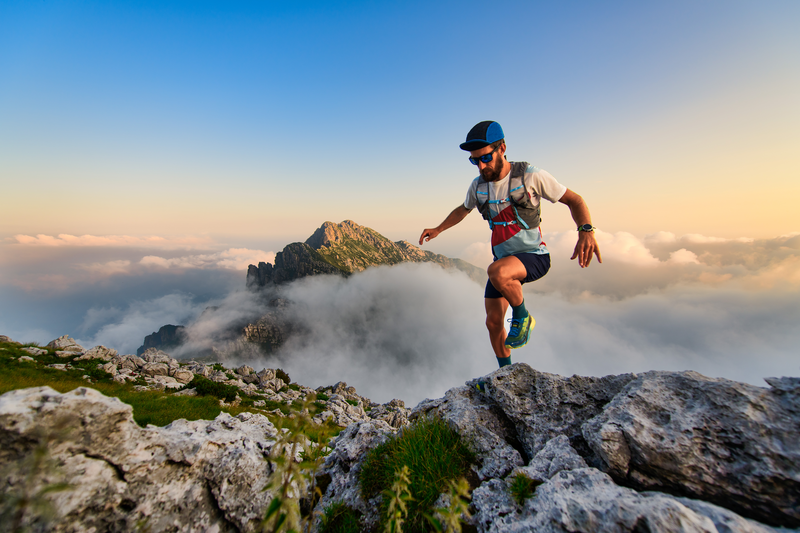
94% of researchers rate our articles as excellent or good
Learn more about the work of our research integrity team to safeguard the quality of each article we publish.
Find out more
MINI REVIEW article
Front. Drug Saf. Regul. , 27 June 2022
Sec. Substance-Based Medical Devices
Volume 2 - 2022 | https://doi.org/10.3389/fdsfr.2022.933471
This article is part of the Research Topic Medical Devices made of substances for human health: a challenge in terms of efficacy, safety and sustainability View all 9 articles
Medical Devices Made of Substances (MDMS) are increasingly used in the healthcare system alongside classic medicinal products and constitute an important field of experimentation and innovation in the biomedical field. In fact, these products are rapidly establishing themselves as a valuable therapeutic resource and are available in various forms including, but not limited to, creams, syrups, nasal or oropharyngeal sprays, and eye drops. MDMS are marketed to treat different diseases and the advantages and benefits of the use of these products can be claimed, once proven their clinical activity. What are the differences between medicinal products and MDMS? The substantial difference lies in the mechanism of action: the first case is based on pharmacological, metabolic, and immunological actions while the second one is based on mechanical, or chemical/physical action. Sometimes the boundaries are not well defined and there is a need for a reassessment and a consensus on the underlying concepts and definitions, also in the light of the increasing ability to recognize molecular mechanisms underneath the action of several substances not acting through an easy recognizable unique target (as a receptor, for example). In the present paper, we discuss the role of eye drops as an example of MDMS used in glaucoma, a widely diffused eye disease. The choice is due to the fact that some products used in this field of application and containing similar substances are marketed either as medicinal products or as medical devices or, using other dosage forms, as food supplements. Accordingly, it is important to underscore in the various cases what may be the principal mode of action and the contribution of additional mechanisms as derived, for example, from system pharmacology data. Their analysis may help to exemplify some of the problems around the sometimes fuzzy border between MDMS and medicinal products suggesting the need for new definitions and regulatory decisions about MDMS.
Medical devices made of substances (MDMS) are health products used to cure or prevent an illness. From the patient’s point of view are hardly distinguishable from conventional medicinal products and they should comply with strict regulatory definitions involving in their therapeutic action only physicochemical and not pharmacological, immunological, or metabolic mechanisms as, usually, medicinal products do.
Indeed, medical devices are vastly used in the healthcare system alongside conventional medicinal products. The EU Medical Devices Regulation (Regulation (EU) 2017/745) recently placed several devices under new classification rules (EUR-Lex - 02017R0745-20200424 - EN - EUR-Lex, 2022). MDMS are designed to treat various diseases, and patients are expected to obtain advantages and benefits with the use of these products. Clinical data are required to claim any therapeutic activity and the safety of the device should be corroborated by a post-market clinical follow-up. It should also be stressed that medical devices may be composed of substances or combination of substances which may give origin to whole body exposure (see Annex VIII of EUR-Lex - 02017R0745-20200424 - EN - EUR-Lex, 2022), going beyond the physicochemical mean thus requiring further refinement of the studies on the mechanism of action.
Even if the European regulatory constraint defines precise boundaries between MDMS and medicinal products in terms of mode of action (physical-chemical versus pharmacological, immunological, metabolic), this definition appears to be limited and insufficient to describe the increased complexity of the biological mechanisms elicited by several substances included some of those present in the MDMS. The regulatory setting is founded on the classical thinking of a pharmacological (immunological, metabolic) action, based on an easy and traceable primary hierarchically organized target and a lock-key interaction between a substance and its target. This definition is not incorrect, but it is presently insufficient to explain the biological and therapeutic effects of several substances and there is the need to expand and overcome this dated setting while defining the mode of action of a medicinal preparation, an MDMS, or, in general, a natural substance. The definition of pharmacological mechanism of action has been recently amended and further detailed in the non-binding guideline proposed by the Medical Device Coordination Group Document (MDCG 2022-5, 2022) substantially expanding the possibility to recognize a mechanism as belonging to pharmacological, immunological, metabolic domain (as in the case, for example of substances of herbal origin) when the principal mode of action is complex and difficult to define or to tribute to a specific substance. On the other hand, the same document is open to the possibility that a product containing such substances having pharmacological action could be qualified as a MDMS if the pharmacological action is ancillary to the principal intended action of the device.
In order to discuss these points, we decided to deal with a pathology, glaucoma, and a substance, citicoline, both topics on which we accumulated some direct experience in the past years.
It is estimated that 67 million people worldwide have glaucoma and glaucoma is the second leading cause of irreversible blindness. Glaucoma is a disease in which increased intraocular pressure is the leading cause of a subsequent degeneration of the axons of retinal ganglion cells (RGCs), which make up the optic nerve. The neurodegenerative process can progress in spite of intraocular pressure control (Davis et al., 2016). The loss of RGCs leads to loss of vision, and if untreated, to blindness (Lavik et al., 2011; Fahmideh et al., 2021). Drugs commonly used for glaucoma treatment aim to decrease intraocular pressure, mostly in form of eye drops, which, according to the clinician intention, should slow the rate of disease progression sufficiently to avoid functional impairment from the disease. Eye drops used in managing glaucoma decrease eye pressure by helping the eye’s fluid to drain better and/or decreasing the amount of fluid made by the eye. Drugs to treat glaucoma are classified by their active ingredient. These include prostaglandin analogs, beta-blockers, alpha agonists, carbonic anhydrase inhibitors, and rho kinase inhibitors. In addition, combination drugs are available for patients who require more than one type of medication. An older class of medications, the cholinergic agonists (such as pilocarpine) are not commonly used nowadays due to their side effects (Weinreb et al., 2014). Considerable efforts have been made to develop neuroprotective glaucoma treatments that prevent optic nerve damage. With the development of neurotrophic, antioxidant, anti-ischemic, anti-inflammatory, antiapoptotic, and immunomodulatory therapeutic approaches, the broad field of neuroprotection in glaucoma shows progress in reducing neurodegeneration and thus stabilizing visual function in experimental studies. Unfortunately, no firm evidence exists that these agents can prevent long-term disease progression in patients with glaucoma, and still, there is a long way from basic research to the clinic (Weinreb et al., 2014; Jünemann et al., 2021). Complementary and alternative medicine is meant to be used as adjuncts to traditional therapy, including oral food supplements and MDMS (usually in the form of eye drops). It is estimated that 5–15% of glaucoma patients, reportedly spending billions of dollars annually, take some form of alternative medicine based only on their impression that it will help treat their glaucoma (John Hetherington, 2013). Nutritional supplementation comprises a broad array of products intended for ingestion to meet essential nutritional requirements. These products can be categorized as vitamins, minerals, herbals, botanicals, amino acids, fatty acids, and other dietary supplements used individually or in combination (Fahmideh et al., 2021). Regarding MDMS used in glaucoma, some of the claims lay on neuroprotection (by restoring the integrity of retinal ganglion cell membrane) and antioxidant activities including topical coenzyme Q10, citicoline, hyaluronic acid, mannitol, and vitamins B12 and E alone or in combinations. It should be mentioned that, so far, no neuroprotective drugs have been approved by the FDA and the clinical studies behind these substances are few and the majority limited to non-randomized ones.
Among the different substances, citicoline is a challenging example, worthy of attention. Indeed, citicoline has been used in several countries for several decades and, based on its properties and route of administration, this substance can be used as a drug, as a food supplement, as a food for special medical purposes, or can be dispensed as an MDMS.
Citicoline, the generic name of the International Nonproprietary Name of cytidine-5′-diphosphocholine (CDP-choline, CDPCho), is a particular molecule with psychic stimulating and nootropic activity (Adibhatla and Hatcher, 2002). In Japan and Europe, citicoline was originally used as a prescription injectable drug for the treatment of cerebrovascular and cognition disorders in people who are healing from a stroke. Nowadays, it is world widely used as an over counter dietary supplement.
Citicoline plays a vital role in the biosynthesis of phospholipids and their precursors and in maintaining the phospholipid components in the cell membranes. Its mechanism of action as well as its biological effects are multifactorial and include, but are not limited to, 1) preservation of cardiolipin and sphingomyelin; 2) restoration of phosphatidylcholine; 3) stimulation of glutathione synthesis; 4) reduction of glutamate concentration; 5) rescue of mitochondrial function, preventing neural apoptosis; 6) synthesis of myelin; 7) improvements of acetylcholine synthesis. These actions (see also Oddone et al., 2021 for a review) may lead to the prevention of endothelial dysfunction and exert a neuroprotective role of the retina (Pascale et al., 2012; Parisi et al., 2018). Thus, the neurotherapeutic effect of citicoline could be multifarious, mainly by improving neuronal membrane integrity, maintaining cellular communications with its environment, reducing oxidative stress, and improving the synthesis of neurotransmitters such as acetylcholine and dopamine. There is, in fact, evidence of a clinical effect of citicoline on several neurodegenerative diseases such as Parkinson’s disease, senile and vascular dementia, and stroke (Vale, 2008; Alvarez-Sabín et al., 2013; Gareri et al., 2017; Mehta et al., 2019).
Indeed, the route of administration, dosage form, and consumption do affect its indication. When this molecule is given, it is metabolized, resulting in the production of choline. The latter is a precursor of acetylcholine, one of the most important neurotransmitters of our nervous system, involved in numerous cognitive functions, such as, for example, memory and attention (Grieb et al., 2015). In fact, nootropic substances generally carry out their actions by promoting the production of neurotransmitters, providing the body with the molecules necessary for their synthesis (Gandolfi et al., 2020).
Recently, due to its neuroprotective properties, citicoline has been proposed and studied as a complementary treatment of glaucoma (both as special food for medical purposes and as MDMS available as eye drops, in the latter case in association with hyaluronic acid, based on its activities in preserving the cell membrane, see below for further comments) (See Table 1).
A recent review extensively summarized the relationship between the cholinergic nervous system and visual function and the potential implications for glaucoma neuroprotection and/or neuroenhancement (Faiq et al., 2019).
Nevertheless, in 2014, EFSA (European Food Security Authority) pronounced on the scientific substantiation of a health claim related to the new food cytidine 5-diphosphocholine and maintenance of normal vision in elderly subjects since middle age. EFSA concluded that a cause-and-effect relationship has not been established between the consumption of CDP-choline and the maintenance of normal vision; therefore, the previously mentioned health claim cannot be supported (Agostoni et al., 2014).
In the aforementioned examples the use of citicoline in symptomatic disease (glaucoma), is proposed whereas its intake by the asymptomatic general population for possible prophylaxis of this disease is not considered as supported.
Citicoline in eye drops can counteract the visual impairment of glaucoma (see also Table 1 summarizing the main characteristics of clinical studies with citicoline in patients with glaucoma). Notably citicoline (2%) eye drop administration can give origin to substantial intravitreal concentrations of the compound (Carnevale et al., 2019). A study highlighted that the combination of citicoline in eye drops reduces eye pressure and slows down both anatomical and functional glaucomatous damage (Rossetti et al., 2020a). The study results showed that if glaucoma patients are accompanied by eye drops containing citicoline, in addition to ocular hypotensive therapy, the glaucomatous damage slows down significantly. Literature data show the positive effects of citicoline in glaucoma and more general in neurodegenerative diseases (Parisi et al., 1999, 2008, 2015; Ottobelli et al., 2013). It is interesting to note that in some studies (Roberti et al., 2014; Parisi et al., 2015, 2019), when glaucomatous patients were treated with citicoline eye drops, the improvement of retinal ganglion cell function (detected by pattern electroretinogram) and neural conduction along the visual pathways (detectable by shortening of visual evoked potentials) were observed. These outcomes demonstrate that citicoline not only prevents the progression of glaucoma but may assist the functional recovery of injured retinal ganglion cells as shown by recovery in the nerve signal conduction in treated patients possibly due to the RGC membrane stabilization (Parisi et al., 2015, 2019).
Once again, all the eye drops preparations containing citicoline used in these studies were medical devices and, as highlighted before, a medical device should not base its activity on pharmacological, immunological, or enzymatic properties. The view is not easy to be reconciled with the observed actions unless played exclusively at the plasma membrane level preserving its integrity.
From a more general point of view it will be important to thoroughly compare the doses used in the various studies following the different ways of administration to gain information on the citicoline eye levels when given topically and systemically.
According to the literature data, there is the possibility that citicoline, given systemically, as a food supplement or food for special medical purposes, may act through its multiple interactions (described in the previous sections and also depicted in Figure 1) which do not fit the definition of pharmacological (immunologic, metabolic) mechanism because the final effect derives from complex interactions that bring about changes in a way that cannot be pinpointed at the single target/receptor level (Bilia et al., 2021).
Figure 1. PPI (protein-protein interaction) Network. The chemical structure of Citicoline was imported into SwissTargetPrediction data library (www.swisstargetprediction.ch) and the target of citicoline was predicted (Gfeller et al., 2014). In DisGenNet (https://disgenet.org/) the keyword of “Glaucoma” was selected to collect the targets of this disease. In order to analyze the interaction between target proteins, the targets of citicoline and glaucoma were selected and imported into the STRING database (https://string-db.org); the interactions were analyzed by selecting “Homo sapiens” as organism and setting the confidence basis to 0.4 as done by Lian and Zheng (Liang et al., 2016; Zheng et al., 2021). The image uses connectivity strength as the driving force for the layout, posing strongly connected nodes closely together, at the same time the more edge enters each node, the more involved each enzyme is in the pathogenesis of glaucoma (edges represent functional associations) (ACDY10: adenylate cyclase type 10; AKT1: protein kinase B (serine-threonine specific protein kinases); CA12: carbonic anhydrase 12; CA2: carbonic anhydrase 2; CA9: carbonic anhydrase 9; CASP3: caspase-3; EDNRA: endothelin receptor type A; EP300: histone acetyltransferase p300; HDAC1: histone deacetylase 1; HSPA1A: heat shock protein family A; MDM2: mouse double minute 2; MMP1: matrix metallo proteinase-1; MMP2: matrix metallo proteinase-2; MMP3: matrix metallo proteinase-3; MMP9: matrix metallopeptidase 9; P2RY6: pyrimidinic receptor P2Y6; VHL: Von Hippel-Lindau tumor suppressor).
As suggested by some of the nodes in Figure 1 there are elements indicating citicoline involvement in glaucoma in pathways known to have an important role in neurodegeneration and apoptosis. Neurodegenerative pathways are of interest in the development of glaucoma since this condition is recognized not only as an ocular disease but also as a neurodegenerative disorder. In these years, many experimental and clinical studies have shown that in glaucoma, neuronal degeneration occurs not only at the level of the retina and optic nerve but also along the entire visual pathway and the brain.
A pathway that acts on glaucoma pathogenesis is caspase-3 (CASP3), citicoline has a potential neuroprotective effect by being involved in apoptosis through the CASP3 target and therefore management of neurodegenerative disorders. The citicoline effect is attributed to the control of neuronal apoptosis and to the induction of the regeneration of newborn RGCs neurites in experimental models including retinal explants and rat optic nerve crush model (Oshitari et al., 2010; Kitamura et al., 2019). In an in vitro study, citicoline administration to rat primary retinal cell cultures protected from apoptosis, by means of a reduced frequency of caspases activation and accumulation of apoptosis markers, in the presence of glutamate-induced excitotoxicity and high glucose challenge (Matteucci et al., 2014). In addition, in a recent study on a methanol-intoxicated retina model in rats, it is hypothesized that citicoline is able to minimize the loss of retinal ganglion cells and the disruption of photoreceptors, to suppress ganglion layer edema, to increase the expression of the antiapoptotic BCL-2 protein, and finally to decrease the expression of the proapoptotic caspase-3 protein (Laksmita et al., 2021).
The treatment with eye drops containing citicoline may be effective in suppressing oxidative stress and controlling inflammation in UVB corneal injury. Not only CASP3 was evaluated but also other targets of the protein-protein interactions as Matrix metalloproteinase (MMP) −2 and −9. In particular, after immunofluorescent staining and Western blot analysis, an increased MMP-2, -9, and caspase-3 in the UVB-only group compared with the UVB/citicoline group have been shown. Citicoline treatment may be effective in suppressing oxidative stress and consequently controlling inflammation in UVB corneal injury. Citicoline exerts this effect by inhibiting lipid peroxidation and increasing antioxidant defense mechanisms (Tokuc et al., 2021).
AKT1 also is an interesting “crowded” node emerging from Figure 1. Indeed the PI3K/AKT pathway plays a role in neurodegeneration and in glaucoma, being involved in retinal ganglion cells and trabecular meshwork cell apoptosis, and in autophagy (He et al., 2018). However, as far as to our knowledge, there are only indirect data showing in animal studies that citicoline may act regulating such pathway in a radiation-induced brain injury rodent model (Abdel-Aziz, Moustafa and Saada., 2021).
As it emerges from the previous paragraphs citicoline has many biological interactions with cellular mechanisms. As depicted in Figure 1 a bioinformatic analysis based on the interaction between targets of citicoline and glaucoma involved genes provides a conspicuous list of plausible targets, including some major crossroad intersections with neurodegeneration, apoptotic processes, vascular and metabolic pathways linked to oxidative stress. The questions arising are: 1) can all these nodes and putative pathways be confirmed by direct experimental data? 2) are these pathways engaged at the doses used clinically and through which route of administration? And, further, do these mechanisms fit exactly any of the present regulatory constraints to distinguish whether the product is an MDMS or a medicinal product?
The findings of the studies briefly summarized above confirm the suggestion stemming out from the bioinformatic model involving multiple targets and a network of various correlators. The relevance of some of these targets were already suggested by clinical studies or literature searching whereas others are presently based only on bioinformatics data and are waiting for an experimental confirmation.
The case of the medical applications of citicoline and in particular of its use of glaucoma is emblematic of the difficulties of classifying some substances neatly and unambiguously as belonging to a regulatory class (medicinal product, medical device based on substances, food supplement, food for special medical purposes) (as also summarized in Figure 2). There are several variables including commercial choices (development investments, market access easiness, price/reimbursement) and the difficulties emerging when examining the mechanism of action of a given substance. As far as the latter point, the new investigational and bioinformatic techniques have opened to the concept of system pharmacology, which may apply to a single substance having multiple targets (as in the citicoline example) as well as to complex mix of substances, as derived from natural sources, for which is not possible to tribute the effect to a an easy and traceable primary hierarchically organized target and a lock-key interaction between a substance and its target, but rather to the resultant balanced action on multiple targets (see also Racchi et al., 2016; Bilia et al., 2021). In some cases these substances have already an history of either food or medical (albeit not registered) use in humans and a known profile of safety. Presently their development either as drugs or as MDMS is paved by difficulties and one is the discrimination between pharmacological and non-pharmacological mode of action that, as shown in the case of citicoline, may not be easy to discriminate and may differ according to associated variables such as the way of administration, the dose, the selection of a target among many possible/available. Provided the demonstration of a clinical activity consistent with the intended proposed use and the compliance to safety standards it may be proposed that such active substances, not fully complying with the present regulatory definitions concerning the mode of action, may follow either the MDMS or the medicinal product registration procedure rather than be confined to the fuzzy domain of food supplements which does not prevent their use but does not impose clinical demonstration of their efficacy and therefore, correctly, does not allow specific claims.
Figure 2. Mode of action of a substance and relationships with regulatory pathways. The figure shows that the advancements in the knowledge of cell signaling pathways and in bioinformatics and system pharmacology have led to the understanding of novel mechanisms underlying the activity of several substances. These mechanisms do not fit the usual definitions used to classify a substance as belonging to a medicinal product or a MDMS domain. A revision of the present criteria when evaluating a mode of action of a substance integrating these new aspects may be needed. See text for further comments.
The way of reasoning here used for citicoline as a case study may be applied also to a mix of substances or to complex substances of natural origin at the cost of exponentially increasing difficulties because of the need to: 1) identify all the potential targets for each single molecule in study and match them with critical pathogenic biological targets/pathways underlying the disease; 2) verify to what extent each target is critical in the development/control of the disease and their hierarchical organization; 3) verify to what extent the activation and/or inhibition of the biological target takes place at the doses/routes of administration used for the intended purposes. It has then to be decided whether these mechanisms involve specific interactions, at the molecular level, with the biological targets and whether their engagement orientates toward the classification as medicinal product or allows also the MDMS classification depending upon the use, the dose, the mode of administration. On the other hand these aspects dealing with system biology and network pharmacology are further blurring the boundaries between drugs and MDs underscoring the need to further develop the scientific debate.
All authors listed have made a substantial, direct, and intellectual contribution to the work and approved it for publication.
The review was written as part of the institutional working duties, the open access publication fees will be paid using the lab. and department funds ad hoc allocated.
The authors declare that the research was conducted in the absence of any commercial or financial relationships that could be construed as a potential conflict of interest.
All claims expressed in this article are solely those of the authors and do not necessarily represent those of their affiliated organizations, or those of the publisher, the editors and the reviewers. Any product that may be evaluated in this article, or claim that may be made by its manufacturer, is not guaranteed or endorsed by the publisher.
Abdel-Aziz, N., Moustafa, E. M., and Saada, H. N. (2021). The Impact of Citicoline on Brain Injury in Rats Subjected to Head Irradiation. Environ. Sci. Pollut. Res. 28 (8), 9742–9752. doi:10.1007/s11356-020-11101-7
Adibhatla, R. M., and Hatcher, J. F. (2002). Citicoline Mechanisms and Clinical Efficacy in Cerebral Ischemia. J. Neurosci. Res. 70, 133–139. doi:10.1002/JNR.10403
Agostoni, C., Berni Canani, R., Fairweather-Tait, S., Heinonen, M., Korhonen, H., La Vieille, S., et al. (2014). Scientific Opinion on the Substantiation of a Health Claim Related to Cytidine 5-diphosphocholine and Maintenance of Normal Vision Pursuant to Article 13(5) of Regulation (EC) No 1924/2006. Efs2 12, 3575. doi:10.2903/J.EFSA.2014.3575
Alvarez-Sabín, J., Ortega, G., Jacas, C., Santamarina, E., Maisterra, O., and Ribo, M. (2013). Long-Term Treatment with Citicoline May Improve Poststroke Vascular Cognitive Impairment. Cerebrovasc. Dis. 35, 146–154. doi:10.1159/000346602
Bilia, A. R., Corazziari, E. S., Govoni, S., Mugelli, A., and Racchi, M. (2021). Special Issue Arnold Vlietinck II: Medical Devices Made of Substances: Possible Innovation and Opportunities for Complex Natural Products. Planta Medica 87, 1110. doi:10.1055/A-1511-8558
Carnevale, C., Manni, G., Roberti, G., Micera, A., Bruno, L., Cacciamani, A., et al. (2019). Human Vitreous Concentrations of Citicoline Following Topical Application of Citicoline 2% Ophthalmic Solution. PLoS One 14, e0224982. doi:10.1371/journal.pone.0224982
Davis, B. M., Crawley, L., Pahlitzsch, M., Javaid, F., and Cordeiro, M. F. (20162016). Glaucoma: the Retina and beyond. Acta Neuropathol. 132 (6 132), 807–826. doi:10.1007/S00401-016-1609-2
EUR-Lex - 02017R0745-20200424 - EN - EUR-Lex (2022). EUR-lex - 02017R0745-20200424 - EN - EUR-Lex. Available at: https://eur-lex.europa.eu/legal-content/EN/TXT/?uri=CELEX:02017R0745-20200424 (Accessed April 1, 2022).
Fahmideh, F., Marchesi, N., Barbieri, A., Govoni, S., and Pascale, A. (2021). Non-drug Interventions in Glaucoma: Putative Roles for Lifestyle, Diet and Nutritional Supplements. Surv. Ophthalmol. 67 (3), 675–696. doi:10.1016/J.SURVOPHTHAL.2021.09.002
Faiq, M. A., Wollstein, G., Schuman, J. S., and Chan, K. C. (2019). Cholinergic Nervous System and Glaucoma: From Basic Science to Clinical Applications. Prog. Retin Eye Res. 72, 100767. doi:10.1016/J.PRETEYERES.2019.06.003
Gandolfi, S., Marchini, G., Caporossi, A., Scuderi, G., Tomasso, L., and Brunoro, A. (20202020). Cytidine 5′-Diphosphocholine (Citicoline): Evidence for a Neuroprotective Role in Glaucoma. Nutrients 12, 793. Page 793 12. doi:10.3390/NU12030793
Gareri, P., Castagna, A., Cotroneo, A. M., Putignano, D., Conforti, R., Santamaria, F., et al. (2017). The Citicholinage Study: Citicoline Plus Cholinesterase Inhibitors in Aged Patients Affected with Alzheimer’s Disease Study. J. Alzheimer’s Dis. 56, 557–565. doi:10.3233/JAD-160808
Gfeller, D., Grosdidier, A., Wirth, M., Daina, A., Michielin, O., and Zoete, V. (2014). SwissTargetPrediction: a Web Server for Target Prediction of Bioactive Small Molecules. Nucleic Acids Res. 42, W32. doi:10.1093/NAR/GKU293
Giraldi, J. P., Virno, M., Covelli, G., Grechi, G., and de Gregorio, F. (19891989). Therapeutic Value of Citicoline in the Treatment of Glaucoma (Computerized and Automated Perimetric Investigation). Int. Ophthalmol. 13 (1 13), 109–112. doi:10.1007/BF02028649
Grieb, P. (2015). Citicoline: A Food that May Improve Memory. Clin. Pract. Rev. Meta-Analysis 2, 67–72. doi:10.12659/MSREV.894711
He, S., Stankowska, D. L., Ellis, D. Z., Krishnamoorthy, R. R., and Yorio, T. (2018). Targets of Neuroprotection in Glaucoma. J. ocular Pharmacol. Ther. official J. Assoc. Ocular Pharmacol. Ther. 34 (1-2), 85–106. doi:10.1089/jop.2017.0041
John Hetherington, J. (2013). Alternative Therapies for Glaucoma | Glaucoma Research Foundation. Available at: https://www.glaucoma.org/treatment/alternative-therapies-for-glaucoma.php (Accessed April 1, 2022).
Jünemann, A. G. M., Grieb, P., and Rejdak, R. (2021). Bedeutung von Citicolin bei der Glaukomerkrankung. Der Ophthalmol. 118, 439. doi:10.1007/S00347-021-01362-Z
Kitamura, Y., Bikbova, G., Baba, T., Yamamoto, S., and Oshitari, T. (20192019). In Vivo effects of Single or Combined Topical Neuroprotective and Regenerative Agents on Degeneration of Retinal Ganglion Cells in Rat Optic Nerve Crush Model. Sci. Rep. 9 (1 9), 1–8. doi:10.1038/s41598-018-36473-2
Laksmita, Y. A., Sidik, M., Siregar, N. C., and Nusanti, S. (2021). Neuroprotective Effects of Citicoline on Methanol-Intoxicated Retina Model in Rats. J. Ocul. Pharmacol. Ther. 37, 534–541. doi:10.1089/JOP.2021.0018
Lanza, M., Carnevale, U. A. G., Mele, L., Sconocchia, M. B., Bartollino, S., and Costagliola, C. (2019). Morphological and Functional Evaluation of Oral Citicoline Therapy in Chronic Open-Angle Glaucoma Patients: A Pilot Study with a 2-Year Follow-Up. Front. Pharmacol. 10, 1117. doi:10.3389/FPHAR.2019.01117
Lavik, E., Kuehn, M. H., and Kwon, Y. H. (20112011). Novel Drug Delivery Systems for Glaucoma. Eye 25 (5 25), 578–586. doi:10.1038/eye.2011.82
Liang, B., Li, C., and Zhao, J. (2016). Identification of Key Pathways and Genes in Colorectal Cancer Using Bioinformatics Analysis. Med. Oncol. 33, 1–8. doi:10.1007/S12032-016-0829-6/FIGURES/2
Marino, P. F., Rossi, G. C. M., Campagna, G., Capobianco, D., and Costagliola, C. (2020). Effects of Citicoline, Homotaurine, and Vitamin E on Contrast Sensitivity and Visual-Related Quality of Life in Patients with Primary Open-Angle Glaucoma: A Preliminary Study. Molecules 25, 5614. doi:10.3390/MOLECULES25235614
Matteucci, A., Varano, M., Gaddini, L., Mallozzi, C., Villa, M., Pricci, F., et al. (2014). Neuroprotective Effects of Citicoline in In Vitro Models of Retinal Neurodegeneration. Int. J. Mol. Sci. 15, 6286. doi:10.3390/IJMS15046286
MDCG 2022-5 (2022). . https://ec.europa.eu/health/latest-updates/mdcg-2022-5-guidance-borderline-between-medical-devices-and-medicinal-products-under-regulation-eu-2022-04-26_en (Accessed April 25, 2022).Guidance on Borderline between Medical Devices and Medicinal Products under Regulation (EU) 2017/745 on Medical Devices
Mehta, A., Mahale, R., Buddaraju, K., Javali, M., Acharya, P., and Srinivasa, R. (2019). Efficacy of Neuroprotective Drugs in Acute Ischemic Stroke: Is it Helpful? J. Neurosci. Rural Pract. 10, 576–581. doi:10.1055/S-0039-1700790/ID/JR_27
Oddone, F., Rossetti, L., Parravano, M., Sbardella, D., Coletta, M., Ziccardi, L., et al. (2021). Citicoline in Ophthalmological Neurodegenerative Disease: A Comprehensive Review. Pharm. (Basel) 14, 281. doi:10.3390/ph14030281
Oshitari, T., Yoshida-Hata, N., and Yamamoto, S. (2010). Effect of Neurotrophic Factors on Neuronal Apoptosis and Neurite Regeneration in Cultured Rat Retinas Exposed to High Glucose. Brain Res. 1346, 43–51. doi:10.1016/J.BRAINRES.2010.05.073
Ottobelli, L., Manni, G. L., Centofanti, M., Iester, M., Allevena, F., and Rossetti, L. (2013). Citicoline Oral Solution in Glaucoma: Is There a Role in Slowing Disease Progression? Ophthalmologica 229, 219–226. doi:10.1159/000350496
Parisi, V., Centofanti, M., Ziccardi, L., Tanga, L., Michelessi, M., Roberti, G., et al. (2015). Treatment with Citicoline Eye Drops Enhances Retinal Function and Neural Conduction along the Visual Pathways in Open Angle Glaucoma. Graefe’s archive Clin. Exp. Ophthalmol. = Albrecht von Graefes Archiv fur klinische und Exp. Ophthalmol. 253, 1327–1340. doi:10.1007/S00417-015-3044-9
Parisi, V., Coppola, G., Centofanti, M., Oddone, F., Maria Angrisani, A., Ziccardi, L., et al. (2008). Evidence of the Neuroprotective Role of Citicoline in Glaucoma Patients. Prog. Brain Res. 173, 541–554. doi:10.1016/S0079-6123(08)01137-0
Parisi, V., Manni, G., Colacino, G., and Bucci, M. G. (1999). Cytidine-5′-diphosphocholine (Citicoline) Improves Retinal and Cortical Responses in Patients with Glaucoma1. Ophthalmology 106, 1126–1134. doi:10.1016/S0161-6420(99)90269-5
Parisi, V., Oddone, F., Roberti, G., Tanga, L., Carnevale, C., Ziccardi, L., et al. (2019). Enhancement of Retinal Function and of Neural Conduction along the Visual Pathway Induced by Treatment with Citicoline Eye Drops in Liposomal Formulation in Open Angle Glaucoma: A Pilot Electrofunctional Study. Adv. Ther. 36, 987–996. doi:10.1007/S12325-019-0897-Z/TABLES/2
Parisi, V., Oddone, F., Ziccardi, L., Roberti, G., Coppola, G., and Manni, G. (2018). Citicoline and Retinal Ganglion Cells: Effects on Morphology and Function. Curr. Neuropharmacol. 16, 919. doi:10.2174/1570159X15666170703111729
Pascale, A., Drago, F., and Govoni, S. (2012). Protecting the Retinal Neurons from Glaucoma: Lowering Ocular Pressure Is Not Enough. Pharmacol. Res. 66, 19–32. doi:10.1016/J.PHRS.2012.03.002
Racchi, M., Govoni, S., Lucchelli, A., Capone, L., and Giovagnoni, E. (2016). Insights into the Definition of Terms in European Medical Device Regulation. Expert Rev. Med. Devices 13, 907–917. doi:10.1080/17434440.2016.1224644
Rejdak, R., Toczołowski, J., Krukowski, J., Kamiński, M., Rejdak, K., Stelmasiak, Z., et al. (2003). Oral Citicoline Treatment Improves Visual Pathway Function in Glaucoma. Med. Sci. Monit. 9.
Roberti, G., Tanga, L., Parisi, V., Sampalmieri, M., Centofanti, M., and Manni, G. (2014). A Preliminary Study of the Neuroprotective Role of Citicoline Eye Drops in Glaucomatous Optic Neuropathy. Indian J. Ophthalmol. 62, 549. doi:10.4103/0301-4738.133484
Rossetti, L., Iester, M., Tranchina, L., Ottobelli, L., Coco, G., Calcatelli, E., et al. (2020a). Can Treatment with Citicoline Eyedrops Reduce Progression in Glaucoma? the Results of a Randomized Placebo-Controlled Clinical Trial. J. Glaucoma 29, 513. doi:10.1097/IJG.0000000000001565
Rossetti, L., Iester, M., Tranchina, L., Ottobelli, L., Coco, G., Calcatelli, E., et al. (2020b). Can Treatment with Citicoline Eyedrops Reduce Progression in Glaucoma? the Results of a Randomized Placebo-Controlled Clinical Trial. J. Glaucoma 29, 513. doi:10.1097/IJG.0000000000001565
Sahin, A. K., Kapti, H. B., and Uzun, A. (2022). Effect of Oral Citicoline Therapy on Retinal Nerve Fiber Layer and Ganglion Cell-Inner Plexiform Layer in Patients with Primary Open Angle Glaucoma. Int. J. Ophthalmol. 15, 483. doi:10.18240/IJO.2022.03.17
Tokuc, E. O., Yuksel, N., Rencber, S. F., Ozturk, A., Duruksu, G., Yazir, Y., et al. (2021). Protective Effects of Citicoline-Containing Eye Drops against UVB-Induced Corneal Oxidative Damage in a Rat Model. Exp. Eye Res. 208, 108612. doi:10.1016/J.EXER.2021.108612
Vale, S. (2008). Current Management of the Cognitive Dysfunction in Parkinson’s Disease: How Far Have We Come? 233(8):941–951. doi:10.3181/0707-MR-193
Virno, M., Pecori-Giraldi, J., Liguori, A., and de Gregorio, F. (2000). The Protective Effect of Citicoline on the Progression of the Perimetric Defects in Glaucomatous Patients (Perimetric Study with a 10-year Follow-Up). Acta Ophthalmol. Scand. 78, 56–57. doi:10.1111/J.1600-0420.2000.TB01107.X
Weinreb, R. N., Aung, T., and Medeiros, F. A. (2014). The Pathophysiology and Treatment of Glaucoma: A Review. JAMA 311, 1901. doi:10.1001/JAMA.2014.3192
Keywords: MDMS, glaucoma, mechanism of action (MOA), system pharmacology, eye drops, food supplements, medicinal product, citicoline
Citation: Marchesi N, Fahmideh F, Barbieri A, Racchi M, Pascale A and Govoni S (2022) Pharmacological Versus Non-Pharmacological and Ancillary Mechanisms in Eye Drops Used in the Treatment of Glaucoma. Front. Drug Saf. Regul. 2:933471. doi: 10.3389/fdsfr.2022.933471
Received: 30 April 2022; Accepted: 08 June 2022;
Published: 27 June 2022.
Edited by:
Juan L. Tamargo, Complutense University of Madrid, SpainReviewed by:
Elisabetta Bigagli, University of Florence, ItalyCopyright © 2022 Marchesi, Fahmideh, Barbieri, Racchi, Pascale and Govoni. This is an open-access article distributed under the terms of the Creative Commons Attribution License (CC BY). The use, distribution or reproduction in other forums is permitted, provided the original author(s) and the copyright owner(s) are credited and that the original publication in this journal is cited, in accordance with accepted academic practice. No use, distribution or reproduction is permitted which does not comply with these terms.
*Correspondence: Annalisa Barbieri, YW5uYWxpc2EuYmFyYmllcmlAdW5pcHYuaXQ=
†These authors have contributed equally to this work
Disclaimer: All claims expressed in this article are solely those of the authors and do not necessarily represent those of their affiliated organizations, or those of the publisher, the editors and the reviewers. Any product that may be evaluated in this article or claim that may be made by its manufacturer is not guaranteed or endorsed by the publisher.
Research integrity at Frontiers
Learn more about the work of our research integrity team to safeguard the quality of each article we publish.