- School of Archaeology, University of Oxford, Oxford, United Kingdom
Stable isotopes are a core method for assessing crop growing conditions in different climatic and soil environments and, thereby, for understanding past agricultural practices. However, isotopic values in plants are altered depending on distinct forms of preservation, including degrees of charring. To investigate this issue in two cereals, common millet (Panicum miliaceum L.) and foxtail millet [Setaria italica (L.) P.Beauv.], we conducted experimental charring to address key questions in millet agriculture and archaeological grain preservation. The results of the experiment under relevant and controlled conditions of charring establish that the relevant temperatures for well-preserved millets occur between 230°C and 250°C and through short-term exposures of up to 6 h of charring. The data confirm that charring increases δ13CVPDB and δ15NAIR values in both common millet and foxtail millet. Husked grains had consistently lower δ13C values than huskless grains, indicating a differential effect of charring on husks and grains, whereas δ15N values are similar regardless of husks. Stable carbon isotope values are relatively similar in uncharred and charred grains, whereas the effects of charring on stable nitrogen isotope values are greater and need to be taken into account when interpreting the values of archaeological grains and palaeodietary analysis in conjunction with human and faunal values. This study significantly advances the understanding of the isotopic effects on archaeological charred grains of common and foxtail millet within their optimal heating preservation window.
Highlights
• Good preservation of common and foxtail millet caryopses occurs at temperatures ranging from 230 to 250 degrees Celsius after 2 to 6 h of heating.
• The most significant differential effects of charring on stable isotopes are observed between husked and huskless grains.
• This work provides a benchmark for comparative studies of millets based on isotopic analysis.
1 Introduction
Isotopic analyses have revolutionized the way in which archaeologists think about agricultural practices in recent decades, enabling new inferences on the growing conditions of plants (Fiorentino et al., 2015) and shaping new models of ancient farming (Styring et al., 2022). From experimental agriculture or traditional crops documented in distinct bioclimatic regions, several studies have sought to frame comparisons between isotopic values of present-day crops and archaeological crop remains. They have also explored the variability between different species, the influence of climate, and the relevance of practices such as irrigation or manuring (Araus et al., 1997; Ferrio et al., 2004; Aguilera et al., 2008; Fraser et al., 2011; Wallace et al., 2013; Bogaard et al., 2016; Are et al., 2018; Bogaard et al., 2018; Lightfoot et al., 2020; Jones et al., 2021).
The stable carbon and nitrogen isotope ratios of plants depend primarily on the photosynthetic pathway, C3, C4, CAM, and plant growth conditions (Farquhar et al., 1982; Araus et al., 1997; Ferrio et al., 2004). However, comparing modern and ancient crops can be challenging due to the potential alterations in their isotopic values resulting from the degradation of organic matter and the degradation of soils. The isotope effect refers to the difference in chemical and physical properties resulting from variations in the atomic mass of an element (Hoefs, 2021). This substitution alters the isotopic values of biological material. An understanding of the extent to which isotopic ratios change due to charring can assist in more accurately estimating the original carbon and nitrogen isotope values, which is crucial for investigating land use and ancient diets (Szpak et al., 2017; Vaiglova et al., 2020, 2022; Stroud et al., 2023).
The most widely analyzed materials for isotopic analysis in archaeological crops are charred grains. This is due to the fact that charring is the most frequent form of preservation of archaeobotanical remains, and also because it preserves biogenic δ13C and δ15N values in complex molecules (Styring et al., 2013). However, charring is a chemical process that produces evident changes in the morphology and structure of the seeds. A myriad of factors determine whether charred remains are preserved in a way that allows taxonomic recognition of their morphology. In light of this, a number of studies have been conducted with the aim of determining the temperature and time variables necessary for optimal seed preservation during charring (Boardman and Jones, 1990; Braadbaart et al., 2004; Braadbaart, 2008; Märkle and Rösch, 2008; Yang et al., 2011b; Charles et al., 2015; Szymanski and Morris, 2015, among others). These studies have focused on examining the surface and internal structures of the grains. Their conclusions indicate that the temperature window for charring is variable in each species (Märkle and Rösch, 2008), or even between husked or huskless grains of cereals of the same species (Boardman and Jones, 1990).
Other papers have explored how temperature and time parameters affect δ13C and δ15N values and which materials are most likely to provide reliable stable isotope measurements, finding that seeds have more positive carbon and nitrogen isotopic ratios after charring than the same fresh material (Aguilera et al., 2008; Fraser et al., 2013a; Nitsch et al., 2015; Styring et al., 2019; Stroud et al., 2023; Varalli et al., 2023). This variation in δ13C and δ15N values has also been determined in millet (Yang et al., 2011a; Fraser et al., 2013a; Dong et al., 2022; Wang et al., 2022; Varalli et al., 2023) –Table 1. In these experimental studies an offset between fresh and charred seeds in common and foxtail millet has been estimated for δ13C values, between +0.2 to +0.5 ‰, being higher and more variable for δ15N values, with values of 0.5-1.3 ‰ (Yang et al., 2011a; Fraser et al., 2013a; Dong et al., 2022; Wang et al., 2022).
Here we aim to investigate the effects of charring on the carbon and nitrogen stable isotope values of the grains of two cereals, common millet (Panicum hereafter) and foxtail millet (Setaria hereafter), to improve our understanding of their past growing conditions and hence ancient agrarian practices. Our goals are: (1) to identify changes in grain morphology and internal structure for establishing an optimal charring window of conditions under which grains are well-preserved on archaeological sites; (2) to determine the effects of this optimal charring on stable carbon and nitrogen isotope values; and (3) to establish whether there is a difference in isotopic values between the husks and the grains (caryopses) in order to inform the selection of archaeological material.
2 Research background
2.1 Brief state-of-art on eastern Asia millets
“Millet” is an umbrella term used to describe different grass species that share a set of structural and functional characteristics. They are small-seeded cereals that produce a large number of grains in their inflorescences, belonging to the C4 photosynthetic pathway, which is typical of warm climatic areas. Millets had different centers of domestication in Asia and Africa, and then progressively expanded to other continents. In particular, Panicum and Setaria were first cultivated in northern China in the early Neolithic (Lu et al., 2009a; Stevens et al., 2021), and during the 2nd millennium BC they spread across Eurasia (Miller et al., 2016; Martin et al., 2021) to Europe (Motuzaite-Matuzeviciute et al., 2013; Filipović et al., 2020). The dispersal of both Chinese millets is associated with social, economic, and human and animal food changes (Lightfoot et al., 2013; Wang et al., 2019; Kirleis et al., 2022; Sun et al., 2024). Millets have recently attracted keen interest in archaeological science with increasing taxonomic identification of preserved macroscopic remains (archaeobotany) but also thanks to recent methodological advances and multi-proxy approaches: phytoliths (Lu et al., 2009b), new analytical biomolecular techniques applied to sediments and artifacts to detect miliacin, a distinctive broomcorn millet compound (Jacob et al., 2008), and stable isotope analysis of human and animal bones (Tafuri et al., 2009).
2.2 Millet grain structure
Grains of Panicum and Setaria have essentially the same structure (Lorenz, 1977). They are formed by a caryopsis encapsulated by two membranous and robust husks: a palea and an upper lemma. The caryopsis is divided into the pericarp, the germ, and the endosperm (Figure 1). The pericarp is the outermost protective layer, made up of one-layered aleurone and endosperm (Serna-Saldivar and Espinosa-Ramírez, 2018; Akanbi et al., 2019). The germ, which includes the embryo and scutellum, is comparatively larger in relation to grain size than in other cereals and also varies between different species of millets (Akanbi et al., 2019). The endosperm contains two different types of cells and starch shapes and sizes (McDonough and Rooney, 1989; Taylor and Belton, 2002; Bean et al., 2018), namely vitreous/corneous/glassy cells and floury/opaque cells. The outer layers of the endosperm are made of vitreous cells which are relatively compact, with large polygonal starch granules pressed together and frequent indentations owing to this dense mesh, and a high concentration of kafirin protein bodies (Watterson et al., 1993; Krishna Kumari and Thayumanavan, 1998; Bean et al., 2018). Proteins become less frequent toward the grain's inner core, which has a less dense and more scattered matrix. Starch-rich cells contain small spherical starch grains and there is a less dense protein mesh in the innermost layers of the grains (Krishna Kumari and Thayumanavan, 1998; Bean et al., 2018). Both Panicum and Setaria contain different proportions of starch granules, the polygonal type being more common in Setaria than in Panicum and vice versa for the spherical type (Krishna Kumari and Thayumanavan, 1998). The major dietary fibers are cellulose, arabinoxylans (AX), b-glucans, other hemicelluloses, pectin, and small amounts of lignin (Serna-Saldivar and Espinosa-Ramírez, 2018). Compounds inside the grains are distributed unequally: husks are particularly rich in cellulose, while the germ contains more lipids than the endosperm.
2.3 Previous charring experiments
Understanding how seeds react to charring has been a frequent concern of the archaeobotanical discipline. Several studies have addressed experimental charring of common millet and foxtail millet grains in controlled conditions to understand how charring modifies their morphology and structure (Märkle and Rösch, 2008; Yang et al., 2011b; Motuzaite-Matuzeviciute et al., 2012; Walsh, 2017; Dong et al., 2022; Liu et al., 2023) and also their carbon and nitrogen isotope ratios (Yang et al., 2011a; Fraser et al., 2013a; Dong et al., 2022). Although in all of these cases different temperatures, times and firing atmospheres have been used to answer the specific questions raised in each paper, the results converge and complement each other. When heated, grain size of both cereals increases from before reaching charring threshold temperatures (Yang et al., 2011b), simultaneously with weight loss (Märkle and Rösch, 2008; Yang et al., 2011b). At temperatures up to 200°C, no structural changes occur in the starch granules (Yang et al., 2011b). The change from a fresh to charred state occurs in both species around 220–235°C in short exposures of 1 h to 4 h (Märkle and Rösch, 2008; Motuzaite-Matuzeviciute et al., 2012), and at lower temperatures after longer exposures: 225°C at an exposure time of 4 h (Märkle and Rösch, 2008) or 24 h at 215°C (Dong et al., 2022). Although distorted, the grains remain identifiable to species up to around 250°C (Walsh, 2017). At higher temperatures grains tend to burst (Märkle and Rösch, 2008; Walsh, 2017; Dong et al., 2022), leading to the disintegration of the grains into ash from 320°C after 4 h of heating (Märkle and Rösch, 2008).
3 Methods
3.1 Sample material
The grain analyzed in the experiments was obtained from traditional varieties cultivated by Celia in the village of Busto (Rois, A Coruña, Spain) and by Luis in the village of Rebollar (Degaña, Oviedo, Spain) in 2012 as part of an ethnobotanical research project (Moreno-Larrazabal et al., 2015; Teira Brión, 2022), and sown annually in the following years. The experimental fields where the traditional varieties were grown were located in Artes, Galicia, Spain (Mercator: 42°34′59′′N 9°00′32′′W), within the biogeographic and climatic Atlantic region. The field is a terraced plot with a long tradition of intense organic manuring (cow, humus, and farmyard manures) and the addition of some chemical fertilizers and rabbit manure from the 1980s until 2013, when it remained uncultivated till the date of the experiment. Millets were sown separately in different fields but sharing very similar growing conditions in terms of soil, drainage, insolation, and humidity. Mechanical means were used to plow the soil, sowing was done by hand and the field was not manured. The plants grew between the 13th of June and September 2020. Common millet and foxtail millet were harvested 85 days and 94 days after sowing, respectively, once the grains were fully ripe. The seeds were naturally sun dried for 5 days and then stored in a traditional granary.
3.2 Charring
The experimental charring methodology was based on previously established protocols for other crop seed types (Fraser et al., 2013a; Charles et al., 2015; Nitsch et al., 2015; Styring et al., 2019; Stroud et al., 2023). A total of 2520 grains were charred in bulk samples of 10 grains each. The different charring conditions were repeated on four sets of samples including a sample of husked and huskless grains of common millet and foxtail millet. The first stage of the experiment comprised grains charred in an oven at four different temperatures, namely at 30-degree intervals between 200 and 290°C. Subsequently, a second round of samples were charred at different temperatures, namely ten-degree intervals between 220°C and 260°C to obtain more detailed data on the processes occurring within this range. As a result, the final experiment comprised seven different charring temperatures: 200, 220, 230, 240, 250, 260, and 290°C. At each temperature three different heating atmospheres were tested: high oxygen availability in uncovered glass beakers; medium oxygen availability with grains covered in 10 cl of sand; and low oxygen availability with grains wrapped in aluminum foil and covered in 25 cl of sand. Each set of grains was charred for 2, 4 or 6 h under each temperature and atmosphere combination.
The grains were charred in a Gallenkamp Plus II oven and temperature fluctuations were monitored using a Datalogger Thermometer with three thermocouples, one located inside the recipients and the other two at the top and at the bottom of the oven respectively (graphs are available as Supplementary material Data Sheet 1). After charring, the grains were cut crosswise with a scalpel to obtain a view of their internal structure and were photographed with a Leica Z6 APO stereo microscope x5.6-x36 magnification with a Leica DFC495 microscopy camera using LAS v4.12 software. Scanning electron microscopes belonging to Research Laboratory for Archaeology and the History of Art (RLAHA) at University of Oxford (JEOL JSM-5510) and the Research Support Infrastructure Network -RIAIDT at Universidade de Santiago de Compostela (ZEISS EVO LS15) were used to image the internal structures of the grains. To record the degree of charring of the outer and inner surface of the grains as a function of time and temperature, a color code system similar to that developed in previous work (Charles et al., 2015; Holguin et al., 2022; Stroud et al., 2023) was created.
3.3 Isotopic analysis
To assess how charring affects isotopic values, only grains for the same ear of each crop were analyzed to avoid possible disparity of results between different plants, and to obtain an average representation of the single ear. A total of 300 grains of Panicum and the same amount of Setaria were selected randomly using a riffle box. Bulk samples of 10 grains, husked—caryopses with paleae and upper lemmas still attached—or huskless, were analyzed. Batches of uncharred fresh grains, and grains charred at optimal conditions for preservation (230 and 250°C after 2 and 4 h respectively and under reducing condition) were analyzed (see Section 4.1). This included batches of husked and huskless grains, to understand the effect of charring on the isotopic composition of grains and grains with husks. The same apparatus for temperature measurement and charring specified in the Section 3.2 was used. All bulk samples were weighed before and after charring to determine their mass loss (see Supplementary material Table 1).
Analysis of the stable carbon and nitrogen isotopic compositions of the samples were conducted at the Research Laboratory for Archaeology and the History of Art at the University of Oxford. Each sample was homogenized within Eppendorf tubes with two steal balls inside using a QIAGEN TissueLyser LT mill for 1 h at an oscillation of 50 pulses per second. The powder obtaining was weighted into tins containers using a Sartorious R200D balance. Carbon and nitrogen isotopic compositions of the common and foxtail millet samples were determined in separate runs on a SerCon Europa EA-GSL sample converter connected to a Sercon 20–22 stable isotope gas-ratio mass spectrometer. Isotopic compositions were calibrated relative to VPDB and AIR scales using the in-house DL glutamic acid monohydrate (δ13C = −10.67 ± 0.07‰, δ15N = −1.73 ± 0.21‰) and Leucine (δ13C = −28.31 ± 0.03‰, δ15N = 6.33 ± 0.07‰) standards for carbon, whereas for nitrogen the glutamic acid and a seal collagen standard (δ13C = −12.54 ± 0.13‰, δ15N = 16.14 ± 0.09‰) were used. To understand precision, accuracy and overall uncertainty check standards were run as well as a duplicate of each tenth sample as minimum. Alanine (δ13C = −27.18 ± 0.16‰, δ15N = −1.50 ± 0.07‰), EMA–P2 (δ13C = −28.19 ± 0.14‰, δ15N = −1.57 ± 0.19‰) Pea protein (δ13C = −26.47 ± 0.08‰, δ15N = 1.97 ± 0.10‰) and seal collagen were used as check standards during the carbon runs, while for the nitrogen run leucine was used instead of seal. Precision, accuracy and overall uncertainly were calculated following Szpak et al. (2017). For the δ13C values precision [u(Rw)] was ± 0.09‰ and accuracy or systematic error [u(bias)] was ± 0.18‰. For δ15N precision was ± 0.14‰ and systematic error [u(bias)] was ± 0.18‰. Overall uncertainty (uc) was ± 0.20‰ for carbon and ± 0.23‰ for nitrogen. All uncorrected raw data from sample and standard measurements are included in Supplementary material Table 2. All statistical tests, calculations, and graphing was conducted in RStudio 2023.12.1, and R version 4.3.3.
4 Results
4.1 Morphological effects of charring
At 200°C grains of Panicum showed no internal or external evidence of starch transformation into solid carbon material resultant from the thermal decomposition of organic matter and release of volatile compounds (Figures 2–4). At 220°C signs of transformation began in the outermost part of the endosperm. Although changes in color took place and a vitrification process started, the cell structure of the core of the Panicum endosperm was very similar to that of the uncharred grains after 2 h. At 220°C the appearance of the grains is vitreous and internal indentations appear. Most relevant changes started at around 230°C from short 2-h exposures. Carbon material and inner voids formed in the outer layers of the endosperm, whereas some areas inside remained dark-brown color, preserving the cells walls (Figure 3). After 4 or 6 h the whole grains were charred. However, germ cells did not show clear evidence of black material. At about 240°C degrees the internal structure and external appearance were very similar to those of well-preserved archaeobotanical grains. Cracks formed regularly in some cases, and surface deformation was evident in a large number of seeds. At 250°C, deep cracks appeared, the caryopsis were heavily distorted, and grains began to form aggregates. The caryopsis compressed by the husk showed visible loss of tissues in irregular shapes. At 260°C (and at higher temperatures, 290°C) the grains burst and were hardly identifiable, resembling popcorn-shapes or amorphous masses. All of these changes occurred in oxidizing, semi-reducing and reducing atmospheres but with some differences –see Figure 2. Differences in grain structure between husked and huskless grains at lower temperatures were observed. At higher temperatures, the compression caused by paleae and upper lemmas resulted in the explosion of the external surface from 240°C onwards and the formation of cracks and more stubby grains. From 260°C pyrogenic material release produced eccentric forms in husked grains.
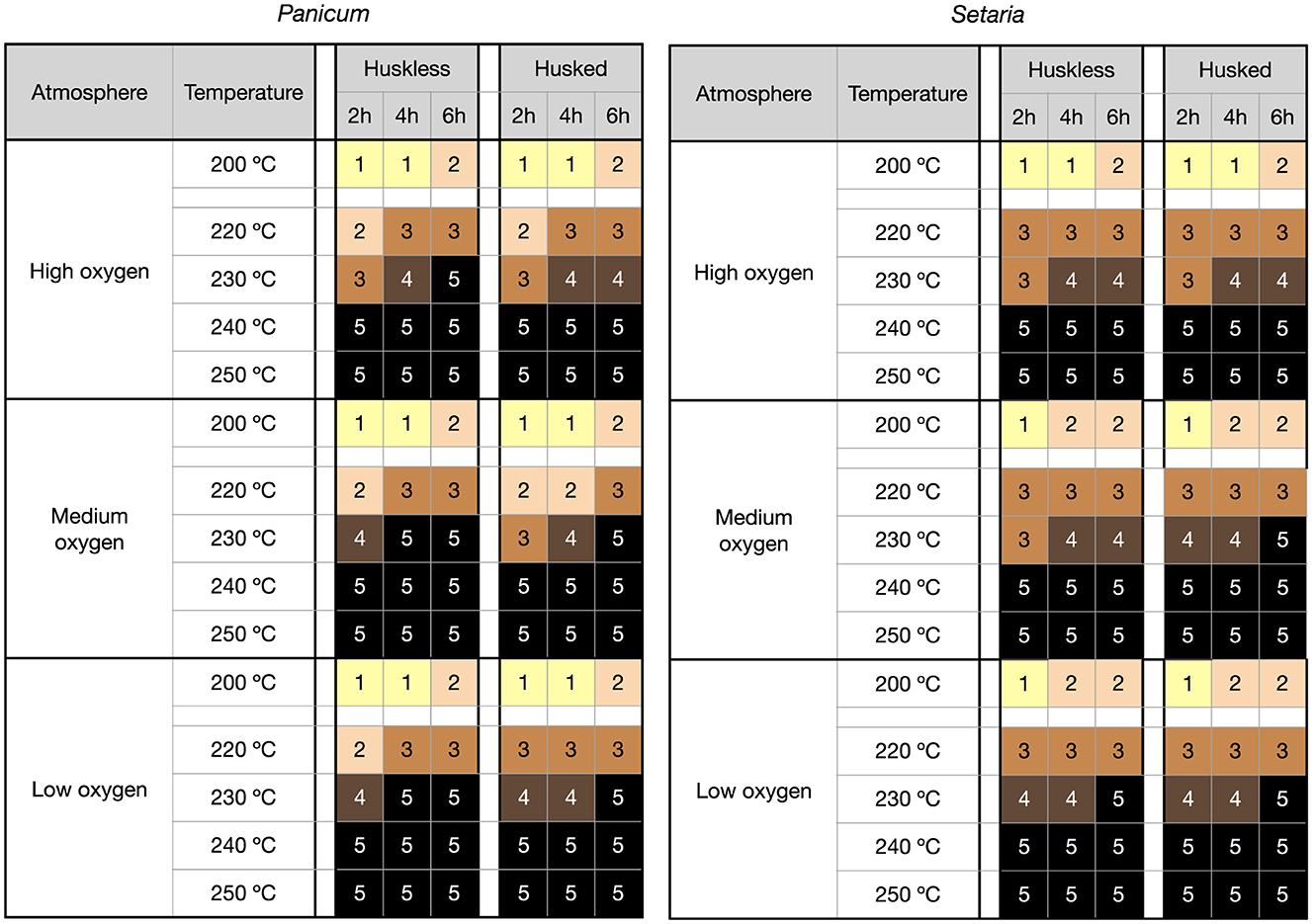
Figure 2. Color code. 1 = yellow, 2 = light brown, 3 = dark brown, 4 = black carbon with brown areas, 5 = black carbon (taken from Charles et al., 2015). Only the temperatures that have registered relevant changes in color are shown. All grains from 240°C to 290°C are in group 5. 260°C and 290°C are not represented as they are redundant with color code 5 and do not provide extra information.
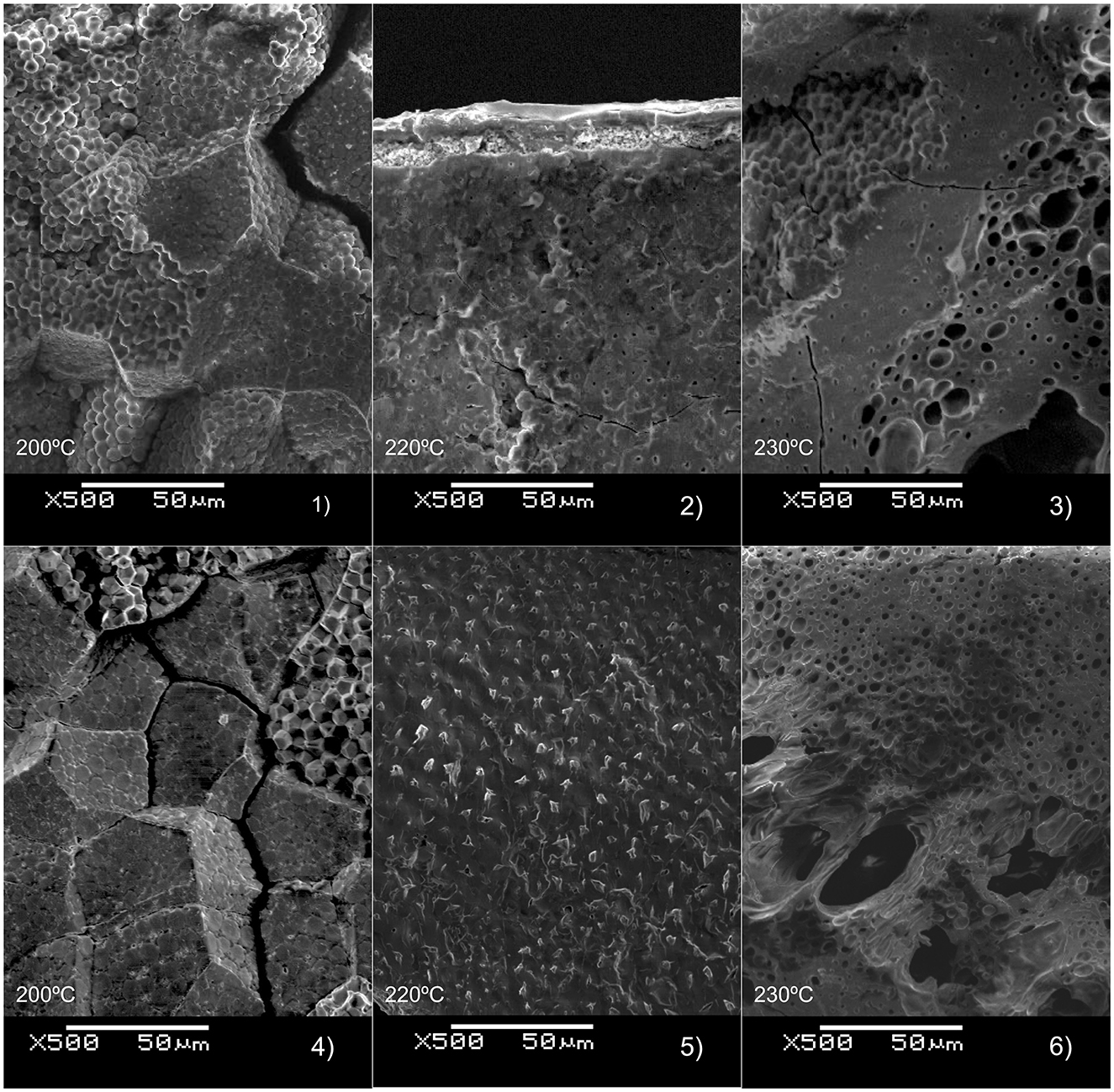
Figure 3. Charring grains of common millet (1–3) and foxtail millet (4–6) in oxidizing atmosphere after 2 hrs of heating at 200, 220, and 230°C. The shape of the floury cells inside the grains are practically intact while at both species the outermost layers species start showed signs of charring at 230°C (3 and 6).
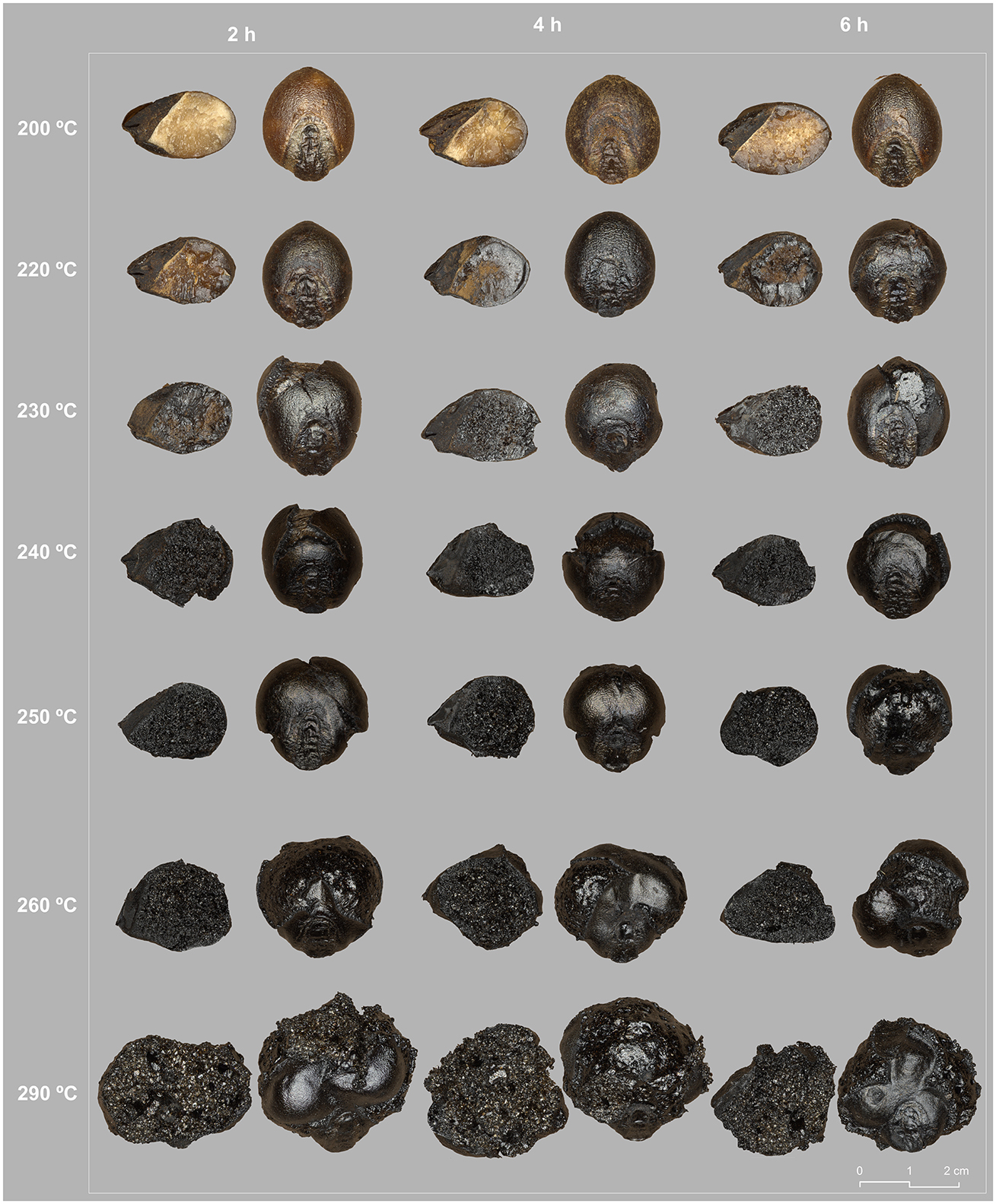
Figure 4. Changes in the external surface and internal structure of the endosperm in common millet as a function of temperature between 200 and 290°C. All seeds have the same scale.
The same pattern was observed in Setaria, but at slightly higher temperatures (Figures 2, 5). Black material was not detected at 200°C or at 220°C in short exposures. The internal structure of the Setaria endosperm cells underwent changes at around 230°C. At this temperature the floury cells still preserved their appearance, even with some indentations caused by stress due to chemical changes—e.g., water or lipid evaporation, whereas vitreous cells became char. After 2 h of heating, only the external layers of the endosperm showed black color, while internal cells remained still uncharred. Between the charred layers of floury cells voids and indentations formed, which led to the beginning of the deformation of the caryopses. The whole endosperm after 4 h or 6 h, depending on the atmosphere, was black in color (Figure 2). The grains were completely made up of pyrogenic material at 240°C. The deformation intensified at 250°C, but grain distortion was more tangible at temperatures above 260°C and higher temperatures, with visible cracks and loss of internal material, but on a smaller scale than observed in Panicum. Low/medium/high oxygen availability atmospheres drove similar grain modification, although grain color darkened quicker under the lower oxygen/higher reducing conditions (Figure 2). As also observed in Panicum, germ cells were more resistant to heating than endosperm cells.
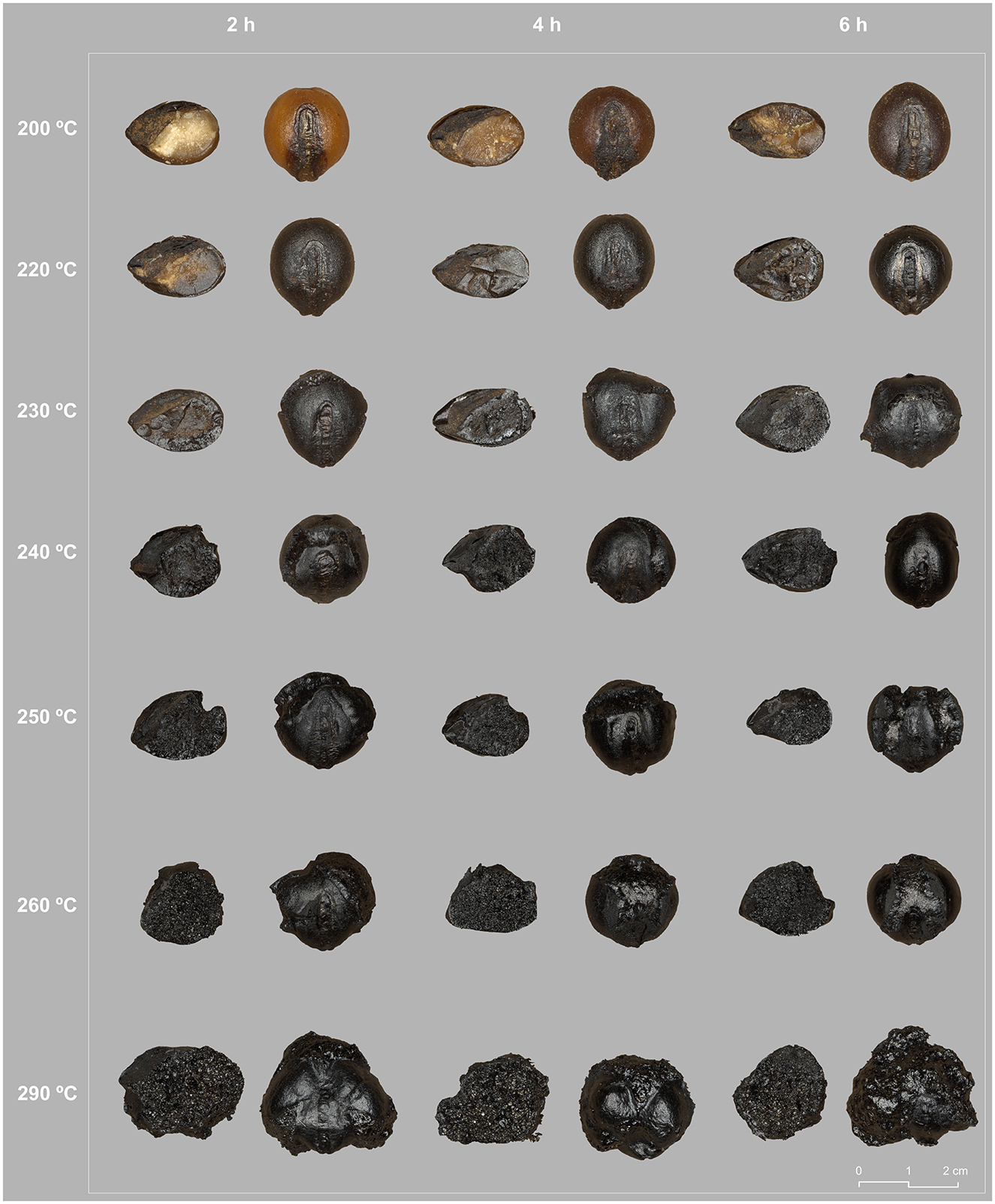
Figure 5. Changes in the external surface and internal structure of the endosperm in foxtail millet as a function of temperature between 200 and 290°C. All seeds have the same scale.
4.2 Stable isotope results
4.2.1 Summary of data
Charring produced a mass loss in grains of both species, being slightly higher in huskless grains than in husked grains: mass losses were around 45% in huskless and 48% in husked grains of Panicum, and around 41% in huskless and 42.5% in husked grains of Setaria after 4 h at 250°C (see Supplementary material Table 1). Additionally, the charring process resulted in an increase in the percentage of carbon (%C) and nitrogen (%N) content compared to that of the original fresh grains (Table 2). Millet grain δ13C and δ15N values from the charring experiment are summarized in Supplementary material Table 3 and Table 3 and represented Supplementary material Data Sheet 2. The δ13C values range from −13.03 to −11.94‰, with Setaria's δ13C values predominantly more positive than Panicum. In both species the δ13C values were more negative in both uncharred and charred husked grains than in huskless grains. The δ15N values range from 9.42 to 15.41‰ with Panicum lower than Setaria.
Comparison of the data by subtracting each sample's value from the mean value of the uncharred samples for each group (Panicum husked, Panicum huskless, Setaria husked, Setaria huskless) shows distinct patterns for temperature and exposure between the species and between the husked and huskless samples (Table 3, Figures 6, 7). The results show that the husked Panicum samples are more negative in δ13C value than the uncharred samples in the low temperature/time batches but increase back toward the uncharred values by the 250°C/4 h batches (Figure 6). This differs from the huskless Panicum samples, as all except one sample are more positive than the mean uncharred value. Husked Setaria samples have more negative δ13C values at 250°C/2 h than the fresh grains. A clear tendency toward increasing δ13C values is only observed until the 250°C /4 h batch. While the huskless Setaria grain samples show an upward trend: the 230°C/4 h batch has higher values than 250°C batches.
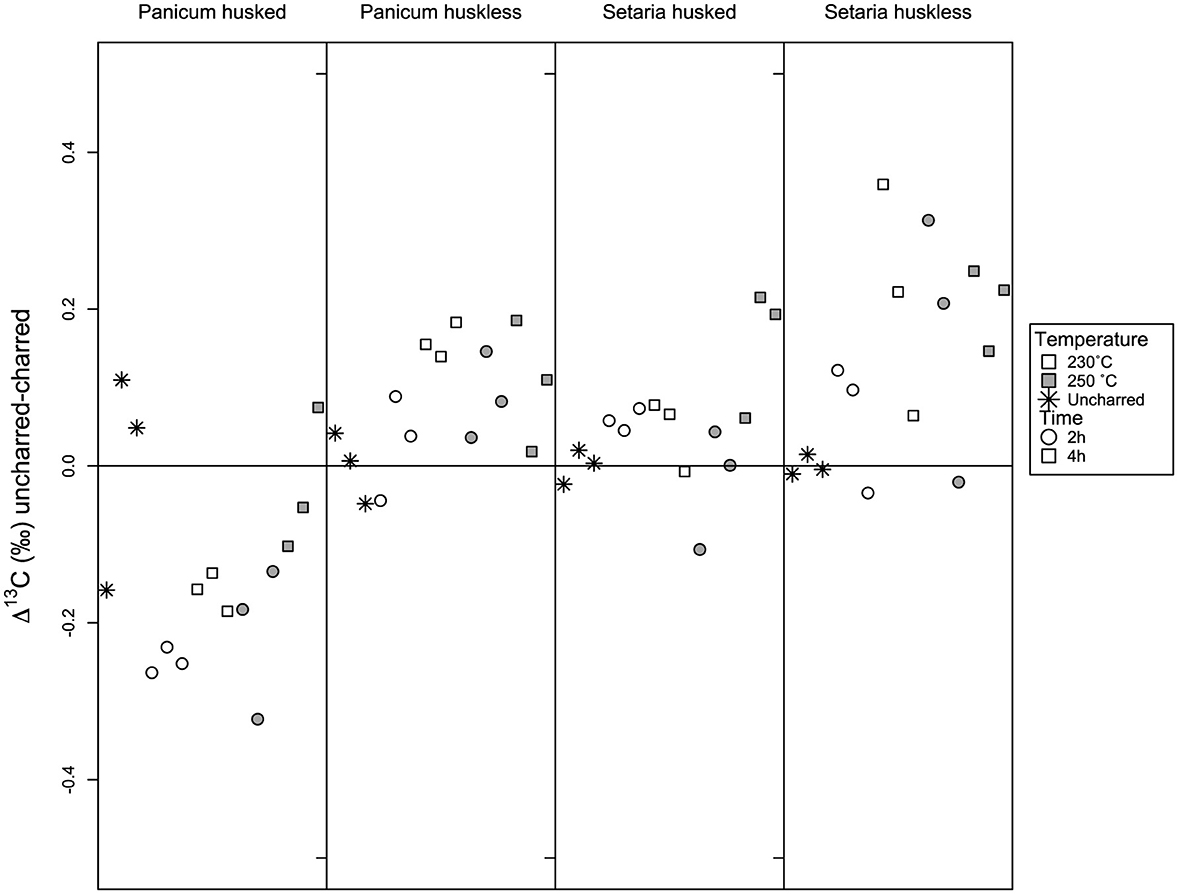
Figure 6. The δ13C value of the huskless and husked charred grains of Panicum and Setaria after heating for 2-4 h at 230-250°C compared to the mean δ13C value of the uncharred replicates.
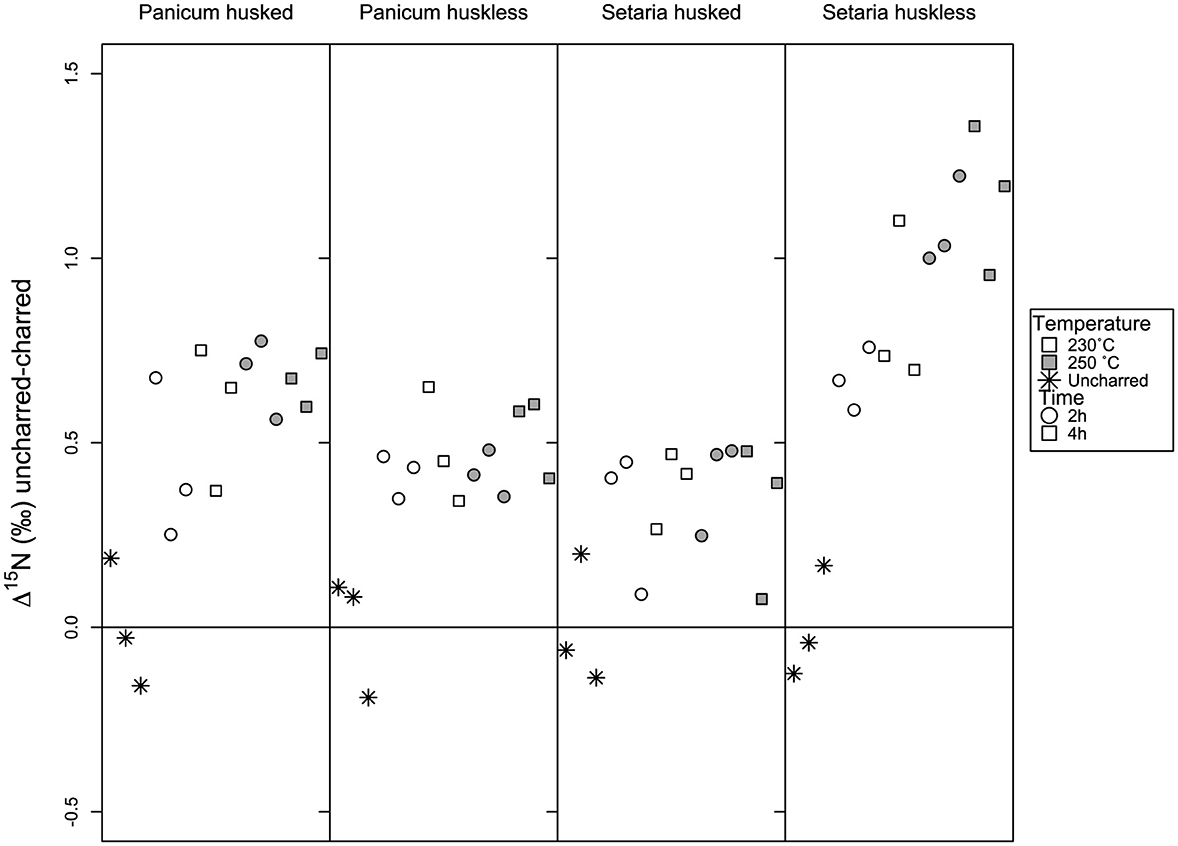
Figure 7. The δ15N value of the huskless and husked charred grains of Panicum and Setaria after heating for 2-4 h at 230-250°C compared to the mean δ15N value of the uncharred replicates.
The nitrogen data, when subtracted from the mean values of the uncharred samples of each group, show more consistency than the carbon data, with all samples higher than the uncharred mean. There is limited difference between the husked Panicum and huskless Panicum data (Figure 7) and limited variation within the charred batches' δ15N values. There is a difference within the husked and huskless Setariaδ15N values, with the husked Setaria behaving in a similar way to the Panicum, while the huskless Setaria shows an upwards trend of increasing δ15N values as time and temperature increase.
4.2.2 Statistics
Several tests were performed to understand the significance of the results from the different parameters of the experiment: fresh or charred grains, husked or huskless grains, temperature, and heating time (Figures 6, 7). Comparison of the husked and huskless samples by species, examining only the charred material, irrespective of time or temperature was conducted using a Welch Two Sample t-test.
Comparison of the husked and huskless Setaria samples (charred only, irrespective of time/temperature) show there is a significant difference between the δ13C values of the two groups [t(19) = −8.2005, p < 0.001], with a difference of −0.35‰. Comparison of the two Panicum groups (husked and huskless, charred only, irrespective of time/temperature) shows a significant difference between the δ13C values of two groups [t(19) = −13.063, p < 0.001] with a difference of −0.48‰.
Comparison of the husked and huskless Setaria (charred only, irrespective of time/temperature) show that there is a significant difference between the δ15N values of charred grain of the two groups [t(18) = −4.648), p = 0.0002], with a difference of−0.39‰. Comparison of the charred husked and huskless Panicum samples shows no significant difference in the δ15N values of two groups [t(18) = 0.97708, p = 0.341] with a difference of 0.06‰.
Comparison between the charred and uncharred data (irrespective of time or temperature) using a linear regression model was conducted for Setaria and Panicum with the combined data of both husked and huskless samples. The carbon stable isotope linear model for Panicum, when using charred vs. uncharred as a predictor of δ13C value, is insignificant (adjusted R2 = −0.032, p = 0.766). The addition of a husked/huskless variable produced a significant model (adjusted R2 = 0.809, p < 0.00001). The charred/uncharred variable within the model was not significant, so a model was re-run with just husked/huskless as the predictor of δ13C values, to test the impact of its exclusion. Such a model explained more of the data (adjusted R2 = 0.812, p < 0.00001) suggesting that charring does not have a significant statistical impact in δ13C value, but whether the grain is husked or huskless has a significant impact. For the Panicum nitrogen stable isotope results, the model shows a significant trend between charred and uncharred (adjusted R2 = 0.683, p < 0.00001). The addition of the husked/huskless variable slightly reduces the model's fitness (adjusted R2 = 0.675, p < 0.00001), suggesting that for nitrogen the husked/huskless variable has limited impact on the δ15N values compared to the charring factor.
The stable carbon isotope linear model for Setaria (charred vs. uncharred) was insignificant with a low R squared value (adjusted R2 = 0.018, p = 0.227). The addition of the independent variable of husked/huskless produces a robust model (adjusted R2 = 0.758, p < 0.0001), with all variables statistically significant. For the nitrogen stable isotope linear regression model for Setaria, when including charred vs. uncharred as a variable, is significant, explaining 48% of variability (adjusted R2 = 0.483, p < 0.00001). The addition of the huskless/husked variable increases the goodness of the model (adjusted R2 = 0.612, p < 0.000001), with all variables significant. In summary, for stable carbon isotope values the most significant variable was the presence or absence of husks in the samples, the linear regression of which best explains the results. For stable nitrogen isotope values, the presence/absence of husk and charring have differing impacts based on species.
The various combinations of temperature (230–250°C) and time (2–4 h) did not yield a robust trend of increasing offset as a function of both factors within the defined window. Consequently, the charred batches that framed the optimum charring window were analyzed together. The offset of the charred grain batches with respect to the fresh grains is expressed in Figures 6, 7. The average offset between fresh grains and charred grains in Panicum for δ13C in husked grains was 0.09‰ ± 0.07 (min = −0.04‰, max = 0.19‰), while the average for husked grains is −0.16‰ ± 0.10 (min = −0.32‰, max = 0.07‰). In Setaria the offset of δ13C was 0.16‰ ± 0.12 (min = −0.03‰, max = 0.36‰) for huskless grains and 0.06‰ ± 0.08 (min = −0.11‰, max = 0.21‰) for husked grains. For nitrogen the average offset in Panicum for δ15N was 0.46‰ ± 0.10 (min = 0.34‰, max = 0.65‰) in huskless grains and 0.59‰ ± 0.17 (min = 0.25‰, max = 0. 78‰) for husked grains; the offset for Setaria was 0.94‰ ± 0.24 (min = 0.58‰, max = 1.36‰) for huskless grains and 0.35‰ ± 0.14 (min = 0.08‰, max = 0.48‰) for husked grains (Table 4).

Table 4. Summary of the average offset of δ13C and δ15N in charred grains compared to the normalized values of uncharred grains.
5 Discussion
5.1 The effect of charring on millet grain morphology
This study shows that the most relevant changes due to charring in Panicum and Setaria grains occur between 230 and 250°C over short time exposures of 2 to 6 h. These charring conditions produced good, low distortion grains which resembled well preserved archaeological grains. Changes in both millets happen in a narrower window of temperatures than observed in other cereals (Charles et al., 2015; Stroud et al., 2023). This could also relate to the tendency of millet to form aggregates (Teira-Brión et al., 2024), possibly due to the layers that make up the caryopsis, the two types of cells and mesh of proteins that constitute their endosperms, their shape and molecular composition, and how they react distinctively to charring. In other cereals such as wheat, barley, rye, rice or oat, the endosperm contains a single type of floury cells, which could result in a similar response to charring and seed deformation. Conversely, millets have distinctive endosperm cells.
The conversion of starch present in the endosperm and cellulose in the pericarp initiates around 220°C (Braadbaart et al., 2004; Braadbaart, 2008). This transformation affects each layer differently. When charring takes place in the floury cells, there is an expansion and bursting of the grains, with cracks and loss of material to the outside. By contrast, the glassy cells layer when subjected to 220°C heating show some signs of vitrification but still preserve tissue structures. When the grains are heated at 230°C for 2 h, voids begin to form between the glassy and floury cells, which still preserve starch granules. However, tissue charring only affects the outermost layers and the cells in the core of the endosperm still contain uncharred tissues; this also occurs in the germ, which is more resistant to heating. The grains heated at 230°C still contain a high proportion of carbohydrates, which is indicative of a starch-rich content (Teira-Brión et al., 2024).
The formation of indentations on the grain surface appeared from 230°C, being a constant feature of grains exposed to 240°C and above. From 250°C the scars are deep and at 260°C, the bursting of the grain is the most characteristic external feature. This is the temperature at which “popcorn” shapes are produced. Some studies of coffee beans suggest that these cracks start to form after 8 min of charring (Charles et al., 2015). Other work indicates that mass loss increases before 40 min at the same temperature (Braadbaart et al., 2004; Braadbaart, 2008). Hence, it is possible that the number of indentations in the experimental millets is correlated to the longer duration of this experimental charring (≥2 h).
As for the temperature window in which we can find identifiable grains, it has been set between 230 and 250°C for the conditions and variables in this experiment. The lower limit obtained for charring differs from that determined by Dong et al. (2022), which suggests that charring would occur from 200°C. However, in this and a related study (Teira-Brión et al., 2024), although changes occurred in the cellular tissues, we were not able to detect charring of the grains at 200°C when heated for 2 to 24 h. The upper limit of 250°C for good grain preservation has also been considered in experiments on Panicum miliaceum and Setaria italica, albeit with larger and less precise temperature gaps of 50°C (Yang et al., 2011b; Walsh, 2017).
The germ was found to be more resistant to charring than the endosperm. Only at temperatures of 260°C was it fully bonded with the endosperm. This could be the origin of a scarce presence of embryos in the millet grains come from the archaeological assemblages, which would have been disarticulated or fragmented (Martín-Seijo et al., 2020). This aspect may also be significant in the isotopic values since the elemental composition in embryos and endosperm differs (see Section 2.2).
Huskless grains are usually interpreted as a sign that they were fully processed and stored clean before consumption (Tereso et al., 2013; Filipović et al., 2019). However, the absence of paleae and lemmas does not necessarily indicate that they were stored without husks. It has been observed that glumes are typically more susceptible to destruction at lower temperatures compared to grains (Boardman and Jones, 1990). In our experimental design, the paleae and upper lemmas demonstrated better resistance to changes in morphology, particularly under oxidizing conditions, but they became extremely fragile and prone to breakage. Differences in the preservation of husks in some archaeological assemblages (e.g., Martín-Seijo et al., 2020) may be explained by the conditions of charring or the recovery methods.
5.2 The isotope effect in Panicum and Setaria grains
Minor deviations in δ13C values of the charred samples compared to the fresh samples are similar to results obtained in other work on isotopes in millets (Yang et al., 2011a; Dong et al., 2022; Wang et al., 2022). In this study, the mean offset has been established as an average value of ±0.16‰ relative to the bulk samples of fresh grains taken from the same inflorescence for Panicum and Setaria.
Since different compounds deplete into specific isotopes at different temperatures, the timing of this reaction in the grains and which compounds are most affected could explain the pattern observed in the charring offset. Grain composition and chemical changes can make a difference in that carbohydrates comprise approximately 74% of the grain, whereas lipids make up 5% (Ravindran, 1991). Starches and sugars have 13C:12C values similar to that of the carbon initially fixed in photosynthesis, whereas lipids are up to 15‰ higher and cellulose tend to be approximately 2‰ lower (Ehleringer, 1981). The 13C depletion observed during charring may be partially attributed to the loss of cellulose, a carbohydrate that is more prevalent in seeds than other compounds with lighter isotopic values, such as lipids. Lipids are known to volatilise at temperatures >150°C, implying that their residues will be higher when charring initiates (Czimczik et al., 2002). The negative δ13C offset of the Panicum husked grains charred at 230°C from the uncharred material has also been observed by other studies among C3 cereals (bread wheat, einkorn, rye) and millets (common millet) (e.g. Nitsch et al., 2015; Dong et al., 2022; Stroud et al., 2023). It is plausible that these values may be linked with a greater prevalence of uncharred tissues, when the temperature is still insufficient to transform all organic compounds.
The δ15N values of the charred samples tend to a higher offset from the uncharred samples than do the δ13C values, with δ15N values tending to be more positive in charred material. This correlation has been widely reported in different studies on other crops, but not in all species and experiments (Fraser et al., 2013a; Nitsch et al., 2015; Styring et al., 2019; Dong et al., 2022; Wang et al., 2022; among others). Variation in δ15N values can have an impact when considering fractionation processes in body tissues, which has an effect between diet and bone collagen in the order of approximately +3‰ (Reitsema, 2013). Therefore, when comparing bones with charred plant remains, the isotope effect must be considered to determine the trophic effect more accurately (Fraser et al., 2013b). Nitrogen is also key for understanding soil nitrogen composition and potential manure supply to the soil (Bogaard et al., 2013). The δ15N offset must be taken into account in the interpretation of manuring levels in the archaeological samples.
A relationship between nitrogen isotopic effect in millets and their different biochemical subtypes within the C4 photosynthetic pathway has been proposed (i.e., Varalli et al., 2023). These authors posit that charred millets of the NAD-ME subtype, such as Panicum miliaceum and Cenchrus americanus, exhibit a higher isotopic offset in δ15N values compared to those from NADP-ME subtype, such as Setaria italica and Sorghum bicolor (Varalli et al., 2023). Conversely, the results obtained within the parameters of the present experimental design differ from those of the previous study. Specifically, the offset for charred Panicum huskless has been shown to increase less than that for the equivalent Setaria batch. Consequently, this contrast suggests the need for further investigation to determine the impact that the biochemical NAD-ME and NADP-ME subtypes have on the isotopic effect. Additional factors must be considered, such as variations in the compound composition of each millet species—even within the same species—which can lead to different isotopic values in the grains. Furthermore, differences in temperature and heating exposure between experiments may reflect different stages of compound depletion, thereby affecting isotopic values.
Although no specific values have been measured for paleae and lemmas, experimental results indicate that grains with intact bracts have lower isotopic values than grains without. Previous works have reported distinct δ13C and δ15N values among leaves compared to grains in Setaria (Lightfoot et al., 2016, 2020). As paleae and lemmas develop before grain formation (Motuzaite-Matuzeviciute et al., 2012), their values are also associated with different growth stages, along with the different components that make up each constituent. Just as each part of a plant has distinct isotopic values, the isotopic signatures of humans and livestock would differ depending on which parts of the plant they consume.
6 Conclusions
This study indicates that ideal conditions for well-preserved charred grains of common millet (Panicum miliaceum) and foxtail millet (Setaria italica) occur from 230°C to 250°C of constant heating over short periods of time (2, 4, and 6 h). Nevertheless, at 230°C the endosperm was not completely charred, with the greatest effect observed in the outermost cell layers. At temperatures above 250°C, the deformation of the seeds prevents their identification to species level. In this window of optimal conditions for the preservation of grain shape, the most influential factors in understanding the effect of charring on stable carbon values in each millet species have been, in descending order of importance, the presence or absence of husks in the grains, and temperature/time, while both factors differ in important for nitrogen depending on species.
The isotopic values of the charred grains exhibited a significantly lower offset from their initial fresh state in carbon than in nitrogen. The average offset in δ13C was up to ±0.16 ‰, whereas the variation in δ15N was higher, with mean values between +0.35‰ and +0.94‰. Consequently, the values of both isotope ratios in archaeological samples may differ from those when the crops were cultivated. In particular, the offset in δ15N should be taken into account when making inferences about soil nitrogen composition and possible manuring.
The experiment reported more negative δ13C and δ15N values in husked grains than in huskless grains in the fresh samples. Although in this study we have not measured how husks contribute to the values obtained, we have verified their influence on the results. The presence or absence of husks can lead to differences in the stable isotopes. Therefore, it is crucial to consider husks as a factor when selecting millet samples for isotopic analysis.
In conclusion, stable isotopes are a sensitive proxy for addressing plant growing conditions in the past. To compare the results obtained in the research on present-day and archaeological remains, it is necessary to comprehend and correct the isotope effect in each species. Experimental charring designs can establish comparative baselines for stable isotope studies, allowing comparisons between modern farming with original archaeobotanical assemblages to infer original carbon and nitrogen composition in ancient crops. To achieve this, data libraries comprising offsets for different species, crop conditions, combustion atmospheres and preservation in different environments are needed. Experimental approaches provide a basis for more accurate understanding of agricultural practice and palaeodiet over time and enable the exploration of potential differences between bioclimatic and geographical regions.
Data availability statement
The datasets presented in this study can be found in online repositories. The names of the repository/repositories and accession number(s) can be found in the article/Supplementary material.
Author contributions
AT-B: Conceptualization, Data curation, Formal analysis, Funding acquisition, Investigation, Methodology, Project administration, Visualization, Writing – original draft, Writing – review & editing. ES: Formal analysis, Resources, Software, Validation, Visualization, Writing – review & editing. MC: Conceptualization, Methodology, Resources, Supervision, Writing – review & editing. AB: Conceptualization, Methodology, Project administration, Supervision, Writing – review & editing.
Funding
The author(s) declare financial support was received for the research, authorship, and/or publication of this article. Andrés Teira-Brión was supported by a Marie Skłodowska-Curie Individual Fellowship H2020-MSCA-IF-2020 (REA grant agreement ID: 101018935).
Acknowledgments
The authors would like to thank Amy Styring for her insightful comments, Peter Ditchfield for sample analysis and Amy Holguin for her assistance in SEM photographs.
Conflict of interest
The authors declare that the research was conducted in the absence of any commercial or financial relationships that could be construed as a potential conflict of interest.
Publisher's note
All claims expressed in this article are solely those of the authors and do not necessarily represent those of their affiliated organizations, or those of the publisher, the editors and the reviewers. Any product that may be evaluated in this article, or claim that may be made by its manufacturer, is not guaranteed or endorsed by the publisher.
Supplementary material
The Supplementary Material for this article can be found online at: https://www.frontiersin.org/articles/10.3389/fearc.2024.1473593/full#supplementary-material
Supplementary material Table 1 | Weight loss.
Supplementary material Table 2 | Uncorrected measurements of standards and samples.
Supplementary material Table 3 | Summary of corrected isotopic values.
Supplementary material Data Sheet 1 | Temperature graphs.
Supplementary material Data Sheet 2 | The δ13C and δ15N results of uncharred and charred batches of the same Panicum and Setaria ears.
References
Aguilera, M., Araus, J. L., Voltas, J., Rodríguez-Ariza, M.O., Molina, F., Rovira, N., et al. (2008). Stable carbon and nitrogen isotopes and quality traits of fossil cereal grains provide clues on sustainability at the beginnings of Mediterranean agriculture. Rapid Commun. Mass Spectromet. 22, 1653–1663. doi: 10.1002/rcm.3501
Akanbi, T. O., Timilsena, Y., and Dhital, S. (2019). “Bioactives from millet: properties and effects of processing on bioavailability,” in Bioactive Factors and Processing Technology for Cereal Foods, eds. J. Wang, B. Sun and R. Tsao (Singapore: Springer Singapore), 171–183.
Araus, J. L., Febrero, A., Buxó, R., RodriGuez-Ariza, M. O., Molina, F., Camalich, M., et al. (1997). Identification of ancient irrigation practices based on the carbon isotope discrimination of plant seeds: a case study from the South-East Iberian Peninsula. J. Archaeol. Sci.24, 729–740. doi: 10.1006/jasc.1997.0154
Are, A. K., Srivastava, R. K., Mahalingam, G., Gorthy, S., Gaddameedi, A., Kunapareddy, A., et al. (2018). “Chapter 3 - application of plant breeding and genomics for improved sorghum and pearl millet grain nutritional quality,” in Sorghum and Millets (Second Edition), eds. J.R.N. Taylor and K.G. Duodu (St. Paul, MN: AACC International Press), 51–68.
Bean, S. R., Zhu, L., Smith, B. M., Wilson, J. D., Ioerger, B. P., and Tilley, M. (2018). “Chapter 6 - Starch and Protein Chemistry and Functional Properties*,” in Sorghum and Millets (Second Edition), eds. J. R. N. Taylor and K. G. (St. Paul, MN: Duodu AACC International Press) 131–170. doi: 10.1016/B978-0-12-811527-5.00006-X
Boardman, S., and Jones, G. (1990). Experiments on the effects of charring on cereal plant components. J. Archaeol.Sci. 17, 1–11. doi: 10.1016/0305-4403(90)90012-T
Bogaard, A., Fraser, R., Heaton, T. H. E., Wallace, M., Vaiglova, P., Charles, M., et al. (2013). Crop manuring and intensive land management by Europe's first farmers. Proc. Nat. Acad. Sci. 110, 12589–12594. doi: 10.1073/pnas.1305918110
Bogaard, A., Hodgson, J., Nitsch, E., Jones, G., Styring, A., Diffey, C., et al. (2016). Combining functional weed ecology and crop stable isotope ratios to identify cultivation intensity: a comparison of cereal production regimes in Haute Provence, France and Asturias, Spain. Vegetat. Hist. Archaeob. 25, 57–73. doi: 10.1007/s00334-015-0524-0
Bogaard, A., Styring, A., Ater, M., Hmimsa, Y., Green, L., Stroud, E., et al. (2018). From traditional farming in morocco to early urban agroecology in northern mesopotamia: combining present-day arable weed surveys and crop isotope analysis to reconstruct past agrosystems in (Semi-)arid regions. Environm. Archaeol. 23, 303–322. doi: 10.1080/14614103.2016.1261217
Braadbaart, F. (2008). Carbonisation and morphological changes in modern dehusked and husked Triticum dicoccum and Triticum aestivum grains. Vegetat. Hist. Archaeob. 17, 155–166. doi: 10.1007/s00334-007-0134-6
Braadbaart, F., Van Der Horst, J., Boon, J. J., and Van Bergen, P. F. (2004). Laboratory simulations of the transformation of emmer wheat as a result of heating. J. Therm. Analy. Calorim. 77, 957–973. doi: 10.1023/B:JTAN.0000041672.45140.e9
Charles, M., Forster, E., Wallace, M., and Jones, G. (2015). “Nor ever lightning char thy grain”1: establishing archaeologically relevant charring conditions and their effect on glume wheat grain morphology. STAR. 1, 1–6. doi: 10.1179/2054892315Y.0000000008
Czimczik, C. I., Preston, C. M., Schmidt, M. W. I., Werner, R. A., and Schulze, E.-D. (2002). Effects of charring on mass, organic carbon, and stable carbon isotope composition of wood. Org. Geochem. 33, 1207–1223. doi: 10.1016/S0146-6380(02)00137-7
Dong, Y., Bi, X., Wu, R., Belfield, E. J., Harberd, N. P., Christensen, B. T., et al. (2022). The potential of stable carbon and nitrogen isotope analysis of foxtail and broomcorn millets for investigating ancient farming systems. Front. Plant Sci. 13. doi: 10.3389/fpls.2022.1018312
Ehleringer, J. R. (1981). “13C/12C fractionation and its utility in terrestrial plant studies,” in Carbon Isotope Techniques, eds. D. C. Coleman and B. Fry. (San Diego: Academic Press), 187–200.
Farquhar, G. D., O'leary, M., and Berry, J. (1982). On the relationship between carbon isotope discrimination and the intercellular carbon dioxide concentration in leaves. Funct. Plant Biol. 9, 121–137. doi: 10.1071/PP9820121
Ferrio, J. P., Alonso, N., Voltas, J., and Araus, J. L. (2004). Estimating grain weight in archaeological cereal crops: a quantitative approach for comparison with current conditions. J. Archaeol. Sci. 31, 1635-1642. doi: 10.1016/j.jas.2004.04.006
Filipović, D., Meadows, J., Corso, M. D., Kirleis, W., Alsleben, A., Akeret, Ö., et al. (2020). New AMS 14C dates track the arrival and spread of broomcorn millet cultivation and agricultural change in prehistoric Europe. Scient. Rep. 10:13698. 10.1038/s41598-020-70495-z doi: 10.1038/s41598-020-70495-z
Filipović, D., Mewis, F., Saalow, L., Schmidt, J.-P., and Kirleis, W. (2019). “Waste disposal in the Bronze Age: plants in pits at Wismar-Wendorf, northern Germany,” in How's Life? Living Conditions in the 2nd and 1st Millennia BCE, eds. M. Dal Corso, W. Kirleis, J. Kneisel, N. Taylor, M. Wieckowska-Lüth and M. Zanon (Leiden: Sidestone Press), 103–130.
Fiorentino, G., Ferrio, J. P., Bogaard, A., Araus, J. L., and Riehl, S. (2015). Stable isotopes in archaeobotanical research. Veget. Hist. Archaeob. 24, 215–227. doi: 10.1007/s00334-014-0492-9
Fraser, R. A., Bogaard, A., Charles, M., Styring, A. K., Wallace, M., Jones, G., et al. (2013a). Assessing natural variation and the effects of charring, burial and pre-treatment on the stable carbon and nitrogen isotope values of archaeobotanical cereals and pulses. J. Archaeol. Sci. 40, 4754–4766. doi: 10.1016/j.jas.2013.01.032
Fraser, R. A., Bogaard, A., Heaton, T., Charles, M., Jones, G., Christensen, B. T., et al. (2011). Manuring and stable nitrogen isotope ratios in cereals and pulses: towards a new archaeobotanical approach to the inference of land use and dietary practices. J. Archaeol. Sci. 38, 2790–2804. doi: 10.1016/j.jas.2011.06.024
Fraser, R. A., Bogaard, A., Schäfer, M., Arbogast, R., and Heaton, T. H. E. (2013b). Integrating botanical, faunal and human stable carbon and nitrogen isotope values to reconstruct land use and palaeodiet at LBK Vaihingen an der Enz, Baden-Württemberg. World Archaeol. 45, 492–517. doi: 10.1080/00438243.2013.820649
Hoefs, J. (2021). Stable Isotope Geochemistry. Cham: Springer Nature. doi: 10.1007/978-3-030-77692-3
Holguin, A., Charles, M., Mithen, S., and Schulting, R. (2022). In a nutshell: Using structural and chemical changes to establish the charring conditions of archaeological hazelnut shells. J. Archaeol. Sci. 144, 105623. doi: 10.1016/j.jas.2022.105623
Jacob, J., Disnar, J.-R., and Bardoux, G. (2008). Carbon isotope evidence for sedimentary miliacin as a tracer of Panicum miliaceum (broomcorn millet) in the sediments of Lake le Bourget (French Alps). Adv. Organic Geochem. 39, 1077–1080. doi: 10.1016/j.orggeochem.2008.04.003
Jones, P. J., O'connell, T. C., Jones, M. K., Singh, R. N., and Petrie, C. A. (2021). Crop water status from plant stable carbon isotope values: a test case for monsoonal climates. Holocene 31, 993-1004. doi: 10.1177/0959683621994649
Kirleis, W., Dal Corso, M., and Filipovic, D. (2022). Millet and What Else? The Wider Context of the Adoption of Millet Cultivation in Europe. Leiden: Sidestone Press.
Krishna Kumari, S., and Thayumanavan, B. (1998). Characterization of starches of proso, foxtail, barnyard, kodo, and little millets. Plant Foods Human Nutr. 53, 47–56. doi: 10.1023/A:1008083020810
Lightfoot, E., Liu, X., and Jones, M. K. (2013). Why move starchy cereals? A review of the isotopic evidence for prehistoric millet consumption across Eurasia. World Archaeol. 45, 574–623. doi: 10.1080/00438243.2013.852070
Lightfoot, E., Przelomska, N., Craven, M., O′Connell, T. C., He, L., Hunt, H. V., et al. (2016). Intraspecific carbon and nitrogen isotopic variability in foxtail millet (Setaria italica). Rapid Commun. Mass Spectrom. 30, 1475–1487. 10.1002/rcm.7583 doi: 10.1002/rcm.7583
Lightfoot, E., Ustunkaya, M. C., Przelomska, N., O'connell, T. C., Hunt, H. V., Jones, M. K., et al. (2020). Carbon and nitrogen isotopic variability in foxtail millet (Setaria italica) with watering regime. Rapid Commun. Mass Spectromet. 34, e8615–e8615. doi: 10.1002/rcm.8615
Liu, Y., Xi, Y., Zhang, F., Wang, Z., Wang, C., Yu, S., et al. (2023). Charring-induced morphological changes of Chinese “Five Grains”: an experimental study. Front. Plant Sci. 14:1063617. doi: 10.3389/fpls.2023.1063617
Lorenz, K. (1977). Proso and foxtail millets- scanning electron microscopy and starch characteristics. LWT Food Sci. Technol. 10, 324–327.
Lu, H., Zhang, J., Liu, K.-B., Wu, N., Li, Y., Zhou, K., et al. (2009a). Earliest domestication of common millet (Panicum miliaceum) in East Asia extended to 10,000 years ago. Proc. Nat. Acad. Sci. 116:372. 10.1073/pnas.0900158106 doi: 10.1073/pnas.0900158106
Lu, H., Zhang, J., Wu, N., Liu, K.-B., Xu, D., and Li, Q. (2009b). Phytoliths analysis for the discrimination of foxtail millet (Setaria italica) and common millet (Panicum miliaceum). PLOS ONE 4, e4448. doi: 10.1371/journal.pone.0004448
Märkle, T., and Rösch, M. (2008). Experiments on the effects of carbonization on some cultivated plant seeds. Veg. Hist. Archaeobot. 17, 257–263. doi: 10.1007/s00334-008-0165-7
Martin, L., Messager, E., Bedianashvili, G., Rusishvili, N., Lebedeva, E., Longford, C., et al. (2021). The place of millet in food globalization during Late Prehistory as evidenced by new bioarchaeological data from the Caucasus. Sci. Rep. 11:13124. 10.1038/s41598-021-92392-9 doi: 10.1038/s41598-021-92392-9
Martín-Seijo, M., Teira-Brión, A., Currás, A., and Rodríguez-Rellán, C. (2020). After the fire: the end of a house life-cycle at the Iron Age site of Nabás (North-western Iberia). Veg. Hist. Archaeobot. 29, 427–446. doi: 10.1007/s00334-019-00750-5
McDonough, C. M., and Rooney, L. W. (1989). Structural characteristics of Pennisetum americanum (pearl millet) using scanning electron and fluorescence microscopy. Food Struct. 8:16.
Miller, N. F., Spengler, R. N., and Frachetti, M. (2016). Millet cultivation across Eurasia: Origins, spread, and the influence of seasonal climate. Holocene 26, 1566–1575. doi: 10.1177/0959683616641742
Moreno-Larrazabal, A., Teira-Brión, A., Sopelana-Salcedo, I., Arranz-Otaegui, A., and Zapata, L. (2015). Ethnobotany of millet cultivation in the north of the Iberian Peninsula. Veg. Hist. Archaeobot. 24, 541–554. doi: 10.1007/s00334-015-0518-y
Motuzaite-Matuzeviciute, G., Hunt, H. V., and Jones, M. K. (2012). Experimental approaches to understanding variation in grain size in Panicum miliaceum (broomcorn millet) and its relevance for interpreting archaeobotanical assemblages. Veget. Hist. Archaeob. 21, 69–77. doi: 10.1007/s00334-011-0322-2
Motuzaite-Matuzeviciute, G., Staff, R. A., Hunt, H. V., Liu, X., and Jones, M. K. (2013). The early chronology of broomcorn millet (Panicum miliaceum) in Europe. Antiquity 87, 1073–1085. doi: 10.1017/S0003598X00049875
Nitsch, E. K., Charles, M., and Bogaard, A. (2015). Calculating a statistically robust δ13C and δ15N offset for charred cereal and pulse seeds. STAR. 1, 1–8. doi: 10.1179/2054892315Y.0000000001
Ravindran, G. (1991). Studies on millets: proximate composition, mineral composition, and phytate and oxalate contents. Food Chem. 39, 99–107. doi: 10.1016/0308-8146(91)90088-6
Reitsema, L. J. (2013). Beyond diet reconstruction: stable isotope applications to human physiology, health, and nutrition. Am. J. Human Biol. 25, 445–456. doi: 10.1002/ajhb.22398
Serna-Saldivar, S. O., and Espinosa-Ramírez, J. (2018). “Chapter 5 - Grain Structure and Grain Chemical Composition,” in Sorghum and Millets (Second Edition), eds. J. R. N. Taylor and K. G. Duodu (St. Paul, MN: AACC International Press) 85–129. doi: 10.1016/B978-0-12-811527-5.00005-8
Stevens, C. J., Shelach-Lavi, G., Zhang, H., Teng, M., and Fuller, D. Q. (2021). A model for the domestication of Panicum miliaceum (common, proso or broomcorn millet) in China. Veget. Hist. Archaeob. 30, 21–33. doi: 10.1007/s00334-020-00804-z
Stroud, E., Charles, M., Bogaard, A., and Hamerow, H. (2023). Turning up the heat: Assessing the impact of charring regime on the morphology and stable isotopic values of cereal grains. J. Archaeol. Sci. 153:105754. doi: 10.1016/j.jas.2023.105754
Styring, A. K., Carmona, C. U., Isaakidou, V., Karathanou, A., Nicholls, G. K., Sarpaki, A., et al. (2022). Urban form and scale shaped the agroecology of early ‘cities' in northern Mesopotamia, the Aegean and Central Europe. J. Agrarian Change 22, 831–854. doi: 10.1111/joac.12497
Styring, A. K., Diop, A. M., Bogaard, A., Champion, L., Fuller, D. Q., Gestrich, N., et al. (2019). Nitrogen isotope values of Pennisetum glaucum (pearl millet) grains: towards a reconstruction of past cultivation conditions in the Sahel, West Africa. Veget. Hist. Archaeob. 28, 663–678. doi: 10.1007/s00334-019-00722-9
Styring, A. K., Manning, H., Fraser, R. A., Wallace, M., Jones, G., Charles, M., et al. (2013). The effect of charring and burial on the biochemical composition of cereal grains: investigating the integrity of archaeological plant material. J. Archaeol. Sci. 40, 4767–4779. doi: 10.1016/j.jas.2013.03.024
Sun, Y., Ritchey, M., Zhong, H., Tang, L., Sergusheva, E., Shi, T., et al. (2024). Variation of millet grain size and cooking techniques across Asia between the late fourth and first millennia BC. Antiquity 98, 401–416. doi: 10.15184/aqy.2024.31
Szpak, P., Metcalfe, J. Z., and Macdonald, R. A. (2017). Best practices for calibrating and reporting stable isotope measurements in archaeology. J. Archaeol. Sci.: Reports 13, 609–616. doi: 10.1016/j.jasrep.2017.05.007
Szymanski, R. M., and Morris, C. F. (2015). Internal structure of carbonized wheat (Triticum spp.) grains: relationships to kernel texture and ploidy. Veget. Hist. Archaeob. 24, 503–515. doi: 10.1007/s00334-014-0508-5
Tafuri, M., Craig, O. E., and Canci, A. (2009). Stable isotope evidence for the consumption of millet and other plants in Bronze Age Italy. Am. J. Phys. Anthropol. 139, 146–153. doi: 10.1002/ajpa.20955
Taylor, J. R. N., and Belton, P. S. (2002). “Sorghum,” in Pseudocereals and Less Common Cereals: Grain Properties and Utilization Potential, eds. P. S. Belton and J. R. N. Taylor. (Berlin, Heidelberg: Springer Berlin Heidelberg), 25–91.
Teira Brión, A. (2022). “Traditional millet cultivation in the Iberian Peninsula: Ethnoarchaeological reflections through the lens of social relations and economic concerns,” in Millet and What Else? The Wider Context of the Adoption of Millet Cultivation in Europe, eds. W. Kirleis, M. Dal Corso and D. Filipović. (Leiden: Sidestone Press), 263–278.
Teira-Brión, A., Kaal, J., and Charles, M. (2024). On the formation of charred millet aggregates in archaeological assemblages. Archaeometry 2024:12983 doi: 10.1111/arcm.12983
Tereso, J. P., Ramil-Rego, P., Pires De Carvalho, T., Almeida-Da-Silva, R., and Vaz, F. C. (2013). Crops and fodder: evidence for storage and processing activities in a functional area at the Roman settlement of Monte Mozinho (northern Portugal). Veg. Hist. Archaeobot. 22, 479–492. doi: 10.1007/s00334-013-0399-x
Vaiglova, P., Gardeisen, A., Buckley, M., Cavanagh, W., Renard, J., Lee-Thorp, J., et al. (2020). Further insight into Neolithic agricultural management at Kouphovouno, southern Greece: expanding the isotopic approach. Archaeol. Anthropol. Sci. 12:43. doi: 10.1007/s12520-019-00960-y
Vaiglova, P., Lazar, N. A., Stroud, E. A., Loftus, E., and Makarewicz, C. A. (2022). Best practices for selecting samples, analyzing data, and publishing results in isotope archaeology. Quat. Int. 650, 86–100. doi: 10.1016/j.quaint.2022.02.027
Varalli, A., D'agostini, F., Madella, M., Fiorentino, G., and Lancelotti, C. (2023). Charring effects on stable carbon and nitrogen isotope values on C4 plants: Inferences for archaeological investigations. J. Archaeol. Sci. 156:105821. doi: 10.1016/j.jas.2023.105821
Wallace, M., Jones, G., Charles, M., Fraser, R., Halstead, P., Heaton, T. H. E., et al. (2013). Stable carbon isotope analysis as a direct means of inferring crop water status and water management practices. World Archaeol. 45, 388–409. doi: 10.1080/00438243.2013.821671
Walsh, R. (2017). Experiments on the effects of charring on Setaria italica (foxtail millet). Vegetat. Hist. Archaeobot. 26, 447–453. doi: 10.1007/s00334-016-0600-0
Wang, T., Wei, D., Chang, X., Yu, Z., Zhang, X., Wang, C., et al. (2019). Tianshanbeilu and the Isotopic Millet Road: reviewing the late Neolithic/Bronze Age radiation of human millet consumption from north China to Europe. Nat. Sci. Rev. 6, 1024-1039. doi: 10.1093/nsr/nwx015
Wang, X., Shang, X., and Hu, Y. (2022). Evaluating the influence of manuring on stable isotope values of foxtail millet: An experimental planting study. Quat. Scie. 42, 1806–1814. doi: 10.11928/j.issn.1001-7410.2022.06.26
Watterson, J. J., Shull, J. M., and Kirleis, A. W. (1993). Quantitation of α-, β-, and γ-kafirins in vitreous and opaque endosperm of Sorghum bicolor. Cereal Chem. 70, 452–457.
Yang, Q., Li, X., Liu, W., Zhou, X., Zhao, K., and Sun, N. (2011a). Carbon isotope fractionation during low temperature carbonization of foxtail and common millets. Org. Geochem. 42, 713–719. doi: 10.1016/j.orggeochem.2011.06.012
Keywords: isotopic analysis, C4 plants, millet, experimental charring, archaeobotany
Citation: Teira-Brión A, Stroud E, Charles M and Bogaard A (2024) The effects of charring on morphology and stable carbon and nitrogen isotope values of common and foxtail millet grains. Front. Environ. Archaeol. 3:1473593. doi: 10.3389/fearc.2024.1473593
Received: 31 July 2024; Accepted: 31 October 2024;
Published: 29 November 2024.
Edited by:
Darren R. Gröcke, Durham University, United KingdomReviewed by:
Alessandra Varalli, UMR7269 Laboratoire Méditerranéen de Préhistoire Europe Afrique (LAMPEA), FranceGiedre Motuzaite Matuzeviciute, Vilnius University, Lithuania
Copyright © 2024 Teira-Brión, Stroud, Charles and Bogaard. This is an open-access article distributed under the terms of the Creative Commons Attribution License (CC BY). The use, distribution or reproduction in other forums is permitted, provided the original author(s) and the copyright owner(s) are credited and that the original publication in this journal is cited, in accordance with accepted academic practice. No use, distribution or reproduction is permitted which does not comply with these terms.
*Correspondence: Andrés Teira-Brión, YW5kcmVzLnRlaXJhYnJpb24mI3gwMDA0MDthcmNoLm94LmFjLnVr; YW5kcmVzLnRlaXJhLmJyaW9uJiN4MDAwNDA7b3V0bG9vay5jb20=