- Department of Pharmaceutics, UCL School of Pharmacy, University College London, London, United Kingdom
Fungal infections, though affecting healthcare globally, receive insufficient attention in clinical and academic settings. Invasive fungal infections, particularly caused by combat wounds, have been identified as a critical threat by the US Department of Defense. Monotherapy with traditional antifungals is often insufficient, and so combination therapies are explored to enhance treatment efficacy. However, systemic combination treatments can result in severe adverse effects, suggesting the need for localised delivery systems, such as drug-loaded electrospun patches, to administer antifungals directly to the infection site. This proof-of-concept study hypothesised that dual amorolfine and terbinafine therapy slowly releasing from electrospun patches would be an effective way of eradicating Candida albicans when the patch was applied directly to the fungal colony. The feasibility of creating electrospun materials loaded with amorolfine and terbinafine for combination antifungal therapy was investigated. Electrospinning was used to fabricate polycaprolactone (PCL) patches with varying drug loadings (2.5%, 5%, and 10% w/w) of amorolfine and terbinafine either individually or in combination. The incorporation of both drugs in the fibres was confirmed, with the drugs predominantly in an amorphous state. Results showed that combination therapy patches had a significantly greater and prolonged antifungal effect compared to monotherapy patches, with larger zones of inhibition and sustained efficacy over at least 7 days. This study therefore demonstrates that PCL-based electrospun patches containing amorolfine and terbinafine provide superior antifungal activity against C. albicans compared to monotherapy patches. This approach could lower required drug doses, reducing adverse effects, and enhance patient compliance due to prolonged drug release, leading to more effective antifungal therapy.
Introduction
Fungal infections are generally neglected both in the clinic and academic research, despite their high global impact (https://gaffi.org/). The US Department of Defence identifies combat wound infections due to invasive fungi as a high priority emerging threat (Turnbull et al., 2021). Candida species are the most prevalent in the wound mycobiome while species belonging to the Curvularia, Malessezia, Aureobasidium, Cladosporium, Ulocladium, Engodontium and Trichtophyton genera can also be found (Dowd et al., 2011). Monotherapy with traditional antifungal agents is often inefficient (Baran et al., 2007), and combination therapies are therefore being explored to provide additive or synergistic antifungal effects (Zhu et al., 2023). However, effective, long-term systemic combination therapy with antifungals can lead to adverse effects. There is therefore a strong need for localised delivery systems administering antifungal therapy directly to the site of action, for example, through a drug-loaded wound dressing. Such dressings can be prepared using electrospinning, a fabrication method which allows for the formation of drug-loaded fibrous materials with appropriate handling properties for implants and wound dressings. Electrospinning of drug-loaded patches has been extensively described for a variety of healthcare applications (Dziemidowicz et al., 2021a; Kellaway et al., 2024), with recent focus on antimicrobial treatments (Akombaetwa et al., 2022; Zhang et al., 2024). While there are published examples of electrospun materials with antifungal properties, such as fluconazole-loaded mucoadhesive patches for vaginal candidiasis (Sharma et al., 2016) or poly (lactic acid)-based carvacrol-releasing membranes (Scaffaro et al., 2018), the field remains largely unexplored.
This study therefore explores the feasibility of fabricating electrospun materials loaded with two therapeutic agents (amorolfine and terbinafine) for antifungal combination therapy. These candidates were incorporated into poly (ε-caprolactone) (PCL) electrospun fibres, either alone or in combination, and their antifungal activity assessed in an extended disk diffusion assay against the pathogen most commonly found in fungal wound infections, Candida albicans. To the best of our knowledge, this is the first report of the development of electrospun fibres as carriers for antifungal drug combinations.
Experimental
Materials and methods
Preparation of electrospun patches. PCL fibres containing no drug (Blank) were prepared by electrospinning a solution of 12% w/v PCL (Mw ∼80 kDa; Sigma-Aldrich) in 2,2,2-trifluoroethanol (TFE; Sigma-Aldrich). This concentration was selected based on the literature and our previous experience of working with PCL (Dziemidowicz et al., 2021b; Dziemidowicz et al., 2023). Solutions were carefully loaded into 5 mL disposable syringes and dispensed through a 21G stainless steel needle connected to a high-voltage direct-current power supply (HCP 14-20000, FuG Elektronik GmbH). Drug-loaded electrospinning solutions were prepared by adding antifungals (amorolfine, terbinafine or a 1:1 w/w mixture of both) directly to the polymer solution and stirring for another 60 min prior to electrospinning. The electrospinning solutions were dispensed at a flow rate of 1.8 and 1.3 mL/h for blank and drug-loaded formulations, respectively. The fibres were collected on baking paper placed on top of a 14.7 cm × 20 cm metal plate collector connected to the grounded electrode. The distance from the needle to the collector was 14 cm. The experiments were conducted at 25°C ± 2°C and relative humidity 35% ± 10%. Full details of the processing parameters are given in Table 1.
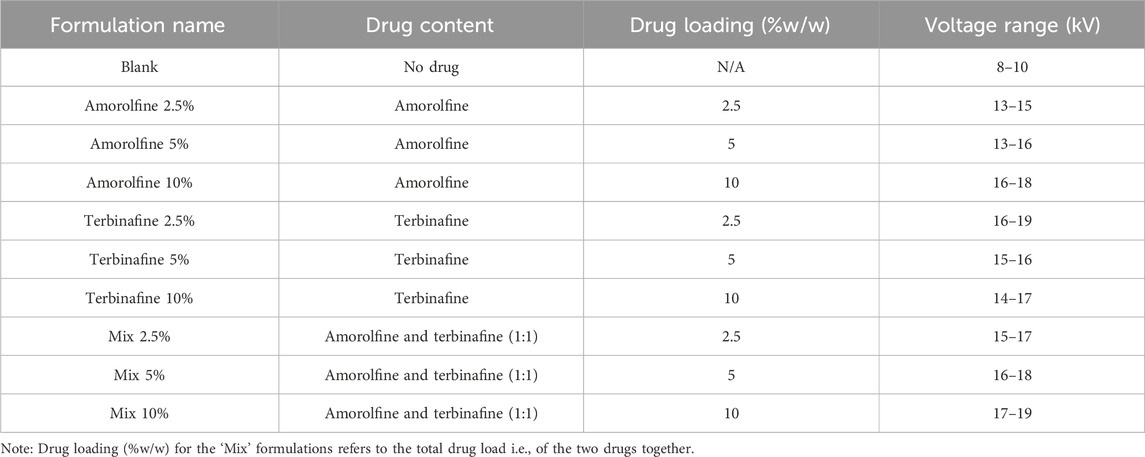
Table 1. Summary of formulation composition and electrospinning parameters used to fabricate antifungal patches.
The fibres were then analysed by scanning electron microscopy (SEM), Fourier-transform infrared (FTIR), X-ray diffraction (XRD), and differential scanning calorimetry (DSC) and thermogravimetric analysis (TGA). Full experimental details as well as characterisation data for monotherapy fibres are provided in the Supplementary Material.
Antifungal testing
Candida albicans (C. albicans) cultured on Sabouraud Dextrose Agar (SDA) plates was used to prepare the fungal inoculum. The colonies were swabbed using a sterile inoculating loop and suspended into 5 mL of 0.85% w/v saline in a sterile vial. The absorbance of the suspension was measured using a UV-visible spectrometer (Biochrom Libra S12) at a wavelength of 530 nm and adjusted by either adding additional saline or fungal colonies until the suspension turbidity was in compliance with a 0.5 McFarland standard measured at the same wavelength.
The duration and potency of the antifungal effect of the electrospun patches was established using the disc diffusion method. The electrospun patches were cut into discs (6 mm in diameter) using a metal hole punch and sterilised using UV irradiation within a laminar flow hood for 20 min on both sides. Once the fungal inoculum was spread on the SDA plate, electrospun patch discs were transferred onto the dish using sterile tweezers and gently pressed down. For each plate used, a blank formulation (no drug loaded) was used as a negative control. The plates were then incubated at 32°C for 24 h, after which a photograph of each plate was taken. The electrospun discs were then moved onto a freshly inoculated plate each day to test the duration of drug release and the antifungal effect of the formulations. Using a ruler the zone of inhibition (ZOI) was measured by placing the end of the ruler at the centre of the drug-loaded disc and reading the distance at which the first fungal colonies on the plate were observed. Each formulation was tested in triplicate, and the results are presented as mean ± SD.
Data were statistically analysed using two-way analysis of variance (ANOVA) followed by Tukey’s multiple comparisons test and t-tests. Differences were considered significant when p < 0.05. GraphPad Prism version 9.5.00 (GraphPad Software, United States) was used for statistical analysis.
Results and discussion
A simple method of blend electrospinning was selected to facilitate future scaled-up manufacture. In this approach, each drug individually or a 1:1 drug mixture was directly added into a polymer solution containing organic solvent. Owing to its well-described biodegradability, biocompatibility and favourable mechanical properties, PCL was chosen as the polymer carrier. As a hydrophobic polymer with slow degradation rate, PCL would be a suitable choice for an extended-release delivery system of poorly soluble drugs such as amorolfine and terbinafine. Moreover, both drugs and the polymer dissolve readily in the electrospinning solvent (TFE), ensuring appropriate physicochemical stability of the electrospinning solution throughout the fabrication process. The electrospinning of the polymer-drug solutions was stable, required minimal optimisation and resulted in the formation of uniform, defect-free, cylindrical fibres (Figure 1A; Supplementary Figure S1.
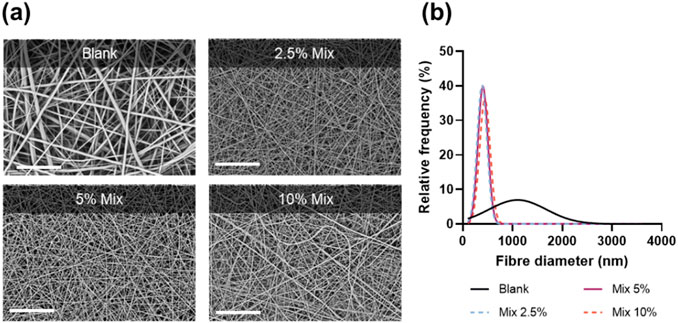
Figure 1. Scanning electron micrographs (A); and fibre diameter size distribution curves (B) for unloaded PCL fibres (blank) and combination therapy patches at different drug loadings (Mix 2.5%, 5%, and 10%). Scale bar = 80 µm.
Fibre diameter measurements (Figure 1B) showed a drastic decrease in fibre diameter upon addition of the drugs, from 1235 ± 639 nm (Blank) to 395 ± 92 nm (Mix 2.5%), 423 ± 128 nm (Mix 5%) and 462 ± 140 nm (Mix 10%). A lower diameter for drug-loaded electrospun fibres is a common phenomenon often attributed to increased conductivity of drug-polymer solutions (Zeng et al., 2003; Zamani et al., 2010; Krogstad and Woodrow, 2014).
Monoaxial electrospinning of drug-polymer blends is particularly attractive for the processing of poorly soluble crystalline materials as it typically leads to the creation of amorphous solid dispersions (ASD), therefore enhancing bioavailability. FTIR spectra (Figure 2A; Supplementary Figure S2) were acquired to investigate the chemical composition of the fabricated formulations.
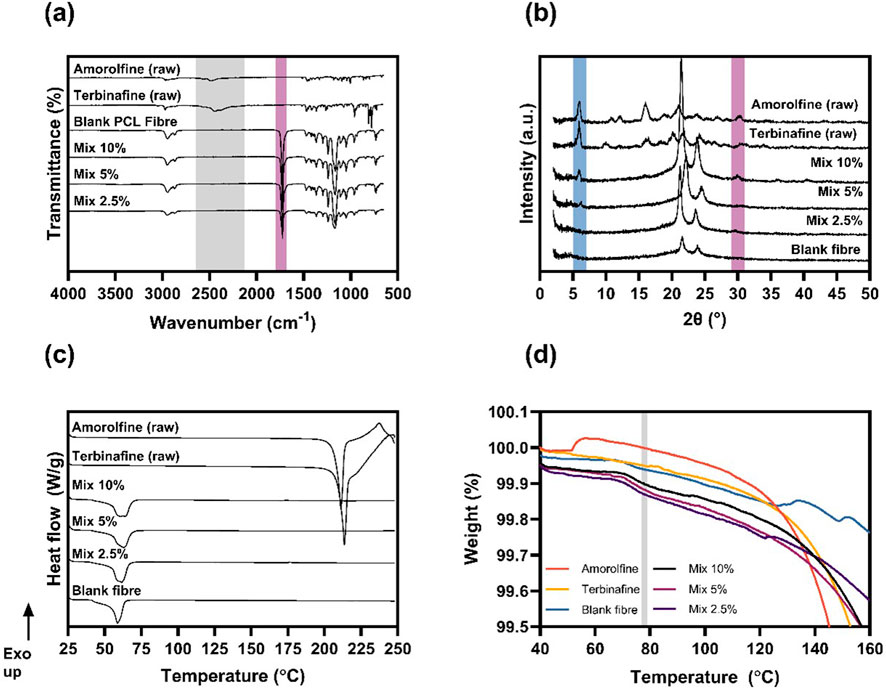
Figure 2. Physicochemical characterisation. FTIR (A), XRD (B) and DSC (C) data confirm the mostly amorphous nature of amorolfine and terbinafine within the electrospun patches. The TGA analysis (D) around the boiling point of TFE (shaded in grey) showed no residual solvent present in electrospun patches.
In amorolfine raw material, the characteristic NH+ stretching vibration of the nitrogen within the morpholine ring is present at around 2480 and 2560 cm−1. Similarly, terbinafine raw material exhibits an amine peak at 2448 cm−1 (Kuminek et al., 2013). The FTIR spectrum of the blank fibres is identical to that of previously reported raw PCL (Chiu et al., 2020). In the drug-loaded formulations, neither amorolfine nor terbinafine peaks were observed. In XRD (Figures 2B, Supplementary Figure S3, S4), sharp Bragg reflections observed for the amorolfine and terbinafine raw materials suggest a crystalline structure of both active ingredients (Thatai and Sapra, 2018). Blank fibres containing only PCL have two broad Bragg reflections, consistent with its nature as a semi-crystalline polymer (Chiu et al., 2020). While the XRD patterns of formulations with the lowest drug loading (Mix 2.5%) resembled that of the blank formulation, a small reflection at 5.9° and a broad peak at 29.8° were detected with Mix 5% and Mix 10%, indicating a small amount of crystalline drug in the fibres. A similar observation was made for terbinafine monotherapy patches (Supplementary Figure S3). DSC analysis (Figure 2C; Supplementary Figure S5) shows sharp melting endotherms for the raw drugs (at 210°C for amorolfine and 213°C for terbinafine), consistent with the literature (Thatai and Sapra, 2018; Kerai et al., 2015; Abd-Elmonem et al., 2023) and confirming a crystalline structure. In both cases, this is followed by an exotherm likely arising from drug degradation. Blank PCL fibres also show a melt, at ca. 60°C. The PCL melt is clearly visible in the drug-loaded fibres (both mono- and mixed), but the distinct drug melting endotherms were not present in any of the fibre formulations, confirming the mostly amorphous nature of the drug-loaded electrospun patches. Additionally, TGA analysis (Figure 2D) was performed to investigate whether residual solvent was present in the materials post-fabrication. The unchanged mass in the TGA curve at 78°C (boiling point of TFE) suggests that the solvent had completely evaporated during the electrospinning process.
Antifungal performance was assessed against C. albicans, one of the most prevalent fungi in chronic wounds (Dowd et al., 2011; Chellan et al., 2010). Monotherapy patches containing amorolfine or terbinafine only were used as controls. While amorolfine-only patches showed some antifungal activity, terbinafine-only patches were generally ineffective against C. albicans, reflecting the known lower activity of terbinafine and previous literature reporting no growth inhibition in C. albicans upon treatment with terbinafine-loaded PCL/gelatin fibres (Paskiabi et al., 2017). In contrast, the Mix patches were very effective and clear ZOIs were seen for the Mix formulations at all loadings (2.5%, 5%, 10%) (Figure 3; Supplementary Tables S1-S3), suggesting a potential additive or synergistic effect of amorolfine and terbinafine. This agrees with a recent study by Schaller et al., who explored these two agents in combination against pathogenic fungi in onychomycosis and confirmed their additive effect against T. rubrum as well as T. interdigitale, encouraging further exploration of electrospun antifungal combination patches for applications in fungal nail infections.
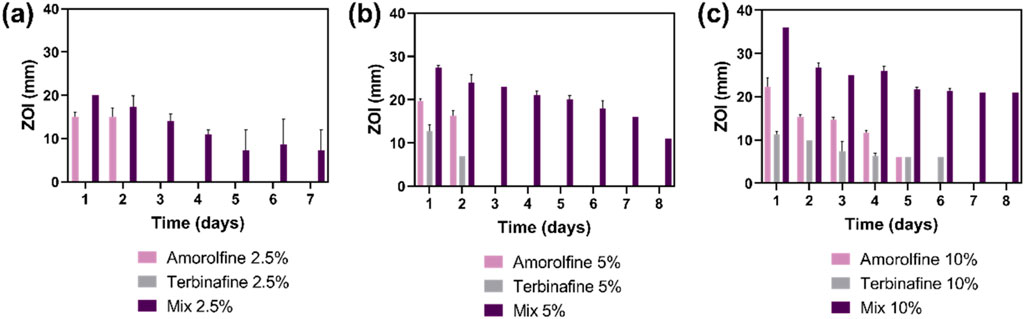
Figure 3. Antifungal effect of electrospun patches presented as zone of inhibition (ZOI) of C. albicans growth in a disc diffusion test. The drug combination formulations gave a larger ZOI occurring over a longer period of time following transfer of patches to freshly fungus-inoculated agar plates, showing a more potent antifungal effect than single-drug formulations (amorolfine and terbinafine) at all tested concentrations (2.5% (A), 5% (B), 10% (C) w/w drug: polymer). Sustained drug release is evidenced by prolonged antifungal effects visible over at least 8 days. Two-way ANOVA showed a significant difference between monotherapy (either amorolfine or terbinafine) and combination therapy (Mix) for 5% and 10% drug loadings at all tested timepoints (p < 0.05). Data are presented as mean ± SD, n = 3.
The ZOI could be correlated to the concentration of terbinafine/amorolfine, where Mix 10% achieved the highest ZOI, followed by Mix 5% and Mix 2.5%. For higher drug concentrations (5% and 10%), the combination patches showed significantly (p < 0.05) higher ZOIs than for monotherapy at all tested timepoints. Combination patches also showed prolonged antifungal effect over at least 7 days.
Conclusion
Combination antifungal PCL-based patches containing both amorolfine and terbinafine were fabricated using blend electrospinning. Morphological and physicochemical characterisation confirmed successful incorporation of both drugs within the fibre meshes, and their predominantly amorphous state in the fibres. The effect of combination therapy with amorolfine and terbinafine was demonstrated against C. albicans, where combination formulations achieved greater and prolonged inhibition of fungal growth over at least 7 days compared to single drug-loaded patches containing the same total drug concentration. In practice, enhanced antifungal activity could enable the use of lower drug doses, reducing/eliminating adverse effects, while prolonged antifungal activity would reduce the frequency of medicine administration, which could lead to improved patient compliance and thereby to more successful therapy.
Data availability statement
The raw data supporting the conclusions of this article will be made available by the authors, without undue reservation.
Author contributions
KD: Data curation, Formal Analysis, Investigation, Methodology, Supervision, Validation, Visualization, Writing–original draft, Writing–review and editing. MM: Writing–review and editing, Data curation, Formal Analysis, Investigation, Methodology. JP: Writing–review and editing, Data curation, Investigation, Methodology. MA: Investigation, Writing–review and editing. GW: Conceptualization, Funding acquisition, Project administration, Resources, Supervision, Writing–review and editing. SM: Conceptualization, Funding acquisition, Project administration, Resources, Supervision, Writing–review and editing.
Funding
The author(s) declare that no financial support was received for the research, authorship, and/or publication of this article.
Acknowledgments
KD thanks the EPSRC for a Doctoral Prize Fellowship (EP/T517793/1). MM was a UCL Laidlaw scholar. JP thanks 2018 British Skin Foundation Research Awards (032/S/18-BSF) for a PhD studentship. MA thanks the EPSRC for a PhD studentship in the Centre for Doctoral Training in Transformative Pharmaceutical Technologies (EP/S023054/1).
Conflict of interest
The authors declare that the research was conducted in the absence of any commercial or financial relationships that could be construed as a potential conflict of interest.
The author(s) declared that they were an editorial board member of Frontiers, at the time of submission. This had no impact on the peer review process and the final decision.
Publisher’s note
All claims expressed in this article are solely those of the authors and do not necessarily represent those of their affiliated organizations, or those of the publisher, the editors and the reviewers. Any product that may be evaluated in this article, or claim that may be made by its manufacturer, is not guaranteed or endorsed by the publisher.
Supplementary material
The Supplementary Material for this article can be found online at: https://www.frontiersin.org/articles/10.3389/fddev.2024.1458009/full#supplementary-material
References
Abd-Elmonem, E. M., Makky, A. M. A., Antar, A., Abd-Elsalam, W. H., and Khalil, I. A. (2023). Corneal targeted amorolfine HCl-mixed micelles for the management of ocular candidiasis: preparation, in vitro characterization, ex vivo and in vivo assessments. J. Drug Deliv. Sci. Technol. 85, 104614. doi:10.1016/j.jddst.2023.104614
Akombaetwa, N., Bwanga, A., Makoni, P. A., and Witika, B. A. (2022). Applications of electrospun drug-eluting nanofibers in wound healing: current and future perspectives. Polym. (Basel) 14 (14), 2931. doi:10.3390/polym14142931
Baran, R., Sigurgeirsson, B., Berker, D. de, Kaufmann, R., Lecha, M., Faergemann, J., et al. (2007). A multicentre, randomized, controlled study of the efficacy, safety and cost-effectiveness of a combination therapy with amorolfine nail lacquer and oral terbinafine compared with oral terbinafine alone for the treatment of onychomycosis with matrix involvement. Br. J. Dermatology 157 (1), 149–157. doi:10.1111/j.1365-2133.2007.07974.x
Chellan, G., Shivaprakash, S., Ramaiyar, S. K., Varma, A. K., Varma, N., Sukumaran, M. T., et al. (2010). Spectrum and prevalence of fungi infecting deep tissues of lower-limb wounds in patients with type 2 diabetes. J. Clin. Microbiol. 48 (6), 2097–2102. doi:10.1128/JCM.02035-09
Chiu, Y.-J., Zhang, Z., Dziemidowicz, K., Nikoletopoulos, C.-G., Angkawinitwong, U., Chen, J.-T., et al. (2020). The effect of solvent vapor annealing on drug-loaded electrospun polymer fibers. Pharmaceutics 12, 139. doi:10.3390/pharmaceutics12020139
Dowd, S. E., Delton Hanson, J., Rees, E., Wolcott, R. D., Zischau, A. M., Sun, Y., et al. (2011). Survey of fungi and yeast in polymicrobial infections in chronic wounds. J. Wound Care 20 (1), 40–47. doi:10.12968/jowc.2011.20.1.40
Dziemidowicz, K., Brocchini, S., and Williams, G. R. A. (2021b). A simple route to functionalising electrospun polymer scaffolds with surface biomolecules. Int. J. Pharm. 597, 120231. doi:10.1016/j.ijpharm.2021.120231
Dziemidowicz, K., Kellaway, S. C., Guillemot-Legris, O., Matar, O., Trindade, R. P., Roberton, V. H., et al. (2023). Development of ibuprofen-loaded electrospun materials suitable for surgical implantation in peripheral nerve injury. Biomater. Adv. 154, 213623. doi:10.1016/j.bioadv.2023.213623
Dziemidowicz, K., Sang, Q., Wu, J., Zhang, Z., Zhou, F., Lagaron, J. M., et al. (2021a). Electrospinning for healthcare: recent advancements. J. Mater Chem. B 9, 939–951. doi:10.1039/D0TB02124E
Kellaway, S. C., Ullrich, M. M., and Dziemidowicz, K. (2024). Electrospun drug-loaded scaffolds for nervous system repair. WIREs Nanomedicine Nanobiotechnology 16 (3), e1965. doi:10.1002/wnan.1965
Kerai, L. V., Hilton, S., and Murdan, S. (2015). UV-curable gel formulations: potential drug carriers for the topical treatment of nail diseases. Int. J. Pharm. 492 (1), 177–190. doi:10.1016/j.ijpharm.2015.07.020
Krogstad, E. A., and Woodrow, K. A. (2014). Manufacturing scale-up of electrospun poly(vinyl alcohol) fibers containing tenofovir for vaginal drug delivery. Int. J. Pharm. 475 (1), 282–291. doi:10.1016/j.ijpharm.2014.08.039
Kuminek, G., Rauber, G. S., Riekes, M. K., Campos, C. E. M. de, Monti, G. A., Bortoluzzi, A. J., et al. (2013). Single crystal structure, solid state characterization and dissolution rate of terbinafine hydrochloride. J. Pharm. Biomed. Anal. 78–79, 105–111. doi:10.1016/j.jpba.2013.02.001
Paskiabi, F. A., Bonakdar, S., Shokrgozar, M. A., Imani, M., Jahanshiri, Z., Shams-Ghahfarokhi, M., et al. (2017). Terbinafine-loaded wound dressing for chronic superficial fungal infections. Mater. Sci. Eng. C 73, 130–136. doi:10.1016/j.msec.2016.12.078
Scaffaro, R., Lopresti, F., D’Arrigo, M., Marino, A., and Nostro, A. (2018). Efficacy of poly(lactic acid)/carvacrol electrospun membranes against Staphylococcus aureus and Candida albicans in single and mixed cultures. Appl. Microbiol. Biotechnol. 102 (9), 4171–4181. doi:10.1007/s00253-018-8879-7
Sharma, R., Garg, T., Goyal, A. K., and Rath, G. (2016). Development, optimization and evaluation of polymeric electrospun nanofiber: a tool for local delivery of fluconazole for management of vaginal candidiasis. Artif. Cells Nanomed Biotechnol. 44 (2), 524–531. doi:10.3109/21691401.2014.966194
Thatai, P., and Sapra, B. (2018). Terbinafine hydrochloride nail lacquer for the management of onychomycosis: formulation, characterization and in vitro evaluation. Ther. Deliv. 9 (2), 99–119. doi:10.4155/tde-2017-0069
Turnbull, I. R., Mazer, M. B., Hoofnagle, M. H., Kirby, J. P., Leonard, J. M., Mejia-Chew, C., et al. (2021). IL-7 immunotherapy in a nonimmunocompromised patient with intractable fungal wound sepsis. Open Forum Infect. Dis. 8 (6), ofab256. doi:10.1093/ofid/ofab256
Zamani, M., Morshed, M., Varshosaz, J., and Jannesari, M. (2010). Controlled release of metronidazole benzoate from poly ε-caprolactone electrospun nanofibers for periodontal diseases. Eur. J. Pharm. Biopharm. 75 (2), 179–185. doi:10.1016/j.ejpb.2010.02.002
Zeng, J., Xu, X., Chen, X., Liang, Q., Bian, X., Yang, L., et al. (2003). Biodegradable electrospun fibers for drug delivery. J. Control. Release 92 (3), 227–231. doi:10.1016/S0168-3659(03)00372-9
Zhang, F., Jacobs, A. I., Woodall, M., Hailes, H. C., Uchegbu, I. F., Fernandez-Reyes, D., et al. (2024). A one-step method for generating antimicrobial nanofibre meshes via coaxial electrospinning. Mater Adv. 5 (13), 5561–5571. doi:10.1039/D4MA00125G
Keywords: electrospinning, patch, drug combination, fungus, infection
Citation: Dziemidowicz K, Meszarik M, Piovesan J, Almatroudi MA, Williams GR and Murdan S (2024) Electrospun patches to deliver combination drug therapy for fungal infections. Front. Drug Deliv. 4:1458009. doi: 10.3389/fddev.2024.1458009
Received: 01 July 2024; Accepted: 20 August 2024;
Published: 16 September 2024.
Edited by:
Khushwant Yadav, SVKM’s Narsee Monjee Institute of Management Studies, IndiaReviewed by:
Franklin Chimaobi Kenechukwu, University of Nigeria, Nsukka, NigeriaS. N. Koteswara Rao G., SVKM’s Narsee Monjee Institute of Management Studies, India
Copyright © 2024 Dziemidowicz, Meszarik, Piovesan, Almatroudi, Williams and Murdan. This is an open-access article distributed under the terms of the Creative Commons Attribution License (CC BY). The use, distribution or reproduction in other forums is permitted, provided the original author(s) and the copyright owner(s) are credited and that the original publication in this journal is cited, in accordance with accepted academic practice. No use, distribution or reproduction is permitted which does not comply with these terms.
*Correspondence: Sudaxshina Murdan, cy5tdXJkYW5AdWNsLmFjLnVr