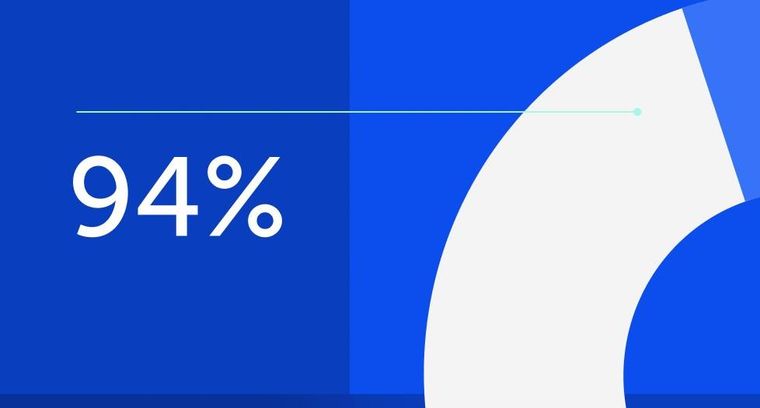
94% of researchers rate our articles as excellent or good
Learn more about the work of our research integrity team to safeguard the quality of each article we publish.
Find out more
ORIGINAL RESEARCH article
Front. Environ. Archaeol., 26 April 2023
Sec. Zooarchaeology
Volume 2 - 2023 | https://doi.org/10.3389/fearc.2023.1145220
This article is part of the Research TopicEditor's Showcase: ZooarchaeologyView all 4 articles
For millennia, Inuit peoples of the Arctic and Subarctic have been challenged by the impacts of climate change on the abundance of key subsistence species. Responses to climate-induced declines in animal populations included switching to alternative food sources and/or migrating to regions of greater availability. We examine these dynamics for the Chugach Inuit (Sugpiat) people of southern coastal Alaska by synthesizing a large body of evidence from archeological sites, including radiocarbon dates and archaeofaunal assemblages, and by applying contemporary knowledge of glaciomarine ecosystems, spatial patterns of resource richness, and ocean-climate induced regime shifts in the Gulf of Alaska. We hypothesize that Chugach groups migrated from Cook Inlet and Prince William Sound to the Kenai Peninsula during periods of low sea surface temperatures (SSTs) to harvest harbor seals, which were seasonally aggregated near tidewater glaciers during pupping season, as well as piscivorous seabirds, Pacific cod, and other species that thrive under cool ocean conditions. During warming phases, the Chugach returned to Cook Inlet and Prince William Sound to fish for salmon and other species that abound during higher SSTs. Drivers of this coupled human-natural system of repeated (pulse) migration include the Pacific Decadal Oscillation (PDO), the dominant pattern of sea surface temperatures in the North Pacific that has been shown to generate step-like regime shifts in the marine food web; and coastal glaciers that structure the functioning of fiord ecosystems and support high levels of biological productivity. The culturally-constructed Chugach niche in the glaciomarine habitat of the Gulf of Alaska was based on intergenerationally transmitted ecological knowledge that enabled a resilient, mobile response to climate and resource variation.
Abrupt migrations and regional abandonments have occurred throughout Arctic and Subarctic culture history, and while long attributed primarily to climate change, recent perspectives from cultural ecology and coupled human-natural systems research foreground Inuit knowledge and agency in initiating these events (Desjardins and Jordan, 2019; Fitzhugh et al., 2019; Desjardins, 2020; Friesen et al., 2020). Holocene climatic variation and core characteristics of high latitude ecosystems including short food chains, geographic heterogeneity, and rapid cycles of animal abundance and decline necessitated modes of Inuit adaptation that were flexible, resilient, and mobile (Krupnik and Crowell, 2020). Thus, Late Dorset (Paleo-Inuit) populations migrated in 800–1000 CE from Foxe Basin to Ellesmere Island, Victoria Island, and Labrador, coinciding with elevated temperatures of the Medieval Warm Period and range shifts in walrus and caribou populations (Friesen et al., 2020). Thule Inuit migrated from Alaska across Canada to Greenland ca. 1250 CE during the early Little Ice Age when sea ice was increasing but bowhead whales—a mainstay of the Thule economy—were abundant in the Eastern Arctic (Mathiassen, 1927; Friesen and Arnold, 2008; Friesen, 2016; Mason, 2020). As temperatures further declined and sea ice blocked whaling, Thule groups turned to caribou, muskoxen, char, and ringed seals taken at their breathing holes or settled around open-water polynyas where walrus were available (Maxwell, 1985; Desjardins, 2020).
These examples illustrate a critical choice that confronted northern human foragers during periods of climate change—whether to remain in place and “prey-switch” to alternative food species, or to migrate where key animals were becoming more abundant. The latter choice, when repeated, is pulse migration—successive movements into and away from a region in phase with climatic and resource cycles, often mediated by interaction with other Indigenous groups or in later times with colonial settlers, whalers, and traders (Hastrup et al., 2018a; Fitzhugh, 2019). For instance, in Northwest Greenland, cyclical Inuit migrations and shifts in subsistence strategies were governed by centennial and decadal climate fluxes affecting the North Water, a polynya between Ellesmere Island and Northwest Greenland (Vibe, 1967; Grønnow, 2017; Hastrup et al., 2018b; Ribeiro et al., 2021). On the coast of Labrador, ancestral Inuit populations expanded south during cold North Atlantic phases to follow harp seals at the sea ice margin, retreating north during warmer periods (Fitzhugh, 1972, 1977, 2020). On the Melville Peninsula, Thule populations moved between maritime and continental environmental zones to maximize access to resources during climate shifts (Finkelstein et al., 2009).
Herein we present a previously unidentified example of pulse migration in the western Subarctic—Chugach (Sugpiat) territorial expansion and contraction on the Gulf of Alaska coast linked with interdecadal to centennial-scale climate variability. The Chugach are a southern Alaska Native people of Inuit ancestry whose homeland includes Kachemak Bay in lower Cook Inlet, the Kenai Peninsula, and Prince William Sound (Crowell et al., 2001) (Figure 1). Today about 1,200 Chugach tribal members reside in the communities of Nanwalek, Port Graham, Seldovia, Chenega Bay, Tatitlek, Valdez, and Cordova, engaging in subsistence economies based on over 50 varieties of marine and terrestrial foods (Stanek, 1985; Stratton and Chisum, 1986; Fall, 1991). Subsistence practices have varied over the last 5,000 years or more, focusing on different suites of coastal fauna (De Laguna, 1956, 1975; Yesner, 1992; Workman, 1996, 1998; Yarborough and Yarborough, 1998; Yarborough, 2000).
Figure 1. The Chugach region of southcentral Alaska showing topography, archaeological sites, contemporary Chugach communities, and migration routes (blue arrows) linking Kachemak Bay, the Kenai Coast, and Prince William Sound. Base map from National Geographic MapMaker (c) National Geographic Society.
We present new field data and an extensive compilation of radiocarbon dates to document Chugach migration to the Gulf of Alaska coast of the Kenai Peninsula (hereafter, the Kenai Coast) from Kachemak Bay and Prince William Sound at various times during the Late Neoglacial Period (0–900 CE), Medieval Warm Period (900–1100 CE), and Little Ice Age (1100–1900 CE) and synthesize multidisciplinary data from published or otherwise publicly available sources to assess climatic variation and changes in marine biota as potential drivers of these population movements. We hypothesize that during intervals of low sea surface temperatures (SSTs) Chugach populations relocated to Kenai Coast villages to subsist on harbor seals, Steller sea lions, murres, puffins, Pacific cod, and other fauna that thrive under cool ocean conditions, and that they returned to Kachemak Bay and Prince William Sound during warm cycles when these species decline and salmon increase along with halibut and other high-value fish. Other potential drivers of these movements include cataclysmic natural events (volcanism and earthquakes) and interaction with Russian and American trading companies. We conclude that Chugach pulse migration was a mobile, resilient response to climatic and environmental change and was a product of human niche construction (HNC), based on the intergenerational transmission of ecological and historical knowledge (Laland and O'Brien, 2010).
To validate the climate-coupled pulse migration model, we characterize differential access to marine food resources in the Chugach region. Crowell et al. (2012) developed a GIS model to examine the spatial correlation of archaeological sites and modern harvest locales for 23 subsistence species in the central Gulf of Alaska from the Alaska Peninsula to Prince William Sound, including Kodiak Island (Table 1). The study area encompassed 17,000 km of shoreline divided for the analysis into 6,800 2.5 km-long segments on which there are 1,959 known Indigenous archaeological villages and camps. Sites in the sample dated to the Ocean Bay (5500–1500 BCE), Kachemak/Palugvik (1500 BCE−1100 CE), and Koniag/Chugach (1100 CE–present) cultural phases (Clark, 1984; Mills, 1994; Reger and Boraas, 1996). Numbers of harvest locales accessible from each segment were computed using GIS buffers. Locales traditionally accessed by kayak such as harbor seal and sea lion haulouts, bird rookeries, and pelagic fishing areas were enumerated within a 10-km radius from any point on the segment, while anadromous fish streams were counted within a 1-km radius, reflecting the cultural pattern of establishing fishing camps at those locations. Resource density or “richness” scores—the sum of accessible harvest locales for all species—were computed for each segment. The 23 resource variables were reduced to six factors explaining 65% of the total resource variation at the 6,800 segments and were highly predictive of site density.
Table 1. Layers and source data for GIS analysis of archaeological sites and subsistence resources in the Gulf of Alaska (Crowell et al., 2012).
To identify spatial patterns of species richness in the northern Gulf of Alaska, we summarize data on birds and marine mammals, including 33,557 transect segments sampled between 1975–2022 in the North Pacific Pelagic Seabird Database (Drew et al., 2005) and benthic fish in 2,883 bottom trawls sampled between 1984–2019 during NOAA Alaska Fisheries Science Center groundfish bottom trawl survey (https://www.fisheries.noaa.gov/alaska/commercial-fishing/alaska-groundfish-bottom-trawl-survey-data, accessed on 12/28/2022). To accommodate plotting of indices on the same scale, for each sample and taxa group we normalize the index by subtracting the mean and dividing by the standard deviation. For each taxon, we summarize the mean species index anomalies within 25 × 25 km hexagonal cells overlaid across the extent of the samples.
To infer the influence of changes in climate conditions on past human use of marine resources, we examine cool and warm periods identified by Pacific Decadal Oscillation (PDO) reconstructions (Gedalof and Smith, 2001) and proxy studies of air temperatures (Wiles et al., 2014) and water temperatures (Praetorius et al., 2015; Helser et al., 2018). The PDO is the dominant pattern of sea surface temperature in the North Pacific (Mantua et al., 1997), whereby strong ocean-atmospheric covariation results in positive (warm) and negative (cool) phases accompanied by step-like alternations in the predominance of various species in the marine food web (Anderson and Piatt, 1999; Benson and Trites, 2002; Overland et al., 2008; Rogers et al., 2013; Litzow and Mueter, 2014). Similar large amplitude trends in the abundance of faunal classes attend centennial-scale climatic phases (Finney et al., 2002; Maschner et al., 2008; Fitzhugh et al., 2022). Due to known variability in marine community response to the PDO over time (Litzow et al., 2019), we used local SST and air temperature reconstructions for their relevance to conditions in the Gulf of Alaska.
To document pulse migrations of ancestral Chugach populations of Kachemak Bay and Prince William Sound to the Kenai Coast during cooler climatic intervals and away from it during warmer intervals we compare climate data and radiocarbon dates from the three areas, dendrochronologically calibrated using the Oxford University OxCal program (Version 4.4, https://c14.arch.ox.ac.uk/oxcal.html; Supplementary Table S1). Dates are presented with two standard deviation error ranges (totaling 95.4% probability) and median intercept dates. All dates are on wood charcoal. Species identifications have been reported for < 5 samples, posing the risk of overestimating age by dating charcoal from the inner growth rings of long-lived species such as Sitka spruce (Picea sitchensis).
We interpret temporal gaps in dates as evidence of abandonment and population movement between areas, defining gaps as intervals of at least 100 years with ≤ 1 median intercepts. All known (published and unpublished) dates from the region with median calibrated ages of 2,000 years or less (n = 172) are included (Workman, 1977; Workman et al., 1980, 1993, 1998; McMahan and Holmes, 1987; Dotter, 1988; Workman and Workman, 1988; Boraas and Klein, 1989, 1992; Haggarty et al., 1991; Reger et al., 1991; Yarborough, 1992, 1997; Dekin et al., 1993; Yarborough and Yarborough, 1996; Martin, 2004). We also interpret apparent population shifts between the three study areas by using numbers of radiocarbon dates as a coarse population proxy and comparing those data to estimated air temperatures for each calendar year from 800 CE to 2010 CE (Wiles et al., 2014). We use cross-correlation to statistically test the strength of this relationship and to account for time lags between climate signals and population movements.
To ground truth our model of Chugach migration and resource use on the Kenai Coast we present results of field studies of the glaciomarine ecology (Arimitsu et al., 2010, 2012) and archaeology (Crowell and Mann, 1998; Crowell et al., 2008) of two glacial fiords, Harris Bay and Aialik Bay, in Kenai Fjords National Park. We describe the ecosystem structure of the fiords based on oceanographic surveys, chlorophyll-a reflectance measurements, acoustic-trawl surveys for zooplankton and forage fish, and surveys of marine birds and mammals, demonstrating the influence of glacial mineral nutrients on primary productivity and the marine food web. Archaeological results including site locations, radiocarbon dates, structures, and artifact assemblages are described for four Chugach villages that were occupied intermittently during cold climate phases of the 12th through 19th centuries. Archaeofaunal assemblages are taxonomically identified and quantified by number of identified specimens (NISP) (Yarborough, 1998; Yesner, 2004, 2010; Hanson, 2007, 2009). We compare taxonomic frequencies to ecosystem proportionalities that characterized PDO phases of the 20th century and to subsistence species harvested by Chugach communities after a 1976 warm shift in the PDO.
The project reflects Chugach community engagement and collaborative co-production of knowledge. In cooperation with the tribal councils of Nanwalek, Port Graham, and Seldovia, residents of those communities were invited to participate in the Smithsonian Arctic Studies Center's Kenai Fjords Oral History and Archaeology Project (2002–2004, 2015–2017), and their contributions have deeply informed the present paper. Elders shared stories about grandparents and great-grandparents who lived or were born at such locations as Nuchek and Chenega in Prince William Sound and Aialik Bay, Nuka Bay, and other locations on the Kenai Coast. Interviews are archived at the Pratt Museum (Homer) and excerpted in the film “Archaeology and Memory: Ancestral Alutiiq Villages of the Outer Kenai Coast, Alaska” (Smithsonian Institution and Pratt Museum, 2003). College students, adults, and high school students from the Kachemak Bay villages participated in four seasons of fieldwork in Aialik Bay and Nuka Bay as paid technicians, advisors, and interns. Chugach elders visited research locations in Aialik Bay and provided on-site interpretation of artifacts, cultural features, and local ecology. Reports and publications have been shared with the communities (e.g., Crowell et al., 2001, 2008; Crowell, 2006, 2010; Maio et al., 2019) and numerous project presentations have been made to local audiences, tribal councils, and school classes in the participating villages. Cultural resource management discussions have been held with the Chugach Alaska Corporation and the English Bay (Nanwalek) and Port Graham village corporations, hosted by the National Park Service.
The coastline of the central Gulf of Alaska is deeply indented by Cook Inlet and Prince William Sound, large embayments formed by Pleistocene ice cap expansion and separated by the Kenai Peninsula (Mann et al., 1998). Kachemak Bay, also of glacial origin, adjoins lower Cook Inlet. The Chugach Mountains rise behind Prince William Sound and the Kenai Mountains extend along the spine of the Kenai Peninsula, both capped with ice fields from which glaciers descend toward the coast. The Gulf of Alaska does not develop seasonal sea ice. The westward-flowing Alaska Current follows the continental shelf break and the Alaska Coastal Current, propelled by large volumes of fresh water from rivers and glaciers, flows along the mainland coast and curls into lower Cook Inlet (Stabeno et al., 2016). Ocean transport is characterized by persistent downwelling except at the mouth of Cook Inlet where strong tidal currents intersect the Alaska Coastal Current, generating upwelling that brings deep nutrient-rich waters to the surface (Drew and Piatt, 2002; Piatt and Springer, 2007). Storms, high tidal energies, and the fast-flowing Alaska Coastal Current enhance vertical mixing along other parts of the coastal shelf (Weingartner, 2007).
Minerals eroded by glaciers from mountain bedrock including iron, inorganic nitrogen, phosphate, and silica are transported to the ocean by meltwater streams, calved ice, and dust storms, that along with marine-derived nutrients fuel plankton blooms, contribute to high and sustained biological productivity in coastal fiords and on the continental shelf, and support a vast trophic web of fish, seabirds, and marine mammals (Parsons, 1986; Syvitski et al., 1987; Childers et al., 2005; Spies, 2007; Hood et al., 2009; Arimitsu et al., 2010, 2012, 2016, 2018; Crusius et al., 2011). Iron-rich glaciogenic dust from the Chugach region is thought to be a significant source of iron fueling primary production in the Gulf of Alaska (Muhs et al., 2013).
Cook Inlet receives sediment from watersheds draining Susitna, Matanuska, Knik, and Tustumena glaciers and from Grewingk and other glaciers on the south shore of Kachemak Bay, generating primary production of up to 300 g C/m2 per year (Larrance et al., 1977; Sambrotto and Lorenzen, 1986; Cooney, 2007). Resurrection Bay on the outer Kenai coast, charged with glacial sediments carried by the Resurrection River, has estimated primary production of 200 g C/m2 per year (Heggie et al., 1977; Sambrotto and Lorenzen, 1986). Prince William Sound receives inputs from Columbia, Harriman, Yale, Harvard, Meares, and other glaciers along its northern and northwestern coasts and has an average production of 185 g C/m2 per year (Goering et al., 1973; Eslinger et al., 2001). Values are lower in the oceanic GOA beyond the shelf, estimated at < 100 g C/m2 per year (Sambrotto and Lorenzen, 1986). Zooplankton biomass including copepods (small crustaceans), euphausiids (krill), hyperiids (amphipods), and pteropods (pelagic snails) declines with distance from the Gulf of Alaska coast, with oceanic values of 1.5–30 g/m2 in summer compared to 60 g/m2 on the continental shelf (Cooney, 1986; Batten et al., 2018).
The Gulf of Alaska provides habitat for at least 287 species of fish, 147 species of birds, and 26 species of marine mammals (Hood and Zimmerman, 1986; Cooney, 2007; Iverson et al., 2007). Important commercial and subsistence fish include sockeye salmon (Oncorrhynchus nerka), pink salmon (Oncorrhynchus gorbuscha), Coho salmon (Oncorrhynchus kisutch), Chinook salmon (Oncorrhynchus tshawytscha), Pacific herring (Hippoglossus stenolepsis), Pacific cod (Gadus macrocephalus), halibut (H. stenolepsis), rockfish (Sebastes spp.), and lingcod (Ophiodon elongatus). Salmon spawn in over 300 coastal watersheds in the study area and there are large commercial salmon fisheries in lower Cook Inlet and Prince William Sound (Alaska Department of Fish and Game, 2022). Most of the Kenai Coast is relatively poor in this resource, dominated by small salmon runs in coastal streams. Abundant seabirds include common murres (Uria aalge), tufted puffins (Fratercula cirrhata), and black-legged kittiwakes (Rissa tridactyla) which have breeding colonies in Kachemak Bay, the Barren Islands, Rocky Bay, the Pye Islands near Nuka Bay, the Chiswell Islands off Aialik Bay, Cape Resurrection, and Prince William Sound (Bailey, 1977; Arimitsu et al., 2021; Alaska Department of Environmental Conservation, 2022).
The marine mammals most important to present-day Chugach hunters are harbor seals (Phoca vitulina) and Steller sea lions (Eumetopias jubatus). Both have undergone severe population declines since the 1970s. Sea lions, with a current estimated western Alaska population of 50,000, are most abundant on the Kenai Coast where they maintain 16 breeding rookeries (Muto et al., 2021). About 69,000 harbor seals utilize scores of terrestrial haulouts for resting, breeding, and pupping (London et al., 2015; Muto et al., 2021) and about 10% rear their pups on ice floes adjacent to tidewater glaciers including rookeries in McCarty Fiord, Northwestern Lagoon, and Aialik Bay on the Kenai coast and College Fjord and Columbia Glacier in Prince William Sound (Hoover-Miller, 1994; Bengtson et al., 2007; Hoover-Miller et al., 2011; Hoover-Miller and Armato, 2018). In the past, Chugach hunters also took harbor porpoises (Phocoena phocoena) and Dall porpoises (Phocoenoides dalli), both common throughout the region (Muto et al., 2021).
Clustering of precontact archaeological sites in areas of high resource density has been demonstrated in several Gulf of Alaska regions (Mobley et al., 1990; Erlandson et al., 1992; Crowell and Mann, 1998; Crowell et al., 2003). Results of a GIS-based statistical analysis of archeological site locations and local availability of 23 marine subsistence species (Crowell et al., 2012) found that resource richness (numbers of harvesting locales for all species) was highest in the outer portions of fiords, where pelagic and benthic fish, seabirds, and marine mammals are typically more abundant, and lowest in the inner reaches of fiords where glacially fed salmon rivers are often the principal resource, augmented at some locations by harbor seal haulouts and seabird colonies (Figure 2A). The pattern is multiscalar, applying to Cook Inlet, Prince William Sound, and Kachemak Bay as well as smaller embayments, likely reflecting the influence of glacial sediment plumes that enter at the heads of fiords but have maximum impact on marine food webs in more distal areas (Arimitsu et al., 2012, 2016). The regionally highest values are in the Cook Inlet upwelling zone including the Kenai Coast, which is consistent with oceanic mixing of glacial and marine-derived nutrients and low turbidity–high salinity conditions that lead to abundant and energetically rich forage communities in this area (Abookire and Piatt, 2005; Speckman et al., 2005).
Figure 2. Results of GIS subsistence resource analysis (Crowell et al., 2012) showing: (A) overall richness scores; (B) Factor 1 dominated by sea lion, herring, cod, halibut, fin whale, and Dall porpoise; (C) Factor 4 dominated by sockeye, coho, and king salmon, steelhead trout; and (D) Factor 6, harbor seal, seabirds, beluga whale.
Archaeological site frequency was highly correlated with resource richness values. Out of 856 coastal segments where sites were present, 776 (90.6%) had richness scores of 10 or greater (Chi square p ≤ 0.0001). All archaeological sites were located on only 13% of the shoreline segments, demonstrating clustering in resource-rich areas, while 87% contained no settlements. The Kenai Coast has some of the highest richness scores in the Chugach region (Figure 2A), although there are fewer known coastal sites than in Kachemak Bay or Prince William Sound due to loss of older sites through tectonic submergence and erosion (Crowell and Mann, 1996, 1998).
Multivariate analysis reduced the 23 resource variables to six factors with Eigen values >1.0 (Table 2). A generalized linear model was fitted with site count as a response to these six factors as explanatory variables. Factors 1 (p = < 0.0001), 4 (p = < 0.0001) and 6 (p = 0.0163) had significant influences on site count per segment. On Factor 1 (dominated by sea lion, herring, cod, halibut, fin whale, and Dall porpoise) Kachemak Bay and the Kenai Coast greatly exceed Prince William Sound (Figure 2B); on Factor 4 (sockeye, coho, and king salmon, steelhead) the Kenai Coast is similar to Kachemak Bay and Prince William Sound, although this parity reflects the number of anadromous streams in each area rather than the size of salmon stocks, which are far higher in Cook Inlet and Prince William Sound (Figure 2C). Factor 6 (harbor seal, seabirds, beluga whale) is consistently highest on the Kenai Coast (Figure 2D).
Spatial patterns of marine mammals, seabirds, and benthic fish in the region are consistent with patterns of resource use inferred from archeological sites, with above-average species indices of all three taxa along much of the Kenai Coast (Figure 3). Taken together, these regional resource comparisons suggest that the Kenai Coast would have been most attractive for human settlement when lower water temperatures boosted its exceptionally rich marine mammal, seabird, and benthic fish stocks. The area was abandoned during periods of warmer SSTs that would have brought declines in those resources but increases to salmon in Cook Inlet and Prince William Sound.
Figure 3. Map of the average species richness index (color, standardized anomalies) of samples within 25 × 25 km hexagonal cells for each taxa grouping. Benthic fish data from groundfish bottom trawl surveys 1984–2019 were used with permission NOAA Alaska Fisheries Science Center, and marine bird and mammal species richness was summarized from the USGS North Pacific Pelagic Seabird Database (Drew et al., 2005).
Growth rings of coastal trees provide a proxy record of climatic flux in the Gulf of Alaska (Barclay et al., 1999). Based on 1,200 years of data from living and subfossil mountain hemlock trees, the Medieval Warm Period (900–1100 CE) peaked in 950 CE and was characterized by an average air temperature (February to August) of 7.7°C (Wiles et al., 2014) (Figure 4). During the Little Ice Age (1100–1900 CE), cooling periods separated by warmer intervals occurred from the 1180s through the 1320s when the average air temperature was 7°C; from 1400s to 1530s when it dipped to 6.7°C; from the 1540s to 1710s when it was 6.8°C; and from the 1810s to 1880s when it was 6.9°C (Wiles et al., 2014). The anthropogenic modern warm period is evident in tree rings after 1900 CE.
Figure 4. Major climatic phases including the Late Neoglacial Period (LNGP), Medieval Warm Period (MWP), Little Ice Age (LIA), and Modern Warm Period (MOD); estimated Gulf of Alaska (GOA) air temperatures since 800 CE from tree ring widths (Wiles et al., 2014); and estimated GOA air temperatures since 1600 CE from tree ring widths with inferred PDO phases (Gedalof and Smith, 2001).
Tree ring chronologies suggest correlation of air temperatures with the PDO index, which together with the 2–3 year El Niño-Southern Oscillation (ENSO) accounts for much of the multiyear variation in the longer climate curve (Wiles et al., 1998; Wilson et al., 2007). The PDO indexes fluctuation in the strength of the cyclonic Aleutian Low, which in positive phases advects warm air and water from subtropical regions northward to the Gulf of Alaska and during negative phases allows a return to cooler air and ocean temperatures (Bond and Harrison, 2000; Mantua and Hare, 2002). Gedalof and Smith (2001) used tree ring studies at six coastal locations from Oregon to Alaska to identify punctuated shifts in air temperatures since 1600 CE that correlate with instrumentally measured changes in PDO state during the 20th century and indicate similar step-like changes during earlier centuries (Figure 4). Wiles et al. (1998) obtained similar results from tree ring chronologies at eight locations on the Kenai Peninsula and Prince William Sound. Both studies indicate negative PDO phases with cooler air temperatures during ~1662–1680, 1696–1712, 1734–1758, 1798–1816, 1840–1923, and 1946–1976 CE. Although poor correspondence among multiple proxy records suggest lower variability of the PDO index during much of the 19th century (Gedalof et al., 2002; Newman et al., 2016), these temperature reconstructions were chosen because they reflect local variation in SSTs in our study area even during periods of lower variability in the PDO index.
Sea surface temperatures along the Alaskan coast are broadly correlated with air temperatures (Wiles et al., 1998; Hare and Mantua, 2000; Danielson et al., 2022). On a millennial timescale, paleotemperatures estimated from alkenones in a Gulf of Alaska bottom core indicated SSTs in the range of 9.8°C in ~0 CE at the end of the Neoglacial Period, rising to 11°C by ~1000 CE during the Medieval Warm Period (Praetorius et al., 2015).
SSTs during the Little Ice Age prior to instrumental records have been estimated from δ18O isotope values in archaeological otoliths of Pacific cod (Helser et al., 2018). Ion microprobe sampling of the core (early life) sections of otoliths recovered at two Aialik Bay sites discussed in this paper—the Early Contact Village, occupied during the 1798–1816 CE cool PDO phase and the Ayalik (Denton) site, occupied during the 1840–1923 CE cool PDO phase—indicate that SSTs in near-shore areas inhabited by juvenile cod averaged about 8.3°C in ~1800 CE, 9.1°C in ~1880 CE, and 11.7°C in 2004. Conversion of the aragonite δ18O to water temperature was calibrated from otoliths in living Aialik Bay cod fitted with electronic tags to measure in situ depths and water temperatures during 1–2 years of free swimming, and derived temperatures were compared to late 20th–early 21st century data from the GAK1 oceanographic buoy in Aialik Bay (Helser et al., 2018). Pacific cod otoliths from the 500-year-old Karluk-1 site on Kodiak Island showed high δ18O values that correspond with low SSTs around 1420 CE and 1800 CE (West et al., 2012).
The reorganization of Northeast Pacific biota that accompanied ocean warming after the 1976 PDO regime shift is widely recognized (Francis and Hare, 1994; McGowan et al., 1998; Anderson and Piatt, 1999; Hare and Mantua, 2000; Benson and Trites, 2002; Mantua and Hare, 2002; Litzow and Mueter, 2014). Prior to the regime shift commercial fish catch data showed an abundance of shrimp but depressed numbers of salmon, halibut, ocean perch, Pacific cod, arrowtooth flounder, and herring (Hare and Mantua, 2000). Ocean warming that began in the winter of 1976–1977 resulted in increased phytoplankton and zooplankton production; increased production of pelagic and demersal predatory fish such as salmon (particularly sockeye and pink), walleye pollock, Pacific cod, hake, Pacific ocean perch, sablefish, arrowtooth flounder, herring, and halibut; declines of key forage species including shrimp, capelin, and sand lance; declines in piscivorous seabirds including murres and kittiwakes; and declines in marine mammals including Steller sea lions, northern fur seals, and harbor seals, due in part to limitations of prey (especially capelin) that were central to their diets prior to 1976. For some species, including salmon (Chinook, chum, pink) and groundfish, these trends reversed with the temporary return of cooler waters in 1989 (Hare and Mantua, 2000).
Paleoenvironmental and archaeofaunal records track trends in fish abundance over longer time periods and greater temperature differentials. A 2,200-year sediment core analyzed for marine-derived δ15N isotope concentrations deposited by spawning sockeye salmon in Karluk Lake on Kodiak Island showed depressed salmon production around 130 CE in the Late Neoglacial Period, a steady upward trend through the Medieval Warm Period to a peak around 1200 CE in the early Little Ice Age, and a gradual decline to a low point around 1820 CE (Finney et al., 2002). Sockeye salmon trends over the last 300 years were studied using the same methods at other lakes on Kodiak Island and the Alaska Peninsula, demonstrating fluctuations that correlated with PDO phases (Finney et al., 2000).
The Pacific cod population paralleled salmon trends during 20th century PDO cycles but varied in the opposite direction during larger temperature anomalies of earlier centuries. On Sanak Island in the western Gulf of Alaska, Pacific cod were the dominant species harvested at eight Neoglacial archaeological sites from 2550 BCE through 520 CE, declined sharply relative to other species (salmon, flatfish, greenlings, sculpins) during the Medieval Warm Period, and increased again during the Little Ice Age (Maschner et al., 2008). Pacific cod and other marine species exceeded salmon in some Little Ice Age stratigraphic levels at Karluk-1 on Kodiak Island despite the site's location at the mouth of one of the region's most productive salmon rivers, suggesting that cod remained available in high numbers throughout the Little Ice Age even as salmon declined (West et al., 2012). At Palugvik and other archaeological sites in Prince William Sound, percentages of Pacific cod in archaeofaunal samples increased and salmon decreased with the onset of the Little Ice Age around 1100 CE (Yarborough, 2000).
While archaeological sequences in Kachemak Bay and Prince William Sound extend back to before 3000 BCE, the Kenai Coast record was degraded by 2 m or more of subsidence during each of two megathrust earthquakes, in 1170 cal. CE and 1964, resulting in severe coastal erosion (Mann and Crowell, 1996; Hutchinson and Crowell, 2007; Maio et al., 2019). The oldest surviving Kenai Coast site is McArthur Pass (SEL-188), where the basal cultural level has a median intercept date of 346 cal. CE (Betts et al., 1991). The 1964 earthquake caused uplift in most parts of Prince William Sound and a similar displacement likely occurred during the 1170 CE event, resulting in little or no damage to the archaeological record. Subsidence of about 1 m occurred at Kachemak Bay in 1964, probably comparable to the earlier earthquake, with possible erosion of sites although no damage has been reported.
To compensate for variable site preservation and compare regional sequences in an equivalent time frame, we include all Chugach tradition dates with calibrated ages of 2,000 years or less (n = 172, Supplementary Table S1; Figure 5). Occupation periods are blocked in gray and occupation gaps (no color) are defined as intervals of at least 100 years with ≤ 1 median intercepts. A single median intercept is allowed within a gap because many dates have long error tails. Inferred migrations to the Kenai Coast are labeled M1 through M5; inferred return migrations to Kachemak Bay and Prince William Sound are labeled R1 through R4. The unequal number of dates available for the three regions—Kachemak Bay (21), Kenai Coast (60), Prince William Sound (91)—is due to their respective research histories.
Figure 5. Inferred occupation periods (gray blocks) and migrations (arrows) between Kachemak Bay, the Kenai Coast, and Prince William Sound, aligned with climate periods. Radiocarbon dates are shown with median calibrated age intercept (red dots) and 2 SD error ranges (95.4% probability). Occupation gaps are defined as intervals of at least 100 years with ≤ 1 median intercepts. LNGP, Late Neoglacial Period; MWP, Medieval Warm Period; LIA, Little Ice Age; MOD, Modern Warm Period.
As shown in Figure 5, all three areas were occupied during all or part of 0–600 CE although there are too few dates to present a clear picture. A gap in Prince William Sound dates from 378–485 cal. CE suggests a possible migration (M1) to the Kenai Coast and settlement at MacArthur Pass (three dates, 386–483 cal. CE) and possibly at other eroded sites, with a return (R1) around 490 cal. CE.
A second possible migration to the Kenai Coast (M2) is marked by a 645–841 cal. CE gap in Prince William Sound dates and a nearly synchronous 612–857 cal. CE gap at Kachemak Bay, suggesting migration from both areas to the Kenai Coast as water temperatures dropped and salmon declined in the Late Neoglacial period. However, there are only two radiocarbon dates in this period from the Kenai Coast, again from MacArthur Pass (690 cal. CE and 820 cal. CE).
A reverse population movement (R2) took place at the start of the Medieval Warm Period as indicated by a gap in Kenai Coast dates (820–1035 cal. CE) and the simultaneous reappearance of populations in Kachemak Bay and Prince William Sound. During this period, air temperatures rose to an average 7.7°C (Wiles et al., 2014), SSTs rose to about 11°C (Praetorius et al., 2015), and Gulf of Alaska salmon production was increasing (Finney et al., 2002).
At the end of the Medieval Warm Period Kachemak Bay was again abandoned for about 300 years, with no recorded dates between 1027–1317 cal. CE. During the same early Little Ice Age interval (1035–1364 cal. CE) numerous sites were established on the outer Kenai coast. The evidence suggests migration to the Kenai Coast (M3) as average air temperature cooled to about 7°C (Wiles et al., 2014). Salmon on Kodiak increased during the early part of this interval and then declined after 1200 CE (Finney et al., 2002). In contrast, Prince William Sound was occupied by at least part of its population throughout the early Little Ice Age with no gap in dates to indicate extensive outmigration. However, archaeofaunal remains from Palugvik (COR-001), Uqciuvit (SEW-056), and Eleanor Island (SEW-440) indicate reduced consumption of salmon and increased consumption of Pacific cod and sea mammals (Yarborough, 2000).
The end of the flourishing early Little Ice Age period of Chugach occupation on the Kenai Coast coincided with a late 1300s eruption of Katmai, Augustine, Redoubt, or another volcano near Cook Inlet that deposited airborne tephra across the Kenai Peninsula (Payne and Blackford, 2008; Maio et al., 2019). A 10–20 cm layer of reddish-brown ash from this eruption (known as Tephra 1) overlies early Little Ice Age cultural strata at Kenai Coast sites including Kiniyak (SEL-119), Yálik (SEL-172), McArthur Pass (SEL-188), and Bear Cove (XBS-030) (Schaff and Johnson, 1990; Crowell, 2010). Ecological disruption caused by the ashfall may explain abandonment of these sites and the century-long gap in Kenai Coast residence that followed (1364–1492 cal. CE). We suggest that Kenai Coast residents relocated to Prince William Sound at this time (R3).
Post-eruption resettlement of the Kenai coast (M4) during the depressed temperatures of the middle Little Ice Age by Chugach migrants from Prince William Sound is evident at sites including SEL-119 (1492 cal. CE), SEL-215 (1502 cal. CE), SEL-381 (1547 cal. CE, 1567 cal. CE), XBS-020 (1562 cal. CE, 1680 cal. CE), and XBS-030 (1640 cal. CE), all with first or renewed occupations in the late 15th to mid-17th centuries. A gap in Prince William Sound dates from 1601–1738 cal. CE) suggests a population movement to the Kenai Coast during this period. There was no Chugach habitation at Kachemak Bay at this time.
The final period of Chugach occupation on the outer Kenai coast (M5) coincided with PDO cool phases of the late Little Ice Age (Wiles et al., 1998; Gedalof and Smith, 2001). Sites inhabited during the 1798–1816 cool cycle included the Early Contact Village (XBS-029) in Aialik Bay (1779 cal. CE; median artifact date 1800), the Northwestern Lagoon site (XBS-020) in Harris Bay (1809 cal. CE), and the Bear Cove site (XBS-030) in Aialik Bay (1826 cal. CE). These settlements were abandoned at the end of the cycle and never reoccupied. During the next cool cycle in 1840–1923, the Home Cove/Agmilek (SEL-130) site in Nuka Bay (median artifact date 1855 CE) and Denton/Ayalik site (XBS-014) in Aialik Bay (median artifact date 1880) were established.
This sequence of migration events may also be examined by plotting the number of calibrated radiocarbon dates in each study area against the air temperature curve available for the period 800–2010 CE (Wiles et al., 2014) (Figure 6). Dates with 1 SD (68.3% probability) error ranges that included a given calendar year were assigned to that year and the sum of these assignments was normalized by subtracting the mean and dividing by the standard deviation of the total number of dates for the region. Normalized variation in the number of radiocarbon dates (color scale) provides a rough proxy for population (Fitzhugh et al., 2022). We included occupation dates for modern villages in the data series based on historical information (Nanwalek, 1850–2010; Port Graham, 1912–2010; Seldovia, 1880–2010; Tatitlek, 1850–2010; Chenega/Chenega Bay, 1850–2010; and Kniklik, 1850–1910). For comparison, periods of occupation identified in Figure 5 are transposed to Figure 6 as horizontal black bars with date ranges. Transitions between occupation phases appear more gradual in Figure 6 than in Figure 5 because of overlapping radiocarbon error estimates, and a time lag of several decades between climate signal and human movement is apparent in several instances.
Figure 6. Normalized numbers of calibrated radiocarbon dates (color scale) for each calendar year plotted on the air temperature curve (Wiles et al., 2014) for the period 800–2010 CE. Dates with 1 SD (68.3% probability) error ranges that included a given calendar year were assigned to that year and this sum was normalized by subtracting the mean and dividing by the standard deviation of the total number of dates for the region. Periods of occupation identified in Figure 5 are shown as horizontal black bars with date ranges.
At Kachemak Bay there were three periods of estimated above-average Chugach population (purple to yellow on the color scale), each coinciding with a peak in the air temperature curve, consistent with the climate-coupled migration model. On the Kenai Coast, the three-century period of moderate to high population that followed migration M3 coincided with the variable but generally lower air temperatures of the early Little Ice Age, also supporting the model. However, a pronounced gap in Kenai Coast dates followed the 14th century volcanic eruption, with moderate population levels (purple) resuming only in the 1490s following migration M4. The eruption gap coincided with low values on the temperature curve and we suggest that moderate to high population levels would have continued on the Kenai Coast during this time if not for the eruption. Occupation in Prince William Sound was more continuous, with population peaks associated with upward trending temperatures during the middle Little Ice Age. We note that the highest values (yellow) immediately followed the Kenai volcanic eruption, supporting our interpretation that the Kenai population shifted to Prince William Sound at this time.
We tested for interaction between air temperatures and numbers of radiocarbon dates per calendar year using cross-correlation, which is a measure of the similarity between two time series incorporating lagged effects. The data series used for correlation excluded the years 1950–2010 since radiocarbon signals are not meaningful in that range. Hypothetically, Kachemak Bay and Prince William Sound should show positive correlation between number of dates and air temperature while these variables should be negatively correlated on the Kenai Coast. The hypothesis is weakly supported (Figure 7) in that Kachemak Bay and Prince William Sound show positive correlations with temperature (0.40 and 0.25, respectively) and with each other (0.49). The Kenai Coast value is near zero (0.08), indicating no statistical correlation. We suggest that the Kenai Coast correlation would have been negative if not for post-eruption abandonment of the area during the very low temperatures of the 14th and 15th centuries, thus losing a significant portion of the data series.
Figure 7. Cross-correlation matrix showing temperature-radiocarbon date frequency correlations for each study area (KB, Kachemak Bay; KC, Kenai Coast; PWS, Prince William Sound) and between areas for the years 800–1950 CE.
Harris Bay (including its inner portion, Northwestern Lagoon) and Aialik Bay are adjacent fiords in Kenai Fjords National Park west of Seward (Figure 8). Coves along their shorelines are cirques of small tributary glaciers drowned by tectonic subsidence over the last 15,000 years (Crowell and Mann, 1998). The Chiswell Islands, prime habitat for sea mammals and seabirds, lie just offshore. Northwestern Glacier expanded during the early Little Ice Age, reaching the moraine shoal that divides Northwestern Lagoon from Harris Bay sometime after 1476 cal. CE, then began to retreat about 1900 CE. Aialik Glacier also advanced during the early Little Ice Age, reaching its terminus ca. 1600 CE and withdrawing a century later, timing similar to the advance and retreat of Holgate Glacier. Anchor, Ogive, and Northwestern glaciers discharge ice floes and meltwater into the head of Northwestern Lagoon. Ice and meltwater enter Aialik Bay from Aialik, Holgate, and Pederson glaciers and from hanging glaciers on the Aialik Peninsula.
Figure 8. Harris Bay and Aialik Bay study areas in Kenai Fjords National Park. Shown are hydroacoustic survey lines, oceanographic measurement stations, water sample and trawl stations, beach seine locations, harbor seal haulouts and concentrations, seabird colonies, salmon streams, and sea lion haulouts (Yarborough, 1998; Arimitsu et al., 2012). Approximate locations of four archaeological sites (XBS-020, XBS-030, XBS-029, XBS-014) are indicated. Base map from National Geographic MapMaker (c) National Geographic Society.
A study of marine ecology and seabird prey dynamics in Aialik Bay and Harris Bay/Northwestern Lagoon was undertaken in 2007–2008 (Arimitsu et al., 2012). Physical and biological characteristics of the fiords were sampled monthly during June–August, including oceanographic surveys, acoustic-trawl surveys for zooplankton and forage fish, and at-sea surveys of marine birds and mammals. The influence of glacial effluents is evident in temperature and salinity profiles of the two fiords (Figure 9). Cool, fresh water introduced by glaciers and meltwater streams pools behind the Little Ice Age cross-bay moraines, while warmer, more saline waters are held outside these sills. This effect is more pronounced in Harris Bay where sill depth is shallower (< 4 m) than in Aialik Bay (< 10 m). Turbidity from glacial silt is highest inside the moraines and water clarity increases from near zero in the stratified freshwater layer near the glaciers to almost 100% in the surface waters of outer fiord areas. Mineral nutrients carried in glacial effluent including nitrite, nitrate, phosphate, and silica diffuse down-fiord and had lower concentrations at the outer stations. Levels of all nutrients except silica were found to decline by about half over the summer months as they were taken up by phytoplankton.
Figure 9. Temperature (C, color) and salinity (lines, range: 24.34–33.00) vertical contours relative to depth (m; y-axis) and distance to tidewater glaciers (km; x-axis) in Northwestern Lagoon/Harris Bay and Aialik Bay during 2008 (Arimitsu et al., 2012). Sample locations are represented by black arrows. Bathymetric features are indicated in black, with minimum sill depth of 4 and 10 m, in Northwestern Lagoon and Aialik Bay, respectively.
Depth-integrated phytoplankton concentrations up to 25 mg/m2 occurred 5 km or more down-fiord from glaciers in relatively clear surface waters, although these blooms were distributed primarily within the shallow sill at Northwestern Lagoon and 10 km or more beyond the deeper sill in Aialik Bay, a contrast that was strongest in June and weakened over the summer (Figure 10). Zooplankton biomass had a similar spatial and temporal pattern, whereas euphausiids (krill) were concentrated in shallow turbid water close to the glaciers in both fiords.
Figure 10. Chlorophyll a vertical contours (mg m−3, color) relative to depth (m; y-axis) and distance to tidewater glaciers (km; x-axis) in Northwestern Lagoon/Harris Bay and Aialik Bay (Arimitsu et al., 2012). Sample locations are represented by black arrows. Photic depth is indicated as black diamonds for 2008, and locations of the prominent marine sills are indicated in solid black, with minimum sill depth of 4 and 10 m, in Northwestern Lagoon and Aialik Bay, respectively. Fluorometer data were not collected at core oceanography stations in July 2007 or August 2008.
A minimum of 28 fish species were captured by small-mesh trawl net, including forage fish such as capelin (Mallotus catervarius), Pacific herring (Clupea pallasii) and Pacific sand lance (Ammodytes personatus). Saffron cod (Eleginus gracilis) was the most abundant juvenile gadid; however, Pacific cod (G. macrocephalus) and walleye pollock (Gadus chalcogrammus) were also present. Mesopelagic fishes included lanternfish (Myctophidae) and the northern smoothtongue (Leuroglossus schmidti). At least 21 fish species were collected in beach seines. Pacific sand lance (61% of the catch), pink salmon (O. gorbuscha, 22% of the total catch), and Pacific herring (5% of the total catch) were the most abundant. Chum salmon (Oncorhynchus keta, 3% of the total catch) and Surf smelt (Hypomesus pretiosus; 2% of the total catch) were also captured. Fish biomass, as measured by acoustic backscatter, was highest inside the Northwestern Lagoon sill, illustrating its containment effect. The relationship between acoustic backscatter and distance to glaciers in Aialik Bay was variable over the summer, with a significant positive correlation in the early season but no significant relationship later in the season. In the middle and late summer, dense forage fish schools moved into inner glacial areas of Aialik Bay.
The spatial distributions of major subsistence species in the two fiords are influenced by the glacial inputs, physical features, oceanographic gradients, and biological relationships described above. Large bottom fish including Pacific cod and halibut feed on forage fish and invertebrates in outer Aialik Bay and spend most of the year in relatively deep water, moving to shallower depths in spring. Pink, chum, and coho salmon spawn in short, glacially sourced streams at the head of Aialik Bay and pink salmon spawn in glacial streams of Harris Bay and Northwestern Lagoon. Herring spawn along low-energy beaches in protected coves in both fiords. The abundance of phytoplankton, zooplankton, and forage fish in outer Aialik Bay during early summer supports large populations of seabirds including cormorants, horned and tufted puffins, and murres, which have nesting colonies in the Chiswell Islands and outer fiord coves. There are several nesting colonies in Northwestern Lagoon/Harris Bay, most in the fiord where primary and secondary productivity are highest.
Marine mammals including sea lions, harbor seals, harbor porpoises, Dall porpoises, and humpback whales feed primarily in the outer reaches of Aialik Bay where macrozooplankton and forage fish abound, and there is a sea lion rookery in the Chiswell Islands (Nishimoto and Rice, 1987). During May–July, over 600 harbor seals congregate at ice floe haulouts near Northwestern, Anchor, Ogive, Aialik, and Pederson glaciers for birthing and rearing pups (Hoover-Miller and Armato, 2018). Overall, the abundance and diversity of subsistence resources increases with distance from glaciers due to the balance of mineral nutrients and water clarity that yields maximum phytoplankton growth. Moraine sills in both fiords modify this effect, to a greater degree in Harris Bay than in Aialik Bay.
Northwestern Lagoon (XBS-020), one of the largest precontact Chugach settlements on the Kenai Coast, is located near the glacial moraine that divides Harris Bay from Northwestern Lagoon (Kent and McCallum, 1991; Crowell and Mann, 1998). Surface features at XBS-020 include 25 shallow, subrectangular pits up to 6 m long interpreted as the imprints of plank-walled summer dwellings; a deep, subrectangular winter house pit (6 m long) with an entrance tunnel; two large oval depressions (9 and 13 m long), possibly multifamily fall houses; and eight circular cache pits for underground food storage (Figure 11A).
Figure 11. Archaeological site maps showing topographic contours, house pits, and other cultural features. (A) Northwestern Lagoon (XBS-020); (B) Bear Cove (XBS-030); (C) Early Contact Village (XBS-029) and Ayalik (Denton) site (XBS-014).
Radiocarbon dates from limited test excavations in 1994 indicate three separate phases of occupation: the early Little Ice Age until the 14th century (five dates, 1201–1360 cal. CE); the middle Little Ice Age (two dates, 1562–1680 cal. CE); and the late Little Ice Age (one date, 1809 cal. CE; Supplementary Table S1). All were periods of cool ocean temperatures, as discussed above. No glass beads or other imported goods were found, suggesting that residents did not interact with Russian fur traders who arrived in the 1790s. During the site's span of occupation Northwestern Glacier advanced down the fiord, reaching its terminus near the village by the late 15th century. Proximity to the glacier and its ice floe harbor seal rookery, combined with the predominance of summer houses, suggests that XBS-020 was primarily a sealing camp.
Bone preservation was poor due to the near-absence of calcium carbonate from marine shells to buffer the naturally high acidity of the soil. Test units yielded only a few fragments of burnt (calcined) marine mammal bone and one identification, a hoary marmot (Marmota caligata) (Yarborough, 1998). The potential for interpreting subsistence practices of the residents is therefore limited, although the small artifact collection includes marine mammal hunting and processing tools—a slate lance preform, semilunar slate knife, and cobble-spall scrapers—in addition to a stone lamp for burning marine mammal oil (Crowell and Mann, 1998).
The Bear Cove site (XBS-030) occupies a beach terrace about 10 km south of the Little Ice Age glacial terminus in Aialik Bay, a position that afforded good access to marine mammals, salmon, cod, halibut, and seabird colonies in the middle and outer fiord, and to the ice floe harbor seal rookery at Aialik Glacier.
Excavations at the site in 1994, 2002, and 2004 revealed two groups of structures (Crowell and Mann, 1998; Crowell, 2010) (Figure 11B). An older house group, located at the back of the terrace, included four subrectangular house pits 4–5 m long with central hearths (Structures 4, 5, 7, and 9), a form consistent with historic semi-subterranean Chugach winter houses with bark-covered roofs (Walker, 1982; Steller, 1988). Structure 8 was larger (10 m long) and consisted of a main room with two hearths and a pit for roasting meat on hot stones covered with seaweed, as this feature was described by Chugach advisor Nick Tanape, Sr. (personal communication, June 25, 2002). The main room was adjoined by a small annex filled with fire-cracked cobbles, indicating a steam bathing room. The size, shape, depth, and interior features of this structure are similar to multifamily Chugach plank houses used during summer and fall (Birket-Smith, 1953; Walker, 1982).
The lowest house floors and cultural strata in this part of the site predate or coincide with the 1170 cal. CE earthquake (three dates, 1035–1176 cal. CE, Supplementary Table S1) and are overlain by gravels washed in by tsunami waves or storms following coseismic subsidence. This event appears to have terminated the original occupation. Residents returned to Bear Cove about a century later, depositing new occupation layers (four dates, 1293–1353 cal. CE, Supplementary Table S1) above the earthquake gravel. These are capped by Tephra 1 from the Cook Inlet eruption, which may have forced a second abandonment of the site. Use of the older house group thus spanned the early Little Ice Age period and included fall, winter, and summer residency.
The younger group consists of 12 subrectangular winter house pits (4–5 m long) along the front edge of the terrace (Figure 11B). Test excavations in Structures 19 and 20 yielded medial dates of 1640 cal. CE and 1840 cal. CE, respectively (Supplementary Table S1). No tephra was found above the house floors, confirming that the structures were built after the eruption. These dates coincide with middle and late Little Ice Age occupations at Northwestern Lagoon and other sites.
Bear Cove artifacts (Figure 12) include triangular slate endblades for sea mammal lances, used to dispatch harpooned animals; barbed endblades for arrows; shouldered and semilunar knives; and a bayonet-form lance point with medial ridge, a chronotype indicative of the period 1000–1200 CE (De Laguna, 1956, 1975; Clark, 1974; Jordan and Knecht, 1988). Stone adze blades, a sea mammal oil lamp, and coal beads were also found, but Russian trade items were absent. Hundreds of pieces of animal bone—evidently from birds, marine mammals, and possibly land mammals - were collected from the house floors and midden but preservation was very poor due to soil acidity. All were tiny fragments burned to the point of calcification and unidentifiable beyond zoological order. Chugach advisors attributed these fragments to the custom of tossing bones into a cooking fire to chase away storms, called pinahsuhtut (“they are hunting for good weather”) (Nick Tanape, Sr., personal communication, June 25, 2002). No analysis of this material was attempted except for a small 1994 sample (n = 74) that included 18 marine mammal fragments, 26 pieces identifiable only as mammal, and a bird carpometacarpus (Yarborough, 1998).
Figure 12. Artifacts from the Bear Cove (XBS-030) site. (A) Slate bayonet-form lance blade with medial ridge; (B–E) slate triangular endblades for lances; (F, G) slate arrowpoints with barbed bases; (H, I) beads made of hard coal; (J–L) slate and greenstone blades for hafted knives; (M, N) slate semilunar knife blades; (O, P) pecked greenstone heads for hafted adzes; (Q) pecked stone seal oil lamp.
The Early Contact Village (XBS-029) is on the west side of Aialik Bay in the productive fiord mouth area, with access by kayak to fishing sites and to seabird colonies, sea lions, and seals in the Chiswell Islands (Crowell and Mann, 1998; Crowell, 2006; Crowell et al., 2008). The site includes a 50 cm-thick midden mound with abundant shell and well-preserved faunal remains, seven house depressions (Structures 1, 2, 3, 4, 10, 12, 16), four cache pits (Structures 5, 7, 11, 14), and two aboveground sod wall features (Structures 6, 13; Figure 11C). Dwellings are a combination of summer and winter houses, the latter including Structure 10 which had an interior hearth, log-reinforced walls, and a side room for storage or sleeping. The stratigraphy of the midden suggests rapid deposition over three seasons—a spring-summer middle level containing abundant faunal remains and implements for hunting and fishing, and fall-winter layers above and below this stratum containing abundant fire-cracked rock but few animal bones or tools.
The Early Contact Village was established shorty before or after 1800 CE, corresponding to the PDO cold phase of 1798–1816 (Gedalof and Smith, 2001). Dating of the site is based on a radiocarbon sample from the lower midden (1779 cal. CE, Supplementary Table S1); a seriation date of 1790–1810 CE derived from glass trade beads (Crowell, 1997); and the presence of other early Russian trade items including a forged iron knife blade, window and bottle glass, and a kopeck coin minted in 1748 (Crowell et al., 2008). Chugach residents might have been trading fish, game, and furs to Russians at Voskresenskii fort, established at what is now Seward in 1793. The majority of artifacts found at the site are Chugach traditional forms including ground slate lance and arrow points, knives, preforms, and debitage; cobble spall scrapers; two-part bone fishhooks; barbed bone dart heads; and bone toggling harpoon heads (Figure 13). One small toggling head (Figure 13FF) may have been for spearing salmon at weirs, a method that has been historically documented at Nanwalek (Stanek, 1999).
Figure 13. Artifacts from the Early Contact Village (XBS-029) site. (A–E) Tips of slate lance blades; (F–K) bases of barbed slate lance blades; (L) flaked slate lance blade preform; (M) slate lance blade preform with flat-ground margins; (N–Q) preforms and finished triangular slate blades; (R, S) stemmed arrow points; (T–Y) small triangular endblades for arrows or harpoons; (AA) bone fishhook shank; (BB, CC) bone fishhook barbs; (DD, EE) bone toggling harpoon heads for seals with slot for slate endblades; (FF) miniature bone toggling harpoon head, possibly for salmon; (GG) tip for bone awl. Items reflecting Russian trade included: (HH) thumbnail scraper flaked from flat clear glass; (II) green glass bottle fragment with retouch; (JJ) Russian half-kopek with double eagle insignia dated 1748; (KK) copper arrow point; (LL) lead finger ring; (MM) forged iron knife blade with tang; (NN) drawn glass trade beads; (OO) wire-wound glass trade beads.
Identification of faunal remains (n = 26,436) from the midden and house excavations (Yarborough, 1998; Yesner, 2004; Hanson, 2007) allows a detailed assessment of subsistence practices (Table 3). Shellfish including mussels, chitons, cockles, and Pacific littleneck clams were harvested from local beaches and rocky intertidal zones. The most frequently taken fish were Gadidae (69.1% of identified specimens), dominated by Pacific cod with a few Alaska pollock. Second were rockfishes (Scorpaenidae, 24%), with other taxa of minor importance including halibut (Pleuronectidae, 3.4%), sculpins (Cottidae, 1.4%), salmon (Salmonidae 1.1%), greenlings or lingcod (Hexagrammidae, 0.9%), and herring (Clupeidae, 0.1%). Spines, rays, ribs, and branchials were not identified to family or genus, accounting for the large number of unidentified fish bones (Hanson, 2007). Cod, rockfish, and other species were taken with two-piece bone fishhooks (Figures 13AA–CC). Capelin, herring, sand lance, and other forage fish identified in the Aialik Bay biological surveys were absent or scarcely represented in the midden because these species are too small for line fishing, and no evidence of nets such as sinkers or floats was found.
Based on a minimum number of individuals (MNI) analysis of the faunal sample from a 1 × 1 m 1994 test pit in the midden (n = 5,757) an estimated 37% by weight of all meat consumed at the site came from Gadidae (Yarborough, 1998). The dominance of cod and minimal presence of other fishes including salmon, pollock, flounder, and halibut is consistent with cool ocean temperatures, estimated from XBS-029 cod otoliths to have averaged about 8.3°C at the time of occupation (Helser et al., 2018), and with cod-dominant biological regimes of the late Little Ice Age (Maschner et al., 2008).
Alcidae were the predominant avian family consumed (89.6% of identified birds) including puffins, murres, auklets, and guillemots, an abundance suggesting that these seabirds had ample forage in the outer fiord. There were relatively few ducks (Anatidae, 4.8%), cormorants (Phalacrocoracidae, 3.6%), loons (Gaviidae, 0.8%), eagles (Accipitridae, 0.5%), ptarmigans (Phasianidae, 0.5%), and shorebirds (Charadriidae, 0.2%) (Yesner, 2004). Alcidae would have been obtained by kayak hunting using bird darts or by cliff harvesting at the rookeries in the Chiswell Islands. While Alcidae do not have much edible meat their feathered pelts were used to make birdskin parkas, and the intensive focus on these birds may have been influenced by Russian colonial demand for such garments (Davydov, 1977, p. 194).
The most important mammalian prey were harbor seals (Phocidae, 44.4%), sea lions (Otaridae, 18.4%), animals identifiable as either seal or sea lion (clade Pinnipedia, 25.2%), and harbor or Dall porpoise (Phocoenidae, 19.9%). There was minor representation of whales, possibly humpback (Cetacea, 2.0%) and a single sea otter bone (Mustelidae, 0.1%). Meat from marine mammals made up and estimated 45% of the diet by weight (Yarborough, 1998).
Age patterning within the Otaridae and Phocidae suggest differences in hunting methods. Sea lions show an age distribution biased toward large, mature animals likely taken at sea (13.4% immature, 26.1% juvenile, 60.5% adult, n = 357), whereas for harbor seals there was a much higher proportion of animals under 4 months of age compared to juveniles and adults (46.2% immature, 29.8% juvenile, 23.9% adult, n = 737) suggesting hunting at the Aialik Glacier or Northwestern Glacier ice floe rookeries after the pups were born. Chugach kayak hunting at Aialik Glacier has been orally documented for the late 19th and early 20th centuries (Stanek, 1985, 1999; Nick Tanape, Sr., personal communication, June 25, 2002).
Terrestrial game taken with arrows, spears, or traps played a minor role in subsistence. Animals included beaver, marmot, and porcupine (Rodentia, 70.0% of identified land mammals); river otter (Mustelidae, 12.5%); wolf or fox (Canidae, 10.0%); mountain goat (Bovidae, 5.0%); and caribou (Cervidae, 2.5%).
Overall, subsistence activities at the Early Contact Village show a strong maritime focus including gathering of intertidal invertebrates; kayak hunting for sea lions, harbor seals, porpoises, and whales with harpoons, darts, and lances in Aialik Bay, the Chiswell Islands, and possibly Harris Bay; sealing at the Aialik or Northwestern rookeries; birding for Alcidae in outer fiord areas and the Chiswell Islands; and hook and line fishing for cod and rockfish. Proportions of these animals in the faunal assemblage are consistent with cool SST conditions.
Cultural features at the Ayalik site (XBS-014), located on the same beach as the Early Contact Village, include seven low mounds of charcoal-stained soil, fire-cracked rock, artifacts, and animal bone (Figure 11C). The mounds are household debris deposited in and around 19th century log cabins (Schaaf, 1989; Crowell and Mann, 1998; Crowell, 2006; Tozzi, 2012). Artifacts recovered at the site (n = 1,550) included factory-made whiteware ceramics; glass beads; glass bottles; firearms (parts of percussion muskets and breech-loading rifles, percussion caps, gunpower cans, lead shot, rifle cartridges); iron and copper tools, parts, and fasteners; and commercial cloth, buttons, and boots. Manufacturing dates for these artifacts span approximately 1850–1920, with a mean of 1880 (Tozzi, 2012). A few items of Chugach manufacture were found including copper spear heads, bird arrow blunt tips made from rifle cartridges, and a bone dart socket.
The XBS-014 site, originally named Denton for its discoverers (Schaaf, 1989), is securely identified as the former Chugach village of Ayalik, whose residents traded seal skins and furs with the Alaska Commercial Company store in English Bay (Nanwalek) during the 1870s (De Laguna, 1956; Luehrmann, 2008; Crowell, 2010) and with the Lowell trading station at Seward, which operated from 1883 to the mid-1890s. Lowell paid Chugach hunting crews for pelts of black bear, fox, mink, sea otter, and river otter obtained along the Kenai coast (Cook and Norris, 1998, p. 70–73). According to the late Nanwalek elders Joe Tanape and Walter Meganack, Sr., men of their fathers' generation traveled by kayak or rowboat to the area for hunting and fishing, joining up with relatives and staying on the coast from October until May (Stanek, 1985, 1999). In the late 19th century, Chugach people from Nuchek and other settlements in Prince William Sound migrated west to Aialik Bay and from there along the Kenai Coast to Kachemak Bay (Johnson, 1984; Stanek, 1999). In 2002, Elenore McMullen of Port Graham recounted the story of her grandmother, Anesia Anahonak (b. 1886), who as a girl traveled with her family to Ayalik during the migration. The group hunted for birds and harbor seals—the latter said to be exceptionally abundant—and fished for cod and “bass” (rockfish). Aialik Bay was remembered as “very cold” but a good area for hunting (Smithsonian Institution and Pratt Museum, 2002; Crowell, 2006).
Oral accounts of the late 19th century climate, subsistence practices, and animal populations at Aialik Bay are confirmed by paleoenvironmental and archaeofaunal data. Residence at Ayalik village coincided with the late Little Ice Age cold PDO period of 1840–1923 (Gedalof and Smith, 2001) when the average water temperature was estimated from δ18O isotope values in cod otoliths to have been about 9.1°C, warmer than the estimated 8.3°C during the Early Contact Village occupation (Helser et al., 2018).
Ayalik faunal remains (n = 18,246) also reflect a relatively cool biological regime, although shifts in species representation are evident (Table 4). Fish remains were predominantly Pacific cod (Gadidae, 60.1% of identified bones) as at Early Contact Village, but flatfishes including flounders, sole, and halibut made up a larger portion of the assemblage (Pleuronectidae, 13.1%), slightly exceeding rockfish (Scorpaenidae, 12.3%). Other fish included sculpins (Cottidae, 8.7%), greenlings (Hexagrammidae, 1.7%), and salmon (Salmonidae, 3.7%) (Hanson, 2009).
Puffins and murres (Alcidae, 49.2%) were the leading birds harvested at Ayalik but eagles (Accipitidria, 17.8%), ducks and geese (Anatidae, 11.4%), and cormorants (Phalacrocoracidae, 10.8%) were all taken in greater numbers than at Early Contact Village, along with loons (Gavidae, 6.0%), gulls (Laridae, 2.6%), grebes (Podicipedidae, 1.3%), and ptarmigan (Phasianidae, 0.9%).
There was a particularly strong emphasis at Ayalik on harbor seals (Phocidae, 78.0%), perhaps due in part to production of seal skins and oil for the Alaska Commercial Company and the “hair seal” industry that flourished in Alaska from the late 1860s to 1914 (Crowell, 2017, 2020). Sea lions (Otariidae, 18.0%), porpoises (Phocoenidae, 3.2%), and unidentified whales (Cetacea, 0.2%) comprised the remainder of the marine mammal bones (Yesner, 2010). The high proportion of very young animals among the harbor seal remains (49% immature, 18% juveniles, 32% adults, n = 1,690) again suggests rookery hunting, while sea lions were selected for larger animals and probably hunted at sea (16.4% immature, 31% juvenile, 52.2% adult, n = 335). Land mammals were a minor component of the subsistence effort including porcupine and marmot (Rodentia, 90.5% of identified land mammals) and caribou (Cervidae, 9.5%).
Overall harvesting patterns at Ayalik were similar to Early Contact Village but with shifts in fishing that suggest reduced populations of Pacific cod and rockfish and larger numbers of halibut and other flatfishes due to increasing water temperatures. Salmon still comprised < 4% of identified fish. The take of Alcidae and other seabirds was reduced, with more hunting of eagles and other terrestrial birds. Harbor seals appear to have been the main source of meat, oil, and skins for subsistence and possibly commercial consumption.
The Little Ice Age faunal assemblages at Early Contact Village and Ayalik contrast with the mix of species consumed by Kachemak Bay and Prince William Sound communities following the warm PDO shift in 1976. In 1981–1983, residents of Nanwalek in Kachemak Bay harvested 250 kg of subsistence foods per household per year, led by salmon (66.5% by edible weight) and followed by trout and other freshwater fish (10.4%), halibut and other flatfish (10.1%), marine mammals (5.9%), and seabirds (0.2%) (Stanek, 1985). At Chenega Bay in Prince William Sound during 1984–1985, residents harvested 462 kg of subsistence foods per household, including 37.2% salmon, 9.1% halibut, 18.7% harbor seals and sea lions, and 18.7% Sitka deer by edible weight (Stratton and Chisum, 1986). Burgeoning salmon and flatfish populations and the resulting subsistence focus on these fishes after 1976 are indicated.
The 1976–1977 regime shift was also accompanied by severe declines in marine mammals, as reflected in subsistence takes at Nanwalek and Chenega Bay. An 80%−90% population crash of harbor seals took place during the 1970s (Ver Hoef and Frost, 2003; Hoover-Miller et al., 2011) and although conditions for the crash were created by commercial overhunting in the 1960s, losses of forage fish after 1976 contributed to the decline of seals and their failure to recover (Crowell, 2020). A Chenega Bay hunter interviewed in 1992 recalled “There was plenty of seal [before] but…Seal Island in the early 80s did not have half of what I seen with my dad [in 1968 or 1970]”; another said “They're really scarce now. I haven't eaten seal in two months” (Haynes and Wolfe, 1999, p. 111–112).
The results of this study substantiate a long-standing pattern of intermittent Chugach migration and occupation on the Kenai Coast during periods of reduced ocean temperatures. Highly productive glacial fiords on the Kenai Coast including Nuka Bay, Harris Bay, and Aialik Bay have baseline populations of pelagic fish, seabirds, and marine mammals that increase during periods of low SSTs, and migration villages were situated in the outer portions of these fiords where marine resources are most concentrated. Archaeofaunal data from the Early Contact Village and Ayalik in Aialik Bay confirm a primary reliance on cold water fauna and negligible consumption of salmon. The Kenai Coast settlements were used for varying periods of time from a few seasons to several centuries, abandoned when the climate warmed, and in most instances reoccupied during subsequent cooling cycles. During the final century of the Little Ice Age, opportunities for trade with Russian and American fur companies may also have influenced Chugach migration and hunting on the Kenai Coast.
Periods of higher temperatures were accompanied by return migrations to Kachemak Bay and Prince William Sound and switching of the principal subsistence focus to salmon, fished at weirs to maximize production. Chugach subsistence economies based on salmon, flatfish, freshwater fish, and terrestrial game, with low sea mammal harvests, characterized modern Kachemak Bay and Prince William Sound communities after the 1976 warm PDO regime shift, demonstrating the pattern of resource switching that accompanied return to these areas during warm phases of previous centuries.
Although Chugach pulse migration may have occurred throughout the Holocene it can be documented for only about the last 2,000 years due to erosion and loss of the early Kenai Coast archaeological record. Catastrophic natural events including the earthquake of 1170 cal. CE and the Cook Inlet volcanic eruption in the late 1300s also disrupted residence on the outer coast, underlining challenges posed by the physical dynamism of the region as well as its climatic and resource fluctuations.
The Chugach response to climatic and environmental change may be characterized as a highly intentional, knowledge-based strategy of mobilization for maximum productivity, with at least part of the population moving to where rising resources were most easily acquired in large quantities. A comparable situation has been identified on Kodiak Island, where the marine mammal-focused Late Kachemak culture transitioned to Koniag culture with onset of the Medieval Warm Period, accompanied by the establishment of extensive fishing villages along the Karluk, Ayakulik, and other rivers on the southwest side of the island where salmon were procured in mass quantities at river weirs (Knecht, 1995; Fitzhugh, 2003; Kopperl, 2003; Steffian et al., 2015). During the coldest Little Ice Age temperatures of the late 18th century these fishing villages were abandoned and the population shifted to the northern and eastern coasts of the island where harbor seals, northern fur seals, sea lions, and humpback whales became principal subsistence targets (Clark, 1974, 1987; Crowell, 1994).
An emergent perspective of this study is that the entire Chugach region was an ecologically heterogenous but culturally unified territory and resource catchment. Indigenous ecological and historical knowledge extended not only to the environs of individual villages but to all parts of the coast including places where prior generations once lived and which could be reoccupied when the climate next turned and the order of animal abundance was reshuffled. Even today, Nanwalek, Port Graham, and Seldovia community members retain the names and locations of the 19th century Kenai Coast villages, oral traditions about the travels of their forebears, and ecological knowledge about fiord biomes on the outer coast (Crowell, 2006, 2010). An elder resident of Nanwalek said, “I did just about anything to hear the stories of our ancestors; Aleuts [Sugpiat] have been survivors—they survived.” A younger man who visited the archaeological excavations in Aialik Bay said, “I kind of got chilled to the bone; part of our people, it's in my blood, part of our people lived here and survived in these bays where there's a lot of ice, wind, and rain.”
Chugach community scholar Nick Tanape, Sr. related stories from his father about how hunters from Kachemak Bay would meet others at Aialik Bay, including people from Prince William Sound. Visiting the seal rookery at Aialik Glacier in 2002, Mr. Tanape said, “It would make a lot of sense to hunt in this area. You can sneak around this floating ice to get to the seals. They would be more abundant here in cooler weather, cooler weather for them, especially in the summer. And there's probably more feed on the bottom for them” (Smithsonian Institution and Pratt Museum, 2002). Tanape noted the plunge in seal and sea lion populations over recent decades, underlining that such environmental cues are closely monitored in contemporary Chugach communities.
The Chugach role in the glacial fiord ecosystem of the central Gulf of Alaska was the outcome of human niche construction (HNC) (Hardesty, 1972; Fitzhugh et al., 2019). In evolutionary ecology, niche refers to the energetic interrelationships of an organism with its habitat and biotic community and niche construction emphasizes the capacity for organisms to modify natural selection and increase fitness (Lewontin, 1983; Kylafis and Loreau, 2011; Odling-Smee et al., 2013). In place of natural selection HNC emphasizes human agency and culture as the means by which long-term survival (sustainability), resilience, and ecosystem integration are achieved (Smith, 2007; Laland and O'Brien, 2010).
For the Chugach, observational knowledge of glaciomarine ecology across a broad region and of cyclical changes in the abundance of key species, acquired over centuries and orally transmitted to successive generations, was the foundation of a niche that afforded flexible modes of energy extraction (subsistence) under fluctuating climatic, biological, and physical conditions. In addition, technologies for ocean travel, fishing, and hunting; habitat-modifying constructions including houses, storage caches, and fish weirs; and social cooperation for food production were significant cultural elements from which an enduring ecosystemic role, or niche, was constructed. Like other northern Indigenous societies, the Chugach shaped their effective environment through both perturbation, e.g., the construction of settlements and modification of animal populations through hunting and fishing; and relocation, including seasonal movements and migrations to alternative habitats to take advantage of resource availabilities (Odling-Smee et al., 2003; Laland and O'Brien, 2010). Over time, enactment of these processes formed a cultural landscape on the Kenai Coast that today includes both physical traces of ancestral settlements (dwellings, middens, artifacts, archaeofaunal remains) and an intangible geography of Chugach place names, memories, and oral traditions (Cook and Norris, 1998; Crowell and Mann, 1998; Pratt and Heyes, 2022).
The original contributions presented in the study are included in the article/Supplementary material, further inquiries can be directed to the corresponding author.
All authors listed have made a substantial, direct, and intellectual contribution to the work and approved it for publication.
Funding for archaeological field work was provided by the U. S. National Park Service, Smithsonian Institution, and Ocean Alaska Science and Learning Center. Support for community coordination was provided by the Alaska Humanities Forum. Funding for the glacier ecosystem study was provided by the U.S. Geological Survey Alaska Science Center with additional support from the Natural Resources Protection Program.
We respectfully acknowledge that this research took place on the traditional lands and waters of the Chugach people and are thankful for the generous support, permissions, and cooperation of Kenai Fjords National Park, the Ocean Alaska Science and Learning Center in Seward, Chugach Alaska Corporation, English Bay (Nanwalek) Corporation, Port Graham Corporation, and the Native Villages of Nanwalek, Port Graham, and Seldovia. We thank members of those communities who contributed oral historical and ecological knowledge and joined in field research conducted for the Smithsonian Institution's Kenai Fjords Oral History and Archaeological Project. All analyses and interpretation presented in this paper, including supporting tables and figures, are original to the authors unless otherwise attributed. We thank NOAA Alaska Fishery Science Center's Resource Assessment and Conservation Engineering Division's Groundfish Assessment Program for permission to use groundfish bottom trawl survey data, and the many contributors of at-sea marine bird and mammal survey data to the USGS North Pacific Pelagic Seabird Database. Any use of trade, firm, or product names is for descriptive purposes only and does not constitute endorsement by the U. S. Government. We thank Gregory C. Wiles (Earth Sciences and Archaeology, The College of Wooster) for permission to access and use the 1,200-year data set of estimated Gulf of Alaska air temperatures (Wiles et al., 2014; online at https://www.ncei.noaa.gov/access/paleo-search/study/16015; accessed on February 3, 2023).
The authors declare that the research was conducted in the absence of any commercial or financial relationships that could be construed as a potential conflict of interest.
All claims expressed in this article are solely those of the authors and do not necessarily represent those of their affiliated organizations, or those of the publisher, the editors and the reviewers. Any product that may be evaluated in this article, or claim that may be made by its manufacturer, is not guaranteed or endorsed by the publisher.
The Supplementary Material for this article can be found online at: https://www.frontiersin.org/articles/10.3389/fearc.2023.1145220/full#supplementary-material
Abookire, A. A., and Piatt, J. F. (2005). Oceanographic conditions structure forage fishes into lipid-rich and lipid-poor communities in lower Cook Inlet, Alaska, USA. Mar. Ecol. Prog. Ser. 287, 229–240. doi: 10.3354/meps287229
Alaska Department of Environmental Conservation (2022). Alaska DEC Open Data: Alaska Seabird Colonies. Available online at: https://data-soa-adec.opendata.arcgis.com/datasets/ADEC::alaska-seabird-colonies-1/explore?location=60.474258.%2C-148.531383%2C8.00 (accessed April 14, 2023).
Alaska Department of Fish Game (2022). Alaska Waters Catalog of Anadromous Fish. Available online at: http://www.adfg.alaska.gov/index.cfm?adfg=maps.data (accessed April 14, 2023).
Anderson, P. J., and Piatt, J. F. (1999). Community reorganization in the Gulf of Alaska following ocean climate regime shift. Mar. Ecol. Prog. Ser. 189, 117–123. doi: 10.3354/meps189117
Arimitsu, M. L., Hobson, K. A., Webber, D. N., Piatt, J. F., Hood, E. W., Fellman, J. B., et al. (2018). Tracing biogeochemical subsidies from glacier runoff into Alaska's coastal marine food webs. Glob. Chang. Biol. 24, 387–398. doi: 10.1111/gcb.13875
Arimitsu, M. L., Piatt, J. F., Madison, E. N., Conaway, J. S., and Hillgruber, N. (2012). Oceanographic gradients and seabird prey community dynamics in glacial fjords. Fish. Oceanogr. 21, 148–169. doi: 10.1111/j.1365-2419.2012.00616.x
Arimitsu, M. L., Piatt, J. F., and Mueter, F. (2016). Influence of glacier runoff on ecosystem structure in Gulf of Alaska fjords. Mar. Ecol. Prog. Ser. 560, 19–40. doi: 10.3354/meps11888
Arimitsu, M. L., Piatt, J. F., Romano, M. D., Madison, E. N., and Conaway, J. S. (2010). Kittlitz's and Marbled Murrelets in Kenai Fjords National Park, South-Central Alaska: At-sea Distribution, Abundance, and Foraging Habitat, 2006–08. U. S. Geological Survey Open-File Report 2010-1181. Reston, VA. doi: 10.3133/ofr20101181
Arimitsu, M. L., Schoen, S., Piatt, J., Marsteller, C., and Drew, G. (2021). Monitoring the Recovery of Seabirds and Forage Fish Following a Major Ecosystem Disruption in Lower Cook Inlet. U.S. Department of the Interior, Bureau of Ocean Energy Management. Anchorage: U. S. Geological Survey, Alaska Science Center.
Bailey, E. P. (1977). Distribution and abundance of marine birds and mammals along the south side of the Kenai Peninsula, Alaska. Murrelet 58, 58–72. doi: 10.2307/3536404
Barclay, D. J., Wiles, G. C., and Calkin, P. E. (1999). A 1,119-year tree ring width chronology from Western Prince William Sound, Southern Alaska. Holocene 9, 79–84. doi: 10.1191/095968399672825976
Batten, S. D., Raitsos, D. E., Daneilson, S., Hopcraft, R., Coyle, K., McQuatters-Gollop, A., et al. (2018). Interannual variability in lower trophic levels on the Alaskan shelf. Deep Sea Res. t II 147, 58–58. doi: 10.1016/j.dsr2.2017.04.023
Bengtson, J. A., Phillips, A. V., Mathews, A., and Simpkins, M. A. (2007). Comparison of survey methods for estimating abundance of harbor seals (Phoca vitulina) in glacial fjords. Fish Bull. 105, 348–355.
Benson, A. J., and Trites, A. W. (2002). Ecological effects of regime shifts in the Bering Sea and eastern North Pacific Ocean. Fish Fish. 3, 95–113. doi: 10.1046/j.1467-2979.2002.00078.x
Betts, R. C., Wooley, C. B., Mobley, C. M., Haggarty, J. C., and Crowell, A. L. (1991). Site Protection and Oil Spill Treatment at SEL-188, An Archaeological Site in Kenai Fjords National Park, Alaska. Anchorage: Exxon Company USA.
Bond, N. A., and Harrison, D. E. (2000). The Pacific Decadal Oscillation, air-sea interaction and central North Pacific winter atmospheric regimes. Geophys. Res. Lett. 27, 731–734. doi: 10.1029/1999GL010847
Boraas, A., and Klein, J. (1989). “Radiocarbon dates from SEL-010: Kachemak Bay, Alaska,” in Paper presented to the Alaska Anthropological Association, Anchorage. Center for Digital Antiquity, The Digital Archaeological Record (tDAR id: 130202). Available online at: https://core.tdar.org/document/130202/radiocarbon-dates-from-sel-010-kachemak-bay-alaska (accessed April 24, 2023).
Boraas, A., and Klein, J. (1992). Archaeology of point west of halibut cove. Anthropol. Pap. Univ. Alsk. 24, 183–204.
Childers, A. R., Whitledge, T. E., and Stockwell, D. A. (2005). Seasonal and interannual variability in the distribution of nutrients and chlorophyll a across the Gulf of Alaska Shelf: 1998–2000. Deep-Sea Res. II 52, 193–216. doi: 10.1016/j.dsr2.2004.09.018
Clark, D. W. (1974). Koniag Prehistory: Archaeological Investigations at Late Prehistoric Sites on Kodiak Island, Alaska. Stuttgart: Verlag W. Kohlhammer. doi: 10.2307/j.ctv1751r
Clark, D. W. (1984). “Prehistory of the Pacific Eskimo region,” in Artic, ed D. Damas Handbook of North American Indians, Vol. 5, General ed W. C. Sturtevant (Washington, DC: Smithsonian Institution), 136–148.
Clark, D. W. (1987). On a misty day you can see back to 1805: ethnohistory and historical archaeology on the southeastern side of Kodiak Island, Alaska. Anthropol. Pap. Univ. Alsk. 21, 105–132.
Cook, L., and Norris, F. (1998). A Stern and Rockbound Coast: Kenai Fjords National Park Historic Resource Study. Anchorage: National Park Service, Alaska Support Office.
Cooney, R. T. (1986). “Zooplankton,” in The Gulf of Alaska: Physical Environment and Biological Resources, eds D. W. Hood, and S. T. Zimmerman. Alaska Office, Ocean Assessments Division, National Oceanic and Atmospheric Administration (Washington, DC: Government Printing Office), 285–303.
Cooney, R. T. (2007). “The transfer of matter and energy through the food web,” in Long-Term Ecological Change in the Northern Gulf of Alaska, ed R. B. Spies (Amsterdam: Elsevier), 60–74.
Crowell, A. L. (1994). “Alutiiq poison dart whaling,” in Anthropology of the North Pacific, eds W. W. Fitzhugh, and V. Chaussonnet (Washington DC: Smithsonian Institution Press), 217–242.
Crowell, A. L. (1997). Archaeology and the Capitalist World System: A Study from Russian American. New York, NY: Plenum Press. doi: 10.1007/978-1-4757-9279-9
Crowell, A. L. (2006). The Outer Coast Project: Kenai Fjords Oral History and Archaeology: Final Technical Report, Grant SI 634019. Seward, Alaska: Ocean Alaska Science and Learning Center.
Crowell, A. L. (2010). Final Report: Smithsonian Archaeological Survey of Nuka Bay, Kenai Fjords National Park. Ocean Alaska Science and Learning Center (OASLC) Grant KEFJ 2007 00029. Seward: National Park Service, Kenai Fjords National Park.
Crowell, A. L. (2017). Ice, seals, and guns: late 19th century Alaska Native commercial sealing in Southeast Alaska. Arct. Anthropol. 53, 11–32. doi: 10.3368/aa.53.2.11
Crowell, A. L. (2020). “The 1960s−1970s harbor seal crash in Alaska: a historical and ecological perspective,” in Arctic Crashes: People and Animals in the Changing North, eds I. Krupnik, and A. L. Crowell (Washington, DC: Smithsonian Scholarly Press), 317–335.
Crowell, A. L., Liddle, J., and Matson, M. (2012). Spatial correlation of archaeological sites and subsistence resources in the Gulf of Alaska. Alsk. Park Sci. 11, 4–9.
Crowell, A. L., and Mann, D. H. (1996). Human populations, sea level change, and the archaeological record of the northern Gulf of Alaska. Arct. Anthropol. 33, 16–37.
Crowell, A. L., and Mann, D. H. (1998). Archaeology and Coastal Dynamics of Kenai Fjords National Park, Alaska. Anchorage: National Park Service.
Crowell, A. L., Matson, M., and Mann, D. H. (2003). Implications of ‘punctuated productivity' for coastal settlement patterns: a GIS study of the Katmai Coast, Gulf of Alaska. Alsk. J. Anthropol. 1, 62–96.
Crowell, A. L., Steffian, A. F., and Pullar, G. L. (2001). Looking Both Ways: Heritage and Identity of the Alutiiq People. Fairbanks: University of Alaska Press.
Crowell, A. L., Yesner, D. R., Eagle, R., and Hansen, D. K. (2008). An historic Alutiiq village on the outer Kenai Coast: subsistence and trade in the early contact period. Alsk. J. Anthropol. 6, 225–251.
Crusius, J., Schroth, A. W., Gassó, S., Moy, C. M., Levy, R. C., Gatica, M., et al. (2011). Glacial flour dust storms in the Gulf of Alaska: hydrologic and meteorological controls and their importance as a source of bioavailable iron. Geophys. Res. Lett. 38, L06602. doi: 10.1029/2010GL046573
Danielson, S. L., Hennon, T. D., Monson, D. H., Suryan, R. M., Campbell, R. W., Baird, S. J., et al. (2022). Temperature variations in the northern Gulf of Alaska across synoptic to century-long time scales. Deep Sea Res. II 203, 105155. doi: 10.1016/j.dsr2.2022.105155
Davydov, G. I. (1977). Two Voyages to Russian America, 1802–1807, ed R. A. Pierce (Transl by C. Bearne). Kingston, ON: The Limestone Press.
De Laguna, F. (1956). Chugach Prehistory: The Archaeology of Prince William Sound, Alaska. Seattle: University of Washington Press.
Dekin, A. A. Jr., Cassell, M. S., Ebert, J. I., Camilli, E., Kerley, J. M., Yarborough, R., et al. (1993). Exxon Valdez Oil Spill Archaeological Damage Assessment, Final Report. Binghamton: The Research Foundation of the State University of New York.
Desjardins, S. (2020). Neo-Inuit strategies for ensuring food security during the Little Ice Age climate change episode, Foxe Basin, Arctic Canada. Quat. Int. 549, 163–175. doi: 10.1016/j.quaint.2017.12.026
Desjardins, S., and Jordan, P. D. (2019). Arctic archaeology and climate change. Annu. Rev. Anthropol. 48, 279–296. doi: 10.1146/annurev-anthro-102317-045901
Dotter, W. R. (1988). “1988 BIA ANSCA SEC. 14(H)1 Field Investigations in Prince William Sound and Adjacent Areas,” in Paper presented at the Annual Meeting of the Alaska Anthropological Association, Fairbanks.
Drew, G. S., and Piatt, J. F. (2002). “Oceanography of lower Cook Inlet,” in Response of Seabirds to Fluctuations in Forage Fish Density: Exxon Valdez Oil Spill Restoration Project Final Report, ed J. Piatt (Anchorage: Alaska Science Center, U. S. Geological Survey), 18–26.
Drew, G. S., Schoen, S. K., Hood, M. D., Arimitsu, M. L., and Piatt, J. F. (2005). North Pacific Pelagic Seabird Database (NPPSD) (ver. 4, 0. January 2023): U.S. Geological Survey Data Release Anchorage. doi: 10.5066/F7WQ01T3
Erlandson, J., Crowell, A. L., Wooley, C., and Haggarty, J. (1992). Spatial and temporal patterns in Alutiiq paleodemography. Arct. Anthropol. 29, 42–62.
Eslinger, D. L., Cooney, R. T., McRoy, C. P., Ward, A., Kline, T. C., Simpson, E. P., et al. (2001). Plankton dynamics: observed and modeled responses to physical conditions in Prince William Sound, Alaska. Fish. Oceanogr. 10 (Supplement 1), 81–96. doi: 10.1046/j.1054-6006.2001.00036.x
Fall, J. A., (ed.). (1991). Subsistence Harvests and Uses in Seven Gulf of Alaska Communities in the Second Year Following the “Exxon Valdez” Oil Spill. Alaska Department of Fish and Game, Division of Subsistence, Technical Paper No. 218. Anchorage.
Finkelstein, S. A., Ross, J. M., and Adams, J. K. (2009). Spatiotemporal variability in Arctic climates of the past millennium: implications for the study of Thule culture on Melville Peninsula, Nunavut. Arct. Antarct. Alp. Res. 41, 442–454. doi: 10.1657/1938-4246-41.4.442
Finney, B. P., Gregory-Eaves, I., Douglas, M. S. V., and Smol, J. P. (2002). Fisheries productivity in the northeastern Pacific Ocean over the past 2, 200. years. Nature 416, 729–733. doi: 10.1038/416729a
Finney, B. P., Gregory-Eaves, I., Sweetman, J., Douglas, M. S. V., and Smol, J. P. (2000). Impacts of climate change and fishing on Pacific salmon abundance over the past 300 years. Science NS 290, 795–799. doi: 10.1126/science.290.5492.795
Fitzhugh, B. (2003). The Evolution of Complex Hunter-Gatherers: Evidence from the North Pacific. New York, NY: Kluwer-Plenum Press. doi: 10.1007/978-1-4615-0137-4
Fitzhugh, B., Brown, W. A., Misarti, N., Takase, K., and Tremayne, A. H. (2022). Human paleodemography and paleoecology of the North Pacific Rim from the mid to late Holocene. Quat. Res. 108, 123–149. doi: 10.1017/qua.2022.35
Fitzhugh, B., Butler, V. L., Bovy, K. M., and Etnier, M. S. (2019). Human ecodynamics: a perspective for the study of long-term change in socioecological systems. J. Archaeol. Sci. Rep. 23, 1077–1094. doi: 10.1016/j.jasrep.2018.03.016
Fitzhugh, W. W. (1972). Environmental Archaeology and Cultural Systems in Hamilton Inlet, Labrador. Smithsonian Contributions to Anthropology No. 16. Washington DC: Smithsonian Institution.
Fitzhugh, W. W. (1977). Population movement and culture change on the Central Labrador coast. Ann. N. Y. Acad. Sci. 288, 481–497. doi: 10.1111/j.1749-6632.1977.tb33638.x
Fitzhugh, W. W. (2019). Paradise gained, lost, and regained: pulse migration and the Inuit archaeology of the Quebec Lower North Shore. Arct. Anthropol. 56, 52–76. doi: 10.3368/aa.56.1.52
Fitzhugh, W. W. (2020). “Riding the harp seal highway: modeling climate, sea ice pulsations, and Inuit migrations in the eastern Subarctic,” in Arctic Crashes: People and Animals in the Changing North, eds I. Krupnik, and A. Crowell (Washington DC: Smithsonian Scholarly Press), 79–99.
Francis, R. C., and Hare, S. R. (1994). Decadal-scale regime shifts in the large marine ecosystems of the North-East Pacific: a case for historical science. Fish. Oceanogr. 3, 279–291. doi: 10.1111/j.1365-2419.1994.tb00105.x
Friesen, T. M. (2016). “Pan-Arctic population movements: the early Paleo-Inuit and Thule Inuit migrations,” in The Oxford Handbook of the Prehistoric Arctic, eds T. M. Friesen, and O. K. Mason (New York, NY: Oxford University Press), 673–691. doi: 10.1093/oxfordhb/9780199766956.013.40
Friesen, T. M., and Arnold, C. (2008). The timing of the Thule migration: new dates from the western Canadian Arctic. Am. Antiq. 73, 527–538. doi: 10.1017/S0002731600046850
Friesen, T. M., Finkelstein, S. A., and Medeiros, A. S. (2020). Climate variability of the Common Era (AD 1-2000) in the eastern North American Arctic: impacts on human migrations. Quat. Int. 549, 142–154. doi: 10.1016/j.quaint.2019.06.002
Gedalof, Z., Mantua, N. J., and Peterson, D. L. (2002). A multi-century perspective of variability in the Pacific Decadal Oscillation: new insights from tree rings and coral. Geophys. Res. Lett. 29, 1–4. doi: 10.1029/2002GL015824
Gedalof, Z., and Smith, D. J. (2001). Interdecadal variability and regime-scale shifts in Pacific North America. Geophys. Res. Lett. 28, 1515–1518. doi: 10.1029/2000GL011779
Goering, J. J., Shiels, W. E., and Patton, C. J. (1973). “Primary production,” in Environmental Studies of Port Valdez, eds D. W. Hood, W. E. Shiels, and E. J. Kelley (Fairbanks, AK: Institute of Marine Sciences, University of Alaska), 251–279. Occasional Publication 3.
Grønnow, B. (2017). The Frozen Saqqaq Sites of Disko Bay, West Greenland. Qeqertasussuk and Qajaa (2400-900 BC). Copenhagen; Chicago, IL: Museum Tusculanum Press and University of Chicago Press. p. 490.
Haggarty, J. C., Wooley, C., Erlandson, J. M., and Crowell, A. L. (1991). The 1990 Exxon Cultural Resource Program: Site Protection and Maritime Cultural Ecology in Prince William Sound and the Gulf of Alaska. Anchorage: Exxon Shipping Company and Exxon Company.
Hanson, D. K. (2007). Analysis of fish remains from Verdant Cove Early Contact Village (XBS-029). Anchorage, AK: Littoral Zooarchaeology.
Hanson, D. K. (2009). Analysis of Fish Remains from the Denton Site (XBS-014), Aialik Bay, Kenai Fjords National Park, Alaska. Anchorage, AK: Littoral Zooarchaeology.
Hardesty, D. L. (1972). The human ecological niche. Am. Anthropol. 74, 458–466. doi: 10.1525/aa.1972.74.3.02a00150
Hare, S. R., and Mantua, N. J. (2000). Empirical evidence for North Pacific regime shifts in 1977 and 1989. Prog. Oceanogr. 47, 103–145. doi: 10.1016/S0079-6611(00)00033-1
Hastrup, K., Mosbech, A., and Grønnow, B. (2018a). Introducing the North Water: histories of exploration, ice dynamics, living resources, and human settlement in the Thule region. Ambio 47 (Suppl. 2), S162–S174. doi: 10.1007/s13280-018-1030-2
Hastrup, K., Oberborbeck Andersen, A., Grønnow, B., and Heide-Jørgensen, M. P. (2018b). Life around the North Water ecosystem: natural and social drivers of change over a millennium. Ambio 47 (Suppl. 2), S213–S225. doi: 10.1007/s13280-018-1028-9
Haynes, T. L., and Wolfe, R. J., (eds.). (1999). Ecology, Harvest, and Use of Harbor Seals and Sea Lions: Interview Materials from Alaska Native Hunters. Technical Paper 249. Juneau: Alaska Department of Fish and Game, Subsistence Division.
Heggie, D. T., Boisseau, D. W., and Burrell, D. C. (1977). Hydrography, Nutrient Chemistry, and Primary Productivity of Resurrection Bay, Alaska 1972-75. Institute of Marine Science Report No. R77-2. Fairbanks: University of Alaska Fairbanks.
Helser, T., Kastelle, C., Crowell, A. L., Ushikubo, T., Orland, I. J., Kozdon, R., et al. (2018). A 200-year archaeozoological record of Pacific cod (Gadus macrocephalus) life history as revealed through ion microprobe oxygen isotope rations in otoliths. J. Archaeol. Sci. Rep. 21, 1236–1246. doi: 10.1016/j.jasrep.2017.06.037
Hood, D. W., and Zimmerman, S. T., (eds.). (1986). The Gulf of Alaska: Physical Environment and Biological Resources. Alaska Office, Ocean Assessments Division, National Oceanic and Atmospheric Administration. Washington, DC: U. S. Government Printing Office. doi: 10.5962/bhl.title.60759
Hood, E. W., Fellman, J. B., Spencer, R. G. M., Hernes, P. J., Edwards, R., D'Amore, D. V., et al. (2009). Glaciers as a source of ancient and labile organic matter to the marine environment. Nature 462, 1044–1047. doi: 10.1038/nature08580
Hoover-Miller, A. (1994). Harbor Seal (Phoca vitulina) Biology and Management in Alaska. Report by Pacific Rim Research (Seward, Alaska) to the Marine Mammal Commission. Washington, DC.
Hoover-Miller, A., and Armato, P. (2018). Harbor seal use of glacier ice and terrestrial haul-outs in the Kenai Fjords, Alaska. Mar. Mamm. Sci. 34, 616–644. doi: 10.1111/mms.12470
Hoover-Miller, A., Atkinson, S., Conton, S., Prewitt, J., and Armato, P. (2011). Persistent decline in abundance of harbor seals Phoca vitulina richardsi over three decades in Aialik Bay, an Alaskan tidewater glacial fjord. Mar. Ecol. Prog. Ser. 424, 259–271. doi: 10.3354/meps08987
Hutchinson, I., and Crowell, A. L. (2007). Recurrence and extent of great earthquakes in southern Alaska during the late Holocene: an integrated analysis of radiocarbon evidence of crustal deformation and village abandonment. Radiocarbon 49, 1323–1385. doi: 10.1017/S0033822200043198
Iverson, S. J., Springer, A. M., and Bodkin, J. L. (2007). “Marine mammals,” in Long-Term Ecological Change in the Gulf of Alaska, ed R. B. Spies (Amsterdam: Elsevier), 114–135.
Johnson, J. F. C. (ed.)., (1984). Chugach Legends. Stories and Photographs of the Chugach Region. Anchorage: Chugach Alaska Corporation.
Jordan, R. H., and Knecht, R. A. (1988). “Archaeological research on western Kodiak Island, Alaska: the development of Koniag culture,” in The Late Prehistoric Development of Alaska's Native People, eds R. D. Shaw, R. K. Harritt, and D. E. Dumond (Anchorage: Alaska Anthropological Association), 225–306.
Kent, R., and McCallum, M. (1991). Report of Investigations for Chugach Alaska Corporation, Sites BLM AA-10720 through BLM AA41502. Bureau of Indian Affairs, Alaska Native Claims Settlement Act (ANCSA) Office. Anchorage.
Knecht, R. A. (1995). The Late Prehistory of the Alutiiq People: Culture Change on the Kodiak Archipelago from 1200–1750 A.D [Unpublished Ph.D. Dissertation]. Philadelphia, PA: Department of Anthropology, Bryn Mawr College.
Kopperl, R. E. (2003). Cultural Complexity and Resource Intensification on Kodiak Island, Alaska [Unpublished Ph.D. Dissertation]. Seattle, WA: Department of Anthropology, University of Washington.
Krupnik, I., and Crowell, A. L., (eds.). (2020). Arctic Crashes: People and Animals in the Changing North. Washington DC: Smithsonian Scholarly Press.
Kylafis, G., and Loreau, M. (2011). Ecological and evolutionary consequences of niche construction for its agent. Ecol. Lett. 14, 1072–1081. doi: 10.1111/j.1461-0248.2008.01220.x
Laland, K. N., and O'Brien, M. J. (2010). Niche construction theory and archaeology. J. Archaeol. Method Theory 17, 303–322. doi: 10.1007/s10816-010-9096-6
Larrance, J. D., Tennant, D. A., Chester, A. J., and Ruffio, P. A. (1977). “Phytoplankton and primary production in the northeast Gulf of Alaska and lower Cook Inlet,” in Environmental Assessment of the Alaskan Continental Shelf, Annual Reports of Principal Investigators for the Year ending March 1977, Vol. X (Boulder, CO: Outer Continental Shelf Environmental Assessment Program (OCSEAP)), 1–136.
Lewontin, R. C. (1983). “Gene, organism and environment,” in Evolution from Molecules to Men, ed D. S. Bendall (Cambridge: Cambridge University Press), 273–285.
Litzow, M. A., Ciannelli, L., Puerta, P., Wettstein, J. J., Rykaczewski, R. R., Opiekun, M., et al. (2019). Nonstationary environmental and community relationships in the North Pacific Ocean. Ecology 100, e02760. doi: 10.1002/ecy.2760
Litzow, M. A., and Mueter, F. J. (2014). Assessing the ecological importance of climate regime shifts: an approach from the North Pacific Ocean. Prog. Oceanogr. 120, 110–119. doi: 10.1016/j.pocean.2013.08.003
London, J. M., Yano, K. M., Richmond, E. L., Withrow, D. E., Dahle, S. P., Jansen, J. K., et al (2015). Observed Haul-out Locations for Harbor Seals in Coastal Alaska. Seattle: Alaska Fisheries Science Center, National Oceanic and Atmospheric Administration. Available online at: http://www.arcgis.com/home/webmap/viewer.html?url=https%3A%2F%2Fwebmap.afsc.noaa.gov%2Fmaps%2Frest%2Fservices%2Fpep_public%2Fakpvwypts%2FFeatureServerandsource=sd
Luehrmann, S. (2008). Alutiiq Villages under Russian and U. S. Rule. Fairbanks: University of Alaska Press.
Maio, C. V., Crowell, A. L., Sullivan, R. M., Buzard, R. M., Whitley, M. W., Bogardus, R. C., et al. (2019). Examining coastal dynamics and archaeological site evidence at a drowned cirque basin influenced by earthquakes and Little Ice Age glaciation. J. Coast. Res. 35, 814–834. doi: 10.2112/JCOASTRES-D-18-00074.1
Mann, D. H., and Crowell, A. L. (1996). A large earthquake occurring 700–800 years ago in Aialik Bay, southern Coastal Alaska. Canad. J. Earth Sci. 33, 117–126. doi: 10.1139/e96-012
Mann, D. H., Crowell, A. L., Hamilton, T. D., and Finney, B. P. (1998). Holocene geologic and climatic history around the Gulf of Alaska. Arct. Anthropol. 35, 112–131.
Mantua, N. J., and Hare, S. R. (2002). The pacific decadal oscillation. J. Oceanogr. 58, 35–44. doi: 10.1023/A:1015820616384
Mantua, N. J., Hare, S. R., Zhang, Y., Wallace, J. M., and Francis, R. C. (1997). A Pacific interdecadal climate oscillation with impacts on salmon production. Bull. Am. Meteorol. Soc. 78, 1069–1079. doi: 10.1175/1520-0477(1997)078<1069:APICOW>2.0.CO;2
Martin, R. L. (2004). “An overview of culturally related radiocarbon dates from Prince William Sound,” in Paper presented at the annual meeting of the Alaska Anthropological Association, 2004, Whitehorse, Yukon.
Maschner, H. D. G., Betts, M. W. Reedy-Maschner, K. L., and Trites, A. W. (2008). A 4500-year time series of Pacific cod (Gadus microcephalus) abundance: archaeology, oceanic regime shifts, and sustainable fisheries. Fish. Bull. 106, 386–394.
Mason, O. F. (2020). The Thule migrations as an analog for the early peopling of the Americas: evaluating scenarios of overkill, climate forcing, and scalar stress. PaleoAmerica 6, 308–356. doi: 10.1080/20555563.2020.1783969
Mathiassen, T. (1927). Archaeology of the Central Eskimos. Report of the Fifth Thule Expedition, 1921–24, Vol. 4. Copenhagen: Gyldendal.
McGowan, J. A., Cayan, D. R., and Dorman, L. M. (1998). Climate-ocean variability and ecosystem response in the northeast Pacific. Science 281, 210–217. doi: 10.1126/science.281.5374.210
McMahan, J. D., and Holmes, C. E. (1987). Report of Archaeological and Historical Investigations at Nuka Island and the Adjacent Kenai Peninsula, Gulf of Alaska. Anchorage: Office of History and Archaeology, Alaska Department of Natural Resources.
Mills, R. O. (1994). Radiocarbon calibration of archaeological dates from the central Gulf of Alaska. Arct. Anthropol. 31, 126–149.
Mobley, C. M., Haggarty, J. C., Utermohle, C. J., Eldridge, M., Reanier, R. E., Crowell, A. L., et al. (1990). The 1989 Exxon Valdez Cultural Resource Program. Anchorage: Exxon Company USA.
Muhs, D. R., Budahn, J. R., Mcgeehin, J. P., Bettis, E. A., Skipp, G., Paces, J. B., et al. (2013). Loess origin, transport, and deposition over the past 10,000 years, Wrangell-St. Elias National Park, Alaska. Aeolian Res. 11, 85–99. doi: 10.1016/j.aeolia.2013.06.001
Muto, M. M., Helker, V. T., Delean, B. J., Young, N. C., Freed, J. C., Angliss, R. P., et al. (2021). Marine Mammal Stock Assessments, 2021. U.S. Department of Commerce, National Oceanic and Atmospheric Administration Technical Memo NMFSAFSC-441. Springfield, VA: National Technical Information Service, USDC.
National Marine Fisheries Service (2006). Harbor Seal Haul-Out Locations and Stellar Sea Lion Sites. Juneau: National Marine Mammal Laboratory, NOAA Alaska Fisheries Science Center, National Marine Fisheries Service.
National Oceanic and Atmospheric Administration. (1988). West Coast of North America: Coastal and Ocean Zones Strategic Assessment: Data Atlas. National Marine Fisheries Service of the National Oceanic and Atmospheric Administration, United States Department of Commerce, Rockville, MD, United States.
Newman, M., Alexander, M. A., Ault, T. R., Cobb, K. M., Deser, C., Di Lorenzo, E., et al. (2016). The Pacific decadal oscillation, revisited. J. Clim. 29, 4399–4427. doi: 10.1175/JCLI-D-15-0508.1
Nishimoto, M., and Rice, B. (1987). A re-survey of Seabirds and Marine Mammals along the South Coast of the Kenai Peninsula, Alaska During the Summer of 1986. Seward, AK: U.S. Fish and Wildlife Service and Kenai Fjords National Park.
Odling-Smee, J., Erwin, D. H., Palkovacs, E. P., Feldman, M. W., and Laland, K. N. (2013). Niche construction theory: a practical guide for ecologists. Q. Rev. Biol. 88, 3–28. doi: 10.1086/669266
Odling-Smee, J., Laland, K. N., and Feldman, M. (2003). Niche Construction. Monographs in Population Biology, No. 37. Princeton, NJ: Princeton University Press.
Overland, J., Rodionov, S., Minobe, S., and Bond, N. (2008). North Pacific regime shifts: definitions, issues, and recent transitions. Prog. Oceanogr. 77, 92–102. doi: 10.1016/j.pocean.2008.03.016
Parsons, T. R. (1986). “Ecological relations,” in The Gulf of Alaska: Physical Environment and Biological Resources, eds D. W. Hood, and S. T. Zimmerman (Washington, DC: Alaska Office, Ocean Assessments Division, National Oceanic and Atmospheric Administration), 561–570.
Payne, R. J., and Blackford, J. J. (2008). Extending the late Holocene tephrochronology of the central Kenai Peninsula, Alaska. Arctic 61, 243–254. doi: 10.14430/arctic22
Piatt, J. F., and Springer, A. M. (2007). “Marine ecoregions of Alaska,” in Long-term Ecological Change in the Northern Gulf of Alaska, ed R. R. Spies (Amsterdam: Elsevier), 522–526.
Praetorius, S. K., Mix, A. C., Walczak, M. H., Wolhowe, M. D., and Addison, J. A. F. G. (2015). North Pacific deglacial hypoxic events linked to abrupt ocean warming. Nature 527, 362–369. doi: 10.1038/nature15753
Pratt, K., and Heyes, S. (eds.), (2022). Memory and Landscape: Indigenous Responses to a Changing North. Edmonton: Athabasca University Press. doi: 10.15215/aupress/9781771993159.01
Reger, D. R., and Boraas, A. (1996). “An overview of the radiocarbon chronology in Cook Inlet prehistory,” in Adventures Through Time: Readings in the Anthropology of Cook Inlet, Alaska, eds N. Y. Davis, and W. E. Davis (Anchorage: Cook Inlet Historical Society), 155–172.
Reger, D. R., McMahan, J. D., and Holmes, C. E. (1991). Effect of Crude Oil Contamination on Some Archaeological Sites in the Gulf of Alaska, 1991 Investigations. Anchorage: Office of History and Archaeology, Alaska Department of Natural Resources.
Ribeiro, S., Limoges, A., Massé, G., Johansen, K. L., Colgan, W., Weckström, K., et al. (2021). Vulnerability of the North Water ecosystem to climate change. Nat. Commun. 12, 1–12. doi: 10.1038/s41467-021-24742-0
Rogers, L. R., Schindler, D. E., Lisi, P. J., Holtgrieve, G. W., Leavitt, P. R., Bunting, L., et al. (2013). Centennial-scale fluctuations and regional complexity characterize Pacific salmon population dynamics over the past five centuries. Proc. Natl. Acad. Sci. U.S.A. 110, 1750–1755. doi: 10.1073/pnas.1212858110
Sambrotto, R. N., and Lorenzen, C. J. (1986). “Phytoplankton and primary production,” in The Gulf of Alaska: Physical Environment and Biological Resources, eds D. W. Hood, and S. T. Zimmerman. U. S. Department of Commerce, National Oceanic and Atmospheric Administration (Washington DC: Government Printing Office), 249–282.
Schaaf, J. (1989). The Denton collection, Verdant Cove, Aialik Bay, Kenai Fjords National Park. Unpublished report. Anchorage: National Park Service, Alaska Regional Office.
Schaff, J., and Johnson, L. (1990). Upland investigations at SEL-188, Kenai Fjords National Park. Anchorage: National Park Service.
Smith, B. D. (2007). Niche construction and the behavioral context of plant and animal domestication. Evol. Anthropol. 16, 188–199. doi: 10.1002/evan.20135
Smithsonian Institution and Pratt Museum (2003). Archaeology and Memory: Ancestral Alutiiq Villages on the Outer Kenai Coast, Alaska. Video (21 minutes). Produced by the Smithsonian Institution (Arctic Studies Center) and Pratt Museum in cooperation with the Native Villages of Nanwalek, Port Graham, and Seldovia. Anchorage: Talking Circle Media.
Speckman, S., Piatt, J., Mintevera, C., and Parrish, J. (2005). Parallel structure among environmental gradients and three trophic levels in a subarctic estuary. Prog. Oceanogr. 66, 25–65. doi: 10.1016/j.pocean.2005.04.001
Spies, R. B. (ed.)., (2007). Long-Term Ecological Change in the Northern Gulf of Alaska. Amsterdam: Elsevier.
Stabeno, P. J., Bell, S., Cheng, W., Danielson, S., Kachel, N. B., Mordy, C. W., et al. (2016). Long-term observations of Alaska Coastal Current in the northern Gulf of Alaska. Deep Sea Res. II 132, 24–40. doi: 10.1016/j.dsr2.2015.12.016
Stanek, R. T. (1985). Patterns of Wild Resource Use in English Bay and Port Graham, Alaska. Technical Paper No. 104. Anchorage, AK: Division of Subsistence, Alaska Department of Fish and Game.
Stanek, R. T. (1999). Ethnographic Overview and Assessment for Nanwalek and Port Graham. Anchorage: Alaska Department of Fish and Game, Division of Subsistence.
Steffian, A. F., Leist, M. A., Haakanson, S. D., and Saltonstall, P. G. (2015). Kal'unek from Karluk: Kodiak Alutiiq History and the Archaeology of the Karluk One Village Site. Fairbanks: University of Alaska Press.
Steller, G. W. (1988). Journey of a Voyage with Bering, 1741–1742, ed O. W. Frost (Transl. by M. A. Engel, and O. W. Frost). Stanford, CA: Stanford University Press. doi: 10.1515/9781503621459
Stratton, L., and Chisum, E. B. (1986). Resource Use Patterns in Chenega, Western Prince William Sound: Chenega in the 1960s and Chenega Bay 1984-1986. Anchorage: Alaska Department of Fish and Game, Division of Subsistence, Technical Paper No. 139.
Syvitski, J. P. M., Burrell, D. C., and Ske, J. M. (1987). Fjords: Processes and Products. New York, NY: Springer-Verlag. doi: 10.1007/978-1-4612-4632-9
Tozzi, N. (2012). Commercial Influence on the Outer Coast Sugpiaq [Unpublished M. A. thesis]. Fairbanks: Department of Anthropology, University of Alaska Fairbanks.
U. S. Fish and Wildlife Service (2006). The Beringian Seabird Colony Database. Junea: U. S. Fish and Wildlife Service, Migratory Bird Management.
Ver Hoef, J. M., and Frost, K. (2003). A Bayesian hierarchical model for monitoring harbor seal changes in Prince William Sound, Alaska. Environ. Ecol. Stat. 10, 201–209.
Vibe, C. (1967). Arctic animals in relation to climate fluctuations: the Danish zoogeographical investigations in Greenland. Medd. Grønl. 170, 1–226.
Walker, A. (1982). An Account of a Voyage to the North-West Coast of America in 1785 and 1786. Edited by R. Fisher and J. M. Bumstead. Seattle, WA: University of Washington Press.
Weingartner, T. (2007). “The physical environment of the Gulf of Alaska,” in Long-Term Ecological Change in the Northern Gulf of Alaska, ed R. B. Spies (Amsterdam: Elsevier), 11–47.
West, C. F., Wischniowski, S., and Johnston, C. (2012). Pacific cod (Gadus macrocephalus) as a paleothermometer: otolith oxygen isotope reconstruction. J. Archaeol. Sci. 39, 3277–3283. doi: 10.1016/j.jas.2012.05.009
Wiles, G. C., D'Arrigo, R. C., and Jacoby, G. C. (1998). Gulf of Alaska atmosphere-ocean variability over centuries inferred from coastal tree-ring records. Clim. Change 38, 289–306. doi: 10.1023/A:1005396027562
Wiles, G. C., D'Arrigo, R. D., Barclay, D., Wilson, R. S., Jarvis, S. K., Vargo, L., et al. (2014). Surface air temperature variability reconstructed with tree rings for the Gulf of Alaska over the past 1, 200. years. Holocene 24, 198–208. doi: 10.1177/0959683613516815
Wilson, R., Wiles, G., and D'Arrigo, R. (2007). Cycles and shifts: 1,300 years of multi-decadal temperature variability in the Gulf of Alaska. Clim. Dyn. 28, 425–440. doi: 10.1007/s00382-006-0194-9
Workman, W. B. (1977). New data on the radiocarbon chronology of the Kachemak Bay sequence. Anthropol. Pap. Univ. Alsk.18, 31–36.
Workman, W. B. (1996). “Human colonization of the Cook Inlet basin before 3, 000. Years Ago,” in Adventures Through Time: Readings in the Anthropology of Cook Inlet, Alaska, eds by N. Y. Davis, and W. E. Davis (Anchorage: Cook Inlet Historical Society), 37–48.
Workman, W. B., Klein, J., Testaguzza, M., and Zollars, P. (1993). “1992 test excavations at the Sylva Site (SEL 245): a stratified late Ocean Bay occupation in upper Kachemak Bay, Kenai Peninsula, Alaska,” in Paper presented at the annual meeting of the Alaska Anthropological Association, Anchorage.
Workman, W. B., Lobdell, J. E., and Workman, K. W. (1980). Recent archaeological work in Kachemak Bay, Gulf of Alaska. Arctic 33, 385–399. doi: 10.14430/arctic2573
Workman, W. B., Workman, K. W., and Yesner, D. R. (1998). “1997 excavations at SEL-027: a later prehistoric site at Port Graham, Kenai Peninsula, Alaska,” in Paper presented at the annual meeting of the Alaska Anthropological Association, Anchorage.
Workman, W. B., and Workman., K. W. (1988). “The last 1,300 years of prehistory in Kachemak Bay: where later is less,” in The Late Prehistoric Development of Alaska's Native People (AURORA Vol. 4), eds R. D. Shaw, R. K. Harritt, and D. E. Dumond (Anchorage: Alaska Anthropological Association), 339–354.
Yarborough, L. F. (1997). “Site Specific Archaeological Restoration at SEW-440 and SEW-488. Exxon Valdez Oil Spill Restoration Project 95007B Final Report, Forest Service Report No. R10-TP-69. Anchorage: USDA Forest Service, Chugach National Forest.
Yarborough, L. F. (1998). “Faunal analysis, kenai fjords archaeological survey,” in Archaeology and Coastal Dynamics of Kenai Fjords National Park, eds A. L. Crowell, and D. H. Mann (Anchorage: National Park Service, Alaska Region), A1–A38.
Yarborough, L. F. (2000). Prehistoric and early historic subsistence patterns along the north Gulf Coast of Alaska [Unpublished Ph.D. Dissertation]. Madison, WI: University of Wisconsin.
Yarborough, M. R., and Yarborough, L. F. (1996). Uqciuvit: A Multicomponent Site in Northwestern Prince William Sound, Alaska. Final Report. Contract No. 53-01114-7-00132. Anchorage: USDA Forest Service, Chugach National Forest.
Yarborough, M. R., and Yarborough, L. F. (1998). Prehistoric maritime adaptations of Prince William Sound and the Pacific Coast of the Kenai Peninsula. Arct. Anthropol. 35, 132–145.
Yesner, D. R. (1992). Evolution of subsistence in the Kachemak Tradition: evaluating the North Pacific maritime stability model. Arct. Anthropol. 29, 167–181.
Keywords: climate change, migration, Inuit, resilience, glacial fiord, glaciomarine ecosystem, human niche construction, Alaska
Citation: Crowell AL and Arimitsu M (2023) Climate change and pulse migration: intermittent Chugach Inuit occupation of glacial fiords on the Kenai Coast, Alaska. Front. Environ. Archaeol. 2:1145220. doi: 10.3389/fearc.2023.1145220
Received: 15 January 2023; Accepted: 04 April 2023;
Published: 26 April 2023.
Edited by:
Cheryl Makarewicz, University of Kiel, GermanyReviewed by:
David Kaniewski, The Université Toulouse III - Paul Sabatier, FranceCopyright © 2023 Crowell and Arimitsu. This is an open-access article distributed under the terms of the Creative Commons Attribution License (CC BY). The use, distribution or reproduction in other forums is permitted, provided the original author(s) and the copyright owner(s) are credited and that the original publication in this journal is cited, in accordance with accepted academic practice. No use, distribution or reproduction is permitted which does not comply with these terms.
*Correspondence: Aron L. Crowell, Y3Jvd2VsbGFAc2kuZWR1
Disclaimer: All claims expressed in this article are solely those of the authors and do not necessarily represent those of their affiliated organizations, or those of the publisher, the editors and the reviewers. Any product that may be evaluated in this article or claim that may be made by its manufacturer is not guaranteed or endorsed by the publisher.
Research integrity at Frontiers
Learn more about the work of our research integrity team to safeguard the quality of each article we publish.