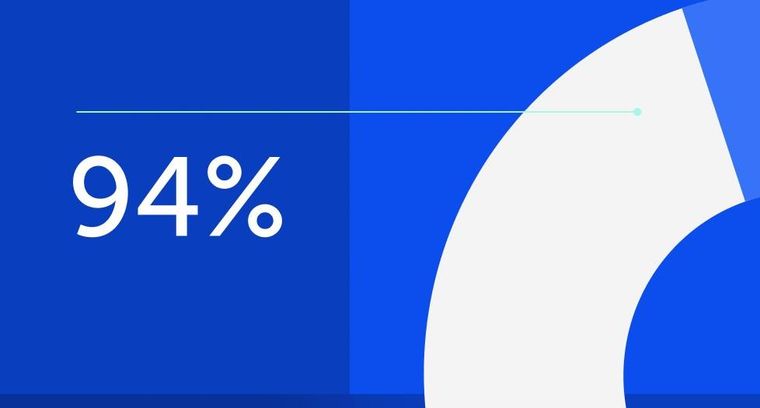
94% of researchers rate our articles as excellent or good
Learn more about the work of our research integrity team to safeguard the quality of each article we publish.
Find out more
ORIGINAL RESEARCH article
Front. Drug Deliv., 22 July 2022
Sec. CNS Drug Delivery
Volume 2 - 2022 | https://doi.org/10.3389/fddev.2022.932576
This article is part of the Research TopicMechanisms of Blood-brain Barrier Transport and Tight Junction RegulationView all 4 articles
Breast cancer resistance protein (BCRP) is expressed by brain capillary endothelial cells and at the interface between two placental syncytiotrophoblast layers in rodents and serves to suppress drug distribution to the brain and the fetus. The purpose of the present study is to determine and compare the apparent impact of a single BCRP molecule on drug transfer between the maternal blood-brain barrier and placental barrier in pregnant mice at different gestation ages. BCRP protein was quantified by liquid chromatography-tandem mass spectrometry. Genistein or dantrolene was continuously administered to pregnant Bcrp−/− or wild-type (WT) mice, and the brain-to-plasma concentration ratio in the mother (Kp,brain) and the fetal-to-maternal ratio of plasma concentrations (Kp,fp) were determined. At gestational day 15.5 (GD15.5), the protein amount of BCRP at the murine placental barrier was estimated to be approximately three times higher than at the maternal blood-brain barrier, but the levels were approximately the same at GD17.5 due to the decline of placental BCRP expression during gestation. On the other hand, the values of Bcrp−/−/WT ratio of Kp,brain for genistein and dantrolene were 6.1 and 3.8, respectively, while the Kp,fp ratios were all less than 2.0. These results indicate that the apparent impact of a single placental BCRP molecule on the restriction of drug distribution is much less than that of a single brain BCRP molecule, probably because the function of placental BCRP is attenuated by bypass transfer through the connexin26 gap junctions between adjacent syncytiotrophoblast layers. The present study also found that the expression amount of BCRP protein at the human placental barrier formed by the monolayer of syncytiotrophoblasts was lower than that in mice, but this species difference appears to be functionally compensated by the murine-specific bypass route through gap junctions, at least in part.
Brain capillary endothelial cells and placental syncytiotrophoblasts (SynT) form the blood-brain and placental barriers to limit drug distribution to the brain and fetus, respectively, by expressing breast cancer resistance protein (BCRP)/ABCG2 (Ohtsuki and Terasaki, 2007; Han et al., 2018), together with multidrug resistance protein 1 (MDR1)/P-glycoprotein/ABCB1, as an efflux pump. Though challenging, prediction of the impact of BCRP on drug distribution to the brain and fetus is important, since BCRP activity is closely associated with the pharmacological actions and toxic effects of drugs in these regions. One approach is to extrapolate the impact of BCRP on drug distribution at a particular site (e.g., the human placental barrier) from available data on BCRP-mediated transport rate at other sites (e.g., the rodent placental barrier, the blood-brain barrier, and in BCRP-overexpressing cells) by correcting for differences in BCRP protein amounts. This approach is especially useful in cases where direct measurement of the impact of BCRP on drug distribution is virtually impossible (Liu et al., 2017). To achieve precise prediction, it is necessary to determine whether the functional impact per single BCRP molecule is equal among various sites expressing BCRP. However, the impact per single BCRP molecule on the brain distribution of BCRP and MDR1 co-substrates is apparently diminished compared with BCRP-overexpressing cells, since the two transporters compensate for each other when the function of one is impaired (Kodaira et al., 2010). It is also necessary to establish whether other factors at the blood-brain or placental barrier also significantly affect the impact of BCRP.
BCRP proteins at the blood-brain and the placental barriers in humans are exposed to the systemic circulation, i.e., the luminal membrane of capillary endothelial cells and the apical microvillous membrane (MVM) of the multinucleated SynT monolayer, respectively (Allikmets et al., 1998; Maliepaard et al., 2001; Zhang et al., 2003). However, the anatomic structure of the chorioallantoic placenta in eutherian mammals varies among animal species (Furukawa et al., 2014), and this is expected to affect the validity of extrapolation of the embryo-fetal developmental toxicity of drugs observed in rodents to humans. The rodent SynT consists of a bilayer, with a maternal-facing layer I (SynT-I) and a fetal-facing layer II (SynT-II) (Coan et al., 2005). We have reported that MDR1 and BCRP proteins in rodent placenta are localized at the apical membrane of the SynT-II layer (Akashi et al., 2016), meaning that these proteins are not directly exposed to the maternal circulation. Regarding the effect of this unique localization of efflux transporters in rodent placenta on fetal drug distribution, we have recently shown that murine placental MDR1 has a minimal influence on the fetal distribution of digoxin, compared with the brain distribution (Fujita et al., 2022). This is probably due to the presence of a bi-directional transfer process through connexin26 gap junctions between SynT-I and SynT-II, which would counter the uni-directional transport by MDR1 from fetal to maternal side. Although the role of rodent placental BCRP in limiting the fetal distribution of substrates such as genistein, nitrofurantoin, and glyburide was confirmed by in vivo studies using Bcrp−/− mice (Enokizono et al., 2007; Zhang et al., 2007; Zhou et al., 2008), the 80% increase in the fetus-to-maternal plasma concentration ratio of genistein in Bcrp−/− mice was much smaller than the 820% increase in the brain-to-plasma concentration ratio (Enokizono et al., 2007). These observations lead to the hypothesis that connexin26 gap junctions also mediate the bi-directional transfer of BCRP substrates between SynT-I and SynT-II, thus diminishing the apparent impact per single BCRP molecule, which would invalidate simple scaling between the blood-brain and the placental barriers in rodents and between the human and rodent placental barriers.
Of course, it is also possible that the smaller effect of BCRP at the murine placenta barrier compared with the blood-brain barrier is attributable to a difference in BCRP protein amounts. The absolute BCRP protein amount has already been determined at the murine blood-brain barrier (Kamiie et al., 2008), but not so far at the murine placental barrier. When quantifying the placental amount of BCRP, the gestational age-dependent expression must be taken into consideration, though this remains controversial; one study showed that the mRNA and protein expression levels of BCRP in murine placenta peak at gestation day (GD) 15.5 (Wang et al., 2006), while another study found no significant change during gestation (Kalabis et al., 2007). The correlation between the absolute amount and the transport activity of BCRP protein should be examined by measuring them at different gestation times. This would also be helpful for estimating the impact of BCRP on the fetal distribution of drugs from the protein amount.
The purpose of the present study is to determine and compare the apparent impact of a single BCRP molecule on the restriction of drug distribution between the blood-brain barrier and the placental barrier in pregnant mice at different gestational ages. The apparent impact per single BCRP molecule at these barriers was calculated as the change in the distributions of dantrolene and genistein, which are BCRP substrates but not MDR1 substrates (Enokizono et al., 2007, 2008), in Bcrp−/− mice divided by the BCRP protein amount.
Uncomplicated term human placental tissues were obtained with written informed consent and with the approval of the Institutional Ethics Committee of Keio University Faculty of Pharmacy (150421–2) and Keio University School of Medicine (20110250). Human placentas were collected from 4 pregnant women who had undergone elective cesarean section due to previous cesarean section or breech presentation and were 40 ± 2 years old (mean ± S.D.) and gestational age of 37 ± 1 week (mean ± S.D.) at the date of elective cesarean section. The placental weight was 550 ± 108 g, (mean ± S.D.) and three of the four neonates were male. Human placental MVM-enriched fraction was prepared by magnesium precipitation; the activity of alkaline phosphatase, an MVM marker, in human MVMs was enriched more than 10-fold (n = 4) relative to the villous homogenate, as previously reported (Fujita et al., 2022).
Bcrp−/− and wild-type (WT) FVB mice were purchased from Taconic (Hudson, NY, United States) and CLEA Japan (Tokyo, Japan), respectively. Mice were maintained under a 12 h/12 h light-dark cycle at 25°C with free access to water and food until use. Female WT and Bcrp−/− mice were mated with male mice of the same genotype. The presence of a vaginal plug was designated as GD0.5. Bcrp−/− mice were born at the expected Mendelian ratio, and they did not demonstrate phenotypic aberrations under standard housing conditions (Jonker et al., 2002). Animal experiments were approved by the Institutional Animal Care Committee (12040) and complied with the standards set out in the Guideline for the Care and Use of Laboratory Animals at Keio University.
Plasma membrane fractions were prepared from the murine placental labyrinth as reported previously (Fujita et al., 2022). Briefly, all placentas within a litter were isolated from pregnant GD15.5 and 17.5 WT and GD17.5 Bcrp−/− mice to purify one plasma membrane-enriched fraction of the placental labyrinth. Homogenates of the placental labyrinth were centrifuged at 10,000 g, and then supernatants were ultra-centrifuged at 124,000 g. Pellets were suspended and layered on top of 38% (w/v) sucrose solution and centrifuged at 10,000 g with a swing-out rotor. The turbid layer at the interface was recovered, suspended, and centrifuged at 10,000 g. The resultant pellets were obtained as a plasma membrane fraction and stored at −80°C until use. Protein concentrations were measured by the Bradford method using Protein Assay reagent (Bio-Rad, Hercules, CA, United States) with bovine serum albumin as a standard.
The expression amounts of transporter proteins were measured by quantifying the absolute amounts of specific (ST) peptides generated from the target transporter proteins using liquid chromatography-tandem mass spectrometry (LC-MS/MS) as described previously (Fujita et al., 2022). The peptide sequence for human BCRP (NP_001244315.1) and murine BCRP (NP_001342406.1) proteins is SSLLDVL*AAR (Uchida et al., 2013). The asterisk (*) indicates the amino acid labeled with 13C and 15N in the internal standard (IS). Briefly, the membrane fractions (100 µg protein) were reduced with dithiothreitol, alkylated with iodoacetamide, and precipitated with methanol and chloroform. The precipitates were dissolved in urea and treated first with lysyl endopeptidase and ProteaseMAX surfactant (Promega, Madison, WI, United States) and secondary N-tosyl-L-phenylalanine chloromethyl ketone-treated trypsin (Promega). The resulting peptide samples were spiked with IS and acidified with formic acid.
The LC-MS/MS system consisted of a high-performance liquid chromatography instrument and an electrospray ionization triple quadrupole mass spectrometer (LCMS-8050; Shimadzu, Kyoto, Japan) operated in the positive ionization mode. Samples were injected into the LC system equipped with an XBridge BEH130 C18 column (1 mm I.D. × 100 mm, 3.5 µm, Waters, Milford, MA, United States) at 40°C and eluted with a linear gradient of acetonitrile containing 0.1% formic acid. MS spectrometric detection was performed by multiplexed selected reaction monitoring (SRM) using 3 sets of transitions (m/z) of precursor and product ions (Q1/Q3: 522.8/644.4, 522.8/529.3, and 522.8/288.2 for ST, 526.3/651.4, 526.3/536.3, and 526.3/288.2 for IS) with the dwell time of 10 msec per transition. A peak was defined as positive when the signal-to-noise ratio of peak intensity was over 3. The amount of the peptide in the sample was first determined for each transition using the peak area ratio (ST/IS) of the positive peak and a calibration curve obtained with known concentrations of a synthetic peptide and was subsequently expressed as the average of 3 positive peaks from different transitions, as presented in Supplementary Table S1.
The femoral vein of pregnant mice at GD15.5 and 17.5 was cannulated with polyethylene tubing (PE-10; BD Biosciences, San Jose, CA, United States) under deep anesthesia with isoflurane. Genistein and dantrolene were administered via the cannula by continuous infusion using a syringe pump (Kd Scientific, Holliston, MA, United States) with a pumping rate of 160 and 70 μl/h, respectively, for 2 h. Syringe pumps were filled with genistein (12.5 mM in DMSO: Tokyo Chemical Industry, Tokyo, Japan) diluted 10 times with 2 mM NaOH in saline or with dantrolene (5.0 mM in DMSO: Fujifilm Wako, Osaka, Japan) diluted 10 times with a mixed solvent of PEG-400 and DMSO (1:1). Maternal blood was intermittently collected from the left jugular vein in experiments to obtain the maternal plasma concentration-time profile.
Dantrolene was also continuously administered to pregnant Bcrp−/− or WT mice from GD14.5 for 24 h using an osmotic pump (Alzet model 2001D, 8 μl/h for 24 h: Durect, Cupertino, CA, United States). The osmotic pump was filled with 7.5 mM dantrolene in propylene glycol and connected with PE10 polyethylene tubing. The osmotic pump was cannulated to the right jugular vein and implanted in the back subcutaneously under deep anesthesia with isoflurane. Maternal blood was collected from a tail vein to determine the maternal plasma concentration during the continuous administration.
At the end of infusion, the maternal blood was collected from the left jugular vein and all fetuses within a litter were decapitated for collection of the fetal blood in an EDTA-treated microhematocrit capillary tube. The cerebrum of the mother was collected and weighed.
Blood was centrifuged at 15,000 rpm for 20 min to obtain plasma. The fetal plasma samples obtained from each litter were pooled in one tube and counted as one sample. The cerebrum was homogenized with water. For genistein and dantrolene determination, the plasma and brain homogenate was diluted with acetonitrile and spiked with IS (daidzein for genistein determination and nitrofurazone for dantrolene determination). The samples were centrifuged at 15,000 g for 10 min at 4°C. The supernatant was evaporated in a vacuum centrifuge. The residue was reconstituted in 0.1% formic acid in methanol for genistein determination or in 0.1% formic acid in acetonitrile with 2 mM ammonium acetate for dantrolene determination and centrifuged at 15,000 g for 1 min at 4°C. Supernatant samples were used for quantification.
The n-octanol/pH 7.4 phosphate buffer solution partition coefficient of drugs was determined by the shaken-flask method according to the OECD guideline for testing of chemicals (OECD, 1995).
Quantification of drugs in samples was done by LC-MS/MS. HPLC separation of genistein was performed on a Scherzo SS-C18 (3.0 mm I.D. × 150 mm, 3.0 µm, Imtakt, Kyoto, Japan) at 40°C with a gradient of mobile phases A: 13 mM ammonium acetate buffer adjusted to pH 4.0 with 20% acetic acid and B: 0.1% formic acid in methanol. HPLC separation of dantrolene was performed on a Capcell Pak C18 UG120 column (4.6 mm I.D. × 150 mm, 5 μm, Osaka Soda, Osaka, Japan) at 40°C with a gradient of mobile phases A: 0.1% formic acid and 2 mM ammonium acetate in water and B: 0.1% formic acid and 2 mM ammonium acetate in acetonitrile. The gradient of mobile phase B was as follows: 0–60% for 5 min (at 0–5 min), 60–70% for 10 min (at 5–15 min), 100% for 4 min (at 15–19 min), and 0% for 4 min (at 19–23 min) at a flow rate of 0.25 ml/min for genistein determination, and 0% for 1 min (at 0–1 min), 0–90% for 3 min (at 1–4 min), 100% for 2 min (at 4–6 min), and 0% for 3 min (at 6–9 min) at a flow rate of 0.40 ml/min for dantrolene determination. The eluted drugs were quantified by SRM, with the mass spectrometer operating in the positive ionization mode, using m/z of Q1/Q3: 269.2/133.2 for genistein, 253.2/91.0 for daidzein, 244.1/114.0 for dantrolene, and 197.2/124.2 for nitrofurazone. The analyte concentration was determined from the peak area ratio to the internal standard by the use of a calibration curve. The lower limits of quantification for genistein and dantrolene were 10 nM and 16 nM, respectively.
Statistical analyses were performed using an unpaired, two-tailed Student’s t-test. The value of p < 0.05 was taken as the criterion for a statistically significant difference.
All data represent the mean ± S.E.M. unless otherwise indicated. The S.E.M. was calculated according to the following law of propagation of errors; given the next functional relationship between several measured variables such as y, x1, x2, …, xn;
If the variables y, x1, x2, …, xn are uncorrelated, standard deviations of y (
where
The S.E.M. of y is given by
where n(y) is assumed to be
The plasma membrane-enriched fraction of murine placental labyrinth at GD15.5 and 17.5 showed positive peaks for BCRP protein at the same retention time as the IS peptide in chromatograms of three SRM transitions, but no peaks were seen in Bcrp−/− mice at GD17.5 (Supplementary Figure S1). As shown in Figure 1, the BCRP expression amount at GD15.5 was 2.6 fmol/μg protein, but this declined to 0.64 fmol/μg protein at GD17.5.
FIGURE 1. BCRP protein amounts in the placenta. The absolute protein expression levels of mouse BCRP in the plasma membrane fraction of the mouse placental labyrinth and human BCRP in the human placental microvillous membrane-enriched fraction are shown. Each column represents the mean ± S.E.M. of four human donors or three independent sample preparations from different mouse dams. The peak data of the peptides were extracted by using three sets of SRM transitions and the expression levels of individual donors or samples were determined as the mean of three quantitative data. *p < 0.05, significant difference between GD 15.5 and 17.5.
In the human term placental MVM fraction, the BCRP expression amount was 0.66 fmol/μg protein (Figure 1), which appears to be similar to that of murine placental labyrinth at GD17.5. However, the murine placental labyrinth is composed of four cell layers from the maternal side: the continuous but fenestrated trophoblast giant cell layer, two syncytiotrophoblast cell layers, SynT-I and SynT-II, and the fetal capillary endothelial cell layer. And all these cell layers do not have microvillous membranes (Coan et al., 2005). Based on the assumption that approximately one-eighth of the plasma membrane fraction was derived from the apical membrane of SynT-II, where the most of BCRP protein in the labyrinth is localized, the estimated BCRP expression amount in the apical membrane of SynT-II can be calculated as indicated in Table 1. The BCRP expression amount in the human term placental MVM fraction is estimated to be 13% of that in the apical membrane of placental SynT-II at GD17.5. Moreover, the BCRP expression amounts in the apical membrane of SynT-II at GD15.5 and 17.5 are estimated to be 310% and 81%, respectively, of that in the luminal membrane of brain capillary endothelial cells of FVB mice (Table 1) (Uchida et al., 2013).
TABLE 1. Estimated protein amount and apparent impact of BCRP at the luminal membrane of brain capillaries and the apical membrane of placental SynT-II in mice.
The apparent impacts of BCRP at the blood-brain and the placental barriers were respectively evaluated from the brain-to-plasma concentration ratio in the mother (Kp,brain) and the fetal-to-maternal ratio of plasma concentrations (Kp,fp) of BCRP-specific substrates, genistein, and dantrolene, after 2 h of continuous administration in WT and Bcrp−/− pregnant mice at GD15.5 and 17.5. The maternal plasma concentration of genistein was almost constant from 0.5 to 2.0 h (Supplementary Figure S2), indicating that the maternal plasma concentration had reached a plateau at the sampling time of brain and fetal plasma. As shown in Figure 2A, Kp,brain of genistein was significantly increased in Bcrp−/− pregnant mice with a Bcrp−/−/WT ratio of Kp,brain of 6.1 (Table 1), which is consistent with a previous observation in non-pregnant mice (Enokizono et al., 2007). The Kp,fp of genistein at GD15.5 was also significantly increased in Bcrp−/− pregnant mice (Figure 2B) with a Bcrp−/−/WT ratio of Kp,fp of 2.0 (Table 1), which is consistent with a previous observation showing a 1.8-fold increase in fetal tissue-to-maternal plasma concentration ratio of genistein in Bcrp−/− mice at 2 weeks’ gestation (Enokizono et al., 2007). However, the Kp,fp of genistein in Bcrp−/− pregnant mice at GD17.5 was not significantly different from that in WT (Figure 2B), suggesting a diminished impact of BCRP in the fetal distribution of genistein at GD17.5 compared with GD15.5. The increase in Kp,fp of genistein in WT mice at GD17.5 is in good agreement with the decline in the amount of BCRP protein during gestation (Figure 1).
FIGURE 2. The ratio of the brain-to-plasma concentration ratio (Kp,brain) (A) and the fetal-to-maternal ratio of plasma concentrations (Kp,fp) (B) of genistein in wild-type (WT: closed bar) and Bcrp−/− (open bar) mice. The Kp values were measured after continuous infusion of 1.25 mM genistein for 2 h at a rate of 160 μL/h. Each column represents the mean ± S.E.M. (n = 3–6 dams). *p < 0.05, **p < 0.01, significant difference between WT and Bcrp−/− mice.
In the case of dantrolene, even though Kp,brain was significantly increased (3.8-fold) in Bcrp−/− pregnant mice, as previously observed in non-pregnant mice (Figure 3A) (Enokizono et al., 2008), Kp,fp at both gestation times showed no significant difference between WT and Bcrp−/− pregnant mice after 2 h of continuous administration (Figure 3B). The maternal plasma dantrolene concentration increased over time during 2 h of continuous administration (Supplementary Figures S3A,B). Considering that the maternal plasma concentration of dantrolene does not reach a steady-state within 2 h, we extended the duration of dantrolene administration to 24 h in pregnant mice at GD15.5. We confirmed that the maternal plasma dantrolene concentration in WT and Bcrp−/− pregnant mice had reached a steady-state by 24 h (Supplementary Figure S3C). However, there was no difference in Kp,fp of dantrolene between WT and Bcrp−/− pregnant mice even after 24 h of continuous administration (Figure 3C), suggesting that placental BCRP does not function effectively to suppress the fetal distribution of dantrolene.
FIGURE 3. The ratio of the brain-to-plasma concentration ratio (Kp,brain) (A) and the fetal-to-maternal ratio of plasma concentrations (Kp,fp) (B,C) of dantrolene in wild-type (WT: closed bar) and Bcrp−/− (open bar) mice. The Kp values were measured after continuous infusion of 0.50 mM dantrolene for 2 h at a rate of 70 μL/h (A,B) or 7.5 mM dantrolene for 24 h at a rate of 8.0 μL/h (C). Each column represents the mean ± S.E.M. (n = 3–9 dams). *p < 0.05, **p < 0.01, significant difference between WT and Bcrp−/− mice.
The above results indicate that placental BCRP does suppress the fetal distribution of genistein, at least when it is expressed at a high level, at GD15.5. The contribution per single molecule of BCRP protein to the suppression of genistein distribution to the fetus at GD15.5 and to the brain can be determined as the Kp ratio −1 divided by the estimated BCRP protein expression amount in Table 1. The placenta-to-brain ratio of the Kp ratio −1 per single molecule of BCRP protein was calculated to be 0.06 at GD15.5, suggesting that BCRP at the placental barrier does work as a functional efflux pump for genistein, but is much less efficient compared to BCRP at the blood-brain barrier.
The n-octanol/pH 7.4 buffer partition coefficients (D7.4) of genistein and dantrolene were experimentally measured. LogD7.4 values for genistein and dantrolene were 3.94 ± 0.01 and 1.56 ± 0.01, respectively (Table 1), and were not concentration-dependent.
In the present study, we quantitatively compared the effect of BCRP on the distribution of BCRP-specific substrate drugs, genistein and dantrolene (Enokizono et al., 2007; Kodaira et al., 2010), between the blood-brain barrier and the placental barrier using Bcrp−/− mice at GD15.5 and 17.5. In all cases, the values of Kp,fp ratio, taken as an index of the suppressive impact of BCRP on fetal drug distribution, were smaller than the values of Kp,brain ratio, taken as an index of the suppressive impact of BCRP on brain drug distribution. For example, the Kp,fp ratio for genistein was the highest at GD15.5 (2.0) but was much smaller than the corresponding Kp,brain ratio of 6.1. These results mean that the suppressive effect of BCRP on the brain distribution is greater than that on the fetal distribution. These findings are consistent with a previous report (Enokizono et al., 2007). However, the primary focus of this study is to clarify whether or not this difference is simply caused by a difference in BCRP protein level at the plasma membrane. We quantified the absolute amount of BCRP protein in the plasma membrane-enriched fraction of murine placental labyrinth by measuring specific peptide fragments of BCRP using LC-MS/MS and found that the BCRP expression amount at the murine placental barrier is similar at GD17.5 or even higher at GD15.5 than that at the blood-brain barrier (Uchida et al., 2013). Accordingly, the difference in the suppressive impact of BCRP between the brain and the placenta cannot be attributed to the difference in the BCRP protein level.
The placenta-to-brain ratio of the Kp ratio −1 per single BCRP protein was much smaller than unity (less than 0.1 in the case of genistein at GD15.5). The lower impact of placental efflux transporters on the fetal distribution cannot be attributed to lower structural integrity of the placental barrier compared with the blood-brain barrier, because we recently reported that the ratio in the case of paclitaxel, MDR1 substrate, is almost equal to unity (Fujita et al., 2022). The Kp,brain ratio −1 of BCRP substrates can be simply expressed as the ratio of the permeability-surface area (PS) product for the efflux mediated by brain BCRP (PSBCRP,BBB) to that for luminal efflux with the exception of BCRP-mediated efflux (PSl.eff), as follows (Uchida et al., 2011):
On the other hand, the Kp,fp ratio −1 should be described differently, since the murine placental barrier consists of a bilayer with SynT-I and SynT-II. The apical membrane of SynT-II expresses BCRP and also connexin26 gap junctions, which form intercellular channels to the basal plasma membrane of SynT-I (Shin et al., 1996). We recently constructed a transplacental kinetic model of MDR1 substrates in mice, which is applicable to BCRP substrates as well, since the localization of MDR1 protein at the apical membrane of SynT-II is the same as that of BCRP (Akashi et al., 2016). According to the model illustrated in Figure 4, the Kp,fp ratio −1 of BCRP substrates can be expressed as follows (Supplementary Text):
where PSBCRP,PB represents the PS product for the efflux mediated by placental BCRP; PSAP2.inf and PSAP2.eff represent the PS product for influx and efflux across the apical membrane of SynT-II, with the exception of BCRP-mediated efflux, respectively, PSBM1.inf represents the PS product for influx across the basal plasma membrane of SynT-I, and PSGJ represents the PS product for the transfer mediated by gap junctions between SynT-I and SynT-II.
FIGURE 4. Pharmacokinetic model illustrating the PS products for transplacental transfer of BCRP substrates in mice.
Genistein and dantrolene are recognized as substrates for BCRP, but not for other efflux transporters, including MDR1 (Enokizono et al., 2007, 2008; Kodaira et al., 2010). Although genistein is reportedly an inhibitor of OATP1B1 and OATP2B1 (Karlgren et al., 2012) and transport of dantrolene via OAT2 and OAT3 has been proposed (Burckhardt et al., 2016), the localization of these transporter proteins at the basal plasma membrane of SynT-I and the apical membrane of SynT-II has not been observed so far. Therefore, although further studies are necessary to clarify if other transporters influence the transplacental transfer of these drugs, it is worth considering the transplacental transfer under the assumption that PS products other than PSGJ in Eqs 4, 5 are equal to the PS product due to passive diffusion (PSdiff). On this assumption, Kp,brain ratio −1 and Kp,fp ratio −1 can be simply expressed as follows:
On the basis of these equations, the apparent impact of placental BCRP on the fetal distribution of drugs, Kp,fp ratio −1, would be diminished due to permeation through the gap junctions. The presence of a transfer process between SynT-I and SynT-II through connexin26 is supported by the observation of decreased fetal transfer of glucose in connexin26-deficient mice (Gabriel et al., 1998). Gap junctions allow the transfer of molecules up to 1 kDa, and the molecular weights of genistein and dantrolene are 270 and 314, respectively. The crystal structure of the human connexin26 gap junction channel shows that the amino-terminal helixes of the six subunits line the intracellular pore entrance to form a funnel, whose narrowest region has a diameter of 1.4 nm (Maeda et al., 2009). This is consistent with our finding that the apparent impact of murine placental MDR1 was diminished for substrate drugs with minimum projection diameters of less than 1.4 nm, such as digoxin and norbuprenorphine (Fujita et al., 2022). The minimum projection diameters of genistein and dantrolene calculated by MarvinSketch 21.16.0 (https://chemaxon.com/) are 0.9 and 0.8 nm, respectively. Accordingly, the lower impact of placental BCRP on the distributions of genistein and dantrolene compared with brain BCRP is explained by the presence of the bypass route between SynT-I and SynT-II through connexin26.
The diminishing effect of PSGJ on the Kp,fp ratio −1 in Eq. 7 would be greater in compounds with lower passive permeability since the PSGJ values of these compounds are more likely to be larger compared with PSdiff. As a matter of fact, a lesser impact of murine placental MDR1 on the fetal distribution has reportedly been observed in drugs with lower passive permeability like digoxin and norbuprenorphine (log D7.4 of 1.26 and 1.18, respectively), compared with higher passive permeability drugs such as paclitaxel and saquinavir (log D7.4 of 6.83 and log P of 4.70, respectively), (Smit et al., 1999; Liao et al., 2017; Fujita et al., 2022). Considering that logD7.4 of dantrolene (1.56) was found to be much smaller than that of genistein (3.94) as shown in Table 1, the negligible impact of placental BCRP on the fetal distribution of dantrolene (Figure 3) may be explained partly by lower passive permeability of this drug.
Kp,fp was expressed as the ratio of the plasmatic clearance in the maternal-to-fetal direction to the sum of the plasmatic clearance in the fetal-to-maternal direction and the fetal metabolic clearance. The transplacental kinetic model mentioned above assumes that the fetal metabolic clearance of the drug is negligible since the expression levels of most drug-metabolizing enzymes are low, but the murine fetal liver exceptionally expresses UDP-glucuronosyltransferases (UGT) 1A6 and CYP3A16 at a similar level and a higher level, respectively, compared with adult liver (Van Groen et al., 2021). Genistein is reported to be metabolized to glucuronide conjugates by several UGTs, including UGT1A6 (Soukup et al., 2016). Dantrolene is metabolized in the liver via oxidative and reductive pathways and several CYP isoforms, including CYP3A, are involved in the oxidative conversion to hydroxydantrolene (Jayyosi et al., 1993). Thus, a contribution of fetal metabolic clearance cannot be completely ruled out at present. However, even if the clearance of these drugs from the fetus were mostly due to metabolism, the clearance in the maternal-to-fetal direction would still be affected by BCRP. Therefore, the attenuating effect of metabolism cannot explain the almost complete loss of the impact of BCRP observed in this study.
The BCRP expression amount at GD15.5 was four times higher than that at GD17.5 (Figure 1) and was functionally confirmed by the observation that the suppressive effect of BCRP on the fetal distribution of genistein was higher at GD15.5 than at GD17.5 (Figure 2B). The restricted permeability of a tracer across fetal cerebral vasculature was observed by GD15.5 in mice (Ben-Zvi et al., 2014), leading to a hypothesis that the formation of the fetal blood-brain barrier decreases the necessity of BCRP at the placental barrier for protecting the fetal brain after GD15.5. The decline in BCRP protein expression at the placental barrier toward term is consistent with previous results, which indicated that peak BCRP protein expression occurs at GD15.5 based on immunoblotting of the S-9 fraction of murine placenta obtained by means of differential centrifugations (Wang et al., 2006). However, such a gestational change was not detected when using total placental cell lysates (Kalabis et al., 2007). Thus, fractionation to concentrate the plasma membrane is crucial for determining the amount of functional BCRP in the placenta, most likely because a part of the protein is not localized at the plasma membrane and thus does not work as an efflux transporter. In humans, investigations using the membrane fraction from a large number of human placental samples consistently showed decreasing protein levels of BCRP toward term (Meyer Zu Schwabedissen et al., 2006; Anoshchenko et al., 2020; Goetzl et al., 2022). Accordingly, the gestational age-dependent decrease of BCRP protein from mid to term gestation appears to be common in both humans and mice.
In the present study, we have determined the absolute amount of BCRP protein at the human as well as the mouse placental barrier. The BCRP expression amount in human placental MVM was determined to be 0.66 fmol/μg protein (Figure 1), which is similar to the previously reported value of ∼1 fmol/μg total membrane protein in human placenta at term (Anoshchenko et al., 2020). The quantification of the absolute amount of protein enables us to compare BCRP protein levels between species. The BCRP expression amount in the human placental barrier at term is much less (∼10%) than that in mouse at GD17.5, which is a near term based on the gestational period of FVB mouse of approximately 18.5 days (Murray et al., 2010). The BCRP protein level in human placenta is several times higher at earlier gestation (Meyer Zu Schwabedissen et al., 2006; Anoshchenko et al., 2020; Goetzl et al., 2022), and this trend is also observed in mice at GD15.5 and 17.5 (Figure 1). Therefore, it is reasonable to consider that the BCRP protein level at the placental barrier is constitutively lower in humans than in mice at least from mid to term gestation. It should be noted that lower protein expression in human placenta does not necessarily imply a lower impact of placental BCRP in humans compared with mice, since the placental BCRP in humans is localized at MVM of the monolayer and is not functionally attenuated by gap junctions, as occurs in mice. It may be also necessary to consider the possible involvement of BCRP expressed in fetal capillaries of the placenta (Han et al., 2018). On the assumption that the Kp ratio −1 per single BCRP protein is similar in human placental barrier and at the murine blood-brain barrier, the values of Kp,fp ratio of genistein and dantrolene in humans are anticipated to be 1.5, and 1.3, respectively. However, further studies will be needed to confirm these estimates.
The absolute amount of BCRP protein determined in the present study will aid in estimating the exposure to substrate drugs. However, BCRP proteins form dimers and oligomers and have been observed to be present as the dimer in human and murine placentas and murine brain capillaries (Kolwankar et al., 2005; Tachikawa et al., 2005; Kalabis et al., 2007). More accurate estimates of the abundance of functional BCRP proteins can be achieved by determining the fraction of dimers/oligomers.
In conclusion, our findings indicate that the apparent contribution of a single BCRP molecule to suppression of the fetal distribution of at least some substrates (genistein and dantrolene) at the murine placental barrier is much smaller than that at the blood-brain barrier. In mice, the apparent impact of BCRP on the fetal distribution of its substrate drugs with low passive permeability and small molecular diameter may be low due to the presence of the bypass route through gap junctions. Although the expression amount of BCRP protein in human placenta is lower than that in mouse placenta, this species difference appears to be functionally compensated by the murine-specific bypass route through gap junctions, at least in part. We also found that changes in BCRP expression at the murine placental barrier during gestation correlate with the function of BCRP in suppressing fetal drug distribution. These findings will be helpful for predicting fetal drug distribution in humans by extrapolation from data obtained using in vitro cells, different tissues, and different animal species.
The raw data supporting the conclusions of this article will be made available by the authors, without undue reservation.
The studies involving human participants were reviewed and approved by the Institutional Ethics Committee of Keio University Faculty of Pharmacy and Keio University School of Medicine. The patients/participants provided their written informed consent to participate in this study. The animal study was reviewed and approved by The Keio University Institutional Animal Care and Use Committee.
Participated in research design: AF, SN, TN, and MT. Conducted experiments: AF and RH. Contributed reagents or analytic tools: TS, SK, TM, and YS. Performed data analysis: AF, SN, RH, TN, and MT. Discussed and edited the manuscript: TS, SK, TM, and YS. Wrote or contributed to the writing of the manuscript: AF and MT.
This work was supported in part by JSPS KAKENHI grant numbers 21H02651, 20K21489, 20K22703, 26282028, and MHLW grant number 20KC2009. It was also funded in part by the Hoansha Foundation and the Mochida Memorial Foundation for Medical and Pharmaceutical Research.
The authors declare that the research was conducted in the absence of any commercial or financial relationships that could be construed as a potential conflict of interest.
All claims expressed in this article are solely those of the authors and do not necessarily represent those of their affiliated organizations, or those of the publisher, the editors, and the reviewers. Any product that may be evaluated in this article, or claim that may be made by its manufacturer, is not guaranteed or endorsed by the publisher.
The Supplementary Material for this article can be found online at: https://www.frontiersin.org/articles/10.3389/fddev.2022.932576/full#supplementary-material
Akashi, T., Nishimura, T., Takaki, Y., Takahashi, M., Shin, B.-C., Tomi, M., et al. (2016). Layer II of Placental Syncytiotrophoblasts Expresses MDR1 and BCRP at the Apical Membrane in Rodents. Reprod. Toxicol. 65, 375–381. doi:10.1016/j.reprotox.2016.09.002
Allikmets, R., Schriml, L. M., Hutchinson, A., Romano-Spica, V., and Dean, M. (1998). A Human Placenta-specific ATP-Binding Cassette Gene (ABCP) on Chromosome 4q22 that Is Involved in Multidrug Resistance. Cancer Res. 58, 5337–5339.
Anoshchenko, O., Prasad, B., Neradugomma, N. K., Wang, J., Mao, Q., and Unadkat, J. D. (2020). Gestational Age-dependent Abundance of Human Placental Transporters as Determined by Quantitative Targeted Proteomics. Drug Metab. Dispos. 48, 735–741. doi:10.1124/dmd.120.000067
Ben-Zvi, A., Lacoste, B., Kur, E., Andreone, B. J., Mayshar, Y., Yan, H., et al. (2014). Mfsd2a Is Critical for the Formation and Function of the Blood-Brain Barrier. Nature 509, 507–511. doi:10.1038/nature13324
Burckhardt, B. C., Henjakovic, M., Hagos, Y., and Burckhardt, G. (2016). Counter-flow Suggests Transport of Dantrolene and 5-OH Dantrolene by the Organic Anion Transporters 2 (OAT2) and 3 (OAT3). Pflugers Arch. - Eur. J. Physiol. 468, 1909–1918. doi:10.1007/s00424-016-1894-6
Coan, P. M., Ferguson-Smith, A. C., and Burton, G. J. (2005). Ultrastructural Changes in the Interhaemal Membrane and Junctional Zone of the Murine Chorioallantoic Placenta across Gestation. J. Anat. 207, 783–796. doi:10.1111/j.1469-7580.2005.00488.x
Enokizono, J., Kusuhara, H., Ose, A., Schinkel, A. H., and Sugiyama, Y. (2008). Quantitative Investigation of the Role of Breast Cancer Resistance Protein (Bcrp/Abcg2) in Limiting Brain and Testis Penetration of Xenobiotic Compounds. Drug Metab. Dispos. 36, 995–1002. doi:10.1124/dmd.107.019257
Enokizono, J., Kusuhara, H., and Sugiyama, Y. (2007). Effect of Breast Cancer Resistance Protein (Bcrp/Abcg2) on the Disposition of Phytoestrogens. Mol. Pharmacol. 72, 967–975. doi:10.1124/mol.107.034751
Fujita, A., Noguchi, S., Hamada, R., Inoue, S., Shimada, T., Katakura, S., et al. (2022). Limited Impact of Murine Placental MDR1 on Fetal Exposure of Certain Drugs Explained by Bypass Transfer between Adjacent Syncytiotrophoblast Layers. Pharm. Res. doi:10.1007/s11095-022-03165-6
Furukawa, S., Kuroda, Y., and Sugiyama, A. (2014). A Comparison of the Histological Structure of the Placenta in Experimental Animals. J. Toxicol. Pathol. 27, 11–18. doi:10.1293/tox.2013-0060
Gabriel, H.-D., Jung, D., Bützler, C., Temme, A., Traub, O., Winterhager, E., et al. (1998). Transplacental Uptake of Glucose Is Decreased in Embryonic Lethal Connexin26-Deficient Mice. J. Cell Biol. 140, 1453–1461. doi:10.1083/jcb.140.6.1453
Goetzl, L., Darbinian, N., Merabova, N., Devane, L. C., and Ramamoorthy, S. (2022). Gestational Age Variation in Human Placental Drug Transporters. Front. Pharmacol. 13, 837694. doi:10.3389/fphar.2022.837694
Han, L. W., Gao, C., and Mao, Q. (2018). An Update on Expression and Function of P-gp/ABCB1 and BCRP/ABCG2 in the Placenta and Fetus. Expert Opin. Drug Metabolism Toxicol. 14, 817–829. doi:10.1080/17425255.2018.1499726
Jayyosi, Z., Villoutreix, J., Ziegler, J. M., Batt, A. M., De Maack, F., Siest, G., et al. (1993). Identification of Cytochrome P-450 Isozymes Involved in the Hydroxylation of Dantrolene by Rat Liver Microsomes. Drug Metab. Dispos. 21, 939–945.
Jonker, J. W., Buitelaar, M., Wagenaar, E., Van der Valk, M. A., Scheffer, G. L., Scheper, R. J., et al. (2002). The Breast Cancer Resistance Protein Protects against a Major Chlorophyll-Derived Dietary Phototoxin and Protoporphyria. Proc. Natl. Acad. Sci. U.S.A. 99, 15649–15654. doi:10.1073/pnas.202607599
Kalabis, G. M., Petropoulos, S., Gibb, W., and Matthews, S. G. (2007). Breast Cancer Resistance Protein (Bcrp1/Abcg2) in Mouse Placenta and Yolk Sac: Ontogeny and its Regulation by Progesterone. Placenta 28, 1073–1081. doi:10.1016/j.placenta.2007.03.010
Kamiie, J., Ohtsuki, S., Iwase, R., Ohmine, K., Katsukura, Y., Yanai, K., et al. (2008). Quantitative Atlas of Membrane Transporter Proteins: Development and Application of a Highly Sensitive Simultaneous LC/MS/MS Method Combined with Novel In-Silico Peptide Selection Criteria. Pharm. Res. 25, 1469–1483. doi:10.1007/s11095-008-9532-4
Karlgren, M., Vildhede, A., Norinder, U., Wisniewski, J. R., Kimoto, E., Lai, Y., et al. (2012). Classification of Inhibitors of Hepatic Organic Anion Transporting Polypeptides (OATPs): Influence of Protein Expression on Drug-Drug Interactions. J. Med. Chem. 55, 4740–4763. doi:10.1021/jm300212s
Kodaira, H., Kusuhara, H., Ushiki, J., Fuse, E., and Sugiyama, Y. (2010). Kinetic Analysis of the Cooperation of P-Glycoprotein (P-gp/Abcb1) and Breast Cancer Resistance Protein (Bcrp/Abcg2) in Limiting the Brain and Testis Penetration of Erlotinib, Flavopiridol, and Mitoxantrone. J. Pharmacol. Exp. Ther. 333, 788–796. doi:10.1124/jpet.109.162321
Kolwankar, D., Glover, D. D., Ware, J. A., and Tracy, T. S. (2005). Expression and Function of ABCB1 and ABCG2 in Human Placental Tissue. Drug Metab. Dispos. 33, 524–529. doi:10.1124/dmd.104.002261
Liao, M. Z., Gao, C., Shireman, L. M., Phillips, B., Risler, L. J., Neradugomma, N. K., et al. (2017). P-gp/ABCB1 Exerts Differential Impacts on Brain and Fetal Exposure to Norbuprenorphine. Pharmacol. Res. 119, 61–71. doi:10.1016/j.phrs.2017.01.018
Liu, H., Huang, L., Li, Y., Fu, T., Sun, X., Zhang, Y.-Y., et al. (2017). Correlation between Membrane Protein Expression Levels and Transcellular Transport Activity for Breast Cancer Resistance Protein. Drug Metab. Dispos. 45, 449–456. doi:10.1124/dmd.116.074245
Maeda, S., Nakagawa, S., Suga, M., Yamashita, E., Oshima, A., Fujiyoshi, Y., et al. (2009). Structure of the Connexin 26 Gap Junction Channel at 3.5 Å Resolution. Nature 458, 597–602. doi:10.1038/nature07869
Maliepaard, M., Scheffer, G. L., Faneyte, I. F., van Gastelen, M. A., Pijnenborg, A. C., Schinkel, A. H., et al. (2001). Subcellular Localization and Distribution of the Breast Cancer Resistance Protein Transporter in Normal Human Tissues. Cancer Res. 61, 3458–3464.
Murray, S. A., Morgan, J. L., Kane, C., Sharma, Y., Heffner, C. S., Lake, J., et al. (2010). Mouse Gestation Length Is Genetically Determined. PLoS One 5, e12418. doi:10.1371/journal.pone.0012418
OECD (1995). Test No. 107: Partition Coefficient (N-octanol/water): Shake Flask Method. OECD. doi:10.1787/9789264069626-en
Ohtsuki, S., and Terasaki, T. (2007). Contribution of Carrier-Mediated Transport Systems to the Blood-Brain Barrier as a Supporting and Protecting Interface for the Brain; Importance for CNS Drug Discovery and Development. Pharm. Res. 24, 1745–1758. doi:10.1007/s11095-007-9374-5
Shin, B.-C., Suzuki, T., Matsuzaki, T., Tanaka, S., Kuraoka, A., Shibata, Y., et al. (1996). Immunolocalization of GLUT1 and Connexin 26 in the Rat Placenta. Cell Tissue Res. 285, 83–89. doi:10.1007/s004410050623
Smit, J. W., Huisman, M. T., van Tellingen, O., Wiltshire, H. R., and Schinkel, A. H. (1999). Absence or Pharmacological Blocking of Placental P-Glycoprotein Profoundly Increases Fetal Drug Exposure. J. Clin. Invest. 104, 1441–1447. doi:10.1172/JCI7963
Soukup, S. T., Helppi, J., Müller, D. R., Zierau, O., Watzl, B., Vollmer, G., et al. (2016). Phase II Metabolism of the Soy Isoflavones Genistein and Daidzein in Humans, Rats and Mice: a Cross-Species and Sex Comparison. Arch. Toxicol. 90, 1335–1347. doi:10.1007/s00204-016-1663-5
Tachikawa, M., Watanabe, M., Hori, S., Fukaya, M., Ohtsuki, S., Asashima, T., et al. (2005). Distinct Spatio-Temporal Expression of ABCA and ABCG Transporters in the Developing and Adult Mouse Brain. J. Neurochem. 95, 294–304. doi:10.1111/j.1471-4159.2005.03369.x
Uchida, Y., Ohtsuki, S., Kamiie, J., and Terasaki, T. (2011). Blood-Brain Barrier (BBB) Pharmacoproteomics: Reconstruction of In Vivo Brain Distribution of 11 P-Glycoprotein Substrates Based on the BBB Transporter Protein Concentration, In Vitro Intrinsic Transport Activity, and Unbound Fraction in Plasma and Brain in Mice. J. Pharmacol. Exp. Ther. 339, 579–588. doi:10.1124/jpet.111.184200
Uchida, Y., Tachikawa, M., Obuchi, W., Hoshi, Y., Tomioka, Y., Ohtsuki, S., et al. (2013). A Study Protocol for Quantitative Targeted Absolute Proteomics (QTAP) by LC-MS/MS: Application for Inter-strain Differences in Protein Expression Levels of Transporters, Receptors, Claudin-5, and Marker Proteins at the Blood-Brain Barrier in ddY, FVB, and C57BL/6J Mice. Fluids Barriers CNS 10, 1–22. doi:10.1186/2045-8118-10-21
Van Groen, B. D., Nicolaï, J., Kuik, A. C., Van Cruchten, S., Van Peer, E., Smits, A., et al. (2021). Ontogeny of Hepatic Transporters and Drug-Metabolizing Enzymes in Humans and in Nonclinical Species. Pharmacol. Rev. 73, 597–678. doi:10.1124/pharmrev.120.000071
Wang, H., Wu, X., Hudkins, K., Mikheev, A., Zhang, H., Gupta, A., et al. (2006). Expression of the Breast Cancer Resistance Protein (Bcrp1/Abcg2) in Tissues from Pregnant Mice: Effects of Pregnancy and Correlations with Nuclear Receptors. Am. J. Physiology-Endocrinology Metabolism 291, E1295–E1304. doi:10.1152/ajpendo.00193.2006
Zhang, W., Mojsilovic-Petrovic, J., Andrade, M. F., Zhang, H., Ball, M., and Stanimirovic, D. B. (2003). Expression and Functional Characterization of ABCG2 in Brain Endothelial Cells and Vessels. FASEB J. 17, 1–24. doi:10.1096/fj.02-1131fje
Zhang, Y., Wang, H., Unadkat, J. D., and Mao, Q. (2007). Breast Cancer Resistance Protein 1 Limits Fetal Distribution of Nitrofurantoin in the Pregnant Mouse. Drug Metab. Dispos. 35, 2154–2158. doi:10.1124/dmd.107.018044
Zhou, L., Naraharisetti, S. B., Wang, H., Unadkat, J. D., Hebert, M. F., and Mao, Q. (2008). The Breast Cancer Resistance Protein (Bcrp1/Abcg2) Limits Fetal Distribution of Glyburide in the Pregnant Mouse: An Obstetric-Fetal Pharmacology Research Unit Network and University of Washington Specialized Center of Research Study. Mol. Pharmacol. 73, 949–959. doi:10.1124/mol.107.041616
zu Schwabedissen, H. E. M., Grube, M., Dreisbach, A., Jedlitschky, G., Meissner, K., Linnemann, K., et al. (2006). Epidermal Growth Factor-Mediated Activation of the MAP Kinase Cascade Results in Altered Expression and Function of ABCG2 (BCRP). Drug Metab. Dispos. 34, 524–533. doi:10.1124/dmd.105.007591
Keywords: breast cancer resistance protein, blood-brain barrier, pharmacokinetic model, placenta, syncytiotrophoblast
Citation: Fujita A, Noguchi S, Hamada R, Shimada T, Katakura S, Maruyama T, Sai Y, Nishimura T and Tomi M (2022) Quantitative Comparison of Breast Cancer Resistance Protein (BCRP/ABCG2) Expression and Function Between Maternal Blood-Brain Barrier and Placental Barrier in Mice at Different Gestational Ages. Front. Drug. Deliv. 2:932576. doi: 10.3389/fddev.2022.932576
Received: 29 April 2022; Accepted: 15 June 2022;
Published: 22 July 2022.
Edited by:
Sumio Ohtsuki, Kumamoto University, JapanCopyright © 2022 Fujita, Noguchi, Hamada, Shimada, Katakura, Maruyama, Sai, Nishimura and Tomi. This is an open-access article distributed under the terms of the Creative Commons Attribution License (CC BY). The use, distribution or reproduction in other forums is permitted, provided the original author(s) and the copyright owner(s) are credited and that the original publication in this journal is cited, in accordance with accepted academic practice. No use, distribution or reproduction is permitted which does not comply with these terms.
*Correspondence: Masatoshi Tomi, dG9taS1tc0BwaGEua2Vpby5hYy5qcA==
Disclaimer: All claims expressed in this article are solely those of the authors and do not necessarily represent those of their affiliated organizations, or those of the publisher, the editors and the reviewers. Any product that may be evaluated in this article or claim that may be made by its manufacturer is not guaranteed or endorsed by the publisher.
Research integrity at Frontiers
Learn more about the work of our research integrity team to safeguard the quality of each article we publish.