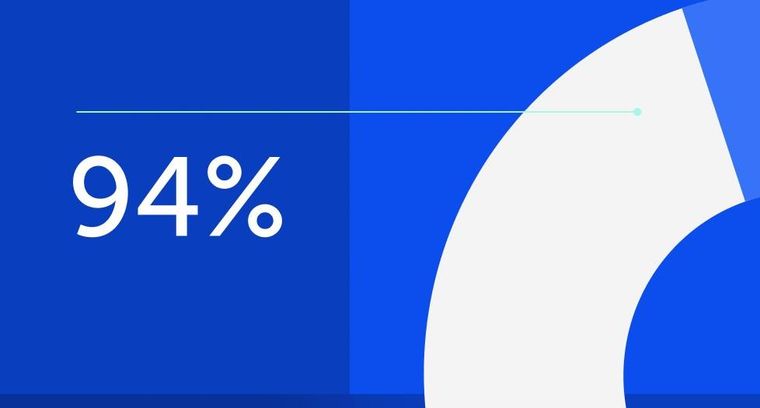
94% of researchers rate our articles as excellent or good
Learn more about the work of our research integrity team to safeguard the quality of each article we publish.
Find out more
REVIEW article
Front. Drug Discov., 25 January 2022
Sec. In silico Methods and Artificial Intelligence for Drug Discovery
Volume 1 - 2021 | https://doi.org/10.3389/fddsv.2021.789710
This article is part of the Research TopicDevelopment of COVID-19 Therapies: Lessons Learnt and Ongoing EffortsView all 13 articles
Covid-19 is particularly mild with children, and its severity escalates with age. Several theories have been proposed to explain these facts. In particular, it was proposed that the lower expression of the viral receptor ACE2 in children protects them from severe Covid-19. However, other works suggested an inverse relationship between ACE2 expression and disease severity. Here we review the seemingly contradicting observations on ACE2 expression at the levels of mRNA, membrane protein and serum protein in humans and rodents and try to reconcile them at the light of the Renin-Angiotensin system (RAS) and bradykinin system, which constitute an integrated inflammatory system connected by common peptidases and interacting receptors. We find that ACE2 level is not monotonically related with age but it reaches a maximum at a young age that depends on the cell type and then decreases, consistently with almost all existing data. The increase with age of the protease Tumor necrosis factor alpha (TNF-α) converting enzyme (TACE), also known as ADAM17 (a disintegrin and metalloproteinase 17) that sheds ACE2 from the cell membrane to the serum predicts that the decrease occurs before and is steeper for ACE2 cell protein than for its mRNA. This negative relation between ACE2 level and Covid-19 severity at old age is not paradoxical but it is consistent with a mathematical model that predicts that higher viral receptor does not necessarily favour virus propagation and it can even slow it down. More importantly, the angiotensin-bradykinin system is characterized by a powerful positive feedback loop that enhances inflammation through the Angiotensin and Bradykinin receptors that upregulate ADAM17, which in turn downregulates ACE2 and upregulates TNF-α and the pro-inflammatory receptor of the cytokine interleukin 6 (IL6). Here we propose that ACE2 contributes essentially to reverse this inflammatory state by downregulating the pro-inflammatory peptides of the angiotensin-bradykinin system, and that failure to do this, possibly induced by the degradation of ACE2 by SARS-COV-2, may underlie both severe CoViD-19 infection and its many post-infection manifestations, including the multi-inflammatory syndrome of children (MIS-C). Within this view, lower severity in children despite lower ACE2 expression may be consistent with their higher expression of the alternative angiotensin II receptor ATR2 and in general of the anti-inflammatory arm of the RAS at young age.
The Covid-19 pandemics (Zhou et al., 2020) has overcome four million confirmed deaths (Dong et al., 2020), and excess death indicates that the real number may be substantially higher. However, in contrast with the high mortality of the elderlies, children predominantly develop a mild form of Covid-19 and their mortality is very low, as part of a general trend of increasing Covid-19 severity with age. Age-stratified mortality rates with respect to detected cases increase very steeply with age, and even more steeply if we consider that most infections are asymptomatic at young age and they tend to go undetected. Figure 1 shows the approximately exponential increase with age of detected cases, hospitalizations, ICU and deaths with respect to the number of seropositives detected in population-wide antibody surveys in Spain at May 10, 2020. One can see that the increase with age is apparent at all levels of severity, except for a drop of ICU at high age that unfortunately is most likely explained by the saturation of the health system during the first pandemic wave.
FIGURE 1. Covid-19 severity increases exponentially with age. Black: Cases detected through positive PCR. Red: PCR-positive patients that required hospitalization. Green: PCR-positive patients in ICU. The drop at high age probably reflects the saturation of the health system during the first wave of the pandemics. Blue: Deceased patients with positive PCR (the real toll estimated with excess deaths is unfortunately much higher). The thin black line reports the fraction of seropositive for each age class, which is used as the normalization for the fractions reported in the figures. Panels (A) and (B) refer to female and male patients, respectively. Data are from Spain but they are qualitatively similar in other countries.
Several theories have been proposed to explain why Covid-19 is so mild with children, recently reviewed by Zimmerman and Curtis (Zimmerman and Curtis, 2020). One group of theories postulates different immune response in children. Cristiani et al. (Cristiani et al., 2020) invoke the strength of the innate immunity of children, which is further reinforced by the frequent infections and the vaccines that they are exposed to, which enhance their trained immunity. However, although the immune response to SARS-CoV-2 of children and adults is markedly different, Pierce et al. argued that the greater severity of hospitalized adults compared to children could not be attributed to their less efficient immune responses (Pierce et al., 2020). A second group of theories is based on factors that put aged adults at increased risk, including co-morbidities and, above all, differences in the endothelial system. The uncompromised state of the endothelial system of children may protect them from the most severe complications of Covid-19 that originate from endothelial inflammation and dysfunction, as reviewed in a Nature news article (Cyranoski, 2020). However, the theory that captured most attention is probably the one based on the variation of the viral receptor ACE2 with age. It was recently observed that ACE2 mRNA and serum protein is lower in children than in adults (Bunyavanich et al., 2020; Muus et al., 2020; Saheb Sharif-Askari et al., 2020; Pavel et al., 2021), and it was proposed that lower receptor levels protect children from severe SARS-CoV-2 infection. Nevertheless, data on ACE2 expression across age are contradictory. Some works found that ACE2 protein in cells is lower in children than in adults (Inde et al., 2020) and others reached the opposite conclusion (Ortiz et al., 2020; Zhang et al., 2021).
Here we go beyond the dichotomy between children and adults and propose an alternative interpretation of the data, supported by observations that indicate that ACE2 mRNA and protein expression are not monotonic with age. ACE2 mRNA starts from zero during foetal life and reaches a maximum at young age, either adolescence or twenties depending on the examined organ (Inde et al., 2020; Muus et al., 2020), after which they decay with age (Chen et al., 2020), as also observed in mice (Booeshaghi and Pachter, 2020). At the protein level, decay of ACE2 was observed in adult rats and mice (Xie et al., 2006; Yoon et al., 2016) and humans (Zhang et al., 2021), with strong inter-individual variation and cell-type dependent maximum age (Inde et al., 2020; Ortiz et al., 2020).
ACE2 cell protein level behaves differently from ACE2 mRNA, due to the shedding of ACE2 from the cell membrane to the serum produced by the metalloprotease ADAM17 (Lambert et al., 2005) (a disintegrin and metalloproteinase 17), also known as Tumor necrosis factor alpha (TNF-α) Converting enzyme (TACE) since it activates this crucial cytokine. The expression of ADAM17 increases through age (Dou et al., 2017; Liu et al., 2019), which predicts increasing ACE2 shedding and implies that the maximum expression is achieved at earlier age for ACE2 protein than for ACE2 mRNA (see below).
Whereas the comparison between children and adults supports a positive correlation between ACE2 level and disease severity, the comparison between young and old adults supports their negative correlation. That lower receptor level is not necessarily a protective factor is predicted by a mathematical model according to which, in some circumstances, viruses propagate in the infected organism more slowly with higher receptor level (Ortega-Cejas et al., 2004) (see section Effect of Receptor Level on Viral Propagation). Most importantly, low ACE2 levels expose the lungs to acute inflammation (Imai et al., 2005), and ACE2 is low in most common chronic pathologies including hypertension, angiocardiopathy, type 2 diabetes, chronic renal failure, pulmonary diseases and liver diseases (Li et al., 2020a; Pagliaro and Penna, 2020). For these reasons, several authors proposed that the severity of Covid-19 is exacerbated by the degradation of ACE2 by the virus (Annweiler et al., 2020; Sun et al., 2020a; Ciaglia et al., 2020; Gurwitz, 2020; Offringa et al., 2020; Verdecchia et al., 2020).
In this paper, we propose the following hypotheses.
1. One of the main functions of ACE2 consists in contributing to reverse the inflammatory state produced by the peptides of the Angiotensin and Bradykinin system and their receptors (we advocate for considering it an integrated system).
2. The activity of this molecular system increases through age at multiple levels, including chromatin remodelling, gene regulation and protein degradation. This hypothesis is highly consistent with the “inflammaging” theory. Key molecular events that contribute to this trend are the decrease of ACE2 at old age, the decrease through age of the alternative Angiotensin receptor ATR2 that is highly expressed in children, and the increase through age of the protease ADAM17/TACE that degrades ACE2 and activates TNF-α. We illustrate how the current data on ACE2 expression are consistent with this hypothesis.
3. The angiotensin-bradykinin system is characterized by a powerful positive feedback loop that amplifies the inflammation by downregulating its negative regulator ACE2 both at the transcription level and at the protein level, the latter through the protease ADAM17/TACE. At the same time, ADAM17 activates inflammatory cytokines such as TNF-α and Interleukin 6 (IL6).
4. The degradation of ACE2 by SARS-CoV-2 may strengthen the positive feedback loop described above. We propose that this is one of the main triggers of the acute inflammation observed in severe Covid-19.
5. The differential activity of the inflammatory system through age and sex may provide an explanation for the extreme differences in severity between groups of Covid-19 patients.
Figure 2 illustrates graphically the pathways of the angiotensin/bradykinin peptides, their inflammatory receptors ATR1, BKR1 and BKR2, their downregulator ACE2 and the negative effect that SARS-Cov-2 has on it.
FIGURE 2. The figure illustrates the positive feedback loop of inflammation, namely: The inflammatrory peptides AngII(1–8), BK(1–8) and LysBK(0–8) signal through the inflammatory receptors ATR1, BKR1 and BKR2, which in turn downregulate the negative regulator ACE2. Our hypotheses are the following: (A) One of the main functions of ACE2 consists in terminating the inflammatory state produced by the peptides of the Angiotensin and Bradykinin system and their receptors. (B) The activity of this inflammatory system increases through age at multiple levels, including chromatin remodelling, gene regulation and protein degradation, and this has the effect of downregulating ACE2. (C) The degradation of ACE2 by SARS-CoV-2 strenghens the positive feedback loop of inflammation, enhancing the acute inflammation observed in severe Covid-19. (D) The differential activity of the inflammatory system through age and sex may provide an explanation for the extreme differences in severity between groups of Covid-19 patients.
The Angiotensin converting enzyme 2 (ACE2), the receptor of SARS, SARS-CoV-2 and other coronaviruses, plays a regulatory role in the RAS and in the Kallikrenin-kinin system (KKS), whose signalling peptides are the family of angiotensin for the RAS and bradykinin for the KKS. These two systems are strongly coupled, since they share some of their main functions (control of blood pressure, blood coagulation, control of inflammatory processes, regulation of the immune system response after infection or traumatic events) and two key enzymes, the carboxy-peptidases ACE and ACE2 that regulate the signal peptides of both systems. Their receptors, which belong to the large family of G-protein coupled receptors (GPCR), may act in a synergistic manner, forming complexes that mutually enhance each other signalling through allosteric interactions (Rukavina Mikusic et al., 2020). Thus, it can be considered that RAS and KKS form an integrated system.
In the RAS, ACE produces the pro-inflammatory and vasoconstrictor peptide Angiotensin II (Ang-II, which we also denote as Ang1-8 since it consists of the first 8 amino acids of the peptide Angiotensin I or Ang1-10), while ACE2 downregulates it, transforming it into the form Ang1-7 that mediates vasodilator and anti-inflammatory effects through the Mas receptor (a GPCR that forms complexes with others of the same system). Moreover, ACE2 transforms Ang1-10, the precursor of Ang1-8, into the form Ang1-9 that is later transformed into Ang1-7 by ACE.
Ang II signals through the pro-inflammatory receptor ATR1, whose action is counteracted by the alternative receptor of AngII, ATR2, which has anti-inflammatory effects. Therefore, the functioning of the RAS arises from a fine dynamical balance between ACE and ACE2 at the level of the production of the signalling peptides, and between ATR1 and ATR2 at the level of the transmission of the signal.
In the KKS, ACE2 degrades Des-Arg-Bradykinin and Lys-Des-Arg-Bradykinin (BK1-8 and LysBK0-8), two peptides that signal through the receptor BKR1 that is expressed upon inflammation, and ACE degrades BK1-9 and LysBK0-9, so that the action of ACE in the KKS is anti-inflammatory, in contrast with its action in the RAS, while it increases blood pressure in both systems. Bradykinin signalling produces vasodilation, decreases blood pressure, increases vascular permeability and induces capillary leakage. These actions can produce the edemas suffered by severe Covid-19 patients. Moreover, bradykinin enhances the inflammation locally by recruiting neutrophils and leukocytes.
The involvement of the KKS in Covid-19 cases has been discussed in recent publications (Garvin et al., 2020; Nicolau et al., 2020; van de Veerdonk et al., 2020). It is consistent with the observation that a fraction of Covid-19 patients present hypotension: 66% of critical patients (Michard and Vieillard-Baron, 2020), and 8% of hospitalized patients compared with 39% with hypertension (Lala et al., 2020).
Both bradykinin and Angiotensin II upregulate the protease ADAM17/TACE that removes ACE2 from the cell membrane, activates TNF-α and transforms the receptor of the cytokine IL6 to a soluble form that enhances inflammation (Matthews et al., 2003; Rose-John, 2012). These processes cause a synergistic positive feedback loop that amplifies inflammation.
We represent in Figure 3 the reduced molecular network of the angiotensin/bradykinin system. The figure represents different peptide species, their transformations through the peptidases ACE and ACE2, the anti-inflammatory receptors ATR2 and Mas, and the metalloprotease ADAM17/TACE.
FIGURE 3. Simplified representation of the Renin-Angiotensin system and the Kallikrein-Bradykinin system, coupled through the peptidases ACE and ACE2 that act on both families of peptides and through the allosteric interactions between the G-protein coupled receptors of both families. Peptides of the angiotensin (Ang) and bradykinin (BK) family are represented as rectangles, coloured arrows indicate their transformations and circles indicate the peptidases that catalyse them (black arrows). Membrane receptors are indicated as triangles and the boxes indicate the main effects of their signalling. The colour code represents inflammatory effects (orange: pro-inflammatory; green: anti-inflammatory, blue: Neutral or not clear at present). Also represented as ellipses are Vitamin D, which downregulates Renin and the down-stream effectors NFkB and NOX, and Interferons α and β, both of which upregulate ACE2 with anti-inflammatory effects, and γ, which downregulates ACE2.
Furthermore, ACE2 has been recently shown through evolutionary rate correlation analysis to possess strong biological interactions with proteins in the coagulation pathway such as Clusterin and Fibrinogen alpha, beta and gamma chains and with proteins involved in cytokine signaling such as X-C Motif Chemokine Receptor 1 (XCR1), Interferon alpha/beta receptor 2 (IFNAR2) and toll-like receptor 8 (TLR8) (Varela et al., 2021).
ACE2 counteracts high blood pressure in the RAS and low blood pressure in the KKS, but it has anti-inflammatory effects in both systems. Interestingly, several studies have shown that ACE2 knockout mice do not present significant hypertension or cardiac anomalies, but they show enhanced response to Ang-II stimulation (Alghamri et al., 2013) and they develop lung edema after acute-inflammation (Imai et al., 2005). Edema is not the mechanical consequence of increased blood pressure, which does not happen in ACE2 KO mice, but it may derive from increased vascular permeability due to high bradykinin levels (van de Veerdonk et al., 2020). This is supported by the observation that attenuation of pulmonary ACE2 activity impairs inactivation of the DABK/BK1R axis and facilitates neutrophil infiltration (Sodhi et al., 2018). These observations connect ACE2 with the termination of the inflammatory process, as further discussed below.
A crucial link between ACE2 and the inflammatory process goes through ADAM17. This metallo-protease sheds ACE2 from the cellular membrane to the serum (Lambert et al., 2005), from which it is rapidly eliminated through urine, and it is triggered by Angiotensin II via AT1Rs (Deshotels et al., 2014; Xu et al., 2017). In addition, bradykinin triggers BKR1s, which also upregulate ADAM17 (Parekh and Sriramula, 2020), establishing a synergistic mechanism that enhances ACE2 degradation. At the same time, ADAM17 activates the cytokine TNF-α and it transforms the receptor of the cytokine IL6 into its soluble form that is pro-inflammatory (Matthews et al., 2003; Rose-John, 2012), initiating a cascade process that, through the transcription factor nuclear factor kB (NFkB), promotes the activation of the inflammatory response.
Here we summarize the main steps with which the RAS and the KKS participate in the inflammation process.
1. The infection activates the RAS, enhancing Ang1-8 production
2. High Ang-II/ATR1 generates a positive feedback by upregulating the expression of ACE, downregulating ACE2 (Koka et al., 2008), and upregulating ADAM17/TACE. This positive feedback loop leads to lower ACE2 and higher local Ang-II production.
3. At the same time, Ang-II/ATR1 enhances the inflammation:
• Ang-II/ATR1 activates the KKS, which induces vasodilation and possible drop of blood pressure, increased capillary leakage and recruitment of neutrophils.
• The Ang-II/ATR1 axis has different effects on the two receptors of the KKS, BKR1 and BKR2. Through IL1 and TNF-α, ATR1 upregulates the BKR1 receptor of DABK, whose level increase through the decrease of ACE2, activating the DABK/BKR1 axis. BKR1 contributes to upregulating ADAM17, producing another positive feedback loop.
• At the same time, the other bradykinin receptor BKR2 is sensitized by ATR1, with which it forms a dimer (Rukavina Mikusic et al., 2020). However, increased ACE downregulates the ligand of BKR2, so the effect on BKR2 signalling is unclear.
• Ang-II/ATR1 upregulates the Vascular Endothelial Growth Factor (VEGF) through TGF-beta and Angiopoietin-2, thus amplifying capillary leakage. At the same time, VEGF may be also activated by BKR2.
• Ang-II/ATR1 and DABK/BKR1 recruit macrophages and neutrophils to the infection.
4. At some point, ACE2 is upregulated and it contributes to reversing the inflammation in a cascade process: ACE2 degrades Ang-II and Ang1-10, downregulating the Ang-II/ATR1 axis, produces Ang1-7, upregulating the anti-inflammatory Ang1-7/Mas, and degrades DABK, diminishing vascular leakage. Downregulation of ATR1 de-sensitives the BKR2 receptor and reduces the level of the BKR1 receptor (whose ligand is also decreased), and, through them, ADAM17-TACE, which in turn decreases TNF-α and the pro-inflammatory IL6 soluble receptor. Moreover, the lower activity of ATR1 reduces ACE, further reducing AngII, and reduces the VEGF.
Consistent with this view, ACE2 is upregulated during inflammation (Hanafy et al., 2011), in part through interferon I stimulation (Ziegler et al., 2020). ACE2 upregulation is also observed in a recent analysis of mRNA expression of bronchoalveolar lavage fluid cells (Garvin et al., 2020) and lung cells (Wu et al., 2020) of Covid-19 patients. However, the ACE2/ACE ratio is decreased in most chronic inflammatory diseases (Li et al., 2020a; Pagliaro and Penna, 2020). As discussed below, we hypothesize that epigenetic mechanisms have a role in the discrepancy between short term activation and chronic reduction of ACE2.
As discussed above, ACE2 plays a key role in reverting the inflammatory process in the context of the RAS and the KKS, and its downregulation favour endothelial damage, capillary leakage and angioedema, as shown by Imai et al. and Kuba et al. among others (Imai et al., 2005; Kuba et al., 2005). It has been shown that SARS-CoV (Haga et al., 2008) and SARS-CoV-2 degrade ACE2. We hypothesize that the degradation of ACE2, especially in presence of low initial levels, or the failure to upregulate ACE2 may prevent the termination of the inflammatory process and perpetuate the propagation of inflammation through the affected organs, leading to organ damage and severe manifestations that can be life threatening.
In a transcriptomic study of lung tissue obtained from patients who died of Covid-19 in China it was found that the viral load was low in all samples. According to the authors, this “suggests that the patient deaths may be related to the host response rather than an active fulminant infection” (Wu et al., 2020).
A retrospective study of almost 48,000 Covid-19 patients discharged from English hospitals (and, accordingly, negative to the virus in their large majority) found rates of hospital readmission and death 3.5 and 7.7 times greater, respectively, than in age- and comorbidities-matched controls, and higher rates of respiratory, diabetes and cardiovascular events, evidencing elevated rates of multi-organ dysfunction in individuals discharged from hospital (Ayoubkhani et al., 2021). These observations indicate that many severe consequences of Covid-19 arise from the immune-inflammatory response rather than being a direct consequence of SARS-COV-2 infection.
A key role in the activation of the inflammatory process is played by ADAM17/TACE, which eliminates ACE2 from the cell membranes preventing its anti-inflammatory function (Lambert et al., 2005), it transforms the pro-inflammatory cytokine TNF-α into the active form and it transforms the receptor of the cytokine IL6 to its soluble form, which is pro-inflammatory, contrasting the anti-inflammatory effect of the membrane bound IL6R (Matthews et al., 2003; Rose-John, 2012).
A recent study on a cohort of 102 Covid-19 patients from Mexico found that ADAM17 is increased in the serum of Covid-19 patients with respect to healthy donors, and in severe patients with respect to mild ones. Possibly as a consequence of this increase, some of the proteins shed in the serum by ADAM17, including TNF-α, its receptors TNFR1 and TNFR2 and T-cell immunoglobulin and mucin domain 3 (TIM3), but not transforming growth factor beta (TGF-β), are increased in the serum of these patients at the protein level while their mRNA is unchanged, which suggests increased ADAM17 activity (Palacios et al., 2021). Moreover, increased level of ADAM17 and its substrates TNFR1 and TIM3 was higher in patients that died of Covid-19 compared with survivors.
These results support the proposed central role that ADAM17 may play in severe Covid-19, and suggest that the dangerous positive feedback loop of ACE2 downregulation through the action of ADAM17 is active in Covid-19 patients, in particular severe ones.
Several studies based on RNA-seq experiments found that the mRNA of ACE2 is absent in the early foetus and it is lower in children than adults (Bunyavanich et al., 2020; Inde et al., 2020; Muus et al., 2020; Saheb Sharif-Askari et al., 2020). A similar observation applies to the ACE2 protein in serum (Pavel et al., 2021). Therefore, it was proposed that the low expression of the virus receptor ACE2 hinders the propagation of SARS-CoV-2 in children organs. However, several studies, including also some of those cited above, indicate that the expression of ACE2 with age is not monotonic, since ACE2 expression decreases at advanced age in several organs after reaching a maximum.
At the mRNA level, decrease of ACE2 mRNA at advanced age was observed in an analysis of several human tissues collected in the GTeX database (Chen et al., 2020) and in mouse lung samples (Booeshaghi and Pachter, 2020). Single cell data of human respiratory cells reported in Figure 3G of Muus et al. (Muus et al., 2020) are also consistent with decrease or stationarity of ACE2 mRNA after a maximum reached at 10–25 years in multiciliated cells and 25–40 years in alveolar type 2 (AT2) cells. A recent preprint by Inde et al. (Inde et al., 2020) also found a maximum of ACE2 mRNA in mouse lungs at few days after birth, evidenced a minimum at approximately 10 days and an increase at least until 9–11 months, which in mice is middle age. For human data, the same preprint found a maximum of ACE2 mRNA short after birth in the hearth and at about 10 years in the testis (Fig. 6C and 6D of (Inde et al., 2020)). Inde et al. also present data of ACE2 mRNA in human lungs in their Figure 3G, which does not show the existence of a maximum but cannot rule it out due to the sparsity of data at advanced age.
Concerning ACE2 protein in cells, which is the relevant molecular species for virus propagation, western blot analysis of rat lungs (Xie et al., 2006) and mouse aorta (Yoon et al., 2016) indicate that ACE2 membrane protein levels decrease with age in adult rodents. These studies did not include juvenile animals and examined three age classes: 2 (3 for rats), 12 and 24 months, corresponding to young adults, middle age and old. In mouse thoracic aorta, the whole anti-inflammatory arm of the RAS to which ACE2 belongs decreases with age, while the pro-inflammatory arm of the RAS increases with age (Yoon et al., 2016).
In humans, a study found that children below 10 years have on the average higher ACE2 protein in AT2 lung cells than adults, although these values vary considerably from cell to cell and from individual to individual (Ortiz et al., 2020). However, this comparison depended on the discretization of ACE2 expression with an arbitrary threshold of more than 1% of positive cells, and it included adults with asthma that downregulates ACE2, whose exclusion would have raised the p-value. Moreover, other cell types did not confirm the same trend as AT2 lung cells. Zhang, Guo et al. (Zhang et al., 2021) found that ACE2 positive cells in lung biopsy samples from 26 children and 24 adults were significantly decreased in patients older than 50 with respect to children. This reduction was observed in bronchial cells but it was not significant in pulmonary alveolar cells, and it was also observed at the mRNA level. The study by Inde et al. (Inde et al., 2020) also found extreme intra- and inter-individual heterogeneity, but it reached the opposite conclusion that ACE2 protein in human lung epithelial cells increases with age; however, also this conclusion was reached measuring the percentage of cells that express ACE2 above a threshold. Inde et al. also plot ACE2 intensities versus the donor age in their Figures 1C,G, but these figures do not suggest a clear increase of ACE2 with age. Furthermore, they did not observe increase of ACE2 with age in AT2 cells, which are the cell type with maximum ACE2 expression in the lungs (Li et al., 2020b).
We conclude that most existing data are consistent with a decrease across age of ACE2 mRNA and protein levels in rodents and several human cell types. Accordingly, we plot in Figure 4A a model in which ACE2 expression starts in the last stage of foetal life, reaches a maximum at young age and then decreases at old age, with data inspired on the data of Muus et al. (Muus et al., 2020) in multiciliated cells. The position of the maximum depends on the cell type and it is influenced by the strong inter- and intra-individual variability evidenced by several studies (Inde et al., 2020; Ortiz et al., 2020).
FIGURE 4. (A): Proposed model of the dependence of ACE2 with age at the three levels of mRNA, protein in the cellular membrane and protein in serum. The mRNA data are inspired by human multiciliated cells reported in Figure 3G of Muus et al. (2020). The cell protein levels result from time-independent mRNA translation and shedding through ADAM17 that we assume to increase with age as exp (age/25y); other functional forms such as power law yield qualitatively similar results. (B): The severity of pediatric cases of Covid-19 in China (Dong, 2020), rescaled to take into account undetected cases, and put in the context of severe Covid-19 cases of children and adults from Figure 1.
For understanding the relation between ACE2 mRNA and protein expression it is necessary to consider the expression of the metalloprotease ADAM17/TACE that sheds ACE2 from the cell membrane to the serum, from which it is rapidly lost through urine. The expression of ADAM17 increases with age (Dou et al., 2017; Liu et al., 2019), consistent with the increase with age of Ang-II and its receptor ATR1 (Yoon et al., 2016) that upregulates ADAM17 (Deshotels et al., 2014; Xu et al., 2017) together with the bradykinin receptor BKR1 (Parekh and Sriramula, 2020).
As a result of mRNA expression and shedding through ADAM17, the stationary concentration of ACE2 cell protein is proportional to the ratio ACE2mRNA/ADAM17. We plot this function in Figure 4A, where we assume that ADAM17 increases exponentially with age as exp (age/25years). Other functional forms such as power law with exponent 1/3 yield qualitatively similar plots. One can see that the maximum of ACE2 cell protein is reached at earlier age than the maximum of ACE2 mRNA, as the consequence of the increase of ADAM17 with age. ACE2 cell protein decreases in rats and mice from 3 to 12 months (Xie et al., 2006; Yoon et al., 2016) while ACE2 mRNA increases in mice of the same age (Inde et al., 2020). Although this comparison involves different species (rats versus mice) or different cell types (aorta versus lungs), it is consistent with the above prediction. ACE2 protein in the serum is proportional to the product of the cell protein times the shedding rate, i.e. it is proportional to ACE2 mRNA averaged over the different cell types. We depict in Figure 4A the proposed behaviour of ACE2 versus age at the levels of mRNA, cell protein and serum protein.
A paediatric study in China observed that the severity of Covid-19 in children (not including the MIS-C syndrome) decreases with age (Dong, 2020): the proportion of severe and critical paediatric cases was 10.6, 7.3, 4.2, 4.1, and 3.0% for the age groups<1, 1–5, 6–10, 11–15 and 16–18 years, respectively (Figure 4B). This decrease of severity from young children to teen-agers is consistent with the drop in the hospitalization rate in Spain from the age class 0–10 to 10–20 that one can see in Figure 1. Covid-19 severity increase with age in adults (Figure 1), therefore severity versus age has a minimum (Figure 4B). Suggestively, the shape of this curve appears inversely related to the ACE2 protein level across age (Figure 4A), although the position of the maximum of ACE2 depends on the cell type. Another recent paper analysed similar data but proposed a direct instead of inverse relationship between Covid-19 severity and ACE2 expression (Inde et al., 2020). However, most existing data suggest that ACE2 expression decreases at high age, although this point is still debated, and the increase of severity with age is a very clear property of Covid-19.
As discussed in more detail in Ref. (Bastolla, 2021), this inverse relationship between ACE2 levels and Covid-19 severity might rationalize not only the influence of age and sex but also most of the other known risk factors of Covid-19 examined in the OpenSAFELY study (Williamson et al., 2020), including diabetes, hypertension, obesity, vitamin D deficit (that may explain at least part of the risk factors connected with ethnicity) and perhaps even the curious protecting effect of smoke that increases ACE2 expression (Saheb Sharif-Askari et al., 2020).
The proposed inverse relationship between ACE2 expression and Covid-19 severity has an exception in children, who experience mild Covid-19 but express less ACE2 than adults. However, children express more than adults the Ang-II receptor ATR2, which competes with ATR1 and counteracts its inflammatory effect (Kaschina et al., 2017).
The decrease of ATR2 with age has been disputed (Gao et al., 2012), but most works support it, and they also concluded that the receptor binding activity of ATR2, which is most relevant in this context, decreases with age. Both ATR1 and ATR2 are GPCR, and they can form complexes between themselves and with other receptors, including BKR2 (Rukavina Mikusic et al., 2020), underscoring complex regulatory interactions and downregulation of ATR1 by ATR2. We propose that the high level of the ATR2 receptor in children may compensate their lower ACE2 level.
This proposal is consistent with the protective influence on Covid-19 severity of angiotensin receptor blockers that block the signaling of ATR1, as evidenced by a meta-analysis of several clinical trials (Guo et al., 2020), and of anti-ATR1 auto-antibodies (Papola et al., 2021), which are known to induce the increase of ATR2 expression (Sun et al., 2020b).
An increasing amount of evidence indicates that inflammation related genes in general, and ACE2 in particular, are subject to epigenetic control through chromatin modifications. Transcriptional memory provides faster and enhanced transcription upon repeated stimulations, and it is a common hallmark of interferon-stimulated genes, mediated by histone variants such as H3.3 and histone modifications that favour transcription (Kamada et al., 2018) and by de-methylation of CpG islands at active promoters, associated with sustained stimulation by the proinflammatory cytokine TNF-α (Zhao et al., 2020) that is activated by ADAM17.
The ACE2 gene has been shown to be subject to transcriptional memory through CpG island demethylation that increases through age (Corley and Ndhlovu, 2020), and ACE2 transcription in samples of patients with comorbidities associated with severe COVID-19 was correlated with the expression of genes related to histone modifications (Pinto et al., 2020). Similarly, genome-wide CRISPR screens identified several chromatin modifiers that facilitate SARS-COV-2 infection (Wei et al., 2020), likely by upregulating ACE2.
The transcriptional memory of the ACE2 gene has been interpreted as evidence of a positive correlation between ACE2 expression and Covid-19 severity (Pruimboom, 2020). However, it is important to note that the transcriptional memory also acts on pro-inflammatory genes (Kamada et al., 2018; Zhao et al., 2020). The reported decrease of the ACE2/ACE ratio in chronic inflammatory diseases associated with severe Covid-19 (Li et al., 2020a; Pagliaro and Penna, 2020) suggests that the pro-inflammatory side prevails in this balance, with the final result to decrease the ACE2/ACE ratio through the transcriptional downregulation of ACE2, which is also consistent with the theory of inflammaging, which postulates that the increase through age of inflammatory processes contributes to the phenomenology of aging and senescence (Franceschi et al., 2007).
Future work should address the complex regulation of ACE2 and other inflammatory and anti-inflammatory genes from a system perspective, considering multiple levels of regulation (epigenetic, transcriptional, post-translational).
The theory according to which lower ACE2 level may protect from severe disease is based on the premise that lower receptor level hinders the propagation of the virus. But is this assumption warranted? Mathematical models of virus propagation, tested with experiments with bacteriophages, suggest that there is an optimal receptor density at which the virus propagates fastest, with viral velocity declining both for lower and for higher receptor density (Ortega-Cejas et al., 2004) (see Figure 5). A mathematical model of the dependence of Covid-19 mortality on receptor density fitted to both Covid-19 and SARS 2003 data of lethality across age and sex suggests that both viruses propagate in a regime in which increasing receptor density slows down their propagation (Bastolla, 2021). Thus, the inverse relation between ACE2 expression and Covid-19 severity is not implausible.
FIGURE 5. Mathematical model of the velocity of viral propagation in an organ as a function of the receptor density (adapted from the formulas presented in Ref. (Ortega-Cejas et al., 2004) tested with experiments on bacteriophage propagation).
It has been recently shown that type-I interferons (IFN-I = IFN-α, IFN-β) upregulate ACE2 expression (Ziegler et al., 2020) and ACE2 has been shown to coevolve with interferon alpha/beta receptor 2 (IFNAR2) throughout mammalian evolution, in the sense that their evolutionary rates are strongly correlated (Varela et al., 2021). Interestingly, IFN-β are used as anti-inflammatory therapy in multiple sclerosis (Kieseier, 2011), supporting their anti-inflammatory role. We conjecture that the upregulation of ACE2 by IFN-I may contribute to stop the inflammatory cascade. This conjecture is consistent with the recent finding that impaired type-I IFN response (Hadjadj et al., 2020), auto-antibodies against IFN-α (Bastard et al., 2020) and genetic variants that hinder interferon activation (Zhang et al., 2020) are associated with more severe Covid-19 cases. Moreover, mutations of the IFN-I receptor have been found to be risk factors of severe Covid-19 through genome wide association studies (GWAS) analysis (Pairo-Castineira et al., 2020). Finally, SARS-COV-2 infection is characterized by low levels of IFN-I (Blanco-Melo et al., 2020), partly because the virus dysregulates IFN-I (Konno et al., 2020). Therefore, we suggest that IFN downregulation cooperates with ACE2 dysregulation by SARS-COV-2 in preventing the arrest of the inflammatory process in severe Covid-19 cases. However, interferon therapy did not provide any reduction of mortality or hospitalization in the Solidarity clinical trial (olidarity Trial Cons, 2020).
Our hypothesis supports several candidate drugs that might alleviate severe complications of CoViD-19 and even its post infection consequences (MIS-C and long Covid-19) by inhibiting the processes that prevent the reversion of the inflammatory state. Luckily for several of these drugs clinical trials are already on-going.
1. Human recombinant ACE2 has been suggested as a potential therapeutic agent against SARS-CoV-2. It can have a double protective effect. On one hand, it can alleviate the inflammatory process, and in the other one it can sequester the virus and difficult its entry in the cells (Batlle et al., 2020; Huang et al., 2021). A recent case report presents very promising results, and clinical trials are ongoing (Zoufaly et al., 2020).
2. Angiotensin receptor blockers (ARB) that block the ATR1 receptor, downregulating the inflammatory process and preventing the positive feedback loop of Ang-II through upregulation of ACE and downregulation of ACE2. They are often discussed together with ACE inhibitors (ACE-I) that reduce the formation of Ang-II. However, ACE has anti-inflammatory effect on the KKS, where it downregulates bradykinin, so that one of the known adverse effects of ACE-I is the upregulation of KKS eventually leading to edema. Moreover, Ang-II can be also formed through other peptidases. The possible therapeutic role of ARB and ACE-I was suggested by several groups already at the time of SARS 2003 and later proposed for SARS-COV-2 (Annweiler et al., 2020; Sun et al., 2020a; Ciaglia et al., 2020; Gurwitz, 2020; Offringa et al., 2020; Verdecchia et al., 2020). Although there was the concern that, by upregulating ACE2, ARB and ACE-I might favour the virus, meta-analysis of several clinical studies suggested that they are associated with lower mortality of COVID-19 in patients with hypertension, with odds ratio 0.57 (95% CI, 0.38–0.84) (Guo et al., 2020). The clinical trials NCT04312009 and NCT04311177 are ongoing to test possible protective effects, although caution is necessary if blood pressure becomes too low, which is a frequent consequence of severe Covid-19 (Lala et al., 2020; Michard and Vieillard-Baron, 2020), probably through the activation of the bradykinin axis.
3. Drugs that enhance the ATR2 receptor. Their effect may be in principle similar to ARB.
4. The product of ACE2, Ang1-7, which exerts anti-inflammatory and anti-fibrotic effects (Chappell and Al Zayadneh, 2017) through the receptor Mas. However, its mechanism of action is still not fully known, it is rapidly degraded by ACE, and it has a moderate affinity for ATR1, thus it might produce effects contrary to the intentions.
5. IFN-I that upregulate ACE2. Although interferon may promote inflammation, IFN-β (type I) is used as anti-inflammatory drug to treat multiple sclerosis (Kieseier, 2011). However, the Solidarity clinical trial did not observe any reduction of Covid-19 mortality upon IFN-I treatment (olidarity Trial Cons, 2020).
6. Other drugs that upregulate ACE2.
7. Inhibitors of TACE/ADAM17, which may prevent the degradation of ACE2 and the increase of TNF-α.
8. Inhibitors of IL-1 and TNF-α, which are successfully used to treat KD and MIS-C, might also be useful to treat Covid-19 for preventing excessive activation of the KKS.
9. Vitamin D, which exerts a protective role by downregulating ATR1 and ADAM17, and in this way limits the inflammatory process and protects ACE2 from excessive shedding. A preprint reporting a retrospective study of almost 5 million people found an association between vitamin D deficiency and Covid-19 severity (Israel et al., 2020), and a clinical study with 76 patients found that a high dose of Calcifediol, a metabolite of vitamin D, significantly reduced the ICU treatment for hospitalized Covid-19 patients (Entrenas Castillo et al., 2020).
Of course, experimental verifications of the above hypothesis are necessary, and clinical trials are urgently needed to test these therapeutic indications.
As reviewed above by us and elsewhere by many other authors, the peptidase ACE2, besides being the cellular receptor of SARS-COV-2 and other coronavirus, exerts a central regulatory role in inflammatory processes by downregulating the main pro-inflammatory peptides of the Renin-angiotensin system (RAS) and the Kinin kallikrein system (KKS) (Figure 3). These two highly integrated signalling systems, whose receptors act synergistically and strengthen each other, activate the inflammatory response, activate cytokines starting from a central controller of inflammation such as TNF-α, which is activated by the same protease TACE/ADAM17 that degrades ACE2 and recruits macrophages and neutrophils. We propose that the main role of ACE2 consists in terminating this inflammatory process. Failure to do so, because of ACE2 degradation or failure to activate it, may exacerbate the inflammatory response leading to organ damage, including edema, and it may be responsible of the most severe consequences of Covid-19 even after the infection has been resolved, as it happens in the Multi-inflammatory syndrome of children (MIS-C).
Because of the central role of ACE2 both for SARS-COV-2 propagation and for controlling the inflammatory process, and because of the very marked increase of Covid-19 with age (Figure 1), a strong effort has been dedicated to elucidate how ACE2 expression changes with age. However, these works have produced conflicting results, that have been interpreted either as support of the theory that the receptor expression favours SARS-COV-2 infection or as support of the opposite theory that high ACE2 expression favours milder disease because of its anti-inflammatory role.
We have shown that these contrasting results on ACE2 expression across age can be reconciled going beyond the dichotomy between adults and children and recognizing that ACE2 expression does not change monotonically with age but it starts in late foetal life, reaches a maximum at a young age that depends on the cell type and exhibits strong inter-individual and inter-cellular variation, and decreases at advanced age. At the protein level, this decrease precedes that of mRNA, Remove starts before and it is more accentuated than at the mRNA level, due to the increase with age of the activity of the protease TACE/ADAM17 that sheds ACE2 from the cell membrane to the serum. This proposed behaviour, represented in Figures 4A, is consistent with essentially all existing data on the ACE2 expression across age in rodents and humans, except some of the figures of the preprint by Inde et al. (Inde et al., 2020).
The complexity and the intricacies of ACE2 regulation will require future approaches that integrate multiple regulatory levels, from chromatin remodelling that plays a key role in transcriptional memory to regulation through transcription factors and post-translational regulation through ADAM17 and other proteases that degrade ACE2. This is an important subject, since the decrease of ACE2 with age, and the simultaneous increase of the pro-inflammatory components of the RAS may play an important role in the theory of inflammaging, which postulates that the increase through age of inflammatory processes contributes to the phenomenology of aging and senescence (Franceschi et al., 2007).
The proposed decrease of ACE2 across age and the observed decrease of the ACE2/ACE ratio in chronic inflammatory diseases often associated with severe Covid-19 (Pagliaro and Penna, 2020; Li et al., 2020a) support a negative relationship between Covid-19 severity and ACE2 expression (Figure 4B). This is not as paradoxical as it seems, since a mathematical model of virus propagation across cells predicts that an increase of the receptor level does not necessarily accelerate viral propagation and it may even slow it down (Ortega-Cejas et al., 2004) (see Figure 5 for illustration). Furthermore, ACE2 is located in the X chromosome and its protein expression is higher in females than in males in murine models (Xie et al., 2006), which may extend the proposed negative correlation also to the comparison between sexs. Interestingly, in a recent analysis of human lung transcriptomic data it was found that the proinflammatory angiotensin receptor ATR1 is significantly more expressed in male tissues than in female ones, while the anti-inflammatory ATR2 has similar expression in both sexes (Hachim Ibrahim et al., 2021), which is also consistent with the hypothesis presented here.
The exception to this proposed negative relationship is constituted by children, who express less ACE2 than adults and experience much milder disease. However, in children the RAS is much less dis-balanced towards the pro-inflammatory side than in adults. In particular children express more ATR2, which is anti-inflammatory.
Of course, experimental work is needed for supporting the hypothesis presented in this review, but we hope that they may help rationalizing apparently conflicting observations.
Publicly available datasets were analyzed in this study. This data can be found here: https://www.mscbs.gob.es/profesionales/saludPublica/ccayes/alertasActual/nCov/documentos/Actualizacion_101_COVID-19.pdf.
UB conceived this study, developed the model of ACE2 expression across age, computed Covid-19 across age and made the figure of the RAS and bradykinin system. All authors provided references and participated in the discussion of the RAS and bradykinin system.
This review paper is related with our experimental study of ACE2 in the serum of Covid-19 patients funded by the Spanish Research Council (CSIC) under the grant CSIC-COV19-108.
The authors declare that the research was conducted in the absence of any commercial or financial relationships that could be construed as a potential conflict of interest.
All claims expressed in this article are solely those of the authors and do not necessarily represent those of their affiliated organizations, or those of the publisher, the editors, and the reviewers. Any product that may be evaluated in this article, or claim that may be made by its manufacturer, is not guaranteed or endorsed by the publisher.
We gratefully acknowledge financial support from the Spanish Research Council (CSIC) under the grant CSIC-COV19-108. We thank Juan Carlos Alonso for interesting discussions.
Alghamri, M. S., Weir, N. M., Anstadt, M. P., Elased, K. M., Gurley, S. B., and Morris, M. (2013). Enhanced Angiotensin II-Induced Cardiac and Aortic Remodeling in ACE2 Knockout Mice. J. Cardiovasc. Pharmacol. Ther. 18, 138–151. doi:10.1177/1074248412460124
Annweiler, C., Cao, Z., Wu, Y., Faucon, E., Mouhat, S., Kovacic, H., et al. (2020). Counter-regulatory 'Renin-Angiotensin' System-Based Candidate Drugs to Treat COVID-19 Diseases in SARS-CoV-2-Infected Patients. Iddt 20, 407–408. doi:10.2174/1871526520666200518073329
Ayoubkhani, D., Khunti, K., Nafilyan, V., Maddox, T., Humberstone, B., Diamond, S. I., et al. (2021). Epidemiology of post-COVID Syndrome Following Hospitalisation with Coronavirus: a Retrospective Cohort Study. Medrxiv Jan. doi:10.1101/2021.01.15.21249885
Bastard, P., Rosen, L. B., Zhang, Q., Michailidis, E., Hoffmann, H-H., Zhang, Y., et al. (2020). Auto-antibodies against Type I IFNs in Patients with Life-Threatening COVID-19. Science, eabd4585. doi:10.1126/science.abd4585
Bastolla, U. (2021). Mathematical Model of SARS-Cov-2 Propagation versus ACE2 Fits COVID-19 Lethality across Age and Sex and Predicts that of SARS. Front. Mol. Biosci. 8, 706122. doi:10.3389/fmolb.2021.706122
Batlle, D., Wysocki, J., and Satchell, K. (2020). Soluble Angiotensin-Converting Enzyme 2: a Potential Approach for Coronavirus Infection Therapy? Clin. Sci. 134, 543–545. doi:10.1042/cs20200163
Blanco-Melo, D., Nilsson-Payant, B. E., Liu, W.-C., Uhl, S., Hoagland, D., Møller, R., et al. (2020). Imbalanced Host Response to SARS-CoV-2 Drives Development of COVID-19. Cell 181, 1036–1045. doi:10.1016/j.cell.2020.04.026
Booeshaghi, A. S., and Pachter, L. Decrease inACE2mRNA Expression in Aged Mouse Lung. Preprint 2020. doi:10.1101/2020.04.02.021451
Bunyavanich, S., Do, A., and Vicencio, A. (2020). Nasal Gene Expression of Angiotensin-Converting Enzyme 2 in Children and Adults. JAMA 323, 2427–2429. doi:10.1001/jama.2020.8707
Chappell, M. C., and Al Zayadneh, E. M. (2017). Angiotensin-(1-7) and the Regulation of Anti-fibrotic Signaling Pathways. J. Cel Signal 2, 134. doi:10.4172/2576-1471.1000134
Chen, J., Jiang, Q., Xia, X., Liu, K., Yu, Z., Tao, W., et al. (2020). Individual Variation of the SARS-CoV-2 Receptor ACE2 Gene Expression and Regulation. Aging Cell 19, e13168. doi:10.1111/acel.13168
Ciaglia, E., Vecchione, C., and Puca, A. A. (2020). COVID-19 Infection and Circulating ACE2 Levels: Protective Role in Women and Children. Front. Pediatr. 8, 206. doi:10.3389/fped.2020.00206
Corley, M. J., and Ndhlovu, L. C. DNA Methylation Analysis of the COVID-19 Host Cell Receptor, Angiotensin I Converting Enzyme 2 Gene (ACE2) in the Respiratory System Reveal Age and Gender Differences. Preprints 2020, 2020030295. doi:10.20944/preprints202003.0295.v1
Cristiani, L., Mancino, E., Matera, L., Nenna, R., Pierangeli, A., Scagnolari, C., et al. (2020). Will Children Reveal Their Secret? the Coronavirus Dilemma. Eur. Respir. J. 55 (4), 2000749. doi:10.1183/13993003.00749-2020
Cyranoski, D. (2020). Why Children Avoid the Worst Coronavirus Complications Might Lie in Their Arteries. Nature 582, 324–325. doi:10.1038/d41586-020-01692-z
Deshotels, M. R., Xia, H., Sriramula, S., Lazartigues, E., and Filipeanu, C. M. (2014). Angiotensin II Mediates Angiotensin Converting Enzyme Type 2 Internalization and Degradation through an Angiotensin II Type I Receptor-dependent Mechanism. Hypertension 64, 1368–1375. doi:10.1161/hypertensionaha.114.03743
Dong, E., Du, H., and Gardner, L. (2020). An Interactive Web-Based Dashboard to Track COVID-19 in Real Time. Lancet Infect. Dis. 20, 533–534. doi:10.1016/s1473-3099(20)30120-1
Dou, H., Feher, A., Davila, A. C., Romero, M. J., Patel, V. S., Kamath, V. M., et al. (2017). Role of Adipose Tissue Endothelial ADAM17 in Age-Related Coronary Microvascular Dysfunction. Atvb 37, 1180–1193. doi:10.1161/atvbaha.117.309430
Entrenas Castillo, M., Entrenas Costa, L. M., Vaquero Barrios, J. M., Alcalá Díaz, J. F., López Miranda, J., Bouillon, R., et al. (2020). "Effect of Calcifediol Treatment and Best Available Therapy versus Best Available Therapy on Intensive Care Unit Admission and Mortality Among Patients Hospitalized for COVID-19: A Pilot Randomized Clinical Study". J. Steroid Biochem. Mol. Biol. 203, 105751. doi:10.1016/j.jsbmb.2020.105751
Franceschi, C., Capri, M., Monti, D., Giunta, S., Olivieri, F., Sevini, F., et al. (2007). Inflammaging and Anti-inflammaging: a Systemic Perspective on Aging and Longevity Emerged from Studies in Humans. Mech. Ageing Dev. 128, 92–105. doi:10.1016/j.mad.2006.11.016
Gao, J., Chao, J., Parbhu, K.-J. K., Yu, L., Xiao, L., Gao, F., et al. (2012). Ontogeny of Angiotensin Type 2 and Type 1 Receptor Expression in Mice. J. Renin Angiotensin Aldosterone Syst. 13, 341–352. doi:10.1177/1470320312443720
Garvin, M. R., Alvarez, C., Miller, J. I., Prates, E. T., Walker, A. M., Amos, B. K., et al. (2020). A Mechanistic Model and Therapeutic Interventions for COVID-19 Involving a RAS-Mediated Bradykinin Storm. Elife 9, e59177. doi:10.7554/eLife.59177
Guo, X., Zhu, Y., and Hong, Y. (2020). Decreased Mortality of COVID-19 with Renin-Angiotensin-Aldosterone System Inhibitors Therapy in Patients with Hypertension: A Meta-Analysis. Hypertension 76, e13–e14. doi:10.1161/HYPERTENSIONAHA.120.15572
Gurwitz, D. (2020). Angiotensin Receptor Blockers as Tentative SARS-CoV-2 Therapeutics. Drug Dev. Res. 81, 537–540. doi:10.1002/ddr.21656
Hachim Ibrahim, Y., Hachim Mahmood, Y., Talaat Iman Mamdouh, López-Ozuna Vanessa, M., Saheb Sharif-Askari, N., Al Heialy, S., Rabih, Halwani., et al. (2021). The Molecular Basis of Gender Variations in Mortality Rates Associated with the Novel Coronavirus (COVID-19) Outbreak. Front. Mol. Biosci. 8, 894. doi:10.3389/fmolb.2021.728409
Hadjadj, J., Yatim, N., Barnabei, L., Corneau, A., Boussier, J., Smith, N., et al. (2020). Impaired Type I Interferon Activity and Inflammatory Responses in Severe COVID-19 Patients. Science 369, 718–724. doi:10.1126/science.abc6027
Haga, S., Yamamoto, N., Nakai-Murakami, C., Osawa, Y., Tokunaga, K., Sata, T., et al. (2008). Modulation of TNF- -converting Enzyme by the Spike Protein of SARS-CoV and ACE2 Induces TNF- Production and Facilitates Viral Entry. Proc. Natl. Acad. Sci. 105, 7809–7814. doi:10.1073/pnas.0711241105
Hanafy, S., Tavasoli, M., and Jamali, F. (2011). Inflammation Alters Angiotensin Converting Enzymes (ACE and ACE-2) Balance in Rat Heart. Inflammation 34, 609–613. doi:10.1007/s10753-010-9269-1
Huang, K. Y., Lin, M. S., Kuo, T. C., Chen, C. L., Lin, C. C., Chou, Y. C., et al. (2021). Humanized COVID-19 Decoy Antibody Effectively Blocks Viral Entry and Prevents SARS-CoV-2 Infection. EMBO Mol. Med. 13, e12828. doi:10.15252/emmm.202012828
Imai, Y., Kuba, K., Rao, S., Huan, Y., Guo, F., Guan, B., et al. (2005). Angiotensin-converting Enzyme 2 Protects from Severe Acute Lung Failure. Nature 436, 112–116. doi:10.1038/nature03712
Inde, Z., Yapp, C., Joshi, G. N., Spetz, J., Fraser, C., Deskin, B., et al. Age-dependent Regulation of SARS-CoV-2 Cell Entry Genes and Cell Death Programs Correlates with COVID-19 Disease Severity. Preprint. Biorxiv. Published 2020. doi:10.1101/2020.09.13.276923
Israel, A., Cicurel, A. A., Feldhamer, I., Dror, Y., Giveon, S. M., Gillis, D., et al. (2020). The Link between Vitamin D Deficiency and Covid-19 in a Large Population. medRxiv, 20188268. 09.04. doi:10.1101/2020.09.04.20188268
Kamada, R., Yang, W., Zhang, Y., Patel, M. C., Yang, Y., Ouda, R., et al. (2018). Interferon Stimulation Creates Chromatin marks and Establishes Transcriptional Memory. Proc. Natl. Acad. Sci. USA 115, E9162–E9171. doi:10.1073/pnas.1720930115
Kaschina, E., Namsolleck, P., and Unger, T. (2017). AT2 Receptors in Cardiovascular and Renal Diseases. Pharmacol. Res. 125, 39–47. doi:10.1016/j.phrs.2017.07.008
Kieseier, B. C. (2011). The Mechanism of Action of Interferon-β in Relapsing Multiple Sclerosis. CNS Drugs 25, 491–502. doi:10.2165/11591110-000000000-00000
Koka, V., Huang, X. R., Chung, A. C. K., Wang, W., Truong, L. D., and Lan, H. Y. (2008). Angiotensin II Up-Regulates Angiotensin I-Converting Enzyme (ACE), but Down-Regulates ACE2 via the AT1-ERK/p38 MAP Kinase Pathway. Am. J. Pathol. 172, 1174–1183. doi:10.2353/ajpath.2008.070762
Konno, Y., Kimura, I., Uriu, K., Fukushi, M., Irie, T., Koyanagi, Y., et al. (2020). SARS-CoV-2 ORF3b Is a Potent Interferon Antagonist Whose Activity Is Increased by a Naturally Occurring Elongation Variant. Cel Rep. 32, 108185. doi:10.1016/j.celrep.2020.108185
Kuba, K., Imai, Y., Rao, S., Gao, H., Guo, F., Guan, B., et al. (2005). A Crucial Role of Angiotensin Converting Enzyme 2 (ACE2) in SARS Coronavirus-Induced Lung Injury. Nat. Med. 11, 875–879. doi:10.1038/nm1267
Lala, A., Johnson, K. W., Januzzi, J. L., Russak, A. J., Paranjpe, I., Richter, F., et al. (2020). Prevalence and Impact of Myocardial Injury in Patients Hospitalized with COVID-19 Infection. J. Am. Coll. Cardiol. 76, 533–546. doi:10.1016/j.jacc.2020.06.007
Lambert, D. W., Yarski, M., Warner, F. J., Thornhill, P., Parkin, E. T., Smith, A. I., et al. (2005). Tumor Necrosis Factor-α Convertase (ADAM17) Mediates Regulated Ectodomain Shedding of the Severe-Acute Respiratory Syndrome-Coronavirus (SARS-CoV) Receptor, Angiotensin-Converting Enzyme-2 (ACE2). J. Biol. Chem. 280, 30113–30119. doi:10.1074/jbc.m505111200
Li, Y., Xu, Q., Ma, L., Wu, D., Gao, J., Chen, G., et al. (2020). Systematic Profiling of ACE2 Expression in Diverse Physiological and Pathological Conditions for COVID-19/SARS-CoV-2. J. Cel Mol Med 24, 9478–9482. doi:10.1111/jcmm.15607
Li, Y., Zhou, W., Yang, L., and You, R. (2020). Physiological and Pathological Regulation of ACE2, the SARS-CoV-2 Receptor. Pharmacol. Res. 157, 104833. doi:10.1016/j.phrs.2020.104833
Liu, H., Wang, H., Cheng, D., Wang, Q., Pei, Z., Zhu, N., et al. (2019). Potential Role of a Disintegrin and Metalloproteinase-17 (ADAM17) in Age-Associated Ventricular Remodeling of Rats. RSC Adv. 9, 14321–14330. doi:10.1039/c9ra01190k
Matthews, V., Schuster, B., Schütze, S., Kallen, K-J., and Rose-John, S. (2003). Cholesterol Depletion of the Plasma Membrane Triggers Shedding of the Human Interleukin-6 Receptor by TACE and Independently of PKC. J. Biol. Chem. 278, 3882939. doi:10.1074/jbc.m210584200
Michard, F., and Vieillard-Baron, A. (2020). Critically Ill Patients with COVID-19: Are They Hemodynamically Unstable and Do We Know Why? Intensive Care Med.
Muus, C., Luecken, M. D., Eraslan, G., Waghray, A., Heimberg, G., Sikkema, L., et al. (2020). Integrated Analyses of Single-Cell Atlases Reveal Age, Sex, and Smoking Status Associations with Cell Type-specific Expression of Mediators of SARS-CoV-2 Viral Entry and Highlights Inflammatory Programs in Putative Target Cells. Preprint bioRxiv, 04925404.19. doi:10.1101/2020.04.19.049254
Nicolau, L. A. D., Magalhães, P. J. C., and Vale, M. L. (2020). What Would Sérgio Ferreira Say to Your Physician in This War against COVID-19: How about Kallikrein/kinin System? Med. Hypotheses 143, 109886. doi:10.1016/j.mehy.2020.109886
Offringa, A., Montijn, R., Singh, S., Paul, M., Pinto, Y. M., and Pinto-Sietsma, S-J. (2020). The Mechanistic Overview of SARS-CoV-2 Using Angiotensin-Converting Enzyme 2 to Enter the Cell for Replication: Possible Treatment Options Related to the Renin-Angiotensin System. Eur. Heart J. Cardiovasc. Pharmacother. 6, 317–325. doi:10.1093/ehjcvp/pvaa053
Ortega-Cejas, V., Fort, J., Méndez, V., and Campos, D. (2004). Approximate Solution to the Speed of Spreading Viruses. Phys. Rev. E Stat. Nonlin Soft Matter Phys. 69, 031909. doi:10.1103/PhysRevE.69.031909
Ortiz, M. E., Thurman, A., Pezzulo, A. A., Leidinger, M. R., Klesney-Tait, J. A., Karp, P. H., et al. (2020). Heterogeneous Expression of the SARS-Coronavirus-2 Receptor ACE2 in the Human Respiratory Tract. EBioMedicine 60, 102976. doi:10.1016/j.ebiom.2020.102976
Pagliaro, P., and Penna, C. (2020). ACE/ACE2 Ratio: A Key Also in 2019 Coronavirus Disease (Covid-19)? Front. Med. 7, 335. doi:10.3389/fmed.2020.00335
Pairo-Castineira, E., Clohisey, S., Klaric, L., Bretherick, A. D., Rawlik, K., Pasko, D., et al. (2020). Genetic Mechanisms of Critical Illness in Covid-19. Nature 591 (7848), 92–98. doi:10.1038/s41586-020-03065-y
Palacios, Y., Ruiz, A., Ramón-Luing, L. A., Ocaña-Guzman, R., Barreto-Rodriguez, O., Sánchez-Monciváis, A., et al. (2021). Severe COVID-19 Patients Show an Increase in Soluble TNFR1 and ADAM17, with a Relationship to Mortality. Ijms 22, 8423. doi:10.3390/ijms22168423
Papola, F., Biancofiore, V., Angeletti, C., Grimaldi, A., Carucci, A. C., Cofini, V., et al. (2021). Anti-AT1R Autoantibodies and Prediction of the Severity of Covid-19. Hum. Immunol. S0198-8859 (21), 00251–00252. doi:10.1016/j.humimm.2021.10.006
Parekh, R. U., and Sriramula, S. (2020). Activation of Kinin B1R Upregulates ADAM17 and Results in ACE2 Shedding in Neurons. Ijms 22, 145. doi:10.3390/ijms22010145
Pavel, A. B., Wu, J., Renert-Yuval, Y., Del Duca, E., Glickman, J. W., Miller, R. L., et al. (2021). SARS-CoV-2 Receptor ACE2 Protein Expression in Serum Is Significantly Associated with Age. Allergy 76, 875–878. doi:10.1111/all.14522
Pierce, C. A., Preston-Hurlburt, P., Dai, Y., Aschner, C. B., Cheshenko, N., Galen, B., et al. (2020). Immune Responses to SARS-CoV-2 Infection in Hospitalized Pediatric and Adult Patients. Sci. Transl. Med. 12, eabd5487. doi:10.1126/scitranslmed.abd5487
Pinto, B. G., Oliveira, A. E. R., Singh, Y., Jimenez, L., Gonçalves, A. N. A., Ogava, R. L. T., et al. (2020). ACE2 Expression Is Increased in the Lungs of Patients with Comorbidities Associated with Severe COVID-19. J. Inf. Dis. 222 (4), 556–563. doi:10.1093/infdis/jiaa332
Pruimboom, L. (2020). Methylation Pathways and SARS-CoV-2 Lung Infiltration and Cell Membrane-Virus Fusion Are Both Subject to Epigenetics. Front. Cel. Infect. Microbiol. 10, 290. doi:10.3389/fcimb.2020.00290
Rose-John, S. (2012). IL-6 Trans-signaling via the Soluble IL-6 Receptor: Importance for the Pro-inflammatory Activities of IL-6. Int. J. Biol. Sci. 8, 1237–1247. doi:10.7150/ijbs.4989
Rukavina Mikusic, N. L., Silva, M. G., Pineda, A. M., and Gironacci, M. M. (2020). Angiotensin Receptors Heterodimerization and Trafficking: How Much Do They Influence Their Biological Function? Front. Pharmacol. 11, 1179. doi:10.3389/fphar.2020.01179
Saheb Sharif-Askari, N., Saheb Sharif-Askari, F., Alabed, M., Temsah, M.-H., Al Heialy, S., Hamid, Q., et al. (2020). Airways Expression of SARS-CoV-2 Receptor, ACE2, and TMPRSS2 Is Lower in Children Than Adults and Increases with Smoking and COPD. Mol. Ther. - Methods Clin. Dev. 18, 1–6. doi:10.1016/j.omtm.2020.05.013
Sodhi, C. P., Wohlford-Lenane, C., Yamaguchi, Y., Prindle, T., Fulton, W. B., Wang, S., et al. (2018). Attenuation of Pulmonary ACE2 Activity Impairs Inactivation of Des-Arg9 bradykinin/BKB1R axis and Facilitates LPS-Induced Neutrophil Infiltration. Am. J. Physiology-Lung Cell Mol. Physiol. 314, L17–L31. doi:10.1152/ajplung.00498.2016
Sun, M. L., Yang, J. M., Sun, Y. P., and Su, G. H. (2020). Inhibitors of RAS Might Be a Good Choice for the Therapy of COVID-19 Pneumonia. Zhonghua Jie He He Hu Xi Za Zhi 43, 219–222. doi:10.3760/cma.j.issn.1001-0939.2020.03.016
Sun, Y., Li, Y., Wang, M., Yue, M., Bai, L., Bian, J., et al. (2020). Increased AT2R Expression Is Induced by AT1R Autoantibody via Two Axes, Klf-5/IRF-1 and circErbB4/miR-29a-5p, to Promote VSMC Migration. Cell Death Dis 11, 432. doi:10.1038/s41419-020-2643-5
van de Veerdonk, F. L., Netea, M. G., van Deuren, M., van der Meer, J. W., de Mast, Q., Brüggemann, R. J., et al. (2020). Kallikrein-kinin Blockade in Patients with COVID-19 to Prevent Acute Respiratory Distress Syndrome. Elife 9, e57555. doi:10.7554/eLife.57555
Varela, A. A., Cheng, S., and Werren, J. H. (2021). Novel ACE2 Protein Interactions Relevant to COVID-19 Predicted by Evolutionary Rate Correlations. PeerJ 9, e12159. doi:10.7717/peerj.12159
Verdecchia, P., Cavallini, C., Spanevello, A., and Angeli, F. (2020). The Pivotal Link between ACE2 Deficiency and SARS-CoV-2 Infection. Eur. J. Intern. Med. 76, 14–20. doi:10.1016/j.ejim.2020.04.037
Wei, J., Alfajaro, M. M., DeWeirdt, P. C., Hanna, R. E., Lu-Culligan, W. J., Cai, W. L., et al. (2021). Genome-wide CRISPR Screens Reveal Host Factors Critical for SARS-CoV-2 Infection. Cell 184, 76–91.e13. doi:10.1016/j.cell.2020.10.028
WHO Solidarity Trial Consortium (2021). WHO Solidarity Trial Consortium. Repurposed Antiviral Drugs for Covid-19 - Interim WHO Solidarity Trial Results. N Engl J Med. 384, 497–511. doi:10.1016/j.cell.2020.10.028
Williamson, E. J., Walker, A. J., Bhaskaran, K., Bacon, S., Bates, C., Morton, C. E., et al. (2020). Factors Associated with COVID-19-Related Death Using OpenSAFELY. Nature 584, 430–436. doi:10.1038/s41586-020-2521-4
Wu, M., Chen, Y., Xia, H., Wang, C., Tan, C. Y., Cai, X., et al. (2020). Transcriptional and Proteomic Insights into the Host Response in Fatal COVID-19 Cases. Proc. Natl. Acad. Sci. U S A. 117, 28336–28343. doi:10.1073/pnas.2018030117
Xie, X., Xudong, X., Chen, J., Junzhu, C., Wang, X., Xingxiang, W., et al. (2006). Age- and Gender-Related Difference of ACE2 Expression in Rat Lung. Life Sci. 78, 2166–2171. doi:10.1016/j.lfs.2005.09.038
Xu, J., Sriramula, S., Xia, H., Moreno-Walton, L., Culicchia, F., Domenig, O., et al. (2017). Clinical Relevance and Role of Neuronal at 1 Receptors in ADAM17-Mediated ACE2 Shedding in Neurogenic Hypertension. Circ. Res. 121, 43–55. doi:10.1161/circresaha.116.310509
Yoon, H. E., Kim, E. N., Kim, M. Y., Lim, J. H., Jang, I. A., Ban, T. H., et al. (2016). Age-associated Changes in the Vascular Renin-Angiotensin System in Mice. Oxid. Med. Cel. Longev. 2016, 6731093. doi:10.1155/2016/6731093
Zhang, Q., Bastard, P., Liu, Z., Le Pen, J., Moncada-Velez, M., Chen, J., et al. (2020). Inborn Errors of Type I IFN Immunity in Patients with Life-Threatening COVID-19. Science 370, eabd4570. doi:10.1126/science.abd4570
Zhang, Z., Guo, L., Huang, L., Zhang, C., Luo, R., Zeng, L., et al. (2021). Distinct Disease Severity between Children and Older Adults with COVID-19: Impacts of ACE2 Expression, Distribution, and Lung Progenitor Cells. Clin. Infect. Dis. 2021, ciaa1911. doi:10.1093/cid/ciaa1911
Zhao, Z., Zhang, Z., Li, J., Dong, Q., Xiong, J., Li, Y., et al. (2020). Sustained TNF-α Stimulation Leads to Transcriptional Memory that Greatly Enhances Signal Sensitivity and Robustness. eLife 9, e61965. doi:10.7554/eLife.61965
Zhou, P., Yang, X.-L., Wang, X.-G., Hu, B., Zhang, L., Zhang, W., et al. (2020). A Pneumonia Outbreak Associated with a New Coronavirus of Probable Bat Origin. Nature 579, 270–273. doi:10.1038/s41586-020-2012-7
Ziegler, C. G. K., Allon, S. J., Nyquist, S. K., Mbano, I. M., Miao, V. N., Tzouanas, C. N., et al. (2020). SARS-CoV-2 Receptor ACE2 Is an Interferon-Stimulated Gene in Human Airway Epithelial Cells and Is Detected in Specific Cell Subsets across Tissues. Cell 181, 1016–e19. doi:10.1016/j.cell.2020.04.035
Zimmerman, P., and Curtis, N. (2020). Why Is COVID-19 Less Severe in Children? A Review of the Proposed Mechanisms Underlying the Age-Related Difference in Severity of SARS-CoV-2 Infections. Arch. Dis. Child. 106, 429–439. doi:10.1136/archdischild-2020-320338
Keywords: COVID-19, SARS-CoV-2, ACE2, renin angiotensin system, bradykinin, TACE/ADAM17, angiotensin receptors
Citation: Bastolla U, Chambers P, Abia D, Garcia-Bermejo M-L and Fresno M (2022) Is Covid-19 Severity Associated With ACE2 Degradation?. Front. Drug. Discov. 1:789710. doi: 10.3389/fddsv.2021.789710
Received: 05 October 2021; Accepted: 30 November 2021;
Published: 25 January 2022.
Edited by:
Juan Fernandez-Recio, Spanish National Research Council (CSIC), SpainReviewed by:
Rupesh Gautam, Maharishi Markandeshwar University, IndiaCopyright © 2022 Bastolla, Chambers, Abia, Garcia-Bermejo and Fresno. This is an open-access article distributed under the terms of the Creative Commons Attribution License (CC BY). The use, distribution or reproduction in other forums is permitted, provided the original author(s) and the copyright owner(s) are credited and that the original publication in this journal is cited, in accordance with accepted academic practice. No use, distribution or reproduction is permitted which does not comply with these terms.
*Correspondence: Ugo Bastolla, dWJhc3RvbGxhQGNibS5jc2ljLmVz
Disclaimer: All claims expressed in this article are solely those of the authors and do not necessarily represent those of their affiliated organizations, or those of the publisher, the editors and the reviewers. Any product that may be evaluated in this article or claim that may be made by its manufacturer is not guaranteed or endorsed by the publisher.
Research integrity at Frontiers
Learn more about the work of our research integrity team to safeguard the quality of each article we publish.