- 1Swedish Cancer Institute, Swedish Medical Center, Seattle, WA, United States
- 2Institute for Systems Biology, Seattle, WA, United States
- 3Swedish Center for Research and Innovation, Swedish Medical Center, Seattle, WA, United States
- 4Department of Anesthesiology, Swedish Medical Center, Seattle, WA, United States
- 5Center for Cardiovascular Analytics, Research and Data Science (CARDS), Providence Heart Institute, Providence Research Network, Portland, OR, United States
- 6Division of Infectious Diseases, Swedish Medical Center, Seattle, WA, United States
- 7Division of Allergy and Infectious Diseases, Department of Medicine, University of Washington, Seattle, WA, United States
Background: Safe, effective, and inexpensive treatment for COVID-19 is an urgent unmet medical need. Zinc and resveratrol have been reported to have antiviral activity, and resveratrol may increase zinc activity at the site of replication by increasing intracellular zinc concentrations.
Methods: A 1:1 randomized, placebo-controlled trial of zinc 150 mg plus resveratrol 4 g daily for 5 days versus placebos in outpatients with SARS-CoV-2 was carried out from 9/21/2020–1/22/2021 in Seattle, Washington. Viral shedding was followed with patient self-collected nasal and saliva samples by measuring qRT-PCR for SARS-CoV-2 N gene days 1–7, 10, and 14. Patients filled out a web-based questionnaire on days 1–14 to report symptoms, vital signs and adherence to the study intervention. The study was posted as Clinical Trials.gov NCT04542993 on 9 September 2020.
Results: A total of 30 participants (14 treatment; 16 placebos) had ≥1 day of the protocol treatment and were evaluable for the primary or secondary outcome. There was no difference in viral shedding between groups, nor in the resolution of symptoms. There was a trend toward a more rapid decrease in symptoms in the treatment group, though this was not statistically significant in the GLM model. Viral shedding was similar between patient self-collected mid-turbinate nasal swabs and expectorated saliva samples with a good correlation.
Conclusion: SARS-CoV-2 shedding and COVID-19 symptoms were not statistically significantly decreased by treatment. Viral shedding correlates well between patient-obtained home nasal swabs and saliva sampling.
1 Introduction
There is a pressing need to develop therapies to inactivate the SARS-CoV-2 virus early in the course of infection to prevent severe illness and mortality. A number of antivirals, monoclonal antibodies, convalescent plasma, and immunotherapies are in clinical use or under investigation, but all of these treatments are costly and require interactions with the medical system. An oral, readily available, easy to tolerate, and inexpensive treatment that does not require in-person appointments to administer is urgently needed.
Zinc, a vital element for human health, has demonstrated antiviral activity for other respiratory viruses (Suara and Crowe, 2004; Krenn, 2009), and has been proposed as a treatment for SARS-CoV-2 (Wessels et al., 2020). Zinc deficiency is common in the elderly (Fosmire, 1990; Kvamme et al., 2015) and has been correlated with increased severity of COVID infection (Jothimani et al., 2020), and advanced age is the strongest risk factor for death from COVID-19 (Centers for Disease Control and Prevention, 2019). Data on the effects of zinc supplementation on COVID-19 are mixed: one retrospective study reported that patients hospitalized with COVID-19 receiving zinc supplementation were more likely to be discharged home and had reduced mortality or transfer to hospice (Carlucci et al., 2020). However, a randomized trial of zinc gluconate utilizing 50 mg daily ± 8 g of ascorbic acid daily versus placebo for 10 days failed to demonstrate a significant impact on COVID-related symptoms in outpatients with mild COVID-19 (Thomas et al., 2021). A study of hospitalized patients with COVID-19 randomized patients to hydroxychloroquine ± 50 mg zinc daily for 5 days. There was no difference in clinical recovery, need for mechanical ventilation, or overall mortality at 28 days (Abd-Elsalam et al., 2021).
Zinc has demonstrated the ability to directly inhibit the activity of RNA-dependent RNA polymerase (RdRp) in the cytoplasm for SARS-1 (2003) and other positive-sense RNA viruses which use RdRp (Kaushik et al., 2017; Read et al., 2019). Zinc is not inherently bioavailable, making it difficult to achieve high intracellular concentrations. When zinc is added to cell lines with an ionophore, such as chloroquine, intracellular zinc levels rise sharply, raising the possibility that a non-toxic ionophore could potentiate its antiviral activity (te Velthuis et al., 2010; Xue et al., 2014) (Supplementary text).
Resveratrol is a naturally occurring polyphenol stilbene with possible antiviral actions (Supplementary text). However, it is unknown whether clinically achievable concentrations of resveratrol would have anti-SARS-CoV-2 activity in vivo. Studies of benign and malignant human prostate tissue suggest that resveratrol might act as an ionophore at clinically safe doses, serving as a means to increase intracellular zinc, thereby potentiating the antiviral property of zinc (Zhang et al., 2009) (Supplementary text).
Zinc has been used in chronic doses of 150 mg daily to treat Wilson’s disease (Brewer and Yuzbasiyan-Gurkan, 1992), though at least 6 weeks of treatment are necessary to cause copper depletion (Sammans and Roberts, 1987). Resveratrol has been shown to be safe and tolerable at doses of up to 4 g daily (la Porte et al., 2010). For a full description of the rationale for dosing zinc and resveratrol, please see the Supplementary text.
In this study, we conducted a 1:1 randomized, placebo-controlled phase I/II trial of 5 days of oral zinc plus resveratrol at maximal single-agent reported safe doses in outpatients with COVID-19. The daily zinc dosage in this study was three times that utilized in the trials mentioned earlier (Abd-Elsalam et al., 2021; Thomas et al., 2021). We monitored viral shedding by both mid-turbinate nasal and saliva sampling (da Silva et al., 2020; Kivela et al., 2021; Butler- LaPorte et al., 2021; Wyllie et al., 2020; Bastos et al., 2021) as well as self-reported symptomatology, including drug toxicities for 14 days.
2 Materials and Methods
2.1 Study Population and Recruitment
We studied ambulatory persons with newly diagnosed SARS-CoV-2 infection and mild-moderate COVID-19 symptoms. Patient eligibility requirements included the following: a positive COVID-19 nasopharyngeal swab within 4 days of randomization, age 18–75 years, not requiring supplemental oxygen, English-speaking, having valid contact information, including email (given remote procedures), consenting to access to their medical records, and living within 25 miles of the medical facility (to allow specimen delivery and pickup). Exclusions included the following: abnormal liver or kidney function, allergy to zinc or resveratrol, prior COVID infection, lung disease requiring supplemental oxygen, cognitive dysfunction, current substance abuse or psychiatric disorder, pregnancy, lactation, and anticoagulant use. Only one patient per household could enroll, as placebo capsules were not visually identical to zinc and resveratrol capsules.
The study team actively screened lists of patients with newly diagnosed SARS-CoV-2 infection, approaching eligible persons via telephone. Informed consent was obtained with electronic consent forms by the principal investigator. Medication (or placebo), a thermometer, pulse oximeter, and materials for obtaining viral samples (see below) were sent to the patient’s home by a commercial delivery. Enrollment occurred between 9/21/2020–1/22/2021, a time when the Delta and Omicron variants were not circulating in the community. The study (Clinical Trials.gov NCT04542993) was approved by the Providence St Joseph Health Care System IRB and carried out within their guidelines and regulations. Study accrual was ended coincident with a marked decrease in eligible patients in the health care system, which coincided with the end of the “third wave” in Washington State.
Participants were randomized 1:1 between zinc plus resveratrol and placebo by a research coordinator using a REDCap database randomization feature. An unblinded research pharmacist dispensed the capsules via home delivery service. The research coordinator and pharmacist were the only unblinded individuals, while all investigators, laboratory scientists, and data analysts were blinded to the study group allocation. For purposes of statistical analysis, the groups were labeled “A” and “B” such that all statistical analyses regarding both viral shedding and symptom reporting were carried out without the knowledge of the participant study group, nor which arm was the intervention arm. Randomization results for two patients were unblinded because of hospitalization.
2.2 Study Procedures
Participants were instructed to take zinc methionine/cysteine (Life Extension) 50 mg capsules orally three times daily and resveratrol (Mega Resveratrol) 2000 mg orally twice daily or placebo capsules for 5 days. Patients were followed clinically and with samples for viral quantification. Clinical follow-up included daily web-based REDCap questionnaires, assessment of the electronic medical record, and direct phone and email conversations. Symptom questionnaires were recorded daily from days 1–14 via the internet in REDCap. Participants were asked to rate their symptoms as none (0 points), very mild (1 point), mild (2 points), moderate (3 points), or severe (4 points). Symptoms assessed included chest congestion, cough, runny nose/sneezing, fatigue, body aches, diarrhea, difficulty breathing, abdominal pain, headache, nausea, difficulty sleeping, and “other”. The total daily symptom score was calculated daily as the severity points for each symptom, summed across all symptoms, with a possible maximum of 44 for the 11 scaled variables. The symptoms were derived from the CDC Covid-19 symptom list (https://www.cdc.gov/coronavirus/2019-ncov/symptoms-testing/symptoms.htmlpatient). Temperature, pulse, and oxygen saturation were also recorded daily by the participant with medical equipment supplied by the study.
Participants were asked to document study medication ingestion on days 1–5 via REDCap and describe any adverse effects related to the study drugs. Participants were assessed at 30 and 90 days for hospitalization and death. Participants were asked to self-collect both nasal and saliva samples for SARS-CoV-2 qRT-PCR testing on days 1–7, 10, and 14. Participants were instructed on how to obtain the samples with written material, a web-based video of the nasal swab procedure, and verbal instruction by phone from a research coordinator and/or nurse. A flocked swab was inserted into the bilateral mid-turbinate area of the nose and rotated five times against the nasal mucosa, then inserted into a sterile tube containing viral transport media. Saliva was collected by expectoration into a dry and sterile 50 cc test tube. Samples were refrigerated at home until retrieval within 72 h. Participants were asked to place samples in a box with cool packs and leave them by the door where they were picked up by the delivery service and brought to the lab the same day and stored at −80°C until analyzed. If a patient was hospitalized, the study drug or placebo intervention was stopped, but follow-up and viral swab and saliva collection continued whenever possible. All data were stored in an encrypted database at the Swedish Cancer Institute.
2.3 Laboratory Analysis
The nasal swabs and saliva samples were thawed and spun at 1,000 x g for 10 min to remove cells and cell debris. The MiRNeasy Kit (Qiagen, Germantown, MD) was used to isolate RNA from 100 ul of either nasal swab transport medium or saliva samples according to the manufacturer’s instruction. RNA was eluted from the membrane with 50 ul of RNAse-free water. To detect and quantify SARS-CoV-2 the CDC protocol (https://www.fda.gov/media/134922/download) was followed using primer pair N1 to measure the SARS-CoV-2 N gene level (Integrated DNA Technologies, Coralville, IA) and qRT-PCR was performed on a CFX-96 qPCR machine (Bio-Rad, Hercules, CA). SARS-CoV-2 RNA N gene was quantified as the cycle threshold (CT), with a value ≤40 cycles indicating positivity. The qRT-PCR was carried out according to the following conditions: 1 cycle at 25°C for 2 min, 1 cycle at 50°C for 15 min, 1 cycle at 95°C for 2 min, and 45 cycles at 95°C for 3 s and 55°C for 30 s. Human RNase was also quantified under the same qRT-PCR conditions as a housekeeper gene, with a CT ≤ 40 indicating the adequacy of the nasal or saliva sample.
2.4 Statistical Analyses
The primary outcome was a reduction in viral shedding based on the cycle threshold (CT) of qRT-PCR. Secondary outcomes included reduction of symptoms, adverse events, incidence and length of hospitalization, days of ventilator support, and drug compliance. Participants were included in the per-protocol analysis if they collected and returned at least 1 sample or documented taking at least 1 dose of the study drug/placebo. Study subjects with an active intervention were compared against placebo subjects across mean SARS-CoV-2 PCR cycle results for nasal and saliva samples, and the sum of daily symptoms, using an independent sample t-test for each test day (days 1, 2, 3, 4, 5, 6, 7, 10, and 14) and Levene’s test for equality of variance. Paired sample t-tests were calculated between nasal and saliva cycle values across each treatment day to investigate potential differences or the equivalence of the two viral measures. To assess the overall decay in viral shedding and COVID-19–related symptoms, we implemented a generalized linear mixed (GLM) model by restricted maximum likelihood method to analyze the repeat measurements of nasal and saliva cycle values and total daily symptom scores. The models are separate for viral shedding and symptoms, and each model includes fixed effects terms for the treatment group, study day, and an interaction term for treatment group and day. The degrees of freedom for each coefficient were calculated using Satterthwaite’s method and the p-values calculated accordingly. The GLM model calculates an intercept (interpreted as the baseline viral N gene PCR CT on day zero prior to the first day of samples collection) and the linear slope of the increase in N gene PCR CT value (interpreted as the exponential decay in viral shedding, with an increasing change of 1 CT estimating half as much viral RNA in the sample). To disaggregate by sex, gender, and age, we included these variables in an adjusted GLM model. In this population, gender and sex were equivalent for all subjects. Thus, only sex was included. Pearson correlation between N gene PCR CT for nasal swabs and saliva samples was performed. The confidence interval of correlation was calculated based on the sample size. For purposes of the correlation analysis, swabs without detectable viral RNA after 45 cycles were assigned a value of 46. In the figure, we indicated the pre-specified value for negative (CT > 40) and all CT values. Pearson correlation coefficient, R, and 95% CI were given, with the p-value. A separate GLM model was run to assess the repeated measures of symptoms over time. Interpretation of the symptoms GLM model is similar to the viral shedding repeated measures, with the total summated symptom/severity score assessed with a linear fit over time. Analyses were performed with IBMSPSS 22, Armonk New York, and R version 4.1.0, with packages “lme4,” “jtools,” “ggpubr,” and “psychometric” (Core Team, 2021). A power calculation was not pre-specified, and a convenience sample was selected for this exploratory Phase ½ study.
3 Results
Of 145 COVID-19 patients who were approached for participation, 45 consented and were enrolled. Exclusions included nine participants who did not submit any viral samples, two whose viral positivity could not be confirmed, two who were discovered to have had a prior episode of COVID-19 after enrollment but prior to initiating any study procedures, and two who were admitted to hospital within 24 h of enrollment and did not take study medication or submit samples. None of these excluded patients was included in the per-protocol analysis as no data were returned to assess endpoints. In addition to the two excluded persons hospitalized prior to initiating the study intervention, no other excluded patients were hospitalized to our knowledge, but contact was not possible for most of these excluded persons. Finally, there were 14 in the treatment group and 16 in the placebo group (Figure 1). For the remaining 30 participants, mean age was 46.3 (SD = 17.4) for the treatment group and 38.5 (SD = 9.9) for the placebo group (p = 0.14 for means; p = 0.002 by Levenes test for equality of variance). The treatment group had six males and eight females. The placebo group had six males and ten females. There were two Hispanic/LatinX, three African Americans, four Asians, and 21 Caucasians. Number of days of symptoms prior to study day 1 was 5.86 (SD = 2.92) for treatment and 5.44 (SD = 1.84) for placebo (p = 0.46) for means (Table 1). None of these participants had received vaccination against SARS-CoV-2.
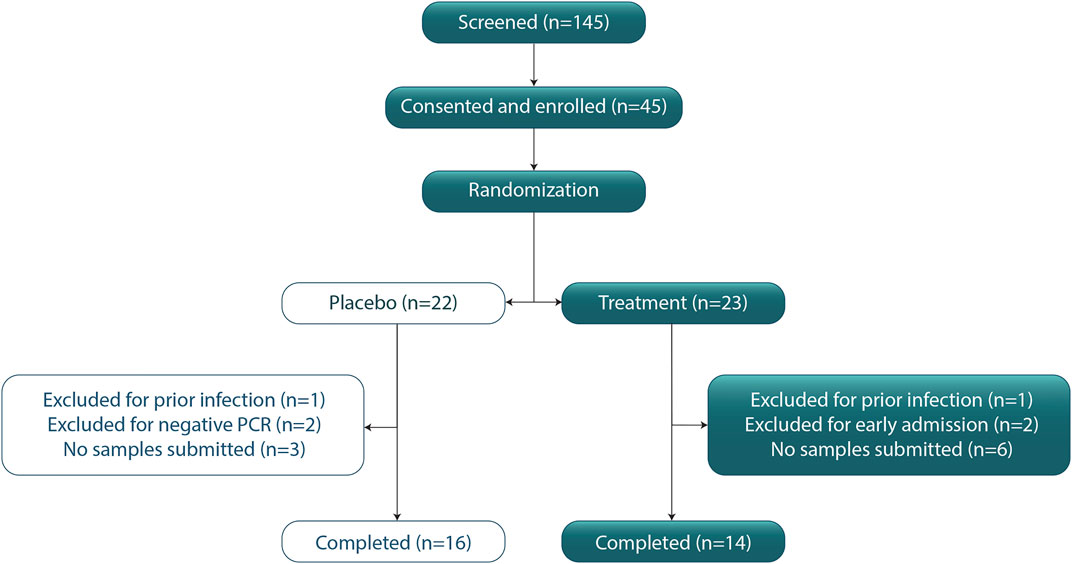
FIGURE 1. CONSORT Diagram. CONSORT diagram indicating patients screened, participants consented, enrolled, and randomized to the study arm. After excluding patients, there were n = 16 persons in the placebo group and n = 14 persons in the treatment (zinc plus resveratrol) group who were randomized and returned at least 1 sample for PCR analysis or submitted 1 symptom screen.
3.1 Virological Assessments
In total, 234/270 (87%) nasal and 230/270 (85%) saliva samples were returned for the 30 included participants for 9 different days (Figure 2). Between the treatment groups, no significant differences were seen in viral load from nasal swabs (Figure 2A) or saliva samples (Figure 2B) across any study collection days. On day 1, the median (IQR) CT for nasal viral load in the treatment group was 27.99 (25.41–31.87) and for the placebo group was 26.23 (24.21–30.52). Median (IQR) treatment saliva viral on day 1 was 27.49 (25.05–30.14), while placebo was 29.18 (24.82–33.97). By day 5, median (IQR) treatment nasal viral was 34.19 (32.36–36.33), and placebo was 31.88 (28.45–34.26). Median (IQR) treatment saliva viral on day 5 was 35.13 (33.57–36.20), while placebo was 34.42 (34.15–35.66). RNase P (housekeeper gene) was present in all returned samples (positive and negative), suggesting the adequacy of these samples.
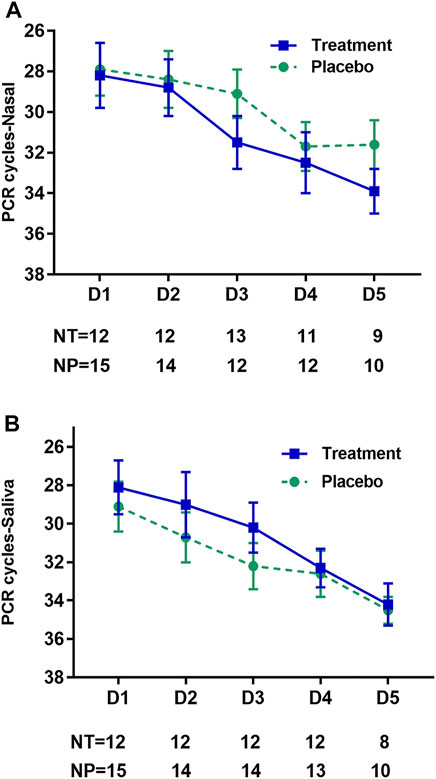
FIGURE 2. Effect of treatment on viral shedding by day of study. Representation of viral shedding data in nasal swab samples (A) and saliva samples (B) by study collection day for all samples which detected virus, N gene PCR cycle threshold (CT) ≤ 40. The mean CT value is indicated on the y-axis for the treatment (zinc plus resveratrol) group by the square (blue) and for the placebo group by the circle (green), with standard error for both groups indicated by the whiskers. On the x-axis is the study day of sampling. The table below the x-axis gives the number of participants contributing specimens at each time point. NT, number in the treatment (zinc plus resveratrol) group; NP, number in the placebo group.
In the GLM model of viral shedding from nasal swabs, viral shedding in both study groups decayed significantly over the study interval from day 1 to day 14, as measured by the SARS-CoV-2 N gene PCR CT value, which had an average increase in CT per day of 1.00 cycles (95% CI 0.64–1.36), p < 0.001 for the zinc plus resveratrol group, and 1.33 cycles (95% CI 1.01–1.66), p < 0.001 for the placebo group, consistent with a dynamic decay in shedding seen in other studies (Weinreich et al., 2021a; Core Team, 2021; Iwanami et al., 2021). The GLM model predicts baseline CT = 28.1 in the placebo group and 29.2 in the treatment group, and these model estimates did not differ statistically (p = 0.70). The slope of the increase in CT was 0.33 less in the treatment compared to placebo groups, which was not statistically significant, p = 0.20. Including age and sex into the model did not alter the estimate of treatment effect (Table 2).
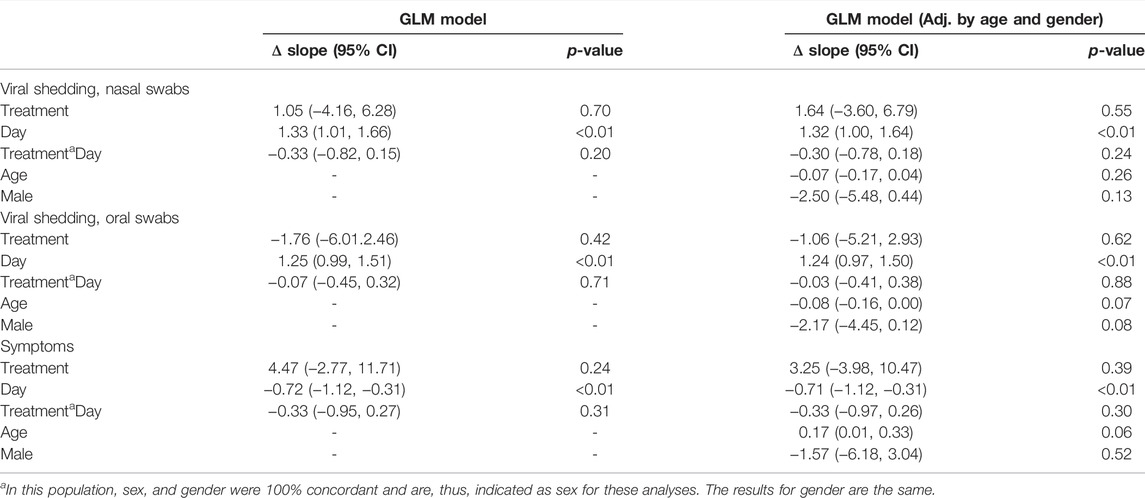
TABLE 2. GLM models, adjusted for age and sex. We present the change in slope of the decay in viral shedding or symptoms and the effect of treatment for unadjusted analysis or adjusted by age, sex, or both. For viral shedding analyses, the change in slope is the rise in CT (cycles per day), and for the symptom analysis, the change in slope is the decrease in symptoms per day.
In the GLM model of viral shedding from saliva samples, viral shedding in both study groups decayed significantly over the study interval from day 1 to day 14, as measured by the SARS-CoV-2 N gene PCR CT value, which had an average increase in CT per day of 1.18 cycles (95% CI 0.90–1.46), p < 0.001 for the treatment group and 1.25 cycles (95% CI 0.99–1.51), p < 0.001 for the placebo group (Table 2). The GLM model predicts baseline CT = 28.2 in the treatment group and 30.0 in the placebo group, and these model estimates did not differ statistically (p = 0.42). The slope of the increase in CT was not different in the treatment compared to placebo groups, p = 0.71. Together, the results indicate that the reduction in shedding over the observation period was not different between the study arms. Including age and sex into the model did not alter the estimate of treatment effect (Table 2).
Of 234 returned nasal swabs and 230 returned saliva samples, 229 paired specimens were available for both sample types (Figure 3). Of paired specimens, 145 (63.3%) were concordant positive (Figure 4A), and 41 (17.9%) were concordant negative, at a CT cutoff of >40. Discordant paired samples included 23 (10.0%) with nasal swab positive and saliva negative and 20 (8.7%) with nasal swab negative and saliva positive. 12/23 (52%) discordant paired samples with nasal swab positive and saliva negative and 14/20 (70%) discordant paired samples with nasal swab negative and saliva positive were noted at CT > 35. Pearson’s correlation coefficient, R was 0.67 (95%CI: 0.59–0.74), p < 0.001. Considering only positive samples (SARS-CoV-2 N gene PCR CT < 40 cycles) in 145 pairs (63%) shows rapid decay of viral shedding in both sample types (Figure 4A). In pairs with at least 1 negative sample, the proportions of nasal negative, saliva negative, or both negative increased throughout the 14-day observation period (Figure 4B). No significant differences were seen in viral load between nasal and saliva samples across any study collection days.
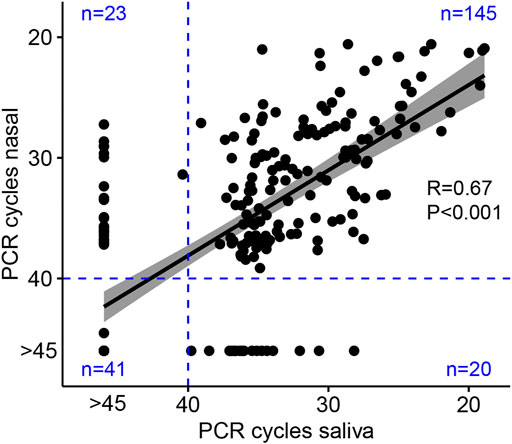
FIGURE 3. Correlation of viral shedding between participant self-collected nasal and saliva samples. Points indicate the cycle threshold (CT) for expectorated saliva sample versus nasal mid-turbinate swab sample collected concurrently on the same day. Linear regression with 95% confidence intervals is plotted, and Pearson’s correlation coefficient (R) is given as a measure of linear association between the two variables. The N gene PCR protocol ran 45 PCR cycles, and pre-specified CT results >40 were negative. Blue lines indicate the pre-specified negative results with numbers of paired daily samples given in each quadrant. Samples which did not amplify after 45 PCR cycles are reassigned a value of CT = 46 for correlation analysis and represented on the figure as “CT > 45”.
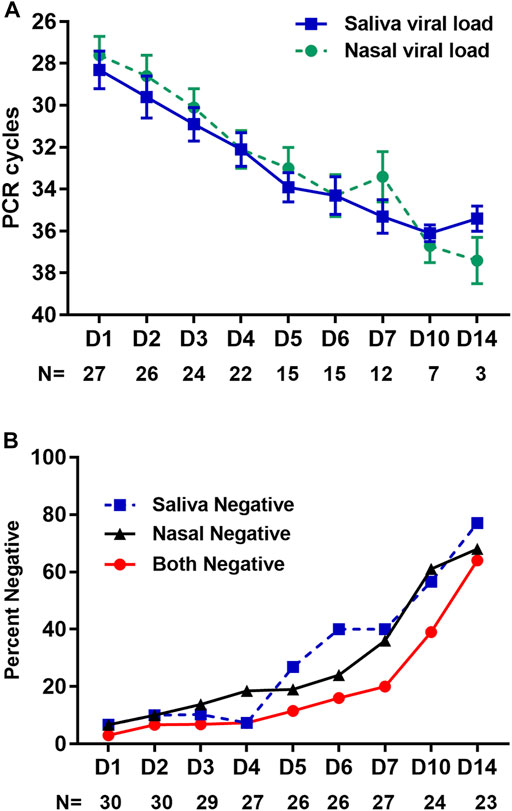
FIGURE 4. Correlation of viral shedding between nasal and saliva samples by day of study. (A) Comparison of viral shedding data in nasal swab and saliva samples. SARS-CoV-2 N gene PCR cycle threshold (CT) is given on the y-axis for participant self-collected nasal mid-turbinate swab (circle, green) versus saliva (square, blue) samples by study collection day on the x-axis. Mean CT is given by the respective symbol and standard error by the whiskers. Below the x-axis is the number of participants contributing specimens at each time point. Results are shown for all samples in which both nasal and saliva SARS-CoV-2 RNA was detected (N gene PCR CT < 40 cycles), n = 145. (B) Percent of samples collected, for which housekeeper gene PCR indicated adequate sampling, yet no detectable viral load was present in saliva or nasal swab, respectively.
In post-hoc analyses, there were no differences when adjusting for age or sex. Day 1 nasal swab CT results correlated with the severity of symptom scores for days 1 through 4 (p <0 .05), while there was only one time point with a statistically significant correlation between any saliva value and symptoms.
3.2 Clinical Assessments
Symptom surveys were returned for 141/196 (72%) days for the treated group, including 62/70 (89%) for the first 5 days, and for 196/224 (88%) for the placebo group, including 76/80 (95%) for the first 5 days. There were no statistically significant differences between treatment and placebo groups with regard to the frequency with which individual symptoms were reported (Figure 5). In the symptom reports under “other” five of 14 (36%) treatment arm and 6/16 (38%) placebo arm participants reported a loss of taste or smell. Fever (≥99°F) was recorded on day 15/123 (12%) in the treatment groups and on day 27/192 (14%) in the placebo group. Hypoxia (Sp02 ≤ 94%) was recorded on day 11/123 (9%) in the treatment group and on day 1/192 (0.5%) in the placebo group. Daily total participant symptom scores which sum number and severity of symptoms were higher in the treatment group on the first 4 days, but thereafter, were numerically higher or equal in the placebo group, though no days found statistically significant group differences (Figure 6).
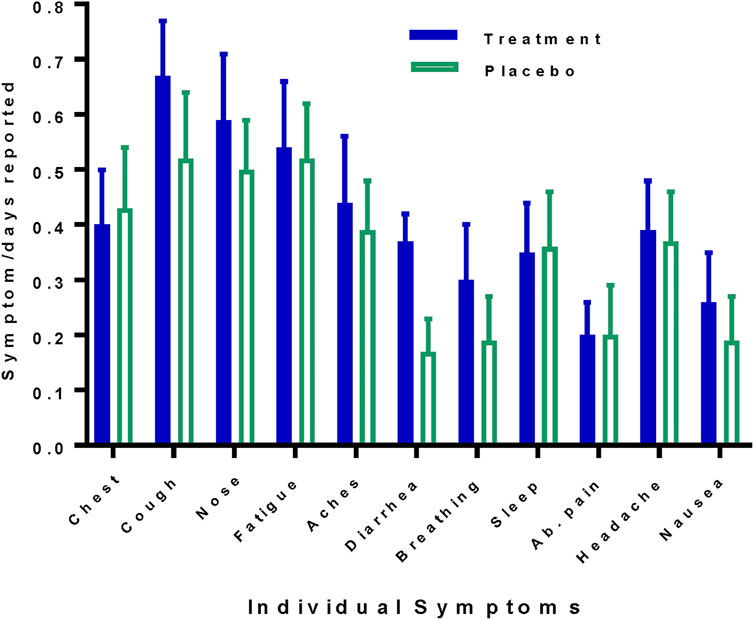
FIGURE 5. Frequency of individual symptoms reported by the study arm. Symptoms are given as the fraction of days reported and shown for the treatment (zinc plus resveratrol) group, solid bars (blue) versus the placebo group, open bars (green). Chest, chest congestion; nose, runny nose; aches, body aches; breathing, difficulty breathing, sleep, trouble sleeping. Whiskers indicate SEs.
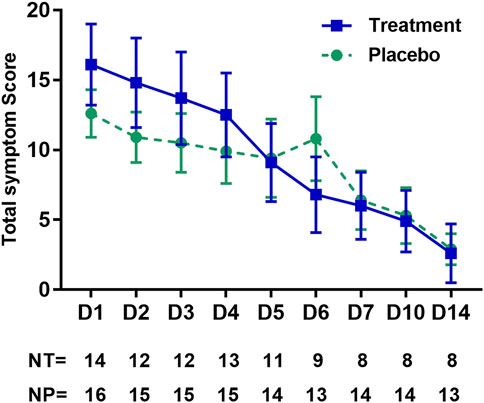
FIGURE 6. Total daily patient-reported symptom scores by day of study. The total symptom score is indicated on the y-axis and is calculated daily for each participant as the severity points for each symptom, summed across all symptoms. Whiskers indicate SEs. Days of collection are indicated on the x-axis, and the number of participants reporting on the given collection day is indicated in the table below the x-axis. NT, number in the treatment (zinc plus resveratrol) group; NP, number in the placebo group.
In the GLM model of symptoms, the score in both study groups decreased significantly over the study interval from day 1 to day 14, as measured by the symptom score (which reflects the number and severity of COVID-19 symptoms). In the zinc plus resveratrol group, the total daily symptom score decreased by 1.1 points per day (95%CI 0.60–1.52), p < 0.001. In the placebo group, the total daily symptom score decreased by 0.72 points per day (95% CI 0.31–1.12), p = 0.003. The GLM model predicts a baseline symptom score of 12.1 in the placebo group and 16.5 in the treatment group, though these model estimates did not differ statistically (p = 0.24). The slope of the decrease in symptom score was 0.33 less in the treatment compared to placebo groups, which was not statistically significant, p = 0.31. Including age and sex into the model did not alter the estimate of treatment effect (Table 2).
Individual symptoms were examined across days between the treatment (zinc plus resveratrol) and placebo groups via assessment of the average severity score on each day. The only significant difference between the treatment and placebo groups was observed for diarrhea, which was greater in the treated group on day 1 (1.6 vs 0.6, p = 0.056), day 2 (1.8 vs 0.7, p = 0.046), day 3 (1.7 vs 0.4, p = 0.006), and day 4 (1.6 vs 0.07, p = 0.014), though a trend was seen for increases in cough, chest congestion, difficulty breathing, diarrhea, and abdominal pain in the treatment group. Two included participants were admitted to the hospital during the study period. One participant, who received active drug, was admitted on day 5 (having taken 4 days of zinc and 4.5 days of resveratrol). She experienced a 46-day hospitalization with 30 days in ICU and 23 days intubated. The other participant, who received placebo, was admitted on day 6. She had an 11-day hospitalization with 5 days in ICU and 4 days intubated. There were no deaths.
3.3 Medication Administration Compliance and Toxicity
In the treatment group, there were 5/70 (7%) self-reported days when capsules were not taken (three patients missed a single day and one missed 2 days) and 6/70 (9%) unknown. In the placebo group, there were 7/80 (9%) days when capsules were not taken (three patients missed 1 day and two patients missed 2 days) and 4/80 (5%) unknown. No symptoms that could be clearly attributed to study drugs were noted. As noted above, during the first 4 days symptom scores for nausea, diarrhea, and abdominal pain trended higher in the treatment group than in the placebo group, and diarrhea had statistically significantly higher scores. These are all known potential side effects of resveratrol. However, symptoms were present at the start of treatment, and the frequency of reports of these symptoms was the same in both groups (Figure 5). The symptom scores declined for all of these, including diarrhea, while the patients were still undergoing treatment. While we cannot exclude some contribution of drug toxicity to symptomatology, this pattern suggests that these symptoms were more disease-related rather than treatment-related.
4 Discussion
In this exploratory Phase 1/2 placebo-controlled RCT, we report on the possible utility of maximal doses of zinc and resveratrol to abate SARS-CoV-2 shedding and to ameliorate COVID-19 symptoms in ambulatory participants with mild—moderate COVID-19. Treatment was well tolerated, with no clear indication of significant toxicity, despite the utilization of much higher daily zinc doses than have been used in other studies for the treatment of SARS-CoV-2 (Abd-Elsalam et al., 2021; Thomas et al., 2021).
We did not detect any statistically significant differences in primary or secondary outcomes. Zinc plus resveratrol at the doses studied was well tolerated but did not reduce viral shedding nor total patient-reported symptoms in this Phase I/II trial. It is notable, however, that total symptom scores and a number of respiratory and gastrointestinal symptoms initially trended more severe in the treatment cohort, but by day 5, they had equalized between the two groups. In the GLM model, the slope of the decrease in symptoms was not different between the treatment and placebo arms, and we concluded that we could not detect any difference in the abatement of symptoms. Thus, further study of zinc plus resveratrol with a larger subject population is necessary to conclude if SARS-CoV-2 shedding or COVID-related symptoms are reduced by the study intervention. It is important to note that current infusion monoclonal antibodies required larger studies to demonstrate subtle reductions in virological shedding (Weinreich et al., 2021a; Weinreich et al., 2021b; Iwanami et al., 2021) despite clear signals in reducing untoward COVID-19 outcomes (Weinreich et al., 2021b). Thus, our Phase 1/2 trial was designed as an early proof of principle and not intended to definitively show efficacy.
Zinc has been noted to possess anti-inflammatory properties mediated through a variety of mechanisms, including modulation of multiple parts of the immune response and a variety of cytokines (Bonaventura et al., 2015; Gammoh and Rink, 2017; Olechnowicz et al., 2018). In addition, the zinc preparation used in this study contains both cysteine and methionine. Methionine helps zinc absorption and bioavailability. Levels of both of these amino acids have been shown to affect immune responses, and it has been postulated that deficiencies of these may affect the body’s immune response to SARS-CoV-2 (Mortaz et al., 2021; Pal et al., 2021) Furthermore, cysteine and methionine metabolism is known to be associated with COVID-19 disease severity, potentially indicating oxidative stress (Bannerman et al., 2021). It is unknown if the levels of these amino acids are altered by the preparation used here. Resveratrol has demonstrated multiple effects on the immune system, including anti-inflammatory properties, and has specifically been shown to reduce acute lung injury in a number of animal models (Malaguernera, 2019; Hoang, 2020; Levy et al., 2020; He et al., 2021; Lee et al., 2021). Thus, it is possible that the current intervention could impact the clinical course of COVID-19 independent of its effect on viral shedding or symptom resolution.
In participants who started the study and completed at least 1 study-related procedure, we were able to achieve over 90% compliance with regard to medication administration and collection of almost 90% of viral sample returns for the participants per protocol. Furthermore, we demonstrated the ability to obtain detailed serial viral shedding nasal and saliva samples from participants without the need for in-person instruction by medical personnel. This extends the work of McCulloch et al. (2020), who evaluated the concordance of a single mid-turbinate swab done by a health care professional with a patient self-collected sample taken at home to our study context in which participants self-collected both nasal swabs and saliva at home.
Exploratory studies of antiviral agents could be simplified by following viral decay in saliva samples alone. Saliva is easier and less uncomfortable for patient self-collection and correlates nicely with self-collected mid-turbinate swabs. A number of studies have documented the equivalence of mid-turbinate and saliva for assessing viral shedding for diagnosis (da Silva et al., 2020; Kivela et al., 2021; Butler- LaPorte et al., 2021; Wyllie et al., 2020; Bastos et al., 2021). A home saliva-based rapid point-of-care diagnostic test has also been developed (Joung et al., 2020). However, to our knowledge, this is the first study in which participants self-obtained serial samples of both at home without health care worker supervision for quantitative PCR (Altamirano et al., 20202020; Hanson et al., 2020; Lindner et al., 2020; Nagura -Ikeda et al., 2020; Tan et al., 2020; Teo et al., 2021; Torres et al., 2021). Our results show a high degree of correlation between these self-collected samples, with only minimal patient training from the study team. Finally, Ott et al. (2020) have demonstrated that saliva samples for SARS-CoV-2 RNA analysis are stable with home refrigeration without the need for RNA stabilization media and viral inactivation buffers reporting RNA stability for samples stored at −80°C for 92 days, 4°C for 21 days, and 30°C for 16 days. This study also demonstrated no change in the viral N gene by RT-qPCR, whether tested on the day of collection or after storage at - 80°C freeze/thaw, room temperature (5 days), or 30°C (3 days) (Ott et al., 2020). Our study moved samples from home refrigeration to −80°C within 72 h. Silva et al. reported a better correlation of saliva shedding with a number of parameters of disease severity and clinical outcomes compared to nasopharyngeal samples (Silva et al., 2021), which was not observed in our study, though we did observe the lower viral shedding in younger age participants that they reported. Further simplification of study procedures with simplified saliva collection and storage, as we showed in this study, will facilitate future outpatient SARS-CoV-2 studies.
The major limitations of this study are the small number of participants and the lag from disease onset to treatment initiation. There is a suggestion that symptoms decreased more rapidly in the treatment group than in the placebo group over the first 4 days. A larger study is needed to conclude whether zinc plus resveratrol might reduce symptoms. Unfortunately, many potential subjects were contacted to enroll the number of participants we accrued, and the study was terminated when the number of potential enrollees in our study dropped dramatically.
Viral respiratory diseases are very dynamic, with viral shedding decaying rapidly over the first week of infection (Wölfel et al., 2020). In the case of influenza, which has a shorter lag time from infection to symptom onset, antiviral treatment should be initiated within the first 2 days of symptoms to demonstrate benefit (Uyeki et al., 2019; Centers for Disease Control and Prevention, 2021). It is possible that earlier treatment with zinc and resveratrol might have a greater impact both on viral shedding and symptoms.
5 Conclusion
Here, we study the utility of a limited-duration, oral, inexpensive, non-toxic, readily available therapy that would not require physical interaction with the medical care system for patients who are quarantined at home. An oral treatment regimen is needed, which could be started by patients at the onset of viral exposure or symptoms and may be useful to ameliorate COVID-19. Zinc plus resveratrol was non-toxic but did not demonstrate efficacy in reducing SARS-CoV-2 shedding or overall COVID-19 symptoms. Though the study numbers are too small to detect differences, a trend toward more rapid symptom abatement of certain symptoms would need to be studied in a larger trial to detect a treatment effect, if one exists. Our remote procedures and paired mid-turbinate nasal swabs and saliva sample collection were feasible without direct medical supervision and showed a good correlation between viral shedding in the different specimen types. The approach presented here could be used to evaluate a larger population and earlier initiation of treatment, including prophylaxis after a high-risk exposure or other oral regimens with possible anti-SARS-CoV-2 activity.
Data Availability Statement
The raw data supporting the conclusion of this article will be made available by the authors, without undue reservation.
Ethics Statement
The studies involving human participants were reviewed and approved by Providence St Joseph Health Institutional Review Board. The patients/participants provided their written informed consent to participate in this study.
Author Contributions
Conceptualization: HK, CN, DK, JS, and JG. Data Curation: HK, KW, KR, JS, and RJ. Analysis: JS and RJ. Investigation: all authors. Funding: HK. Methodology: HK, KW, CN, JS, RJ, and JG. Supervision: HK and JG. Writing—original draft: HK. Writing—review and editing: all authors. Final approval of the manuscript: all authors.
Funding
This study was supported by the Kaplan Cancer Research Fund.
Conflict of Interest
JDG reports research support from Lilly, Gilead, and Regeneron; grants from the NIH, BARDA (administered by Merck), and Viracor to his institution; and speaker or consulting personal fees from Lilly, Gilead, and Mylan.
The remaining authors declare that the research was conducted in the absence of any commercial or financial relationships that could be construed as a potential conflict of interest.
Publisher’s Note
All claims expressed in this article are solely those of the authors and do not necessarily represent those of their affiliated organizations, or those of the publisher, the editors, and the reviewers. Any product that may be evaluated in this article, or claim that may be made by its manufacturer, is not guaranteed or endorsed by the publisher.
Acknowledgments
The authors would like to thank the study participants for their participation in this study and the Swedish Hospital Medical Center COVID-19 Research Steering Committee for their assistance in carrying out this study.
Supplementary Material
The Supplementary Material for this article can be found online at: https://www.frontiersin.org/articles/10.3389/fddsv.2022.910124/full#supplementary-material
References
Abd-Elsalam, S., Soliman, S., Esmail, E. S., Khalaf, M., Mostafa, E. F., Medhat, M. A., et al. (2021). Do zinc Supplements Enhance the Clinical Efficacy of Hydroxychloroquine?: a Randomized, Multicenter Trial. Biol. Trace Elem. Res. 199, 3642–3646. doi:10.1007/s12011-020-02512-1
Altamirano, J., Govindarajan, P., and Blomkalns, A. J. (2020). Assessment of Sensitivity and Specificity of Patient-Collected Lower Nasal Specimens for Severe Acute Respiratory Syndrome Coronavirus 2 Testing. JAMA Netw. Open 3, e2012005. doi:10.1001/jamanetworkopen.2020.12005
Bannerman, B. P., Julvez, J., Oarga, J., Blundell, T. L., Moreno, P., Floto, R. A., et al. (2021). Integrated human/SARS-CoV-2 Metabolic Models Present Novel Treatment Strategies against COVID-19. Life Sci. Alliance 4, e202000954. doi:10.26508/lsa.202000954
Bastos, M. L., Perlman-Arrow, S., Menzies, D., and Campbell, J. R. (2021). The Sensitivity and Costs of Testing for SARS-CoV-2 Infection with Saliva versus Nasopharyngeal Swabs. Ann. Int. Med. 174, 501–510. doi:10.7326/m20-6569
Bonaventura, P., Benedetti, G., Albarede, F., and Miossec, P. (2015). Zinc and its Role in Immunity and Inflammation. Autoimmun. Rev. 14, 277–285. doi:10.1016/j.autrev.2014.11.008
Brewer, G. J., and Yuzbasiyan-Gurkan, V. (1992). Wilson Disease. Medicine 71, 139–164. doi:10.1097/00005792-199205000-00004
Butler-LaPorte, G., Lawandi, A., and Schiller, I. (2021). Comparison of Saliva and Nasopharyngeal Swab Nucleic Acid Amplification Testing for Detection of SARS-COV-2: A Systematic Review and Meta-Analysis. JAMA Int. Med. 181, 353–360. doi:10.1001/jamainternmed.2020.8876
Carlucci, P., Ahuja, T., Petrilli, C., Rajagopalan, H., Jones, S., and Rahimian, J. (2020). Zinc Sulfate in Combination with a Zinc Ionophore May Improve Outcomes in Hospitalized COVID-19 Patients. J. Med. Microbiol. 69, 1228–1234. doi:10.1099/jmm.0.001250
Centers for Disease Control and Prevention (2019). Risk for COVID-19 Infection, Hospitalization, and Death By Age Group. Available at: https://www.cdc.gov/coronavirus/2019-ncov/covid-data/investigations-discovery/hospitalization-death-by-age.html (Accessed Sept 5, 2021).
Centers for Disease Control and Prevention (2021). Influenza Antiviral Medications: Summary for Clinicians. Available at: https://www.cdc.gov/flu/professionals/antivirals/summary-clinicians.htm (Accessed August 13, 2021).
Core Team, R. (2021). R: A Language and Environment for Statistical Computing. Vienna, Austria: R Foundation for Statistical Computing. Available at: https://www.R-project.org.
da Silva, R. C. M., Marinho, L. C. N., Silva, D. N. de. A., de Lima, K. C., Pinh, F. Q., and Martins, A. R. L. de. A. (2020). Saliva as a Possible Tool for the SARS-CoV-2 Detection: A Review. Travel Med. Inf. Dis. 38, 101920. doi:10.1016/j.tmaid.2020.101920
Gammoh, N. Z., and Rink, L. (2017). Zinc in Infection and Inflammation. Nutrients 9, 624. doi:10.3390/nu9060624
Hanson, K. E., Barker, A. P., Hillyard, D. R., Gilmore, N., Barrett, J. W., Orlandi, R. R., et al. (2020). Self-collected Anterior Nasal and Saliva Specimens versus Health Care Worker-Collected Nasopharyngeal Swabs for the Molecular Detection of SARS-CoV-2. J. Clin. Micro 158, e10824–20. doi:10.1128/JCM.01824-20
He, Y-Q., Zhou, C-C., Yu, L-Y., Wang, L., Deng, J-L., He, Y-L., et al. (2021). Natural Product Derived Phytochemicals in Managing Acute Lung Injury by Multiple Mechanisms. Pharmacol. Res. 163, 105224. doi:10.1016/j.phrs.2020.105224
Hoang, T. (2020). An Approach of Fatty Acids and Resveratrol in the Prevention of COVID-19 Severity. Phtother Res. 2020, 1–5. doi:10.1002/ptr.6956
Iwanami, S., Ejima, K., Kim, K. S., Noshita, K., Fujita, Y., Miyazaki, T., et al. (2021). Detection of Signficiant Antiviral Drug Effects on COVID-19 with Reasonable Sample Sizes in Randomized Controlled Trials: A Modeling Study. PLoS Med. 18, e1003660. doi:10.1371/journal.pmed.1003660
Jothimani, D., Kailasam, E., Danielraj, S., Nallathambi, B., Ramachandran, H., Sekar, P., et al. (2020). COVID-19: Poor Outcomes in Patients with Zinc Deficiency. Intl J. Inf. Dis. 100, 343–349. doi:10.1016/j.ijid.2020.09.014
Joung, J., Saito, M., Kim, N.-G., Woolley, A. E., Segel, M., Barretto, R. P. J., et al. (2020). Detection of SARS-CoV-2 with SHERLOCK One-Pot Testing. N. Eng. J. Med. 383, 1492–1494. doi:10.1056/nejmc2026172
Kaushik, N., Subramani, C., Anang, S., Muthumohan, R., Shalimar, , Nayak, B., et al. (2017). Zinc Salts Block Hepatitis E Virus Replication by Inhibiting the Activity of Viral RNA-dependent RNA Polymerase. J. Virol. 91, e00754–17. doi:10.1128/JVI.00754-17
Kivela, J. M., Jarva, H., Lappalainen, M., and Kurkela, S. (2021). Saliva-based Testing for Diagnosis of SARS-CoV-2 Infection: A Meta-Analysis. J. Med. Virol. 93, 1256–1258. doi:10.1002/jmv.26613
Krenn, B. M. (2009). Antiviral Activity of the Zinc Ionophores Pyrithione and Hinokitiol against Picornavirus Infections. J. Virol. 83, 58–64. doi:10.1128/jvi.01543-08
Kvamme, J. M., Gronli, O., Jacobsen, B. K., and Florholmen, J. (2015). Risk of Malnutrition and Zinc Deficiency in Community-Living Elderly Men and Women: the Tromsø Study. Pub Health Nutr. 18, 1907–1913. doi:10.1017/s1368980014002420
la Porte, C., Voduc, N., Zhang, G., Sequin, I., Tardiff, D., Singhal, C. N., et al. (2010). Steady-state Pharmacokinetics and Tolerability of Trans-resveratrol 2000 Mg Twice Daily with Food, Quercetin and Alcohol (Ethanol) in Healthy Human Subjects. Clin. Pharmacokin 49, 449–454. doi:10.2165/11531820-000000000-00000
Lee, J. W., Su, Y., Baloni, P., Chen, D., Palovitch-Bedzyk, A. J., Yuan, D., et al. (2021). Integrated Analysis of Plasma and Single Immune Cells Uncovers Metabolic Changes in Individuals with COVID-19. Nat. Biotechnol. 40, 110–120. doi:10.1038/s41587-021-01020-4
Levy, E., Delvin, E., Morcil, V., and Spahis, S. (2020). Can Phytotherapy with Polyphenols Serve as a Powerful Approach for the Prevention and Therapy Tool of Novel Coronavirus Disease 2019 (COVID-19). Am. J. Physiol. Endo Metab. 319, E689–E708. doi:10.1152/ajpendo.00298.2020
Lindner, A. K., Nikolai, O., Kausch, F., Wintel, M., Hommes, F., Gertier, M., et al. (2020). Head-to-head Comparison of SARS-CoV-2 Antigen-Detecting Rapid Test with Self-Collected Anterior Nasal Swab versus Professional-Collected Nasopharyngeal Swab. Eur. Respir. J. 59, 1–8. doi:10.1183/13993003.03961-2020
Malaguernera, L. (2019). Influence of Resveratrol on the Immune Response. Nutrients 11, 946. doi:10.3390/nu
McCulloch, D. J., Kim, A. E., Wilcox, N. C., Logue, J. K., Greninger, A. L., Englund, J. A., et al. (2020). Comparison of Unsupervised Home Self-Collected Midnasal Swabs with Clinician-Collected Nasopharyngeal Swabs for Detection of SARS-CoV-2 Infection. JAMA Netw. Open 3, e2016382. doi:10.1001/jamanetworkopen.2020.16382
Mortaz, E., Bezemer, G., Alipoor, S. D., Varahram, M., Mumby, S., Folkerts, G., et al. (2021). Nutritional Impact and its Potential Consequences on COVID-19 Severity. Front. Nutri 8, 698617. doi:10.3389/fnut.2021.698617
Nagura-Ikeda, M., Imai, K., Tabata, S. E., Miyoshi, K., Murahara, N., Mizuno, T., et al. (2020). Clinical Evaluation of Self-Collected Saliva by Quantitative Reverse Transcription-PCR (RT-qPCR), Direct RT-qPCR, Reverse Transcription-Loop-Mediated Isothermal Amplification, and a Rapid Antigen Test to Diagnose COVID-19. J. Clin. Micro 58, e01438–20. doi:10.1128/jcm.01438-20
Olechnowicz, J., Tinkov, A., Skalny, A., and Suliburska, J. (2018). Zinc Status Is Associated with Inflammation, Oxidative Stress, Lipid, and Glucose Metabolism. J. Physiol. Sci. 68, 19–31. doi:10.1007/s12576-017-0571-7
Ott, I. M., Strine, M. S., Watkins, A. E., Boot, M., Kalinich, C. C., Harden, C. A., et al. (2020). Simply Saliva: Stability of SARS-CoV-2 Detection Negates the Need for Expensive Collection Devices. Emerg. Infect. Dis. 27, 1146–1150. doi:10.1011/2020.08.03.20165233
Pal, A., Squitti, R. S., Picozza, M., Pawar, A., Rongioletti, M., Dutta, A. K., et al. (2021). Zinc and COvid-19: Basis of Current Clinical Trials. Biol. Trace Elem. Res. 199, 2882–2892. doi:10.1007/s12011-020-02437-9
Read, S. A., Obeid, S., Ahlenstiel, C., and Ahlenstiel, G. (2019). The Role of Zinc in Antiviral Immunity. Adv. Nutr(Bethesda, Md.) 10, 696–710. doi:10.1093/advances/nmz013
Sammans, S., and Roberts, D. C. K. (1987). The Effect of Zinc Supplements on Plasma Zinc and Copper Levels and the Reported Symptoms in Healthy Volunteers. Med. J. Austria 148, 247–249. doi:10.5694/j.1326-5377.1987.tb120232.x
Silva, J., Lucas, C., Sundaram, M., Israelow, B., Wong, P., Klein, J., et al. (2021). Saliva Viral Load Is a Dynamic Unifying Correlate of COVID-19 Severity and Mortality. MedRxiv, 1–38. doi:10.1101/2021.01.04.21249236
Suara, R. O., and Crowe, J. E. (2004). Effect of Zinc Salts on Respiratory Syncytial Virus Replication. Antimicrob. Agents Chemother. 48, 783–790. doi:10.1128/AAC.48.3.783-790.2004
Tan, S. Y., Tey, H. L., Lim, E. T., Toh, S. T., Chan, Y. H., Tan, P. T., et al. (2020). The Accuracy of Health Care Worker versus Self Collected (2-in-1) Oropharyngeal and Bilateral Mid-turbinate (OPMT) Swabs and Saliva Samples for SARS-CoV-2. PLOS One 15 (12), e0244417. doi:10.1371/journal.pone.0244417
te Velthuis, A. J., van den Worm, S. H. E., Sims, A. C., Banc, R. S., Snijder, E. J., van, , et al. (2010). Zn(2+) Inhibits Coronavirus and Arterivirus RNA Polymerase Activity In Vitro and Zinc Ionophores Block the Replication of These Viruses in Cell Culture. PLoS Pathog. 6, e1001176. doi:10.1371/journal.ppat.1001176
Teo, A. K., Choudhury, Y., Tan, I. B., Cher, T. C., Chew, S. H., Wan, Z. Y., et al. (2021). Saliva Is More Sensitive Than Nasopharyngeal or Nasal Swabs for Diagnosis of Asymptomatic and Mild COVID-19 Infection. Sci. Rep. 11, 3134. doi:10.1038/s41598-021-82787-z
Thomas, S., Patel, D., Bittel, B., Wolski, K., Wang, Q., Kumar, A., et al. (2021). Effect of High Dose Zinc and Ascorbic Acid Supplementation versus Usual Care on Symptom Length and Reduction in Ambulatory Patients with Sars-CoV-2 Infection. The COVID A to Z Randomzied Clinical Trial. JAMA Open Netw. 4, e21039. doi:10.1001/jamanetworkopen.2021.0369
Torres, M., Collins, K., Corbit, M., Ramirez, M., Winters, C. R., Katz, L., et al. (2021). Comparison of Saliva and Nasopharyngeal Swab SARS-CoV-2 RT-qPCR in a Community Setting. J. Infect. 82, 84–123. doi:10.1016/j.jinf.2020.11.015
Uyeki, T. M., Bernstein, T., Bradley, H. H., Englund, J. S., File, J. A., Fry, A. M., et al. (2019). Clinical Practice Guidelines by the Infectious Diseases Society of America: 2018 Update on Diagnosis, Treatment, Chemoprophylaxis, and Institutional Outbreak Management of Seasonal Influenza A. Clin. Infect. Dis. 2019, e1–e47. doi:10.1093/cid/ciy866
Weinreich, D. M., Sivapalasingame, S. S., Norton, T., Ali, S., Gao, H., Bhore, R., et al. (2021b). REGEN-COV Antibody Combintion and Outcomes I Outpatients with COVID-19. N. Eng. J. Med. 385 (e81), 1–12. doi:10.1056/NEJMoa2108163
Weinreich, D. M., Sivapalasingame, S. S., Norton, T., Ali, S., Gao, H., Bhore, R., et al. (2021a). REGN-COV2, a Neutralizing Antibody Cocktail, in Outpatients with Covid-19. N. Engl. J. Med. 384, 238–251. doi:10.1056/nejmoa2035002
Wessels, I., Rolles, B., and Rink, L. (2020). The Potential Impact of Zinc Supplementation on COVID-19 Pathogenesis. Front. Immunol. 11, 1–11. doi:10.3389/fimmu.2020.01712
Wölfel, R., Corman, V. M., Guggemos, W., Seilmaier, M., Zange, S., Muller, M. A., et al. (2020). Virological Assessment of Hospitalized Patients with COVID-19. Nature 581 (7809), 465–469. doi:10.1038/s41586-020-2196-x
Wyllie, A. L., Fournier, J., Casanovas-Massana, A., Campbell, M., Tokuyama, M., Vijayakumar, P., et al. (2020). Saliva or Nasopharyngeal Swab Specimens for Detection of SARS-CoV-2. N. Eng. J. Med. 383, 13–16. doi:10.1056/NEJMc2016359
Xue, J., Moyar, A., Peng, B., Wu, J., Hannafon, B. N., Ding, W- Q., et al. (2014). Chloroquine Is a Zinc Ionophore. PloS One 9, e109180. doi:10.1371/journal.pone.0109180
Keywords: COVID-19, zinc, resveratrol, saliva, SARS-CoV-2
Citation: Kaplan HG, Wang K, Reeves KM, Scanlan JM, Nunn CC, Kieper DA, Mark JL, Lee I, Liu R, Jin R, Bolton MJ and Goldman JD (2022) Reszinate—A Phase 1/2 Randomized Clinical Trial of Zinc and Resveratrol Utilizing Home Patient-Obtained Nasal and Saliva Viral Sampling. Front. Drug. Discov. 2:910124. doi: 10.3389/fddsv.2022.910124
Received: 31 March 2022; Accepted: 07 June 2022;
Published: 15 July 2022.
Edited by:
Weiwei Xue, Chongqing University, ChinaReviewed by:
Haibo Wu, Chongqing University, ChinaYuwei Wang, Shaanxi University of Chinese Medicine, China
Copyright © 2022 Kaplan, Wang, Reeves, Scanlan, Nunn, Kieper, Mark, Lee, Liu, Jin, Bolton and Goldman. This is an open-access article distributed under the terms of the Creative Commons Attribution License (CC BY). The use, distribution or reproduction in other forums is permitted, provided the original author(s) and the copyright owner(s) are credited and that the original publication in this journal is cited, in accordance with accepted academic practice. No use, distribution or reproduction is permitted which does not comply with these terms.
*Correspondence: Henry G. Kaplan, aGFuay5rYXBsYW5Ac3dlZGlzaC5vcmc=