- 1ArchDepth Research Group, Bonn Center for Dependency and Slavery Studies, University of Bonn, Bonn, Germany
- 2Geoarchaeology and Quaternary Science, Institute of Geography and Geology, University of Würzburg, Würzburg, Germany
- 3Nasca-Palpa Management Plan, Peruvian Ministry of Culture, Nasca, Peru
- 4Historical Mapping Laboratory, Faculty of Economic Sciences and Administration, Universidad de la República Uruguay, Montevideo, Uruguay
- 5Department of Ancient American Studies and Ethnology, Institute of Archaeology and Cultural Anthropology, University of Bonn, Bonn, Germany
- 6National Scientific and Technical Research Council (CONICET), Universidad Nacional de Cuyo, Mendoza, Argentina
- 7Department of Christian Archaeology and Byzantine Art History, University of Marburg, Marburg, Germany
- 8Global Heritage Lab, University of Bonn, Bonn, Germany
- 9Commission for Archaeology of Non-European Cultures, German Archaeological Institute, Bonn, Germany
This paper presents an integrative and interdisciplinary approach to the study of ancient agricultural terraces and food production systems. Our approach consists of (1) a resource dependency theoretical framework and (2) the application of a variety of archaeological and geoscientific methods, including archaeological and geomorphological surveys, archaeological excavations, drone surveys, mapping based on satellite imagery and high-resolution digital elevation models (DEMs), geographic information system (GIS) applications, soil testing, phytolith analysis, radiocarbon dating, and calculations of food supply capacity and labor requirements. We apply these to the prehispanic site of Cutamalla (3,300 m asl) in the southern Peruvian Andes, which serves as an ideal and pioneering case study. Previous research has focused primarily on the settlement of Cutamalla, particularly through large-scale archaeological excavations, but less attention has been paid to the extensive farming terraces surrounding the settlement and the close relationship between agricultural and settlement activities. By analyzing both the terrace and settlement levels, we take a new perspective and introduce the term agricultural terrace-settlement system for such complexes. Our results show that the residential occupation of Cutamalla and the use of the surrounding farming terraces coincided: the agricultural terrace-settlement system was intensively used for a relatively short period of about 200 years (~250–40 BCE) during the Formative Late Paracas and transitional Initial Nasca periods, long before the famous Inka terrace agricultural systems. There is no evidence of reoccupation of the site and subsequent reuse of the agricultural system. Our data also document the large extent of agricultural terraces around Cutamalla (221 ha) and that maize was likely a major crop grown there. Finally, we place these findings in their broader socio-economic and ecological context. Cutamalla was an important regional center and economic hub during a very dynamic period characterized by significant population growth and increased violence. Not only a more humid climate, but probably also forced collective labor were cornerstones of substantial agricultural production in Cutamalla and the region.
1 Introduction
Global food consumption would be quite different and much poorer without crops indigenous to the Americas. The significance of mountainous environments for the beginnings of agriculture was first discussed by Vavilov (1992), and indeed the tropical Andes are a key area for the domestication, cultivation, and processing of crops (Denevan, 2001; Pearsall, 2008; Piperno, 2011), including the potato (Solanum tuberosum), squashes (e.g., Cucurbita moschata), quinoa (Chenopodium quinoa), kaniwa (Chenopodium pallidicaule), and olluco (Ullucus tuberosus). Along a wild-domesticated continuum with different stages of domestication and cultivation, Andean communities developed diverse plant management practices that resulted in complex relationships and dependencies between humans and non-humans (Gremillion, 2014; Lema, 2014a,b, 2015). Important bases for this agrobiodiversity are the natural biodiversity of the Andean region and the domestication of its landscapes for human purposes. According to Erickson (2019), domestication involves controlling not only plants and animals, but also the environment to manage resources. Andean “domesticated landscapes” (Erickson, 2019) therefore encompass a variety of farming systems, irrigation systems, and associated features.
Basic Andean farming systems include simple fields (Spanish: chacras), raised fields (Spanish: camellones; Quechua: waru waru; Aymara: suka kollus), sunken gardens (Spanish: chacras hundidas, ojos de agua, or pozas; Quechua: q'ochas; Aymara: q'otanas), and terraces (Spanish: andenes; Quechua: anchakas; Aymara: pata pata or lucris; Treacy, 1994; Erickson, 2019). Water management systems include canal irrigation, aqueducts, reservoirs, check dams, channelized rivers, filtration galleries (Quechua: pukios), artificial pastures (Spanish: bofedales; Aymara: oqhos), and the use of fog oases (Spanish: lomas; Canziani, 2002, 2021; Mächtle et al., 2009; Lane, 2014; Beresford-Jones et al., 2015). Associated features include storage facilities, boundary markers such as walls, trails with stairs and ramps, way stations (Quechua: tambos), and stone-walled corrals to herd South American camelids and, nowadays, mostly animals introduced from the Old World, such as cattle and sheep. Such infrastructure is necessary for the many mobile aspects of agriculture, requiring the constant movement of people, animals, water, raw materials, tools, and crops (Erickson, 2019; Mader et al., 2022, 2023a; Beresford-Jones et al., 2023). It goes without saying that the complexity of the agricultural systems is “rooted” in millennia of indigenous knowledge and experience.
In this paper we focus on ancient terrace agriculture, addressing research desiderata such as periods of terrace construction and use, extent and architectural characteristics of farming terraces, and agricultural practices (e.g., irrigation and crop cultivation). Moreover, these analyses and data have implications to discuss paleoenvironmental conditions and their impact on terrace agriculture, drawing conclusions on larger socio-economic-ecological dynamics. The majority of ancient agricultural terraces in our larger study area, the upper northern Nasca Drainage (Llauta, Laramate, and Ocaña River valleys), are situated at elevations between 1,300 and 3,800 m above sea level (asl), thereby covering several ecological zones, microenvironments, and climates. This diversity enables different farming practices and economic opportunities, entailing a concomitant diversity of crops and other raw materials.
Given the omnipresence of terraces in the Andes, relatively few comprehensive studies have been conducted on this form of agrarian land use, especially for periods prior to the Inka Late Horizon (1450–1532 CE) and in contrast to other Andean farming agrosystems mentioned above (Denevan, 2001; Erickson, 2006, 2019; Williams, 2006). Reasons for this lack of research may be the ordinary character of agricultural terraces compared to other archaeological sites, such as large settlements and sacred buildings, and that methods appropriate for the detailed documenting of these extensive kinds of land modification and management have been developed rather recently. Although aerial imagery has been used in archaeology and physical geography for a long time, recent advances in the collection and analysis of geospatial data only now enable the large-scale documentation of terrace agricultural systems. Such advances comprise the availability of high-resolution satellite imagery, the production of high-resolution drone imagery for photogrammetry, and the production of detailed digital elevation models (DEMs).
Another focus of this paper is on forms of dependency and labor necessary for the management of terrace agricultural systems. To reconstruct patterns of land use, dependency, and labor, we follow an integrative approach which complements digital documentation and modeling through geospatial data with methods from archaeology, physical geography, and archaeobotany. We thus integrate data from surveys, architectural documentation, excavations, remote sensing, soil testing, radiocarbon dating, phytolith analysis, and estimations of agricultural production. We apply this approach to the prehispanic agricultural terraces and settlement of Cutamalla (~250–40 BCE), which serves as a uniquely detailed case study and prime example of an archaeological complex comprising terrace agriculture and settlement. We define such complexes as agricultural terrace-settlement systems. Cutamalla is situated in the southern highlands of Peru, in the quechua zone at an elevation of 3,300 m asl, approximately 6 km southeast of the modern village of Laramate, in the province of Lucanas, Ayacucho (Figure 1). The settlement of Cutamalla extends along a ridge above the Laramate River and consists of two sectors: domestic Sector A is located in the southern part, while public Sector B is in the northern part (Reindel et al., 2015; Reindel and Isla, 2017, 2018; Mader et al., 2018, 2023a; Mader, 2019a). The slopes around the settlement are covered with extensive ancient agricultural terraces belonging to the Te-07 terrace agricultural system (Figure 2), with two other terrace systems (Te-09 and Te-10) to the south.
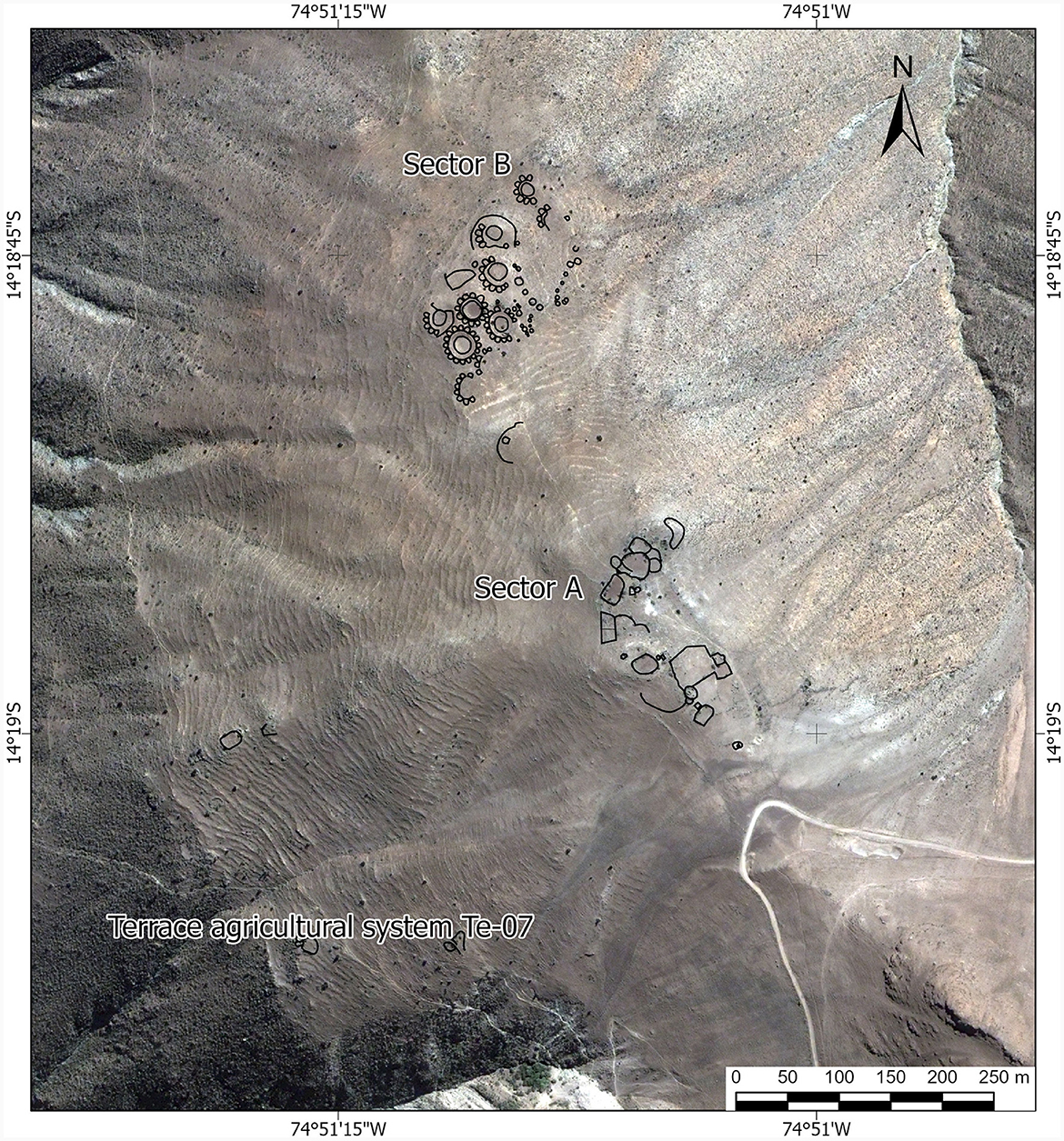
Figure 2. Settlement structures (Sectors A and B) and terrace agricultural system Te-07 in Cutamalla.
The quechua zone (2,300–3,500 m asl), where Cutamalla is located, is the most favorable ecological tier for agriculture in the western central Andes (Pulgar Vidal, 1981; Sandweiss and Richardson, 2008). The reduced availability of oxygen in the air due to the low atmospheric pressure becomes perceptible in quechua elevations. Nevertheless, factors that favor agricultural production here include a temperate climate (although with strong diurnal temperature fluctuations), fertile soils, and seasonal rainfall, mainly from December to March, averaging 250–300 mm, which allows for rainfed and irrigated forms of agriculture. Thus, there is a wide spectrum of crops cultivated in the quechua, which also marks the upper boundary for the cultivation of maize (Zea mays), one of the most significant agricultural products in this ecological tier. Other important crops comprise many species of beans, squashes, and root vegetables such as the potato (Solanum tuberosum) and arracacha (Arracacia xanthorrhiza).
In our broader study area of the upper northern Nasca Drainage, terrace farming systems are often clearly associated with settlements, as evidenced by their proximity and corresponding radiocarbon data and archaeological material culture (although surface finds on terraces are scarce and often non-diagnostic). Thus, settlement data provide valuable contextual information about agricultural terraces, and vice versa. Based on high-resolution archaeological surveys and excavations, the regional prehispanic settlement history is well-documented, from its beginnings during the Archaic period (8000–3500 BCE) to the arrival of the Spaniards in 1532 CE (Reindel, 2009; Unkel et al., 2012; Soßna, 2015; Isla and Reindel, 2017; Beresford-Jones et al., 2023; Mader et al., 2023b). As in most Andean regions, there is no high-resolution documentation of the land use history in our study area. The approach presented in this paper aims to fill this gap by studying ancient terrace farming systems and forms of dependency.
2 Monumental landscapes of everyday life: Andean terrace agricultural systems
Terrace farming is the most impressive, large-scale agricultural landscape modification and the most visible evidence of land use change throughout the ancient Andes (Donkin, 1979; Denevan, 2001). In short, Andean terraces are “a monumental landscape of everyday life” (Erickson, 2019), a designation that emphasizes their importance to prehispanic societies and illustrates the widespread spatial and temporal extent of these agricultural practices. Land use, cultivation, and crop management in the prehispanic Andes certainly went beyond mere economic activities and were embedded in the daily lives and socio-cultural practices of these communities. Most agricultural terraces are located at altitudes between 500 and 4,000 m asl, where the sharp slopes of the Andes make terracing essential for agriculture. This land management strategy creates flat field surfaces in a stepwise manner for all sorts of farming activities, including soil accumulation and conservation, water distribution, cultivation of crops, and livestock breeding, and can also prevent erosion and landslides (Donkin, 1979; Treacy and Denevan, 1994).
When talking about terracing in the Andes, the highly engineered terraces at Inka sites such as Machu Picchu, Cusco, Ollantaytambo, Pisaq, and Tipon inevitably come into mind (Kendall, 2004; Kendall and Rodríguez, 2009; D'Altroy, 2015; Castro et al., 2019; Ortloff, 2019; Canziani, 2021). Ancient Andean terracing, however, took many forms and patterns, depending on the ecological zone, local terrain, availability of building material and other resources, type of agriculture, economic needs, organization of labor and society, and time period with its respective knowledge of terrace construction. Terraces thus range from simple lynchets (earth terraces) to elaborate bench terraces (stone-faced platforms), and from isolated terraces to complex terraced landscapes. Denevan (2001) distinguishes between four types of terracing in the Andes: cross-channel terraces and check dams, sloping-field terraces, bench terraces, and broad-field terraces. Another pioneer in the examination and characterization of Andean terrace agricultural systems was Kendall (2004, 2005, 2013), Kendall and Rodríguez (2009), with a specific focus on applied archaeology and the promotion of agricultural sustainability through the reuse of ancient terraces. Indeed, the abandonment, conservation, and revitalization of ancient agricultural terraces have been long-standing research themes defining approaches to Andean farming systems (Guillet, 1987; Llerena et al., 2004; Londoño et al., 2017).
For the construction of terraces, Andean farmers and workers mostly used local materials, such as fieldstones (Erickson, 2019). The average size of stones used in building terrace walls can vary considerably and seemingly developed from using bigger stones to smaller stones over the prehispanic period, at least in our study area on the western flank of the Peruvian Andes. The height and quality of terrace walls and the area of arable land can also show some variation. All these variables are related to different parameters, including slope inclination, form of farming, and time period with its terrace knowledge, experience, and standards. Terraces often support not just fields but also irrigation canals, paths and stairs, buildings and wall niches for storage and refuge, and towers for supervision. In a broader context, Andean terrace agricultural systems are usually directly connected to settlements and other architectural features such as corrals, water reservoirs, boundary markers, and roads (Denevan, 2001; Erickson, 2019).
Andean agricultural regimes, and prehispanic terraced agriculture in particular, mostly entailed irrigation systems. There were basically two types of water supply for farming terraces: irrigation agriculture and rainfed agriculture, although combinations of both forms exist (Williams, 2006; Aguirre-Morales, 2009; Erickson, 2019). Irrigation canals distributed water over terraces from different sources, including water reservoirs and dams, rivers, and alpine headwaters. Either way, highland water management further upstream was crucial for agriculture down all the Andean slopes, particularly in the arid desert of the Pacific coast. Such large-scale irrigation and water management practices required a great deal of organization, cooperation, and coordination among farming communities (Lane, 2014). In cases of ancient rainfed terraces, which cannot be used today because of the dry climate, this evidence of rainfall farming can even serve as a proxy for more humid climatic conditions and climate change in the past.
Andean societies developed and experimented with various agricultural strategies from the beginning of farming ~10,000 BCE in the region (Piperno and Stothert, 2003; Piperno, 2011; Leon, 2014), bringing about massive changes in the environment in terms of land use and the management of crops and livestock. The most substantial disruption to indigenous agricultural practices in the Andes was certainly the European invasion in the sixteenth century with the introduction of plants, animals, and diseases from the Old World as well as new forms of authority and power, socio-economic organization, land distribution, dependency and oppression, and labor exploitation in the context of colonialism. In spite of these disastrous changes and consequences, Andean farmers at the present time manage to maintain some altered versions of native agrarian structures, crop cultivation, animal keeping, and labor organization. With the exception of a few more densely populated highland zones such as the Cusco region, however, only a fraction of ancient farming terraces are still in use nowadays and they are often in rather poor condition, modified, or even demolished because of modern and mechanized methods of agriculture. In contrast, the soil fertility of ancient terraced fields can remain high (Nanavati et al., 2016; Sandor et al., 2022), even if they have been abandoned or poorly maintained (for research with different findings, see Goodman-Elgar, 2008). In general, whether the properties of agricultural soils improve or degrade over time is related to a combination of environmental, agricultural, and social factors (Sandor and Homburg, 2017).
3 Dependency and labor in the ancient Andes
The construction, use, and maintenance of terrace agricultural systems in the prehispanic Andes unavoidably involved human labor and different degrees of socio-political-economic dependencies among people to carry out and organize such activities. Conditions of labor and dependency can vary substantially across space and time in response to diverse aspects, including the natural environment, resource availability, demography, political economy, social organization and norms, legislation, moral standards and values, ethnicity, gender, and age. Labor and dependency relations, in particular at an interpersonal level, are difficult to determine based on the archaeological record alone and without any direct written sources. This paper therefore presents and applies the theoretical-methodological concept of “resource dependency” by which we provide an analytical framework to study past forms of human cooperation and exploitation (Mader et al., 2023a,b).
Resource dependency was recently defined as entailing two elementary types of structural dependency which constantly interact within their particular ecological and socio-cultural contexts (Mader et al., 2023a). The first type concerns human dependencies on resources of any kind, including the control of access to those resources, while the second concerns dependencies between people, seen in all sorts of work relations, labor exploitation, and beyond. These relations of dependency are frequently asymmetrical, as in the notion of “asymmetrical dependency,” basically describing institutionalized circumstances in which certain actors are able to command the actions and resource use of others (Winnebeck et al., 2023). Hence, asymmetrical dependency comprises phenomena of coercion such as tributary labor, servitude, and—perhaps its strongest form—slavery. The relations between humans and resources, depicted in the first and most fundamental type of dependency in the concept of resource dependency, are structurally asymmetrical because humans and resources represent different kinds of actors with different agencies (on material and relational agency, see Van Oyen, 2018).
The concept of resource dependency understands resources in a comprehensive way, using a broad definition which encompasses every kind of resource, including land, raw materials, food, labor or human resources, knowledge, experience, technology, infrastructure, and many forms of investment such as time, money, and other capital. All these types of resources are highly relevant in regard to terraced agriculture, monetary forms of capital most probably being the only exception in prehispanic times. Two points here in particular make terrace agricultural systems an effective focus for a study of resource dependencies. First, control over such resources involves the organization of labor, and may be a source of power and unequal wealth accumulation. Second, different types of resources are linked through dependency relations, and labor can be considered a key component in this analytical framework.
Non-monetary traditional forms of Andean labor can be divided into three general categories: ayni, mink'a, and mit'a (in Quechua), although the terms may vary regionally (Erickson, 2019; Sammells, 2019). Ayni (also written as ayniy or aini) is a more voluntary form of communal labor, especially in the context of agricultural activities, characterized by the delayed and equitable exchange of labor and goods among community members and families (Mayer, 2002; Wutich et al., 2017). Mink'a (also written as minka or minga), mingaco, or faena covers communal labor in many sectors, including agriculture, construction, and infrastructure (Erickson, 2019; Sammells, 2019). Work is often coordinated by a local leader or host, who provides feasts and drinks, portions of the harvest, and so on, in exchange for these collective efforts. Mit'a (also spelled mita, especially in its amplified from during the colonial period) is a compulsory tribute or tax to the state in the form of collective labor, comparable to types of forced and unpaid labor for public projects, such as corvée and statute labor (D'Altroy and Earle, 1985; D'Altroy, 2015). This form of labor service is primarily associated with Tawantinsuyu, the Inka empire, and was performed on its state lands—including fields, pastures, farms, roads, and mines—and for the expansion of the state.
Since there are no direct written records from prehispanic times, interdisciplinary archaeological research is necessary to examine forms of dependency and labor in the ancient Andes, in particular for periods before the Inka Late Horizon (1450–1532 CE). Our starting point for approaching these dependency and labor relations is the study of several resources, as defined above, related to agriculture and within the theoretical-methodological framework of resource dependency. We do so using multiple lines of evidence, ranging from the landscape to the settlement, household, and burial levels, and a methodological toolkit, detailed below, that integrates archaeological, archeobotanical, geoarchaeological, and digital techniques.
4 Materials and methods
4.1 Archaeological and geomorphological survey
Over recent years, the Nasca-Palpa Archaeological Project has conducted systematic surveys on regional and local scales in the study area (Reindel, 2009; Soßna, 2015; Isla and Reindel, 2017; Mader et al., 2023b). Archaeological sites, including ancient farming terraces, have been identified by extensive, regional pedestrian surveys and aerial archaeology (satellite and drone images). Many settlements and terrace agricultural systems, such as Cutamalla, have been surveyed intensively with a high degree of coverage. Collecting and analyzing surface artifacts and test-pitting have been integral parts of the process at all stages of our surveys (Banning, 2002). Another important aspect of our archaeological and geomorphological surveys was to locate appropriate spots for soil testing. In Cutamalla, we looked for well-preserved terrace walls and terraces with no or few signs of erosion of their fills and different slope positions.
4.2 Archaeological excavation
Various areas of Cutamalla were excavated between 2007 and 2021, including large-scale archaeological excavations (15 units of 10 × 10 m) and test excavations (two units of different dimensions) in Sectors A and B of the settlement (for details on archaeological excavation methods, see Mader, 2013, 2019a; Reindel et al., 2015; Reindel and Isla, 2017, 2018).
4.3 Mapping of agricultural terraces based on satellite images
In order to accurately map the terrace agricultural systems Te-07, Te-09, and Te-10 in Cutamalla and its surroundings, we tested and implemented various methods using different data sets in a geographic information system (GIS) environment. As a first step, a high-resolution QuickBird satellite image (spatial resolution: 60 cm) covering the agricultural areas was manually georeferenced using Google Earth data. The satellite imagery was then carefully examined for distinct patterns, such as parallel lines indicating the presence of terraces. In this way, individual terrace walls and boundaries were manually identified and digitized. Due to the sometimes poor lighting conditions of the QuickBird satellite imagery, satellite imagery from Google Earth Pro was occasionally used for mapping purposes. Overall, there were some difficulties in identifying terraces, particularly in areas covered by vegetation. Animal tracks and geological formations were also sometimes difficult to distinguish from terrace walls. In some areas, satellite imagery indicated that the area was likely to be terraced, but the terrace walls were not visible in sufficient detail to be mapped.
4.4 Photogrammetric documentation and DEM generation
High-resolution DEMs can be used to determine the micro-topography of a site and are considered an excellent tool for locating agricultural terraces (Spanò et al., 2018). For Cutamalla, a high-resolution DEM was acquired in 2011 by aerial photogrammetry using an unmanned aerial vehicle (UAV) as part of the Nasca-Palpa Project (Friedli and Theiler, 2014). The main advantages of creating DEMs based on UAV flights, especially for small study areas, are the low effort required for implementation and the cost and time savings during fieldwork (Uysal et al., 2015; Ferrarese et al., 2019). In Cutamalla, two Falcon 8 UAVs, each equipped with a Sony NEX 5 compact digital camera, L1 GPS antenna, and MEMS IMU, were used to capture the images. A total of 790 images were taken at an altitude of 60 m above ground. The images were georeferenced using manually distributed targets as ground control points (GCPs) and measured using differential carrier-phase-based GNSS. The photogrammetric processing of the images into the DEM and orthophoto was performed using the Pix4Dmapper (Pix4D) software. The resulting DEM has a spatial resolution of 5 cm (for more details, see Friedli and Theiler, 2014) and covers Sectors A and B of the settlement and part of the terrace agricultural system Te-07.
4.5 GIS-based identification and analysis of agricultural terraces from a high-resolution DEM
GIS-based identification of agricultural terraces from high-resolution DEMs is mainly based on the slope differences (Spanò et al., 2018; Zhao et al., 2021). In order to map the terraces surrounding the settlement structures, the DEM of Cutamalla was first preprocessed and the slope gradients and profile curvature were calculated (Figure 3). Since the terrain is very complex and the individual terraces are inconsistent in size and often highly deformed, we divided the area into homogeneous sub-areas in terms of slope characteristics in order to extract as many terraces as possible. The terraced areas can be easily identified by the slope, for which an upper limit of 18.5° was set (Figures 3A, 4). However, due to the heterogeneity of the terrain, the limit varies slightly in other areas. In the case of curvature, the positive and negative extremes occur mainly on the terrace walls, so they are easy to extract. In Cutamalla, a value of −0.1 was used to identify the lower end of a terrace wall (Figure 3B). Based on these threshold values for slope and curvature, the raster data were analyzed, automatically classified, vectorized, and converted into shapefiles (i.e., terrace areas and walls). In some cases, where the terrain was extremely rough, such as on deformed terraces, manual corrections had to be made.
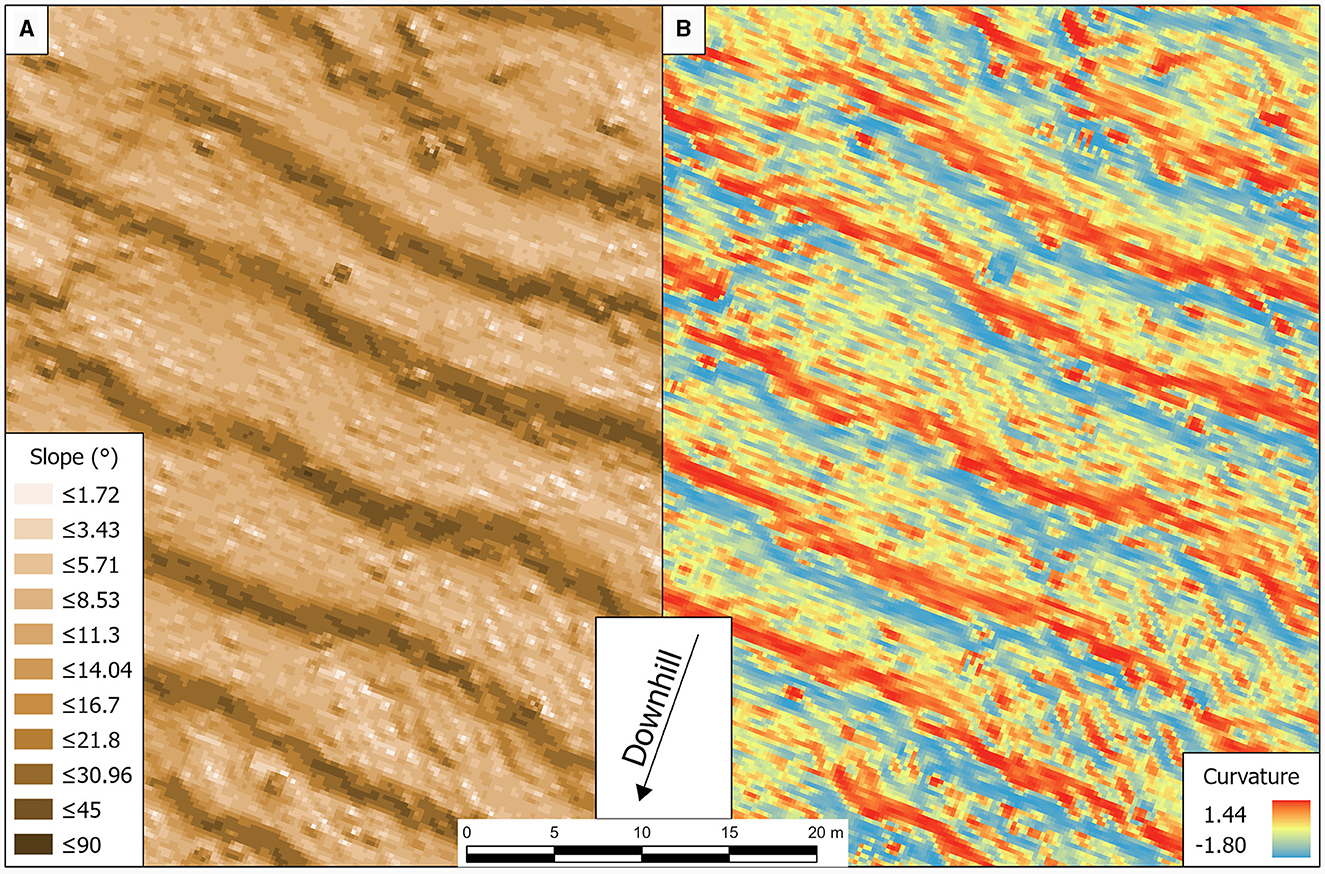
Figure 3. Comparison of slope gradients in ° (A) and curvatures (B) of a selected area of terrace agricultural system Te-07.
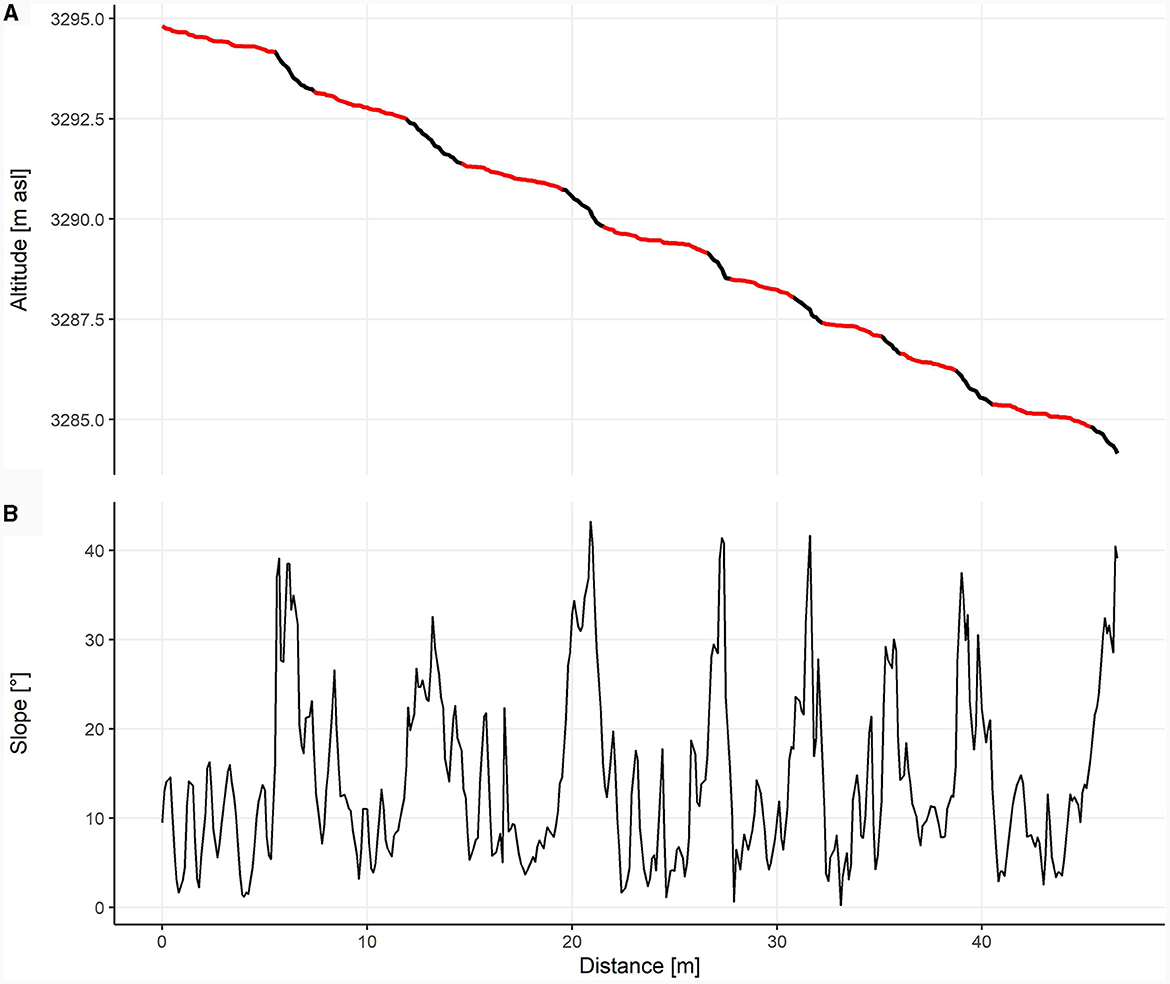
Figure 4. Terrain profile 1 (A) and the corresponding slopes (B). Potential terrace areas (slope < 18.5°) are colored red. The location of the profile is shown in Figure 6.
In addition, further information can be derived from the analysis of the DEM, such as the general characteristics of the study area (including elevation, slope, aspect, and topographic water index) and the agricultural terraces (including location, shape, and size). The average height of the terrace walls and the width of the terraces can be determined from the slope profiles of the terrace areas. For Cutamalla, we evaluated a total of five terrain profiles with an average length of 73.4 m (Figure 4). Moreover, the outcomes of the DEM analyses were used to validate the mapping derived from satellite imagery. In this way, misinterpretations due to vegetation or animal tracks could be detected and corrected. The final step will be to validate the terrace systems mapped in the GIS with actual field observations through surveys. This will allow any necessary adjustments or refinements to be made to improve the accuracy of the mapping.
4.6 Soil testing and sediment analysis of agricultural terrace soils
Pedological investigations of ancient agricultural terraces provide valuable insights into soil properties that can help us to reconstruct ancient land management, soil conservation, and agricultural practices, and assess associated long-term effects on soil development and quality (Kemp et al., 2006; Goodman-Elgar, 2008; Nanavati et al., 2016; Sandor et al., 2022; Handley et al., 2023). We excavated soil pits in a total of three terraces within the Cutamalla terrace system Te-07 (profiles Te-07.1, Te-07.2, and Te-07.4), accompanied by a reference soil profile (Te-07.3) with no archaeological evidence or actual use. Soil field properties were described based on the German soil survey manual, KA5 (Ad-hoc-AG Boden, 2005) and were later transferred to the FAO guidelines (FAO, 2006; IUSS Working Group WRB, 2015).
A total of 17 soil samples were taken and subjected to further laboratory treatment to determine various soil properties. The individual profiles were sampled at consistent intervals (10–20 cm), with emphasis placed on the laboratory analysis on the topsoil horizons (Ap, Ah). Physico-chemical soil analyses were performed at the Laboratory for Geomorphology and Soil Science at the University of Würzburg. Sample pretreatment and water content determination were in line with the international norm (ISO 11465, 1993; ISO 11464, 2006). Grain size distributions were measured using laser diffractometry (HORIBA Partica LA-960V2) for grain sizes < 2 mm, after sample partition by dry sieving. Sample preparation for grain size analysis included the removal of organic matter with H2O2 and dispersion with sodium pyrophosphate (Na4P2O7). Spectrophotometric color measurements were undertaken using a ColorLite SPH−870 with a MA38 measuring adapter (Meyer-Heintze et al., 2020). The pH values were measured in a 1:2.5 suspension in 0.01 M CaCl2 solution. Total carbon, nitrogen, and sulfur contents were measured on milled samples using the CNS analyzer vario EL cube* (Elementar). The carbon/nitrogen (C/N) ratios were calculated to evaluate the mineralization rates in the soil. Soil organic matter contents (SOM mass-%) were determined by means of loss on ignition (LOI) at 550°C (Dean, 1974).
4.7 Phytolith analysis
Sub-fossil biological remains within ancient terrace soil sequences offer significant insights into crop selection, fertilization, and irrigation practices (Handley et al., 2023). While past examinations of plant macro remains and flotation analyses from settlement areas in Cutamalla have yielded limited results, underscoring the challenging preservation conditions for organic materials in this semi-arid region, we have chosen to prioritize phytolith analysis. Phytoliths, microscopic silica structures formed in plants, can endure in the soil for extended periods (Qader et al., 2023). Analyzing phytoliths therefore provides a unique perspective on ancient agricultural practices, unveiling details about plant species, land use, and cereal processing strategies, as well as paleoenvironmental conditions (Korstanje and Cuenya, 2010; Meister et al., 2017; Dietrich et al., 2019; Kirchner et al., 2022). Specifically, the distinctive presence of wavy-topped rondels found in the cobs and fruit cases of maize serve as a diagnostic indicator for cultivated maize (Bozarth, 1998; Pearsall et al., 2003; Piperno, 2006; Handley et al., 2023).
We conducted phytolith analysis from all soil samples at the University of Würzburg following the extraction procedures outlined by Albert et al. (1999). Counting was performed using a KERN OBE-114 microscope at 400x magnification. Images of selected phytoliths were recorded using a KERN ODC 825 microscope camera. The morphological identification of phytoliths was based on the relevant literature (e.g., Twiss, 1992; Bozarth, 1998; Pearsall et al., 2003; Piperno, 2006). The International Code for Phytolith Nomenclature was followed where possible (Neumann et al., 2019). Phytoliths that could not be assigned to a clear description were recorded as weathered morphotypes. The phytolith concentrations were calculated as described by Albert et al. (1999).
4.8 Radiocarbon dating
Radiocarbon samples allow us to place the terraces in time and to compare chronologies to nearby residential occupations at the Cutamalla settlement. We compiled, corrected, and added context information for all 22 dates from the site (Supplementary material A; Unkel et al., 2012; Mader et al., 2018; Reindel and Isla, 2018). Here we present four sediment bulk samples from terraces of the associated terrace system Te-07, which were processed at the Curt-Engelhorn-Centre of Archaeometry in Mannheim and pretreated with the acid-alkali-acid method to remove contamination by carbonates and humic acids. In OxCal 4.4 (Bronk Ramsey, 2009a), we calibrated dates with a site-specific mixed curve of SHCal20 (Hogg et al., 2020) and IntCal20 (Reimer et al., 2020) that reflects modern mixtures of air from the Northern and Southern Hemispheres at Cutamalla (Figure 5), following recently published methods (Ancapichún et al., 2022). We represent this non-normal distribution as an inline array in the OxCal code (Supplementary material B).
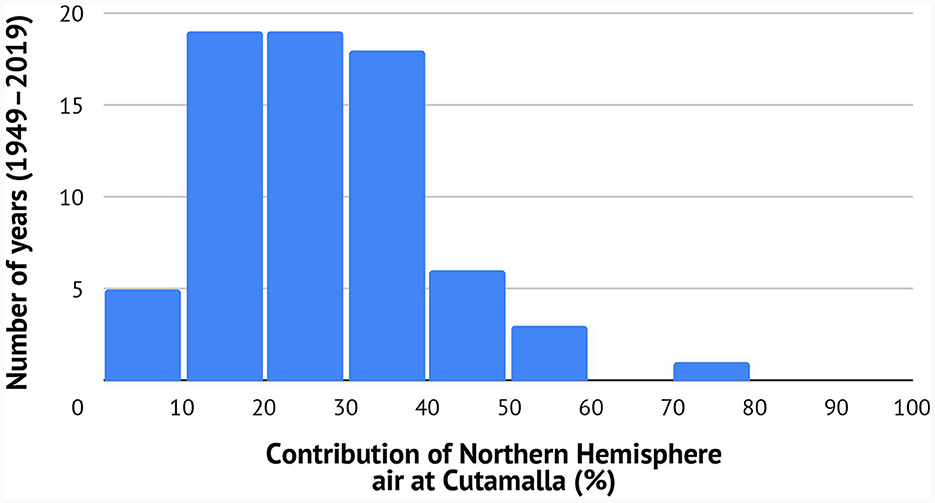
Figure 5. Contribution of Northern Hemisphere air at Cutamalla (14.316°S, 74.852°W) during the span 1949–2019 CE.
Bayesian modeling is especially important in this case because many of the dates fall on a calibration curve reversal, so that despite low error ranges, individual calibrations are bimodal and imprecise. Calibrations become even less precise when using a mixed calibration curve. These situations can be dealt with by incorporating stratigraphic priors (e.g., Rose et al., 2022; Osborn et al., 2023). At Cutamalla, excavations of five structures (UA 6, 7, 11, 12, and 17) have multiple radiocarbon samples. Within each structure, dates from lower strata were modeled as older and dates from the same strata were grouped in a phase (Supplementary material B). All dates were grouped as part of an overall phase representing the site's occupation, summarized as a kernel density estimate (KDE; Bronk Ramsey, 2017). The four terrace dates do not have direct stratigraphic relationships to the other dates, so they were calibrated but not modeled. We report modeled results in italics and medians with a leading tilde (~).
4.9 Calculation of food supply capacity and labor force
We estimate food supply capacity through crop production from the Cutamalla terrace system (Te-07) and surrounding systems (Te-09 and Te-10) based on three values: (1) agricultural area (cf. Section 5.3), (2) estimated late prehistoric crop yields for maize and potatoes (500–1,200 kg/ha for maize, Schroeder, 2001; 430–2,700 kg/ha for potatoes, Hastorf, 1993), and (3) annual calorie consumption per person (Meister et al., 2018). The latter is calculated from the ratio of the minimum daily requirement of calories per person in Peru in 2021 (1,793 kcal/day; FAOSTAT, 2023) and the average caloric value of each crop, using 3,400 kcal/kg for maize and 3,200 kcal/kg for potatoes to model mixed cultivation (Hastorf, 1993).
Hastorf (1993) suggests that farming 50 ha required the full-time labor of 25 people during the entire cultivation season in the prehispanic central Andes (see also Costin, 2015). We use these figures as a guideline for a rough estimate of the labor required to cultivate the terraced fields at Cutamalla and its surroundings.
5 Results
5.1 Archaeological surveys and excavations
Cutamalla (PAP-767) was recorded in 2006 by a regional archaeological survey of the Nasca-Palpa Project (Reindel, 2010), followed by site surveys and excavations on different scales. Archaeological excavations provided rich data on the chronology, layout, and use of the settlement, as well as a great number of archaeological finds such as ceramics, lithic artifacts, malacological material, animal skeletal remains, and human burials (Reindel and Isla, 2017, 2018). Cutamalla is the type site for an architectural pattern called the flower structure, a multi-functional stone building consisting of an inner circular patio ~20 m in diameter surrounded by smaller D-shaped units (Mader, 2013, 2019a; Reindel and Isla, 2017, 2018; Mader et al., 2023a). The flower structures (n = 10) are located in Sector B of the settlement (Figure 2).
Cutamalla dates primarily to the Formative Late Paracas (370–200 BCE) and transitional Initial Nasca (120 BCE−90 CE) phases (Reindel, 2009; Unkel et al., 2012; Mader et al., 2018; Reindel and Isla, 2018). Archaeological evidence suggests that the site was an important center of economic production and distribution to other regions. This evidence includes the abundance of agricultural terraces, work areas, obsidian artifacts at all stages of the lithic reduction sequence, and large quantities of ceramic spindle whorls and camelid skeletal remains (Reindel and Isla, 2017; Mader, 2019a,b; Mader et al., 2022, 2023a). Luxury goods such as jewelry, however, are rare, and the burials discovered so far (n = 5) are rather simple, usually containing only one grave good such as an obsidian projectile point or, in one case, a shell bead. Furthermore, the settlement layout supports the interpretation that Cutamalla was occupied by commoners such as farmers and other workers.
Since our analyses combine settlement and landscape levels, data on the settlement were complemented by surveys of the surrounding terrace agricultural systems Te-07, Te-09 (Ruyruncural), and Te-10 (Figure 5). The architecture of the agricultural terraces in these three systems is very similar and simple, sometimes associated with rather small stone buildings at the margins of terraced fields. Canals for irrigation were not identified by our field surveys, suggesting rainfed agriculture. The terrace walls were constructed with fairly large stones (up to 0.5 m in diameter). This pattern of using large stones in the walls is typical of Formative agricultural terraces in the region. The terrace walls at Cutamalla are quite eroded and some of them have collapsed. In terms of construction technique, at least two rows of stones were used, with remaining average heights ranging between 0.6 and 1.3 m. There is no indication that the walls were built with mortar, mud, and vegetal material, or that the stones were carved. Only a few artifacts, almost none of them diagnostic, were recovered from the surface of the terraced fields, but it is clear from their proximity and overall arrangement that the terraces of the systems Te-07, Te-09, Te-10, and sectors A and B of the settlement belong to the same complex and period.
5.2 Radiocarbon chronology of the Cutamalla settlement
The Bayesian model indicates that the starting and ending boundaries of occupation at Cutamalla were ~250 BCE (390–190, 95%) and ~40 BCE (100 BCE–20 CE, 95%; Figure 6). The initial occupation of the site was probably around ~230–210 BCE, if we look at the peak of the probability distribution and disregard the long leading tail that seems to be due to the calibration curve reversal. Surprisingly, the model's boundaries were not affected by including stratigraphic priors within each architectural unit, but these do offer much more precise estimates for individual contexts. Likewise, the site-specific calibration curve had a very minor effect on the results, but it will be important for more precise chronologies. One date is near the lower limit of acceptable agreement indices (60%), but its stratigraphic position is modeled correctly so we retain it (MAMS-24515). We manually removed one early outlier (Hd-26861) after unsuccessful attempts to include it with a charcoal outlier model (Bronk Ramsey, 2009b). The resulting Bayesian model has acceptable agreement indices (Amodel = 85%, Aoverall = 81%).
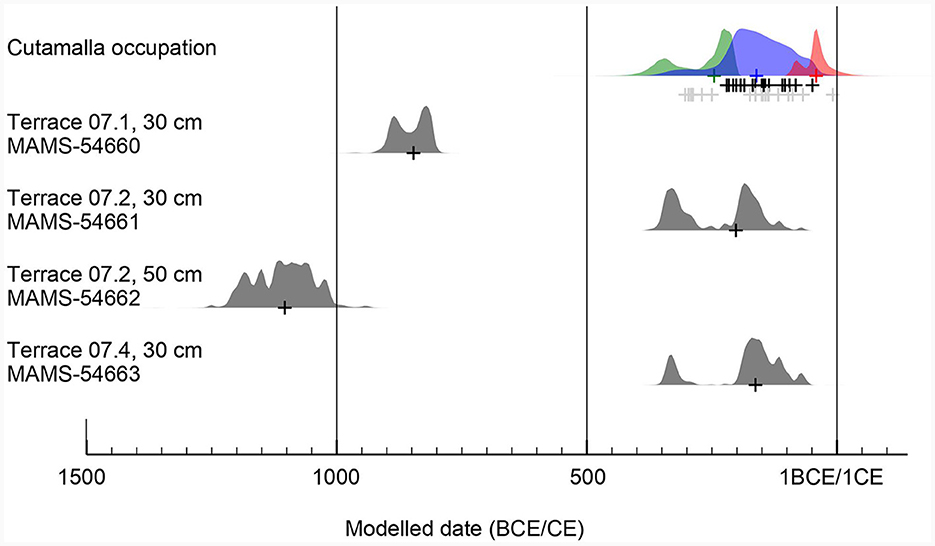
Figure 6. Probability curves for the Bayesian model of 21 dates from Cutamalla, showing the boundaries (in green and red) and the KDE plot (in blue, following Bronk Ramsey, 2017) and unmodeled calibrations of the four terrace dates.
The site's principal occupation lasted only ~190 years (110–340, 95%) or ~7.0 generations (4.0–12.6, 95%), assuming 27-year generations (Wang et al., 2023). The shorter end of this span seems more likely, if we disregard the imprecision caused by the calibration curve reversal. In any case, this is shorter than initially suggested by comparing the site's diagnostic ceramics to the regional culture history (Unkel et al., 2012). One possible reason for this is that the culture history does not allow for temporal gaps or overlaps in ceramic styles, which seem quite plausible (Carmichael, 2019). Future Bayesian models should allow for this, as well as the possibility of notable inter-site temporal variability in terms of ceramic styles.
5.3 Mapped terrace systems
The study area in Cutamalla covered by the DEM is 28.46 ha, of which 10.75 ha could be identified as terrace agricultural land (Figure 7), with the maximum slope of the terraces ranging from 20 to 25°. An area of 1.32 ha is characterized by very flat, non-terraced terrain outside the settlement areas, which may also have been used for agriculture in the past. In very steep areas (average slope >25–30°), terrace structures were almost non-existent. The terraces identified are generally very heterogeneous. Larger terraces predominate in flatter areas, smaller terraces on steeper slopes. In total, 316 terrace walls with a total length of 10.57 km were mapped. Based on the slope profile analysis, the average terrace width is 4.8 m, the average terrace wall height is 0.83 m, and the average terrace slope is 9.49°, although the values vary considerably between individual terraces (Figure 4). In general, the slopes increase significantly at the edges of the terraces, while the terraced areas are characterized by low slopes. Irregularities on the surface, characterized by deviations of the slope values, probably show terrace structures that have collapsed due to erosion. DEM analysis also identified areas in the south of the system where terraces were likely misidentified from the satellite imagery when, in fact, they were animal tracks (Figure 7).
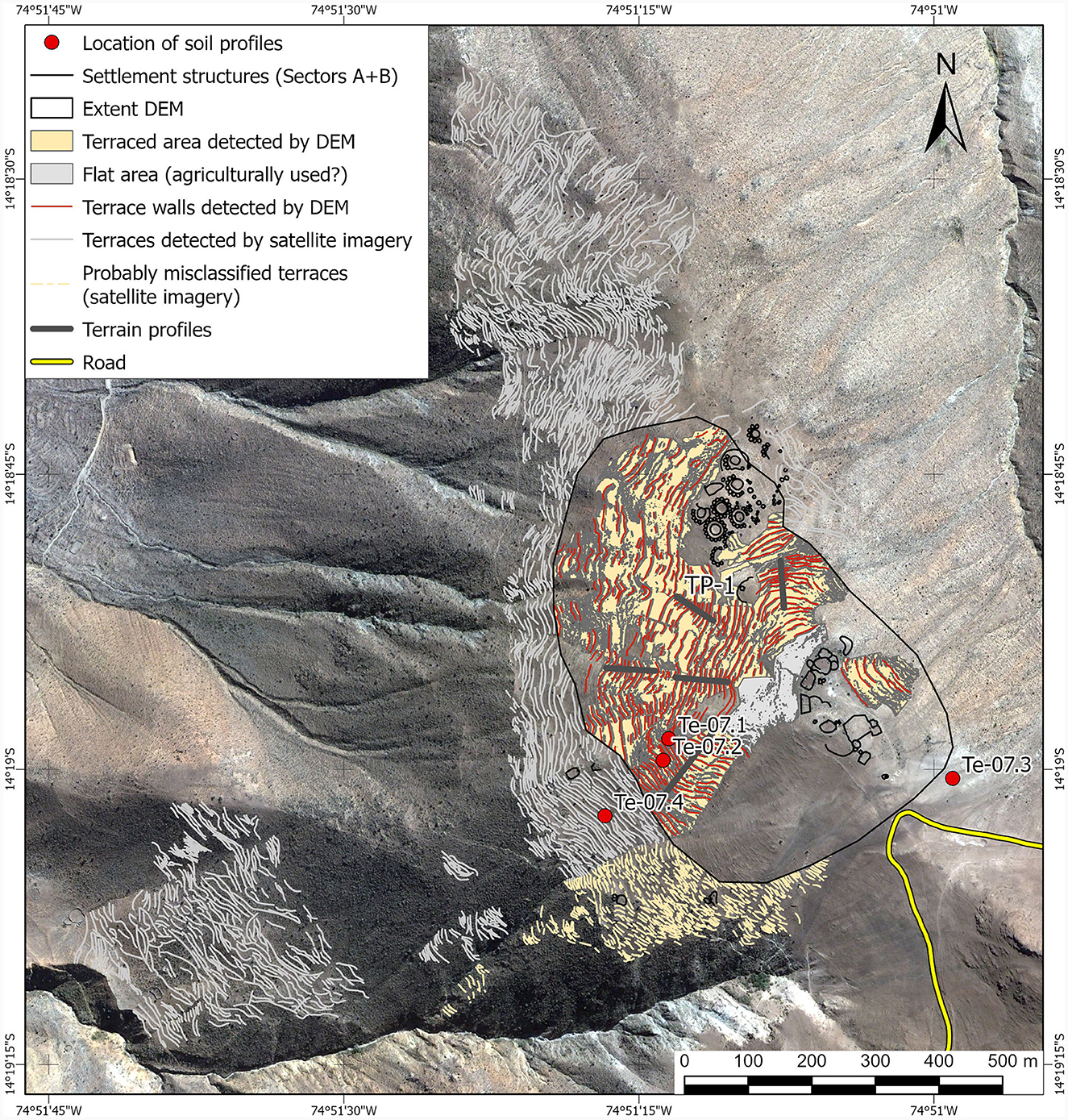
Figure 7. Results of the DEM analysis of terrace surfaces and walls of terrace agricultural system Te-07. The terraced walls shown outside the DEM coverage were mapped using satellite imagery. Note the locations of the investigated soil and terrain profiles.
Based on the mapping of the extended area using satellite imagery, the total terrace agricultural system Te-07 measures 32 ha and consists of 2,069 terrace walls with a total length of 68.5 km (Figures 7–9). The terrace agricultural system Te-09 (Ruyruncural) measures ~135 ha and consists of 852 terrace walls with a total length of 68.3 km. The terrace agricultural system Te-10 measures ~54 ha, with 1,221 terrace walls and a total length of 62.3 km (Figures 8, 9). All three systems together cover a total area of 221 ha. While Te-07 is a more coherent terrace agricultural system, Te-09 and Te-10 often represent scattered subsystems of terraces.
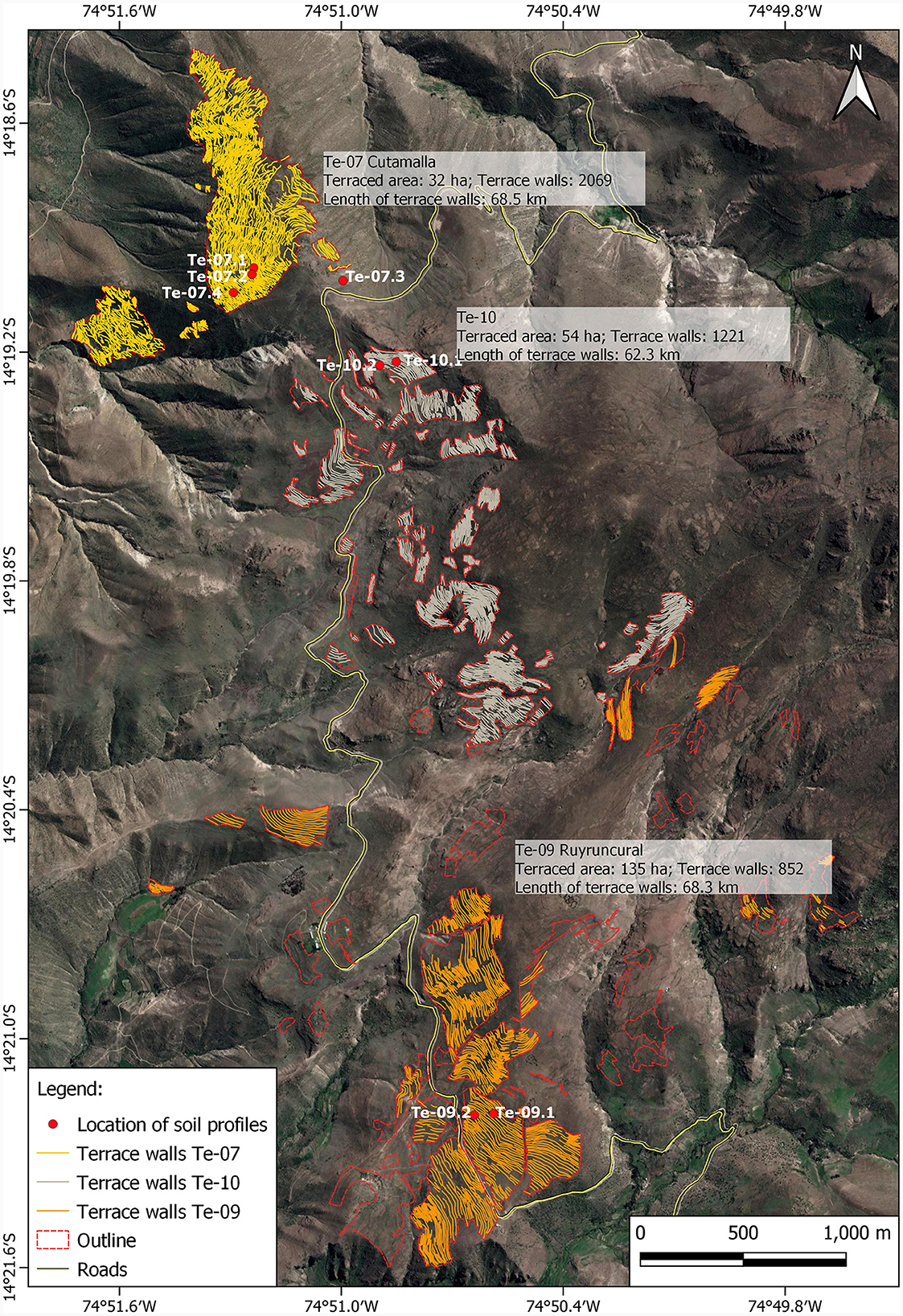
Figure 8. Location and detailed mapping of the agricultural terrace systems Te-07, Te-09, and Te-10 in the surroundings of the Cutamalla archaeological complex. Note that only soil profiles from Te-07 are described and analyzed in this paper.
5.4 Terrace and reference soil profiles
The terrace profiles Te-07.1 (14.3163°S, 74.8539°W, 3,268 m asl), Te-07.2 (14.3166°S, 74.8540°W, 3,257 m asl), and Te-07.4 (14.3174°S, 74.8549°W, 3,203 m asl) are located ~200–400 m south-west of the domestic settlement (Sector A) of Cutamalla at upper and middle slope positions along a 400 m long, south-west facing slope with an average inclination of 18°. The sampled terraces showed very few signs of erosion. Soil control profile Te-07.3 (14.3169°S, 74.8499°W, 3,291 m asl) is located approximately 150 m south-east of Sector A, where no terracing or archaeological structures are visible (Figure 7).
The reference soil group of all three terrace profiles Te-07.1, Te-07.2, and Te-07.4 is a Terric Anthrosol (Loamic, Escalic). The soil structure of all profiles is homogeneous in the upper 30–50 cm and consists of coarse and blocky subangular clods with moderate to strong cohesion. Roots are abundant in the upper 30–60 cm of the profiles.
Terrace profile Te-07.1 shows a Ap-AC-C profile sequence, starting with a compact, brownish-black Ap (0–30 cm) plow horizon on its surface (Figure 10A). The AC (30–40 cm) is a transitional horizon to the parent material, with a very diffuse lower boundary. C (>40 cm) consists of the parent material of the soil, which is a strongly weathered granodiorite. A radiocarbon-dated bulk sediment sample taken from the base of the Ap horizon (30 cm) revealed an age of ~850 BCE (910–800 BCE, 95%; Figure 6).
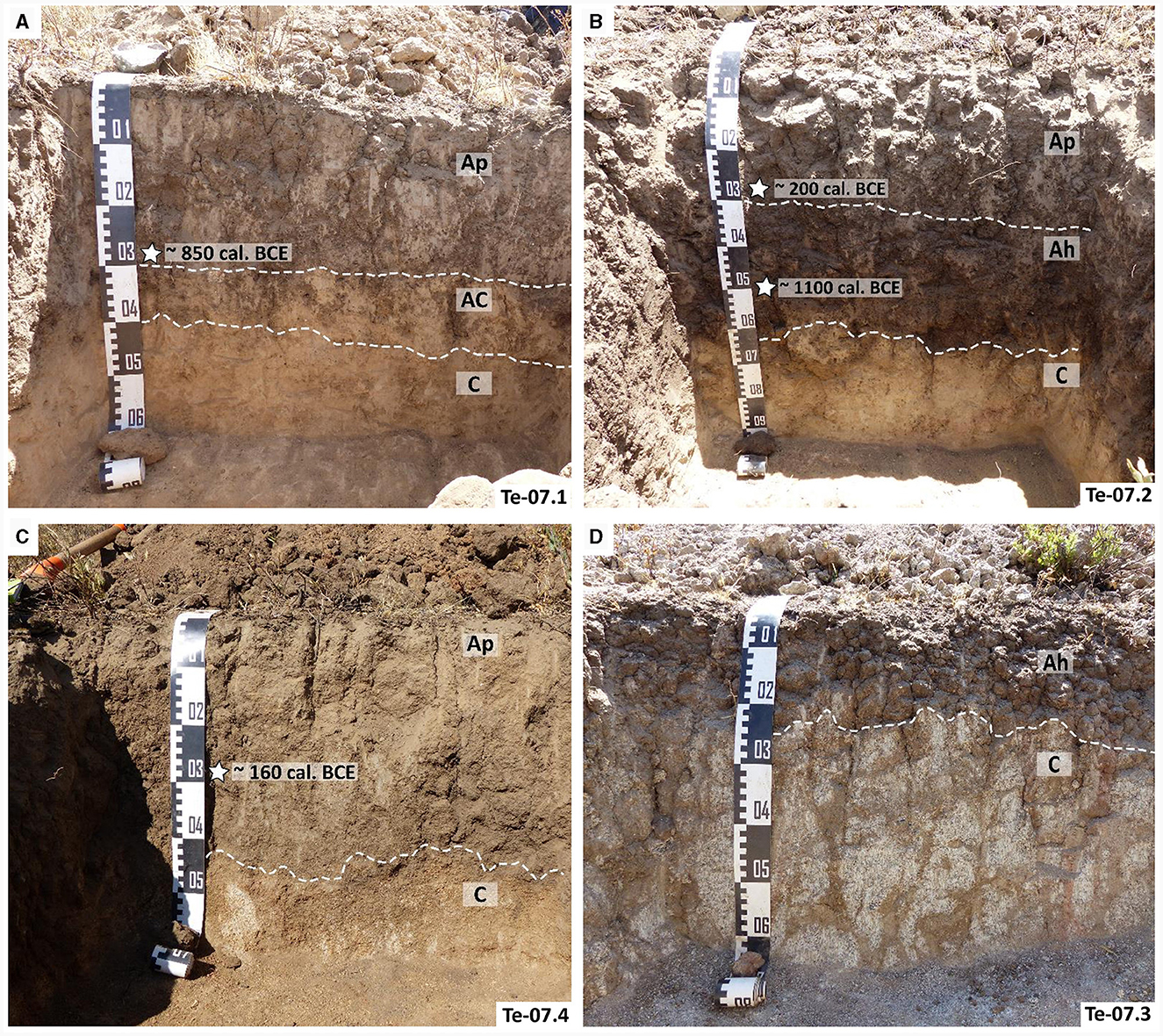
Figure 10. Photographs showing horizons and radiocarbon ages of terrace soils Te-07.1 (A), Te-07.2 (B), Te-07.4 (C), and soil control profile Te-07.3 (D).
Despite their proximity, terrace profile Te-07.2 shows significant morphological differences in profile thickness and horizontation. Characterized by a Ap-Ah-C profile sequence, it starts with a compact, brownish-black Ap (0–30 cm) plow horizon on its surface (Figure 10B). The underlying slightly compacted Ah (30–65 cm) is brownish-black in color and has a higher humus content and a more developed soil structure. It represents the former soil surface before terracing. C (>65 cm) consists of the parent material of the soil, which is a strongly weathered granodiorite. A radiocarbon-dated bulk sediment sample taken from the base of the Ap horizon (30 cm) revealed an age of ~200 BCE (370–100 BCE, 95%). A second bulk sample taken from the Ah horizon (50 cm) dates to ~1100 BCE (1220–1010 BCE, 95%; Figure 6).
Terrace profile Te-07.4 shows an Ap-C profile sequence, starting with a compact, brownish-black Ap (0–45 cm) plow horizon (Figure 10C). Its lower boundary is diffuse and transitions gradually into the C horizon (>25 cm) representing the parent material of the soil, a highly weathered granodiorite. A radiocarbon-dated bulk sediment sample taken from the Ap horizon (30 cm) revealed an age of ~160 BCE (350–60 BCE, 95%; Figure 6).
The reference soil group of soil control profile Te-07.3 is a Someric Phaeozem (Loamic). Two horizons (Ah-C) could be distinguished (Figure 10D). Ah (0–25 cm) is the topsoil horizon, rich in humus and brownish-black in color. Its lower boundary is diffuse and transitions gradually into the C horizon (>25 cm) representing the parent material of the soil, a granodiorite in a highly to moderately weathered state.
The four profiles were composed of a similar sandy/silt loam soil material with slightly varying contents of sand (mean = 56.3%, σ = 10.2%, n = 17), silt (mean = 37.9%, σ = 8.3%, n = 17), and clay (mean = 5.8%, σ = 2.4%, n = 17; Figure 11). Soil pH generally increases with depth, ranging from 5.8 to 7, being slightly acidic to neutral. Organic matter is largely constant within each profile, averaging 8% (σ = 2.6%, n = 17). The C/N ratios vary between 8.7 and 13.5 (mean = 10.6, σ = 1.2, n = 17) and gradually decrease toward the parent material. The highest values were reached in the topsoil horizons of terrace profiles Te-07.1 and Te-07.2.
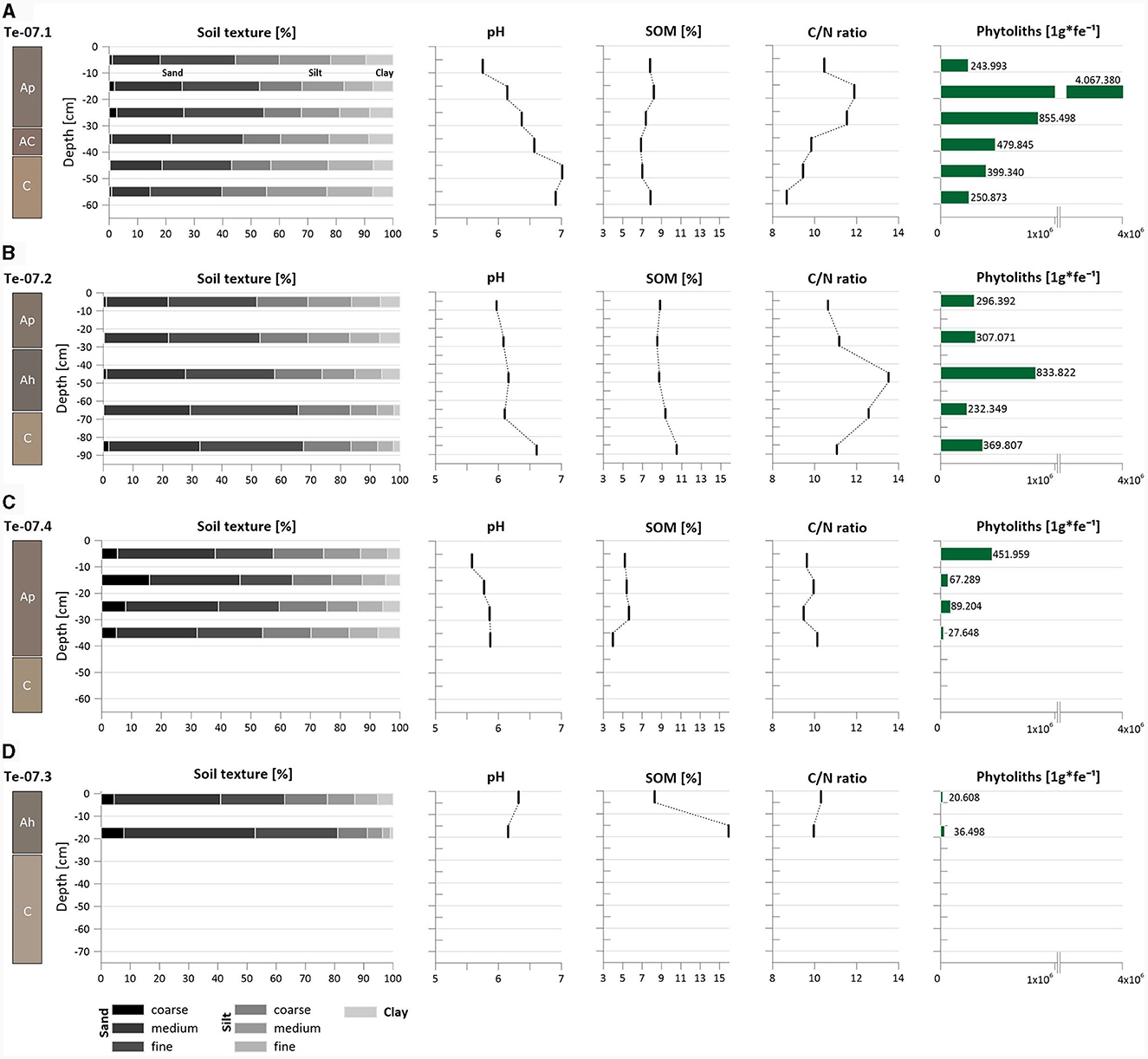
Figure 11. Laboratory results for the terrace soil profiles Te-07.1 (A), Te-07.2 (B), Te-07.4 (C), and soil control profile Te-07.3 (D): grain size, pedo-chemical analytical data, and total phytolith amounts.
Phytolith concentrations vary considerably within each profile, with the highest concentrations of up to 4,067,380 phytoliths per 1 g of sediment in terrace profile Te-07.1 and the lowest amounts of only 20,608 phytoliths per 1 g of sediment in soil control profile Te-07.3. In general, the phytolith concentrations of the terrace profiles Te-07.1, Te-07.2, and Te-07.4 decrease downslope and with increasing distance to the settlement structures of Cutamalla (Figure 11). In terms of phytolith morphotypes, Elongate SUM (mean = 34.7%, σ = 12.4%, n = 17) are the most abundant morphotypes, followed by Pooideae short cell Rondels including Zea mays diagnostic Rondels elongated/wavy top (mean = 14.4%, σ = 7.8%, n = 17), which are slightly decreasing with depth (Figure 12). Another diagnostic morphotype of Zea mays, Rondel tall (mean = 1.0%, σ = 1.2%, n = 17), shows a prominent signal in terrace profiles Te-07.2 and Te-07.4. Other short cells of Poaceae, such as Bilobate (mean = 0.6%, σ = 1.0%, n = 17), or Saddle (mean = 0.6%, σ = 1.5%, n = 17), occur frequently. Weathered morphotypes are very common (mean = 34.4%, σ = 14.7%, n = 17) and tend to increase with depth.
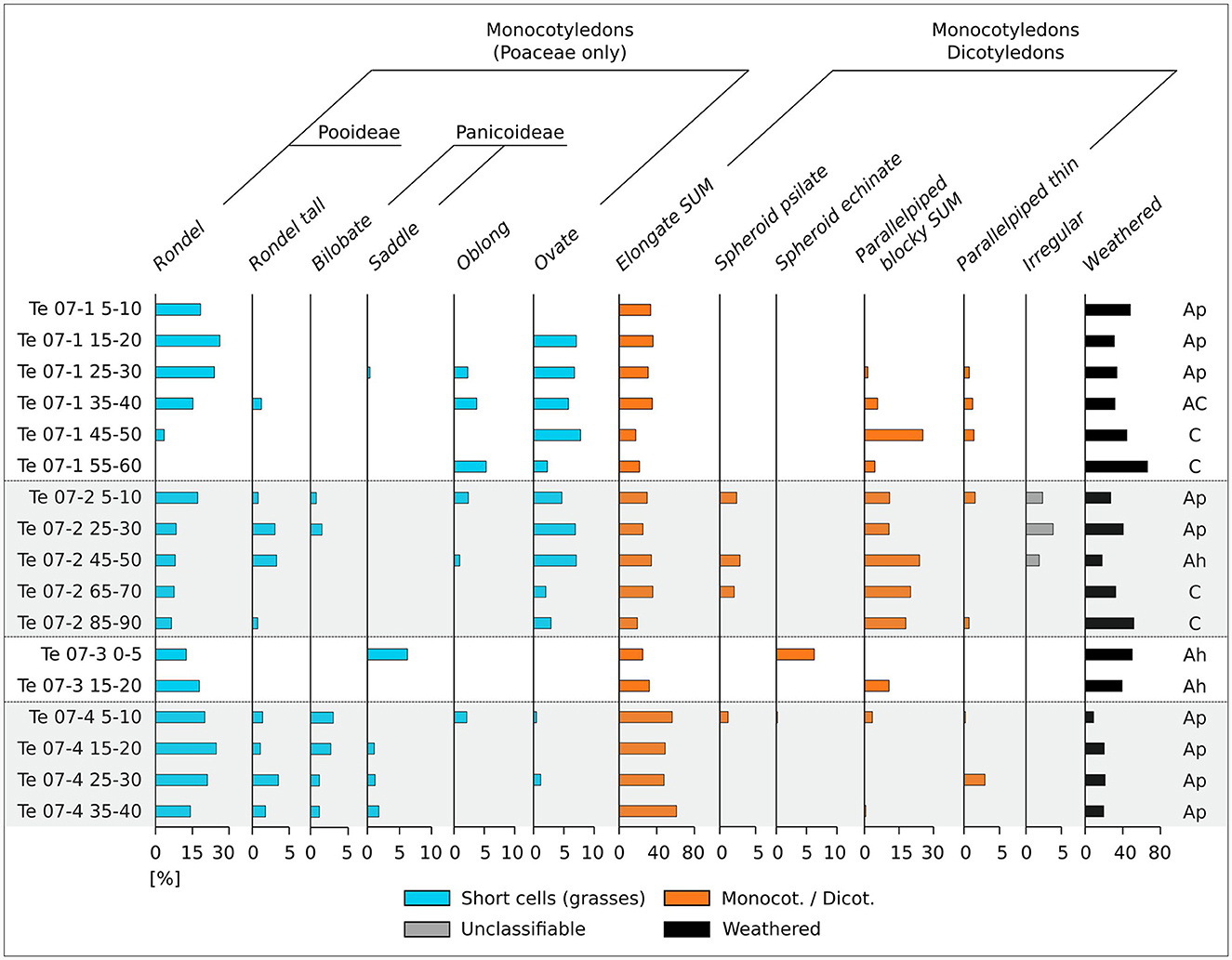
Figure 12. Distribution of most abundant phytolith morphotypes within the studied soil profiles in Cutamalla.
5.5 Agricultural production, food supply capacity, and labor force
The maize production from the Te-07 terrace system has a feeding capacity of between 83 and 199 people per year (mean = 141 people). When all three terrace systems are combined, this capacity increases almost 7-fold, to between 574 and 1,378 people per year (mean = 975 people; Table 1). The supply capacity of potato production is generally higher: average potato production can support 1,707 people per year (min = 465 people, max = 2,950 people). Assuming mixed maize and potato cultivation in systems Te-07, Te-09, and Te-10, the food supply capacity is 1,341 people, taking into account the average yields of both crops. About 110 people were needed to cultivate the total of about 220 ha over the entire growing season.
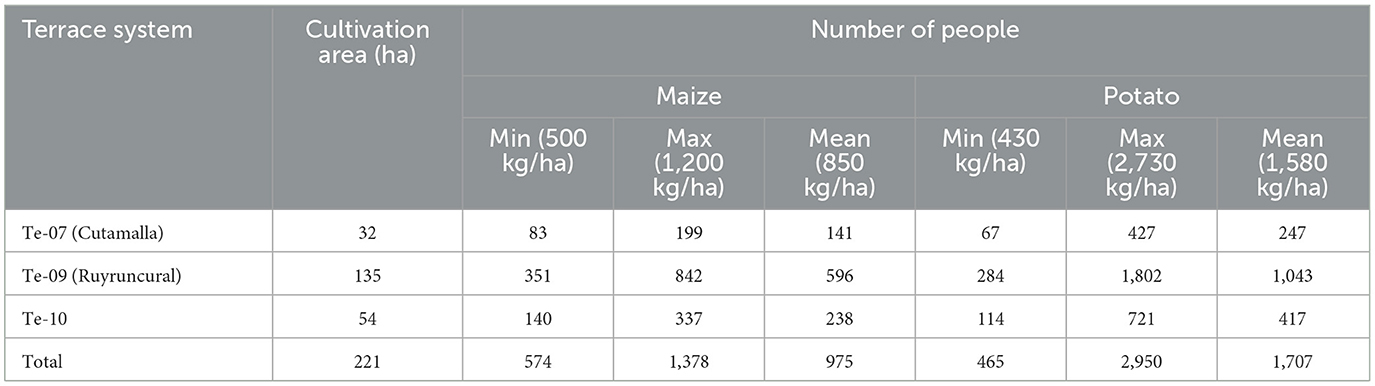
Table 1. Total number of people that can be fed annually from the Cutamalla terrace systems, based on estimated minimum and maximum maize and potato yields.
6 Discussion
Exploring the complex picture of ancient Andean agricultural practices and land use dynamics is only possible through an integrative approach that combines different methods from archaeological and geoscientific disciplines. In this paper, we have used such an integrative approach to study the Cutamalla agricultural terrace-settlement system, producing several dense datasets on the chronology, extent, use, and socio-economic organization of this key site in the southern Peruvian Andes. Our results provide evidence for intensive terrace cultivation and sophisticated agricultural strategies at Cutamalla. Our work also shows that the terraced agricultural system Te-07 at Cutamalla was clearly related to its settlement, as evidenced by their close proximity and corresponding radiocarbon data and archaeological finds. We have defined such complexes as agricultural terrace-settlement systems because they must be understood as a single analytical unit. Accordingly, settlement and terrace data complement each other in terms of chronology, social organization, and economic use (e.g., cultivated crops, food storage, and processing by people living and working in these complexes). The Cutamalla agricultural terrace-settlement system represents a large complex of terraced fields with a total area of 221 ha (including Te-09 and Te-10). The system was also associated with other architectural features such as corrals, boundary walls, and paths. Our study, which applies such an integrative approach to this large agricultural terrace-settlement system, is unique in its design and implementation and will be groundbreaking for future research on farming terraces. The mutual complementation of data from agricultural terraces and settlements is crucial in this approach to achieve a comprehensive understanding of these complexes. This is only possible by combining the results of archaeological and geomorphological surveys, archaeological excavations, detailed mapping, soil testing, radiocarbon dating, and other methods outlined here.
While mapping of agricultural terraces proves valuable for illustrating the extent of anthropogenic impact during the prehispanic period (Reindel, 2016), the use of GIS-based approaches for analyzing prehispanic terrace systems in the Peruvian Andes has been somewhat limited, with the analysis of high-resolution terrain models notably absent from existing studies. Through our integrated mapping approach, utilizing satellite imagery and high-resolution DEMs derived from drone flights, we efficiently and cost-effectively mapped abandoned terrace systems on the basis of terrace walls over larger areas. Furthermore, we quantified the total cultivated area and terrace characteristics, including average terrace width, average terrace wall height, and average terrace slope. Despite the success of our approach, methodological challenges persist, particularly in identifying terraces due to factors such as vegetation cover or extremely rough terrain. As a result, the final validation of mapping results will require pedestrian surveys. In future endeavors, the high-resolution DEMs will be also used to estimate the total amount of soil and debris trapped on terraced slopes, as well as anthropogenic redeposition (Paliaga et al., 2022). Machine learning approaches will be explored to facilitate and accelerate future mapping using satellite imagery and DEMs (Ding et al., 2021; Winzeler et al., 2023).
In terms of soil reference groups, Anthrosols have formed in the terraces of the Cutamalla System, a soil group that has been heavily modified by human activities such as harvesting, irrigation, fertilization, or plowing (Zech et al., 2014; IUSS Working Group WRB, 2015). In Cutamalla, the terrace soils are characterized by relatively thick and homogeneous plowing horizons overlying the parent material, resulting from prolonged agricultural use, and are therefore different from the naturally formed soils of the area. Terrace profile Te-07.2 is the only one where a buried Ah horizon is preserved, probably representing the remnant of an old soil surface prior to terracing. In general, the system provides a favorable environment for agriculture. Soil pH and nutrient availability allow the development of micro-organisms and roots, the germination of seeds, and the cultivation of common Andean products. The silty-sandy to loamy nature of the soil provides moderate to good conditions for soil moisture retention, while the C/N ratios generally indicate fertile soils with high-quality organic matter (Zavaleta García, 1992; Blume et al., 2010). The high level of organic matter in the plow horizons also suggests that maintaining a high and constant level of organic matter in the topsoil of the terraces was one of the central measures of prehispanic terrace management. In general, the Cutamalla terraces show little sign of degradation, which could be due to a short duration of use (see below), long fallow periods, or grazing of animals on the crop stubble (Nanavati et al., 2016). In summary, the analysis of parameters such as grain size, pH, organic matter, and C/N ratio in ancient agricultural soils provides valuable insights into various aspects of the soil, its formation, and ancient agricultural practices. This study also reveals details of soil fertility and quality, which are influenced by factors such as water retention, soil acidity, the amount and state of decomposition of organic matter, and the availability of nutrient to the plants. When integrated with other archaeological and environmental data, these parameters enhance our site-specific understanding of soil conditions in relation to specific land-use practices and paleoenvironmental conditions. In future studies, it would be beneficial to explore additional soil parameters, including but not limited to the total phosphorus content, plant-available phosphorus content, bulk density, and concentrations of plant-available cations.
Phytolith concentrations in terrace profiles Te-07.1, Te-07.2, and Te-07.4 are indicative of agricultural use as they are significantly higher than in soil control profile Te-07.3 (Meister et al., 2017). The significant increase in phytolith concentrations from profile Te-07.4 to Te-07.1 indicates that the terraces near the settlement were used significantly longer or more intensely than the more distant terraces. This also means that the terrace agricultural system Te-07 has probably been systematically extended downhill over the course of its use. The distribution of phytolith concentrations within a terrace profile also gives an indication of the degree of mixing of the soil material due to agricultural use, as the highest phytolith concentrations in natural soils are usually found in the upper humic horizon and decrease with depth (Qader et al., 2023). The significant frequency of the diagnostic morphotypes Rondel elongated/wavy top and Rondel tall of Zea mays in the terrace soils indicates the cultivation of maize as a main crop (Bozarth, 1998; Pearsall et al., 2003; Piperno, 2006; Logan et al., 2012; Handley et al., 2023), although Cross-shaped phytoliths, typically produced in the leaves of maize plants (Piperno, 2006; Logan et al., 2012), occur in very limited numbers. To verify the phytolith data, forthcoming studies should employ a combination of microfossils, encompassing phytoliths and starch grains, covering a wide range of economic taxa (Korstanje and Babot, 2007; Korstanje and Cuenya, 2010). In addition to maize, starch analysis allows the identification of other important Andean crops, such as potato, sweet potato, and bean (Gismondi et al., 2019).
Radiocarbon dating helps place ancient agricultural terraces within a broader cultural and historical context. By dating the organic materials or bulk sediments associated with the terrace fills and buried soils, it is possible to link the terrace construction or use to specific cultural periods (Brown et al., 2021). This information aids in understanding the societal and cultural significance of these agricultural practices during a certain historic period. The two terrace dates of profiles Te-07.2 and Te-07.4 are very similar, with bimodal distributions. The largest part of their distributions is contemporary with residential occupations at Cutamalla, so we consider it likely that residents built and used these terraces during the first half of Cutamalla's residential occupation (the blue KDE in Figure 6). Both of these samples were taken from plow horizons, suggesting that the organic carbon in the soil samples probably originated from the plant mass of the crops cultivated on the terraces. The older age from the plow horizon of profile Te-07.1 could be from mixing deeper soil material during terrace construction or use, as plowing leads to a mixing of soil components and the movement of sediments from deeper parts into the upper profile areas (Brown et al., 2021). The second and older age from the Te-07.2 profile is from the humic horizon of the buried soil underlying the plow horizon. This is a pre-terracing surface, and therefore represents an older phase of soil formation. We cannot rule out that the earliest terraces were constructed before the main settlement phase; however, this is unlikely due to the absence of evidence supporting such an early occupation at Cutamalla. The potential extension of the terrace system Te-07 downhill during its utilization, as suggested by the phytolith data, remains uncertain given the available ages. Additionally, considering the current data, the possibility that the terraces were cultivated in later periods cannot be ruled out. However, if they were used for an extended duration, the thin Ap horizons would likely have undergone thorough mixing, resulting in somewhat younger ages. Ongoing processing of additional radiocarbon samples from terrace systems Te-09 and Te-10 may contribute to refining the terrace chronology, although the similar terrace architecture observed in all three agricultural systems implies concurrent use with the Cutamalla settlement occupation. To establish a more precise chronological framework for terrace construction, use, and abandonment in Cutamalla, further dating will be essential. Acabado (2009), for example, utilized multiple radiocarbon dates within one terrace and Bayesian modeling techniques to unravel the chronological history of terraces. Ideally, combining radiocarbon dating with other methods, such as optically stimulated luminescence (OSL) dating from terrace fills and sediments beneath terrace walls, would provide supplementary information and facilitate the verification of stratigraphic consistency (Beckers et al., 2013; Kinnaird et al., 2017).
Compared to later Inka terrace agriculture in the Andean region (Kendall and Rodríguez, 2009; Castro et al., 2019; Ortloff, 2019), we want to emphasize that the terrace-settlement system at Cutamalla was less standardized in terms of architecture, layout, and distribution, which seems logical due to the different political, economic, and socio-cultural context. It is interesting to note that none of the farming terraces at Cutamalla are still in use today. There is also no evidence of their reuse after Cutamalla was abandoned. When we asked local farmers about this, they said that there was simply not enough water to farm, meaning modern conditions are probably drier than when the terraces were used. This is consistent with paleoenvironmental and paleoclimatic reconstructions of the region, which suggest a dry period that began around 1 CE, shortly after occupation ended at Cutamalla (Mächtle and Eitel, 2013; Fehren-Schmitz et al., 2014; Schittek et al., 2015; Mächtle et al., 2017). However, we are only beginning to understand the relationships between climate and environmental change, agricultural practices, and settlement activities at local and regional scales (Branch et al., 2023). More interdisciplinary research is needed to obtain a reliable picture of climate-land use-settlement dynamics through time and space.
Our calculations of food supply capacity and labor in the Cutamalla agricultural terrace-settlement system show that Te-07, Te-09, and Te-10 produced yields sufficient to feed ~1,341 people, assuming mixed maize and potato cultivation, while requiring ~110 people to work in these systems for the entire growing season. These figures are preliminary estimates that can be refined by future research. Exploring ethnographic studies of agriculture in the greater Cutamalla area would be valuable for comparing crop cultivation practices, especially for maize and potatoes. Unfortunately, such studies are currently unavailable. We plan to generate ethnographic data and develop sustainable agricultural practices with local farmers (comunidades campesinas), with a stronger focus on community archaeology to provide new insights and perspectives in the region. Both refining our calculations of food supply capacity and labor force and more fully integrating community archaeology are promising avenues. As preliminary as they are, our food supply and labor figures suggest that the agricultural terraces around Cutamalla emerged in the context of a larger socio-economic organization, since they fed significantly more people than the people who worked on them. At present, we do not have an estimate of the population of the Cutamalla settlement, but the extent of the terraces in relation to the settlement itself implies the storage of a significant amount of agricultural production and/or the export of food to other settlements and ecological zones. The latter scenario fits well with the model of economic directness (Mader et al., 2023a) that describes the socio-economic organization of the Paracas culture, especially in its final stages, and that also takes into account the archaeological data from Cutamalla. Key features of this model include population growth and the flow of important goods, such as obsidian artifacts and camelid products, from the highlands to the Pacific coastal desert. Crops grown on the farming terraces of Cutamalla and other agricultural terrace-settlement systems of the same period likely corresponded to another flow of goods directed from the highlands to the coast, articulated by camelid caravans (Mader et al., 2022).
According to these commodity flows, the coast was not only a major consumption area for a variety of goods, but also a region where a group of more powerful and privileged people resided, as evidenced by higher percentages of fine-ware ceramics, high-quality textiles, richer burial goods, and examples of monumental architecture (Mader, 2019a; Tantaleán, 2021; Mader et al., 2023a). The highlands, in contrast, did not have these characteristics during the Late Paracas and Initial Nasca periods, as can also be observed in the Cutamalla data. In general, these periods were characterized by a common religious-political ideology (Tantaleán, 2021) and increased violence and conflict in the region, as evidenced by defensive architecture such as walls and ditches; a variety of weapons such as projectile points, clubs, and sling stones; and signs of trauma on human skeletal remains such as fractures, mutilations, and cut marks (Tomasto, 2009; Soßna, 2015; Mader, 2019a). The reliance on important highland resources across the western Andean flank was a basis for dependencies between people and different groups. In order to control access to resources, people had to cooperate, and the larger socio-political-economic context suggests that this included the exploitation of some segments of society, particularly in the highlands, through compulsory and forced forms of collective labor such as mit'a (D'Altroy and Earle, 1985). Conversely, it seems likely that voluntary forms of communal labor such as ayni and mink'a (Mayer, 2002; Erickson, 2019; Sammells, 2019) did not play a major role. In these respects, different levels of dependency, political-ideological coercion, and the use of violence were likely key elements in mobilizing the labor force that worked on the agricultural terraces around Cutamalla and produced, processed, and distributed highland resources.
7 Conclusions and future directions
Our study of the Cutamalla agricultural terrace-settlement system provides comprehensive and unique insights into ancient Andean agricultural strategies. Importantly, our analyses show that the occupation of the Cutamalla settlement and the use of its surrounding agricultural terraces coincided: they were in use for a relatively short period of about 200 years or seven generations (~250–40 BCE) during the Formative Late Paracas and transitional Initial Nasca periods, and there is no evidence for subsequent reuse of this terrace-settlement system. We also document the wide extent of agricultural terraces around Cutamalla and demonstrate that maize was most likely a major crop grown there. Moreover, we contextualize these findings within a larger framework of socio-economic organization, dependency and labor relations, and human-environment interactions. The Late Paracas and Initial Nasca periods were not only very dynamic, with significant population growth, violence, and likely forced collective labor, but also had more humid environmental conditions that allowed for these agricultural practices on a large scale in the region.
Finally, Cutamalla serves as a pioneering case study of an ancient agricultural terrace-settlement system, but it is only one of many such agricultural systems in the region. Our team currently analyzes data from 15 other terrace farming systems from different prehispanic periods, using further methods such as starch analysis, phosphorus analysis, additional numerical dating techniques (OSL), machine learning approaches, and community archaeology to incorporate ethnographic data and collectively develop sustainable agricultural practices for the region. Thereby, our integrative approach will be expanded in the future. These studies of terrace agriculture across space and time may help to refine some of the conclusions for Cutamalla and the larger region, and will certainly help to explore changes and continuities in land use in prehispanic South America.
Data availability statement
The original contributions presented in the study are included in the article/Supplementary material, further inquiries can be directed to the corresponding author/s.
Ethics statement
Written informed consent was obtained from the individual(s) for the publication of any potentially identifiable images or data included in this article.
Author contributions
CM: Conceptualization, Data curation, Formal analysis, Funding acquisition, Investigation, Methodology, Project administration, Resources, Supervision, Validation, Visualization, Writing—original draft, Writing—review & editing. PG: Data curation, Formal analysis, Investigation, Methodology, Software, Visualization, Writing—original draft. MB: Data curation, Writing—review & editing. CB: Formal analysis, Visualization, Writing—review & editing. EH: Data curation, Investigation, Visualization, Writing—review & editing. JI: Data curation, Formal analysis, Investigation, Methodology, Project administration, Writing—review & editing. FL: Formal analysis, Validation, Writing—review & editing. ML: Data curation, Investigation, Software, Writing—review & editing. EM: Data curation, Formal analysis, Investigation, Methodology, Software, Visualization, Writing—original draft, Writing—review & editing. RO: Data curation, Investigation, Writing—review & editing. EF: Investigation, Writing—review & editing. PS: Data curation, Investigation, Visualization, Writing—review & editing. A-KW: Data curation, Investigation, Methodology, Visualization, Writing—review & editing. MR: Conceptualization, Data curation, Formal analysis, Funding acquisition, Investigation, Methodology, Project administration, Resources, Supervision, Writing—review & editing. JM: Conceptualization, Data curation, Formal analysis, Funding acquisition, Investigation, Methodology, Project administration, Resources, Supervision, Validation, Visualization, Writing—original draft, Writing—review & editing.
Funding
The author(s) declare financial support was received for the research, authorship, and/or publication of this article. This research was funded by the Groundcheck Program of the German Archaeological Institute (DAI) and the German Research Foundation (DFG) within the framework of the German Excellence Strategy—Cluster of Excellence Bonn Center for Dependency and Slavery Studies (BCDSS) EXC 2036/1-2020, project number: 390683433. Additional support was provided by an Argelander Grant (Christian Mader) from the University of Bonn and by a Research Award (Julia Meister) of the Frithjof Voss Foundation. This publication was supported by the Open Access Publication Fund of the University of Würzburg.
Acknowledgments
We are very grateful to the topic editors and organizers of the workshop “New theoretical and methodological approaches to changing land use in the South Central Andes during the last three millennia” (Max Planck Institute of Geoanthropology, Jena, Germany), where an earlier version of this paper was presented in November 2022. Many people helped us during the fieldwork in Peru, for which we would especially like to thank the Comunidad Campesina de Laramate, Pablo M. Segura, Oliver Huarcaya, Lener Huarcaya, and Edilshon Champe. We would also like to thank Santiago Ancapichún for calculating the air mixture at Cutamalla, Hanna M. Schubert for compiling literature, and Laura Grüner, Jana Scherg, and Tom Wolf for their help with the laboratory analyses. Finally, special thanks are due to Katharine Thomas for proofreading the manuscript, and to the reviewers whose comments greatly improved our article.
Conflict of interest
The authors declare that the research was conducted in the absence of any commercial or financial relationships that could be construed as a potential conflict of interest.
Publisher's note
All claims expressed in this article are solely those of the authors and do not necessarily represent those of their affiliated organizations, or those of the publisher, the editors and the reviewers. Any product that may be evaluated in this article, or claim that may be made by its manufacturer, is not guaranteed or endorsed by the publisher.
Supplementary material
The Supplementary Material for this article can be found online at: https://www.frontiersin.org/articles/10.3389/fearc.2024.1328315/full#supplementary-material
References
Acabado, S. (2009). A Bayesian approach to dating agricultural terraces: a case from the Philippines. Antiquity 83, 801–814. doi: 10.1017/S0003598X00099002
Ad-hoc-AG Boden ed (2005). Bodenkundliche Kartieranleitung. KA5 [Manual of Soil Mapping]. Stuttgart: Schweizerbart Science.
Aguirre-Morales, M. (2009). Excavaciones en los andenes de Andamarca, cuenca del río Negromayo, Lucanas, Ayacucho. Arqueol. Soc. 20, 223–267. doi: 10.15381/arqueolsoc.2009n20.e12687
Albert, R. M., Lavi, O., Estroff, L., Weiner, S., Tsatskin, A., Ronen, A., et al. (1999). Mode of occupation of Tabun Cave, Mt Carmel, Israel during the Mousterian period: a study of the sediments and phytoliths. J. Archaeol. Sci. 26, 1249–1260. doi: 10.1006/jasc.1999.0355
Ancapichún, S., Pawlyta, J., Rakowski, A. Z., and Sieczkowska, D. (2022). Influence of air parcels from Northern and Southern Hemispheres on radiocarbon-based Inca chronology. Radiocarbon 64, 1431–1446. doi: 10.1017/RDC.2022.87
Beckers, B., Schütt, B., Tsukamoto, S., and Frechen, M. (2013). Age determination of Petra's engineered landscape – optically stimulated luminescence (OSL) and radiocarbon ages of runoff terrace systems in the Eastern Highlands of Jordan. J. Archaeol. Sci. 40, 333–348. doi: 10.1016/j.jas.2012.06.041
Beresford-Jones, D., Mader, C., Lane, K., Cadwallader, L., Gräfingholt, B., Chauca, G., et al. (2023). Beyond Inca roads: archaeological mobilities from the high Andes to the Pacific in southern Peru. Antiquity 97, 194–212. doi: 10.15184/aqy.2022.168
Beresford-Jones, D., Pullen, A. G., Whaley, O. Q., Moat, J., Chauca, G., Cadwallader, L., et al. (2015). Re-evaluating the resource potential of lomas fog oasis environments for Preceramic hunter-gatherers under past ENSO modes on the south coast of Peru. Quat. Sci. Rev. 129, 196–215. doi: 10.1016/j.quascirev.2015.10.025
Blume, H.-P., Brümmer, G. W., Horn, R., Kandeler, E., Kögel-Knabner, I., Kretzschmar, R., et al. (2010). Scheffer/Schachtschabel: Lehrbuch der Bodenkunde. Berlin; Heidelberg: Springer. doi: 10.1007/978-3-662-49960-3
Bozarth, S. (1998). Maize (Zea mays) cob phytoliths from a central Kansas Great Bend Aspect archaeological site. Plains Anthropol. 43, 279–286. doi: 10.1080/2052546.1998.11931908
Branch, N., Ferreira, F., Lane, K., Wade, A., Walsh, D., Handley, J., et al. (2023). Adaptive capacity of farming communities to climate change in the Peruvian Andes: past, present and future (preliminary findings of the ACCESS project). Rev Glaciares Ecosistemas Montaña 8, 51–67. doi: 10.35424/rearam352017/25f
Bronk Ramsey, C. (2009a). Bayesian analysis of radiocarbon dates. Radiocarbon 51, 337–360. doi: 10.1017/S0033822200033865
Bronk Ramsey, C. (2009b). Dealing with outliers and offsets in radiocarbon dating. Radiocarbon 51, 1023–1045. doi: 10.1017/S0033822200034093
Bronk Ramsey, C. (2017). Methods for summarizing radiocarbon datasets. Radiocarbon 59, 1809–1833. doi: 10.1017/RDC.2017.108
Brown, A. G., Fallu, D., Walsh, K., Cucchiaro, S., Tarolli, P., Zhao, P., et al. (2021). Ending the Cinderella status of terraces and lynchets in Europe: the geomorphology of agricultural terraces and implications for ecosystem services and climate adaptation. Geomorphology 379:107579. doi: 10.1016/j.geomorph.2020.107579
Canziani, J. (2002). “Las lomas de Atiquipa: un caso de paisaje cultural en la costa sur del Perú,” in Paisajes culturales en los Andes, ed E. Mujica (Lima: UNESCO), 159–177.
Carmichael, P. H. (2019). Stages, periods, epochs, and phases in Paracas and Nasca chronology: another look at John Rowe's Ica valley master sequence. Ñawpa Pacha 39, 145–179. doi: 10.1080/00776297.2019.1623468
Castro, J., Vallejo, L. E., and Estrada, N. (2019). The optimal design of the retaining walls built by the Incas in their agricultural terraces. J. Cult. Herit. 36, 232–237. doi: 10.1016/j.culher.2018.09.013
Costin, C. L. (2015). “The cost of conquest: assessing the impact of Inka tribute demands on the Wanka of highland Peru,” in Surplus: The Politics of Production and the Strategies of Everyday Life, eds C. T. Morehart, and K. De Lucia (Boulder, CO: University Press of Colorado) ,45–72.
D'Altroy, T. N., and Earle, T. K. (1985). Staple finance, wealth finance, and storage in the Inka political economy. Curr. Anthropol. 26, 187–206. doi: 10.1086/203249
Dean, W. E. (1974). Determination of carbonate and organic matter in calcareous sediments and sedimentary rocks by loss on ignition; comparison with other methods. SEPM JSR 44, 242–248. doi: 10.1306/74D729D2-2B21-11D7-8648000102C1865D
Denevan, W. M. (2001). Cultivated Landscapes of Native Amazonia and the Andes. Oxford: Oxford University Press.
Dietrich, L., Meister, J., Dietrich, O., Notroff, J., Kiep, J., Heeb, J., et al. (2019). Cereal processing at Early Neolithic Göbekli Tepe, southeastern Turkey. PLoS ONE 14:e0215214. doi: 10.1371/journal.pone.0215214
Ding, H., Na, J., Jiang, S., Zhu, J., Liu, K., Fu, Y., et al. (2021). Evaluation of three different machine learning methods for object-based artificial terrace mapping—a case study of the Loess Plateau, China. Remote Sens. 13:1021. doi: 10.3390/rs13051021
Donkin, R. A. (1979). Agricultural Terracing in the Aboriginal New World. Tucson, AZ: University of Arizona Press.
Erickson, C. L. (2006). “Intensification, political economy, and the farming community,” in Agricultural Strategies, eds C. Stanish, and M. Joyce (Los Angeles, CA: Cotsen Institute of Archaeology Press), 334–363.
Erickson, C. L. (2019). “The domesticated landscapes of the Andes,” in The Andean World, eds K. S. Fine-Dare, and L. J. Seligmann (New York, NY: Routledge), 29–43.
FAOSTAT (2023). Minimum Daily Requirement Of Calories, 2021. Available online at: https://ourworldindata.org/grapher/minimum-requirement-calories (accessed September 28, 2023).
Fehren-Schmitz, L., Haak, W., Mächtle, B., Masch, F., Llamas, B., Tomasto, E., et al. (2014). Climate change underlies global demographic, genetic, and cultural transitions in pre-Columbian southern Peru. Proc. Natl. Acad. Sci. U. S. A. 111, 9443–9448. doi: 10.1073/pnas.1403466111
Ferrarese, F., Pappalardo, S. E., Cosner, A., Brugnaro, S., Alum, K., Dal Pozzo, A., et al. (2019). “Mapping agricultural terraces in Italy: methodologies applied in the MAPTER project,” in World Terraced Landscapes: History, Environment, Quality of Life, eds M. Varotto, L. Bonardi, and P. Tarolli (Cham: Springer), 179–194.
Friedli, E., and Theiler, P. W. (2014). “Documentation of remote archaeological sites: a comparison between long-range laser scanning and UAV photogrammetry,” in FIG Congress 2014 Engaging the Challenges – Enhancing the Relevance (Kuala Lumpur), 1–15.
Gismondi, A., D'Agostino, A., Canuti, L., Di Marco, G., Basoli, F., and Canini, A. (2019). Starch granules: a data collection of 40 food species. Plant Biosyst. 153, 273–279. doi: 10.1080/11263504.2018.1473523
Goodman-Elgar, M. (2008). Evaluating soil resilience in long-term cultivation: a study of pre-Columbian terraces from the Paca valley, Peru. J. Archaeol. Sci. 35, 3072–3086. doi: 10.1016/j.jas.2008.06.003
Gremillion, K. (2014). “Plant domestication and cultivation in archaeology,” in Encyclopedia of Global Archaeology, ed C. Smith (New York, NY: Springer), 5964–5973.
Guillet, D. (1987). Terracing and irrigation in the Peruvian highlands. Curr. Anthropol. 28, 409–430. doi: 10.1086/203546
Handley, J., Branch, N., Meddens, F. M., Simmonds, M., and Iriarte, J. (2023). Pre-Hispanic terrace agricultural practices and long-distance transfer of plant taxa in the southern-central Peruvian Andes revealed by phytolith and pollen analysis. Veget. Hist. Archaeobot. doi: 10.1007/s00334-023-00946-w
Hastorf, C. A. (1993). Agriculture and the Onset of Political Inequality Before the Inka. Cambridge: Cambridge University Press.
Hogg, A. G., Heaton, T. J., Hua, Q., Palmer, J. G., Turney, C. S., Southon, J., et al. (2020). SHCal20 Southern Hemisphere calibration, 0–55,000 years cal BP. Radiocarbon 62, 759–778. doi: 10.1017/RDC.2020.59
Isla, J., and Reindel, M. (2017). “Palpa and Lucanas: cultural development under changing climatic conditions on the western slope of the Andes in southern Peru,” in The Andes: Geography, Diversity, and Sociocultural Impacts, ed C. D. Allen (New York, NY: Nova), 53–119.
ISO 11465 (1993). Soil Quality - Determination of Dry Matter and Water Content on a Mass Basis - Gravimetric Method. Geneva.
IUSS Working Group WRB (2015). World Reference Base for Soil Resources 2014, update 2015. International Soil Classification System for Naming Soils and Creating Legends for Soil Maps. World Soil Resources Reports No. 106. Rome: FAO.
Kemp, R., Branch, N., Silva, B., Meddens, F., Williams, A., Kendall, A., et al. (2006). Pedosedimentary, cultural and environmental significance of paleosols within pre-hispanic agricultural terraces in the southern Peruvian Andes. Quat. Int. 158, 13–22. doi: 10.1016/j.quaint.2006.05.013
Kendall, A. (2004). “Restauración de canales y andenes agrícolas prehispánicos en los Andes usando tecnología tradicional y apropiada,” in Conservación y abandono de andenes, eds C. A. Llerena, M. Inbar, and M. A. Benavides (Lima: Universidad Nacional Agraria La Molina), 98–106.
Kendall, A. (2005). Applied archaeology: revitalizing indigenous agricultural technology within an Andean community. Public Archaeol. 4, 205–221. doi: 10.1179/146551805793156185
Kendall, A. (2013). “Applied archaeology in the Andes: the contribution of pre-Hispanic agricultural terracing to environmental and rural development strategies,” in Humans and the Environment: New Archaeological Perspectives for the Twenty-First Century, eds M. I. J. Davies, and F. N. M'Mbogori (Oxford: Oxford University Press), 153–168.
Kendall, A., and Rodríguez, A. (2009). Desarrollo y perspectivas de los sistemas de andenería de los Andes centrales del Perú. Lima: Institut Français d'Études Andines.
Kinnaird, T., Bolòs, J., Turner, A., and Turner, S. (2017). Optically-stimulated luminescence profiling and dating of historic agricultural terraces in Catalonia (Spain). J. Archaeol. Sci. 78, 66–77. doi: 10.1016/j.jas.2016.11.003
Kirchner, A., Herrmann, N., Matras, P., Müller, I., Meister, J., and Schattner, T. G. (2022). A pedo-geomorphological view on land use and its potential in the surroundings of the ancient Hispano-Roman city Munigua (Seville, SW Spain). E&G Q. Sci. J. 71, 123–143. doi: 10.5194/egqsj-71-123-2022
Korstanje, M. A., and Babot, M. P. (2007). “Microfossils characterization from south Andean economic plants,” in Plants, People and Places Recent Studies in Phytolithic Analysis, eds M. Madella and D. Zurro (Oxford: Oxbow), 41–72.
Korstanje, M. A., and Cuenya, P. (2010). Ancient agriculture and domestic activities: a contextual approach studying silica phytoliths and other microfossils in soils. Environ. Archaeol. 15, 43–63. doi: 10.1179/146141010X12640787648739
Lane, K. (2014). “Water technology in the Andes,” in Encyclopaedia of the History of Science, Technology, and Medicine in Non-Western Cultures, ed H. Selin (Dordrecht: Springer), 1–24.
Lema, V. S. (2014a). Boceto para un esquema: domesticación y agricultura temprana en el Noroeste argentino. Rev. Española Antropol. Am. 44, 465–494. doi: 10.5209/rev_REAA.2014.v44.n2.50726
Lema, V. S. (2014b). Hacia una cartografía de la crianza: domesticidad y domesticación en comunidades andinas. Espaço Ameríndio 8, 59–82. doi: 10.22456/1982-6524.44644
Lema, V. S. (2015). Non-domesticated cultivation in the Andes: plant management and nurturing in the Argentine northwest. Veget. Hist. Archaeobot. 24, 143–150. doi: 10.1007/s00334-014-0494-7
Leon, E. (2014). “Andes: origins and development of agriculture,” in Encyclopedia of Global Archaeology, ed C. Smith (New York, NY: Springer), 230–234.
Llerena, C. A., Inbar, M., and Benavides, M. A. eds. (2004). Conservación y abandono de andenes. Lima: Universidad Nacional Agraria La Molina.
Logan, A. L., Hastorf, C. A., and Pearsall, D. M. (2012). “Let's drink together”: early ceremonial use of maize in the Titicaca Basin. Lat. Am. Antiq. 23, 235–258. doi: 10.7183/1045-6635.23.3.235
Londoño, A. C., Williams, P. R., and Hart, M. L. (2017). A change in landscape: lessons learned from abandonment of ancient Wari agricultural terraces in southern Peru. J. Environ. Manage. 202, 532–542. doi: 10.1016/j.jenvman.2017.01.012
Mächtle, B., and Eitel, B. (2013). Fragile landscapes, fragile civilizations: how climate determined societies in the pre-Columbian south Peruvian Andes. Catena 103, 62–73. doi: 10.1016/j.catena.2012.01.012
Mächtle, B., Eitel, B., Schukraft, G., and Ross, K. (2009). “Built on sand: climatic oscillation and water harvesting during the Late Intermediate period,” in New Technologies for Archaeology: Multidisciplinary Investigations in Palpa and Nasca, Peru, eds M. Reindel, and G. A. Wagner (Berlin: Springer), 39–46.
Mächtle, B., Schittek, K., Reindel, M., and Eitel, B. (2017). Cambios paleoclimáticos y su influencia sobre el desarrollo cultural en el sur del área central andina. Rev. Arqueol. Am. 35, 33–50.
Mader, C. (2013). Zur Siedlungsgeschichte des Formativums (1700 bis 200 vor Christus) im südlichen Hochland von Peru: eine Bestandsaufnahme (master's thesis). University of Bonn, Bonn, Germany.
Mader, C. (2019a). Sea Shells in the Mountains and Llamas on the Coast: The Economy of the Paracas Culture (800 to 200 BC) in Southern Peru. Wiesbaden: Harrassowitz.
Mader, C. (2019b). “The economic organisation of the Paracas culture (800–200 BC) in southern Peru,” in Excavated Worlds: 40 Years of Archaeological Research on Four Continents (Bonn: German Archaeological Institute). 64–73.
Mader, C., Hölzl, S., Heck, K., Reindel, M., and Isla, J. (2018). The llama's share: highland origins of camelids during the Late Paracas period (370 to 200 BCE) in south Peru demonstrated by strontium isotope analysis. J. Archaeol. Sci. Rep. 20, 257–270. doi: 10.1016/j.jasrep.2018.04.032
Mader, C., Reindel, M., and Isla, J. (2022). “Camelids as cargo animals by the Paracas culture (800–200 BC) in the Palpa valleys of southern Peru,” in Caravans in Socio-Cultural Perspective: Past and Present, eds P. B. Clarkson, and C. M. Santoro (London: Routledge), 174–192.
Mader, C., Reindel, M., and Isla, J. (2023a). Economic directness in the western Andes: a new model of socioeconomic organization for the Paracas culture in the first millennium BC. Lat. Am. Antiq. 34, 385–403. doi: 10.1017/laq.2022.40
Mader, C., Reindel, M., Isla, J., Behl, M., Meister, J., and Hölzl, S. (2023b). In the land of the apu: Cerro Llamocca as a sacred mountain and central place in the pre-Columbian Andes of southern Peru. J. Archaeol. Sci. Rep. 49:104045. doi: 10.1016/j.jasrep.2023.104045
Mayer, E. (2002). The Articulated Peasant: Household Economies in the Andes. Boulder, CO: Westview Press.
Meister, J., Krause, J., Müller-Neuhof, B., Portillo, M., Reimann, T., and Schütt, B. (2017). Desert agricultural systems at EBA Jawa (Jordan): integrating archaeological and paleoenvironmental records. Quat. Int. 434, 33–50. doi: 10.1016/j.quaint.2015.12.086
Meister, J., Rettig, R., and Schütt, B. (2018). Ancient runoff agriculture at Early Bronze Age Jawa (Jordan): water availability, efficiency and food supply capacity. J. Archaeol. Sci. Rep. 22, 359–371. doi: 10.1016/j.jasrep.2016.06.033
Meyer-Heintze, S., Sprafke, T., Krech, M., Beigel, R., Nadler, M., Kriens, B., et al. (2020). Pedosedimentary and geoarcheological archives from clay-dominated sinkhole infillings in Middle Franconia, Germany. Catena 195:104893. doi: 10.1016/j.catena.2020.104893
Nanavati, W. P., French, C., Lane, K., Oros, O. H., and Beresford-Jones, D. (2016). Testing soil fertility of prehispanic terraces at Viejo Sangayaico in the upper Ica catchment of south-central highland Peru. Catena 142, 139–152. doi: 10.1016/j.catena.2016.03.007
Neumann, K., Strömberg, C. A. E., Ball, T., Albert, R. M., Vrydaghs, L., and Cummings, L. S. (2019). International code for phytolith nomenclature (ICPN) 2.0. Ann. Bot. 124, 189–199. doi: 10.1093/aob/mcz064
Ortloff, C. R. (2019). Tipon: insight into Inka hydraulic engineering practice. Lat. Am. Antiq. 30, 724–740. doi: 10.1017/laq.2019.70
Osborn, J., Hundman, B., Weinberg, C., and Espino Huaman, R. (2023). Reassessing the chronology of Topará emergence and Paracas decline on the Peruvian south coast: a Bayesian approach. Radiocarbon. 65, 930–952. doi: 10.1017/RDC.2023.67
Paliaga, G., Luino, F., Turconi, L., Profeta, M., Vojinovic, Z., Cucchiaro, S., et al. (2022). Terraced landscapes as NBSs for geo-hydrological hazard mitigation: towards a methodology for debris and soil volume estimations through a LiDAR survey. Remote Sens. 14:3586. doi: 10.3390/rs14153586
Pearsall, D. M. (2008). “Plant domestication and the shift to agriculture in the Andes,” in The Handbook of South American Archaeology, eds H. Silverman, and W. H. Isbell (New York, NY: Springer), 105–120.
Pearsall, D. M., Chandler-Ezell, K., and Chandler-Ezell, A. (2003). Identifying maize in neotropical sediments and soils using cob phytoliths. J. Archaeol. Sci. 30, 611–627. doi: 10.1016/S0305-4403(02)00237-6
Piperno, D. R. (2006). Phytoliths: A Comprehensive Guide for Archaeologists and Paleoecologists. Lanham, MD: AltaMira Press.
Piperno, D. R. (2011). The origins of plant cultivation and domestication in the New World tropics: patterns, process, and new developments. Curr. Anthropol. 52, 453–470. doi: 10.1086/659998
Piperno, D. R., and Stothert, K. E. (2003). Phytolith evidence for early Holocene Cucurbita domestication in southwest Ecuador. Science 299, 1054–1057. doi: 10.1126/science.1080365
Pulgar Vidal, J. (1981). Geografía del Perú: las ocho regiones naturales del Perú. Lima: Editorial Universo.
Qader, W., Mir, S. H., Meister, J., Dar, R. A., Madella, M., and Rashid, I. (2023). Sedimentological perspective on phytolith analysis in palaeoecological reconstruction. Earth Sci. Rev. 244:104549. doi: 10.1016/j.earscirev.2023.104549
Reimer, P. J., Austin, W. E. N., Bard, E., Bayliss, A., Blackwell, P. G., Ramsey, C. B., et al. (2020). The IntCal20 Northern Hemisphere radiocarbon age calibration curve (0–55 cal kBP). Radiocarbon 62, 725–757. doi: 10.1017/RDC.2020.41
Reindel, M. (2009). “Life at the edge of the desert: archaeological reconstruction of the settlement history in the valleys of Palpa, Peru,” in New Technologies for Archaeology: Multidisciplinary Investigations in Palpa and Nasca, Peru, eds M. Reindel, and G. A. Wagner (Berlin: Springer), 439–461.
Reindel, M. (2010). Archäologische Forschungen der Jahre 2007 und 2008 im Anden-Transekt, Süd-Peru. Zeitschrift für Archäologie Außereuropäischer Kulturen 3, 207–224. doi: 10.34780/en7a-l6av
Reindel, M. (2016). Palpa, Peru: Neue Forschungen zur Paracas-Kultur (800–200 v. Chr.) im Hochland Süd-Perus. e-Forschungsberichte des Deutschen Archäologischen Instituts 2, 81–92.
Reindel, M., and Isla, J. (2017). Nuevo patrón arquitectónico Paracas en Lucanas, sierra sur del Perú. Bol. Arqueol. PUCP 22, 227–254. doi: 10.18800/boletindearqueologiapucp.201701.009
Reindel, M., and Isla, J. (2018). De Paracas a Nasca: nuevas evidencias desde la vertiente occidental de la sierra de Lucanas, Ayacucho. Bol. Arqueol. PUCP 25, 229–254. doi: 10.18800/boletindearqueologiapucp.201802.008
Reindel, M., Isla, J., Gorbahn, H., and Otten, H. (2015). Paracas en Palpa: los fundamentos del poder de la cultura Nasca. Per. Archaeol. 2, 37–64.
Rose, H. A., Müller-Scheeßel, N., Meadows, J., and Hamann, C. (2022). Radiocarbon dating and Hallstatt chronology: a Bayesian chronological model for the burial sequence at Dietfurt an der Altmühl ‘Tennisplatz', Bavaria, Germany. Archaeol. Anthropol. Sci. 14:72. doi: 10.1007/s12520-022-01542-1
Sammells, C. A. (2019). “Production, trade, reciprocity, and markets,” in The Andean World, eds K. S. Fine-Dare, and L. J. Seligmann (New York, NY: Routledge), 251–265.
Sandor, J. A., and Homburg, J. A. (2017). Anthropogenic soil change in ancient and traditional agricultural fields in arid to semiarid regions of the Americas. J. Ethnobiol. 37, 196–217. doi: 10.2993/0278-0771-37.2.196
Sandor, J. A., Huckleberry, G., Hayashida, F. M., Parcero-Oubiña, C., Salazar, D., Troncoso, A., et al. (2022). Soils in ancient irrigated agricultural terraces in the Atacama desert, Chile. Geoarchaeology 37, 96–119. doi: 10.1002/gea.21834
Sandweiss, D. H., and Richardson, J. B. (2008). “Central Andean environments,” in The Handbook of South American Archaeology, eds H. Silverman, and W. H. Isbell (New York, NY: Springer), 93–104.
Schittek, K., Forbriger, M., Mächtle, B., Schäbitz, F., Wennrich, V., Reindel, M., et al. (2015). Holocene environmental changes in the highlands of the southern Peruvian Andes (14° S) and their impact on pre-Columbian cultures. Clim. Past 11, 27–44. doi: 10.5194/cp-11-27-2015
Schroeder, S. (2001). Understanding variation in prehistoric agricultural productivity: the importance of distinguishing among potential, available, and consumptive yields. Am. Antiq. 66, 517–525. doi: 10.2307/2694249
Soßna, V. (2015). Climate and Settlement in Southern Peru: The Northern Río Grande de Nasca Drainage between 1500 BCE and 1532 CE. Wiesbaden: Reichert.
Spanò, A., Sammartano, G., Calcagno Tunin, F., Cerise, S., and Possi, G. (2018). GIS-based detection of terraced landscape heritage: comparative tests using regional DEMs and UAV data. Appl. Geomat. 10, 77–97. doi: 10.1007/s12518-018-0205-7
Tantaleán, H. (2021). “The Paracas society of prehispanic Peru,” in Oxford Research Encyclopedia of Latin American History (Oxford: Oxford University Press), 1–27.
Tomasto, E. (2009). “Talking bones: bioarchaeological analysis of individuals from Palpa,” in New Technologies for Archaeology: Multidisciplinary Investigations in Palpa and Nasca, Peru, eds M. Reindel, and G. A. Wagner (Berlin: Springer), 141–158.
Treacy, J. M. (1994). Las chacras de Coporaque: andenería y riego en el valle del Colca. Lima: Instituto de Estudios Peruanos.
Treacy, J. M., and Denevan, W. M. (1994). “The creation of cultivable land through terracing,” in The Archaeology of Garden and Field, eds N. F. Miller, and K. L. Gleason (Philadelphia, PA: University of Pennsylvania Press), 91–110.
Twiss, P. C. (1992). “Predicted world distribution of C3 and C4 grass phytoliths,” in Phytolith Systematics: Emerging Issues, eds G. Rapp, and S. C. Mulholland (New York, NY: Springer), 113–128.
Unkel, I., Reindel, M., Gorbahn, H., Isla, J., Kromer, B., and Soßna, V. (2012). A comprehensive numerical chronology for the pre-Columbian cultures of the Palpa valleys, south coast of Peru. J. Archaeol. Sci. 39, 2294–2303. doi: 10.1016/j.jas.2012.02.021
Uysal, M., Toprak, A. S., and Polat, N. (2015). DEM generation with UAV Photogrammetry and accuracy analysis in Sahitler hill. Measurement 73, 539–543. doi: 10.1016/j.measurement.2015.06.010
Van Oyen, A. (2018). “Material agency,” in The Encyclopedia of Archaeological Sciences, ed. S. L. López Varela (Oxford: Wiley), 1–5. doi: 10.1002/9781119188230.saseas0363
Vavilov, N. I. (1992). Origin and Geography of Cultivated Plants. Cambridge: Cambridge University Press.
Wang, R. J., Al-Saffar, S. I., Rogers, J., and Hahn, M. W. (2023). Human generation times across the past 250,000 years. Sci. Adv. 9:eabm7047. doi: 10.1126/sciadv.abm7047
Williams, P. R. (2006). “Agricultural innovation, intensification, and sociopolitical development,” in Agricultural Strategies, eds C. Stanish, and M. Joyce (Los Angeles, CA: Cotsen Institute of Archaeology Press), 309–333.
Winnebeck, J., Sutter, O., Hermann, A., Antweiler, C., and Conermann, S. (2023). The analytical concept of asymmetrical dependency. J. Global Slav. 8, 1–59. doi: 10.1163/2405836X-00801002
Winzeler, H. E., Owens, P. R., Kharel, T., Ashworth, A., and Libohova, Z. (2023). Identification and delineation of broad-base agricultural terraces in flat landscapes in northeastern Oklahoma, USA. Land 12:486. doi: 10.3390/land12020486
Wutich, A., Beresford, M., and Carvajal, C. (2017). Ayni real and imagined: reciprocity, indigenous institutions, and development discourses in contemporary Bolivia. J. Latin Am. Caribb. Anthropol. 22, 475–494. doi: 10.1111/jlca.12292
Zech, W., Schad, P., and Hintermaier-Erhard, G. (2014). Böden der Welt: ein Bildatlas. Berlin: Springer.
Keywords: Andes, terrace agriculture, dependency, Late Paracas, Initial Nasca, GIS and remote sensing, soil testing, phytolith analysis
Citation: Mader C, Godde P, Behl M, Binder C, Hägele E, Isla J, Leceta F, Lyons M, Marsh E, Odenthal R, Fernengel E, Stryjski P, Weber A-K, Reindel M and Meister J (2024) An integrative approach to ancient agricultural terraces and forms of dependency: the case of Cutamalla in the prehispanic Andes. Front. Environ. Archaeol. 3:1328315. doi: 10.3389/fearc.2024.1328315
Received: 26 October 2023; Accepted: 30 January 2024;
Published: 14 February 2024.
Edited by:
Celeste Samec, National Scientific and Technical Research Council (CONICET), ArgentinaReviewed by:
Patricia Aparicio Martinez, University of Toronto, CanadaValeria Leticia Franco Salvi, CCT CONICET Córdoba, Argentina
Gabriel Prieto, University of Florida, United States
Copyright © 2024 Mader, Godde, Behl, Binder, Hägele, Isla, Leceta, Lyons, Marsh, Odenthal, Fernengel, Stryjski, Weber, Reindel and Meister. This is an open-access article distributed under the terms of the Creative Commons Attribution License (CC BY). The use, distribution or reproduction in other forums is permitted, provided the original author(s) and the copyright owner(s) are credited and that the original publication in this journal is cited, in accordance with accepted academic practice. No use, distribution or reproduction is permitted which does not comply with these terms.
*Correspondence: Christian Mader, Y2hyaXN0aWFuLm1hZGVyJiN4MDAwNDA7dW5pLWJvbm4uZGU=; Julia Meister, anVsaWEubWVpc3RlciYjeDAwMDQwO3VuaS13dWVyemJ1cmcuZGU=