- 1Kalms Consulting GmbH, Berlin, Germany
- 2Institute for Theoretical Biology (ITB), Charité—Universitätsmedizin Berlin, Corporate Member of Freie Universität Berlin, Humboldt-Universität zu Berlin and Berlin Institute of Health, Berlin, Germany
- 3Molecular Cancer Research Center (MKFZ), Medical Department of Hematology, Oncology, and Tumour Immunology, Charité—Universitätsmedizin Berlin, Corporate Member of Freie Universität Berlin, Humboldt-Universität zu Berlin and Berlin Institute of Health, Berlin, Germany
- 4Institute for Systems Medicine and Faculty of Human Medicine, MSH Medical School Hamburg, Hamburg, Germany
- 5Fraunhofer Institute for Software and Systems Engineering ISST, Dortmund, Germany
Circadian medicine, the study of the effects of time on health and disease has seen an uprising in recent years as a means to enhance health and performance, and optimize treatment timing. Our endogenous time generating system -the circadian clock- regulates behavioural, physiological and cellular processes. Disruptions of the clock, via external factors like shift work or jet lag, or internal perturbations such as genetic alterations, are linked to an increased risk of various diseases like obesity, diabetes, cardiovascular diseases and cancer. By aligning an individual's circadian clock with optimal times for performing daily routines, physical and mental performance, and also the effectiveness of certain therapies can be improved. Despite the benefits of circadian medicine, the lack of non-invasive tools for characterizing the clock limits the potential of the field. TimeTeller is a non-invasive molecular/digital tool for the characterization of circadian rhythms and prediction of daily routines, including treatment timing, to unlock the potential of circadian medicine and implementing it in various settings. Given the multiple known and potentially yet unknown dependent health factors of individual circadian rhythms, the utility of this emerging biomarker is best exploited in data driven, personalized medicine use cases, using health information across lifestyle, care, and research settings.
Introduction
Humans are a rhythmic species that evolved a time-keeping mechanism which allows them to adapt to environmental cycles, including day-night and feeding-fasting cycles, and to better anticipate opportunities and challenges—the circadian clock. The human circadian clock regulates a number of cellular and molecular mechanisms and plays a vital role in maintaining human health (1). Around 50% of human genes follow 24 h rhythms (circadian) in their expression in at least one tissue (2). Disruption of circadian rhythms (CR) is associated with diseases including sleep disorders, depression, diabetes, neurodegenerative diseases, obesity and cancer (3). Disruption may be caused by conflicting external or internal signals that are not in synchrony with the internal circadian time (4). Almost all biological functions are ruled by and follow a CR (5). Prominent examples are sleep–wake and feeding–fasting cycles, as well as cognitive performance, body temperature, insulin sensitivity, glucose metabolism, hormone secretion and blood pressure. The ultimate goal of such temporal organization is to keep us in balance and in synchrony with the changing environment.
The Nobel Prize for Physiology or Medicine was awarded in 2017 for the discoveries made in the past decades on the genetic and molecular mechanisms of the biological clock (5–11). Even though mounting evidence points to a crucial role of the biological clock in the proper functioning of the organism, the application of this knowledge to the maintenance of health and treatment optimization is still scarce. Published studies have shown that timing treatment based on the circadian rhythm can have a significant impact on the efficacy and toxicity of anti-cancer treatments. This approach is commonly referred to as circadian medicine or chronotherapy. The concept of chronotherapy in cancer is at least partially based on the fact that cancer cells have different circadian rhythms compared to normal cells (12, 13). Chronotherapy aims to exploit these differences in circadian rhythms by administering treatments at times when cancer cells are most vulnerable, and healthy cells are least vulnerable, thereby reducing toxicity and enhancing efficacy. Several studies reported significant reduction in side effects, such as nausea, vomiting, and fatigue and an increase in effectiveness of the treatment, leading to better outcomes and increased survival (14).
Despite the apparent benefits of circadian medicine, the lack of available non-invasive tools for characterizing the clock in humans limits the practical application of this field (15). The pattern of CR shows large variations and relies on several factors including: genetics, age (16), sex hormones (17), and light exposure depending on the intensity, duration, and timing of light applied (18). In the following, we provide an overview of the relevance of CRs for health maintenance and treatment optimization, as well as on the recent efforts made to develop experimental and computational tools for the characterization of CRs (Figure 1A). Finally, we will focus on a recent tool that our group has developed, which allows to profile and monitor CRs in humans and discuss its potential applications in the circadian medicine field and optimization of health. With the development of smart mobile devices and most recently wearable smart devices, biomarker and biofeedback-based lifestyle and health applications emerged, gained traction and are by now a given for significant parts of the population. This spurs a development of more granular, lower threshold health and disease management solutions and services that expand the digital health spectrum beyond remote patient monitoring. Telehealth services focused on a small number of high burden diseases to multivariate data driven approaches to personalized health and treatment management that blend a variety of person/patient generated health data.
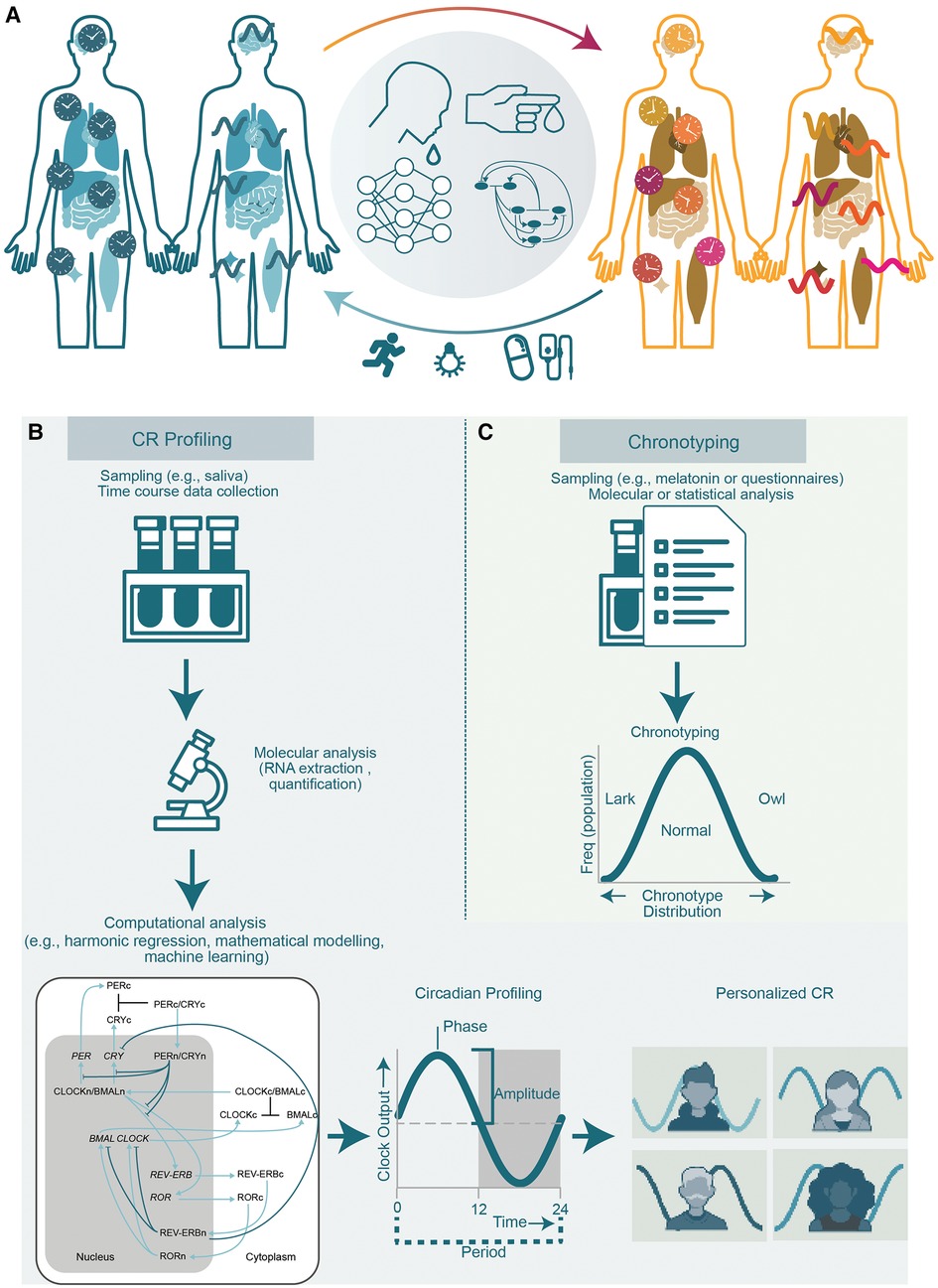
Figure 1. The circadian system and methods for determining CRs and chronotyping. (A) The role of the circadian clock in health and disease. A proper functioning clock ensures correct timing of physiological events such as sleep/wake cycles and contributes to sustain a healthy life (left). The disruption of CRs (right) is associated with numerous diseases including sleep disorders, neurodegenerative diseases, and cancer. Various circadian relevant clinical applications are being studied and implemented to diminish the negative effects of circadian disruption e.g., by the usage of zeitgebers like bright light therapy or physical exercise to reset the clock, and timely administration of drug compounds. For this purpose, methodologies (centre) using molecular data retrieved from time course collection of biological samples for e.g., from blood or saliva in combination with computational and mathematical modelling of the clock network are needed to generate a personalized profile of the CR. (B) CR profiling by repetitive and representative sampling, molecular and computational analysis to generate a personalized circadian profile. (C) Molecular analysis of biological samples or questionnaires and correlation to populational data for typification of an individual into chronotypes.
The endogenous biological clock
A proper functioning clock ensures the timely regulation of crucial physiological processes such as hormonal activity, core body temperature, sleep/wake cycles, immunological activity, and metabolism (19). At the molecular level, the mammalian circadian clock consists of transcriptional and translational interlinked feedback loops of 14 elements including positive regulators (CLOCK, BMAL, ROR), and transcriptional repressors (PERs, CRYs, REV-ERB) (20), resulting in a self-sustained oscillatory system. This 24 h rhythmic oscillation regulates the expression of various target genes also known as clock-controlled genes. In the main pacemaker, the SCN (suprachiasmatic nucleus), located in the brain, the expression of the gene BMAL1 (also knowns as ARNTL) peaks around the middle of the active phase typically mid-day, and the expression of the gene PER2 peaks around the middle of the resting phase, in the middle of the night, with a 12 h-shift to the peak of BMAL1 (21). In human peripheral tissues, genes of the PER family peak around dawn in humans while results for BMAL1 vary heavily across studies suggesting a higher variation than observed for PER2 expression patterns.
The CR is generally stable over time and only adapts to seasonal changes due to the fluctuation in light exposure (22) or can be disturbed by profound and lasting fluctuations of daily routines. While the CR is robust to alterations like temperature fluctuations, the re-alignment of the CR to a new-time zone is not immediate and may require several days to adjust commonly referred to as jet lag (23). Today, with the help of extensive research at the molecular level, it is known that the CR does not simply regulate sleep-wake cycles, but also influences numerous cellular processes, including DNA damage response, metabolism, and cell proliferation (24). This knowledge allows for a range of potential clinical applications including optimization of daily activities such as physical exercise, or light exposure, and therapeutic administration of drugs considering the CR of the individual and the drug (Figure 1A).
Circadian regulation, dysregulation and adjustment: time matters in health and disease
The circadian clock influences and times important functions in our bodies, including hormone release, eating schedules and digestion, body temperature and sleep. In humans, the clock can be adjusted by various external signals. These timing cues, also called zeitgebers (external or environmental cue that synchronizes the circadian clock), can reset the circadian clock. The timely usage of zeitgebers during the day allows for the maintenance or reestablishment of a robust CR and improves health and quality of life (25). The multitude of dependent health effects and the high impact of the CR on everyday life warrants a digital twin approach for people to understand and give them control over utilization of the CR and (potentially) dependent data. As there is not just one CR, but different individuals and health vs. disease status express different rhythm patterns, the generation of computational models of its interdependence with lifestyle, health and care effects will evolve over time. Extending beyond sensor-based measurements of common bio-signals like pulse, blood oxygenation, motion and derived measures, CRs will likely be included in future parameter sets of personal health and fitness dashboards as for e.g., included in common smart device platforms. Users, no matter if they are just interested people or patients under treatment, can be provided with CR data based on initial and potentially repeated individual calibration from for e.g., saliva sampling or wearable sensing. Service providers that can be healthcare providers, payers, or health IT solution/device/drug vendors or combinations of these can offer research, lifestyle, or clinical decision support services for e.g., based on computational models that correlate personal health events and signals, treatment (scheduling) data and CR for upstream evidence generation and downstream clinical decision support. In the following, we provide a brief overview of physiological and behavioural aspects that are CR-dependent (sleep, aging) and/or influence the CR (light, meal times, exercise) and can thus be used as zeitgebers, to enhance or even shift the CR, in case of a dysregulation.
Circadian rhythms and aging
An interesting, and particularly relevant application of enhancing the CR relates to aging. Aging is characterized by a continuous decline in physiological and molecular processes (e.g., endocrine, behavioural and locomotor activities) and results in increased susceptibility to aging-related diseases such as neurodegenerative diseases and cancer (26, 27). Aging may lead to circadian alterations accompanied by sleep disturbances, and reduced cognitive performance (28). Pre-clinical studies on aging animal models have shown a decline in the phase and amplitude of core clock genes such as PER2 and BMAL1. Thus, a healthy CR may exert profound influences on whole-body rejuvenation and the appropriate usage of zeitgebers may support it. Stimuli including social interaction, physical exercise, stress and caffeine can all act as clock synchronizers in the body (29).
Circadian regulation of sleep
The circadian system regulates the timing of sleep and wake up and aligns the human physiology with it. During sleep several important functions take place including clearance of toxins, cellular repair, memory consolation and information processing (30). Furthermore, sleep has been shown to improve the mood, alertness and concentration, boost the immunity and reduce the risk of other chronic diseases such as heart problems (31). Continuous exposure of the CRs to conflicting zeitgebers like artificial light for example due to night-shift work or jet lag can result in disruption of sleep thereby affecting these processes (32).
Light therapy against circadian dysregulation
Light is one of the strongest zeitgebers, which can align the period of two or more oscillators (for example, the SCN to the environmental light/dark cycles) a process known as entrainment (33). Unlike entrainment, synchronization does not require oscillators, but rather aligns the phases throughout the body. Environmental light is perceived via intrinsically photosensitive retinal ganglion cells (ipRGCs) in the retina. The information is then transmitted to the central pacemaker in the brain (SCN), entraining it to a 24 h solar day. Subsequently this information is transmitted to the rest of the body via humoral and autonomic nervous system signals. Mistimed light exposure may lead to misalignment of CRs and negatively impact human health, being associated to mood and sleep disorders, neurodegenerative diseases or cancer (34). In recent years, light therapy has been increasingly implemented as an assisting/supportive therapy for various medical conditions, from depression and sleep disorders (35) to cancer (36). For cancer patients, light therapy has proven to help overcome side effects of the treatment such as treatment-related fatigue. Recently, new applications of light therapy are emerging in internal medicine, especially relevant in intensive care units where artificial light differences between day and night can be minimal. This may result in patients developing a fragmented sleep-wake cycle with a negative impact on their recovery and wellbeing, or even cause delirium (37).
Feed/fasting cycles as synchronizers of the circadian clock
Also feed/fasting regimes can be used to synchronize the circadian clock (35). Biological processes like metabolism are closely linked to our CR (38). Meal time is an important factor leading both to circadian disruption or regulation. Peripheral CRs, like in the liver, are more profoundly affected by the timing of food intake than the timing of light (39). Previous studies on humans and animal models suggested that the consumption of a larger portion of caloric intake during the first half of wakeful hours may be preferred for better blood glucose regulation and weight control (reviewed in (40)). In addition, caloric restriction that involves time-restricted feeding of less than 6 h has been suggested to improve longevity in mammals (41, 42). Therefore, consolidating eating periods to defined intervals (so-called time-restricted eating) is another behavioural intervention that can support robust CRs in peripheral organs and improve health.
Circadian dependence of physical activity
Finally, also physical exercise is both influenced by the clock and can act as a clock enhancer and synchronizer. Several physiological features which influence sports performance show circadian variation, including core body temperature, hormone levels, blood pressure, and heart rate variability (43). Core-clock genes (e.g., BMAL1), influence skeletal muscle function. Physical exercise is an important enhancer of the CRs. Several studies point to a daily variation of exercise performance. Our group also previously reported a correlation between PER2 peak timing and strength performance whereas amplitude of BMAL1 seems to correlate with overall daily variation of exercise performance (44). Thus, the personal CR can be used to determine the variation of athletic performance throughout the day and predict the optimal time window for peak athletic performance. The extent and duration of the effect depends on the intensity and duration of the exercise (45).
Regulation of circadian rhythms
A well-functioning circadian clock, in synchrony with an individual's behavioural habits, can improve general health and wellbeing, will improve overall fitness, and reduce recovery or therapy time in patients. To avoid or overcome CR disruption, a person's individual internal rhythm can be profiled and used to adjust relevant external/internal factors. The efficiency of activities such as sleep, sports, or medicine intake can be optimized based on the internal timing. Interventions such as light therapy, exercise, and shifting the individual rhythm pharmacologically (for example with melatonin supplements) have been used in recent research to revert the effects of disrupted CRs (46). A disruption of normal CRs (e.g., due to constant shift work or genetic disruption of clock mechanism) has been shown to negatively affect an individual's wellbeing, metabolism, physical and cognitive performance and may lead to disease including sleep disorders, obesity, depression, neurodegeneration and cancer as it facilitates tumorigenesis and the establishment of cancer hallmarks (47, 48). Cancer cells can be arrhythmic, rhythmic in-phase or rhythmic out-of-phase with healthy tissues (14, 49) (e.g., metastatic spread of breast cancer accelerates during sleep) (50). Altered circadian rhythmicity in cancer cells allows for chronotherapy with the following rationale: identification of a timepoint of lowest toxicity to non-cancer cells, at which the maximum tolerated dose can be administered to impact tumour cells, while sparing healthy cells, for a given patient patient. The severity of side effects could be reduced by CR-based treatment schedule optimization. A proof of principle was recently published by our group showcasing a mathematical model of the circadian clock and drug pharmacology to optimize irinotecan administration timing in colorectal cancer to reduce its toxicity (51). Proper timing of chemotherapie to increase drug-tolerability is particularly relevant for anticancer drugs, as they are often administered close to their maximum-tolerated dose (52, 53).
Measuring CR: molecular and physiological measurements
Various approaches can be used to investigate circadian rhythmicity in humans both in research, as well as in a clinical context. However, a clear distinction of their 1) information value (a) CR vs. outputs of the CR; b) chronotyping vs. CR profiling) and 2) usability are extremely relevant to consider (see Table 1).
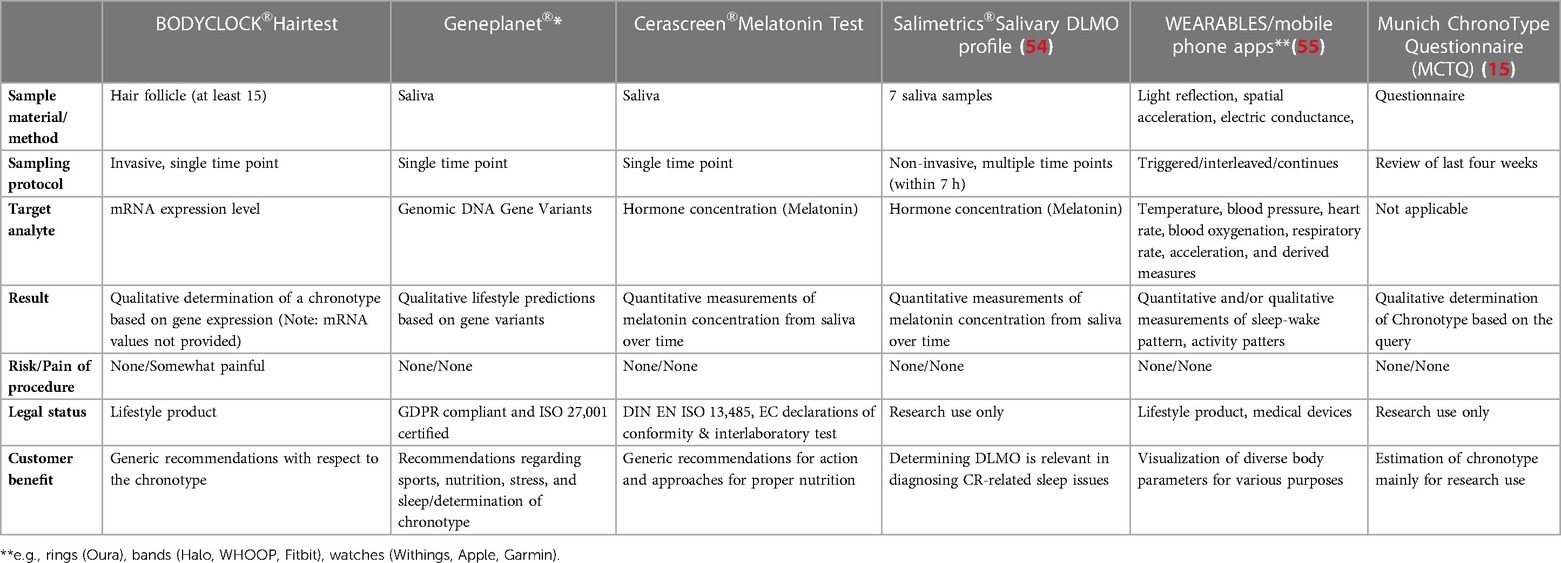
Table 1. Selection of commercial and scientific tools for circadian clock assessment in humans. *Sleep recommendations.
1a) Measurements of cortisol, analysis of genomic DNA or transcriptome, often are invasive and measure single time points to assess chronotypes (e.g., early bird vs. night owl) (54). Generic chronotherapy (e.g., morning vs. evening drug application) considers neither the CR of the individual nor of the drug activity. 1b) Core body temperature, rating scales such as morningness-eveningness questionnaire or actigraphy (15) (e.g., via rings (Oura), bands (Halo, WHOOP) or watches (Withings)) measure outputs of the CR not the CR itself (56). Thus, the approaches described under 1a) or 1b) are not suitable for individualised chronotherapy. 2) Methods like the Dim Light Melatonin secretion Onset time (DLMO) profiling often require an overnight stay at the clinic, are time-consuming and interfere with the sleep time of the test person, and are thus unrealistic for routine clinical use (57).
There is currently no user-friendly (non-invasive, cost-effective) and practicable method on the market (lifestyle or research use only (RUO) products) that would allow to model the CR based on the expression of core-clock genes (57) and thus be useful to advance the field of circadian medicine.
Wearable device-based measurements and derived parameters and models have evolved from specific purpose devices and platforms to multi-purpose devices, platforms and ecosystems. Whether wearable devices have the potential to accurately and consistently capture changes in the CR over time, remains to be demonstrated as wearables can only measure the outputs of the CR like body temperature, heart rate and activity, but not the underling factors like the circadian gene and protein expression network that generates these circa 24 h rhythms. One of the benefits of wearable devices is that they provide continuous and long-term monitoring of CR outputs, which may reveal alterations that might not be apparent during shorter periodic measurements of genes and protein expression.
To date, the most prevalent smart device OS platforms, Google Android and Apple iOS offer health data capture, exploration, management, integration and utilization capabilities through Google Fit and Apple Health that interface with a breadth of vendors and signal modalities. In addition, interoperability platforms and services exist that facilitate combination of signal and device modalities beyond single platform integration. The future will likely bring open, trusted, federated, interoperable and data sovereign personal health data ecosystems, as mandated in the EU by the European Health Data Space regulation. Already today, individuals can choose from a variety of apps and wearable devices to monitor combinations of bio-signals and obtain analytic insights into sleep quality in correlation to habits, as well as derived personal lifestyle and health advice as to optimize sleep quality, for instance.
The need for a non-invasive in-vitro diagnostic for CR-profiling: TimeTeller
To allow for CR consideration a specific tool is needed that ideally is 1) non-invasive to allow easy access without ethical concerns, 2) user-friendly so that no specialized medical personnel is required, 3) can be performed at home to keep the time commitment for participants low and increase compliance, 4) provides informative output with recommendations, which are simple and easy to follow, 5) allows for monitoring via repetitive analysis, 6) ideally certified and approved for medical purposes to be accessible not only for research, but also in the healthcare sector.
In the following we describe “TimeTeller” as an in vitro diagnostic (IVD) tool with the possibility to integrate CR profiling in any scientific field and question (Figure 2). The TimeTeller IVD allows to profile the individual CR in a non-invasive and user-friendly way, which can be carried out at home and does not require neither medical supervision nor assistance. It is based on saliva samples, which are collected over a period of 48 h, only during daytime with a four-hour sampling interval, in a total of 8 samples (44, 51). The collected saliva samples can be kept at room temperature for a few days (or 4 °C for several weeks) for subsequent molecular analyses (Figure 2A).
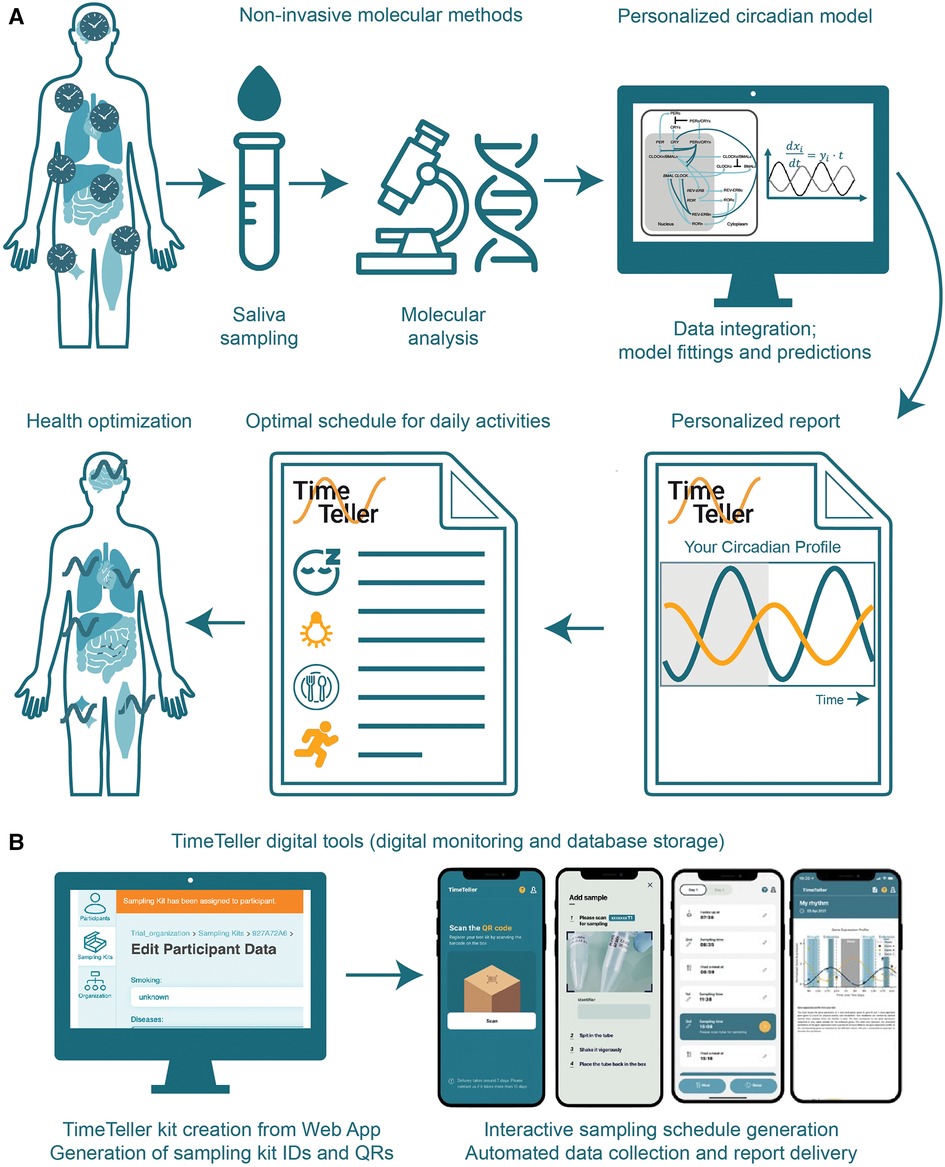
Figure 2. TimeTeller: a non-invasive method for profiling the CR and prediction of optimal times for daily activities. (A) TimeTeller pipeline for saliva collection at defined times, molecular and computational analysis and report generation with circadian profile and recommendations to optimize health. (B) Digital tools to manage sampling logistics (left) and digital patient support to increase compliance (right).
Molecular characterization of the expression of core-clock genes (e.g., BMAL1, PER2) are used as inputs for profiling and modelling the circadian clock using computational algorithms suitable to analyse circadian time series data such as harmonic regression and mathematical models (Figure 1B) (44). The profile of a person's CR defined by amplitude, period, phase, mean level (mesor) can thus be generated by measuring gene activity via RNA quantification in saliva samples and using computational circadian models (44). However, the accuracy of saliva-based methods can be affected by factors such as the timing of sampling, the consumption of food and drinks, and the use of medication.
For more detailed predictions for example, related to the determination of cytotoxicity times for a certain drug and patient, mathematical models based on ordinary differential equations can be used to mathematically represent the molecular processes such as transcription, translation, post-translational regulations and degradation processes of the core-clock network (Figure 2A) integrated in the metabolic network of a given drug (51). The addition of recommendations for sleeping, exercise and meal times provides a holistic picture of the personalised CR (1, 51, 58). Additional web and mobile applications allow for a followup of the sampling collection and processes steps and support patient compliance by generation sampling reminders (Figure 2B).
Significant changes to daily routines for example the disruption of sleep patterns due to jetlag or light exposure, will be reflected in the CR profile. Furthermore, perturbations or misalignment of the CR and daily routines that may manifest among others in sleep disorders can be identified (23).
In comparison to the previously available methods for circadian clock assessment (Figure 1C and Table 1), the TimeTeller tool is more comprehensive since it provides a quantified circadian profile characterised by an amplitude, peak and phase based on gene expression data of selected clock genes. Furthermore, it is non-invasive and does not require medical assistance. In addition, the informative profile of individual CRs have several, highly relevant, future health-related clinical and non-clinical applications like 1) monitoring disease progression (bio-marker), 2) optimizing medical treatment timing according to drug targets and 3) optimizing performance/efficiency by improving sports performance, or sleep quality. Hence TimeTeller may be applied to optimize treatment timing in patients, as well as for optimizing physical and cognitive performance in healthy individuals.
Applications from health prevention to treatment timing
Based on CR-profiles determined by TimeTeller, computational tools can generate personalized predictions relevant to health, wellbeing and physical performance, as well as drug metabolism and ultimately suggest optimal times for daily activities like sleep (59), sports (44), light exposure, and medicine intake (1) (Figure 1).
TimeTeller can be used to profile the CR and identify perturbations or misalignments of the CR not only in healthy (44), but also in individuals suffering from different types of pathologies e.g., Parkinson's disease (60–62), type 2 diabetes (63), cancer (36, 64–66), depression (67). Based on the profiled CR, behavioural recommendations related to the timing of feeding-fasting (68), physical exercise (69), sleep-wake (70–72), or light-dark cycles (18), can be provided to re-align the CR with the environment of the individual. Changes to CR in response to sleep-(71, 72) and meal-timing (68) can be monitored via core-clock gene expression using the TimeTeller technology.
This increases well-being and diminishes certain symptoms that are typically associated with the above-mentioned diseases and/or their treatment: fatigue, sleep disorders and CRs disorders. These symptoms of circadian dysregulation are recognized as a disease by the International Statistical Classification of Diseases and Related Health Problems 10th Revision under (ICD-10-CM Code: G47: Sleep disorder/G47.20: CR sleep disorder). This is particularly relevant as the CR differs for each of us and generic recommendations do not take individual variations into account (17, 59).
Clinical application of CRs: drug development
The overall failure rate in drug development is 96%, and 90% or higher, during clinical development (73). High attrition rates can be seen in every phase of drug development or therapeutic area and have remained at a similar level over the last few decades (74). 40%–50% of compounds fail due to lack of clinical efficacy while 30% show unmanageable toxicity (73). Expanding the drug developmental scope by considering the dimension “time” has the potential to significantly improve these statistics (48).
Chronotherapy uses the circadian rhythmicity (CR-dependencies) of cellular processes in the administration of treatment to maximize effectiveness and minimize side effects in drug development and treatment. To this end, treatments for a broad range of medical conditions and their links to the CRs have been investigated including, but not limited to: allergy, arthritis, asthma, hyperlipidaemia, hypertension, cancer, and neurodegeneration (48). The therapeutic relevance of the circadian clock gained an increased attention in recent years. One reason being that 56 of the top 100 best-selling, FDA approved drugs in the United States, target genes that are expressed in a circadian manner, including all top 7 best-selling drugs (USA) (75). This is expected as a total of 43% of all protein-coding genes show CRs (75). Additionally, nearly 50% of the top 100 drugs also have a half-life time of less than 6 h, an important requirement to manage the drug concentration in the body in a timely defined manner (75). Of note, a study that screened over 1,000 molecules from an FDA-approved drug library found that approximately 5% of the drugs screened altered the circadian period (76). However, currently, the CR does not play an adequate role in drug development, even though the attrition rate, defined as the loss of new candidate drugs during the process from pre-clinical to clinical and through their clinical development, is on a high level. Multiple phase III clinical trials testing chronotherapy vs. conventional treatment schedules showed an improved anticancer treatment tolerability of up to fivefold and a nearly double efficacy in experimental studies (77, 78). Similar chronopharmacological effects have been shown for various drugs (77). A recent systematic review reported that 61% of analysed studies described significant decrease in toxicity of chemotherapy (14). Considering this body of knowledge, the CR has the potential to become an essential clinical parameter in drug development to reduce high attrition rates.
Along with the development of stratified, precision, or even individualized medicine, data driven approaches to drug development, real world evidence generation, and clinical decision support emerge and will shorten cycles, as well as improve targeting of selection, dosing, and potentially even timing based on chronobiology. Data ecosystems that span people, care providers, and in some constellations pharma and/or payers will scale up such that real time treatment management becomes available to everyone in need, while safeguarding data utilization within the stated purpose, by the authorized roles and for the agreed period. Dynamic and adaptive treatment schedules that make time-dependent interactions between drugs, individual physiology, and diseases manageable for individuals and their care providers will gradually replace the “x times daily” type of current intake schedules.
Discussion
With the recent advances in methods for molecular analysis techniques and the development of digital tools, circadian considerations are expected to become an integral part of healthcare and disease management. By focusing on the internal biological clock, which governs the timing of everyday behaviours, circadian medicine has the potential to revolutionize the way we approach healthcare.
A combination of molecular analysis and digital tools can be used to characterize and monitor the CR, but there is still a need to develop user friendly tools that can make this process easier. In the future, tools like TimeTeller, which allow for a full characterization of the CR, will allow to adjust daily routines to optimize health and improve treatment efficacy. The CR specifically warrants research into how an initially identified CR pattern will be carried forward using continuous or spot check measurements, and into hypothesis generation and validation through interoperable, transparent and data sovereign utilization of CR and associated data across lifestyle, care, and research settings using machine learning approaches.
Conclusion
As personalized medicine continues to advance, it is essential for clinicians and researchers to recognize the significance of the CR and to have tools available to measure and monitor it, both to detect possible CR dysregulations that can act as a potential biomarker for diagnostics, and to design individualized treatment plans tailored to the specific needs of each patient. For example, lifestyle modifications such as altering timings of meals, and physical exercise may be recomended to a patient with sleep disorders to achieve a healthier sleep cycle. Similarly, adjusting the patients' treatment administration schedule based on their CR may enable a reduction of side effects and an increase in treatment efficacy. The future of personalized medicine looks bright, and circadian medicine has the potential to make a real difference in patients' lives. Time matters and it's time to use it!
Data availability statement
The original contributions presented in the study are included in the article/Supplementary Material, further inquiries can be directed to the corresponding authors.
Ethics statement
Ethical review and approval was not required for this study in accordance with the local legislation and institutional requirements.
Author contributions
AR conceptualized the work, attained the funding, carried out the investigation related to TimeTeller, prepared the initial draft, reviewed and revised the manuscript. BD prepared the initial draft, reviewed and revised the manuscript. MY carried out the investigation related to TimeTeller, visualization, reviewed and revised the manuscript. SD reviewed and revised the manuscript. All authors contributed to the article and approved the submitted version.
Funding
The work in the group of AR was funded by the Dr. Rolf M. Schwiete Stiftung. MY was additionally funded by the Berlin School of Integrative Oncology (BSIO) graduate program of the Charité Medical University Berlin and by the Einstein Foundation. The TimeTeller project was additionally funded by the I4H (Inventors for Health) Program of the Stiftung Charité, and by the Digital Health Accelerator (DHA) Program from the Berlin Institute of Health (BIH) at the Charité Medical University of Berlin (grants to AR). The funders played no role in study design, data collection, analysis and interpretation of data, or the writing of this manuscript.
Acknowledgments
We are grateful to all members of Relógio group involved in the TimeTeller project, as well as to Marvin Stolz and Craig Garner, from the I4H Program of the Stiftung Charité, Dorothée Marie-Louise Doepfer and Tim Huse from the Digital Health Accelerator Program of the BIH for critical discussion. Parts of the illustrations used in the figures have been adapted from the TimeTeller project webpage (www.time-teller.eu), in addition some panels contain modified versions of vector icons obtained from Flaticon (https://www.flaticon.com/).
Conflict of interest
AR has filled patents (PCT/EP2021/073798 and PCT/EP2021/073802) related to TimeTeller and biomarkers for characterizing the circadian clock in humans. BD works for Kalms Consulting GmbH. SD works for Fraunhofer Institute for Software and Systems Engineering ISST. TimeTeller was recently founded as a spin-off of the Charité Medical University of Berlin. MY declares that the research was conducted in the absence of any commercial or financial relationships that could be construed as a potential conflict of interest.
Publisher's note
All claims expressed in this article are solely those of the authors and do not necessarily represent those of their affiliated organizations, or those of the publisher, the editors and the reviewers. Any product that may be evaluated in this article, or claim that may be made by its manufacturer, is not guaranteed or endorsed by the publisher.
References
1. Hesse J, Malhan D, Yalçin M, Aboumanify O, Basti A, Relogio A. An optimal time for treatment-predicting circadian time by machine learning and mathematical modelling. Cancers (Basel). (2020) 12(11):3103. doi: 10.3390/cancers12113103
2. Ruben MD, Wu G, Smith DF, Schmidt RE, Francey LJ, Lee YY, et al. A database of tissue-specific rhythmically expressed human genes has potential applications in circadian medicine. Sci Transl Med. (2018) 10(458):eaat8806. doi: 10.1126/scitranslmed.aat8806
3. Montaruli A, Castelli L, Mule A, Scurati R, Esposito F, Galasso L, et al. Biological rhythm and chronotype: new perspectives in health. Biomolecules. (2021) 11(4):487. doi: 10.3390/biom11040487
4. Potter GD, Skene DJ, Arendt J, Cade JE, Grant PJ, Hardie LJ. Circadian rhythm and sleep disruption: causes, metabolic consequences, and countermeasures. Endocr Rev. (2016) 37(6):584–608. doi: 10.1210/er.2016-1083
5. Vosshall LB, Price JL, Sehgal A, Saez L, Young MW. Block in nuclear localization of period protein by a second clock mutation, timeless. Science. (1994) 263(5153):1606–9. doi: 10.1126/science.8128247
6. Zehring WA, Wheeler DA, Reddy P, Konopka RJ, Kyriacou CP, Rosbash M, et al. P-element transformation with period locus DNA restores rhythmicity to mutant, arrhythmic Drosophila melanogaster. Cell. (1984) 39(2 Pt 1):369–76. doi: 10.1016/0092-8674(84)90015-1
7. Bargiello TA, Jackson FR, Young MW. Restoration of circadian behavioural rhythms by gene transfer in Drosophila. Nature. (1984) 312(5996):752–4. doi: 10.1038/312752a0
8. Siwicki KK, Eastman C, Petersen G, Rosbash M, Hall JC. Antibodies to the period gene product of Drosophila reveal diverse tissue distribution and rhythmic changes in the visual system. Neuron. (1988) 1(2):141–50. doi: 10.1016/0896-6273(88)90198-5
9. Hardin PE, Hall JC, Rosbash M. Feedback of the Drosophila period gene product on circadian cycling of its messenger RNA levels. Nature. (1990) 343(6258):536–40. doi: 10.1038/343536a0
10. Liu X, Zwiebel LJ, Hinton D, Benzer S, Hall JC, Rosbash M. The period gene encodes a predominantly nuclear protein in adult Drosophila. J Neurosci. (1992) 12(7):2735–44. doi: 10.1523/JNEUROSCI.12-07-02735.1992
11. Price JL, Blau J, Rothenfluh A, Abodeely M, Kloss B, Young MW. Double-time is a novel Drosophila clock gene that regulates PERIOD protein accumulation. Cell. (1998) 94(1):83–95. doi: 10.1016/S0092-8674(00)81224-6
12. Relogio A, Thomas P, Medina-Perez P, Reischl S, Bervoets S, Gloc E, et al. Ras-mediated deregulation of the circadian clock in cancer. PLoS Genet. (2014) 10(5):e1004338. doi: 10.1371/journal.pgen.1004338
13. Zhou L, Zhang Z, Nice E, Huang C, Zhang W, Tang Y. Circadian rhythms and cancers: the intrinsic links and therapeutic potentials. J Hematol Oncol. (2022) 15(1):21. doi: 10.1186/s13045-022-01238-y
14. Printezi MI, Kilgallen AB, Bond MJG, Stibler U, Putker M, Teske AJ, et al. Toxicity and efficacy of chronomodulated chemotherapy: a systematic review. Lancet Oncol. (2022) 23(3):e129–e43. doi: 10.1016/S1470-2045(21)00639-2
15. Ruan W, Yuan X, Eltzschig HK. Circadian rhythm as a therapeutic target. Nat Rev Drug Discov. (2021) 20(4):287–307. doi: 10.1038/s41573-020-00109-w
16. Cain SW, Dennison CF, Zeitzer JM, Guzik AM, Khalsa SB, Santhi N, et al. Sex differences in phase angle of entrainment and melatonin amplitude in humans. J Biol Rhythms. (2010) 25(4):288–96. doi: 10.1177/0748730410374943
17. Anderson ST, FitzGerald GA. Sexual dimorphism in body clocks. Science. (2020) 369(6508):1164–5. doi: 10.1126/science.abd4964
18. Te Kulve M, Schlangen LJM, van Marken Lichtenbelt WD. Early evening light mitigates sleep compromising physiological and alerting responses to subsequent late evening light. Sci Rep. (2019) 9(1):16064. doi: 10.1038/s41598-019-52352-w
19. Ayyar VS, Sukumaran S. Circadian rhythms: influence on physiology, pharmacology, and therapeutic interventions. J Pharmacokinet Pharmacodyn. (2021) 48(3):321–38. doi: 10.1007/s10928-021-09751-2
20. Takahashi JS. Transcriptional architecture of the mammalian circadian clock. Nat Rev Genet. (2017) 18(3):164–79. doi: 10.1038/nrg.2016.150
21. Hughey JJ, Butte AJ. Differential phasing between circadian clocks in the brain and peripheral organs in humans. J Biol Rhythms. (2016) 31(6):588–97. doi: 10.1177/0748730416668049
22. Laje R, Agostino PV, Golombek DA. The times of our lives: interaction among different biological periodicities. Front Integr Neurosc. (2018) 12:10. doi: 10.3389/fnint.2018.00010
23. Sack RL, Auckley D, Auger RR, Carskadon MA, Wright KP Jr., Vitiello MV, et al. Circadian rhythm sleep disorders: part I, basic principles, shift work and jet lag disorders. An American academy of sleep medicine review. Sleep. (2007) 30(11):1460–83. doi: 10.1093/sleep/30.11.1460
24. Rijo-Ferreira F, Takahashi JS. Genomics of circadian rhythms in health and disease. Genome Med. (2019) 11(1):82. doi: 10.1186/s13073-019-0704-0
25. Campbell PD, Miller AM, Woesner ME. Bright light therapy: seasonal affective disorder and beyond. Einstein J Biol Med. (2017) 32:E13–25.31528147
26. Wang K, Liu H, Hu Q, Wang L, Liu J, Zheng Z, et al. Epigenetic regulation of aging: implications for interventions of aging and diseases. Signal Transduct Target Ther. (2022) 7(1):374. doi: 10.1038/s41392-022-01211-8
27. Tabibzadeh S. Circadiomic medicine and aging. Ageing Res Rev. (2021) 71:101424. doi: 10.1016/j.arr.2021.101424
28. Zhu Y, Liu Y, Escames G, Yang Z, Zhao H, Qian L, et al. Deciphering clock genes as emerging targets against aging. Ageing Res Rev. (2022) 81:101725. doi: 10.1016/j.arr.2022.101725
29. Tahara Y, Aoyama S, Shibata S. The mammalian circadian clock and its entrainment by stress and exercise. J Physiol Sci. (2017) 67(1):1–10. doi: 10.1007/s12576-016-0450-7
30. Krueger JM, Frank MG, Wisor JP, Roy S. Sleep function: toward elucidating an enigma. Sleep Med Rev. (2016) 28:46–54. doi: 10.1016/j.smrv.2015.08.005
31. Walker WH 2nd, Walton JC, DeVries AC, Nelson RJ. Circadian rhythm disruption and mental health. Transl Psychiatry. (2020) 10(1):28. doi: 10.1038/s41398-020-0694-0
32. Archer SN, Laing EE, Moller-Levet CS, van der Veen DR, Bucca G, Lazar AS, et al. Mistimed sleep disrupts circadian regulation of the human transcriptome. Proc Natl Acad Sci U S A. (2014) 111(6):E682–91. doi: 10.1073/pnas.1316335111
33. Dunster GP, Hua I, Grahe A, Fleischer JG, Panda S, Wright KP Jr., et al. Daytime light exposure is a strong predictor of seasonal variation in sleep and circadian timing of university students. J Pineal Res. (2023) 74(2):e12843. doi: 10.1111/jpi.12843
34. Lunn RM, Blask DE, Coogan AN, Figueiro MG, Gorman MR, Hall JE, et al. Health consequences of electric lighting practices in the modern world: a report on the national toxicology Program's Workshop on shift work at night, artificial light at night, and circadian disruption. Sci Total Environ. (2017) 607-608:1073–84. doi: 10.1016/j.scitotenv.2017.07.056
35. van Maanen A, Meijer AM, van der Heijden KB, Oort FJ. The effects of light therapy on sleep problems: a systematic review and meta-analysis. Sleep Med Rev. (2016) 29:52–62. doi: 10.1016/j.smrv.2015.08.009
36. Ancoli-Israel S, Rissling M, Neikrug A, Trofimenko V, Natarajan L, Parker BA, et al. Light treatment prevents fatigue in women undergoing chemotherapy for breast cancer. Support Care Cancer. (2012) 20(6):1211–9. doi: 10.1007/s00520-011-1203-z
37. Schmidt S, Hancke L, Haussmann R, Luetz A. [Chronobiological interventions for prevention and treatment of delirium in critically ill patients]. Nervenarzt. (2022) 93(9):901–11. doi: 10.1007/s00115-022-01348-5
38. Eckel-Mahan K, Sassone-Corsi P. Metabolism and the circadian clock converge. Physiol Rev. (2013) 93(1):107–35. doi: 10.1152/physrev.00016.2012
39. Stokkan KA, Yamazaki S, Tei H, Sakaki Y, Menaker M. Entrainment of the circadian clock in the liver by feeding. Science. (2001) 291(5503):490–3. doi: 10.1126/science.291.5503.490
40. Chaix A, Manoogian ENC, Melkani GC, Panda S. Time-Restricted eating to prevent and manage chronic metabolic diseases. Annu Rev Nutr. (2019) 39:291–315. doi: 10.1146/annurev-nutr-082018-124320
41. Acosta-Rodriguez VA, de Groot MHM, Rijo-Ferreira F, Green CB, Takahashi JS. Mice under caloric restriction self-impose a temporal restriction of food intake as revealed by an automated feeder system. Cell Metab. (2017) 26(1):267–77 e2. doi: 10.1016/j.cmet.2017.06.007
42. Mitchell SJ, Bernier M, Mattison JA, Aon MA, Kaiser TA, Anson RM, et al. Daily fasting improves health and survival in male mice independent of diet composition and calories. Cell Metab. (2019) 29(1):221–8 e3. doi: 10.1016/j.cmet.2018.08.011
43. Teo W, Newton MJ, McGuigan MR. Circadian rhythms in exercise performance: implications for hormonal and muscular adaptation. J Sports Sci Med. (2011) 10(4):600–6.24149547
44. Basti A, Yalcin M, Herms D, Hesse J, Aboumanify O, Li Y, et al. Diurnal variations in the expression of core-clock genes correlate with resting muscle properties and predict fluctuations in exercise performance across the day. BMJ Open Sport Exerc Med. (2021) 7(1):e000876. doi: 10.1136/bmjsem-2020-000876
45. Wolff CA, Esser KA. Exercise timing and circadian rhythms. Curr Opin Physiol. (2019) 10:64–9. doi: 10.1016/j.cophys.2019.04.020
46. Sulli G, Manoogian ENC, Taub PR, Panda S. Training the circadian clock, clocking the drugs, and drugging the clock to prevent, manage, and treat chronic diseases. Trends Pharmacol Sci. (2018) 39(9):812–27. doi: 10.1016/j.tips.2018.07.003
47. Sulli G, Lam MTY, Panda S. Interplay between circadian clock and cancer: new frontiers for cancer treatment. Trends Cancer. (2019) 5(8):475–94. doi: 10.1016/j.trecan.2019.07.002
48. Cederroth CR, Albrecht U, Bass J, Brown SA, Dyhrfjeld-Johnsen J, Gachon F, et al. Medicine in the fourth dimension. Cell Metab. (2019) 30(2):238–50. doi: 10.1016/j.cmet.2019.06.019
49. Basti A, Malhan D, Dumbani M, Dahlmann M, Stein U, Relogio A. Core-Clock genes regulate proliferation and invasion via a reciprocal interplay with MACC1 in colorectal cancer cells. Cancers (Basel). (2022) 14(14):3458. doi: 10.3390/cancers14143458
50. Diamantopoulou Z, Castro-Giner F, Schwab FD, Foerster C, Saini M, Budinjas S, et al. The metastatic spread of breast cancer accelerates during sleep. Nature. (2022) 607(7917):156–62. doi: 10.1038/s41586-022-04875-y
51. Hesse J, Martinelli J, Aboumanify O, Ballesta A, Relogio A. A mathematical model of the circadian clock and drug pharmacology to optimize irinotecan administration timing in colorectal cancer. Comput Struct Biotechnol J. (2021) 19:5170–83. doi: 10.1016/j.csbj.2021.08.051
52. Cassidy J. Chemotherapy administration: doses, infusions and choice of schedule. Ann Oncol. (1994) 5(Suppl 4):25–9.; discussion 9–30. doi: 10.1093/annonc/5.suppl_4.S25
53. Marangolo M, Bengala C, Conte PF, Danova M, Pronzato P, Rosti G, et al. Dose and outcome: the hurdle of neutropenia (review). Oncol Rep. (2006) 16(2):233–48.16820898
54. Lewy AJ, Cutler NL, Sack RL. The endogenous melatonin profile as a marker for circadian phase position. J Biol Rhythms. (1999) 14(3):227–36. doi: 10.1177/074873099129000641
55. Ancoli-Israel S, Cole R, Alessi C, Chambers M, Moorcroft W, Pollak CP. The role of actigraphy in the study of sleep and circadian rhythms. Sleep. (2003) 26(3):342–92. doi: 10.1093/sleep/26.3.342
56. Haffen E. [Measuring circadian rhythm]. Encephale. (2009) 35(Suppl 2):S63–7. doi: 10.1016/S0013-7006(09)75536-8
57. Rahman SA. Are we ready to assess circadian phase at home? Sleep. (2015) 38(6):849–50. doi: 10.5665/sleep.4722
58. Gaspar LS, Alvaro AR, Carmo-Silva S, Mendes AF, Relogio A, Cavadas C. The importance of determining circadian parameters in pharmacological studies. Br J Pharmacol. (2019) 176(16):2827–47. doi: 10.1111/bph.14712
59. Kim DW, Zavala E, Kim JK. Wearable technology and systems modeling for personalized chronotherapy. Curr Opin Syst Biol. (2020) 21:9–15. doi: 10.1016/j.coisb.2020.07.007
60. Yalcin M, Malhan D, Basti A, Peralta AR, Ferreira JJ, Relogio A. A computational analysis in a cohort of Parkinson's Disease patients and clock-modified colorectal cancer cells reveals common expression alterations in clock-regulated genes. Cancers (Basel). (2021) 13(23):5978. doi: 10.3390/cancers13235978
61. Breen DP, Vuono R, Nawarathna U, Fisher K, Shneerson JM, Reddy AB, et al. Sleep and circadian rhythm regulation in early Parkinson disease. JAMA Neurol. (2014) 71(5):589–95. doi: 10.1001/jamaneurol.2014.65
62. Endo T, Matsumura R, Tokuda IT, Yoshikawa T, Shigeyoshi Y, Node K, et al. Bright light improves sleep in patients with Parkinson's Disease: possible role of circadian restoration. Sci Rep. (2020) 10(1):7982. doi: 10.1038/s41598-020-64645-6
63. Jakubowicz D, Wainstein J, Landau Z, Raz I, Ahren B, Chapnik N, et al. Influences of breakfast on clock gene expression and postprandial glycemia in healthy individuals and individuals with diabetes: a randomized clinical trial. Diabetes Care. (2017) 40(11):1573–9. doi: 10.2337/dc16-2753
64. Redd WH, Valdimarsdottir H, Wu LM, Winkel G, Byrne EE, Beltre MA, et al. Systematic light exposure in the treatment of cancer-related fatigue: a preliminary study. Psychooncology. (2014) 23(12):1431–4. doi: 10.1002/pon.3553
65. Wu LM, Amidi A, Valdimarsdottir H, Ancoli-Israel S, Liu L, Winkel G, et al. The effect of systematic light exposure on sleep in a mixed group of fatigued cancer survivors. J Clin Sleep Med. (2018) 14(1):31–9. doi: 10.5664/jcsm.6874
66. Fox RS, Baik SH, McGinty H, Garcia SF, Reid KJ, Bovbjerg K, et al. Feasibility and preliminary efficacy of a bright light intervention in ovarian and endometrial cancer survivors. Int J Behav Med. (2021) 28(1):83–95. doi: 10.1007/s12529-020-09861-0
67. Bunney BG, Li JZ, Walsh DM, Stein R, Vawter MP, Cartagena P, et al. Circadian dysregulation of clock genes: clues to rapid treatments in major depressive disorder. Mol Psychiatry. (2015) 20(1):48–55. doi: 10.1038/mp.2014.138
68. Wehrens SMT, Christou S, Isherwood C, Middleton B, Gibbs MA, Archer SN, et al. Meal timing regulates the human circadian system. Curr Biol. (2017) 27(12):1768–75 e3. doi: 10.1016/j.cub.2017.04.059
69. Facer-Childs ER, Middleton B, Skene DJ, Bagshaw AP. Resetting the late timing of “night owls” has a positive impact on mental health and performance. Sleep Med. (2019) 60:236–47. doi: 10.1016/j.sleep.2019.05.001
70. Maurer LF, Ftouni S, Espie CA, Bisdounis L, Kyle SD. The acute effects of sleep restriction therapy for insomnia on circadian timing and vigilance. J Sleep Res. (2021) 30(4):e13260. doi: 10.1111/jsr.13260
71. Ackermann K, Plomp R, Lao O, Middleton B, Revell VL, Skene DJ, et al. Effect of sleep deprivation on rhythms of clock gene expression and melatonin in humans. Chronobiol Int. (2013) 30(7):901–9. doi: 10.3109/07420528.2013.784773
72. Moller-Levet CS, Archer SN, Bucca G, Laing EE, Slak A, Kabiljo R, et al. Effects of insufficient sleep on circadian rhythmicity and expression amplitude of the human blood transcriptome. Proc Natl Acad Sci U S A. (2013) 110(12):E1132–41. doi: 10.1073/pnas.1217154110
73. Sun D, Gao W, Hu H, Zhou S. Why 90% of clinical drug development fails and how to improve it? Acta Pharm Sin B. (2022) 12(7):3049–62. doi: 10.1016/j.apsb.2022.02.002
74. Kola I, Landis J. Can the pharmaceutical industry reduce attrition rates? Nat Rev Drug Discovery. (2004) 3(8):711–6. doi: 10.1038/nrd1470
75. Zhang R, Lahens NF, Ballance HI, Hughes ME, Hogenesch JB. A circadian gene expression atlas in mammals: implications for biology and medicine. Proc Natl Acad Sci U S A. (2014) 111(45):16219–24. doi: 10.1073/pnas.1408886111
76. Tamai TK, Nakane Y, Ota W, Kobayashi A, Ishiguro M, Kadofusa N, et al. Identification of circadian clock modulators from existing drugs. EMBO Mol Med. (2018) 10(5):e8724. doi: 10.15252/emmm.201708724
77. Dallmann R, Okyar A, Levi F. Dosing-Time makes the poison: circadian regulation and pharmacotherapy. Trends Mol Med. (2016) 22(5):430–45. doi: 10.1016/j.molmed.2016.03.004
Keywords: circadian rhythms, circadian medicine, personalized treatment, health maintenance, performance optimization, diagnostic tools, computational predictive models, TimeTeller
Citation: Dose B, Yalçin M, Dries SPM and Relógio A (2023) TimeTeller for timing health: The potential of circadian medicine to improve performance, prevent disease and optimize treatment. Front. Digit. Health 5:1157654. doi: 10.3389/fdgth.2023.1157654
Received: 2 February 2023; Accepted: 27 March 2023;
Published: 18 April 2023.
Edited by:
Mohamed Elgendi, ETH Zürich, SwitzerlandReviewed by:
Ming Huang, Nara Institute of Science and Technology (NAIST), JapanColin K. Drummond, Case Western Reserve University, United States
Yongbo Liang, Guilin University of Electronic Technology, China
© 2023 Dose, Yalçin, Dries and Relógio. This is an open-access article distributed under the terms of the Creative Commons Attribution License (CC BY). The use, distribution or reproduction in other forums is permitted, provided the original author(s) and the copyright owner(s) are credited and that the original publication in this journal is cited, in accordance with accepted academic practice. No use, distribution or reproduction is permitted which does not comply with these terms.
*Correspondence: Angela Relógio angela.relogio@charite.de angela.relogio@medicalschool-hamburg.de
Specialty Section: This article was submitted to Personalized Medicine, a section of the journal Frontiers in Digital Health