- 1Department of Pharmaceutical Chemistry, Institute of Pharmaceutical Sciences, University of Graz, Graz, Austria
- 2Department of Medicine, University of Maryland School of Medicine, Baltimore, MD, United States
- 3Field of Excellence BioHealth, University of Graz, Graz, Austria
Enzymatic oxidation or autooxidation of esterified polyunsaturated fatty acids (PUFA) residues within phospholipids in cell membranes or circulating lipoprotein particles leads to the formation of a broad range of oxidized phospholipid (OxPL) species. Chronically elevated OxPL levels present in circulation and atherosclerotic plaques are thought to induce proinflammatory and injurious effects on blood- and vessel wall cells. However, analysis of the structure-activity relationship also identified specific OxPL products exhibiting prominent anti-inflammatory, pro-survival and barrier protective properties. This minireview will briefly summarize rapidly accumulating evidence pointing to the importance of OxPLs in pathology, where they can play multiple roles of biomarkers, drug targets and drug leads.
Introduction
Membrane PLs contain the major cellular pool of PUFAs, which can be oxidized enzymatically or non-enzymatically thus producing biologically active oxidized phospholipids (OxPLs, Figure 1) (Hajeyah et al., 2020; Spickett, 2020). During the last two decades OxPLs have been extensively characterized as drivers of pathology in multiple inflammatory and metabolic conditions. This minireview will focus on the emerging role of OxPLs in medicine and pharmacology, where they are increasingly recognized as disease markers, drug targets and drug leads.
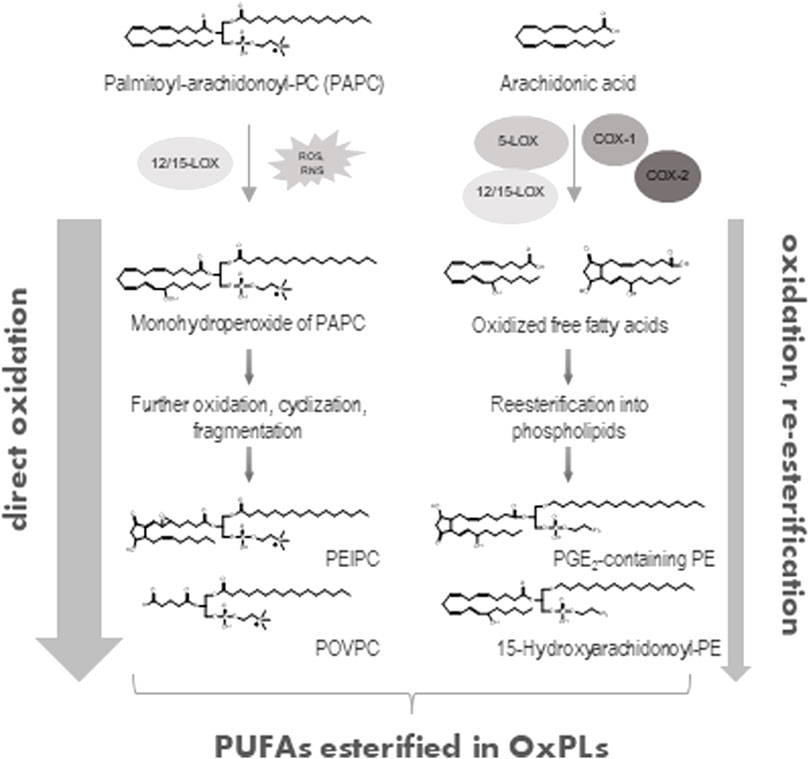
FIGURE 1. Generation of OxPLs. Direct oxidation of PUFA-containing phospholipids either via enzymatic (12/15-lipoxygenase, 12/15-LOX) or non-enzymatic (ROS- or RNS-mediated) reactions represents the major way of formation of OxPLs (left part). Alternatively, free, unesterified PUFAs (e.g., arachidonic acid) can be directly oxidized by lipoxygenases (5- or 12/15-LOX) or cycloxygenases (COX-1 or COX-2) and then esterified to a lyso-PL with generation of OxPLs (right part). Only few examples of possible OxPL structures are shown.
Oxidized phospholipids as biomarkers of chronic vascular disease and acute inflammation
Oxidative stress is a major mechanism underlying atherogenesis and cardiovascular disease. OxPLs represent a group of oxidation-generated lipids, some of which can covalently bind to proteins and form so-called oxidation-specific epitopes recognized by the innate and adaptive immunity. Such molecules accumulate in OxLDL and atherosclerotic plaques (Gugiu et al., 2008; Gonen et al., 2019) and stimulate chronic inflammation and deposition of lipids (Podrez et al., 2007; Bartolini Gritti and Binder, 2016). OxPLs are especially abundant in circulating apoB-100-containing lipoproteins LDL and Lp(a), where they are present both as free lipids and covalent complexes with apoB or apo(a) (Leibundgut et al., 2013). An established immune assay for circulating OxPLs is based on a monoclonal antibody E06, which recognizes oxidized phosphatidylcholine. Levels of OxPLs are normalized to the apoB-100 and therefore the readout is referred to as an OxPL-apoB ratio (Tsimikas et al., 2005). Multiple clinical association studies using the OxPL-apoB assay have shown correlation of OxPL-apoB levels with the progression, acute cardiovascular events, and efficiency of therapy of cardiovascular disease (Taleb et al., 2011; Byun et al., 2015; Capoulade et al., 2015; Byun et al., 2017). In addition, generation of OxPL products has been reported in various non-infectious (Philippova et al., 2019; Ademowo et al., 2020a; Solati et al., 2021) and infectious (Imai et al., 2008; Matt et al., 2015) diseases including COVID-19 (Akpinar et al., 2021). Importantly, several disease associations mentioned above have been independently confirmed by mass spectrometry-based methods, although such studies are relatively scarce in comparison to immune methods. An advantage of mass spectrometry is the ability to quantify different classes of OxPLs. Available publications on the clinical measurements of different classes and molecular species of OxPLs by ELISA or mass spectrometry are summarized in the Table 1.
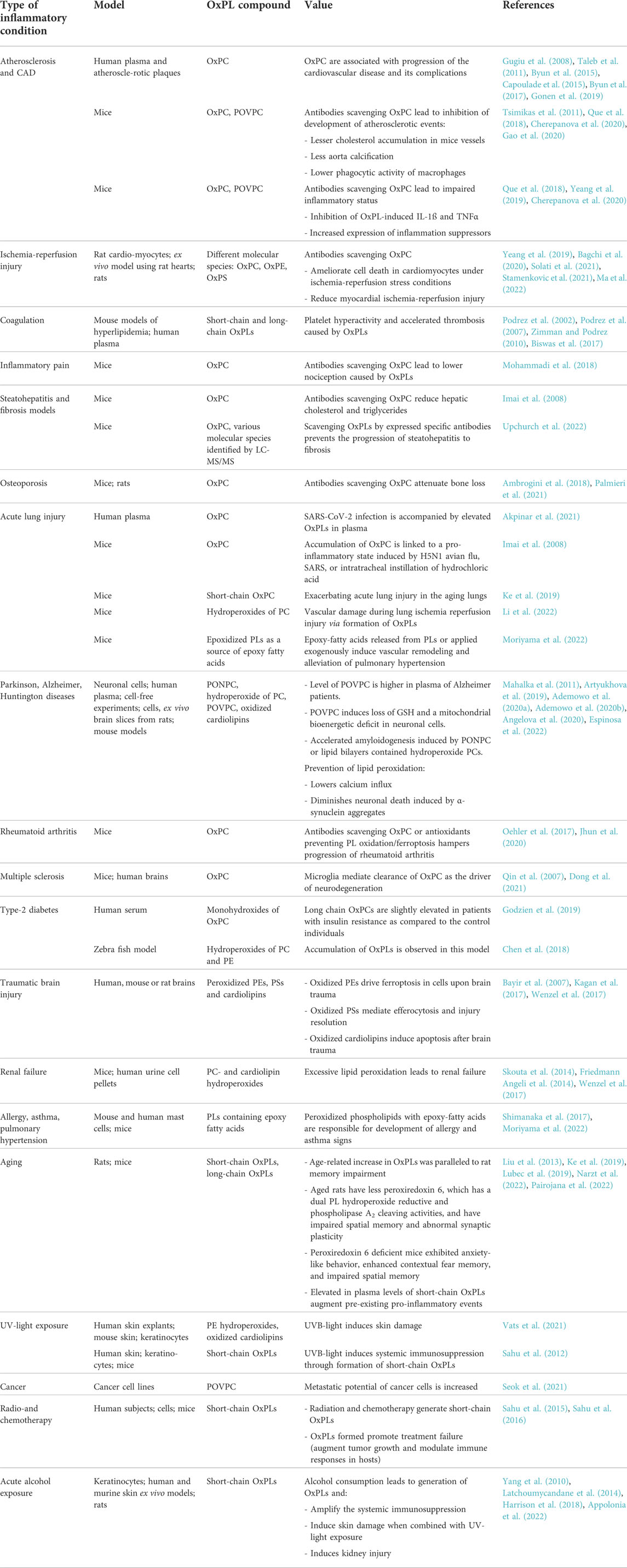
TABLE 1. Role of elevated OxPL products in various inflammatory diseases in humans and rodent models.
Open question: How direct is the relation between circulating levels of OxPLs and their pathological impact?
It is incorrect to limit the analysis of OxPLs solely with quantification of their circulating concentrations because the pathological impact depends on the balance of plasma concentrations and activity of protective mechanisms. We have developed an antibody-based in vitro test showing that human plasma contains components that either degrade or physically mask OxPLs thus potentially preventing interaction of OxPLs with cellular targets (Bochkov et al., 2016). This “masking” assay demonstrated a significant negative correlation between the masking capacity and atherosclerosis risk factors such as age, smoking, hypertension and family history of CAD (Bochkov et al., 2016). Furthermore, masking capacity negatively correlated with the presence of cardiovascular disease and acute events. In other words, cardiovascular risk burden in patients is accompanied with a weaker masking activity for circulating OxPLs, which can promote disease progression. Further work is needed to establish if a combination of the OxPL-apoB and masking assays can increase their diagnostic power.
Oxidized phospholipids as potential drug targets
The interest to OxPLs is not limited by their role as oxidative stress biomarkers. A large body of data suggests that OxPLs are likely to be active drivers of disease. By analogy with the effects of protein modifications on protein functions, one can say that oxidation induces a gain of function changes in PLs because OxPLs can trigger biological effects that cannot be induced by their non-oxidized precursors. Multiple (patho)physiological activities of OxPLs have been described including those related to innate and acquired immunity, blood clotting, atheroma formation, pain control, etc. (Bochkov et al., 2010; Karki and Birukov, 2021; Zhivaki and Kagan, 2022).
Animal models confirmed the importance of OxPLs in conditions of acute and chronic inflammation such as atherosclerosis (Que et al., 2018), nonalcoholic fatty liver disease (Sun et al., 2020; Upchurch et al. 2022), ischemia-reperfusion injury (Yeang et al., 2019) and osteoporosis (Ambrogini et al., 2018). In combination with human disease association data descried above, these results strongly support the causative involvement of OxPLs in the disease pathogenesis. Therefore, it is tempting to speculate that therapy directed at the neutralization of toxic and pro-inflammatory effects of OxPLs may help to cure human disease.
There are several experimental approaches targeting OxPLs in vivo. In addition to passive immunization with antibodies to OxPLs (Oehler et al., 2017), titers of natural anti-OxPL antibodies can be elevated by the immunization with Streptococcus pneumonia (Binder et al., 2003). Another approach is the use of anti-inflammatory and anti-atherogenic apoAI-mimetic peptides, which have high affinity to OxPLs and can inhibit their negative effects (Van Lenten et al., 2008; Getz and Reardon, 2011; Oehler et al., 2017). Furthermore, a major part of OxPCs in circulation is bound to Lp(a) and is responsible for pro-inflammatory properties of this lipoprotein (Bergmark et al., 2008; Scipione et al., 2015). Recent clinical trials show that circulating levels of Lp(a) and associated OxPLs can be effectively reduced by RNA-based approaches (Tsimikas et al., 2021). Another promising strategy is a reduction of the pro-inflammatory activities of truncated OxPLs by removing their oxidized acyl chains by administration of a highly conserved host lipase, acyloxyacyl hydrolase (AOAH) (Zou et al., 2021) or platelet-activating factor (PAF) acetylhydrolases (PAF-AHs) (McIntyre, 2012). Last but not least, it is known that pro-inflammatory signaling pathways induced by OxPLs at least partially differ from the pathways activated by classical inflammatory mediators such as TNFα, IL-1β, etc. (Bochkov et al., 2002a; Gargalovic et al., 2006). Thus, selective targeting of OxPLs-induced signal transduction potentially can prevent negative effects of OxPLs without impairing the normal inflammatory signaling.
Oxidized phospholipids as potential drug leads
Well-investigated families of lipid mediators, e.g., prostanoids, induce variable and often functionally opposite effects mediated by different receptors expressed in different cell types. In full agreement with this mode of action, under different biological conditions OxPLs can be either toxic and pro-inflammatory, or they can exhibit protective effects (Figure 2). It has been shown that OxPLs inhibit activation of Toll-like receptor 4 (TLR4) (Bochkov et al., 2002b), well recognized for its role in triggering sepsis. The effect of OxPLs in vivo was strong enough to protect animals from lethal doses of LPS (Bochkov et al., 2002b). An important anti-LPS mechanism is the mutually exclusive binding (antagonism) of OxPLs with TLR4 and its accessory proteins MD-2, CD14 and LBP (Bochkov et al., 2002b; Erridge et al., 2006).
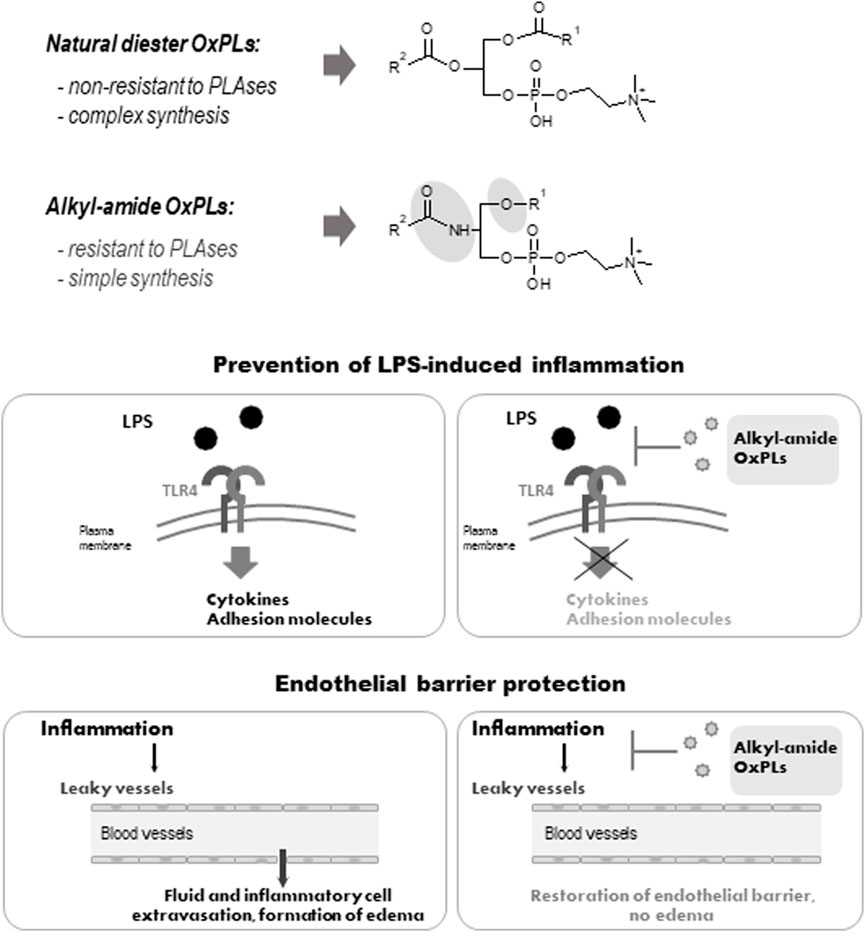
FIGURE 2. Structure and biological activity of alkyl-amide OxPLs. Fatty acid residues are linked via an sn-1 ether or an sn-2 amide bond of alkyl-amide OxPLs respectivelly, which simplifies their synthesis (upper panel). Alkyl-amide OxPLs inhibit LPS-induced inflammation (middle panel) and enhance lung endothelial barrier (lower panel) in vitro and in vivo (Oskolkova et al., 2021).
Another potentially beneficial property of OxPLs is their ability to enhance the lung endothelial barrier under basal conditions and after treatment with pathological stimuli such as bacteria, edemagenic bioactive peptides, inflammatory cytokines (TNFα, IL-6) and pathologic mechanical forces (Nonas et al., 2008; Karki and Birukov, 2020). These barrier-protective properties may be beneficial in treatment of sepsis-associated inflammation and its frequent complication—pulmonary edema. As compared to other molecules enhancing lung barrier, such as prostaglandins E2, and I2, the barrier-enhancing action of OxPAPC continues for significantly longer time. The efficiency of OxPLs in the prevention of sepsis and lung edema in animal models, as well as underlying molecular mechanisms, have been reviewed recently (Karki and Birukov, 2021).
The data presented above show that non-truncated OxPLs exhibit a unique combination of beneficial properties. They inhibit inflammation induced by bacteria, and at the same time act as long-acting enhancers of endothelial lung barrier. Such a combination may be especially beneficial for treatment of ARDS, where both bacterial inflammation and lung edema play an important role (Karki and Birukov, 2021). However, in vivo application of OxPLs is complicated by rapid degradation of diacyl OxPLs by phospholipases A1/A2. To improve pharmacokinetics, we have synthesized OxPLs in which fatty acid residues are bound with glycerol via an alkyl and an amide bonds. These bonds are not non-physiological: a large proportion of cellular phospholipids contains sn-1 alkyl bond, while an amide bond is typical for sphingolipids, which are also abundant in cells. We found that alkyl-amide OxPLs had good solubility, improved in vivo stability and demonstrated anti-LPS and barrier protective effects that were similar to the action of diacyl OxPAPC in vitro and in animal models (Figure 2). Thus, alkyl-amide OxPLs represent an improved hit for further development of a drug with polypharmacological activity simultaneously protecting against bacterial sepsis and pulmonary edema.
Given the deleterious effects of chronically elevated OxPLs in cardiovascular pathologies, could modified OxPLs still be beneficial for treatment of patients with pre-existing condition (hyperlipidemia, atherosclerosis, diabetes) suffering from ARDS and other inflammatory syndromes? Both sepsis and lung edema are acute conditions and require short-term application of OxPLs. Therefore, it is unlikely that treatment of such patients with OxPLs-based drugs for hours or days could aggravate concomitant chronic pathologies such as atherosclerosis, which develop during decades.
Conclusion
Similar to other families of lipid mediators, OxPLs may serve as biomarkers of oxidative stress and cardiovascular pathology. Furthermore, OxPLs induce chronic pathological effects, which may be a target for pharmacological neutralization. On the other hand, OxPLs are pharmacological lead structures demonstrating a unique combination of TLR-inhibitory and lung barrier-protective properties. This combination ideally fits for a short-term therapy of systemic inflammation and lung edema. In summary, available data characterize OxPLs as promising structures for further biological analysis and justify their further pharmacological development.
Author contributions
OVO prepared the figures and wrote parts of the manuscript AAB prepared the figures, discussed modifications, edited parts of manuscript. KGB conceived the idea, edited the manuscript and discussed modifications. VNB conceived the idea, wrote the manuscript and discussed modifications.
Funding
This work was supported in part by NIH grants HL076259, HL146829 for KB, HL152761, HL155051 for AB and Pan-European Network in Lipidomics and EpiLipidomics (COST Grant CA19105) funded by the Horizon 2020 Framework Programme of the European Union.
Conflict of interest
The authors declare that the research was conducted in the absence of any commercial or financial relationships that could be construed as a potential conflict of interest.
Publisher’s note
All claims expressed in this article are solely those of the authors and do not necessarily represent those of their affiliated organizations, or those of the publisher, the editors and the reviewers. Any product that may be evaluated in this article, or claim that may be made by its manufacturer, is not guaranteed or endorsed by the publisher.
Abbreviations
AOAH, acyloxyacyl hydrolase; apo(a), apolipoprotein a; apoB-100, apolipoprotein B-100; ARDS, acute respiratory distress syndrome; CAD, coronary artery disease; KOdiA-PC, 1-palmitoyl-2-(5-keto-6-octene-dioyl)-sn-glycero-3-phosphocholine; KDdiA-PC, 1-palmitoyl-2-(4-keto-dodec-3-enadioyl)-sn-glycero-3-phosphocholine; LBP, lipopolysaccharide binding protein; LDL, low density lipoproteins; Lp(a), lipoprotein (a); LPS, bacterial wall lipopolysaccharide; OxLDL, oxidized low-density lipoprotein; OxPAPC, oxidized 1-palmitoyl-2-arachidonoyl-sn-glycero-3-phosphocholine; OxPE, oxidized phosphatidylethanolamine; OxPL, oxidized phospholipid; OxPS, oxidized phosphatidylserine; PAF, platelet-activating factor; PAF-AH, PAF acetylhydrolase; PL, phospholipid; PEIPC, 1-palmitoyl-2-(5′,6′-epoxyisoprostane E2)-sn-glycero-3-phosphocholine; PGPC, 1-palmitoyl-2-(9′-glutaroyl)-sn-glycero-3-phosphocholine; PONPC, 1-palmitoyl-2-(9′-oxononanoyl)-sn-glycero-3-phosphocholine; POVPC, 1-palmitoyl-2-(5′-oxovaleroyl)-sn-glycero-3-phosphocholine; PUFA, polyunsaturated fatty acid; ROS, reactive oxygen species; RNS, reactive nitrogen species; TLR, Toll-like receptor.
References
Ademowo, O. S., Dias, I. H. K., Diaz-Sanchez, L., Sanchez-Aranguren, L., Stahl, W., and Griffiths, H. R. (2020). Partial mitigation of oxidized phospholipid-mediated mitochondrial dysfunction in neuronal cells by oxocarotenoids. J. Alzheimers Dis. 74, 113–126. doi:10.3233/JAD-190923
Ademowo, O. S., Sharma, P., Cockwell, P., Reis, A., Chapple, I. L., Griffiths, H. R., et al. (2020). Distribution of plasma oxidised phosphatidylcholines in chronic kidney disease and periodontitis as a co-morbidity. Free Radic. Biol. Med. 146, 130–138. doi:10.1016/j.freeradbiomed.2019.10.012
Akpinar, S., Oran, M., Dogan, M., Celikkol, A., Erdem, I., and Turgut, B. (2021). The role of oxidized phospholipids in COVID-19-associated hypercoagulopathy. Eur. Rev. Med. Pharmacol. Sci. 25, 5304–5309. doi:10.26355/eurrev_202108_26551
Ambrogini, E., Que, X., Wang, S., Yamaguchi, F., Weinstein, R. S., Tsimikas, S., et al. (2018). Oxidation-specific epitopes restrain bone formation. Nat. Commun. 9, 2193. doi:10.1038/s41467-018-04047-5
Angelova, P. R., Choi, M. L., Berezhnov, A. V., Horrocks, M. H., Hughes, C. D., De, S., et al. (2020). Alpha synuclein aggregation drives ferroptosis: An interplay of iron, calcium and lipid peroxidation. Cell Death Differ. 27, 2781–2796. doi:10.1038/s41418-020-0542-z
Appolonia, C. N., Wolf, K. M., Zawatsky, C. N., and Cinar, R. (2022). Chronic and binge alcohol ingestion increases truncated oxidized phosphatidylcholines in mice lungs due to increased oxidative stress. Front. Physiol. 13, 860449. doi:10.3389/fphys.2022.860449
Artyukhova, M. A., Tyurina, Y. Y., Chu, C. T., Zharikova, T. M., Bayir, H., Kagan, V. E., et al. (2019). Interrogating Parkinson's disease associated redox targets: Potential application of CRISPR editing. Free Radic. Biol. Med. 144, 279–292. doi:10.1016/j.freeradbiomed.2019.06.007
Bagchi, A. K., Surendran, A., Malik, A., Jassal, D. S., Ravandi, A., and Singal, P. K. (2020). IL-10 attenuates OxPCs-mediated lipid metabolic responses in ischemia reperfusion injury. Sci. Rep. 10, 12120. doi:10.1038/s41598-020-68995-z
Bartolini Gritti, B., and Binder, C. J. (2016). Oxidation-specific epitopes are major targets of innate immunity in atherothrombosis. Hamostaseologie 36, 89–96. doi:10.5482/HAMO-14-11-0069
Bayir, H., Tyurin, V. A., Tyurina, Y. Y., Viner, R., Ritov, V., Amoscato, A. A., et al. (2007). Selective early cardiolipin peroxidation after traumatic brain injury: An oxidative lipidomics analysis. Ann. Neurol. 62, 154–169. doi:10.1002/ana.21168
Bergmark, C., Dewan, A., Orsoni, A., Merki, E., Miller, E. R., Shin, M. J., et al. (2008). A novel function of lipoprotein [a] as a preferential carrier of oxidized phospholipids in human plasma. J. Lipid Res. 49, 2230–2239. doi:10.1194/jlr.M800174-JLR200
Binder, C. J., Horkko, S., Dewan, A., Chang, M. K., Kieu, E. P., Goodyear, C. S., et al. (2003). Pneumococcal vaccination decreases atherosclerotic lesion formation: Molecular mimicry between Streptococcus pneumoniae and oxidized LDL. Nat. Med. 9, 736–743. doi:10.1038/nm876
Biswas, S., Zimman, A., Gao, D., Byzova, T. V., and Podrez, E. A. (2017). TLR2 plays a key role in platelet hyperreactivity and accelerated thrombosis associated with hyperlipidemia. Circ. Res. 121, 951–962. doi:10.1161/CIRCRESAHA.117.311069
Bochkov, V., Schoenenberger, A. W., Oskolkova, O., Toth, U., Stockl, J., Majdic, O., et al. (2016). Novel immune assay for quantification of plasma protective capacity against oxidized phospholipids. Biomark. Med. 10, 797–810. doi:10.2217/bmm-2016-0096
Bochkov, V. N., Kadl, A., Huber, J., Gruber, F., Binder, B. R., and Leitinger, N. (2002). Protective role of phospholipid oxidation products in endotoxin-induced tissue damage. Nature 419, 77–81. doi:10.1038/nature01023
Bochkov, V. N., Mechtcheriakova, D., Lucerna, M., Huber, J., Malli, R., Graier, W. F., et al. (2002). Oxidized phospholipids stimulate tissue factor expression in human endothelial cells via activation of ERK/EGR-1 and Ca(++)/NFAT. Blood 99, 199–206. doi:10.1182/blood.v99.1.199
Bochkov, V. N., Oskolkova, O. V., Birukov, K. G., Levonen, A. L., Binder, C. J., and Stockl, J. (2010). Generation and biological activities of oxidized phospholipids. Antioxid. Redox Signal. 12, 1009–1059. doi:10.1089/ars.2009.2597
Byun, Y. S., Lee, J. H., Arsenault, B. J., Yang, X., Bao, W., DeMicco, D., et al. (2015). Relationship of oxidized phospholipids on apolipoprotein B-100 to cardiovascular outcomes in patients treated with intensive versus moderate atorvastatin therapy: The TNT trial. J. Am. Coll. Cardiol. 65, 1286–1295. doi:10.1016/j.jacc.2015.01.050
Byun, Y. S., Yang, X., Bao, W., DeMicco, D., Laskey, R., Witztum, J. L., et al. (2017). Oxidized phospholipids on apolipoprotein B-100 and recurrent ischemic events following stroke or transient ischemic attack. J. Am. Coll. Cardiol. 69, 147–158. doi:10.1016/j.jacc.2016.10.057
Capoulade, R., Chan, K. L., Yeang, C., Mathieu, P., Bosse, Y., Dumesnil, J. G., et al. (2015). Oxidized phospholipids, lipoprotein(a), and progression of calcific aortic valve stenosis. J. Am. Coll. Cardiol. 66, 1236–1246. doi:10.1016/j.jacc.2015.07.020
Chen, Z., Zang, L., Wu, Y., Nakayama, H., Shimada, Y., Shrestha, R., et al. (2018). Lipidomic profiling on oxidized phospholipids in type 2 diabetes mellitus model zebrafish. Anal. Sci. 34, 1201–1208. doi:10.2116/analsci.18P281
Cherepanova, O. A., Srikakulapu, P., Greene, E. S., Chaklader, M., Haskins, R. M., McCanna, M. E., et al. (2020). Novel autoimmune IgM antibody attenuates atherosclerosis in IgM deficient low-fat diet-fed, but not western diet-fed apoe(-/-) mice. Arterioscler. Thromb. Vasc. Biol. 40, 206–219. doi:10.1161/ATVBAHA.119.312771
Dong, Y., D'Mello, C., Pinsky, W., Lozinski, B. M., Kaushik, D. K., Ghorbani, S., et al. (2021). Oxidized phosphatidylcholines found in multiple sclerosis lesions mediate neurodegeneration and are neutralized by microglia. Nat. Neurosci. 24, 489–503. doi:10.1038/s41593-021-00801-z
Erridge, C., Webb, D. J., and Spickett, C. M. (2006). Toll-like receptor 4 signalling is neither sufficient nor required for oxidised phospholipid mediated induction of interleukin-8 expression. Atherosclerosis 193 (1), 77–85. doi:10.1016/j.atherosclerosis.2006.08.032
Espinosa, Y. R., Barrera Valderrama, D. I., Carlevaro, C. M., and Llanos, E. J. (2022). Molecular basis of the anchoring and stabilization of human islet amyloid polypeptide in lipid hydroperoxidized bilayers. Biochim. Biophys. Acta. Gen. Subj. 1866, 130200. doi:10.1016/j.bbagen.2022.130200
Friedmann Angeli, J. P., Schneider, M., Proneth, B., Tyurina, Y. Y., Tyurin, V. A., Hammond, V. J., et al. (2014). Inactivation of the ferroptosis regulator Gpx4 triggers acute renal failure in mice. Nat. Cell Biol. 16, 1180–1191. doi:10.1038/ncb3064
Gao, D., Ashraf, M. Z., Zhang, L., Kar, N., Byzova, T. V., and Podrez, E. A. (2020). Cross-linking modifications of HDL apoproteins by oxidized phospholipids: Structural characterization, in vivo detection, and functional implications. J. Biol. Chem. 295, 1973–1984. doi:10.1074/jbc.RA119.008445
Gargalovic, P. S., Gharavi, N. M., Clark, M. J., Pagnon, J., Yang, W. P., He, A., et al. (2006). The unfolded protein response is an important regulator of inflammatory genes in endothelial cells. Arterioscler. Thromb. Vasc. Biol. 26, 2490–2496. doi:10.1161/01.ATV.0000242903.41158.a1
Getz, G. S., and Reardon, C. A. (2011). Apolipoprotein A-I and A-I mimetic peptides: A role in atherosclerosis. J. Inflamm. Res. 4, 83–92. doi:10.2147/JIR.S12983
Godzien, J., Kalaska, B., Adamska-Patruno, E., Siroka, J., Ciborowski, M., Kretowski, A., et al. (2019). Oxidized glycerophosphatidylcholines in diabetes through non-targeted metabolomics: Their annotation and biological meaning. J. Chromatogr. B Anal. Technol. Biomed. Life Sci. 1120, 62–70. doi:10.1016/j.jchromb.2019.04.053
Gonen, A., Choi, S. H., Miu, P., Agatisa-Boyle, C., Acks, D., Taylor, A. M., et al. (2019). A monoclonal antibody to assess oxidized cholesteryl esters associated with apoAI and apoB-100 lipoproteins in human plasma. J. Lipid Res. 60, 436–445. doi:10.1194/jlr.D090852
Gugiu, B. G., Mouillesseaux, K., Duong, V., Herzog, T., Hekimian, A., Koroniak, L., et al. (2008). Protein targets of oxidized phospholipids in endothelial cells. J. Lipid Res. 49, 510–520. doi:10.1194/jlr.M700264-JLR200
Hajeyah, A. A., Griffiths, W. J., Wang, Y., Finch, A. J., and O'Donnell, V. B. (2020). The biosynthesis of enzymatically oxidized lipids. Front. Endocrinol. 11, 591819. doi:10.3389/fendo.2020.591819
Harrison, K. A., Romer, E., Weyerbacher, J., Ocana, J. A., Sahu, R. P., Murphy, R. C., et al. (2018). Enhanced platelet-activating factor synthesis facilitates acute and delayed effects of ethanol-intoxicated thermal burn injury. J. Invest. Dermatol. 138, 2461–2469. doi:10.1016/j.jid.2018.04.039
Imai, Y., Kuba, K., Neely, G. G., Yaghubian-Malhami, R., Perkmann, T., van Loo, G., et al. (2008). Identification of oxidative stress and Toll-like receptor 4 signaling as a key pathway of acute lung injury. Cell 133, 235–249. doi:10.1016/j.cell.2008.02.043
Jhun, J., Moon, J., Ryu, J., Shin, Y., Lee, S., Cho, K. H., et al. (2020). Liposome/gold hybrid nanoparticle encoded with CoQ10 (LGNP-CoQ10) suppressed rheumatoid arthritis via STAT3/Th17 targeting. PLoS One 15, e0241080. doi:10.1371/journal.pone.0241080
Kagan, V. E., Mao, G., Qu, F., Angeli, J. P., Doll, S., Croix, C. S., et al. (2017). Oxidized arachidonic and adrenic PEs navigate cells to ferroptosis. Nat. Chem. Biol. 13, 81–90. doi:10.1038/nchembio.2238
Karki, P., and Birukov, K. G. (2021). Oxidized phospholipids in control of endothelial barrier function: Mechanisms and implication in lung injury. Front. Endocrinol. 12, 794437. doi:10.3389/fendo.2021.794437
Karki, P., and Birukov, K. G. (2020). Oxidized phospholipids in healthy and diseased lung endothelium. Cells 9, E981. doi:10.3390/cells9040981
Ke, Y., Karki, P., Kim, J., Son, S., Berdyshev, E., Bochkov, V. N., et al. (2019). Elevated truncated oxidized phospholipids as a factor exacerbating ALI in the aging lungs. FASEB J. 33, 3887–3900. doi:10.1096/fj.201800981R
Latchoumycandane, C., Nagy, L. E., and McIntyre, T. M. (2014). Chronic ethanol ingestion induces oxidative kidney injury through taurine-inhibitable inflammation. Free Radic. Biol. Med. 69, 403–416. doi:10.1016/j.freeradbiomed.2014.01.001
Leibundgut, G., Scipione, C., Yin, H., Schneider, M., Boffa, M. B., Green, S., et al. (2013). Determinants of binding of oxidized phospholipids on apolipoprotein (a) and lipoprotein (a). J. Lipid Res. 54, 2815–2830. doi:10.1194/jlr.M040733
Li, W., Terada, Y., Tyurina, Y. Y., Tyurin, V. A., Bery, A. I., Gauthier, J. M., et al. (2022). Necroptosis triggers spatially restricted neutrophil-mediated vascular damage during lung ischemia reperfusion injury. Proc. Natl. Acad. Sci. U. S. A. 119, e2111537119. doi:10.1073/pnas.2111537119
Liu, J., Li, W., Chen, R., and McIntyre, T. M. (2013). Circulating biologically active oxidized phospholipids show on-going and increased oxidative stress in older male mice. Redox Biol. 1, 110–114. doi:10.1016/j.redox.2012.11.011
Lubec, J., Smidak, R., Malikovic, J., Feyissa, D. D., Korz, V., Hoger, H., et al. (2019). Dentate gyrus peroxiredoxin 6 levels discriminate aged unimpaired from impaired rats in a spatial memory task. Front. Aging Neurosci. 11, 198. doi:10.3389/fnagi.2019.00198
Ma, X. H., Liu, J. H., Liu, C. Y., Sun, W. Y., Duan, W. J., Wang, G., et al. (2022). ALOX15-launched PUFA-phospholipids peroxidation increases the susceptibility of ferroptosis in ischemia-induced myocardial damage. Signal Transduct. Target. Ther. 7, 288. doi:10.1038/s41392-022-01090-z
Mahalka, A. K., Maury, C. P., and Kinnunen, P. K. (2011). 1-Palmitoyl-2-(9'-oxononanoyl)-sn-glycero-3-phosphocholine, an oxidized phospholipid, accelerates Finnish type familial gelsolin amyloidosis in vitro. Biochemistry 50, 4877–4889. doi:10.1021/bi200195s
Matt, U., Sharif, O., Martins, R., and Knapp, S. (2015). Accumulating evidence for a role of oxidized phospholipids in infectious diseases. Cell. Mol. Life Sci. 72, 1059–1071. doi:10.1007/s00018-014-1780-3
McIntyre, T. M. (2012). Bioactive oxidatively truncated phospholipids in inflammation and apoptosis: Formation, targets, and inactivation. Biochim. Biophys. Acta 1818, 2456–2464. doi:10.1016/j.bbamem.2012.03.004
Mohammadi, M., Oehler, B., Kloka, J., Martin, C., Brack, A., Blum, R., et al. (2018). Antinociception by the anti-oxidized phospholipid antibody E06. Br. J. Pharmacol. 175, 2940–2955. doi:10.1111/bph.14340
Moriyama, H., Endo, J., Kataoka, M., Shimanaka, Y., Kono, N., Sugiura, Y., et al. (2022). Omega-3 fatty acid epoxides produced by PAF-AH2 in mast cells regulate pulmonary vascular remodeling. Nat. Commun. 13, 3013. doi:10.1038/s41467-022-30621-z
Narzt, M. S., Kremslehner, C., Golabi, B., Nagelreiter, I. M., Malikovic, J., Hussein, A. M., et al. (2022). Molecular species of oxidized phospholipids in brain differentiate between learning- and memory impaired and unimpaired aged rats. Amino Acids 54, 1311–1326. doi:10.1007/s00726-022-03183-z
Nonas, S., Birukova, A. A., Fu, P., Xing, J., Chatchavalvanich, S., Bochkov, V. N., et al. (2008). Oxidized phospholipids reduce ventilator-induced vascular leak and inflammation in vivo. Crit. Care 12, R27. doi:10.1186/cc6805
Oehler, B., Kistner, K., Martin, C., Schiller, J., Mayer, R., Mohammadi, M., et al. (2017). Inflammatory pain control by blocking oxidized phospholipid-mediated TRP channel activation. Sci. Rep. 7, 5447. doi:10.1038/s41598-017-05348-3
Oskolkova, O. V., Hodzic, A., Karki, P., Gesslbauer, B., Ke, Y., Hofer, D. C., et al. (2021). Oxidized phospholipids on alkyl-amide scaffold demonstrate anti-endotoxin and endothelial barrier-protective properties. Free Radic. Biol. Med. 174, 264–271. doi:10.1016/j.freeradbiomed.2021.07.041
Pairojana, T., Phasuk, S., Suresh, P., and Liu, I. Y. (2022). Behavioral and synaptic phenotypes of female Prdx6(-/-) mice. Antioxidants (Basel) 11, 1201. doi:10.3390/antiox11061201
Palmieri, M., Almeida, M., Nookaew, I., Gomez-Acevedo, H., Joseph, T. E., Que, X., et al. (2021). Neutralization of oxidized phospholipids attenuates age-associated bone loss in mice. Aging Cell 20, e13442. doi:10.1111/acel.13442
Philippova, M., Oskolkova, O. V., Bicker, W., Schoenenberger, A. W., Resink, T. J., Erne, P., et al. (2019). Analysis of fragmented oxidized phosphatidylcholines in human plasma using mass spectrometry: Comparison with immune assays. Free Radic. Biol. Med. 144, 167–175. doi:10.1016/j.freeradbiomed.2019.05.027
Podrez, E. A., Byzova, T. V., Febbraio, M., Salomon, R. G., Ma, Y., Valiyaveettil, M., et al. (2007). Platelet CD36 links hyperlipidemia, oxidant stress and a prothrombotic phenotype. Nat. Med. 13, 1086–1095. doi:10.1038/nm1626
Podrez, E. A., Poliakov, E., Shen, Z., Zhang, R., Deng, Y., Sun, M., et al. (2002). Identification of a novel family of oxidized phospholipids that serve as ligands for the macrophage scavenger receptor CD36. J. Biol. Chem. 277, 38503–38516. doi:10.1074/jbc.M203318200
Qin, J., Goswami, R., Balabanov, R., and Dawson, G. (2007). Oxidized phosphatidylcholine is a marker for neuroinflammation in multiple sclerosis brain. J. Neurosci. Res. 85, 977–984. doi:10.1002/jnr.21206
Que, X., Hung, M. Y., Yeang, C., Gonen, A., Prohaska, T. A., Sun, X., et al. (2018). Oxidized phospholipids are proinflammatory and proatherogenic in hypercholesterolaemic mice. Nature 558, 301–306. doi:10.1038/s41586-018-0198-8
Sahu, R. P., Ferracini, M., and Travers, J. B. (2015). Systemic chemotherapy is modulated by platelet-activating factor-receptor agonists. Mediat. Inflamm. 2015, 820543. doi:10.1155/2015/820543
Sahu, R. P., Harrison, K. A., Weyerbacher, J., Murphy, R. C., Konger, R. L., Garrett, J. E., et al. (2016). Radiation therapy generates platelet-activating factor agonists. Oncotarget 7, 20788–20800. doi:10.18632/oncotarget.7878
Sahu, R. P., Turner, M. J., DaSilva, S. C., Rashid, B. M., Ocana, J. A., Perkins, S. M., et al. (2012). The environmental stressor ultraviolet B radiation inhibits murine antitumor immunity through its ability to generate platelet-activating factor agonists. Carcinogenesis 33, 1360–1367. doi:10.1093/carcin/bgs152
Scipione, C. A., Sayegh, S. E., Romagnuolo, R., Tsimikas, S., Marcovina, S. M., Boffa, M. B., et al. (2015). Mechanistic insights into Lp(a)-induced IL-8 expression: A role for oxidized phospholipid modification of apo(a). J. Lipid Res. 56, 2273–2285. doi:10.1194/jlr.M060210
Seok, J. K., Hong, E. H., Yang, G., Lee, H. E., Kim, S. E., Liu, K. H., et al. (2021). Oxidized phospholipids in tumor microenvironment stimulate tumor metastasis via regulation of autophagy. Cells 10, 558. doi:10.3390/cells10030558
Shimanaka, Y., Kono, N., Taketomi, Y., Arita, M., Okayama, Y., Tanaka, Y., et al. (2017). Omega-3 fatty acid epoxides are autocrine mediators that control the magnitude of IgE-mediated mast cell activation. Nat. Med. 23, 1287–1297. doi:10.1038/nm.4417
Skouta, R., Dixon, S. J., Wang, J., Dunn, D. E., Orman, M., Shimada, K., et al. (2014). Ferrostatins inhibit oxidative lipid damage and cell death in diverse disease models. J. Am. Chem. Soc. 136, 4551–4556. doi:10.1021/ja411006a
Solati, Z., Surendran, A., Edel, A., Roznik, M., Allen, D., and Ravandi, A. (2021). Increase in plasma oxidized phosphatidylcholines (OxPCs) in patients presenting with ST-elevation myocardial infarction (STEMI). Front. Med. 8, 716944. doi:10.3389/fmed.2021.716944
Spickett, C. M. (2020). Formation of oxidatively modified lipids as the basis for a cellular epilipidome. Front. Endocrinol. 11, 602771. doi:10.3389/fendo.2020.602771
Stamenkovic, A., O'Hara, K. A., Nelson, D. C., Maddaford, T. G., Edel, A. L., Maddaford, G., et al. (2021). Oxidized phosphatidylcholines trigger ferroptosis in cardiomyocytes during ischemia-reperfusion injury. Am. J. Physiol. Heart Circ. Physiol. 320, H1170–H1184. doi:10.1152/ajpheart.00237.2020
Sun, X., Seidman, J. S., Zhao, P., Troutman, T. D., Spann, N. J., Que, X., et al. (2020). Neutralization of oxidized phospholipids ameliorates non-alcoholic steatohepatitis. Cell Metab. 31, 189–206. doi:10.1016/j.cmet.2019.10.014
Taleb, A., Witztum, J. L., and Tsimikas, S. (2011). Oxidized phospholipids on apoB-100-containing lipoproteins: A biomarker predicting cardiovascular disease and cardiovascular events. Biomark. Med. 5, 673–694. doi:10.2217/bmm.11.60
Tsimikas, S., Brilakis, E. S., Miller, E. R., McConnell, J. P., Lennon, R. J., Kornman, K. S., et al. (2005). Oxidized phospholipids, Lp(a) lipoprotein, and coronary artery disease. N. Engl. J. Med. 353, 46–57. doi:10.1056/NEJMoa043175
Tsimikas, S., Miyanohara, A., Hartvigsen, K., Merki, E., Shaw, P. X., Chou, M. Y., et al. (2011). Human oxidation-specific antibodies reduce foam cell formation and atherosclerosis progression. J. Am. Coll. Cardiol. 58, 1715–1727. doi:10.1016/j.jacc.2011.07.017
Tsimikas, S., Moriarty, P. M., and Stroes, E. S. (2021). Emerging RNA therapeutics to lower blood levels of Lp(a): JACC focus seminar 2/4. J. Am. Coll. Cardiol. 77, 1576–1589. doi:10.1016/j.jacc.2021.01.051
Upchurch, C. M., Yeudall, S., Pavelec, C. M., Merk, D., Greulich, J., Manjegowda, M., et al. (2022). Targeting oxidized phospholipids by AAV-based gene therapy in mice with established hepatic steatosis prevents progression to fibrosis. Sci. Adv. 8, eabn0050. doi:10.1126/sciadv.abn0050
Van Lenten, B. J., Wagner, A. C., Jung, C. L., Ruchala, P., Waring, A. J., Lehrer, R. I., et al. (2008). Anti-inflammatory apoA-I-mimetic peptides bind oxidized lipids with much higher affinity than human apoA-I. J. Lipid Res. 49, 2302–2311. doi:10.1194/jlr.M800075-JLR200
Vats, K., Kruglov, O., Mizes, A., Samovich, S. N., Amoscato, A. A., Tyurin, V. A., et al. (2021). Keratinocyte death by ferroptosis initiates skin inflammation after UVB exposure. Redox Biol. 47, 102143. doi:10.1016/j.redox.2021.102143
Wenzel, S. E., Tyurina, Y. Y., Zhao, J., St Croix, C. M., Dar, H. H., Mao, G., et al. (2017). PEBP1 wardens ferroptosis by enabling lipoxygenase generation of lipid death signals. Cell 171, 628–641. doi:10.1016/j.cell.2017.09.044
Yang, L., Latchoumycandane, C., McMullen, M. R., Pratt, B. T., Zhang, R., Papouchado, B. G., et al. (2010). Chronic alcohol exposure increases circulating bioactive oxidized phospholipids. J. Biol. Chem. 285, 22211–22220. doi:10.1074/jbc.M110.119982
Yeang, C., Hasanally, D., Que, X., Hung, M. Y., Stamenkovic, A., Chan, D., et al. (2019). Reduction of myocardial ischaemia-reperfusion injury by inactivating oxidized phospholipids. Cardiovasc. Res. 115, 179–189. doi:10.1093/cvr/cvy136
Zhivaki, D., and Kagan, J. C. (2022). Innate immune detection of lipid oxidation as a threat assessment strategy. Nat. Rev. Immunol. 22, 322–330. doi:10.1038/s41577-021-00618-8
Zimman, A., and Podrez, E. A. (2010). Regulation of platelet function by class B scavenger receptors in hyperlipidemia. Arterioscler. Thromb. Vasc. Biol. 30, 2350–2356. doi:10.1161/ATVBAHA.110.207498
Keywords: endothelium, permeability, lung injury, inflammation, toll-like receptor inhibition, endothelial cell barrier protection, alkyl-amide oxidized phospholipids
Citation: Oskolkova OV, Birukova AA, Birukov KG and Bochkov VN (2022) Oxidized phospholipids are biomarkers, drug targets, and drug leads. Front. Drug. Discov. 2:1043708. doi: 10.3389/fddsv.2022.1043708
Received: 13 September 2022; Accepted: 19 October 2022;
Published: 03 November 2022.
Edited by:
Xin Wang, The University of Manchester, United KingdomReviewed by:
Aaron Zefrin Fernandis, Merck Sharp & Dohme Corp,SingaporeCopyright © 2022 Oskolkova, Birukova, Birukov and Bochkov. This is an open-access article distributed under the terms of the Creative Commons Attribution License (CC BY). The use, distribution or reproduction in other forums is permitted, provided the original author(s) and the copyright owner(s) are credited and that the original publication in this journal is cited, in accordance with accepted academic practice. No use, distribution or reproduction is permitted which does not comply with these terms.
*Correspondence: Konstantin G. Birukov, a2JpcnVrb3ZAc29tLnVtYXJ5bGFuZC5lZHU=; Valery N. Bochkov, dmFsZXJ5LmJvY2hrb3ZAdW5pLWdyYXouYXQ=