- 1Institute for Bioengineering Research, University of Kansas, Lawrence, KS, United States
- 2Department of Mechanical Engineering, University of Kansas, Lawrence, KS, United States
- 3Bioengineering Program, University of Kansas, Lawrence, KS, United States
- 4Department of Civil Engineering, University of Kansas, Lawrence, KS, United States
- 5Department of Molecular Biosciences, University of Kansas, Lawrence, KS, United States
- 6School of Dentistry, Department of Periodontics, University of Missouri-Kansas City, Kansas City, MO, United States
By 2060, nearly 100 million people in the USA will be over the age of 65 years. One-third of these older adults will have root caries, and nearly 80% will have dental erosion. These conditions can cause pain and loss of tooth structure that interfere with eating, speaking, sleeping, and quality of life. Current treatments for root caries and dental erosion have produced unreliable results. For example, the glass-ionomer-cement or composite-resin restorations used to treat these lesions have annual failure rates of 44% and 17%, respectively. These limitations and the pressing need to treat these conditions in the aging population are driving a focus on microinvasive strategies, such as sealants and varnishes. Sealants can inhibit caries on coronal surfaces, but they are ineffective for root caries. For healthy, functionally independent elders, chlorhexidine varnish applied every 3 months inhibits root caries, but this bitter-tasting varnish stains the teeth. Fluoride gel inhibits root caries, but requires prescriptions and daily use, which may not be feasible for some older patients. Silver diamine fluoride can both arrest and inhibit root caries but stains the treated tooth surface black. The limitations of current approaches and high prevalence of root caries and dental erosion in the aging population create an urgent need for microinvasive therapies that can: (a) remineralize damaged dentin; (b) inhibit bacterial activity; and (c) provide durable protection for the root surface. Since cavitated and non-cavitated root lesions are difficult to distinguish, optimal approaches will treat both. This review will explore the multi-factorial elements that contribute to root surface lesions and discuss a multi-pronged strategy to both repair and protect root surfaces. The strategy integrates engineered peptides, novel polymer chemistry, multi-scale structure/property characterization and predictive modeling to develop a durable, microinvasive treatment for root surface lesions.
Introduction
Clinical need
Older people have a significantly increased risk of poor oral health and untreated dental disease (1, 2). Treatment costs are often a substantial barrier to dental care for older adults. Reduced mobility and lack of convenient access to care can add to the challenges of accessing dental treatment. Access to appropriate oral health care can be particularly challenging for older adults who are cognitively impaired or living in long-term care facilities (2). A chronic lack of access to oral health care translates to high levels of untreated dental disease for older adults (1, 2). This problem is expected to grow as more working-age adults transition into retirement and potentially, lose their employer-provided dental insurance (2).
By 2060, the Census Bureau projects the U.S. population will grow to 404 million people, a quarter of whom will be over 65 years. One-third of these older adults will have root caries (3) and nearly 80% will have dental erosion (4). These conditions can cause pain, discomfort and loss of tooth structure that can interfere with eating, speaking, sleeping, smiling, socializing and quality of life.
The increased risk of dental erosion and root caries in older adults has been linked to several factors including gingival recession. Gingival recession becomes more prevalent with age, increasing tooth-root exposure and the risk of irreversible damage to the tooth structure (5–7). Tooth-root exposure means that dentin and cementum are exposed to the oral environment. Cementum is sufficiently porous to allow diffusion of acids and enzymes derived from saliva, gingival crevicular fluid and bacteria (8). Dentin is also a porous composite material and loss of mineral as a result of acid and enzyme exposure increases the porosity (9). Over time, acidic foods, beverages, occlusal stress, and abrasion lead to erosion of the exposed tooth-root.
The root caries process starts when acid is released by bacteria causes mineral to dissolve (9) and the composition of dentin and cementum increases the risk of mineral dissolution. As opposed to enamel with a composition (by weight) of more than 96% inorganic and 4% organic, the inorganic phase in dentin and cementum is much lower. Dentin is 65% inorganic minerals, 35% organic matrix and water while cementum, the outer mineral tissue layer of tooth-root, is 50%–55% inorganic, 45%–50% organic and water (10). Type I collagen constitutes about 90% of the organic phase for both dentin and cementum (10). If demineralization is not intercepted or stymied by remineralization at the early stages of the caries process, destruction of the inorganic and organic phases will progress leading to cavitation (10).
Reduced salivary flow or altered salivary composition can increase caries risk, and numerous diseases common in aging populations, such as hypertension, diabetes, Alzheimer's disease, Parkinson's disease, stroke, and rheumatoid arthritis carry an attendant risk of salivary dysfunction. In addition, a wide variety of medications, including antihypertensives, antidepressants, tranquilizers, diuretics, and antihistamines, also contribute to salivary dysfunction.
Targeted microorganisms
Microorganisms in the oral cavity exist mainly as biofilms on saliva-coated surfaces such as teeth and restorative materials. Adhesion of primary microorganisms to such surfaces is a key interaction in the initiation of biofilm development. This initial colonization involves interaction of bacterial cell-surface proteins with dental pellicle, a 0.1–1.0 µm thick acellular layer rich in mucinous glycoproteins (11).
Streptococcus mutans, a Gram-positive, facultatively anaerobic microorganism, is among the first colonizers of the pellicle. The adhesion of this “pioneer” organism creates an environment that promotes binding of other oral bacteria (12, 13). Ultimately, these activities lead to the formation of a micro-ecosystem (biofilm). S. mutans is a primary causative agent of dental caries—higher counts of Streptococcus mutans or lactobacilli species are noted with higher prevalence of root caries (7). S. mutans is both a “pioneer” organism in biofilm formation and produces acids and enzymes that damage the tooth.
In addition to the high prevalence of root caries and dental erosion, nearly 60% of the population 65 years and older will have periodontal disease (14). Porphyromonas gingivalis, a Gram-negative anaerobic microorganism, plays a prominent role in inflammatory periodontal disease such as periodontitis (15). Periodontitis is characterized by destruction of the periodontal ligament, and alveolar bone. P. gingivalis can attach to a variety of substrates in the oral cavity including synthetic materials, soft tissues and other bacteria (16). Furthermore, P. gingivalis expresses virulence factors that allow it to evade host responses and to colonize and spread within the tissues (17). It is theorized that periodontal inflammation results from the interaction of the host immune system and a dysbiotic subgingival biofilm (8). Dysbiosis potentially results from the interaction of “keystone” organisms such as Porphyromonas gingivalis and Filifactor alocis with subgingival pathogenic microbes (8).
Quorum sensing
Many bacteria communicate with one another using chemical signaling molecules that may be beneficial in mixed microbial communities (18). A particularly well understood type of cell-cell communication is quorum sensing. Quorum-sensing systems become activated at a critical density or “quorum” and cause changes in gene expression that switch on certain group activities. For example, P. gingivalis uses the AI-2 quorum-sensing signal to switch on biofilm formation. AI-2 signals are synthesized by the LuxS signal synthase, which cleaves S-ribosylhomocysteine into 4,5-dihydroxy-2,3-pentanedione (DPD), which further derivatizes to become AI-2 (19). Many different bacteria have LuxS enzymes and can produce AI-2, thus AI-2 may be important for coordinating interactions between different species. In P. gingivalis, AI-2 is necessary for the formation of mixed biofilms with other species such as Streptococcus gordonii (20). S. mutans also encodes a luxS AI-2 synthase gene that is important for biofilm formation (21). Thus AI-2 might be an essential cell-cell communication molecule that is used for coordinating the formation of mixed biofilms in the oral cavity.
In addition to AI-2, S. mutans encodes a second quorum sensing system that relies on a signal called competence stimulating peptide (CSF) (22). Unlike AI-2, which is found in many species, CSF is found only in S. mutans. CSF is a post-translationally modified 21 amino acid peptide that is encoded by the comC gene. Other genes involved in CSF signaling are comAB, which codes for the CSF secretion apparatus, and comDE, which codes for the membrane-localized CSF receptor and cognate response regulator, respectively. At a sufficient population density CSF signaling induces natural competence, which is the uptake of DNA from the environment. Natural competence may be important for acquiring DNA that has been released from nearby bacteria, such as antibiotic resistance genes that might be beneficial in certain conditions. CSF has also been shown to regulate other behaviors, such as production of antimicrobials. Thus, CSF is another type of quorum-sensing system that may be important for competing with other strains or species in polymicrobial communities.
Quorum sensing has been the target of much effort to develop novel therapeutics that function by blocking critical cell-cell interactions rather than essential functions that are targeted by classic antibiotic approaches. These types of “anti-virulence” therapeutics are novel in concept because they may block destructive properties of the microbes (e.g., establishment of caries infections) but do not block essential processes that are needed to survive. It is thought that such anti-virulence therapeutics might escape the normal selective pressures that quickly lead to resistance in the case of classic antibiotic therapies, and ultimately be a more effective treatment strategy. However, basic studies of such therapeutics are lacking, particularly studies in the context of more complex multi-species and multi-strain communities, and such studies are needed to demonstrate their efficacy and determine the best strategies for treating infections.
Non-cavitated and cavitated lesions on root surfaces
Cavitated and non-cavitated root carious lesions are difficult to distinguish in practice (23). In general, non-cavitated carious lesions can be described as surfaces that appear macroscopically intact. A cavitated carious lesion presents as a surface with a distinct discontinuity or break in the surface integrity (23).
Non-carious root lesions may be caused by erosion, abrasion and/or occlusal stress (24). (Figure 1: Clinical Image) Restoration of non-carious root lesions may be required to relieve hypersensitivity, to prevent further loss of tooth structure, and to improve esthetics (24, 25).
Root caries is a cavitation occurring below the cementoenamel junction that involves both cementum and dentin but not enamel (7). (Figure 2: Clinical Image) These lesions are insidious—overhanging enamel leads to surfaces that are not readily accessible for cleaning. The overhanging enamel provides a niche that collects and retains food particles, e.g., fermentable carbohydrates—bacteria will thrive in these nutrient-rich environments. Dentin and cementum will be exposed to low-pH biofilm for extended periods (26, 27). As noted recently, the following factors are associated with an increased risk of root cares: older people, lower socioeconomic status, gingival recession, tooth root exposure, tobacco use and poor oral hygiene (7). Elderly patients with compromised functional capabilities or in settings where regular dental care is not possible are especially susceptible to root caries.
Current treatments
Root-surface lesions are more challenging to treat than those at the coronal surface. The efficacy of glass-ionomer-cement or composite-resin restorations is hampered by moisture-control difficulties and the non-retentive saucer-shaped cavity preparations that are often required to conserve tooth-root structure (26, 27). The restoration will generally be bonded to dentin and/or cementum—dentin and cementum are particularly challenging substrates for bonding (24). For example, in non-carious cervical lesions a large fraction of the substrate may be sclerotic dentin—sclerotic dentin is resistant to acid-etching which is commonly used with adhesive bonding. Inadequate bonding leads to a fragile adhesive seal—a seal that is readily damaged by acids, enzymes, and oral fluids.
Inadequate bonding, non-retentive saucer-shaped cavity preparations and moisture contamination contribute to the high failure rates of root-surface glass-ionomer-cement or composite-resin restorations—annual failure rates are 44% and 17%, respectively (26). The repair of cervical lesions with proximal extensions is exceptionally challenging—the repair of these lesions requires extensive removal of sound tooth structure (25). The longevity of these restorations is significantly shortened (25). The failures lead to a vicious cycle of repeated restorations, with each cycle weakening the tooth and increasing the risk of endodontic treatment or extraction.
Given the prevalence of root-surface lesions in the aging population, these limitations are driving a focus on microinvasive strategies to arrest or reverse the carious lesions. Fluoride gel (5,000 parts-per-million) inhibits root caries, but success requires filling prescriptions and daily use, which may not be feasible for some older patients (23, 28). For healthy, functionally independent elders, chlorhexidine varnish applied every 3 months inhibits root caries, but this varnish stains the teeth brown and its bitter taste discourages regimen compliance (28). Silver diamine fluoride (SDF) can arrest and inhibit root caries, but stains the teeth black (23, 28).
Next generation treatments: peptide-polymer hybrids
There is increasing interest in approaches that integrate biologically instructive roles into dental biomaterials—the ultimate goal of these approaches is to prevent oral disease and/or restore oral health (29–31). Biomolecules are essential in physiological processes, and smaller molecules such as peptides offer the potential to mimic their function while also, serving as uniquely versatile molecular tools to couple biologically instructive roles in material systems (32, 33). Our group as well as others have explored peptide-based approaches to repair mineralized dental tissues damaged by caries, trauma or periodontal diseases and derivatized dental materials with a wide range of bioactivities including antimicrobial activity to prevent infection (29, 34–38).
Our initial efforts focused on mineralization as next generation treatment options for dental biomaterials. Dental enamel is the hardest and most highly mineralized tissue—enamel can withstand 770 N and nearly one million cycles per year (29). Enamel biomineralization has been an inspiration for scientists to understand the amelogenesis, i.e., enamel formation process and to replicate this intricate biological process. Amelogenin protein, a major constituent of the developing enamel matrix, plays a critical role in the oriented growth of enamel mineral (39–41). There have been several studies to correlate the functional domains of amelogenin and utilize this knowledge to remineralize and restore tooth structure using biomolecules as a biomimetic guide. To develop amelogenin derived functional peptides, we developed a bioinformatics scoring matrix approach to identify short amino acid similarity regions across the full length amelogenin protein. In this search, we used our combinatorial phage display library of selected calcium phosphate binding peptides (42). In our prior research, we demonstrated that these peptides can mediate remineralization function and remineralize a cementum-like hydroxyapatite mineral layer on demineralized root dentin (34, 43).
Another active area of peptide incorporation in the dental biomaterials focuses on antimicrobial peptides (AMPs) to prevent infection. With the rapidly proliferating concern on antibiotic resistant strains, the non-antibiotic-based approaches are an active area of research. AMPs gained significant attention as small molecules that are an integral part of host defense systems found among all life forms. These small peptides have excellent antibacterial, antibiofilm properties, and low bacterial strain resistance. We engineered biomaterial surfaces with AMPs and developed localized delivery approaches for their easy deployment on biomaterials including dental implants (44, 45). We developed machine learning approaches to classify antimicrobial peptides and enrich sequence domains for effective antimicrobial search with enhanced properties (46, 47).
Resin-based composite restorations are among the most commonly applied restorative materials used to treat defective tooth tissues. With their improved formulations, composites have become increasingly popular in dentistry, however they suffer from recurrent dental caries occurring at the margin between the composite and the tooth. The cariogenic bacteria, S. mutans, adheres to the tooth/adhesive/composite interface and creates a microenvironment promoting the subsequent attachment and growth of bacteria leading to biofilm formation. Acidic environment produced by the bacterial activity demineralizes the tooth surface and erodes the dental adhesive resulting in enlargements of the gaps between the tooth and the composite. Despite dental adhesive polymers possessing broad and versatile properties, they lack biological functionalities such as remineralization and antimicrobial. Building on our prior research (46–55), we developed a multi-pronged strategy to both repair and protect exposed dentin (50). This multi-pronged strategy: (a) remineralizes damaged dentin; (b) inhibits bacterial attack; and (c) provides durable protection. Since cavitated and non-cavitated root lesions are difficult to distinguish, our multi-pronged, microinvasive strategy will be suitable for treating both lesion types.
Polymer-peptide conjugates are generally hybrid soft materials, which are designed to achieve synergistic behavior of both components. There have been several studies on hydrogel-based materials, however low mechanical properties and rapid erosion of these conjugates inhibit their application as dental restorative materials. Our recent investigations have led to a synergistic approach to design a peptide-polymer hybrid system. Our strategy includes polymer-tethered peptides to promote remineralization, polymer-tethered antimicrobial peptides to inhibit S mutans activity, and novel self-strengthening polymers (Figure 3). The alkoxysilane-containing polymers achieve self-strengthening, i.e., intrinsic network reinforcement in both neutral and acidic conditions (56, 57). The intrinsic network reinforcement leads to enhanced mechanical properties and hydrolytic stability (56–59). The tethered peptides present bioactive cues to inhibit bacterial activity and promote remineralization at the lesion site (50) (Figure 4).
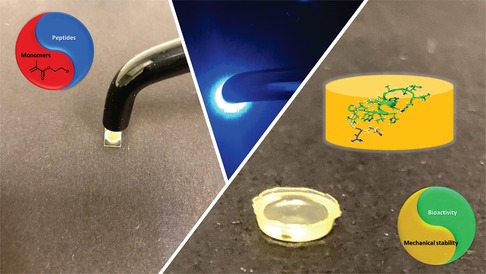
Figure 4. Formulation polymerized with dental curing light to produce polymer with tethered peptides to provide bioactive cues to inhibit bacterial activity and promote remineralization.
Peptide-mediated remineralization
Our prior art was built upon incorporating a phage-display-selected hydroxyapatite binding peptide (HABP: CMLPHHGAC) (42). Among the several HABPs identified using the combinatorial library selection, this peptide was shown to bind and simultaneously exhibit control over calcium phosphate mineralization. We demonstrated that this peptide also binds to dentin and mediates the remineralization at the dentin-adhesive interface (53). We also explored our amelogenin derived peptides to provide treatment at the lesion site and demonstrated that peptide-mediated remineralization diffuses into the substrates and can be tuned for increased nucleation sites (55). The repertoire of calcium-phosphate-binding peptides that we developed allows us to develop peptide-polymer hybrids that can be tuned to promote remineralization (Figure 5) of the damaged dentin with different kinetics and morphology as well as availability at the lesion sites (50).
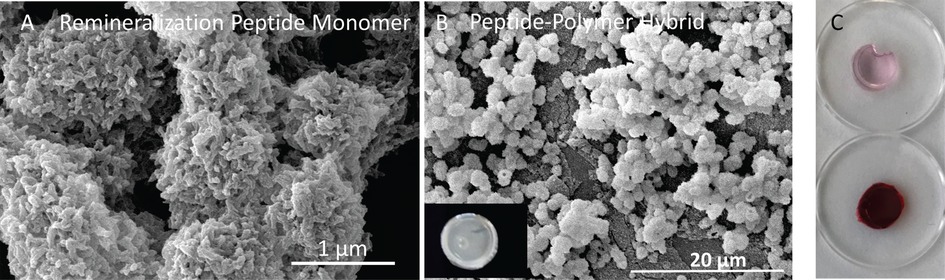
Figure 5. (A) SEM images of the minerals formed in the presence of K-GSGGG-HABP monomer; (B) K-HABP:AMPM7 integrated polymer disc samples was monitored under SEM after completing the overnight mineralization reaction; (C) peptide integrated polymer discs after mineralization were stained with alizarin red. The same type of discs without mineralization were used as controls.
Antibacterial activity
Agents such as chlorhexidine (CHX), fluoride, and quaternary ammonium salts (QAMs) have been incorporated in dental materials to prevent caries (60–63). The antimicrobial activity of these agents is generally achieved through gradual release—gradual release can lead to inconsistent dosage and short-term effectiveness. In addition, these agents can discolor the tooth, interfere with taste (64–66), drive development of bacterial resistance (67, 68), and display toxicity to host tissues (60, 62, 64). Our approach of incorporating antimicrobial peptides (AMPs) offers a therapeutic alternative that can be delivered at the site as an integral part of the polymeric network (50–52, 69, 70). AMPs provide early-stage protection and also bridge between innate and acquired immunity (71). Despite these advances, the vast potential of AMPs is quite limited — the limitation is related, in part, to the concerns associated with their systemic delivery which requires high concentrations and raises toxicity concerns. Our approach provides an alternative delivery strategy to deploy peptides on the sites and increases their availability and preserves their antibacterial efficacy.
Dual peptide-polymer hybrid
We have developed a dual peptide-polymer hybrid with tunable properties (50). The peptide-polymer hybrid was produced by mixing two peptides, i.e., an antimicrobial peptide and mineralization mediator peptide, tethering them to monomers and co-polymerizing the peptide-tethered monomers. In brief, HABP and AMP were synthesized using oligomeric spacers to tether them to a methacrylic acid (MA) as peptide-monomers. The sequences for the peptide-monomers are: MA-K-GSGGG-CMLPHHGAC and MA-K-GGG-KWKRWWWWR-NH2 for HABP and AMPM7, respectively (50). The peptides become an integral part of the polymer and their antimicrobial and remineralizing properties are displayed simultaneously. The tethered peptides remineralize the damaged tooth structure and inhibit bacterial activity, i.e., S. mutans, while the polymer provides durable protection to the prepared tooth surface.
Integrated experimental & computational approaches for rational treatment design
The durability of treatments for root surface lesions, and in general, other treatments for dental disease and/or trauma are impacted by a complex bio-chemo-mechanical environment. This complexity is manifold at the interface between the treatments and the native tooth structure.
Spatial scales
The treatments are characterized by a complex system of different material components—the material components have varying mechanical properties and are arranged in a variety of morphologies. The mechano-morphological properties of these material components can be considered at different spatial and temporal scales. From the viewpoint of spatial scales, material systems can be broadly considered at:
(1) The macro-scale defined at the millimeter level spanning 0.1 mm–10 mm. At this scale, the interfaces between native materials and the treatment appears as a thin layer with variable mechano-morphology.
(2) The micro-scale defined at the micrometer level spanning 0.1 µm–100 µm. At this scale, the interfaces between native materials and the treatment appears as a complex mechano-morphology construct. Such a micro-mechano-morphology is characterized by ill-defined structure-property relationships, including heterogeneity and/or phase separations, inter-digitation, defects, presence of pore fluid, and others.
(3) The atomic-scales defined at the nanometer level spanning 0.1 nm–100 nm. The mechano-morphology at this scale can be surmised to consist of a variety of covalent, ionic and hydrogen bonds among the multi-atomic components, i.e., the native apatitic mineralite, collagen and the introduced treatment materials.
Temporal scales
From the viewpoint of temporal scales, the complex mechano-morphological composition and the dynamic external loading produced at the occlusal surfaces indicates that the behavior at the interface between the treatment and native structure is both rate- and time-dependent. Thus, the durability and long-term performance of the treated root surface lesions depends upon the rate-dependence (visco-elastic-damage-plastic) behavior as well as the time-related bio-chemo-mechanical transformations of the various material components.
Clearly, the characterization and modeling of treatment constructs pose significant challenges. These challenges are not only for the laboratory analytical techniques, but also for mathematical modeling necessary for informing the design of effective treatments. A practical way to understand the impact of the complex mechano-morphologies at the different spatial and temporal scales is to develop integrated experimental and modeling approaches. For example, we have discussed the spatial scales issues for dentin-adhesive (d-a) interfaces using macro- and micro-scale elastic models and the temporal issues using micro-scale visco-elastic-damage-plastic models [see discussion in (72)]. Similarly, experimental techniques at appropriate scales may be used to characterize mechano-morphology properties of the native materials as discussed in the following references (73–77).
Indeed, appropriate mathematical models can be a significant component of the iterative scheme for developing effective treatments. Along this line, we have been developing mathematical models of bio-chemo-mechanical behavior of adhesives and their performances at the d-a interface. For tractable analyses of this highly complex multi-scalar construct, we have developed methods applicable at different spatial-scales, time- and rate- dependency. We have applied this approach to connect molecular-level data to model time- and rate-dependent stress/strain relationships in adhesives, as well as in adhesive-collagen constructs such as hybrid-layer mimics (58, 59, 73–75, 78, 79).
The models exploit recent developments in continuum physics that aim to connect mechanisms at nano- and micro-scales to larger scales (80–83). These models aid the rational design of our adhesive formulation by predicting their constitutive behaviors under relevant oral conditions (73–75, 78, 79, 84–86). Further, using µ-scale structure-property measurements (87) we have developed 3D Finite Element (µFE) models (87–90). The µFE models can be used to assess how the change in adhesive properties effects the performance of the d-a interface by directly utilizing the predicted constitutive behaviors.
The stress analysis at macro-scales (tooth scale) can only assess occlusal-loading stresses at the d-a interface, while micro-scale stress analysis identifies the locations where stresses concentrate. The (µFE) models have been used to perform micromechanical stress analyses (88–90). These analyses show that the different material phases at the d-a interface experience different stress amplitudes under functional load (88, 89). As a result, the different material phases reach their failure strengths at different overall stress levels. Thus, the overall failure behavior of the d-a interface is not necessarily determined by the weakest component—instead, failure is determined by the component whose stress concentration is closest to its failure strength (90).
We have also used µmechanical stress-analysis (Figure 6) to show the effects of such stress concentrations on the mechanisms that govern overall fatigue failure behavior of the d-a interface (82). Predictions were compared to experimental data to illustrate the predictive power of our methodology (91). The modeling is used to predict properties and forecast behavior of the adhesive under conditions relevant to function in the mouth. Feedback from the modeling informs refinement of the polymer formulations and promotes targeted design optimization of the adhesive.
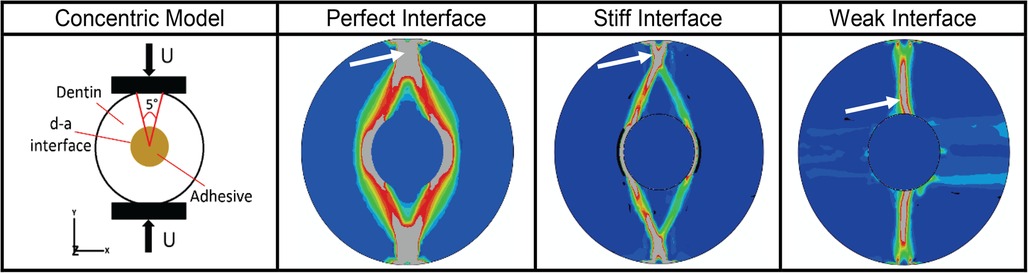
Figure 6. Results from micromechanical stress analysis as of d-a interface for different types of potential interfacial conditions based upon finite element in-silico analyses of a concentric model of d-a interface formed by creating a hole in a cylindrical dentin sample. Panel 1 shows the schematic of the concentric model and its external loading regime. Panels 2 to 4 give the octahedral shear strain (blue-low, red-high) at post failure showing fracture zone (gray – indicated by white arrow) for three ideal cases of d-a interfacial conditions. For perfect and stiff interfaces, respectively, the fracture initiates from the load application zone on the sample periphery and propagates towards the interface, while for a weak interface, the fracture initiates from inside at the d-a interface and propagates outwards towards the loading location.
Feasible integrated, iterative experimental and mathematical modelling approaches are required to develop a comprehensive understanding of how treatments perform in function. For example, to address the issues related to spatial scales by using independent macro- and micro-scale models as well as temporal issues by developing rate-dependent models—these models as well as experimental validation are necessary for a comprehensive understanding. The current efforts, which are typically based upon classical elastic continuum mechanics or experimental approaches based upon the classical theories are inadequate. Refined modeling and experiments, that aim to bridge the response at various scales such as the micromechanically based models that have the capability of connecting the micro-scale mechanisms to the macro-scale phenomena (82, 85, 92, 93) can advance our understanding of the effectiveness of proposed treatments.
Summary
By 2060, 100 million US citizens will be over 65 and one-third of these older adults will have root caries and nearly 80% will have dental erosion—conditions that cause pain and loss of tooth structure that can interfere with eating, speaking, sleeping and quality of life. Several factors lead to older adults' significantly increased risk of root caries and dental erosion. Gingival recession becomes more prevalent with age, increasing tooth-root exposure and the risk of irreversible damage to the tooth. Reduced salivary flow or altered salivary composition can increase caries risk, and numerous diseases as well as medications common in aging populations contribute to salivary dysfunction.
Current restorative treatments for root caries and dental erosion have produced unreliable results such as high annual failure rates. These limitations and the pressing need to treat these conditions in the aging population are driving a focus on microinvasive strategies, such as sealants, varnishes, and gels. Sealants are effective for coronal caries, but ineffective for root caries. The frequent application required for chlorhexidine varnish and fluoride gels may not be feasible for elders with compromised functional capabilities or individuals living in settings where regular dental care is not possible. The bitter-tasting chlorhexidine varnish must be applied every 3 months. Fluoride gels require prescriptions and daily use. Silver diamine fluoride can both arrest and inhibit root caries but stains the treated tooth surface black.
The limitations of current approaches as well as the high prevalence of root caries and dental erosion in the aging population create an urgent need for alternative treatments. Peptide-polymer hybrids offer a multi-pronged, microinvasive strategy for repairing and protecting exposed tooth-root surfaces. This multi-pronged strategy includes: (a) polymer-tethered peptides to promote remineralization of damaged dentin; (b) polymer-tethered antimicrobial peptides to inhibit S mutans; and (c) self-strengthening polymers to provide durable protection. The tethered peptides present bioactive cues to promote remineralization and inhibit bacterial activity at the lesion site without staining the treated surface black. The polymers offer hydrolytic stability and enhanced mechanical properties under both neutral and acidic conditions.
While the peptide-polymer hybrids offer promise, the design and production of these materials pose significant challenges. For example, the properties of polymers depend on a large parameter space and optimization of these properties is typically a laborious, iterative process. Features such as composition, polymerization, and processing parameters are systematically changed and the effects determined by measuring the properties of the new polymer. This process will only become more intractable as additional biological parameters are included. One approach to these challenges is multi-scale characterization coupled with modeling to provide insights beyond what could be accomplished if either of the approaches were applied independently. With their ability to enable in silico parameter optimization, computational models to correlate system parameters with material properties can be indispensable for streamlining this process.
Finally, there are multiple unresolved questions and challenges that must be addressed to meet the unprecedented demand for new restorative treatments for root caries and dental erosion. These questions and challenges include a reliable, reproducible, and quantitative understanding of the substrate at atomic, molecular and macro-scales. Diagnostic tools that can assess the extent of damaged dentin in vivo. Laboratory results must be validated by representative and reproducible in vivo models (94). In vitro models that predict degradation must be calibrated with clinical data (94). Advanced imaging modalities that can reveal subtle compositional differences that occur in the substrate, i.e., dentin and cementum, and restorative material during clinical function are required (94, 95). Multiscale imaging, advanced technologies, and computational modeling are required to measure the interactions of materials with the tissue microenvironment (96).
Contribution to the field statement
By 2060, nearly 100 million people in the USA will be over age 65 years. Nearly 30 million of these older adults will have root caries and about 80 million will have dental erosion. These conditions can cause pain and loss of tooth structure that can interfere with eating, speaking, sleeping, and quality of life. Several factors lead to older adults significantly increased risk of root caries and dental erosion. Gingival recession becomes more prevalent with age, increasing tooth-root exposure and the risk of irreversible damage to the tooth. Altered salivary composition or reduced salivary flow can increase caries risk; numerous diseases and medications common in aging populations carry an attendant risk of salivary dysfunction. Elderly patients in settings where regular dental care is not possible are especially susceptible to root caries. Current treatments for root caries and dental erosion have produced unreliable results—these limitations and the pressing need to treat these conditions in the aging population are driving a focus on microinvasive strategies. Drawing on discoveries in peptide engineering, polymer chemistry, and predictive modeling, we propose a multi-pronged strategy to remineralize damaged dentin, inhibit bacterial activity and provide durable protection for root surface lesions.
Author contributions
PS, contributed to conception, design, data analysis, and interpretation and drafted and critically revised the manuscript QY contributed to conception, data acquisition, analysis, and interpretation and critically revised the manuscript; AM contributed to conception, design, data analysis, and interpretation and critically revised the manuscript; JRC contributed to conception and design and critically revised the manuscript; CMC contributed to conception and design and critically revised the manuscript; CT contributed to conception and design, data analysis, and interpretation, critically revised the manuscript. All authors contributed to the article and approved the submitted version.
Funding
Research reported in this publication was supported by the National Institute of Dental & Craniofacial Research of the National Institutes of Health under Award Number R01DE025476.
Acknowledgments
The content is solely the responsibility of the authors and does not necessarily represent the official views of the National Institutes of Health. The research was also supported, in part, by a Research GO award from the University of Kansas.
Conflict of interest
The authors declare that the research was conducted in the absence of any commercial or financial relationships that could be construed as a potential conflict of interest.
Publisher's note
All claims expressed in this article are solely those of the authors and do not necessarily represent those of their affiliated organizations, or those of the publisher, the editors and the reviewers. Any product that may be evaluated in this article, or claim that may be made by its manufacturer, is not guaranteed or endorsed by the publisher.
References
1. Peres MA, Macpherson LMD, Weyant RJ, Daly B, Venturelli R, Mathur MR, et al. Oral diseases: a global public health challenge. Lancet. (2019) 394(10194):249–60. doi: 10.1016/S0140-6736(19)31146-8
2. National Institutes of Health. Oral health in America: Advances and challenges. Bethesda, MD: US Department of Health and Human Services, National Institutes of Health, National Institute of Dental and Craniofacial Research (2021).
3. Griffin SO, Griffin PM, Swann JL, Zlobin N. Estimating rates of new root caries in older adults. J Dent Res. (2004) 83(8):634–8. doi: 10.1177/154405910408300810
4. Okunseri C, Wong MCM, Yau DTW, McGrath C, Szabo A. The relationship between consumption of beverages and tooth wear among adults in the United States. J Public Health Dent. (2015) 75(4):274–81. doi: 10.1111/jphd.12096
5. Wierichs RJ, Meyer-Lueckel H. Systematic review on noninvasive treatment of root caries lesions. J Dent Res. (2015) 94(2):261–71. doi: 10.1177/0022034514557330
6. Gostemeyer G, Schulze F, Paris S, Schwendicke F. Arrest of root carious lesions via sodium fluoride, chlorhexidine and silver diamine fluoride in vitro. Materials (Basel). (2017) 11(1):9. doi: 10.3390/ma11010009
7. Zhang J, Sardana D, Wong MCM, Leung KCM, Lo ECM. Factors associated with dental root caries: a systematic review. JDR Clin Trans Res. (2020) 5(1):13–29. doi: 10.1177/2380084419849045
8. Cobb CM, Sottosanti JS. A re-evaluation of scaling and root planing. J Periodontol. (2021) 92(10):1370–8. doi: 10.1002/JPER.20-0839
9. Cai J, Burrow MF, Manton DJ, Tsuda Y, Sobh EG, Palamara JEA. Effects of silver diamine fluoride/potassium iodide on artificial root caries lesions with adjunctive application of proanthocyanidin. Acta Biomater. (2019) 88:491–502. doi: 10.1016/j.actbio.2019.02.020
10. El Gezawi M, Wolfle UC, Haridy R, Fliefel R, Kaisarly D. Remineralization, regeneration, and repair of natural tooth structure: influences on the future of restorative dentistry practice. ACS Biomater Sci Eng. (2019) 5(10):4899–919. doi: 10.1021/acsbiomaterials.9b00591
11. Liljemark WF, Bloomquist C. Human oral microbial ecology and dental caries and periodontal diseases. Crit Rev Oral Biol Med. (1996) 7(2):180–98. doi: 10.1177/10454411960070020601
12. Rickard AH, Gilbert P, High NJ, Kolenbrander PE, Handley PS. Bacterial coaggregation: an integral process in the development of multi-species biofilms. Trends Microbiol. (2003) 11(2):94–100. doi: 10.1016/s0966-842x(02)00034-3
13. Kim D, Barraza JP, Arthur RA, Hara A, Lewis K, Liu Y, et al. Spatial mapping of polymicrobial communities reveals a precise biogeography associated with human dental caries. Proc Natl Acad Sci U S A. (2020) 117(22):12375–86. doi: 10.1073/pnas.1919099117
14. Fellows JL, Atchison KA, Chaffin J, Chavez EM, Tinanoff N. Oral health in America: implications for dental practice. J Am Dent Assoc. (2022) 153(7):601–9. doi: 10.1016/j.adaj.2022.04.002
15. Oliveira RR, Fermiano D, Feres M, Figueiredo LC, Teles FR, Soares GM, et al. Levels of candidate periodontal pathogens in subgingival biofilm. J Dent Res. (2016) 95(6):711–8. doi: 10.1177/0022034516634619
16. Gerits E, Verstraeten N, Michiels J. New approaches to combat Porphyromonas Gingivalis biofilms. J Oral Microbiol. (2017) 9(1):1300366. doi: 10.1080/20002297.2017.1300366
17. Romero-Lastra P, Sanchez MPAC, Llama-Palacios A, Figuero E, Herrera D, Sanz M. Gene expression of Porphyromonas Gingivalis atcc 33277 when growing in an in vitro multispecies biofilm. Plos One. (2019) 14(8):e0221234. doi: 10.1371/journal.pone.0221234
18. Abisado RG, Benomar S, Klaus JR, Dandekar AA, Chandler JR. Bacterial quorum sensing and microbial community interactions. mBio. (2018) 9(3):e02331–17. doi: 10.1128/mBio.02331-17
19. Schauder S, Shokat K, Surette MG, Bassler BL. The luxs family of bacterial autoinducers: biosynthesis of a novel quorum-sensing signal molecule. Mol Microbiol. (2001) 41(2):463–76. doi: 10.1046/j.1365-2958.2001.02532.x
20. McNab R, Ford SK, El-Sabaeny A, Barbieri B, Cook GS, Lamont RJ. Luxs-Based signaling in Streptococcus Gordonii: autoinducer 2 controls carbohydrate metabolism and biofilm formation with Porphyromonas Gingivalis. J Bacteriol. (2003) 185(1):274–84. doi: 10.1128/JB.185.1.274-284.2003
21. Yoshida A, Ansai T, Takehara T, Kuramitsu HK. Luxs-Based signaling affects Streptococcus Mutans biofilm formation. Appl Environ Microbiol. (2005) 71(5):2372–80. doi: 10.1128/AEM.71.5.2372-2380.2005
22. Li YH, Lau PC, Lee JH, Ellen RP, Cvitkovitch DG. Natural genetic transformation of Streptococcus Mutans growing in biofilms. J Bacteriol. (2001) 183(3):897–908. doi: 10.1128/JB.183.3.897-908.2001
23. Slayton RL, Urquhart O, Araujo MWB, Fontana M, Guzman-Armstrong S, Nascimento MM, et al. Evidence-based clinical practice guideline on nonrestorative treatments for carious lesions: a report from the American dental association. J Am Dent Assoc. (2018) 149(10):837–49. e19. doi: doi: 10.1016/j.adaj.2018.07.002
24. Kubo S, Yokota H, Yokota H, Hayashi Y. Challenges to the clinical placement and evaluation of adhesively-bonded, cervical composite restorations. Dent Mater. (2013) 29(1):10–27. doi: 10.1016/j.dental.2012.08.003
25. Wierichs RJ, Kramer EJ, Meyer-Lueckel H. Risk factors for failure of class V restorations of carious cervical lesions in general dental practices. J Dent. (2018) 77:87–92. doi: 10.1016/j.jdent.2018.07.013
26. Schwendicke F, Gostemeyer G. Cost-effectiveness of root caries preventive treatments. J Dent. (2017) 56:58–64. doi: 10.1016/j.jdent.2016.10.016
27. Schwendicke, F, Splieth CH, Bottenberg P, Breschi L, Campus G, Domejean S, et al. How to intervene in the caries process in adults: proximal and secondary caries? An EFCD-ORCA-DGZ expert Delphi consensus statement. Clin Oral Investig. (2020) 24(9):3315–21. doi: 10.1007/s00784-020-03431-0
28. Tan HP, Lo EC, Dyson JE, Luo Y, Corbet EF. A randomized trial on root caries prevention in elders. J Dent Res. (2010) 89(10):1086–90. doi: 10.1177/0022034510375825
29. Fischer NG, Munchow EA, Tamerler C, Bottino MC, Aparicio C. Harnessing biomolecules for bioinspired dental biomaterials. J Mater Chem B. (2020) 8(38):8713–47. doi: 10.1039/d0tb01456g
30. Datta LP, Manchineella S, Govindaraju T. Biomolecules-derived biomaterials. Biomaterials. (2020) 230:119633. doi: 10.1016/j.biomaterials.2019.119633
31. Diekwisch TG, Berman BJ, Anderton X, Gurinsky B, Ortega AJ, Satchell PG, et al. Membranes, minerals, and proteins of developing vertebrate enamel. Microsc Res Tech. (2002) 59(5):373–95. doi: 10.1002/jemt.10218
32. Kim J, Arola DD, Gu L, Kim YK, Mai S, Liu Y, et al. Functional biomimetic analogs help remineralize apatite-depleted demineralized resin-infiltrated dentin via a bottom-up approach. Acta Biomater. (2010) 6(7):2740–50. doi: 10.1016/j.actbio.2009.12.052
33. Margolis HC, Beniash E, Fowler CE. Role of macromolecular assembly of enamel matrix proteins in enamel formation. J Dent Res. (2006) 85(9):775–93. doi: 10.1177/154405910608500902
34. Gungormus M, Oren EE, Horst JA, Fong H, Hnilova M, Somerman MJ, et al. Cementomimetics-constructing a cementum-like biomineralized microlayer via amelogenin-derived peptides. Int J Oral Sci. (2012) 4(2):69–77. doi: 10.1038/Ijos.2012.40
35. Mounit MMF, Matat MA, Lei YP, Snead ML. Recombinant amelogenin protein induces apical closure and pulp regeneration in open-apex, nonvital permanent canine teeth. J Endodont. (2016) 42(3):402–12. doi: 10.1016/j.joen.2015.11.003
36. Mukherjee K, Ruan Q, Nutt S, Tao J, De Yoreo JJ, Moradian-Oldak J. Peptide-Based bioinspired approach to regrowing multilayered aprismatic enamel. ACS Omega. (2018) 3(3):2546–57. doi: 10.1021/acsomega.7b02004
37. Su M, Yao S, Gu L, Huang Z, Mai S. Antibacterial effect and bond strength of a modified dental adhesive containing the peptide nisin. Peptides. (2018) 99:189–94. doi: 10.1016/j.peptides.2017.10.003
38. Moussa DG, Aparicio C. Present and future of tissue engineering scaffolds for dentin-pulp Complex regeneration. J Tissue Eng Regen Med. (2019) 13(1):58–75. doi: 10.1002/term.2769
39. Paine ML, Luo W, Zhu DH, Bringas P, Snead ML Jr. Functional domains for amelogenin revealed by compound genetic defects. J Bone Miner Res. (2003) 18(3):466–72. doi: 10.1359/jbmr.2003.18.3.466
40. Snead ML, Zhu DH, Lei YP, White SN, Snead CM, Luo W, et al. Protein self-assembly creates a nanoscale device for biomineralization. Mater Sci Eng C. (2006) 26(8):1296–300. doi: 10.1016/j.msec.2005.08.030
41. Chen CL, Bromley KM, Moradian-Oldak J, DeYoreo JJ. In situ afm study of amelogenin assembly and disassembly dynamics on charged surfaces provides insights on matrix protein self-assembly. J Am Chem Soc. (2011) 133(43):17406–13. doi: 10.1021/ja206849c
42. Gungormus M, Fong H, Kim IW, Evans JS, Tamerler C, Sarikaya M. Regulation of in vitro calcium phosphate mineralization by combinatorially selected hydroxyapatite-binding peptides. Biomacromolecules. (2008) 9(3):966–73. doi: 10.1021/bm701037x
43. Yuca E, Karatas AY, Seker UO, Gungormus M, Dinler-Doganay G, Sarikaya M, et al. In vitro labeling of hydroxyapatite minerals by an engineered protein. Biotechnol Bioeng. (2011) 108(5):1021–30. doi: 10.1002/bit.23041
44. Yucesoy DT, Hnilova M, Boone K, Arnold PM, Snead ML, Tamerler C. Chimeric peptides as implant functionalization agents for titanium alloy implants with antimicrobial properties. JOM. (2015) 67(4):754–66. doi: 10.1007/s11837-015-1350-7
45. Yazici H, O'Neill MB, Kacar T, Wilson BR, Oren EE, Sarikaya M, et al. Engineered chimeric peptides as antimicrobial surface coating agents toward infection-free implants. ACS Appl Mater Interfaces. (2016) 8(8):5070–81. doi: 10.1021/acsami.5b03697
46. Boone K, Camarda K, Spencer P, Tamerler C. Antimicrobial peptide similarity and classification through rough set theory using physicochemical boundaries. BMC Bioinform. (2018) 19:469. doi: 10.1186/s12859-018-2514-6
47. Boone K, Wisdom C, Camarda K, Spencer P, Tamerler C. Combining genetic algorithm with machine learning strategies for designing potent antimicrobial peptides. BMC Bioinform. (2021) 22(1):239. doi: 10.1186/s12859-021-04156-x
48. Tamerler C, Khatayevich D, Gungormus M, Kacar T, Oren EE, Hnilova M, et al. Molecular biomimetics: gepi-based biological routes to technology. Biopolymers. (2010) 94(1):78–94. doi: 10.1002/bip.21368
49. Yuca E, Utku FS, Spencer P, Tamerler C. Chimeric biomolecules: biomolecular recognition-based self-organization at the bio-material interfaces. In: Spencer P, Misra A, editors. Material-Tissue interfacial phenomena. Amsterdam: Woodhead Publishing (2017). p. 285–324.
50. Yuca E, Xie SX, Song L, Boone K, Kamathewatta N, Woolfolk SK, et al. Reconfigurable dual peptide tethered polymer system offers a synergistic solution for next generation dental adhesives. Int J Mol Sci. (2021) 22(12):6552. doi: 10.3390/ijms22126552
51. Xie SX, Boone K, VanOosten SK, Yuca E, Song LY, Ge XP, et al. Peptide mediated antimicrobial dental adhesive system. Appl Sci-Basel. (2019) 9(3):557. doi: 10.3390/app9030557
52. Xie S-X, Song L, Yuca E, Boone K, Sarikaya R, VanOosten SK, et al. Antimicrobial peptide–polymer conjugates for dentistry. ACS Appl Polymer Mater. (2020) 2(3):1134–44. doi: 10.1021/acsapm.9b00921
53. Ye Q, Spencer P, Yuca E, Tamerler C. Engineered peptide repairs defective adhesive-dentin interface. Macromol Mater Eng. (2017) 302(5):1600487. doi: 10.1002/mame.201600487
54. Spencer P, Ye Q, Kamathewatta NJB, Woolfolk SK, Bohaty BS, Misra A, et al. Chemometrics-Assisted Raman spectroscopy characterization of tunable polymer-peptide hybrids for dental tissue repair. Front Mater. (2021) 8:681415. doi: 10.3389/fmats.2021.681415
55. Woolfolk SK, Cloyd AK, Ye Q, Boone K, Spencer P, Snead ML, et al. Peptide-Enabled nanocomposites offer biomimetic reconstruction of silver diamine fluoride-treated dental tissues. Polymers (Basel). (2022) 14(7):1368. doi: 10.3390/polym14071368
56. Song L, Ye Q, Ge X, Misra A, Spencer P. Mimicking nature: self-strengthening properties in a dental adhesive. Acta Biomater. (2016) 35:138–52. doi: 10.1016/j.actbio.2016.02.019
57. Song L, Ye Q, Ge X, Misra A, Tamerler C, Spencer P. Self-strengthening hybrid dental adhesive via visible-light irradiation triple polymerization. Rsc Adv. (2016) 6(57):52434–47. doi: 10.1039/C6RA09933E
58. Song LY, Ye Q, Ge XP, Misra A, Tamerler C, Spencer P. New silyl-functionalized bisgma provides autonomous strengthening without leaching for dental adhesives. Acta Biomater. (2019) 83:130–9. doi: 10.1016/j.actbio.2018.10.033
59. Song LY, Sarikaya R, Ye Q, Misra A, Tamerler C, Spencer P. Multifunctional monomer acts as co-initiator and crosslinker to provide autonomous strengthening with enhanced hydrolytic stability in dental adhesives. Dent Mater. (2020) 36(2):284–95. doi: 10.1016/j.dental.2019.11.007
60. Pietrokovski Y, Nisimov I, Kesler-Shvero D, Zaltsman N, Beyth N. Antibacterial effect of composite resin foundation material incorporating quaternary ammonium polyethyleneimine nanoparticles. J Prosthet Dent. (2016) 116(4):603–9. doi: 10.1016/j.prosdent.2016.02.022
61. Cheng L, Zhang K, Melo MA, Weir MD, Zhou X, Xu HH. Anti-biofilm dentin primer with quaternary ammonium and silver nanoparticles. J Dent Res. (2012) 91(6):598–604. doi: 10.1177/0022034512444128
62. Frassetto A, Breschi L, Turco G, Marchesi G, Di Lenarda R, Tay FR, et al. Mechanisms of degradation of the hybrid layer in adhesive dentistry and therapeutic agents to improve bond durability–a literature review. Dent Mater. (2016) 32(2):e41–53. doi: 10.1016/j.dental.2015.11.007
63. Xia W, Razi MRM, Ashley P, Abou Neel EA, Hofmann MP, Young AM. Quantifying effects of interactions between polyacrylic acid and chlorhexidine in dicalcium phosphate - forming cements. J Mater Chem B. (2014) 2(12):1673–80. doi: 10.1039/c3tb21533d
64. Cieplik F, Jakubovics NS, Buchalla W, Maisch T, Hellwig E, Al-Ahmad A. Resistance toward chlorhexidine in oral bacteria - is there cause for concern? Front Microbiol. (2019) 10:587. doi: 10.3389/fmicb.2019.00587
65. Sajjan P, Laxminarayan N, Kar P, Sajjanar M. Chlorhexidine as an antimicrobial agent in dentistry -a review. Oral Health Dent Manag. (2019) 15:93–100. doi: 10.4172/2247-2452.1000879
66. Liao Y, Chen J, Brandt BW, Zhu Y, Li J, van Loveren C, et al. Identification and functional analysis of genome mutations in a fluoride-resistant Streptococcus Mutans strain. PLoS One. (2015) 10(4):e0122630. doi: 10.1371/journal.pone.0122630
67. Sundheim G, Langsrud S, Heir E, Holck AL. Bacterial resistance to disinfectants containing quaternary ammonium compounds. Int Biodeter Biodegr. (1998) 41(3–4):235–9. doi: 10.1016/S0964-8305(98)00027-4
68. Sterzenbach T, Helbig R, Hannig C, Hannig M. Bioadhesion in the oral cavity and approaches for biofilm management by surface modifications. Clin Oral Investig. (2020) 24(12):4237–60. doi: 10.1007/s00784-020-03646-1
69. Sarikaya R, Song L, Yuca E, Xie SX, Boone K, Misra A, et al. Bioinspired multifunctional adhesive system for next generation bio-additively designed dental restorations. J Mech Behav Biomed Mater. (2021) 113:104135. doi: 10.1016/j.jmbbm.2020.104135
70. Spencer P, Misra A, Ye Q, Picking WD, Boone K, Kamathewatta N, et al. Modulating the mechanochemistry of peptide-polymer hybrids for precision tissue repair. Encyclopedia of Materials: Plastics and Polymers. (2022) 3:397–406. doi: 10.1016/B978-0-12-820352-1.00208-X
71. Mai S, Mauger MT, Niu LN, Barnes JB, Kao S, Bergeron BE, et al. Potential applications of antimicrobial peptides and their mimics in combating caries and pulpal infections. Acta Biomater. (2017) 49:16–35. doi: 10.1016/j.actbio.2016.11.026
72. Misra A, Singh V, Parthasarathy R. Material-tissue interfacial phenomena: Challenges in mathematical modeling. In: Spencer P, Misra A, editors. Material-tissue interfacial phenomena. Amsterdam: Woodhead Publishing (2017). p. 253–64.
73. Misra A, Parthasarathy R, Ye Q, Singh V, Spencer P. Swelling equilibrium of dentin adhesive polymers formed on the water-adhesive phase boundary: experiments and micromechanical model. Acta Biomater. (2014) 10(1):330–42. doi: 10.1016/j.actbio.2013.09.017
74. Singh V, Misra A, Parthasarathy R, Ye Q, Spencer P. Viscoelastic properties of collagen-adhesive composites under water-saturated and dry conditions. J Biomed Mater Res A. (2015) 103(2):646–57. doi: 10.1002/jbm.a.35204
75. Singh V, Misra A, Parthasarathy R, Ye Q, Park J, Spencer P. Mechanical properties of methacrylate-based model dentin adhesives: effect of loading rate and moisture exposure. J Biomed Mater Res B Appl Biomater. (2013) 101(8):1437–43. doi: 10.1002/jbm.b.32963
76. Marangos O, Misra A, Spencer P, Bohaty B, Katz JL. Physico-Mechanical properties determination using microscale homotopic measurements: application to sound and caries-affected primary tooth dentin. Acta Biomater. (2009) 5(4):1338–48. doi: 10.1016/j.actbio.2008.10.023
77. Marangos O, Misra A, Spencer P, Katz JL. Scanning acoustic microscopy investigation of frequency-dependent reflectance of acid- etched human dentin using homotopic measurements. IEEE Trans Ultrason Ferroelectr Freq Control. (2011) 58(3):585–95. doi: 10.1109/TUFFC.2011.1841
78. Parthasarathy R, Misra A, Song L, Ye Q, Spencer P. Structure-Property relationships for wet dentin adhesive polymers. Biointerphases. (2018) 13(6):061004. doi: 10.1116/1.5058072
79. Misra A, Parthasarathy R, Singh V, Spencer P. Micro-Poromechanics model of fluid-saturated chemically active fibrous Media. ZAMM J Appl Math Mech/Z Angew Math Mech. (2015) 95(2):215–34. doi: 10.1002/zamm.201300071
80. Nejadsadeghi N, Misra A. Extended granular micromechanics approach: a micromorphic theory of degree N. Math Mech Solids. (2020) 25(2):407–29. doi: 10.1177/1081286519879479
81. Misra A, Placidi L, Turco E. Variational methods for continuum models of granular materials. In: Altenbach H, Öchsner A, editors. Encyclopedia of Continuum mechanics. Berlin, Heidelberg: Springer Berlin Heidelberg (2019). p. 1–11.
82. Misra A, Sarikaya R. Computational analysis of tensile damage and failure of mineralized tissue assisted with experimental observations. Proc Inst Mech Eng Part H. (2020) 234(3):289–98. doi: 10.1177/0954411919870650
83. Parthasarathy R, Misra A, Ouyang LZ. Finite-Temperature stress calculations in atomic models using moments of position. J Condens Matter Phys. (2018) 30(26):265901. doi: 10.1088/1361-648X/aac52f
84. Misra A, Singh V. Micromechanical model for viscoelastic materials undergoing damage. Continuum Mech Therm. (2013) 25(2–4):343–58. doi: 10.1007/s00161-012-0262-9
85. Misra A, Singh V. Nonlinear granular micromechanics model for multi-axial rate-dependent behavior. Int J Solids Struct. (2014) 51(13):2272–82. doi: 10.1016/j.ijsolstr.2014.02.034
86. Misra A, Singh V. Thermomechanics-Based nonlinear rate-dependent coupled damage-plasticity granular micromechanics model. Continuum Mech Therm. (2015) 27(4-5):787–817. doi: 10.1007/s00161-014-0360-y
87. Misra A, Marangos O, Parthasarathy R, Spencer P. Micro-scale analysis of compositional and mechanical properties of dentin using homotopic measurements. Biomed Imagine Comput Modeling Biomech. (2013) 4:131–41. doi: 10.1007/978-94-007-4270-3_7
88. Misra A, Spencer P, Marangos O, Wang Y, Katz JL. Micromechanical analysis of dentin/adhesive interface by the finite element method. J Biomed Mater Res B Appl Biomater. (2004) 70(1):56–65. doi: 10.1002/jbm.b.30012
89. Misra A, Spencer P, Marangos O, Wang Y, Katz JL. Parametric study of the effect of phase anisotropy on the micromechanical behavior of dentin/adhesive interfaces. J R Soc Interface. (2005) 2:145–57. doi: 10.1098/rsif.2005.0029
90. Singh V, Misra A, Marangos O, Park J, Ye Q, Kieweg SL, et al. Fatigue life prediction of dentin-adhesive interface using micromechanical stress analysis. Dent Mater. (2011) 27(9):e187–95. doi: 10.1016/j.dental.2011.05.010
91. Spencer P, Ye Q, Park J, Topp EM, Misra A, Marangos O, et al. Adhesive/dentin interface: the weak link in the composite restoration. Ann Biomed Eng. (2010) 38(6):1989–2003. doi: 10.1007/s10439-010-9969-6
92. Yang Y, Ching WY, Misra A. Higher-Order Continuum theory applied to fracture simulation of nano-scale intergranular glassy film. Journal of Nanomechanics and Micromechanics. (2011) 1(2):60–71. doi: 10.1061/(ASCE)NM.2153-5477.0000030
93. Timofeev D, Barchiesi E, Misra A, Placidi L. Hemivariational Continuum approach for granular solids with damage-induced anisotropy evolution. Math Mech Solids. (2021) 26(5):738–70. doi: 10.1177/1081286520968149
94. Zhang A, Ye N, Aregawi W, Zhang L, Salah M, VanHeel B, et al. A review of mechano-biochemical models for testing composite restorations. J Dent Res. (2021) 100(10):1030–8. doi: 10.1177/00220345211026918
95. Bauer LJ, Mustafa HA, Zaslansky P, Mantouvalou I. Chemical mapping of teeth in 2d and 3d: x-ray fluorescence reveals hidden details in dentine surrounding fillings. Acta Biomater. (2020) 109:142–52. doi: 10.1016/j.actbio.2020.04.008
Keywords: peptide-mediated remineralization, elderly population, root surfaces, peptide-tethered polymer, cervical, peptide-mediated antimicrobial
Citation: Spencer P, Ye Q, Misra A, Chandler JR, Cobb CM and Tamerler C (2022) Engineering peptide-polymer hybrids for targeted repair and protection of cervical lesions. Front. Dent. Med 3:1007753. doi: 10.3389/fdmed.2022.1007753
Received: 30 July 2022; Accepted: 11 November 2022;
Published: 25 November 2022.
Edited by:
Victor Pinheiro Feitosa, Faculdade Paulo Picanço, BrazilReviewed by:
Jeffrey Platt, Indiana University, United StatesMonica Yamauti, Hokkaido University, Japan
© 2022 Spencer, Ye, Misra, Chandler, Cobb and Tamerler. This is an open-access article distributed under the terms of the Creative Commons Attribution License (CC BY). The use, distribution or reproduction in other forums is permitted, provided the original author(s) and the copyright owner(s) are credited and that the original publication in this journal is cited, in accordance with accepted academic practice. No use, distribution or reproduction is permitted which does not comply with these terms.
*Correspondence: Paulette Spencer, cHNwZW5jZXJAa3UuZWR1; Qiang Ye, eWVxQGt1LmVkdQ==
Specialty Section: This article was submitted to Dental Materials, a section of the journal Frontiers in Dental Medicine