- 1Department of Medicine, Li Ka Shing Faculty of Medicine, The University of Hong Kong, Queen Mary Hospital, Hong Kong Special Administrative Region, Pokfulam, China
- 2Department of Ophthalmology, Li Ka Shing Faculty of Medicine, The University of Hong Kong, Hong Kong Special Administrative Region, Pokfulam, China
Since the COVID-19 outbreak in China in 2019, the pandemic has spread globally. There is no definitive cure, but vaccines have greatly protected humans from symptomatic infections and severe complications. However, vaccine efficacy has been greatly reduced by the advent of SARS-CoV-2 variants worldwide. The World Health Organization has classified the variants into two groups: variants of concern (Alpha, Beta, Gamma, Delta, Omicron) and variants of interest (Lambda, Mu). Clinical trials and modifications of vaccines are currently undertaken to improve their clinical efficacies. This is particularly worrying in immunocompromised patients since breakthrough infections with multiple lineages of variants can pose a continuous threat of severe diseases in these vulnerable subjects, though there is no evidence showing immunocompromised patients are at a higher risk of vaccine-associated adverse events. However, there is no consensus on the schedule, benefits, and risks as well as contraindications (both absolute and relative) of receiving booster vaccinations. This review looks into the efficacy and safety of COVID-19 vaccination booster to guide clinical decisions on when and who to receive booster vaccination.
Introduction
Since the outbreak of COVID-19 infection in late 2019 in China, it has spread globally causing massive morbidity and mortality. It spreads through contact, droplets, and aerosol transmission (PriyankaChoudhary et al., 2020). The morbidity and mortality rates in developed countries slowed down because COVID-19 vaccines provided immune protection against SARS-CoV-2 (Arbel et al., 2021; Yan et al., 2021a). In spite of the high efficacy and safety profile of vaccines as reported in various studies, some patient groups are still undecided whether to receive vaccinations (Abu-Farha et al., 2021; Yigit et al., 2021a; Choudhary et al., 2021; Zhou et al., 2021). Previous studies and guidelines have documented the special considerations for different clinical special populations, and medical staff should assist in the risk–benefit analysis to make a proper clinical decision (Yan et al., 2021b; Furer et al., 2021; Powers et al., 2021; Soiza et al., 2021).
Worldwide, a significant portion of people in developed countries has completed COVID-19 vaccination schedule, while some in developing and under-developed countries have not completed basic vaccination schedules due to resources scarcity and income disparity (The Lancet Infectious Diseases, 2021; Van De Pas et al., 2022). Vaccination does not confer sufficient lifelong protection: post-vaccination humoral responses decrease after 3–6 months (Chemaitelly et al., 2021; Levin et al., 2021; Erice et al., 2022). Early studies on booster vaccination show that the infection rate in the booster cohort is lower compared with those without receiving a booster (Bar-On et al., 2021). Of even greater concern, vaccine efficacy dwindles when it comes to Delta- and Omicron-related variant infections, which is associated with higher viral load and transmissibility (Campbell et al., 2021; Torjesen, 2021). Patients without sufficient protective efficacy run a higher risk of symptomatic infection, severe hospitalization, mortality, and long COVID-19 syndrome (Yan et al., 2021c).
This prompts a key question: who should receive a booster 6 months after the last dose since vaccination per se may be associated with risks? This paper evaluates the risk and benefits of booster vaccination to assist clinical decision making. Literature data were retrieved from electronic databases (PubMed, Medline, Scopus, Cochrane, Google Scholar) on December 14, 2021, with the following keywords: COVID-19, Vaccine, Booster, and Variants. Related articles are reviewed to address the key question.
COVID-19 Variants
Since the outbreak in 2019, various variants have been identified worldwide. The transmissibility and mortality of SARS-CoV-2 have been changing with the new mutations. Various reports have shown the reduced efficacy of vaccines to neutralize SARS-CoV-2 variants. WHO has proposed the classification of SARS-CoV-2 variants into two major types: variants of concern (VOC) and variants of interest (VOI) (WHO, 2021a). Their characteristics are listed in Table 1 (WHO, 2021a). In view of the emerging variants globally, vaccine efficacy against different variant strains are recorded in Table 2.
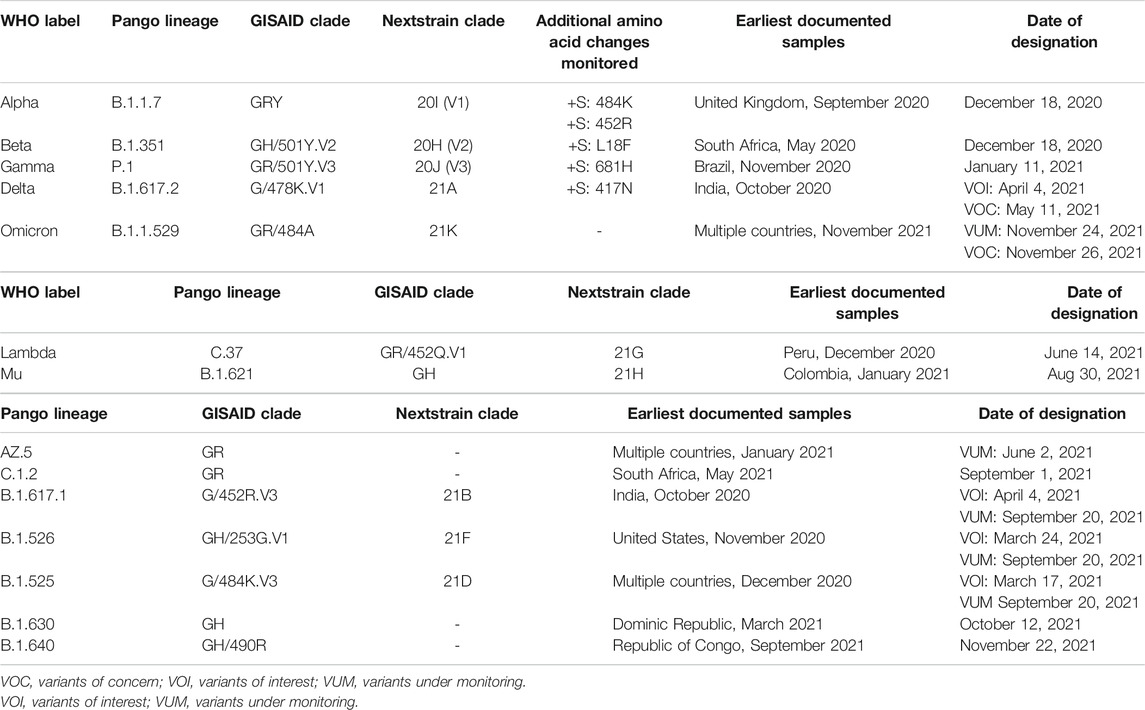
TABLE 1. Summary of characteristics of SARS-CoV-2 variants by the WHO Classification (WHO, 2021a). a) Variants of concern.
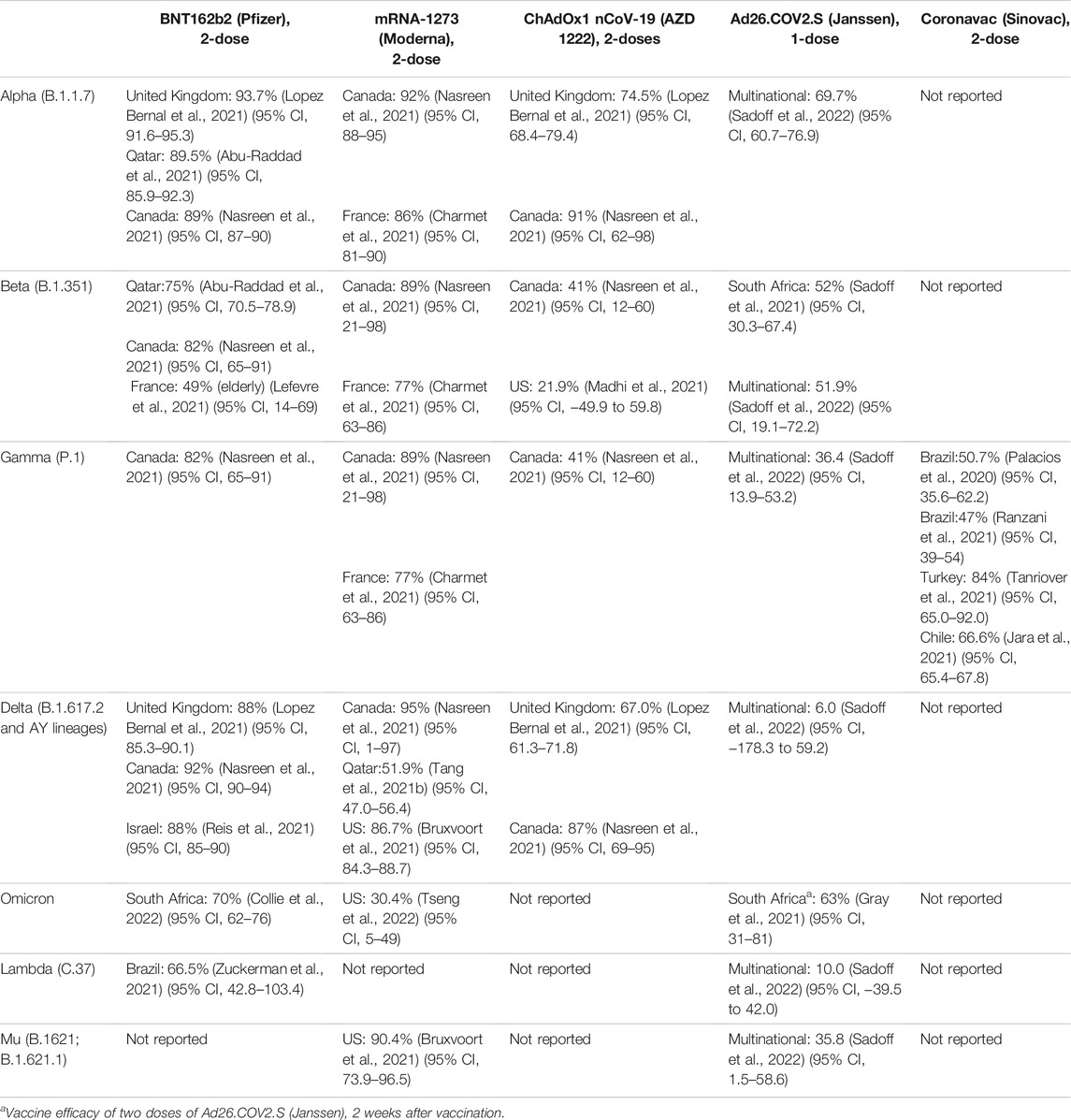
TABLE 2. COVID-19 vaccine efficacy against symptomatic infection 14 days after complete vaccination schedule (without booster) against variant strains 2 weeks after administration, stratified by region.
Benefits of Booster Vaccination
Waning humoral responses have been reported worldwide 6 months after completion of vaccination (one to three doses, depending on vaccination design) (Levin et al., 2021; Chemaitelly et al., 2021; Shrotri et al., 2021). This is particularly evident in men, participants older than 65 years old, or people with immunosuppression (Levin et al., 2021) for BNT162b2 (Pfizer-BioNTech) recipients. In spite of the waning antibody titer after 6 months of completion of BNT162b2 (Pfizer-BioNTech) vaccination, the protection against hospitalization and death persists at a robust level. The estimated effectiveness of BNT162b2 (Pfizer-BioNTech) against SARs-CoV-2 infection peaked at 77.5% (95% CI, 76.4–78.6) in the first month after the second dose, but it progressively dropped to 20% 5 months after the second dose (Chemaitelly et al., 2021). Thus, reinfection by SARS-CoV-2 is possible (Cromer et al., 2021). Vaccine protection against major variants of SARS-CoV-2 is summarized in Tables 2–8. One of the major concerns is that all vaccines express the ancestral SARS-CoV-2 spike protein, whereas currently circulating variants such as Delta variant possess several mutations to evade the response, resulting in a 4-fold lower neutralizing antibody response to Delta-variant infections (Kent and Juno, 2021).
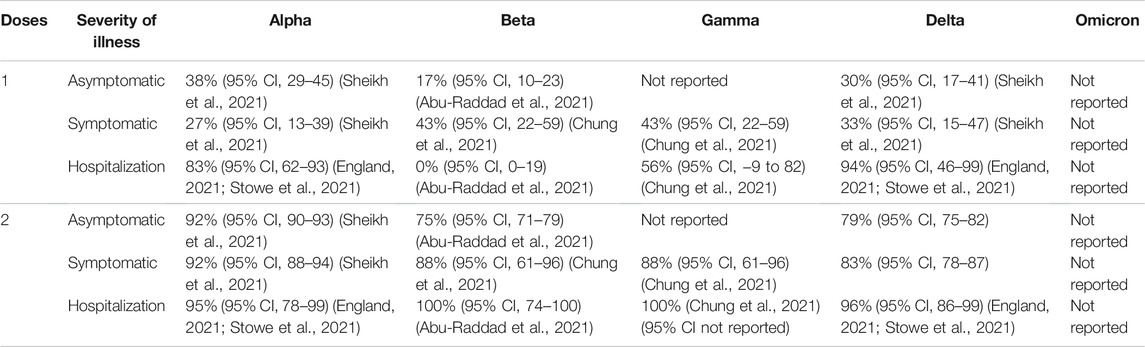
TABLE 3. BNT162-b2 (Pfizer-BioNTech) vaccine efficacy (without booster) against different clinical severity in variant strain infection 2 weeks after administration.
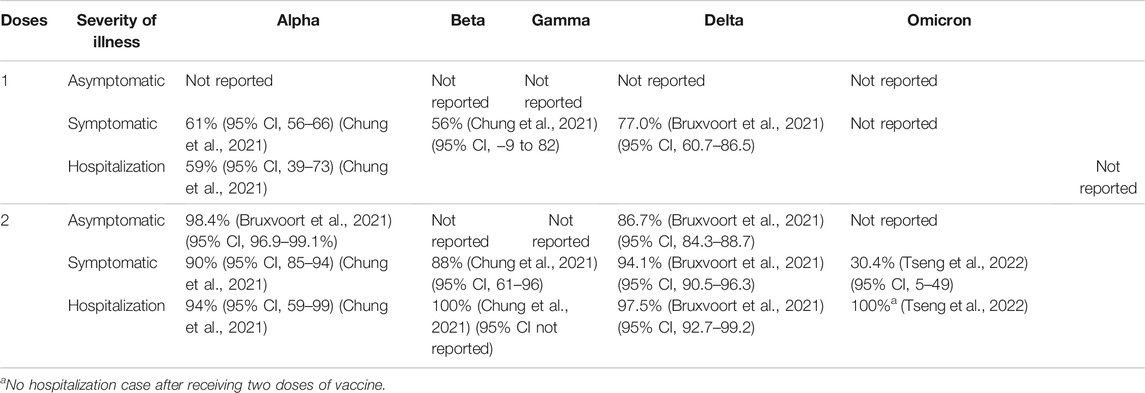
TABLE 4. mRNA-1273 (Moderna) vaccine efficacy (without booster) against different clinical severity in variant strain infection 2 weeks after administration.
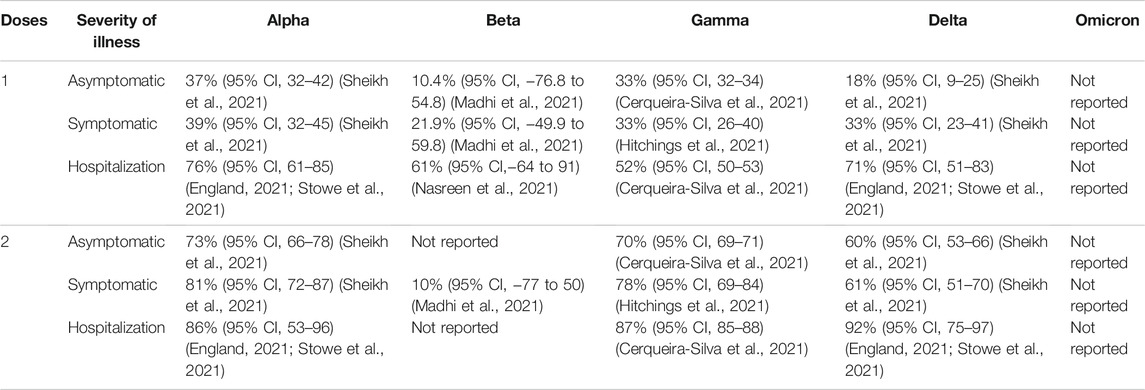
TABLE 5. ChAdOx1 nCoV-19 (AZD 1222) vaccine efficacy (without booster) against different clinical severity in variant strain infection 2 weeks after administration.
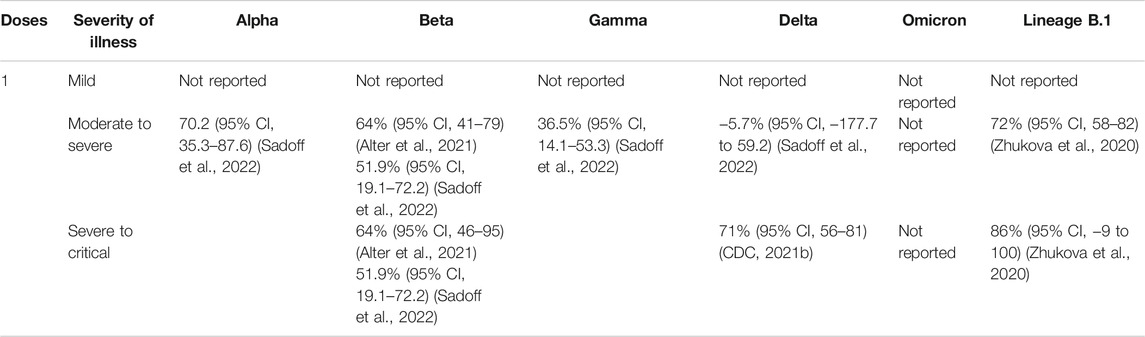
TABLE 6. Ad26.COV2.S (Janssen) vaccine efficacy (without booster) against different clinical severity in variant strain infection 2 weeks after administration.

TABLE 7. Sputnik V COVID-19 Vaccine (Gam-COVID-Vac) vaccine efficacy (without booster) against different clinical severity in variant strain infection 2 weeks after administration.
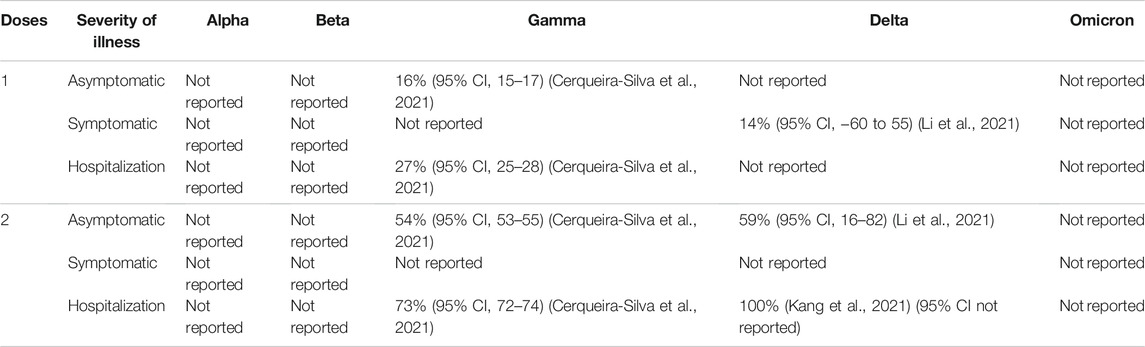
TABLE 8. Coronavac (Sinovac) COVID-19 vaccine efficacy (without booster) against different clinical severity in variant strain infection 2 weeks after administration.
Vaccination Efficacy Against Variants of Concern
VOC include Alpha (B.1.1.7), Beta (B.1.351), Gamma (P.1), Delta (B.1.617.2), and Omicron (B.1.1.529) variants. They have been associated with higher transmissibility and reduced vaccination efficacy. Despite being infected with B.1.1.7 (Alpha) and B.1.351 (Beta) variants after mRNA-based vaccination, the protection against severe, critical, or fatal COVID-19 cases remains 96% in Qatar (Chemaitelly et al., 2021). The vaccine efficacy against symptomatic infection quickly drops from over 90% before the spread of Delta variant to 42–80% after the spread of the variant (Tang et al., 2021a; Pouwels et al., 2021; Sheikh et al., 2021). This efficacy also dwindles as time elapses (Barouch et al., 2021; Ciabattini et al., 2021; Naaber et al., 2021; Pegu et al., 2021).
However, recent studies have shown that the mRNA-based booster vaccine still induces a robust immune response to variants, though weaker when compared with its prototype. The mRNA-based booster vaccination is safe and well tolerated (Ebinger et al., 2021). It boosts antibody production to neutralize variant strains especially wild-type D614G (p < 0.0001). The neutralization titers against B.1.351 (Beta), P.1 (Gamma), and B.1.617.2 (Delta) are either low or undetectable 1 month after vaccination (Ebinger et al., 2021). The vaccine-mediated protection against variants in the respiratory tract is durable but delayed (Gagne et al., 2021). It is dependent on anamnestic antibody responses which can be maximized by a booster dose (Gagne et al., 2021).
Upon receiving BNT162b2 (Pfizer-BioNTech) booster vaccination, the infection and severe illness rates are lower, compared with those without booster vaccination in Israel (Bar-On et al., 2021). A third-dose booster vaccine in Israel showed a significant reduction of confirmed infection and severe illness by a factor of 11.3 and 19.9, respectively (Bar-On et al., 2021). This finding is consistent with another serological study which showed that the occurrence of breakthrough infection with SARS-CoV-2 correlated with neutralizing antibody titers during the peri-infection period (Bergwerk et al., 2021). Therefore, an elevated titer is protective against breakthrough infections. This is particularly important in ambulatory and inpatient settings in less developed countries where the resources are scarce and the hospital wards are usually crowded to favor transmission. A similar booster recommendation is recommended in recipients of CoronaVac since over one-fifth of recipients become seronegative 2 months after the second dose of vaccination (Yigit et al., 2021b). Vaccination protects patients in ambulatory and inpatient care settings (Thompson et al., 2021). A full-dose mRNA-based vaccination (even without booster) is associated with 90% (95% CI, 86–93) effectiveness against intensive care unit (ICU) admission and 91% (95% CI, 89–93) effectiveness against emergency care visits. The effectiveness of Ad26.COV2.S is 68% (95% CI, 50–79) against hospitalization and 73% (95% CI, 59–82) against emergency care visits.
Various studies show a close association between infection rate and serological titer of circulating antibody levels after vaccination (Bergwerk et al., 2021; Khoury et al., 2021). The vaccination regimen of ChAdOx1 nCoV-19 also recommends a booster regime since it induces multifunctional antibody responses, including antibody-dependent neutrophil/monocyte phagocytosis, complement activation, and natural killer activation (Barrett et al., 2021).
Some special populations are at high risks of breakthrough infections. The incidence risk ratio in the immunocompromised patient is 1.66 (95% CI, 1.17–2.35) even after full-dose mRNA-based vaccination. An increased incidence is observed over time, showing the decreasing protective efficacy as time elapsed. However, among those with COVID-19 infection, vaccination significantly reduces the risk of death (hazard ratio 0.20, 95% CI, 0.08–0.49) (Liu et al., 2021a).
Vaccination Efficacy Against Variant of Interest
VOI include Lambda (C.37) and Mu (B.1.621) variants. A study using a micro-neutralization assay following mRNA vaccine demonstrated a 1.6-fold reduction of neutralizing titers compared with the wild-type virus, increasing the likelihood of infection and disease transmission after vaccination (Zuckerman et al., 2021). The Lambda variant harbors two key mutations in the receptor-binding domain (RBD), L452Q and F490S, changing the antigenicity and infectivity. Cell line studies show that the convalescent and vaccine-based sera recorded 1.3- to 2.5-fold lower neutralizing antibody titers (Wang et al., 2021). The reason of partial escape from neutralizing antibodies in vaccinated individuals behind is closely related to the increased affinity between RBD and angiotensin-converting enzyme 2 (ACE2) binding, leading to increased processing of spike protein to yield a higher fusion activity and syncytium formation in these variants (Moghaddar et al., 2021). The antibody titer drop after 6 months against Lambda variant is 3-fold, leading to breakthrough infections (Liu et al., 2021b). The rapidly dropping antibody titers against Lambda and Mu infections can be countered by a third-dose booster to cope with the surge of variants transmission.
The Mu variant demonstrates a remarkable resistance to antibodies by convalescent plasma and vaccine-induced protection (Uriu et al., 2021). Pseudovirus model serological assay was performed on Mu variant infection. It was 9.1 times as resistant as the parenteral virus in response to mRNA-based vaccination (BioNTech), 2.0 times as resistant to neutralization by convalescent serum, and 1.5 times as resistant to neutralization by vaccine serum as the Beta variant. Similar resistance has been reported in inactivated vaccine-elicited antibodies (Xie et al., 2021).
Risks of Booster Vaccination
A booster vaccination is not without risks. Myocarditis has been reported worldwide, particularly in young male recipients after receiving the second dosage of BNT162b2 (Pfizer-Biotech) vaccine (Mevorach et al., 2021). The risk ratio 30 days after the second dose in fully vaccinated recipients (without booster) is 2.35 (95% CI, 1.10–5.02), while the risk ratio rises to 8.96 (95% CI, 4.50–17.83) in male subjects between 16 and 19 years old. It is uncertain whether the third dose will trigger further myocardial damage. However, severe complications such as myocarditis or allergy from the first two doses of BNT162b2 are relatively rare (Barda et al., 2021). Guillain–Barre syndrome has also been reported in adenovirus-vectored COVID-19 vaccines (WHO, 2021b). For the majority of fully vaccinated recipients who have no significant associated complications, a booster vaccination may be safer.
The booster may greatly safeguard the effect offered by the first two dosages. When serum antibody titer drops below the protective threshold, a “start over” vaccination may still be necessary, which may also trigger complications. Studies also reveal that antibody levels in the aged population are relatively lower after full vaccination, though no severe complications are observed (Wei et al., 2021). Therefore, a booster vaccination may be necessary for this population.
Overall, vaccination is very safe, though common transient side effects happen, and a booster is a good option that significantly consolidates the protective effect to fight against this long-term pandemic. It is recommended that the earliest time to receive a booster is 6 months after the initial vaccination (Chemaitelly et al., 2021; Levin et al., 2021). A future study evaluating long-term serum antibody titer and booster clinical trials are needed for guidance on booster vaccination.
Whether to Advocate Booster Vaccination
Though booster vaccination is associated with both benefits and risks, overall it is safe and effective. The BNT162b2 third-dose booster vaccination in Israel showed that the side effects were mild and self-limiting, including immunocompromised patients and senior citizens (Shapiro Ben David et al., 2021). The most common side effects were fatigue (19.6%), myalgia (9.2%), and fever (8.1%) in immunocompromised patients and fatigue (21.3%), myalgia (9.9%), and fever (9.2%) in senior citizens. Over two-thirds of the recipients developed a better or similar response compared to the second dose. This is consistent with the study in the United Kingdom which showed that although there were numerous local or systemic side effects in the short term, the vaccine efficacy exceeded 60% within 2–3 weeks (Menni et al., 2021).
One concern of advocating booster vaccination is related to equity (The Lancet Infectious Diseases, 2021). The income disparity between developed countries and developing countries results in a competition for gaining access to vaccinations. Up to August 9, 2021, over 80% of the vaccines were distributed to high-income countries, while only 20% were in low-income countries with only 3% of the African population fully vaccinated (without a booster) (Kherabi et al., 2021; The Lancet Infectious Diseases, 2021). This results in increasing reporting of variants in different countries, in particular from low-income countries (see Supplementary Appendix with reports of different variants reported internationally). Vaccines thus should be made available to other countries before offering domestic booster vaccinations to reduce variants transmission and new variants from evolving (Dyer, 2021; Schaefer et al., 2021).
The vaccination schedule should be individualized for different populations, such as healthy individuals without previous SARS-CoV-2 infection and COVID-19 survivors with prior SARS-CoV-2 infection. The underlying reason is related to the waning trajectory of antibody titers in different populations. After a two-dose schedule of mRNA-based vaccination, healthy subjects developed similar antibody levels to COVID-19 survivors who recover from the infection for 1 year and receive single-dose mRNA-based vaccination (Gluck et al., 2021). None of the study participants experienced reinfection. The half-life of anti-SARS-CoV-2 IgG antibody ranges between 85 and 158 days (Lumley et al., 2021; Dan et al., 2021; den Hartog et al., 2021), while some studies also show the immunity may last over 300 days (Gluck et al., 2021; Lee et al., 2021). There is uncertainty about the booster schedule for different populations, and this may create a burden on healthcare service and spark controversy on vaccine equity between developing countries and developed countries (The Lancet Infectious Diseases, 2021). Overall, vaccine boosters quickly increase antibody levels since the large number of memory B cells and plasma cells derived from previous immunity drives rapid antibody productions after booster vaccination (Ebinger et al., 2021; Gobbi et al., 2021; Liao et al., 2021; Turner et al., 2021). Booster vaccination thus is protective against SARS-CoV-2 infection, but the booster schedule requires further studies.
Though vaccine recipients may have more persistent nasopharynx-homing SARS-CoV-2-specific T cells compared to infection-naïve subjects (Neidleman et al., 2021), a further booster vaccination still induces a stronger immune response while giving manageable side effects (Ebinger et al., 2021).
Current Recommendation on Booster Vaccination
The US Center for disease Control and Prevention and French National Authority of Health have recommended prioritization of booster vaccination for high-risk groups, subjects aged over 65 years, subjects between 50 and 64 years with underlying medical conditions, healthcare workers, and residents of long-term care facilities (Burki, 2021; Kherabi et al., 2021). The United Kingdom is currently considering a third-shot booster vaccination for all adults (Burki, 2021).
Healthcare workers are at high risk of infection. The British study of BNT162b2 vaccine showed vaccine effectiveness of 70% (95% CI 55–85) 21 days after the first dose and 85% (95% CI 74–96) 7 days after two doses in healthcare staff when the dominant variant was Alpha (B.1.1.7) (Hall et al., 2021).
The timing to receive booster vaccination is subject to debate. A study focusing on the immunogenicity and efficacy of ChAdOx1 nCoV-19 (AZD1222) recommends a 3-month interval to receive a booster since a high protective efficacy is maintained until 3 months (Voysey et al., 2021). Efficacy was higher with a 12-week prime–boost interval (vaccine efficacy 81.3%, 95% CI 60.3–91.2), compared with a 6-week interval (vaccine efficacy 55.1%, 95% CI 33.0–69.9). Meanwhile, BNT162b2 (Pfizer) vaccine recommends a 6-month interval to receive a booster (CDC, 2021a).
Whether subjects with previous SARS-CoV-2 should receive a three-dose vaccination schedule and when they should receive the booster vaccination as antibody titers drop are still under debate. The antibody responses to the first vaccine dose in individuals with pre-existing immunity are equal to or exceed the titers found in naïve individuals after the second dose of BNT162b2 (Pfizer-BioNTech). The antibody titers against VOC, Delta variant (1.617.2), in COVID-19 recoverers (at least 11 months after complete resolution of the previous infection) receiving one-dose ChAdOx1 nCoV-19 vaccine are similar to or higher than the counterparts receiving two-dose BNT162b2 (Pfizer-BioNTech) (Havervall et al., 2021). BBV152 (Bharat Biotech) vaccine of India also induces a similar response in COVID-19 recoverers, suggesting one-dose vaccinations can give similar antibody levels as two-dose vaccinations (Kumar et al., 2021). Their adverse reaction to vaccination is higher than those of healthy adults (Krammer et al., 2021). COVID-19 recoverers receiving a second-dose vaccination show no additional benefits since they have reached the peak of their immunity after the first dose (Lozano-Ojalvo et al., 2021). These studies suggest that one-dose vaccinations in COVID-19 recoverers is sufficient to confer protection non-inferior to healthy individuals with two-dose vaccinations (Cavalcanti et al., 2021; Vicenti et al., 2021), and they should be put lower on the vaccination priority list (Saadat et al., 2021). Despite the similar efficacy, the post-vaccine symptoms are more prominent for those with prior infection after the first dose (Ebinger et al., 2021).
Contraindications for Vaccination
The absolute and relative contraindications of vaccination are documented in Tables 9 and 10. Absolute contraindications include mainly severe allergic reactions to its constituents shown in Table 9 (USCDC, 2021a). Relative contraindications and recommended actions are listed in Table 10. Subjects with relative contraindications are recommended to discuss individual risk profiles to plan their vaccination decision. Counseling should include risk factors, relative contraindications, benefits and risks of vaccinations, alternative vaccines, and risks of without vaccinations. Table 11 documents the ingredients of 24 COVID-19 vaccines with emergency use authorizations by national regulatory authorities (as of October 26, 2021).
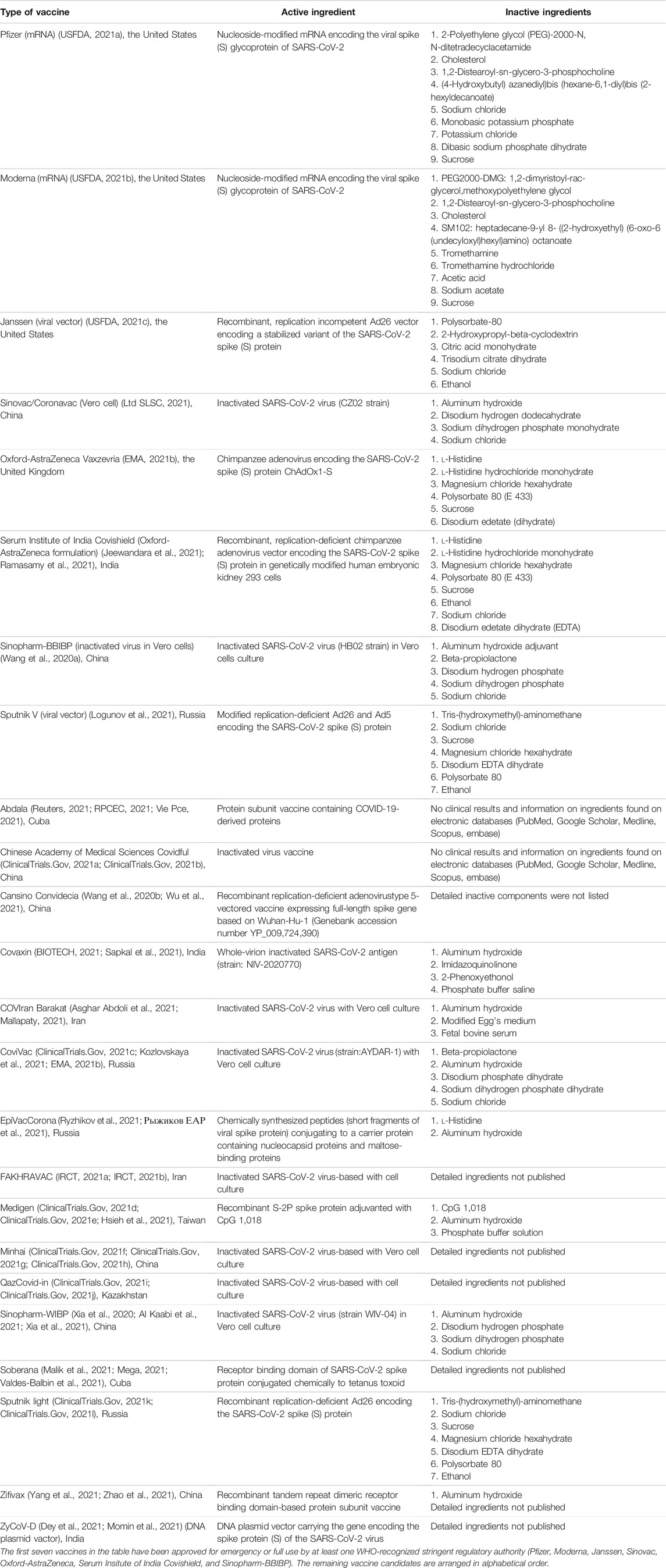
TABLE 11. Components of 24 COVID-19 vaccines with emergency use authorizations by national regulatory authorities (as at October 26, 2021).
Recommendations for Booster Vaccination
Heterologous vaccination (Com-COV study) is safe and induces robust immunity without serious adverse events (Borobia et al., 2021; Liu et al., 2021c; Moghnieh et al., 2021). This has been performed in subjects receiving “BNT162b2 (Pfizer/BioNTech) plus ChAdOx1 nCoV-19 (AZD1222)” and “BNT162b2 (Pfizer/BioNTech) plus BBIBP-Cor-V (Sinopharm).” The interim analysis documents that heterologous ChAdOx/BNT immunization regimen with 10–12 weeks vaccination interval is well tolerated and slightly more immunogenic compared to homologous BNT/BNT vaccination with 3-week intervals. A recent randomized controlled trial showed that heterologous vaccination with other vaccines after the initial two doses (either two-dose ChAdOx-1 or BioNTech) yields a higher SARS-CoV-2 anti-spike IgG titer and stronger cellular response (Munro et al., 2021). This may be a viable choice for countries without a stable source of vaccines or in immunocompromised patients who could not produce sufficient protective antibodies (Borobia et al., 2021; Liu et al., 2021c; Hillus et al., 2021; Moghnieh et al., 2021). Initial SARS-CoV-2 vaccination response can predict booster response; thus, reassessment of antibody response may be a viable choice whether to receive homologous or heterologous vaccination (Perkmann et al., 2021).
Timing of vaccinations is vital in certain patients after specific treatments. Suboptimal immunological response has been found in patients receiving BNT162b2 (Pfizer-BioNTech) after rituximab administration (Kant and Geetha, 2021). Delaying vaccination for 6 months after rituximab administration or B-cell reconstitution has been suggested in previous studies (Kant et al., 2021). Vaccine booster schedule should be individualized according to the half-life of immunity decline. A British study shows that an extended interval before the second dose of ChAdOx1 nCoV-19 leads to increased antibody titers, while a third dose of ChAdOx1 nCoV-19 induces antibody that correlates with higher efficacy after second dose due to robust T-cell responses (Flaxman et al., 2021).
Patients receiving immunosuppressants or with chronic kidney impairment receiving renal replacement therapy also have suboptimal anti-SARS-CoV-2 antibodies after the second dose (Boyarsky et al., 2021a; Boyarsky et al., 2021b; Bensouna et al., 2021; Kamar et al., 2021; Peled et al., 2021; Werbel et al., 2021). A booster dose of mRNA-1273 vaccine induces serological response in 49% of renal recipients who are refractory to produce antibodies after two doses (Benotmane et al., 2021). This is similar in patients receiving solid organ transplants on immunosuppressants and with negative antibody titers before the third dose: 25% of them develop high-positive antibodies after a third dose, while over two-thirds of them remain negative (Werbel et al., 2021). A significant proportion of patients who fail to develop immunity after a third-dose booster is on triple immunosuppressants (Benotmane et al., 2021). The SENCOVAC study shows that an absence of antibody protection is associated with kidney transplant recipients due to their immunosuppression therapy (odds ratio 20.56, p < 0.01), while receiving BNT162b2 increases the chance of antibody response (odds ratio 6.03, p = 0.02) (Quiroga et al., 2021). These patients are advised to adopt persistent isolation measures and consider booster vaccines to optimize protection against COVID-19 infection (Quiroga et al., 2021). Use of rituximab is common in cancer or autoimmune disease treatments leading to failure of immunological response (Yahav et al., 2021). Heterologous vaccination leads to stronger induction of antibodies and CD4 T cells in immunocompromised patients: SARS-CoV-2-specific antibodies and T-cells response after second vaccination were induced 100% and 70.6% in transplant recipients (Baker et al., 2021; Schmidt et al., 2021).
A recent systematic review compared the relative likelihood of non-responders (Galmiche et al., 2021). The proportion of non-responders is higher among solid organ transplant recipients (range 18–100%), hematological malignancy (range 14–61%), cancers (2–36%), and dialysis usage (2–30%). Risk factors of failure of antibody induction include older age, use of corticosteroids, immunosuppressants, and anti-CD20 agents.
Currently, a new approach is consideration of immunosuppressant dosage adjustment or additional booster to maximize immunological response induction (Albach et al., 2021; Yan et al., 2021b; Connolly et al., 2021; Mackintosh et al., 2021; Yahav et al., 2021). With vaccination-refractory in immunocompromised subjects (solid organ transplant recipients) (Chavarot et al., 2021), recommendation of an additional dose is encouraged since a third vaccine dose increases the seropositivity prevalence from 40% to 68% (Husain and Argyropoulos, 2021). The seropositivity is protective against symptomatic infection, while it is unlikely to carry a significant risk of adverse events (Husain and Argyropoulos, 2021).
Cancer patients benefit from third-dose vaccinations since they run a high risk of failed induction of immune memory (Peeters et al., 2021). The CANVAX Cohort Study shows that immune responses to SARS-CoV-2 vaccines are moderately impaired in patients with cancer, while antibody testing may be effective to identify immune-inert patients to receive booster vaccinations (Naranbhai et al., 2021). A third-dose BNT162b2 (Pfizer/BioNTech) vaccination demonstrated a median 3-fold increase of neutralizing antibody response with mild adverse events (Shroff et al., 2021). This should be similarly considered in cancer patients with active anti-neoplastic treatment (Peeters et al., 2021). In view of the breakthrough infections with multiple lineages of variants, immunocompromised patients are at risk of severe diseases (Deng et al., 2021; D'Amelio et al., 2021). In terms of safety, immunocompromised patients are not at an increased risk of vaccine-related adverse events (Mackintosh et al., 2021).
Recommendation for Breakthrough Infection
Breakthrough infections have been reported worldwide. Breakthrough infections are mild in healthy adults (Abbasi, 2021). Thus, management of breakthrough infections should include a thorough clinical history review to determine the presence of risk factors and appropriate actions (Figure 1).
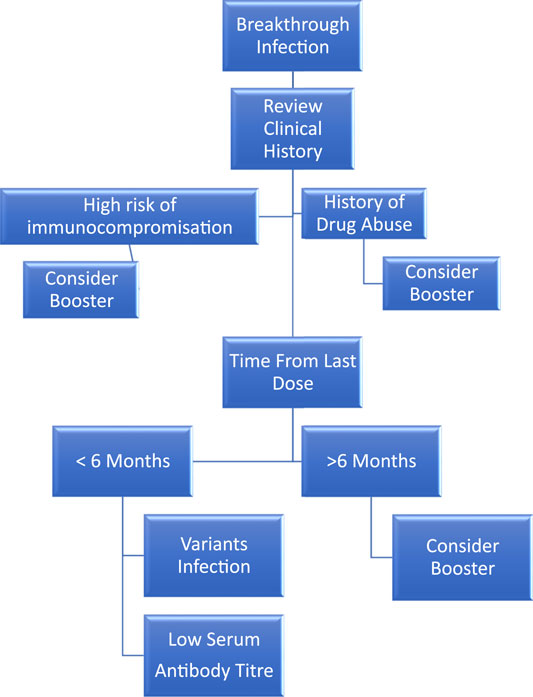
FIGURE 1. Management algorithm for COVID-19 breakthrough infection. Common reasons include the following: (A) Abuse of drugs. (B) Baseline multiple comorbidities. (C) Changing strains. (D) Decreasing antibody level. (E) Elderly.
Common reasons related to this include: 1) infection of variant strains (Bosch et al., 2021); 2) low circulating antibody levels as time elapsed from the previous second dose (Chemaitelly et al., 2021; Levin et al., 2021); 3) insufficient induction of antibody due to multiple comorbidities or immunocompromised state (Albach et al., 2021; Arya et al., 2021); 4) history of substance abuse (Wang et al., 2022); and 5) old age (Butt et al., 2021; Glatman-Freedman et al., 2021).
Within 6 months after the last dose of mRNA-based vaccination, the antibody is still protective against COVID-19 disease (El Sahly et al., 2021; Thomas et al., 2021). Consideration of vaccine-resistant variant strain infection should be the top priority. Low serum antibody level leading to insufficient protective efficacy is the common reason for breakthrough infections. Booster vaccination after 6 months, regardless of antibody level, should be considered (Ebinger et al., 2021; Gobbi et al., 2021; Liao et al., 2021; Turner et al., 2021), though there is evidence suggesting breakthrough infections do not necessarily correlate with lack of vaccine-induced immunity (Duarte et al., 2021).
Patients with multiple comorbidities leading to the immunocompromised state should consider booster vaccination, with either homologous or heterologous vaccines (Baker et al., 2021; Schmidt et al., 2021). The multiple comorbidities include solid organ transplant patients on immunosuppressants (Werbel et al., 2021), autoimmune diseases with failed induction of antibody despite full-dose vaccination schedule (Yahav et al., 2021), chronic kidney disease with renal replacement therapy (Boyarsky et al., 2021a; Boyarsky et al., 2021b; Bensouna et al., 2021; Kamar et al., 2021; Peled et al., 2021; Werbel et al., 2021), poorly controlled hypertension, diabetes mellitus (Brosh-Nissimov et al., 2021), and cancers (Peeters et al., 2021).
Conclusion
This paper provides an updated evaluation of booster vaccination: its necessity, concerns, and benefits on variant strains. It gives a detailed description of the efficacy and safety of vaccination on variant strains. However, there are limited data on the effect of booster vaccination on Omicron strains at the time of writing, and Omicron-strain vaccination has been under development by vaccine manufacturers; thus, clinical trials of newly modified vaccines have not started. Booster vaccination brings benefits to the waning immunity and protective efficacy of COVID-19 vaccines. Booster vaccination induces immunological memory by elevating circulating anti-SARS-CoV-2 antibody level. Its associated risk is manageable, while risk–benefit analysis should be evaluated by medical health staff to manage comorbidities and rule out contraindications for vaccinations. Special populations should have an alternative vaccination schedule to boost their protective antibodies against multiple lineages of SARS-CoV-2 variants. These patients are not at a higher risk of vaccine-associated adverse events. More research is required on the schedule of booster vaccination and the type of booster vaccine for special populations.
Checklist Declaration
1) Not in contravention of the European Respiratory Society (ERS) policy on tobacco
2) No funding on this project, and therefore no issue of copyright transfer
3) Completed International Committee of Medical Journal Editors (ICMJE) conflict of interest disclosure and submitted as an attachment
4) Consented submission with email address specified
Author Contributions
Literature search, study designs, figures, data collections, data analysis, data interpretation, and manuscript writing were done by MZY, MY, and C-LL. MZY and MY contributed equally to this work. All authors have read and agreed to the published version of the manuscript.
Conflict of Interest
The authors declare that the research was conducted in the absence of any commercial or financial relationships that could be construed as a potential conflict of interest.
Publisher’s Note
All claims expressed in this article are solely those of the authors and do not necessarily represent those of their affiliated organizations or those of the publisher, the editors, and the reviewers. Any product that may be evaluated in this article, or claim that may be made by its manufacturer, is not guaranteed or endorsed by the publisher.
Supplementary Material
The Supplementary Material for this article can be found online at: https://www.frontiersin.org/articles/10.3389/fddsv.2022.858006/full#supplementary-material
References
Abbasi, J. (2021). COVID-19 mRNA Vaccines Blunt Breakthrough Infection Severity. JAMA 326 (6), 473. doi:10.1001/jama.2021.12619
Abu-Farha, R., Mukattash, T., Itani, R., Karout, S., Khojah, H. M. J., Abed Al-Mahmood, A., et al. (2021). Willingness of Middle Eastern Public to Receive COVID-19 Vaccines. Saudi Pharm. J. 29 (7), 734–739. doi:10.1016/j.jsps.2021.05.005
Abu-Raddad, L. J., Chemaitelly, H., and Butt, A. A.National Study Group for C-V (2021). Effectiveness of the BNT162b2 Covid-19 Vaccine against the B.1.1.7 and B.1.351 Variants. N. Engl. J. Med. 385 (2), 187–189. doi:10.1056/nejmc2104974
Al Kaabi, N., Zhang, Y., Xia, S., Yang, Y., Al Qahtani, M. M., Abdulrazzaq, N., et al. (2021). Effect of 2 Inactivated SARS-CoV-2 Vaccines on Symptomatic COVID-19 Infection in Adults: A Randomized Clinical Trial. JAMA 326 (1), 35–45. doi:10.1001/jama.2021.8565
Albach, F. N., Burmester, G. R., and Biesen, R. (2021). Successful BNT162b2 Booster Vaccinations in a Patient with Rheumatoid Arthritis and Initially Negative Antibody Response. Ann. Rheum. Dis. 80 (10), 1361–1362. doi:10.1136/annrheumdis-2021-220834
Alter, G., Yu, J., Liu, J., Chandrashekar, A., Borducchi, E. N., Tostanoski, L. H., et al. (2021). Immunogenicity of Ad26.COV2.S Vaccine against SARS-CoV-2 Variants in Humans. Nature 596 (7871), 268–272. doi:10.1038/s41586-021-03681-2
Arbel, R., Hammerman, A., Sergienko, R., Friger, M., Peretz, A., Netzer, D., et al. (2021). BNT162b2 Vaccine Booster and Mortality Due to Covid-19. N. Engl. J. Med. 385 (26), 2413–2420. doi:10.1056/nejmoa2115624
Arya, A., Li, M., Aburjania, N., Singh, P., Royer, T., Moss, S., et al. (2021). COVID-19 in Solid Organ Transplantation: Disease Severity and Clinical Update. Transplant. Proc. 53 (4), 1227–1236. doi:10.1016/j.transproceed.2021.02.014
Asghar Abdoli, R. A., Aminianfar, H., Kianmehr, Z., Azimi, E., Emamipour, N., Jamshidi, H., et al. (2021). Safety and Potency of COVIran Barekat Inactivated Vaccine Candidate for SARS-CoV-2: A Preclinical Study. bioRxiv.
Baker, M. C., Mallajosyula, V., Davis, M. M., Boyd, S. D., Nadeau, K. C., and Robinson, W. H. (2021). Effective Viral Vector SARS-CoV-2 Booster Vaccination in a Patient with Rheumatoid Arthritis after Initial Ineffective mRNA Vaccine Response. Arthritis Rheumatol. 74 (3), 541–542. doi:10.1002/art.41978
Bar-On, Y. M., Goldberg, Y., Mandel, M., Bodenheimer, O., Freedman, L., Kalkstein, N., et al. (2021). Protection of BNT162b2 Vaccine Booster against Covid-19 in Israel. N. Engl. J. Med. 385 (15), 1393–1400. doi:10.1056/nejmoa2114255
Barchuk, A., Cherkashin, M., Bulina, A., Berezina, N., Rakova, T., Kuplevatskaya, D., et al. (2021). Vaccine Effectiveness against Referral to Hospital and Severe Lung Injury Associated with COVID-19: A Population-Based Case-Control Study in St. Petersburg, Russia. medRxiv.
Barda, N., Dagan, N., Ben-Shlomo, Y., Kepten, E., Waxman, J., Ohana, R., et al. (2021). Safety of the BNT162b2 mRNA Covid-19 Vaccine in a Nationwide Setting. N. Engl. J. Med. 385 (12), 1078–1090. doi:10.1056/nejmoa2110475
Barouch, D. H., Stephenson, K. E., Sadoff, J., Yu, J., Chang, A., Gebre, M., et al. (2021). Durable Humoral and Cellular Immune Responses 8 Months after Ad26.COV2.S Vaccination. N. Engl. J. Med. 385 (10), 951–953. doi:10.1056/nejmc2108829
Barrett, J. R., Belij-Rammerstorfer, S., Dold, C., Ewer, K. J., Folegatti, P. M., Gilbride, C., et al. (2021). Phase 1/2 Trial of SARS-CoV-2 Vaccine ChAdOx1 nCoV-19 with a Booster Dose Induces Multifunctional Antibody Responses. Nat. Med. 27 (2), 279–288. doi:10.1038/s41591-020-01179-4
Benotmane, I., Gautier, G., Perrin, P., Olagne, J., Cognard, N., Fafi-Kremer, S., et al. (2021). Antibody Response after a Third Dose of the mRNA-1273 SARS-CoV-2 Vaccine in Kidney Transplant Recipients with Minimal Serologic Response to 2 Doses. JAMA 326 (11), 1063–1065. doi:10.1001/jama.2021.12339
Bensouna, I., Caudwell, V., Kubab, S., Acquaviva, S., Pardon, A., Vittoz, N., et al. (2021). SARS-CoV-2 Antibody Response after a Third Dose of the BNT162b2 Vaccine in Patients Receiving Maintenance Hemodialysis or Peritoneal Dialysis. Am. J. Kidney Dis. 79 (2), 185–192. doi:10.1053/j.ajkd.2021.08.005
Bergwerk, M., Gonen, T., Lustig, Y., Amit, S., Lipsitch, M., Cohen, C., et al. (2021). Covid-19 Breakthrough Infections in Vaccinated Health Care Workers. N. Engl. J. Med. 385 (16), 1474–1484. doi:10.1056/nejmoa2109072
BIOTECH, B. (2021). Fact Sheet for Vaccination Recipients and Caregivers: Covaxin SARS-CoV-2 Vaccine. Available from: https://www.bharatbiotech.com/images/covaxin/covaxin-factsheet.pdf.
Borobia, A. M., Carcas, A. J., Pérez-Olmeda, M., Castaño, L., Bertran, M. J., García-Pérez, J., et al. (2021). Immunogenicity and Reactogenicity of BNT162b2 Booster in ChAdOx1-S-Primed Participants (CombiVacS): a Multicentre, Open-Label, Randomised, Controlled, Phase 2 Trial. Lancet 398 (10295), 121–130. doi:10.1016/S0140-6736(21)01420-3
Bosch, W., Cowart, J. B., Bhakta, S., Carter, R. E., Wadei, H. M., Shah, S. Z., et al. (2021). COVID-19 Vaccine-Breakthrough Infections Requiring Hospitalization in Mayo Clinic Florida through August 2021. Clin. Infect. Dis. 2, ciab932. doi:10.1093/cid/ciab932
Boyarsky, B. J., Werbel, W. A., Avery, R. K., Tobian, A. A. R., Massie, A. B., Segev, D. L., et al. (2021). Antibody Response to 2-Dose SARS-CoV-2 mRNA Vaccine Series in Solid Organ Transplant Recipients. JAMA 325 (21), 2204–2206. doi:10.1001/jama.2021.7489
Boyarsky, B. J., Werbel, W. A., Avery, R. K., Tobian, A. A. R., Massie, A. B., Segev, D. L., et al. (2021). Immunogenicity of a Single Dose of SARS-CoV-2 Messenger RNA Vaccine in Solid Organ Transplant Recipients. JAMA 325 (17), 1784–1786. doi:10.1001/jama.2021.4385
Brosh-Nissimov, T., Orenbuch-Harroch, E., Chowers, M., Elbaz, M., Nesher, L., Stein, M., et al. (2021). BNT162b2 Vaccine Breakthrough: Clinical Characteristics of 152 Fully Vaccinated Hospitalized COVID-19 Patients in Israel. Clin. Microbiol. Infect. 27 (11), 1652–1657. doi:10.1016/j.cmi.2021.06.036
Bruxvoort, K. J., Sy, L. S., Qian, L., Ackerson, B. K., Luo, Y., Lee, G. S., et al. (2021). Effectiveness of mRNA-1273 against delta, Mu, and Other Emerging Variants of SARS-CoV-2: Test Negative Case-Control Study. BMJ 375, e068848. doi:10.1136/bmj-2021-068848
Burki, T. (2021). Booster Shots for COVID-19-The Debate Continues. Lancet Infect. Dis. 21 (10), 1359–1360. doi:10.1016/s1473-3099(21)00574-0
Butt, A. A., Nafady-Hego, H., Chemaitelly, H., Abou-Samra, A.-B., Khal, A. A., Coyle, P. V., et al. (2021). Outcomes Among Patients with Breakthrough SARS-CoV-2 Infection after Vaccination. Int. J. Infect. Dis. 110, 353–358. doi:10.1016/j.ijid.2021.08.008
Campbell, F., Archer, B., Laurenson-Schafer, H., Jinnai, Y., Konings, F., Batra, N., et al. (2021). Increased Transmissibility and Global Spread of SARS-CoV-2 Variants of Concern as at June 2021. Euro Surveill. 26 (24), 1. doi:10.2807/1560-7917.ES.2021.26.24.2100509
Cavalcanti, E., Isgrò, M. A., Rea, D., Di Capua, L., Trillò, G., Russo, L., et al. (2021). Vaccination Strategy and Anti - SARS-CoV-2 S Titers in Healthcare Workers of the INT - IRCCS "Fondazione Pascale" Cancer Center (Naples, Italy). Infect. Agents Cancer 16 (1), 32. doi:10.1186/s13027-021-00375-2
CDC, U. (2021). CDC Statement on ACIP Booster Recommendations. Available from:https://www.cdc.gov/media/releases/2021/p0924-booster-recommendations-.html.
CDC, U. (2021). Comparative Effectiveness of Moderna, Pfizer-BioNTech, and Janssen (Johnson & Johnson) Vaccines in Preventing COVID-19 Hospitalizations Among Adults without Immunocompromising Conditions — United States, March–August 2021. Morbidity and Mortality Weekly Report. MMWR 70 (38), 1337–1343.
Cerqueira-Silva, T., de Araújo Oliveira, V., Pescarini, J., Júnior, J. B., Machado, T. M., Ortiz, R. F., et al. (2021). The Effectiveness of Vaxzevria and CoronaVac Vaccines: A Nationwide Longitudinal Retrospective Study of 61 Million Brazilians (VigiVac-COVID19). medRxiv.
Charmet, T., Schaeffer, L., Grant, R., Galmiche, S., Chény, O., Von Platen, C., et al. (2021). Impact of Original, B.1.1.7, and B.1.351/P.1 SARS-CoV-2 Lineages on Vaccine Effectiveness of Two Doses of COVID-19 mRNA Vaccines: Results from a Nationwide Case-Control Study in France. The Lancet Reg. Health - Europe 8, 100171. doi:10.1016/j.lanepe.2021.100171
Chavarot, N., Morel, A., Leruez-Ville, M., Vilain, E., Divard, G., Burger, C., et al. (2021). Weak Antibody Response to Three Doses of mRNA Vaccine in Kidney Transplant Recipients Treated with Belatacept. Am. J. Transpl. 21 (12), 4043–4051. doi:10.1111/ajt.1681410.1111/ajt.16814
Chemaitelly, H., Tang, P., Hasan, M. R., AlMukdad, S., Yassine, H. M., Benslimane, F. M., et al. (2021). Waning of BNT162b2 Vaccine Protection against SARS-CoV-2 Infection in Qatar. N. Engl. J. Med. 385 (24), e83. doi:10.1056/nejmoa2114114
Choudhary, O. P., Choudhary, P., and Singh, I. (2021). India's COVID-19 Vaccination Drive: Key Challenges and Resolutions. Lancet Infect. Dis. 21 (11), 1483–1484. doi:10.1016/s1473-3099(21)00567-3
Chung, H., He, S., Nasreen, S., Sundaram, M. E., Buchan, S. A., Wilson, S. E., et al. (2021). Effectiveness of BNT162b2 and mRNA-1273 Covid-19 Vaccines against Symptomatic SARS-CoV-2 Infection and Severe Covid-19 Outcomes in Ontario, Canada: Test Negative Design Study. BMJ 374, n1943. doi:10.1136/bmj.n1943
Ciabattini, A., Pastore, G., Fiorino, F., Polvere, J., Lucchesi, S., Pettini, E., et al. (2021). Evidence of SARS-CoV-2-specific Memory B Cells Six Months after Vaccination with the BNT162b2 mRNA Vaccine. Front. Immunol. 12, 740708. doi:10.3389/fimmu.2021.740708
ClinicalTrials.Gov (2021). A Phase 1/2 Safety and Immunogenicity Trial of COVID-19 Vaccine COVIVAC - NCT04830800. Available from: https://clinicaltrials.gov/ct2/show/NCT04830800.
ClinicalTrials.Gov (2021). A Safety and Immunogenicity Study of Inactivated SARS-CoV-2 Vaccine (Vero Cells) in Healthy Population Aged 18 Years and above (COVID-19) - NCT04758273. Available from: https://clinicaltrials.gov/ct2/show/NCT04758273.
ClinicalTrials.Gov (2021). A Study to Evaluate MVC-Cov1901 Vaccine against COVID-19 in Adult (COVID-19) - NCT04695652. Available from: https://clinicaltrials.gov/ct2/show/NCT04695652.
ClinicalTrials.Gov (2021). A Study to Evaluate Safety and Immunogenicity of Inactivated SARS-CoV-2 Vaccine (Vero Cells) in Healthy Population Aged 18 Years and above (COVID-19) - NCT04756323. Available from: https://clinicaltrials.gov/ct2/show/NCT04756323.
ClinicalTrials.Gov (2021). A Study to Evaluate the Efficacy, Safety and Immunogenicity of SARS-CoV-2 Vaccine (Vero Cells), Inactivated in Healthy Adults Aged 18 Years and Older (COVID-19) - NCT04852705. Available from: https://clinicaltrials.gov/ct2/show/NCT04852705.
ClinicalTrials.Gov (2021). A Study to Evaluate the Safety and Immunogenicity of MVC-Cov1901 against COVID-19. Available from: https://clinicaltrials.gov/ct2/show/NCT04487210.
ClinicalTrials.Gov (2021). An Open Study on the Safety, Tolerability, and Immunogenicity of "Sputnik Light" Vaccine - NCT04713488. Available from: https://www.clinicaltrials.gov/ct2/show/NCT04713488.
ClinicalTrials.Gov (2021). Immunogenicity, Efficacy and Safety of QazCovid-In® COVID-19 Vaccine -NCT04691908. Available from: https://www.clinicaltrials.gov/ct2/show/NCT04691908.
ClinicalTrials.Gov (2021). Reactogenicity, Safety and Immunogenicity of QazCovid-In® COVID-19 Vaccine - NCT04530357. Available from: https://clinicaltrials.gov/ct2/show/study/NCT04530357?draw=2.
ClinicalTrials.Gov (2021). Safety and Immunogenicity Study of an Inactivated SARS-CoV-2 Vaccine for Preventing against COVID-19 - NCT04412538. Available from: https://www.clinicaltrials.gov/ct2/show/NCT04412538.
ClinicalTrials.Gov (2021). Study to Evaluate Efficacy, Immunogenicity and Safety of the Sputnik-Light (SPUTNIK-LIGHT) - NCT04741061. Available from: https://www.clinicaltrials.gov/ct2/show/NCT04741061.
ClinicalTrials.Gov (2021). The Efficacy, Safety and Immunogenicity Study of Inactivated SARS-CoV-2 Vaccine for Preventing against COVID-19 - NCT04659239. Available from: https://www.clinicaltrials.gov/ct2/show/NCT04659239.
Collie, S., Champion, J., Moultrie, H., Bekker, L.-G., and Gray, G. (2022). Effectiveness of BNT162b2 Vaccine against Omicron Variant in South Africa. N. Engl. J. Med. 386 (5), 494–496. doi:10.1056/nejmc2119270
Connolly, C. M., Teles, M., Frey, S., Boyarsky, B. J., Alejo, J. L., Werbel, W. A., et al. (2021). Booster-dose SARS-CoV-2 Vaccination in Patients with Autoimmune Disease: a Case Series. Ann. Rheum. Dis. 81 (2), 291–293. doi:10.1136/annrheumdis-2021-221206
Cromer, D., Juno, J. A., Khoury, D., Reynaldi, A., Wheatley, A. K., Kent, S. J., et al. (2021). Prospects for Durable Immune Control of SARS-CoV-2 and Prevention of Reinfection. Nat. Rev. Immunol. 21 (6), 395–404. doi:10.1038/s41577-021-00550-x
D'Amelio, R., Asero, R., Cassatella, M. A., Lagana, B., Lunardi, C., Migliorini, P., et al. (2021). Anti-COVID-19 Vaccination in Patients with Autoimmune-Autoinflammatory Disorders and Primary/Secondary Immunodeficiencies: The Position of the Task Force on Behalf of the Italian Immunological Societies. Biomedicines 9 (9), 1. doi:10.3390/biomedicines9091163
Dan, J. M., Mateus, J., Kato, Y., Hastie, K. M., Yu, E. D., Faliti, C. E., et al. (2021). Immunological Memory to SARS-CoV-2 Assessed for up to 8 Months after Infection. Science 371 (6529), 1. doi:10.1126/science.abf4063
den Hartog, G., Vos, E. R. A., van den Hoogen, L. L., van Boven, M., Schepp, R. M., Smits, G., et al. (2021). Persistence of Antibodies to SARS-CoV-2 in Relation to Symptoms in a Nationwide Prospective Study. Clin. Infect. Dis. 73 (12), 2155–2162. doi:10.1093/cid/ciab172
Deng, X., Evdokimova, M., O'Brien, A., Rowe, C. L., Clark, N. M., Harrington, A., et al. (2021). Breakthrough Infections with Multiple Lineages of SARS-CoV-2 Variants Reveals Continued Risk of Severe Disease in Immunosuppressed Patients. Viruses 13 (9), 1. doi:10.3390/v13091743
Dey, A., Chozhavel Rajanathan, T. M., Chandra, H., Pericherla, H. P. R., Kumar, S., Choonia, H. S., et al. (2021). Immunogenic Potential of DNA Vaccine Candidate, ZyCoV-D against SARS-CoV-2 in Animal Models. bioRxiv.
Duarte, L. F., Gálvez, N. M. S., Iturriaga, C., Melo-González, F., Soto, J. A., Schultz, B. M., et al. (2021). Immune Profile and Clinical Outcome of Breakthrough Cases after Vaccination with an Inactivated SARS-CoV-2 Vaccine. Front. Immunol. 12, 742914. doi:10.3389/fimmu.2021.742914
Dyer, O. (2021). Covid-19: Rich Countries' Booster Plans Will Impede Global Vaccination, Experts Say. BMJ 374, n2353. doi:10.1136/bmj.n2353
Ebinger, J. E., Fert-Bober, J., Printsev, I., Wu, M., Sun, N., Prostko, J. C., et al. (2021). Antibody Responses to the BNT162b2 mRNA Vaccine in Individuals Previously Infected with SARS-CoV-2. Nat. Med. 27 (6), 981–984. doi:10.1038/s41591-021-01325-6
El Sahly, H. M., Baden, L. R., Essink, B., Doblecki-Lewis, S., Martin, J. M., Anderson, E. J., et al. (2021). Efficacy of the mRNA-1273 SARS-CoV-2 Vaccine at Completion of Blinded Phase. N. Engl. J. Med. 385 (19), 1774–1785. doi:10.1056/nejmoa2113017
EMA (2021). Signal Assessment Report on Embolic and Thrombotic Events (SMQ) with COVID-19 Vaccine (ChAdOx1-S [recombinant]) – COVID-19 Vaccine AstraZeneca (Other Viral Vaccines). Available from: https://www.ema.europa.eu/en/documents/prac-recommendation/signal-assessment-report-embolic-thrombotic-events-smq-covid-19-vaccine-chadox1-s-recombinant-covid_en.pdf.
EMA (2021). Summary of Product Characteristics - Astrazeneca Covid-Vaccine. Available from: https://www.ema.europa.eu/en/documents/product-information/vaxzevria-previously-covid-19-vaccine-astrazeneca-epar-product-information_en.pdf.
England, P. H. (2021). SARS-CoV-2 Variants of Concern and Variants under Investigation in England Technical Briefing 17. Available from: https://assets.publishing.service.gov.uk/government/uploads/system/uploads/attachment_data/file/1001354/Variants_of_Concern_VOC_Technical_Briefing_17.pdf.
Erice, A., Varillas-Delgado, D., and Caballero, C. (2022). Decline of Antibody Titres 3 Months after Two Doses of BNT162b2 in Non-immunocompromised Adults. Clin. Microbiol. Infect. 28 (1), 139.e1–139.e4. doi:10.1016/j.cmi.2021.08.023
Flaxman, A., Marchevsky, N. G., Jenkin, D., Aboagye, J., Aley, P. K., Angus, B., et al. (2021). Reactogenicity and Immunogenicity after a Late Second Dose or a Third Dose of ChAdOx1 nCoV-19 in the UK: a Substudy of Two Randomised Controlled Trials (COV001 and COV002). Lancet 398 (10304), 981–990. doi:10.1016/S0140-6736(21)01699-8
Furer, V., Eviatar, T., Zisman, D., Peleg, H., Paran, D., Levartovsky, D., et al. (2021). Immunogenicity and Safety of the BNT162b2 mRNA COVID-19 Vaccine in Adult Patients with Autoimmune Inflammatory Rheumatic Diseases and in the General Population: a Multicentre Study. Ann. Rheum. Dis. 80 (10), 1330–1338. doi:10.1136/annrheumdis-2021-220647
Gagne, M., Corbett, K. S., Flynn, B. J., Foulds, K. E., Wagner, D. A., Andrew, S. F., et al. (2021). Protection from SARS-CoV-2 Delta One Year after mRNA-1273 Vaccination in Nonhuman Primates Is Coincident with an Anamnestic Antibody Response in the Lower Airway. bioRxiv.
Galmiche, S. N. L., Tartour, E., de Lamballerie, X., Wittkop, L., Loubet, P., and Launay, O. (2021). Immunological and Clinical Efficacy of COVID-19 Vaccines in Immunocompromised Populations: a Systematic Review. Clin. Microbiol. Infect. 28 (2), 163–177. doi:10.1016/j.cmi.2021.09.036
Glatman-Freedman, A., Bromberg, M., Dichtiar, R., Hershkovitz, Y., and Keinan-Boker, L. (2021). The BNT162b2 Vaccine Effectiveness against New COVID-19 Cases and Complications of Breakthrough Cases: A Nation-wide Retrospective Longitudinal Multiple Cohort Analysis Using Individualised Data. EBioMedicine 72, 103574. doi:10.1016/j.ebiom.2021.103574
Gluck, V., Grobecker, S., Kostler, J., Tydykov, L., Bertok, M., Weidlich, T., et al. (2021). Immunity after COVID-19 and Vaccination: Follow-Up Study over 1 Year Among Medical Personnel. Infection, 1–8. doi:10.1007/s15010-021-01703-9
Gobbi, F., Buonfrate, D., Moro, L., Rodari, P., Piubelli, C., Caldrer, S., et al. (2021). Antibody Response to the BNT162b2 mRNA COVID-19 Vaccine in Subjects with Prior SARS-CoV-2 Infection. Viruses 13 (3). doi:10.3390/v13030422
Gray, G. E., Collie, S., Garrett, N., Goga, A., Champion, J., Zylstra, M., et al. (2021). Vaccine Effectiveness against Hospital Admission in South African Health Care Workers Who Received a Homologous Booster of Ad26.COV2 during an Omicron COVID19 Wave: Preliminary Results of the Sisonke 2 Study. medRxiv.
Hall, V. J., Foulkes, S., Saei, A., Andrews, N., Oguti, B., Charlett, A., et al. (2021). COVID-19 Vaccine Coverage in Health-Care Workers in England and Effectiveness of BNT162b2 mRNA Vaccine against Infection (SIREN): a Prospective, Multicentre, Cohort Study. Lancet 397 (10286), 1725–1735. doi:10.1016/S0140-6736(21)00790-X
Havervall, S., Marking, U., Greilert-Norin, N., Ng, H., Gordon, M., Salomonsson, A.-C., et al. (2021). Antibody Responses after a Single Dose of ChAdOx1 nCoV-19 Vaccine in Healthcare Workers Previously Infected with SARS-CoV-2. EBioMedicine 70, 103523. doi:10.1016/j.ebiom.2021.103523
Hillus, D., Schwarz, T., Tober-Lau, P., Hastor, H., Thibeault, C., Kasper, S., et al. (2021). Safety, Reactogenicity, and Immunogenicity of Homologous and Heterologous Prime-Boost Immunisation with ChAdOx1-nCoV19 and BNT162b2: A Prospective Cohort Study. medRxiv.
Hitchings, M. D. T., Ranzani, O. T., Dorion, M., D’Agostini, T. L., de Paula, R. C., de Paula, O. F. P., et al. (2021). Effectiveness of the ChAdOx1 Vaccine in the Elderly during SARS-CoV-2 Gamma Variant Transmission in Brazil. medRxiv.
Hsieh, S.-M., Liu, W.-D., Huang, Y.-S., Lin, Y.-J., Hsieh, E.-F., Lian, W.-C., et al. (2021). Safety and Immunogenicity of a Recombinant Stabilized Prefusion SARS-CoV-2 Spike Protein Vaccine (MVC COV1901) Adjuvanted with CpG 1018 and Aluminum Hydroxide in Healthy Adults: A Phase 1, Dose-Escalation Study. EClinicalMedicine 38, 100989. doi:10.1016/j.eclinm.2021.100989
Husain, S. A., and Argyropoulos, C. P. (2021). Boosters and Optimizing SARS-CoV-2 Vaccine for Transplantation: No Time to Wait. Am. J. Transpl. 22 (1), 328–329. doi:10.1111/ajt.16788
IRCT (2021). Comparison of the Safety, Efficacy and Immunogenicity of Fakhravac and Sinopharm SARS-CoV-2 Vaccines, in Adults Aged 18 and over; a Phase III Randomised, Non-inferiority Clinical Trial. Available from: https://en.irct.ir/trial/57980.
IRCT (2021). Phase 2 Trial of Safety and Immunogenicity of 10 Micro Gram Inactivated SARS-CoV-2 Vaccine (FAKHRAVAC), Two Doses Two Weeks Apart in Adults Aged 18-70 Years: a Randomized, Double-Blind, Placebo-Controlled, Clinical Trial. Available from: https://en.irct.ir/trial/56027.
Jara, A., Undurraga, E. A., Gonzalez, C., Paredes, F., Fontecilla, T., Jara, G., et al. (2021). Effectiveness of an Inactivated SARS-CoV-2 Vaccine in Chile. N. Engl. J. Med. 385 (10), 875–884. doi:10.1056/nejmoa2107715
Jeewandara, C., Kamaladasa, A., Pushpakumara, P. D., Jayathilaka, D., Aberathna, I. S., Danasekara, D. R. S. R., et al. (2021). Immune Responses to a Single Dose of the AZD1222/Covishield Vaccine in Health Care Workers. Nat. Commun. 12 (1), 4617. doi:10.1038/s41467-021-24579-7
Kamar, N., Abravanel, F., Marion, O., Couat, C., Izopet, J., and Del Bello, A. (2021). Three Doses of an mRNA Covid-19 Vaccine in Solid-Organ Transplant Recipients. N. Engl. J. Med. 385 (7), 661–662. doi:10.1056/nejmc2108861
Kang, Y., Li, Y., Sun, L., Deng, A., Hu, T., Zhang, J., et al. (2021). Effectiveness of Inactivated COVID-19 Vaccines against COVID-19 Pneumonia and Severe Illness Caused by the B.1.617.2 (Delta) Variant: Evidence from an Outbreak in Guangdong, China. SSRN [Epub ahead of print]. doi:10.2139/ssrn.3895639
Kant, S., and Geetha, D. (2021). Impact of Rituximab on Humoral Response to COVID-19 Booster Vaccine and Antibody Kinetics in Patients with Anti-neutrophil Cytoplasmic Antibody Vasculitis. Kidney Int. 100 (5), 1124–1127. doi:10.1016/j.kint.2021.08.020
Kant, S., Kronbichler, A., Salas, A., Bruchfeld, A., and Geetha, D. (2021). Timing of COVID-19 Vaccine in the Setting of Anti-CD20 Therapy: A Primer for Nephrologists. Kidney Int. Rep. 6 (5), 1197–1199. doi:10.1016/j.ekir.2021.03.876
Kent, S. J., and Juno, J. A. (2021). Vaccination after Prior COVID-19 Infection: Implications for Dose Sparing and Booster Shots. EBioMedicine 72, 103586. doi:10.1016/j.ebiom.2021.103586
Kherabi, Y., Fiolet, T., Rozencwajg, S., Salaün, J.-P., and Peiffer-Smadja, N. (2021). COVID-19 Vaccine Boosters: What Do We Know So Far? Anaesth. Crit. Care Pain Med. 40, 100959. doi:10.1016/j.accpm.2021.100959
Khoury, D. S., Cromer, D., Reynaldi, A., Schlub, T. E., Wheatley, A. K., Juno, J. A., et al. (2021). Neutralizing Antibody Levels Are Highly Predictive of Immune protection from Symptomatic SARS-CoV-2 Infection. Nat. Med. 27 (7), 1205–1211. doi:10.1038/s41591-021-01377-8
Kozlovskaya, L. I., Piniaeva, A. N., Ignatyev, G. M., Gordeychuk, I. V., Volok, V. P., Rogova, Y. V., et al. (2021). Long-term Humoral Immunogenicity, Safety and Protective Efficacy of Inactivated Vaccine against COVID-19 (CoviVac) in Preclinical Studies. Emerging Microbes & Infections 10 (1), 1790–1806. doi:10.1080/22221751.2021.1971569
Krammer, F., Srivastava, K., Team, P., and Simon, V. (2021). Robust Spike Antibody Responses and Increased Reactogenicity in Seropositive Individuals after a Single Dose of SARS-CoV-2 mRNA Vaccine. medRxiv, 1.
Kumar, N. P., Padmapriyadarsini, C., Uma Devi, K. R., Banurekha, V. V., Nancy, A., Girish Kumar, C. P., et al. (2021). Antibody Responses to the BBV152 Vaccine in Individuals Previously Infected with SARS-CoV-2: A Pilot Study. Indian J. Med. Res. 153 (5&6), 671–676. doi:10.4103/ijmr.IJMR_2066_21
Lee, H. K., Knabl, L., Knabl, L., Kapferer, S., Pateter, B., Walter, M., et al. (2021). Robust Immune Response to the BNT162b mRNA Vaccine in an Elderly Population Vaccinated 15 Months after Recovery from COVID-19. medRxiv.
Lefevre, B., Tondeur, L., Madec, Y., Grant, R., Lina, B., van der Werf, S., et al. (2021). Beta SARS-CoV-2 Variant and BNT162b2 Vaccine Effectiveness in Long-Term Care Facilities in France. Lancet Healthy Longev 2 (11), e685–e687. doi:10.1016/s2666-7568(21)00230-0
Levin, E. G., Lustig, Y., Cohen, C., Fluss, R., Indenbaum, V., Amit, S., et al. (2021). Waning Immune Humoral Response to BNT162b2 Covid-19 Vaccine over 6 Months. N. Engl. J. Med. 385 (24), e84. doi:10.1056/nejmoa2114583
Li, X.-N., Huang, Y., Wang, W., Jing, Q.-L., Zhang, C.-H., Qin, P.-Z., et al. (2021). Effectiveness of Inactivated SARS-CoV-2 Vaccines against the Delta Variant Infection in Guangzhou: a Test-Negative Case-Control Real-World Study. Emerging Microbes & Infections 10 (1), 1751–1759. doi:10.1080/22221751.2021.1969291
Liao, Y., Zhang, Y., Zhao, H., Pu, J., Zhao, Z., Li, D., et al. (2021). Intensified Antibody Response Elicited by Boost Suggests Immune Memory in Individuals Administered Two Doses of SARS-CoV-2 Inactivated Vaccine. Emerging Microbes & Infections 10 (1), 1112–1115. doi:10.1080/22221751.2021.1937328
Liu, C., Lee, J., Ta, C., Soroush, A., Rogers, J. R., Kim, J. H., et al. (2021). A Retrospective Analysis of COVID-19 mRNA Vaccine Breakthrough Infections – Risk Factors and Vaccine Effectiveness. medRxiv.
Liu, H., Wei, P., Zhang, Q., Aviszus, K., Linderberger, J., Yang, J., et al. (2021). The Lambda Variant of SARS-CoV-2 Has a Better Chance than the Delta Variant to Escape Vaccines. bioRxiv.
Liu, X., Shaw, R. H., Stuart, A. S. V., Greenland, M., Aley, P. K., Andrews, N. J., et al. (2021). Safety and Immunogenicity of Heterologous versus Homologous Prime-Boost Schedules with an Adenoviral Vectored and mRNA COVID-19 Vaccine (Com-COV): a Single-Blind, Randomised, Non-inferiority Trial. The Lancet 398 (10303), 856–869. doi:10.1016/s0140-6736(21)01694-9
Logunov, D. Y., Dolzhikova, I. V., Shcheblyakov, D. V., Tukhvatulin, A. I., Zubkova, O. V., Dzharullaeva, A. S., et al. (2021). Safety and Efficacy of an rAd26 and rAd5 Vector-Based Heterologous Prime-Boost COVID-19 Vaccine: an Interim Analysis of a Randomised Controlled Phase 3 Trial in Russia. The Lancet 397 (10275), 671–681. doi:10.1016/s0140-6736(21)00234-8
Lopez Bernal, J., Andrews, N., Gower, C., Gallagher, E., Simmons, R., Thelwall, S., et al. (2021). Effectiveness of Covid-19 Vaccines against the B.1.617.2 (Delta) Variant. N. Engl. J. Med. 385 (7), 585–594. doi:10.1056/nejmoa2108891
Lozano-Ojalvo, D., Camara, C., Lopez-Granados, E., Nozal, P., Del Pino-Molina, L., Bravo-Gallego, L. Y., et al. (2021). Differential Effects of the Second SARS-CoV-2 mRNA Vaccine Dose on T Cell Immunity in Naive and COVID-19 Recovered Individuals. Cell Rep. 36 (8), 109570. doi:10.1016/j.celrep.2021.109570
Ltd SLSC (2021). COVID-19 Vaccine (Vero Cell), Inactivated (Brief Version) Conditional Market Authorization. Available from: https://www.covidvaccine.gov.hk/pdf/CoronaVac_ENG_PI_brief.pdf.
Lumley, S. F., Wei, J., O'Donnell, D., Stoesser, N. E., Matthews, P. C., Howarth, A., et al. (2021). The Duration, Dynamics, and Determinants of Severe Acute Respiratory Syndrome Coronavirus 2 (SARS-CoV-2) Antibody Responses in Individual Healthcare Workers. Clin. Infect. Dis. 73 (3), e699–e709. doi:10.1093/cid/ciab004
Mackintosh, J. A., Lipman, M., Lowe, D. M., and Renzoni, E. A. (2021). Protecting the Vulnerable: SARS-CoV-2 Vaccination in Immunosuppressed Patients with Interstitial Lung Disease. Lancet Respir. Med. 9 (9), 947–949. doi:10.1016/s2213-2600(21)00326-x
Madhi, S. A., Baillie, V., Cutland, C. L., Voysey, M., Koen, A. L., Fairlie, L., et al. (2021). Efficacy of the ChAdOx1 nCoV-19 Covid-19 Vaccine against the B.1.351 Variant. N. Engl. J. Med. 384 (20), 1885–1898. doi:10.1056/NEJMoa2102214
Malik, J. A., Mulla, A. H., Farooqi, T., Pottoo, F. H., Anwar, S., and Rengasamy, K. R. R. (2021). Targets and Strategies for Vaccine Development against SARS-CoV-2. Biomed. Pharmacother. 137, 111254. doi:10.1016/j.biopha.2021.111254
Mallapaty, S. (2021). Iran Hopes to Defeat COVID with home-grown Crop of Vaccines. Nature 596 (7873), 475. doi:10.1038/d41586-021-02216-z
Mega, E. R. (2021). Can Cuba Beat COVID with its Homegrown Vaccines?. Nature [Epub ahead of print]. doi:10.1038/d41586-021-01126-4
Menni, C., Klaser, K., May, A., Polidori, L., Capdevila, J., Louca, P., et al. (2021). Vaccine Side-Effects and SARS-CoV-2 Infection after Vaccination in Users of the COVID Symptom Study App in the UK: a Prospective Observational Study. Lancet Infect. Dis. 21 (7), 939–949. doi:10.1016/s1473-3099(21)00224-3
Mevorach, D., Anis, E., Cedar, N., Bromberg, M., Haas, E. J., Nadir, E., et al. (2021). Myocarditis after BNT162b2 mRNA Vaccine against Covid-19 in Israel. N. Engl. J. Med. 385 (23), 2140–2149. doi:10.1056/nejmoa2109730
Moghaddar, M., Radman, R., and Macreadie, I. (2021). Severity, Pathogenicity and Transmissibility of Delta and Lambda Variants of SARS-CoV-2, Toxicity of Spike Protein and Possibilities for Future Prevention of COVID-19. Microorganisms 9 (10), 1. doi:10.3390/microorganisms9102167
Moghnieh, R., Mekdashi, R., El-Hassan, S., Abdallah, D., Jisr, T., Bader, M., et al. (2021). Immunogenicity and Reactogenicity of BNT162b2 Booster in BBIBP-CorV-Vaccinated Individuals Compared with Homologous BNT162b2 Vaccination: Results of a Pilot Prospective Cohort Study from Lebanon. Vaccine 39 (46), 6713–6719. doi:10.1016/j.vaccine.2021.10.007
Momin, T., Kansagra, K., Patel, H., Sharma, S., Sharma, B., Patel, J., et al. (2021). Safety and Immunogenicity of a DNA SARS-CoV-2 Vaccine (ZyCoV-D): Results of an Open-Label, Non-randomized Phase I Part of Phase I/II Clinical Study by Intradermal Route in Healthy Subjects in India. EClinicalMedicine 38, 101020. doi:10.1016/j.eclinm.2021.101020
Munro, A. P. S., Janani, L., Cornelius, V., Aley, P. K., Babbage, G., Baxter, D., et al. (2021). Safety and Immunogenicity of Seven COVID-19 Vaccines as a Third Dose (Booster) Following Two Doses of ChAdOx1 nCov-19 or BNT162b2 in the UK (COV-BOOST): A Blinded, Multicentre, Randomised, Controlled, Phase 2 Trial. Lancet 398 (10318), 2258–2276. doi:10.1016/S0140-6736(21)02717-3
Naaber, P., Tserel, L., Kangro, K., Sepp, E., Jürjenson, V., Adamson, A., et al. (2021). Dynamics of Antibody Response to BNT162b2 Vaccine after Six Months: a Longitudinal Prospective Study. Lancet Reg. Health - Europe 10, 100208. doi:10.1016/j.lanepe.2021.100208
NACI (2021). Recommendations on the Use of COVID-19 Vaccines. Available from: https://www.canada.ca/en/public-health/services/immunization/national-advisory-committee-on-immunization-naci/recommendations-use-covid-19-vaccines.html?hq_e=el&hq_m=2188311&hq_l=1&hq_v=91d220e044.
Naranbhai, V., Pernat, C. A., Gavralidis, A., St Denis, K. J., Lam, E. C., Spring, L. M., et al. (2021). Immunogenicity and Reactogenicity of SARS-CoV-2 Vaccines in Patients with Cancer: The CANVAX Cohort Study. J. Clin. Oncol. 40 (1), 12–23. doi:10.1200/JCO.21.01891
Nasreen, S., Chung, H., He, S., Brown, K. A., Gubbay, J. B., Buchan, S. A., et al. (2022). Effectiveness of COVID-19 Vaccines against Symptomatic SARS-CoV-2 Infection and Severe Outcomes with Variants of Concern in Ontario. Nat. Microbiol. 7 (3), 379–385. doi:10.1038/s41564-021-01053-0
Nasreen, S., Chung, H., He, S., Brown, K. A., Gubbay, J. B., Buchan, S. A., et al. (2021). Effectiveness of mRNA and ChAdOx1 COVID-19 Vaccines against Symptomatic SARS-CoV-2 Infection and Severe Outcomes with Variants of Concern in Ontario. medRxiv, 2021.
Neidleman, J., Luo, X., McGregor, M., Xie, G., Murray, V., Greene, W. C., et al. (2021). mRNA Vaccine-Induced T Cells Respond Identically to SARS-CoV-2 Variants of Concern but Differ in Longevity and Homing Properties Depending on Prior Infection Status. Elife 10, 10. doi:10.7554/eLife.72619
Palacios, R., Patiño, E. G., de Oliveira Piorelli, R., Conde, M. T. R. P., Batista, A. P., Zeng, G., et al. (2020). Double-Blind, Randomized, Placebo-Controlled Phase III Clinical Trial to Evaluate the Efficacy and Safety of Treating Healthcare Professionals with the Adsorbed COVID-19 (Inactivated) Vaccine Manufactured by Sinovac - PROFISCOV: A Structured Summary of a Study Protocol for a Randomised Controlled Trial. Trials 21 (1), 853. doi:10.1186/s13063-020-04775-4
Peeters, M., Verbruggen, L., Teuwen, L., Vanhoutte, G., Vande Kerckhove, S., Peeters, B., et al. (2021). Reduced Humoral Immune Response after BNT162b2 Coronavirus Disease 2019 Messenger RNA Vaccination in Cancer Patients under Antineoplastic Treatment. ESMO Open 6 (5), 100274. doi:10.1016/j.esmoop.2021.100274
Pegu, A., O'Connell, S. E., Schmidt, S. D., O'Dell, S., Talana, C. A., Lai, L., et al. (2021). Durability of mRNA-1273 Vaccine-Induced Antibodies against SARS-CoV-2 Variants. Science 373 (6561), 1372–1377. doi:10.1126/science.abj4176
Peled, Y., Ram, E., Lavee, J., Segev, A., Matezki, S., Wieder-Finesod, A., et al. (2021). Third Dose of the BNT162b2 Vaccine in Heart Transplant Recipients: Immunogenicity and Clinical Experience. J. Heart Lung Transpl. 41 (2), 148–157. doi:10.1016/j.healun.2021.08.010
Perkmann, T., Perkmann-Nagele, N., Mucher, P., Radakovics, A., Repl, M., Koller, T., et al. (2021). Initial SARS-CoV-2 Vaccination Response Can Predict Booster Response for BNT162b2 but Not for AZD1222. Int. J. Infect. Dis. 110, 309–313. doi:10.1016/j.ijid.2021.07.063
Pouwels, K. B., Pritchard, E., Matthews, P. C., Stoesser, N., Eyre, D. W., Vihta, K. D., et al. (2021). Effect of Delta Variant on Viral burden and Vaccine Effectiveness against New SARS-CoV-2 Infections in the UK. Nat. Med. 27 (12), 2127–2135. doi:10.1038/s41591-021-01548-7
Powers, A. C., Aronoff, D. M., and Eckel, R. H. (2021). COVID-19 Vaccine Prioritisation for Type 1 and Type 2 Diabetes. Lancet Diabetes Endocrinol. 9 (3), 140–141. doi:10.1016/s2213-8587(21)00017-6
Priyanka, , Choudhary, O. P., Singh, I., and Patra, G. (2020). Aerosol Transmission of SARS-CoV-2: The Unresolved Paradox. Trav. Med. Infect. Dis. 37, 101869. doi:10.1016/j.tmaid.2020.101869
Quiroga, B., Soler, M. J., Ortiz, A., Vaquera, S. M., Mantecon, C. J. J., Useche, G., et al. (2021). Safety and Immediate Humoral Response of COVID-19 Vaccines in Chronic Kidney Disease Patients: the SENCOVAC Study. Nephrol. Dial. Transpl. 12, gfab313. doi:10.1093/ndt/gfab313
Ramasamy, M. N., Minassian, A. M., Ewer, K. J., Flaxman, A. L., Folegatti, P. M., Owens, D. R., et al. (2021). Safety and Immunogenicity of ChAdOx1 nCoV-19 Vaccine Administered in a Prime-Boost Regimen in Young and Old Adults (COV002): a Single-Blind, Randomised, Controlled, Phase 2/3 Trial. Lancet 396 (10267), 1979–1993. doi:10.1016/S0140-6736(20)32466-1
Ranzani, O. T., Hitchings, M. D. T., Dorion, M., D’Agostini, T. L., de Paula, R. C., de Paula, O. F. P., et al. (2021). Effectiveness of the CoronaVac Vaccine in Older Adults during a Gamma Variant Associated Epidemic of Covid-19 in Brazil: Test Negative Case-Control Study. BMJ 374, n2015. doi:10.1136/bmj.n2015
Reis, B. Y., Barda, N., Leshchinsky, M., Kepten, E., Hernan, M. A., Lipsitch, M., et al. (2021). Effectiveness of BNT162b2 Vaccine against Delta Variant in Adolescents. N. Engl. J. Med. 385 (22), 2101–2103. doi:10.1056/nejmc2114290
Reuters (2021). Cuba Says Abdala Vaccine 92.28% Effective against Coronavirus. Available from: https://www.reuters.com/business/healthcare-pharmaceuticals/cuba-says-abdala-vaccine-9228-effective-against-coronavirus-2021-06-21/.
RPCEC (2021). ABDALA Clinical Study - Phase III. Available from: https://rpcec.sld.cu/en/trials/RPCEC00000359-En.
Ryzhikov, A. B., Ryzhikov, E. А., Bogryantseva, M. P., Danilenko, E. D., Imatdinov, I. R., Nechaeva, E. A., et al. (2021). Immunogenicity and Protectivity of the Peptide Candidate Vaccine against SARS-CoV-2. Ann. RAMS 76 (1), 5–19. doi:10.15690/vramn1528
Saadat, S., Rikhtegaran Tehrani, Z., Logue, J., Newman, M., Frieman, M. B., Harris, A. D., et al. (2021). Binding and Neutralization Antibody Titers after a Single Vaccine Dose in Health Care Workers Previously Infected with SARS-CoV-2. JAMA 325 (14), 1467–1469. doi:10.1001/jama.2021.3341
Sadoff, J., Gray, G., Vandebosch, A., Cardenas, V., Shukarev, G., Grinsztejn, B., et al. (2022). Final Analysis of Efficacy and Safety of Single-Dose Ad26.COV2.S. N. Engl. J. Med. 9, NEJMoa2117608. doi:10.1056/nejmoa2117608
Sadoff, J., Gray, G., Vandebosch, A., Cárdenas, V., Shukarev, G., Grinsztejn, B., et al. (2021). Safety and Efficacy of Single-Dose Ad26.COV2.S Vaccine against Covid-19. N. Engl. J. Med. 384 (23), 2187–2201. doi:10.1056/nejmoa2101544
Sapkal, G. N., Yadav, P. D., Ella, R., Deshpande, G. R., Sahay, R. R., Gupta, N., et al. (2021). Inactivated COVID-19 Vaccine BBV152/COVAXIN Effectively Neutralizes Recently Emerged B.1.1.7 Variant of SARS-CoV-2. J. Trav. Med 28 (4). doi:10.1093/jtm/taab051
Schaefer, G. O., Leland, R. J., and Emanuel, E. J. (2021). Making Vaccines Available to Other Countries before Offering Domestic Booster Vaccinations. JAMA 326 (10), 903–904. doi:10.1001/jama.2021.13226
Schmidt, T., Klemis, V., Schub, D., Schneitler, S., Reichert, M. C., Wilkens, H., et al. (2021). Cellular Immunity Predominates over Humoral Immunity after Homologous and Heterologous mRNA and Vector-Based COVID-19 Vaccine Regimens in Solid Organ Transplant Recipients. Am. J. Transpl. 21 (12), 3990–4002. doi:10.1111/ajt.16818
Shapiro Ben David, S., Shamir-Stein, N. a., Baruch Gez, S., Lerner, U., Rahamim-Cohen, D., and Ekka Zohar, A. (2021). Reactogenicity of a Third BNT162b2 mRNA COVID-19 Vaccine Among Immunocompromised Individuals and Seniors - A Nationwide Survey. Clin. Immunol. 232, 108860. doi:10.1016/j.clim.2021.108860
Sheikh, A., McMenamin, J., Taylor, B., Robertson, C., Public Health, S., and the, E. I. I. C. (2021). SARS-CoV-2 Delta VOC in Scotland: Demographics, Risk of Hospital Admission, and Vaccine Effectiveness. Lancet 397 (10293), 2461–2462. doi:10.1016/S0140-6736(21)01358-1
Shroff, R. T., Chalasani, P., Wei, R., Pennington, D., Quirk, G., Schoenle, M. V., et al. (2021). Immune Responses to Two and Three Doses of the BNT162b2 mRNA Vaccine in Adults with Solid Tumors. Nat. Med. 27 (11), 2002–2011. doi:10.1038/s41591-021-01542-z
Shrotri, M., Navaratnam, A. M. D., Nguyen, V., Byrne, T., Geismar, C., Fragaszy, E., et al. (2021). Spike-antibody Waning after Second Dose of BNT162b2 or ChAdOx1. The Lancet 398 (10298), 385–387. doi:10.1016/s0140-6736(21)01642-1
Soiza, R. L., Scicluna, C., and Thomson, E. C. (2021). Efficacy and Safety of COVID-19 Vaccines in Older People. Age Ageing 50 (2), 279–283. doi:10.1093/ageing/afaa274
Stowe, J. A. N., Gower, C., Gallagher, E., Utsi, L., Simmons, R., et al. (2021). Effectiveness of COVID-19 Vaccines against Hospital Admission with the Delta (B.1.617.2) Variant. Public Health England. Available from: https://khub.net/documents/135939561/479607266/Effectiveness+of+COVID-19+vaccines+against+hospital+admission+with+the+Delta+%28B.1.617.2%29+variant.pdf/1c213463-3997-ed16-2a6f-14e5deb0b997?t=1623689315431.
Tang, P., Hasan, M. R., Chemaitelly, H., Yassine, H. M., Benslimane, F. M., Khatib, H. A. A., et al. (2021). BNT162b2 and mRNA-1273 COVID-19 Vaccine Effectiveness against the Delta (B.1.617.2) Variant in Qatar. medRxiv, 2021.
Tang, P., Hasan, M. R., Chemaitelly, H., Yassine, H. M., Benslimane, F. M., Al Khatib, H. A., et al. (2021). BNT162b2 and mRNA-1273 COVID-19 Vaccine Effectiveness against the SARS-CoV-2 Delta Variant in Qatar. Nat. Med. 27 (12), 2136–2143. doi:10.1038/s41591-021-01583-4
Tanriover, M. D., Doğanay, H. L., Akova, M., Güner, H. R., Azap, A., Akhan, S., et al. (2021). Efficacy and Safety of an Inactivated Whole-Virion SARS-CoV-2 Vaccine (CoronaVac): Interim Results of a Double-Blind, Randomised, Placebo-Controlled, Phase 3 Trial in Turkey. The Lancet 398 (10296), 213–222. doi:10.1016/s0140-6736(21)01429-x
The Lancet Infectious Diseases, D. (2021). COVID-19 Vaccine Equity and Booster Doses. Lancet Infect. Dis. 21 (9), 1193. doi:10.1016/s1473-3099(21)00486-2
Thomas, S. J., Moreira, E. D., Kitchin, N., Absalon, J., Gurtman, A., Lockhart, S., et al. (2021). Safety and Efficacy of the BNT162b2 mRNA Covid-19 Vaccine through 6 Months. N. Engl. J. Med. 385 (19), 1761–1773. doi:10.1056/nejmoa2110345
Thompson, M. G., Stenehjem, E., Grannis, S., Ball, S. W., Naleway, A. L., Ong, T. C., et al. (2021). Effectiveness of Covid-19 Vaccines in Ambulatory and Inpatient Care Settings. N. Engl. J. Med. 385 (15), 1355–1371. doi:10.1056/nejmoa2110362
Torjesen, I. (2021). Covid-19: Omicron May Be More Transmissible Than Other Variants and Partly Resistant to Existing Vaccines, Scientists Fear. BMJ 375, n2943. doi:10.1136/bmj.n2943
Tseng, H. F., Ackerson, B. K., Luo, Y., Sy, L. S., Talarico, C. A., Tian, Y., et al. (2022). Effectiveness of mRNA-1273 against SARS-CoV-2 Omicron and delta Variants. medRxiv.
Turner, J. S., Kim, W., Kalaidina, E., Goss, C. W., Rauseo, A. M., Schmitz, A. J., et al. (2021). SARS-CoV-2 Infection Induces Long-Lived Bone Marrow Plasma Cells in Humans. Nature 595 (7867), 421–425. doi:10.1038/s41586-021-03647-4
Uriu, K., Kimura, I., Shirakawa, K., Takaori-Kondo, A., Nakada, T. A., Kaneda, A., et al. (2021). Neutralization of the SARS-CoV-2 Mu Variant by Convalescent and Vaccine Serum. N. Engl. J. Med. 385 (25), 2397–2399. doi:10.1056/nejmc2114706
USCDC (2021). ACIP COVID-19 Vaccines. Available from: https://www.cdc.gov/vaccines/acip/meetings/downloads/slides-2021-04-23/06-COVID-Oliver-508.pdf.
USCDC (2021). COVID-19 Vaccines while Pregnant or Breastfeeding. Available from: https://www.cdc.gov/coronavirus/2019-ncov/vaccines/recommendations/pregnancy.html.
USCDC (2021). Interim Clinical Considerations for Use of COVID-19 Vaccines Currently Approved or Authorized in the United States. Available from: https://www.cdc.gov/vaccines/covid-19/clinical-considerations/covid-19-vaccines-us.html?CDC_AA_refVal=https%3A%2F%2Fwww.cdc.gov%2Fvaccines%2Fcovid-19%2Finfo-by-product%2Fclinical-considerations.html#Contraindications.
USFDA (2021). Fact Sheet for Healthcare Providers Administering Vaccine (Vaccination Providers) Emergency Use Authorization (Eua) of the Janssen Covid-19 Vaccine to Prevent Coronavirus Disease 2019 (Covid-19). Available from: https://www.fda.gov/media/146304/download.
USFDA (2021). Fact Sheet for Healthcare Providers Administering Vaccine (Vaccination Providers) Emergency Use Authorization (Eua) of the Moderna Covid-19 Vaccine to Prevent Coronavirus Disease 2019 (Covid-19). Available from: https://www.fda.gov/media/144637/download.
USFDA (2021). Fact Sheet for Healthcare Providers Administering Vaccine (Vaccination Providers) Emergency Use Authorization (Eua) of the Pfizer-Biontech Covid-19 Vaccine to Prevent Coronavirus Disease 2019 (Covid-19). Available from: https://www.fda.gov/media/144413/download.
Valdes-Balbin, Y., Santana-Mederos, D., Quintero, L., Fernández, S., Rodriguez, L., Sanchez Ramirez, B., et al. (2021). SARS-CoV-2 RBD-Tetanus Toxoid Conjugate Vaccine Induces a Strong Neutralizing Immunity in Preclinical Studies. ACS Chem. Biol. 16 (7), 1223–1233. doi:10.1021/acschembio.1c00272
Van De Pas, R., Widdowson, M.-A., Ravinetto, R., N Srinivas, P., N Srinivas, T. J., Fofana, T. O., et al. (2022). COVID-19 Vaccine Equity: a Health Systems and Policy Perspective. Expert Rev. Vaccin. 21 (1), 25–36. doi:10.1080/14760584.2022.2004125
Vicenti, I., Gatti, F., Scaggiante, R., Boccuto, A., Zago, D., Basso, M., et al. (2021). Single-dose BNT162b2 mRNA COVID-19 Vaccine Significantly Boosts Neutralizing Antibody Response in Health Care Workers Recovering from Asymptomatic or Mild Natural SARS-CoV-2 Infection. Int. J. Infect. Dis. 108, 176–178. doi:10.1016/j.ijid.2021.05.033
Vie Pce (2021). Aprueba El Cecmed El Autorizo De Uso De Emergencia Del Candidato Vacunal Cubano Abdala. Available from: https://www.cecmed.cu/noticias/aprueba-cecmed-autorizo-uso-emergencia-candidato-vacunal-cubano-abdala.
Voysey, M., Costa Clemens, S. A., Madhi, S. A., Weckx, L. Y., Folegatti, P. M., Aley, P. K., et al. (2021). Single-dose Administration and the Influence of the Timing of the Booster Dose on Immunogenicity and Efficacy of ChAdOx1 nCoV-19 (AZD1222) Vaccine: a Pooled Analysis of Four Randomised Trials. Lancet 397 (10277), 881–891. doi:10.1016/S0140-6736(21)00432-3
Wang, H., Zhang, Y., Huang, B., Deng, W., Quan, Y., Wang, W., et al. (2020). Development of an Inactivated Vaccine Candidate, BBIBP-CorV, with Potent Protection against SARS-CoV-2. Cell 182 (3), 713–721. doi:10.1016/j.cell.2020.06.008
Wang, L., Wang, Q., Davis, P. B., Volkow, N. D., and Xu, R. (2022). Increased Risk for COVID-19 Breakthrough Infection in Fully Vaccinated Patients with Substance Use Disorders in the United States between December 2020 and August 2021. World Psych. 21, 124–132. doi:10.1002/wps.20921
Wang, M., Zhang, L., Li, Q., Wang, B., Liang, Z., Sun, Y., et al. (2021). Reduced Sensitivity of the SARS-CoV-2 Lambda Variant to Monoclonal Antibodies and Neutralizing Antibodies Induced by Infection and Vaccination. Emerg. Microbes Infect. 11, 1–30. doi:10.1080/22221751.2021.2008775
Wang, Q., Zhuang, P., Huang, H., Li, L., Liu, L., Webber, H. C., et al. (2020). Mouse γ-Synuclein Promoter-Mediated Gene Expression and Editing in Mammalian Retinal Ganglion Cells. J. Neurosci. 40 (20), 3896–3914. doi:10.1523/jneurosci.0102-20.2020
Wei, J., Stoesser, N., Matthews, P. C., Ayoubkhani, D., Studley, R., Bell, I., et al. (2021). Antibody Responses to SARS-CoV-2 Vaccines in 45,965 Adults from the General Population of the United Kingdom. Nat. Microbiol. 6 (9), 1140–1149. doi:10.1038/s41564-021-00947-3
Werbel, W. A., Boyarsky, B. J., Ou, M. T., Massie, A. B., Tobian, A. A. R., Garonzik-Wang, J. M., et al. (2021). Safety and Immunogenicity of a Third Dose of SARS-CoV-2 Vaccine in Solid Organ Transplant Recipients: A Case Series. Ann. Intern. Med. 174 (9), 1330–1332. doi:10.7326/l21-0282
WHO (2021). COVID-19 Subcommittee of the WHO Global Advisory Committee on Vaccine Safety (GACVS): Updated Guidance Regarding Myocarditis and Pericarditis Reported with COVID-19 mRNA Vaccines. Available from: https://www.who.int/news/item/09-07-2021-gacvs-guidance-myocarditis-pericarditis-covid-19-mrna-vaccines.
WHO (2021). Statement of the WHO Global Advisory Committee on Vaccine Safety (GACVS) COVID-19 Subcommittee on Reports of Guillain-Barré Syndrome (GBS) Following Adenovirus Vector COVID-19 Vaccines. Available from: https://www.who.int/news/item/26-07-2021-statement-of-the-who-gacvs-covid-19-subcommittee-on-gbs.
WHO (2021). The Sinovac-CoronaVac COVID-19 Vaccine: What You Need to Know. Available from: https://www.who.int/news-room/feature-stories/detail/the-sinovac-covid-19-vaccine-what-you-need-to-know.
WHO (2021). Tracking SARS-CoV-2 Variants. Available from: https://www.who.int/en/activities/tracking-SARS-CoV-2-variants/.
Wu, S., Huang, J., Zhang, Z., Wu, J., Zhang, J., Hu, H., et al. (2021). Safety, Tolerability, and Immunogenicity of an Aerosolised Adenovirus Type-5 Vector-Based COVID-19 Vaccine (Ad5-nCoV) in Adults: Preliminary Report of an Open-Label and Randomised Phase 1 Clinical Trial. Lancet Infect. Dis. 21 (12), 1654–1664. doi:10.1016/s1473-3099(21)00396-0
Xia, S., Duan, K., Zhang, Y., Zhao, D., Zhang, H., Xie, Z., et al. (2020). Effect of an Inactivated Vaccine against SARS-CoV-2 on Safety and Immunogenicity Outcomes. JAMA 324 (10), 951–960. doi:10.1001/jama.2020.15543
Xia, S., Zhang, Y., Wang, Y., Wang, H., Yang, Y., Gao, G. F., et al. (2021). Safety and Immunogenicity of an Inactivated SARS-CoV-2 Vaccine, BBIBP-CorV: a Randomised, Double-Blind, Placebo-Controlled, Phase 1/2 Trial. Lancet Infect. Dis. 21 (1), 39–51. doi:10.1016/s1473-3099(20)30831-8
Xie, X., Han, J. B., Ma, G., Feng, X. L., Li, X., Zou, Q. C., et al. (2021). Emerging SARS-CoV-2 B.1.621/Mu Variant Is Prominently Resistant to Inactivated Vaccine-Elicited Antibodies. Zool Res. 42 (6), 789–791. doi:10.24272/j.issn.2095-8137.2021.343
Yahav, D., Rozen-Zvi, B., Mashraki, T., Atamna, A., Ben-Zvi, H., Bar-Haim, E., et al. (2021). Immunosuppression Reduction when Administering a Booster Dose of the BNT162b2 mRNA SARS-CoV-2 Vaccine in Kidney Transplant Recipients without Adequate Humoral Response Following Two Vaccine Doses: Protocol for a Randomised Controlled Trial (BECAME Study). BMJ Open 11 (10), e055611. doi:10.1136/bmjopen-2021-055611
Yan, Z. P., Yang, M., and Lai, C. L. (2021). COVID-19 Vaccines: A Review of the Safety and Efficacy of Current Clinical Trials. Pharmaceuticals (Basel) 14 (5). doi:10.3390/ph14050406
Yan, Z., Yang, M., and Lai, C.-L. (2021). COVID-19 Vaccinations: A Comprehensive Review of Their Safety and Efficacy in Special Populations. Vaccines 9 (10), 1097. doi:10.3390/vaccines9101097
Yan, Z., Yang, M., and Lai, C.-L. (2021). Long COVID-19 Syndrome: A Comprehensive Review of its Effect on Various Organ Systems and Recommendation on Rehabilitation Plans. Biomedicines 9 (8), 966. doi:10.3390/biomedicines9080966
Yang, S., Li, Y., Dai, L., Wang, J., He, P., Li, C., et al. (2021). Safety and Immunogenicity of a Recombinant Tandem-Repeat Dimeric RBD-Based Protein Subunit Vaccine (ZF2001) against COVID-19 in Adults: Two Randomised, Double-Blind, Placebo-Controlled, Phase 1 and 2 Trials. Lancet Infect. Dis. 21 (8), 1107–1119. doi:10.1016/s1473-3099(21)00127-4
Yigit, M., Ozkaya-Parlakay, A., Cosgun, Y., Ince, Y. E., Bulut, Y. E., and Senel, E. (2021). Should a Third Booster Dose Be Scheduled after Two Doses of CoronaVac? A Single-center Experience. J. Med. Virol. 94 (1), 287–290. doi:10.1002/jmv.27318
Yigit, M., Ozkaya-Parlakay, A., and Senel, E. (2021). Evaluation of COVID-19 Vaccine Refusal in Parents. Pediatr. Infect. Dis. J. 40 (4), e134–e136. doi:10.1097/inf.0000000000003042
Zhao, X., Zheng, A., Li, D., Zhang, R., Sun, H., Wang, Q., et al. (2021). Neutralisation of ZF2001-Elicited Antisera to SARS-CoV-2 Variants. Lancet Microbe 2 (10), e494. doi:10.1016/s2666-5247(21)00217-2
Zhou, Y., Zhang, J., Wu, W., Liang, M., and Wu, Q.-S. (2021). Willingness to Receive Future COVID-19 Vaccines Following the COVID-19 Epidemic in Shanghai, China. BMC Public Health 21 (1), 1103. doi:10.1186/s12889-021-11174-0
Zhukova, A., Blassel, L., Lemoine, F., Morel, M., Voznica, J., and Gascuel, O. (2020). Origin, Evolution and Global Spread of SARS-CoV-2. C R Biol. [Epub ahead of print]. doi:10.5802/crbiol.29
Zuckerman, N., Nemet, I., Kliker, L., Atari, N., Lustig, Y., Bucris, E., et al. (2021). The SARS-CoV-2 Lambda Variant and its Neutralisation Efficiency Following Vaccination with Comirnaty, Israel, April to June 2021. Euro Surveill. 26 (45), 1. doi:10.2807/1560-7917.ES.2021.26.45.2100974
Рыжиков ЕАР, А. Б., Богрянцева, М. П., Усова, С. В., Даниленко, Е. Д., Нечаева, Е. А., Пьянков, О. В., et al. (2021). A Single Blind, Placebo-Controlled Randomized Study of the Safety, Reactogenicity and Immunogenicity of the “EpiVacCorona” Vaccine for the Prevention of COVID-19, in Volunteers Aged 18–60 Years (Phase I–II). Moscow: Russian Journal of Infection and Immunity.
Keywords: COVID-19, vaccinations, booster, risks analysis, review
Citation: Yan MZ, Yang M and Lai C-L (2022) Review of Clinical Trials of COVID-19 Vaccination Booster in SARS-CoV-2 Variants Era: To Take It or Not To Take It. Front. Drug. Discov. 2:858006. doi: 10.3389/fddsv.2022.858006
Received: 19 January 2022; Accepted: 22 February 2022;
Published: 22 March 2022.
Edited by:
Bruno Villoutreix, Institut National de la Santé et de la Recherche Médicale (INSERM), FranceReviewed by:
Om Prakash Choudhary, Central Agricultural University, IndiaObasanjo Afolabi Bolarinwa, University of KwaZulu Natal, South Africa
Copyright © 2022 Yan, Yang and Lai. This is an open-access article distributed under the terms of the Creative Commons Attribution License (CC BY). The use, distribution or reproduction in other forums is permitted, provided the original author(s) and the copyright owner(s) are credited and that the original publication in this journal is cited, in accordance with accepted academic practice. No use, distribution or reproduction is permitted which does not comply with these terms.
*Correspondence: Michael Zhipeng Yan, dTM1Mzc4MjFAY29ubmVjdC5oa3UuaGs=; Ching-Lung Lai, aHJtZWxjbEBoa3UuaGs=
†These authors share first authorship