- 1Department of Restorative Dentistry, Faculty of Dentistry, Al Salam University, Tanta, Egypt
- 2Conservative Dentistry Department, Faculty of Dentistry, Tanta University, Tanta, Egypt
- 3Department of Pharmaceutical Microbiology, Faculty of Pharmacy, Tanta University, Tanta, Egypt
Aim: The aim to this study is to evaluate the biocompatibility and antibiofilm actions of two nano-hydroxy apatite (NHA).
Methodology: Nano-hydroxy apatites are biomaterials use in direct contact with living tissues. Therefore, they should be tested for their safety beside assessment of their minimum inhibitory (MIC) and minimum bactericidal concentration (MBC) using broth microdilution method. One hundred and twenty extracted bovine incisors were collected and cleaned to ensure the absence of any defects. Enamel blocks with different size (2 × 2 × 3 mm) and (5 × 5 × 2 mm) were prepared from their labial surfaces using an isomet saw. Enamel blocks are used for detecting the suitable concentration will be used in the following experiments using energy dispersive X-ray analysis (EDX). The remaining enamel blocks divided into 5 equal groups to detect inhibitory effect against bacterial adhesion to the initial enamel caries like lesions using viable count technique beside the antibiofilm activity against mature biofilm of Streptococcus mutans (S. mutans) using confocal laser microscopy. The remaining enamel blocks were used as a representing data for detecting surface topography for each group by using the scanning electron microscopy (SEM).
Result: The data showed safety of NHA suspensions. Additionally, only NHA suspension of large nanoparticle size (NHA-LPS) had MIC of 1.25 mg/ml against S. mutans. Also, have the higher percentages of Ca and P in the enamel blocks. Furthermore, the lowest level of bacterial adhesion was recorded in (group III) treated by NHA-LPS which was non-significantly different with the positive control group V. Biofilm thickness in group IV treated with NHA-small particle size (SPS) recorded high biofilm thickness followed by group III. Interestingly, group III showed greater killing effect against mature biofilm which is slightly higher than the positive control group V. In group III, surface topography revealed very smooth enamel surface with closed pores. Accordingly, NHA-LPS suspension had antiadhesive, antibacterial, and antibiofilm effect against cariogenic S. mutans representing a promising possibility to be recommended for safe effective remineralization.
Introduction
Dental caries is the most widespread disease that affects all ages (1). It is defined as dynamic, complex, multifactorial (2) biofilm-related oral disease (3) that starts with microbial shifts within the complex biofilm and is affected by salivary flow and composition, increased rate of dietary sugar, exposure to fluoride (F) and preventive behavior (4).
Bacteria play an important role in dental caries; they are organized into a yellowish film known as dental plaque on teeth surfaces. Most caries active bacteria appear to be Streptococcus mutans (S. mutans), Lactobacillus, Veillonella and Actinomyces species (5). Research on the bacteriology of dental caries has focused on the S. mutans and its ability to ferment sucrose to produce significant amounts of acid and extracellular polysaccharides (plaque) (5).
The adhesion of bacteria may be affected by a variety of microbiological, physical, chemical, and material-related parameters (6–9). The adhering parameters can be significantly influenced by the topography of substrate surface (10) where different studies (11–13) showed that the roughened enamel surface could significantly increase susceptibility to bacterial adhesion (14).
Continuous balanced demineralization and remineralization are natural dynamic processes in enamel. If the balance is interrupted and demineralization process dominates (15), it may eventually lead to increase in the porosity, surface roughness (15), and development of carious lesions (15). Thus, it is a major advancement to treat early caries lesions by non-invasive methods that depend on remineralization (16), utilizing a lot of products used as remineralizing agent such as chewing gums, toothpastes, mouth rinses, fissure sealant, and remineralizing pastes (17).
Over the last few decades, fluoride varnish is known to enhance a remineralization as a non-invasive treatment (18). However, reservations have been expressed about total intake of fluoride and fluorosis (17). Recently, alternative remineralizing materials have been suggested such as casein phosphopeptide-amorphous calcium phosphate (CCP-ACP), nano-hydroxyapatite (NHA); both of them are reported to have anticariogenic effects (19, 20), in addition to tri-calcium phosphate, ACP Technology, and Xylitol spray (21).
Later, nanotechnology was used in dentistry for developing preventive therapeutic agents and bioactive dental restorative materials (1). NHA are biomaterials manufactured for the purpose of medical use in direct contact with living tissues. Therefore, they should be tested for their safety and usefulness prior to their applications. Both of phosphate (PO4) and calcium (Ca) crystals are found in NHAs similar to those present in enamel, dentin, and cementum so the later were recommended as remineralizing agents because of being an important source for Ca and PO4 ions (22). Therefore, it was interesting to test the potential of two different NHA products in reducing the bacterial adhesion to the initial carious lesions on the enamel surface and their effect against the mature biofilm of S. mutans.
Materials and Methods
Material used in this study is NHA suspension of large nanoparticle size (NHA-LPS) (length: 86.64 nm, width: 17.43 nm) which is prepared at the Institute of Nano-science and Nanotechnology, Kafrelsheikh University, Kafrelsheikh, Egypt (23). NHA-SPS is a ready-made powder (length 30–40 nm, width: 8–12) from Nano-stream, Egypt (NHA paste Desensibilize Nano P, FGMD dental Product, Brazil). Sodium Fluoride (NaF) Varnish 5% was obtained from President Dental, Germany. Demineralizing solution (2.2 mMol calcium chloride [CaCl2], 2.2 mMol sodium phosphate, 0.05 M acetic acid, 1 M potassium hydroxide [pH 4.2]) (24) and artificial saliva (0.0856% sodium chloride, 0.1200% potassium chloride, 0.0052 magnisum chloride × 6 H2O, 0.0148 CaCl2 × 2 H2O, 0.2000 Mannitol, 0.0456 KH2PO4, 0.1000 Carbomer 974P, 0.4000 NaOH 10%, and Purified water) (25) both are made in faculty of pharmacy Tanta University. Dexorotatory 1,4-Dithiothreitol (DTT), brain heart infusion broth (BHI), Mitis salivarius agar, Resazurin blue stain, acridine orange fluoresces green (AO), and propidium iodide fluoresces red (PI) were obtained from SIGMA-ALDRICH, Germany.
Test microorganism, the test bacterial strain (gram +ve S. mutans ATCC® 25175™) was obtained from Microbiological Resources Centre (MIRCEN), Cairo, Egypt.
Cell line WI-38 (ATCC® CCL-75™) VACSERA which is normal fibroblast derived from lungs was obtained from Alexandria Medical Research Institute.
This research was designed as a controlled in vitro study. All the study steps were performed in the laboratories of the Faculties of Dentistry and Pharmacy, Tanta University.
Approval of this research was obtained from Research Ethics Committee, Faculty of Dentistry, Tanta University. The design and procedures of the present study were accomplished according to the research guidelines adopted by research Ethics Committee, Faculty of Dentistry, Tanta University.
Preparation of the Powder Aqueous Suspension
Nano-Hydroxyapatite Synthesis and Characterization
(a) NHA-LPS (length: 86.64 nm, width: 17.43 nm) was prepared at the Institute of Nano-science and Nanotechnology, Kafrelsheikh University, Kafrelsheikh, Egypt (23).
(b) NHA-SPS is a ready-made powder (length 30–40 nm, width: 8–12) from Nano-stream, Egypt.
For making suspension ready for application, the two NHA powders were dissolved in distilled water according to required concentrations.
Cytotoxicity Test
Fibroblast cells were cultured in a standard condition (5% CO2, 37 ± 1°C) in a Steri-cycleTM 381. Cells were removed from culture asks with 0.25% trypsin-ethylene di amine tetra acetic acid solution (Lonza, USA) and seeded in 96-well at-bottomed plates (Nunc, Nunclon™ Surface Roskilde, Denmark) in a concentration of 1 × 104 cells per well in 100 μl Dulbecco's Modified Eagle Medium (Lonza, USA). Approximately 200 mg/ml of tested powder was mixed properly in the first well by suction and pipetting 5–8 times. Approximately 100 μl was withdrawn from the first well and transferred to the second, mixed as previously done then transferred to third and so on till the concentration of the tested powder reach to 0.39 mg/ml with leaving last raw as a negative control (without adding any powder). Each tested powder was incubated in standard conditions for 5 min and 1 h, then the medium with the tested powders was discarded and the wells were washed with phosphate-buffered saline (pH 7). The cell viability was determined using 3-(4, 5-Dimethylthiazol-2-yl)-2, 5-diphenyltetrazolium bromide (MTT) dye (SIGMA-ALDRICH, Germany). Approximately 100 μl MTT was added to each well and plates were incubated for 3 h at 37 ± 1°C in 5% CO2. The MTT solution was then discarded and 100 μl of dimethyl sulfoxide analytical grade (Stanlab®Lublin, Poland) was added in each well. After shaking for 10 min, the absorbance values were recorded at 490 nm using an Epoch® microplate spectrophotometer (BIORAD, Japan). The changes in cell morphology were evaluated using the inverted phase contrast microscope CK × 41 (Olympus, Tokyo, Japan). Cell viability was calculated according to the formula: V = (Ab:As) 100%, where V is cell viability percentage, Ab is the mean absorbance of the test enamel block, and As is mean Absorbance of the blank (26).
Determination of Minimum Inhibitory and Minimum Bactericidal Concentration Test
The microtiter broth dilution method is used for detecting MIC and MBC of both powders in which the chosen strain was grown in BHI broth and the bacterial growth was adjusted to 0.5 McFarland standard. The NHA powder (200 mg/ml) solution under investigation was prepared and diluted in BHI broth by dispensing 100 μl of the broth into all wells of the microtiter plate. In the first well, 200 mg was added and properly mixed by suction and pipetting 5–8 times. About 200 μl was serially diluted. A total of 100 μl was withdrawn from the first well and transferred to the second, mixed as previously done then transferred to third and so on till the concentration of the tested powder reach to 0.39 mg/ml. Then 100 μl was discarded from the last well. Approximately 10 μl from Resazurin blue stain (0.001% [w/v]) was used as a color indicator (27). Also, 10 μl from the diluted bacteria (0.5 McFarland) was added by micropipette into all wells except final row as a negative control. Then the plates were incubated in 37°C for 12–18 h. Approximately 10 μl of the wells content was directly plated on the mitis salivarius agar. Wherever the least concentration results in no colony growth was considered as MBC. On the other hand, the least concentration that the bacterial growth was considered as MIC (28).
Teeth Selection
One hundred and twenty freshly extracted bovine incisors in death were collected, cleaned, polished, and carefully examined using a magnifying glass lens to ensure the absence of any defects (29). The selected teeth were kept in 0.1% thymol solution (SIGMA-ALDRICH, Germany) at 4°C to be used within 1 month (30).
Enamel Blocks Preparation
Fifty enamel blocks (2 × 2 × 3 mm) were randomly selected from the extracted bovine teeth and prepared from the labial surfaces of the teeth using an isomet saw (5000 Linear Precision Sawbuehler® LTD, USA) for the detection of S. mutans adhesion to enamel surfaces (31). Another 20 enamel blocks (5 × 5 × 2 mm) are used for Energy Dispersive X-ray analysis (EDX) (JEOL JSM-6510LV, Japan) to detect which concentration will be used (30 and 100 mg/ml) for both powders. Also, another 10 enamel blocks (5 × 5 × 2 mm) representing the different groups were used to detect the surface topography by the scanning electron microscopy (SEM) (FESEM, JEOL JSM-6510LV, Japan), while the other 40 enamel blocks (5 × 5 × 2 mm) were used to detect the thickness of mature biofilm and live/dead bacteria by the confocal laser microscopy. All enamel blocks were covered by 2 layers of nail polish except top of the enamel blocks (32). All were kept in a daily fresh mix of artificial saliva in an incubator (Binder CB 150 CO2 Incubator, China) at 37°C until used for a maximum of 21 days (33). The enamel blocks were randomly divided into 5 equal groups according to the material used for treatment as presented in the experimental design of the study (Table 1).
Lesion Creation
The enamel blocks were removed from the artificial saliva and immersed individually in 10 ml of demineralizing solution in test tubes which composed of (2.2 mMol calcium chloride [CaCl2], 2.2 mMol sodium phosphate, 0.05 M acetic acid, 1 M potassium hydroxide [pH 4.2]) (24) at 37°C for 96 h to create initial enamel caries like lesions. The pH of the solution was checked daily using digital pH meter and adjusted to 4.2 to avoid super saturation (34).
The tested materials were applied to groups II–V according to manufacturer instructions as follows (Table 2).
Elemental analysis for 20 enamel blocks with initial caries was performed by EDX to measure Ca and P ions levels to detect which concentration will be used in the study. Blocks were treated by 30 and 100 mg/ml of NHA-LPS suspension or NHA-SPS suspensions (n = 5).
Detection of Antiadhesive Effect of NHA Preparations Against S. mutans Adhesion to Enamel Surfaces
All the enamel blocks were autoclaved at 115.5°C for 40 min to ensure complete sterilization then washed with distilled water (35). Sterile swabs of bacterial suspension equivalent to 0.5 McFarland of standard bacteria S. mutans were spread on the mitis salivarius agar which is a selective media for the tested organism (36). The desired enamel blocks were then placed on the agar surface and incubated in CO2 incubator for 48 h at 37°C. The enamel blocks were taken from the medium and washed with 15 cc normal sterile saline solution and placed in 1 cc sterile saline solution (37) and for 5 min in the vortex with 2,800 rpm, to remove the bacteria from the surface of enamel blocks and to spread it in normal saline (37). Serial dilutions of 1:10, 1:100, and 1:1,000 of the above suspension was prepared in normal sterile saline (38) and 0.1 cc of each dilution was spread on the surface of mitis salivarius agar (39). Incubation was performed in 37°C for 18–24 h, then the grown colonies were counted and the results were reported in terms of colonies forming unit (CFU)/ml (37).
Evaluation of the Antibacterial Effect of the Tested Materials on Mature Biofilm
All enamel blocks were autoclaved at 115.5°C for 40 min for sterilization (35), washed with distilled water, and allowed to dry in their corresponding test tubes. BHI broth was used as a nutrient for the culture of S. mutans (40). All the enamel blocks were incubated for 14 days at 37°C in an anaerobic condition for making mature biofilm. The medium was changed daily to renew media and provide new nutrition to allow bacterial growth and biofilm maturation (40). The biofilm formed on the surface was used in the subsequent experiments. A randomly selected 8 enamel blocks were used to represent each group. The corresponding materials were applied to the mature biofilm as previously mentioned.
Preparation of Sterile Saliva
Undiluted sterile saliva was prepared according to De Jong and Van der Hoeven (41) with some modifications (41). Approximately 25 ml of stimulated whole saliva (SWS) was collected every day from a single volunteer to minimize salivary composition variations between individuals. The volunteer was asked to chew on sugar-free gum to stimulate the saliva production. The collection was done using ice-chilled test tubes. The aggregation of protein in the SWS enamel blocks was minimized by adding DTT to a concentration of 2.5 mM. Upon addition of DTT, the saliva was stirred slowly for 10 min before it was centrifuged at 864 × g for 30 min. The supernatant obtained was then filter-sterilized through a disposable 0.2 μm low protein-binding filter (Supor® EKV Membrane Filters) into sterile test tubes. The sterile SWS was then stored at −20°C in ice. Prior to use, it was thawed and centrifuged once again to remove any precipitate (42).
Staining of Biofilm for Examination by Confocal Microscope
After applying materials on mature biofilms, all biofilms on enamel blocks were stained with 1 ml of 0.01% AO in a dark environment for 30 min and rinsed with distilled water to remove excess dye then stained again by PI in a dark environment for 30 min and rinsed with distilled water then allowed to dry (40).
Laser and Stain Specification
Confocal illumination was performed using argon laser which was adjusted to 525 nm laser for AO and to 460 nm emission for PI. The fluorescence from the stained cell was viewed under confocal scanning laser microscope (LSM 710, Carl Zeiss, Jena, Germany). PI dye has the ability to enter only through the membranes of dead cells and binds with bacterial RNA and DNA or one with reversibly damage emitting red fluorescence. While AO stains nucleic acids of the live cells and exhibits green fluorescence after the staining procedure (43). For the AO staining, the excitation and emission wavelengths for RNA were 460 and 650 nm, respectively, and for PI, the excitation and emission wavelengths were 525 and 595 nm, respectively (44). After the staining procedure, the corresponding enamel blocks were immediately subjected to confocal laser scanning microscope. Available Lens: 10×, 20×, 40× oil & 63× oil. Confocal images were analyzed and quantitated (live/dead ratios) using Software version: ZEN imaging software (ZEN 2012 blue edition) and the image stacks were viewed with LSM browser (45). The percentage of death of biofilm cells determined by LIVE/DEAD technique was obtained as follows:
Percentage of death biofilm cells = biofilm volume of red fluorescence/total biofilm volume of green and red fluorescence × 100% (46).
Scanning Electron Microscopy Analysis to Detect Surface Topography
Ten enamel blocks, one represented each group were gold coated with Sputter Coating Evaporator (SPI Module—Sputter Carbon/Gold Coater, Japan) and examined under SEM (FESEM, JEOL JSM-6510LV, Japan) magnifications (×5,000) at 20 KV. The most representative images of enamel surface topography were captured.
Statistical Analysis
The data related to the effect of different materials on bacterial colonies and thickness of mature biofilm were collected, tabulated, and statistically analyzed. Numerical variables were expressed by descriptive statistics as mean, standard deviations, and range. Regression analyses were performed to analyze the relation between the viability and concentration. One-way ANOVA and Tukey's test were used to compare different groups and different concentrations. The p-value <0.05 was considered significant difference & p-value <0.001 was considered highly significant difference. Statistical analyses were performed using Statistical Package for Social Sciences (SPSS version 25). The null hypothesis is that all the materials under investigation will exhibit the same effect.
Results
Cytotoxicity Test
Cytotoxicity test of different concentrations of NHA powders on the viability of normal fibroblasts following incubation for 5 min or 1 h. There is no recorded cytotoxicity for both powders when tested at 10 mg/ml with no significant difference (p > 0.05) in regard to incubation time as shown in Figures 1, 2. Increasing the concentration of the test NHA to 30 mg/ml for 5 min resulted in a slight but non-significant reduction in the cellular viability (97 and 87%) for NHA-LPS and NHA-SPS suspensions, respectively. Furthermore, extending the incubation time to 1 h revealed also slight but non-significant (p > 0.05) decrease in the viability of fibroblasts as shown in Figure 3, indicating safety of both powders at the test concentrations (10 and 30 mg/ml) and incubation period, as revealed by ANOVA test which recorded non-significant difference (p > 0.05). Moreover, a significant concentration-dependent decrease in the viability was also observed for both powders tested above 30 mg/ml recording an IC50 at concentrations ranged between 80 and 90 mg/ml.
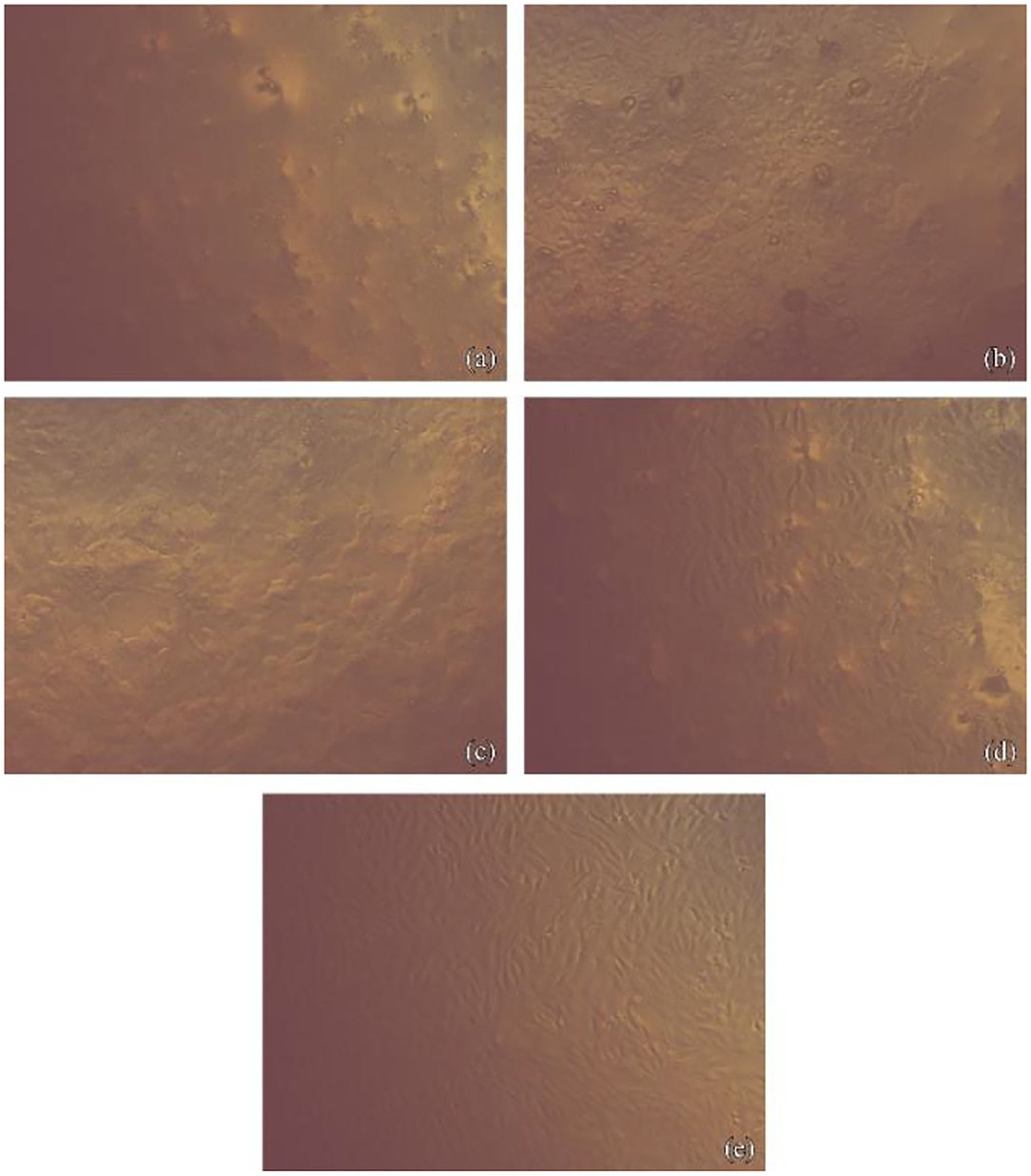
Figure 1. Fibroblasts cells imaged with phase-contrast microscope showing their morphology after contact with NHA-SPS suspension. (a) For 1 h at concentration 10 gm/ml, Images show lowest cell density with loss of normal spindle morphology. About 50% of cells became rounded, no granules in the cytoplasm. Cell lysis and empty spaces show between cells. (b) For 1 h at concentration 2.5 gm/ml, images show little spindle cells shrunk, separating from the substrate and visible intraplasmic granules. (c) For 5 min at concentration 10 gm/ml, images show lowest cell density with the loss of normal spindle morphology. About 50% of cells became rounded, no granules in the cytoplasm. Cell lysis and empty spaces show between cells. (d) For 5 min at concentration 2.5 gm/ml, images show little spindle cells shrunk, separating from the substrate, and visible intraplasmic granules. (e) Normal fibroblast cells as a control.
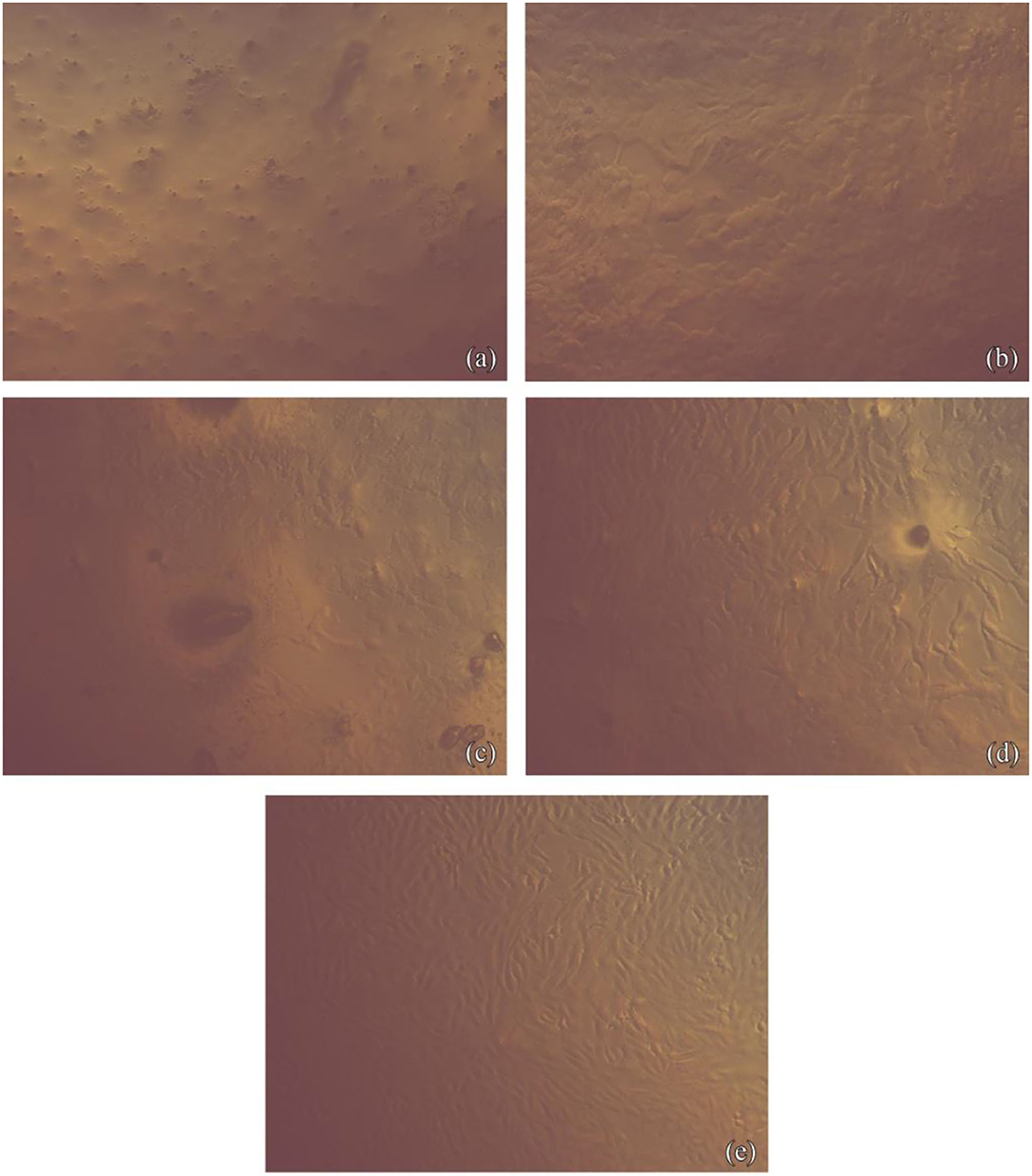
Figure 2. Fibroblasts cells imaged with phase-contrast microscope showing their morphology after contact with NHA-LPS suspension. (a) For 1 h at concentration 10 gm/ml, images show lowest cell density with the loss of normal spindle morphology. About 50% of cells became rounded, no granules in the cytoplasm. Cell lysis and empty spaces show between cells. (b) For 1 h at concentration 2.5 gm/ml, images show little spindle cells shrunk, separating from the substrate and visible intraplasmic granules. (c) For 5 min at concentration 10 gm/ml, images show lowest cell density with the loss of normal spindle morphology. Approximately 50% of cells became rounded, no granules in the cytoplasm. Cell lysis and empty spaces show between cells. (d) For 5 min at concentration 2.5 gm/ml, images show little spindle cells shrunk, separating from the substrate, and visible intraplasmic granules. (e) Normal fibroblast cells as a control.
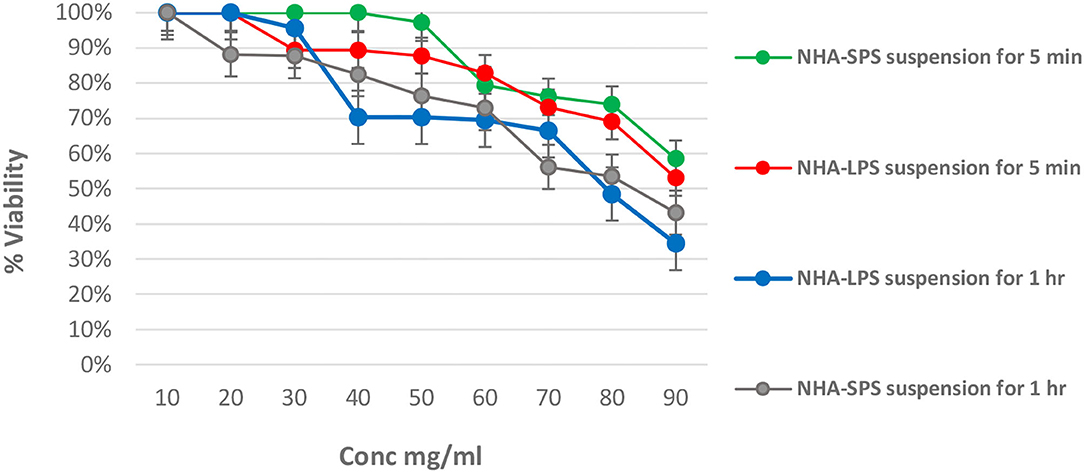
Figure 3. Effect of test NHA suspensions on the viability of normal fibroblasts at different time intervals. (A) For NHA-SPS suspension for 5 min. (B) NHA-LPS suspension for 5 min. (C) NHA-LPS suspension for 1 h. (D) NHA-SPS suspension for 1 h.
Minimum Inhibitory Concentration and Minimum Bactericidal Concentration
Broth microdilution assay revealed that only NHA-LPS suspension had MIC (1.25 mg/ml) and MBC (2.5 mg/ml) against S. mutans as presented in Table 3. On the other hand, NHA-SPS suspension did not inhibit the growth of the tested organism below 10 mg/ml suggesting that it may have high inhibitory concentrations and also indicating the potency of NHA-LPS suspension.
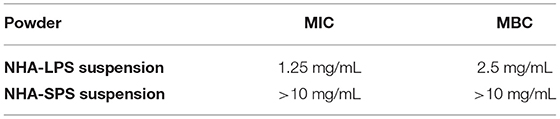
Table 3. Minimum inhibitory concentration (MIC) and minimum bactericidal concentration (MBC) of test NHA suspensions.
Elemental Analysis of Ca and P
Table 4 and Figure 4 presented the elemental analysis of Ca and P percentages (W%) detected by EDX in the initial caries like lesions induced in the enamel blocks and treated by both NHA suspensions, at 30 and 100 mg/ml. The results showed no significant differences for the percentages of Ca and P in the treated enamel blocks between the two test concentrations of both suspensions as recorded in Table 3. ANOVA test recorded the p-values > 0.05 except for p percentages in the NHA-SPS suspension where these was a significant difference between the two concentration (p-value < 0.05). Overall, the percentages of Ca and P were higher in enamel blocks treated with NHA-LPS aqueous suspension.

Table 4. Mean values (wt. %) of Ca and P elements in the initial caries like lesions induced in the enamel blocks and treated by NHA suspensions for 10 days, as well as the statistical analysis recorded by ANOVA test.
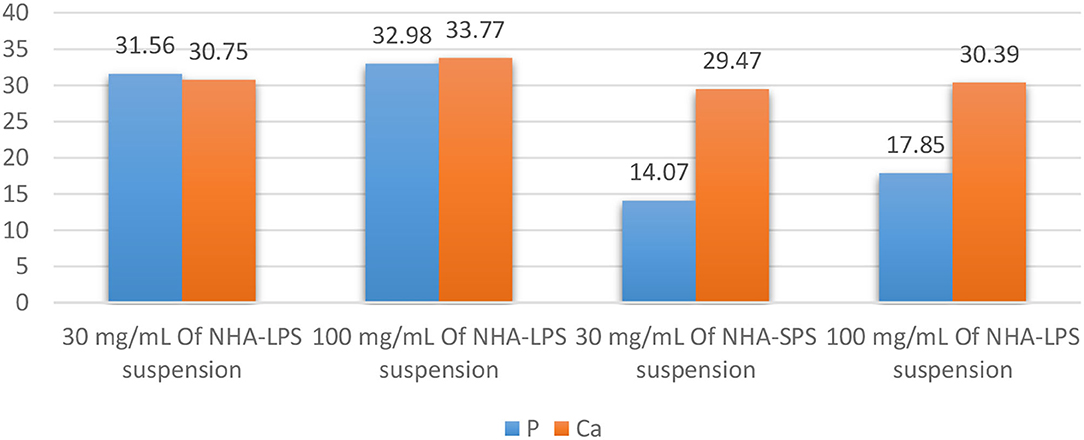
Figure 4. Bar chart showing the mean (P) and (Ca) values (wt. %) throughout the steps of treating sample by 3 and 10% of 1st and 2nd aqueous suspension.
Bacterial Adhesion Test
Regarding bacterial adhesion test (Figure 5), the mean values (CFU/ml) in all groups are presented in Table 5 and Figure 6. The highest level of bacterial adhesion was found on the untreated initial enamel caries like lesions in group I (4.65). However, nearly comparable values were found when the initial enamel caries like lesions was treated with Desensibilize Nano p paste in the group II (positive control 1) and NHA-SPS suspension in group IV and recording values of (3.33 and 3.69) for both groups, respectively. On the other hand, a markedly lower value (0.69) was found in group III when the initial enamel caries like lesions was treated by NHA-LPS suspension. Additionally, no bacterial adhesion was found in the group V (positive control 2) where the initial enamel caries like lesions was treated by NaF varnish. ANOVA test revealed a highly significant difference between the 5 tested groups (p = 0.000). Thus, pairwise Tukey's test was performed to compare between each two groups. It revealed highly statistically significant values of bacterial adhesion in group III and group V vs. all other tested groups. While groups I, II, and IV recorded no significant difference compared to each other.
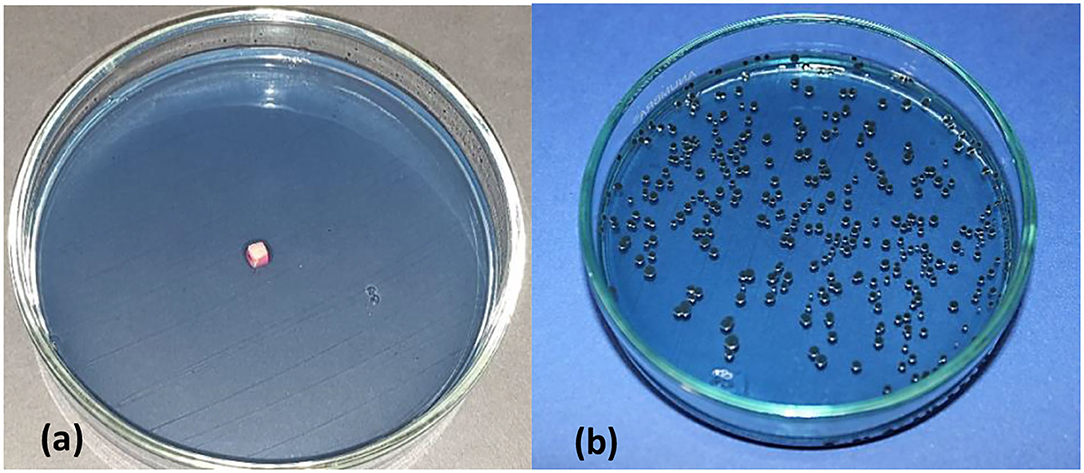
Figure 5. Mitis salivarius containing petridish, showing treated enamel block sample covered with a thin layer of S. mutans bacterial suspension (a), viable count of S. mutans following vortexing of enamel block, serial dilution and incubation for 18–24 h (b).
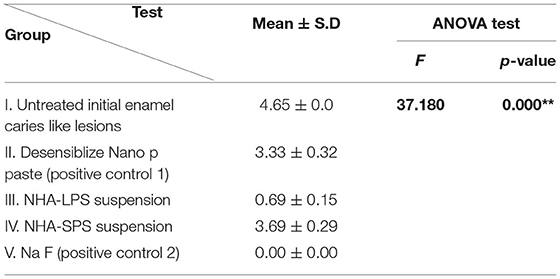
Table 5. Mean values of the logarithmic number of bacterial colonies (log10 CFU/ml), representing bacteria adhered to the enamel surface in all test groups and statistical analysis.
Biofilm Thickness
Regarding the effect of test materials on biofilm thickness, the mean values (μm) were presented in Table 6, and Figures 7, 8. Groups could be arranged in descending order as group IV treated with NHA-SPS suspension (334.80), group III treated with NHA-LPS suspension (309.20), group I untreated initial enamel caries like lesions (230.90), group II treated by Desensibilize Nano p paste (183.70), and the lowest biofilm thickness was found in group V treated by NaF varnish (121.20). The ANOVA test revealed a statistically significant difference between the five tested groups (p = 0.009). The pairwise Tukey's test was performed to compare each two groups. It revealed a statistically significant (p = 0.027) higher value of the biofilm thickness in group IV vs. group V (p = 0.007). While no significant difference was observed between the other tested groups (p > 0.05).
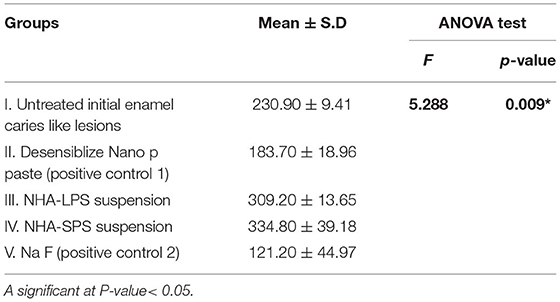
Table 6. Mean values of bacterial biofilm thickness (μm) in all tested groups and statistical analysis.
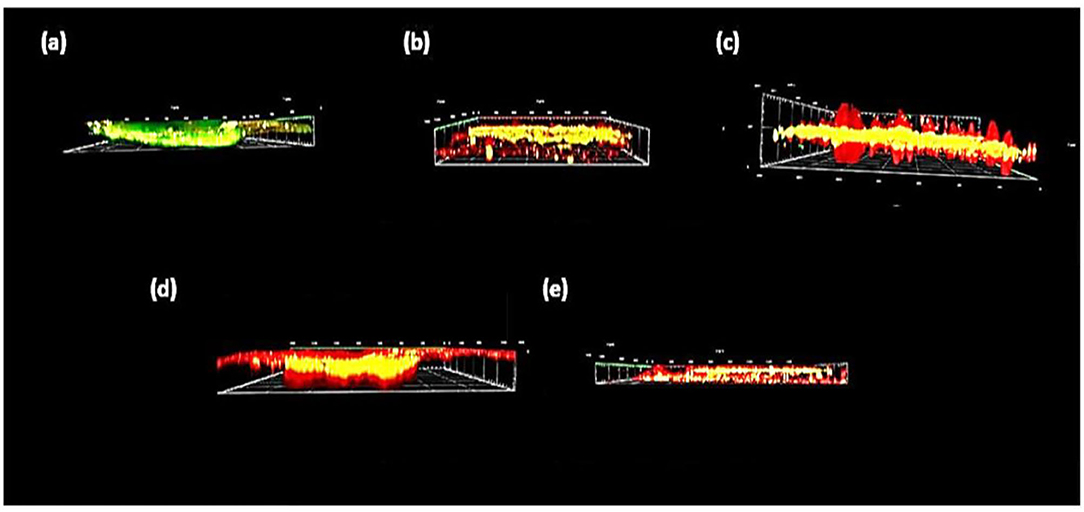
Figure 8. Side view of confocal scanning microscopic images of biofilm thickness obtained from virtual addition of multiple horizontally captured images at different depth levels. (a) Untreated enamel caries like lesions (group I), (b) desensibilize nano p (group II), (c) NHA-LPS suspension (group III), (d) NHA-SPS suspension (group IV), and (e) na F varnish (group V).
Antibiofilm Action
Concerning the antibiofilm action of test materials, this was demonstrated by statistical analysis of the average percentage of dead bacteria to the total bacterial count on the enamel surface of each group. As shown in Table 7 and Figure 9, group III recorded a very high percentage of dead bacteria (98.33%) which was slightly higher than the positive control group V (96.25%). However, the lowest percent of dead bacteria (17.94%) was recorded in group I. Representative confocal microscopic images (Figure 10) denoting the level of living to dead bacteria in all tested groups were presented, showing dead bacteria in red or yellow while live bacteria in green color. The pairwise Tukey's test compared between each two groups, it revealed a high statistically significant difference between group I vs. each of the other groups (p < 0.001) beside the presence of statistically significant difference recorded between the other groups.
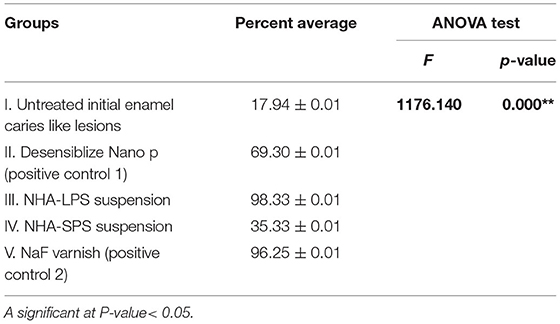
Table 7. Statistical analysis of the average percentage values of dead bacterial in all tested groups.
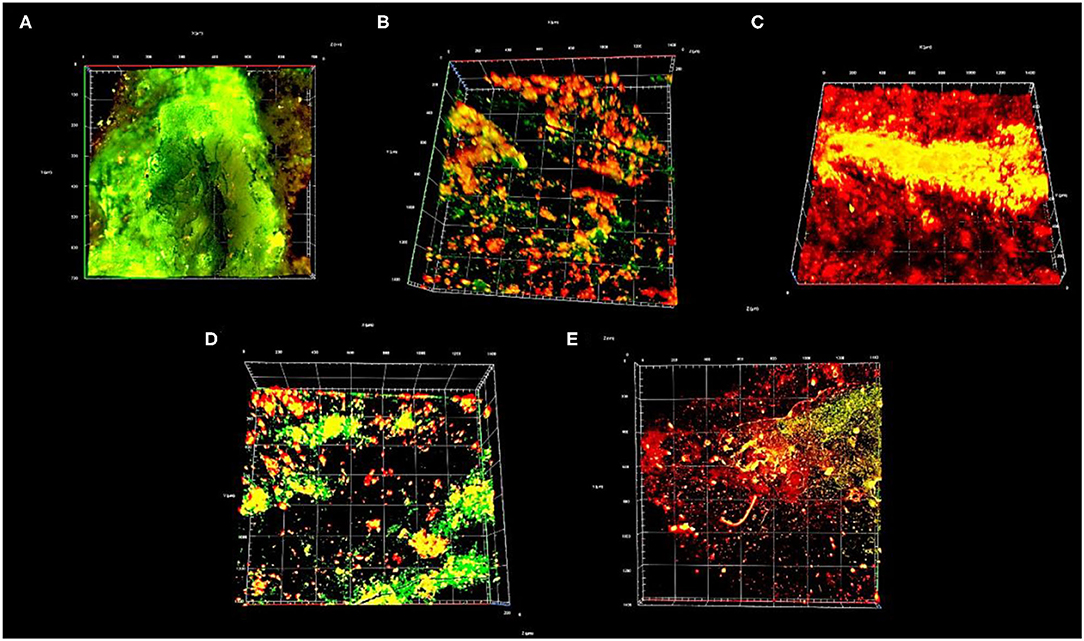
Figure 10. Top view 3D image of confocal scanning microscope showing live (green) and dead (red or yellow) bacteria. (A) Untreated enamel caries like lesions (group I), (B) Desensibilize nano p (group II), (C) NHA-LPS suspension (group III), (D) NHA-SPS suspension (group IV), and (E) Sodium Fluoride (NaF) varnish (group V).
Scanning Electron Microscopy Evaluation
Scanning electron microscopy evaluation of the surface topography was performed as representative samples for all the studied groups and presented in Figure 11. Surface topography of the initial enamel caries like lesions, after acid etching (group I), revealed a lot of pores due to partial decalcification and dissolution of the prisms or interprisms. Initial enamel caries like lesions treated with group II of Nano p paste (positive control of NHA) showed multiple remnants of coating NHA appeared on the surface resulting in roughness of enamel surface (Figure 11B). On the other hand, using NHA-LPS and NHA-SPS suspensions (group III and IV, respectively) resulted in remineralized lesions with filling the dark spaces but very smooth enamel surface was observed following treatment with NHA-LPS suspensions (group III) as shown in Figures 11C, D. However, group IV (the positive control; NaF varnish) showed that some of the pores were closed but still pores could be seen opened indicating weak remineralization effect (Figure 11E).
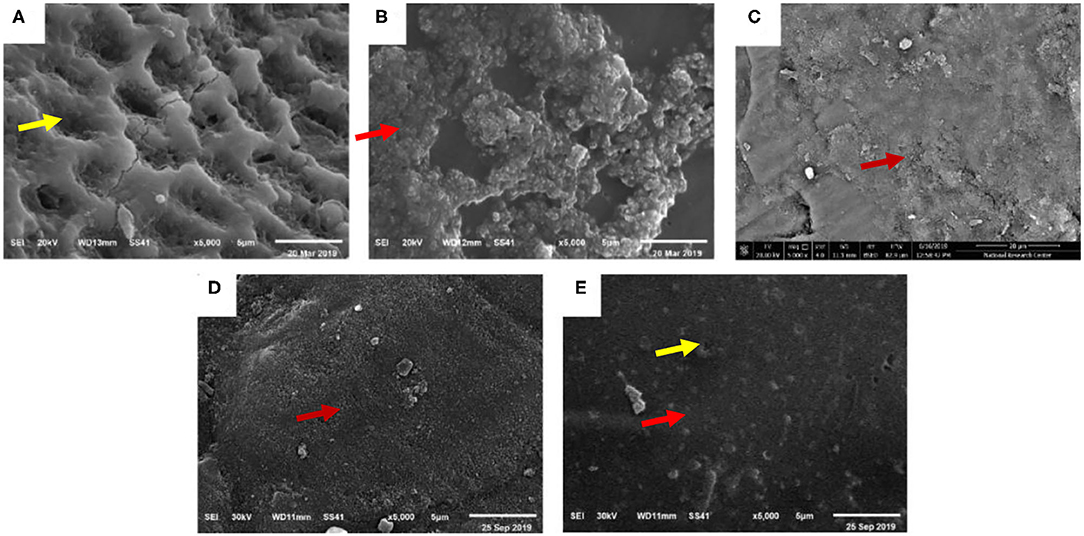
Figure 11. Scanning electron microscopy (SEM) image × 5,000 showing, (A) outer surface of untreated enamel caries like lesions showing obvious dissolution of the prisms or interprisms creating micro surface irregularities showed by yellow arrow (group I), (B) Desensibilize nano p (group II) showed smooth enamel surface showed by red arrow, (C) NHA-LPS suspension showing very smooth enamel surface and synthetic enamel surface showed by red arrow (group III), (D) NHA-SPS suspension showing few remnants of NHA showed by red arrow (group IV), and (E) NaF varnish showing some closed pores showed by red arrow and others still opened showed by yellow arrow (group V).
Discussion
Dental caries is a multifactorial biofilm-related disease (3) where bacteria play an important role due to being organized into a yellowish biofilm on teeth surfaces. Most caries active bacteria include S. mutans, Lactobacillus, Veillonella and Actinomyces species (5). Thus, acidogenic and aciduric S. mutans were selected for this study considering it as a major etiological challenge in the development of dental caries (47). This pathogen is known to be the main initiator of dental caries. It can produce glycosyltransferase (GTFs) and water insoluble extracellular polymeric substance from sucrose, leading to adhesion to tooth surface and contribution to form a biofilm (37).
Nano-hydroxyapatite has been incorporated in different commercial oral care preparation. According to the manufacturers, the maximum incorporation of NHA powder was 100 mg/ml while the concentration reached 200 mg/ml in paste and aqueous suspension. Therefore, we decided to test the effect of different concentration of NHA-LPS and NHA-SPS suspensions on the viability of normal fibroblasts using MTT assay at different time intervals to determine the safe concentration that could be used in further tests such as remineralization, antibacterial, and antibiofilm. It was found that both test suspensions showed no recorded cytotoxicity at concentrations ranged between 10 and 30 mg/ml, indicating safety of both powders at this range. Similar data were reported by Zhao and co-workers (48), Szymonowicz et al. (26), and Lamkhao et al. (49).
Concerning the antibacterial effect of NHA aqueous suspensions, the present study showed that NHA-LPS suspension recorded MBC of 2.5 mg/ml and MIC of 1.25 mg/ml. However, NHA-SPS suspension had no direct antibacterial action, Ciobanu et al. (50) combined silver with NHA to add an antibacterial effect while no MIC or MBC recorded even at high concentration (200 mg/ml) (51). Similar results were reported by Lim et al. (52). However, Lamkhao et al. (49) evaluated the antibacterial activity of different hydroxyapatite preparations using well diffusion method on nutrient agar plates. They reported that commercial and filtering hydroxy-apatite (HA) did not have any activity against Gram-positive bacteria while microwave-HA showed superior antibacterial effect. This might be due to the method of synthesis and nano-shape and particle size of NHA powder (48, 49).
Regarding EDX experiment, it was performed in order to aid in selecting the suitable remineralization concentration from the safe range that would also be evaluated against S. mutans mature biofilm. The results revealed slightly high remineralizing effect exerted following the use of 30 mg/ml of NHA-LPS suspensions without the significant difference in the concentration of Ca and P ions between both tested concentrations (30 and 100 mg/ml). So based on these data, the suggested concentration was 30 mg/ml for both test suspensions due to effectivity and safety. In another study, it was found that 100 mg/ml showed the optimal remineralizing effect (53) but it was not recommended in ours due to very high toxicity and absence of significant difference compared to the other concentration in regard to the remineralization. Also, NHA was found to drive the incorporation of large amounts of Ca and PO4 ions from oral environment into the crystal lattice forming NHA minerals. This remineralized enamel will be significantly resistant to future acid demineralization (54).
Microbial adhesion is considered the first step in biofilm formation which is known to be affected by a variety of microbiological-, physical-, chemical-, and material-related parameters (3, 6, 9). Also, it can be significantly influenced by the topography of substrate surface (10) where different studies (11, 13) reported that the roughened enamel surface could significantly increase the susceptibility to bacterial adhesion (14). Interestingly, the results of adhesion test in the present work showed that NHA-LPS suspension had anti-adherence activity because it recorded the least logarithmic number of bacterial colonies (0.69) with no significant difference compared to the positive control (NaF varnish; 0) while great significant differences were recorded with other groups indicating strong anti-adherence activity. Consequently, the application of this powder could decrease the biofilm formation and hence prevent the dental caries initiation. This came in an agreement with other studies reported that NHA introduced in the dental research field could block the specific bacterial adhesins, reduce the bacterial adherence, and interfere with the bacterial biofilm formation (13, 55–57).
Regarding mature biofilm thickness recorded for all test groups, it was found that group III (treated with NHA-LPS suspension) and group IV (treated with NHA-SPS suspension) showed significantly higher data (309.20 and 334.80 μm, respectively) compared to the positive control groups (group II; 183.70 and group V; 21.20 μm).
On the other hand, group III recorded very high level of dead bacteria (98.33%) which was significantly different with group IV (35.33%) while non-significantly different with group V (96.25%) indicating potent antimicrobial activity of NHA-LPS suspension that not only inhibited the bacterial growth but also killed live bacteria. Guerreiro-Tanomaru and co-workers (58) mentioned that the use of 100 mg/ml of NHA resulted in just one-log reduction in the mature biofilm of Enterococcus faecalis. Interestingly, our findings indicated potent antibiofilm activity of NHA-LPS that was used at 30 mg/ml and produced 98.33% dead bacteria. However, the increased biofilm thickness was explained by Miseon et al. (59) who reported that NHA can enhance biofilm thickness by inducing the transcription of glucosyltransferase enzyme leading to an increase in the production of insoluble glucans. In addition, NHA nanoparticles can act as a filler (22, 55) because it can fill the micropores and depressions of enamel surface (60) creating a new layer of synthetic enamel around the tooth surface, rather than hardening the existing layer, that chemically changes into calcium halo phosphate [Ca5(PO4)] (55) which was confirmed by our SEM results that revealed hard and smooth enamel surface following treatment by NHA-LPS suspension. This is reported to be more effective than NaF which forms hydroxyapatite particles, through providing Ca, PO4, and F ions to demineralized enamel (61). Moreover, NaF just provided surface coating deposits covering the pores of demineralized teeth forming a surface-zone of remineralization (superficial layer) without filling the lesion body (62). In addition, NaF was reported to have a little retention time on the enamel surface after which it dissolved and hence it had a limited local effect (63).
In conclusion, also limitation of this study as it was an in-vitro study so it cannot simulate cycles of re/demineralization in oral cavity, teeth used are bovine teeth not human, the used bacteria was only one strain of S. mutans that caused initiation of dental caries and the initial enamel caries was made chemically not by bacterial effect, the data of the present work showed that NHA-LPS suspension had potent antibacterial and antiadhesive actions that could prevent biofilm formation. This was caused by its bactericidal effect against mature biofilm of S. mutans beside its remineralizing effect; it can fill pores of initial enamel caries rather than closing pores superficially like NaF. Last, this powder was characterized by very low cost, safe, and locally synthesized in Egypt. Additionally, further preclinical as well as clinical studies are required in future to evaluate the antibacterial, antibiofilm, and remineralizing effect of NHA-LPS with larger particle size rather than that usually used (20–40 μl).
Data Availability Statement
The original contributions presented in the study are included in the article/supplementary material, further inquiries can be directed to the corresponding author/s.
Ethics Statement
Ethical approval was granted by Research Ethics Committee of Faculty of Dentistry, Tanta University, Egypt, on February 2020. Written informed consent for participation was not needed from the owners due to use of enamel from carcass of cows.
Author Contributions
All authors listed have made a substantial, direct, and intellectual contribution to the work and approved it for publication.
Conflict of Interest
The authors declare that the research was conducted in the absence of any commercial or financial relationships that could be construed as a potential conflict of interest.
Publisher's Note
All claims expressed in this article are solely those of the authors and do not necessarily represent those of their affiliated organizations, or those of the publisher, the editors and the reviewers. Any product that may be evaluated in this article, or claim that may be made by its manufacturer, is not guaranteed or endorsed by the publisher.
Acknowledgments
The authors would like to thank Dr. Ola Mohammed Elborady Lecturer, Institute of Nano Science and Nanotechnology, Kafrelsheikh University for helping them in the syntheses NHA powder.
Abbreviations
F, Fluoride ion; S. mutans, streptococcus mutans; NHA, Nano-hydroxyapatite; CPP-ACP, The casein phosphopeptide-amorphous calcium phosphate; Ca, Calcium; Po4, phosphate; cc, cubic centimeter; NaF, sodium fluoride; CaCL2, Calcium chloride; SEM, Scanning Electron Microscope; EDX, Energy Dispersive X-ray Analysis; ICNARA, the first international conference on novel anticaries and remineralization agent; MIRCEN, Microbiological Resources Centre; EDTA, ethylene di amine tetra acetic acid; CFU, Colony forming assay; DTT, D1,4-Dithiothreitol; AO, Acridine Orange fluoresces green; PI, Propidium iodide fluoresces red; MIC, Minimum Inhibitory Concentration; MBC, Minimum bactericidal effect; TEM, Transmission electron microscopy; MTT, 3-(4, 5-Dimethylthiazol-2-yl)-2, 5-diphenyltetrazolium bromide for dye; BHI, of brain heart infusion broth; SWS, Stimulated Whole Saliva; mM, Millimolar; μl, Microliter; GTFs, Glycosyltransferase enzyme.
References
1. Hamdy TM. Application of nanotechnology in dental caries management. EC Dent Sci. (2017) 16:52–5.
2. Pikatek-Jakubek K, Nowak J, Bołtacz-Rzepkowska E. Influence of infiltration technique and selected demineralization methods on the roughness of demineralized enamel: an in vitro study. Adv Clin Exp Med. (2017) 26:1179–88. doi: 10.17219/acem/66209
3. Pandit S, Kim J-E, Jung K-H, Chang K-W, Jeon J-G. Effect of sodium fluoride on the virulence factors and composition of Streptococcus mutans biofilms. Arch Oral Biol. (2011) 56:643–9. doi: 10.1016/j.archoralbio.2010.12.012
4. Selwitz RH, Ismail AI, Pitts NB. Dental caries. Lancet. (2007) 369:51–9. doi: 10.1016/S0140-6736(07)60031-2
5. Badjatia S, Badjatia RG, Thanveer K, Krishnan ACG. Effects of fluoride varnish on Streptococcus mutans count in saliva. Int J Clin Pediatr Dent. (2017) 10:62. doi: 10.5005/jp-journals-10005-1409
6. Flint SH, Bremer PJ, Brooks JD. Biofilms in dairy manufacturing plant-description, current concerns and methods of control. Biofouling. (1997) 11:81–97. doi: 10.1080/08927019709378321
7. Ahn S-J, Lee S-J, Kook J-K, Lim B-S. Experimental antimicrobial orthodontic adhesives using nanofillers and silver nanoparticles. Dent Mater. (2009) 25:206–13. doi: 10.1016/j.dental.2008.06.002
8. Anami LC, Pereira CA, Guerra E, e Souza RO de A, Jorge AOC, Bottino MA. Morphology and bacterial colonisation of tooth/ceramic restoration interface after different cement excess removal techniques. J Dent. (2012) 40:742–9. doi: 10.1016/j.jdent.2012.05.005
9. Hannig C, Gaeding A, Basche S, Richter G, Helbig R, Hannig M. Effect of conventional mouthrinses on initial bioadhesion to enamel and dentin in situ. Caries Res. (2013) 47:150–61. doi: 10.1159/000345083
10. Edwards KJ, Rutenberg AD. Microbial response to surface microtopography: the role of metabolism in localized mineral dissolution. Chem Geol. (2001) 180:19–32. doi: 10.1016/S0009-2541(01)00303-5
11. Bruinsma GM, der Mei HC, Busscher HJ. Bacterial adhesion to surface hydrophilic and hydrophobic contact lenses. Biomaterials. (2001) 22:3217–24. doi: 10.1016/S0142-9612(01)00159-4
12. Reyes CD, Garcia AJ. A centrifugation cell adhesion assay for high-throughput screening of biomaterial surfaces. J Biomed Mater Res Part A An Off J Soc Biomater Japanese Soc Biomater Aust Soc Biomater Korean Soc Biomater. (2003) 67:328–33. doi: 10.1002/jbm.a.10122
13. Mei L, Busscher HJ, van der Mei HC, Ren Y. Influence of surface roughness on streptococcal adhesion forces to composite resins. Dent Mater. (2011) 27:770–8. doi: 10.1016/j.dental.2011.03.017
14. Wang C, Zhao Y, Zheng S, Xue J, Zhou J, Tang Y, et al. Effect of enamel morphology on nanoscale adhesion forces of streptococcal bacteria: an AFM study. Scanning. (2015) doi: 10.1002/sca.21218
15. Brar GS, Arora AS, Khinda VIS, Kallar S, Arora K. Topographic assessment of human enamel surface treated with different topical sodium fluoride agents: Scanning electron microscope consideration. Indian J Dent Res. (2017) 28:617. doi: 10.4103/ijdr.IJDR_675_16
16. Reynolds EC. Calcium phosphate-based remineralization systems: scientific evidence? Aust Dent J. (2008) 53:268–73. doi: 10.1111/j.1834-7819.2008.00061.x
17. Ebadifar A, Nomani M, Fatemi SA. Effect of nano-hydroxyapatite toothpaste on microhardness ofartificial carious lesions created on extracted teeth. J Dent Res Dent Clin Dent Prospects. (2017) 11:14. doi: 10.15171/joddd.2017.003
18. Aziznezhad M, Alaghemand H, Shahande Z, Pasdar N, Bijani A, Eslami A, et al. Comparison of the effect of resin infiltrant, fluoride varnish, and nano-hydroxy apatite paste on surface hardness and streptococcus mutans adhesion to artificial enamel lesions. Electron Physician. (2017) 9:3934. doi: 10.19082/3934
19. Vyavhare S, Sharma DS, Kulkarni VK. Effect of three different pastes on remineralization of initial enamel lesion: an in vitro study. J Clin Pediatr Dent. (2015) 39:149–60. doi: 10.17796/jcpd.39.2.yn2r54nw24l03741
20. Kalra DD, Kalra RD, Kini P V, Prabhu CRA. Non-fluoride remineralization: An evidence-based review of contemporary technologies. J Dent Allied Sci. (2014) 3:24. doi: 10.4103/2277-4696.156525
21. Tyagi SP, Garg P, Sinha DJ, Singh UP others. An update on remineralizing agents. J Interdiscip Dent. (2013) 3:151. doi: 10.4103/2229-5194.131200
22. El Assal D-W, Saafan A-M, Moustafa D-H, Al-Sayed M-A. The effect of combining laser and nanohydroxy-apatite on the surface properties of enamel with initial defects. J Clin Exp Dent. (2018) 10:e425. doi: 10.4317/jced.54371
23. Abdelnabi A, Hamza MK, El-Borady OM, Hamdy TM. Effect of different formulations and application methods of coral calcium on its remineralization ability on carious enamel. Open Access Maced J Med Sci. (2020) 8:94–9. doi: 10.3889/oamjms.2020.4689
24. Mehta AB, Kumari V, Jose R, Izadikhah V. Remineralization potential of bioactive glass and casein phosphopeptide-amorphous calcium phosphate on initial carious lesion: an in-vitro pH-cycling study. J Conserv Dent JCD. (2014) 17:3. doi: 10.4103/0972-0707.124085
25. Dimić ID, Cvijović-Alagić IL, Rakin MB, Perić-Grujić AA, Rakin MP, Bugarski BM, et al. Effect of the pH of artificial saliva on ion release from commercially pure titanium. Acta Period Technol. (2013) 2013:207–15. doi: 10.2298/APT1344207D
26. Szymonowicz M, Korczynski M, Dobrzynski M, Zawisza K, Mikulewicz M, Karuga-Kuzniewska E, et al. Cytotoxicity evaluation of high-temperature annealed nanohydroxyapatite in contact with fibroblast cells. Materials (Basel). (2017) 10:590. doi: 10.3390/ma10060590
27. Buranrat B, Prawan A, Kukongviriyapan V. Optimization of Resazurin-based Assay for Cytotoxicity Test in Cholangiocarcinoma Cells. KKU Res J. (2008) 8:73–80. doi: 10.1016/j.mimet.2013.12.011
29. Yassen GH, Platt JA, Hara AT. Bovine teeth as substitute for human teeth in dental research: a review of literature. J Oral Sci. (2011) 53:273–82. doi: 10.2334/josnusd.53.273
30. Nogueira RD, Silva CB, Lepri CP, Palma-Dibb RG, Geraldo-Martins VR. Evaluation of surface roughness and bacterial adhesion on tooth enamel irradiated with high intensity lasers. Braz Dent J. (2017) 28:24–9. doi: 10.1590/0103-6440201701190
31. Karlinsey RL, Pfarrer AM. Fluoride plus functionalized β-TCP: a promising combination for robust remineralization. Adv Dent Res. (2012) 24:48–52. doi: 10.1177/0022034512449463
32. Melo MAS, Guedes SFF, Xu HHK, Rodrigues LKA. Nanotechnology-based restorative materials for dental caries management. Trends Biotechnol. (2013) 31:459–67. doi: 10.1016/j.tibtech.2013.05.010
33. Raum K, Kempf K, Hein HJ, Schubert J, Maurer P. Preservation of microelastic properties of dentin and tooth enamel in vitro—a scanning acoustic microscopy study. Dent Mater. (2007) 23:1221–8. doi: 10.1016/j.dental.2006.11.009
34. Hegde MN, Devadiga D, Jemsily PA. Comparative evaluation of effect of acidic beverage on enamel surface pre-treated with various remineralizing agents: an In vitro study. J Conserv Dent JCD. (2012) 15:351. doi: 10.4103/0972-0707.101902
35. Dominici JT, Eleazer PD, Clark SJ, Staat RH, Scheetz JP. Disinfection/sterilization of extracted teeth for dental student use. J Dent Educ. (2001) 65:1278–80. doi: 10.1002/j.0022-0337.2001.65.11.tb03488.x
36. Saito T, Ueda O, Teramoto S, Oguchi H, Yanagisawa S, Miyazawa H. Bacteriological evaluation of mutans streptococci using modified mitis-salivarius-bacitracin (MSB) agar medium in primary dentition period. Pediatr Dent J. (2007) 17:53–7. doi: 10.1016/S0917-2394(07)70095-2
37. Chau NPT, Pandit S, Jung J-E, Jeon J-G. Evaluation of Streptococcus mutans adhesion to fluoride varnishes and subsequent change in biofilm accumulation and acidogenicity. J Dent. (2014) 42:726–34. doi: 10.1016/j.jdent.2014.03.009
38. de Carvalho FG, Vieira BR, Santos RL dos, Carlo HL, Lopes PQ, de Lima BASG. In vitro effects of nano-hydroxyapatite paste on initial enamel carious lesions. Pediatr Dent. (2014) 36:85E–−89E.
39. Arakawa T, Fujimaru T, Ishizaki T, Takeuchi H, Kageyama M, Ikemi T, et al. Unique functions of hydroxyapatite with mutans streptococci adherence. Quintessence Int (Berl). (2010) 41:12.
40. Chandra PVR, Kumar VH, Reddy SJ, Kiran DR, Krishna MN, Kumar GV. Biofilm forming capacity of Enterococcus faecalis on Gutta-percha points treated with four disinfectants using confocal scanning laser microscope: an in vitro study. Dent Res J (Isfahan). (2015) 12:331. doi: 10.4103/1735-3327.161446
41. De Jong MH, der Hoeven JS. The growth of oral bacteria on saliva. J Dent Res. (1987) 66:498–505. doi: 10.1177/00220345870660021901
42. Rahim ZHA, Fathilah AR, Irwan S, Wan Nordini Hasnor WI. An artificial mouth system (NAM model) for oral biofilm research. Res J Microbiol. (2008) 3:466–73. doi: 10.3923/jm.2008.466.473
43. Rosenberg M, Azevedo NF, Ivask A. Propidium iodide staining underestimates viability of adherent bacterial cells. Sci Rep. (2019) 9:6483. doi: 10.1038/s41598-019-42906-3
44. Hussain H, Ahmad S, Abd. Razak MF, Wan Mohamud WN, Bakar J, Ghazali HM. Determination of cell viability using acridine orange/propidium iodide dual-spectrofluorometry assay. Cogent Food Agric. (2019) 5:1582398. doi: 10.1080/23311932.2019.1582398
45. Mohamed MM, Fouad SA, Elshoky HA, Mohammed GM, Salaheldin TA. Antibacterial effect of gold nanoparticles against Corynebacterium pseudotuberculosis. Int J Vet Sci Med. (2017) 5:23–9. doi: 10.1016/j.ijvsm.2017.02.003
46. Wang Z, Shen Y, Haapasalo M. Dynamics of dissolution, killing and inhibition of dental plaque biofilm. Front Microbiol. (2020) 11:964. doi: 10.3389/fmicb.2020.00964
47. Yu OY, Zhao IS, Mei ML, Lo ECM, Chu CH. Caries-arresting effects of silver diamine fluoride and sodium fluoride on dentine caries lesions. J Dent. (2018) 78:65–71. doi: 10.1016/j.jdent.2018.08.007
48. Zhao X, Ng S, Heng BC, Guo J, Ma L, Tan TTY, et al. Cytotoxicity of hydroxyapatite nanoparticles is shape and cell dependent. Arch Toxicol. (2013) 87:1037–52. doi: 10.1007/s00204-012-0827-1
49. Lamkhao S, Phaya M, Jansakun C, Chandet N, Thongkorn K, Rujijanagul G, et al. Synthesis of hydroxyapatite with antibacterial properties using a microwave-assisted combustion method. Sci Rep. (2019) 9:1–9. doi: 10.1038/s41598-019-40488-8
50. Ciobanu CS, Massuyeau F, Constantin LV, Predoi D. Structural and physical properties of antibacterial Ag-doped nano-hydroxyapatite synthesized at 100 C. Nanoscale Res Lett. (2011) 6:1–8. doi: 10.1186/1556-276X-6-613
51. Seyedmajidi S, Rajabnia R, Seyedmajidi M. Evaluation of antibacterial properties of hydroxyapatite/bioactive glass and fluorapatite/bioactive glass nanocomposite foams as a cellular scaffold of bone tissue. J Lab Physicians. (2018) 10:265. doi: 10.4103/JLP.JLP_167_17
52. Lim PN, Teo EY, Ho B, Tay BY, Thian ES. Effect of silver content on the antibacterial and bioactive properties of silver-substituted hydroxyapatite. J Biomed Mater Res Part A. (2013) 101:2456–64. doi: 10.1002/jbm.a.34544
53. Huang SB, Gao SS, Yu HY. Effect of nano-hydroxyapatite concentration on remineralization of initial enamel lesion in vitro. Biomed Mater. (2009) 4:0–5. doi: 10.1088/1748-6041/4/3/034104
54. Jindal S, Gupta N, Gupta P, Arora V, Mehta N. Reverse The Adverse: A Review. Int J Adv Heal Sci. (2015) 1:21–4.
55. Pepla E, Besharat LK, Palaia G, Tenore G, Migliau G. Nano-hydroxyapatite and its applications in preventive, restorative and regenerative dentistry: a review of literature. Ann Stomatol (Roma). (2014) 5:108. doi: 10.11138/ads/2014.5.3.108
56. Hannig C, Basche S, Burghardt T, Al-Ahmad A, Hannig M. Influence of a mouthwash containing hydroxyapatite microclusters on bacterial adherence in situ. Clin Oral Investig. (2013) 17:805–14. doi: 10.1007/s00784-012-0781-6
57. Kensche A, Holder C, Basche S, Tahan N, Hannig C, Hannig M. Efficacy of a mouthrinse based on hydroxyapatite to reduce initial bacterial colonisation in situ. Arch Oral Biol. (2017) 80:18–26. doi: 10.1016/j.archoralbio.2017.03.013
58. Guerreiro-Tanomaru JM, Vazquez-Garcia FA, Bosso-Martelo R, Bernardi MIB, Faria G, Tanomaru Filho M. Effect of addition of nano-hydroxyapatite on physico-chemical and antibiofilm properties of calcium silicate cements. J Appl Oral Sci. (2016) 24:204–10. doi: 10.1590/1678-775720150422
59. Park M, Sutherland JB, Rafii F. Effects of nano-hydroxyapatite on the formation of biofilms by Streptococcus mutans in two different media. Arch Oral Biol. (2019) 107:104484. doi: 10.1016/j.archoralbio.2019.104484
60. Haghgoo R, Ahmadvand M, Moshaverinia S. Remineralizing effect of topical novamin and nano-hydroxyapatite on caries-like lesions in primary teeth. J Contemp Dent Pract. (2016) 17:645–9. doi: 10.5005/jp-journals-10024-1905
61. Jones N, Ray B, Ranjit KT, Manna AC. Antibacterial activity of ZnO nanoparticle suspensions on a broad spectrum of microorganisms. FEMS Microbiol Lett. (2008) 279:71–6. doi: 10.1111/j.1574-6968.2007.01012.x
62. Amaechi BT. Remineralization therapies for initial caries lesions. Curr Oral Heal Reports. (2015) 2:95–101. doi: 10.1007/s40496-015-0048-9
Keywords: MIC, bacterial adhesion, initial enamel caries like lesions, remineralization, cytotoxicity
Citation: El-Gar YHA, Etman WM, Genaid TM and Al-Madboly LA (2022) Potent Antibacterial and Antibiofilm Activities of a Synthetic Remineralizing Preparation of Nano-Hydroxyapatite Against Cariogenic Streptococcus mutans Using an Ex-vivo Animal Model. Front. Dent. Med. 3:738326. doi: 10.3389/fdmed.2022.738326
Received: 08 July 2021; Accepted: 27 January 2022;
Published: 01 March 2022.
Edited by:
Jae-Sung Kwon, Yonsei University, South KoreaReviewed by:
Vijayakumar Sekar, Shandong University, ChinaDinesh Rokaya, Walailak University International College of Dentistry, Thailand
Uriel Zapata, EAFIT University, Colombia
Copyright © 2022 El-Gar, Etman, Genaid and Al-Madboly. This is an open-access article distributed under the terms of the Creative Commons Attribution License (CC BY). The use, distribution or reproduction in other forums is permitted, provided the original author(s) and the copyright owner(s) are credited and that the original publication in this journal is cited, in accordance with accepted academic practice. No use, distribution or reproduction is permitted which does not comply with these terms.
*Correspondence: Yasmeen H. Abo El-Gar, yassemen.hashem@sue.edu.eg; Lamiaa A. Al-Madboly, lamia.youssif@pharm.tanta.edu.eg