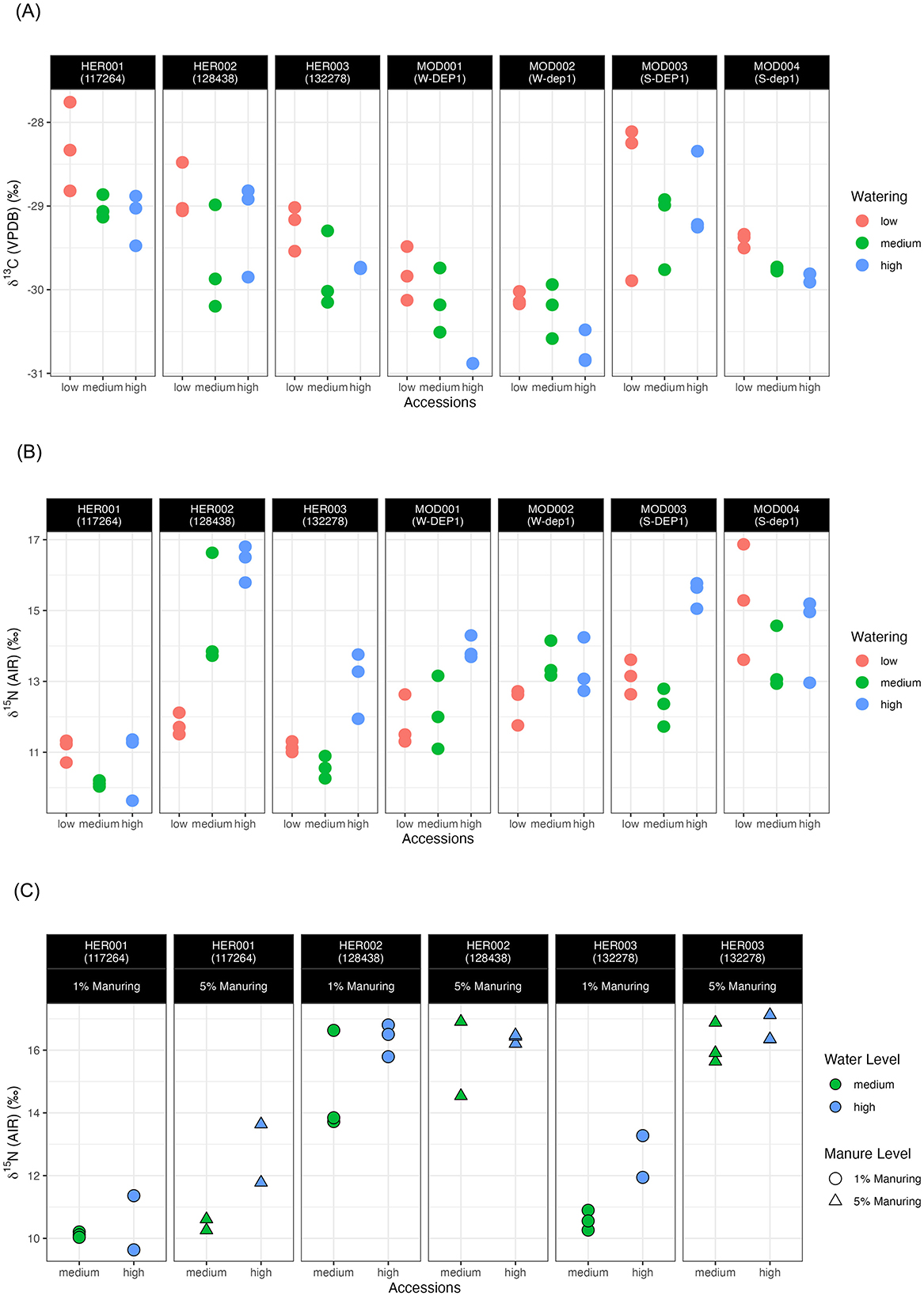
Figure 2. δ13C values (A) and δ15N values (B) of 1% manuring pot experiment samples; (C) δ15N values of heritage landraces grown under 1% and 5% manuring levels from the pot experiment.
For all accessions at the 1% manuring level, a tendency toward higher δ13C values can be observed at the low watering level compared with the medium and high watering levels. However, the δ13C values of HER002 (128438) and MOD003 (S-DEP1) increase when moving from the medium to the high watering level. A Spearman's rank-order correlation test was conducted to compare the δ13C values of plants grown under three watering regimes. A negative correlation was found in one heritage landrace, HER001 (rs = −0.738, p = 0.023) and three modern accessions MOD001 (rs = −0.794, p = 0.019), MOD002 (rs = −0.738, p = 0.023), and MOD004 (rs = −0.949, p = 9.585e−05) (Table 5). The means of the 1% manuring level heritage and modern accessions at three watering levels are shown in Table 4.
The δ15N values of heritage accessions ranged from +9.6‰ to +16.8‰ at the 1% manuring level and from +10.3‰ to +17.1‰ at 5% manuring level (Figure 2B, Table 4, Supplementary material 3). The δ15N values of modern accessions ranged from +11.1‰ to +16.9‰. The range of the δ15N values of HER002 covered the range of all the modern accessions. Overall, higher water input raised the δ15N values of all accessions from the low to the high watering level and the medium to the high watering level (Figure 2B), with HER001 and MOD004 being exceptions. A negative correlation was observed in one heritage accession HER002 (rs = 0.843, p = 0.004) and one modern accession MOD001 (rs = 0.738, p = 0.023) using Spearman's rank-order correlation analysis (Table 5).
Turning to the effect of manuring on δ15N values, plants grown at the 5% manuring level showed a tendency toward higher δ15N values compared with those grown at the 1% manuring level, as observed in HER001 and HER003 but not HER002. For heritage landraces the manuring effect on δ15N values was significant for the medium watering level samples (CI = 95%, p = 0.03595) but not the high watering level samples (CI = 95%, p = 0.1388) (Figure 2C, Table 5).
3.2 Modern fields
Based on Interquartile Range (IQR) analysis performed in R, three samples (LY083, SHC30N, and SH325) from fields were identified as outliers and excluded in the following discussion.
Overall, modern field rice δ13C values ranged from −28.6‰ to −25.5‰ (Figure 3, Table 6, Supplementary material 3), which were about 2.5‰ higher than samples grown in the pot experiment. For paddy rice, δ13C values from South Korea samples ranged from −27.4‰ to −26.3‰. Shanghai samples had a range of −28.6‰ to −26.8‰ and −27.4‰ to −26.7‰ in 2017 and 2019, respectively. Shandong paddy rice in 2020 ranged from −28.3‰ to −27.8‰. For dry rice collected in Shandong in 2019, samples had a range of −26.5‰ to −25.9‰ at Location 1, −26.1‰ to −25.5‰ at Location 2 and −27.2‰ to −25.8‰ at Location 3.
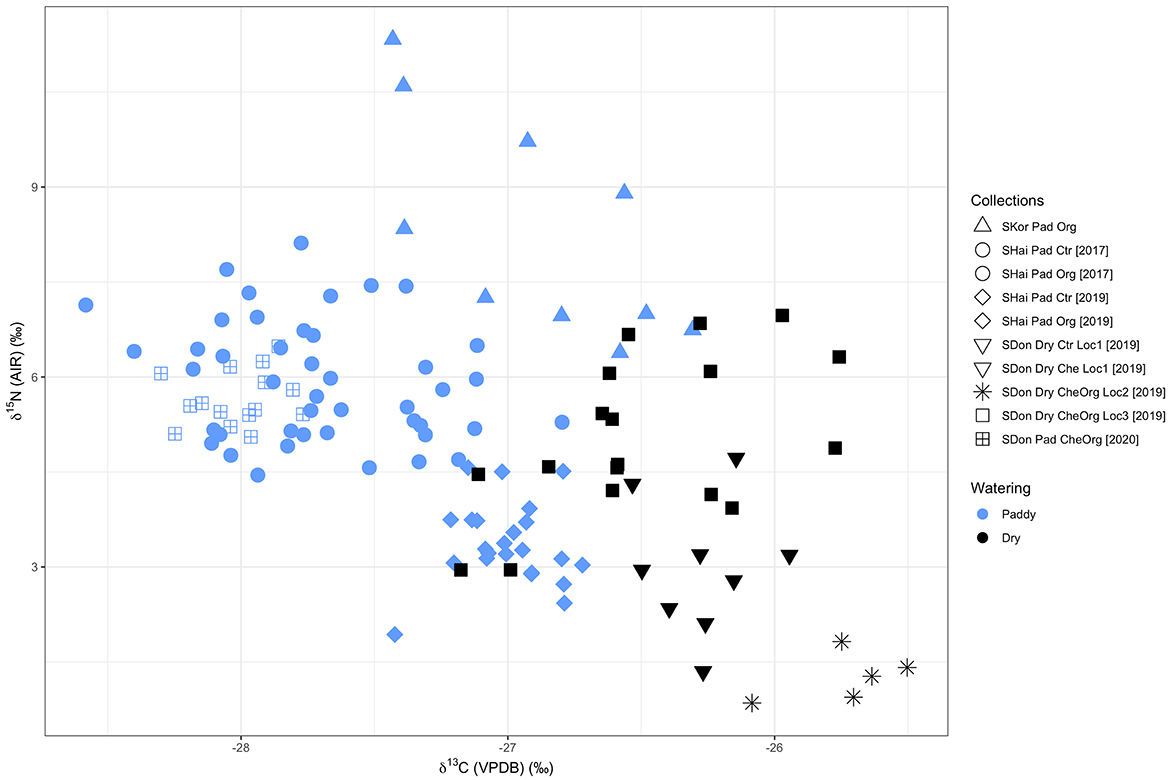
Figure 3. Stable isotope values of modern fields samples coded by watering regimes. SKor, South Korea; SHai, Shanghai; SDon, Shandong; Pad, Paddy; Org, Organic Manure; Ctr, Control; Che, Chemical fertilizer; Loc, Location.
The δ13C values of Shandong dry rice 2020 (mean = −27.5 ± 0.1‰, n = 10) separated from most dry rice samples in this study and overlapped with the range of paddy rice, which were in accordance with their actual growing condition due to drainage problems (see Field collections, above). Therefore, these samples cannot be considered as “normal” dry rice and were excluded in the following discussion. The South Korea samples were grown under the same watering and manuring treatment in an organic farm over the long term, with one sample collected from one field, which provides a good reference for the expected range in δ13C values of rice grains grown under the same conditions in modern fields. In terms of watering treatment and its potential effect on rice δ13C values, the values of paddy field samples (mean = −27.5 ± 0.5‰, n = 92) and dry field samples (mean = −26.3 ± 0.4‰, n = 32) show a significant difference (CI = 95%, p < 2.2e−16) based on the Mann-Whitney U test (Table 7).
Modern field samples had δ15N values ranging from +0.9‰ to +11.3‰ (Figure 4, Table 6, Supplementary material 3), mostly lower than samples grown in the pot experiment. For paddy rice, the δ15N values of the South Korea samples ranged from +6.4‰ to +11.3‰. Shanghai samples had a range of δ15N values from +4.5‰ to +8.1‰ and from +1.9‰ to +4.6‰ in 2017 and 2019, respectively. Shandong paddy rice in 2020 ranged from 5.1‰ to 6.5‰. For dry rice, samples collected in Shandong in 2019 had a range of +1.3‰ to +4.7‰ at Location 1, +0.9‰ to +1.8‰ at Location 2 and +3.0‰ to +7.0‰ at Location 3.
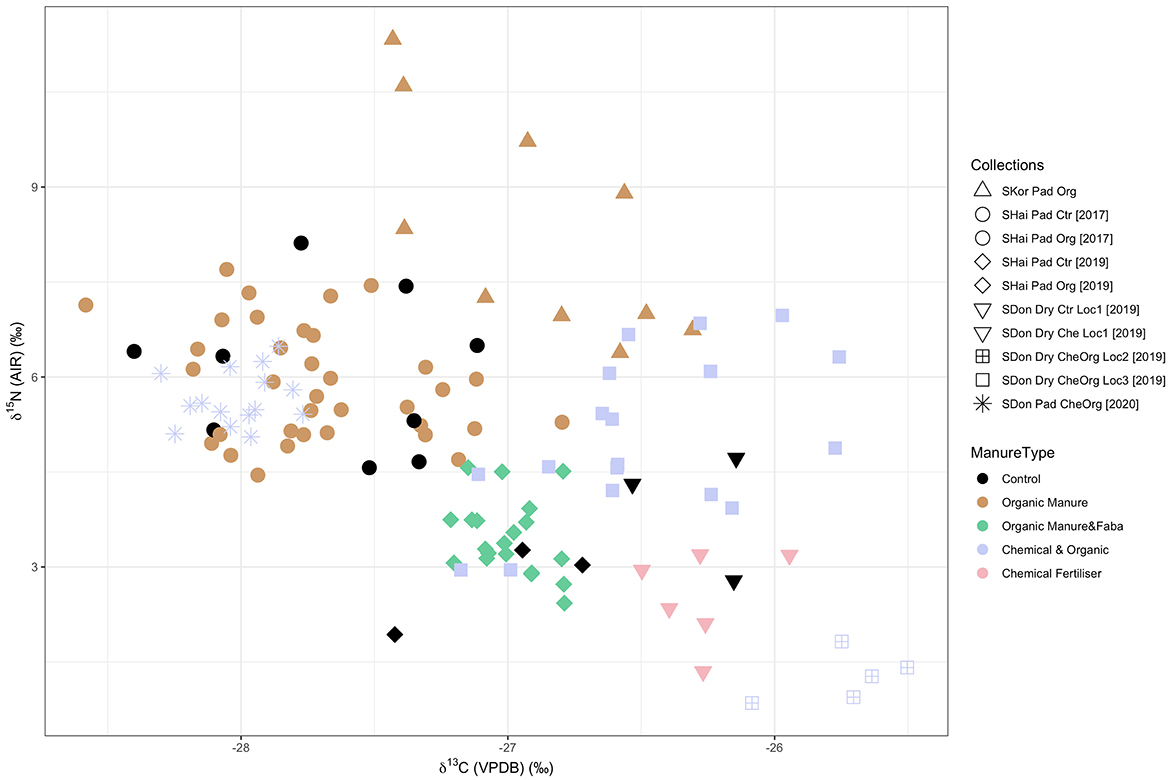
Figure 4. Stable isotope values of modern fields samples coded by manuring regimes. SKor, South Korea; SHai, Shanghai; SDon, Shandong; Pad, Paddy; Org, Organic Manure; Ctr, Control; Che, Chemical fertilizer; Loc, Location.
In terms of watering effects on δ15N values, the control group of paddy rice from the Shanghai 2017 collection (mean = +6.1 ± 1.2‰, n = 9) had higher values than the control group of dry rice from Shandong (Location 1) (mean = +3.9 ± 1.0‰, n = 3) (Figure 5, Table 6). A significant difference between the two groups was observed using the Mann-Whitney U test (CI = 95%, p = 0.03636) (Table 7).
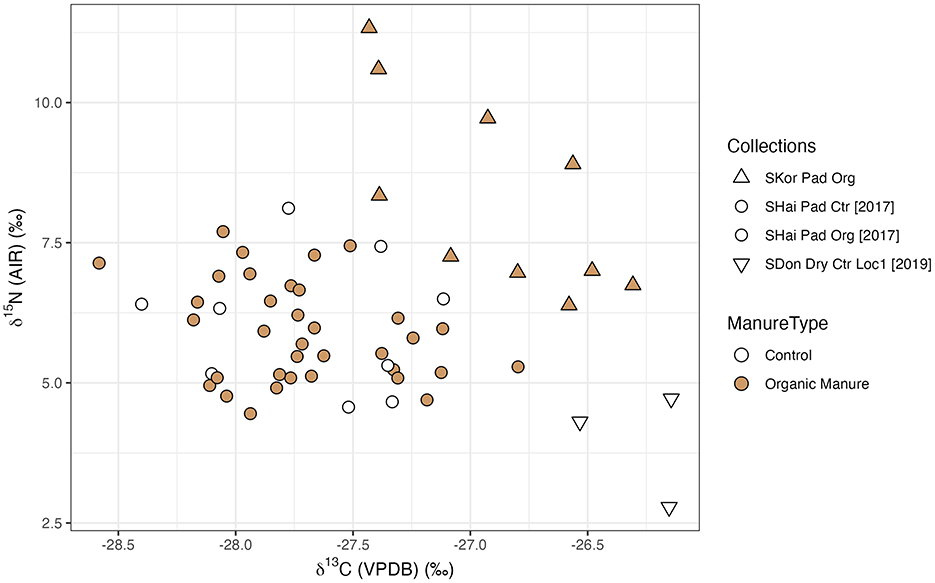
Figure 5. Stable isotope values of modern fields samples (control groups and organic manured groups). SKor, South Korea; SHai, Shanghai; SDon, Shandong; Pad, Paddy; Org, Organic Manure; Ctr, Control; Loc, Location.
Turning to the effect of manuring on δ15N values, the organic rice samples of various manuring regimes from Shanghai had very similar δ15N values in the year 2017, with average values ranging from +5.7 ± 0.8‰ (n = 9, Runbao 5 kg) to +6.1 ± 1.1‰ (n = 9, Runbao 40 kg) (Figure 6). The δ15N values of the control group (mean = +6.2 ± 1.1‰, n = 8) almost covered the whole range of organic manured groups. The Mann-Whitney U test also showed no difference between the control group and the organic manure group (Table 7). For samples collected in Shanghai in 2019, likely due to the application of green manure of Vicia faba, the mean values of the δ15N values of rice (+2.7 ± 0.7‰ to +4.0 ± 0.5‰) were lower than those of soils from 2018 (+4.2 ± 0.4‰ to +5.3 ± 0.4‰) (Supplementary Table 7). On the other hand, the average δ15N values of organic groups in 2019 were still similar to each other and did not differ much from the control group (Table 6).
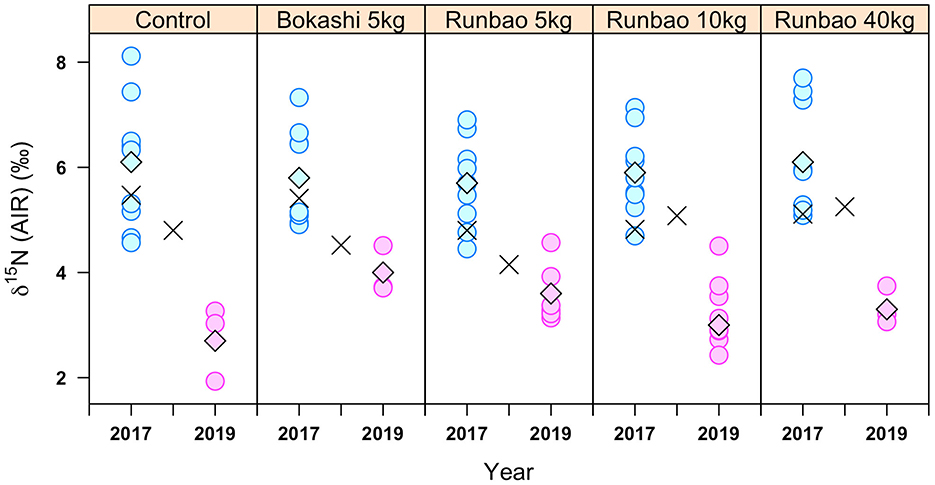
Figure 6. δ15N values of Shanghai 2017 and 2019 rice samples. Diamonds show mean values of each manuring treatment. Black crosses are values of soils from 2017 and 2018.
Since both watering and manuring potentially have a positive effect on δ15N values while watering has a negative effect on δ13C values, statistical tests were applied to see whether there was a consistent correlation between the δ13C values and the δ15N values of unmanured/organic manured paddy rice (without legume green manure). The distribution of the data was first checked using the Shapiro-Wilk test. Only the δ15N values of Bokashi 5 kg group did not follow a normal distribution. The non-parametric Spearman rank correlation test was conducted on this group while the remaining groups were analyzed using the Pearson correlation test. A negative Pearson correlation between the δ13C values and the δ15N values was seen in South Korea samples (CI = 95%, p = 0.02205), Runbao 10 kg (CI = 95%, p = 0.004017), and Runbao 40 kg (CI = 95%, p = 0.05117) samples of the Shanghai 2017 collection (Figure 7, Table 7). In contrast, there was no correlation in the control, Bokashi 5 kg and Runbao 5 kg groups of Shanghai 2017 collection. South Korea samples received long-term intensive organic manure and Runbao 10 kg, 40 kg groups from Shanghai received short-term but more intensive organic manure. The implication is that the negative correlation between δ13C values and δ15N values can possibly be another indicator suggesting the application of intensive manuring.
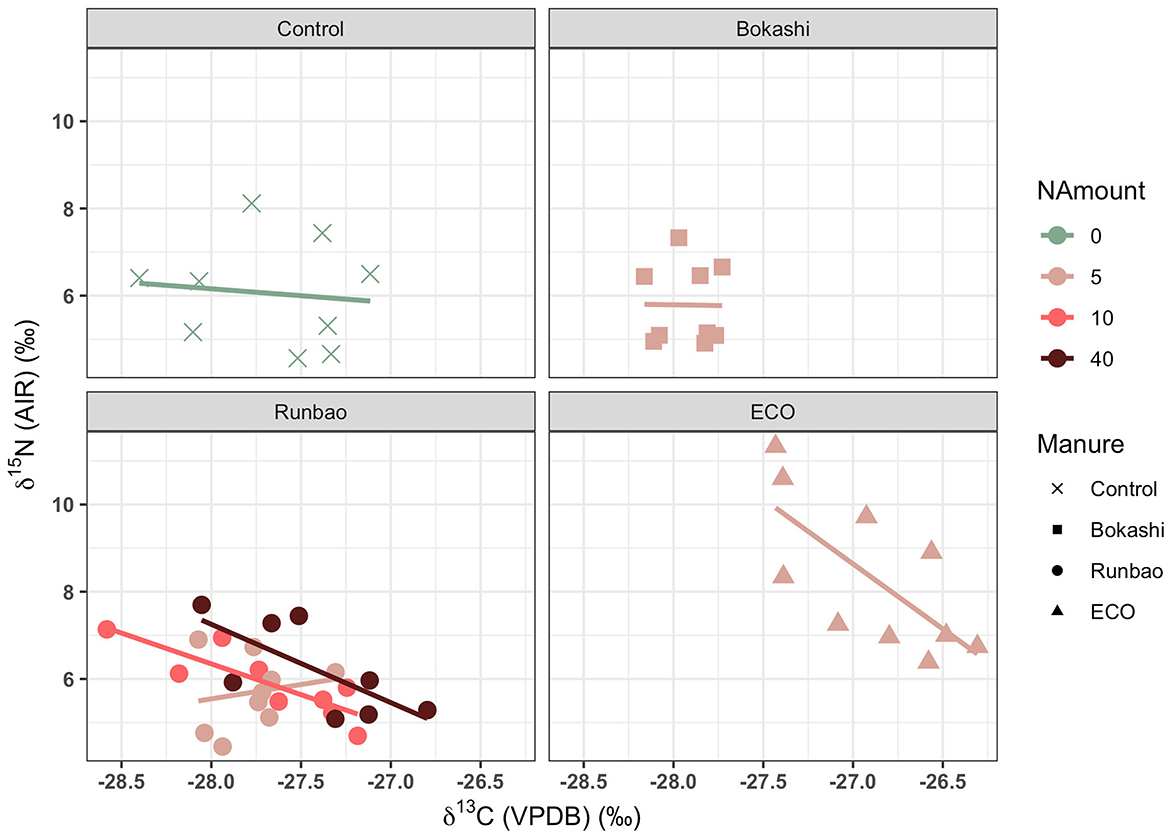
Figure 7. Correlation between the δ13C values and the δ15N values of Shanghai 2017 samples and South Korea samples (Unit of NAmount: kg/666.7 m2).
4 Discussion
4.1 Effect of watering treatments on δ13C values
Under dry conditions, stomata tend to close to decrease evaporation, resulting in a reduced stomatal conductance. C3 plants such as rice prefer to use 12C for photosynthesis, causing the remaining carbon to be enriched in 13C. The lower stomatal conductance prevents 12C in the air to freely enter stomata, which further raised the concentration of 13C (Farquhar and Richards, 1984; Farquhar et al., 1989; Ehleringer et al., 1993; Wallace et al., 2013; Styring et al., 2016). Consequently, rice under a dry condition would have higher δ13C values than rice under a wet condition.
For most pot experiment samples, watering does indeed have a negative effect on δ13C values. However, the δ13C values of HER002 (128438) and MOD003 (S-DEP1) increase from the medium to the high watering level. This might be due to their physiological features since they grew more quickly and thus their medium watering level samples experienced a shorter period under the waterlogged condition. In addition, pot experiment samples showed larger variations in the δ13C values between accessions than between watering levels. This indicated that the archaeological application of δ13C values to reveal wetness should primarily be used for comparison within local sequences since variations in water use efficiency between populations can be factored out. Paddy rice samples from the pot experiment generally have higher δ13C values than those from fields, which was possibly associated with the rather high relative humidity (80%) and high temperature (28°C to 30°C) in the glasshouse where the pot experiment was conducted.
For field samples, paddy rice also exhibited lower δ13C values than dry rice as expected. The δ13C values of most paddy rice samples were lower than −26.5‰, while most dry rice samples were higher than −26.5‰ (Figure 3). The δ13C values of paddy field rice in this study were comparable to those from previous reported values. Supplementary Table 2 summarizes previous studies with δ13C values of wet rice grains (or roots and shoots) from pot experiments or fields in East Asia, Southeast Asia, South Asia as well as Australia and United States. The mean values of paddy rice varied from −29.2‰ to −26.3‰, which in general were similar as the range of the field samples in this study (−28.6‰ to −26.3‰).
There was no difference in the δ13C values of paddy rice at different locations, in accordance with previous findings that δ13C values alone did not distinguish geographic origins (Chung et al., 2017). The distribution of the Shanghai 2019 samples and South Korea samples largely overlapped with the distribution of the Shanghai 2017 samples. Similarly, the range of Shandong 2020 samples was covered by Shanghai 2017 samples. It was the same case for the dry rice field samples as samples from location 3 covered the distribution of samples from location 1 and overlapped with samples from location 2.
4.2 Effect of watering treatments on δ15N values
The anaerobic conditions prevalent in paddy fields impede nitrification and nitrate production while increasing denitrification. Denitrification transforms nitrate to N2 and leads to an accumulation of 15N in the remaining nitrate. Thus, ammonium becomes the dominant N species in paddy rice, which is directly absorbed by plants. 15N-enriched nitrate can also be utilized by paddy rice as a secondary N resource (Lim et al., 2015; Denk et al., 2017). As a result, paddy rice shows higher δ15N values than dry rice.
The significantly higher δ15N values of the paddy rice control group (mean = +6.1 ± 1.2‰, n = 9) compared with the dry rice control group (mean = +3.9 ± 1.0‰, n = 3) suggested that waterlogged conditions elevated the δ15N values of rice grains. Previous findings analyzed charred rice grains from the Late Yangshao period to Eastern Zhou period (around 3,500 BC to 221 BC) in the Central Plains, China, and suggested that manuring was applied to rice plants throughout the studied periods based on δ15N values (Wang et al., 2022; Tao et al., 2022a,b, 2023). However, the mean value of the modern paddy rice control group in this study was higher than that of the Er'ligang period (+5.4 ± 2.5‰) and the Yinxu period (+6.0 ± 2.4‰) at the site of Huqiugang (Supplementary Table 8). In addition, the range of the δ15N values of the modern paddy rice control group (+1.9‰ to +8.1‰) overlapped with the range of the remaining sites (+7.0 ± 1.7‰ to +8.0 ± 1.4‰) as summarized in Supplementary Table 8. Consequently, paddy conditions could also produce δ15N values similar to at least part of the data reported in these studies, and whether the data can be fully explained by manuring effect requires reconsideration. Whereas light irrigation has no effect on the δ15N values of dryland crops (Bogaard et al., 2013; Sanborn et al., 2021), paddy conditions have a significant positive effect on the δ15N values of rice.
4.3 Effect of manuring treatments on δ15N values
In the glasshouse pot experiment, two manuring levels (the 1% and 5%) were applied to three heritage accessions, of which two showed higher δ15N values of rice grains at the 5% manuring level compared with the 1% manuring level. Significant differences in δ15N values were found at the medium watering level but not the high watering level as shown by the Mann-Whitney test, suggesting that manuring did have a positive effect on paddy rice grains, though the influence may vary among accessions and needs to be considered together with watering regimes.
Intensive organic manure in initial seasons did not increase the δ15N values of rice grains from fields in this study. This finding is similar to Fraser et al. (2011), where short-term experiments did not show marked manuring effect on cereal δ15N values. The δ15N values of most organic rice from fields were above 5‰, which was an organic rice threshold suggested by Chung et al. (2017). On the other hand, paddy rice from the control group Shanghai 2017 collection (mean = +6.1 ± 1.2‰, n = 9) and from the chemical and organic group Shandong 2020 collection (mean = +5.7 ± 0.4‰, n = 15) were also above this threshold. Consequently, manuring is not the only factor for interpreting δ15N values of rice grains and currently no solid threshold of manuring levels can be suggested.
In addition, the negative correlation of δ13C values and δ15N values was found in South Korea samples where long-term intensive organic manure had been applied, and in some of the Shanghai 2017 samples which received one season's high input of organic manure. This finding indicates that the effect of intensive manuring on the δ15N values may be identifiable, but more data are needed where wetness and manuring vary independently.
4.4 Differentiating watering and manuring regimes of rice
This study opens a new way for understanding the watering and manuring status of archaeological rice remains. Based on the δ13C values, it is possible to differentiate the relative wet/dry status of rice (Figure 3, Table 7) and to infer management practices such as drainage. Combining the results of the δ13C values and δ15N values makes long term application of organic manure discernible for dry rice (Figure 4, Table 8) and future study of dry rice receiving only organic manure will further refine this framework. Turning to paddy rice, since both paddy conditions and organic manure increase the δ15N values of rice grains, the effect of these two factors may mask each other when the manure input is of short term or at relatively low level. However, long-term application of intensive organic manure has the potential to be diagnosed through a negative correlation between the δ13C values and δ15N values (Figure 7, Table 8). Future studies could focus on paddy and dry rice samples receiving different durations of organic manure application to understand how δ15N values are affected by long-term manuring. Experimental growth of paddy rice with varying watering and manuring regimes is another important approach to check whether the positive effect of organic manuring on δ15N values can be differentiated from the effect caused by denitrification in paddy fields.
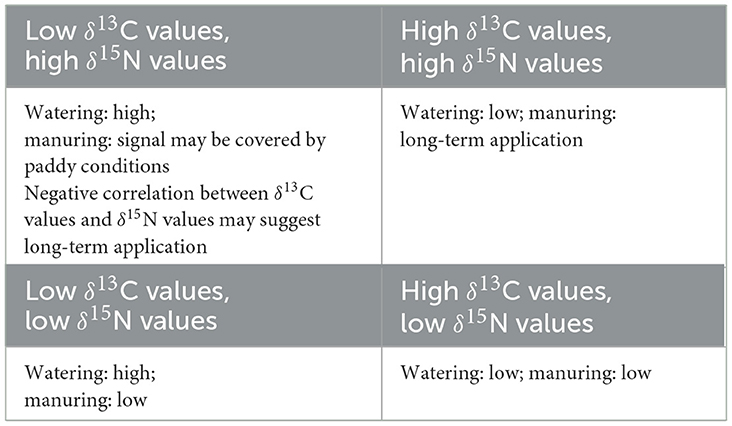
Table 8. Indications of the combination of δ13C values and δ15N values on rice watering and manuring regimes.
5 Conclusions
This study shows that stable carbon and nitrogen isotope values of rice grains are useful indicators of water status, with potential in combination to disentangle watering and manuring practices of archaeological rice remains. In the glasshouse pot growing experiment, we found that δ13C values tended to have a negative correlation with watering levels, evident in one heritage accession and three modern accessions. Medium and high watering level samples showed lower δ13C values than low watering level samples across all of the accessions. Similarly, the δ13C values of experimental rice from paddy fields in eastern China and South Korea were significantly lower than those from dry fields. Field samples analyzed in this study also provide reference values for future research on archaeological rice remains. Dry rice had a mean δ13C value of −26.3 ± 0.4‰ while that of paddy rice was −27.5 ± 0.5‰. For the mean δ15N value, that of the control group of dry rice and paddy rice was +3.9 ± 1.0‰ and +6.1 ± 1.2‰, respectively.
Paddy conditions also tended to increase the δ15N values of rice grown in the glasshouse pot experiment. A similar trend was found between unmanured groups of experimental paddy rice and dry rice from fields. Organic manure also increased the δ15N values of rice grains, as shown by the pot rice with 1% and 5% manuring levels under medium watering level. Nevertheless, the effect of intensive manuring was not observed in initial growing seasons in field experiments, echoing results from the early years of newly established manuring experiments in Europe (Fraser et al., 2011). Therefore, it may take a number of years for manuring effects on the δ15N values of rice to develop.
Data availability statement
The original contributions presented in the study are included in the article/Supplementary material, further inquiries can be directed to the corresponding author.
Author contributions
RW: Conceptualization, Data curation, Formal analysis, Funding acquisition, Investigation, Methodology, Project administration, Resources, Software, Validation, Visualization, Writing – original draft, Writing – review & editing. EB: Methodology, Resources, Writing – review & editing. YD: Investigation, Writing – review & editing. SF: Resources, Writing – review & editing. XF: Resources, Writing – review & editing. NH: Resources, Writing – review & editing. FY: Resources, Writing – review & editing. MC: Conceptualization, Formal analysis, Investigation, Methodology, Project administration, Resources, Supervision, Validation, Writing – review & editing. AB: Conceptualization, Formal analysis, Funding acquisition, Investigation, Methodology, Project administration, Resources, Supervision, Validation, Writing – review & editing.
Funding
The author(s) declare financial support was received for the research, authorship, and/or publication of this article. This research was supported by the Chinese Scholarship Council for the study at the University of Oxford, by St Peter's College Graduate Awards and the School of Archaeology's Meyerstein Fund for fieldwork expenses, and by the University of Oxford's John Fell Fund for conducting the glasshouse pot experiment.
Acknowledgments
The authors would like to dedicate this paper to Dr Alison Weisskopf, whose thought-provoking work touched a long-neglected aspect and led us to view rice-growing landscapes in new ways. We thank colleagues at Bad Lauchstädt (Dr Ines Merbach) and Askov (Professor Bent Christensen) who advised on the base nutrient solution for pot-grown rice. We thank Dr Hyunyoung Kim for the access to samples from Buyeo, South Korea and the introduction of growth information. We thank Dr Jianli Liao for the isotopic data of soils from the Anyway Farm. We thank Professor Guiyun Jin, Yanquan Song, Xuyuan Zhou, Lianguang Zhang, Zongzi Pan, Guilong Zhang, and Zhicai Shang who provided opportunities and assistance for us to investigate samples from Linyi City, Shandong province, China and assisted in sample collection. We thank Lechun Lai, Gang Wu, and Hong Pan who helped with sample preparation. We thank Dr Chulmin Kim, Dr Peter Ditchfield, Dr Amy Styring, David Peat, Dr Elizabeth Stroud, and Corrie Hyland for help and advice on the pot experiment, sample analysis and/or data processing. We thank the reviewers and editors for their comments. This research was supported by the Chinese Scholarship Council, St Peter's College Graduate Awards, the School of Archaeology's Meyerstein Fund and the University of Oxford's John Fell Fund.
Conflict of interest
The authors declare that the research was conducted in the absence of any commercial or financial relationships that could be construed as a potential conflict of interest.
Publisher's note
All claims expressed in this article are solely those of the authors and do not necessarily represent those of their affiliated organizations, or those of the publisher, the editors and the reviewers. Any product that may be evaluated in this article, or claim that may be made by its manufacturer, is not guaranteed or endorsed by the publisher.
Supplementary material
The Supplementary Material for this article can be found online at: https://www.frontiersin.org/articles/10.3389/fearc.2024.1488754/full#supplementary-material
References
Aguilera, M., Ferrio, J. P., Pérez, G., Araus, J. L., and Voltas, J. (2012). Holocene changes in precipitation seasonality in the western mediterranean basin: a multi-species approach using δ13C of archaeobotanical remains. J. Quarter. Sci. 27, 192–202. doi: 10.1002/jqs.1533
Araus, J. L., Febrero, A., Buxo, R., Camalich, M. D., Martin, D., Molina, F., et al. (1997). Changes in carbon isotope discrimination in grain cereals from different regions of the western mediterranean basin during the past seven millennia. Palaeoenvironmental evidence of a differential change in aridity during the late holocene. Glob. Chang. Biol. 3, 107–118. doi: 10.1046/j.1365-2486.1997.00056.x
Araus, J. L., Febrero, A., Catala, M., Molist, M., Voltas, J., and Romagosa, I. (1999). Crop water availability in early agriculture: evidence from carbon isotope discrimination of seeds from a tenth millennium BP site on the euphrates. Glob. Chang. Biol. 5, 201–212. doi: 10.1046/j.1365-2486.1999.00213.x
Araus, J. L., Ferrio, J. P., Voltas, J., Aguilera, M., and Buxó, R. (2014). Agronomic conditions and crop evolution in ancient near east agriculture. Nat. Commun. 5:3953. doi: 10.1038/ncomms4953
Bogaard, A., Fraser, R., Heaton, T. H., Wallace, M., Vaiglova, P., Charles, M., et al. (2013). Crop manuring and intensive land management by Europe's first farmers. Proc. Nat. Acad. Sci. 110, 12589–12594. doi: 10.1073/pnas.1305918110
Bogaard, A., Heaton, T. H. E., Poulton, P., and Merbach, I. (2007). The impact of manuring on nitrogen isotope ratios in cereals: archaeological implications for reconstruction of diet and crop management practices. J. Archaeol. Sci. 34, 335–343. doi: 10.1016/j.jas.2006.04.009
Bogaard, A., Hodgson, J., Nitsch, E., Jones, G., Styring, A., Diffey, C., et al. (2016). Combining functional weed ecology and crop stable isotope ratios to identify cultivation intensity: a comparison of cereal production regimes in Haute Provence, France and Asturias, Spain. Veg. Hist. Archaeobot. 25, 57–73. doi: 10.1007/s00334-015-0524-0
Cai, D., and Fu, T. (1985). A study on the effect of split–applied n fertilizer with isotope 15N dilution Method. J. Nanjing Agric. Univ. 2, 82–88.
Cao, Z. (2008). Study of prehistoric irrigated paddys and ancient paddy soils in China. Acta Pedol. Sinica 45, 784–791.
Centritto, M., Lauteri, M., Monteverdi, M. C., and Serraj, R. (2009). Leaf gas exchange, carbon isotope discrimination, and grain yield in contrasting rice genotypes subjected to water deficits during the reproductive stage. J. Exp. Bot. 60, 2325–2339. doi: 10.1093/jxb/erp123
Chen, T., Zhao, Y., Zhang, W., Yang, S., Ye, Z., and Zhang, G. (2016). Variation of the light stable isotopes in the superior and inferior grains of rice (Oryza sativa L.) with different geographical origins. Food Chem. 209, 95–98. doi: 10.1016/j.foodchem.2016.04.029
Choi, J. Y., Platts, A. E., Fuller, D. Q., Hsing, Y. I., Wing, R. A., and Purugganan, M. D. (2017). The rice paradox: multiple origins but single domestication in Asian rice. Mol. Biol. Evol. 34, 969–979. doi: 10.1093/molbev/msx049
Christensen, B. T., Jensen, J. L., Dong, Y., and Bogaard, A. (2022). Manure for Millet: Grain δ15N Values as Indicators of Prehistoric Cropping Intensity of Panicum Miliaceum and Setaria Italica. J. Archaeol. Sci. 139:105554. doi: 10.1016/j.jas.2022.105554
Chung, I., Park, S., Lee, K., An, M., Lee, J., Oh, Y., et al. (2017). Authenticity testing of environment-friendly Korean rice (Oryza Sativa L.) using carbon and nitrogen stable isotope ratio analysis'. Food Chem. 234, 425–430. doi: 10.1016/j.foodchem.2017.05.014
De, P. K. (1939). The role of blue-green algae in nitrogen fixation in rice-fields. Proc. R. Soc. London Biol. Sci. 127, 121–139. doi: 10.1098/rspb.1939.0014
Denk, T. R. A., Mohn, J., Decock, C., Lewicka-Szczebak, D., Harris, E., Butterbach-Bahl, K., et al. (2017). The nitrogen cycle: a review of isotope effects and isotope modeling approaches. Soil Biol. Biochem. 105, 121–137. doi: 10.1016/j.soilbio.2016.11.015
Ding, J. (2004). The origin of paddy fields and rice agriculture in the Neolithic lower Yangtze river basin. Southeast Culture. 2004, 19–23.
Ding, J. (2010). Paddy field and rice farming during the Majiabang culture period. J. Jiaxing Univ. 22, 22–27. doi: 10.3969/i.issn.1008-6781.2010.05.003
Dong, Y., Bi, X., Wu, R., Belfield, E. J., Harberd, N. P., Christensen, B. T., et al. (2022). The potential of stable carbon and nitrogen isotope analysis of foxtail and broomcorn millets for investigating ancient farming systems. Front. Plant Sci. 13:1018312. doi: 10.3389/fpls.2022.1018312
Ehleringer, J. R., Hall, A. E., and Farquhar, G. D. (1993). Stable Isotopes and Plant Carbon-Water Relations. San Diego: Academic Press.
Farquhar, G. D., Ehleringer, J. R., and Hubick, K. T. (1989). Carbon isotope discrimination and photosynthesis. Annu. Rev. Plant Biol. 40, 503–537. doi: 10.1146/annurev.pp.40.060189.002443
Farquhar, G. D., O'Leary, M. H., and Berry, J. A. (1982). On the relationship between carbon isotope discrimination and the intercellular carbon dioxide concentration in leaves. Funct. Plant Biol. 9, 121–137.
Farquhar, G. D., and Richards, R. A. (1984). Isotopic composition of plant carbon correlates with water-use efficiency of wheat genotypes. Funct. Plant Biol. 11, 539–552. doi: 10.1071/PP9840539
Ferrio, J. P., Araus, J. L., Buxó, R., Voltas, J., and Bort, J. (2005). Water management practices and climate in ancient agriculture: inferences from the stable isotope composition of archaeobotanical remains. Veg. Hist. Archaeobot. 14, 510–517. doi: 10.1007/s00334-005-0062-2
Fraser, R. A., Bogaard, A., Heaton, T., Charles, M., Jones, G., Christensen, B. T., et al. (2011). Manuring and stable nitrogen isotope ratios in cereals and pulses: towards a new archaeobotanical approach to the inference of land use and dietary practices. J. Archaeol. Sci. 38, 2790–2804. doi: 10.1016/j.jas.2011.06.024
Fraser, R. A., Bogaard, A., Schäfer, M., Arbogast, R., and Heaton, T. H. E. (2013). Integrating botanical, faunal and human stable carbon and nitrogen isotope values to reconstruct land use and palaeodiet at LBK Vaihingen an Der Enz, Baden-Württemberg. World Archaeol. 45, 492–517. doi: 10.1080/00438243.2013.820649
Fuller, D. Q., Etten, J., Manning, K., Castillo, C., Kingwell-Banham, E., Weisskopf, A., et al. (2011). The contribution of rice agriculture and livestock pastoralism to prehistoric methane levels: an archaeological assessment. Holocene 21, 743–759. doi: 10.1177/0959683611398052
Fuller, D. Q., and Qin, L. (2009). Water management and labour in the origins and dispersal of Asian rice. World Archaeol. 41, 88–111. doi: 10.1080/00438240802668321
Fuller, D. Q., Qin, L., Zheng, Y., Zhao, Z., Chen, X., Hosoya, L. A., et al. (2009). The domestication process and domestication rate in rice: spikelet bases from the Lower Yangtze. Science 323, 1607–1610. doi: 10.1126/science.1166605
Fuller, D. Q., Sato, Y. I., Castillo, C., Qin, L., Weisskopf, A. R., Kingwell-Banham, E. J., et al. (2010). Consilience of genetics and archaeobotany in the entangled history of rice. Archaeol. Anthropol. Sci. 2, 115–131. doi: 10.1007/s12520-010-0035-y
Gao, Q., Sun, J., Tong, H., Wang, W., Zhang, Y., Zhang, G., et al. (2018). Evaluation of rice drought stress response using carbon isotope discrimination. Plant Physiol. Biochem. 132, 80–88. doi: 10.1016/j.plaphy.2018.08.030
Gao, Y. (2017). A Study of the Prehistoric Rice Agriculture in Taihu Region. PhD Thesis. Peking University.
Gealy, D. R., and Fischer, A. J. (2010). 13C discrimination: a stable isotope method to quantify root interactions between C3 rice (Oryza Sativa) and C4 barnyardgrass (Echinochloa Crus-Galli) in flooded fields. Weed Sci. 58, 359–368. doi: 10.1614/WS-D-09-00053.1
Gealy, D. R., and Gealy, G. S. (2011). 13Carbon isotope discrimination in roots and shoots of major weed species of southern US rice fields and its potential use for analysis of rice-weed root interactions. Weed Sci. 59, 587–600. doi: 10.1614/WS-D-10-00140.1
Gross, B. L., and Zhao, Z. (2014). Archaeological and genetic insights into the origins of domesticated rice. Proc. Nat. Acad. Sci. 111, 6190–6197. doi: 10.1073/pnas.1308942110
Guo, X. R. (2014). Research of prehistoric plant remains from Guangfulin Site in Shanghai. Master's thesis. Shandong University
Gupta, N. S., Leng, Q., Yang, H., Cody, G. D., Fogel, M. L., Liu, W., et al. (2013). Molecular preservation and bulk isotopic signals of ancient rice from the Neolithic Tianluoshan site, Lower Yangtze River Valley, China. Org. Geochem. 63, 85–93. doi: 10.1016/j.orggeochem.2013.08.006
Hedges, R. E., and Reynard, L. M. (2007). Nitrogen isotopes and the trophic level of humans in archaeology. J. Archaeol. Sci. 34, 1240–1251. doi: 10.1016/j.jas.2006.10.015
Hu, J., Zhu, L., Zhong, C., Zhang, J., Cao, X., Yu, S., et al. (2017). Effects of dissolved oxygen on nitrogen transformation in paddy soil and metabolism of rice: a review. Chinese J. Ecol. 36, 2019–2028. doi: 10.13292/j.1000-4890.201707.005
Huang, H., and Shi, Z. (1964). Effects of green manure in raising the fertility of rice paddy fields. Chin. J. Soil Sci. 4, 57–59.
Huang, X., Qian, Q., Liu, Z., Sun, H., He, S., Luo, D., et al. (2009). Natural variation at the DEP1 locus enhances grain yield in rice. Nat. Genet. 41, 494–497. doi: 10.1038/ng.352
Impa, S.M., Nadaradjan, S., Boominathan, P., Shashidhar, G., Bindumadhava, H.Y., and Sheshshayee, M.S. (2005). Carbon isotope discrimination accurately reflects variability in WUE measured at a whole plant level in rice. Crop Sci. 45, 2517–2522. doi: 10.2135/cropsci2005.0119
Ishikawa, R., Castillo, C. C., and Fuller, D. Q. (2020). Genetic evaluation of domestication-related traits in rice: implications for the archaeobotany of rice origins. Archaeol. Anthropol. Sci. 12:197. doi: 10.1007/s12520-020-01112-3
Kano-Nakata, M., Tatsumi, J., Inukai, Y., Asanuma, S., and Yamauchi, A. (2014). Effect of various intensities of drought stress on δ13C variation among plant organs in rice: comparison of two cultivars. Am. J. Plant Sci. 5:1686. doi: 10.4236/ajps.2014.511183
Kaushal, R., and Ghosh, P. (2018). Stable oxygen and carbon isotopic composition of rice (Oryza sativa L.) grains as recorder of relative humidity. J. Geophys. Res. Biogeosci. 123, 423–439. doi: 10.1002/2017JG004245
Kaushal, R., Ghosh, P., and Geilmann, H. (2016). Fingerprinting environmental conditions and related stress using stable isotopic composition of rice (Oryza Sativa L.) grain organic matter. Ecol. Indic. 61, 941–951. doi: 10.1016/j.ecolind.2015.10.050
Kaushal, R., Ghosh, P., and Pokharia, A. K. (2019). Stable isotopic composition of rice grain organic matter marking an abrupt shift of hydroclimatic condition during the cultural transformation of Harappan civilization. Quater. Int. 512, 144–154. doi: 10.1016/j.quaint.2019.04.017
Korenaga, T., Musashi, M., Nakashita, R., and Suzuki, Y. (2010). Statistical analysis of rice samples for compositions of multiple light elements (H, C, N, and O) and their stable isotopes. Anal. Sci. 26, 873–878. doi: 10.2116/analsci.26.873
Ladha, J. K., and Reddy, P. M. (2003). Nitrogen fixation in rice systems: state of knowledge and future prospects. Plant Soil 252, 151–167. doi: 10.1023/A:1024175307238
Ladha, J. K., Watanabe, I., and Roger, P. A. (1983). 28 Biological nitrogen fixation in wetland rice. Women Rice Farm. 481, 515–566.
Laursen, K. H., Mihailova, A., Kelly, S. D., Epov, V. N., Bérail, S., Schjørring, J. K., et al. (2013). Is it really organic?–Multi-isotopic analysis as a tool to discriminate between organic and conventional plants. Food Chem. 141, 2812–2820. doi: 10.1016/j.foodchem.2013.05.068
Laza, M. R., Kondo, M., Ideta, O., Barlaan, E., and Imbe, T. (2006). Identification of quantitative trait loci for δ13C and productivity in irrigated lowland rice. Crop Sci. 46, 763–773. doi: 10.2135/cropsci2005.05.0084
Ley, S., Yeh, T., Liu, F., Wang, L., and Ts'ui, S. (1959). The effects of nitrogen-fixing blue-green algae on the yields of rice plant. Acta Hydrobiol. Sin. 4, 440–444.
Li, H., Sun, Y., Yang, Y., Cui, Y., Ren, L., Li, H., et al. (2022). Water and soil management strategies and the introduction of wheat and barley to northern China: an isotopic analysis of cultivation on the Loess Plateau. Antiquity 96, 1478–1494. doi: 10.15184/aqy.2022.138
Li, Y. (2012). Studies on effects and mechanisms of water conditions and nitrogen fertilizer levels on nitrogen use efficiency in a rice cropping system. Zhejiang University, PhD thesis.
Lim, S., Kwak, J., Lee, K., Chang, S., Yoon, K., Kim, H., et al. (2015). Soil and plant nitrogen pools in paddy and upland ecosystems have contrasting δ15N. Biol. Fertil. Soils 51, 231–239. doi: 10.1007/s00374-014-0967-y
Liu, H., Wang, A., Chen, J., and Yin, K. (2012). Stable carbon and nitrogen isotope characters in rice paddy ditch and wetland system. Trans. CSAE 28, 220–224.
Liu, L., Lee, G., Jiang, L., and Zhang, J. (2007). Evidence for the early beginning (c. 9000 cal. BP) of rice domestication in China: a response. Holocene 17, 1059–1068. doi: 10.1177/0959683607085121
Liu, X. (2021). The system construction and theory exploration of the archaeology of agricultural fields. J. Guangxi Univ. National. 43, 153–164. doi: 10.1080/03090728.2021.1974192
Ma, Y., Yang, X., Huan, X., Wang, W., Ma, Z., Li, Z., et al. (2016). Rice bulliform phytoliths reveal the process of rice domestication in the Neolithic Lower Yangtze River region. Quarter. Int. Domestic. East Asia 426, 126–132. doi: 10.1016/j.quaint.2016.02.030
Nayak, A., Basa, K., Boivin, N., Fuller, D., Mohanty, R., Kingwell-Banham, E., et al. (2022). A stable isotope perspective on archaeological agricultural variability and Neolithic experimentation in India. J. Archaeol. Sci. 141:105591. doi: 10.1016/j.jas.2022.105591
Nitsch, E., Andreou, S., Creuzieux, A., Gardeisen, A., Halstead, P., Isaakidou, V., et al. (2017). A bottom-up view of food surplus: using stable carbon and nitrogen isotope analysis to investigate agricultural strategies and diet at Bronze Age Archontiko and Thessaloniki Toumba, northern Greece. World Archaeol. 49, 105–137. doi: 10.1080/00438243.2016.1271745
Pang, H., and Chen, C. (2002). Wild Rice Resources in China. Nanning: Guangxi Science and Technology Press, 2–9.
Qiu, Z. (2015). Study of the development of rice agriculture and environmental changes in the prehistoric Taihu Basin. [PhD thesis]. University of Chinese Academy of Sciences.
Qiu, Z., Jiang, H., and Ding, J. (2013). Analysis of plant remains at the Neolithic Jiangli Site, Kunshan City, Jiangsu Province. Cult. Relics 1, 90–96.
Qiu, Z., Jiang, H., Ding, J., Hu, Y., and Shang, X. (2014). Pollen and phytolith evidence for rice cultivation and vegetation change during the mid-late holocene at the Jiangli Site, Suzhou, East China. PLoS ONE 9:e86816. doi: 10.1371/journal.pone.0086816
Robinson, D. (2001). δ15N as an integrator of the nitrogen cycle. Trends Ecol. Evol. 16, 153–162. doi: 10.1016/S0169-5347(00)02098-X
Sanborn, L. H., Reid, R. E., Bradley, A. S., and Liu, X. (2021). The effect of water availability on the carbon and nitrogen isotope composition of a C4 plant (pearl millet, Pennisetum glaucum). J. Archaeol. Sci. Rep. 38:103047. doi: 10.1016/j.jasrep.2021.103047
Sano, Y., Morishima, H., and Oka, H. (1980). Intermediate perennial-annual populations of Oryza perennis found in Thailand and their evolutionary significance. J. Plant Res. 93, 291–305. doi: 10.1007/BF02488735
Shao, C., Liu, G., Wang, J., Yue, C., and Lin, W. (2008). Differential proteomic analysis of leaf development at rice (Oryza sativa) seedling stage. Agric. Sci. China 7, 1153–1160. doi: 10.1016/S1671-2927(08)60159-5
Shoda, S., Nasu, H., Yamazaki, K., Murakami, N., Na, G., Ahn, S., et al. (2021). Dry or wet? Evaluating the initial rice cultivation environment on the Korean peninsula. Agronomy 11:929. doi: 10.3390/agronomy11050929
Stroud, E., Bogaard, A., and Charles, M. (2021). A stable isotope and functional weed ecology investigation into Chalcolithic cultivation practices in Central Anatolia: Çatalhöyük, Çamlibel Tarlasi and Kuruçay. J. Archaeol. Sci. 38:103010. doi: 10.1016/j.jasrep.2021.103010
Styring, A. K., Ater, M., Hmimsa, Y., Fraser, R., Miller, H., Neef, R., et al. (2016). Disentangling the effect of farming practice from aridity on crop stable isotope values: a present-day model from Morocco and its application to early farming sites in the eastern Mediterranean. Anthropocene Rev. 3, 2–22. doi: 10.1177/2053019616630762
Styring, A. K., Charles, M., Fantone, F., Hald, M. M., McMahon, A., Meadow, R. H., et al. (2017). Isotope evidence for agricultural extensification reveals how the world's first cities were fed. Nat. Plants 3:17076. doi: 10.1038/nplants.2017.76
Styring, A. K., Diop, A. M., Bogaard, A., Champion, L., Fuller, D. Q., Gestrich, N., et al. (2019a). Nitrogen isotope values of Pennisetum glaucum (pearl millet) grains: towards a reconstruction of past cultivation conditions in the Sahel, West Africa. Veget Hist Archaeobot 28, 663–678. doi: 10.1007/s00334-019-00722-9
Styring, A. K., Höhn, A., Linseele, V., and Neumann, K. (2019b). Direct evidence for agricultural intensification during the first two millennia AD in northeast Burkina Faso. J. Archaeol. Sci. 108:104976. doi: 10.1016/j.jas.2019.104976
Sullivan, J. H., Warkentin, M., and Wallace, L. (2021). So many ways for assessing outliers: what really works and does it matter? J. Bus. Res. 132, 530–543. doi: 10.1016/j.jbusres.2021.03.066
Szpak, P., Longstaffe, F. J., Millaire, J. F., and White, C. D. (2014). Large variation in nitrogen isotopic composition of a fertilized legume. J. Archaeol. Sci. 45, 72–79. doi: 10.1016/j.jas.2014.02.007
Szpak, P., Metcalfe, J. Z., and Macdonald, R. A. (2017). Best practices for calibrating and reporting stable isotope measurements in archaeology. J. Archaeol. Sci. 13, 609–616. doi: 10.1016/j.jasrep.2017.05.007
Tao, D., Xu, J., Wu, Q., Gu, W., Wei, Q., Zhou, Y., et al. (2022a). Human diets, crop patterns, and settlement hierarchies in third millennium BC China: bioarchaeological perspectives in Zhengluo region. J. Archaeol. Sci. 145:105647. doi: 10.1016/j.jas.2022.105647
Tao, D., Zhang, R., Xu, J., Wu, Q., Wei, Q., Gu, W., et al. (2022b). Agricultural extensification or intensification: Nitrogen isotopic investigation into late Yangshao agricultural strategies in the middle Yellow River area. J. Archaeol. Sci. 44:103534. doi: 10.1016/j.jasrep.2022.103534
Tao, D., Zhang, R., Xu, J., Zheng, L., Zhang, G., and Zhang, G. (2023). Understanding the nature of early agriculture in the Central Plains of China (ca. 2600BC−221BC): Non-urban settlement perspective through archaeobotanical and stable isotope analysis. J. Archaeol. Sci. 51:104162. doi: 10.1016/j.jasrep.2023.104162
Tian, D., Sun, Y., Ritchey, M. M., Xi, T., Ren, M., Ma, J., et al. (2022). Varying cultivation strategies in eastern Tianshan corresponded to growing pastoral lifeways between 1300 BCE and 300 CE. Front. Ecol. Evol. 10:966366. doi: 10.3389/fevo.2022.966366
Vaiglova, P., Coleman, J., Diffey, C., Tzevelekidi, V., Fillios, M., Pappa, M., et al. (2021). Exploring diversity in neolithic agropastoral management in mainland greece using stable isotope analysis. Environ. Archaeol. 28, 62–85. doi: 10.1080/14614103.2020.1867292
Wallace, M., Jones, G., Charles, M., Fraser, R., Halstead, P., Heaton, T. H. E., et al. (2013). Stable carbon isotope analysis as a direct means of inferring crop water status and water management practices. World Archaeol. 45, 388–409. doi: 10.1080/00438243.2013.821671
Wallace, M. P., Jones, G., Charles, M., Fraser, R., Heaton, T. H., and Bogaard, A. (2015). Stable carbon isotope evidence for Neolithic and Bronze Age crop water management in the eastern Mediterranean and southwest Asia. PLoS ONE 10:e0127085. doi: 10.1371/journal.pone.0127085
Wang, X., Zhao, Z., Zhong, H., Chen, X., and Hu, Y. (2022). Manuring and land exploitation in the Central Plains of late Longshan (2200–1900 BCE) China: Implications of stable isotopes of archaeobotanical remains. J. Archaeol. Sci. 148:105691. doi: 10.1016/j.jas.2022.105691
Wang, Y., Song, S., Zhang, Y., Mei, S., Lu, X., Zheng, Y., et al. (2023). Brief report of the excavation of ancient rice paddies at the Shi'ao site, Yuyao city, Zhejiang. Archaeology 5, 3–21.
Wang, Z., Guo, X., Huang, S., Cao, X., Qiu, R., and Liu, C. (2016). Relationships between stable C and N isotope compositions of rice plants, rice yield and quality. Trans. Chin. Soc. Agric. Mach. 47, 160–171. doi: 10.6041/j.issn.1000-1298.2016.12.020
Weisskopf, A., Qin, L., Ding, J., Ding, P., Sun, G., and Fuller, D. Q. (2015). Phytoliths and rice: from wet to dry and back again in the Neolithic Lower Yangtze. Antiquity 89, 1051–1063. doi: 10.15184/aqy.2015.94
Wu, R. (2017). Preliminary Discussion of Neolithic Water Chestnut (Trapa) Remains from the Tianluoshan Site, China. Master's thesis. Zhejiang University.
Wu, Y., Jiang, L., Zheng, Y., Wang, C., and Zhao, Z. (2014). Morphological trend analysis of rice phytolith during the early Neolithic in the Lower Yangtze. J. Archaeol. Sci. 49, 326–331. doi: 10.1016/j.jas.2014.06.001
Xi, Y. (2014). Effects of green manure species and planting methods on rice yield, nutrient uptake and soil fertility. Master's thesis. Huazhong Agricultural University.
Xiao, Y. (2017). Study on Water, Carbon and Nitrogen Variations in Wetland Plant–Soil Continuum Based on Stable Isotope Techniques. PhD thesis. Wuhan University.
Xiao, Z. (1963). Preliminary study of regimes of green manure applied to paddy fields in Hunan Province. Sci. Agric. Sinica 9, 8–12.
Xiong, Q., Zhong, L., Chen, X., Zhu, C., Peng, X., He, X., et al. (2017). Effects of drought–floods abrupt alternation during panicle initiation stage on δ13C and δ15N stable isotope ratios of leaves in double–season super hybrid rice. J. Nucl. Agric. Sci. 31, 559–565.
Yoneda, M., Kikuchi, Y., Hiroo, N., and Yamazaki, K. (2019). “Background in stable isotope ecology and foundamental research in experimental paddy fields (in Japanese),” in Agricultural Culture Complex (II), ed. H. Shitara (Tokyo: Yuzankaku).
Yoneyama, T., Kouno, K., and Yazaki, J. (1990). Variation of natural 15N abundance of crops and soils in Japan with special reference to the effect of soil conditions and Fertilizer application. Soil Sci. Plant Nutr. 36, 667–675. doi: 10.1080/00380768.1990.10416804
Yoshida, T., and Ancajas, R. R. (1973). Nitrogen-fixing activity in upland and flooded rice fields. Soil Sci. Soc. Am. J. 37, 42–46. doi: 10.2136/sssaj1973.03615995003700010017x
Yuan, M., Liu, Q., Zhang, S., Chen, W., and Yu, H. (2011). Effects of biological nitrogen fixation and plow-down of green manure crop on rice yield and soil nitrogen in paddy field. Acta Pedol. Sinica 48, 797–803.
Yun, S., Lim, S., Lee, G., Lee, S., Kim, H., Ro, H., et al. (2011). Natural 15N abundance of paddy rice (Oryza sativa L.) grown with synthetic fertilizer, livestock manure compost, and hairy vetch. Biol. Fertil. Soils 47, 607–617. doi: 10.1007/s00374-011-0571-3
Zhang, G., Ji, Y., Liu, G., Ma, J., and Xu, H. (2014). Carbon isotope fractionation during CH4 transport in paddy fields. Sci. China Earth Sci. 57, 1664–1670. doi: 10.1007/s11430-014-4879-3
Zhang, J., Jiang, L., Yu, L., Huan, X., Zhou, L., Wang, C., et al. (2024). Rice's trajectory from wild to domesticated in East Asia. Science 384, 901–906. doi: 10.1126/science.ade4487
Zhao, Y. (2017). Effects of tillage and fertilization on anammox and denitrification rates in paddy soils. Master's thesis. Southwest University.
Zhao, Z. (2018). New thinking on the study of rice agriculture origin in China. Agric. Archaeol. 4, 7–17.
Zheng, Y., Chen, X., and Ding, P. (2014). Studies on the archaeological paddy fields at Maoshan site in Zhejiang. Quat. Sci. 34, 85–96. doi: 10.3969/j.issn.1001-7410.2014.01.11
Zheng, Y., and Jiang, L. (2007). Ancient rice remains discovered at the Shangshan site and its significance. Archaeology 9, 19–25.
Zhu, S., Sun, A., Zhang, Z., Wang, Z., and Du, P. (2013). Effects of different water-saving irrigation modes on rice tillering, height and yield in cold area. Water Saving Irrig. 12, 16–19.
Zhuang, Y., Ding, P., and French, C. (2014). Water management and agricultural intensification of rice farming at the late-Neolithic site of Maoshan, Lower Yangtze River, China. Holocene 24, 531–545. doi: 10.1177/0959683614522310
Keywords: stable isotope analysis, rice farming, watering regimes, manuring regimes, Lower Yangtze River basin
Citation: Wu R, Belfield EJ, Dong Y, Feng S, Fu X, Harberd NP, Yang F, Charles M and Bogaard A (2025) Rice stable carbon and nitrogen isotope values as potential indicators of watering and manuring regimes. Front. Environ. Archaeol. 3:1488754. doi: 10.3389/fearc.2024.1488754
Received: 30 August 2024; Accepted: 16 December 2024;
Published: 09 January 2025.
Edited by:
Darren R. Gröcke, Durham University, United KingdomReviewed by:
Xinyi Liu, Washington University in St. Louis, United StatesFerran Antolín, Deutsches Archäologisches Institut, Germany
Asta Rand, Memorial University of Newfoundland, Canada
Copyright © 2025 Wu, Belfield, Dong, Feng, Fu, Harberd, Yang, Charles and Bogaard. This is an open-access article distributed under the terms of the Creative Commons Attribution License (CC BY). The use, distribution or reproduction in other forums is permitted, provided the original author(s) and the copyright owner(s) are credited and that the original publication in this journal is cited, in accordance with accepted academic practice. No use, distribution or reproduction is permitted which does not comply with these terms.
*Correspondence: Rubi Wu, cnViaS53dTIwMjNAaG90bWFpbC5jb20=