- 1Centre for Human Drug Research (CHDR), Leiden, Netherlands
- 2Leiden University Medical Centre (LUMC), Leiden, Netherlands
- 3Leiden Academic Centre for Drug Research (LACDR), Leiden, Netherlands
The pharmacological activity assessment of novel immunomodulatory drugs in early-stage drug development is challenging as healthy volunteers do not express relevant immune biomarkers. Alternatively, the immune system can be challenged with keyhole limpet hemocyanin (KLH), a suitable antigen for studying adaptive immune responses. This report systemically reviews the KLH challenge in clinical studies focusing on the characterization of the KLH-driven systemic and local immune responses, identification of the KLH-induced biomarkers, and the evaluation of the effect of pharmacological interventions and diseases on the KLH response. A systematic literature review was carried out in PubMed spanning from 1967 to 2022. The systemic humoral KLH responses could be characterized by ELISA after 3 weeks following immunization. For the systemic cellular and molecular immune responses multiple KLH immunizations and the use of novel techniques such as flow cytometry and ELISpot yield optimal results. The objective evaluation of dermal KLH rechallenge allows for more accurate and sensitive quantification of the local response compared to subjective scoring. For the local cellular and molecular assays after KLH dermal rechallenge we also advocate the use of multiple KLH immunizations. Furthermore, oral KLH feeding, age, physical activity, alcohol consumption, stress, as well as certain auto-immune diseases also play a role in the KLH-induced immune response. Importantly, based on the KLH challenges, the effect of (novel) immunomodulatory drugs could be demonstrated in healthy volunteers, providing valuable information for the clinical development of these compounds. This review underlines the value of KLH challenges in clinical studies, but also the need for standardized and well-controlled methodology to induce and evaluate KLH responses.
Systematic Review Registration: https://www.crd.york.ac.uk/prospero/, identifier CRD42022335419
Introduction
The evaluation of pharmacological activity of immunomodulatory investigational drugs in the early phase of clinical development is challenging as healthy volunteers do not express biomarkers related to immunological disorders. A workaround is to challenge the immune system by activating T cells and/or B cells in healthy volunteers (Saghari et al., 2020). Subsequently, the effect of these investigational drugs on the adaptive immune system can be quantified.
Keyhole limpet hemocyanin (KLH) is a suitable immunization antigen for studying the adaptive immune system (Saghari et al., 2020; Swaminathan et al., 2014). KLH is derived from the hemolymph of the marine mollusk, Megathura crenulata, which can be found in the Pacific coastal waters of California and Mexico. Hemocyanins are metalloproteins (copper-containing molecules) with as main function the transport of oxygen within many mollusk species (Harris et al., 1999; Swaminathan et al., 2014). KLH is used extensively as an immunostimulant in clinical research as it drives a strong humoral and cell-mediated immune response, is harmless to human subjects, and is available as a clinical grade product. KLH induces a T cell-dependent response, which makes it an effective agent for studying the effect of novel immunomodulatory drugs on T cell-mediated immunity (Wimmers et al., 2017). KLH was first clinically introduced in 1967 to study the immunocompetence of humans (Swanson and Schwartz, 1967). KLH has proven to be effective in bladder cancer immunotherapy (Lammers et al., 2012; Perabo and Müller, 2004) and is also registered as a treatment modality for the disease. KLH is also used as a carrier protein, as an immunostimulatory challenge agent driving an immune response, or as an adjuvant in cancer vaccines or along with immunomodulatory drugs against autoimmune disorders (Gandhi et al., 2009; Jia et al., 2013; Jurincic-Winkler et al., 2000; Schumacher, 2001; Swaminathan et al., 2014). However, the exact immunological actions are still unknown, KLH doses and regimens are not standardized, and the endpoints to characterize KLH responses have not been optimized. Swaminathan et al. have systematically reviewed the use of KLH in 16 clinical studies (Swaminathan et al., 2014). This review provided an overview of KLH doses, routes of administration, and high-level response monitoring. As sequel, we performed an in-depth systematic review focusing on the KLH response characterization in clinical studies, extending the scope of Swaminathan’s review by inclusion of a significantly larger number of clinical studies, and focusing on 1) the various approaches for characterization of the systemic (humoral and cellular/molecular) and local (planimetric and cellular/molecular) immune response driven by KLH, 2) identification of the most robust biomarkers for monitoring of a KLH response, based on response size and variability, and 3) evaluation of the effect of pharmacological interventions and diseases on the KLH response.
Methods
A systematic literature review was carried out spanning a period from 1967 up to the 20th of February 2022 in PubMed. The systematic review was registered on PROSPERO (identifier CRD42022335419). No systematic review protocol was prepared. Figure 1 provides a schematic outline based on the PRISMA 2020 statement flow diagram for systematic reviews of the search steps for the identification, screening, and inclusion process. The PRISMA 2020 checklist for the systematic review report can be found in the Supplementary Table S1. The execution of the database search, screening and data extraction were all performed by a single author (MS). The search query contained the keywords keyhole limpet hemocyanin, immunotherapy, and response and it encompassed any derivatives from these keywords. The exact search strategy is given in Supplementary Table S2. The outcomes were defined as any immune system response following a KLH challenge subdivided into four categories: systemic humoral response, systemic cellular/molecular response, local planimetric response, and local cellular/molecular response. The outcomes for the evaluation of the effect of pharmacological interventions and diseases on the KLH response were similarly approached and included the presence of at least two groups for comparison (e.g., treatment vs. no treatment or disease vs. no disease). The KLH immunization and the local rechallenge strategy was tabulated per article and included data on the KLH formulation, the use of an adjuvant, the immunization dose, the immunization route, the number of immunizations, the interval between immunizations, and the skin rechallenge dose. The immune system response following KLH immunization was also tabulated per article subdivided into the four categories (systemic humoral response, systemic cellular/molecular response, local planimetric response, and local cellular/molecular response) and included the measurement assay/technique used and the response size and variability per category. Lastly, the outcomes for evaluation of the effect of (pharmacological) interventions and/or diseases on the KLH challenge were also tabulated and included data on the intervention and/or patient population, the comparison between groups examined, and the differences in immune system responses observed between groups.
The initial search resulted in a total of 1,605 records. The titles and abstracts of those records were screened for eligibility. Only the records where KLH was studied in humans were included. The records in which no KLH immunization was performed or no clinical use of KLH was mentioned, no original trial was reported, or only KLH-pulsed cells were used were excluded. Other exclusion criteria were non-English articles and KLH used as a conjugate. A total of 142 records remained, of which 11 articles were not retrievable. The full-text reports of the remaining 131 records were screened for eligibility using the same in- and exclusion criteria. A total of 57 studies were included in this systematic review of which 45 studies were relevant for objectives 1 and 2, and 43 studies for objective 3.
As no validated and widely adopted reference material was available for many of the outcomes the effects were analyzed as the mean fold change compared to baseline. The variability was also reported as the fold change compared to baseline mean. A few outcomes in a couple of studies had no baseline measurement, in these cases the outcomes were analyzed as the mean fold change compared to untreated or placebo instead. For some outcomes, e.g., the local planimetric induration and erythema responses, it was possible to keep the reporting similar to the original article as the methods and reference material used were similar across studies. Furthermore, not many studies had included numbers of the analyses performed in a tabulated form or within the article text, but only had a graphical presentation of the analyses. In these cases, the mean fold change over baseline was estimated from the graphical presentations using graphical software tools. In the majority of the cases only partial information from selected studies was needed for this systematic review, namely the KLH challenge outcomes, therefore the GRADE guidelines could not be implemented and the individual articles could not be graded in their entirety, including the risk of bias.
Results
KLH immunization and rechallenge
KLH has been used in the clinical studies in various formulations, doses, routes of administration, and immunization regimens (number of immunizations and interval). Table 1 displays the use of the KLH challenge model for human studies in which healthy volunteers were immunized with KLH without any other interventions. Figure 2 summarizes the KLH challenge in terms of the formulation, the immunization dose, the immunization route, and the number of immunizations across the studies displayed in Table 1. There are currently two clinical grade KLH formulations available: High Molecular Weight (HMW) and subunit KLH (Harris et al., 1999; Lebrec et al., 2014; Saghari et al., 2020; Swaminathan et al., 2014). HMW-KLH is the native KLH protein consisting of multiple subunits with a size of approximately 4–8 MDa. Each subunit has a size of approximately 350–390 kDa. HMW-KLH was used in 30 out of 45 studies and subunit KLH in 14 out of 45 studies (Table 1 and Figure 2). A study performed by Miller et al. used both KLH formulations (Miller et al., 2005). They compared three different KLH formulations; HMW-KLH, subunit KLH, and subunit KLH with Montanide ISA-51 as an adjuvant. HMW-KLH and subunit KLH with Montanide ISA-51 showed a comparable immune response in healthy participants, and were more potent compared to subunit KLH alone. The immunogenicity of KLH is presumably related to the carbohydrate and peptide epitopes (Geyer et al., 2005; Harris et al., 1999). Because of the lower immunogenicity, subunit KLH is often used concurrently with an adjuvant, such as aluminum hydroxide (Edelman, 1980). Out of the 9 studies that used an adjuvant together with subunit KLH, participants were immunized with subunit KLH adsorbed to aluminum hydroxide in 7 studies (Boulton et al., 2012; Poirier et al., 2016; Saghari et al., 2020; Saghari et al., 2022; Smith et al., 2004a; Smith et al., 2004b; Spazierer et al., 2009).
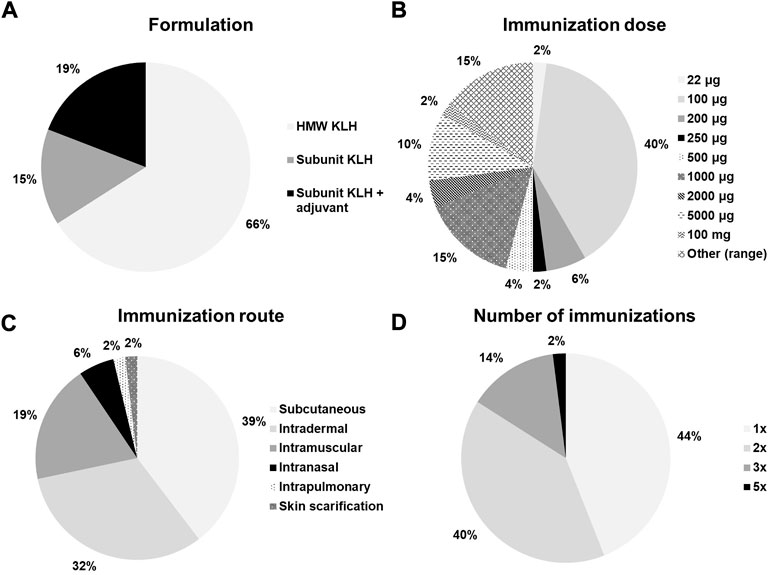
FIGURE 2. Ratios of (A) KLH formulation, (B) immunization dose, (C) immunization route, and (D) number of immunizations across all studies with a KLH challenge in healthy subjects without any other immunomodulatory interventions. HWM, high molecular weight; KLH, keyhole limpet hemocyanin.
Immunizations with doses of 1 μg–5,000 μg have been reported, with 100 μg being the most frequently used dose (Figure 2). (Birdsall and Rossen, 1983; Boulton et al., 2012; Husby et al., 1994; Kantele et al., 1999; Kantele et al., 2011; Kraus et al., 2004; Lange et al., 2003; Moldoveanu et al., 2004; Ochs et al., 1988; Otterhaug et al., 2021; Paty et al., 1975; Smith et al., 2004a; Smith et al., 2004b; Spazierer et al., 2009; Poirier et al., 2016; Saghari et al., 2020; Saghari et al., 2022; Snyder et al., 1993; Waldo et al., 1994) Higher KLH immunization doses have been used in earlier studies and in studies using subunit KLH alone compared to studies using HMW-KLH or subunit KLH with an adjuvant (Table 1). Notably, Belson et al. used a high KLH immunization dose of 5,000 μg causing a participant withdrawal rate of approximately 38% due to large local reactions following a single subcutaneous administration (Belson et al., 2016). Overall, there seems to be a dose-effect relationship following KLH immunization as the maximum response sizes appear to be greater when higher immunization doses are used (Table 2 and Table 3). The number of KLH immunizations also appears to increase the maximum response size. Out of 45 studies, 22 studies immunized participants once (Ashorn et al., 1986; Belson et al., 2016; Boelens et al., 2004; Brunner et al., 1973; Miller et al., 2005; Moldoveanu et al., 2004; Paty et al., 1975; Falconer et al., 1992; Ford and Burger, 1983; Palestine et al., 1985; Snyder et al., 1993; Waldo et al., 1994; Kondratenko et al., 1997; Rentenaar et al., 2002; Schuyler et al., 1997; Smith et al., 2004a; Smith et al., 2004b; Poirier et al., 2016; Saghari et al., 2020; Saghari et al., 2022; Ward et al., 1990; Yang et al., 2021), 20 studies immunized participants twice (Salvaggio et al., 1969; Curtis and Hersh, 1972; Powell et al., 1978; Volkman et al., 1981; Birdsall and Rossen, 1983; Ford and Burger, 1983; Ochs et al., 1988; Bird et al., 1990; Ward et al., 1990; Husby et al., 1994; Kantele et al., 1999; Valdez et al., 2000; Lange et al., 2003; Kraus et al., 2004; Kapp et al., 2010; Kantele et al., 2011; Milgrom et al., 2012; Ferbas et al., 2013; Giesecke et al., 2018; Otterhaug et al., 2021), and 8 studies immunized participants more than 2 times (Table 1 and Figure 2). (Salvaggio et al., 1969; Waldo et al., 1994; Fijter et al., 1996; Diaz-Sanchez et al., 1999; Spazierer et al., 2009; Boulton et al., 2012; Hostmann et al., 2015; Giesecke et al., 2018) Spazierer et al. demonstrated that both the systemic humoral as well as the cell-mediated immune response increase in strength after a subsequent KLH immunization (Spazierer et al., 2009). The response was already observed 14 days after the initial immunization, however, the response size on antigen-specific antibodies and proliferation of peripheral blood mononuclear cells (PBMCs) increased and peaked on day 57 (4 weeks after the third and last immunization). The maximum systemic humoral response had increased 24-fold for anti-KLH immunoglobulin M (IgM, range 8–38-fold), 10,000-fold for anti-KLH IgG1 (range 4,000–19,000-fold) and 40-fold for anti-KLH IgG4 (range 10–110-fold) compared to the pre-immunization anti-KLH antibody titers (Table 2). Similarly, Giesecke et al. demonstrated an increase in anti-KLH antibody responses after a secondary immunization up to 18 months after the primary immunization, though the sample size was small (n = 3) (Giesecke et al., 2018). Moreover, they also found that the secondary immune response occurred faster with an increase of the anti-KLH IgG antibodies 1 week after the secondary immunization, compared to an increase of the anti-KLH IgG antibody responses 2 weeks after the primary immunization.
Subcutaneous KLH injection is the most frequently used administration route (21 out of 45 studies, Table 1 and Figure 2). (Curtis and Hersh, 1972; Brunner et al., 1973; Paty et al., 1975; Volkman et al., 1981; Ford and Burger, 1983; Bird et al., 1990; Ward et al., 1990; Snyder et al., 1993; Husby et al., 1994; Waldo et al., 1994; Fijter et al., 1996; Kantele et al., 1999; Rentenaar et al., 2002; Kraus et al., 2004; Miller et al., 2005; Kapp et al., 2010; Kantele et al., 2011; Hostmann et al., 2015; Belson et al., 2016; Giesecke et al., 2018; Yang et al., 2021) Other frequently used routes of administration include intramuscular and intradermal injections (Ashorn et al., 1986; Boelens et al., 2004; Birdsall and Rossen, 1983; Bird et al., 1990; Curtis and Hersh, 1972; Falconer et al., 1992; Ford and Burger, 1983; Kondratenko et al., 1997; Lange et al., 2003; Ochs et al., 1988; Otterhaug et al., 2021; Palestine et al., 1985; Powell et al., 1978; Salvaggio et al., 1969; Smith et al., 2004a; Smith et al., 2004b; Moldoveanu et al., 2004; Spazierer et al., 2009; Kapp et al., 2010; Boulton et al., 2012; Milgrom et al., 2012; Ferbas et al., 2013; Hostmann et al., 2015; Poirier et al., 2016; Giesecke et al., 2018; Saghari et al., 2020; Saghari et al., 2022). Intranasal KLH inhalation has also been reported, however, sufficient penetration of KLH through the mucosal tissue likely requires higher (cumulative) KLH doses in order to exert measurable systemic immune responses (Salvaggio et al., 1969; Waldo et al., 1994; Diaz-Sanchez et al., 1999). Intradermal KLH administration is the preferential administration route when analyzing the skin response after KLH rechallenge (Salvaggio et al., 1969; Curtis and Hersh, 1972; Brunner et al., 1973; Palestine et al., 1985; Ashorn et al., 1986; Husby et al., 1994; Waldo et al., 1994; Valdez et al., 2000; Rentenaar et al., 2002; Lange et al., 2003; Smith et al., 2004a; Boelens et al., 2004; Smith et al., 2004b; Moldoveanu et al., 2004; Spazierer et al., 2009; Boulton et al., 2012; Milgrom et al., 2012; Hostmann et al., 2015; Belson et al., 2016; Saghari et al., 2020; Saghari et al., 2022). The arm is most often used for KLH administration as it is easily accessible and convenient for the (subjective) evaluation of the skin rechallenge.
Assays for quantification of systemic humoral responses following KLH immunization
The systemic humoral response after immunization with KLH was investigated in 36 out of 45 studies. Analysis methods varied from enzyme-linked immunosorbent assay (ELISA) to hemagglutination assay (HA), radioimmunoassay (RIA), and cytometric bead array (CBA) (Table 2). The majority of the studies had used ELISA to quantify KLH-specific antibodies (Volkman et al., 1981; Palestine et al., 1985; Bird et al., 1990; Ward et al., 1990; Falconer et al., 1992; Husby et al., 1994; Waldo et al., 1994; Fijter et al., 1996; Kondratenko et al., 1997; Schuyler et al., 1997; Rentenaar et al., 2002; Lange et al., 2003; Smith et al., 2004a; Boelens et al., 2004; Smith et al., 2004b; Kraus et al., 2004; Moldoveanu et al., 2004; Miller et al., 2005; Spazierer et al., 2009; Kapp et al., 2010; Boulton et al., 2012; Milgrom et al., 2012; Hostmann et al., 2015; Poirier et al., 2016; Giesecke et al., 2018; Saghari et al., 2020; Otterhaug et al., 2021; Yang et al., 2021; Saghari et al., 2022).
Earlier studies used HA or RIA to identify antibodies. A disadvantage of HA is the difficulty to distinguish between the different types of antibodies, therefore often the total anti-KLH antibody response was measured (Salvaggio et al., 1969; Curtis and Hersh, 1972; Brunner et al., 1973; Paty et al., 1975; Ochs et al., 1988). Birdsall et al. used RIA to quantify the humoral immune response which is a more specific method compared to HA and also based on the binding of antibodies in the sample sera to a known concentration of antigen (Birdsall and Rossen, 1983). Similar to ELISA, RIA also allows for the quantification of the various subtypes of KLH-specific antibodies, however, the antigen is radiolabeled as opposed to an enzyme linked color change in ELISA. The simplicity, practicality, and no need for special equipment or radioactive labels have made ELISA the gold standard for detection and quantification of protein biomarkers (Aydin, 2015; Thiha and Ibrahim, 2015).
A more recent study performed by Ferbas et al. showed the course of anti-KLH IgG and IgM production by B cells in serum with a CBA method (Ferbas et al., 2013). With CBA, beads with various fluorescence intensities are used and conjugated to human Ig subclasses. Subsequently, the samples are analyzed with a flow cytometer (Morgan et al., 2004).
The KLH-specific antibody responses were analyzed differently across the studies included in this review. The comparison of optical density (OD) values of experimental sera in precalculated dilutions to negative control and OD values of a positive control included on the same ELISA plate was used in several studies (Bird et al., 1990; Schuyler et al., 1997; Smith et al., 2004a; Smith et al., 2004b; Kraus et al., 2004; Milgrom et al., 2012; Ferbas et al., 2013; Saghari et al., 2020). Other studies analyzing the anti-KLH antibody production in sera also prepared standard curves for each studied antibody isotype with established KLH antibody concentrations in mg/L, thereby being able to calculate KLH antibody levels (Palestine et al., 1985; Fijter et al., 1996; Kondratenko et al., 1997; Diaz-Sanchez et al., 1999; Valdez et al., 2000; Boelens et al., 2004; Miller et al., 2005; Spazierer et al., 2009; Poirier et al., 2016; Giesecke et al., 2018; Otterhaug et al., 2021). Another method used was to compare the OD values of the sample sera to a reference serum (Ward et al., 1990; Kapp et al., 2010; Hostmann et al., 2015). This reference serum contained high-antibody titer sera from immunized subjects defined to contain 1,000 arbitrary units.
Systemic humoral KLH response size and variability
Various anti-KLH antibody subtypes (e.g., IgG, IgM, IgA, IgE) were studied (Table 2). Anti-KLH IgG antibodies were measured in all studies characterizing the systemic humoral immune response, of which 8 studies also included the IgG subtypes (IgG1-4) (Bird et al., 1990; Falconer et al., 1992; Schuyler et al., 1997; Boelens et al., 2004; Miller et al., 2005; Spazierer et al., 2009; Kapp et al., 2010; Hostmann et al., 2015). Anti-KLH IgM antibodies were analyzed in 20 studies (Salvaggio et al., 1969; Brunner et al., 1973; Volkman et al., 1981; Birdsall and Rossen, 1983; Palestine et al., 1985; Ward et al., 1990; Husby et al., 1994; Waldo et al., 1994; Fijter et al., 1996; Kondratenko et al., 1997; Schuyler et al., 1997; Smith et al., 2004a; Boelens et al., 2004; Kraus et al., 2004; Moldoveanu et al., 2004; Miller et al., 2005; Spazierer et al., 2009; Kapp et al., 2010; Boulton et al., 2012; Milgrom et al., 2012; Ferbas et al., 2013; Hostmann et al., 2015; Giesecke et al., 2018; Saghari et al., 2020) and anti-KLH IgA antibodies were analyzed in 10 studies (Birdsall and Rossen, 1983; Husby et al., 1994; Waldo et al., 1994; Fijter et al., 1996; Schuyler et al., 1997; Diaz-Sanchez et al., 1999; Boelens et al., 2004; Kapp et al., 2010; Milgrom et al., 2012; Hostmann et al., 2015; Giesecke et al., 2018). Some older studies only analyzed the total, non KLH-specific Ig response (Curtis and Hersh, 1972; Brunner et al., 1973; Paty et al., 1975). The response sizes as well as the variability of the antibody subtypes varied between studies. This could be attributed to differences in the analytical and statistical methodology and the study setup. However, it is evident that the KLH response size increases with increasing immunization dose and the number of immunizations. All studies tested anti-KLH antibodies at baseline. The antibody titer was consistently lower compared to post-dose values. KLH is a neoantigen and as such little to no background signal is expected to occur. However, as no validated reference material is available for the KLH-specific antibody assessment in humans, it is impossible to state with certainty that the baseline anti-KLH antibody titers are undetectable. Given the increases observed in the KLH-specific antibody assays across the studies that used calibration curves or defined study specific reference sera containing a high anti-KLH antibody titer, it is possible to suggest that the KLH-specific antibody titers were at the very least low in baseline samples. Three weeks after immunization was the most frequently used interval for antibody assessment, ranging from one to 8 weeks with some also analyzing antibodies after 1 or 5 years (Bird et al., 1990; Ward et al., 1990; Giesecke et al., 2018).
It is difficult to say which anti-KLH antibody shows the strongest response as comparison between studies is complex due to variations in the immunization dose, the interval between immunization and sampling, and the differences in endpoints and analytical methods between studies. Overall, the maximum anti-KLH IgG response increases to a greater extent from baseline values than the IgM response. We previously showed that the anti-KLH IgG response is stronger than the IgM response after one intramuscular immunization with KLH (Saghari et al., 2020). Anti-KLH IgG increased 4.9-fold (range 1.3–9.3-fold) compared to a 1.4-fold (range 1–2.4-fold) increase in IgM. Both antibody titers started to increase from 7 to 14 days after immunization and remained constant until day 28. Similarly, Smith et al. showed a 5-fold (range 4.7–5.3-fold) increase in anti-KLH IgG titers compared to a 1.7-fold (range 1.6–1.8-fold) increase in anti-KLH IgM titers (Smith et al., 2004a). These changes were observed 3 weeks post KLH immunization. Both studies used a 100 μg subunit KLH formulation with alum as adjuvant for the intramuscular immunization. Miller et al. used 3 different formulations of KLH (Miller et al., 2005). Both HMW KLH and subunit KLH with Montanide ISA-51 as adjuvant showed a stronger increase in antigen specific IgG compared to IgM. HMW KLH induced an increase of 37.6-fold in IgG1 (range 11.8–87-fold), 6-fold in IgG2 (range 1.3–10.7-fold) and only 2.9-fold in IgM (range 0.1–5.6-fold). Subunit KLH with Montanide ISA-51 induced comparable responses with an increase of 67.4-fold in IgG1 (range 8.5–126.3-fold), 7.4-fold IgG2 (range 2.4–12.5-fold) and 5.9-fold IgM (range 0.8–11-fold).
IgE does not seem to be produced after immunization with KLH. A study performed by Schuyler et al. did not detect anti-KLH IgE antibody levels after immunization with KLH (Schuyler et al., 1997). The anti-KLH antibody response was analyzed between atopic asthmatics and non-atopic asthmatics after KLH immunization by instillation into a subsegment of the lingula of the left lung. Anti-KLH IgG1, IgG4, IgA1 and IgM antibodies were detected in serum. The levels of IgG1 (38 IU/ml), IgM (280 IU/ml) and IgA1 (25 IU/ml) peaked after 12 days and decreased thereafter for IgM (200 IU/ml) and IgA1 (18 IU/ml) displaying a difference in the peak time for each (sub)type of anti-KLH antibody as the IgM and IgA antibody response increased early followed by the IgG antibody response. Overall, the response size increased 175-fold for anti-KLH IgM (range 125–225-fold), 35-fold for anti-KLH IgG1 (range 21–49-fold), 1.5-fold for anti-KLH IgG4 (range 1.3–1.7-fold), and 35-fold for anti-KLH IgA (range 23–47-fold). A study by Ward et al. also demonstrated differences in the peak times of various anti-KLH antibodies (Ward et al., 1990). Peak anti-KLH IgM responses (8.5-fold increase) were observed 7 days after immunization and peak anti-KLH IgG responses (76-fold increase) 21 days after immunization. Spazierer et al. showed that the anti-KLH IgM antibody reaction was higher at 2–4 weeks after immunization, plateauing in the 2 weeks after that, whereas IgG1 continued to increase until day 57 (Spazierer et al., 2009). IgM increased 24-fold (range 8–38-fold), IgG1 increased 10,000-fold (range 4,000–19000-fold) and IgG4 increased 40-fold (range 10–110-fold) compared to baseline.
Boelens et al. found a difference in the anti-KLH IgG titers between the IgG subtypes (Boelens et al., 2004). An increase of 12-fold in total IgG (range 3–16-fold), 1.6-fold in IgG1 (range 1.1–1.9-fold), 5-fold in IgG2 (range 3–11-fold), and 3-fold in IgG3 subtypes (range 1.5–33-fold) was reported compared to baseline. No IgG4 anti-KLH antibodies were detected. This increase of IgG1, IgG2 and IgG3 anti-KLH antibodies and no change in the anti-KLH IgG4 antibodies was also described by Bird et al. (Bird et al., 1990) Total anti-KLH IgG showed a 23-fold increase in serum antibody (range 15–40-fold), of which an increase of 58-fold (range 30–120-fold) in IgG1, 4-fold (range 1.8–12.5-fold) in IgG2, 3-fold (range 1.5-3-fold) in IgG3 and undetectable IgG4 was observed. After a secondary immunization a year later, the anti-KLH IgG4 antibody response showed an increase of 78-fold (range 40–100-fold). The rise in anti-KLH IgG4 titers after the secondary immunization could be attributed to an increase in T helper cells after a secondary immunization with KLH. This could lead to the class switching of B cells and possibly the proliferation of more IgG4-producing plasma cells. Potentially, these IgG4-producing plasma cells were only able to mature during the secondary response as the primary anti-KLH IgG4 antibody response might have been insufficient to stimulate B cell differentiation (Bird et al., 1990).
Assays for quantification of systemic cellular and molecular responses following KLH immunization
A total of 26 studies characterized aspects of the systemic cell-mediated immunity following KLH immunization, using ex vivo restimulation of immune cells isolated from KLH-immunized volunteers (Table 2). (Curtis and Hersh, 1972; Brunner et al., 1973; Paty et al., 1975; Powell et al., 1978; Ford and Burger, 1983; Palestine et al., 1985; Ashorn et al., 1986; Snyder et al., 1993; Husby et al., 1994; Fijter et al., 1996; Kondratenko et al., 1997; Kantele et al., 1999; Valdez et al., 2000; Rentenaar et al., 2002; Lange et al., 2003; Boelens et al., 2004; Smith et al., 2004b; Kraus et al., 2004; Moldoveanu et al., 2004; Miller et al., 2005; Spazierer et al., 2009; Kapp et al., 2010; Kantele et al., 2011; Ferbas et al., 2013; Hostmann et al., 2015; Giesecke et al., 2018) These studies used conventional in vitro lymphocyte proliferation assays, where PBMCs were incubated with KLH to induce the proliferation of T cells and the release of cytokines. The incubation time with KLH varied from 4 to 8 days. Three different assays were used, a thymidine incorporation assay (TIA, 19 studies) (Curtis and Hersh, 1972; Brunner et al., 1973; Paty et al., 1975; Ford and Burger, 1983; Palestine et al., 1985; Ashorn et al., 1986; Snyder et al., 1993; Waldo et al., 1994; Kondratenko et al., 1997; Kantele et al., 1999; Valdez et al., 2000; Rentenaar et al., 2002; Lange et al., 2003; Boelens et al., 2004; Smith et al., 2004b; Kraus et al., 2004; Moldoveanu et al., 2004; Miller et al., 2005; Spazierer et al., 2009), an enzyme-linked immunosorbent spot assay (ELISpot, 6 studies) (Husby et al., 1994; Fijter et al., 1996; Miller et al., 2005; Kantele et al., 2011; Ferbas et al., 2013; Giesecke et al., 2018), and flow cytometry (FC, 2 studies) (Kapp et al., 2010; Hostmann et al., 2015). Although TIA is the most widely used assay to measure T cell proliferation after KLH rechallenge, a main disadvantage is the use of radioactive labels, therefore more modern techniques are now used (Phetsouphanh et al., 2015). ELISpot is often used for the detection of cytokine secreting cells, however, it can also be used for the determination of antibody secreting cells (Ji and Forsthuber, 2014). FC is currently widely used for rapid specific protein characterization analyses and phosphorylation states of individual cells (Phetsouphanh et al., 2015).
Spazierer et al. used the multiplex Luminex method for the quantification of cytokines secreted in cell culture (Vignali, 2000; Spazierer et al., 2009). They found an increase in IL-5 secretion (15-fold, range 1–80-fold), IL-10 secretion (12-fold, range 1–20-fold), IL-13 secretion (120-fold, range 10–500-fold), and IFN-γ secretion (15-fold, range 1–30-fold). Importantly, most cytokines are released by Type 2 T helper (Th2) cells.
An older technique used to quantify the systemic cellular response is the leukocyte adherence inhibition assay (LAI) (Koppi et al., 1979). Powell et al. reported a LAI response of 35-fold (range 26–45-fold) in subjects immunized with KLH compared to baseline indicative of cell-mediated immunity (Powell et al., 1978).
Systemic cellular and molecular KLH response sizes and variability
The proliferative responses of PBMCs after KLH immunization were all increased by 1.5- to 26-fold from baseline (Table 2). The ex vivo sample workup plays a role in the variability observed between studies. Factors such as the PBMC or T cell isolation, the incubation time, and the ex vivo KLH stimulation protocol were expectedly variable between studies. However, within single studies there was also a rather moderate to large variability of the proliferative responses. Spazierer et al. observed a mean proliferation response of 4-fold with limited variability (min-max 3.3-fold–4.7-fold) (Spazierer et al., 2009), whereas Lange et al. observed a stronger proliferation response size of 25-fold with a substantially higher variability (min-max 16-fold–80-fold) (Lange et al., 2004).
KLH-driven B cell responses by ELISpot were evaluated in multiple studies. Giesecke et al. and Ferbas et al. showed a 44-fold (variability unclear) increase in plasmablasts and a 1,250-fold (range 1–2,600-fold) increase in B cells, respectively (Ferbas et al., 2013; Giesecke et al., 2018). Several studies characterized the B cell response by analyzing the antibody type produced by the cells (IgG, IgM, IgA) (Husby et al., 1994; Fijter et al., 1996; Kantele et al., 2011). KLH responses were detected, but there was no consistency between the studies in which antibody producing cell type was the most or least increased.
Kapp et al. analyzed the cytokine production by CD4+CD154+ T cells (Kapp et al., 2010). Immunization with KLH resulted in the induction of a T cell subset secreting IL-2 (30-fold increase, range 10–50-fold), IL-4 (10-fold increase, range 4–16-fold), IL-10 (2-fold increase, range 1–10-fold), IL-17 (3-fold increase, range 1-7-fold), TNF (30-fold increase, range 5–50-fold), and IFN-γ (21-fold increase, range 5–45-fold) compared to baseline. The induction of T cells secreting IL-2, IL-4, IL-10, TNF and IFN-γ after KLH immunization was reported by Hostmann et al (Hostmann et al., 2015), reporting T cell responses for IL-2 of 140-fold (range 90–170-fold), IL-4 of 36-fold (range 22–40-fold), IL-10 of 12-fold (range 5–17-fold), TNF of 7-fold (range 5–10-fold), and IFN-γ of 20-fold (range 10–30-fold).
Miller et al. analyzed IFN-γ release by ELISpot and showed an increase of 10-fold (range 5–20-fold) compared to baseline indicating activation of the adaptive immune system (Miller et al., 2005).
The systemic cellular responses upon KLH immunization seem to be heavily dependent on the number of immunizations and, to a lesser extent, on the immunization dose. All but one study that had used ELISpot, FC, and/or Multiplex analyses had immunized subjects with KLH at least twice (Husby et al., 1994; Fijter et al., 1996; Spazierer et al., 2009; Kapp et al., 2010; Kantele et al., 2011; Ferbas et al., 2013; Hostmann et al., 2015; Giesecke et al., 2018). Miller et al. immunized subjects with KLH only once before ELISpot analysis of IFN-γ release, however, the 1,000 μg KLH dose was rather high when compared to the most frequently used dose of 100 μg (Miller et al., 2005). Notably, the observed IFN-γ release was lower compared to the results reported by Spazierer et al. (10-fold increase vs. 15-fold increase) where subjects were immunized with 100 μg KLH three times (cumulative dose of 300 μg) (Spazierer et al., 2009). Though, it should be noted that the IFN-γ analysis method differed between the studies (ELISpot vs. Multiplex).
Although a little less evident, similar findings can be observed for TIA across studies supporting the hypothesis that multiple KLH immunizations are more effective for increased systemic cellular responses compared to higher doses of KLH. Several studies that immunized subjects with 100 μg KLH twice showed larger increases in TIA responses (7.7-fold–25-fold increase) (Husby et al., 1994; Kantele et al., 1999; Lange et al., 2003; Kraus et al., 2004) compared to Kondratenko et al. where subjects were immunized with 200 μg KLH only once (6.1-fold increase) (Kondratenko et al., 1997). Other studies used even higher single KLH immunization doses (500 μg up to 5,000 μg), however, the TIA responses were nevertheless lower overall (2.5-fold–7.5-fold increase) (Palestine et al., 1985; Ashorn et al., 1986; Rentenaar et al., 2002; Boelens et al., 2004).
Techniques for evaluation of skin responses following intradermal KLH administration
The antigen-specific cell-mediated immunity can be studied locally by challenging the skin with intradermal KLH, after an initial immunization. This T cell-driven inflammatory response usually takes more than 12 h to develop, and the maximal response time usually occurs between 24 and 72 h. The effects induced by the intradermal KLH rechallenge are likely to be driven by a mixed reaction of innate immune responses (Spazierer et al., 2009), T cell-driven delayed-type hypersensitivity (DTH) (Spazierer et al., 2009; Belson et al., 2016; Saghari et al., 2020; Saghari et al., 2022), and partially Th2 cell-type late-phase skin response effects (Spazierer et al., 2009).
A total of 20 studies evaluated a skin rechallenge to investigate the local cell-mediated immune response (Table 3). (Salvaggio et al., 1969; Curtis and Hersh, 1972; Brunner et al., 1973; Palestine et al., 1985; Ashorn et al., 1986; Husby et al., 1994; Waldo et al., 1994; Valdez et al., 2000; Lange et al., 2003; Smith et al., 2004a; Boelens et al., 2004; Smith et al., 2004b; Moldoveanu et al., 2004; Spazierer et al., 2009; Boulton et al., 2012; Milgrom et al., 2012; Belson et al., 2016; Saghari et al., 2020) The studies varied in the initial KLH immunization dose/regimen, as well as the rechallenge timing and KLH dose (Table 1). KLH skin rechallenge doses ranging from 1 to 1,000 μg have been reported, with 10 μg being most frequently used for the skin rechallenge. Most studies evaluated the KLH skin responses induced by an intradermal injection 2–3 weeks post initial immunization. Subsequently, the response was evaluated at 24–72 h post-challenge, commonly at 48 h. The skin response was predominantly evaluated by (subjective) planimetric scoring and measurement of induration (18 studies) and/or erythema (3 studies) with either a ruler or ballpoint pen technique (Table 3). (Sokal, 1975) A positive response was sometimes scored categorically with a positive reaction defined as induration with a mean diameter of greater than 5 mm (Valdez et al., 2000; Boulton et al., 2012; Milgrom et al., 2012).
The method of measuring diameter index of the skin rechallenge response and the ballpoint technique or ruler technique (Sokal, 1975) both suffer from a lot of inter-rater variability (Pouchot et al., 1997; Saghari et al., 2020). An objective, non-invasive method for evaluation of induration and erythema would be favored. A few studies used objective methods to quantify the skin rechallenge response, such as laser speckle contrast imaging (LSCI), laser doppler imaging (LDI), multispectral imaging (MI), colorimetry and erythema index calculated from photographs (Table 3). (Belson et al., 2016; Saghari et al., 2020; Saghari et al., 2022) LSCI and LDI both measure the cutaneous blood flow by using different laser techniques (Deegan and Wang, 2019). MI captures images of a defined location without exposure to ambient light and illuminates this region with multidirectional light (Linming et al., 2018). MI-based endpoints can include wrinkles, erythema, elevations, and depressions assessments. Colorimetry captures reflected light from the skin and measures the light intensity, usually utilizing the CIELab color space coding system (Ly et al., 2020).
Local planimetric KLH response sizes and variability
Induration was observed following the intradermal KLH rechallenge with a mean diameter of 5.6–51.8 mm across studies (Table 3). Importantly, higher intradermal rechallenge KLH doses lead to larger induration reactions. Smith et al. observed a mean induration response of 5.6 mm (range 1.0–10.2 mm) in subjects immunized with 100 µg KLH and rechallenged with 1 µg intradermally (Smith et al., 2004b) whereas Belson et al. showed a mean induration response of 51.8 mm (range 35.8–75.1 mm) in subjects immunized with 5,000 µg KLH and rechallenged with 100 µg intradermally (Belson et al., 2016). Furthermore, the erythema response after skin rechallenge was always larger compared to the induration response (Salvaggio et al., 1969; Boelens et al., 2004; Belson et al., 2016). This finding is consistent with literature, however, as induration is a widely accepted measure of skin rechallenge response it is advantageous to at least use the induration index as outcome when assessing the skin responses following an intradermal KLH rechallenge (Kimura et al., 2005).
Several studies objectively scored the KLH skin rechallenge response by imaging techniques (Table 3). We used LSCI, MI, colorimetry and photography to score cutaneous blood perfusion and erythema in two separate studies following an intradermal KLH rechallenge (Saghari et al., 2020; Saghari et al., 2022). Interestingly, in the initial study we were unable to detect a positive skin rechallenge response using subjective evaluation, since we used a single KLH immunization and a low KLH rechallenge dose (Saghari et al., 2020). However, due to the increased sensitivity of the applied imaging techniques, significant KLH-dependent changes in cutaneous blood flow (1.4-fold increase, range 1.0–1.9-fold) and erythema (1.4-fold increase, range 1.2–1.9-fold) were detected compared to placebo-treated subjects. Belson et al. used LDI to evaluate the skin rechallenge response (Belson et al., 2016). They demonstrated that LDI measurements showed increased inter-subject variability in the area of flare, compared to the results of induration diameter and erythema. The LDI measurements of the area of flare were 29.5 cm2 (range 20.6–42.3 cm2) after 48 h and 2.2 cm2 (range 0.7–9.7 cm2) after 120 h. These imaging devices could become important measurement instruments for objectively studying the skin reactions in future clinical trials. Notably, two out of three studies that performed categorical scoring of induration (>5 mm) after the intradermal KLH rechallenge had poor responder rates (8% and 25%) (Boulton et al., 2012; Milgrom et al., 2012). The third study had a responder rate of 80%, however, the intradermal KLH dose of 1,000 μg was rather high compared to the most frequently used dose of 10 μg. Considering both the high non-responder rate and increased inter-rater variability when scoring the skin response subjectively, imaging techniques are preferred for analysis of the skin reactions as they provide more sensitive, objective quantification of the skin reactions.
Evaluation of local cellular and molecular responses following KLH administration
The local cellular and molecular responses following a local KLH rechallenge have been rarely studied: only 3 out of 45 studies evaluated these responses (Table 3). (Diaz-Sanchez et al., 1999; Spazierer et al., 2009; Belson et al., 2016) The challenged skin can be harvested by performing skin (punch) biopsies, the subsequent sample can be subjected to a multitude of analyses such as immunohistochemistry, immunofluorescence, qPCR and more. Another method is to assess the local cellular and molecular skin response by inducing suction blisters. The suction blister exudate can be aspirated and analyzed for the presence of immune cells by FC and cytokine concentrations by ELISA (Belson et al., 2016).
Diaz-Sanchez et al. analyzed the local molecular immune response in nasal fluid samples after intranasal KLH immunizations (Diaz-Sanchez et al., 1999). They did not find any significant KLH-induced changes in IL-4 and IFN-γ concentrations in nasosorption samples. Moreover, the increase in the systemic humoral response was small which could possibly indicate that aerosol immunization induces a weaker immune response compared to an intramuscular, subcutaneous, or intradermal immunization with KLH.
Local cellular and molecular KLH response sizes and variability
Belson et al. evaluated the local KLH responses in both skin punch biopsies and skin suction blisters (Belson et al., 2016). The skin biopsies were examined by single chromogenic immunohistochemical staining, to quantify the number and activation of T cells. Following the KLH rechallenge, CD3+ and LAG3+ cells were detected in the biopsies at larger numbers compared to control skin (treated with PBS) or unchallenged skin. There was a 16-fold (range 14–19-fold) increase in CD3+ cells and 20-fold (range 12–39-fold) more LAG3+ T cells compared to the control skin after 48 h. In parallel, the suction blisters were induced at the site of the KLH skin rechallenge for the harvesting of immune cells. Multi-color FC showed a 60-fold (range 15–130-fold) increase in leucocyte numbers in KLH treated compared to untreated skin. The cells within the suction blister exudate were dominated by lymphocytes (mean lymphocyte percentage of 72.6%). Additionally, FC showed that the lymphocytes in the blister fluid were predominantly CD4+ T helper cells, of which 42% had a central memory phenotype and 44% had an effector memory phenotype (indicated by CD4+CCR7+CD45RA−). A shift in the absolute mean cell numbers from central memory CD4+ T cells towards the effector memory CD4+ T cells was observed between 48 h and 120 h after the skin rechallenge.
Spazierer et al. examined the local cellular and molecular immune response by skin biopsies (Spazierer et al., 2009). Eosinophils and IgE positive cells were analyzed in skin biopsies with immunohistochemical staining. They showed a 70-fold (range 1–140-fold) increase in eosinophils and a 75-fold (range 1–180-fold) increase in IgE positive cells compared to placebo. The eosinophilic and IgE cell positive infiltrate in KLH rechallenged skin is indicative of a local Th2 response (Vercelli, 2000; Platts-Mills et al., 2001; Poulsen and Hummelshoj, 2007; Spencer and Weller, 2010). Furthermore, they also observed increased cytokines in the rechallenged skin compared to placebo, including a 2-fold IL-1β increase (range 1-9-fold), 9-fold IL-4 increase (range 5–12-fold), 19-fold IL-13 increase (range 8–50-fold), 10-fold IL-17 increase (range 3–24-fold), 15-fold IL-22 increase (range 1–45-fold), 4-fold IL-23 p19 increase (range 1-6-fold), and 5-fold IFN-γ increase (range 2-8-fold). The high local levels of IL-4 and IL-13 suggest a Th2 response driven largely by a late-phase skin reaction rather than a DTH response demonstrated by the reaction peak observed at 24 h post intradermal rechallenge whereas a DTH reaction peak is expected 48–72 h after induction (Dannenberg, 1991; Vukmanovic-Stejic et al., 2006). Based on the low IL-33 levels, a known Th2 response promoter, it seems unlikely that the Th2 response was induced by this cytokine. Moreover, the importance of increased IL-17 and IL-22 levels compared to placebo remain to be elucidated as their role in the KLH-induced late-phase skin reactions are unknown.
Effect of (pharmacological) interventions and disease on KLH responses
KLH challenges have been used extensively to study the influences of environmental, psychological, and physical factors as well as the effect of diseases and (immunomodulatory) drugs on the adaptive immune system. A total of 43 studies were identified in which the KLH challenges were utilized in intervention studies and/or patient populations (Table 4). Out of these studies, 26 focused primarily on the effect of interventions on the KLH challenge model (Palestine et al., 1985; Snyder et al., 1993; Husby et al., 1994; Waldo et al., 1994; Schuyler et al., 1997; Abrams et al., 1999; Diaz-Sanchez et al., 1999; Kantele et al., 1999; Smith et al., 2004a; Smith et al., 2004b; Smith et al., 2004c; Kraus et al., 2004; Moldoveanu et al., 2004; Smith et al., 2005; Weide et al., 2009; Bingham et al., 2010; Kapp et al., 2010; Boulton et al., 2012; Gallegos et al., 2013; Kaufman et al., 2014; Hostmann et al., 2015; Poirier et al., 2016; Swaminathan et al., 2019; Otterhaug et al., 2021; Yang et al., 2021; Saghari et al., 2022). The remaining 17 studies evaluated the KLH responses in various patient populations (Salvaggio et al., 1969; Paty et al., 1975; Berd et al., 1984; Ochs et al., 1988; Sidell et al., 1990; Falconer et al., 1992; Wishahi et al., 1995; Fijter et al., 1996; Kondratenko et al., 1997; Valdez et al., 2000; Rentenaar et al., 2002; Lange et al., 2003; Boelens et al., 2004; Miller et al., 2005; Grant et al., 2008; Spazierer et al., 2009; Ferbas et al., 2013).
Several clinical trials investigated whether oral KLH feeding would affect subsequent immunization and skin rechallenge response outcomes (Table 4). (Husby et al., 1994; Kantele et al., 1999; Kraus et al., 2004; Moldoveanu et al., 2004; Kapp et al., 2010; Hostmann et al., 2015) Immune tolerance after oral KLH administration was inconsistent across studies: some showed systemic T cell tolerance development after oral KLH administration (Husby et al., 1994; Kapp et al., 2010; Hostmann et al., 2015) whereas others did not (Kraus et al., 2004; Moldoveanu et al., 2004). Kapp et al. included both orally primed and non-primed healthy volunteers that were subsequently immunized with KLH (Kapp et al., 2010). Oral KLH priming induced immune tolerance (decreased CD4+ T cell IL-2, IFN-γ, and TNF cytokine secretion, increased CD4+ T cell IL-4 and IL-10 secretion, and decreased CD4+CLA+ T cells). The KLH-specific systemic CD4+ T cell response shifted from a Th type 1 toward a Th type 2 cytokine pattern and the B cell response was amplified after immunization. Their findings are largely consistent with Hostmann et al. showing a decreased pro-inflammatory phenotype in KLH-specific CD4+ T cells (decreased CD4+ T cell IL-2, IL-17, and IFN-γ cytokine secretion, and CD4+CLA+ T cells, increased CD4+ T cell IL-10 cytokine secretion, skin rechallenge and humoral response unaltered) (Hostmann et al., 2015). The differences observed between the oral KLH studies may be attributed to KLH doses used for oral and parenteral administration (Kapp et al., 2010). Studies where higher oral and lower parenteral doses of KLH were used displayed decreased skin rechallenge responses and reduced PBMC proliferation (Husby et al., 1994), possibly confirming an oral KLH dose-dependent effect. Low doses of oral KLH induced systemic T cell responses and modulated the systemic immune responses induced by parenteral KLH (Kapp et al., 2010; Hostmann et al., 2015).
The immune response as evoked by the KLH challenge diminishes with increased age (Smith et al., 2004a; Grant et al., 2008), decreased physical activity (Smith et al., 2004a; Grant et al., 2008), increased alcohol consumption (Smith et al., 2004c), and increased stress (Table 4). (Smith et al., 2004b; Smith et al., 2004c; Smith et al., 2005; Gallegos et al., 2013) Physically fit older adults have increased humoral and skin rechallenge responses after KLH challenge compared to sedentary older adults (Smith et al., 2004a), but interestingly, the humoral response can be restored in previously sedentary older adults when physical exercise is introduced compared to stretching exercises (Grant et al., 2008). Distress during KLH immunization impairs the skin rechallenge response to KLH, but not the humoral or lymphocyte proliferation response (Smith et al., 2004b; Smith et al., 2004c) whereas alcohol consumption during the intradermal KLH skin rechallenge decreases the skin rechallenge response (Smith et al., 2004c) hinting toward different mechanisms and targets for stress and alcohol consumption to alter the KLH challenge response. Furthermore, Smith et al. concluded that a distress phenotype together with HLA-DQ2+ or HLA-DQ5+ genotype possibly contributes to the skin rechallenge response as they found a decreased skin rechallenge response in subjects with HLA-DQ2+ genotype and an increased skin rechallenge response in subjects with HLA-DQ5+ genotype (Smith et al., 2005).
KLH challenge responses have been evaluated in various patient populations, compared to healthy volunteers. Patients with atopic characteristics tend to have increased responses following KLH immunization and subsequent intradermal KLH skin rechallenge (Salvaggio et al., 1969; Falconer et al., 1992; Spazierer et al., 2009). The humoral response is overall not upregulated. Falconer et al. observed increased anti-KLH IgG4 and decreased anti-KLH IgG1 compared to healthy volunteers (Falconer et al., 1992), however, Spazierer et al. was not able to find this discrepancy in the IgG1 and IgG4 subclasses between healthy controls and atopic patients (Spazierer et al., 2009). Patients with systemic lupus erythematosus (SLE), human immunodeficiency virus (HIV), and common variable immunodeficiency disorder (CVID) all have a decreased humoral and cell-mediated response following KLH challenge (Paty et al., 1975; Ochs et al., 1988; Kondratenko et al., 1997; Valdez et al., 2000; Lange et al., 2003; Ferbas et al., 2013). This is explained by the immunodeficiencies (polyclonal B cell activation with a shift toward immature B cells in SLE, decrease in CD4+ T cells in HIV, and decreases in antibody levels in CVID) in all these patient populations.
A couple of investigational medicinal products and registered drugs have been evaluated for their modulatory effect on the KLH-driven immune responses (Table 4). (Berd et al., 1984; Palestine et al., 1985; Sidell et al., 1990; Abrams et al., 1999; Weide et al., 2009; Bingham et al., 2010; Boulton et al., 2012; Kaufman et al., 2014; Poirier et al., 2016; Otterhaug et al., 2021; Yang et al., 2021; Saghari et al., 2022) Palestine et al. showed that cyclosporine administration in uveitis patients suppressed the KLH skin rechallenge response, but did not alter the humoral and lymphocyte proliferation response (Palestine et al., 1985). Weide et al. investigated whether mRNA immunotherapy therapy consisting of Melan-A, Tyrosinase, gp100, Mage-A1, Mage-A3, and Survivin would have an effect on the KLH challenge in metastatic melanoma patients (Weide et al., 2009). They showed a decrease in Foxp3+CD4+ regulatory T cells indicating that the mRNA mixture was able to inhibit the regulatory T cell signals induced by KLH immunization. Boulton et al. administered fingolimod, a S1PR modulator present on lymphocytes, in healthy volunteers and observed a decreased humoral response following KLH immunization (Boulton et al., 2012). Otterhaug et al. performed an innovative study in which they gave fimaporfin, a synthetic light-activated compound that localizes to endosomes and lysosomes and induces endosomal content release into the cell cytosol after activation, intradermally to healthy volunteers and exposed them to a light source thereafter (Otterhaug et al., 2021). Interestingly, subjects treated with fimaporfin exhibited an increased humoral response to KLH immunization. The increase of antibody production may possibly be explained by an enhancement of CD4+ T-cell responses, potentially stimulating antibody production by plasma cells (Parker, 1993). Several other studies used targeted therapies in combination with a KLH challenge to evaluate the immune response. These compounds included monoclonal antibodies against CD28 (VEL-101) (Poirier et al., 2016), and CD20 (rituximab) (Bingham et al., 2010), and chimeric proteins against CD28 and ICOS (acazicolcept) (Yang et al., 2021) and CD80 and CD86 (abatacept) (Abrams et al., 1999). We demonstrated that a novel monoclonal antibody targeted against CD134/OX40 ligand (amlitelimab) was able to suppress the immune response following a KLH challenge, both on a systemic as well as a local level (Saghari et al., 2022). Subsequently, this compound also proved effective in inadequately controlled moderate-to-severe atopic dermatitis patients (Bain et al., 2021) and was sold to Sanofi S.A. for $1.5 billion (White, 2021) underlining the potential predictive value of the KLH challenge in healthy volunteers for future clinical efficacy.
Discussion
KLH has been shown to be an effective immunostimulatory antigen for human clinical studies. Swaminathan et al. has previously set up a framework including the various formulations, doses and routes of administration (Swaminathan et al., 2014). The aim of this systematic review was to expand this framework and to characterize the local and systemic immune response of the immunization with KLH, and to define the optimal biomarkers for KLH immunization readout based on the response size and variability. Furthermore, we have also summarized the effect of a multitude of interventions and diseases on the in vivo KLH challenge in humans.
KLH immunization drives a robust systemic humoral response. All studies included in this systematic review report an anti-KLH IgG response, whereas the anti-KLH IgM and IgA were also increased after KLH immunization. The maximal systemic humoral response is reached approximately 3 weeks after KLH immunization. Anti-KLH IgM antibody levels rise first, anti-KLH IgG antibody levels rise thereafter and in time overtake the anti-KLH IgM response. The systemic cellular response is commonly examined with lymphocyte proliferation assays to assess the T cell responses and cytokine production. FC and ELISpot assays are used more frequently over the past years and give more specific and accurate results. The ex vivo proliferation of KLH-specific T cells, most commonly CD4+ cells, and cytokine production (IFN-γ, IL-10 and IL-4) have been studied most often. Importantly, the number of KLH immunizations and, less evidently, the immunization dose profoundly influences the systemic cellular responses. Taken together, we recommend implementing KLH-specific antibody assessments using ELISA when performing a KLH challenge in clinical trials. This humoral assay should be performed on samples collected at least before and 3 weeks after KLH immunization. When analyzing systemic cellular and molecular immune responses we advise multiple KLH immunizations and the preferential use of novel techniques such as FC and ELISpot over TIA. As too few studies have performed KLH-specific systemic immune response analyses it is hard to make suggestions as to which immune cell types and specific cytokines should be analyzed.
The local skin response upon the KLH rechallenge was quantified mostly subjectively, by measuring the induration diameter. Although subjective evaluation of induration diameter index of the skin rechallenge response is widely used throughout literature, this technique suffers from a lot of inter-rater variability (Pouchot et al., 1997; Saghari et al., 2020). When studying the skin rechallenge response with objective imaging techniques, the local KLH response can be quantified more accurately and with higher sensitivity. Although the local cellular and molecular immune responses upon KLH rechallenge have not been investigated in many studies, this approach has provided valuable mechanistic insight into the local KLH response. Local activation of T cells after KLH cutaneous rechallenge was observed by the increased presence of CD3+ and LAG3+ T cells (Belson et al., 2016). Furthermore, increased eosinophils and IgE positive cells in KLH rechallenged skin indicate a local Th2 response (Spazierer et al., 2009). This Th2 response is likely driven by a late-phase skin reaction as high local levels of IL-4 and IL-13 were detected and the reaction peak was observed at 24 h after rechallenge, whereas a DTH reaction peak is usually observed after 48–72 h. Between 48 and 120 h after the skin rechallenge, a shift from central memory towards effector memory CD4+ T cells was observed (Belson et al., 2016). Based on the literature discussed in this review, we recommend performing both a subjective evaluation of the dermal KLH rechallenge (current gold standard) as well as objective measurement of the local inflammation by using imaging techniques. Evaluations should be performed at least before, 24 h and 48 h after dermal KLH rechallenge. If possible, the addition of multiple time windows after rechallenge could prove valuable, including 2 and 6 h (innate response) and possibly 72 and 120 h (late DTH response) after intracutaneous KLH administration. For the local cellular and molecular assays after KLH dermal rechallenge we also advocate the use of multiple KLH immunizations in order to increase the systemic KLH-specific immune cell pool. Too few studies have analyzed local cellular and molecular immune responses in order to make recommendations on which cell types and cytokines to analyze.
Lastly, we have summarized the effect of environmental, psychological, and physical factors and (investigational) drugs on the KLH response as well as the impact of disease. Oral KLH feeding induced immune tolerance when administered orally and parenterally depending on the KLH dosing regimen used (Husby et al., 1994; Kapp et al., 2010; Hostmann et al., 2015). Factors such as age, physical activity, alcohol consumption, and stress all play a role in the immune response following KLH challenge. Atopy seems to partially increase the immune responses following KLH immunization and a subsequent intradermal KLH skin rechallenge, possibly due to a stronger immune response after repeated contact with an antigen. In contrast, SLE, HIV, and CVID patients showed a decreased humoral and cell-mediated KLH response. KLH challenges have proven their value in healthy volunteer trials evaluating the effects of immunomodulatory drugs. Based on the implementation of KLH challenges in the earliest stages of drug development, pharmacologically active doses of the investigational drugs could be identified, which facilitated dose selection for the subsequent phase 1B/phase 2A studies in patient populations. The best example of this is the recent success of Kymab’s OX40 ligand blocker amlitelimab, which was effective in suppressing KLH-driven responses in healthy volunteers (Saghari et al., 2022), and subsequently showed improvements in symptoms of moderate-to-severe atopic dermatitis patients (Bain et al., 2021). In the meanwhile, the compound has been acquired by Sanofi (White, 2021).
A limitation of this systematic review was the difficulty in generalizing the response sizes and variabilities due to the differences in analytical and statistical methods, or reference material in the studies. Some studies reported the range as the standard deviation from the mean whereas others reported the range as the 95% confidence interval. A few studies reported the range as the standard error of the mean. Another constraint of this review was that the response sizes and variabilities in many studies were based on estimations from graphical presentations as no concrete numbers were mentioned within the article text or tables. We analyzed these data as best as we were able to from the data at hand. Finally, it is important to stress that most KLH responses have been presented as fold-increase compared to baseline. Conceptually, this approach is questionable since KLH is regarded to be a neoantigen to most study participants, which means that at baseline no KLH-specific immunoglobulins or immune cells should be detected. However, for the authors this was the only way to systematically present the KLH responses over the wide range of clinical studies.
In conclusion, this systematic review provides an overview of the systemic and local responses to immunization with KLH and discusses the preferred imaging, planimetric, cellular and molecular biomarkers for the KLH response characterization. Whereas the circulating KLH-specific immunoglobulins are a very common endpoint for KLH challenge studies, the systemic KLH-specific immune cells have been evaluated less frequently. Importantly, these KLH-specific cells are rare in the circulation, so from a methodological point-of-view the detection of these cells is challenging. Evaluation of the skin response to a local KLH rechallenge yields important information since it is a measure for a specific T cell response at the tissue level. Although subjective evaluation of skin responses to KLH is already being done for decades, our review shows the importance of state-of-the-art imaging techniques to capture the often-mild perfusion increase, erythema and induration caused by KLH. Only a few studies evaluated the cellular and molecular responses to a dermal KLH rechallenge. Since blister exudate- or biopsy-based response characterization provides mechanistic insight into the immune responses driven by KLH, we advocate the implementation of invasive KLH response evaluation in future clinical trials. Based on the KLH challenges, the effect of immunomodulatory drugs could be demonstrated already in healthy volunteers, providing valuable information for the clinical development of these compounds. Moreover, based on the KLH challenge responses the functional immune status of different patient populations could be discriminated from healthy controls, providing novel insight into the pathophysiology of these diseases. Taken together, our review underlines the potential value of KLH challenges in clinical studies, but also the need for standardized and well-controlled methodology to induce and evaluate KLH responses.
Data availability statement
The original contributions presented in the study are included in the article/Supplementary Material, further inquiries can be directed to the corresponding author.
Author contributions
MS performed the database search, screening and data extraction. All authors wrote the manuscript.
Conflict of interest
The authors declare that the research was conducted in the absence of any commercial or financial relationships that could be construed as a potential conflict of interest.
Publisher’s note
All claims expressed in this article are solely those of the authors and do not necessarily represent those of their affiliated organizations, or those of the publisher, the editors and the reviewers. Any product that may be evaluated in this article, or claim that may be made by its manufacturer, is not guaranteed or endorsed by the publisher.
Supplementary material
The Supplementary Material for this article can be found online at: https://www.frontiersin.org/articles/10.3389/fddsv.2022.992087/full#supplementary-material
References
Abrams, J. R., Lebwohl, M. G., Guzzo, C. A., Jegasothy, B. V., Goldfarb, M. T., Goffe, B. S., et al. (1999). CTLA4Ig-mediated blockade of T-cell costimulation in patients with psoriasis vulgaris. J. Clin. Investig. 103, 1243–1252. doi:10.1172/JCI5857
Ashorn, R. G. I. I. G., Vandenbark, A. A. A. A., Acott, K. M. M. M., and Krohn, K. J. E. J. E. J. (1986). Dialysable leukocyte extracts (transfer factor) augment nonspecifically keyhole limpet haemocyanin and horseshoe crab haemocyanin skin reactivity in unimmunized human recipients. Scand. J. Immunol. 23, 161–167. doi:10.1111/j.1365-3083.1986.tb01954.x
Aydin, S. (2015). A short history, principles, and types of ELISA, and our laboratory experience with peptide/protein analyses using ELISA. Peptides 72, 4–15. doi:10.1016/j.peptides.2015.04.012
Bain, S., Schaefer-Jansen, E., Delepine, A., Pham, N., and Lauscher, F. (2021). New, late-breaking data at EADV highlights emerging clinical profile of amlitelimab (formerly KY1005) in adults with inadequately controlled moderate-to-severe atopic dermatitis. Paris, France: Sanofi Press Release. Available at: https://www.sanofi.com/en/media-room/press-releases/2021/2021-09-30-14-30-00-2306183.
Belson, A., Schmidt, T., Fernando, D., Hardes, K., Scott, N., Brett, S., et al. (2016). Characterisation of the clinical and activated T cell response to repeat delayed-type hypersensitivity skin challenges in human subjects, with KLH and PPD, as a potential model to test T cell-targeted therapies. Inflamm. Res. 65, 389–404. doi:10.1007/s00011-016-0923-4
Berd, D., Maguire, H. C., and Mastrangelo, M. J. (1984). Potentiation of human cell-mediated and humoral immunity by low-dose cyclophosphamide. Cancer Res. 44, 5439–5443.
Bingham, C. O., Looney, R. J., Deodhar, A., Halsey, N., Greenwald, M., Codding, C., et al. (2010). Immunization responses in rheumatoid arthritis patients treated with rituximab: Results from a controlled clinical trial. Arthritis Rheum. 62, 64–74. doi:10.1002/art.25034
Bird, P., Calvert, J. E., and Amlot, P. L. (1990). Distinctive development of IgG4 subclass antibodies in the primary and secondary responses to keyhole limpet haemocyanin in man. Immunology 69, 355–360.
Birdsall, H. H., and Rossen, R. D. (1983). Characterization of anti-fab’ antibodies in human sera: Identification of soluble immune complexes that contain hidden anti-KLH and blocking anti-immunoglobulins following immunization with keyhole limpet haemocyanin. Clin. Exp. Immunol. 53, 497–504.
Boelens, P. G., Fonk, J. C. M., Houdijk, A. P. J., Scheper, R. J., Haarman, H. J. T. H. M., Meijer, S., et al. (2004). Primary immune response to keyhole limpet haemocyanin following trauma in relation to low plasma glutamine. Clin. Exp. Immunol. 136, 356–364. doi:10.1111/j.1365-2249.2004.02447.x
Boulton, C., Meiser, K., David, O. J., and Schmouder, R. (2012). Pharmacodynamic effects of steady-state fingolimod on antibody response in healthy volunteers: A 4-week, randomized, placebo-controlled, parallel-group, multiple-dose study. J. Clin. Pharmacol. 52, 1879–1890. doi:10.1177/0091270011427908
Brunner, C. M. M., Horwitz, D. A., Shann, M. K., Sturgill, B. A., and Davis, J. S. (1973). Clinical and immunologic studies in identical twins discordant for systemic lupus erythematosus. Am. J. Med. 55, 249–254. doi:10.1016/0002-9343(73)90176-9
Curtis, J. E., and Hersh, E. M. (1972). The human secondary immune response to Keyhole limpet haemocyanin. Clin. Exp. Immunol. 10, 171–177.
Dannenberg, A. M. (1991). Delayed-type hypersensitivity and cell-mediated immunity in the pathogenesis of tuberculosis. Immunol. Today 12, 228–233. doi:10.1016/0167-5699(91)90035-R
Deegan, A. J., and Wang, R. K. (2019). Microvascular imaging of the skin. Phys. Med. Biol. 64, 07TR01. doi:10.1088/1361-6560/ab03f1
Diaz-Sanchez, D., Garcia, M. P. P., Wang, M., Jyrala, M., and Saxon, A. (1999). Nasal challenge with diesel exhaust particles can induce sensitization to a neoallergen in the human mucosa. J. Allergy Clin. Immunol. 104, 1183–1188. doi:10.1016/s0091-6749(99)70011-4
Falconer, A. E., Friedmann, P. S., Bird, P., and Calvert, J. E. (1992). Abnormal immunoglobulin G subclass production in response to keyhole limpet haemocyanin in atopic patients. Clin. Exp. Immunol. 89, 495–499. doi:10.1111/j.1365-2249.1992.tb06987.x
Ferbas, J., Belouski, S. S., Horner, M., Kaliyaperumal, A., Chen, L., Boyce, M., et al. (2013). A novel assay to measure B cell responses to keyhole limpet haemocyanin vaccination in healthy volunteers and subjects with systemic lupus erythematosus. Br. J. Clin. Pharmacol. 76, 188–202. doi:10.1111/bcp.12172
Fijter, J. W. W. de, Eijgenraam, J. W., Braam, C. A., Holmgren, J., Daha, M. R., van Es, L. A., et al. (1996). Deficient IgA1 immune response to nasal cholera toxin subunit B in primary IgA nephropathy. Kidney Int. 50, 952–961. doi:10.1038/ki.1996.396
Ford, D., and Burger, D. (1983). Precursor frequency of antigen-specific T cells: Effects of sensitization in vivo and in vitro. Cell. Immunol. 79, 334–344. doi:10.1016/0008-8749(83)90075-8
Gallegos, A. M., Hoerger, M., Talbot, N. L., Krasner, M. S., Knight, J. M., Moynihan, J. A., et al. (2013). Toward identifying the effects of the specific components of mindfulness-based stress reduction on biologic and emotional outcomes among older adults. J. Altern. Complement. Med. 19, 787–792. doi:10.1089/acm.2012.0028
Gandhi, R. T., O'Neill, D., Bosch, R. J., Chan, E. S., Bucy, R. P., Shopis, J., et al. (2009). A randomized therapeutic vaccine trial of canarypox-HIV-pulsed dendritic cells vs. canarypox-HIV alone in HIV-1-infected patients on antiretroviral therapy. Vaccine 27, 6088–6094. doi:10.1016/j.vaccine.2009.05.016
Geyer, H., Wuhrer, M., Resemann, A., and Geyer, R. (2005). Identification and characterization of keyhole limpet hemocyanin N-glycans mediating cross-reactivity with Schistosoma mansoni. J. Biol. Chem. 280, 40731–40748. doi:10.1074/jbc.M505985200
Giesecke, C., Meyer, T., Durek, P., Maul, J., PreiB, J., Jacobs, J. F. M., et al. (2018). Simultaneous presence of non- and highly mutated keyhole limpet hemocyanin (KLH)-Specific plasmablasts early after primary KLH immunization suggests cross-reactive memory B cell activation. J. Immunol. 200, 3981–3992. doi:10.4049/jimmunol.1701728
Grant, R. W., Mariani, R. A., Vieira, V. J., FleshnerM., , Smith, T. P., Keylock, K. T., et al. (2008). Cardiovascular exercise intervention improves the primary antibody response to keyhole limpet hemocyanin (KLH) in previously sedentary older adults. Brain Behav. Immun. 22, 923–932. doi:10.1016/j.bbi.2008.01.006
Harris, J. R., Markl, J., and Keyhole limpet hemocyanin (Klh), (1999). Keyhole limpet hemocyanin (KLH): A biomedical review. Micron 30, 597–623. doi:10.1016/s0968-4328(99)00036-0
Hostmann, A., Meyer, T., Maul, J., Preiss, J., Boortz, B., Thiel, A., et al. (2015). Preexisting antigen-specific immune responses are modulated by oral KLH feeding in humans. Eur. J. Immunol. 45, 1991–1996. doi:10.1002/eji.201445024
Husby, S., Mestecky, J., Moldoveanu, Z., Holland, S., and Elson, C. O. (1994). Oral tolerance in humans. T cell but not B cell tolerance after antigen feeding. J. Immunol. 152, 4663–4670.
Ji, N., and Forsthuber, T. G. (2014). ELISPOT techniques. Methods Mol. Biol. 1304, 63–71. doi:10.1007/7651_2014_111
Jia, T., Pan, Y., Li, J., and Wang, L. (2013). Strategies for active TNF-α vaccination in rheumatoid arthritis treatment. Vaccine 31, 4063–4068. doi:10.1016/j.vaccine.2013.06.101
Jurincic-Winkler, C. D., Metz, K. A., Beuth, J., and Klippel, K. F. (2000). Keyhole limpet hemocyanin for carcinoma in situ of the bladder: A long-term follow-up study. Eur. Urol. 37, 45–49. doi:10.1159/000052392
Kantele, A., Zivny, J., Elson, C. O., Mestecky, J., and Kantele, J. M. (2011). Humoral immune response to keyhole limpet haemocyanin, the protein carrier in cancer vaccines. Clin. Dev. Immunol. 2011, 614383. doi:10.1155/2011/614383
Kantele, A., Zivny, J., Häkkinen, M., Elson, C. O., and Mestecky, J. (1999). Differential homing commitments of antigen-specific T cells after oral or parenteral immunization in humans. J. Immunol. 162, 5173–5177.
Kapp, K., Maul, J., Hostmann, A., Mundt, P., Preiss, J. C., Wenzel, A., et al. (2010). Modulation of systemic antigen-specific immune responses by oral antigen in humans. Eur. J. Immunol. 40, 3128–3137. doi:10.1002/eji.201040701
Kaufman, M., Pardo, G., Rossman, H., Sweetser, M. T., Forrestal, F., and Duda, P. (2014). Natalizumab treatment shows no clinically meaningful effects on immunization responses in patients with relapsing-remitting multiple sclerosis. J. Neurol. Sci. 341, 22–27. doi:10.1016/j.jns.2014.03.035
Kimura, M., Comstock, G. W., and Mori, T. (2005). Comparison of erythema and induration as results of tuberculin tests. Int. J. Tuberc. Lung Dis. 9, 853–857.
Kondratenko, I., Amlot, P. L., Webster, A. D. B., and Farrant, J. (1997). Lack of specific antibody response in common variable immunodeficiency (CVID) associated with failure in production of antigen-specific memory T cells. MRC Immunodeficiency Group. Clin. Exp. Immunol. 108, 9–13. doi:10.1046/j.1365-2249.1997.d01-993.x
Koppi, T. A., Maluish, A. E., and Halliday, W. J. (1979). The cellular mechanism of leukocyte adherence inhibition. J. Immunol. 123, 2255–2260.
Kraus, T. A., Toy, L., Chan, L., Childs, J., and Mayer, L. (2004). Failure to induce oral tolerance to a soluble protein in patients with inflammatory bowel disease. Gastroenterology 126, 1771–1778. doi:10.1053/j.gastro.2004.03.076
Lammers, R. J. M., Witjes, W. P. J., Janzing-Pastors, M. H. D., Caris, C. T. M., and Witjes, J. A. (2012). Intracutaneous and intravesical immunotherapy with keyhole limpet hemocyanin compared with intravesical mitomycin in patients with non-muscle-invasive bladder cancer: Results from a prospective randomized phase III trial. J. Clin. Oncol. 30, 2273–2279. doi:10.1200/JCO.2011.39.2936
Lange, C. G., Lederman, M. M., Medvik, K., Asaad, R., Wild, M., Kalayjian, R., et al. (2003). Nadir CD4+ T-cell count and numbers of CD28+ CD4+ T-cells predict functional responses to immunizations in chronic HIV-1 infection. AIDS 17, 2015–2023. doi:10.1097/00002030-200309260-00002
Lange, C. G., Xu, Z., Patterson, B. K., Medvik, K., Harnisch, B., Asaad, R., et al. (2004). Proliferation responses to HIVp24 during antiretroviral therapy do not reflect improved immune phenotype or function. AIDS 18, 605–613. doi:10.1097/00002030-200403050-00004
Lebrec, H., Hock, M. B., Sundsmo, J. S., Mytych, D. T., Chow, H., Carlock, L. L., et al. (2014). T-cell-dependent antibody responses in the rat: Forms and sources of keyhole limpet hemocyanin matter. J. Immunotoxicol. 11, 213–221. doi:10.3109/1547691X.2013.822948
Linming, F., Wei, H., Anqi, L., Yuanyu, C., Heng, X., Sushmita, P., et al. (2018). Comparison of two skin imaging analysis instruments: The VISIA® from Canfield vs the ANTERA 3D®CS from Miravex. Skin. Res. Technol. 24, 3–8. doi:10.1111/srt.12381
Ly, B. C. K., Dyer, E. B., Feig, J. L., Chien, A. L., and Bino, S. (2020). Research techniques made simple: Cutaneous colorimetry: A reliable technique for objective Skin Color measurement. J. Investig. Dermatol. 140, 3–12. doi:10.1016/j.jid.2019.11.003
Milgrom, H., Kesler, K., Byron, M., Harbeck, R., Holliday, R., and Leung, D. Y. M. (2012). Response to cutaneous immunization with low-molecular-weight subunit keyhole limpet hemocyanin. Int. Arch. Allergy Immunol. 157, 269–274. doi:10.1159/000328784
Miller, J. S., Curtsinger, J., Berthold, M., Malvey, K., Bliss, R. L., Le, C. T., et al. (2005). Diminished neo-antigen response to keyhole limpet hemocyanin (KLH) vaccines in patients after treatment with chemotherapy or hematopoietic cell transplantation. Clin. Immunol. 117, 144–151. doi:10.1016/j.clim.2005.07.005
Moldoveanu, Z., Oliver, F., Mestecky, J., and Elson, C. O. (2004). Oral tolerance in humans: Failure to suppress an existing immune response by oral antigen administration. Ann. N. Y. Acad. Sci. 1029, 299–309. doi:10.1196/annals.1309.051
Morgan, E., Varro, R., Sepulveda, H., Ember, J. A., Apgar, J., Wilson, J., et al. (2004). Cytometric bead array: A multiplexed assay platform with applications in various areas of biology. Clin. Immunol. 110, 252–266. doi:10.1016/j.clim.2003.11.017
Ochs, H. D. D., Junker, A. K., Collier, A. C., Virant, F. S., Handsfield, H. H., and Wedgwood, R. J. (1988). Abnormal antibody responses in patients with persistent generalized lymphadenopathy. J. Clin. Immunol. 8, 57–63. doi:10.1007/BF00915157
Otterhaug, T., Janetzki, S., Welters, M. J. P., Hakerud, M., Nedberg, A. G., Edwards, V. T., et al. (2021). Photochemical internalization enhanced vaccination is safe, and gives promising cellular immune responses to an HPV peptide-based vaccine in a phase I clinical study in healthy volunteers. Front. Immunol. 11, 3361. doi:10.3389/fimmu.2020.576756
Palestine, A. G., RobergeF., , Charous, B. L., Lane, H. C., Fauci, A. S., and Nussenblatt, R. B. (1985). The effect of cyclosporine on immunization with tetanus and keyhole limpet hemocyanin (KLH) in humans. J. Clin. Immunol. 5, 115–121. doi:10.1007/BF00915009
Parker, D. C. (1993). T cell-dependent B cell activation. Annu. Rev. Immunol. 11, 331–360. doi:10.1146/annurev.iy.11.040193.001555
Paty, J. G. G., Sienknecht, C. W., Townes, A. S., Hanissian, A. S., Miller, J. B., and Masi, A. T. (1975). Impaired cell-mediated immunity in systemic lupus erythematosus (SLE). A controlled study of 23 untreated patients. Am. J. Med. 59, 769–779. doi:10.1016/0002-9343(75)90462-3
Perabo, F. G. E., and Müller, S. C. (2004). Current and new strategies in immunotherapy for superficial bladder cancer. Urology 64, 409–421. doi:10.1016/j.urology.2004.04.026
Phetsouphanh, C., Zaunders, J. J., and Kelleher, A. D. (2015). Detecting antigen-specific T cell responses: From bulk populations to single cells. Int. J. Mol. Sci. 16, 18878–18893. doi:10.3390/ijms160818878
Platts-Mills, T. A. E., Vaughan, J. W., Blumenthal, K., Woodfolk, J. A., and Sporik, R. B. (2001). Decreased prevalence of asthma among children with high exposure to cat allergen: Relevance of the modified Th2 response. Mediat. Inflamm. 10, 288–291. doi:10.1080/09629350152700902
Poirier, N., Blancho, G., Hiance, M., Mary, C., Van Assche, T., Lempoels, J., et al. (2016). First-in-Human study in healthy subjects with FR104, a pegylated monoclonal antibody fragment antagonist of CD28. J. Immunol. 197, 4593–4602. doi:10.4049/jimmunol.1601538
Pouchot, J., GrAslAnd, A., Collet, C., Coste, J., Esdaile, J. M., and Vinceneux, P. (1997). Reliability of tuberculin skin test measurement. Ann. Intern. Med. 126, 210–214. doi:10.7326/0003-4819-126-3-199702010-00005
Poulsen, L. K., and Hummelshoj, L. (2007). Triggers of IgE class switching and allergy development. Ann. Med. 39, 440–456. doi:10.1080/07853890701449354
Powell, A. E., Sloss, A. M., and Smith, R. N. (1978). Leukocyte-adherence inhibition: A specific assay of cell-mediated immunity dependent on lymphokine-mediated collaboration between T lymphocytes. J. Immunol. 120, 1957–1966.
Rentenaar, R. J., van Diepen, F. N. J., Meijer, R. T., Surachno, S., Wilmink, J. M., Schellekens, P. T. A., et al. (2002). Immune responsiveness in renal transplant recipients: Mycophenolic acid severely depresses humoral immunity in vivo. Kidney Int. 62, 319–328. doi:10.1046/j.1523-1755.2002.00425.x
Saghari, M., Gal, P., Gilbert, S., Yateman, M., Porter-Brown, B., Brennan, N., et al. (2022). OX40L inhibition suppresses KLH-driven immune responses in healthy volunteers: A randomized controlled trial demonstrating proof-of-pharmacology for KY1005. Clin. Pharmacol. Ther. 111, 1121–1132. doi:10.1002/cpt.2539
Saghari, M., Gal, P., Ziagkos, D., Burggraaf, J., Powell, J. F., Brennan, N., et al. (2020). A randomized controlled trial with a delayed‐type hypersensitivity model using keyhole limpet haemocyanin to evaluate adaptive immune responses in man. Br. J. Clin. Pharmacol. 87, 1953–1962. doi:10.1111/bcp.14588
Salvaggio, J., Castro-Murillo, E., and Kundur, V. (1969). Immunologic response of atopic and normal individuals to keyhole limpet hemocyanin. J. Allergy 44, 344–354. doi:10.1016/0021-8707(69)90026-4
Schumacher, K. (2001). Keyhole limpet hemocyanin (KLH) conjugate vaccines as novel therapeutic tools in malignant disorders. J. Cancer Res. Clin. Oncol. 127, R1–R2. doi:10.1007/BF01470991
Schuyler, M., Lyons, C. R., Masten, B., and Bice, D. (1997). Immunoglobulin response to intrapulmonary immunization of asthmatics. Immunology 91, 167–175. doi:10.1046/j.1365-2567.1997.00234.x
Sidell, N., Connor, M. J., Chang, B., Lowe, N. J., and Borok, M. (1990). Effects of 13-cis retinoic acid therapy on human antibody responses to defined protein antigens. J. Investig. Dermatol. 95, 597–602. doi:10.1111/1523-1747.ep12505593
Smith, A. J., Vollmer-Conna, U., Bennett, B., Hickie, I. B., and Lloyd, A. R. (2004). Influences of distress and alcohol consumption on the development of a delayed-type hypersensitivity skin test response. Psychosom. Med. 66, 614–619. doi:10.1097/01.psy.0000130962.28801.af
Smith, A., Vollmer-Conna, U., Bennett, B., Wakefield, D., Hickie, I., and Lloyd, A. (2004). The relationship between distress and the development of a primary immune response to a novel antigen. Brain Behav. Immun. 18, 65–75. doi:10.1016/s0889-1591(03)00107-7
Smith, A., Vollmer-Conna, U., Geczy, A., Dunckley, H., Bennett, B., Hickie, I., et al. (2005). Does genotype mask the relationship between psychological factors and immune function? Brain Behav. Immun. 19, 147–152. doi:10.1016/j.bbi.2004.06.005
Smith, T. P., Kennedy, S. L., and Fleshner, M. (2004). Influence of age and physical activity on the primary in vivo antibody and T cell-mediated responses in men. J. Appl. Physiol. 97, 491–498. doi:10.1152/japplphysiol.01404.2003
Snyder, B. K. K., Roghmann, K. J. J., and Sigal, L. H. H. (1993). Stress and psychosocial factors: Effects on primary cellular immune response. J. Behav. Med. 16, 143–161. doi:10.1007/BF00844890
Sokal, J. E. (1975). Editorial: Measurement of delayed skin-test responses. N. Engl. J. Med. 293, 501–502. doi:10.1056/NEJM197509042931013
Spazierer, D., Skvara, H., DawidM., , FallahiN., , Gruber, K., Rose, K., et al. (2009). T helper 2 biased de novo immune response to Keyhole Limpet Hemocyanin in humans. Clin. Exp. Allergy 39, 999–1008. doi:10.1111/j.1365-2222.2008.03177.x
Spencer, L. A., and Weller, P. F. (2010). Eosinophils and Th2 immunity: Contemporary insights. Immunol. Cell. Biol. 88, 250–256. doi:10.1038/icb.2009.115
Swaminathan, A., Harrison, S. L., Ketheesan, N., van den Boogaard, C. H. A., Dear, K., Allen, M., et al. (2019). Exposure to solar UVR suppresses cell-mediated immunization responses in humans: The Australian ultraviolet radiation and immunity study. J. Investig. Dermatol. 139, 1545–1553. doi:10.1016/j.jid.2018.12.025
Swaminathan, A., Lucas, R. M., Dear, K., and McMichael, A. J. (2014). Keyhole limpet haemocyanin - a model antigen for human immunotoxicological studies. Br. J. Clin. Pharmacol. 78, 1135–1142. doi:10.1111/bcp.12422
Swanson, M. A., and Schwartz, R. S. (1967). Immunosuppressive therapy. The relation between clinical response and immunologic competence. N. Engl. J. Med. 277, 163–170. doi:10.1056/NEJM196707272770401
Thiha, A., and Ibrahim, F. (2015). A colorimetric enzyme-linked immunosorbent assay (ELISA) detection platform for a point-of-care dengue detection system on a lab-on-compact-disc. Sensors 15 (5), 11431–11441. doi:10.3390/s150511431
Valdez, H., Smith, K. Y., LandAy, A., Connick, E., Kuritzkes, D. R., Kessler, H., et al. (2000). Response to immunization with recall and neoantigens after prolonged administration of an HIV-1 protease inhibitor-containing regimen. ACTG 375 team. AIDS Clinical Trials Group. AIDS 14, 11–21. doi:10.1097/00002030-200001070-00002
Vercelli, D. (2000). One cytokine, two isotypes: A trojan horse, pandora’s box, and an evolving paradigm. Am. J. Respir. Crit. Care Med. 162, S86–S90. doi:10.1164/ajrccm.162.supplement_2.ras-6
Vignali, D. A. A. (2000). Multiplexed particle-based flow cytometric assays. J. Immunol. Methods 243, 243–255. doi:10.1016/s0022-1759(00)00238-6
Volkman, D. J., Lane, H. C., and Fauci, A. S. (1981). Antigen-induced in vitro antibody production in humans: A model for B cell activation and immunoregulation. Proc. Natl. Acad. Sci. U. S. A. 78, 2528–2531. doi:10.1073/pnas.78.4.2528
Vukmanovic-Stejic, M., Reed, J. R., Lacy, K. E., Rustin, M. H. A., and Akbar, A. N. (2006). Mantoux Test as a model for a secondary immune response in humans. Immunol. Lett. 107, 93–101. doi:10.1016/j.imlet.2006.08.002
Waldo, F. B., Wall Bake, A. W. v. d., Mestecky, J., and Husby, S. (1994). Suppression of the immune response by nasal immunization. Clin. Immunol. Immunopathol. 72, 30–34. doi:10.1006/clin.1994.1103
Ward, M. M. M., Hall, R. P. P., and Pisetsky, D. S. S. (1990). Serum interleukin-2 receptor responses to immunization. Clin. Immunol. Immunopathol. 57, 120–124. doi:10.1016/0090-1229(90)90027-n
Weide, B., Pascolo, S., Scheel, B., Derhovanessian, E., Pflugfelder, A., Eigentler, T. K., et al. (2009). Direct injection of protamine-protected mRNA: Results of a phase 1/2 vaccination trial in metastatic melanoma patients. J. Immunother. 32, 498–507. doi:10.1097/CJI.0b013e3181a00068
White, S. (2021). Sanofi buys Kymab for up to $1.5 billion to expand in immunotherapy. Reuters. Available at: https://www.reuters.com/article/us-sanofi-immunotherapy-idUSKBN29G0RT.
Wimmers, F., de Haas, N., Scholzen, A., Schreibelt, G., Simonetti, E., Eleveld, M. J., et al. (2017). Monitoring of dynamic changes in Keyhole Limpet Hemocyanin (KLH)-specific B cells in KLH-vaccinated cancer patients. Sci. Rep. 7, 43486. doi:10.1038/srep43486
Wishahi, M. M., Ismail, I. M., Ruebben, H., and Otto, T. (1995). Keyhole-limpet hemocyanin immunotherapy in the bilharzial bladder: A new treatment modality? Phase II trial: Superficial bladder cancer. J. Urology 153, 926–928. doi:10.1016/s0022-5347(01)67605-5
Yang, J., Lickliter, J. D., Hillson, J. L., Means, G. D., Sanderson, R. J., Carley, K., et al. (2021). First-in-human study of the safety, tolerability, pharmacokinetics, and pharmacodynamics of ALPN-101, a dual CD28/ICOS antagonist, in healthy adult subjects. Clin. Transl. Sci. 14, 1314–1326. doi:10.1111/cts.12983
Keywords: keyhole limpet hemocyanin, immunotherapy, immune response, challenge model, late-phase skin reaction, delayed-type hypersensitivity
Citation: Saghari M, Jansen MAA, Grievink HW, Rissmann R and Moerland M (2022) Characterization of KLH-driven immune responses in clinical studies: A systematic review. Front. Drug. Discov. 2:992087. doi: 10.3389/fddsv.2022.992087
Received: 03 August 2022; Accepted: 14 October 2022;
Published: 25 October 2022.
Edited by:
Stephen Geoffrey Ward, University of Bath, United KingdomReviewed by:
Zhifang Zhou, Jiangnan University, ChinaSayed K. Goda, University of Derby, United Kingdom
Copyright © 2022 Saghari, Jansen, Grievink, Rissmann and Moerland. This is an open-access article distributed under the terms of the Creative Commons Attribution License (CC BY). The use, distribution or reproduction in other forums is permitted, provided the original author(s) and the copyright owner(s) are credited and that the original publication in this journal is cited, in accordance with accepted academic practice. No use, distribution or reproduction is permitted which does not comply with these terms.
*Correspondence: Matthijs Moerland, mmoerland@chdr.nl