- 1School of Dental Sciences, Translational and Clinical Research Institute, Faculty of Medical Sciences, Newcastle University, Newcastle-Upon-Tyne, United Kingdom
- 2National Centre for Molecular Hydrodynamics, and Soft Matter Biomaterials and Bio-Interfaces, University of Nottingham, Nottinghamshire, United Kingdom
- 3School of Dentistry, University Dental Hospital, Cardiff University, Cardiff, United Kingdom
Dental erosion continues to be a significant global health concern affecting nearly 30% of adults worldwide. With increasing soft drink consumption predominantly driving its prevalence, strategies for prevention and control are often implemented when erosion is severe, or rates are high in the populace. While factors affecting dental erosion such as pH on enamel have received much attention, the effect of dietary acid concentration when factored out to a commercially available pH has yet to be determined. Furthermore, understanding these effects on dentine, which is known to be more susceptible to erosion than enamel can unravel structure-property relationships between acid characteristics and hard tissue types. This study aimed to develop structure-property relationships between dietary acid concentration, and pH, on the nano-textural and nano-mechanical properties of human enamel and dentine during short-term simulated drinking. To achieve this, a novel sample preparation methodology and analysis approach was developed by applying atomic force microscopy (AFM) in quantitative imaging mode. This enabled simultaneous measurement of enamel and dentine morphology and mechanical properties. Flow-cells were used to simulate drinking, exposing polished and smear layer-free human enamel and dentine to 30 s repeated cycles of unbuffered citric acid 6% (pH = 1.88) and 1% (w/v) (pH = 2.55) and commercially available buffered pH = 3.8 states, for up to 180 s. The same 50 µm × 50 µm area of specimen morphology was analysed using in-house developed nanotextural analysis using the bearing area curve (BAC) with a focus on roughness (Ra), normalised peak (PA) and valley areas (VA). Mechanical properties were simultaneously measured for stiffness (N/m) after each 30 s. While all studies agree pH is a major factor in the erosion of enamel, here its dominance over the treatment time varied, with concentration surpassing the importance of pH after initial acid contact. Conversely, dentine erosion showed concentration-dependent changes in morpho-mechanical properties only. These results not only highlight the dynamic process of erosion but also how the interplay between acid characteristics and dental tissue type impacts the progression of very early-stage erosion.
Introduction
Dental erosion continues to be a significant global health concern with up to 29% of young adults (18–35-year-olds) exhibiting some erosion, of which 3% show severe erosion (1–4). While the causes of erosion are multifactorial, the consumption of fruit juices and carbonated soft drinks is particularly significant due their acidic pH; this leads to demineralisation of first the enamel, and eventually the dentine (5). Many laboratory studies have been conducted with the aim of better understanding the effect of acids on these mineralised tissues, but these studies tend to focus observations on either enamel (6–16) or dentine in isolation (17–26), with very few focussing on erosion of both tissues under the same conditions (27–32).
The application of remineralising agents such as fluoride in toothpastes (33–35) and in water sources (36, 37) have provided a moderate reduction in erosive wear in populations (38–40); Government guidelines even provide public health (41) advice on the condition and method of drinking, such as reducing the temperature of erosive drinks, and encouraging the use of straws (42). However, rates remain high. Identifying structure-property relationships between dietary acid characteristics and erosive wear has received much attention. The erosive severity of dietary acids based on; pH (29, 43–45), titratable acidity (44, 46), buffering capacity (15, 47), undissociated acid concentration (48, 49), acid dissociation constants (50–52), acid concentration (7), and phosphate/carbonate contents (45, 53) have highlighted their dynamic impact on dental erosion. Reduction in acid pH and increases in acid concentration have increased erosion on dental hard tissues (7, 15, 27, 29, 31, 44–46, 49–52) while improving buffering properties have shown a significant reduction in erosive severity (43, 45, 47–49, 54). While these studies have shown the impact of varying solution properties on erosion studies have applied varying degrees of erosive severity, experimental approaches, and have not approached their research questions from a dietary perspective. For example, the impact of drink properties such as concentration and pH of those found in commercially available products.
From the literature, four major problems in understanding the effect of dietary acid erosion on dental hard tissues have been identified. Firstly, as off-the-shelf products tend to have various acid concentrations and pH (45, 53, 55), the effects of pH and concentration on erosion are often convoluted. These can be deconvoluted by analysing the effect of unbuffered and buffered acids of similar concentrations. Secondly, a better understanding can be achieved by focusing on the dental erosion at the nanoscale, in combination with time-resolved, in situ acid exposure, as very early changes can be detected, sequentially, on the same area. Thirdly, traditional measurements are based on roughness (13, 56–62), step-height changes (63–65) and hardness (54, 66–69), all of which are limited by the detection method and do not translate well for dentine compared to enamel (70). Utilising more complex surface analysis methods designed for multi-stratified surfaces can provide a more meaningful measure of surface features, such as the bearing area curve analysis (71–73). Finally, with enamel being the focus of dental erosion studies as it is normally the first structure to be attacked by acids, the morpho-mechanical analysis of dentine erosion has received less attention. Most studies that have focused on dentine erosion have approached experiments from an endodontic perspective (74–78), in which root dentine specimens are exposed to high concentrations of various acids or chelates, revealing the extremes of eroded morphology to enhance bonding procedures (79–81).
To overcome these problems, this study aimed to deconvolute the effect of dietary acid pH and concentration on human enamel and dentine erosion. This was conducted to identify the effect of acid concentration when pH is factored out to a typical, commercially available pH (pH = 3.8) (82), highlighting the impact of these 2 variables on erosive severity. To understand this effect in greater detail, novel advanced nanoscale techniques with complex textural surface and mechanical analysis, combined with a simulated drinking model during short-term repeated exposures have been applied.
Methods
Sample preparation
Enamel and dentine specimens (n = 25 per dental tissue type) were cut from healthy enamel and dentine taken from 40 erupted human 3rd molars in the occlusal plane, using a water-cooled diamond saw (Skilldent, Skillbond UK), to produce 0.5 mm3 sections. This was conducted in accordance with local and national rules dictated by Newcastle University Biomedicine Biobank, and fully anonymised in compliance with the ethical and legal framework of the Human Tissue Act (HTA) of 2004. Each section was embedded in polyester resin (Sigma-Aldrich, UK) and was cut out using the water-cooled diamond saw to produce 0.75 mm3 embedded sections for polishing. Specimens were then polished perpendicular to the buccal surface using 1,200 grit silicon carbide paper (Norton, Northants, UK) to reach the embedded mineralised surface. This was then followed by 1 µm, 0.3 µm and 0.05 µm aluminium oxide solutions (BUEHLER®, Illinois, USA) in sequence (BUEHLER® Metaserv Twin Polisher, Illinois, USA). This enabled approximately 0.05 mm to be removed from the initial enamel and dentine surface providing consistency in section depth, allowing a 0.5 mm2 2-dimensional hard tissue surface for acid exposure. Each section was then ultrasonicated (Langford Electronics Ltd, UK) in Chloramine-T (1% w/v) (Sigma-Aldrich, UK) at 4°C and transferred to fresh Chloramine-T (1% w/v) solutions and stored at 4°C before use.
Dietary acid solutions
Citric acid solutions of concentrations 1% (w/v) and 6% (w/v) were produced by diluting citric acid (Sigma-Aldrich, UK) in distilled water. The solution pH was measured using a pH meter (Orion 4 Star, Thermo Electron Ltd, Massachusetts, USA) at room temperature (22°C). Next these solutions were divided in half and one half was buffered to pH = 3.8 by adding 1 M NaOH (Sigma) to the solution. All acidic solutions were freshly made on the day of analysis. The polished enamel and dentine sections were then randomly divided into five study groups containing five specimens each for each acid solution. Group 1 and 2 specimens were exposed to citric acid 6% (w/v) pH = 1.88 and citric acid 1% (w/v) pH = 2.55. Group 3 and 4 specimens were exposed to citric acid 6% (w/v) and citric acid 1% (w/v) both at pH = 3.8. Group 5 specimens were exposed to a control solution consisting of Dulbecco's phosphate-buffered saline (PBS) (pH 7.20)(Lonza, BioWhittaker®).
In-vitro Simulated Drinking Model
Specimens were attached to a circular glass coverslip (AGL46R13-1, Agar Scientific, Essex, UK) using 2-part curable adhesive (Araldite Instant, Huntsman Advanced Materials, UK) and placed individually into an AFM flow-cell (JPK Instruments, Bruker, FR). 1 ml of PBS solution was injected into the flow-cell for baseline analysis. 1 ml of dietary acid solutions was then injected, left for 30 s, and then eluted using a 1 ml syringe (Terumo HT-SLWC-0R2W 1 ml Syringe, Tokyo, JP), washed twice with fresh PBS and further replaced with PBS for analysis to stop the erosive process. This continued, performing quantitative imaging analysis after each 30 s interval under PBS hydrated conditions until each specimen experienced a total of 180 s acid exposure.
AFM imaging, textural and mechanical analysis
AFM imaging and mechanical analysis were performed using a Nanowizard 3 AFM (JPK Instruments, Bruker, FR), operating in quantitative imaging mode (QI™), using gold coated non-conductive silicon nitride cantilevers (NP-10, Bruker, Camarillo CA, USA). AFM cantilever spring constant calibration was performed using the in-built software calibration procedure for all probes providing an average spring constant of 0.204 ± 0.107 N/m. All quantitative images were obtained at a resolution of 256 × 256 and scans were performed over the same 50 µm × 50 µm areas after each acid exposure in PBS. Average roughness (Ra) was measured using integrated JPK software. Bearing area curves (BAC) were calculated by extracting two-dimensional line traces at 12.5 µm, 25 µm and 37.5 µm in both the x- and y-direction from each topographic image and importing them into using in house developed Visual Basic® code. From this code the BAC parameter peak area (PA) (area occupied by material peaks) and valley area (VA) (area occupied by material voids) were calculated within defined regions each BAC (67). Stiffness (N/m) was extracted from resulting force distance curves from each pixel in the 256 × 256 quantitative image using dedicated software (JPK Data Processing Software).
Statistical analysis
Average roughness (Ra), peak area (PA), valley area (VA) and stiffness were all found to be non-normally distributed, so non-parametric statistics were used for analysis. Statistically significant differences in all analysed parameters for each acid sub-group and exposure time were conducted by Kruskal-Wallis test (P < 0.001). A post-hoc Tukey's test was used to identify significant differences between acid pH, concentration, any interaction between pH and concentration, and the control specimens. Medians with interquartile ranges were used to identify statistically significant differences at a 95% confidence level. As the specimens were derived from different human donors there were some baseline variations in the PA, VA and stiffness. Consequently, we normalised these data to the value measured for each specimen before they were exposed to an acid solution, t = 0 s.
Results
Typical topography images for enamel specimens treated with each solution are shown in Figure 1, with the stiffness maps for these enamel specimens in Figure 2. Likewise, typical topography and stiffness images for dentine specimens treated with each solution are shown in Figures 3, 4, respectively. Quantitative roughness, normalised peak and valley area and normalised stiffness data are plotted as a function of exposure time with enamel shown in Figures 5A,C,E,G and dentine shown in Figures 5B,D,F,H respectively.
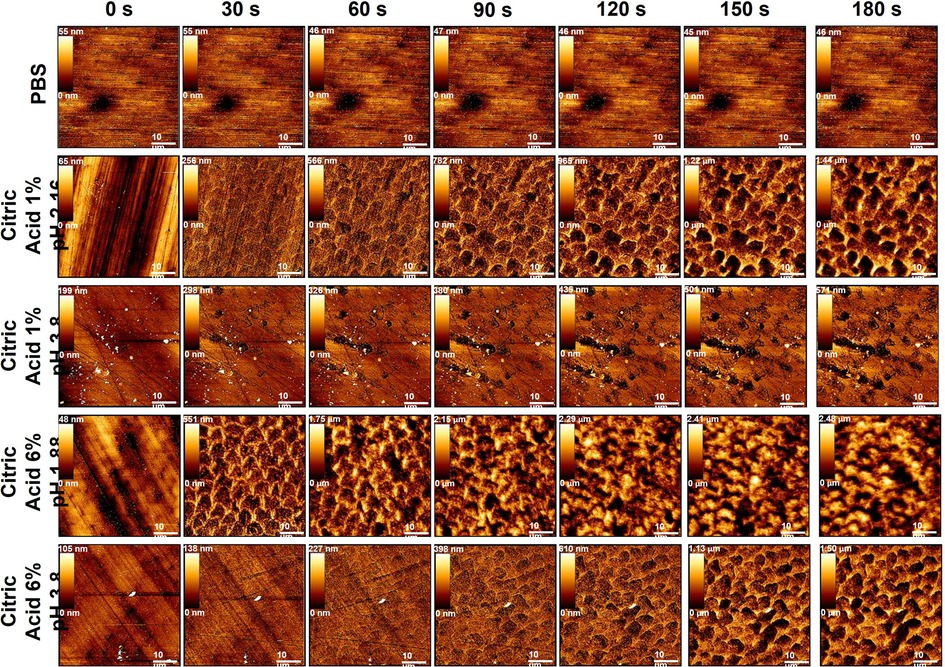
Figure 1 Showing example topographic AFM imaging of human enamel exposed to all dietary solutions (top) throughout the exposure regime starting from 0 s (top) to 180 s (bottom) at 30 s intervals.
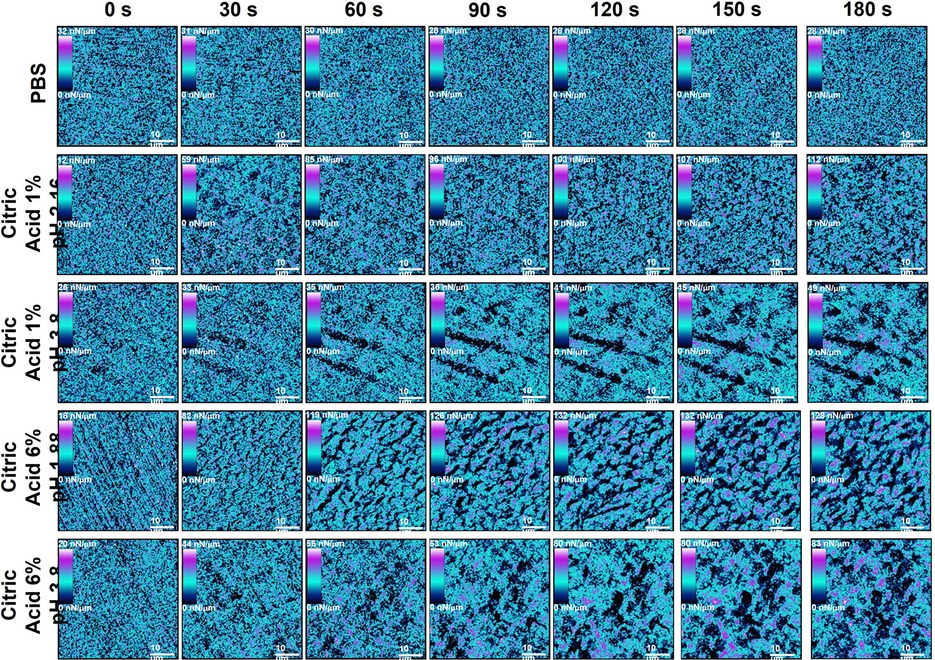
Figure 2 Showing example stiffness AFM mapping of human enamel exposed to all dietary solutions (top) throughout the exposure regime starting from 0 s (top) to 180 s (bottom) at 30 s intervals.
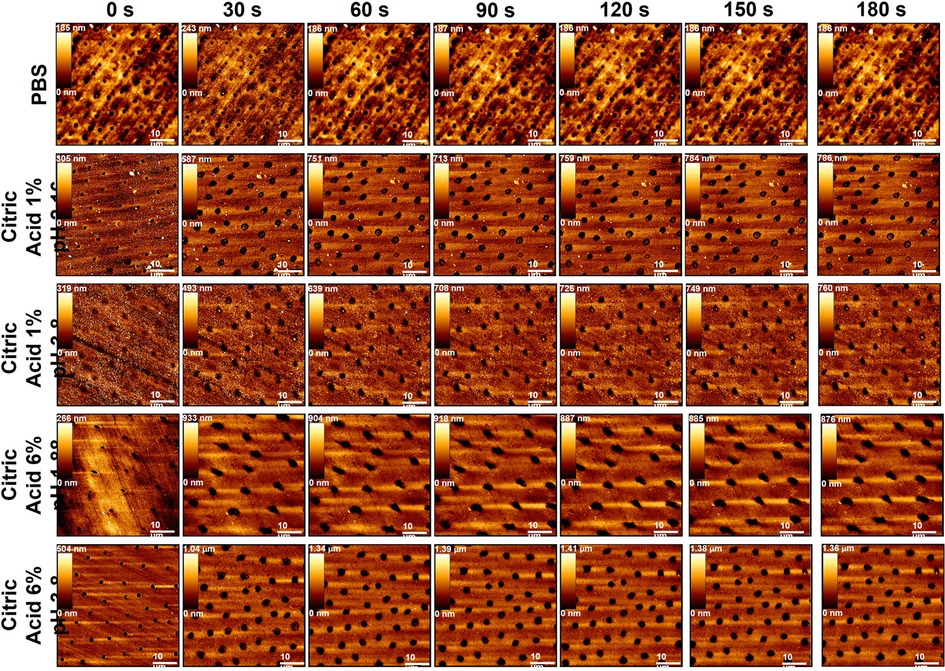
Figure 3 Showing example topographic AFM imaging of human dentine exposed to all dietary solutions (top) throughout the exposure regime starting from 0 s (top) to 180 s (bottom) at 30 s intervals.
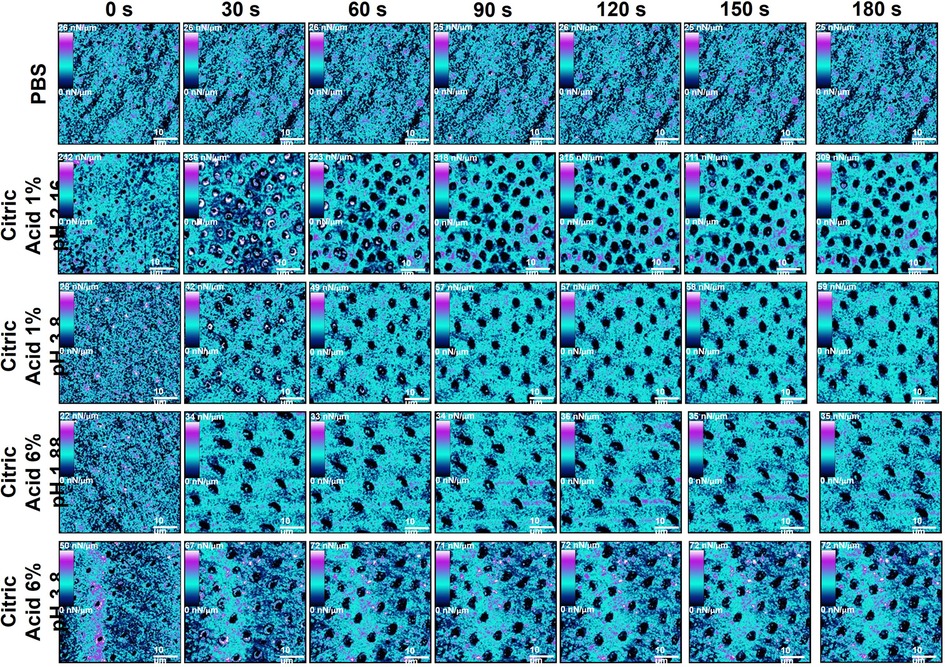
Figure 4 Showing example stiffness AFM mapping of human dentine exposed to all dietary solutions (top) throughout the exposure regime starting from 0 s (top) to 180 s (bottom) at 30 s intervals.
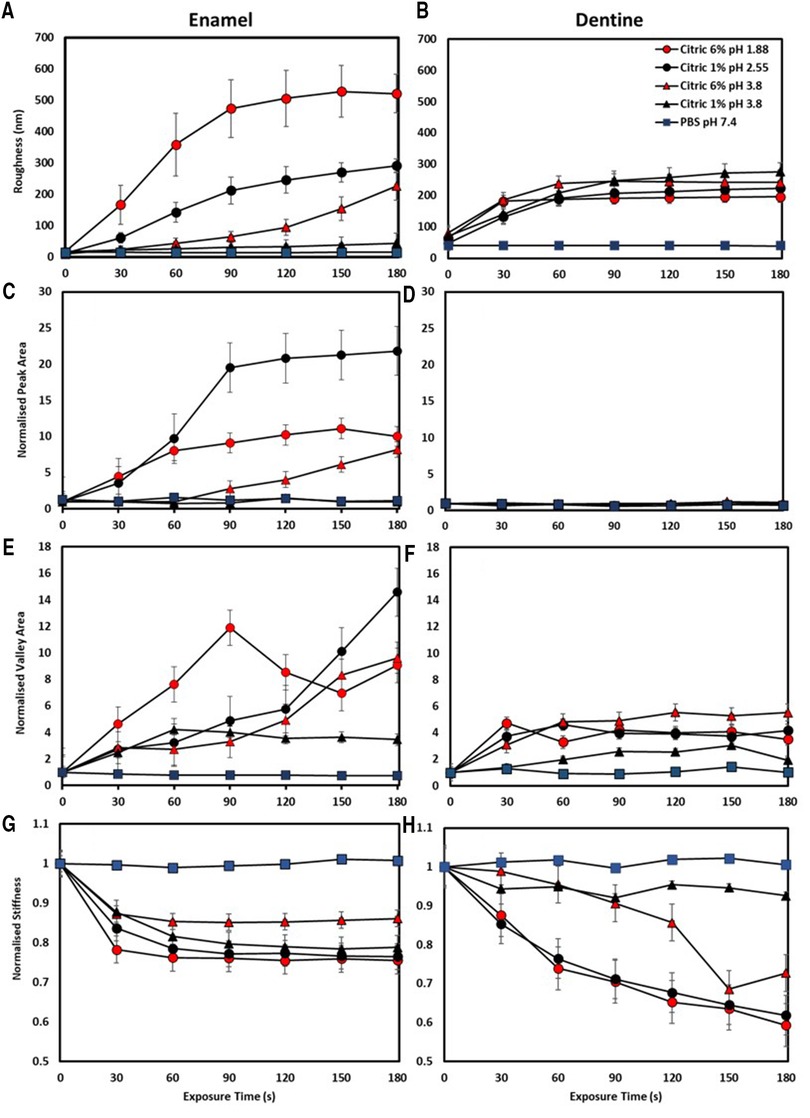
Figure 5 Showing median and interquartile ranges of n = 25 human enamel (left) and n = 25 dentine (right) exposed to all dietary solutions with (A,B) showing roughness (nm), (C,D) normalised peak area, (E,F) normalised valley area and (G,H) normalised stiffness. A post-hoc Tukeys test was used to identify statistically significant differences (P < 0.05).
Enamel erosion
For enamel specimens treated with PBS there were no obvious changes in morphology over the 180 s of exposure while there are clear changes evident for all acid-treated samples shown in Figure 1. When comparing the morphological changes with respect to acid concentration and pH, surface changes occur faster with enamel prisms becoming obvious sooner as acid concentration increases, and pH of the solution decreases.
The solution pH significantly affected roughness at each time point (P < 0.001) with the 6% pH = 1.88 solution producing the greatest increase in roughness, significantly so when compared to PBS treatment (P < 0.05), Figure 5A. Acid concentration also significantly affected roughness, with the 6% solutions always producing greater roughening than the 1% solutions (P < 0.001) at either pH. There was significant interaction between pH and concentration on the increase in roughness due to exposure to the solutions (P < 0.001).
The PA and VA results further clarify the effect of acid pH and concentration on enamel erosion, shown in Figures 5C,E. Specimens treated with either citric acid 6% pH = 1.88 and 1% pH = 2.55 showed a steep rise in PA up to 60 s, after which the PA for the 6% pH = 1.88 plateaued while the 1% pH = 2.55 continued to rise, to a higher value, until 90 s of exposure. No significant rise in PA was seen for the 1% pH = 3.8 treated specimens, which corresponds to the lack of distinct prismatic structure visible in the topography images shown in Figure 1. However, 6% pH = 3.8 citric acid-treated specimens showed a different PA behaviour, with a rise in PA, corresponding with the appearance of enamel prisms, seen after 90 s exposure. Similar behaviour was seen for the VA data, with the 6% citric acid pH = 1.88 producing the greatest increase in this parameter up to 90 s, after which there was a decrease. For the 1% pH = 2.55 and the 6% pH = 3.8 acid treated specimens VA increased over the full 180 s, while the 1% pH = 3.8 acid led to a plateau in VA after 60 s of exposure. As PA and VA both show how the enamel erosion results in the formation of peaks and valleys within the prisms, by using these BAC-derived textural parameters it is possible to elucidate the more complex relationships in enamel erosion between acid concentration and pH than using Ra alone.
Treatment with PBS resulted in no change in stiffness shown in Figures 2, 5D (P > 0.05), while clear changes were obvious when any of the acid solutions were used. The stiffness images showed that the changes in stiffness were associated with the appearance of the enamel prisms. Treatment with citric acid 6% pH = 1.88 and 1% pH = 2.55 acid solutions led to a similar reduction in stiffness, with an initial rapid decrease found after 30 s exposure, followed by a much slower decrease for the remaining 180 s, shown in Figure 5G. Interestingly, a greater reduction in stiffness was observed on specimens exposed to 1% buffered acid when compared to 6% buffered acid.
Dentine erosion
Like that found for enamel there was no obvious effect on the dentine specimens due to exposure to PBS, shown in Figures 3, 4. While the specimen preparation method resulted in exposed dentinal tubules, confirming we were not testing enamel in the early time periods of this experiment, the overall appearance and stiffness of the PBS-treated specimens remained the same. In contrast, treating the dentine specimens with any of the acidic solutions for 30 s led to appreciable tubule widening compared to the baseline, t = 0 s, images. After 30 s of exposure no clear differences in the topography image was seen for any of the acid solution groups.
The tubular structure of the dentine surface made the roughness measurement insensitive to any differences in erosion due to either pH or concentration. In general, the overall behaviour for each acid solution was the same with an increase in Ra up to 90 s followed by a plateau, with no significant effect due to differences in pH (P > 0.05) or concertation (P = 0.166).
No significant change in PA was measured for specimens exposed to any of the acidic solutions, shown in Figure 5D. VA analysis shown Figure 5F, showed that dentine exposed to citric acid 6% pH = 1.88 and 1% pH = 2.55 exhibited the greatest change while specimens exposed to the pH = 3.8 acids showed a more progressive increase in normalised VA across the treatment regime compared to pH = 1.88 and pH = 2.55 exposed specimens, also increasing with concentration. The fact that roughness and PA were insensitive to the effects of acid exposure while VA showed some differences in behaviour highlights the difference in behaviour seen between enamel and dentine. Acid exposure resulted in the exposure of the enamel prisms which eroded more towards the prism centre than the edge, certainly at early exposure times, meaning that roughness data was composed of both material peaks and valleys. Dentine eroded differently, with only tubule widening seen, which corresponded to the roughness data only comprising material valleys.
The change in dentine stiffness due to exposure to the different acid solutions shown in Figure 5H, clearly demonstrated that both pH and concentration were significant factors. Treatment with the citric acid 1% pH = 3.8 solution resulted in the lowest reduction in stiffness after 180 s, while the 6% solution, at the same pH, caused significantly more reduction in stiffness (P < 0.05) at times after 120 s of exposure. Both citric acid 6% pH = 1.88 and 1% pH = 2.55 acid solutions, despite having different pHs, produced identical behaviour in the stiffness, both producing significantly more reduction in stiffness than either pH = 3.8 acid solution.
Discussion
Applying nanoscale textural and mechanical analysis enabled the effect of dietary acid pH and concentration on human enamel and dentine during short-term dietary acid exposure to be explored. While all studies agree pH is a major factor in the erosion of enamel (7, 29, 44, 45, 83–92), here its dominance over the treatment time varied, with acid concentration surpassing the importance of pH after initial acid contact. Conversely, dentine erosion showed concentration-dependent changes in morpho-mechanical properties only. These results not only highlight the dynamic process of erosion, but how the interplay between acid characteristics and dental tissue type impact the progression of very early-stage erosion.
Dietary acids are predominantly carboxylic and once in solution their dissociated ionic components play different roles in the erosive process (29). Mineral destabilisation of biological hydroxyapatite (bHAP) occurs through protonation (H+) of carbonate and phosphate groups of the crystal lattice, termed direct acid attack (93). This weakens the coordination of the surrounding calcium ion (49, 94) where the anionic component (R-COO−) subsequently adheres and decalcifies bHAP by chelation (50, 94, 95) through the adhesion-decalcification concept (93). For citric acid both mechanisms of erosion are known to occur on separate territories of bHAP at the Nernst layer (48, 49) i.e., the acid – enamel or dentine interface, which is static, and diffusion controlled (94). Additionally, undissociated acid (inactive acid) can diffuse into bHAP, acting as a mobile carrier for dissociation in enamel and dentine's water content (93). Further direct acid attack and chelation occur here in the subsurface as a high concentration of dissociated ions at the mineralisation front are maintained, termed subsurface erosion (48, 93). Buffering dietary acids to match the typical pH of commercially available fruit juices (pH = 3.8) reduces phosphate and carbonate group dissociation on the bHAP crystal lattice via direct acid attack (49) creating a higher diffusion gradient for subsurface erosion at the Nernst layer (49). As buffered citric acid approaches its pKa value (96), subsurface erosion is postulated to dominate the erosive activity.
Previous enamel erosion investigations have shown concentration-dependent mineral loss (27, 97, 98) with before and after dietary acid challenges showing increases in the prevalence of key-hole structures (61, 99–101), roughness (53, 56, 58, 102, 103), BAC parameters (73, 103), and reduction in mechanical properties (49, 69, 94, 104–106). Only one study has monitored increases in roughness during consecutive citric acid exposure of enamel on the same area using AFM (61). The study used relatively long-term treatment times (90–250 min) with no mechanical analysis or simulated drinking. Profilometric studies into short-term dietary acid enamel erosion have determined increases in BAC parameters (73), although again, the effects of pH and concentration were not deconvoluted.
Buffering dietary acids to a commercially relevant pH = 3.8 still enables dissolution of enamel surfaces (105) as it is far lower than the critical dissolution pH. For enamel, we propose buffering dietary acids to pH = 3.8 while maintaining the same concentration causes subsurface erosion to dominate the erosive process, as opposed to pH-driven direct acid attack. This is evident in pH = 3.8 acid exposed enamels delayed increases in roughness, BAC derived normalised PA, VA, and normalised stiffness reduction, compared to citric 6% pH = 1.88 and 1% pH = 2.55 acid exposed enamel, at the same concentration, shown in Figures 1, 5A,C,E,G. Citric 6% pH = 1.88 and 1% pH = 2.55 acid exposed enamel erosion was dominated by pH during initial exposure (30–90 s), causing saturation at the Nernst layer thereafter (94). At later exposure times (90–180 s) subsurface erosion dominates the morpho-mechanical changes of enamel.
Most research into dentine erosion has been applied from an endodontic perspective (74, 77, 107–111) using high concentrations of citric (92, 97), phosphoric (97), nitric (112) and lactic acids (92, 113), generating a rough surface to enhance bonding procedures. Here, dentine exhibited a very different trend in morpho-mechanical properties during erosion compared to enamel. The effect of tissue structure, organic components, ratio and microphase separation of the mineral phases (intratubular and peritubular dentine) must be considered when comparing the erosive characteristics of enamel and dentine. These structures have enabled them to function for specific roles, with enamel providing a hard surface for mastication (114) and dentine withstanding compression (115). While both substrates can be regarded as polymer reinforced composites, their degrees of mineral and organic constituents vary according to their associated roles. Historically, dentine has been regarded as more susceptible to erosion than enamel, as dissolution of its mineral component occurs at a higher pH (pH = 6.3 compared to enamel's pH = 5.5) (116). Moreover, dentine's carbonate content is greater in peritubular compared to intertubular dentine (117) increasing its susceptibility to direct acid attack (92, 112). This is evident by the immediate increase in observable tubule diameter and quantitative valley area analysis for all acid exposed dentine specimens shown in Figures 3, 5E respectively. After a certain depth of mineral is removed by demineralisation, mineral loss has been shown to significantly decrease (17, 19) and, in the absences of a collagen matrix, the rate of dissolution is markedly higher (17, 118). The surrounding liquid phase protects the mineral components even further, as collagen provides a buffering effect to increase the local pH (119, 120). De-bound mineral is prevented from immediate removal, increasing calcium and phosphate saturation at the Nernst-layer (48). This protective effect of collagen, preventing substantial erosion is a vital aspect in overall tooth protection from acid erosion. Here, increased acid concentration was found to remove peritubular dentine and cause exposure and subsequent collapse of the extra cellular collagen matrix faster than that of 1% citric acid solutions. This subsequently limited the changes of roughness, BAC derived normalised PA and VA and normalised stiffness measurements thereafter.
The collapse of the collagen matrix was found to play a significant role in the prevention of further changes in roughness and BAC derived parameters after the erosion of peritubular dentine. While erosion of peritubular dentine created significant changes in morphological parameters after initial exposure treatment, plateaux's were obtained after complete peritubular dentine removal. Intertubular dentine was postulated to protect any further demineralisation, as no changes in roughness and BACs were obtained. This occurred at a shorter exposure time for 6% citric acid exposed specimens (30 s), while 1% acid exposed specimens exhibited this at 60–90 s treatment in either pH = 2.55 or pH = 3.8 states. The stiffness of dentine erosion was shown to reflect a similar trend to that of the morphological parameters. Increased acid concentration exposed specimens yielded the largest reductions in stiffness over the exposure time. Again, the collapse of the collagen matrix was found to play a significant role in the prevention of further changes in stiffness after the erosion of peritubular dentine. This occurred at 30 s for 6% acid exposed dentine, while 1% exposed specimens plateaued at 60–90 s, previously un-reported.
Fundamental differences in enamel and dentine's mineral phase (106, 121–125) and proteinaceous material (121, 122, 126–128) give rise to these qualitative and quantitative differences in morphological and mechanical properties upon demineralisation. While carbonate substitution exhibited by bHAP increases the susceptibility of enamel to erosion compared to HAP (50, 119, 129, 130), increased carbonate substitution occurs in dentine compared to enamel (5%–6% compared to 3% respectively) (119), making dentine even more susceptible to erosion. Here, dietary acid pH i.e., direct acid attack dominated the erosive capacity of acids in very early-stage erosion of enamel, while concentration i.e., subsurface erosion surpassed the importance of pH after this. For dentine, we postulate the evolutionary role collagen plays in enabling bulk compression compliance also plays an effective and efficient role in protecting further morpho-mechanical changes during low pH dietary acid erosion.
If dietary acid solutions were buffered to a far lower pH = 2.3 to match the pH of cola drinks (131), we would expect pH driven direct acid attack to dominate the erosive process far more compared to concentration-driven subsurface erosion. This is due to the greater presence of dissociated H+ ions in solution for pH 2.3 dietary acid solutions and a reduction in undissociated acid for diffusion and subsequent dissociation into the subsurface. Enamel's delayed reduction in morpho-mechanical properties exposed to citric 6% pH = 3.8 solutions shown here would occur much faster in the exposure regime for those exposed to pH = 2.3. For dentine, we would expect concentration-driven subsurface erosion to dominate the erosive process at later exposure times with pH driven direct acid attack dominating the erosive process during initial acid contact. This would erode peritubular dentine almost instantly with the buffering properties of the collagenous network taking longer to reduce direct acid attack.
AFM is a powerful tool to image and quantify morpho-mechanical changes in dental hard and soft tissues subject to dynamic processes such as erosion (61) and oral biofilm development (132, 133). Operating in QI™ mode enabled careful control of probe-surface contact force, allowing no surface damage during imaging while simultaneously measuring sample stiffness. While previous studies have utilised this technique on dry dental specimens (134), here, for the first time, it was used on PBS hydrated specimens, after each acid exposure, using a simulated drinking model.
AFM cantilevers with low spring constants on relatively hard tissues such as enamel and dentine were chosen to minimise sample damage during analysis as it was expected that eroded specimens would become increasingly softer after each acid exposure. This meant that there was considerable variability between sample stiffness values measured between different specimens with different probes. Only once the data were normalised to the initial median stiffness prior to acid exposure did the overall trend become apparent. Since the same nominal force was applied across all surfaces and exposure times, enamel and dentine's resistance to deformation decreased during erosion, exhibited by the normalised reduction shown in Figures 5G,H, characteristic of surface softening.
This study has potential limitations. Improving the sample size by applying a power analysis could enhance statistical analysis between tissue type, dietary acid variables and analysed parameters. Due to the limited number of intact dental specimens that could be obtained for each specimen cohort n = 5 per group was sufficient. This enabled significant differences in analysed parameters to be obtained. Future investigators may wish to apply a power analysis in their study with a ready supply of intact dental specimens. Other limitations such as improving simulated drinking and the in vitro model to more reflect that the oral environment could be made. Utilising this novel platform investigators could reduce dietary acid exposure times to reflect sipping and apply artificial or pooled human saliva with varying mucin types and concentrations. Moreover, other dental hard tissue structures can be assessed such as prismatic or aprismatic enamel, enamel-dentine junctions, mantle, globular, sclerotic, primary, secondary, and tertiary dentine by sectioning at different depths. The effect of diseases could also be assessed enabling structure-property relationships to be developed on varying types amelogenesis imperfecta e.g., hypocalcification, hypoplasia, porphyria as well as fluorosis and celiac disease. While pH and concentration were the only variables assessed in this study, investigators may wish to understand the effect of other dietary acid properties such as titratable acidity, buffering capacity, undissociated acid concentration, acid dissociation constants and phosphate/carbonate contents, unravelling other structure-property relationships.
Future studies can now utilise this novel sample preparation methodology and nano-scale analysis protocol to determine the effect of other dietary acids on a variety of dental hard tissue types. Including, utilising the novel platform to determine the effect of agents to prevent, arrest or even remineralise eroded tissue structures.
Conclusion
This investigation sought to uncover the effect dietary acid concentration and pH play in the erosion of human enamel and dentine during very early-stage exposure. By developing novel approaches to sample preparation and simulated drinking, simultaneous nano- textural and nano-mechanical analysis of enamel and dentine were shown to exhibit very different responses to dietary acid erosion. Interestingly, for enamel, pH-driven direct acid attack was shown to dominate the erosive capacity of acids during initial exposure, while concentration-driven subsurface erosion was shown to dominate thereafter. For dentine, concentration was shown to dominate the erosive capacity of dietary acids. Moreover, while dentine has a higher critical pH of bHAP dissolution compared to enamel, here we have shown dentine to be less susceptible to dietary acid erosion, due to the protective effects of the collagen matrix.
Data availability statement
The raw data supporting the conclusions of this article will be made available by the authors, without undue reservation.
Author contributions
JP, JF, PJW and MJG contributed to conception, design, data acquisition, analysis, and interpretation and drafted the manuscript. All authors contributed to the article and approved the submitted version.
Funding
This work was funded by a BBSRC Industrial CASE Partnership with GSK BB/G530517/1 and supported by C. Fowler (GSK).
Conflict of interest
The authors declare that the research was conducted in the absence of any commercial or financial relationships that could be construed as a potential conflict of interest.
Publisher's note
All claims expressed in this article are solely those of the authors and do not necessarily represent those of their affiliated organizations, or those of the publisher, the editors and the reviewers. Any product that may be evaluated in this article, or claim that may be made by its manufacturer, is not guaranteed or endorsed by the publisher.
References
1. Strużycka I, Rusyan E, Bogusławska-Kapała A. Prevalence of dental erosion in young adults aged 18 years in Poland. Przegl Epidemiol. (2014) 68(4):689–93. doi: 10.5604/12321966.1228948
2. Al-Dlaigan Y, Shaw L, Smith A. Dental erosion in a group of British 14-year-old, school children. Part I: prevalence and influence of differing socioeconomic backgrounds. Br Dent J. (2001) 190:145–9. doi: 10.1038/sj.bdj.4800908
3. Nunn JH, Gordon PH, Morris AJ, Pine CM, Walker A. Dental erosion – changing prevalence? A review of British national childrens’ surveys. Int J Paediatr Dent. (2003) 13(2):98–105. doi: 10.1046/j.1365-263X.2003.00433.x
4. Aidi HE, Bronkhorst EM, Charlotte M, Huysmans DNJM, Truin GT. Dynamics of tooth erosion in adolescents: a 3-year longitudinal study. J Dent. (2010) 38(2):131–7. doi: 10.1016/j.jdent.2009.09.012
5. Zimmer S, Kirchner G, Bizhang M, Benedix M. Influence of various acidic beverages on tooth erosion. Evaluation by a new method. PloS One. (2015) 10(6):e0129462. doi: 10.1371/journal.pone.0129462
6. Lippert F, Parker DM, Jandt KD. Susceptibility of deciduous and permanent enamel to dietary acid-induced erosion studied with atomic force microscopy nanoindentation. Eur J Oral Sci. (2004) 112(1):61–6. doi: 10.1111/j.0909-8836.2004.00095.x
7. Hughes JA, West NX, Parker DM, Van Den Braak MH, Addy M. Effects of pH and concentration of citric, malic, and lactic acids on enamel, in vitro. J Dent. (2000) 28(2):147–52. doi: 10.1016/S0300-5712(99)00060-3
8. von Fraunhofer JA, Rogers MM. Dissolution of dental enamel in soft drinks. Gen Dent. (2004) 52(4):308–12.15366295
9. Khamverdi Z, Vahedi M, Abdollahzadeh S, Ghambari MH. Effect of a common diet and regular beverage on enamel erosion in various temperatures: an in-vitro study. J Dent. (2013) 10(5):411.
10. Derceli JDR, Faraoni JJ, Pereira-da-Silva MA, Palma-Dibb RG. Analysis of the early stages and evolution of dental enamel erosion. Braz Dent J. (2016) 27:313–7. doi: 10.1590/0103-6440201600667
11. Tahmassebi JF, Kandiah P, Sukeri S. The effects of fruit smoothies on enamel erosion. Eur Arch Paediatr Dent. (2014) 15(3):175–81. doi: 10.1007/s40368-013-0080-1
12. Kitchens M, Owens B. Effect of carbonated beverages, coffee, sports and high energy drinks, and bottled water on the in vitro erosion characteristics of dental enamel. J Clin Pediatr Dent. (2007) 31(3):153–9. doi: 10.17796/jcpd.31.3.1157l653t8206100
13. Mullan F, Mylonas P, Parkinson C, Bartlett D, Austin RS. Precision of 655 nm confocal Laser profilometry for 3D surface texture characterisation of natural human enamel undergoing dietary acid mediated erosive wear. Dent Mater. (2018) 34(3):531–7. doi: 10.1016/j.dental.2017.12.012
14. Rugg-Gunn AJ, Maguire A, Gordon PH, McCabe JF, Stephenson G. Comparison of erosion of dental enamel by four drinks using an intra-oral appliance. Caries Res. (1998) 32(5):337–43. doi: 10.1159/000016469
15. Owens B, Kitchens M. The erosive potential of soft drinks on enamel surface substrate: an. Scanning electron microscopy investigation. J Contemp Dent Pract. (2007) 8(7):11–20. doi: 10.5005/jcdp-8-7-11
16. Eisenburger M, Shellis RP, Addy M. Comparative study of wear of enamel induced by alternating and simultaneous combinations of abrasion and erosion in vitro. Caries Res. (2003) 37(6):450–5. doi: 10.1159/000073399
17. Hara AT, Ando M, Cury JA, Serra MC, Gonzalez-Cabezas C, Zero DT. Influence of the organic matrix on root dentine erosion by citric acid. Caries Res. (2005) 39(2):134–8. doi: 10.1159/000083159
18. Zarella BL, Cardoso CAB, Pelá VT, Kato MT, Tjäderhane L, Buzalaf MAR. The role of matrix metalloproteinases and cysteine-cathepsins on the progression of dentine erosion. Arch Oral Biol. (2015) 60(9):1340–5. doi: 10.1016/j.archoralbio.2015.06.011
19. Ganss C, Klimek J, Starck C. Quantitative analysis of the impact of the organic matrix on the fluoride effect on erosion progression in human dentine using longitudinal microradiography. Arch Oral Biol. (2004) 49(11):931–5. doi: 10.1016/j.archoralbio.2004.05.010
20. Domiciano SJ, Colucci V, Campos Serra M. Effect of two restorative materials on root dentine erosion. J Biomed Mater Res B Appl Biomed. (2010) 93(2):304–8. doi: 10.1002/jbm.b.31532
21. Görduysus M, Küçükkaya S, Bayramgil NP, Görduysus MÖ. Evaluation of the effects of two novel irrigants on intraradicular dentine erosion, debris and smear layer removal. Restor Dent Endod. (2015) 40(3):216–22. doi: 10.5395/rde.2015.40.3.216
22. Ulusoy Öİ, Görgül G. Effects of different irrigation solutions on root dentine microhardness, smear layer removal and erosion. Aust Endod J. (2013) 39(2):66–72. doi: 10.1111/j.1747-4477.2010.00291.x
23. Azzopardi A, Bartlett DW, Watson TF, Sherriff M. The surface effects of erosion and abrasion on dentine with and without a protective layer. Br Dent J. (2004) 196(6):351–4. doi: 10.1038/sj.bdj.4811083
24. Mancini M, Armellin E, Casaglia A, Cerroni L, Cianconi L. A comparative study of smear layer removal and erosion in apical intraradicular dentine with three irrigating solutions: a scanning electron microscopy evaluation. J Endod. (2009) 35(6):900–3. doi: 10.1016/j.joen.2009.03.052
25. Vanuspong W, Eisenburger M, Addy M. Cervical tooth wear and sensitivity: erosion, softening and rehardening of dentine; effects of pH, time and ultrasonication. J Clin Periodontol. (2002) 29(4):351–7. doi: 10.1034/j.1600-051X.2002.290411.x
26. Viola KS, Coaguila-Llerena H, Rodrigues EM, Santos CS, Chávez-Andrade GM, Magro MG, et al. Different formulations of peracetic acid: effects on smear layer removal, dentine erosion, cytotoxicity and antibiofilm activity. J Appl Oral Sci. (2022) 30. doi: 10.1590/1678-7757-2021-0575
27. West NX, Hughes JA, Addy M. Erosion of dentine and enamel in vitro by dietary acids: the effect of temperature, acid character, concentration and exposure time. J Oral Rehabil. (2000) 27(10):875–80. doi: 10.1046/j.1365-2842.2000.00583.x
28. Hall AF, Buchanan CA, Millett DT, Creanor SL, Strang R, Foye RH. The effect of saliva on enamel and dentine erosion. J Dent. (1999) 27(5):333–9. doi: 10.1016/S0300-5712(98)00067-0
29. West NX, Hughes JA, Addy M. The effect of pH on the erosion of dentine and enamel by dietary acids in vitro. J Oral Rehabil. (2001) 28(9):860–4. doi: 10.1046/j.1365-2842.2001.00778.x
30. Alghilan MA, Cook NB, Platt JA, Eckert GJ, Hara AT. Susceptibility of restorations and adjacent enamel/dentine to erosion under different salivary flow conditions. J Dent. (2015) 43(12):1476–82. doi: 10.1016/j.jdent.2015.10.007
31. Shellis RP, Barbour ME, Jones SB, Addy M. Effects of pH and acid concentration on erosive dissolution of enamel, dentine, and compressed hydroxyapatite. Eur J Oral Sci. (2010) 118(5):475–82. doi: 10.1111/j.1600-0722.2010.00763.x
32. Wetton S, Hughes J, West N, Addy M. Exposure time of enamel and dentine to saliva for protection against erosion: a study in vitro. Caries Res. (2006) 40(3):213–7. doi: 10.1159/000092228
33. Cury JA, Tenuta LMA. Evidence-based recommendation on toothpaste use. Braz Oral Res. (2014) 28:1–7. doi: 10.1590/S1806-83242014.50000001
34. Jones S, Burt BA, Petersen PE, Lennon MA. The effective use of fluorides in public health. Bull W H O. (2005) 83:670–6.16211158
35. Marinho VCC. Evidence-based effectiveness of topical fluorides. Adv Dent Res. (2008) 20(1):3–7. doi: 10.1177/154407370802000102
36. Harrison PT. Fluoride in water: a UK perspective. J Fluorine Chem. (2005) 126(11–12):1448–56. doi: 10.1016/j.jfluchem.2005.09.009
37. Cheng KK, Chalmers I, Sheldon TA. Adding fluoride to water supplies. Br Med J. (2007) 335(7622):699–702. doi: 10.1136/bmj.39318.562951.BE
38. Hughes JA, West NX, Addy M. The protective effect of fluoride treatments against enamel erosion in vitro. J Oral Rehabil. (2004) 31(4):357–63. doi: 10.1046/j.1365-2842.2003.01240.x
39. Lussi A, Buzalaf MAR, Duangthip D, Anttonen V, Ganss C, João-Souza SH, et al. The use of fluoride for the prevention of dental erosion and erosive tooth wear in children and adolescents. Eur Arch Paediatr Dent. (2019) 20(6):517–27. doi: 10.1007/s40368-019-00420-0
40. Amaechi BT, Higham SM. Dental erosion: possible approaches to prevention and control. J Dent. (2005) 33(3):243–52. doi: 10.1016/j.jdent.2004.10.014
41. Office for Health Improvement and Disparities, Department of Health and Social Care, NHS England, and NHS Improvement (2014).
42. Edwards M, Ashwood RA, Littlewood SJ, Brocklebank LM, Fung DE. A video fluoroscopic comparison of straw and cup drinking: the potential influence on dental erosion. Br Dent J. (1998) 185(5):244–9. doi: 10.1038/sj.bdj.4809782
43. Owens BM. The potential effects of pH and buffering capacity on dental erosion. Gen Dent. (2007) 55(6):527–31.18050578
44. Cairns AM, Watson M, Creanor SL, Foye RH. The pH and titratable acidity of a range of diluting drinks and their potential effect on dental erosion. J Dent. (2002) 30(7–8):313–7. doi: 10.1016/S0300-5712(02)00044-1
45. Larsen MJ, Nyvad B. Enamel erosion by some soft drinks and orange juices relative to their pH, buffering effect and contents of calcium phosphate. Caries Res. (1999) 33(1):81–7. doi: 10.1159/000016499
46. Aykut-Yetkiner ARZU, Wiegand A, Bollhalder A, Becker K, Attin T. Effect of acidic solution viscosity on enamel erosion. J Dent Res. (2013) 92(3):289–94. doi: 10.1177/0022034512473115
47. Edwards M, Creanor SL, Foye RH, Gilmour WH. Buffering capacities of soft drinks: the potential influence on dental erosion. J Oral Rehabil. (1999) 26(12):923–7. doi: 10.1046/j.1365-2842.1999.00494.x
48. Shellis RP, Barbour ME, Jesani A, Lussi A. Effects of buffering properties and undissociated acid concentration on dissolution of dental enamel in relation to pH and acid type. Caries Res. (2013) 47(6):601–11. doi: 10.1159/000351641
49. Barbour ME, Shellis RP. An investigation using atomic force microscopy nanoindentation of dental enamel demineralization as a function of undissociated acid concentration and differential buffer capacity. Phys Med Biol. (2007) 52(4):899. doi: 10.1088/0031-9155/52/4/003
50. Featherstone JDB, Lussi A. Understanding the chemistry of dental erosion. In: Lussi A (Bern), editor. Dental erosion. Switzerland: Karger Publishers (2006). Vol. 20. p. 66–76.
51. Lussi A, Jaeggi T, Schärer S. The influence of different factors on in vitro enamel erosion. Caries Res. (1993) 27(5):387–93. doi: 10.1159/000261569
52. Azadi-Schossig P, Becker K, Attin T. Chelating effect of citric acid is negligible for development of enamel erosions. Clin Oral Investig. (2016) 20(7):1577–87. doi: 10.1007/s00784-015-1634-x
53. Fujii M, Kitasako Y, Sadr A, Tagami J. Roughness and pH changes of enamel surface induced by soft drinks in vitro-applications of stylus profilometry, focus variation 3D scanning microscopy and micro pH sensor. Dent Mater J. (2011) 30(3):1105140145. doi: 10.4012/dmj.2010-204
54. Attin T, Meyer K, Hellwig E, Buchalla W, Lennon AM. Effect of mineral supplements to citric acid on enamel erosion. Arch Oral Biol. (2003) 48(11):753–9. doi: 10.1016/S0003-9969(03)00156-0
55. Grenby TH, Phillips A, Desai T, Mistry M. Laboratory studies of the dental properties of soft drinks. Br J Nutr. (1989) 62(2):451–64. doi: 10.1079/BJN19890045
56. Nekrashevych Y, Hannig M, Stosser L. Assessment of enamel erosion and protective effect of salivary pellicle by surface roughness analysis and scanning electron microscopy. Oral Health Prev Dent. (2004) 2(1):5–12.15641759
57. Mullan F, Austin RS, Parkinson CR, Hasan A, Bartlett DW. Measurement of surface roughness changes of unpolished and polished enamel following erosion. PLoS One. (2017) 12(8):e0182406. doi: 10.1371/journal.pone.0182406
58. Zhang XZ, Anderson P, Dowker SEP, Elliott JC. Optical profilometric study of changes in surface roughness of enamel during in vitro demineralization. Caries Res. (2000) 34(2):164–74. doi: 10.1159/000016585
59. Nekrashevych Y, Stösser L. Protective influence of experimentally formed salivary pellicle on enamel erosion. Caries Res. (2003) 37(3):225–31. doi: 10.1159/000070449
60. Azrak B, Callaway A, Kurth P, Willershausen B. Influence of bleaching agents on surface roughness of sound or eroded dental enamel specimens. J Esthet Restor Dent. (2010) 22(6):391–9. doi: 10.1111/j.1708-8240.2010.00372.x
61. Parkinson CR, Shahzad A, Rees GD. Initial stages of enamel erosion: an in situ atomic force microscopy study. J Struct Biol. (2010) 171(3):298–302. doi: 10.1016/j.jsb.2010.04.011
62. Machado C, Lacefield W, Catledge A. Human enamel nanohardness, elastic modulus and surface integrity after beverage contact. Braz Dent J. (2008) 19:68–72. doi: 10.1590/S0103-64402008000100012
63. Elton V, Cooper L, Higham SM, Pender N. Validation of enamel erosion in vitro. J Dent. (2009) 37(5):336–41. doi: 10.1016/j.jdent.2009.01.006
64. Paepegaey AM, Barker ML, Bartlett DW, Mistry M, West NX, Hellin N, et al. Measuring enamel erosion: a comparative study of contact profilometry, non-contact profilometry and confocal laser scanning microscopy. Dent Mater. (2013) 29(12):1265–72. doi: 10.1016/j.dental.2013.09.015
65. Ablal MA, Milosevic A, Preston AJ, Higham SM. A novel approach to study in situ enamel erosion and abrasion lesions. J Dent. (2017) 59:78–85. doi: 10.1016/j.jdent.2017.02.013
66. Wongkhantee S, Patanapiradej V, Maneenut C, Tantbirojn D. Effect of acidic food and drinks on surface hardness of enamel, dentine, and tooth-coloured filling materials. J Dent. (2006) 34(3):214–20. doi: 10.1016/j.jdent.2005.06.003
67. Tantbirojn D, Huang A, Ericson MD, Poolthong S. Change in surface hardness of enamel by a cola drink and a CPP–ACP paste. J Dent. (2008) 36(1):74–9. doi: 10.1016/j.jdent.2007.10.008
68. Devlin H, Bassiouny MA, Boston D. Hardness of enamel exposed to Coca-Cola® and artificial saliva. J Oral Rehabil. (2006) 33(1):26–30. doi: 10.1111/j.1365-2842.2006.01533.x
69. Barbour ME, Parker DM, Allen GC, Jandt KD. Human enamel erosion in constant composition citric acid solutions as a function of degree of saturation with respect to hydroxyapatite. J Oral Rehabil. (2005) 32(1):16–21. doi: 10.1111/j.1365-2842.2004.01365.x
70. Rees JS, Jacobsen PH. The elastic moduli of enamel and dentine. Clin Mater. (1993) 14(1):35–9. doi: 10.1016/0267-6605(93)90045-9
71. Stewart M. A new approach to the use of bearing area curve. Dearborn, MI: Society of Manufacturing Engineers (1990). 1–11.
72. Zhu S, Huang P. Influence mechanism of morphological parameters on tribological behaviors based on bearing ratio curve. Tribol Int. (2017) 109:10–8. doi: 10.1016/j.triboint.2016.12.014
73. Field J, German M, Waterhouse P. Using bearing area parameters to quantify early erosive tooth surface changes in enamel: a pilot study. J Dent. (2013) 41(11):1060–7. doi: 10.1016/j.jdent.2013.08.015
74. Niu W, Yoshioka T, Kobayashi C, Suda H. A scanning electron microscopic study of dentinal erosion by final irrigation with EDTA and NaOCl solutions. Int Endod J. (2002) 35(11):934–9. doi: 10.1046/j.1365-2591.2002.00594.x
75. Teixeira CS, Felippe MCS, Felippe WT. The effect of application time of EDTA and NaOCl on intracanal smear layer removal: an SEM analysis. Int Endod J. (2005) 38(5):285–90.15876291
76. Simezo AP, da Silveira Bueno CE, Cunha RS, Pelegrine RA, Rocha DGP, de Martin AS, et al. Comparative analysis of dentinal erosion after passive ultrasonic irrigation versus irrigation with reciprocating activation: an environmental scanning electron study. J Endod. (2017) 43(1):141–6. doi: 10.1016/j.joen.2016.09.016
77. Haapasalo M, Shen Y, Qian W, Gao Y. Irrigation in endodontics. Dent Clin. (2010) 54(2):291–312. doi: 10.1016/j.cden.2009.12.001
78. Elbahary S, Haj-Yahya S, Khawalid M. Effects of different irrigation protocols on dentin surfaces as revealed through quantitative 3D surface texture analysis. Sci Rep. (2020) 10(1):22073. doi: 10.1038/s41598-020-79003-9
79. de Siqueira FSF, Hilgemberg B, Araujo LCR, Hass V, Bandeca MC, Gomes JC, et al. Improving bonding to eroded dentin by using collagen cross-linking agents: 2 years of water storage. Clin Oral Investig. (2020) 24(2):809–22. doi: 10.1007/s00784-019-02918-9
80. Jaeggi T, Grüninger A, Lussi A. Restorative therapy of erosion. Dental Erosion. (2006) 20:200–14. doi: 10.1159/000093364
81. Forgerini TV, Ribeiro JF, Rocha RDO, Soares FZ, Lenzi TL. Role of etching mode on bonding longevity of a universal adhesive to eroded dentin. J Adhes Dent. (2017) 19(1):69–75. doi: 10.3290/j.jad.a37723
82. Tahmassebi J, Duggal MS, Malik-Kotru G, Curzon MEJ. Soft drinks and dental health: a review of the current literature. J Dent. (2006) 34(1):2–11. doi: 10.1016/j.jdent.2004.11.006
83. Dawes C. What is the critical pH and why does a tooth dissolve in acid? J Can Dent Assoc. (2003) 69(11):722–5.14653937
84. Ranjitkar S, Kaidonis JA, Townsend GC, Vu AM, Richards LC. An in vitro assessment of the effect of load and pH on wear between opposing enamel and dentine surfaces. Arch Oral Biol. (2008) 53(11):1011–6. doi: 10.1016/j.archoralbio.2008.05.013
85. Herkströter FM, Witjes M, Arends J. Demineralization of human dentine compared with enamel in a pH-cycling apparatus with a constant composition during de-and remineralization periods. Caries Res. (1991) 25(5):317–22. doi: 10.1159/000261385
86. Jensdottir T, Holbrook P, Nauntofte B, Buchwald C, Bardow A. Immediate erosive potential of cola drinks and orange juices. J Dent Res. (2006) 85(3):226–30. doi: 10.1177/154405910608500304
87. Jensdottir T, Bardow A, Holbrook P. Properties and modification of soft drinks in relation to their erosive potential in vitro. J Dent. (2005) 33(7):569–75. doi: 10.1016/j.jdent.2004.12.002
88. Johansson AK, Johansson A, Birkhed D, Omar R, Baghdadi S, Khan N, et al. Dental erosion associated with soft-drink consumption in young Saudi men. Acta Odontol Scand. (1997) 55(6):390–7. doi: 10.3109/00016359709059205
89. Jandt KD. Probing the future in functional soft drinks on the nanometre scale—towards tooth friendly soft drinks. Trends Food Sci Technol. (2006) 17(5):263–71. doi: 10.1016/j.tifs.2005.11.016
90. Heurich E, Beyer M, Jandt KD, Reichert J, Herold V, Schnabelrauch M, et al. Quantification of dental erosion—a comparison of stylus profilometry and confocal laser scanning microscopy (CLSM). Dent Mater. (2010) 26(4):326–36. doi: 10.1016/j.dental.2009.12.001
91. Hannig C, Hamkens A, Becker K, Attin R, Attin T. Erosive effects of different acids on bovine enamel: release of calcium and phosphate in vitro. Arch Oral Biol. (2005) 50(6):541–52. doi: 10.1016/j.archoralbio.2004.11.002
92. Kinney JH, Balooch M, Haupt DL Jr, Marshall SJ, Marshall GW Jr. Mineral distribution and dimensional changes in human dentin during demineralization. J Dent Res. (1995) 74(5):1179–84. doi: 10.1177/00220345950740050601
93. Yoshida Y, Van Meerbeek B, Nakayama Y, Yoshioka M, Snauwaert J, Abe Y, et al. Adhesion to and decalcification of hydroxyapatite by carboxylic acids. J Dent Res. (2001) 80(6):1565–9. doi: 10.1177/00220345010800061701
94. Barbour ME, Lussi A, Shellis RP. Screening and prediction of erosive potential. Caries Res. (2011) 45(Suppl. 1):24–32. doi: 10.1159/000325917
95. Buonocore MG. Dissolution rates of enamel and dentin in acid buffers. J Dent Res. (1961) 40(3):561–70. doi: 10.1177/00220345610400032501
96. National Centre for Biotechnology Information. PubChem Compound Summary for CID 311, Citric Acid (2022). Available at: https://pubchem.ncbi.nlm.nih.gov/compound/Citric-Acid (Retrieved November 22, 2022).
97. Marshall GW Jr, Inai N, Magidi ICW, Balooch M, Kinney JH, Tagami J, et al. Dentin demineralization: effects of dentin depth, pH and different acids. Dent Mater. (1997) 13(5–6):338–43. doi: 10.1016/S0109-5641(97)80104-2
98. Marshall SJ, Balooch M, Breunig T, Kinney JH, Tomsia AP, Inai N, et al. Human dentin and the dentin-resin adhesive interface. Acta Mater. (1998) 46(7):2529–39. doi: 10.1016/S1359-6454(98)80037-2
99. Zheng J, Xiao F, Qian LM, Zhou ZR. Erosion behavior of human tooth enamel in citric acid solution. Tribol Int. (2009) 42(11–12):1558–64. doi: 10.1016/j.triboint.2008.12.008
100. Zheng J, Xiao F, Zheng L, Qian LM, Zhou ZR. Erosion behaviors of human tooth enamel at different depth. Tribol Int. (2010) 43(7):1262–7. doi: 10.1016/j.triboint.2009.12.008
101. Cheng ZJ, Wang XM, Cui FZ, Ge J, Yan JX. The enamel softening and loss during early erosion studied by AFM, SEM and nanoindentation. Biomed Mater. (2009) 4(1):015020. doi: 10.1088/1748-6041/4/1/015020
102. Ren YF, Amin A, Malmstrom H. Effects of tooth whitening and orange juice on surface properties of dental enamel. J Dent. (2009) 37(6):424–31. doi: 10.1016/j.jdent.2009.01.011
103. Whitehead SA, Lo LY, Watts DC, Wilson NHF. Changes of surface texture of enamel in vivo. J Oral Rehabil. (1997) 24(6):449–53. doi: 10.1046/j.1365-2842.1997.00517.x
104. Beyer M, Reichert J, Bossert J, Sigusch BW, Watts DC, Jandt KD. Acids with an equivalent taste lead to different erosion of human dental enamel. Dent Mater. (2011) 27(10):1017–23. doi: 10.1016/j.dental.2011.07.001
105. Barbour ME, Parker DM, Allen GC, Jandt KD. Human enamel dissolution in citric acid as a function of pH in the range 2.30 ≤ pH≤6.30–a nanoindentation study. Eur J Oral Sci. (2003) 111(3):258–62. doi: 10.1034/j.1600-0722.2003.00039.x
106. Finke M, Hughes JA, Parker DM, Jandt KD. Mechanical properties of in situ demineralised human enamel measured by AFM nanoindentation. Surf Sci. (2001) 491(3):456–67. doi: 10.1016/S0039-6028(01)01311-5
107. Soares CJ, Pereira JC, Valdivia ADCM, Novais VR, Meneses MS. Influence of resin cement and post configuration on bond strength to root dentine. Int Endod J. (2012) 45(2):136–45. doi: 10.1111/j.1365-2591.2011.01953.x
108. Calt S, Serper A. Time-dependent effects of EDTA on dentin structures. J Endod. (2002) 28(1):17–9. doi: 10.1097/00004770-200201000-00004
109. Torabinejad M, Khademi AA, Babagoli J, Cho Y, Johnson WB, Bozhilov K, et al. A new solution for the removal of the smear layer. J Endod. (2003) 29(3):170–5. doi: 10.1097/00004770-200303000-00002
110. Mjör IA, Nordahl I. The density and branching of dentinal tubules in human teeth. Arch Oral Biol. (1996) 41(5):401–12. doi: 10.1016/0003-9969(96)00008-8
111. Chu CY, Kuo TC, Chang SF, Shyu YC, Lin CP. Comparison of the microstructure of crown and root dentin by a scanning electron microscopic study. J Dent Sci. (2010) 5(1):14–20. doi: 10.1016/S1991-7902(10)60003-7
112. Marshall GW Jr. Dentin: microstructure and characterization. Quintessence Int (Berl). (1993) 24(9):606–17.
113. Sanches RP, Otani C, Damião AJ, Miyakawa W. AFM Characterization of bovine enamel and dentine after acid-etching. Micron. (2009) 40(4):502–6. doi: 10.1016/j.micron.2008.12.001
114. Goel VK, Khera SC, Singh K. Clinical implications of the response of enamel and dentin to masticatory loads. J Prosthet Dent. (1990) 64(4):446–54. doi: 10.1016/0022-3913(90)90041-A
116. De Menezes M, Turssi CP, Hara AT, Messias DCF, Serra MC. Abrasion of eroded root dentine brushed with different toothpastes. Clin Oral Investig. (2004) 8(3):151–5. doi: 10.1007/s00784-004-0262-7
117. Xu C, Wang Y. Chemical composition and structure of peritubular and intertubular human dentine revisited. Arch Oral Biol. (2012) 57(4):383–91. doi: 10.1016/j.archoralbio.2011.09.008
118. Kleter GA, Damen JJM, Everts V, Niehof J, Ten Cate JM. The influence of the organic matrix on demineralization of bovine root dentin in vitro. J Dent Res. (1994) 73(9):1523–9. doi: 10.1177/00220345940730090701
119. Lussi A, Schlüter N, Rakhmatullina E, Ganss C. Dental erosion–an overview with emphasis on chemical and histopathological aspects. Caries Res. (2011) 45(Suppl. 1):2–12. doi: 10.1159/000325915
120. Breschi L, Gobbi P, Mazzotti G, Falconi M, Ellis TH, Stangel I. High resolution SEM evaluation of dentin etched with maleic and citric acid. Dent Mater. (2002) 18(1):26–35. doi: 10.1016/S0109-5641(01)00017-3
121. Berkovitz BK, Holland GR, Moxham BJ. Oral anatomy, histology and embryology E-book. Edinburgh, London, New York, Oxford, Toronto: Elsevier Health Sciences (2017).
123. Driessens FCM, Verbeeck RMH. The probable phase composition of the mineral in sound enamel and dentine. Bull Soc Chim Belg. (1982) 91(7):573–96. doi: 10.1002/bscb.19820910702
124. Theuns HM, Van Dijk JWE, Jongebloed WL, Groeneveld A. The mineral content of human enamel studied by polarizing microscopy, microradiography and scanning electron microscopy. Arch Oral Biol. (1983) 28(9):797–803. doi: 10.1016/0003-9969(83)90035-3
125. Chiego DJ. Essentials of oral histology and embryology E-book: A clinical approach. Michigan, USA: Elsevier Health Sciences (2018).
126. Reyes-Gasga J, Martínez-Piñeiro EL, Bres EF. Crystallographic structure of human tooth enamel by electron microscopy and x-ray diffraction: hexagonal or monoclinic. J Microsc. (2012) 248(1):102–9. doi: 10.1111/j.1365-2818.2012.03653.x
127. Jeng YR, Lin TT, Hsu HM, Chang HJ, Shieh DB. Human enamel rod presents anisotropic nanotribological properties. J Mech Behav Biomed Mater. (2011) 4(4):515–22. doi: 10.1016/j.jmbbm.2010.12.002
128. Habelitz S, Marshall SJ, Marshall GW Jr, Balooch M. Mechanical properties of human dental enamel on the nanometre scale. Arch Oral Biol. (2001) 46(2):173–83. doi: 10.1016/S0003-9969(00)00089-3
129. Featherstone JDB, Mayer I, Driessens FCM, Verbeeck RMH, Heijligers HJM. Synthetic apatites containing na, mg, and CO3 and their comparison with tooth enamel mineral. Calcif Tissue Int. (1983) 35(1):169–71. doi: 10.1007/BF02405026
130. Lussi A, Hellwig E, Zero D, Jaeggi T. Erosive tooth wear: diagnosis, risk factors and prevention. Am J Dent. (2006) 19(6):319.17212071
131. Seow WK, Thong KM. Erosive effects of common beverages on extracted premolar teeth. Aust Dent J. (2005) 50(3):173–8. doi: 10.1111/j.1834-7819.2005.tb00357.x
132. Pattem J, Swift T, Rimmer S, Holmes T, MacNeil S, Shepherd J. Development of a novel micro-bead force spectroscopy approach to measure the ability of a thermo-active polymer to remove bacteria from a corneal model. Sci Rep. (2021) 11(1):1–11. doi: 10.1038/s41598-020-79139-8
133. Pattem J, Davrandi M, Aguayo S, Allan E, Spratt D, Bozec L. A multi-scale biophysical approach to develop structure-property relationships in oral biofilms. Sci Rep. (2018) 8(1):1–10. doi: 10.1038/s41598-018-23798-1
Keywords: tooth erosion, enamel, dentine, in vitro, atomic force microscopy
Citation: Pattem J, Field J, Waterhouse PJ and German MJ (2022) The dynamic interplay of dietary acid pH and concentration during early-stage human enamel and dentine erosion. Front. Dent. Med 3: 1040565. doi: 10.3389/fdmed.2022.1040565
Received: 9 September 2022; Accepted: 28 November 2022;
Published: 15 December 2022.
Edited by:
Nelson Patricio Barrera, Pontificia Universidad Católica de Chile, Chile© 2022 Pattem, Field, Waterhouse and German. This is an open-access article distributed under the terms of the Creative Commons Attribution License (CC BY). The use, distribution or reproduction in other forums is permitted, provided the original author(s) and the copyright owner(s) are credited and that the original publication in this journal is cited, in accordance with accepted academic practice. No use, distribution or reproduction is permitted which does not comply with these terms.
*Correspondence: Jacob Pattem, amFjb2IucGF0dGVtQG5vdHRpbmdoYW0uYWMudWs=
Specialty Section: This article was submitted to Dental Materials, a section of the journal Frontiers in Dental Medicine