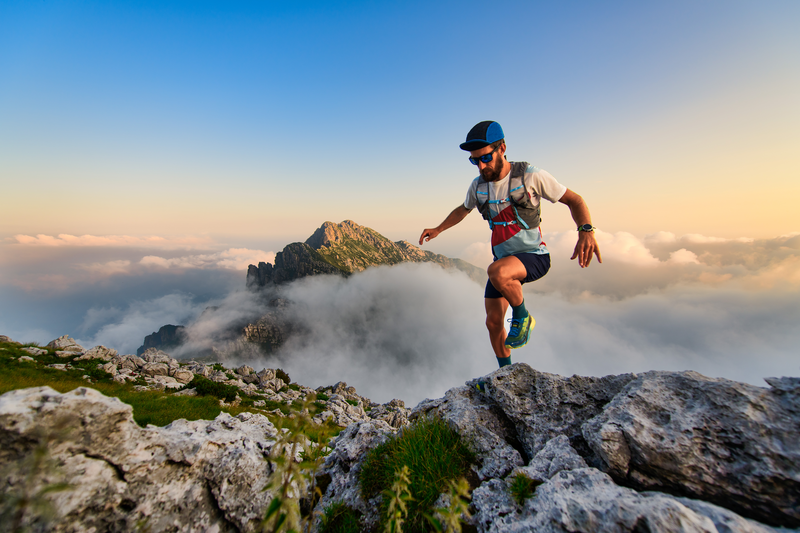
94% of researchers rate our articles as excellent or good
Learn more about the work of our research integrity team to safeguard the quality of each article we publish.
Find out more
ORIGINAL RESEARCH article
Front. Environ. Archaeol. , 14 January 2025
Sec. Landscape and Geological Processes
Volume 3 - 2024 | https://doi.org/10.3389/fearc.2024.1474357
This article is part of the Research Topic Natural Resource Exploitation in Mountain Environments: New Theoretical and Methodological Approaches View all 6 articles
Introduction: This study, conducted on the Plateau des Glières, in the northern French Alps, investigates the evolution of vegetation composition over the past 7000 years. This site, within a montane ecosystem, offers a new opportunity to understand the human and landscape interactions under the influence of climate. To study these interactions as a whole and by integrating their complexity, we used two conceptual frameworks: the agroecosystem and the trajectory.
Methods: The analyses conducted are based on a detailed analysis of pollen, NPP, macro-charcoal, and geochemical data from peat cores.
Results: The vegetation history of the Plateau des Glières is dominated by forest, due to its location within the montane belt (1,435 m). However, the vegetation composition trajectory of the Glières reveals three regimes: two are characterized by an evolutionary trend of the system, and between them, the a third regime is characterized by a “dynamic steady state” of the system. The first regime, corresponding to the Neolithic period, is initially characterized by a closed forested environment with a progressive trend toward a more open landscape due to the first agro-pastoral activities. The second regime is recorded from the very end of the Neolithic until the High Roman Empire. It corresponds to a relatively stationary system, characterized by the progressive development of pastoral activities leading to low impacts on the landscape. This long period (4300 to 1800 cal. BP) is characterized by the sustainability of the agroecosystem that developed. After this long pseudo-stable phase, a tipping point in the vegetation composition trajectory is highlighted. The third regime, spanning from the Migration Period to the present day, is the result of the intensification and diversification of agro-pastoral activities (pastoralism, cereal crops, and fruit trees). The landscape that we can see today in the Plateau des Glières is the legacy of this trajectory characterizing the interactions between ecosystems and human societies, i.e., the socio-ecosystem.
Discussion: While the agroecosystem trajectory reconstructed on the Plateau des Glières is rather consistent with the altitudinal model of anthropization previously proposed for this region, the vegetation response to the activities is unusual because it shows a long phase of ecosystem stability despite the relatively high human pressure.
Human societies have had a significant impact on the European Alpine landscape since the Neolithic period, when the first agro-pastoral activities began. A number of palaeoecological studies carried out in the Alpine chain have identified these early human impacts on the vegetation cover dynamics: the studies are based on the presence of indicators such as Cerealia and Plantago-type pollens (Finsinger and Tinner, 2007, 2006; Stahli et al., 2006), an increase in forest fires (Dietre et al., 2017; Pini et al., 2017; Schwörer et al., 2015; Wick and Tinner, 1997) and a decrease in arboreal pollen (van der Knaap et al., 2012). However, the intensity of these indicators depends on local conditions and remains relatively low at the Alpine scale. This low signal of anthropization means that is often difficult to detect in palaeorecords. These practices have only spread throughout the European Alpine chain since the Bronze Age (David, 2010a; Dziomber et al., 2023; Giguet-Covex et al., 2023; Guiter et al., 2005; Pini et al., 2017; Stahli et al., 2006; Tinner et al., 2003, 1996; Vugt et al., 2022; Walsh et al., 2007). However, the chronology of the different phases of anthropization differs between Alpine areas and, in particular, between different vegetation belts. Indeed, on the basis of sedaDNA analyses (Giguet-Covex et al., 2023), a model of altitudinal dynamics of agro-pastoral activity with different impacts on vegetation cover and erosion processes has been proposed for the north-western Alps. In this model of mountain anthropization, the Montane belt area, characterized by dense forests, does not record significant agricultural and pastoral activities until the Middle Ages, around 1,500 years ago (Bajard et al., 2016). These dynamics suggest the existence of a development trajectory for agro-pastoral activities that follows the altitudinal gradient (Giguet-Covex et al., 2023). However, in this previous study, most of the sites were at high altitude, that is, covering Montane and lower Sub-Alpine belts, and only two lakes were included.
To improve our understanding of socio-ecological trajectories of Montane belts, a new study has been carried out on a peat bog located at 1,435 m a.s.l of altitude, on the Plateau des Glières. The plateau has also been investigated by historians and archaeologists, providing additional information on the history of human occupation and, above all, on the human practices established.
In a multi-disciplinary approach, this palaeo-environmental study has the advantage of providing temporal continuity and temporal depth, going back over 7,000 years, that is, including the Neolithic period. Such temporal depth enables us to analyse the long-term evolution of the socio-ecosystem (including pre-anthropogenic impacts) and to discuss this evolution from a new perspective based on the concept of a trajectory reconstructed using a statistical approach. This analysis of the trajectory will provide a better understanding of the current state of the mountain's socio-ecosystems. The palaeo-environmental study is based on the combined analysis of pollen grains, spores of coprophilous fungi, macro-charcoals and geochemical elements archived in the peat deposits. The aim of this multi-proxy approach is (i) to reconstruct local and regional changes in vegetation cover over the last 7000 cal. BP; (ii) to identify agro-pastoral activities and their impact on the local vegetation and erosion dynamics; (iii) to distinguish between natural, climatically-induced, and human-induced (e.g., pastoralism, crops) vegetation dynamics; (iv) to increase the spatial resolution and to fill a gap in the palaeoecological data for this vegetation belt, and thus gain a better understanding of the spatio-temporal dynamics of landscapes in the north-western Alps.
The Col des Glières peat bog is located at an altitude of 1,435 m a.s.l (Figure 1) on the Plateau des Glières in the Bornes-Aravis massif, in the northern Pre-Alps. The Bornes massif is predominantly composed of sedimentary rock, particularly alternating between marls and limestone. More precisely, the Plateau des Glières, especially the peat bog, is made of Flysch and Marls, which are bordered by two mountains mostly made of limestones (Montagnes des Frêtes and Montagnes des Auges on the north-western and south-eastern parts of the Plateau, respectively). The vegetation of the plateau is characterized by mesophilic grassland used for mowing or grazing, wet meadows composed by Molina sp. and acidiphilous meadows with Nardus stricta (Figure 1). Numerous wetlands have also been identified, covering a large part of the plateau and Champ Laitier, further north. The slopes of Montagne des Frêtes, Sous-dine and Les Auges are covered by Picea-Vaccinium myrtillus forests, while the few rocky escarpments are covered by Pinus uncinata heathlands. Finally, the lower slopes are mainly characterized by Fagus-Abies forests. However, a few small areas have been colonized by Quercus sp. and Alnus sp.
Figure 1. Location and current vegetation around the Col des Glières peat bog. This map is based on the CNRS vegetation map—Annecy panel and the map of wetlands in Haute-Savoie.
Archaeological prospections were led on the Plateau des Glières to make an inventory of (1) the ancient and recent paths linking this area to the surrounding villages, (2) the remains of crossing structures (natural or carved passageways, retaining walls, ramps, etc.), and (3) of the occupation structures (ruins, shelters, etc.) (Mélo, 2025; Laissus, 2018). These prospections were performed based on land registers, aerial photographies and fieldwork campaigns. However, no excavations have yet been carried out in the structures. This limits the chronological interpretations, which are only based on land registers (Mappe Sarde 1730 and Etat-Major in 1868–1870) and written historical archives, which takes us back to the 13th century. This exploration work led to the identification of 67 remains of cabins, sometimes isolated in the landscape and forming perceptible agricultural units. Most of these ruins are associated with other structures such as retaining walls, enclosures, cellars or gardens. The presence of abandoned remains found in the buildings, such as cast-iron cauldrons, as well as the presence of cellars, underlines the pastoral use and, above all, cheese-making on the plateau, in the recent period. Other archaeological work close to the area (in North-Western Alps) or in similar massifs, is also included in the discussion to put our palaeoenvironmental results into perspective.
This study is based on the analysis of twin cores (GLI20-I A and GLI20-I B; N° IGSN CNRS0000023721) sampled using a Russian corer in the Plateau des Glières peat bog (Alps, France) in August 2020; both cores are from the same location, with only around 50 cm between them (45.96559900° N, 6.33330000° E, 1420 m a.s.l.). All the information relative to the coring system, scheme and section lengths can be found in the cybercarotheque website (https://cybercarotheque.fr/index.php?mission=&date1=&date2=&projet=&carotte=gli&repository=&recherche=Rechercher). The total master-core, that is, integrating the sections from the two cores GLI20-I A and GLI20-I B, measures 313.5 cm in length.
Avaatech XRF core scanning was performed on both cores, GLI20-I A and GLI20-I B, to correlate them and to obtain records of lead pollutions and the erosion dynamic. The upper 16 cm of core GLI20-I A are missing in the XRF signals. This is due to Sphagnum sp. moss accumulation, characterized by very low density which prevents the sensor from being correctly positioned on the sediment surface (protected by the ultralene) thus preventing attenuation of the signal in the air. The light elements, Aluminum (Al), Silicium (Si), Sulfur (S), Potassium (K), Titanium (Ti) and Calcium (Ca) were measured at 10 kV and 1 mA for 30 s, while the heavy elements Iron (Fe), Nickel (Ni), Copper (Cu), Zinc (Zn), Bromine (Br), Rubidium (Rb), Zirconium (Zr) and Lead (Pb) were measured at 30 kV and 0.75 mA for 30 s. In this study, only well-measured terrigenous (silicates and carbonates) elements that provide information regarding changes in sources of erosion were selected (i.e., Ti, Sr). Lead pollution is represented by ln (Pb/Rb), with Rubidium (trace element as lead) normalization eliminating lead inputs from rock erosion and the logarithmic expression allowing matrix effects to be reduced (water content, density, particle size) on the XRF measurement. This curve provides additional information that can be used to adjust or validate the age-depth model (lead pollution related to lead in gasoline, charcoal combustion and mining activities for the Roman period).
Nine sediment samples taken along the twin cores and corresponding to plant macroremains (Table 1) were dated with radiocarbon analyses (14C) at Poznan Radiocarbon laboratory (Table 1, “Poz“) and at LSCE lab (Table 1, “Sac”). The Clam (Blaauw, 2010) running on Rstudio (Posit Team, 2022) was then used to calibrate these dates with a confidence interval of 0.95 and to produce the age-depth model. The robustness of the age-depth model was then tested by comparing it with the XRF record of lead normalized by the rubidium (ln [Pb/Rb]). Rubidium is a purely clastic element not affected by weathering processes. It is thus used in the normalization to avoid lead inputs from the bedrock and to only track atmospheric inputs related to past pollutions: the maximum use of leaded gasolines in 1974 and the hemispheric pollution during the Roman Period recorded as far as the Greenland (Rosman et al., 1997). The age model was constrained over the first 10 cm by an additional date (1950 CE) based on pollen analysis (see below Section 3.2).
Forty-seven 1 cm3 samples, taken every 5–10 cm, were processed using standard methods (Faegri and Iversen, 1989) for preparing palynological samples. Carbonates, organic matter and silica present in the bulk sediments were destroyed by HCl, NaOH and HF, respectively. Then, pollen grains were isolated from the remaining organic and silicate residues by treatment with ZnCl2. Finally, acetolysis was carried out before the samples were mixed with glycerol. The residual preparations were then mounted on slides. A minimum of 500 pollen grains (except for five samples: 9.5, 19.5, 29.5, 39.5, and 49.5 cm with a grain count of between 319 and 466) per slide were counted using a Leica DM 1000 LED microscope with a ×63 lens and identified using pollen atlases (Beug, 2004; Reille, 1998, 1995, 1992) in order to estimate the pollen assemblage of each sample as accurately as possible.
A total of 19,464 pollen grains were counted and 86 pollen types were identified from the 38 upper core samples. The nine samples from the clays at the base of the sequence, that is, between 239.5 and 304.5 cm (Figure 2; Units 7 and 8) are sterile.
Figure 2. Age-depth model, lithological description and geochemical analyses of GLI20-I A. The age-depth model is based on radiocarbon dating and is validated or adjusted with the widely recorded lead pollutions during the Roman and Contemporary Periods. Yellow to orange boxes show old lead pollutions recorded in high altitude lakes in the western Alps. 1. Lake Laouchets Bis in Haut-Giffre Massif, Giguet-Covex, 2025; 2. Lake Anterne in Haut-Giffre Massif, Giguet-Covex et al., 2012; 3. Lake Robert in Belledonne Massif, Elbaz-Poulichet et al., 2020; 4. Lake Meidsee in Switzerland, Thevenon et al., 2011; and 5. Lake Serre de l'Homme in the Ecrin Massif, Giguet-Covex et al., 2019 and in Greenland, Rosman et al., 1997. The Munsell code references associated to each unit are written in brown.
The five main saprophytic and coprophilous fungal spore types (Sporormiella, Podospora, Sordariaceae, Coniochaeta, and Cercophora) were identified and counted on pollen slides using a ×40 lens. Fungal spore identification was carried out using the plates published by Van Geel (2002) and Cugny et al. (2010), as well as the NPPD (Non-Pollen Palynomorphs Database, “http://non-pollen-palynomorphs.uni-goettingen.de”). The counting procedure followed the method proposed by Etienne and Jouffroy-Bapicot (2014). The ecological interpretation of the selected fungal spores is based on the available literature (Dietre et al., 2012; Doyen and Etienne, 2017; Ejarque et al., 2011; López-Vila et al., 2014). Results are expressed in concentrations (number per gram of sediment) and fluxes (number per cm2 per year) based on estimated peat accumulation rates derived from the age-depth model (Figure 2).
The pollen and fungal diagrams (Figures 3, 4) were produced using the TILIA program (Grimm, 1990). The species abundances are expressed as a percentage of the total sum (base sum) of pollen grains corresponding to terrestrial species (arboreal, shrub and non-arboreal), excluding pteridophyte spores, Potamogeton pollen grains, Sparganium-type and Cyperaceae, which in the context of a wetland study may be over-represented in the pollen assemblages. The percentage of these excluded taxa (“tex”) is therefore calculated as %t = (sum of “tex” grains counted/(base sum + sum of “tex” grains counted)) ×100.
Figure 3. Simplified pollen diagram of the Glières sequence (in %). An exaggeration of a factor of 5 is represented for taxa that never reach 15%. Proportions for taxa with <1% over the whole sequence are represented by dots. Other trees and shrubs: Acer, Carpinus, Olea, Salix, Buxus, Ephedra Fragilis, Juniperus/Cupressus, Ilex Aquifolium, and Sambucus.
Figure 4. Diagram of spores of coprophilous fungi from the Glières sequence. This diagram shows both the results in terms of concentration (number/g of sediment) and flux (number/cm2/year).
Finally, the division of the different Local Pollen Assemblage Zones (LPAZ) is the result of a CONISS statistical analysis (Grimm, 1987) (Stratigraphically Constrained Cluster Analysis using the incremental sum-of-squares method), which groups assemblages by degree of similarity.
Two agro-pastoral activity indexes are also calculated following the same method as proposed in Rapuc et al. (2024) that is, based on a 0%−100% standardization of cultural and pastoral indicators from pollen and coprophilous fungi spore analyses and the calculation of an average value of these indicators. The first calculated index reflects the intensity of local activities (here, pastoralism) and includes spores of Sporormiella and ruderal, nitrophilous, adventice or apophyte pollen taxa, that is, Plantago-type, Rumex-type, Amaranthaceae/Chenopodiaceae and Urtica pollen types. Spores of Podospora were not included to avoid a relative overestimation due to its highly local representation (directly on the peat) compared to Sporormiella which reflects more the catchment scale (Etienne et al., 2013). The second index is called “regional index” because it represents arable farming developed more probably at lower altitude. This index includes Cerealia, Secale, Vitis, Cannabis/Humulus, Juglans and Castanea. Because of the relatively important abundance of Cerealia pollen in the modern samples (>3%), while there is no cultivation on the Plateau, we included cereals in the “regional” index (i.e., areas beyond the Plateau, including especially lower vegetation belts). The method used here differs from the Land Use Probability (LUP) calculation proposed by Deza-Araujo et al. (2022) for similar geographical areas, mostly because it also includes coprophilous fungi spore analyses, which required the standardization of all the data before their integration through an average [and not a sum as in Deza-Araujo et al. (2022)]. Our method of calculation means that it is not recommended to make comparisons between sites (i.e., to compare absolute values), but only within sites. However, our interests are rather (i) to evidence phases of relative changes in activity intensity on the study site and (ii) then, to compare these phases (with their relative intra-site intensities) between different areas (i.e., the different vegetation belts, e.g., with the two on-site indexes and between different mountain massifs based on the literature using the same (Rapuc et al., 2024) or similar approach (Giguet-Covex et al., 2023).
Eighty-seven contiguous sediment subsamples with 2–5 cm thick were taken along cores. Charcoal particles were extracted by soaking samples in sodium hydroxide (10% NaOH), in hydrogen peroxide (35% H2O2) to remove organic matter and by sieving the samples at 150 μm (Umbanhowar and McGrath, 1998). Charcoal particles were counted and measured under a binocular loupe at 40× magnification with a reticule grid. Charcoal identification was based on criteria defined by Rhodes (1998). The past local fire activity was reconstructed by calculating the charcoal accumulation rates in mm2/cm2/year.
An NMDS analysis was conducted utilizing the “MetaMDS” function within the Vegan package on the RStudio software (Posit Team, 2022). This analysis, which was specifically designed for the study of ecological communities, is considered to be the most robust gradient analysis and ordination method for studying this type of variable (Minchin, 1987). The NMDS ellipses were generated on the basis of the cultural periods to which the samples belong (Table 2). In order to combine the proportions of pollen taxa and the concentrations of spores of coprophilous fungi on the same NMDS, the two types of data were standardized. A threshold of 0.3% was applied to all pollen taxa in order to prevent taxa with a low occurrence from masking the overall dynamics.
The core retrieved from the Glières peatbog is composed of eight lithological units (Figure 2). The lower unit (U8, 313.5–260.5 cm) contains a mix of clayey and silty-sandy sediments with some gravel in the lower part of the unit (313.5–300.5 cm) and light gray mottled clays at the top (266.5–260.5 cm). Unit U7 (260.5–240.5 cm) is characterized by dark gray clays with the presence of small fragments of organic matter. From 240.5 to 161 cm, unit U6 is divided into three sub-units. Unit U6c is composed of a mix of organic (peat) and clastic sediments that are particularly rich in iron (Fe) and strontium (Sr). Unit U6b is more fibrous and highly enriched in all minerogenic elements presented in Figure 2 (Si, K, Ti, Fe, Rb, Zr, Sr). The last sub-unit, U6a, is characterized by very organic and compact peat accumulation, but is less fibrous than U6b. Unit U5 (161–138 cm) is made up of a fibrous and compact brown peat. Units U4 (138–132 cm) and U2 (116–16 cm) correspond to a homogeneous blond peat, but U2 is also characterized by a small increase in lithogenic elements (mostly Ti, Rb, Zr and Sr, Figure 2) in the upper 70 cm, with a sharper increase at the top, that is, from 35 cm to 16 cm-depth. Unit 3 (132–116 cm) is similar to unit 5. Finally, the first unit (U1 - 15–0 cm) is characterized by sphagnum moss accumulation.
The generated age-depth model reveals that the entire sequence (310 cm) covers more than 7,400 years (Figure 2). Three 14C dates were excluded from the analysis. The date at a depth of 67 cm is an age very similar (but younger) to that at 60 cm. To satisfy the principle of stratigraphic superposition, this date (67 cm) was excluded from the construction of the age-depth model. Without this date, the age-depth model better matches with the chronology of Roman lead pollution recorded within the other sequences in the Alps and in Greenland (Elbaz-Poulichet et al., 2020; Giguet-Covex et al., 2019, 2012; Rosman et al., 1997; Thevenon et al., 2011) (Figure 2). The 14C date at 156.9 cm-depth is older than the 14C date at 162 cm-depth. Consequently, this date was also rejected. Finally, the deepest 14C date (302.7 cm) appears to be significantly younger than the dates above so it too has been rejected.
The period covered by the palynological analysis (upper 229.5 cm) corresponds to the interval 7000 to 0 cal. BP. However, the age-depth model (Figure 2) presents an uncertainty in the dating of the last 200 years, corresponding to the top of the core (<15 cm). The upper centimeters of the peat core are characterized by low density sphagnum moss, which prevents accurate measurement with the XRF Core Scanner. Consequently, the Pb/Rb curve (Figure 2) does not record the lead pollution peak observed just before the oil crisis (1970′s) (Arnaud et al., 2012; Bindler, 2011; Boutron et al., 1991; Moor et al., 1996; Rosman et al., 2000; Shotyk et al., 1996). However, this part of the core has also been sampled for pollen analysis (samples 9.5 and 14.5 cm). A pollen sample taken at a depth of 9.5 cm indicates a phase of forest re-conquest, which is characteristic of the period of agricultural abandonment (around mid-20th century) and the start of mechanization (Elleaume et al., 2022). Based on this hypothesis, we set the date at 1950 CE at 9.5 cm with an uncertainty of 10 years.
The first zone, from 7000 to 5300 cal. BP (229.5–194.5 cm), is characterized by the presence of mesothermophilous species (Quercus, Ulmus, and Tilia), which gradually decrease throughout this phase (Figure 3). From 6600 cal. BP, the period is marked by the gradual decline of Abies and the appearance of Fagus, followed by the appearance of Picea at the end of the period, around 5200 cal. BP. Alnus and Betula also increase gradually throughout the period. The percentage of Arboreal Pollen (AP), which averaged 84% over the entire period, was much higher than herbaceous cover, despite the decline in mesothermophilous species and Abies. Monolete spores, which are quite abundant at the beginning of the period, decline sharply until they almost disappear at the end of the zone. Cyperaceae, which are relatively abundant (~10–15%), also decline during this period. Non-Arboreal Pollen (NAP) are dominated by Poaceae, Ranunculaceae-type, Rubiaceae and Asteraceae Cichorioïdeae but their abundances remain low (<10%). No indicators of agro-pastoral activities are recorded during this period, except the low occurrence of Amaranthaceae (<1%).
The spores of strict coprophilous fungi (Sporormiella, Podospora) are not very abundant during this phase, but Sporormiella and Sordariaceae tend to increase after 6000 cal. BP (fluxes and concentrations) (Figure 4). Cercophora, on the other hand, shows the opposite trend, with a concentration reaching its highest value but declining sharply until the end of the period.
The second period begins in 5300 cal. BP (194.5 cm) and lasts until around 4300 cal. BP (164.5 cm). This zone is characterized by major changes in the pollen assemblage. Arboreal pollen counts are the lowest of the entire sequence (65%) (Figure 3). Abies and mesothermophilous species (Tilia, Ulmus) declined sharply. Except for Corylus the proportions of which decline but remain relatively high, they are almost non-existent. The beginning of this period is also marked by the progressive transition phase between Abies and Picea. However, other deciduous species such as Betula, Alnus, Quercus and Fagus are relatively stable or increase slightly. Like the arboreal pollen, monolete spores sharply decreased during this period. In contrast, herbaceous species, particularly Poaceae and Cyperaceae (over 20%), expand. Ranunculaceae-type and Rosaceae-type are also well-represented, but to a lesser extent, with proportions of <15% and 5%, respectively. A weak Cerealia pollen signal is also observed around 4700 cal. BP. It is also important to note that the first Plantago-type pollen grains are identified during this period, although in very small proportions (<1%).
Finally, the spores of strict coprophilic fungi (Sporormiella, Podospora) and Sordariaceae are also much more abundant than in the previous zone (Figure 4). Concentrations and fluxes of Sordariaceae are the highest of the entire sequence. Sporormiella and Podospora also have very high concentrations and fluxes. Podospora only appears during this period. Cercophora presents low and stable concentrations and fluxes and disappears almost completely at the end of this zone.
The third zone, spanning from 4300 to 2000 cal. BP (164.5–59.5 cm), is divided into two sub-zones.
The first sub-zone of LPAZ 3 is characterized by the highest rates of arboreal pollen of the entire sequence (>95%) (Figure 3). Picea, as well as Abies which increase again during this period, each represent more than 20% of the pollen assemblage, while Fagus and Alnus each represent more than 12%. Pollens of Pinus, Betula, Corylus, and Quercus are also abundant, but to a lesser extent (<10%). Herbaceous taxa, in contrast to the previous period decrease and are not very abundant (<15%). Plantago-type pollen grains are also identified during this period, although in very small proportions (<1%). Cerealia pollen counts remain like those of the previous zone. However, Plantago-type and Cerealia are not recorded in all sample from this period. This phase is also marked by an increase of specific aquatic taxa such as Drosera and Sparganium-type (Supplementary Figure 1).
The proportions of spores of coprophilous fungi (except Coniochaeta) significantly decrease at the very beginning of this phase (Figure 4).
At the beginning of this period, Fagus and Abies decrease significantly, by more than 10% (Figure 3). In contrast, Alnus increases sharply, before decreasing in the middle of the period, around 2700 cal. BP, in parallel with the gradual expansion of Fagus. The other arboreal taxa are stable during this period. Thus, this sub-zone is still characterized by high levels of tree pollen, with significant proportions of Fagus, Alnus, Abies and Picea (over 15%). Regarding herbaceous taxa, whose total abundance does not exceed 16%, values of Plantago-type continue to increase throughout the period. However, although some Cerealia grains are identified, their proportion decreases significantly compared with the two previous zones. During this sub-zone, the Cyperaceae values are the lowest of the entire sequence (<12%).
Similar to the previous period, the spores of strict coprophilous fungi have relatively low concentrations but higher fluxes (Figure 4). Sporormiella, Sordariaceae, and Coniochaeta increase significantly during the period, as do Plantago-type pollen grains.
The fourth period, spanning from 2000 to 1000 cal. BP (59.5–34.5 cm), is characterized by a gradual decline of arboreal pollen in favor of an increase in herbaceous taxa, until the end of the sequence (from 10% to 20%) (Figure 3). Both Picea and Quercus decline (slightly) during the first part of the period. Around 1300 cal. BP, Alnus and Quercus increase again, followed by Picea at the very end of the period. This zone is also characterized by the appearance of Juglans and Castanea in the pollen assemblages. The appearance of these two taxa is also accompanied by a modest increase of Plantago-type and Cerealia and the first occurrences of Cannabis/Humulus at the end of the zone. Cerealia are continuously identified from this period.
At the same time, around 1200 cal. BP, both fluxes and concentrations of Sporormiella, Sordariaceae, and Coniochaeta increase significantly (Figure 4).
The fifth zone, which extends from 1000 to 200 cal. BP (34.5–14.5 cm), is characterized by a significant decrease of tree values mostly affecting deciduous trees (except the cultivated or human-promoted tree taxa, Castanea and Juglans) (Figure 3). This decrease is accompanied by an increase in herbaceous values. Although it fluctuates greatly, Pinus shows a general increasing trend during this period. The rates of Poaceae are very high in comparison to previous periods but never exceed those observed during LPAZ 2. Pollen indicators of agro-pastoral activities (Plantago-type, Cerealia, Rumex-type, Cannabis/Humulus, Amaranthaceae, Vitis) are also more abundant during this period. Other herbaceous taxa (Apiaceae, Asteraceae Asteroïdeae, Fabaceae) are also more abundant and more diverse. Finally, the Cyperaceae are once again more abundant.
Regarding the spores of coprophilous fungi, on the one hand the concentrations of Sporormiella are the highest in the sequence, as are those of Sordariaceae and Coniochaeta (Figure 4). On the other hand, the fluxes, although also high, are no higher than during the previous phase.
The last zone is characterized by a re-increase in tree pollen taxa, particularly Picea and Pinus (Figure 3). Pollen grains of Pinus have never been so abundant within the sequence. Conversely, the proportions of Abies have never been so low (<2%). Deciduous taxa, such as Quercus, Corylus, and Fagus are tending to decrease. Herbaceous taxa, such as Poaceae and Plantago-type, decrease at the end of the sequence. Cerealia and Cannabis/Humulus also decline slightly during this period, with Cannabis/Humulus, finally disappearing. On the other hand, Cerealia levels remain quite high (1.9%).
The spores of coprophilous fungi decrease during this period and are almost absent in the last sample, except the Sordariaceae which are recorded but in very low concentrations (<250 spores/g of sediment) and fluxes (<5 spores/cm2/year) (Figure 4).
The NMDS analysis reveals different vegetation compositions in the Glières sequence according to the different cultural periods (Figure 5). The temporal trajectory of the composition shows three main regimes. The first regime, corresponding to the Neolithic period, is characterized by a closed and forested environment with a progressive trajectory along axis 1 toward a more open landscape due to the first agro-pastoral activities (Figure 5). In the second regime, the trajectory undergoes a pseudo stable phase over a period of more than 2,500 years (until the High Roman Empire) which echoes the concept of Ecological Dynamic Regime, defined by Sánchez-Pinillos et al. (2023) as “the dynamic property of steady states.” In greater detail, this phase is characterized by the progressive development of pastoral activities leading to low impacts on the vegetation cover which highlight the sustainability of the agroecosystem that developed. After this long phase, that is, from the Migration Period, ca. 1500 cal. BP, a tipping point in the vegetation composition trajectory is highlighted leading to a new operating regime following axis 2 up to the present day. This regime is characterized by taxa indicative of agro-pastoral activities, in particular cereal crops and fruit trees. The trajectory of the Plateau des Glières thus shows a major tipping period in the socio-ecosystem during the Migration Period/Early Middle-Ages, highlighting the significant and non-reversible impact of human activities on the vegetation composition from that time onwards. While this general trend is obvious, short-term dynamics are more complex to decipher, due to the limited number of samples available for each period (except for the Iron Age). The evolution of this trajectory and its major changes will be discussed in relation to the dynamics operating in other sites in the area and in the western European Alps generally.
Figure 5. Non-metric multidimensional scaling analysis (NMDS) of the Glières samples. The samples are categorized according to the Local Pollen Assemblage Zone (LPAZ) and the cultural period to which they belong. The species panel presents the different individuals (pollen and fungal taxa) from the NMDS analysis and the second panel presents the different samples characterized according to their belonging to a LPAZ (shape) and to a cultural period (color). Due to the small number of samples for the High and Late Middle-Ages categories, they have been combined together in the Middle-Ages category.
From 7000 to 5300 cal. BP, the trajectory is along axis 1 of the NMDS (Figure 5). This trajectory is influenced by the dynamics of forest taxa such as mesothermophilous trees (Ulmus, Corylus, Tilia, and Quercus) and Abies. These taxa, combined with high abundances of monolete spores and saprophytic fungi (Cercophora), suggest a wet forested landscape. From 8200 cal. BP, the mesothermophilous trees are gradually replaced by Abies in Montane belt areas of the northern French Alps (David et al., 2002; David, 2010b; de Beaulieu et al., 1993). Indeed, the 8.2 ka event led to high humidity levels (Finsinger and Tinner, 2006; Magny et al., 2003; Tinner and Lotter, 2001, 2006), which facilitated the expansion of Abies and subsequently Fagus. In the north-western Alps, Fagus appeared between 7500 and 7000 cal. BP (David et al., 2002; Joannin et al., 2013; Rey et al., 2022, 2013; Schwörer et al., 2015; Thöle et al., 2016; Tinner et al., 1996; Wick and Möhl, 2006), which correlates closely with the beginning of the dynamic recorded in the Glières sequence. Since 6500 cal. BP on the Glières sequence, Abies forests exhibited a gradual decline allowing the expansion of Picea, especially from 5500 cal. BP. These changes coincides with the other records from the north-western Alps, although slight temporal discrepancies are also observed [e.g., 6000 cal. BP at Lake Annecy (David et al., 2002), and at Survilly (David, 2010b) around 5700 cal. BP]. These discrepancies may be due to local variations and/or to the variations in pollinic rainfalls recorded in each site and/or uncertainties in the age-depth models. This change in forest composition also coincides with what has been observed in the Alps, where Picea first colonized the eastern part at the beginning of the Holocene (Henne et al., 2011) and migrated progressively to the western part (van der Knaap et al., 2005) around 8500 cal. BP, arriving in the Swiss Alps between 7000 and 6000 cal. BP (Finsinger and Tinner, 2007; Rey et al., 2022, 2013; Schwörer et al., 2015; Thöle et al., 2016; Tinner et al., 1996). Although the appearance of Picea is relatively synchronous between all sites in the north-western Alps, the rapidity of the shift in abundance, when Picea becomes more abundant than Abies, varies depending on the sites. This delay may be explained by the difference in altitudes and by the spatial representativity of the pollen rain fall (local vs. regional) in the archives and by the fact that this major change in forest composition is thought to be the result of a combination and succession of anthropogenic and climatic disturbances (de Beaulieu et al., 1993; Wick and Möhl, 2006). In the western Swiss Alps, the increase in anthropogenic fires around 6000 cal. BP could explain the decline of Abies, which is very sensitive to such disturbances (Schwörer et al., 2015; Tinner et al., 1999; Tinner and Lotter, 2006). Conversely, these dynamics, followed by the colder and wetter conditions, that characterize the transition toward the Neoglacial period, probably favored the expansion of Picea (Pini et al., 2017; Rey et al., 2013; Schwörer et al., 2015; Wick and Tinner, 1997).
In the Glières sequence, concomitantly with the appearance of Picea around 5500 cal. BP, a significant increase in erosion is recorded affecting the silicate substratum but even more the carbonated ones, as evidenced by the detrital elements and Sr, respectively (Figure 6). Such an increase in erosion is reported at exactly the same time at lake Anterne located 35 km from the Glières Plateau. Although human impact cannot be totally excluded, this dynamic can be interpreted as a response to the transition toward the Neoglacial period characterized by colder and wetter climatic conditions, whether for Anterne (Giguet-Covex et al., 2011; Pansu et al., 2015) or the Glières Plateau. Indeed, first evidences of human activities are not recorded before 5300 cal. BP at Glières and even later at Anterne (possibly from ca. 4800 cal. BP; Giguet-Covex et al., 2014).
Figure 6. Summary of the results obtained from the various analyses (pollen, spores of coprophilous fungi, macro-charcoal, lithology, geochemistry) carried out on the Glières sequence. From left (oldest samples) to right (most recent). BA, Bronze Age; RP, Roman Period; MP, Migration Period; MA, Middle Ages; MOD, Modern Period; CP, Contemporary Period.
From the beginning of the important increase of Picea and decrease of Abies around 4700 cal. BP, the general vegetation composition trajectory seems mostly influenced by the development of the first agro-pastoral activities at a local scale (i.e., Podospora, Sporormiella, Poaceae, Plantago-type, local pastoral index) but also possibly at lower altitudes (i.e., Cerealia, regional agricultural index) (Figures 5, 6). However, the Abies/Picea succession recorded in the Glières reflects a regional dynamic triggered by several local anthropogenic activities in the area but not specifically those recorded on the Plateau des Glières. Indeed, no increase in fire signal is recorded in the Glières sequence, as is usually observed in other Alpine sites (Schwörer et al., 2015).
From 5300 to 4300 cal. BP, a first phase of landscape opening is recorded, leading to high levels of Poaceae and an increase in taxa richness (Figure 6). Although, this could be due to a change in edaphic conditions, which facilitated the development of a more hygrophilous vegetation (with abundant Cyperaceae and Poaceae taxa), the hypothesis of first human disturbances, locally on the Plateau, is favored. Many pieces of evidence provided by our record and synthesized through the agro-pastoral indexes lead us to this conclusion, which is otherwise supported by other palaeoenvironmental and archaeological research in the region. Pastoralism is suggested by the increase (flux and concentration) of spores of the strict coprophilous fungi, Sporormiella and Podospora (Figure 4), which only develop on the excrement of herbivores (Cugny et al., 2010; Etienne et al., 2013). While Sporormiella may originate from the entire catchment area, the high amount of Podospora spores, is interpreted as being due to the immediate presence of animals, probably directly on the peat bog (Etienne et al., 2013). Secondly, although the Plantago-type pollen does not occur in significant amounts during this period, it is present and its proportions increases more significantly at the end of this phase, that is, around the transition with the Bronze Age (Figure 3). This result may be interpreted as a delayed response of the vegetation to these first pastoral activities. Although, the presence of strict coprophilous fungi spores may not only be caused by domestic herbivores but also wild ones, the hypothesis of an anthropogenic impact on the vegetation trajectory is preferred based on the appearance of Cerealia pollen grains. Cereals were probably grown at lower altitudes, and attest to farming activities not very far from the Plateau des Glières. These results can also suggest a certain mobility of the mountain population in the area, with a subsistence based on both raising livestock and cultivating crops.
Such agricultural and pastoral activities are known in the Alps during the Late Neolithic period, from archaeological research and other palaeoenvironmental records. Several “sheepfold caves,” which were occupied in the Early and/or Late Neolithic, are known in the pre-Alps and Sub-Alpine massifs (Argant et al., 1991; Delhon et al., 2024; Ginestet et al., 1984; Martín and Tornero, 2024; Nicod, 2008; Nicod and Picavet, 2003; Remicourt, 2009). In particular, <8 km from the Glières Plateau around 620 m a.s.l. (Figure 7), the cave called “La Balme-de-Thuy” was occupied from the Mid-Neolithic period (Ginestet et al., 1984; Remicourt, 2009). At a more regional scale, archaeological excavations in Grotte de Diois (Vercors massif) have confirmed the presence of several herds between 6450 and 3950 cal. BP (Argant et al., 1991; Brochier, 1986; Carrier and Mouthon, 2010). In addition, some palynological studies in the Northern French Alps also mention the increase in pastoral and agricultural evidence, at the end of the Neolithic period. Indeed, further east, on the other side of the Swiss border, Plantago-type and Sporormiella are recorded in the Lake Champex at the same time and altitude (1467 m a.s.l) as in the Glières Plateau (Rey et al., 2022, Valais region). In addition, this phase coincides with the detection of sheep and cattle DNA between 5400 and 4400 cal. BP, in lake La Thuile (874 m) (Giguet-Covex et al., 2023) (Figure 7). The differences in the agro-pastoral intensity index observed between this lake (which is barely noticeable compared to the other periods at this site) and the Glières Plateau (Figure 7) may reflect more intense activity in the Glières than at Lake La Thuile or it may more probably be due to lake taphonomic differences in the indicators used for each site. At a more regional scale, some studies in the Swiss Alps mention the appearance of Cerealia, Plantago-type or spore of coprophilous fungi in the Late Neolithic (Dietre et al., 2017; Garcés Pastor et al., 2022; Gobet et al., 2003; Hafner and Schwörer, 2018; Pini et al., 2017; Schwörer et al., 2015; Stahli et al., 2006; Tinner et al., 2003). An increase in the frequency of anthropogenic fires due to the practice of slash-and-burn, has also been observed in the Swiss Alps and the Jura (Dietre et al., 2020, 2017; Doyen et al., 2016, 2013; Gobet et al., 2003; Schwörer et al., 2015). However, this practice does not seem to occur in the Glières Plateau as no significant increase in the fire signal has been observed (Figure 6). At a broader scale, agro-pastoral practices have also been documented further west in the Massif Central since the beginning of the Neolithic and seem to have intensified at the end of the Neolithic with the occurrence of Cerealia and the increase in spores of coprophilous fungi (Dendievel et al., 2019; Miras et al., 2018).
Figure 7. Summary of vegetation dynamics and agro-pastoral activities in the north-western Alps. This figure shows the results of six additional sequences in the north-western Alps. All these sequences are from lake sites, except for the Glières. La Thuile (Bajard et al., 2015a) is located at the transition between the Collinean and Montane belts, while Gers (Bajard et al., 2020) and Bénit (Bajard et al., 2018) are in the Montane belt, and Anterne (Giguet-Covex et al., 2014) and Les Laouchets (Giguet-Covex, 2025) are in the Sub-Alpine belt. Pollen data are presented in percentages (Trees/Shrubs, Plantago-type, Cerealia), DNA results in number of reads (Plantago, Cattle, Sheep, Goats) except for Trees/Shrubs which are also presented in %. Data on spores of coprophilous fungi are presented in flux (nb/cm2/year). Each taxon associated with a type of data is represented using the same scale for all sites (except the tree/shrubs results for the Anterne DNA analysis and the Sporormiella results from Lake Gers). The agro-pastoral intensity Index is an index calculated based on the aggregation of all (agro) pastoral activity proxies when available: pollen taxa (Amaranthaceae, Plantago-type, Rumex-type, Urtica), DNA analysis (Plantago, Rumex, Cattle, Sheep) and Sporormiella. RO, Roman Period; MP, Migration Period; MOD, Modern and Contemporary Period.
It now seems that evidence of these potential Neolithic activities can be found, with varying degrees of intensity, over a wide range of altitudinal belts in the northern French Alps. Could this recurrence confirm that agro-pastoral activities were practiced on a regional scale during the Late Neolithic, but with very local impacts on the landscape? During the Neolithic, small communities would have spread within Alpine territory, heterogeneously engaging in agro-pastoral activities in the area. This explains the variability of the anthropogenic signals recorded at sites in the northern French Alps, highlighting the development of these pioneering activities with very local impacts on vegetation.
From the end of the Neolithic period to the High Roman Empire, a pseudo stable phase is recorded, with small fluctuations of the vegetation composition around an average (Figure 5). During this phase, the gradual development of pastoral (local) and agricultural (regional) activity moderately influenced the landscape.
The Bronze Age and Iron Age periods correspond to a phase with a high level of forest cover, which persisted for more than two millennia (Figures 3, 6).
Low intensity agro-pastoral activities were identified on the Glières Plateau and below until the Mid-Late Bronze age period. In contrast, numerous studies have identified increasing agro-pastoral practices during the Bronze Age in the Alps and Jura (Doyen et al., 2013; Dziomber et al., 2023; Garcés Pastor et al., 2022; Pini et al., 2017; Stahli et al., 2006; Tinner et al., 2003, 1996). However, these sites are situated at either lower or higher altitudes than the Glières Plateau. At that time, it seems that people preferred to invest in higher, more naturally open environments. A comparable situation to that at Glières, is observed at Lake Gers (1,450 m a.s.l) and Lake la Thuile (Bajard et al., 2020, 2015a,b; Giguet-Covex et al., 2023) (Figure 7).
From 3000 cal. BP (Late Bronze/Early Iron Age), concomitantly with the increase in the richness of herbaceous species, we observe an increase in intensity toward moderate activity intensities in the Glières (increase of Sporormiella, Sordaria, Plantago-type, agro-pastoral index) (Figure 6). In the Iron Age, around 2300 cal. BP the rise in the fire signal could also indicate the first important human-triggered land clearance, which mostly affected deciduous trees (especially Fagus), to develop the pastoral activities as suggested by the concomitant increase in local pastoral activity index.
Signals of moderately intensive human activity have been recorded during the Iron Age in Montane belt sites such as lakes La Thuile, Champex and Gers (Bajard et al., 2020, 2015b; Rey et al., 2022) (Figure 7). At high altitude (Anterne, Savine), the signal is much stronger (sometimes slightly later: end of the Iron Age) suggesting more intense pastoral activities (Giguet-Covex et al., 2014; Sabatier et al., 2017). The signal of pastoral activities recorded in the Glières seems weaker than in the Sub-Alpine or Alpine belts but greater than that recorded on sites in the Montane belts. This record may be interpreted as reflecting a specific local dynamic due to the topography of the Glières Plateau. Indeed, this flat topography may have favored early clearing of the forest, compared with other sites located in the same vegetation belt.
Although we have only one sample representing this period, it is characterized by the first appearance of Juglans and Castanea (around 2000 cal. BP). These two species, grown at lower altitudes, are indicative of the beginning of the footprint of the Roman civilization on the Alpine landscape. Doyen et al. (2016) also observed the development of these taxa in the peri-Alpine Lake Paladru. In addition, cultivated species originating at lower altitudes (Cerealia and Vitis) are detected (Figure 6). The fire signal is lower than during the Iron Age but is still at a high level. This may be due to the progressive abandonment of the slash-and-burn system for a system aimed at maintaining the open landscape (Doyen, 2013). Despite the occurrence of Plantago-type pollen grains, pastoralism does not appear to have been an important activity in the Glières Plateau.
For this period, numerous studies in the Alps have observed a development and diversification of agriculture (Tinner et al., 1996), that are characterized by varying degrees of intensity depending on the altitudinal belts (Giguet-Covex et al., 2023). Several Sub-Alpine/Alpine sites in the Swiss, Italian and French Alps recorded an increase of the agro-pastoral indicators during this period (Dziomber et al., 2023; Finsinger and Tinner, 2007; Pini, 2002; Thöle et al., 2016). In the Northern French Alps, pastoral activities are also mainly recorded in these high altitudinal belts, as for instance at lakes Anterne, Verney and Savine (Giguet-Covex et al., 2023; Sabatier et al., 2017). At lower altitudes, like the Plateau des Glières, the Swiss Lake Champex sequence also recorded an increase in agro-pastoral indicators (Rey et al., 2022). The authors suggest that the surrounding area may have been used as an “intermediate seasonal stopover to reach the grazing grounds at higher altitude.” This hypothesis is supported by the results of DNA analyses of numerous sites located at intermediate altitudes in the northern French Alps, such as lakes La Thuile (874 m) and Gers (1,450 m a.s.l) which also suggest moderate local pastoral activity (Giguet-Covex et al., 2023) (Figure 7). Pastoral activities also developed in peri-Alpine areas (Arnaud et al., 2016; Doyen et al., 2016, 2013). All this evidence reflects the expansion of livestock farming and pastoralism within the territory with the exploitation of all vegetation belts. However, there are some particularities, such as in the Bornes-Aravis massif as shown by the Glières sequence and the lake Bénit sequence. Indeed, no significant proportions of pastoral indicators are recorded for this period (Bajard et al., 2018) (Figure 7). These differences suggest greater local variability in human activities in the lower/intermediate belts, compared to the higher altitudes.
This period marks a transition between the dynamics observed previously and the Middle Ages. The trajectory is progressively negatively oriented along axis 2 of the NMDS (Figure 5), with the influence of cultivated taxa (Cerealia, Vitis, Castanea, Cannabis/Humulus). Indicators of local pastoral activity (local agro-pastoral index, Sporormiella, Plantago-type, Rumex-type) appear to increase during this transition (Figure 6), as they do in other sites at similar altitude, such as lakes La Thuile and Gers (Figure 7). In addition, at lower altitudes, agrarian activities increase, as evidenced by Cerealia, fruit trees and the regional index of activity (Figure 7). This last observation is more evident at Lake La Thuile probably because of its lower altitude, which would have favored the local development of these cultures, especially cereals as well as hemp and green beans as evidenced by pollen and sedaDNA analyses, respectively.
The temporal resolution of the Glières sequence does not provide a precise record of environmental dynamics and human activities during the medieval period. It is therefore difficult to cross-reference palaeoecological information with recent historical and/or archaeological works carried out on the plateau (Laissus, 2018, 2025). However, the trajectory illustrated by the NMDS analysis (Figure 5) highlights a profound change in the composition of the vegetation, with significant increases of cultivated taxa (Cannabis/Humulus, Castanea, Juglans, Cerealia, Secale, and Vitis) and pastoral indicators (Sporomiella, Sordariaceae, Plantago-type). The average of all these indicators represented by the agro-pastoral activity index (Figure 7) shows the most intense phase of agro-pastoral activities on the local scale and even on a more regional scale (with the cultivated taxa). The fire signal (concentrations and fluxes) also suggests an intensification of the human impact on the landscape in the High Middle Ages. In addition, this period is characterized by the greatest richness of pollinic taxa and constitutes the second major phase of landscape opening in this sequence (after the Late Neolithic).
In addition, Fagus declines sharply from the beginning of the medieval period and never recovers to its previous levels. This decline is probably the result of human activity. Fagus was an important component of ancient mountain forests and an excellent source of firewood (Rochel, 2017). It is therefore possible that its decline was directly linked to its use in charcoal pits to then produce the required resource in the glass and/or metallurgical industries from the end of Antiquity (Gouriveau, 2020). In geochemical measurements, titanium (Ti) and strontium (Sr) slightly increases throughout the medieval period, reflecting the first, but moderate, increase in erosion since the beginning of local human activities (Figure 6). This erosion phase affects more the silicated substratum than the carbonated ones compared to the early and probably natural erosion phase (Early to Late-Neolithic). This result may indicate that human activities and/or land clearance are more concentrated on the flat part of the Plateau des Glières made of flysch and marls, while the steep calcareous slopes on either side of the plateau are less exploited. This increase in erosion is in line with the results obtained by Rapuc et al. (2024) at a larger scale, that is, in the Arve and Rhône catchment areas upstream of Lake Bourget (including the Glières Plateau), who observed an increase in human triggered erosion during the Middle Ages. In addition, the oldest medieval archives dating to the 13th century revealed that the plateau was seasonally used for pastoralism until the 18th century (Laissus, 2018; Mélo, 2025).
Such an intensification of agro-pastoral practices is commonly recorded during the Middle Ages in various Alpine regions (Bajard, 2017; Garcés Pastor et al., 2022; Tinner et al., 1996; van der Knaap et al., 2012). From the High Middle Ages, the practices initiated in Antiquity became more established and diversified in the Jura (Doyen et al., 2013) and throughout the Alpine chain (Finsinger and Tinner, 2007, 2006; Giguet-Covex et al., 2023; Rey et al., 2013; Thöle et al., 2016; van Vugt et al., 2022). Whether at low altitude in peri-alpine lakes (Doyen et al., 2016; Messager et al., 2022) or at mid and high altitudes (Bajard et al., 2020, 2018; Giguet-Covex et al., 2014) in the north-western Alps, all the indicators of agro-pastoral activities increase and highlight a phase of agricultural intensification (Figure 7).
The single sample documenting the Late Middle Ages seems to show that these dynamics declined during this period. The fire signal, which has been analyzed at a higher resolution, shows a clear decrease in intensity at the end of the Middle Ages. These dynamics are consistent with other records in the same altitudinal belt, such as those from lakes Bénit and Gers, but run contrary to the other records from lower and upper vegetation belts in the North-Western Alps discussed in the previous sections (Doyen et al., 2016; Giguet-Covex et al., 2023; Rapuc et al., 2024) (Figure 7).
During the 20th century, a period of reforestation is observed in the Glières sequence. There is a marked decline of agro-pastoral indicators (Sporormiella, Plantago-type, Cerealia, Cannabis/Humulus) and agro-pastoral activity index (Figures 6, 7) indicating the abandonment of cultivated land and pastures in lower and intermediate belts and leading to a gradual landscape closure. Despite a marked reduction in species richness associated with this reforestation phase, some herbaceous plants are still present, characterizing the grassland ecosystems that currently exist on the plateau. Tree species in decline (Abies, Fagus, Quercus, and Corylus) during this period are no longer present in the catchment area, as shown in Figure 1. On the contrary, the species currently present (Picea, Pinus) show a strong recolonization of the site despite the maintenance of pastoral activity on the plateau and the development of recreational activities (Nordic skiing in particular). This reforestation phase in subsequent to the abandonment of agricultural land is known throughout the Alpine chain (David, 2010b; de Beaulieu et al., 1993; Elleaume et al., 2022) (Figure 7). A decline in agro-pastoral activities is also documented through the comparison between the historical land registers (Mappe Sarde and Etat-Major map) and contemporary aerial photographies, showing less cabins but an increase of ruins of buildings (Laissus, 2018). However, formerly grazed areas were maintained and their expansion encouraged, particularly in Alpine and Sub-Alpine zones (Bajard, 2017; Doyen et al., 2013; Richer, 2009; Stahli et al., 2006; van der Knaap et al., 2012).
Despite this reforestation dynamic, which is considerably modifying the landscape, the NMDS analysis (Figure 5) does not show any trend toward a return to a vegetation composition characteristic of a pre-agropastoral system state. These results can be interpreted as reflecting the cumulative effects of changes in the landscape over the last five millennia, in other words they are the legacy of history.
This study has shown that the evolution of the vegetation on the Plateau des Glières, located at the transition between the Montane and Sub-Alpine belts, has been influenced by numerous factors. The comparison of this new sequence with other studies carried out in the area highlights spatial and temporal differences that are partly linked to altitude, as identified by Giguet-Covex et al. (2023). However, we also suggest that some of these differences are due to the specific topography of the Plateau des Glières. Indeed, although the plateau is predominantly characterized by forest ecosystems, its topographical context may have favored (agro-)pastoral activities earlier than suggested by the other pollen records located at the same altitude in this area.
The Glières peatbog records indicate that a first phase of anthropization associated with pastoral activities and cereal crops, probably at lower altitude, seems to have occurred during the Late Neolithic. This confirms the hypotheses formulated about possible anthropization on other sites throughout all the altitudinal belts in the sector. However, these activities seem to be more pronounced in Glières sequence. The next phases of agro-pastoral activities on the Glières Plateau are recorded from the Bronze Age to the beginning of the Iron Age. In contrast to Sub-Alpine/Alpine sites, the Montane site of Plateau des Glières experienced moderate pastoral pressure, which lead to a moderate ecosystem response characterized by a dynamic steady state. Such agroecosystem can be defined as sustainable. Then, the Migration Period represented a major tipping point in the Glières vegetation composition trajectory, as in other Alpine areas within the same vegetation belt, leading to the fragmentation of the regional and local landscape by diversified land uses. These changes in the landscape are linked to the development of fruit tree cultivations and agricultural practices, which gradually expanded during the medieval period and continued into the modern period. From this tipping period, the agro-pastoral pressure increased greatly, preventing the system from remaining as stable as it was in the second regime phase. By revealing these three regimes in the agroecosystem trajectory, this study highlights that pastoral resources (regime 2) has been exploited for more than two millennia without profoundly impacting ecosystems, creating a stable dynamic of interaction between human and the environment. This long phase of stability may also have been favored by the flat topography of the Glières, by limiting soil erosion processes and thus by reducing disturbances to vegetation dynamic. This history points to good management practices that could inspire current policies.
The new palynological sequence of the Plateau des Glières contributes to a better understanding of the agro-pastoral history and human impacts on landscapes in the north-western Alps, by filling temporal and spatial (especially, altitudinal) gaps. However, the complex spatial dynamic of human-landscape interactions cannot be fully captured only based on the study of natural archives, as their availability is limited. Furthermore, pollen records are not spatially resolved and spatially-quantitative because they do not consider the dispersion and deposition patterns of pollen grains, which can lead to low detection of agro-pastoral activities. To meet this challenge, a useful approach would be to apply modeling to pollen data.
The original contributions presented in the study are included in the article/Supplementary material, further inquiries can be directed to the corresponding author.
AJ: Conceptualization, Data curation, Formal analysis, Investigation, Visualization, Writing – original draft. EM: Conceptualization, Funding acquisition, Investigation, Project administration, Supervision, Writing – review & editing. ED: Formal analysis, Investigation, Writing – review & editing. DE: Validation, Writing – review & editing. LM: Validation, Writing – review & editing. CG-C: Conceptualization, Formal analysis, Funding acquisition, Investigation, Writing – original draft.
The author(s) declare financial support was received for the research, authorship, and/or publication of this article. The PCR project (Ecosystèmes montagnards du Moyen-Age à nos jours, trois cas hauts-savoyards: Sales, Salève, Glières) provided financial support for this study, covering the cost of pollen analysis and macro-carbon analysis, and radiocarbon dates and human-resource for a 6-month internship to carry out the pollen analysis.
We thank the PCR project (Ecosystèmes montagnards du Moyen-Age à nos jours, trois cas hauts-savoyards: Sales, Salève, Glières). We would also like to thank the Syndicat Mixte des Glières and the DDT for their support in coring the peat bog. We also thank Fanny Canone for the preparation of the pollen samples. We express our gratitude to Rhoda Allanic for correcting the English text.
The authors declare that the research was conducted in the absence of any commercial or financial relationships that could be construed as a potential conflict of interest.
All claims expressed in this article are solely those of the authors and do not necessarily represent those of their affiliated organizations, or those of the publisher, the editors and the reviewers. Any product that may be evaluated in this article, or claim that may be made by its manufacturer, is not guaranteed or endorsed by the publisher.
The Supplementary Material for this article can be found online at: https://www.frontiersin.org/articles/10.3389/fearc.2024.1474357/full#supplementary-material
Argant, J., Heinz, C., and Brochier, J.-L. (1991). Pollens, charbons de bois et sédiments: l'action humaine et la végétation, le cas de la grotte d'Antonnaire (Montmaur-en-Diois, Drôme). ArchéoSciences, revue d'Archéométrie 16, 29–40. doi: 10.3406/arsci.1991.1255
Arnaud, F., Poulenard, J., Giguet-Covex, C., Wilhelm, B., Révillon, S., Jenny, J.-P., et al. (2016). Erosion under climate and human pressures: an alpine lake sediment perspective. Quat. Sci. Rev. 152, 1–18. doi: 10.1016/j.quascirev.2016.09.018
Arnaud, F., Révillon, S., Debret, M., Revel, M., Chapron, E., Jacob, J., et al. (2012). Lake Bourget regional erosion patterns reconstruction reveals Holocene NW European Alps soil evolution and paleohydrology. Quat. Sci. Rev. 51, 81–92. doi: 10.1016/j.quascirev.2012.07.025
Bajard, M. (2017). Trajectoires d'évolution des sols et des agroécosystèmes de montagne de l'Holocène à l'Anthropocène Reconstitution des pédopaysages des Alpes du Nord occidentales à partir d'archives sédimentaires lacustres.
Bajard, M., Etienne, D., Quinsac, S., Dambrine, E., Sabatier, P., Frossard, V., et al. (2018). Legacy of early anthropogenic effects on recent lake eutrophication (Lake Bénit, northern French Alps). Anthropocene 24, 72–87. doi: 10.1016/j.ancene.2018.11.005
Bajard, M., Poulenard, J., Sabatier, P., Bertrand, Y., Crouzet, C., Ficetola, G. F., et al. (2020). Pastoralism increased vulnerability of a subalpine catchment to flood hazard through changing soil properties. Palaeogeogr. Palaeoclimatol. Palaeoecol. 538:109462. doi: 10.1016/j.palaeo.2019.109462
Bajard, M., Sabatier, P., David, F., Develle, A.-L., Reyss, J.-L., Fanget, B., et al. (2015b). Erosion record in Lake La Thuile sediments (Prealps, France): evidence of montane landscape dynamics throughout the Holocene. Holocene 26:9750. doi: 10.1177/0959683615609750
Bajard, M., Sabatier, P., David, F., Develle, A.-L., Reyss, J. L., Fanget, B., et al. (2015a). “Holocene reconstitution of Lake La Thuile Landscape (874 m a.s.l, Massif des Bauges, France) Evidence of human-controlled of erosion in the mountain belt,” in Sols et matières organiques: mémoires et héritages, Collection Edytem, eds. E. Chalmin, E. Dambrine, B. David, P. Faivre, and J. Poulenard (Edytem), 41–56.
Beug, H.-J. (2004). Beug: Leitfaden der Pollenbestimmung für Mitteleuropa und angrenzende gebiete. Munich: Verlag Friedrich Pfeil.
Bindler, R. (2011). Contaminated lead environments of man: reviewing the lead isotopic evidence in sediments, peat, and soils for the temporal and spatial patterns of atmospheric lead pollution in Sweden. Environ. Geochem. Health 33, 311–329. doi: 10.1007/s10653-011-9381-7
Blaauw, M. (2010). R-Code for “Classical” Age-Modelling (CLAM V1.0) of Radiocarbon Sequences [WWW Document], n.d. Available at: https://doi.pangaea.de/10.1594/PANGAEA.873023 (accessed October 20, 2022).
Boutron, C. F., Görlach, U., Candelone, J.-P., Bolshov, M. A., and Delmas, R. J. (1991). Decrease in anthropogenic lead, cadmium and zinc in Greenland snows since the late 1960s. Nature 353, 153–156. doi: 10.1038/353153a0
Brochier, J.-L. (1986). “Sédiments – documents archéologiques: la grotte d'Antonnaire – grotte bergerie,” in Culture et milieu des premiers paysans de la Moyenne Vallée du Rhône (Lyon: ARENERA; Centre d'Archéologie préhistorique de Valence), 32–33.
Carrier, N., and Mouthon, F. (2010). Chapitre II. — L'homme et les Alpes du Néolithique à l'an Mil, in: Paysans des Alpes: Les communautés montagnardes au Moyen Âge, Histoire. Rennes: Presses universitaires de Rennes. doi: 10.4000/books.pur.128
Cugny, C., Mazier, F., and Galop, D. (2010). Modern and fossil non-pollen palynomorphs from the Basque mountains (western Pyrenees, France): the use of coprophilous fungi to reconstruct pastoral activity. Veg. Hist. Archaeobot. 19, 391–408. doi: 10.1007/s00334-010-0242-6
David, F. (2010a). Expansion of green alder (Alnus alnobetula [Ehrh] K. Koch) in the northern French Alps: a palaeoecological point of view. Compt. Rend. Biol. 333, 424–428. doi: 10.1016/j.crvi.2010.01.002
David, F. (2010b). An example of the consequences of human activities on the evolution of subalpine landscapes. Compt. Rend. Palevol. 9, 229–235. doi: 10.1016/j.crpv.2010.06.002
David, F., Farjanel, G., and Jolly, M.-P. (2002). Enregistrement de l'histoire de la végétation tardiglaciaire et holocène dans un grand lac: le lac d'Annecy (France). GPQ 54, 91–103. doi: 10.7202/004783ar
de Beaulieu, J.-L., Kostenzer, J., and Reich, K. (1993). Dynamique forestière holocène dans la haute vallée de l'Arve (Haute-Savoie) et migrations de Abies et Picea dans les Alpes occidentales. Festschrift Zoller. Dissert. Botan. 196, 387–398.
Delhon, C., Martin, L., and Thiébault, S. (2024). Neolithic shepherds and sheepfold caves in Southern France and adjacent areas: an overview from 40 years of bioarchaeological analyses. Quat. Int. 683–684, 61–75. doi: 10.1016/j.quaint.2023.03.004
Dendievel, A.-M., Dietre, B., Cubizolle, H., Hajdas, I., Kofler, W., Oberlin, C., et al. (2019). Holocene palaeoecological changes and agro-pastoral impact on the “La Narce du Béage” mire (Massif Central, France). Holocene. 29:6. doi: 10.1177/0959683619831416
Deza-Araujo, M., Morales-Molino, C., Conedera, M., Henne, P. D., Krebs, P., Hinz, M., et al. (2022). A new indicator approach to reconstruct agricultural land use in Europe from sedimentary pollen assemblages. Palaeogeogr. Palaeoclimatol. Palaeoecol. 599:111051. doi: 10.1016/j.palaeo.2022.111051
Dietre, B., Gauthier, É., and Gillet, F. (2012). Modern pollen rain and fungal spore assemblages from pasture woodlands around Lake Saint-Point (France). Rev. Palaeobot. Palynol. 186, 69–89. doi: 10.1016/j.revpalbo.2012.07.002
Dietre, B., Reitmaier, T., Walser, C., Warnk, T., Unkel, I., Hajdas, I., et al. (2020). Steady transformation of primeval forest into subalpine pasture during the Late Neolithic to Early Bronze Age (2300–1700 BC) in the Silvretta Alps, Switzerland. Holocene 30, 355–368. doi: 10.1177/0959683619887419
Dietre, B., Walser, C., Kofler, W., Kothieringer, K., Hajdas, I., Lambers, K., et al. (2017). Neolithic to Bronze Age (4850–3450 cal. BP) fire management of the Alpine Lower Engadine landscape (Switzerland) to establish pastures and cereal fields. Holocene 27, 181–196. doi: 10.1177/0959683616658523
Doyen, E. (2013). 15 000 ans d'évolution des écosystèmes sous contrôle climatique et anthropique entre Jura et Bas Dauphiné: végétation, feu et érosion des sols.
Doyen, E., Bégeot, C., Simonneau, A., Millet, L., Chapron, E., Arnaud, F., et al. (2016). Land use development and environmental responses since the Neolithic around Lake Paladru in the French Pre-alps. J. Archaeol. Sci. Rep. 7, 48–59. doi: 10.1016/j.jasrep.2016.03.040
Doyen, E., and Etienne, D. (2017). Ecological and human land-use indicator value of fungal spore morphotypes and assemblages. Veg. Hist. Archaeobot. 26, 357–367. doi: 10.1007/s00334-016-0599-2
Doyen, E., Vannière, B., Berger, J.-F., Arnaud, F., Tachikawa, K., Bard, E., et al. (2013). Land-use changes and environmental dynamics in the upper Rhone valley since Neolithic times inferred from sediments in Lac Moras. Holocene 23, 961–973. doi: 10.1177/0959683612475142
Dziomber, L., Gobet, E., Leunda, M., Gurtner, L., Vogel, H., Tournier, N., et al. (2023). Palaeoecological multiproxy reconstruction captures long-term climatic and anthropogenic impacts on vegetation dynamics in the Rhaetian Alps. Rev. Palaeobot. Palynol. 321:105020. doi: 10.1016/j.revpalbo.2023.105020
Ejarque, A., Miras, Y., and Riera, S. (2011). Pollen and non-pollen palynomorph indicators of vegetation and highland grazing activities obtained from modern surface and dung datasets in the eastern Pyrenees. Rev. Palaeobot. Palynol. 167, 123–139. doi: 10.1016/j.revpalbo.2011.08.001
Elbaz-Poulichet, F., Guédron, S., Anne-Lise, D., Freydier, R., Perrot, V., Rossi, M., et al. (2020). A 10,000-year record of trace metal and metalloid (Cu, Hg, Sb, Pb) deposition in a western Alpine lake (Lake Robert, France): deciphering local and regional mining contamination. Quat. Sci. Rev. 228:106076. doi: 10.1016/j.quascirev.2019.106076
Elleaume, N., Lachello, R., Blanchet, C., Giguet-Covex, C., Etienne, D., Pérès, C., et al. (2022). Interdisciplinary insights into a 500-year trajectory of an alpine socio-ecological system in Montaimont, France. Region. Environ. Change 22:1902. doi: 10.1007/s10113-022-01902-6
Etienne, D., and Jouffroy-Bapicot, I. (2014). Optimal counting limit for fungal spore abundance estimation using Sporormiella as a case study. Veg. Hist. Archaeobot. 23, 743–749. doi: 10.1007/s00334-014-0439-1
Etienne, D., Wilhelm, B., Sabatier, P., Reyss, J.-L., and Arnaud, F. (2013). Influence of sample location and livestock numbers on Sporormiella concentrations and accumulation rates in surface sediments of Lake Allos, French Alps. J. Paleolimnol. 49, 117–127. doi: 10.1007/s10933-012-9646-x
Finsinger, W., and Tinner, W. (2006). Holocene vegetation and land-use changes in response to climatic changes in the forelands of the southwestern Alps, Italy. J. Quat. Sci. 21, 243–258. doi: 10.1002/jqs.971
Finsinger, W., and Tinner, W. (2007). Pollen and plant macrofossils at Lac de Fully (2135 m a.s.l.): holocene forest dynamics on a highland plateau in the Valais, Switzerland. Holocene 17, 1119–1127. doi: 10.1177/0959683607082552
Garcés Pastor, S., Coissac, É., Lavergne, S., Schwörer, C., Theurillat, J.-P., Heintzman, P., et al. (2022). High resolution ancient sedimentary DNA shows that alpine plant diversity is associated with human land use and climate change. Nat. Commun. 13:6559. doi: 10.1038/s41467-022-34010-4
Giguet-Covex, C., Arnaud, F., Enters, D., Poulenard, J., Millet, L., Francus, P., et al. (2012). Frequency and intensity of high-altitude floods over the last 3.5 ka in northwestern French Alps (Lake Anterne). Quat. Res. 77, 12–22. doi: 10.1016/j.yqres.2011.11.003
Giguet-Covex, C., Arnaud, F., Poulenard, J., Disnar, J.-R., Delhon, C., Francus, P., et al. (2011). Changes in erosion patterns during the Holocene in a currently treeless subalpine catchment inferred from lake sediment geochemistry (Lake Anterne, 2063 m a.s.l., NW French Alps): the role of climate and human activities. Holocene 21, 651–665. doi: 10.1177/0959683610391320
Giguet-Covex, C., Bajard, M., Chen, W., Walsh, K. J., Rey, P.-J., Messager, E., et al. (2023). Long-term trajectories of mountain agro-ecosystems in the North-Western Alps. Reg. Environ. Change 23:58. doi: 10.1007/s10113-023-02030-5
Giguet-Covex, C., Ficetola, G. F., Poulenard, J., Bajard, M., Fouinat, L., Gielly, L., et al. (2019). New insights on lake sediment DNA from the catchment: importance of taphonomic and analytical issues on the record quality. Sci. Rep. 9, 1–22. doi: 10.1038/s41598-019-50339-1
Giguet-Covex, C., Pansu, J., Arnaud, F., Rey, P.-J., Griggo, C., Gielly, L., et al. (2014). Long livestock farming history and human landscape shaping revealed by lake sediment DNA. Nat. Commun. 5:3211. doi: 10.1038/ncomms4211
Ginestet, J.-P., Bintz, P., Chaix, L., Evin, J., and Olive, C. (1984). L'abri sous roche de la Vieille Église, La Balme-de-Thuy (Haute-Savoie), premiers résultats. BSPF 81, 320–342. doi: 10.3406/bspf.1984.8595
Gobet, E., Tinner, W., Hochuli, P. A., van Leeuwen, J. F. N., and Ammann, B. (2003). Middle to Late Holocene vegetation history of the Upper Engadine (Swiss Alps): the role of man and fire. Veg. Hist. Archaeobot. 12, 143–163. doi: 10.1007/s00334-003-0017-4
Gouriveau, E. (2020). Résilience des écosystèmes: approche multiproxy de l'impact environnemental des activités humaines passées et récentes dans les Vosges du Nord (mines, verreries, activités militaires et agro-pastorales).
Grimm, E. C. (1987). CONISS: a FORTRAN 77 program for stratigraphically constrained cluster analysis by the method of incremental sum of squares. Comp. Geosci. 13, 13–35. doi: 10.1016/0098-3004(87)90022-7
Grimm, E. C. (1990). TILIA and TILIA GRAPH. PC spreadsheet and graphics software for pollen data. INQUA, working group on data-handling methods. Newsletter 4, 5–7.
Guiter, F., Andrieu-Ponel, V., Beaulieu, D., Ponel, J.-L., Nicoud, P., Blavoux, G., et al. (2005). Impact anthropique sur la végétation de la région d'Évian depuis le Néolithique moyen : l'enregistrement pollinique de la Beunaz (971 m n.g.f., Haute-Savoie, France). Comp. Rend. Biol. 328, 661–673. doi: 10.1016/j.crvi.2005.03.002
Hafner, A., and Schwörer, C. (2018). Vertical mobility around the high-alpine Schnidejoch Pass. Indications of Neolithic and Bronze Age pastoralism in the Swiss Alps from paleoecological and archaeological sources. Quat. Int. 484, 3–18. doi: 10.1016/j.quaint.2016.12.049
Henne, P. D., Elkin, C. M., Reineking, B., Bugmann, H., and Tinner, W. (2011). Did soil development limit spruce (Picea abies) expansion in the Central Alps during the Holocene? Testing a palaeobotanical hypothesis with a dynamic landscape model. J. Biogeogr. 38, 933–949. doi: 10.1111/j.1365-2699.2010.02460.x
Joannin, S., Vannière, B., Galop, D., Peyron, O., Haas, J. N., Gilli, A., et al. (2013). Climate and vegetation changes during the Lateglacial and early–middle Holocene at Lake Ledro (southern Alps, Italy). Clim. Past 9, 913–933. doi: 10.5194/cp-9-913-2013
Laissus, D. (2018). “Territoire et occupation humaine du plateau des Glières du Moyen Age à nos jours. Première approche documentaire et premiers jalons chronologiques,” in Circulations et Occupations Humaines de La Montagne Dans Le Massif Des Bornes: Le Plateau Des Glières (Annecy, Lyon: Rapport d'opération Archéologique).
Laissus, D. (2025). “Chapitre 3 : Derrière les apparences. L'empreinte de la propriété des montagnes (massif des Bornes),” in Écosystèmes Montagnards Du Moyen Âge à Nos Jours, eds. A.-M. Granet, C. Guffond, D. Laissus (Presse Universitaire de Grenoble, collection; La Pierre et l'Ecrit).
López-Vila, J., Montoya, E., Cañellas-Boltà, N., and Rull, V. (2014). Modern non-pollen palynomorphs sedimentation along an elevational gradient in the south-central Pyrenees (southwestern Europe) as a tool for Holocene paleoecological reconstruction. Holocene 24, 327–345. doi: 10.1177/0959683613518593
Magny, M., Bégeot, C., Guiot, J., Marguet, A., and Billaud, Y. (2003). Reconstruction and palaeoclimatic interpretation of mid-Holocene vegetation and lake-level changes at Saint-Jorioz, Lake Annecy, French Pre-Alps. Holocene 13, 265–275. doi: 10.1191/0959683603hl612rp
Martín, P., and Tornero, C. (2024). Sheepfold caves under study: a review of zooarchaeological approaches to old and new-fashioned research questions. Quat. Int. 683–684, 51–60. doi: 10.1016/j.quaint.2023.06.011
Mélo, A. (2025). “Chapitre 2: Des sociétés en leurs montagnes. Mobilité(s) et constructions des territoires montagnards sur le plateau des Glières (massif des Bornes, Haute-Savoie),” in Écosystèmes montagnards du Moyen Âge à nos jours, eds. A.-M. Granet, C. Guffond, and D. Laissus (Presse Universitaire de Grenoble; La Pierre et l'Ecrit).
Messager, E., Giguet-Covex, C., Doyen, E., Etienne, D., Gielly, L., Sabatier, P., et al. (2022). Two millennia of complexity and variability in a perialpine socioecological system (Savoie, France): the contribution of palynology and sedaDNA analysis. Front. Ecol. Evol. 10:866781. doi: 10.3389/fevo.2022.866781
Minchin, P. R. (1987). An evaluation of the relative robustness of techniques for ecological ordination. Vegetation 69, 89–107. doi: 10.1007/BF00038690
Miras, Y., Mariani, M., Ledger, P., Mayoral, A., Chassiot, L., Lavrieux, M., et al. (2018). Holocene vegetation dynamics and first land-cover estimates in the auvergne mountains (Massif Central, France): key tools to landscape management. Interdisc. Archaeol. Nat. Sci. Archaeol. IX, 179–190. doi: 10.24916/iansa.2018.2.5
Moor, H. C., Schaller, T., and Sturm, M. (1996). Recent changes in stable lead isotope ratios in sediments of lake zug, Switzerland. Environ. Sci. Technol. 30, 2928–2933. doi: 10.1021/es950895t
Nicod, P.-Y. (2008). “Les premières sociétés agropastorales dans les Alpes occidentales,” in Premiers bergers des Alpes: de la préhistoire à l'Antiquité, eds. J.-P. Jospin, and T. Favrie (Gollion: Infolio), 44–51.
Nicod, P.-Y., and Picavet, R. (2003). “La stratigraphie de la Grande Rivoire (Isère, France) et la question de la néolithisation alpine,” in ConstellaSion : hommage à Alain Gallay, Cahiers d'archéologie romande (Cahiers d'archéologie romande), 147.
Pansu, J., Giguet-Covex, C., Ficetola, G. F., Gielly, L., Boyer, F., Zinger, L., et al. (2015). Reconstructing long-term human impacts on plant communities: an ecological approach based on lake sediment DNA. Mol. Ecol. 24, 1485–1498. doi: 10.1111/mec.13136
Pini, R. (2002). A high-resolution late-glacial – Holocene pollen diagram from Pian di Gembro (Central Alps, Northern Italy). Veg. Hist. Archaeobot. 11:38. doi: 10.1007/s003340200038
Pini, R., Ravazzi, C., Raiteri, L., Guerreschi, A., Castellano, L., Comolli, R., et al. (2017). From pristine forests to high-altitude pastures: an ecological approach to prehistoric human impact on vegetation and landscapes in the western Italian Alps. J. Ecol. 105, 1580–1597. doi: 10.1111/1365-2745.12767
Posit Team, (2022). RStudio: Integrated Development Environment for R. Available at: http://www.rstudio.com/
Rapuc, W., Giguet-Covex, C., Bouchez, J., Sabatier, P., Gaillardet, J., Jacq, K., et al. (2024). Human-triggered magnification of erosion rates in European Alps since the Bronze Age. Nat. Commun. 15:1246. doi: 10.1038/s41467-024-45123-3
Reille, M. (1992). Pollen et spores d'Europe et d'Afrique du Nord. Laboratoire de Botanique Historique et Palynologie.
Reille, M. (1995). Pollen et spores d'Europe et d'Afrique du Nord: Supplement 1, 1995. Laboratoire de Botanique historique et Palynologie.
Reille, M. (1998). Pollen et spores d'Europe et d'Afrique du Nord: Supplément 2, 1998. Laboratoire de botanique historique et palynologie.
Remicourt, M. (2009). Nouvelles données sur la couche 5B de l'abri-sous-roche de la Vieille Église, La Balme-de-Thuy (Haute-Savoie). Néolithique ancien ou Mésolithique final? BSPF 106, 15–23. doi: 10.3406/bspf.2009.13827
Rey, F., Brugger, S. O., Gobet, E., Andenmatten, R., Bonini, A., Inniger, H., et al. (2022). 14,500 years of vegetation and land use history in the upper continental montane zone at Lac de Champex (Valais, Switzerland). Veg. Hist. Archaeobot. 31, 377–393. doi: 10.1007/s00334-021-00859-6
Rey, F., Schwörer, C., Gobet, E., Colombaroli, D., van Leeuwen, J. F., Schleiss, S., et al. (2013). Climatic and human impacts on mountain vegetation at Lauenensee (Bernese Alps, Switzerland) during the last 14,000 years. Holocene 23, 1415–1427. doi: 10.1177/0959683613489585
Richer, S. (2009). From Pollen to People: The Interaction Between People and Their Environment in the Mid to High-Altitudes of the Southern French Alps. University of York.
Rochel, X. (2017). Une biogéographie historique: Forêts et industries dans le comté de Bitche au xviiie siècle. Histoiremesure XXXII, 9–38. doi: 10.4000/histoiremesure.6076
Rosman, K. J. R., Chisholm, W., Hong, S., Candelone, J.-P., and Boutron, C. F. (1997). Lead from carthaginian and Roman Spanish mines isotopically identified in Greenland Ice dated from 600 B.C. to 300 A.D. Environ. Sci. Technol. 31, 3413–3416. doi: 10.1021/es970038k
Rosman, K. J. R., Ly, C., Van de Velde, K., and Boutron, C. F. (2000). A two century record of lead isotopes in high altitude Alpine snow and ice. Earth Planet. Sci. Lett. 176, 413–424. doi: 10.1016/S0012-821X(00)00013-3
Sabatier, P., Wilhelm, B., Ficetola, G. F., Moiroux, F., Poulenard, J., Develle, A.-L., et al. (2017). 6-kyr record of flood frequency and intensity in the western mediterranean Alps – Interplay of solar and temperature forcing. Quat. Sci. Rev. 170, 121–135. doi: 10.1016/j.quascirev.2017.06.019
Sánchez-Pinillos, M., Kéfi, S., De Cáceres, M., and Dakos, V. (2023). Ecological dynamic regimes: Identification, characterization, and comparison. Ecol. Monogr. 93:e1589. doi: 10.1002/ecm.1589
Schwörer, C., Colombaroli, D., Kaltenrieder, P., Rey, F., and Tinner, W. (2015). Early human impact (5000-3000 BC) affects mountain forest dynamics in the Alps. J. Ecol. 103, 281–295. doi: 10.1111/1365-2745.12354
Shotyk, W., Cheburkin, A. K., Appleby, P. G., Fankhauser, A., and Kramers, J. D. (1996). Two thousand years of atmospheric arsenic, antimony, and lead deposition recorded in an ombrotrophic peat bog profile, Jura Mountains, Switzerland. Earth Planet. Sci. Lett. 145, E1–E7. doi: 10.1016/S0012-821X(96)00197-5
Stahli, M., Finsinger, W., Tinner, W., and Allgower, B. (2006). Wildfire history and fire ecology of the Swiss National Park (Central Alps): new evidence from charcoal, pollen and plant macrofossils. Holocene 16, 805–817. doi: 10.1191/0959683606hol967rp
Thevenon, F., Guédron, S., Chiaradia, M., Loizeau, J.-L., and Poté, J. (2011). (Pre-) historic changes in natural and anthropogenic heavy metals deposition inferred from two contrasting Swiss Alpine lakes. Quat. Sci. Rev. 30, 224–233. doi: 10.1016/j.quascirev.2010.10.013
Thöle, L., Schwörer, C., Colombaroli, D., Gobet, E., Kaltenrieder, P., Van Leeuwen, J., et al. (2016). Reconstruction of Holocene vegetation dynamics at Lac de Bretaye, a high-mountain lake in the Swiss Alps. Holocene 26, 380–396. doi: 10.1177/0959683615609746
Tinner, W., Ammann, B., and Germann, P. (1996). Treeline fluctuations recorded for 12,500 years by soil profiles, pollen, and plant macrofossils in the central swiss alps. Arctic Alpine Res. 28:12003159. doi: 10.1080/00040851.1996.12003159
Tinner, W., Hubschmid, P., Wehrli, M., Ammann, B., and Conedera, M. (1999). Long-term forest fire ecology and dynamics in southern Switzerland. J. Ecol. 87, 273–289. doi: 10.1046/j.1365-2745.1999.00346.x
Tinner, W., and Lotter, A. (2001). Central European vegetation response to abrupt climate change at 8.2 ka. Geology 29, 551–554. doi: 10.1130/0091-7613(2001)029>0551:CEVRTA<2.0.CO;2
Tinner, W., and Lotter, A. F. (2006). Holocene expansions of Fagus silvatica and Abies alba in Central Europe: where are we after eight decades of debate? Quat. Sci. Rev. 25, 526–549. doi: 10.1016/j.quascirev.2005.03.017
Tinner, W., Lotter, A. F., Ammann, B., Conedera, M., Hubschmid, P., van Leeuwen, J. F. N., et al. (2003). Climatic change and contemporaneous land-use phases north and south of the Alps 2300 BC to 800 AD. Quat. Sci. Rev. 22, 1447–1460. doi: 10.1016/S0277-3791(03)00083-0
van der Knaap, W. O., van Leeuwen, J. F. N., Finsinger, W., Gobet, E., Pini, R., Schweizer, A., et al. (2005). Migration and population expansion of Abies, Fagus, Picea, and Quercus since 15000 years in and across the Alps, based on pollen-percentage threshold values. Quat. Sci. Rev. 24, 645–680. doi: 10.1016/j.quascirev.2004.06.013
van der Knaap, W. O., van Leeuwen, J. F. N., Goslar, T., Krisai, R., and Tinner, W. (2012). Human impact on vegetation at the Alpine tree-line ecotone during the last millennium: lessons from high temporal and palynological resolution. Veg. Hist. Archaeobot. 21, 37–60. doi: 10.1007/s00334-011-0315-1
Van Geel, B. (2002). “Non-pollen palynomorphs,” in Tracking Environmental Change Using Lake Sediments, Developments in Paleoenvironmental Research, eds J. P. Smol, H. J. B. Birks, W. M. Last, R. S. Bradley, and K. Alverson (Dordrecht: Springer Netherlands), 99–119. doi: 10.1007/0-306-47668-1_6
van Vugt, L., Garcés-Pastor, S., Gobet, E., Brechbühl, S., Knetge, A., Lammers, Y., et al. (2022). Pollen, macrofossils and sedaDNA reveal climate and land use impacts on Holocene mountain vegetation of the Lepontine Alps, Italy. Quat. Sci. Rev. 296:107749. doi: 10.1016/j.quascirev.2022.107749
Vugt, L., Garcés Pastor, S., Gobet, E., Brechbühl, S., Knetge, A., Lammers, Y., et al. (2022). Pollen, macrofossils and sedaDNA reveal climate and land use impacts on Holocene mountain vegetation of the. Quat. Sci. Rev. 296:107749. doi: 10.1016/j.quascirev.2022.107749
Walsh, K. J., Mocci, F., and Palet-Martinez, J. M. (2007). “Nine thousand years of human/landscape dynamics in a high altitude zone in the southern French Alps (Parc National des Ecrins (Hautes-Alpes (05)),” in Interpretation of Sites and Material Culture from Mid-high Altitude Mountain Environments: Proceedings of the 2004 EAA Session on Alpine Archaeology. Preistoria Alpina. Vol. 42, eds. P. Della Casa and K. Walsh (Museo Tridentino di Scienze Naturali), 922.
Wick, L., and Möhl, A. (2006). The mid-Holocene extinction of silver fir (Abies alba) in the Southern Alps: a consequence of forest fires? Palaeobotanical records and forest simulations. Veg. Hist. Archaeobot. 15, 435–444. doi: 10.1007/s00334-006-0051-0
Wick, L., and Tinner, W. (1997). Vegetation changes and timberline fluctuations in the central Alps as indicators of Holocene climatic oscillations. Arct. Alpine Res. 29, 445–458. doi: 10.2307/1551992
Bajard, M., Sabatier, P., David, F., Develle, A.-L., Reyss, J.-L., Fanget, B., et al. (2016). Erosion record in Lake La Thuile sediments (Prealps, France): evidence of montane landscape dynamics throughout the Holocene. Holocene 26, 350–364. doi: 10.1177/0959683615609750
Rhodes, A. N. (1998). A method for the preparation and quantification of microscopic charcoal from terrestrial and lacustrine sediment cores. Holocene 8, 113–117.
Keywords: vegetation history, vegetation trajectories, human activity, agro-pastoralism, Alps, pollen, coprophilous fungi, macro-charcoal
Citation: Julien A, Messager E, Doyen E, Etienne D, Marquer L and Giguet-Covex C (2025) A 7,000 years trajectory of socio-ecosystems in the montane belt of the northern French Alps. Front. Environ. Archaeol. 3:1474357. doi: 10.3389/fearc.2024.1474357
Received: 01 August 2024; Accepted: 13 December 2024;
Published: 14 January 2025.
Edited by:
Nicholas Branch, University of Reading, United KingdomReviewed by:
Simon Kenneth Stoddart, University of Cambridge, United KingdomCopyright © 2025 Julien, Messager, Doyen, Etienne, Marquer and Giguet-Covex. This is an open-access article distributed under the terms of the Creative Commons Attribution License (CC BY). The use, distribution or reproduction in other forums is permitted, provided the original author(s) and the copyright owner(s) are credited and that the original publication in this journal is cited, in accordance with accepted academic practice. No use, distribution or reproduction is permitted which does not comply with these terms.
*Correspondence: Andréa Julien, YW5kcmVhLmp1bGllbkB1bml2LXNtYi5mcg==
Disclaimer: All claims expressed in this article are solely those of the authors and do not necessarily represent those of their affiliated organizations, or those of the publisher, the editors and the reviewers. Any product that may be evaluated in this article or claim that may be made by its manufacturer is not guaranteed or endorsed by the publisher.
Research integrity at Frontiers
Learn more about the work of our research integrity team to safeguard the quality of each article we publish.