- 1Faculty of Medicine Dentistry and Health Sciences, The University of Melbourne, Parkville, VIC, Australia
- 2Department of Paediatric Stomatology, Faculty of Health Sciences, University of Talca, Talca, Chile
- 3Faculty of Dentistry, University of Toronto, Toronto, ON, Canada
One hundred years ago, histopathology pioneer Bernhard Gottlieb described developmentally disrupted teeth as having “chalky enamel” and “chalky spots” that “crumble” easily. He also asked pivotal questions about the pathogenesis of “enamel hypoplasia” that remained enigmatic for almost a century. Today, breakthrough pathomechanistic investigations of chalky enamel are revealing surprising answers, and an allied translational initiative—The D3 Group for developmental dental defects (“D3s”) —is converting such scientific knowledge into social good surrounding prevention of tooth decay. Molar hypomineralisation (MH) affects 1-in-5 children worldwide and is well-evidenced, but poorly recognised, as a principal risk factor for childhood tooth decay. Given MH is causally linked to infantile illness, an exciting corollary is that medical prevention would lead to substantial reductions in decay. Here we reflect on the past century of chalky teeth research and retrace the path leading to recognition of MH as a global health concern. Five research eras, today's four major D3s, and diverse experimental attacks are outlined alongside translational wins that have benefitted global health. Addressing hopes for medical prevention of MH, this centennial year's pathomechanistic discovery is contextualised against past accomplishments and new opportunities. Finally, we note the translational value of accessible infographics for guiding future work, and forecast exciting prospects for the next century.
100 Years in a Nutshell
One hundred years ago, eminent medical scientist Bernhard Gottlieb described developmentally disrupted teeth as having “chalky enamel” and “chalky spots” that “crumble” easily (1, 2). He also asked pivotal questions about the pathogenesis of so-called “enamel hypoplasia” that remained enigmatic for almost a century – why are lesions scattered among normal enamel and do they reflect cellular or extracellular disruption? Today, breakthrough pathomechanistic investigations of chalky enamel are revealing surprising answers, and an allied translational initiative – The D3 Group (D3G) for developmental dental defects (“D3s”) – is converting such scientific knowledge into social good surrounding prevention of tooth decay (3–9)1. The primary target of these efforts is molar hypomineralisation (MH), a developmental disorder of unknown cause (idiopathic) defined pathologically by discolored enamel lesions termed demarcated opacities. MH affects the back teeth (2-year molars, 6-year molars, or both)2 of 1-in-5 children worldwide and is well-evidenced, but poorly recognised, as a principal risk factor for childhood tooth decay3,4. Given MH is causally linked to infantile illness, an exciting corollary is that medical prevention would lead to substantial reductions in decay and dental caries – a worldwide blight incurring massive social and economic tolls5.
Here we reflect briefly on the past century of chalky teeth research and retrace the path leading to recognition of MH as a significant concern for global health. Five research eras, today's four major D3s, and diverse experimental attacks are outlined alongside translational wins that have brought social good worldwide. Addressing hopes for medical prevention of MH, the pathomechanistic breakthrough—fortuitously reported in this centennial year for chalky teeth—is contextualised against past accomplishments and new opportunities. Finally, we note the translational value of accessible infographics for guiding future work, and forecast exciting prospects for the next century.
Three Pioneers of the “Enamel Hypoplasia” Research Era
“Chalky teeth” sound like a dental matter. But actually they may be the fossilised legacy of paediatric medical problems, as is the case with MH. This was realised by Gottlieb who is considered a founder of oral biology having instigated the field of oral histopathology during his medical training. His 1920 investigation of enamel defects in children overwhelmed by various contemporary diseases (e.g., rickets, syphillis, gastroenteritis, measles) led to the conclusion that injury of enamel-forming cells (ameloblasts) was secondary to defective calcification of extracellular enamel (1, 2). Six decades later, more-nuanced studies of infantile sheep by the pioneer of a later era—dentist-turned-researcher Grace Suckling—prompted the opposite conclusion, and the notion that enamel hypomineralisation results from primary injuries to ameloblasts became dogma until now (10–12). Gottlieb applied “chalky” descriptors both to immature enamel (as seen histologically) and to erupted teeth, as back then “enamel hypoplasia” embraced what today are known separately as hypoplasias (pits, grooves) and hypomineralised opacities.
Another early contributor to MH history was Joseph Turner, a dentist whose findings about “localized enamel hypoplasia” became memorialised with the term “Turner teeth.” In 1909 he reported that patches of discoloured enamel in permanent (adult) teeth often coincided with predecessor primary (baby, or deciduous)6 teeth that had been abscessed, prompting the conclusion that an abscess-related inflammatory process had earlier damaged the enamel organ of the developing adult tooth (13). Later investigators substantiated this idea and added traumatised baby teeth—usually intruded front teeth (incisors) in fallen toddlers—as a second means of disrupting enamel development locally in successional adult teeth (14). Hereafter we refer to these two types of ascribed disruption as local causes of demarcated opacities (as opposed to the idiopathic, systemic causation of MH).
Our third pioneer of the “enamel hypoplasia” era is physiologist May Mellanby who—together with husband Edward, a medical physiologist and discoverer of vitamin D—hypothesised that enamel defects associated with rickets reflect a nutritional insufficiency (15, 16). This led to a famous series of investigations that inspired global health improvements, as elaborated below.
Five Research Eras
With hindsight, five research eras can be recognised as stepping stones to the present translational mix of D3s, chalky teeth and MH (Figure 1). The “enamel hypoplasia” era ended in 1949 with dentist Veikko Hurme's breakthrough report about “discrete enamel opacities,” later renamed demarcated opacities by Suckling (17, 18). This followed other investigators' delineation of fluoride-related opacities and amelogenesis imperfecta (AI) from true hypoplasia – so-called enamel pits and grooves (19, 20). Thus, the field now comprised four principal D3s, as holds today.
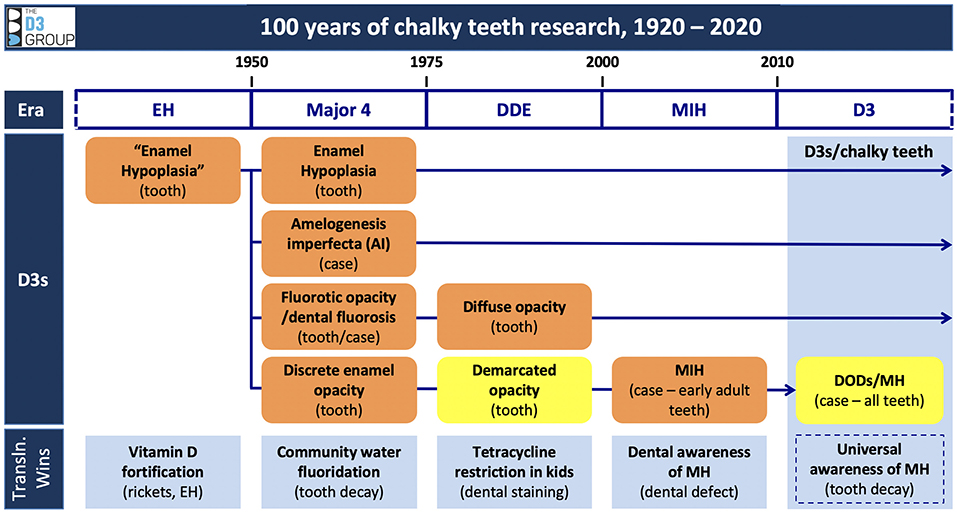
Figure 1. A century of chalky teeth research leading to D3s and MH. This infographic depicts the research eras, major D3s, and translational (Transln.) wins referred to in the text. Our present focus on demarcated opacities, demarcated opacity disorders (DODs) and MH is highlighted in yellow. Note that some D3 descriptors relate to affected individuals, others to dental pathology (case and tooth level, respectively). This portrayal encourages researchers to consider MH in context of the other major D3s, realising for example that the dental phenotype of an AI child might be influenced by MH (indeed, why wouldn't MH affect 1-in-5 AI children?). Conversely, should a child suffer over several years serial bouts of whatever causes MH, their dental phenotype could well resemble AI. Another consideration is that multiple types of D3 (hypoplasia, demarcated and diffuse opacities) may appear on the same tooth. EH, enamel hypoplasia; DODs, demarcated opacity disorders. Taken from a D3G working document (Hubbard, unpublished) with permission.
The “major 4” era, which ended with Suckling's emergence in the 1970s, featured fragmentary progress clouded by terminological indiscipline. Contrastingly, Suckling orchestrated a remarkably cohesive set of investigations that advanced pathomechanistic understanding greatly and produced a standardised clinico-scientific terminology—the developmental defects of enamel (DDE) index—that remains foundational to the D3 field (10, 18)7. The DDE era defined further hallmarks of MH, being idiopathic demarcated opacities in baby and adult teeth that are prone to degradation (so-called “post-eruptive breakdown”), plus a sporadic phenotype (affecting 1 to all 4 molars of any type) that seemingly contradicts systemic causation (21, 22). However, a case descriptor for the common clinical presentations of idiopathic demarcated opacities was lacking.
The “MIH” era commenced in 2001 when the term “molar-incisor hypomineralisation” (MIH) was introduced by European paediatric dentists to describe cases involving early adult teeth – namely 6-year molars, with or without co-affected incisors (23). Together with streamlining of the DDE index, a suite of pioneering studies by dentist-turned-researcher Birgitta Jalevik triggered numerous investigations ranging from epidemiology and clinical impacts to ultrastructure of chalky enamel. Detrimentally however, the restriction to early adult teeth sidelined 2- and 12-year molars—which, like 6-year molars, are the principal loci for childhood tooth decay4–and the inclusion of incisors raised scientific concerns about aetiological confounding. The latter issue reflects clinical inability to distinguish idiopathic demarcated opacities from those consequent to trauma or infection, which is an issue for all successional teeth – i.e., adult incisors, canines, and premolars (10, 24, 25).
The “D3” era started a decade later with a biochemical investigation of chalky enamel directed toward medical prevention of MH and allied decay, and launch of D3G's translational education resource and public-facing “Chalky Teeth Campaign.” Being scientifically driven and prevention-centric, the ensuing D3 movement co-opted the term (idiopathic) MH to encompass the complete baby and adult dentitions and also avoid the aetiological complications surrounding adult incisors (3–5, 7–9, 26)8,9.
Four Major D3S of Enamel
Although demarcated opacities are center-stage of the MH story, it seems opportune to address their delineation (differential diagnosis) from the other 3 major D3s of enamel and also detangle some terminological confusion that frequents the literature. It is imperative that case descriptors (AI, dental fluorosis, MIH, MH) are distinguished from tooth-level pathology such as enamel opacities (Figure 1). For example, common misnomers such as “MIH molars” and “MIH enamel” overlook the fact that—consequent upon the sporadic phenotype—affected molars in MIH children usually contain both normal and abnormal enamel, and those children may also have partner molars that aren't hypomineralised.
Based on historical understanding of causation and pathogenesis, major D3s have long been divided into primary groupings of genetic (AI) and acquired (the rest). AI comes in many flavors and is a key comparator for MH because, although rare, it can be devastating for affected families as most if not all teeth are disrupted (3). That some manifestations of AI feature demarcated opacities also holds aetiological interest for MH (27, 28). Diffuse opacities are a hallmark of fluoride overexposure (dental fluorosis) and usually can be distinguished from demarcated opacities through clinical appearance. However, as Suckling stressed, an individual tooth may have both demarcated and diffuse opacities and the latter may reflect causes other than fluoride. Distinguishing features of demarcated opacities (distinct borders, discolouration, hard or chalky texture, acid lability) were reported by Hurme and then correlated to enamel cell biology by Suckling. Crucially, she linked diffuse and demarcated opacities to the secretory and hardening phases of enamel formation, respectively (10, 11, 17). Many investigators have since added a wealth of detail to these fundamentals, as follows.
A Multitude of Experimental Approaches and Adaptations
The foregoing D3 learnings involved a battery of experimental approaches, many adapted from mainstream soft-tissue science to accommodate the technical challenges posed by dental enamel – our hardest tissue. To exemplify how MH studies have ranged from population level to nanoscale, it's notable that light microscopic histology as pioneered by Gottlieb remains informative today, boosted by modernisations including polarised light microscopy, immunofluorescence, scanning and transmission electron microscopy (11, 29–32). Biophysical investigations have grown from Hurme's simplistic acid dissolution of chalky spots to advanced analyses of chemical composition and hardness (30, 33–35). With time, biochemical approaches were used to query pathogenesis of chalky enamel at protein level (26, 36–39). Together, these tooth-level analyses showed that chalky opacities exhibit elevated porosity and carbonate levels (i.e., more chalk-like), plus high amounts of protein consistent with incomplete hardening.
Animal models added pathophysiological insights, ranging from Mellanby's rachitic puppies and Suckling's gastroenteritic lambs through to toxicological and gene-knockout studies in rats and mice (10, 15, 40, 41). Further understanding came from human population and genetics studies (epidemiology, twins, single nucleotide polymorphisms), which together reinforced longstanding belief that idiopathic demarcated opacities (and so MH) are primarily of acquired/non-genetic origin (17, 42–46). As such, this bodes well for medical preventability of MH – notably, even partial alleviation of severe phenotypes (i.e., hard-white, rather than chalky opacities) would normalise risk of decay4. However, as with other acquired conditions, we must expect that complex genetics may also influence outcomes to some degree.
Five Translational Successes
It seems remarkable (indeed inspirational) that such a boutique field as D3s has inspired a string of translational successes, two of which have benefitted global health through integration of medicine and dentistry (Figure 1). While common practice today, vitamin D-balanced lifestyles trace back to the Mellanbys' discovery that cod liver oil cured rickets in dogs – one manifestation of which was “enamel hypoplasia” (15, 16). These findings were amplified and then translated to population level through vitamin D-rich foods, leading to containment of rickets and consequent improvements to dental health—namely less hypoplasia and allied decay—by the 1940s (47). A second medico-dental win followed introduction of tetracycline antibiotics in the early 1950s. While tetracyclines provided a magic bullet for many paediatric infections, unsightly grey/brown staining of tooth enamel was an unacceptable side-effect. The causal link to tooth development was soon recognised and medical restriction of tetracycline use to people older than 8 years was implemented worldwide over the course of 2 decades. The translational success of community water fluoridation—another nutritional intervention that led to global reductions in tooth decay—needs no elaboration other than to note progression from initial clinical observations to successful management at population level took several decades (3).
More modestly, the MIH era successfully translated problem-awareness (and allied clinical guidelines) from a select few researchers and practitioners out to the wider dental community10. The D3 movement in turn is extending awareness of MH and the all-important connection to childhood tooth decay more broadly across the healthcare sector and society. By publicising MH as a medically-acquired disorder, this prevention-centric initiative is using a medico-dental platform to argue for well-funded cross-disciplinary research (3)5.
Three DS and a Social-Good Movement Driven by Science
It struck the first author that, if the real world was to get a grip on the 3-layered “MH problem” —comprising MH itself, allied educational gaps, and lack of scientific understanding—there would need to be an accessible language embedded within a cross-sector translational approach. Initially this inspired coining of “D3” as an abstract substitute for the technical tongue-twister it stands for (3). D3G has since developed a “translational lingo” (e.g., “chalky teeth,” “chalky molars,” “Molar Hypomin”) combining clinico-scientific and public faces, and mined the historical record to put “chalky terminology” on a solid scientific and social footing1,11,12. These and other social-good aspects of the D3 movement reflect D3G's philanthropic origins, whereas the scientific “pointy end” draws on career scientists working collaboratively with practitioners and other stakeholders. Unique outcomes of this scientifically-driven translational approach include an international cross-sector network embodying numerous researchers of diverse ilk (“D3G's research army”), a “one-stop” online-education resource featuring tools for researchers and affected children alike, and a nascent practice-based research network (3, 9)8,13,14.
D3G's aspiration to prevent MH through paediatric medical intervention reflects expertise of the biomedical research team at its core. They attacked this lofty goal by addressing some basic questions about chalky opacities, hoping that answers would eventually elevate idiopathic MH to a disorder of known causation as follows.
One Unexpected Protein and a 100-Year Breakthrough
Gottlieb's century-old questions about opacity localisation within enamel, together with Suckling's intrigue over 6-year molars being affected sporadically, led dentally-trained biochemist Mike Hubbard to reason that MH pathogenesis involves 2 mechanistic levels: (1) a local (dental) pathomechanism responsible for chalky enamel; and (2) a systemic (medical) disruption that triggers the localised dental event. Using proteomics and an inside-out strategy to address the local pathomechanism, chalky enamel was found to contain numerous proteins when the opacity surface was visibly broken, but only one prevalent protein if the surface layer was intact and so impervious to oral fluid (26). That the latter “fossilised protein” was serum albumin—and not the principal enamel protein amelogenin—was unexpected given long-established connections between retained amelogenin and defective enamel (37, 38). Such presence of albumin also had to be treated cautiously given a contentious history concerning experimental artifact (26, 39, 48).
Two follow-up studies in 2020 overcame the artifact concerns and addressed medical-onset and dental-outcome perspectives, respectively. The collective biochemical findings provided cohesive evidence that the local pathomechanism does indeed involve exposure of unhardened enamel to serum albumin (7, 8). This discovery, plus evidence that albumin resists the proteolytic attack normally used to remove amelogenin—thereby enabling enamel to harden—provides breakthrough insight to the pathogenesis of chalky enamel. The ensuing pathomechanistic model, termed “mineralisation poisoning,” involves persistent blockage of enamel hardening by albumin and plausibly accounts for the clinical characteristics of demarcated opacities and MH. It also answers Gottlieb's question by establishing that, at its ultimate crux, MH involves direct disruption of immature enamel and not injury to enamel cells as held by dogma – the latter may well be involved in penultimate pathologic steps however. This fundamental advance holds significance for future clinical management and opens an exciting research avenue toward medical prevention of MH (6–8).
Several Infographics and a Symposium to Guide Future Research and Learning
If the scientific path to victory over MH is to be smoothed, shortcomings exemplified by today's muddled literature must be addressed with a focus on standardisation (e.g., common terminology and definitions extending across laboratory and clinical practice). This isn't to say that off-track activities should be stifled, rather that a strong consensus should be identified from which to base future thinking and communication. We hope that by condensing a century of learning into a series of accessible infographics (e.g., the working documents depicted in Figures 1, 2), the field will soon gain a consistent understanding of past achievements and then develop a robust framework for future work. Translationally, such educational tools should be dynamic, readily available online, and adaptable to various audiences. Cross-disciplinary dialogue will be required to achieve consensus and marry the demands of scientific method with the realities of clinical practice, as exemplified by proposed lab-friendly clinical descriptors italicised in Figure 2. Fittingly, the century is closing with D3G's inaugural international workshop and symposium, which bring unprecedented opportunity to involve all stakeholders in such discourse pertaining to MH and chalky teeth15.
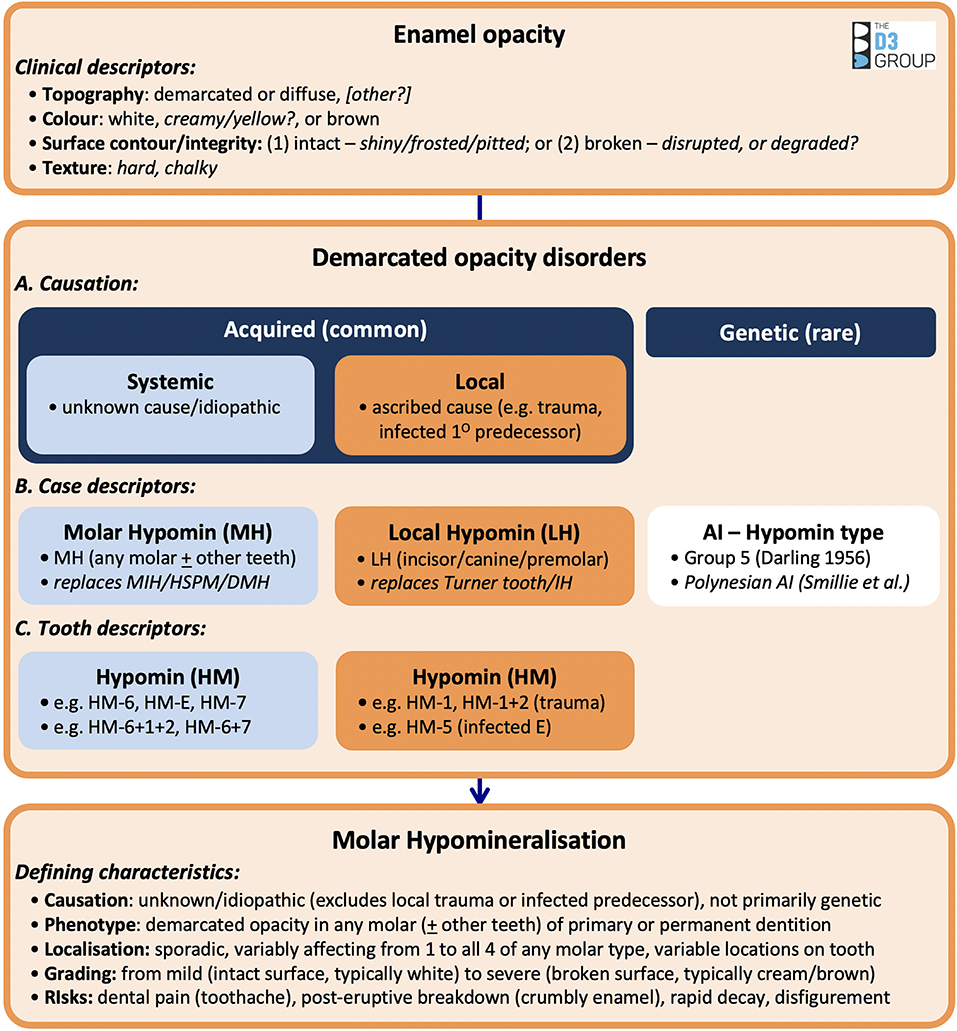
Figure 2. Charting 100 years of learning about demarcated opacity disorders. This infographic depicts key aspects of enamel opacities and MH discussed in the text – some facets still requiring clinico-scientific consensus are italicised. The layout encourages researchers to think “inside-out” – from tooth-level pathology to whole-dentition phenotype – rather than starting with case descriptors such as MH. Consequently, MH and other manifestions of demarcated opacities (e.g., Turner teeth) are logically denominated together as “demarcated opacity disorders,” thereby encouraging consideration of how disparate causal events (ascribed and idiopathic) can trigger the same dental pathology (11). Researchers' needs to assign hypomineralisation status to individual teeth in both dentitions, and to record combinatorial phenotypes, are also embraced. HSPM, hypomineralised second primary molar; DMH, deciduous molar hypomineralisation; IH, incisor hypomineralisation; HM-6, hypomineralised first adult molar; HM-E, hypomineralised second baby molar; HM-7, hypomineralised second adult molar; HM-1+2, hypomineralised first and second adult incisors; HM-5, hypomineralised second premolar. Taken from a D3G working document (Hubbard, unpublished) with permission.
Some Closing Thoughts About the Next Century
The past century has seen chalky teeth research grow from pioneering histology into a comprehensive international endeavor framed around science translation and social good. Gottlieb's “chalky spots” have been illuminated in molecular detail, providing pathomechanistic insight to his visionary intrigue about teeth erupting with isolated patches of crumbly enamel. Strikingly, the field now has protein biomarkers with aetiological and clinical potential, spurring exciting thoughts about digital dentistry workflows connecting seamlessly to laboratory and epidemiological investigations. The emergent handle on MH pathogenesis opens the door to concerted trans-disciplinary attack on medical prevention, bolstered by integrated educational and advocacy programs. If translational-research cards are played well, coming years may see funders hunting for D3 researchers—rather than the opposite situation we all suffer today—and timescales for translation to social good may decrease from decades to years. We close by tipping our hats to the multitude of investigators who elevated the D3 field to what it is today, and encourage researchers of all station to consider adding “translational D3ology” to their future interests.
Data Availability Statement
The original contributions presented in the study are included in the article, further inquiries can be directed to the corresponding author.
Author Contributions
MH conceived, drafted, and critically revised the manuscript. VP and BG made underpinning intellectual contributions and critical revisions. All authors contributed to the article and approved the submitted version.
Funding
This work was supported by the Melbourne Research Unit for Facial Disorders at the University of Melbourne (to MH and D3G).
Conflict of Interest
MH is founder/director of The D3 Group for developmental dental defects (www.thed3group.org), a charitable network.
The authors declare that the research was conducted in the absence of any commercial or financial relationships that could be construed as a potential conflict of interest.
Acknowledgments
We thank colleagues (Erin Mahoney, Louise Messer, Alan Mighell, Garry Nervo, Tim Wright) for refining the manuscript and acknowledge the international D3 community for multifarious contributions that catalysed and continue to shape the D3 movement.
Abbreviations
D3, developmental dental defect; MH, molar hypomineralisation; D3G, The D3 Group for developmental dental defects; AI, amelogenesis imperfecta; DDE, developmental defect of enamel; MIH, molar-incisor hypomineralisation.
Footnotes
1. ^www.thed3group.org/social-impact
2. ^We use the classical terms 2-year/6-year/12-year molars (corresponding to second primary/first permanent/second permanent molars, respectively) because of the following translational benefits: (1) aetiologically, it is useful to align dental outcomes with the time course of medical onset in babies and young children; (2) clinically, and for early screening in particular, it is useful to align with tooth-eruption ages; (3) for public communication, these colloquial terms are logical and simple.
3. ^www.thed3group.org/prevalence
4. ^www.thed3group.org/health-risks
5. ^www.chalkyteeth.org/prevention.html
6. ^We use the public-friendly terms “baby” and “adult” teeth to facilitate translational communication and also to align medical onset of MH (hypomineralised 2-year/6-year molars) with “sick babies”.
7. ^www.thed3group.org/grace-suckling
10. ^www.thed3group.org/specialist-4
11. ^www.thed3group.org/what-are-chalky-teeth
12. ^www.chalkyteeth.org/speak-chalky-teeth.html
13. ^www.thed3group.org/international
14. ^www.chalkyteeth.org/we-fight-chalky-teeth-practices.html
References
3. Hubbard MJ, Mangum JE, Perez VA, Nervo GJ, and Hall RK. Molar hypomineralisation: a call to arms for enamel researchers. Front Physiol. (2017) 8:546. doi: 10.3389/fphys.2017.00546
4. Hubbard MJ. Molar hypomineralization: what is the US experience? J Am Dent Assoc. (2018) 149:329–30. doi: 10.1016/j.adaj.2018.03.013
5. Hubbard MJ. Chalky teeth 100 years on: what comes next? J Am Dent Assoc. (2020) 151:803–5. doi: 10.1016/j.adaj.2020.07.022
6. Hubbard MJ, Mangum JE, Perez VA, and Williams R. A breakthrough in understanding the pathogenesis of molar hypomineralisation: the mineralisation-poisoning model. Front Physiol. (Forthcoming).
7. Perez VA, Mangum JE, and Hubbard MJ. Pathogenesis of molar hypomineralisation: aged albumin demarcates chalky regions of hypomineralised enamel. Front Physiol. (2020) 11:1–8. doi: 10.3389/fphys.2020.579015
8. Williams R, Perez VA, Mangum JE, and Hubbard MJ. Pathogenesis of molar hypomineralisation: hypomineralised 6-year molars contain traces of fetal serum albumin. Front Physiol. (2020) 11:1–9. doi: 10.3389/fphys.2020.00619
9. Hubbard MJ. Molar hypomineralization: what is the U.S. experience (revisited)? Pediatr Dent. (2020) 42:414–6.
10. Suckling GW. Developmental defects of enamel–historical and present-day perspectives of their pathogenesis. Adv Dent Res. (1989) 3:87–94. doi: 10.1177/08959374890030022901
11. Suckling GW, Nelson DG, and Patel MJ. Macroscopic and scanning electron microscopic appearance and hardness values of developmental defects in human permanent tooth enamel. Adv Dent Res. (1989) 3:219–33. doi: 10.1177/08959374890030022301
12. Babajko S, Jedeon K, Houari S, Loiodice S, and Berdal A. Disruption of steroid axis, a new paradigm for molar incisor hypomineralization (MIH). Front Physiol. (2017) 8:343. doi: 10.3389/fphys.2017.00343
13. Turner JG. Injury to the teeth of succession by abcess of the temporary teeth. Br Dent J. (1909) 30:1233–7.
14. Andreasen JO, and Ravin JJ. Enamel changes in permanent teeth after trauma to their primary predecessors. Scand J Dent Res. (1973) 81:203–9. doi: 10.1111/j.1600-0722.1973.tb00330.x
15. Mellanby M. The influence of diet on teeth formation. Lancet. (1918) 192:767–70. doi: 10.1016/S0140-6736(01)04455-5
16. Mellanby E. An experimental investigation of rickets. Lancet. (1919) 193:407–12. doi: 10.1016/S0140-6736(01)25465-8
17. Hurme VO. Developmental opacities of teeth in a New England community; their relation to fluorine toxicosis. Am J Dis Child. (1949) 77:61–75. doi: 10.1001/archpedi.1949.02030040068005
19. Kronfeld R, and Schour I. Neonatal dental hypoplasia. J Am Dent Assoc. (1939) 26:18–32. doi: 10.14219/jada.archive.1939.0041
20. Weinmann JP, Svoboda JF, and Woods RW. Hereditary disturbances of enamel formation and calcification. J Am Dent Assoc. (1945) 32:397–418. doi: 10.14219/jada.archive.1945.0063
21. Suckling GW, Pearce EI, and Cutress TW. Developmental defects of enamel in New Zealand children. N Z Dent J. (1976) 72:201–10.
22. Murray JJ, and Shaw L. Classification and prevalence of enamel opacities in the human deciduous and permanent dentitions. Arch Oral Biol. (1979) 24:7–13. doi: 10.1016/0003-9969(79)90168-7
23. Weerheijm KL, Jalevik B, and Alaluusua S. Molar-incisor hypomineralisation. Caries Res. (2001) 35:390–1. doi: 10.1159/000047479
24. Wogelius P, Haubek D, and Poulsen S. Prevalence and distribution of demarcated opacities in permanent 1st molars and incisors in 6 to 8-year-old Danish children. Acta Odontol Scand. (2008) 66:58–64. doi: 10.1080/00016350801926941
25. Balmer R, Toumba KJ, Munyombwe T, Godson J, and Duggal MS. The prevalence of incisor hypomineralisation and its relationship with the prevalence of molar incisor hypomineralisation. Eur Arch Paediatr Dent. (2015) 16:265–9. doi: 10.1007/s40368-014-0171-7
26. Mangum JE, Crombie FA, Kilpatrick N, Manton DJ, and Hubbard MJ. Surface integrity governs the proteome of hypomineralized enamel. J Dent Res. (2010) 89:1160–5. doi: 10.1177/0022034510375824
27. Darling AI. Some observations on amelogenesis imperfecta and calcification of the dental enamel. Proc R Soc Med. (1956) 49:759–65. doi: 10.1177/003591575604901007
28. Smillie AC, Rodda JC, and Kawasaki K. Some aspects of hereditary defects of dental enamel, including some observations on pigmented Polynesian enamel. N Z Dent J. (1986) 82:122–5.
29. Kostlan J, and Plackova A. The histological investigation of the developmental hypomineralized areas of the enamel and their comparison with the carious lesion. Arch Oral Biol. (1962) 7:317–26. doi: 10.1016/0003-9969(62)90022-5
30. Jalevik B. Enamel hypomineralization in permanent first molars. A clinical, histo-morphological and biochemical study. Swed Dent J Suppl. (2001) 1–86.
31. Rodd HD, Morgan CR, Day PF, and Boissonade FM. Pulpal expression of TRPV1 in molar incisor hypomineralisation. Eur Arch Paediatr Dent. (2007) 8:184–8. doi: 10.1007/BF03262594
32. Xie ZH, Mahoney EK, Kilpatrick NM, Swain MV, and Hoffman M. On the structure-property relationship of sound and hypomineralized enamel. Acta Biomater. (2007) 3:865–72. doi: 10.1016/j.actbio.2007.05.007
33. Mahoney EK, Rohanizadeh R, Ismail FS, Kilpatrick NM, and Swain MV. Mechanical properties and microstructure of hypomineralised enamel of permanent teeth. Biomaterials. (2004) 25:5091–100. doi: 10.1016/j.biomaterials.2004.02.044
34. Crombie FA, Manton DJ, Palamara JE, Zalizniak I, Cochrane NJ, and Reynolds EC. Characterisation of developmentally hypomineralised human enamel. J Dent. (2013) 41:611–8. doi: 10.1016/j.jdent.2013.05.002
35. Natarajan AK, Fraser SJ, Swain MV, Drummond BK, and Gordon KC. Raman spectroscopic characterisation of resin-infiltrated hypomineralised enamel. Anal Bioanal Chem. (2015) 407:5661–71. doi: 10.1007/s00216-015-8742-y
36. Robinson C, Kirkham J, Brookes SJ, and Shore RC. The role of albumin in developing rodent dental enamel: a possible explanation for white spot hypoplasia. J Dent Res. (1992) 71:1270–4. doi: 10.1177/00220345920710060101
37. Wright JT, Hall KI, and Yamauche M. The enamel proteins in human amelogenesis imperfecta. Arch Oral Biol. (1997) 42:149–59. doi: 10.1016/S0003-9969(96)00096-9
38. Takagi Y, Fujita H, Katano H, Shimokawa H, and Kuroda T. Immunochemical and biochemical characteristics of enamel proteins in hypocalcified amelogenesis imperfecta. Oral Surg Oral Med Oral Pathol Oral Radiol Endod. (1998) 85:424–30. doi: 10.1016/S1079-2104(98)90068-3
39. Farah RA, Monk BC, Swain MV, and Drummond BK. Protein content of molar-incisor hypomineralisation enamel. J Dent. (2010) 38:591–6. doi: 10.1016/j.jdent.2010.04.012
40. Jedeon K, De La Dure-Molla M, Brookes SJ, Loiodice S, Marciano C, Kirkham J, et al. Enamel defects reflect perinatal exposure to bisphenol A. Am J Pathol. (2013) 183:108–18. doi: 10.1016/j.ajpath.2013.04.004
41. Nakayama Y, Holcroft J, and Ganss B. Enamel hypomineralization and structural defects in amelotin-deficient mice. J Dent Res. (2015) 94:697–705. doi: 10.1177/0022034514566214
42. Mahoney EK, and Morrison DG. Further examination of the prevalence of MIH in the Wellington region. N Z Dent J. (2011) 107:79–84.
43. Teixeira R, Andrade NS, Queiroz LCC, Mendes FM, Moura MS, Moura L, et al. Exploring the association between genetic and environmental factors and molar incisor hypomineralization: evidence from a twin study. Int J Paediatr Dent. (2018) 28:198–206. doi: 10.1111/ipd.12327
44. Bussaneli DG, Restrepo M, Fragelli CMB, Santos-Pinto L, Jeremias F, Cordeiro RCL, et al. Genes regulating immune response and amelogenesis interact in increasing the susceptibility to molar-incisor hypomineralization. Caries Res. (2019) 53:217–27. doi: 10.1159/000491644
45. Silva MJ, Kilpatrick NM, Craig JM, Manton DJ, Leong P, Burgner D, et al. Etiology of hypomineralized second primary molars: a prospective twin study. J Dent Res. (2019) 98:77–83. doi: 10.1177/0022034518792870
46. Vieira AR. On the genetics contribution to molar incisor hypomineralization. Int J Paediatr Dent. (2019) 29:2–3. doi: 10.1111/ipd.12439
47. Mellanby M, and Mellanby H. The reduction in dental caries in 5-year-old London school-children, 1929-47. Br Med J. (1948) 2:409–13. doi: 10.1136/bmj.2.4573.409
Keywords: global health, paediatric disorders, dental defects, dental caries, medical prevention, developmental biomarkers, serum albumin, biomineralization
Citation: Hubbard MJ, Perez VA and Ganss B (2021) 100 Years of Chalky Teeth Research: From Pioneering Histopathology to Social Good. Front. Dent. Med. 1:632534. doi: 10.3389/fdmed.2020.632534
Received: 23 November 2020; Accepted: 15 December 2020;
Published: 15 January 2021.
Edited by:
Figen Seymen, Istanbul University, TurkeyReviewed by:
Jan Kühnisch, LMU Munich University Hospital, GermanyAna Petar Vukovic, University of Belgrade, Serbia
Ola B. Al-Batayneh, Jordan University of Science and Technology, Jordan
Copyright © 2021 Hubbard, Perez and Ganss. This is an open-access article distributed under the terms of the Creative Commons Attribution License (CC BY). The use, distribution or reproduction in other forums is permitted, provided the original author(s) and the copyright owner(s) are credited and that the original publication in this journal is cited, in accordance with accepted academic practice. No use, distribution or reproduction is permitted which does not comply with these terms.
*Correspondence: Michael J. Hubbard, bWlrZS5odWJiYXJkJiN4MDAwNDA7dW5pbWVsYi5lZHUuYXU=