- 1Department of Anthropology, University of Utah, Salt Lake City, UT, United States
- 2Natural History Museum of Utah, Salt Lake City, UT, United States
- 3Institute of Ecology and Evolution, University of Oregon, Eugene, OR, United States
- 4Department of Geology and Geophysics, University of Utah, Salt Lake City, UT, United States
- 5Department of Anthropology, University of Connecticut, Storrs, CT, United States
- 6Human Origins Program, National Museum of Natural History, Smithsonian Institution, Washington, DC, United States
- 7Department of Earth Sciences, National Museums of Kenya, Nairobi, Kenya
- 8Origins Centre, University of the Witwatersrand, Johannesburg, South Africa
For many animals, migration is an important strategy for navigating seasonal bottlenecks in resource availability. In the savannas of eastern Africa, herds of grazing animals, including blue wildebeest (Connochaetes taurinus), Thomson's gazelle (Eudorcas thomsonii), and plains zebra (Equus quagga), travel hundreds of kilometers annually tracking suitable forage and water. However, we know nearly nothing about migration among the extinct species that often dominated Late Pleistocene communities. Using serially sampled 87Sr/86Sr and δ13C, we characterize the prehistoric movement and diet of the enigmatic wildebeest Rusingoryx atopocranion from two localities (Karungu and Rusinga Island) in the Lake Victoria Basin of western Kenya. We find clear evidence for migration in all four individuals studied, with three 87Sr/86Sr series demonstrating high-amplitude fluctuations and all falling outside the modeled isoscape 87Sr/86Sr ranges of the fossil localities from which they were recovered. This suggests that R. atopocranion exhibited migratory behavior comparable to that of its closest living relatives in the genus Connochaetes. Additionally, individuals show seasonally-variable δ13C, with a higher browse intake than modern and fossil eastern African alcelaphins indicating behavioral differences among extinct taxa otherwise unrecognized by comparison with extant related species. That this species was highly migratory aligns with its morphology matching that of an open grassland migrant: it had open-adapted postcranial morphology along with a unique cranial structure convergent with lambeosaurine dinosaurs for calling long distances. We further hypothesize that its migratory behavior may be linked to its extinction, as R. atopocranion disappears from the Lake Victoria Basin fossil sequence coincident with the refilling of Lake Victoria sometime after 36 ka, potentially impeding its past migratory routes. This study characterizes migration in an extinct eastern African species for the first time and shapes our ecological understanding of this unique bovid and the ecosystems in which Middle Stone Age humans lived.
1. Introduction
Seasonal migration plays a key role in eastern African ecosystems, most famously in the Greater Serengeti Ecosystem (GSE) of Kenya and Tanzania. Seasonal migrants in the GSE are primarily grazers tracking high-quality grass seasonally (Holdo et al., 2009; Anderson et al., 2010). Migration mitigates competition during times of scarcity and allows migratory populations to sustain far higher population density than nonmigratory ones, even when comparing populations of the same species (Fryxell et al., 1988; Staver and Hempson, 2020). The three key seasonal migrants in this ecosystem are blue wildebeest (Connochaetes taurinus), Thomson's gazelle (Eudorcas thomsonii), and plains zebra (Equus quagga), which migrate hundreds of kilometers annually between short grasslands in the southern GSE in wet seasons and wooded tall grasslands in the northern GSE (the Mara region) in dry seasons (Estes, 1991, 2014) (Figure 1). Numbering in the millions, C. taurinus is the dominant species in this migration (Estes, 2014). An additional historic migratory system brought large mammals from the Lambwe Valley of the eastern Lake Victoria Basin to the Mara region annually (Allsopp and Baldry, 1972), indicating that the migratory systems of the GSE once had a much larger reach prior to colonial practices of population and land control.
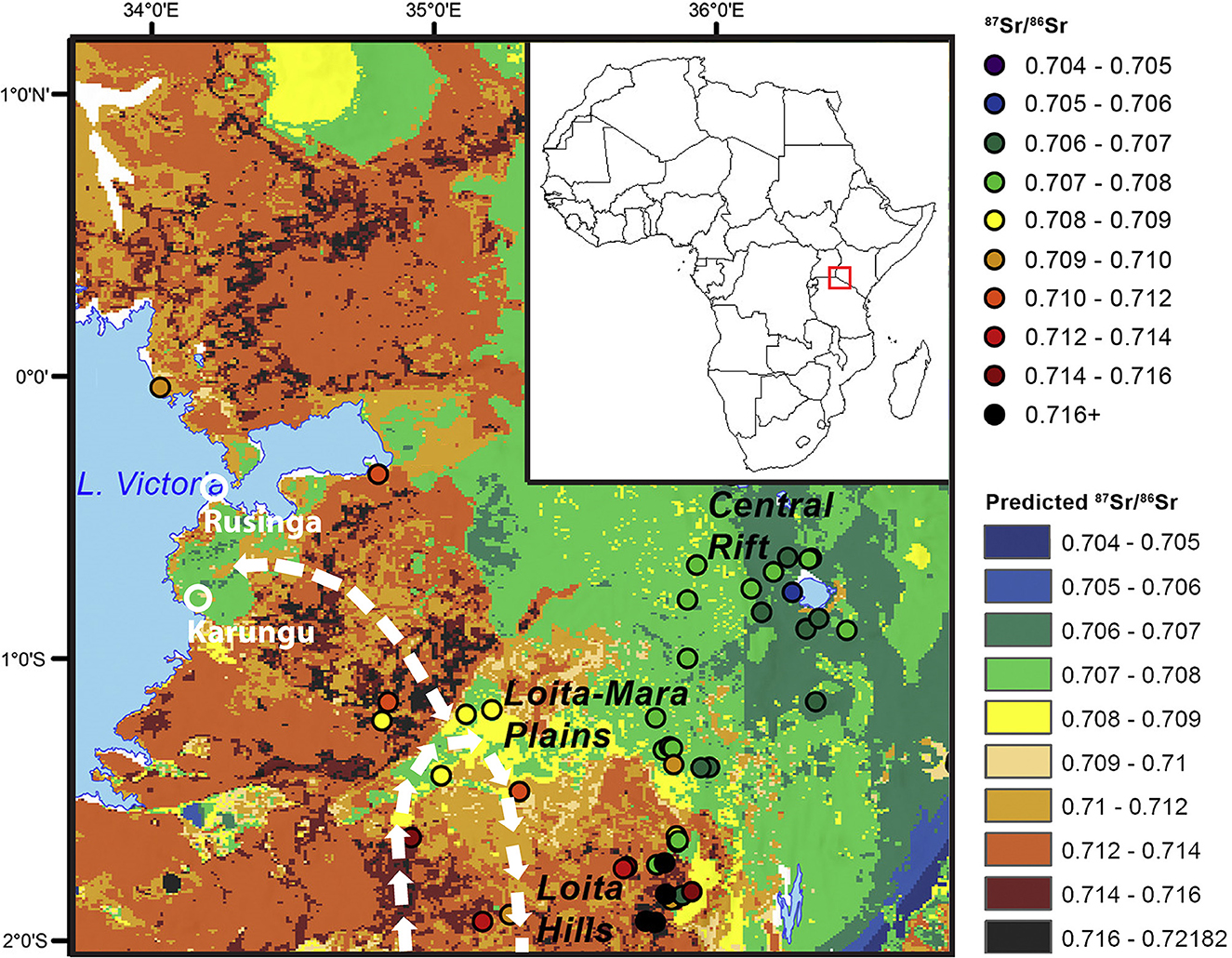
Figure 1. 87Sr/86Sr isoscape for the eastern Lake Victoria Basin (modified from Janzen et al., 2020), with Karungu and Rusinga labeled. Modern and historical migration paths noted with white arrows. Position within Africa shown on inset.
Wildebeest migrations in the GSE and elsewhere are well-studied today, but our knowledge of prehistoric migration in Africa is limited to only a few studies (Faith and Thompson, 2013; Copeland et al., 2016; Tucker et al., 2020). The GSE is the largest grassland ecosystem in modern eastern Africa, surrounded by a heterogeneous array of semideserts, bushlands, woodlands, and forests (Burgess et al., 2004). However, there is strong fossil and isotopic evidence that the GSE was greatly expanded during the Last Glacial Period (115–11.7 ka; LGP), with C4 plant communities dominating most archaeological and paleontological sites in eastern Africa (Marean, 1992; Steele, 2007; Faith et al., 2012, 2015; Tryon et al., 2012, 2016; Beverly et al., 2015). Bulk sampled enamel δ13C of ungulates and rodents also indicates the dominance of C4 grasses in this region during the LGP (Faith et al., 2015; Tryon et al., 2016). In this expanded GSE, C. taurinus was joined by two extinct wildebeest species: the strange-headed Rusingoryx atopocranion and the giant Megalotragus sp. (Faith et al., 2011, 2015; Tryon et al., 2012). Though closely related to one another, these species varied in size, ecomorphology, and, most strikingly, in head shape, with R. atopocranion characterized by nasal cavities housed within bony cranial crests convergent with lambeosaurine dinosaurs allowing it to produce low-frequency calls (O'Brien et al., 2016). Additionally, stable isotopic analyses suggest that these wildebeests may have varied in diet, with R. atopocranion and Megalotragus sp. having lower δ13C values than other alcelaphins (Faith et al., 2015; Garrett et al., 2015), though this has only been evaluated with bulk sampling. Additional stable isotope work will help resolve the paleoecology of this unique species. This in turn can help to shed light on the ecosystems in which Middle Stone Age humans lived and the seasonal availability of large game (Ambrose and Lorenz, 1990; Marean, 1997).
In this study, we employ stable strontium and carbon isotopes from serially sampled molars to evaluate the paleoecology of R. atopocranion during the LGP. 87Sr/86Sr will shed light on migration and δ13C will inform on diet and dietary seasonality. We analyze four individuals from the eastern Lake Victoria Basin localities of Karungu and Rusinga, including three individuals from a catastrophic death assemblage at Rusinga thought to have come from the same bachelor herd (O'Brien et al., 2016; Jenkins et al., 2017). Our results shed light on the behavioral ecology of this unique bovid and characterize the migratory paleoecology of an extinct eastern African species for the first time.
2. Materials and methods
2.1. Sampling
Four Rusingoryx atopocranion specimens from the eastern Lake Victoria Basin localities of Karungu and Rusinga were selected for analysis. These two localities are among the region's most fossiliferous assemblages from the Late Pleistocene and have well-established chronologies (Tryon et al., 2012; Faith et al., 2015; Jenkins et al., 2017). The paleontology, archaeology, and geology of both localities have been the subject of ongoing research by the Lake Victoria Prehistory Project since 2009. Rusinga is an island in Lake Victoria connected to mainland Kenya during much of the LGP (Tryon et al., 2016; Beverly et al., 2020), with the Pleistocene Wasiriya Beds yielding abundant large mammal fauna collected across multiple stratigraphic intervals spanning ~100–36 ka (Tryon et al., 2010, 2012). Rusinga's fossil record is dominated by R. atopocranion, with this single species accounting for 34% of the recovered mammalian fauna (Tryon et al., 2016). Karungu is a set of Late Pleistocene exposures on the Kenyan mainland with stratigraphic sequences correlated to the Wasiriya Beds of Rusinga Island (Faith et al., 2015). Its ungulate fauna consists of abundant alcelaphins, also dominated by R. atopocranion (Faith et al., 2015).
All faunal specimens from Rusinga and Karungu are curated by the Earth Sciences Department at the National Museums of Kenya in Nairobi, Kenya. The Karungu specimen (LVP 2015-462) originates from the locale of Kisaaka (~100–33 ka), and the Rusinga specimens (RUP 12-22, RU-2007-1004, and KNM-RU-49553) are all from the catastrophic death assemblage known as Bovid Hill at Wakondo. Bovid Hill preserves the remains of dozens of R. atopocranion individuals in a channel deposit dated to 68 ± 5 ka by optically stimulated luminescence (Jenkins et al., 2017). These individuals are thought to have come from a bachelor herd killed by either human hunting or a mass drowning event. As these three specimens come from the same site, care was taken to ensure they belong to different individuals: they are at different wear stages and have differing mesowear and unique occlusal morphology. The four specimens selected for sampling are among the most complete second and third molars from these localities, with complete enamel surfaces and lacking substantial cracks, discoloration, or other damages. First molars were avoided due to their mineralization occurring during gestation or while nursing, leading to their geochemical signals reflecting those of their mothers rather than their own diet and water intake (Balasse et al., 2001). Identifications were confirmed using fossil and modern comparative collections. Summary descriptions of each specimen are given in Table 1.
Each molar was cleaned with acetone and cotton swabs, and the outermost layer of enamel was removed using a handheld drill with a 1.2 mm diameter diamond bit. Then, ~8 mg of enamel was removed with the drill as powder into a microcentrifuge tube in 2–5 mm serial increments along the growth axis from apex to cervix. Sampling lines were ~5 mm by 1 mm. Each tooth was sampled 11 to 13 times (Figure 2). The powder samples were then shipped to the University of Utah for processing. Each aliquot of powdered tooth enamel was divided, with one portion for strontium analysis and the other for carbonate analysis. All remaining enamel powder was retained for future research.
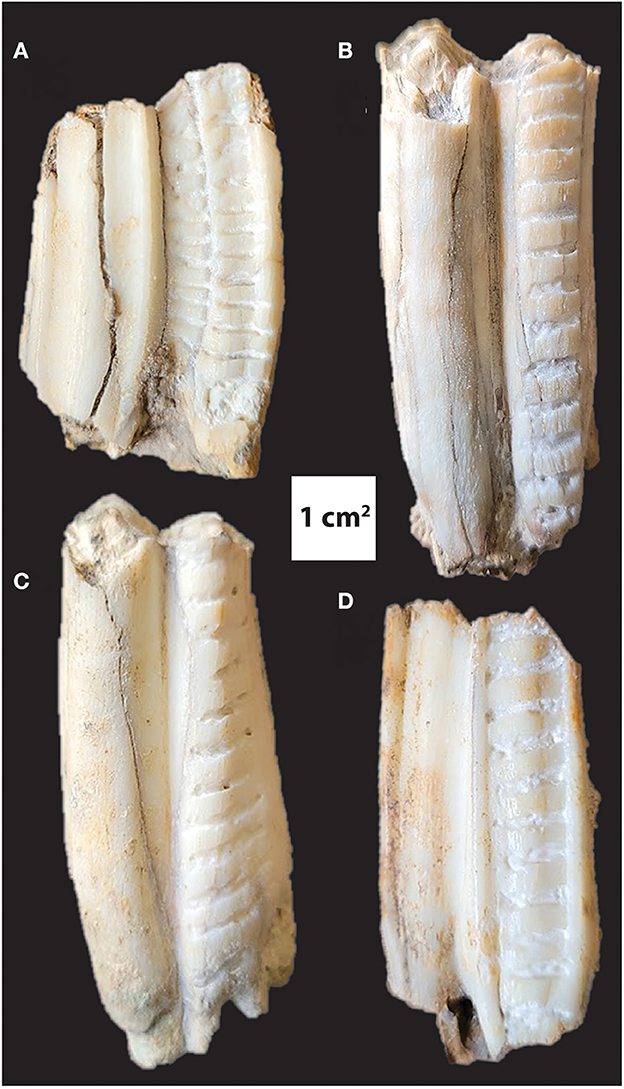
Figure 2. Photos of each specimen after sampling: LVP 2015-462 (A), RUP 12-22 (B), KNM-RU-49553 (C), and RU-2007-1004 (D).
2.2. 87Sr/86Sr analysis
A 2 ± 0.25 mg sample of enamel powder was taken from each sample for inductively-coupled plasma mass spectrometry (ICP-MS) 87Sr/86Sr analysis in the ICP-MS Metals Laboratory in the University of Utah Department of Geology and Geophysics following the methods of Mackey and Fernandez (2011). Samples were first digested in concentrated nitric acid. Then, an aliquot of each sample was combined with indium and diluted with 2.4% nitric acid, and elemental concentrations were measured using an ICP-MS Agilent. Using calculated elemental concentrations, dissolved enamel solutions were diluted to a minimum of 200 ppb Sr and purified using a PrepFAST automated inline dilution system. Samples were then dried, suspended in 2.4% nitric acid, and then run on a Multicollector ICP-MS to measure 87Sr/86Sr isotope ratios.
The resulting 87Sr/86Sr values were then synthesized for each individual and used to determine the degree to which individuals were migratory, which areas migratory individuals moved through, and how this varied seasonally. Both the raw 87Sr/86Sr values and the amplitude of variation through each tooth were considered for these purposes. Values were compared to those of the isoscape model produced by Janzen et al. (2020), shown in Figure 1. Based on differences in geology and other factors (see Bataille et al., 2018, 2020; Janzen et al., 2020 for variables used), the modeled local 87Sr/86Sr range (values within <5 km radius of locality) for the Karungu locale of Kisaaka is ~0.707–0.708. The isoscape does not predict 87Sr/86Sr values for islands, so two East African mole rat (Tachyoryctes splendens) fossils from the Rusinga LGP locales of Nyamita and Wakondo were evaluated, producing 87Sr/86Sr values of 0.70495 and 0.70502, respectively. The assumption here is that the home ranges of these animals is small, and should therefore provide a more localized signal of the Sr values of Rusinga. In addition to the Wasiriya Beds, Rusinga hosts early Miocene lavas and sediments, which Janzen et al. (2020) show to have a mean value of 0.70668 elsewhere in the Kenyan rift system. Finally, the Miocene complexes immediately south of Rusinga on the mainland have values from 0.707 to 0.708. Therefore, a conservative estimate for the range of values on Rusinga is 0.704–0.708. Any values outside these ranges for the two localities are interpreted here as indicative of migration.
2.3. Carbonate analysis
Samples of 250 ± 25 μg were used for paired δ13C and δ18O analyses. Samples were treated with 0.1 M ammonium hydroxide buffered acetic acid for 30 mins and stirred every 10 mins on a vortex mixer. Samples were then centrifuged, and the supernatant was removed. Next, samples were rinsed three times with 18.2 MΩ-cm resistance Milli-Q water. All remaining supernatant was removed, and samples were dried for 12 hours in a 50°C oven. Paired δ13C and δ18O analyses were conducted in the Stable Isotope Ratio Facility for Environmental Research in the University of Utah Department of Biology. Enamel powder samples were reacted with 100% H3PO4 at 50°C in glass vials for 8 hours using a Thermo Finnigan GasBench II. CO2 was extracted using a GC-TC PAL autosampler connected to a Conflo IV. 13C/12C and 18O/16O ratios were measured using a Thermo Finnigan MAT 253, with data corrected with fossil internal laboratory carbonate standards and for the temperature-dependent acid fractionation factor for fossils (α = 1.00699322, at 50°C). All results were reported relative to the VPDB standard (see Appendix A of Yang et al., 2020 for protocol).
δ13C will inform diet and intra-annual dietary variation. Percent C4 is calculated using a dual endpoint mixing model, with 100% C4 at 4.1‰ and 100% C3 at−12.5‰ (following Codron et al., 2007; Cerling et al., 2015; Rowan et al., 2017; Abraham et al., 2019; Robinson et al., 2021):
The results for Rusingoryx atopocranion will be compared to those of alcelaphins in modern eastern Africa using data from Cerling et al. (2015).
Intra-annual variation in diet will be considered in tandem with 87Sr/86Sr results. Variation in δ13C is most substantial in nonmigrant species due to dietary switching (i.e., consuming unpreferred vegetation during dry seasons), while migrants often show little variation as they track preferred resources seasonally (Abraham et al., 2019). Though measured in this study, interpreting the δ18O results from a single species is complex (Blumenthal et al., 2017, 2019), especially for a species like R. atopocranion that is likely water-dependent like its modern relatives (Kingdon and Hoffman, 2013). Thus, δ18O results are not interpreted in detail here and will require further investigation incorporating water-independent taxa from the same localities.
3. Results
Results for 87Sr/86Sr, δ13C, and δ18O are summarized in Table 2.
3.1. 87Sr/86Sr results
87Sr/86Sr results are shown for each individual in Figure 3. The majority of measurements fall outside the modeled isoscape range for the localities (0.707–0.708 for Karungu; 0.704–0.708 for Rusinga), indicating that most of their tooth development occurred elsewhere. However, for three of the four specimens, 87Sr/86Sr values overlap with the local signal at one or two points in the series, likely indicating that Rusinga or Karungu were part of each individual's migratory path during the period of tooth formation. In addition, all individuals but RU-2007-1004 exhibit high amplitude in values (>0.001), consistent with seasonal migratory behavior.
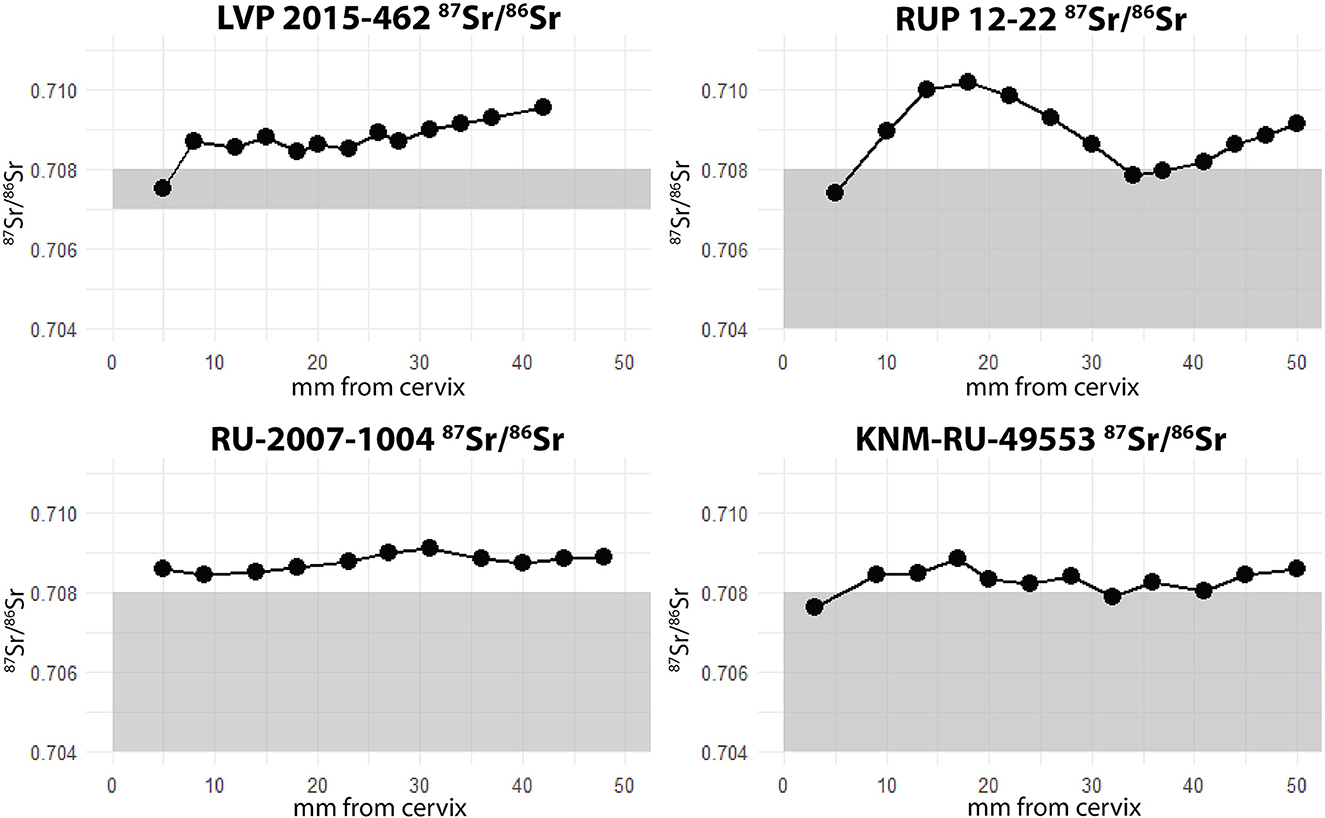
Figure 3. 87Sr/86Sr results for all Rusingoryx atopocranion individuals analyzed. Modeled range at each locality highlighted in gray.
Individual RU-2007-1004 has a low amplitude relative to the other three specimens, and does not overlap with the local range at Rusinga. Therefore, it can be inferred that it traveled relatively little during the period of tooth development, though outside Rusinga, before migrating or dispersing to Rusinga in later life before its death. Alternatively, it traveled exclusively within an area of uniform strontium isotope values. This is consistent with the fission-fusion systems of many modern bovids, especially those living in bachelor herds like the one these individuals presumably derive from (Estes, 1991).
3.2. δ13C results
δ13C results for each individual are shown in Figure 4. All individuals have a majority C4 grazing signal throughout each tooth, though they vary to some extent throughout the year, and all incorporated some C3 browse into their diet, with individual samples varying from 63 to 86% C4. Despite generally falling into the range of grazing, Rusingoryx atopocranion has lower δ13C values than any modern alcelaphin species in eastern Africa, falling outside the 1σ ranges of each (Cerling et al., 2015). This is consistent with the findings by Faith et al. (2015) and Garrett et al. (2015) that R. atopocranion has lower δ13C values than other alcelaphins at eastern Lake Victoria Basin fossil sites. Comparison of R. atopocranion results to those of modern alcelaphins is shown in Figure 5, calculated using the means of serial samples from each individual and combined with data from Faith et al. (2015) and Garrett et al. (2015). Rusingoryx atopocranion has significantly lower δ13C than all modern alcelaphins in eastern Africa as well as fossil hartebeest (Alcelaphus buselaphus), blue wildebeest (Connochaetes taurinus), and extinct eastern African blesbok (Damaliscus hypsodon) (two-sample t-test p < 0.004 for all), though it overlaps in range with the extinct giant wildebeest (Megalotragus sp.). RUP 12-22, the specimen with the strongest migratory signal, also has the strongest evidence for diet-switching, with 87Sr/86Sr ranging from 0.70742 to 0.71019 and δ13C ranging from −2.0 to 1.8‰.
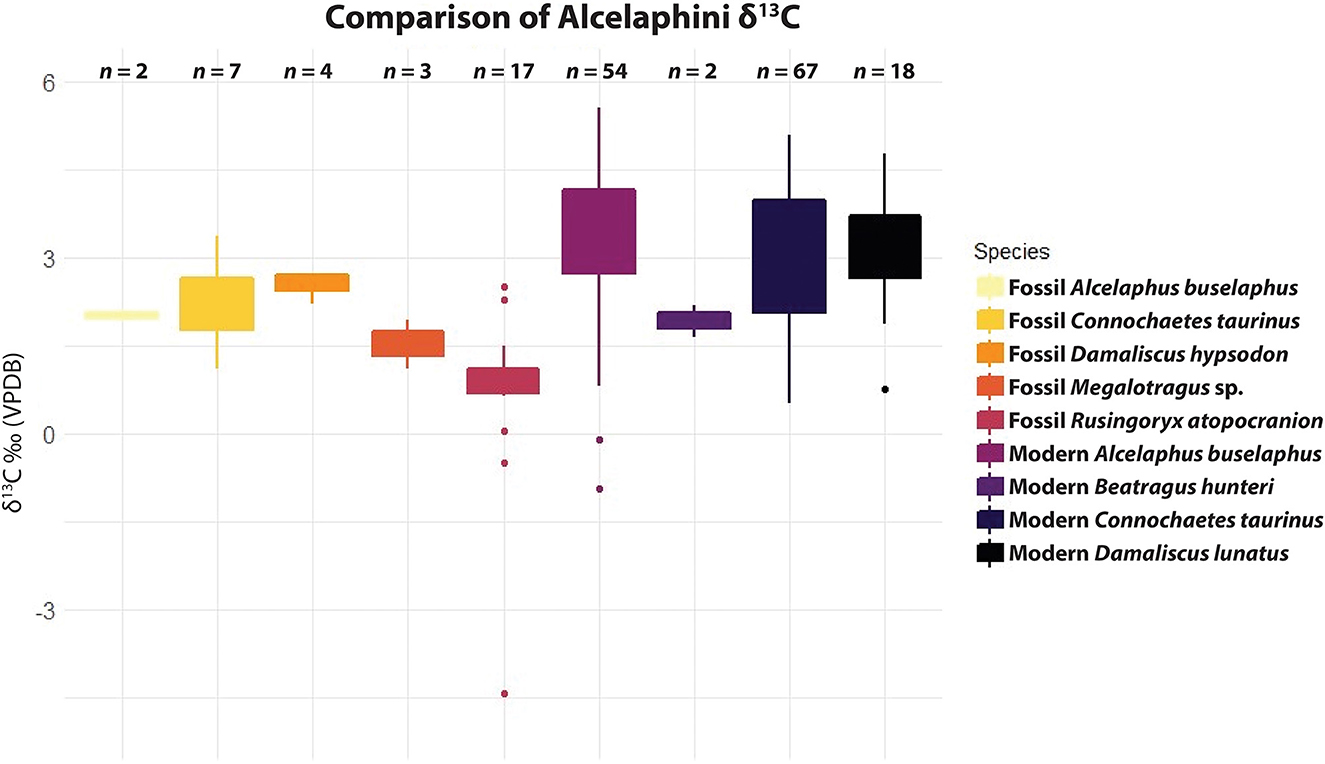
Figure 5. Rusingoryx atopocranion δ13C results compared to fossil alcelaphins from the eastern Lake Victoria Basin using data from Faith et al. (2015) and Garrett et al. (2015), and modern eastern African alcelaphins using data from Cerling et al. (2015). Garrett et al. (2015) corrected values to modern levels by subtracting 1.4‰, so 1.4‰ was added back to these values.
4. Discussion
4.1. Migration
We provide the first direct evidence of migration in a Pleistocene eastern African animal, the extinct wildebeest Rusingoryx atopocranion, at the LGP localities of Karungu and Rusinga. A majority of 87Sr/86Sr values for all R. atopocranion individuals differ from those modeled for the localities at which their fossils were found. Additionally, three out of four individuals analyzed have notably high amplitude fluctuations, indicating movement during the period of tooth formation. Lake Victoria was desiccated during the time when these animals lived, allowing for movement to and from islands like Rusinga (Beverly et al., 2020), but the high 87Sr/86Sr values of Rusinga individuals (>0.708) do not suggest that these individuals roamed over the dry bed of Lake Victoria during tooth development, as Quaternary lake sediments would likely have low 87Sr/86Sr values like those of Rusinga (~0.705). However, more information is needed regarding the true geologic nature of the sediments and bedrock underlying Lake Victoria. Rather, the 87Sr/86Sr values of all four specimens are consistent with those of several higher-elevation areas to the north, east, and south of Rusinga and Karungu, such as the Uyoma and Homa Peninsulas (Figure 1). Movement from far more distant places is also possible, as much of western Kenya has values ranging from 0.708 to 0.710 (Janzen et al., 2020), as detected in the serial samples. Additionally, when considering the delayed attenuation rate of Sr in bovid enamel (Lazzerini et al., 2021), the actual amplitude of geologic strontium in the areas traveled by R. atopocranion may be much higher, in which case the distance of migration may have been far greater.
Two individuals from Bovid Hill (RUP 12-22 and KNM-RU-49553) have patterns possibly indicating traveling to Rusinga twice during the period of tooth formation (Figure 3), perhaps one year apart if the growth rate in other medium-sized bovids can be applied to R. atopocranion (Milhaud and Nezit, 1991; Zazzo et al., 2010). This is consistent with them having been part of the same herd, as argued by Jenkins et al. (2017), or at least having followed a similar migration timing through the time of tooth formation.
That R. atopocranion is revealed to be a migrant is perhaps unsurprising, as its closest living relatives, the blue and black wildebeests of the genus Connochaetes, were highly migratory historically. Additionally, R. atopocranion possesses morphological adaptations for migration, including unique cranial morphology distinguishing it from all other ungulates. This is most apparent in its strange, elongated and sinuous nasal cavity convergent with that of lambeosaurine dinosaurs, suitable for calling long distances (O'Brien et al., 2016). This behavior would be advantageous to animals traveling long distances to maintain communication with conspecifics. Its postcranial morphology also indicates that this species was exceptionally adapted for open environments, with some limb structures outside the range of variation in modern African Bovidae, including shorter relative phalanx length than any other taxon (Kovarovic et al., 2021). This is consistent with animals traveling across open grasslands seasonally.
4.2. Diet
Though primarily grazing, Rusingoryx atopocranion incorporated more browse into its diet than any modern eastern African alcelaphin, including its closest relative in the region, Connochaetes taurinus (Figure 5). The relatively low δ13C values of R. atopocranion may seem contradictory to the morphology of this species, being highly hypsodont (Faith et al., 2011) and open-adapted postcranially (Kovarovic et al., 2021). Additionally, Faith et al. (2011) find that its mesowear clusters with other grazers. Indeed, R. atopocranion and other extinct eastern African ungulates, which are typically characterized by extreme hypsodonty (R. atopocranion and D. hypsodon) or massive body size (Megalotragus sp. and S. antiquus), are often thought to be highly specialized C4-grazers (Faith, 2014). Our δ13C values for R. atopocranion instead suggest a more varied diet, consistent with other ungulate species in semi-arid environments that seasonally consume herbaceous dicots, including Grévy's zebra (Equus grevyi) and eastern African oryx (Oryx beisa) (Cerling et al., 2015), both of which frequently co-occur with R. atopocranion (Faith et al., 2013). The most arid-associated alcelaphin in modern eastern Africa, hirola (Beatragus hunteri), has the lowest δ13C values of any eastern African alcelaphin today (Figure 5), though even these values are more positive than those of R. atopocranion.
A perhaps surprising finding is that these migratory individuals exhibited diet-switching. This is best demonstrated in the individual RUP 12-22, which has particularly high amplitude in both 87Sr/86Sr and δ13C. Today, African savanna herbivores often have a tradeoff between migration and diet-switching: to survive dry seasons, grazers typically either have to migrate to follow high-quality grass forage or temporarily switch to incorporating more browse in their diets (Abraham et al., 2019). For R. atopocranion, migration and diet-switching both occurred in the same individuals. This suggests that the seasonal ecological pressures on this species may have been different during the LGP than those experienced by modern large herbivores in the region.
4.3. Implications for extinction
Rusingoryx atopocranion is most associated in the fossil record with a guild of extinct and endangered hypsodont grazers affiliated with semi-arid environments, including Damaliscus hypsodon, Megalotragus sp., Oryx beisa, Syncerus antiquus, Ceratotherium simum, and Equus grevyi (Faith et al., 2013). That these species are absent or rare in eastern Africa today is likely due to their preferred ecosystems being more widespread during the LGP than today. The combination of a colder climate (Osmaston, 1989; Faith et al., 2016a), a hyperabundance of grazing ungulates (Faith et al., 2012, 2015; Tryon et al., 2012), and the expansion of grassy environments across much of the eastern Lake Victoria Basin (Steele, 2007; Beverly et al., 2015; Tryon et al., 2016) created a non-analog faunal community in which R. atopocranion was the dominant species (Faith et al., 2016b). Many of these most-associated species became extinct or extirpated around the Pleistocene-Holocene transition (Faith, 2014) when precipitation increased at the onset of the African Humid Period and woody ecosystems expanded.
However, R. atopocranion disappears earlier, with its last appearance in the Lake Victoria Basin (the only region in which it is presently known) predating 36 ka at Karungu, Mfangano, and Rusinga (Tryon et al., 2012; Faith et al., 2015). It is notably absent at the well-sampled nearby site of Kibogo (~36–12 ka; ~95 km NE from Rusinga), where the dominant alcelaphins are blue wildebeest and hartebeest (Faith et al., 2020). The faunal evidence from Kibogo (Faith et al., 2020) as well as Holocene zooarchaeological assemblages from the Lake Victoria Basin (Chritz et al., 2019) indicate that grasses were still widely available at later dates, suggesting that factors other than declining forage availability may have been to blame in this species' disappearance from the Lake Victoria Basin. Using the results of this study, a possible additional factor for the extinction of R. atopocranion can be deduced: migration. Migrating long distances would be possible in the eastern Lake Victoria Basin so long as grassy ecosystems persisted and there were no obstacles along the migration path. From 100–36 ka, this would have likely been the case, as all lines of evidence suggest reduced lake levels (Beverly et al., 2020) and extensive grasslands (Marean, 1992; Steele, 2007; Faith et al., 2012, 2015; Tryon et al., 2012, 2016; Beverly et al., 2015). However, the previously desiccated Lake Victoria filled after 36 ka (Beverly et al., 2020). In addition to making Rusinga an island directly cutting it off from the mainland, making movement difficult, this also established the Winam Gulf and other waterways blocking long-distance travel through much of the region. For this reason, we hypothesize that the demise of R. atopocranion in the Lake Victoria Basin may be linked to its migratory behavior. Finally, as argued by Kovarovic et al. (2021), the re-establishment of Lake Victoria would have been associated with increased humidity and precipitation in the region, possibly leading to expansion of woodier environments that may have further impeded migratory pathways.
5. Conclusions
The paleoecology of Rusingoryx atopocranion has been extensively studied due to the abundance of well-preserved specimens from the Lake Victoria Basin and its unique morphology. This study adds a new dimension to our understanding of this enigmatic species through the application of serially sampled strontium and carbon isotopes, revealing insights into its migratory behavior and diet. We find the first direct evidence of prehistoric migration in eastern Africa, consistent with seasonal migration within the eastern Lake Victoria Basin. Additionally, we report a uniquely high browsing component in the diet of this species that is perhaps unanticipated by its morphology but is similar to arid-adapted ungulates in eastern Africa. These results offer insight into the behavior of this species on an individual level and possibly into its extinction.
Data availability statement
The original contributions presented in the study are included in the article/supplementary material, further inquiries can be directed to the corresponding author.
Author contributions
KO'B and JF conceived the project. KO'B, JF, and CT provided funding. KO'B, LA, KP, and DF conducted stable isotope analyses. KO'B wrote the manuscript. All authors provided manuscript feedback and gave final approval for paper publication.
Funding
Collection of fossil specimens in the Lake Victoria Basin was made possible with support from the National Museums of Kenya (NMK) and with funding provided by the National Geographic Society (9284-13 and 8762-10), the National Science Foundation (BCS-1013199 and BCS-1013108), the Leakey Foundation, the Geological Society of America, the University of Minnesota, Baylor University, New York University, the University of Queensland, Harvard University, and the American School of Prehistoric Research. Isotopic analyses were funded by a National Science Foundation (NSF) Doctoral Dissertation Research Improvement Grant (#2234426), an NSF Graduate Research Fellowship (fellow ID 2020294514), a Graduate Research Grant from the Leakey Foundation, the Dawson Grant from the Society of Vertebrate Paleontology, the University of Utah Global Change and Sustainability Center, and the Wilkes Center for Climate Science and Policy.
Acknowledgments
We thank John Rowan and Anneke Janzen for their roles in conceiving this project, Suvankar Chakraborty for his assistance with processing isotope samples, and Sally Reynolds for the invitation to submit this manuscript to this special issue.
Conflict of interest
The authors declare that the research was conducted in the absence of any commercial or financial relationships that could be construed as a potential conflict of interest.
Publisher's note
All claims expressed in this article are solely those of the authors and do not necessarily represent those of their affiliated organizations, or those of the publisher, the editors and the reviewers. Any product that may be evaluated in this article, or claim that may be made by its manufacturer, is not guaranteed or endorsed by the publisher.
References
Abraham, J. O., Hempson, G. P., and Staver, A. C. (2019). Drought-response strategies of savanna herbivores. Ecol. Evol. 12, 259. doi: 10.1002/ece3.5270
Allsopp, R., and Baldry, D. A. T. (1972). A general description of the Lambwe Valley area of South Nyanza District, Kenya. B. World Health Organ. 47, 691–697.
Ambrose, S. H., and Lorenz, K. G. (1990). “Social and ecological models for the Middle Stone Age in southern Africa,” in The Emergence of Modern Humans: An Archaeological Perspective, ed P. Mellars (Edinburgh: Edinburgh University Press).
Anderson, T., Hopcraft, J., Eby, S., Ritchie, M., Grace, J., and Olff, H. (2010). Landscape-scale analyses suggest both nutrient and antipredator advantages to Serengeti herbivore hotspots. Ecology 91, 1519–1529. doi: 10.1890/09-0739.1
Balasse, M., Bocherens, H., Mariotti, A., and Ambrose, S. (2001). Detection of dietary changes by intra-tooth carbon and nitrogen isotopic analysis: an experimental study of dentine collagen of cattle (Bos taurus). J. Archaeol. Sci. 28, 235–245. doi: 10.1006/jasc.1999.0535
Bataille, C., Crowley, B., Wooller, M., and Bowen, G. (2020). Advances in global bioavailable strontium isoscapes. Palaeogeogr. Palaeocl. 555, 49. doi: 10.1016/j.palaeo.2020.109849
Bataille, C., Von Holstein, I., Laffoon, J., Willmes, M., Liu, X., and Davies, G. (2018). A bioavailable strontium isoscape for Western Europe: a machine learning approach. PLOS ONE 13, 7386. doi: 10.1371/journal.pone.0197386
Beverly, E., Driese, S. G., Peppe, D. J., Arellano, L. N., Blegen, N., Faith, J. T., et al. (2015). Reconstruction of a semi-arid Late Pleistocene paleocatena from the Lake Victoria region, Kenya. Quaternary Res. 84, 368–381. doi: 10.1016/j.yqres.2015.08.002
Beverly, E. J., White, J. D., Peppe, D. J., Faith, J. T., Blegen, N., and Tryon, C. A. (2020). Rapid Pleistocene desiccation and the future of Africa's Lake Victoria. Earth Planet Sc. Lett. 530, 115883. doi: 10.1016/j.epsl.2019.115883
Blumenthal, S., Cerling, T., Smiley, T., Badgley, C., and Plummer, T. (2019). Isotopic records of climate seasonality in equid teeth. Geochim. et Cosmochim. Ac. 260, 329–348. doi: 10.1016/j.gca.2019.06.037
Blumenthal, S. A., Levin, N. E., Brown, F. H., Brugal, J.-P., Chritz, K. L., Harris, J. M., et al. (2017). Aridity and hominin environments. P. Natl. Acad. Sci. USA 114, 7331–7336. doi: 10.1073/pnas.1700597114
Burgess, N. D., Hales, J. D., Underwood, E., Dinerstein, E., Olson, D., Itoua, I., et al. (2004). Terrestrial Ecoregions of Africa and Madagascar: A Continental Assessment. Washington, DC: Island Press.
Cerling, T. E., Andanje, S. A., Blumenthal, S. A., Brown, F. H., Chritz, K. L., Harris, J. M., et al. (2015). Dietary changes of large herbivores in the Turkana Basin, Kenya from 4 to 1 Ma. P. Natl. Acad. Sci. USA 112, 11467–11472. doi: 10.1073/pnas.1513075112
Chritz, K., Cerling, T., Freeman, K., Hildebrand, E., Janzen, A., and Prendergast, M. (2019). Climate, ecology, and the spread of herding in eastern Africa. Quaternary Sci. Rev. 204, 119–132. doi: 10.1016/j.quascirev.2018.11.029
Codron, D., Codron, J., Lee-Thorp, J. A., Sponheimer, M., De Ruiter, D., Sealy, J., et al. (2007). Diets of savanna ungulates from stable carbon isotope composition of faeces. J. Zool. 273, 21–29. doi: 10.1111/j.1469-7998.2007.00292.x
Copeland, S. R., Cawthra, H. C., Fisher, E. C., Lee-Thorp, J., Cowling, R. M., Le Roux, P. J., et al. (2016). Strontium isotope investigation of ungulate movement patterns on the Pleistocene Paleo-Agulhas Plain of the Greater Cape Floristic Region, South Africa. Quaternary Sci. Rev. 141, 65–84. doi: 10.1016/j.quascirev.2016.04.002
Estes, R. D. (1991). The Behavior Guide to African Mammals. Los Angeles, CA: University of California Press.
Faith, J., Potts, R., Plummer, T., Bishop, L., Marean, C., and Tryon, C. (2012). New perspectives on Middle Pleistocene change in the large mammal faunas of East Africa: Damaliscus hypsodon sp nov (Mammalia, Artiodactyla) from Lainyamok, Kenya. Palaeogeogr. Palaeocl. 361, 84–93. doi: 10.1016/j.palaeo.2012.08.005
Faith, J. T. (2014). Late Pleistocene and Holocene mammal extinctions on continental Africa. Earth Sci. Rev. 128, 105–121. doi: 10.1016/j.earscirev.2013.10.009
Faith, J. T., Choiniere, J. N., Tryon, C. A., Peppe, D. J., and Fox, D. L. (2011). Taxonomic status and paleoecology of Rusingoryx atopocranion (Mammalia, Artiodactyla), an extinct Pleistocene bovid from Rusinga Island, Kenya. Quaternary Res. 75, 697–707. doi: 10.1016/j.yqres.2010.11.006
Faith, J. T., Patterson, D. B., Blegen, N., O'Neill, C. J., Marean, C. W., Peppe, D. J., et al. (2016a). Size variation in Tachyoryctes splendens (East African mole-rat) and its implications for late Quaternary temperature change in equatorial East Africa. Quaternary Sci. Rev. 140, 39–48. doi: 10.1016/j.quascirev.2016.03.017
Faith, J. T., Rowan, J., O'Brien, K., Blegen, N., and Peppe, D. J. (2020). Late Pleistocene mammals from Kibogo, Kenya: systematic paleontology, paleoenvironments, and non-analog associations. J. Vertebr. Paleontol. 40, E1841781. doi: 10.1080/02724634.2020.1841781
Faith, J. T., and Thompson, J. C. (2013). Fossil evidence for seasonal calving and migration of extinct blue antelope (Hippotragus leucophaeus) in southern Africa. J. Biogeogr. 40, 2108–2118. doi: 10.1111/jbi.12154
Faith, J. T., Tryon, C. A., and Peppe, D. J. (2016b). “Environmental change, ungulate biogeography, and their implications for early human dispersals in equatorial East Africa,” in Africa from MIS 6-2. Population Dynamics and Paleoenvironments, eds S. Jones and B. Stewart (Dordrecht: Springer), 233–245. doi: 10.1007/978-94-017-7520-5_13
Faith, J. T., Tryon, C. A., Peppe, D. J., Beverly, E. J., Blegen, N., Blumenthal, S., et al. (2015). Paleoenvironmental context of the Middle Stone Age record from Karungu, Lake Victoria Basin, Kenya, and its implications for human and faunal dispersals in East Africa. J. Hum. Evol. 83, 28–45. doi: 10.1016/j.jhevol.2015.03.004
Faith, J. T., Tryon, C. A., Peppe, D. J., and Fox, D. L. (2013). The fossil history of Grévy's zebra (Equus grevyi) in equatorial East Africa. J. Biogeogr. 40, 359–369. doi: 10.1111/j.1365-2699.2012.02796.x
Fryxell, J., Greever, J., and Sinclair, A. (1988). Why are migratory ungulates so abundant? Am. Nat. 131, 781–798. doi: 10.1086/284822
Garrett, N. D., Fox, D. L., Mcnulty, K. P., Faith, J. T., Peppe, D. J., Van Plantinga, A., et al. (2015). Stable isotope paleoecology of Late Pleistocene Middle Stone Age humans from equatorial East Africa, Lake Victoria Basin, Kenya. J. Hum. Evol. 82, 1–14. doi: 10.1016/j.jhevol.2014.10.005
Holdo, R. M., Holt, R. D., and Fryxell, J. M. (2009). Opposing rainfall and plant nutritional gradients best explain the wildebeest migration in the Serengeti. Am. Nat. 173, 431–445. doi: 10.1086/597229
Janzen, A., Bataille, C., Copeland, S., Quinn, R., Ambrose, S., Reed, D., et al. (2020). Spatial variation in bioavailable strontium isotope ratios (Sr-87/Sr-86) in Kenya and northern Tanzania: implications for ecology, paleoanthropology, and archaeology. Palaeogeogr. Palaeocl. 560, 957. doi: 10.1016/j.palaeo.2020.109957
Jenkins, K. E., Nightingale, S., Faith, J. T., Peppe, D. J., Michel, L. A., Driese, S. G., et al. (2017). Evaluating the potential for tactical hunting in the Middle Stone Age: insights from a bonebed of the extinct bovid, Rusingoryx atopocranion. J. Hum. Evol. 108, 72–91. doi: 10.1016/j.jhevol.2016.11.004
Kingdon, J., and Hoffman, M. (2013). Mammals of Africa. Volume VI: Pigs, Hippopotamuses, Chevrotain, Giraffes, Deer and Bovids. London: Bloomsbury Publishing.
Kovarovic, K., Faith, J., Jenkins, K., Tryon, C., and Peppe, D. (2021). Ecomorphology and ecology of the grassland specialist, Rusingoryx atopocranion (Artiodactyla: Bovidae), from the Late Pleistocene of western Kenya. Quaternary Res. 101, 187–204. doi: 10.1017/qua.2020.102
Lazzerini, N., Balter, V., Coulon, A., Tacail, T., Marchina, C., Lemoine, M., et al. (2021). Monthly mobility inferred from isoscapes and laser ablation strontium isotope ratios in caprine tooth enamel. Sci. Rep. UK 11, 2277. doi: 10.1038/s41598-021-81923-z
Mackey, G. N., and Fernandez, D. P. (2011). High Throughput Sr Isotope Analysis Using an Automated Column Chemistry System. San Francisco, CA: American Geophysical Union.
Marean, C. W. (1992). Implications of late Quaternary mammalian fauna from Lukenya Hill (south-central Kenya) for paleoenvironmental change and faunal extinctions. Quaternary Res. 37, 239–255. doi: 10.1016/0033-5894(92)90085-W
Marean, C. W. (1997). Hunter-gatherer foraging strategies in tropical grasslands: model building and testing in the East African Middle and Later Stone Age. J. Anthropol. Archaeol. 16, 189–225. doi: 10.1006/jaar.1997.0309
Milhaud, G., and Nezit, J. (1991). Molar development in sheep - morphology, radiography, microhardness. Recl. Med. Vet. 167, 121–127.
O'Brien, H. D., Faith, J. T., Jenkins, K. R., Peppe, D. J., Plummer, T. W., Jacobs, Z. L., et al. (2016). Unexpected convergent evolution of nasal domes between Pleistocene bovids and Cretaceous hadrosaur dinosaurs. Curr. Biol. 26, 503–508. doi: 10.1016/j.cub.2015.12.050
Osmaston, H. (1989). “Glaciers, glaciations and equilibrium line altitudes on Kilimanjaro,” in Quaternary and Environmental Research on East African Mountains, ed W. C. Mahaney (Rotterdam: Balkema), 7–30.
Robinson, J., Rowan, J., Barr, W., and Sponheimer, M. (2021). Intrataxonomic trends in herbivore enamel δ13C are decoupled from ecosystem woody cover. Nat. Ecol. Evol. 5, 995. doi: 10.1038/s41559-021-01455-7
Rowan, J., Locke, E. M., Robinson, J. R., Campisano, C. J., Wynn, J., and Reed, K. E. (2017). Fossil Giraffidae (Mammalia, Artiodactyla) from Lee Adoyta, Ledi-Geraru, and Late Pliocene dietary evolution in giraffids from the Lower Awash Valley, Ethiopia. J. Mamm. Evol. 24, 359–371. doi: 10.1007/s10914-016-9343-z
Staver, A., and Hempson, G. (2020). Seasonal dietary changes increase the abundances of savanna herbivore species. Sci. Adv. 6, 2848. doi: 10.1126/sciadv.abd2848
Steele, T. E. (2007). “Late Pleistocene of Africa,” in Encyclopedia of Quaternary Science, ed S. A. Elias (Amsterdam: Elsevier).
Tryon, C. A., Faith, J. T., Peppe, D. J., Beverly, E. J., Blegen, N., Blumenthal, S., et al. (2016). The Pleistocene prehistory of the Lake Victoria Basin. Q. Int. 404, Part B, 100-114. doi: 10.1016/j.quaint.2015.11.073
Tryon, C. A., Faith, J. T., Peppe, D. J., Fox, D. L., McNulty, K. P., Jenkins, K., et al. (2010). The Pleistocene archaeology and environments of the Wasiriya Beds, Rusinga Island, Kenya. J. Hum. Evol. 59, 657–671. doi: 10.1016/j.jhevol.2010.07.020
Tryon, C. A., Peppe, D. J., Faith, J. T., Van Plantinga, A., Nightengale, S., and Ogondo, J. (2012). Late Pleistocene artefacts and fauna from Rusinga and Mfangano Islands, Lake Victoria, Kenya. Azania Archaeol. Res. Afr. 47, 14–38. doi: 10.1080/0067270X.2011.647946
Tucker, L., Favreau, J., Itambu, M., Larter, F., Mollel, N., Mwambwiga, A., et al. (2020). Initial assessment of bioavailable strontium at Oldupai Gorge, Tanzania: potential for early mobility studies. J. Archaeol. Sci. 114, 254. doi: 10.1016/j.jas.2019.105066
Yang, D., Uno, K., Souron, A., Mcgrath, K., Pubert, E., and Cerling, T. (2020). Intra-tooth stable isotope profiles in warthog canines and third molars: implications for paleoenvironmental reconstructions. Chem. Geol. 554, 499. doi: 10.1016/j.chemgeo.2020.119799
Keywords: carbon, Kenya, seasonality, stable isotopes, strontium
Citation: O'Brien K, Podkovyroff K, Fernandez DP, Tryon CA, Ashioya L and Faith JT (2023) Migratory behavior in the enigmatic Late Pleistocene bovid Rusingoryx atopocranion. Front. Environ. Archaeol. 2:1237714. doi: 10.3389/fearc.2023.1237714
Received: 09 June 2023; Accepted: 07 July 2023;
Published: 01 August 2023.
Edited by:
Sally Christine Reynolds, Bournemouth University, United KingdomReviewed by:
Noel Amano, Max Planck Institute of Geoanthropology, GermanyEmma Loftus, Ludwig Maximilian University of Munich, Germany
Copyright © 2023 O'Brien, Podkovyroff, Fernandez, Tryon, Ashioya and Faith. This is an open-access article distributed under the terms of the Creative Commons Attribution License (CC BY). The use, distribution or reproduction in other forums is permitted, provided the original author(s) and the copyright owner(s) are credited and that the original publication in this journal is cited, in accordance with accepted academic practice. No use, distribution or reproduction is permitted which does not comply with these terms.
*Correspondence: Kaedan O'Brien, kaedan.obrien@anthro.utah.edu